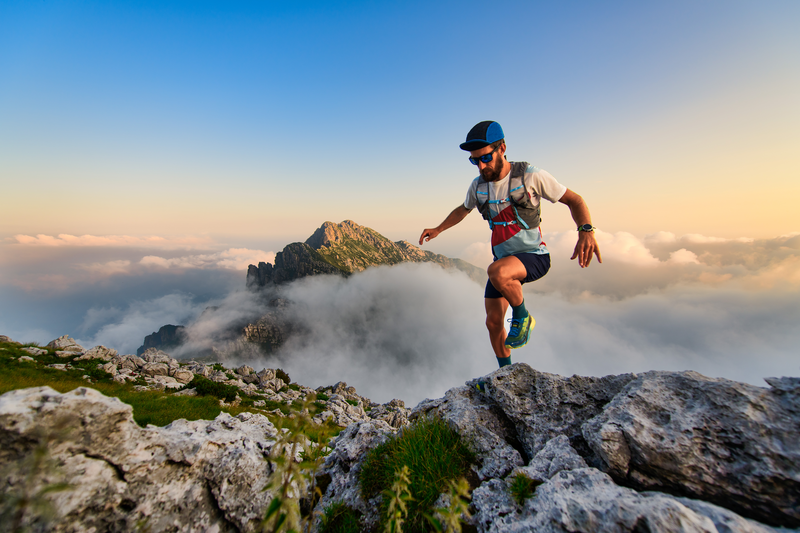
94% of researchers rate our articles as excellent or good
Learn more about the work of our research integrity team to safeguard the quality of each article we publish.
Find out more
SYSTEMATIC REVIEW article
Front. Pharmacol. , 09 June 2022
Sec. Pharmacology of Anti-Cancer Drugs
Volume 13 - 2022 | https://doi.org/10.3389/fphar.2022.908079
This article is part of the Research Topic Drug-Resistance in Cancer Cells: A New Wine in an old Bottle View all 15 articles
Digestive system tumours, including stomach, colon, esophagus, liver and pancreatic tumours, are serious diseases affecting human health. Although surgical treatment and postoperative chemoradiotherapy effectively improve patient survival, current diagnostic and therapeutic strategies for digestive system tumours lack sensitivity and specificity. Moreover, the tumour’s tolerance to drug therapy is enhanced owing to tumour cell heterogeneity. Thus, primary or acquired treatment resistance is currently the main hindrance to chemotherapy efficiency. N6-methyladenosine (m6A) has various biological functions in RNA modification. m6A modification, a key regulator of transcription expression, regulates RNA metabolism and biological processes through the interaction of m6A methyltransferase (“writers”) and demethylase (“erasers”) with the binding protein decoding m6A methylation (“readers”). Additionally, m6A modification regulates the occurrence and development of tumours and is a potential driving factor of tumour drug resistance. This review systematically summarises the regulatory mechanisms of m6A modification in the drug therapy of digestive system malignancies. Furthermore, it clarifies the related mechanisms and therapeutic prospects of m6A modification in the resistence of digestive system malignancies to drug therapy.
Digestive system tumours predominantly include stomach, colon, esophagus, liver and pancreatic tumours. Currently, these tumours have high morbidity and mortality rates. Among patients with digestive system tumours, the elderly account for approximately 68.5% (Sung et al., 2021). Surgery, including open, laparoscopic and endoscopic surgeries, is currently the standard treatment for digestive system tumours (Chesney et al., 2021). To date, several treatment strategies, including chemotherapy and radiation, have enhanced the disease-free and overall survival rates of patients with cancer (Zhang et al., 2020a). However, owing to the heterogeneity of cancer cells, primary or acquired treatment resistance is often observed, leading to treatment failure (Dagogo-Jack and Shaw, 2018). Additionally, the occurrence and development of digestive system tumours are reported to be related to the activation of oncogenes, inactivation of tumour suppressor genes and activation of abnormal cell signalling pathways. Furthermore, epigenetic processes regulate gene expression via DNA methylation, histone modification and RNA modification, thereby affecting the occurrence and development of tumours in the digestive system (Kong et al., 2020a).
Liver cancer is the fourth most common cause of cancer-related death worldwide, with hepatocellular carcinoma (HCC) as the most prevalent form, and the incidence of liver cancer is reported to be increasing globally (Kong et al., 2019; Chen et al., 2021a; Kong et al., 2021). HCC is the second most common cause of cancer-related death, but its current treatment strategies and outcomes are poor (Chen et al., 2021a; Llovet et al., 2021). Several drugs, such as Sorafenib (SOR) and Lenvatinib, have been approved for the first-line systemic therapy of advanced or unresectable patients with HCC (Al-Salama et al., 2019; Kant et al., 2021). Thus, the identification of new drug targets for the treatment of HCC is important, especially in tumour immuno-targeted therapy. Currently, systematic therapies, including immune checkpoint inhibitors (ICIs), tyrosine kinase inhibitors and monoclonal antibodies, have been reporting better outcomes than traditional HCC therapies (Llovet et al., 2021). Over the past five years, significant advances have been made in overall survival and the quality of life (Llovet et al., 2018). However, various challenges continue to exist in the treatment of HCC, such as drug resistance.
Pancreatic cancer, a highly lethal malignancy with a 5-year survival rate of approximately 10% in the United States, is becoming an increasingly common cause of cancer-associated death (Mizrahi et al., 2020). Currently, surgical resection remains the only option to cure pancreatic cancer. However, adjuvant chemotherapy has made significant progress in improving the prognosis of patients with pancreatic cancer (Wang et al., 2021a). Adjuvant chemotherapy regimens include FOLFIRINOX [5-fluorouracil (FU), folate, irinotecan and oxaliplatin (OX)] and gemcitabine combined with sodium protein, sodium and paclitaxel and have been shown to prolong overall survival (Mizrahi et al., 2020). Currently, many clinical trials are evaluating the effectiveness of immunotherapy strategies in pancreatic cancer, including ICIs; cancer vaccines; adoptive cell metastasis; and combinations with other immunotherapy agents, chemoradiotherapy, or other molecule-targeted agents. However, the therapeutic outcomes of these strategies remain poor (Schizas et al., 2020). Therefore, the molecular mechanism of pancreatic cancer requires further exploration.
Colorectal cancer (CRC) is the third most common cancer type and has the third-highest rate of cancer-related deaths in the United States (Kong et al., 2020b). CRC ranks 2nd to 4th in the global incidence of cancer depending on region, cancer type or gender (Sawicki et al., 2021). It remains one of the deadliest diseases worldwide due to the lack of early detection methods and appropriate drug treatment strategies (Pavitra et al., 2021). Surgery remains the preferred treatment method for CRC (Chen et al., 2018). Additionally, chemotherapy has become an effective treatment to prolong the survival of patients with CRC. Currently, the commonly used treatment regimens are FOLFOX (OX + calcium folate and FU), CAPEOX (OX + capecitabine) and FOLFIRI (irinotecan + calcium folate and FU) (Dekker et al., 2019). However, the treatment and prognosis of CRC have been unsatisfactory, especially for patients with metastasis. Therefore, the identification of novel drug targets and the improvement of drug resistance could effectively improve the prognosis and survival rate of patients with CRC.
Gastric cancer is the fifth most common cancer worldwide and the third most common cause of cancer-associated deaths (Smyth et al., 2020). Gastric cancer is a multifactorial disease wherein environmental and genetic factors influence its occurrence and development. It is also a highly invasive and heterogeneous malignant tumour (Machlowska et al., 2020). Presently, surgery is the first-line of treatment for gastric cancer (Johnston and Beckman, 2019). Postoperative adjuvant radiotherapy/chemotherapy and targeted therapy have become a routine course of treatment for gastric cancer. Furthermore, active early screening could effectively aid in the early diagnosis of gastric cancer. However, the early diagnosis of gastric cancer remains a challenge due to the poor specificity of diagnostic markers and the cost of screening (Johnston and Beckman, 2019). Therefore, the development of new diagnostic and therapeutic targets is vital to the treatment of gastric cancer.
N6-methyladenosine (m6A) is considered to be the most common, abundant and conserved internal transcriptional modification, especially in eukaryotic messenger RNA (mRNA) (Huo et al., 2020). m6A modification exists in mRNA and various non-coding RNAs (Ma et al., 2019; Huang et al., 2020). m6A is modified by m6A methyltransferase (writer), removed by m6A methylase (eraser) and recognized by reading proteins (reader). It regulates RNA metabolism, including translation, splicing, export, degradation and microRNA (miRNA) processing. Recently, m6A RNA modification has been proved to play a key role in tumour development (Yan et al., 2021). The alteration of m6A levels regulates the expression of tumour-related genes, such as BRD4, MYC, SOCS2 and epidermal growth factor receptor (EGFR), thereby promoting the pathogenesis and development of tumours (He et al., 2019). Many studies report that the dysregulation of m6A is associated with the progression and drug resistance of various cancers, suggesting that m6A regulatory factors can be used as therapeutic targets in cancer treatment and biomarkers in overcoming drug resistance (Xu et al., 2020a).
m6A writers are composed of KIAA1429 (VIRMA), METTL3, RBM15, WTAP, ZC3H13, METTL16, METTL14, and CBLL1 (Shen et al., 2020). As a heterodimer, METTL3/METTL14 can be catalysed and bound by WTAP, which interacts with METTL3/METTL14 and regulates the translation stability of mRNA (Schöller et al., 2018). KIAA1429 plays a key role in guiding the deposition of regionally selective m6A (Hu et al., 2020) and regulating the expression of sex-lethal genes by the selective splicing of pre-mRNA using WTAP (Bansal et al., 2014). METTL16, a newly discovered RNA m6A methyltransferase, acts as an RNA-binding protein (RBP) and plays a key role in SAM homeostasis by regulating SAM synthase MAT2A mRNA (Doxtader et al., 2018). RBM15, an RBP, can regulate Notch, Wnt and other signalling pathways and affect the development of various tumour cells (Wang et al., 2021b). ZC3H13 plays the role of a tumour suppressor, mainly inhibiting tumour occurrence by regulating the Ras–ERK signalling pathway (Zhu et al., 2019a). The mechanism of action of m6A writers in tumours is shown in Figure 1.
FIGURE 1. Molecular mechanisms of m6A modification in digestive tumors. m6A modification is a dynamic and reversible process. Methyltransferase complexes (writers) catalyze m6A methylation, demethylase (erasers) reverse m6A methylation, and m6A binding protein (readers) promote its function. m6A methylation is involved in carcinogenesis and chemotherapy resistance of digestive tumors.
m6A erasers are predominantly composed of ALKBH5 and FTO (Huo et al., 2020). ALKBH5 regulates RNA metabolism through m6A demethylation, such as pre-mRNA processing and mRNA decay and translation (Qu et al., 2022). It participates in the modification of oncogene or tumour suppressor gene mRNA in an m6A-dependent manner. Moreover, ALKBH5 regulates the transcriptome of tumours, causing changes in cell proliferation, survival, invasion and metastasis; drug sensitivity; tumour stem cell status; and tumour immunity (Qu et al., 2022). Given that ALKBH5 has high substrate specificity in tumours, targeting ALKBH5 has promising potential in cancer treatment (Wang et al., 2020a). On understanding ALKBH5 structure, mediated carcinogenesis and drug reaction mechanism, ALKBH5-targeted therapy could be applied in clinical practice. FTO, a demethylase, was originally identified to be involved in the development of obesity and type 2 diabetes. This gene encodes the FTO protein, which belongs to the ALKB dioxygenase family that is dependent on Fe2+ and 2-oxoglutarate (Gerken et al., 2007). The dysregulation of FTO demethylation has been identified as a driver of various diseases, including cancer, metabolic diseases and neuropsychiatric disorders (Annapoorna et al., 2019). Studies have found that the abnormal expression of FTO is increasingly associated with various diseases, especially cancer. Thus, the development of FTO modulators has potential therapeutic applications. Recent studies report that inhibitors that interfere with FTO activity show significant therapeutic effects in different cancers, thus providing a new strategy for identifying drugs that target external transcriptomic RNA methylation in drug discovery (Zhou et al., 2021). The mechanism of action of m6A erasers in tumours is illustrated in Figure 1.
m6A readers can be divided into three types based on binding m6A-containing transcription: YTH domain (YTH family protein), HNRNP family (hnRNPC, hnRNPG and hnRNPA2B1) and common RNA-binding domain and its flanking regions (IGF2BPs and hnRNPA2B1) (Shi et al., 2021; Huang et al., 2022). Additionally, FXR family, IGF2BP family, eIF family and G3BPs family (44). Heterogeneous nuclear ribonucleoproteins (hnRNPs) are a large family of RBPs that are involved in the many aspects of nucleic acid metabolism, including alternative splicing, mRNA stabilization and transcription and translation regulation (Geuens et al., 2016). hnRNP family proteins are abnormally expressed in most tumours and play a role in promoting tumour occurrence and development. The YTH domain protein family is the main “reader” of m6A modification while the YTH domain can recognize and bind m6A-containing RNA. YTH family proteins have different functions to determine the metabolic fate of m6A-modified RNAs (Shi et al., 2021). YTHDF1 selectively recognises m6A-modified mRNA through the YTH domain, promotes its loading into ribosomes and interacts with initiation factors to promote its translation through the N-terminal domain (Wang et al., 2015). Conversely, YTHDF2 selectively binds m6A-modified RNA and regulates its degradation by recruiting CCR4–NOT complexes to accelerate RNA de-enylation (Zhao et al., 2017). YTHDF3 acts as an assigner, following which YTHDF1 and YTHDF2 competitively interact with YTHDF3, thus determining the fate of the mRNA transcript (Jin et al., 2020). YTHDC1, a widely expressed nuclear protein, is located in YT bodies near nuclear spots and phosphorylated by members of the SRC and TEC tyrosine kinase families in the cytoplasm, leading to its conversion function in RNA splicing (Rafalska et al., 2004). Like other proteins in the YTH family, YTHDC2 can recognize and bind to the m6A fragment of mRNA to play a regulatory role (Wojtas et al., 2017). YTHDC2 can improve the translation efficiency of its target, thus affecting the occurrence of tumours (Hsu et al., 2017). The mechanism of m6A readers in tumours is shown in Figure 1.
The prognosis of digestive tract tumours has been significantly improved in recent years. However, the early diagnosis of digestive tract tumours remains elusive, and the phenomenon of drug resistance persists. Moreover, the underlying aetiology of these malignancies remains unclear, therefore, the epigenetic factors that promote the occurrence and development of digestive malignancies should be elucidated and novel biomarkers or effective therapeutic targets should be simultaneously identified (Seebacher et al., 2019). Numerous studies report that m6A modification promotes the occurrence and development of tumours by regulating oncogene expression and inhibiting genes (Huo et al., 2020). Through epigenetic modification, m6A can promote and inhibit tumorigenesis, playing a “double-edged sword” role (He et al., 2019). Furthermore, the m6A regulatory protein is a therapeutic target for cancer and plays an important biological role in the resistance of malignant tumours to chemotherapy (Yu et al., 2019). Additionally, studies show that the mechanisms of drug resistance in malignant tumours are complex and diverse. m6A-dependent RNA modification has also received extensive attention as a potential determinant of tumour heterogeneity and chemotherapy response.
Presently, the identification of efficient and safe chemical drugs for m6A modification is under study. In particular, few drugs based on natural products are characterised by novel structures, various biological activities and reliable safety (Lu and Wang, 2020). Therefore, an m6A regulator based on natural product discovery is considered the future research direction. Furthermore, modern drug discovery platforms, which are characterized by a combination of web-based pharmacology, chemical databases derived from natural resources, computer-aided design and chemical modifications, have been recognized to aid in the development of new drugs that target m6A regulators (Zhang et al., 2021a). Recently, natural products have been used as the chemical libraries of m6A-targeted anticancer drugs, subsequently becoming potential anti-tumour drugs. For example, curcumin is a natural phenolic compound that down-regulates the expression of ALKBH5 and enhances the expression of m6A modified TRAF4-mRNA (Chen et al., 2021b). Resveratrol is a natural polyphenol with antioxidant, anti-inflammatory, heart-protective and anticancer properties that can be used in combination with curcumin to reduce m6A modifications, thereby effectively improving normal growth performance and intestinal mucosal integrity (Gan et al., 2019). Quercetin, another flavonoid, has various biological functions, including anti-cancer activity. It can inhibit the proliferation, migration and invasion of HeLa and SiHa cells synergistically with cisplatin by inhibiting METTL3 expression (Xu et al., 2021). Recent studies suggest that betaine plays an important role in the methylation of m6A. Zhang et al. found that betaine inhibited the expression of m6A methylases, METTL3 and METTL14, in HepG2 cells, but promoted the expression of demethylases, FTO and ALKBH5 Zhang et al. (2019). In addition to these natural products, other active natural products have also been shown to have anti-M6A bioactivity and anti-cancer activity. Fusarium acid decreases p53 expression in HCC HepG2 cells by down-regulating the m6A methylation of p53-mRNA (Ghazi et al., 2021). Although many studies have shown promising prospects for the development of targeted m6A modification drugs, only a few have potential drug-capabilities and can be used as therapeutic targets for cancer treatment. The currently developed m6A modification inhibitors and activators still have disadvantages, such as poor target specificity, efficacy, safety and pharmacokinetics (Huff et al., 2021). Hence, developing novel drugs is vital to cancer treatment.
Thanks to the rapid development of science and technology, especially artificial intelligence (AI) technology and computer technology, the newly discovered drugs have the advantages of fast speed, easy use and cost-saving. Presently, AI-assisted technology has been widely used in drug candidate discovery and development (Paul et al., 2021). Chen et al. (2012) developed a series of FTO inhibitors using AI techniques. The natural product rhein was identified as the first cell-based FTO inhibitor, which also inhibited ALKBH2 activity. Additionally, they designed and synthesised eight luciferin molecules whose structures were similar to two luciferin molecules. The structure-activity relationship of these fluorescent FTO inhibitors was elucidated by the X-ray crystal structure of FTO/luciferin complexes. These studies demonstrate advancement in identifying novel chemical CLASS FTO inhibitors with strictly defined physicochemical properties by combining structure-based drug design with high-throughput in vitro inhibition test systems. Additionally, Huang et al. identified Entacapone as an FTO inhibitor by combining several methods, including structure-based hierarchical virtual screening strategies, biochemical experiments, in vivo experiments and transcriptome sequencing analysis (Peng et al., 2019). Chen et al. also found two effective FTO inhibitors CS1 and CS2 using structure-based virtual screening (Su et al., 2020). Using molecular docking, Lan et al. identified a cage-like molecular activator of METTL3/14, the photocured substituent-linked MPCH. The drug activates METTL3/14 and results in m6A hypermethylation under short periods of ultraviolet light exposure (Lan et al., 2021a). Currently, AI has aided in the development of revolutionary approaches to drug discovery, design and development, therefore, targeting m6A modification regulators could be a possibility, thereby proving its therapeutic potential in cancer treatment.
Liver cancer is the sixth most common cancer worldwide, including HCC and cholangiocarcinoma (CCA) subtypes. Recent studies have shown that m6A regulatory factor is closely related to the development of HCC and is expected to be a potential therapeutic target for HCC (Xu et al., 2019). Moreover, HCC is often too advanced for surgical treatment by the time it is diagnosed (Kong et al., 2022). SOR, a bisaryl urea multikinase inhibitor, is the first molecularly targeted drug approved by the Food and Drug Administration (FDA) for the clinical treatment of HCC (Kong et al., 2021). SOR has strong antitumour and antiangiogenic effects, effectively improving the survival rate of patients with advanced HCC (Palazzo et al., 2010; Iacovelli et al., 2015). However, during treatment, changes in epigenetic modifications occur due to the heterogeneity of HCC. This phenomenon suggests that acquired or primary SOR resistance is a major obstacle to the survival of patients with HCC (Zhu et al., 2017a). As an important member of RNA modification, m6A modification plays an important role in regulating drug resistance during HCC treatment. As the most famous m6A methyltransferase, METTL3 has been identified as a key regulator in many biological processes, including cell cycle, apoptosis, migration, invasion, differentiation and inflammatory response (Maldonado López and Capell, 2021). Kong et al. (2022) showed that the lncRNA LINC01273 can promote the resistance of HCC to SOR. LINC01273 increases the stability of miR-600 by acting as a “reservoir” and enhances the inhibition of miR-600 on METTL3 mRNA, resulting in the downregulation of METTL3 and drug resistance of HCC cells to SOR. Additionally, METTL3 increases the m6A level of LINC01273 and decreases the stability of LINC01273 in recognizing YTHDF2. Therefore, the dysregulation of the LINC01273/miR-600/METTL3 axis could be a potential cause of SOR resistance in HCC cells. Notably, METTL3 is often reported as an oncogene and is upregulated in most tumours (Zeng et al., 2020). This could be associated with the dual regulatory role of the m6A regulatory factor. Lin et al. (2020) also found that METTL3 expression is significantly downregulated in SOR-resistant HCC cells. The deletion or depletion of METTL3 promotes the expression of SOR drug resistance and angiogenesis genes and activates autophagy-related pathways. The downregulation of METTL3 expression leads to the decreased stability of FOXO3-mRNA, which promotes the resistance of HCC to SOR. This indicates that METTL3 is a negative regulator of SOR resistance and could be related to the bidirectional action of METTL3, and its internal mechanism is worth further study. Additionally, Xu et al. (2020b) showed that m6A modification promotes the drug resistance of HCC to SOR by regulating the expression level of circRNA-SORE, especially via an m6A modification site in circRNA-SORE. Furthermore, the m6A level of circRNA-SORE was increased in SOR-resistant HepG2 cells and the level of circRNA-SORE was significantly decreased on METTL3/14 knockout. These results indicated that m6A modification promotes HCC resistance to SOR through the circRNA expression level. Currently, SOR has become the first-line drug for patients with advanced liver cancer. Despite the wide use of SOR, it has certain disadvantages in its clinical application (Llovet et al., 2008; Cervello et al., 2012). Some patients acquire resistance to SOR in the course of treatment, which affects the overall survival time of patients with HCC (Zhu et al., 2017b). Therefore, further studies on the mechanism of drug resistance could improve and overcome this obstacle. The mechanism of m6A regulatory factors in HCC is shown in Figure 2 and Table 1.
FIGURE 2. The regulatory mechanism of m6A modification on chemotherapy resistance in HCC. METTL3, as an m6A writer, is down-regulated in HCC and regulated by LncRNA and miRNA. Meanwhile, METTL3 also regulates the stability of FOXO-mRNA and promotes the resistance of HCC to sorafenib.
Pancreatic cancer is one of the leading causes of cancer-related deaths in the Western world, owing to its advanced nature, early metastasis and limited response to chemotherapy or radiation. Adjuvant chemotherapy after surgical resection is the preferred treatment for early pancreatic cancer (Zeng et al., 2019). Although gemcitabine remains a cornerstone in the treatment of early-stage advanced pancreatic cancer, its clinical efficacy is poor due to molecular mechanisms, epigenetic modifications, limitations in cell uptake and activation and chemotherapeutic resistance development within weeks of treatment initiation (Amrutkar and Gladhaug, 2017). Current studies on the mechanism of drug resistance in pancreatic cancer report that m6A modification plays an important role in the drug resistance of pancreatic cancer to chemotherapy drugs. The deletion of METTL3, an m6A writer, enhances the sensitivity of pancreatic cancer cells to gemcitabine, 5-FU, cisplatin and radiotherapy. Furthermore, METTL3 could promote the resistance of pancreatic cancer to gemcitabine, 5-FU and cisplatin via several key pathways, including the MAPK cascade, ubiquitin-dependent processes, RNA splicing and cellular process regulation (Taketo et al., 2018). METTL14, another regulator of m6A writer, forms a functional heterodimer with METTL3, which is further catalysed and stabilised by WTAP, promoting the effect of m6A modification (Schöller et al., 2018). Furthermore, METTL14, one of the key methyltransferases, is an RNA-binding scaffold that recognizes the substrate of the m6A methyltransferase complex and has 20% sequence homology with METTL3. Among m6A methyltransferases, METTL14 is speculated to have a methyltransferase function that aids in RNA binding and METTL3 stabilisation (Wang et al., 2017). Kong et al. (2020a) showed that METTL14 is upregulated in pancreatic cancer tissues, with METTL14 knockdown in pancreatic cancer cells enhancing its sensitivity to cisplatin therapy. METTL14 regulates the sensitivity of pancreatic cancer cells to cisplatin treatment via the AMPKα, ERK1/2, and mTOR signalling pathways and improves autophagy via the mTOR signalling pathway. Additionally, METTL14 expression is closely associated with gemcitabine-resistant treatment and upregulated in gemcitabine-resistant human pancreatic cancer cells. METTL14 increases the expression of cytidine deaminase, an enzyme that inhibits gemcitabine. Therefore, METTL14 knockdown significantly increases the sensitivity of gemcitabine in drug-resistant cells (Zhang et al., 2021b). Although the expression of METTL14 is increased in drug-resistant pancreatic cancer cells, its expression regulation mechanism remains unclear. Therefore, further studies on the mechanism of METTL14 resistance are required.
ALKBH5, an m6A eraser, is downregulated in pancreatic cancer. The overexpression of ALKBH5 can inhibit the proliferation, migration and invasive activities of pancreatic cancer (Guo et al., 2020). Compared with existing tumour markers, ALKBH5 shows good prognostic ability, and its expression level is positively correlated with the prognosis of The Cancer Genome Atlas cohort patients (Cho et al., 2018). In patients with pancreatic cancer treated with gemcitabine, ALKBH5 expression is down regulated, whereas its overexpression induces the pancreatic cancer cells’ sensitivity to chemotherapy. The sensitivity of pancreatic cancer cells to gemcitabine is affected by the regulation of Wnt inhibitor 1 and the Wnt pathway (Tang et al., 2020). Unlike readers and writers, only two m6A demethylases, FTO and ALKBH5, are known that rely on Fe (II) and α-ketoglutaric acid (Dai et al., 2018). The downregulation of m6A promotes the resistance of FTO and ALKBH5 to PARPi. Moreover, m6A was confirmed to play an important regulatory role in treatments related to DNA damage response, including radiotherapy, chemotherapy and therapy targeting mutations related to DNA damage repair. Considering that the crystal structures of FTO and ALKBH5 have been determined, the development of drugs targeting FTO and ALKBH5 is a potential research direction (Han et al., 2010; Aik et al., 2014). The mechanism of the m6A regulatory factor in pancreatic cancer is shown in Figure 3 and Table 2.
FIGURE 3. The regulatory mechanism of m6A modification on chemotherapy resistance in pancreatic cancer. METTL3 and METTL14 are up-regulated in pancreatic cancer and promote drug resistance to gemcitabine, 5-fluorouracil and cisplatin through MAPK cascade, ubiquitin-dependent process, RNA splicing, AMPK α, ERK1/2, and mTOR signaling pathways. The down-regulated expression of ALKBH5 in pancreatic cancer affects the sensitivity of pancreatic cancer cells to gemcitabine by regulating Wnt inhibitor 1 and the Wnt pathway.
Radical surgical resection is the preferred treatment regimen for CRC, and radiotherapy and chemotherapy, as a routine treatment strategy after surgery, can effectively improve the survival of patients (Liu et al., 2019; Kong et al., 2020b). OX, a third-generation platinum drug, is widely used as a first-line chemotherapy agent for CRC. However, repeated long-term dosing induces chemotherapeutic resistance by increasing the expression of multidrug-resistant proteins, glutathione and excision repair cross-complement and promoting cell export and excision repair nucleotides (Allen and Johnston, 2005). Lan et al. (2021b) found that total m6A RNA content and critical methyltransferase METTL3 expression were increased in the CRC tissues of patients with OX resistance. The overexpression of METTL3 enhances the resistance of CRC cells to OX via TRAF5-mediated necrosis. Patients with CRC often develop resistance to 5-FU. Studies showed that miRNAs in exosomes secreted by cancer-associated fibroblasts (CAFs) are associated with sensitivity to 5-FU (Hu et al., 2019). METTL3 promotes miR-181d-5p secretion through the DiGeorge Syndrome Critical Region 8 (DGCR8) in CAFs. CAF-derived exosomes enhance 5-FU resistance in CRC cells via the METTL3/miR-181d-5p axis. Thus, a novel role of exosome miR-181d-5p secreted by CAFs was revealed. METTL3-dependent m6A methylation is upregulated in CRC, thereby promoting miR-181d-5p processing by DGCR8, resulting in increased miR-181d-5plevels, and inhibiting 5-FU sensitivity by targeting NCALD (Pan et al., 2022). METTL3 also promotes CRC resistance to 5-FU through circ-0000677 (Liu et al., 2022a). Additionally, METTL3 catalyses transition adenosine methylation and promotes pre-mRNA preferential splicing and p53 protein’s R273H mutation, leading to acquired drug resistance in colon cancer cells (Uddin et al., 2019).
Cisplatin is a platinum-based chemotherapy drug that has been clinically proven to treat various malignant tumours (Dasari and Tchounwou, 2014). Although cisplatin therapy has achieved good prognosis and survival rates in patients with cancer, problems of drug resistance and considerable side effects remain (Galluzzi et al., 2012). YTHDF1, an m6A “reader”, is an important regulator of tumour progression (Chen et al., 2021c). The expression of YTHDF1 is significantly upregulated in CRC, and its overexpression can reduce the sensitivity of colon cancer cells to cisplatin. YTHDF1 promotes the synthesis of GLS1 protein by binding to GLS1 3′UTR, which causes cisplatin resistance in colon cancer cells (Chen et al., 2021d). Yang et al. (2021) analysed CRC cells using proteomic and transcriptomic analyses to identify proteins involved in multidrug resistance in CRC. Results showed that IGF2BP3 expression was upregulated in CRC, whereas IGF2BP3 knockout significantly improved the sensitivity of CRC to adriamycin. Therefore, IGF2BP3 could be a potential biomarker to predict the occurrence of CRC multidrug resistance. Targeting IGF2BP3 could also be a potential chemotherapy strategy to prevent the development of multidrug resistance in CRC. FTO, an m6A eraser, blocks the ability of cancer stem cells through its N-6,2′-O-dimethyladenosine demethylase activity. The downregulation of FTO expression in CRC enhances the m6A modification level of mRNA, leading to increased tumorigenicity and chemotherapy resistance in vivo (Relier et al., 2021).
Immunotherapy and targeted therapy are current strategies for the treatment of CRC. Programmed cell death-1 (PD-1) checkpoint blocking immunotherapy has achieved impressive clinical success in treating various cancers (Lipson et al., 2015). However, limited or nonresponsive to PD-1 antibody therapy remains a challenge (Ganesh et al., 2019). In immunotherapy-resistant CRC, the deletion of METTL3 and METTL14 enhances the response of CRC and melanoma to PD-1 therapy. This could be attributed to the downregulated expression of METTL3 or METTL14, YTHDF2-stabilized STAT1 and Irf1 mRNA, activated IFN-γ–STAT1–IRF1 signalling pathway and enhanced sensitivity of CRC against PD-1 treatment (Wang et al., 2020b). Cetuximab is an FDA-approved monoclonal antibody against EGFR that is recommended for patients with metastatic CRC and wild-type KRAS/NRAS/BRAF tumours; however, its efficacy remains unsatisfactory, especially in patients with potentially metastatic CRC and adjuvant therapy progression (Benson et al., 2021). Additionally, PHLDB2 is upregulated in CRC and promotes the migration and invasion of cancer cells. METTL14 regulates PHLDB2 and promotes its expression, whereas PHLDB2 upregulation stabilises EGFR and promotes its nuclear translocation, leading to EGFR signal transduction activation and cetuximab resistance (Luo et al., 2022). Therefore, PHLDB2 is a potential therapeutic target for CRC. Hao et al. report that MIR100HG expression is closely related to the markers of epithelial–mesenchymal transformation in CRC and can serve as a positive regulator of epithelial–mesenchymal transformation. MIR100HG maintains cetuximab resistance in vitro and in vivo and promotes the invasion and metastasis of CRC cells. Furthermore, hnRNPA2B1 binds with MIR100HG and maintains the mRNA stability of TCF7L2. hnRNPA2B1 identifies the TCF7L2 mRNA m6A site via the MIR100HG hnRNPA2B1/TCF7L2 axis and enhances CRC resistance to cetuximab (Liu et al., 2022b). Therefore, targeted therapy combined with MIR100HG and immune checkpoint blockade could be a potential therapeutic strategy to improve the immunotherapy response of patients with CRC. The mechanism of m6A regulation in CRC is shown in Figure 4 and Table 3.
FIGURE 4. The regulatory mechanism of m6A modification on chemotherapy resistance in CRC. In CRC, m6A Writers, Erasers, and Readers are dysregulated and promote CRC resistance to cisplatin, 5-fluorouracil, oxaliplatin, doxorubicin, and targeted agents by regulating the stability of downstream gene expression.
Endoscopic resection is the standard treatment regimen for early gastric cancer. Non-inchoate operable gastric cancer is treated by surgery while sequential chemotherapy is used for advanced gastric cancer. The first-line treatment also includes platinum drugs and FU double chain (Smyth et al., 2020). OX, a first-line treatment for advanced gastric cancer, has been widely used in clinical settings; however, drug resistance mainly causes treatment failure (Boku et al., 2019; Harada et al., 2021). Li et al. (2022a) showed that CD133+ stem cell-like cells are the main subgroup of OX resistance whereas PARP1 is the central gene mediating OX resistance in gastric cancer. PARP1 can effectively repair the DNA damage caused by OX, leading to the occurrence of drug resistance. Moreover, METTL3 expression is upregulated in CD133+ stem cells. METTL3 recruits YTHDF1 to enhance the stability of PARP1 mRNA, thus playing a role in the repair of PARP1-mediated DNA damage and the development of OX resistance in gastric cancer cells. In addition to OX, cisplatin, either alone or in combination with other chemotherapeutic agents, is a first-line chemotherapy drug for patients with advanced gastric cancer (Kang et al., 2020). However, cisplatin resistance remains a major challenge in the treatment of advanced gastric cancer (Wang et al., 2016). Zhu et al. (2022) found that lncRNA LINC00942 is upregulated in gastric cancer and associated with poor prognosis. LINC00942 upregulates MSI2 expression by blocking the interaction between MSI2 and SCFβ−TrCP E3 ubiquitin ligase, ultimately inhibiting its ubiquitination. Subsequently, LINC00942 enhances C-Myc mRNA stability in an m6A-dependent manner and enhances cisplatin resistance in gastric cancer. Therefore, blocking the LINC00942–MSI2–C-Myc axis could be a novel therapeutic strategy for patients with chemotherapy-resistant gastric cancer. Another lncRNA, ARHGAP5-AS1, is upregulated in gastric cancer and associated with poor prognosis. ARHGAP5-AS1 enhances the stability of ARHGAP5 mRNA by recruiting METTL3 and modifying ARHGAP5 mRNA. Therefore, the upregulation of ARHGAP5 could promote cisplatin chemotherapy resistance in gastric cancer (Zhu et al., 2019b). Hence, targeting the ARHGAP5-AS1/ARHGAP5 axis is a promising strategy for overcoming chemotherapy resistance in gastric cancer. Recently, a special relationship between the tumour microenvironment infiltration of immune cells and m6A modification has been revealed, which cannot be explained by the mechanism of RNA degradation. Therefore, a comprehensive understanding of the characteristics of cell infiltration in the tumour microenvironment mediated by multiple m6A regulatory factors could aid in our understanding of the tumour microenvironment immune regulation. Zhang et al. (2020b) comprehensively analysed the m6A landscape associated with immunophenotype in 1,938 gastric cancer samples and constructed an m6A scoring system called the m6Ascore to quantify the m6A characteristics associated with immune cell infiltration in individual patients with GC. When the m6A score was low, the neoantigen load was increased and immune infiltration was high, indicating that the immune checkpoint blockade (PD-1 and PD-L1) has good clinical efficacy. Therefore, m6A modification plays an important role in the diversity and complexity of the tumour microenvironment. Additionally, Feng et al. (2021) speculate that proton pump inhibitors could be a promising therapeutic strategy to further improve the sensitivity of gastric cancer cells to antitumour drugs. For example, omeprazole pre-treatment can enhance the inhibitory effect of 5-FU, DDP and TAX on gastric cancer cells, increase the total m6A level of gastric cancer cells and inhibit autophagy, thereby improving the anti-tumour efficiency of chemotherapy drugs. The mechanism of m6A regulation in gastric cancer is shown in Table 4.
Esophageal cancer is a malignant tumour with a high degree of malignancy and mortality (Jain and Dhingra, 2017; Miller et al., 2020). m6A methylation is an important epigenetic modification involved in the physiological and pathological mechanisms of cancer. However, its role in esophageal cancer remains unclear. Current studies show that m6A modification plays a complex role in the occurrence, development and biological function of esophageal cancer, and it is a research hotspot in epigenetics. m6A modification also has therapeutic potential as an early diagnostic marker and therapeutic target in esophageal cancer. METTL3 has been identified as a decisive inducer of cancer progression, which is up-regulated in esophageal cancer and promotes epithelial-mesenchymal transformation, invasion and migration by regulating miR-20a-5p expression and inhibiting NFIC transcription (Liang et al., 2021). Furthermore, METTL3 was attributed to altered m6A levels in esophageal cancer, and its upregulation was significantly associated with cancer progression. Moreover, the deletion of METTL3 induces the G2/M arrest of esophageal cancer cells via the P21 signalling pathway (Zou et al., 2021). Therefore, METTL3 is a potential target molecule in esophageal cancer treatment. ALKBH5, an m6A regulatory factor, is down-regulated in esophageal cancer. The overexpression of ALKBH5 inhibits esophageal cancer cell proliferation and promotes ESCC cell apoptosis (Li et al., 2021a). Liu et al. revealed a METTL14-miR-99a-5p-TRIB2 positive feedback loop in esophageal cancer that enhances tumour stem cell characterisation and drug resistance of ESCC cells. METTL14 has been shown to play an antitumour role through its N6‐methyladenosine modification function. In esophageal cancer, METTL14 downregulation eliminated the inhibitory effect of Mir-99a-5p on TRIB2 expression by blocking Mir-99a-5p maturation, which subsequently increased the radiation-resistance of ESCC (Liu et al., 2021). Using METTL14’s effect on radiation therapy, Li et al. analysed 15 m6A regulatory factors and identified three new molecular subtypes associated with clinical features and esophageal cancer prognosis. By constructing a protein-protein interaction network for the three novel molecular subtypes and analysing their related genes, eight potential drugs (such as gefitinib, nalatinib, and imatinib) that closely interacted with these genes were identified. This study provides a valuable reference for identifying potential targets and drugs for esophageal cancer treatment (Li et al., 2022b). Currently, studies on m6A modification are limited to the interaction mechanism of esophageal cancer, and there is a lack of research on drug therapy. Therefore, further studies are needed to promote the application of m6A modification in clinical practice, such as the combination of m6A with chemotherapy and immunotherapy.
m6A is one of the richest modifications that determine the fate of RNA. Currently, m6A modification is closely related to tumorigenesis and plays an important role in the fate of tumour cells, including tumour proliferation and metastasis, tumour cell apoptosis, autophagy and iron death. The abnormal levels of m6A modification during the progression of apoptosis, autophagy, ferroptosis, necrosis and pyroptosis have been detected in gastrointestinal tumours (Zhi et al., 2022). Apoptosis, a type of cell death, is closely related to m6A modification (Zhi et al., 2022), wherein it regulates apoptosis by regulating apoptosis-related gene expression, silencing methylation or demethylase genes and reducing YTHDF2-mediated transcripts (Liu et al., 2022c). For example, METTL3 inhibits the apoptosis of non-small cell lung cancer (NSCLC) cells by promoting miR-1246 maturation and down-regulating PEG3 expression levels (Huang et al., 2021). In a recent study of lncRNAs containing m6A, LNC942 was observed to directly recruit METTL14, a core member of the m6A methyltransferase complex, and associated with increased levels of m6A methylation modification in breast cancer cells. Further, the LNC942-METTL14-CXCR4/CYP1B1 signal axis accelerated cell proliferation and colony formation, and reduced cell apoptosis rate (Sun et al., 2020).
Autophagy is a degradation process involving the lysosomal cytoplasmic content and autophagy-associated (ATG) proteins and transcription factors. It is also closely influenced by different stimulators and inhibitors. Autophagy can promote the resistance of tumour cells to chemotherapy and enable tumour cells to survive (Levy et al., 2017). Various studies have revealed the potential correlation between m6A modification and autophagy mechanism. Kong et al. found that METTL14 expression was higher in pancreatic cancer tissues than in non-tumour tissues, with METTL14 downregulation increasing the sensitivity of pancreatic cancer cells to cisplatin. Compared with the control group, the apoptosis and autophagy of tumour cells were significantly enhanced after METT14 gene knockout (Kong et al., 2020a). YTHDF1 expression is an independent prognostic factor for patients with HCC. Multiple HCC models confirmed that YTHDF1 cannot inhibit the autophagy, growth and metastasis of HCC (Li et al., 2021b). These findings highlight the interaction between autophagy and m6A regulators, but the relationship between m6A and autophagy remains unclear.
Ferroptosis is a novel pro-inflammatory programmed cell death pathway that plays a key role in the clearance of malignant cells. It is caused by the inhibition of the xCT/GSH/GPX4 axis and characterised by iron hyperplasia, lipid peroxidation and the compression of mitochondrial membrane density (Mou et al., 2019). Current studies on ferroptosis modified with m6A have focused on the interference of reader and writer factors on lipid peroxidation or antioxidant enzymes. However, few studies have detected the level of m6A erasers and their correlation with abnormal ferroptosis execution in cancer cells. In NSCLC, METTL3 is involved in cisplatin-mediated ferroptosis via m6A enrichment in FSP1 mRNA (Song et al., 2021). Thus, the relationship between m6A modification and iron death suggests that targeting m6A to induce ferrous iron death could be a promising therapeutic strategy.
m6A RNA modification has attracted attention in epigenetic research and is involved in many biological processes and disease progressions. From the perspective of epigenetics, m6A modification provides novel insights into the pathogenesis of many diseases, especially tumours. However, further studies are required to understand the dynamic nature of m6A RNA modification in post-transcriptional regulation (Chen and Wong, 2020). m6A RNA modification plays an important role in promoting or inhibiting the growth, proliferation, migration, invasion, specific metastasis, drug resistance and prognosis of digestive tumours through three effector factors, writers, erasers and readers. With increasing studies on the network mechanism of m6A modification regulation, the related mechanism of m6A modification on tumour drug resistance will be clarified (Qiu et al., 2022).
Multiple studies showed that m6A-modified regulatory factors are resistant to SOR in HCC. In CRC, OX, cisplatin and other drug resistance are observed. Resistant to gemcitabine, 5-FU and cisplatin are also observed in pancreatic cancer. Additionally, m6A plays an important role in the regulation of OX and cisplatin resistance in gastric cancer. However, these studies are preliminary, requiring more systematic studies. Furthermore, translational studies are needed to further clarify the use of m6A alone or in combination with other therapies for the treatment of digestive tumours.
Immunotherapy is a new cancer treatment strategy that has been widely used to treat various solid tumours, including various digestive tract tumours and other solid tumours (Kong et al., 2021; Lee et al., 2021). In recent years, promising progress has been made in tumour immunotherapy with m6A modification, among which, ICIs harness the patient’s immune system, offering a novel method of cancer treatment. However, immunotherapy, such as ICIs, also presents drug resistance in some patients. For example, from the perspective of an m6A “writer”, Wang et al. found that the deletion of methyltransferases METTL3 and METTL14 inhibits m6A modification and enhances pMR-MSI-L response to PD-1 therapy in patients with CRC and melanoma, significantly delaying tumour growth and prolonging patient survival Wang et al. (2020b). However, large-scale basic research is needed to further clarify the specific mechanism of m6A modification in immunotherapy.
m6A plays various roles in different tumour types, suggesting the complexity and diversity of m6A modifications in drug resistance. Recent studies also showed that m6A-modified regulatory factors have potential as therapeutic targets and can enhance the sensitivity of tumour cells to anticancer drugs, providing a new research direction in solving the problem of anticancer drug resistance. This review also highlights the positive prospects of targeted m6A modifications.
The original contributions presented in the study are included in the article/supplementary material, further inquiries can be directed to the corresponding author.
ZX, FK, and XZ contributed to conceive and design the study. ZX and KW performed the systematic searching. ZX and FK extracted the data. ZX and XZ wrote the manuscript. KW and FK supervised the manuscript. All of the authors read and approved the final manuscript.
This project was supported by the grants from Science and Technology Plan Project of Taizhou (Nos. 21ywb36 and 20ywb41).
The authors declare that the research was conducted in the absence of any commercial or financial relationships that could be construed as a potential conflict of interest.
All claims expressed in this article are solely those of the authors and do not necessarily represent those of their affiliated organizations, or those of the publisher, the editors and the reviewers. Any product that may be evaluated in this article, or claim that may be made by its manufacturer, is not guaranteed or endorsed by the publisher.
Aik, W., Scotti, J. S., Choi, H., Gong, L., Demetriades, M., Schofield, C. J., et al. (2014). Structure of Human RNA N⁶-methyladenine Demethylase ALKBH5 Provides Insights into its Mechanisms of Nucleic Acid Recognition and Demethylation. Nucleic Acids Res. 42 (7), 4741–4754. doi:10.1093/nar/gku085
Al-Salama, Z. T., Syed, Y. Y., and Scott, L. J. (2019). Lenvatinib: A Review in Hepatocellular Carcinoma. Drugs 79 (6), 665–674. doi:10.1007/s40265-019-01116-x
Allen, W. L., and Johnston, P. G. (2005). Role of Genomic Markers in Colorectal Cancer Treatment. J. Clin. Oncol. 23 (20), 4545–4552. doi:10.1200/jco.2005.19.752
Amrutkar, M., and Gladhaug, I. P. (2017). Pancreatic Cancer Chemoresistance to Gemcitabine. Cancers (Basel) 9 (11). doi:10.3390/cancers9110157
Annapoorna, P. K., Iyer, H., Parnaik, T., Narasimhan, H., Bhattacharya, A., and Kumar, A. (2019). FTO: An Emerging Molecular Player in Neuropsychiatric Diseases. Neuroscience 418, 15–24. doi:10.1016/j.neuroscience.2019.08.021
Bansal, H., Yihua, Q., Iyer, S. P., Ganapathy, S., Proia, D. A., Proia, D., et al. (2014). WTAP Is a Novel Oncogenic Protein in Acute Myeloid Leukemia. Leukemia 28 (5), 1171–1174. doi:10.1038/leu.2014.16
Benson, A. B., Venook, A. P., Al-Hawary, M. M., Arain, M. A., Chen, Y. J., Ciombor, K. K., et al. (2021). Colon Cancer, Version 2.2021, NCCN Clinical Practice Guidelines in Oncology. J. Natl. Compr. Canc Netw. 19 (3), 329–359. doi:10.6004/jnccn.2021.0012
Boku, N., Ryu, M. H., Kato, K., Chung, H. C., Minashi, K., Lee, K. W., et al. (2019). Safety and Efficacy of Nivolumab in Combination with S-1/capecitabine Plus Oxaliplatin in Patients with Previously Untreated, Unresectable, Advanced, or Recurrent Gastric/gastroesophageal Junction Cancer: Interim Results of a Randomized, Phase II Trial (ATTRACTION-4). Ann. Oncol. 30 (2), 250–258. doi:10.1093/annonc/mdy540
Cervello, M., McCubrey, J. A., Cusimano, A., Lampiasi, N., Azzolina, A., and Montalto, G. (2012). Targeted Therapy for Hepatocellular Carcinoma: Novel Agents on the Horizon. Oncotarget 3 (3), 236–260. doi:10.18632/oncotarget.466
Chen, B., Xiong, L., Chen, W. D., Zhao, X. H., He, J., Zheng, Y. W., et al. (2018). Photodynamic Therapy for Middle-Advanced Stage Upper Gastrointestinal Carcinomas: A Systematic Review and Meta-Analysis. World J. Clin. Cases 6 (13), 650–658. doi:10.12998/wjcc.v6.i13.650
Chen, B., Ye, F., Yu, L., Jia, G., Huang, X., Zhang, X., et al. (2012). Development of Cell-Active N6-Methyladenosine RNA Demethylase FTO Inhibitor. J. Am. Chem. Soc. 134 (43), 17963–17971. doi:10.1021/ja3064149
Chen, M., and Wong, C. M. (2020). The Emerging Roles of N6-Methyladenosine (m6A) Deregulation in Liver Carcinogenesis. Mol. Cancer 19 (1), 44. doi:10.1186/s12943-020-01172-y
Chen, P., Liu, X. Q., Lin, X., Gao, L. Y., Zhang, S., and Huang, X. (2021). Targeting YTHDF1 Effectively Re-sensitizes Cisplatin-Resistant Colon Cancer Cells by Modulating GLS-Mediated Glutamine Metabolism. Mol. Ther. Oncolytics 20, 228–239. doi:10.1016/j.omto.2021.01.001
Chen, W., Zhang, Z., Fang, X., Xiong, L., Wen, Y., Zhou, J., et al. (2021). Prognostic Value of the ALBI Grade Among Patients with Single Hepatocellular Carcinoma without Macrovascular Invasion. Med. Baltim. 100 (24), e26265. doi:10.1097/md.0000000000026265
Chen, Y., Wu, R., Chen, W., Liu, Y., Liao, X., Zeng, B., et al. (2021). Curcumin Prevents Obesity by Targeting TRAF4‐induced Ubiquitylation in M 6 A‐dependent Manner. EMBO Rep. 22 (5), e52146. doi:10.15252/embr.202052146
Chen, Z., Zhong, X., Xia, M., and Zhong, J. (2021). The Roles and Mechanisms of the m6A Reader Protein YTHDF1 in Tumor Biology and Human Diseases. Mol. Ther. Nucleic Acids 26, 1270–1279. doi:10.1016/j.omtn.2021.10.023
Chesney, T. R., Haas, B., Coburn, N., Mahar, A. L., Davis, L. E., Zuk, V., et al. (2021). Association of Frailty with Long-Term Homecare Utilization in Older Adults Following Cancer Surgery: Retrospective Population-Based Cohort Study. Eur. J. Surg. Oncol. 47 (4), 888–895. doi:10.1016/j.ejso.2020.09.009
Cho, S. H., Ha, M., Cho, Y. H., Ryu, J. H., Yang, K., Lee, K. H., et al. (2018). ALKBH5 Gene Is a Novel Biomarker that Predicts the Prognosis of Pancreatic Cancer: A Retrospective Multicohort Study. Ann. Hepatobiliary Pancreat. Surg. 22 (4), 305–309. doi:10.14701/ahbps.2018.22.4.305
Dagogo-Jack, I., and Shaw, A. T. (2018). Tumour Heterogeneity and Resistance to Cancer Therapies. Nat. Rev. Clin. Oncol. 15 (2), 81–94. doi:10.1038/nrclinonc.2017.166
Dai, D., Wang, H., Zhu, L., Jin, H., and Wang, X. (2018). N6-methyladenosine Links RNA Metabolism to Cancer Progression. Cell. Death Dis. 9 (2), 124. doi:10.1038/s41419-017-0129-x
Dasari, S., and Tchounwou, P. B. (2014). Cisplatin in Cancer Therapy: Molecular Mechanisms of Action. Eur. J. Pharmacol. 740, 364–378. doi:10.1016/j.ejphar.2014.07.025
Dekker, E., Tanis, P. J., Vleugels, J. L. A., Kasi, P. M., and Wallace, M. B. (2019). Colorectal Cancer. Lancet 394 (10207), 1467–1480. doi:10.1016/s0140-6736(19)32319-0
Doxtader, K. A., Wang, P., Scarborough, A. M., Seo, D., Conrad, N. K., and Nam, Y. (2018). Structural Basis for Regulation of METTL16, an S-Adenosylmethionine Homeostasis Factor. Mol. Cell. 71 (6), 1001–e4. e4. doi:10.1016/j.molcel.2018.07.025
Feng, S., Qiu, G., Yang, L., Feng, L., Fan, X., Ren, F., et al. (2021). Omeprazole Improves Chemosensitivity of Gastric Cancer Cells by m6A Demethylase FTO-Mediated Activation of mTORC1 and DDIT3 Up-Regulation. Biosci. Rep. 41 (1). doi:10.1042/bsr20200842
Galluzzi, L., Senovilla, L., Vitale, I., Michels, J., Martins, I., Kepp, O., et al. (2012). Molecular Mechanisms of Cisplatin Resistance. Oncogene 31 (15), 1869–1883. doi:10.1038/onc.2011.384
Gan, Z., Wei, W., Wu, J., Zhao, Y., Zhang, L., Wang, T., et al. (2019). Resveratrol and Curcumin Improve Intestinal Mucosal Integrity and Decrease m6A RNA Methylation in the Intestine of Weaning Piglets. ACS Omega 4 (17), 17438–17446. doi:10.1021/acsomega.9b02236
Ganesh, K., Stadler, Z. K., Cercek, A., Mendelsohn, R. B., Shia, J., Segal, N. H., et al. (2019). Immunotherapy in Colorectal Cancer: Rationale, Challenges and Potential. Nat. Rev. Gastroenterol. Hepatol. 16 (6), 361–375. doi:10.1038/s41575-019-0126-x
Gerken, T., Girard, C. A., Tung, Y. C., Webby, C. J., Saudek, V., Hewitson, K. S., et al. (2007). The Obesity-Associated FTO Gene Encodes a 2-oxoglutarate-dependent Nucleic Acid Demethylase. Science 318 (5855), 1469–1472. doi:10.1126/science.1151710
Geuens, T., Bouhy, D., and Timmerman, V. (2016). The hnRNP Family: Insights into Their Role in Health and Disease. Hum. Genet. 135 (8), 851–867. doi:10.1007/s00439-016-1683-5
Ghazi, T., Nagiah, S., and Chuturgoon, A. A. (2021). Fusaric Acid Decreases P53 Expression by Altering Promoter Methylation and m6A RNA Methylation in Human Hepatocellular Carcinoma (HepG2) Cells. Epigenetics 16 (1), 79–91. doi:10.1080/15592294.2020.1788324
Guo, X., Li, K., Jiang, W., Hu, Y., Xiao, W., Huang, Y., et al. (2020). RNA Demethylase ALKBH5 Prevents Pancreatic Cancer Progression by Posttranscriptional Activation of PER1 in an m6A-YTHDF2-dependent Manner. Mol. Cancer 19 (1), 91. doi:10.1186/s12943-020-01158-w
Han, Z., Niu, T., Chang, J., Lei, X., Zhao, M., Wang, Q., et al. (2010). Crystal Structure of the FTO Protein Reveals Basis for its Substrate Specificity. Nature 464 (7292), 1205–1209. doi:10.1038/nature08921
Harada, K., Sakamoto, N., Ukai, S., Yamamoto, Y., Pham, Q. T., Taniyama, D., et al. (2021). Establishment of Oxaliplatin-Resistant Gastric Cancer Organoids: Importance of Myoferlin in the Acquisition of Oxaliplatin Resistance. Gastric Cancer 24 (6), 1264–1277. doi:10.1007/s10120-021-01206-4
He, L., Li, H., Wu, A., Peng, Y., Shu, G., and Yin, G. (2019). Functions of N6-Methyladenosine and its Role in Cancer. Mol. Cancer 18 (1), 176. doi:10.1186/s12943-019-1109-9
Hsu, P. J., Zhu, Y., Ma, H., Guo, Y., Shi, X., Liu, Y., et al. (2017). Ythdc2 Is an N6-Methyladenosine Binding Protein that Regulates Mammalian Spermatogenesis. Cell. Res. 27 (9), 1115–1127. doi:10.1038/cr.2017.99
Hu, J. L., Wang, W., Lan, X. L., Zeng, Z. C., Liang, Y. S., Yan, Y. R., et al. (2019). CAFs Secreted Exosomes Promote Metastasis and Chemotherapy Resistance by Enhancing Cell Stemness and Epithelial-Mesenchymal Transition in Colorectal Cancer. Mol. Cancer 18 (1), 91. doi:10.1186/s12943-019-1019-x
Hu, Y., Ouyang, Z., Sui, X., Qi, M., Li, M., He, Y., et al. (2020). Oocyte Competence Is Maintained by m6A Methyltransferase KIAA1429-Mediated RNA Metabolism during Mouse Follicular Development. Cell. Death Differ. 27 (8), 2468–2483. doi:10.1038/s41418-020-0516-1
Huang, H., Weng, H., and Chen, J. (2020). The Biogenesis and Precise Control of RNA m6A Methylation. Trends Genet. 36 (1), 44–52. doi:10.1016/j.tig.2019.10.011
Huang, S., Luo, S., Gong, C., Liang, L., Xiao, Y., Li, M., et al. (2021). MTTL3 Upregulates microRNA-1246 to Promote Occurrence and Progression of NSCLC via Targeting Paternally Expressed Gene 3. Mol. Ther. Nucleic Acids 24, 542–553. doi:10.1016/j.omtn.2021.02.020
Huang, W., Kong, F., Li, R., Chen, X., and Wang, K. (2022). Emerging Roles of m6A RNA Methylation Regulators in Gynecological Cancer. Front. Oncol. 12, 827956. doi:10.3389/fonc.2022.827956
Huff, S., Tiwari, S. K., Gonzalez, G. M., Wang, Y., and Rana, T. M. (2021). m6A-RNA Demethylase FTO Inhibitors Impair Self-Renewal in Glioblastoma Stem CellsA-RNA Demethylase FTO Inhibitors Impair Self-Renewal in Glioblastoma Stem Cells. ACS Chem. Biol. 16 (2), 324–333. doi:10.1021/acschembio.0c00841
Huo, F. C., Zhu, Z. M., and Pei, D. S. (2020). N6 -methyladenosine (M6 A) RNA Modification in Human Cancer. Cell. Prolif. 53 (11), e12921. doi:10.1111/cpr.12921
Iacovelli, R., Sternberg, C. N., Porta, C., Verzoni, E., de Braud, F., Escudier, B., et al. (2015). Inhibition of the VEGF/VEGFR Pathway Improves Survival in Advanced Kidney Cancer: a Systematic Review and Meta-Analysis. Curr. Drug Targets 16 (2), 164–170. doi:10.2174/1389450115666141120120145
Jain, S., and Dhingra, S. (2017). Pathology of Esophageal Cancer and Barrett's Esophagus. Ann. Cardiothorac. Surg. 6 (2), 99–109. doi:10.21037/acs.2017.03.06
Jin, D., Guo, J., Wu, Y., Yang, L., Wang, X., Du, J., et al. (2020). m6A Demethylase ALKBH5 Inhibits Tumor Growth and Metastasis by Reducing YTHDFs-Mediated YAP Expression and Inhibiting miR-107/lats2-Mediated YAP Activity in NSCLCA Demethylase ALKBH5 Inhibits Tumor Growth and Metastasis by Reducing YTHDFs-Mediated YAP Expression and Inhibiting miR-107/lats2-Mediated YAP Activity in NSCLC. Mol. Cancer 19 (1), 40. doi:10.1186/s12943-020-01161-1
Johnston, F. M., and Beckman, M. (2019). Updates on Management of Gastric Cancer. Curr. Oncol. Rep. 21 (8), 67. doi:10.1007/s11912-019-0820-4
Kang, Y. K., Chin, K., Chung, H. C., Kadowaki, S., Oh, S. C., Nakayama, N., et al. (2020). S-1 Plus Leucovorin and Oxaliplatin versus S-1 Plus Cisplatin as First-Line Therapy in Patients with Advanced Gastric Cancer (SOLAR): a Randomised, Open-Label, Phase 3 Trial. Lancet Oncol. 21 (8), 1045–1056. doi:10.1016/s1470-2045(20)30315-6
Kant, R., Yang, M. H., Tseng, C. H., Yen, C. H., Li, W. Y., Tyan, Y. C., et al. (2021). Discovery of an Orally Efficacious MYC Inhibitor for Liver Cancer Using a GNMT-Based High-Throughput Screening System and Structure-Activity Relationship Analysis. J. Med. Chem. 64 (13), 8992–9009. doi:10.1021/acs.jmedchem.1c00093
Kong, F., Liu, X., Zhou, Y., Hou, X., He, J., Li, Q., et al. (2020). Downregulation of METTL14 Increases Apoptosis and Autophagy Induced by Cisplatin in Pancreatic Cancer Cells. Int. J. Biochem. Cell. Biol. 122, 105731. doi:10.1016/j.biocel.2020.105731
Kong, F., Zou, H., Liu, X., He, J., Zheng, Y., Xiong, L., et al. (2020). miR-7112-3p Targets PERK to Regulate the Endoplasmic Reticulum Stress Pathway and Apoptosis Induced by Photodynamic Therapy in Colorectal Cancer CX-1 Cells. Photodiagnosis Photodyn. Ther. 29, 101663. doi:10.1016/j.pdpdt.2020.101663
Kong, F. H., Miao, X. Y., Zou, H., Xiong, L., Wen, Y., Chen, B., et al. (2019). End-stage Liver Disease Score and Future Liver Remnant Volume Predict Post-hepatectomy Liver Failure in Hepatocellular Carcinoma. World J. Clin. Cases 7 (22), 3734–3741. doi:10.12998/wjcc.v7.i22.3734
Kong, F. H., Ye, Q. F., Miao, X. Y., Liu, X., Huang, S. Q., Xiong, L., et al. (2021). Current Status of Sorafenib Nanoparticle Delivery Systems in the Treatment of Hepatocellular Carcinoma. Theranostics 11 (11), 5464–5490. doi:10.7150/thno.54822
Kong, H., Sun, J., Zhang, W., Zhang, H., and Li, H. (2022). Long Intergenic Non-protein Coding RNA 1273 Confers Sorafenib Resistance in Hepatocellular Carcinoma via Regulation of Methyltransferase 3. Bioengineered 13 (2), 3108–3121. doi:10.1080/21655979.2022.2025701
Lan, H., Liu, Y., Liu, J., Wang, X., Guan, Z., Du, J., et al. (2021). Tumor-Associated Macrophages Promote Oxaliplatin Resistance via METTL3-Mediated m6A of TRAF5 and Necroptosis in Colorectal Cancer. Mol. Pharm. 18 (3), 1026–1037. doi:10.1021/acs.molpharmaceut.0c00961
Lan, L., Sun, Y. J., Jin, X. Y., Xie, L. J., Liu, L., and Cheng, L. (2021). A Light-Controllable Chemical Modulation of M6 A RNA Methylation. Angew. Chem. Int. Ed. Engl. 60 (33), 18116–18121. doi:10.1002/anie.202103854
Lee, J. C., Green, M. D., Huppert, L. A., Chow, C., Pierce, R. H., and Daud, A. I. (2021). The Liver-Immunity Nexus and Cancer Immunotherapy. Clin. Cancer Res. 28, 5–12. doi:10.1158/1078-0432.ccr-21-1193
Levy, J. M. M., Towers, C. G., and Thorburn, A. (2017). Targeting Autophagy in Cancer. Nat. Rev. Cancer 17 (9), 528–542. doi:10.1038/nrc.2017.53
Li, H., Wang, C., Lan, L., Yan, L., Li, W., Evans, I., et al. (2022). METTL3 Promotes Oxaliplatin Resistance of Gastric Cancer CD133+ Stem Cells by Promoting PARP1 mRNA Stability. Cell. Mol. Life Sci. 79 (3), 135. doi:10.1007/s00018-022-04129-0
Li, J., Liu, H., Dong, S., Zhang, Y., Li, X., and Wang, J. (2021). ALKBH5 Is Lowly Expressed in Esophageal Squamous Cell Carcinoma and Inhibits the Malignant Proliferation and Invasion of Tumor Cells. Comput. Math. Methods Med. 2021, 1001446. doi:10.1155/2021/1001446
Li, J., Zhu, H., Yang, Q., Xiao, H., Wu, H., Fang, Z., et al. (2022). Identification of Molecular Subtypes and Potential Small-Molecule Drugs for Esophagus Cancer Treatment Based on m6A Regulators. J. Oncol. 2022, 5490461. doi:10.1155/2022/5490461
Li, Q., Ni, Y., Zhang, L., Jiang, R., Xu, J., Yang, H., et al. (2021). HIF-1α-induced Expression of m6A Reader YTHDF1 Drives Hypoxia-Induced Autophagy and Malignancy of Hepatocellular Carcinoma by Promoting ATG2A and ATG14 Translation. Signal Transduct. Target Ther. 6 (1), 76. doi:10.1038/s41392-020-00453-8
Liang, X., Zhang, Z., Wang, L., Zhang, S., Ren, L., Li, S., et al. (2021). Mechanism of Methyltransferase like 3 in Epithelial-Mesenchymal Transition Process, Invasion, and Metastasis in Esophageal Cancer. Bioengineered 12 (2), 10023–10036. doi:10.1080/21655979.2021.1994721
Lin, Z., Niu, Y., Wan, A., Chen, D., Liang, H., Chen, X., et al. (2020). RNA M6 A Methylation Regulates Sorafenib Resistance in Liver Cancer through FOXO3-Mediated Autophagy. Embo J. 39 (12), e103181. doi:10.15252/embj.2019103181
Lipson, E. J., Forde, P. M., Hammers, H. J., Emens, L. A., Taube, J. M., and Topalian, S. L. (2015). Antagonists of PD-1 and PD-L1 in Cancer Treatment. Semin. Oncol. 42 (4), 587–600. doi:10.1053/j.seminoncol.2015.05.013
Liu, H., Li, D., Sun, L., Qin, H., Fan, A., Meng, L., et al. (2022). Interaction of lncRNA MIR100HG with hnRNPA2B1 Facilitates m6A-dependent Stabilization of TCF7L2 mRNA and Colorectal Cancer Progression. Mol. Cancer 21 (1), 74. doi:10.1186/s12943-022-01555-3
Liu, L., Li, H., Hu, D., Wang, Y., Shao, W., Zhong, J., et al. (2022). Insights into N6-Methyladenosine and Programmed Cell Death in Cancer. Mol. Cancer 21 (1), 32. doi:10.1186/s12943-022-01508-w
Liu, Q., Huang, Q., Liu, H., He, F. J., Liu, J. H., Zhou, Y. Y., et al. (2022). SUMOylation of Methyltransferase‐like 3 Facilitates Colorectal Cancer Progression by Promoting Circ_0000677 in an M 6 A‐dependent Manner. J Gastro Hepatol 37, 700–713. doi:10.1111/jgh.15775
Liu, X., Hou, X., Zhou, Y., Li, Q., Kong, F., Yan, S., et al. (2019). Downregulation of the Helicase Lymphoid-specific (HELLS) Gene Impairs Cell Proliferation and Induces Cell Cycle Arrest in Colorectal Cancer Cells. Onco Targets Ther. 12, 10153–10163. doi:10.2147/ott.s223668
Liu, Z., Wu, K., Gu, S., Wang, W., Xie, S., Lu, T., et al. (2021). A Methyltransferase-like 14/miR-99a-5p/tribble 2 Positive Feedback Circuit Promotes Cancer Stem Cell Persistence and Radioresistance via Histone Deacetylase 2-mediated Epigenetic Modulation in Esophageal Squamous Cell Carcinoma. Clin. Transl. Med. 11 (9), e545. doi:10.1002/ctm2.545
Llovet, J. M., Kelley, R. K., Villanueva, A., Singal, A. G., Pikarsky, E., Roayaie, S., et al. (2021). Hepatocellular Carcinoma. Nat. Rev. Dis. Prim. 7 (1), 6. doi:10.1038/s41572-020-00240-3
Llovet, J. M., Montal, R., Sia, D., and Finn, R. S. (2018). Molecular Therapies and Precision Medicine for Hepatocellular Carcinoma. Nat. Rev. Clin. Oncol. 15 (10), 599–616. doi:10.1038/s41571-018-0073-4
Llovet, J. M., Ricci, S., Mazzaferro, V., Hilgard, P., Gane, E., Blanc, J. F., et al. (2008). Sorafenib in Advanced Hepatocellular Carcinoma. N. Engl. J. Med. 359 (4), 378–390. doi:10.1056/NEJMoa0708857
Lu, J. J., and Wang, Y. T. (2020). Identification of Anti-cancer Compounds from Natural Products. Chin. J. Nat. Med. 18 (7), 481–482. doi:10.1016/s1875-5364(20)30057-1
Luo, M., Huang, Z., Yang, X., Chen, Y., Jiang, J., Zhang, L., et al. (2022). PHLDB2 Mediates Cetuximab Resistance via Interacting with EGFR in Latent Metastasis of Colorectal Cancer. Cell. Mol. Gastroenterol. Hepatol. 13 (4), 1223–1242. doi:10.1016/j.jcmgh.2021.12.011
Ma, S., Chen, C., Ji, X., Liu, J., Zhou, Q., Wang, G., et al. (2019). The Interplay between m6A RNA Methylation and Noncoding RNA in Cancer. J. Hematol. Oncol. 12 (1), 121. doi:10.1186/s13045-019-0805-7
Machlowska, J., Baj, J., Sitarz, M., Maciejewski, R., and Sitarz, R. (2020). Gastric Cancer: Epidemiology, Risk Factors, Classification, Genomic Characteristics and Treatment Strategies. Int. J. Mol. Sci. 21 (11). doi:10.3390/ijms21114012
Maldonado López, A., and Capell, B. C. (2021). The METTL3-m6A Epitranscriptome: Dynamic Regulator of Epithelial Development, Differentiation, and Cancer. Genes. 12 (7), 1019. doi:10.3390/genes12071019
Miller, K. D., Fidler-Benaoudia, M., Keegan, T. H., Hipp, H. S., Jemal, A., and Siegel, R. L. (2020). Cancer Statistics for Adolescents and Young Adults, 2020. CA Cancer J. Clin. 70 (1), 443–459. doi:10.3322/caac.2159010.3322/caac.21637
Mizrahi, J. D., Surana, R., Valle, J. W., and Shroff, R. T. (2020). Pancreatic Cancer. Lancet 395 (10242), 2008–2020. doi:10.1016/s0140-6736(20)30974-0
Mou, Y., Wang, J., Wu, J., He, D., Zhang, C., Duan, C., et al. (2019). Ferroptosis, a New Form of Cell Death: Opportunities and Challenges in Cancer. J. Hematol. Oncol. 12 (1), 34. doi:10.1186/s13045-019-0720-y
Palazzo, A., Iacovelli, R., and Cortesi, E. (2010). Past, Present and Future of Targeted Therapy in Solid Tumors. Curr. Cancer Drug Targets 10 (5), 433–461. doi:10.2174/156800910791517145
Pan, S., Deng, Y., Fu, J., Zhang, Y., Zhang, Z., and Qin, X. (2022). N6-methyladenosine U-pregulates miR-181d-5p in E-xosomes D-erived from C-ancer-associated F-ibroblasts to I-nhibit 5-FU S-ensitivity by T-argeting NCALD in C-olorectal C-ancer. Int. J. Oncol. 60 (2). doi:10.3892/ijo.2022.5304
Paul, D., Sanap, G., Shenoy, S., Kalyane, D., Kalia, K., and Tekade, R. K. (2021). Artificial Intelligence in Drug Discovery and Development. Drug Discov. Today 26 (1), 80–93. doi:10.1016/j.drudis.2020.10.010
Pavitra, E., Dariya, B., Srivani, G., Kang, S. M., Alam, A., Sudhir, P. R., et al. (2021). Engineered Nanoparticles for Imaging and Drug Delivery in Colorectal Cancer. Semin. Cancer Biol. 69, 293–306. doi:10.1016/j.semcancer.2019.06.017
Peng, S., Xiao, W., Ju, D., Sun, B., Hou, N., Liu, Q., et al. (2019). Identification of Entacapone as a Chemical Inhibitor of FTO Mediating Metabolic Regulation through FOXO1. Sci. Transl. Med. 11 (488), 11. doi:10.1126/scitranslmed.aau7116
Qiu, X., Li, X., Yan, Y., Cai, Y., Liang, Q., Peng, B., et al. (2022). Identification of m6A-Associated Gene DST as a Prognostic and Immune-Associated Biomarker in Breast Cancer Patients. Int. J. Gen. Med. 15, 523–534. doi:10.2147/ijgm.s344146
Qu, J., Yan, H., Hou, Y., Cao, W., Liu, Y., Zhang, E., et al. (2022). RNA Demethylase ALKBH5 in Cancer: from Mechanisms to Therapeutic Potential. J. Hematol. Oncol. 15 (1), 8. doi:10.1186/s13045-022-01224-4
Rafalska, I., Zhang, Z., Benderska, N., Wolff, H., Hartmann, A. M., Brack-Werner, R., et al. (2004). The Intranuclear Localization and Function of YT521-B Is Regulated by Tyrosine Phosphorylation. Hum. Mol. Genet. 13 (15), 1535–1549. doi:10.1093/hmg/ddh167
Relier, S., Ripoll, J., Guillorit, H., Amalric, A., Achour, C., Boissière, F., et al. (2021). FTO-mediated Cytoplasmic m6Am Demethylation Adjusts Stem-like Properties in Colorectal Cancer Cell. Nat. Commun. 12 (1), 1716. doi:10.1038/s41467-021-21758-4
Sawicki, T., Ruszkowska, M., Danielewicz, A., Niedźwiedzka, E., Arłukowicz, T., and Przybyłowicz, K. E. (2021). A Review of Colorectal Cancer in Terms of Epidemiology, Risk Factors, Development, Symptoms and Diagnosis. Cancers (Basel) 13 (9). doi:10.3390/cancers13092025
Schizas, D., Charalampakis, N., Kole, C., Economopoulou, P., Koustas, E., Gkotsis, E., et al. (2020). Immunotherapy for Pancreatic Cancer: A 2020 Update. Cancer Treat. Rev. 86, 102016. doi:10.1016/j.ctrv.2020.102016
Schöller, E., Weichmann, F., Treiber, T., Ringle, S., Treiber, N., Flatley, A., et al. (2018). Interactions, Localization, and Phosphorylation of the m6A Generating METTL3-METTL14-WTAP Complex. Rna 24 (4), 499–512. doi:10.1261/rna.064063.117
Seebacher, N. A., Stacy, A. E., Porter, G. M., and Merlot, A. M. (2019). Clinical Development of Targeted and Immune Based Anti-cancer Therapies. J. Exp. Clin. Cancer Res. 38 (1), 156. doi:10.1186/s13046-019-1094-2
Shen, H., Lan, Y., Zhao, Y., Shi, Y., Jin, J., and Xie, W. (2020). The Emerging Roles of N6-Methyladenosine RNA Methylation in Human Cancers. Biomark. Res. 8, 24. doi:10.1186/s40364-020-00203-6
Shi, R., Ying, S., Li, Y., Zhu, L., Wang, X., and Jin, H. (2021). Linking the YTH Domain to Cancer: the Importance of YTH Family Proteins in Epigenetics. Cell. Death Dis. 12 (4), 346. doi:10.1038/s41419-021-03625-8
Smyth, E. C., Nilsson, M., Grabsch, H. I., van Grieken, N. C., and Lordick, F. (2020). Gastric Cancer. Lancet 396 (10251), 635–648. doi:10.1016/s0140-6736(20)31288-5
Song, Z., Jia, G., Ma, P., and Cang, S. (2021). Exosomal miR-4443 Promotes Cisplatin Resistance in Non-small Cell Lung Carcinoma by Regulating FSP1 m6A Modification-Mediated Ferroptosis. Life Sci. 276, 119399. doi:10.1016/j.lfs.2021.119399
Su, R., Dong, L., Li, Y., Gao, M., Han, L., Wunderlich, M., et al. (2020). Targeting FTO Suppresses Cancer Stem Cell Maintenance and Immune Evasion. Cancer Cell. 38 (1), 79–e11. e11. doi:10.1016/j.ccell.2020.04.017
Sun, T., Wu, Z., Wang, X., Wang, Y., Hu, X., Qin, W., et al. (2020). LNC942 Promoting METTL14-Mediated m6A Methylation in Breast Cancer Cell Proliferation and Progression. Oncogene 39 (31), 5358–5372. doi:10.1038/s41388-020-1338-9
Sung, H., Ferlay, J., Siegel, R. L., Laversanne, M., Soerjomataram, I., Jemal, A., et al. (2021). Global Cancer Statistics 2020: GLOBOCAN Estimates of Incidence and Mortality Worldwide for 36 Cancers in 185 Countries. CA Cancer J. Clin. 71 (3), 209–249. doi:10.3322/caac.21660
Taketo, K., Konno, M., Asai, A., Koseki, J., Toratani, M., Satoh, T., et al. (2018). The Epitranscriptome m6A Writer METTL3 Promotes Chemo- and Radioresistance in Pancreatic Cancer Cells. Int. J. Oncol. 52 (2), 621–629. doi:10.3892/ijo.2017.4219
Tang, B., Yang, Y., Kang, M., Wang, Y., Wang, Y., Bi, Y., et al. (2020). m6A Demethylase ALKBH5 Inhibits Pancreatic Cancer Tumorigenesis by Decreasing WIF-1 RNA Methylation and Mediating Wnt signalingA Demethylase ALKBH5 Inhibits Pancreatic Cancer Tumorigenesis by Decreasing WIF-1 RNA Methylation and Mediating Wnt Signaling. Mol. Cancer 19 (1), 3. doi:10.1186/s12943-019-1128-6
Uddin, M. B., Roy, K. R., Hosain, S. B., Khiste, S. K., Hill, R. A., Jois, S. D., et al. (2019). An N6-Methyladenosine at the Transited Codon 273 of P53 Pre-mRNA Promotes the Expression of R273H Mutant Protein and Drug Resistance of Cancer Cells. Biochem. Pharmacol. 160, 134–145. doi:10.1016/j.bcp.2018.12.014
Wang, J., Wang, J., Gu, Q., Ma, Y., Yang, Y., Zhu, J., et al. (2020). The Biological Function of m6A Demethylase ALKBH5 and its Role in Human Disease. Cancer Cell. Int. 20, 347. doi:10.1186/s12935-020-01450-1
Wang, J., Xu, R., Li, J., Bai, Y., Liu, T., Jiao, S., et al. (2016). Randomized Multicenter Phase III Study of a Modified Docetaxel and Cisplatin Plus Fluorouracil Regimen Compared with Cisplatin and Fluorouracil as First-Line Therapy for Advanced or Locally Recurrent Gastric Cancer. Gastric Cancer 19 (1), 234–244. doi:10.1007/s10120-015-0457-4
Wang, K., Miao, X., Kong, F., Huang, S., Mo, J., Jin, C., et al. (2021). Integrating Network Pharmacology and Experimental Verification to Explore the Mechanism of Effect of Zuojin Pills in Pancreatic Cancer Treatment. Drug Des. Devel Ther. 15, 3749–3764. doi:10.2147/dddt.s323360
Wang, L., Hui, H., Agrawal, K., Kang, Y., Li, N., Tang, R., et al. (2020). m6 A RNA Methyltransferases METTL3/14 Regulate Immune Responses to Anti-PD-1 therapyA RNA Methyltransferases METTL3/14 Regulate Immune Responses to Anti-PD-1 Therapy. Embo J. 39 (20), e104514. doi:10.15252/embj.2020104514
Wang, X., Huang, J., Zou, T., and Yin, P. (2017). Human m6A Writers: Two Subunits, 2 Roles. RNA Biol. 14 (3), 300–304. doi:10.1080/15476286.2017.1282025
Wang, X., Tian, L., Li, Y., Wang, J., Yan, B., Yang, L., et al. (2021). RBM15 Facilitates Laryngeal Squamous Cell Carcinoma Progression by Regulating TMBIM6 Stability through IGF2BP3 Dependent. J. Exp. Clin. Cancer Res. 40 (1), 80. doi:10.1186/s13046-021-01871-4
Wang, X., Zhao, B. S., Roundtree, I. A., Lu, Z., Han, D., Ma, H., et al. (2015). N(6)-methyladenosine Modulates Messenger RNA Translation Efficiency. Cell. 161 (6), 1388–1399. doi:10.1016/j.cell.2015.05.014
Wojtas, M. N., Pandey, R. R., Mendel, M., Homolka, D., Sachidanandam, R., and Pillai, R. S. (2017). Regulation of m6A Transcripts by the 3'→5' RNA Helicase YTHDC2 Is Essential for a Successful Meiotic Program in the Mammalian Germline. Mol. Cell. 68 (2), 374–e12. e12. doi:10.1016/j.molcel.2017.09.021
Xu, J., Wan, Z., Tang, M., Lin, Z., Jiang, S., Ji, L., et al. (2020). N6-methyladenosine-modified CircRNA-SORE Sustains Sorafenib Resistance in Hepatocellular Carcinoma by Regulating β-catenin Signaling. Mol. Cancer 19 (1), 163. doi:10.1186/s12943-020-01281-8
Xu, K., Sun, Y., Sheng, B., Zheng, Y., Wu, X., and Xu, K. (2019). Role of Identified RNA N6-Methyladenosine Methylation in Liver. Anal. Biochem. 578, 45–50. doi:10.1016/j.ab.2019.05.005
Xu, W., Xie, S., Chen, X., Pan, S., Qian, H., and Zhu, X. (2021). Effects of Quercetin on the Efficacy of Various Chemotherapeutic Drugs in Cervical Cancer Cells. Drug Des. Devel Ther. 15, 577–588. doi:10.2147/dddt.s291865
Xu, Z., Peng, B., Cai, Y., Wu, G., Huang, J., Gao, M., et al. (2020). N6-methyladenosine RNA Modification in Cancer Therapeutic Resistance: Current Status and Perspectives. Biochem. Pharmacol. 182, 114258. doi:10.1016/j.bcp.2020.114258
Yan, Y., Liang, Q., Xu, Z., and Yi, Q. (2021). Integrative Bioinformatics and Experimental Analysis Revealed Down-Regulated CDC42EP3 as a Novel Prognostic Target for Ovarian Cancer and its Roles in Immune Infiltration. PeerJ 9, e12171. doi:10.7717/peerj.12171
Yang, Z., Zhao, F., Gu, X., Feng, L., Xu, M., Li, T., et al. (2021). Binding of RNA m6A by IGF2BP3 Triggers Chemoresistance of HCT8 Cells via Upregulation of ABCB1. Am. J. Cancer Res. 11 (4), 1428–1445.
Yu, S., Li, X., Liu, S., Yang, R., Liu, X., and Wu, S. (2019). N6-Methyladenosine: A Novel RNA Imprint in Human Cancer. Front. Oncol. 9, 1407. doi:10.3389/fonc.2019.01407
Zeng, C., Huang, W., Li, Y., and Weng, H. (2020). Roles of METTL3 in Cancer: Mechanisms and Therapeutic Targeting. J. Hematol. Oncol. 13 (1), 117. doi:10.1186/s13045-020-00951-w
Zeng, S., Pöttler, M., Lan, B., Grützmann, R., Pilarsky, C., and Yang, H. (2019). Chemoresistance in Pancreatic Cancer. Int. J. Mol. Sci. 20 (18). doi:10.3390/ijms20184504
Zhang, B., Wu, Q., Li, B., Wang, D., Wang, L., and Zhou, Y. L. (2020). m6A Regulator-Mediated Methylation Modification Patterns and Tumor Microenvironment Infiltration Characterization in Gastric Cancer A Regulator-Mediated Methylation Modification Patterns and Tumor Microenvironment Infiltration Characterization in Gastric Cancer. Mol. Cancer 19 (1), 53. doi:10.1186/s12943-020-01170-0
Zhang, C., Ou, S., Zhou, Y., Liu, P., Zhang, P., Li, Z., et al. (2021). m6A Methyltransferase METTL14-Mediated Upregulation of Cytidine Deaminase Promoting Gemcitabine Resistance in Pancreatic CancerA Methyltransferase METTL14-Mediated Upregulation of Cytidine Deaminase Promoting Gemcitabine Resistance in Pancreatic Cancer. Front. Oncol. 11, 696371. doi:10.3389/fonc.2021.696371
Zhang, L., Qi, Y., ALuo, Z., Zhang, Z., and Zhou, L. (2019). Betaine Increases Mitochondrial Content and Improves Hepatic Lipid Metabolism. Food Funct. 10 (1), 216–223. doi:10.1039/c8fo02004c
Zhang, R., Li, X., Zhang, X., Qin, H., and Xiao, W. (2021). Machine Learning Approaches for Elucidating the Biological Effects of Natural Products. Nat. Prod. Rep. 38 (2), 346–361. doi:10.1039/d0np00043d
Zhang, X., Xie, K., Zhou, H., Wu, Y., Li, C., Liu, Y., et al. (2020). Role of Non-coding RNAs and RNA Modifiers in Cancer Therapy Resistance. Mol. Cancer 19 (1), 47. doi:10.1186/s12943-020-01171-z
Zhao, B. S., Wang, X., Beadell, A. V., Lu, Z., Shi, H., Kuuspalu, A., et al. (2017). m6A-dependent Maternal mRNA Clearance Facilitates Zebrafish Maternal-To-Zygotic transitionA-dependent Maternal mRNA Clearance Facilitates Zebrafish Maternal-To-Zygotic Transition. Nature 542 (7642), 475–478. doi:10.1038/nature21355
Zheng, F., Du, F., Zhao, J., Wang, X., Si, Y., Jin, P., et al. (2021). The Emerging Role of RNA N6-Methyladenosine Methylation in Breast Cancer. Biomark. Res. 9 (1), 39. doi:10.1186/s40364-021-00295-8
Zhi, Y., Zhang, S., Zi, M., Wang, Y., Liu, Y., Zhang, M., et al. (2022). Potential Applications of N 6 ‐methyladenosine Modification in the Prognosis and Treatment of Cancers via Modulating Apoptosis, Autophagy, and Ferroptosis. Wiley Interdiscip. Rev. RNA, e1719. doi:10.1002/wrna.1719
Zhou, L. L., Xu, H., Huang, Y., and Yang, C. G. (2021). Targeting the RNA Demethylase FTO for Cancer Therapy. RSC Chem. Biol. 2 (5), 1352–1369. doi:10.1039/d1cb00075f
Zhu, D., Zhou, J., Zhao, J., Jiang, G., Zhang, X., Zhang, Y., et al. (2019). ZC3H13 Suppresses Colorectal Cancer Proliferation and Invasion via Inactivating Ras-ERK Signaling. J. Cell. Physiol. 234 (6), 8899–8907. doi:10.1002/jcp.27551
Zhu, L., Zhu, Y., Han, S., Chen, M., Song, P., Dai, D., et al. (2019). Impaired Autophagic Degradation of lncRNA ARHGAP5-AS1 Promotes Chemoresistance in Gastric Cancer. Cell. Death Dis. 10 (6), 383. doi:10.1038/s41419-019-1585-2
Zhu, Y. J., Zheng, B., Wang, H. Y., and Chen, L. (2017). New Knowledge of the Mechanisms of Sorafenib Resistance in Liver Cancer. Acta Pharmacol. Sin. 38 (5), 614–622. doi:10.1038/aps.2017.5
Zhu, Y. J., Zheng, B., Wang, H. Y., and Chen, L. (2017). New Knowledge of the Mechanisms of Sorafenib Resistance in Liver Cancer. Acta Pharmacol. Sin. 38 (5), 614–622. doi:10.1038/aps.2017.5
Zhu, Y., Zhou, B., Hu, X., Ying, S., Zhou, Q., Xu, W., et al. (2022). LncRNA LINC00942 Promotes Chemoresistance in Gastric Cancer by Suppressing MSI2 Degradation to Enhance c‐Myc mRNA Stability. Clin. Transl. Med 12 (1), e703. doi:10.1002/ctm2.703
Keywords: digestive system tumors, N6-methyladenosine, drug resistance, chemotherapy, immunotherapy
Citation: Xia Z, Kong F, Wang K and Zhang X (2022) Role of N6-Methyladenosine Methylation Regulators in the Drug Therapy of Digestive System Tumours. Front. Pharmacol. 13:908079. doi: 10.3389/fphar.2022.908079
Received: 30 March 2022; Accepted: 17 May 2022;
Published: 09 June 2022.
Edited by:
Leonardo Freire-De-Lima, Federal University of Rio de Janeiro, BrazilReviewed by:
Keyang Xu, Zhejiang Chinese Medical University, ChinaCopyright © 2022 Xia, Kong, Wang and Zhang. This is an open-access article distributed under the terms of the Creative Commons Attribution License (CC BY). The use, distribution or reproduction in other forums is permitted, provided the original author(s) and the copyright owner(s) are credited and that the original publication in this journal is cited, in accordance with accepted academic practice. No use, distribution or reproduction is permitted which does not comply with these terms.
*Correspondence: Xin Zhang, engxMzYwNTc2QDEyNi5jb20=
†These authors have contributed equally to this work and share first authorship
Disclaimer: All claims expressed in this article are solely those of the authors and do not necessarily represent those of their affiliated organizations, or those of the publisher, the editors and the reviewers. Any product that may be evaluated in this article or claim that may be made by its manufacturer is not guaranteed or endorsed by the publisher.
Research integrity at Frontiers
Learn more about the work of our research integrity team to safeguard the quality of each article we publish.