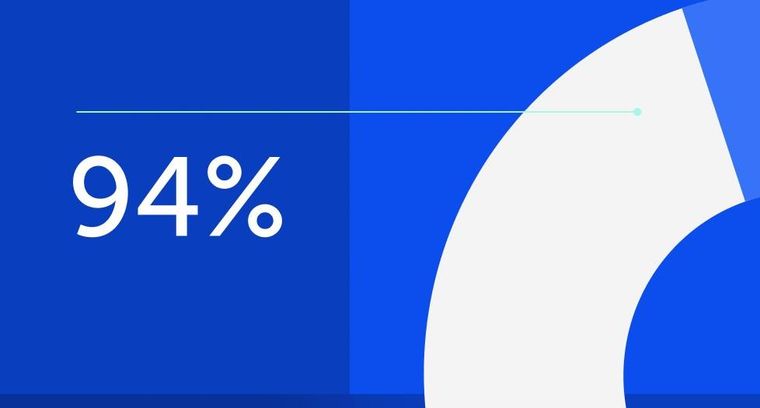
94% of researchers rate our articles as excellent or good
Learn more about the work of our research integrity team to safeguard the quality of each article we publish.
Find out more
REVIEW article
Front. Pharmacol., 16 May 2022
Sec. Experimental Pharmacology and Drug Discovery
Volume 13 - 2022 | https://doi.org/10.3389/fphar.2022.907981
A correction has been applied to this article in:
Corrigendum: Role of selective histone deacetylase 6 inhibitor ACY-1215 in cancer and other human diseases
The deacetylation process regulated by histone deacetylases (HDACs) plays an important role in human health and diseases. HDAC6 belongs to the Class IIb of HDACs family, which mainly modifies non-histone proteins located in the cytoplasm. HDAC6 plays a key role in tumors, neurological diseases, and inflammatory diseases. Therefore, targeting HDAC6 has become a promising treatment strategy in recent years. ACY-1215 is the first orally available highly selective HDAC6 inhibitor, and its efficacy and therapeutic effects are being continuously verified. This review summarizes the research progress of ACY-1215 in cancer and other human diseases, as well as the underlying mechanism, in order to guide the future clinical trials of ACY-1215 and more in-depth mechanism researches.
Epigenetics, first defined by Professor C.H. Waddington, refers to the heritable modification of gene expression and regulation while does not involve DNA sequence changes, and its related research have been accelerated rapidly in the 21st century (Waddington, 2012; Cavalli and Heard, 2019). Epigenetics includes DNA methylation, histone modification, nucleosome remodeling, and RNA-mediated targeted regulation. They regulate many biological processes that lead to cancer and other human diseases (Dawson and Kouzarides, 2012). Histone acetylation was first identified in 1963 and functionally characterized as a positive regulator of transcription by Vincent Allfrey and colleagues in 1964 (Phillips, 1963; Allfrey et al., 1964). The balance between acetylation and deacetylation is important in regulating gene expression. Histone deacetylases (HDACs) mediate deacetylation, promote the return of chromatin to a suppressed, higher-order structure, which obviously reduces DNA accessibility to the transcription machine. As a result, it will increase transcriptional silencing, and then affect cell fate. Therefore, over acetylation of normally silenced regions or deacetylation of normally active transcription regions may lead to various diseases (Timmermann et al., 2001).
There are 18 subtypes of HDACs in mammals: Class I (HDAC1, HDAC2, HDAC3 and HDAC8), Class II (HDAC4, HDAC5, HDAC6, HDAC7, HDAC9 and HDAC10), Class III (SIRT1, SIRT2, SIRT3, SIRT4, SIRT5, SIRT6 and SIRT7), and Class IV (HDAC11) (de Ruijter et al., 2003). By removing acetyl groups from ε-amino-lysine of proteins (Gallinari et al., 2007), HDACs not only alter transcription, but also promote the establishment or elimination of other post-translational lysine modifications such as methylation and ubiquitination. Biological processes induced by HDACs have a significant impact on human health, and HDACs abnormalities have been documented to play a key role in many human diseases, including cancer, neurological diseases, inflammatory diseases, and heart diseases (Seto and Yoshida, 2014; Zhou et al., 2021).
In the HDACs family, HDAC6 is the most special as it is the only HDAC with two functional deacetylase domains and a ubiquitin binding zinc finger motif (Verdel et al., 2000; Grozinger et al., 1999; Zhang et al., 2006), containing 1215 amino acid residues (Figure 1). HDAC6 mainly targets proteins located in the cytoplasm. Through the direct deacetylation of tubulin, cortactin and HSP90, or by binding with some chaperonin, HDAC6 regulates the cell response to some important phenomena (Hubbert et al., 2002; Matsuyama et al., 2002; Valenzuela-Fernández et al., 2008; Wang et al., 2018a). Deacetylation of microtubules by HDAC6 is necessary for cell movement, cell cycle regulation, and processing of misfolded proteins (Hubbert et al., 2002; Kawaguchi et al., 2003). The deacetylation of HSP90 by HDAC6 plays an important role in the ubiquitin-proteasome system and protein folding (Yu et al., 2002; Bali et al., 2005; Kovacs et al., 2005). HDAC6 plays an important role in cancer, neurological diseases, inflammatory diseases, and other diseases (Porter et al., 2017; Cosenza and Pozzi, 2018; Ke et al., 2018; Li et al., 2018; LoPresti, 2020; Shen and Kozikowski, 2020). Therefore, inhibitors targeting HDAC6 may be promising treatment modalities.
FIGURE 1. Structure of HDAC6. NLS, nuclear localization sequence. CD, catalytic domains. NES, nuclear export signal. SE14, cytoplasmic retention signal. UBP, ubiquitin-binding zinc finger domain.
HDACs inhibitors are divided into pan-inhibitor and selective inhibitor. HDACs inhibitor has three functional groups. The typical pharmacophore characteristics of HDACs inhibitor is consist of zinc-binding group (ZBG), linker and cap group (Figure 2A). To date, five HDACs inhibitors have been approved: vorinostat (SAHA, Zolinza), romidepsin (FK228, Istodax), panobinostat (LBH589, Farydak), belinostat (PXD101, Beleodaq), and chidamide (HBI8000, Epidaza) (Figure 3) (Whittaker et al., 2010; Duvic and Vu, 2007; Garnock-Jones, 2015; Ning et al., 2012). However, the five HDACs inhibitors are all pan-inhibitors. Due to adverse toxicity such as fatigue, diarrhea, and thrombocytopenia, their clinical application is limited (Falkenberg and Johnstone, 2014; Minucci and Pelicci, 2006). To develop potentially less toxic and more effective treatments, studies on selective HDACs inhibitors are gradually deepening (Zhao et al., 2021; He et al., 2020). Chemical modification of the cap group allows for isomer selective HDACs inhibitors (Krämer et al., 2014). A series of compounds containing urea-based branched linkers with hydroxamate as ZBG have been identified as selective HDAC6 inhibitors (Bergman et al., 2012), such as Tubasatin A, Nexturastat A, ACY-1215 (ricolinostat), ACY-241 (citarinostat), ACY-738, ACY-775, ACY-1083, KA2507, CKD-504,CKD-506 etc (Zhao et al., 2021; Pulya et al., 2021). Among whom, ACY-1215 is a typical representative, having an IC50 of 4.7 nM against HDAC6 (Figure 2B). As an effective and the first oral bioavailable selective HDAC6 inhibitor, ACY-1215 is at least 10 times more selective against HDAC6 than other HDACs and is basically non-toxic (Santo et al., 2012; Amengual et al., 2021). ACY-1215 has been studied for long and its importance as anti-cancer agent has already been established through various papers. However, a comprehensive review specifically summarizing its role on cancer and other human diseases is lacking. What’s more, recent studies have found its potential applications in other human diseases and revealed several novel mechanisms. Therefore, we summarized the current study progress of ACY-1215 in cancer and other human diseases.
Imbalance of non-histone acetylation is common in human cancers, with changes in the structure or expression of histone acetyltransferases and HDACs occurring in many cancers (Marks et al., 2004). Since the first application of ACY-1215 in multiple myeloma (MM) in 2012 (Santo et al., 2012), ACY-1215 has shown satisfactory efficacy in various tumors. And its molecular mechanism has been gradually revealed (Figures 4, 5).
FIGURE 4. Biological processes regulated by ACY-1215. (A) Acetylate tubulin and increase microtubule stability. (B) Acetylate GRP78, inhibit misfolded or unfolded protein in the aggregate pathway and inhibit protein degradation. (C) Acetylate HSP90 and reduce polyubiquitinated protein transportation. (D) Reduce tau hyperphosphorylation and promote clearance. (E) Reduce F-actin depended cell migration by deacetylating cortactin.
Accumulation of unfolded or misfolded proteins in the endoplasmic reticulum (ER) causes an unfolded protein response, which is a part of ER stress (Senft and Ronai, 2015). Acetylated GRP78 inhibits misfolded or unfolded protein transport along microtubules to centrosomes in the aggregate pathway, inhibiting protein degradation, and then leading to excessive ER stress. Unfolded protein response may induce apoptosis if ER homeostasis cannot be restored (Pfaffenbach and Lee, 2011). MM can be effectively treated with proteasome inhibitors such as bortezomib or carfilzomib, but myeloma cells can overcome proteasome inhibition through alternative aggresome and autophagy pathways to escape from death (Richardson et al., 2017). By deacetylating HSP90, HDAC6 binds and transports polyubiquitinated protein aggregates (Liu et al., 2021). Tereu et al. (Hideshima et al., 2005) found that selective inhibition of HDAC6 increased tubulin acetylation, which in turn inhibited the aggresome pathway, therefore leading to accumulation of polyubiquitinated proteins and apoptosis in MM cells. In 2012, Santo et al. (Santo et al., 2012) applied ACY-1215 for the first time in the treatment of MM, and they found that the combination of low-dose ACY-1215 and bortezomib could produce synergistic anti-MM activity. Compared with pan-HDAC inhibitors, the highly selective ACY-1215 has improved security. Further studies by (Mishima et al., 2015) showed that ACY-1215 inhibited aggresome formation and autophagy caused by carfilzomib induced inhibition of the proteasome pathway, and then induced additional ER stress, thus promoting MM cell apoptosis. In cholangiocarcinoma, reduction of autophagy caused by ACY-1215 decreases proliferation and increases cilia expression (Peixoto et al., 2020). In primary lymphoma, head and neck cancer and melanoma, ACY-1215 and bortezomib have also been demonstrated to have strong synergic effects by dual targeting protein degradation pathways (Amengual et al., 2015; Peng et al., 2017; Hattori et al., 2021).
ACY-1215 has also been demonstrated to be an important regulator of apoptosis pathways in tumors. ACY-1215 inhibits cell proliferation and promotes apoptosis by targeting MAPK/ERK, PI3K/AKT and other cell signaling pathways. In non-Hodgkin’s lymphoma, ACY-1215 causes inactivation of AKT and ERK1/2, leading to increased DNA damage and ultimately tumor cells death (Lee et al., 2019a). In melanoma cells, ACY-1215 accelerates cell death by inhibiting ERK activation (Peng et al., 2017; Sundaramurthi et al., 2022). In head and neck carcinoma cell, the suppression of p-Chk1 activity caused by ACY-1215 leads to synergistically enhanced apoptosis via mitotic catastrophe in a p53-dependent manner (Miyake et al., 2022). Enhanced transcriptional activity of p53 by ACY-1215 is also found in triple-negative breast cancer (Cao et al., 2022). In cholangiocarcinoma, ACY-1215 suppresses GRP78 translocation to the cell surface via PI3K/AKT pathway, which inhibits proliferation and promotes apoptosis (Kim et al., 2022). Growth inhibition has also been observed in colon cancer cells, prostate cancer cells, glioma cells and gallbladder cancer cells (Tan et al., 2019; Corno et al., 2020; Huang et al., 2020; Ruan et al., 2021). Besides, ACY-1215 in combination with immunosuppressant IMiD (Hideshima et al., 2015) and inhibitor JQ1 (Carew et al., 2019), a member of the Bromine domain and extra terminal protein family, down-regulated proto-oncogene c-Myc expression and induced co-cytotoxicity in MM.
Abnormal cell cycle and strong migration ability of tumor cells lead to rapid proliferation and high degree of malignancies. The change of microtubule dynamics can lead to cell cycle stagnation. ACY-1215 induces apoptosis and G0/G1 cell cycle arrest by increasing tubulin acetylation in melanoma cells (Wang et al., 2018b). In non-small cell carcinoma cell lines A549, LL2, and H1299, inhibition of HDAC6 by ACY-1215 leads to G2 phase arrest and increased apoptosis (Deskin et al., 2020). In ARID1A-deficient endometrial carcinoma, the G2/M cell cycle checkpoint and ATM/ATR-mediated DNA damage checkpoints is disrupted, while the migratory and invasive phenotype can be reversed by ACY-1215 (Megino-Luque et al., 2022). In triple-negative breast cancer, ACY-1215 results in G1 cell cycle arrest and apoptosis (Cao et al., 2022), and enhances the anti-tumor effect of eribulin through tubulin acetylation (Oba et al., 2021). Moreover, F-actin depended cell migration is also reduced when cortactin deacetylation is inhibited (Li et al., 2018). ACY-1215 inhibits the proliferation and migration of high-grade serous ovarian cancer cells and tektin4-deficient triple-negative breast cancer cells (Ali et al., 2020; Ge et al., 2021).
Immunotherapy is an important part of cancer therapy (van den Bulk et al., 2018; Yang, 2015). Programmed death ligand 1 (PD-L1) expression is significantly increased in ACY-1215 combination therapy in colorectal cancer cells (Ryu et al., 2018). (Lee et al., 2018) further verified that the combination of ACY-1215 and oxaliplatin could not only induce the synergistic upregulation of PD-L1, but also decreased the level of Bcl-2 protein and some other kinase. In ovarian clear cell carcinoma, ACY-1215 was found to activate CD4 and CD8 T cells and increase IFNγ+ CD4 and CD8 T cells, as a result enhancing the immune killing effect (Fukumoto et al., 2019). In melanoma patients, ACY-1215 downregulates mTORC1/2 signaling, reduces yield of Treg and production of Th2 cytokines, thereby, altering T-cell function (Laino et al., 2019). The combination of ACY-1215 and JQ1 in the treatment of xenograft tumors derived from human and mouse small-cell lung cancer cell lines showed significant tumor growth inhibition by provoking NK-cell-mediated immunity (Liu et al., 2018).
The antitumor effects of HDAC6 inhibitors were also demonstrated in other selective HDAC6 inhibitors, such as ACY-241 (Ray et al., 2018; Cosenza et al., 2020; Awad et al., 2021; Park et al., 2021) and KA2507 (Tsimberidou et al., 2021).
In addition to bind and transport polyubiquitinated proteins for aggregation, HDAC6 can also regulate domain receptors for cytoskeletal proteins such as tau, IIp45 (invasion inhibitory protein 45) and EGFR (epidermal growth factor receptor) through protein-protein interactions (Pulya et al., 2021). HDAC6-mediated acetylation of multiple non-histones is associated with different functions including intracellular transport, neurotransmitter release, and aggregation formation (Chen et al., 2010; Kalinski et al., 2019). Dysregulation of HDAC6 results in alterations in excitatory-inhibitory equilibrium, synaptic transmission, memory, and protein processing. HDAC6 inhibitors regulate a variety of events including growth cone function, synaptic plasticity, transport and autophagosome degradation (LoPresti, 2020). Inhibition of HDAC6 restores α -tubulin acetylation and mitochondrial transport (Perry et al., 2017). In addition, HDAC6 inhibitors promote degradation of protein aggregates and protection from neuronal oxidative stress (Wang et al., 2019; Zeb et al., 2019). Therefore, ACY-1215 may play a vital role in neurodegeneration and peripheral neuropathy.
Neurodegenerative diseases are a kind of nervous system diseases closely related to aging (Hou et al., 2019). Low acetylation is present during neurodegeneration (Sharma et al., 2019). HDAC6 may not only lead to deterioration of learning and memory, but also increase Aβ and tau phosphorylation levels (Liu et al., 2020). Extracellular aggregation of Aβ plaques and intracellular neurofibrillary tangles composed of hyperphosphorylated tau protein in the human cortex and limbic regions contribute to the development of Alzheimer’s disease (AD). Tau usually binds to and stabilizes microtubules. But in AD and related neurodegenerative diseases, significantly increased HDAC6 reduces tubulin acetylation, as a result, tau is hyperphosphorylated and aggregates into neurofibrillary tangles, which eventually leads to neuron loss, synaptic dysfunction, and cognitive decline (Hempen and Brion, 1996; Yan, 2014; Tiwari et al., 2019; Li et al., 2021). (Zhang et al., 2014) found that ACY-1215 effectively reduced the behavioral defects of AD mice by reducing Aβ deposition and tau hyperphosphorylation, as well as promoting autophagy clearance. (Mao et al., 2017) further found in drosophila that by increasing the acetylation of tubulin, ACY-1215 could rescue microtubules defects and neuromuscular junction growth anomalies caused by tau overexpression. The same results were also noticed in another selective HDAC6 inhibitor CKD-504 (Choi et al., 2020).
Cognitive impairment of the nervous system is directly related to axon damage. (Wang et al., 2019) found that by increasing tubulin acetylation, ACY-1215 decreased mitochondrial transport and mitochondrial dysfunction and increased synaptic density, thus ameliorating cisplatin-induced brain damage in mice. The same conclusion was found in hippocampus mitochondria (Ma et al., 2018). In HIV-positive patients, binding of GP120 to neuronal microtubules and reduced tubulin acetylation levels decreased the rate of axon transport of brain-derived neurotrophic factor. Wenzel et al. showed that ACY-1215 blocked GP120-mediated tubulin deacetylation and axon transport reduction (Avdoshina et al., 2017; Wenzel et al., 2019).
In addition to affecting cognitive function, axon transport disorders are associated with peripheral neuropathy (Pareyson et al., 2015; Prior et al., 2018). Peripheral neuropathy is a chronic, debilitating disease that involves peripheral nerve damage in varies diseases such as Charcot-Marie-Tooth (CMT) disease, chemotherapy neurotoxicity, mitochondrial disease, and diabetes (Colloca et al., 2017). Studies have found that mechanical abnormal pain occurs due to mitochondrial damage in neurons (Ma et al., 2019). ACY-1215 can effectively reverse cisplatin-induced mechanical abnormal pain, and the effect still exists 1 week after completion of treatment (Krukowski et al., 2017). CMT2 is a non-demyelinating axonal disease characterized by muscle weakness and atrophy (Morena et al., 2019). What’s more, ACY-1215 ameliorates mitochondrial transport deficits by increasing tubulin acetylation, which in turn rescue axon transport deficits and then reverse motor and sensory deficits in a mouse model for mutant “small heat shock protein B1”-induced CMT2 at both behavioral and electrophysiological levels (Benoy et al., 2017). The effect of ACY-1215 on CMT has also been demonstrated in CKD-504 (Ha et al., 2020; Smith et al., 2022).
Generally, inflammation is a defensive response of lesion present in living tissue (Shi and Pamer, 2011). However, dysregulated, or excessive inflammation can be harmful. Through regulating cell signaling pathways, inflammatory cytokines, and inflammatory cells (Ran and Zhou, 2019; Lee et al., 2020), HDAC6 inhibitors have great potential as a treatment for inflammatory diseases, including rheumatoid arthritis (Oh et al., 2017), inflammatory bowel disease (Lu et al., 2016; Do et al., 2017), and respiratory inflammation (Ren et al., 2016). In addition, ACY-1215 has also shown to promising results in acute liver failure (ALF), osteoarthritis, and skin inflammation (Figure 6).
Gong et al. (Jiao et al., 2017; Zhang et al., 2018; Chen et al., 2021) applied ACY-1215 to a mouse model of ALF. They showed that ACY-1215 improved liver tissue damage and liver function in ALF, reduced the expression level of inflammatory factor TNFα-mRNA and NF-κB-P65 protein, and reduced NLRP3 inflammasome through regulation of ATM/F-actin signaling pathway. In vitro, ACY-1215 mitigated LPS-induced macrophage inflammation via the TLR4-MAPK/NF-κB pathway (Zhang et al., 2019a). Furthermore, ACY-1215 can also activate AMPK signaling pathway, enhance autophagy, regulate mitochondrial mediated oxidative stress, improve glucose metabolism and lipid metabolism, and thereby reduce apoptosis and inflammatory response in ALF (Zhang et al., 2019b; Chen et al., 2019; Chen et al., 2020a; Wang et al., 2021). In osteoarthritis, ACY-1215 inhibits the STAT3 and NF-κB pathway in chondrocytes leading to anti-inflammatory and chondroprotective effects (Cheng et al., 2019), as well as inhibits VEGF expression through the PI3K/AKT pathway and then promotes osteoblast apoptosis (Li et al., 2019). In addition, ACY-1215 improves neuropathic pain by blocking MyD88-dependent pro-inflammatory pathways (Chen et al., 2022). HDAC6 inhibitors inhibit inflammation by inhibiting NF-κB signaling, reducing the production of various pro-inflammatory cytokines and chemokines, and inhibiting the inflammatory response of monocytes/macrophages, which was also demonstrated in CKD-506 (Choi et al., 2018; Lee et al., 2020; Park et al., 2020; Park et al., 2021). Another interesting finding was that ACY-1215 inhibited the function of impaired CD8 T cells during skin inflammation, preventing the development of contact hypersensitivity and graft-versus-host disease like-diseases in vivo by regulating CD8 T cell activation and function (Tsuji et al., 2015).
Because of ACY-1215’s involvement of various pathway, attempts in varies disease models have yielded inspiring results. In polycystic liver disease, ACY-1215 diminished liver cyst development and fibrosis by reducing cholangiocyte proliferation and cyst growth both in vitro and in vivo (Gradilone et al., 2014). ACY-1215 with pasireotide synergistically reduced cyst growth and increased length of primary cilia in vivo, and decreased cell proliferation and inhibited cAMP levels in vitro (Lorenzo Pisarello et al., 2018). In a mouse model of polycystic kidney disease, ACY-1215 treatment reduced cAMP and cyst growth (Yanda et al., 2017). And ACY-1215 mitigated renal fibrosis by suppressing transforming growth factor-β1 and epidermal growth factor receptor signaling pathways in obstructive nephropathy (Chen et al., 2020b). In glucocorticoid-induced osteoporosis, ACY-1215 reverses dexamethasone-induced inhibition of osteoblast proliferation and differentiation (Wang et al., 2020). In rats with cardiac ischemia-reperfusion injury, ACY-1215 might reduce infarct size through modulating hypoxia inducible factor-1α expression (Lin et al., 2020). Although this part of the study did not have a more in-depth mechanism discussion, it also provided a new treatment idea for the diseases.
HDAC6 regulates various biological processes involved in proteasome degradation, cell migration, microtubule dynamics, apoptosis, and axon growth, and it also participates in a variety of signaling pathways in pathological responses to diseases. Targeting the above biological process by inhibiting the functions and activities of HDAC6 are well studied in different cancers, neurodegenerative diseases, epigenetic rare diseases, and inflammatory diseases. To date, many selective HDAC6 inhibitors have been have been reported in preclinical studies and have entered clinical trials (Tables 1, 2). However, except for ACY-1215, the present application range of other HDAC6 inhibitors is limited. Studies on ACY-241 (Ray et al., 2018; Cosenza et al., 2020; Awad et al., 2021; Park et al., 2021) and KA2507 (Tsimberidou et al., 2021) mainly focused on tumors, CKD-504 (Choi et al., 2020; Ha et al., 2020; Jeong et al., 2022; Smith et al., 2022) focused on neurological diseases, and CKD-506 (Choi et al., 2018; Park et al., 2020; Bae et al., 2021) focused on inflammatory diseases. Although ACY-241 and KA2507 show higher selectivity over ACY-1215 on HDAC6, its studies on other diseases needs further research (Table 3).
It has been more than 10 years since the discovery and application of ACY-1215. Currently, there are more than 10 phase I/II clinical trials related to ACY-1215. The existing trial results show that at the recommended dose of ACY-1215 of 160 mg daily, the combination with bortezomib/lenalidomide and dexamethasone of MM therapy has a higher treatment response and without adverse events (Yee et al., 2016; Vogl et al., 2017). The safety and efficacy of ACY-1215 in patients with recurrent and refractory lymphatic malignancies were also demonstrated (Amengual et al., 2021). Meanwhile, ACY-1215 could have meaningful clinical impact on preventing or attenuating taxane-induced peripheral neuropathy (Lee et al., 2019b). While, there are currently no phase III clinical trials of ACY-1215 ongoing. Therefore, phase III clinical trials of the above or clinical trials on the application of ACY-1215 on other diseases need further investigation.
At present, ACY-1215 has achieved significant therapeutic effects among various diseases in cell and animal models, and the involved pathway is relatively clear. However, there are still some objective problems that cannot be ignored. On the one hand, the limitations of the disease model itself lead to the distance gap from laboratory to clinic, and on the other hand, the safety and efficacy of ACY-1215 still need more clinical trials to prove. The latest researches also showed that ACY-1215 could improve the developmental competence of somatic cell nuclear transfer embryos (Gao et al., 2022) and promote the generation of megakaryocyte progenitors (Jiang et al., 2022).
In the present review, we summarized the research progress of ACY-1215 in cancer and other human diseases, as well as its related mechanisms. This review will guide researchers to further explore the clinical application of ACY-1215 to various diseases and further reveal its underlying molecular mechanisms.
JL drafted the manuscript and drew diagrams. MY revised the manuscript. SF revised the manuscript. DL proposed the topic of the article and made important revisions to the manuscript. YT made important revisions to the manuscript. All authors read and approved the final manuscript.
This work was supported by Scientific research project of Hunan Provincial Health Commission (No. 202103030980) and the Fundamental Research Funds for the Central Universities of Central South University (No. 2022ZZTS0860).
The authors declare that the research was conducted in the absence of any commercial or financial relationships that could be construed as a potential conflict of interest.
All claims expressed in this article are solely those of the authors and do not necessarily represent those of their affiliated organizations, or those of the publisher, the editors and the reviewers. Any product that may be evaluated in this article, or claim that may be made by its manufacturer, is not guaranteed or endorsed by the publisher.
Ali, A., Zhang, F., Maguire, A., Byrne, T., Weiner-Gorzel, K., Bridgett, S., et al. (2020). HDAC6 Degradation Inhibits the Growth of High-Grade Serous Ovarian Cancer Cells. Cancers (Basel) 12 (12). doi:10.3390/cancers12123734
Allfrey, V. G., Faulkner, R., and Mirsky, A. E. (1964). Acetylation and Methylation of Histones and Their Possible Role in the Regulation of Rna Synthesis. Proc. Natl. Acad. Sci. U. S. A. 51 (5), 786–794. doi:10.1073/pnas.51.5.786
Amengual, J. E., Johannet, P., Lombardo, M., Zullo, K., Hoehn, D., Bhagat, G., et al. (2015). Dual Targeting of Protein Degradation Pathways with the Selective HDAC6 Inhibitor ACY-1215 and Bortezomib Is Synergistic in Lymphoma. Clin. Cancer Res. 21 (20), 4663–4675. doi:10.1158/1078-0432.CCR-14-3068
Amengual, J. E., Lue, J. K., Ma, H., Lichtenstein, R., Shah, B., Cremers, S., et al. (2021). First-in-Class Selective HDAC6 Inhibitor (ACY-1215) Has a Highly Favorable Safety Profile in Patients with Relapsed and Refractory Lymphoma. Oncologist 26 (3), 184–e366. doi:10.1002/onco.13673
Avdoshina, V., Caragher, S. P., Wenzel, E. D., Taraballi, F., Mocchetti, I., and Harry, G. J. (2017). The Viral Protein Gp120 Decreases the Acetylation of Neuronal Tubulin: Potential Mechanism of Neurotoxicity. J. Neurochem. 141 (4), 606–613. doi:10.1111/jnc.14015
Awad, M. M., Le Bruchec, Y., Lu, B., Ye, J., Miller, J., Lizotte, P. H., et al. (2021). Selective Histone Deacetylase Inhibitor ACY-241 (Citarinostat) Plus Nivolumab in Advanced Non-small Cell Lung Cancer: Results from a Phase Ib Study. Front. Oncol. 11, 696512. doi:10.3389/fonc.2021.696512
Bae, D., Lee, J. Y., Ha, N., Park, J., Baek, J., Suh, D., et al. (2021). CKD-506: A Novel HDAC6-Selective Inhibitor that Exerts Therapeutic Effects in a Rodent Model of Multiple Sclerosis. Sci. Rep. 11 (1), 14466. doi:10.1038/s41598-021-93232-6
Bali, P., Pranpat, M., Bradner, J., Balasis, M., Fiskus, W., Guo, F., et al. (2005). Inhibition of Histone Deacetylase 6 Acetylates and Disrupts the Chaperone Function of Heat Shock Protein 90: a Novel Basis for Antileukemia Activity of Histone Deacetylase Inhibitors. J. Biol. Chem. 280 (29), 26729–26734. doi:10.1074/jbc.C500186200
Benoy, V., Vanden Berghe, P., Jarpe, M., Van Damme, P., Robberecht, W., and Van Den Bosch, L. (2017). Development of Improved HDAC6 Inhibitors as Pharmacological Therapy for Axonal Charcot-Marie-Tooth Disease. Neurotherapeutics 14 (2), 417–428. doi:10.1007/s13311-016-0501-z
Bergman, J. A., Woan, K., Perez-Villarroel, P., Villagra, A., Sotomayor, E. M., and Kozikowski, A. P. (2012). Selective Histone Deacetylase 6 Inhibitors Bearing Substituted Urea Linkers Inhibit Melanoma Cell Growth. J. Med. Chem. 55 (22), 9891–9899. doi:10.1021/jm301098e
Cao, W., Shen, R., Richard, S., Liu, Y., Jalalirad, M., Cleary, M. P., et al. (2022). Inhibition of Triple-negative B-reast C-ancer P-roliferation and M-otility by R-eactivating P-53 and I-nhibiting O-veractivated Akt. Oncol. Rep. 47 (2). doi:10.3892/or.2021.8252
Carew, J. S., Espitia, C. M., Zhao, W., Visconte, V., Anwer, F., Kelly, K. R., et al. (2019). Rational Cotargeting of HDAC6 and BET Proteins Yields Synergistic Antimyeloma Activity. Blood Adv. 3 (8), 1318–1329. doi:10.1182/bloodadvances.2018026484
Cavalli, G., and Heard, E. (2019). Advances in Epigenetics Link Genetics to the Environment and Disease. Nature 571 (7766), 489–499. doi:10.1038/s41586-019-1411-0
Chen, C., Liu, A., Lu, Q., Luo, L., Li, J., Ke, J., et al. (2022). HDAC6 Inhibitor ACY-1215 Improves Neuropathic Pain and its Comorbidities in Rats of Peripheral Nerve Injury by Regulating Neuroinflammation. Chem. Biol. Interact. 353, 109803. doi:10.1016/j.cbi.2022.109803
Chen, Q., Wang, Y., Jiao, F., Cao, P., Shi, C., Pei, M., et al. (2021). HDAC6 Inhibitor ACY1215 Inhibits the Activation of NLRP3 Inflammasome in Acute Liver Failure by Regulating the ATM/F-actin Signalling Pathway. J. Cell. Mol. Med. 25 (15), 7218–7228. doi:10.1111/jcmm.16751
Chen, Q., Wang, Y., Jiao, F., Shi, C., Pei, M., Wang, L., et al. (2020). Histone Deacetylase 6 Inhibitor ACY1215 Ameliorates Mitochondrial Dynamic and Function Injury in Hepatocytes by Activating AMPK Signaling Pathway in Acute Liver Failure Mice. Histol. Histopathol. 35 (9), 1047–1058. doi:10.14670/HH-18-237
Chen, Q., Wang, Y., Jiao, F. Z., Shi, C. X., and Gong, Z. J. (2019). Histone Deacetylase 6 Inhibitor ACY1215 Offers a Protective Effect through the Autophagy Pathway in Acute Liver Failure. Life Sci. 238, 116976. doi:10.1016/j.lfs.2019.116976
Chen, S., Owens, G. C., Makarenkova, H., and Edelman, D. B. (2010). HDAC6 Regulates Mitochondrial Transport in Hippocampal Neurons. PloS one 5 (5), e10848. doi:10.1371/journal.pone.0010848
Chen, X., Yu, C., Hou, X., Li, J., Li, T., Qiu, A., et al. (2020). Histone Deacetylase 6 Inhibition Mitigates Renal Fibrosis by Suppressing TGF-β and EGFR Signaling Pathways in Obstructive Nephropathy. Am. J. Physiol. Ren. Physiol. 319 (6), F1003–f1014. doi:10.1152/ajprenal.00261.2020
Cheng, C., Shan, W., Huang, W., Ding, Z., Cui, G., Liu, F., et al. (2019). ACY-1215 Exhibits Anti-inflammatory and Chondroprotective Effects in Human Osteoarthritis Chondrocytes via Inhibition of STAT3 and NF-Κb Signaling Pathways. Biomed. Pharmacother. 109, 2464–2471. doi:10.1016/j.biopha.2018.11.017
Choi, E. W., Song, J. W., Ha, N., Choi, Y. I., and Kim, S. (2018). CKD-506, a Novel HDAC6-Selective Inhibitor, Improves Renal Outcomes and Survival in a Mouse Model of Systemic Lupus Erythematosus. Sci. Rep. 8 (1), 17297. doi:10.1038/s41598-018-35602-1
Choi, H., Kim, H. J., Yang, J., Chae, S., Lee, W., Chung, S., et al. (2020). Acetylation Changes Tau Interactome to Degrade Tau in Alzheimer's Disease Animal and Organoid Models. Aging Cell. 19 (1), e13081. doi:10.1111/acel.13081
Colloca, L., Ludman, T., Bouhassira, D., Baron, R., Dickenson, A. H., Yarnitsky, D., et al. (2017). Neuropathic Pain. Nat. Rev. Dis. Prim. 3, 17002. doi:10.1038/nrdp.2017.2
Corno, C., Arrighetti, N., Ciusani, E., Corna, E., Carenini, N., Zaffaroni, N., et al. (2020). Synergistic Interaction of Histone Deacetylase 6- and MEK-Inhibitors in Castration-Resistant Prostate Cancer Cells. Front. Cell. Dev. Biol. 8, 610. doi:10.3389/fcell.2020.00610
Cosenza, M., Civallero, M., Marcheselli, L., Sacchi, S., and Pozzi, S. (2020). Citarinostat and Momelotinib Co-target HDAC6 and JAK2/STAT3 in Lymphoid Malignant Cell Lines: a Potential New Therapeutic Combination. Apoptosis 25 (5-6), 370–387. doi:10.1007/s10495-020-01607-3
Cosenza, M., and Pozzi, S. (2018). The Therapeutic Strategy of HDAC6 Inhibitors in Lymphoproliferative Disease. Int. J. Mol. Sci. 19 (8). doi:10.3390/ijms19082337
Dawson, M. A., and Kouzarides, T. (2012). Cancer Epigenetics: from Mechanism to Therapy. Cell. 150 (1), 12–27. doi:10.1016/j.cell.2012.06.013
de Ruijter, A. J., van Gennip, A. H., Caron, H. N., Kemp, S., and van Kuilenburg, A. B. (2003). Histone Deacetylases (HDACs): Characterization of the Classical HDAC Family. Biochem. J. 370 (Pt 3), 737–749. doi:10.1042/BJ20021321
Deskin, B., Yin, Q., Zhuang, Y., Saito, S., Shan, B., and Lasky, J. A. (2020). Inhibition of HDAC6 Attenuates Tumor Growth of Non-small Cell Lung Cancer. Transl. Oncol. 13 (2), 135–145. doi:10.1016/j.tranon.2019.11.001
Do, A., Reid, R. C., Lohman, R. J., Sweet, M. J., Fairlie, D. P., and Iyer, A. (2017). An HDAC6 Inhibitor Confers Protection and Selectively Inhibits B-Cell Infiltration in DSS-Induced Colitis in Mice. J. Pharmacol. Exp. Ther. 360 (1), 140–151. doi:10.1124/jpet.116.236711
Duvic, M., and Vu, J. (2007). Vorinostat: a New Oral Histone Deacetylase Inhibitor Approved for Cutaneous T-Cell Lymphoma. Expert Opin. Investig. Drugs 16 (7), 1111–1120. doi:10.1517/13543784.16.7.1111
Falkenberg, K. J., and Johnstone, R. W. (2014). Histone Deacetylases and Their Inhibitors in Cancer, Neurological Diseases and Immune Disorders. Nat. Rev. Drug Discov. 13 (9), 673–691. doi:10.1038/nrd4360
Fukumoto, T., Fatkhutdinov, N., Zundell, J. A., Tcyganov, E. N., Nacarelli, T., Karakashev, S., et al. (2019). HDAC6 Inhibition Synergizes with Anti-PD-L1 Therapy in ARID1A-Inactivated Ovarian Cancer. Cancer Res. 79 (21), 5482–5489. doi:10.1158/0008-5472.CAN-19-1302
Gallinari, P., Di Marco, S., Jones, P., Pallaoro, M., and Steinkühler, C. (2007). HDACs, Histone Deacetylation and Gene Transcription: from Molecular Biology to Cancer Therapeutics. Cell. Res. 17 (3), 195–211. doi:10.1038/sj.cr.7310149
Gao, S., Wang, Z., Ma, J., Wang, J., Xing, X., Shen, C., et al. (2022). Effect of ACY-1215 on Cytoskeletal Remodeling and Histone Acetylation in Bovine Somatic Cell Nuclear Transfer Embryos. Theriogenology 183, 98–107. doi:10.1016/j.theriogenology.2022.02.018
Garnock-Jones, K. P. (2015). Panobinostat: First Global Approval. Drugs 75 (6), 695–704. doi:10.1007/s40265-015-0388-8
Ge, L. P., Jin, X., Yang, Y. S., Liu, X. Y., Shao, Z. M., Di, G. H., et al. (2021). Tektin4 Loss Promotes Triple-Negative Breast Cancer Metastasis through HDAC6-Mediated Tubulin Deacetylation and Increases Sensitivity to HDAC6 Inhibitor. Oncogene 40 (12), 2323–2334. doi:10.1038/s41388-021-01655-2
Gradilone, S. A., Habringer, S., Masyuk, T. V., Howard, B. N., Masyuk, A. I., and Larusso, N. F. (2014). HDAC6 Is Overexpressed in Cystic Cholangiocytes and its Inhibition Reduces Cystogenesis. Am. J. Pathol. 184 (3), 600–608. doi:10.1016/j.ajpath.2013.11.027
Grozinger, C. M., Hassig, C. A., and Schreiber, S. L. (1999). Three Proteins Define a Class of Human Histone Deacetylases Related to Yeast Hda1p. Proc. Natl. Acad. Sci. U. S. A. 96 (9), 4868–4873. doi:10.1073/pnas.96.9.4868
Ha, N., Choi, Y. I., Jung, N., Song, J. Y., Bae, D. K., Kim, M. C., et al. (2020). A Novel Histone Deacetylase 6 Inhibitor Improves Myelination of Schwann Cells in a Model of Charcot-Marie-Tooth Disease Type 1A. Br. J. Pharmacol. 177 (22), 5096–5113. doi:10.1111/bph.15231
Hattori, K., Takano, N., Kazama, H., Moriya, S., Miyake, K., Hiramoto, M., et al. (2021). Induction of Synergistic Non-apoptotic Cell Death by Simultaneously Targeting Proteasomes with Bortezomib and Histone Deacetylase 6 with Ricolinostat in Head and Neck Tumor Cells. Oncol. Lett. 22 (3), 680. doi:10.3892/ol.2021.12941
He, X., Li, Z., Zhuo, X. T., Hui, Z., Xie, T., and Ye, X. Y. (2020). Novel Selective Histone Deacetylase 6 (HDAC6) Inhibitors: A Patent Review (2016-2019). Recent Pat. Anticancer Drug Discov. 15 (1), 32–48. doi:10.2174/1574892815666200217125419
Hempen, B., and Brion, J. P. (1996). Reduction of Acetylated Alpha-Tubulin Immunoreactivity in Neurofibrillary Tangle-Bearing Neurons in Alzheimer's Disease. J. Neuropathol. Exp. Neurol. 55 (9), 964–972. doi:10.1097/00005072-199609000-00003
Hideshima, T., Bradner, J. E., Wong, J., Chauhan, D., Richardson, P., Schreiber, S. L., et al. (2005). Small-molecule Inhibition of Proteasome and Aggresome Function Induces Synergistic Antitumor Activity in Multiple Myeloma. Proc. Natl. Acad. Sci. U. S. A. 102 (24), 8567–8572. doi:10.1073/pnas.0503221102
Hideshima, T., Cottini, F., Ohguchi, H., Jakubikova, J., Gorgun, G., Mimura, N., et al. (2015). Rational Combination Treatment with Histone Deacetylase Inhibitors and Immunomodulatory Drugs in Multiple Myeloma. Blood Cancer J. 5 (5), e312. doi:10.1038/bcj.2015.38
Hou, Y., Dan, X., Babbar, M., Wei, Y., Hasselbalch, S. G., Croteau, D. L., et al. (2019). Ageing as a Risk Factor for Neurodegenerative Disease. Nat. Rev. Neurol. 15 (10), 565–581. doi:10.1038/s41582-019-0244-7
Huang, P., Almeciga-Pinto, I., Jarpe, M., van Duzer, J. H., Mazitschek, R., Yang, M., et al. (2017). Selective HDAC Inhibition by ACY-241 Enhances the Activity of Paclitaxel in Solid Tumor Models. Oncotarget 8 (2), 2694–2707. doi:10.18632/oncotarget.13738
Huang, Z., Xia, Y., Hu, K., Zeng, S., Wu, L., Liu, S., et al. (2020). Histone Deacetylase 6 Promotes Growth of Glioblastoma through the MKK7/JNK/c-Jun Signaling Pathway. J. Neurochem. 152 (2), 221–234. doi:10.1111/jnc.14849
Hubbert, C., Guardiola, A., Shao, R., Kawaguchi, Y., Ito, A., Nixon, A., et al. (2002). HDAC6 Is a Microtubule-Associated Deacetylase. Nature 417 (6887), 455–458. doi:10.1038/417455a
Jeong, H. S., Kim, H. J., Kim, D. H., Chung, K. W., Choi, B. O., and Lee, J. E. (2022). Therapeutic Potential of CKD-504, a Novel Selective Histone Deacetylase 6 Inhibitor, in a Zebrafish Model of Neuromuscular Junction Disorders. Mol. Cells 45 (4), 231–242. doi:10.14348/molcells.2022.5005
Jiang, J., Qin, J., Li, J., Lin, X., Zhang, B., Fan, Z., et al. (2022). Ricolinostat Promotes the Generation of Megakaryocyte Progenitors from Human Hematopoietic Stem and Progenitor Cells. Stem Cell. Res. Ther. 13 (1), 54. doi:10.1186/s13287-022-02722-5
Jiao, F. Z., Zhang, H. Y., Yang, F., Wang, L. W., and Gong, Z. J. (2017). Protective Effect of the Histone Deacetylase Inhibitor ACY1215 against Brain Edema in Mice with Acute Liver Failure. Zhonghua Gan Zang Bing Za Zhi 25 (9), 695–700. doi:10.3760/cma.j.issn.1007-3418.2017.09.009
Kalinski, A. L., Kar, A. N., Craver, J., Tosolini, A. P., Sleigh, J. N., Lee, S. J., et al. (2019). Deacetylation of Miro1 by HDAC6 Blocks Mitochondrial Transport and Mediates Axon Growth Inhibition. J. Cell. Biol. 218 (6), 1871–1890. doi:10.1083/jcb.201702187
Kawaguchi, Y., Kovacs, J. J., McLaurin, A., Vance, J. M., Ito, A., and Yao, T. P. (2003). The Deacetylase HDAC6 Regulates Aggresome Formation and Cell Viability in Response to Misfolded Protein Stress. Cell. 115 (6), 727–738. doi:10.1016/s0092-8674(03)00939-5
Ke, B., Chen, Y., Tu, W., Ye, T., Fang, X., and Yang, L. (2018). Inhibition of HDAC6 Activity in Kidney Diseases: a New Perspective. Mol. Med. 24 (1), 33. doi:10.1186/s10020-018-0027-4
Kim, C., Lee, S., Kim, D., Lee, D. S., Lee, E., Yoo, C., et al. (2022). Blockade of GRP78 Translocation to the Cell Surface by HDAC6 Inhibition Suppresses Proliferation of Cholangiocarcinoma Cells. Anticancer Res. 42 (1), 471–482. doi:10.21873/anticanres.15505
Kovacs, J. J., Murphy, P. J., Gaillard, S., Zhao, X., Wu, J. T., Nicchitta, C. V., et al. (2005). HDAC6 Regulates Hsp90 Acetylation and Chaperone-dependent Activation of Glucocorticoid Receptor. Mol. Cell. 18 (5), 601–607. doi:10.1016/j.molcel.2005.04.021
Krämer, O. H., Mahboobi, S., and Sellmer, A. (2014). Drugging the HDAC6-HSP90 Interplay in Malignant Cells. Trends Pharmacol. Sci. 35 (10), 501–509. doi:10.1016/j.tips.2014.08.001
Krukowski, K., Ma, J., Golonzhka, O., Laumet, G. O., Gutti, T., van Duzer, J. H., et al. (2017). HDAC6 Inhibition Effectively Reverses Chemotherapy-Induced Peripheral Neuropathy. Pain 158 (6), 1126–1137. doi:10.1097/j.pain.0000000000000893
Laino, A. S., Betts, B. C., Veerapathran, A., Dolgalev, I., Sarnaik, A., Quayle, S. N., et al. (2019). HDAC6 Selective Inhibition of Melanoma Patient T-Cells Augments Anti-tumor Characteristics. J. Immunother. Cancer 7 (1), 33. doi:10.1186/s40425-019-0517-0
Lee, D. H., Kim, G. W., and Kwon, S. H. (2019). The HDAC6-Selective Inhibitor Is Effective against Non-hodgkin Lymphoma and Synergizes with Ibrutinib in Follicular Lymphoma. Mol. Carcinog. 58 (6), 944–956. doi:10.1002/mc.22983
Lee, D. H., Won, H. R., Ryu, H. W., Han, J. M., and Kwon, S. H. (2018). The HDAC6 Inhibitor ACY-1215 E-nhances the A-nticancer A-ctivity of O-xaliplatin in C-olorectal C-ancer C-ells. Int. J. Oncol. 53 (2), 844–854. doi:10.3892/ijo.2018.4405
Lee, E. K., Tan-Wasielewski, Z., Matulonis, U. A., Birrer, M. J., Wright, A. A., Horowitz, N., et al. (2019). Results of an Abbreviated Phase Ib Study of the HDAC6 Inhibitor Ricolinostat and Paclitaxel in Recurrent Ovarian, Fallopian Tube, or Primary Peritoneal Cancer. Gynecol. Oncol. Rep. 29, 118–122. doi:10.1016/j.gore.2019.07.010
Lee, J. W., Lee, S. M., Chun, J., Im, J. P., Seo, S. K., Ha, N., et al. (2020). Novel Histone Deacetylase 6 Inhibitor CKD-506 Inhibits NF-Κb Signaling in Intestinal Epithelial Cells and Macrophages and Ameliorates Acute and Chronic Murine Colitis. Inflamm. Bowel Dis. 26 (6), 852–862. doi:10.1093/ibd/izz317
Li, L., Liu, F., Huang, W., Wang, J., Wan, Y., Li, M., et al. (2019). Ricolinostat (ACY-1215) Inhibits VEGF Expression via PI3K/AKT Pathway and Promotes Apoptosis in Osteoarthritic Osteoblasts. Biomed. Pharmacother. 118, 109357. doi:10.1016/j.biopha.2019.109357
Li, T., Zhang, C., Hassan, S., Liu, X., Song, F., Chen, K., et al. (2018). Histone Deacetylase 6 in Cancer. J. Hematol. Oncol. 11 (1), 111. doi:10.1186/s13045-018-0654-9
Li, Y., Sang, S., Ren, W., Pei, Y., Bian, Y., Chen, Y., et al. (2021). Inhibition of Histone Deacetylase 6 (HDAC6) as a Therapeutic Strategy for Alzheimer's Disease: A Review (2010-2020). Eur. J. Med. Chem. 226, 113874. doi:10.1016/j.ejmech.2021.113874
Lin, C. F., Hsu, K. C., HuangFu, W. C., Lin, T. E., Huang, H. L., and Pan, S. L. (2020). Investigating the Potential Effects of Selective Histone Deacetylase 6 Inhibitor ACY1215 on Infarct Size in Rats with Cardiac Ischemia-Reperfusion Injury. BMC Pharmacol. Toxicol. 21 (1), 21. doi:10.1186/s40360-020-0400-0
Liu, P., Xiao, J., Wang, Y., Song, X., Huang, L., Ren, Z., et al. (2021). Posttranslational Modification and beyond: Interplay between Histone Deacetylase 6 and Heat-Shock Protein 90. Mol. Med. 27 (1), 110. doi:10.1186/s10020-021-00375-3
Liu, Y., Li, Y., Liu, S., Adeegbe, D. O., Christensen, C. L., Quinn, M. M., et al. (2018). NK Cells Mediate Synergistic Antitumor Effects of Combined Inhibition of HDAC6 and BET in a SCLC Preclinical Model. Cancer Res. 78 (13), 3709–3717. doi:10.1158/0008-5472.CAN-18-0161
Liu, Z., Hao, K. M., Wang, H. Y., and Qi, W. X. (2020). Histone Deacetylase-6 Modulates Amyloid Beta-Induced Cognitive Dysfunction Rats by Regulating PTK2B. Neuroreport 31 (10), 754–761. doi:10.1097/WNR.0000000000001481
LoPresti, P. (2020). HDAC6 in Diseases of Cognition and of Neurons. Cells 10 (1). doi:10.3390/cells10010012
Lorenzo Pisarello, M., Masyuk, T. V., Gradilone, S. A., Masyuk, A. I., Ding, J. F., Lee, P. Y., et al. (2018). Combination of a Histone Deacetylase 6 Inhibitor and a Somatostatin Receptor Agonist Synergistically Reduces Hepatorenal Cystogenesis in an Animal Model of Polycystic Liver Disease. Am. J. Pathol. 188 (4), 981–994. doi:10.1016/j.ajpath.2017.12.016
Lu, L. F., Kim, D. H., Lee, I. H., Hong, J., Zhang, P., Yoon, I. N., et al. (2016). Potassium Acetate Blocks Clostridium difficile Toxin A-Induced Microtubule Disassembly by Directly Inhibiting Histone Deacetylase 6, Thereby Ameliorating Inflammatory Responses in the Gut. J. Microbiol. Biotechnol. 26 (4), 693–699. doi:10.4014/jmb.1511.11063
Ma, J., Huo, X., Jarpe, M. B., Kavelaars, A., and Heijnen, C. J. (2018). Pharmacological Inhibition of HDAC6 Reverses Cognitive Impairment and Tau Pathology as a Result of Cisplatin Treatment. Acta Neuropathol. Commun. 6 (1), 103. doi:10.1186/s40478-018-0604-3
Ma, J., Trinh, R. T., Mahant, I. D., Peng, B., Matthias, P., Heijnen, C. J., et al. (2019). Cell-specific Role of Histone Deacetylase 6 in Chemotherapy-Induced Mechanical Allodynia and Loss of Intraepidermal Nerve Fibers. Pain 160 (12), 2877–2890. doi:10.1097/j.pain.0000000000001667
Mao, C. X., Wen, X., Jin, S., and Zhang, Y. Q. (2017). Increased Acetylation of Microtubules Rescues Human Tau-Induced Microtubule Defects and Neuromuscular Junction Abnormalities in Drosophila. Dis. Model Mech. 10 (10), 1245–1252. doi:10.1242/dmm.028316
Marks, P. A., Richon, V. M., Miller, T., and Kelly, W. K. (2004). Histone Deacetylase Inhibitors. Adv. Cancer Res. 91, 137–168. doi:10.1016/S0065-230X(04)91004-4
Matsuyama, A., Shimazu, T., Sumida, Y., Saito, A., Yoshimatsu, Y., Seigneurin-Berny, D., et al. (2002). In Vivo destabilization of Dynamic Microtubules by HDAC6-Mediated Deacetylation. EMBO J. 21 (24), 6820–6831. doi:10.1093/emboj/cdf682
Megino-Luque, C., Siso, P., Mota-Martorell, N., Navaridas, R., de la Rosa, I., Urdanibia, I., et al. (2022). ARID1A-deficient Cells Require HDAC6 for Progression of Endometrial Carcinoma. Mol. Oncol.
Minucci, S., and Pelicci, P. G. (2006). Histone Deacetylase Inhibitors and the Promise of Epigenetic (And More) Treatments for Cancer. Nat. Rev. Cancer 6 (1), 38–51. doi:10.1038/nrc1779
Mishima, Y., Santo, L., Eda, H., Cirstea, D., Nemani, N., Yee, A. J., et al. (2015). Ricolinostat (ACY-1215) Induced Inhibition of Aggresome Formation Accelerates Carfilzomib-Induced Multiple Myeloma Cell Death. Br. J. Haematol. 169 (3), 423–434. doi:10.1111/bjh.13315
Miyake, K., Takano, N., Kazama, H., Kikuchi, H., Hiramoto, M., Tsukahara, K., et al. (2022). Ricolinostat Enhances Adavosertib-induced M-itotic C-atastrophe in TP53-mutated H-ead and N-eck S-quamous C-ell C-arcinoma C-ells. Int. J. Oncol. 60 (5). doi:10.3892/ijo.2022.5344
Morena, J., Gupta, A., and Hoyle, J. C. (2019). Charcot-Marie-Tooth: From Molecules to Therapy. Int. J. Mol. Sci. 20 (14). doi:10.3390/ijms20143419
Ning, Z. Q., Li, Z. B., Newman, M. J., Shan, S., Wang, X. H., Pan, D. S., et al. (2012). Chidamide (CS055/HBI-8000): a New Histone Deacetylase Inhibitor of the Benzamide Class with Antitumor Activity and the Ability to Enhance Immune Cell-Mediated Tumor Cell Cytotoxicity. Cancer Chemother. Pharmacol. 69 (4), 901–909. doi:10.1007/s00280-011-1766-x
Oba, T., Ono, M., Matoba, H., Uehara, T., Hasegawa, Y., and Ito, K. I. (2021). HDAC6 Inhibition Enhances the Anti-tumor Effect of Eribulin through Tubulin Acetylation in Triple-Negative Breast Cancer Cells. Breast Cancer Res. Treat. 186 (1), 37–51. doi:10.1007/s10549-020-06033-2
Oh, B. R., Suh, D. H., Bae, D., Ha, N., Choi, Y. I., Yoo, H. J., et al. (2017). Therapeutic Effect of a Novel Histone Deacetylase 6 Inhibitor, CKD-L, on Collagen-Induced Arthritis In Vivo and Regulatory T Cells in Rheumatoid Arthritis In Vitro. Arthritis Res. Ther. 19 (1), 154. doi:10.1186/s13075-017-1357-2
Pareyson, D., Saveri, P., Sagnelli, A., and Piscosquito, G. (2015). Mitochondrial Dynamics and Inherited Peripheral Nerve Diseases. Neurosci. Lett. 596, 66–77. doi:10.1016/j.neulet.2015.04.001
Park, J. K., Jang, Y. J., Oh, B. R., Shin, J., Bae, D., Ha, N., et al. (2020). Therapeutic Potential of CKD-506, a Novel Selective Histone Deacetylase 6 Inhibitor, in a Murine Model of Rheumatoid Arthritis. Arthritis Res. Ther. 22 (1), 176. doi:10.1186/s13075-020-02258-0
Park, S. J., Joo, S. H., Lee, N., Jang, W. J., Seo, J. H., and Jeong, C. H. (2021). ACY-241, an HDAC6 Inhibitor, Overcomes Erlotinib Resistance in Human Pancreatic Cancer Cells by Inducing Autophagy. Arch. Pharm. Res. 44 (12), 1062–1075. doi:10.1007/s12272-021-01359-x
Peixoto, E., Jin, S., Thelen, K., Biswas, A., Richard, S., Morleo, M., et al. (2020). HDAC6-dependent Ciliophagy Is Involved in Ciliary Loss and Cholangiocarcinoma Growth in Human Cells and Murine Models. Am. J. Physiol. Gastrointest. Liver Physiol. 318 (6), G1022–G1033. doi:10.1152/ajpgi.00033.2020
Peng, U., Wang, Z., Pei, S., Ou, Y., Hu, P., Liu, W., et al. (2017). ACY-1215 Accelerates Vemurafenib Induced Cell Death of BRAF-Mutant Melanoma Cells via Induction of ER Stress and Inhibition of ERK Activation. Oncol. Rep. 37 (2), 1270–1276. doi:10.3892/or.2016.5340
Perry, S., Kiragasi, B., Dickman, D., and Ray, A. (2017). The Role of Histone Deacetylase 6 in Synaptic Plasticity and Memory. Cell. Rep. 18 (6), 1337–1345. doi:10.1016/j.celrep.2017.01.028
Pfaffenbach, K. T., and Lee, A. S. (2011). The Critical Role of GRP78 in Physiologic and Pathologic Stress. Curr. Opin. Cell. Biol. 23 (2), 150–156. doi:10.1016/j.ceb.2010.09.007
Phillips, D. M. (1963). The Presence of Acetyl Groups of Histones. Biochem. J. 87 (2), 258–263. doi:10.1042/bj0870258
Porter, N. J., Mahendran, A., Breslow, R., and Christianson, D. W. (2017). Unusual Zinc-Binding Mode of HDAC6-Selective Hydroxamate Inhibitors. Proc. Natl. Acad. Sci. U. S. A. 114 (51), 13459–13464. doi:10.1073/pnas.1718823114
Prior, R., Van Helleputte, L., Klingl, Y. E., and Van Den Bosch, L. (2018). HDAC6 as a Potential Therapeutic Target for Peripheral Nerve Disorders. Expert Opin. Ther. Targets 22 (12), 993–1007. doi:10.1080/14728222.2018.1541235
Pulya, S., Amin, S. A., Adhikari, N., Biswas, S., Jha, T., and Ghosh, B. (2021). HDAC6 as Privileged Target in Drug Discovery: A Perspective. Pharmacol. Res. 163, 105274. doi:10.1016/j.phrs.2020.105274
Ran, J., and Zhou, J. (2019). Targeted Inhibition of Histone Deacetylase 6 in Inflammatory Diseases. Thorac. Cancer 10 (3), 405–412. doi:10.1111/1759-7714.12974
Ray, A., Das, D. S., Song, Y., Hideshima, T., Tai, Y. T., Chauhan, D., et al. (2018). Combination of a Novel HDAC6 Inhibitor ACY-241 and Anti-PD-L1 Antibody Enhances Anti-tumor Immunity and Cytotoxicity in Multiple Myeloma. Leukemia 32 (3), 843–846. doi:10.1038/leu.2017.322
Ren, Y., Su, X., Kong, L., Li, M., Zhao, X., Yu, N., et al. (2016). Therapeutic Effects of Histone Deacetylase Inhibitors in a Murine Asthma Model. Inflamm. Res. 65 (12), 995–1008. doi:10.1007/s00011-016-0984-4
Richardson, P. G., Moreau, P., Laubach, J. P., Maglio, M. E., Lonial, S., and San-Miguel, J. (2017). Deacetylase Inhibitors as a Novel Modality in the Treatment of Multiple Myeloma. Pharmacol. Res. 117, 185–191. doi:10.1016/j.phrs.2016.11.020
Ruan, Y., Wang, L., and Lu, Y. (2021). HDAC6 Inhibitor, ACY1215 Suppress the Proliferation and Induce Apoptosis of Gallbladder Cancer Cells and Increased the Chemotherapy Effect of Gemcitabine and Oxaliplatin. Drug Dev. Res. 82 (4), 598–604. doi:10.1002/ddr.21780
Ryu, H. W., Shin, D. H., Lee, D. H., Won, H. R., and Kwon, S. H. (2018). A Potent Hydroxamic Acid-Based, Small-Molecule Inhibitor A452 Preferentially Inhibits HDAC6 Activity and Induces Cytotoxicity toward Cancer Cells Irrespective of P53 Status. Carcinogenesis 39 (1), 72–83. doi:10.1093/carcin/bgx121
Santo, L., Hideshima, T., Kung, A. L., Tseng, J. C., Tamang, D., Yang, M., et al. (2012). Preclinical Activity, Pharmacodynamic, and Pharmacokinetic Properties of a Selective HDAC6 Inhibitor, ACY-1215, in Combination with Bortezomib in Multiple Myeloma. Blood 119 (11), 2579–2589. doi:10.1182/blood-2011-10-387365
Senft, D., and Ronai, Z. A. (2015). UPR, Autophagy, and Mitochondria Crosstalk Underlies the ER Stress Response. Trends Biochem. Sci. 40 (3), 141–148. doi:10.1016/j.tibs.2015.01.002
Seto, E., and Yoshida, M. (2014). Erasers of Histone Acetylation: the Histone Deacetylase Enzymes. Cold Spring Harb. Perspect. Biol. 6 (4), a018713. doi:10.1101/cshperspect.a018713
Sharma, S., Sarathlal, K. C., and Taliyan, R. (2019). Epigenetics in Neurodegenerative Diseases: The Role of Histone Deacetylases. CNS Neurol. Disord. Drug Targets 18 (1), 11–18. doi:10.2174/1871527317666181004155136
Shen, S., and Kozikowski, A. P. (2020). A Patent Review of Histone Deacetylase 6 Inhibitors in Neurodegenerative Diseases (2014-2019). Expert Opin. Ther. Pat. 30 (2), 121–136. doi:10.1080/13543776.2019.1708901
Shi, C., and Pamer, E. G. (2011). Monocyte Recruitment during Infection and Inflammation. Nat. Rev. Immunol. 11 (11), 762–774. doi:10.1038/nri3070
Smith, A. S. T., Kim, J. H., Chun, C., Gharai, A., Moon, H. W., Kim, E. Y., et al. (2022). HDAC6 Inhibition Corrects Electrophysiological and Axonal Transport Deficits in a Human Stem Cell-Based Model of Charcot-Marie-Tooth Disease (Type 2D). Adv. Biol. (Weinh) 6 (2), e2101308. doi:10.1002/adbi.202101308
Sundaramurthi, H., García-Mulero, S., Tonelotto, V., Slater, K., Marcone, S., Piulats, J. M., et al. (2022). Uveal Melanoma Cell Line Proliferation Is Inhibited by Ricolinostat, a Histone Deacetylase Inhibitor. Cancers (Basel) 14 (3). doi:10.3390/cancers14030782
Tan, Y., Zhang, S., Zhu, H., Chu, Y., Zhou, H., Liu, D., et al. (2019). Histone Deacetylase 6 Selective Inhibitor ACY1215 Inhibits Cell Proliferation and Enhances the Chemotherapeutic Effect of 5-fluorouracil in HCT116 Cells. Ann. Transl. Med. 7 (1), 2. doi:10.21037/atm.2018.11.48
Timmermann, S., Lehrmann, H., Polesskaya, A., and Harel-Bellan, A. (2001). Histone Acetylation and Disease. Cell. Mol. Life Sci. 58 (5-6), 728–736. doi:10.1007/pl00000896
Tiwari, S., Atluri, V., Kaushik, A., Yndart, A., and Nair, M. (2019). Alzheimer's Disease: Pathogenesis, Diagnostics, and Therapeutics. Int. J. Nanomedicine 14, 5541–5554. doi:10.2147/IJN.S200490
Tsimberidou, A. M., Beer, P. A., Cartwright, C. A., Haymaker, C., Vo, H. H., Kiany, S., et al. (2021). Preclinical Development and First-In-Human Study of KA2507, a Selective and Potent Inhibitor of Histone Deacetylase 6, for Patients with Refractory Solid Tumors. Clin. cancer Res. official J. Am. Assoc. Cancer Res. 27 (13), 3584–3594. doi:10.1158/1078-0432.ccr-21-0238
Tsuji, G., Okiyama, N., Villarroel, V. A., and Katz, S. I. (2015). Histone Deacetylase 6 Inhibition Impairs Effector CD8 T-Cell Functions during Skin Inflammation. J. Allergy Clin. Immunol. 135 (5), 1228–1239. doi:10.1016/j.jaci.2014.10.002
Valenzuela-Fernández, A., Cabrero, J. R., Serrador, J. M., and Sánchez-Madrid, F. (2008). HDAC6: a Key Regulator of Cytoskeleton, Cell Migration and Cell-Cell Interactions. Trends Cell Biol. 18 (6), 291–297.
van den Bulk, J., Verdegaal, E. M., and de Miranda, N. F. (2018). Cancer Immunotherapy: Broadening the Scope of Targetable Tumours. Open Biol. 8 (6). doi:10.1098/rsob.180037
Verdel, A., Curtet, S., Brocard, M. P., Rousseaux, S., Lemercier, C., Yoshida, M., et al. (2000). Active Maintenance of mHDA2/mHDAC6 Histone-Deacetylase in the Cytoplasm. Curr. Biol. 10 (12), 747–749. doi:10.1016/s0960-9822(00)00542-x
Vogl, D. T., Raje, N., Jagannath, S., Richardson, P., Hari, P., Orlowski, R., et al. (2017). Ricolinostat, the First Selective Histone Deacetylase 6 Inhibitor, in Combination with Bortezomib and Dexamethasone for Relapsed or Refractory Multiple Myeloma. Clin. Cancer Res. 23 (13), 3307–3315. doi:10.1158/1078-0432.CCR-16-2526
Waddington, C. H. (2012). The Epigenotype. 1942. Int. J. Epidemiol. 41 (1), 10–13. doi:10.1093/ije/dyr184
Wang, D., Wang, B., Liu, Y., Dong, X., Su, Y., and Li, S. (2019). Protective Effects of ACY-1215 against Chemotherapy-Related Cognitive Impairment and Brain Damage in Mice. Neurochem. Res. 44 (11), 2460–2469. doi:10.1007/s11064-019-02882-6
Wang, F., Zhong, B. W., and Zhao, Z. R. (2018). ACY 1215, a Histone Deacetylase 6 Inhibitor, Inhibits Cancer Cell Growth in Melanoma. J. Biol. Regul. Homeost. Agents 32 (4), 851–858.
Wang, N., Wang, H., Chen, J., Wang, F., Wang, S., Zhou, Q., et al. (2020). ACY-1215, a HDAC6 I-nhibitor, D-ecreases the D-examethasone-induced S-uppression of O-steogenesis in MC3T3-E1 C-ells. Mol. Med. Rep. 22 (3), 2451–2459. doi:10.3892/mmr.2020.11319
Wang, X. X., Wan, R. Z., and Liu, Z. P. (2018). Recent Advances in the Discovery of Potent and Selective HDAC6 Inhibitors. Eur. J. Med. Chem. 143, 1406–1418. doi:10.1016/j.ejmech.2017.10.040
Wang, Y., Li, X., Chen, Q., Jiao, F., Shi, C., Pei, M., et al. (2021). Histone Deacetylase 6 Regulates the Activation of M1 Macrophages by the Glycolytic Pathway during Acute Liver Failure. J. Inflamm. Res. 14, 1473–1485. doi:10.2147/JIR.S302391
Wenzel, E. D., Speidell, A., Flowers, S. A., Wu, C., Avdoshina, V., and Mocchetti, I. (2019). Histone Deacetylase 6 Inhibition Rescues Axonal Transport Impairments and Prevents the Neurotoxicity of HIV-1 Envelope Protein Gp120. Cell. Death Dis. 10 (9), 674. doi:10.1038/s41419-019-1920-7
Whittaker, S. J., Demierre, M. F., Kim, E. J., Rook, A. H., Lerner, A., Duvic, M., et al. (2010). Final Results from a Multicenter, International, Pivotal Study of Romidepsin in Refractory Cutaneous T-Cell Lymphoma. J. Clin. Oncol. 28 (29), 4485–4491. doi:10.1200/JCO.2010.28.9066
Yan, J. (2014). Interplay between HDAC6 and its Interacting Partners: Essential Roles in the Aggresome-Autophagy Pathway and Neurodegenerative Diseases. DNA Cell. Biol. 33 (9), 567–580. doi:10.1089/dna.2013.2300
Yanda, M. K., Liu, Q., and Cebotaru, L. (2017). An Inhibitor of Histone Deacetylase 6 Activity, ACY-1215, Reduces cAMP and Cyst Growth in Polycystic Kidney Disease. Am. J. Physiol. Ren. Physiol. 313 (4), F997–f1004. doi:10.1152/ajprenal.00186.2017
Yang, Y. (2015). Cancer Immunotherapy: Harnessing the Immune System to Battle Cancer. J. Clin. Invest. 125 (9), 3335–3337. doi:10.1172/JCI83871
Yee, A. J., Bensinger, W. I., Supko, J. G., Voorhees, P. M., Berdeja, J. G., Richardson, P. G., et al. (2016). Ricolinostat Plus Lenalidomide, and Dexamethasone in Relapsed or Refractory Multiple Myeloma: a Multicentre Phase 1b Trial. Lancet Oncol. 17 (11), 1569–1578. doi:10.1016/S1470-2045(16)30375-8
Yu, X., Guo, Z. S., Marcu, M. G., Neckers, L., Nguyen, D. M., Chen, G. A., et al. (2002). Modulation of P53, ErbB1, ErbB2, and Raf-1 Expression in Lung Cancer Cells by Depsipeptide FR901228. J. Natl. Cancer Inst. 94 (7), 504–513. doi:10.1093/jnci/94.7.504
Zeb, A., Park, C., Rampogu, S., Son, M., Lee, G., and Lee, K. W. (2019). Structure-Based Drug Designing Recommends HDAC6 Inhibitors to Attenuate Microtubule-Associated Tau-Pathogenesis. ACS Chem. Neurosci. 10 (3), 1326–1335. doi:10.1021/acschemneuro.8b00405
Zhang, L., Liu, C., Wu, J., Tao, J. J., Sui, X. L., Yao, Z. G., et al. (2014). Tubastatin A/ACY-1215 Improves Cognition in Alzheimer's Disease Transgenic Mice. J. Alzheimers Dis. 41 (4), 1193–1205. doi:10.3233/JAD-140066
Zhang, W. B., Yang, F., Wang, Y., Jiao, F. Z., Zhang, H. Y., Wang, L. W., et al. (2019). Inhibition of HDAC6 Attenuates LPS-Induced Inflammation in Macrophages by Regulating Oxidative Stress and Suppressing the TLR4-Mapk/nf-Κb Pathways. Biomed. Pharmacother. 117, 109166. doi:10.1016/j.biopha.2019.109166
Zhang, W. B., Zhang, H. Y., Jiao, F. Z., Wang, L. W., Zhang, H., and Gong, Z. J. (2018). Histone Deacetylase 6 Inhibitor ACY-1215 Protects against Experimental Acute Liver Failure by Regulating the TLR4-Mapk/nf-Κb Pathway. Biomed. Pharmacother. 97, 818–824. doi:10.1016/j.biopha.2017.10.103
Zhang, W. B., Zhang, H. Y., Wang, Y., Jiao, F. Z., Wang, L. W., and Gong, Z. J. (2019). Quantitative Proteomic Analysis Reveals the Sites Related to Acetylation and Mechanism of ACY-1215 in Acute Liver Failure Mice. Front. Pharmacol. 10, 653. doi:10.3389/fphar.2019.00653
Zhang, Y., Gilquin, B., Khochbin, S., and Matthias, P. (2006). Two Catalytic Domains Are Required for Protein Deacetylation. J. Biol. Chem. 281 (5), 2401–2404. doi:10.1074/jbc.C500241200
Zhao, Y., Liang, T., Hou, X., and Fang, H. (2021). Recent Development of Novel HDAC6 Isoform-Selective Inhibitors. Curr. Med. Chem. 28 (21), 4133–4151. doi:10.2174/0929867327666201111142653
Keywords: histone deacetylase 6, histone deacetylase inhibitor, ACY-1215, cancer, neurological diseases, inflammatory diseases
Citation: Li J, Yu M, Fu S, Liu D and Tan Y (2022) Role of Selective Histone Deacetylase 6 Inhibitor ACY-1215 in Cancer and Other Human Diseases. Front. Pharmacol. 13:907981. doi: 10.3389/fphar.2022.907981
Received: 31 March 2022; Accepted: 02 May 2022;
Published: 16 May 2022.
Edited by:
Yuhei Nishimura, Mie University, JapanReviewed by:
A. Ganesan, University of East Anglia, United KingdomCopyright © 2022 Li, Yu, Fu, Liu and Tan. This is an open-access article distributed under the terms of the Creative Commons Attribution License (CC BY). The use, distribution or reproduction in other forums is permitted, provided the original author(s) and the copyright owner(s) are credited and that the original publication in this journal is cited, in accordance with accepted academic practice. No use, distribution or reproduction is permitted which does not comply with these terms.
*Correspondence: Deliang Liu, ZGVsaWFuZ2xpdUBjc3UuZWR1LmNu; Yuyong Tan, dGFueXV5b25nQGNzdS5lZHUuY24=
Disclaimer: All claims expressed in this article are solely those of the authors and do not necessarily represent those of their affiliated organizations, or those of the publisher, the editors and the reviewers. Any product that may be evaluated in this article or claim that may be made by its manufacturer is not guaranteed or endorsed by the publisher.
Research integrity at Frontiers
Learn more about the work of our research integrity team to safeguard the quality of each article we publish.