- 1Department of Obstetrics and Gynaecology, The Faculty of Medicine, Prince of Wales Hospital, The Chinese University of Hong Kong, Hong Kong, China
- 2Department of Obstetrics and Gynaecology, Shenzhen Baoan Women’s and Children’s Hospital, Shenzhen University, Shenzhen, China
- 3Department of Ophthalmology and Visual Sciences, Hong Kong Eye Hospital, The Chinese University of Hong Kong, Hong Kong, China
- 4Department of Obstetrics and Gynecology, School of Medicine, Renji Hospital, Shanghai Jiao Tong University, Shanghai, China
- 5Department of Orthopaedics and Traumatology, The Faculty of Medicine, Prince of Wales Hospital, The Chinese University of Hong Kong, Hong Kong, China
- 6Li Ka Shing Institute of Health Sciences; School of Biomedical Sciences; and Chinese University of Hong Kong-Sichuan University Joint Laboratory in Reproductive Medicine, Prince of Wales Hospital, The Chinese University of Hong Kong, Hong Kong, China
With a rich abundance of natural polyphenols, green tea has become one of the most popular and healthiest nonalcoholic beverages being consumed worldwide. Epigallocatechin-3-gallate (EGCG) is the predominant catechin found in green tea, which has been shown to promote numerous health benefits, including metabolic regulation, antioxidant, anti-inflammatory, and anticancer. Clinical studies have also shown the inhibitory effects of EGCG on cancers of the male and female reproductive system, including ovarian, cervical, endometrial, breast, testicular, and prostate cancers. Autophagy is a natural, self-degradation process that serves important functions in both tumor suppression and tumor cell survival. Naturally derived products have the potential to be an effective and safe alternative in balancing autophagy and maintaining homeostasis during tumor development. Although EGCG has been shown to play a critical role in the suppression of multiple cancers, its role as autophagy modulator in cancers of the male and female reproductive system remains to be fully discussed. Herein, we aim to provide an overview of the current knowledge of EGCG in targeting autophagy and its related signaling mechanism in reproductive cancers. Effects of EGCG on regulating autophagy toward reproductive cancers as a single therapy or cotreatment with other chemotherapies will be reviewed and compared. Additionally, the underlying mechanisms and crosstalk of EGCG between autophagy and other cellular processes, such as reactive oxidative stress, ER stress, angiogenesis, and apoptosis, will be summarized. The present review will help to shed light on the significance of green tea as a potential therapeutic treatment for reproductive cancers through regulating autophagy.
Introduction
Male and female reproductive cancers remain one of the biggest health concerns worldwide due to the high prevalence, yet therapeutic management remains a great challenge. A low survival rate reflects the lack of efficient treatments and risks of recurrence (Garcia-Aranda and Redondo, 2019; Hanna et al., 2020; Hawsawi et al., 2020). Current treatments for reproductive cancers bring fertility-related side effects. The risk of infertility associated with gynecologic malignancies is high, with a successful pregnancy rate of only above 40% (Poorvu et al., 2019). Hysterectomy is one of the most common management of ovarian and endometrial cancers, removing the critical reproductive organs. In breast cancer, a negative impact on the reproductive system has been reported after chemotherapy (Hickey et al., 2009). Radiation therapy that is directed to the reproductive organs disturbs hormone production and imposes fertility risk. Adjuvant therapy for testicular cancer affects fecundity (Kim et al., 2010; Poorvu et al., 2019). Recently, modulation of autophagy is proposed as a novel approach to tackle cancer. In this review article, we will report the therapeutic effects of green tea, particularly EGCG, on female and male reproductive cancers and its possible mechanisms behind it. In addition, this review will emphasize the crosstalk of EGCG between its regulated anti-carcinogenic properties with autophagy on highlighting its role as an autophagy modulator toward reproductive cancer. Importantly, we hope to provide a perspective on green tea catechins and EGCG as potential autophagy modulator for the treatment of reproductive cancer.
Incidence, Mortality, and Prevalence of Reproductive Cancer
Cancer is a major leading burden of disease worldwide, accounting for nearly 10 million deaths in 2020, or nearly one in six deaths. In 2050, an estimated 6.9 million new cancers will be diagnosed in adults aged 80 years or older worldwide (20.5% of all cancer cases) (Pilleron et al., 2021). Among both male and female, reproductive cancer contributes to a high incidence and mortality on the causes of death worldwide (Garcia-Aranda and Redondo, 2019; Hawsawi et al., 2020). The following cancers are exclusive to the female reproductive system including cancer originating at the ovary (ovarian cancer), cervix uteri (cervical cancer), and corpus uteri (endometrial cancer). While these cancers are exclusive to the male reproductive system, these include cancer originating at the prostate gland (prostate cancer) and the testis (testicular cancer). As for breast cancer, this can affect both males and females.
Ovarian cancer cells arise from epithelial, stromal, or germ cells of the ovary (Horowitz et al., 2020). The cells disseminate through the perineal cavity and metastasize to the omentum (Yeung et al., 2015). On the first hand, cancer cells from primary tumor cells can passively be disseminated to a newly established site, with enhanced cancer cells adhesion, invasiveness, motility, and a supportive microenvironment. On the other hand, it can undergo a hematogenous metastatic mechanism via epithelial-mesenchymal transition (Yeung et al., 2015). In 2020, ovarian cancer accounts for 313,959 new cases, and 207,252 deaths. The crude death rate of ovarian cancer was 5.4 per 100,000 female population. The age-standardized death rate of ovarian cancer was 4.2 per 100,000 standard population (WHO, 2022). Diagnosis of ovarian cancer is often delayed; it could be more treatable if it is diagnosed earlier (Evans et al., 2007).
For cervical cancer, it has been widely accepted that Human papillomavirus (HPV) infection is an attributable factor (Walboomers et al., 1999). HPV oncoproteins E6 and E7 block p53 and retinoblastoma protein, which function as tumor suppressors, as well as engage in multi-step carcinogenesis such as viral DNA replication and proliferation, leading to invasive cervical cancer development (Walboomers et al., 1999; Yugawa and Kiyono, 2009; Den Boon et al., 2015; Gupta and Mania-Pramanik, 2019). Worldwide, cervical cancer is reportedly the fourth most incident cancer and the fourth leading cause of cancer-related death, with 604,127 new cases and 341,831 deaths in women in 2020. Early on, typically no symptoms are seen. Later, symptoms may include abnormal vaginal bleeding, pelvic pain, or pain during sexual intercourse (WHO, 2022).
Endometrial cancer can be endometrioid (type 1), arising from excess stimulation of estrogen and atypical glandular hyperplasia; or non-endometrioid (type 2) (Lu and Broaddus, 2020; Terzic et al., 2021). Type 1 tumors are associated with significantly enhanced oncogene mutations and tumor suppressor genes; hence, it resembles proliferative endometrium (Hecht and Mutter, 2006; Morice et al., 2016). Type 2 tumors are mainly associated with enhanced TP53 and HER2/neu mutations (Morice et al., 2016; Terzic et al., 2021). In 2020, endometrial cancers newly occurred in 417,367 women and caused 97,370 deaths. This makes it the third most common cause of death in cancers which only affect women, behind cervical and ovarian cancer. Endometrial cancer arises from the endometrium (the lining of the uterus or womb) and is associated with excessive estrogen exposure, high blood pressure, and diabetes. And often, regular screening in those at normal risk is not common.
Breast cancer originates within the inner lining of ducts or the lobules, then disseminates to lymph nodes or other nearby sites. The major cause of mortalities includes metastasis with enhanced cells, or with dysregulated immune responses (Zheng et al., 2017). In 2020, breast cancer is the most diagnosed cancer, with 2.3 million cases and 685,000 deaths globally (Sung et al., 2021). It affects 1 in 7 (14%) women worldwide, making it the world’s most prevalent cancer (Balasubramanian et al., 2019). Breast cancer occurs in every country of the world in women at any age after puberty but with increasing rates in later life (WHO, 2021).
Genetic and environmental factors contribute to the development of testicular germ cell tumors, supported by ethical and geographic evidence (Al-Obaidy et al., 2020). It is also reported that isochromosome 12p alternation is especially associated with the progression and invasiveness of the tumors; and it can be related to abnormalities in malignant transformation, oxidative damage, and DNA repairing (Reuter, 2005; Cheng et al., 2017; Al-Obaidy et al., 2020). Globally, testicular cancer affected about 74,458 new cases in 2020, which resulted in 9,334 deaths in 2020. As found, rates are lower in the developing than the developed world. Onset most commonly occurs in males 20–34 years old, rarely before 15 years old (Hayes-Lattin and Nichols, 2009). Most often, treatment outcomes are better when the disease remains localized.
Prostate cancer development can be via intraepithelial neoplasia which is the hyperplasia of luminal cells and progressive loss of basal cells. On the other hand, it is also reported as an androgen-dependent adenocarcinoma, that is characterized by the strong phenotype of lumina cells but without basal cells. In contrast, it can also be castration-resistant and independent of androgens (Testa et al., 2019; Tong, 2021). Prostate cancer is the fourth most incident cancer worldwide, with 1,414,259 new cases and 375,304 deaths in 2020. In males, it was the second most common cancer and had the highest prevalence in 2020. Factors that increase the risk of prostate cancer include older age, family history, and race. About 99% of cases occur after age 50 (Rawla, 2019).
Overall, reproductive cancers comprised 28.11% of incidence, 17.34% of mortality, and 38.05% of prevalence (5 years) of all cancers in the world in 2020, demonstrating its relevance as a public health problem (Table 1). The molecular mechanism of reproductive related cancers had been extensively reviewed, which is a complex carcinogenesis process accompanied by related pathophysiology (Zheng et al., 2017; Gupta and Mania-Pramanik, 2019; Testa et al., 2019; Al-Obaidy et al., 2020; Horowitz et al., 2020; Terzic et al., 2021). These include enhanced growth factors and oncogenes but resisting apoptotic factors and tumor suppressors, as a result of enhanced invasiveness and metastasis. These processes are regulated by different signal transduction systems such as the cell cycle and DNA repair system (Torgovnick and Schumacher, 2015; Li et al., 2020). Noteworthily, there is a rapid growth of research that focuses on the autophagy roles in cancer pathophysiology. Our team previously conducted a comprehensive and systematic analysis on the roles of autophagy in cervical, endometrial, and ovarian cancer progression and chemoresistance (Tam et al., 2021). We agreed with the argumentative roles of autophagy, which are inconsistent with those proposed in previous cancer studies (Janku et al., 2011; Mowers et al., 2017; Lim and Murthy, 2020). In this review, we extend the research to explore autophagy modulators in reproductive cancer.
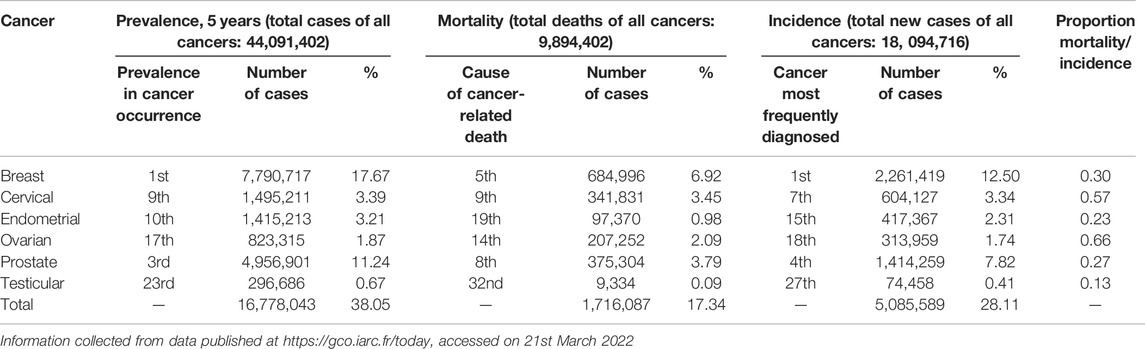
TABLE 1. Incidence, mortality, and prevalence of male and female reproductive cancers worldwide in 2020.
The Role of Autophagy in Reproductive Cancer
In normal physiological conditions, autophagy typically would be maintained at a minimal level of activity. Mainly, cells utilize basal levels of autophagy to aid in the maintenance of biological function, homeostasis, quality-control of cell contents, and elimination of old proteins and damaged organelles for cell survival (Levine and Yuan, 2005; Mizushima et al., 2008). Autophagy happens in lysosome which has a membrane to limit the leakage of the degradative enzyme. There are micro- and macro-autophagy. The former engulfs the cytosolic compound directly by lysosomes, and the latter involves the sequestration of cargoes within an autophagosome, which is a double membrane cytosolic vesicle formed from a phagophore. Macro-autophagy can be specific and targets organelles or invasive microbes, as well as can be nonspecific. The degradation process of autophagosome happens in autolysosome and macromolecules will be released back into the cytosol (Levine and Kroemer, 2008; Mizushima et al., 2008; Mizushima and Levine, 2020). Additionally, the regulation of autophagy can modulate cancer cells’ growth or death.
During the initiation stage of autophagy, phagophore assembly site (PAS) is formed, along with UNC51-like kinase (ULK)1/2 and autophagy-related protein (ATG)13 which translocate to the endoplasmic reticulum to facilitate the formation of class III PI3K-Beclin1-VPS34 complex as phagophore. Phagophore undergoes elongation to form autophagosome, which requires two ubiquitin-like conjugate systems: ATG12-ATG5-ATG16 multimeric system and Phosphatidylethanolamine (PE) to microtubule-associated protein 1 light chain (LC)3 conjugate system. ATG7 and ATG4 facilitate the binding of LC3-PE, which are essential for the expansion of the autophagosome membrane to recognize autophagic cargos. During the maturation stage, the cargo sequestration is completed. The resulting autophagosome fuses with a lysosome to form an autolysosome, meanwhile, lysosomal enzyme degrades the autophagosome contents. At last, the cargo is broken down and released into the cytosol. The lysosome is recycled during the degradation stage (Levine and Kroemer, 2008; Mizushima et al., 2008; Feng et al., 2014; Kaur and Debnath, 2015; Mizushima and Levine, 2020). Based on our previous findings, we reported that several autophagy regulators, consisting of ULK1, Beclin1, ATG5, p62, and LC-3BII, were related to the growth of cervical, endometrial, and ovarian cancer (Tam et al., 2021). Similarly, these autophagy regulators, including Beclin1 and LC-3B, were also shown to modulate prostate adenocarcinoma and breast cancer (Naponelli et al., 2015; Tyutyunyk-Massey and Gewirtz, 2020) while p62, LC3B, and Beclin1 played critical rolesin the survival of testicular cancer (Nakkas et al., 2021). Therefore, by targeting these autophagy regulatory factors directly or indirectly, it could potentially be used to inhibit the progression of reproductive cancer tumors.
Promotion of Cancer Growth
Autophagy is a physiological cellular process for the degradation and elimination of misfolded proteins and damaged organelles that function in adaptation to starvation, development, cell death, and tumor suppression (Klionsky et al., 2021). This process allows unneeded proteins to be degraded and the amino acids recycled for the synthesis of proteins that are essential for survival. Toward cancer survival, autophagy plays dual roles in contributing to the growth of cancer as well as tumor suppression (Klionsky et al., 2021). Such that, the growth of cancer can be promoted by maintaining the survival of tumor cells that have been starved, or to degrade apoptotic mediators, through autophagy (Su et al., 2013). This can be induced by cytotoxic and metabolic stresses, including hypoxia and nutrient deprivation, to activate autophagy for recycling of ATP and to maintain cellular biosynthesis and survival, which promotes HIF-1α-dependent and -independent activation (Semenza, 2010). This activation would increase the expression of angiogenic factors, such as vascular endothelial growth factor (VEGF), platelet-derived growth factor, and nitric oxide synthase. Also, the induction of autophagy by miRNA-4673 has been found to improve the resistance of cancer cells to radiation (Dokumcu et al., 2018). In addition, suppression of autophagy by deletion of Beclin 1 enhances cell death (Degenhardt and White, 2006; White and Dipaola, 2009). Autophagy presents the cytoprotective ability to cancer cells and favors cell survival by coping with the intracellular and environmental stress. Therefore, potent inhibitors to limit the progression of autophagy can be an alternative strategy (Amaravadi et al., 2019; Mizushima and Levine, 2020), such as Metformin in prostate cancer (Farrow et al., 2014); Chloroquine in prostate, breast, and endometrial cancer (Solomon and Lee, 2009; Farrow et al., 2014; Nunez-Olvera et al., 2019); Bortezomib and HDAC6 inhibitor Panobinostat in breast cancer (Maycotte and Thorburn, 2014); Elaiophyl in ovarian cancer (Zhao et al., 2015) etc. Autophagy inhibitors should be used only if cancer cells are more reliant on autophagy than normal cells (Mizushima and Levine, 2020).
Promotion of Cancer Cell Death
Various studies have shown the regulation of autophagy would contribute to the expression of tumor suppressor proteins or oncogenes (Avalos et al., 2014). The previous study has shown xenografts with a lowered expression of Beclin1, a protein that regulates autophagy, to be tumor-prone (Qu et al., 2003). However, another study demonstrated when Beclin1 was overexpressed, the tumor development was inhibited (Liang et al., 1999). Likewise, autophagy can indirectly prevent the release of proinflammatory HMGB1 by limiting necrosis and chronic inflammation to protect against tumorigenesis (Tang et al., 2010). Autophagy brings cytotoxicity effects to promote tumor cell death, which can be completed through degradation of oncogenic proteins (Janku et al., 2011), or via reducing metabolic stress, regulation of oxidative stress, or metastasis during tumorigenesis. DNA would be damaged and lead to genomic instability (Mathew et al., 2009; Mizushima and Levine, 2020). It responds to a range of cellular stresses, including nutrient deprivation, organelle damage, and abnormal protein accumulation. Autophagy inducers may be beneficial when there are excess abnormal macromolecules and organelles (Mizushima and Levine, 2020), for example, Rapamycin in breast cancer (Danko et al., 2021); Trastuzumab in HER2 positive breast cancer (Maycotte and Thorburn, 2014); Atorvastatin in prostate cancer (Farrow et al., 2014); Metformin in cervical, breast and endometrial cancer (Lu G. et al., 2021), etc.
As mentioned, some anticancer drugs have been shown to regulate autophagy (Cuomo et al., 2019). However, targeting these autophagy modulators as a therapeutical strategy remains a huge obstacle (Levy et al., 2017; Amaravadi et al., 2019). Different side effects were reported, ranging from diarrhea, and sensitization to chemotherapy and anemia (Buzun et al., 2021). Autophagy inhibitors had also been reported to sensitize immune response in therapy, as well as influence tumor stroma and normal cell function (Gewirtz, 2016; Noguchi et al., 2020). Hence, natural product as autophagy modulator has been proposed, owing to their safe profiles whilst showing high effectiveness to diminish tumor cells (Zhang Q. Y. et al., 2018; Rahman et al., 2020; Al-Bari et al., 2021). Natural product to regulate autophagy is also a novel therapeutic approach in improving fertility (Zhu et al., 2019; Park et al., 2021). In addition, natural products can be used to target multiple pathways, thus providing greater flexibility and potential for a variety of cancer types and stages.
Green Tea
Tea is brewed from the leaves and buds of the plant Camellia sinensis. With extreme popularity particularly in Asia, it is ranked the second most common beverage consumed worldwide (behind water) (Graham, 1992). In general, teas from this plant can be grouped into green, black, or oolong tea. Among the tea consumption, approximately 20% is in the form of green tea. As green tea has not undergone the same withering and oxidation process as black or oolong tea, it contains the highest amounts of natural polyphenols (catechins) (around 5–7 mg/g in green tea; while that in black tea is only around 0–3 mg/g). These catechins account for approximately 30–40% of the extractable solids of dried green tea leaves, alkaloids (such as caffeine and theobromine), carbohydrates, tannins, and minerals (such as fluoride and aluminum) (Kochman et al., 2020). These catechins have been shown to be more powerful antioxidants, both in vitro and in vivo, when compared to vitamins C and vitamins E (Cabrera et al., 2006; Khan and Mukhtar, 2008; Chacko et al., 2010). The four-major green tea catechins include (−)-epicatechin (EC), (−)-epigallocatechin (EGC), (−)-epicatechin-3-gallate (ECG), and (−)-epigallocatechin-3-gallate (EGCG), which EGCG is the most abundant and biologically active (Schramm, 2013) (Supplementary Figure S1). Green tea has been shown to regulate a range of health issues such as improves digestive and brain function, promotes metabolism, as well as fights and protects against cardiovascular disease, diabetes, cancer and others (Cabrera et al., 2006; Khan and Mukhtar, 2008; Chacko et al., 2010; Kochman et al., 2020). Based on previous studies, the consumption of green tea daily was found to be correlated with a slightly lower risk of death from cardiovascular causes (Xi et al., 2015) and a reduced risk of stroke (Zhang et al., 2015). As EGCG is a powerful antioxidant, it is believed to be an important determinant in the therapeutic qualities of green tea. As the structure of EGCG possessed a gallic acid, the presence of the gallate moiety and the extra phenol ring on EGCG enhance its anticancer property, which traps electrons, as a scavenger of free radicals and reduces oxidative stress (Du et al., 2012; Chu et al., 2017). This powerful antioxidant property of EGCG can suppress neovascularization and regulate vessel permeability, thereby cutting off the blood supply to cancerous cells (Maiti et al., 2003).
Potential Anticancer Effect of Green Tea
Green tea catechin was shown to target cell cycle regulatory proteins, inhibit cell division, stimulate cell cycle arrest mechanism and promote apoptosis, thus is effective against the progression of the tumor. As green tea catechins, in particular, EGCG, are powerful antioxidants, it is believed they are important determinants in the therapeutic qualities of green tea. As the structure of EGCG possessed a gallic acid, the presence of the gallate moiety and the extra phenol ring on EGCG enhance its anticancer property, which traps electrons, as a scavenger of free radicals and reduces oxidative stress (Du et al., 2012; Chu et al., 2017). This powerful antioxidant property of EGCG can suppress neovascularization and regulating vessel permeability, thereby cutting off the blood supply to cancerous cells (Maiti et al., 2003). EGCG also regulated various transcription factors, protein kinases, and inflammatory cytokines to mediate the invasion, metastasis, and progression of cancerous cells (Shirakami and Shimizu, 2018; Kochman et al., 2020). The summary of the anticancer properties of green tea is shown in Figure 1.
Experimental Studies
Toward ovarian cancer, experimental studies uncovered the mechanisms of green tea, which are mainly involved in regulating cell proliferation and apoptosis in cells or xenograft models. Green tea targeted CD44 as a safe nanomedicine treatment, thus inhibiting tumor progression and recurrence (Bae et al., 2017). It had been demonstrated that EGCG efficacies via targeting a number of genes including proliferative related genes JUN, FADD, NFKB1, HIF1α, and matrix metalloproteinases (MMPs) (Xinqiang et al., 2017), apoptotic related genes Bcl-2, Bax, caspases (Panji et al., 2021a) as well as downregulated PTEN/AKT/mTOR pathway to inhibit proliferation in vitro and in vivo (Qin et al., 2020). EGCG promoted DNA damage response, reduced DNA synthesis, and enhanced oxidative stress to induce apoptosis in cells (Mazumder et al., 2012) (Rao and Pagidas, 2010) (Chan et al., 2006).
Towards cervical cancer, green tea extract was reported to suppress TGF-β induced epithelial–mesenchymal transition via reactive oxygen species (ROS) generation, as well as inhibited proliferation and induced apoptosis via VEGF, NF-KB, and Akt pathways in cell lines or mice with HeLa xenografts (Singh et al., 2011; Panji et al., 2021b; Khojaste and Ahmadizadeh, 2021). EGCG reduced DNMT to regulate DNA hypomethylation (Khan et al., 2015), induced cell cycle arrest (Ahn et al., 2003), and downregulated p53 expression to regulate proliferation and apoptosis (Muthusami et al., 2013). EGCG inhibited hypoxia-induced HIF-1α to inhibit VEGF, thus regulating angiogenesis (Zhang et al., 2006). Toward endometrial cancer, the effect of the prodrug of EGCG (ProEGCG) exhibited anti-proliferative, pro-apoptotic, and anti-tumor actions via ERK/JNK, Akt and PI3K/AKT/mTOR/HIF1α signaling pathway (Wang Q. et al., 2018; Man et al., 2020).
Toward breast cancer, the anti-proliferative and pro-apoptotic effects of green tea catechins were achieved via inhibiting VEGF transcription (Sartippour et al., 2002), increasing Bax/Bcl-2 ratio (Li W. et al., 2016) or inducing p27 (kip1) CK1(Kavanagh et al., 2001), cell cycle arrest (Liu et al., 2017), caspases (Mbuthia et al., 2017) and p53 (Santos et al., 2021). In particular, EGCG mediated multiple signaling pathways to inhibit cell proliferation, migration, and invasion, thus limiting tumor progression, which included PI3K, Akt, NF-κB, MAPK, Wnt (Pianetti et al., 2002; Pan et al., 2007) and estrogen receptor (Belguise et al., 2007; Li et al., 2010) signaling pathways. Additionally, EGCG served as an antiangiogenic inhibitor that downregulated the VEGF pathway (Sartippour et al., 2002; Braicu et al., 2013; Crew et al., 2015), as well as an immune modulator that targeted IL-6 and TNF-α (Jang et al., 2013). Several studies also revealed the anti-invasive ability of catechins through mediating MMPs(Roomi et al., 2005a), VASP(Zhang et al., 2009), FOXO3a (Belguise et al., 2007), or p53 (Santos et al., 2021) expressions. In addition to acting as a chemo-preventive agent, green tea catechins also reversed chemotherapy resistance (Esmaeili, 2016; Tuntiteerawit et al., 2020).
While in testicular cancer, in vitro study revealed the potential of green tea extract to inhibit MMP and regulated invasion (Roomi et al., 2007). Similarly, studies on prostate cancer showed that green tea catechins suppressed cell proliferation and induced apoptosis by an increased Bax/Bcl-2 ratio (Yang et al., 2019) and via regulating the cell cycle (Wang et al., 2012), PI3K, Akt and Erk pathways (Siddiqui et al., 2004). Furthermore, green tea catechins suppressed invasion and migration by TIMPs and MMPs(Deb et al., 2019; Fan et al., 2021); inhibited tumor angiogenesis by downregulating IGF-1 (Harper et al., 2007), HIF1α (Thomas and Kim, 2005) and VEGF (Roomi et al., 2015b); and targets NK-κB (Saedmocheshi et al., 2019), COX-2 (Adhami et al., 2007), interleukin family (Mukherjee et al., 2014), androgen receptor (Stanislawska et al., 2019) to suppress prostate cancer cell growth. A summary of studies based on animal models about the anti-cancer effects of EGCG and green tea catechins on reproductive cancers can be found in Table 2.
These experimental studies demonstrated the ability of EGCG to regulate multi-targets and multi-signaling pathways. Some of these targeted pathways presented potential mechanism of actions of EGCG to regulate autophagy in reproductive cancers. In particular, studies showed that the inhibition of autophagy was conducted via inhibiting the MAPK pathway mechanistically (Han et al., 2021; Tam et al., 2021). The Akt/mTor signaling pathway act as the main autophagy regulator for the survival of reproductive cancers (Amaral et al., 2018; Nunez-Olvera et al., 2019; Tam et al., 2021; Wang et al., 2021). Moreover, the NF-
Clinical Trials
Currently, there are very few clinical trials performed to validate whether green tea possesses chemo-preventive or curative activity in significantly reducing cancer development. In clinical trials on prostate cancer, the majority of studies showed significant beneficial effects of green tea extracts on reducing cancer progression than placebo (Bettuzzi et al., 2006; Brausi et al., 2008; Mclarty et al., 2009; Ram Kumar and Schor, 2018). While in breast cancer, the studies by Samavat et al. demonstrated the consumption of green tea extracts significantly reduced breast tumors in female patients ((Samavat et al., 2017; Samavat et al., 2019). However, it showed no significant effects when conducted by another study group (Crew et al., 2012; Crew et al., 2015)). These results remained inconsistent owing to the presence of numerous confounding variables in these study designs, such as diet and population differences. In addition, prospective and case-control studies had demonstrated the potential of green tea in reducing the risk of ovarian cancer (Zhang et al., 2002; Yoon et al., 2008; Lee et al., 2013), cervical cancer (Jia et al., 2012; Paul et al., 2019), endometrial cancer (Bandera et al., 2010), breast cancer (Dai et al., 2010; Iwasaki et al., 2010; Iwasaki et al., 2014; Li M. et al., 2016; Yuan et al., 2017), and prostate cancer (Kurahashi et al., 2008; Erdrich et al., 2015; Lassed et al., 2016; Lee et al., 2017; Sawada, 2017; Beynon et al., 2019). A summary of studies based on clinical trials about the anti-cancer effects of EGCG and green tea catechins on reproductive cancers can be found in Table 3.
Regulation of Autophagy by EGCG and Green Tea Catechins in Reproductive Cancer
As autophagy is regulated by different autophagy-related proteins that could be potential molecular targets in reproductive cancers. Likewise, EGCG has demonstrated its potential to modulate these factors in current literatures. EGCG restored autophagic flux via downregulating Beclin1, Atg5, and p62 in myocardial ischemia/reperfusion injury rats (Xuan and Jian, 2016). Also, EGCG was reported to inhibit dimerization of LC-3BI, promoted LC3B-II production, and activated autophagy in hepatocellular carcinoma cells (Zhao et al., 2017). By promoting the upregulation of Atg5, Beclin-1, and LC3B-II protein levels, EGCG induced apoptosis and autophagy in cisplatin-resistant oral cancer (Yuan et al., 2017). Also, other studies showed that EGCG enhanced LC3 transition and induced Beclin-1in lymphoma (Tsai et al., 2017). Whereas, it regulated Atg5 and Beclin-1 to induce autophagy in subarachnoid hemorrhage (Chen et al., 2018). This regulation of EGCG on the protein level of Atg5 was essentially critical in governing the autophagy and apoptosis switch, as lysosomes would not be able to fuse with phagosome when it was absent (Yousefi et al., 2006). However, the concrete evidence on the modulating effect of EGCG and green tea catechin on autophagy regulation remained controversial. Their role in determining the onset of autophagy might also be dependent on the integration of signaling networks, in which autophagy and cell growth often shared a series of direct or indirect signaling pathways (Neufeld, 2012; Rizzi et al., 2014).
MAPK had been shown to act as a prognostic factor to regulate the incidence of several reproductive cancers ((Eritja et al., 2017; Lim et al., 2019)). EGCG inhibited various transcriptional factors of mitogen-activated protein kinase (MAPK), including c-Jun, and ERK1/2, to inhibit proliferation, and migration and promote apoptosis (Katiyar et al., 2001; Maeda-Yamamoto et al., 2003). As matrix metalloproteinases (MMPs) were correlated with tumor invasion via MAPK, MMPs were reduced upon the treatment with EGCG to revoke the activation of ERK and JNK, which prevented fibrosis, invasion, and migration of cancer cells (Kim et al., 2004; Zhen et al., 2006; Ho et al., 2007; Zeng and Harris, 2014). Autophagy also acts as an adaptive resistance mechanism upon the inhibition of MAPK (Lee et al., 2021). MAPK signaling pathway did not solely regulate autophagy (Raufi et al., 2021). The significance of autophagy against MAPK inhibition was also dependent to the cell’s ability to produce autolysosomes (Kochetkova et al., 2017). Autophagic mechanism was activated dependent on MAPK/JNK pathway after therapeutic stress in endometrial cancer (Eritja et al., 2017). In ovarian cancer, the stimulation of tumor cell proliferation via the MAPK/ERK1/2/mTor pathway induced autophagy (Lim et al., 2019). Likewise, the promotion of autophagy was found to inhibit tumor cell growth in a breast cancer study via the inhibition of MAPK-regulated mTOR activity, and the phosphorylation of ERK was hindered (Sun et al., 2013). Moreover, the downregulation of p-ERK1/2 protein via the MAPK/ERK pathway induced early autophagy and apoptosis for anti-tumor activities in breast and cervical cancer (Hanyu et al., 2020; Liu et al., 2020). On the contrary, the promotion of MAPK/ERK pathway was shown to inhibit autophagy on promoting metastasis (Li et al., 2021).
In another aspect, EGCG targeted PI3K/AKT/mTOR pathway via inhibiting phosphorylation of AKT and mTOR to exert anti-proliferation and pro-apoptosis effects (Liu et al., 2013; Liu et al., 2016). EGCG also inhibited the binding of EGF to EGFR by reducing receptor dimerization and inhibiting EGFR phosphorylation (Adachi et al., 2007). These regulations indirectly inhibited AKT activities to induce cell cycle arrest (Sah et al., 2004; Hou et al., 2005; Shimizu et al., 2005) As a result, EGCG was shown to increase caspases and anti-apoptotic proteins level, and decrease AKT and pro-apoptotic levels (Moradzadeh et al., 2017). The inhibition of the PI3K/AKT/mTOR pathway promoted autophagy for the anti-proliferation activity in endometrial cancer cells (Lin et al., 2019). The blockade of the AKT/mTOR pathway activated the compensatory MEK/ERK pathway to promote autophagy and apoptosis induced cell death and cell cycle arrest in prostate, cervical and ovarian cancers (Zhou et al., 2015; Xu et al., 2016; Butler et al., 2017; Qin et al., 2018). Another study also showed that the downregulation of p-AKT inhibited cancer cell survival, along with the upregulation of p-JNK1/2-induced apoptosis, synergistically lead to autophagy-induced cell death in the breast cancer cells (Innajak et al., 2016). The promotion of apoptosis and autophagic cell death via inhibiting the PI3K/AKT/mTOR signaling pathway could have induced stress to attenuate MAPK/ERK activity in autophagy inhibition (Reddy et al., 2020).
In addition, EGCG also suppressed anti-apoptotic related protein through the inhibition of the NF-
EGCG can work like various chemotherapeutic drugs, which enhanced autophagy-induced cancer cell death (Denton et al., 2015). Currently, EGCG as an autophagy inducer was only studied in breast, cervical, and prostate cancers. The summary on the finding of EGCG and green tea catechins on autophagy is shown in Table 4. In breast cancer, EGCG inhibited tumor growth in vitro and in vivo via activating autophagy directly, thus regulating glucose metabolism (Wei et al., 2018). Another study in the breast cancer cell line showed that EGCG induced autophagy via enhancing autophagosome formation, hence inhibiting proinflammatory mediators HMGB1 (Li et al., 2011). The regulated stages of autophagy in breast cancer were shown to be dependent on the EGCG concentration. EGCG in low concentration upregulated Beclin1, Atg5, and LC3B-II/LC3B-I levels, promoting autophagosomes; However, when EGCG is under high concentration, it would only upregulate Beclin1 and Atg5 to enhance the fusion of the autophagosome with lysosomes to form autolysomes, which resulted in a downregulated LC3B-II/LC3B-I levels. Whereas, high concentrations of EGCG were shown to activate later stages of autophagy in breast cancer cells (Wei et al., 2018).
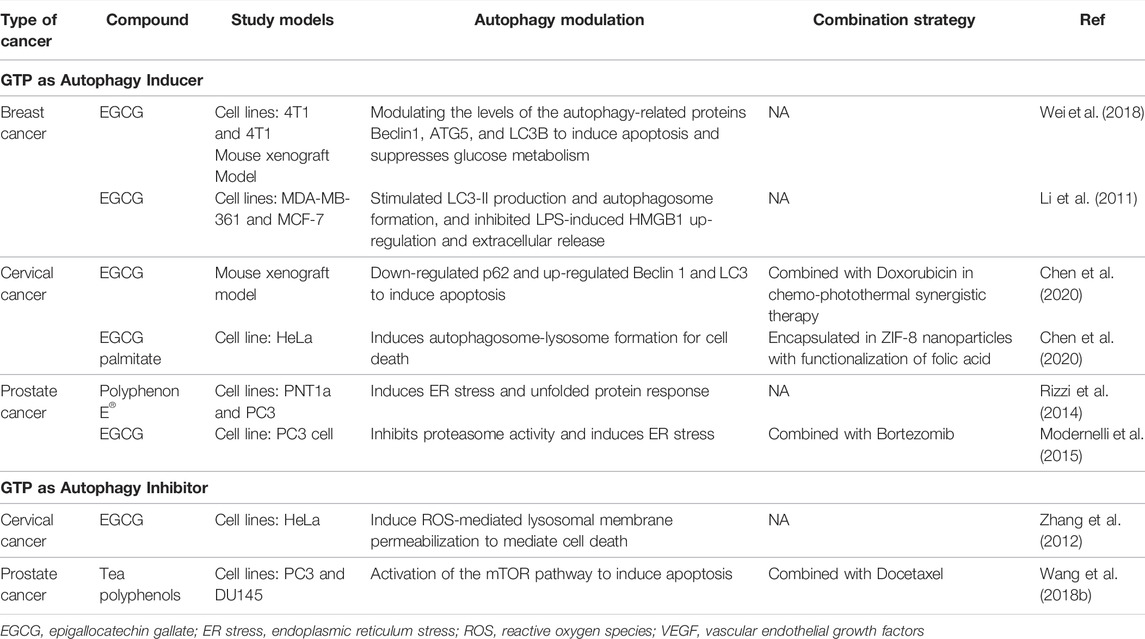
TABLE 4. Green tea polyphenol (EGCG) as autophagy modulator in cancer study from 2011 to 2022 January.
In cervical cancer, the combination of EGCG and Doxorubicin, which is chemotherapy, was investigated as an autophagy modulator that further improved the therapeutic efficacy of photothermal therapy (Chen et al., 2020). Photothermal therapy solely induced hyperthermia damage to intracellular components and activated autophagy which inhibited apoptotic-induced cancer cell death (Zhang Y. et al., 2018; Li et al., 2019; Chen et al., 2020). However, an autophagy enhancer could suppress inflammation and cancer progression, and therefore promote autophagic-induced cell death. When autophagic flux and the formation of autophagosomes were activated, this would downregulate p62 while upregulating both Beclin1 and LC3 in tumors. Additionally, EGCG also played roles to inhibit the toxins generated from Doxorubicin in vitro and in vivo, and limited chemo-resistance, resulting in greater efficiency (Shan et al., 2019; Chen et al., 2020)
In prostate cancer, Bortezomib is an effective proteasome inhibitor against tumor growth via induction of apoptosis in vitro and in vivo (Papandreou and Logothetis, 2004), followed by activation of unfolded protein regulator, ER stress, and autophagy (Modernelli et al., 2015; Takenokuchi et al., 2015). The proteasome is mechanistically related to autophagy as both pathways act as protein degradation systems and maintain intracellular proteostasis (Zhu et al., 2010). EGCG potently suppressed proteasome activity in prostate cancer (Kazi et al., 2004), and could potentially induce autophagy as Bortezomib. A study hence showed that co-treatment of Bortezomib with EGCG exacerbated autophagy via induction of LC3B transition and an autophagic flux in prostate cancer cells (Modernelli et al., 2015). The therapeutic efficacy of green tea as an autophagy inducer depended on the stage of cancer. Green tea polyphenol activated autophagy in the initial stage of prostate cancer in vitro, demonstrated by an increased interconversion of LC3B-I to LC3B-II, which indicated the accumulation of inactivated autophagosomes (Rizzi et al., 2014). However, in advanced stages of prostate cancer in vitro, although green tea increased the level of LC3B, there was no change in the interconversion level of LC3B-I to LC3B-II to indicate a net autophagic flux (Mizushima and Yoshimori, 2007; Rizzi et al., 2014).
Autophagy degrades unusual macromolecules and organelles that are used to induce apoptosis. An upregulated level of autophagy was shown to limit drug-induced apoptosis in prostate cancer (Herman-Antosiewicz et al., 2006). Induction of autophagy favored cancer cells’ survival under metabolic stress conditions stimulated by chemotherapy or radiation therapy (Ozpolat and Benbrook, 2015). Therefore, a downregulated level of autophagy could enhance the pro-apoptotic properties of chemotherapy. In prostate cancer, patients developed resistance against first-line therapy such as docetaxel (Armstrong and Gao, 2015), which could be due to the induction of autophagy (Wang Q. et al., 2018). Green tea polyphenol inhibited the induced-autophagy events and promoted apoptosis via activation of the mTOR signaling pathway. LC3-II was reduced to enhance the p-mTOR expression level (Wang Q. et al., 2018). The schematic diagram of the chemo-preventive mechanisms of EGCG and green tea catechins is shown in Figure 2.
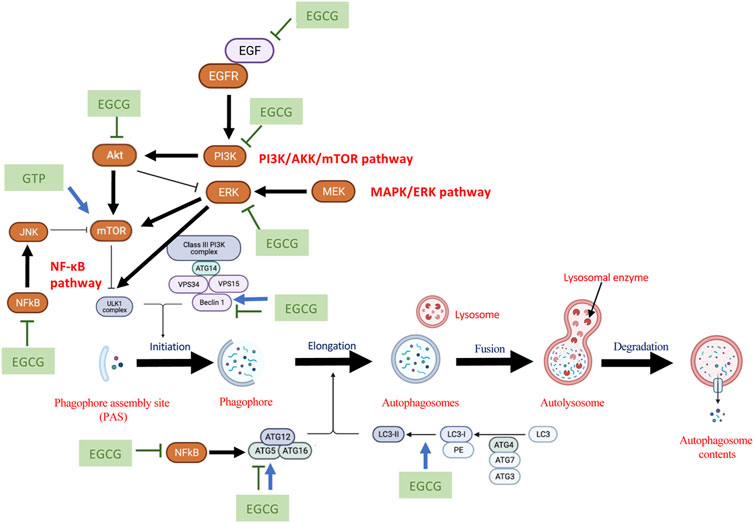
FIGURE 2. The schematic diagram of potential chemopreventive mechanisms of EGCG and green tea catechins to regulate autophagy. The schematic diagram was created using BioRender.com.
Potential of EGCG as an Autophagy Modulator in Reproductive Cancer
Pharmacological actions of autophagy modulator are not tumor-selective, which could impose a risk of healthy tissue being transformed into malignant tissue (Gewirtz, 2016; Noguchi et al., 2020). Although autophagy was shown to induce cancer cell death, the anti-oxidative properties of green tea polyphenols inhibited stress-induced autophagy cell death in healthy ovarian granulosa cells. Activities and concentrations of antioxidant SOD and GSH-PX were upregulated. MDA levels, Beclin1, as well as the autophagic vacuoles in cytoplasmic were reduced (Yang et al., 2020). EGCG reduced ROS to limit the enzyme that cleaved LC3-I to form LC3-II, and inhibited LC3-II formation, indicating a reduced level of autophagosome formation and autophagy in healthy ovarian cells (Zhang S. et al., 2020; Yang et al., 2020). Autophagy was induced by environmental stress such as chemicals and toxins (Lee et al., 2011). EGCG shows the potential to regulate autophagy and protect healthy cells against death from chemotherapy.
Reproductive cancer treatments could interfere with the reproductive processes and fertility. EGCG was shown to improve fertility and protect it from reproductive cancers. Its molecular mechanism against infertility is based on regulating ROS, enhancing the antioxidant enzymes’ expression and activity (Roychoudhury et al., 2017; Zhang Y. et al., 2020). From the male fertility aspect, EGCG was able to protect germ cell loss, as well as diminish testes lesions (Ge et al., 2015), sperm deformity (Spinaci et al., 2008), and spermatogenic cell apoptosis (Ge et al., 2015). EGCG in a low concentration improved motility and capacitation of sperm. The addition of EGCG in thawing sperm for in-vitro fertilization after chemotherapy significantly increased penetration rate and fertilization efficiency (Rahman et al., 2018). DNA damage on semen from ROS was protected, and the fertility rate was significantly increased. While on the other hand, from the female fertility aspect, EGCG improved developmental capacity and the porcine oocytes’ maturation quality in vitro. EGCG in premature cultures of oocytes delayed oxidation and reduced ROS in vitro (Roth et al., 2008; Balazi et al., 2019). EGCG inhibitory actions of AMPK, cAMP, calciumion, and ferric iron prevented chromosome impairment, low-density lipoprotein oxidation, and spontaneous mutation. This reduced ROS and regulated gene expression to improve fertility (Rahman et al., 2018).
Current Limitation and Future Perspectives of EGCG Development
Autophagy modulators can act as tumor activators or suppressors, which complicates the discovery stage in terms of pharmacological, technical, and experimental natures. It must be solved before implement as a chemotherapeutic modulator in the clinic. The autophagy strategy should be customizable and used depending on cancer cell types and tumorigenesis stages. Natural products such as cancer therapies attract growing attention due to their high effectiveness to diminish tumor cells and low toxicity to normal cells (Zhang Q. Y. et al., 2018). Their autophagy roles have been put forward (Rahman et al., 2020; Al-Bari et al., 2021). We reviewed and proposed the use of green tea EGCG as an autophagy modulator in reproductive cancer studies. EGCG exerts extraordinary anti-carcinogenic properties, attributes to its multi-targets and multi-pathways functional properties from anti-proliferative, proapoptotic, anti-invasive, anti-angiogenesis, and anti-oxidative effects (Chacko et al., 2010; Gan et al., 2018; Kochman et al., 2020). It was tested with a safe profile and benefited the preservation of fertility (Rahman et al., 2018; Opuwari and Monsees, 2020), yet it still requires more validation using animal studies and clinical trials to prove the efficacies of EGCG. Fertility-related side effects of EGCG for reproductive cancers in clinical use also needs to be investigated further.
However, the hydroxyl group on EGCG brings low bioavailability in the human body (Du et al., 2012; Chu et al., 2017). EGCG is not stable and is readily oxidized in a neutral or alkaline environment. A basic environment disfavors the protons on phenol groups, causing it to generate phenoxide anion which acts on electrophilic agents such as free radicals in the body (Lam et al., 2004). With innovations and technologies, this could be overcome via different delivery methods including prodrug and encapsulation approaches. Lam et al., team’s derived a prodrug of EGCG (ProEGCG) with enhanced EGCG stability by replacing the -OH hydroxyl groups of EGCG into -COOCH3 acetyl acetoxy groups of ProEGCG (Lam et al., 2004) (Figure 3). ProEGCG showed greater bioavailability and stability than EGCG on breast cancer in vitro and in vivo (Landis-Piwowar et al., 2005; Landis-Piwowar et al., 2007). Its potency to inhibit tumor growth was to a greater extent than EGCG, with an enhanced inhibitory effect on proteasome and induction abilities of caspase3/7 and PARP proteins, thus to inducing apoptosis. In prostate cancer, ProEGCG limited tumor progression and angiogenesis, as well as induced apoptosis in vivo (Lee et al., 2008). Besides these, ProEGCG was shown to enhance the sensitization of leukemia to chemotherapy via caspase 3 activity and cleavage of PARP (Davenport et al., 2010). More recently, our team reported that ProEGCG acts as an angiogenesis inhibitor in endometrial cancer models in vitro and in vivo, and downregulated HIF-1α and VEGFA via PI3K/AKT/mTOR pathway (Wang J. et al., 2018). ProEGCG in low doses could inhibit cancer activity in endometrial cancer cells. Based on our finding, it was shown to upregulate the phosphorylation of JNK and p38 MAPK and downregulate that of AKT and ERK to ultimately inhibit tumor cell proliferation, micro-vessels formation and promote apoptotic activity in vitro and in vivo (Man et al., 2020). Importantly, this anticancer effect was significantlysuperior when compared with EGCG, with no toxicity being found.
On the other hand, a nanostructure-based drug delivery system improves the bioavailability of even low doses of EGCG. Chitosan nanoparticles (Dube et al., 2010; Li et al., 2014), liposomes (Fang et al., 2006; Dag and Oztop, 2017), nanoliposomes (De Pace et al., 2013) encapsulation material were used as the carriers of EGCG in different studies. Improved chemical structure stability via sustainable release of bioactive and the enhanced permeability of chemicals through the cellular membrane were resulted (Cai et al., 2018). In breast cancer, EGCG nanoparticles FA-NPS-PEG and FA-PEG-NPS were synthesized. It showed improved cellular uptake and anti-proliferation effect in vitro (Zeng et al., 2017). In cervical cancer, EGCG was delivered in a photothermal responsive zeolitic imidazolate framework using polydopamine-PEG nanoparticles (ZIF-PDA-PEG). Infrared laser irradiation assisted the releases of drugs, solving co-loading and multiple drug resistance problems, as well as showed higher efficiency in inducing apoptosis and autophagy (Chen et al., 2020).
Future studies can also focus on combining EGCG with other anticancer drugs to achieve a synergistic enhancement of the anti-cancer effects (Suganuma et al., 2011; Fujiki et al., 2015). In ovarian cancer, green tea with Paclitaxel or other nutrients as combination strategy synergistically inhibited cancer cells (Xinqiang et al., 2017; Panji et al., 2021a). Meanwhile, EGCG in combination with Cruciferous vegetables or Sulforaphane were shown to overcome Cisplatin or Paclitaxel chemotherapy resistance in ovarian cancer (Mazumder et al., 2012; Chen et al., 2013b; a). In cervical cancer, EGCG was treated synergistically with Enoacin or Eugenol-Amarogentin against cancer tumor growth (Pal et al., 2018; Mcdonnell et al., 2019). It was also treated to enhance Cisplatin effects in chemotherapy (Kilic et al., 2015). In breast cancer, EGCG demonstrated synergistic effects with Dexamethasone, U021, Tamoxifen, Quercetin or Paclitaxel (Guo et al., 2006; Sartippour et al., 2006; Li et al., 2009; Luo et al., 2010), thus enhanced cancer cell death. As discussed, tea polyphenol was proposed to be used with other chemotherapies to synergistically advance the therapeutic efficiency of autophagy modulating effect, such as with Docetaxel or Bortezomib in prostate cancer (Modernelli et al., 2015; Wang Q. et al., 2018), with Paclitaxel in ovarian cancer (Panji et al., 2021a), or with Doxorubicin in cervical cancer (Chen et al., 2020).
Conclusion
The role of autophagy has been shown to play a dual role in cancer. On one hand, autophagy may help to protect against cancer, while on the other hand, it may promote the growth of cancer. As autophagy plays an important role in cell death, these autophagy modulators may be a potential target to induce tumor cell death in a manner independent of necrosis or apoptosis. However, targeting these autophagy modulators as therapeutical strategy remained a huge obstacle. Thus, natural products can be used to target multiple pathways, which provide greater flexibility and potential for a variety of cancer types and stages. Green tea catechins and EGCG have been shown to play an important role in modulating autophagy in reproductive cancer cells. A number of in vitro and in vivo studies have confirmed the anti-inflammatory and antioxidant properties of polyphenolic compounds to induce autophagic death in reproductive cancer cells. However, it still required more validation using animal studies and clinical trials. Nevertheless, the current evidence strongly suggests the biologically active ingredients of green tea, namely EGCG, to be a valuable and promising agent in reproductive cancer chemoprevention.
Author Contributions
SH, YL, GC-WM, and CW wrote the first draft of the manuscript. XC, KC, YZ, YL, and XG contributed sections of the manuscript. All authors contributed to manuscript revision, read, and approved the submitted version.
Conflict of Interest
The authors declare that the research was conducted in the absence of any commercial or financial relationships that could be construed as a potential conflict of interest.
Publisher’s Note
All claims expressed in this article are solely those of the authors and do not necessarily represent those of their affiliated organizations, or those of the publisher, the editors, and the reviewers. Any product that may be evaluated in this article, or claim that may be made by its manufacturer, is not guaranteed or endorsed by the publisher.
Supplementary Material
The Supplementary Material for this article can be found online at: https://www.frontiersin.org/articles/10.3389/fphar.2022.906746/full#supplementary-material
Supplementary Figure S1 | Chemical structures of the four-major green tea catechins include (−)-epicatechin (EC), (−)-epigallocatechin (EGC), (−)-epicatechin-3-gallate (ECG), and (−)-epigallocatechin-3- gallate (EGCG).
References
Adachi, S., Nagao, T., Ingolfsson, H. I., Maxfield, F. R., Andersen, O. S., Kopelovich, L., et al. (2007). The Inhibitory Effect of (-)-epigallocatechin Gallate on Activation of the Epidermal Growth Factor Receptor Is Associated with Altered Lipid Order in HT29 Colon Cancer Cells. Cancer Res. 67, 6493–6501. doi:10.1158/0008-5472.CAN-07-0411
Adhami, V. M., Malik, A., Zaman, N., Sarfaraz, S., Siddiqui, I. A., Syed, D. N., et al. (2007). Combined Inhibitory Effects of Green Tea Polyphenols and Selective Cyclooxygenase-2 Inhibitors on the Growth of Human Prostate Cancer Cells Both In Vitro and In Vivo. Clin. Cancer Res. 13, 1611–1619. doi:10.1158/1078-0432.CCR-06-2269
Adhami, V. M., Siddiqui, I. A., Ahmad, N., Gupta, S., and Mukhtar, H. (2004). Oral Consumption of Green Tea Polyphenols Inhibits Insulin-like Growth Factor-I-Induced Signaling in an Autochthonous Mouse Model of Prostate Cancer. Cancer Res. 64, 8715–8722. doi:10.1158/0008-5472.CAN-04-2840
Adhami, V. M., Siddiqui, I. A., Sarfaraz, S., Khwaja, S. I., Hafeez, B. B., Ahmad, N., et al. (2009). Effective Prostate Cancer Chemopreventive Intervention with Green Tea Polyphenols in the TRAMP Model Depends on the Stage of the Disease. Clin. Cancer Res. 15, 1947–1953. doi:10.1158/1078-0432.CCR-08-2332
Ahmad, N., Gupta, S., and Mukhtar, H. (2000). Green Tea Polyphenol Epigallocatechin-3-Gallate Differentially Modulates Nuclear Factor kappaB in Cancer Cells versus Normal Cells. Arch. Biochem. Biophys. 376, 338–346. doi:10.1006/abbi.2000.1742
Ahn, W. S., Huh, S. W., Bae, S. M., Lee, I. P., Lee, J. M., Namkoong, S. E., et al. (2003). A Major Constituent of Green Tea, EGCG, Inhibits the Growth of a Human Cervical Cancer Cell Line, CaSki Cells, through Apoptosis, G(1) Arrest, and Regulation of Gene Expression. DNA Cell Biol. 22, 217–224. doi:10.1089/104454903321655846
Al-Bari, M. A. A., Ito, Y., Ahmed, S., Radwan, N., Ahmed, H. S., and Eid, N. (2021). Targeting Autophagy with Natural Products as a Potential Therapeutic Approach for Cancer. Int. J. Mol. Sci. 22, 9807. doi:10.3390/ijms22189807
Al-Obaidy, K. I., Chovanec, M., and Cheng, L. (2020). Molecular Characteristics of Testicular Germ Cell Tumors: Pathogenesis and Mechanisms of Therapy Resistance. Expert Rev. Anticancer Ther. 20, 75–79. doi:10.1080/14737140.2020.1717337
Amaral, C., Augusto, T. V., Tavares-Da-Silva, E., Roleira, F. M. F., Correia-Da-Silva, G., and Teixeira, N. (2018). Hormone-dependent Breast Cancer: Targeting Autophagy and PI3K Overcomes Exemestane-Acquired Resistance. J. Steroid Biochem. Mol. Biol. 183, 51–61. doi:10.1016/j.jsbmb.2018.05.006
Amaravadi, R. K., Kimmelman, A. C., and Debnath, J. (2019). Targeting Autophagy in Cancer: Recent Advances and Future Directions. Cancer Discov. 9, 1167–1181. doi:10.1158/2159-8290.CD-19-0292
Armstrong, C. M., and Gao, A. C. (2015). Drug Resistance in Castration Resistant Prostate Cancer: Resistance Mechanisms and Emerging Treatment Strategies. Am. J. Clin. Exp. Urol. 3, 64–76.
Ávalos, Y., Canales, J., Bravo-Sagua, R., Criollo, A., Lavandero, S., and Quest, A. F. (2014). Tumor Suppression and Promotion by Autophagy. Biomed. Res. Int. 2014, 603980. doi:10.1155/2014/603980
Bae, K. H., Tan, S., Yamashita, A., Ang, W. X., Gao, S. J., Wang, S., et al. (2017). Hyaluronic Acid-Green Tea Catechin Micellar Nanocomplexes: Fail-Safe Cisplatin Nanomedicine for the Treatment of Ovarian Cancer without Off-Target Toxicity. Biomaterials 148, 41–53. doi:10.1016/j.biomaterials.2017.09.027
Balasubramanian, R., Rolph, R., Morgan, C., and Hamed, H. (2019). Genetics of Breast Cancer: Management Strategies and Risk-Reducing Surgery. Br. J. Hosp. Med. (Lond) 80, 720–725. doi:10.12968/hmed.2019.80.12.720
Balazi, A., Sirotkin, A. V., Foldesiova, M., Makovicky, P., Chrastinova, L., Makovicky, P., et al. (2019). Green Tea Can Supress Rabbit Ovarian Functions In Vitro and In Vivo. Theriogenology 127, 72–79. doi:10.1016/j.theriogenology.2019.01.010
Bandera, E. V., Williams-King, M. G., Sima, C., Bayuga-Miller, S., Pulick, K., Wilcox, H., et al. (2010). Coffee and Tea Consumption and Endometrial Cancer Risk in a Population-Based Study in New Jersey. Cancer Causes Control 21, 1467–1473. doi:10.1007/s10552-010-9575-9
Belguise, K., Guo, S., and Sonenshein, G. E. (2007). Activation of FOXO3a by the Green Tea Polyphenol Epigallocatechin-3-Gallate Induces Estrogen Receptor Alpha Expression Reversing Invasive Phenotype of Breast Cancer Cells. Cancer Res. 67, 5763–5770. doi:10.1158/0008-5472.CAN-06-4327
Bettuzzi, S., Brausi, M., Rizzi, F., Castagnetti, G., Peracchia, G., and Corti, A. (2006). Chemoprevention of Human Prostate Cancer by Oral Administration of Green Tea Catechins in Volunteers with High-Grade Prostate Intraepithelial Neoplasia: A Preliminary Report from a One-Year Proof-Of-Principle Study. Cancer Res. 66, 1234–1240. doi:10.1158/0008-5472.CAN-05-1145
Beynon, R. A., Richmond, R. C., Santos Ferreira, D. L., Ness, A. R., May, M., Smith, G. D., et al. (2019). Investigating the Effects of Lycopene and Green Tea on the Metabolome of Men at Risk of Prostate Cancer: The ProDiet Randomised Controlled Trial. Int. J. Cancer 144, 1918–1928. doi:10.1002/ijc.31929
Braicu, C., Gherman, C. D., Irimie, A., and Berindan-Neagoe, I. (2013). Epigallocatechin-3-gallate (EGCG) Inhibits Cell Proliferation and Migratory Behaviour of Triple Negative Breast Cancer Cells. J. Nanosci. Nanotechnol. 13, 632–637. doi:10.1166/jnn.2013.6882
Brausi, M., Rizzi, F., and Bettuzzi, S. (2008). Chemoprevention of Human Prostate Cancer by Green Tea Catechins: Two Years Later. A Follow-Up Update. Eur. Urol. 54, 472–473. doi:10.1016/j.eururo.2008.03.100
Butler, D. E., Marlein, C., Walker, H. F., Frame, F. M., Mann, V. M., Simms, M. S., et al. (2017). Inhibition of the PI3K/AKT/mTOR Pathway Activates Autophagy and Compensatory Ras/Raf/MEK/ERK Signalling in Prostate Cancer. Oncotarget 8, 56698–56713. doi:10.18632/oncotarget.18082
Buzun, K., Gornowicz, A., Lesyk, R., Bielawski, K., and Bielawska, A. (2021). Autophagy Modulators in Cancer Therapy. Int. J. Mol. Sci. 22, 5804. doi:10.3390/ijms22115804
Cabrera, C., Artacho, R., and Giménez, R. (2006). Beneficial Effects of Green Tea-Aa Review. J. Am. Coll. Nutr. 25, 79–99. doi:10.1080/07315724.2006.10719518
Cai, Z. Y., Li, X. M., Liang, J. P., Xiang, L. P., Wang, K. R., Shi, Y. L., et al. (2018). Bioavailability of Tea Catechins and its Improvement. Molecules 23, 2346. doi:10.3390/molecules23092346
Chacko, S. M., Thambi, P. T., Kuttan, R., and Nishigaki, I. (2010). Beneficial Effects of Green Tea: a Literature Review. Chin. Med. 5, 13. doi:10.1186/1749-8546-5-13
Chan, M. M., Soprano, K. J., Weinstein, K., and Fong, D. (2006). Epigallocatechin-3-gallate Delivers Hydrogen Peroxide to Induce Death of Ovarian Cancer Cells and Enhances Their Cisplatin Susceptibility. J. Cell Physiol. 207, 389–396. doi:10.1002/jcp.20569
Chen, H., Landen, C. N., Li, Y., Alvarez, R. D., and Tollefsbol, T. O. (2013b). Epigallocatechin Gallate and Sulforaphane Combination Treatment Induce Apoptosis in Paclitaxel-Resistant Ovarian Cancer Cells through hTERT and Bcl-2 Down-Regulation. Exp. Cell Res. 319, 697–706. doi:10.1016/j.yexcr.2012.12.026
Chen, H., Landen, C. N., Li, Y., Alvarez, R. D., and Tollefsbol, T. O. (2013a). Enhancement of Cisplatin-Mediated Apoptosis in Ovarian Cancer Cells through Potentiating G2/M Arrest and P21 Upregulation by Combinatorial Epigallocatechin Gallate and Sulforaphane. J. Oncol. 2013, 872957. doi:10.1155/2013/872957
Chen, X., Tong, R., Liu, B., Liu, H., Feng, X., Ding, S., et al. (2020). Duo of (-)-Epigallocatechin-3-Gallate and Doxorubicin Loaded by Polydopamine Coating ZIF-8 in the Regulation of Autophagy for Chemo-Photothermal Synergistic Therapy. Biomater. Sci. 8, 1380–1393. doi:10.1039/c9bm01614g
Chen, Y., Chen, J., Sun, X., Shi, X., Wang, L., Huang, L., et al. (2018). Evaluation of the Neuroprotective Effect of EGCG: a Potential Mechanism of Mitochondrial Dysfunction and Mitochondrial Dynamics after Subarachnoid Hemorrhage. Food Funct. 9, 6349–6359. doi:10.1039/c8fo01497c
Cheng, L., Lyu, B., and Roth, L. M. (2017). Perspectives on Testicular Germ Cell Neoplasms. Hum. Pathol. 59, 10–25. doi:10.1016/j.humpath.2016.08.002
Choan, E., Segal, R., Jonker, D., Malone, S., Reaume, N., Eapen, L., et al. (2005). A Prospective Clinical Trial of Green Tea for Hormone Refractory Prostate Cancer: An Evaluation of the Complementary/alternative Therapy Approach. Urol. Oncol. 23, 108–113. doi:10.1016/j.urolonc.2004.10.008
Chu, C., Deng, J., Man, Y., and Qu, Y. (2017). Green Tea Extracts Epigallocatechin-3-Gallate for Different Treatments. Biomed. Res. Int. 2017, 5615647. doi:10.1155/2017/5615647
Chuu, C. P., Chen, R. Y., Kokontis, J. M., Hiipakka, R. A., and Liao, S. (2009). Suppression of Androgen Receptor Signaling and Prostate Specific Antigen Expression by (-)-Epigallocatechin-3-Gallate in Different Progression Stages of LNCaP Prostate Cancer Cells. Cancer Lett. 275, 86–92. doi:10.1016/j.canlet.2008.10.001
Crew, K. D., Brown, P., Greenlee, H., Bevers, T. B., Arun, B., Hudis, C., et al. (2012). Phase IB Randomized, Double-Blinded, Placebo-Controlled, Dose Escalation Study of Polyphenon E in Women with Hormone Receptor-Negative Breast Cancer. Cancer Prev. Res. (Phila) 5, 1144–1154. doi:10.1158/1940-6207.CAPR-12-0117
Crew, K. D., Ho, K. A., Brown, P., Greenlee, H., Bevers, T. B., Arun, B., et al. (2015). Effects of a Green Tea Extract, Polyphenon E, on Systemic Biomarkers of Growth Factor Signalling in Women with Hormone Receptor-Negative Breast Cancer. J. Hum. Nutr. Diet. 28, 272–282. doi:10.1111/jhn.12229
Cuomo, F., Altucci, L., and Cobellis, G. (2019). Autophagy Function and Dysfunction: Potential Drugs as Anti-cancer Therapy. Cancers (Basel) 11, 1465. doi:10.3390/cancers11101465
Dag, D., and Oztop, M. H. (2017). Formation and Characterization of Green Tea Extract Loaded Liposomes. J. Food Sci. 82, 463–470. doi:10.1111/1750-3841.13615
Dai, Q., Shu, X. O., Li, H., Yang, G., Shrubsole, M. J., Cai, H., et al. (2010). Is Green Tea Drinking Associated with a Later Onset of Breast Cancer? Ann. Epidemiol. 20, 74–81. doi:10.1016/j.annepidem.2009.09.005
Dankó, T., Petővári, G., Sztankovics, D., Moldvai, D., Raffay, R., Lőrincz, P., et al. (2021). Rapamycin Plus Doxycycline Combination Affects Growth Arrest and Selective Autophagy-dependent Cell Death in Breast Cancer Cells. Int. J. Mol. Sci. 22, 8019. doi:10.3390/ijms22158019
Davenport, A., Frezza, M., Shen, M., Ge, Y., Huo, C., Chan, T. H., et al. (2010). Celastrol and an EGCG Pro-drug Exhibit Potent Chemosensitizing Activity in Human Leukemia Cells. Int. J. Mol. Med. 25, 465–470. doi:10.3892/ijmm_00000366
De Pace, R. C., Liu, X., Sun, M., Nie, S., Zhang, J., Cai, Q., et al. (2013). Anticancer Activities of (-)-Epigallocatechin-3-Gallate Encapsulated Nanoliposomes in MCF7 Breast Cancer Cells. J. Liposome Res. 23, 187–196. doi:10.3109/08982104.2013.788023
Deb, G., Shankar, E., Thakur, V. S., Ponsky, L. E., Bodner, D. R., Fu, P., et al. (2019). Green Tea-Induced Epigenetic Reactivation of Tissue Inhibitor of Matrix Metalloproteinase-3 Suppresses Prostate Cancer Progression through Histone-Modifying Enzymes. Mol. Carcinog. 58, 1194–1207. doi:10.1002/mc.23003
Degenhardt, K., and White, E. (2006). A Mouse Model System to Genetically Dissect the Molecular Mechanisms Regulating Tumorigenesis. Clin. Cancer Res. 12, 5298–5304. doi:10.1158/1078-0432.CCR-06-0439
Den Boon, J. A., Pyeon, D., Wang, S. S., Horswill, M., Schiffman, M., Sherman, M., et al. (2015). Molecular Transitions from Papillomavirus Infection to Cervical Precancer and Cancer: Role of Stromal Estrogen Receptor Signaling. Proc. Natl. Acad. Sci. U. S. A. 112, E3255–E3264. doi:10.1073/pnas.1509322112
Denton, D., Xu, T., and Kumar, S. (2015). Autophagy as a Pro-death Pathway. Immunol. Cell Biol. 93, 35–42. doi:10.1038/icb.2014.85
Djavaheri-Mergny, M., Amelotti, M., Mathieu, J., Besançon, F., Bauvy, C., Souquère, S., et al. (2006). NF-kappaB Activation Represses Tumor Necrosis Factor-Alpha-Induced Autophagy. J. Biol. Chem. 281, 30373–30382. doi:10.1074/jbc.M602097200
Dökümcü, K., Simonian, M., and Farahani, R. M. (2018). miR4673 Improves Fitness Profile of Neoplastic Cells by Induction of Autophagy. Cell Death Dis. 9, 1068. doi:10.1038/s41419-018-1088-6
Du, G. J., Zhang, Z., Wen, X. D., Yu, C., Calway, T., Yuan, C. S., et al. (2012). Epigallocatechin Gallate (EGCG) Is the Most Effective Cancer Chemopreventive Polyphenol in Green Tea. Nutrients 4, 1679–1691. doi:10.3390/nu4111679
Dube, A., Nicolazzo, J. A., and Larson, I. (2010). Chitosan Nanoparticles Enhance the Intestinal Absorption of the Green Tea Catechins (+)-catechin and (-)-epigallocatechin Gallate. Eur. J. Pharm. Sci. 41, 219–225. doi:10.1016/j.ejps.2010.06.010
Erdrich, S., Bishop, K. S., Karunasinghe, N., Han, D. Y., and Ferguson, L. R. (2015). A Pilot Study to Investigate if New Zealand Men with Prostate Cancer Benefit from a Mediterranean-Style Diet. PeerJ 3, e1080. doi:10.7717/peerj.1080
Eritja, N., Chen, B. J., Rodríguez-Barrueco, R., Santacana, M., Gatius, S., Vidal, A., et al. (2017). Autophagy Orchestrates Adaptive Responses to Targeted Therapy in Endometrial Cancer. Autophagy 13, 608–624. doi:10.1080/15548627.2016.1271512
Esmaeili, M. A. (2016). Combination of siRNA-Directed Gene Silencing with Epigallocatechin-3-Gallate (EGCG) Reverses Drug Resistance in Human Breast Cancer Cells. J. Chem. Biol. 9, 41–52. doi:10.1007/s12154-015-0144-2
Evans, J., Ziebland, S., and Mcpherson, A. (2007). Minimizing Delays in Ovarian Cancer Diagnosis: an Expansion of Andersen's Model of 'total Patient Delay'. Fam. Pract. 24, 48–55. doi:10.1093/fampra/cml063
Evans, S., Dizeyi, N., Abrahamsson, P. A., and Persson, J. (2009). The Effect of a Novel Botanical Agent TBS-101 on Invasive Prostate Cancer in Animal Models. Anticancer Res. 29, 3917–3924.
Fan, X., Zhou, J., Bi, X., Liang, J., Lu, S., Yan, X., et al. (2021). L-theanine Suppresses the Metastasis of Prostate Cancer by Downregulating MMP9 and Snail. J. Nutr. Biochem. 89, 108556. doi:10.1016/j.jnutbio.2020.108556
Fang, J. Y., Lee, W. R., Shen, S. C., and Huang, Y. L. (2006). Effect of Liposome Encapsulation of Tea Catechins on Their Accumulation in Basal Cell Carcinomas. J. Dermatol Sci. 42, 101–109. doi:10.1016/j.jdermsci.2005.12.010
Farrow, J. M., Yang, J. C., and Evans, C. P. (2014). Autophagy as a Modulator and Target in Prostate Cancer. Nat. Rev. Urol. 11, 508–516. doi:10.1038/nrurol.2014.196
Feng, Y., He, D., Yao, Z., and Klionsky, D. J. (2014). The Machinery of Macroautophagy. Cell Res. 24, 24–41. doi:10.1038/cr.2013.168
Fujiki, H., Sueoka, E., Watanabe, T., and Suganuma, M. (2015). Synergistic Enhancement of Anticancer Effects on Numerous Human Cancer Cell Lines Treated with the Combination of EGCG, Other Green Tea Catechins, and Anticancer Compounds. J. Cancer Res. Clin. Oncol. 141, 1511–1522. doi:10.1007/s00432-014-1899-5
Gan, R. Y., Li, H. B., Sui, Z. Q., and Corke, H. (2018). Absorption, Metabolism, Anti-cancer Effect and Molecular Targets of Epigallocatechin Gallate (EGCG): An Updated Review. Crit. Rev. Food Sci. Nutr. 58, 924–941. doi:10.1080/10408398.2016.1231168
García-Aranda, M., and Redondo, M. (2019). Immunotherapy: A Challenge of Breast Cancer Treatment. Cancers (Basel) 11, 1822. doi:10.3390/cancers11121822
Ge, J., Han, B., Hu, H., Liu, J., and Liu, Y. (2015). Epigallocatechin-3-O-Gallate Protects against Hepatic Damage and Testicular Toxicity in Male Mice Exposed to Di-(2-ethylhexyl) Phthalate. J. Med. Food 18, 753–761. doi:10.1089/jmf.2014.3247
Gewirtz, D. A. (2016). The Challenge of Developing Autophagy Inhibition as a Therapeutic Strategy. Cancer Res. 76, 5610–5614. doi:10.1158/0008-5472.CAN-16-0722
Graham, H. N. (1992). Green Tea Composition, Consumption, and Polyphenol Chemistry. Prev. Med. 21, 334–350. doi:10.1016/0091-7435(92)90041-f
Guo, S., Lu, J., Subramanian, A., and Sonenshein, G. E. (2006). Microarray-assisted Pathway Analysis Identifies Mitogen-Activated Protein Kinase Signaling as a Mediator of Resistance to the Green Tea Polyphenol Epigallocatechin 3-gallate in Her-2/neu-Overexpressing Breast Cancer Cells. Cancer Res. 66, 5322–5329. doi:10.1158/0008-5472.CAN-05-4287
Gupta, S., Hastak, K., Afaq, F., Ahmad, N., and Mukhtar, H. (2004). Essential Role of Caspases in Epigallocatechin-3-Gallate-Mediated Inhibition of Nuclear Factor Kappa B and Induction of Apoptosis. Oncogene 23, 2507–2522. doi:10.1038/sj.onc.1207353
Gupta, S. M., and Mania-Pramanik, J. (2019). Molecular Mechanisms in Progression of HPV-Associated Cervical Carcinogenesis. J. Biomed. Sci. 26, 28. doi:10.1186/s12929-019-0520-2
Han, Z., Liu, D., Chen, L., He, Y., Tian, X., Qi, L., et al. (2021). PNO1 Regulates Autophagy and Apoptosis of Hepatocellular Carcinoma via the MAPK Signaling Pathway. Cell Death Dis. 12, 552. doi:10.1038/s41419-021-03837-y
Hanna, T. P., King, W. D., Thibodeau, S., Jalink, M., Paulin, G. A., Harvey-Jones, E., et al. (2020). Mortality Due to Cancer Treatment Delay: Systematic Review and Meta-Analysis. BMJ 371, m4087. doi:10.1136/bmj.m4087
Hanyu, X., Lanyue, L., Miao, D., Wentao, F., Cangran, C., and Hui, S. (2020). Effect of Ganoderma Applanatum Polysaccharides on MAPK/ERK Pathway Affecting Autophagy in Breast Cancer MCF-7 Cells. Int. J. Biol. Macromol. 146, 353–362. doi:10.1016/j.ijbiomac.2020.01.010
Harper, C. E., Patel, B. B., Wang, J., Eltoum, I. A., and Lamartiniere, C. A. (2007). Epigallocatechin-3-Gallate Suppresses Early Stage, but Not Late Stage Prostate Cancer in TRAMP Mice: Mechanisms of Action. Prostate 67, 1576–1589. doi:10.1002/pros.20643
Hastak, K., Gupta, S., Ahmad, N., Agarwal, M. K., Agarwal, M. L., and Mukhtar, H. (2003). Role of P53 and NF-kappaB in Epigallocatechin-3-Gallate-Induced Apoptosis of LNCaP Cells. Oncogene 22, 4851–4859. doi:10.1038/sj.onc.1206708
Hawsawi, Y. M., Zailaie, S. A., Oyouni, A. A. A., Alzahrani, O. R., Alamer, O. M., and Aljohani, S. A. S. (2020). Prostate Cancer and Therapeutic Challenges. J. Biol. Res. Thessal. 27, 20. doi:10.1186/s40709-020-00128-z
Hayes-Lattin, B., and Nichols, C. R. (2009). Testicular Cancer: a Prototypic Tumor of Young Adults. Semin. Oncol. 36, 432–438. doi:10.1053/j.seminoncol.2009.07.006
Hecht, J. L., and Mutter, G. L. (2006). Molecular and Pathologic Aspects of Endometrial Carcinogenesis. J. Clin. Oncol. 24, 4783–4791. doi:10.1200/JCO.2006.06.7173
Herman-Antosiewicz, A., Johnson, D. E., and Singh, S. V. (2006). Sulforaphane Causes Autophagy to Inhibit Release of Cytochrome C and Apoptosis in Human Prostate Cancer Cells. Cancer Res. 66, 5828–5835. doi:10.1158/0008-5472.CAN-06-0139
Hickey, M., Peate, M., Saunders, C. M., and Friedlander, M. (2009). Breast Cancer in Young Women and its Impact on Reproductive Function. Hum. Reprod. Update 15, 323–339. doi:10.1093/humupd/dmn064
Ho, Y. C., Yang, S. F., Peng, C. Y., Chou, M. Y., and Chang, Y. C. (2007). Epigallocatechin-3-gallate Inhibits the Invasion of Human Oral Cancer Cells and Decreases the Productions of Matrix Metalloproteinases and Urokinase-Plasminogen Activator. J. Oral Pathol. Med. 36, 588–593. doi:10.1111/j.1600-0714.2007.00588.x
Horowitz, M., Esakov, E., Rose, P., and Reizes, O. (2020). Signaling within the Epithelial Ovarian Cancer Tumor Microenvironment: the Challenge of Tumor Heterogeneity. Ann. Transl. Med. 8, 905. doi:10.21037/atm-2019-cm-08
Hou, Z., Sang, S., You, H., Lee, M. J., Hong, J., Chin, K. V., et al. (2005). Mechanism of Action of (-)-Epigallocatechin-3-Gallate: Auto-oxidation-dependent Inactivation of Epidermal Growth Factor Receptor and Direct Effects on Growth Inhibition in Human Esophageal Cancer KYSE 150 Cells. Cancer Res. 65, 8049–8056. doi:10.1158/0008-5472.CAN-05-0480
Hsu, A., Bruno, R. S., Löhr, C. V., Taylor, A. W., Dashwood, R. H., Bray, T. M., et al. (2011). Dietary Soy and Tea Mitigate Chronic Inflammation and Prostate Cancer via NFκB Pathway in the Noble Rat Model. J. Nutr. Biochem. 22, 502–510. doi:10.1016/j.jnutbio.2010.04.006
Innajak, S., Mahabusrakum, W., and Watanapokasin, R. (2016). Goniothalamin Induces Apoptosis Associated with Autophagy Activation through MAPK Signaling in SK-BR-3 Cells. Oncol. Rep. 35, 2851–2858. doi:10.3892/or.2016.4655
Iwasaki, M., Inoue, M., Sasazuki, S., Miura, T., Sawada, N., Yamaji, T., et al. (2010). Plasma Tea Polyphenol Levels and Subsequent Risk of Breast Cancer Among Japanese Women: A Nested Case-Control Study. Breast Cancer Res. Treat. 124, 827–834. doi:10.1007/s10549-010-0916-x
Iwasaki, M., Mizusawa, J., Kasuga, Y., Yokoyama, S., Onuma, H., Nishimura, H., et al. (2014). Green Tea Consumption and Breast Cancer Risk in Japanese Women: A Case-Control Study. Nutr. Cancer 66, 57–67. doi:10.1080/01635581.2014.847963
Jang, J. Y., Lee, J. K., Jeon, Y. K., and Kim, C. W. (2013). Exosome Derived from Epigallocatechin Gallate Treated Breast Cancer Cells Suppresses Tumor Growth by Inhibiting Tumor-Associated Macrophage Infiltration and M2 Polarization. BMC Cancer 13, 421. doi:10.1186/1471-2407-13-421
Janku, F., Mcconkey, D. J., Hong, D. S., and Kurzrock, R. (2011). Autophagy as a Target for Anticancer Therapy. Nat. Rev. Clin. Oncol. 8, 528–539. doi:10.1038/nrclinonc.2011.71
Jia, Y., Hu, T., Hang, C. Y., Yang, R., Li, X., Chen, Z. L., et al. (2012). Case-control Study of Diet in Patients with Cervical Cancer or Precancerosis in Wufeng, a High Incidence Region in China. Asian Pac J. Cancer Prev. 13, 5299–5302. doi:10.7314/apjcp.2012.13.10.5299
Katiyar, S. K., Afaq, F., Azizuddin, K., and Mukhtar, H. (2001). Inhibition of UVB-Induced Oxidative Stress-Mediated Phosphorylation of Mitogen-Activated Protein Kinase Signaling Pathways in Cultured Human Epidermal Keratinocytes by Green Tea Polyphenol (-)-Epigallocatechin-3-Gallate. Toxicol. Appl. Pharmacol. 176, 110–117. doi:10.1006/taap.2001.9276
Kaur, J., and Debnath, J. (2015). Autophagy at the Crossroads of Catabolism and Anabolism. Nat. Rev. Mol. Cell Biol. 16, 461–472. doi:10.1038/nrm4024
Kaur, S., Greaves, P., Cooke, D. N., Edwards, R., Steward, W. P., Gescher, A. J., et al. (2007). Breast Cancer Prevention by Green Tea Catechins and Black Tea Theaflavins in the C3(1) SV40 T,t Antigen Transgenic Mouse Model Is Accompanied by Increased Apoptosis and a Decrease in Oxidative DNA Adducts. J. Agric. Food Chem. 55, 3378–3385. doi:10.1021/jf0633342
Kavanagh, K. T., Hafer, L. J., Kim, D. W., Mann, K. K., Sherr, D. H., Rogers, A. E., et al. (2001). Green Tea Extracts Decrease Carcinogen-Induced Mammary Tumor Burden in Rats and Rate of Breast Cancer Cell Proliferation in Culture. J. Cell Biochem. 82, 387–398. doi:10.1002/jcb.1164
Kazi, A., Wang, Z., Kumar, N., Falsetti, S. C., Chan, T. H., and Dou, Q. P. (2004). Structure-activity Relationships of Synthetic Analogs of (-)-Epigallocatechin-3-Gallate as Proteasome Inhibitors. Anticancer Res. 24, 943–954.
Khan, M. A., Hussain, A., Sundaram, M. K., Alalami, U., Gunasekera, D., Ramesh, L., et al. (2015). (-)-Epigallocatechin-3-gallate Reverses the Expression of Various Tumor-Suppressor Genes by Inhibiting DNA Methyltransferases and Histone Deacetylases in Human Cervical Cancer Cells. Oncol. Rep. 33, 1976–1984. doi:10.3892/or.2015.3802
Khan, N., Bharali, D. J., Adhami, V. M., Siddiqui, I. A., Cui, H., Shabana, S. M., et al. (2014). Oral Administration of Naturally Occurring Chitosan-Based Nanoformulated Green Tea Polyphenol EGCG Effectively Inhibits Prostate Cancer Cell Growth in a Xenograft Model. Carcinogenesis 35, 415–423. doi:10.1093/carcin/bgt321
Khan, N., and Mukhtar, H. (2008). Multitargeted Therapy of Cancer by Green Tea Polyphenols. Cancer Lett. 269, 269–280. doi:10.1016/j.canlet.2008.04.014
Khojaste, E., and Ahmadizadeh, C. (2021). Catechin Metabolites along with Curcumin Inhibit Proliferation and Induce Apoptosis in Cervical Cancer Cells by Regulating VEGF Expression In-Vitro. Nutr. Cancer 74 (3), 1048–1057. doi:10.1080/01635581.2021.1936082
Kilic, U., Sahin, K., Tuzcu, M., Basak, N., Orhan, C., Elibol-Can, B., et al. (2015). Enhancement of Cisplatin Sensitivity in Human Cervical Cancer: Epigallocatechin-3-Gallate. Front. Nutr. 1, 28. doi:10.3389/fnut.2014.00028
Kim, C., Mcglynn, K. A., Mccorkle, R., Zheng, T., Erickson, R. L., Niebuhr, D. W., et al. (2010). Fertility Among Testicular Cancer Survivors: a Case-Control Study in the U.S. J. Cancer Surviv 4, 266–273. doi:10.1007/s11764-010-0134-x
Kim, H. S., Kim, M. H., Jeong, M., Hwang, Y. S., Lim, S. H., Shin, B. A., et al. (2004). EGCG Blocks Tumor Promoter-Induced MMP-9 Expression via Suppression of MAPK and AP-1 Activation in Human Gastric AGS Cells. Anticancer Res. 24, 747–753.
Kim, S. J., Amankwah, E., Connors, S., Park, H. Y., Rincon, M., Cornnell, H., et al. (2014). Safety and Chemopreventive Effect of Polyphenon E in Preventing Early and Metastatic Progression of Prostate Cancer in TRAMP Mice. Cancer Prev. Res. (Phila) 7, 435–444. doi:10.1158/1940-6207.CAPR-13-0427-T
Kitamura, M., Nishino, T., Obata, Y., Furusu, A., Hishikawa, Y., Koji, T., et al. (2012). Epigallocatechin Gallate Suppresses Peritoneal Fibrosis in Mice. Chem. Biol. Interact. 195, 95–104. doi:10.1016/j.cbi.2011.11.002
Klionsky, D. J., Abdelmohsen, K., Abe, A., Abedin, M. J., Abeliovich, H., Acevedo Arozena, A., et al. (2021). Guidelines for the Use and Interpretation of Assays for Monitoring Autophagy. Autophagy 17, 1–382. doi:10.1080/15548627.2015.1100356
Kochetkova, E. Y., Blinova, G. I., Bystrova, O. A., Martynova, M. G., Pospelov, V. A., and Pospelova, T. V. (2017). Targeted Elimination of Senescent Ras-Transformed Cells by Suppression of MEK/ERK Pathway. Aging (Albany NY) 9, 2352–2375. doi:10.18632/aging.101325
Kochman, J., Jakubczyk, K., Antoniewicz, J., Mruk, H., and Janda, K. (2020). Health Benefits and Chemical Composition of Matcha Green Tea: A Review. Molecules 26, 85. doi:10.3390/molecules26010085
Kumar, N. B., Pow-Sang, J., Spiess, P. E., Park, J., Salup, R., Williams, C. R., et al. (2016). Randomized, Placebo-Controlled Trial Evaluating the Safety of One-Year Administration of Green Tea Catechins. Oncotarget 7, 70794–70802. doi:10.18632/oncotarget.12222
Kurahashi, N., Sasazuki, S., Iwasaki, M., and Inoue, M. (2008). Green Tea Consumption and Prostate Cancer Risk in Japanese Men: A Prospective Study. Am. J. Epidemiol. 167, 71–77. doi:10.1093/aje/kwm249
Lam, W. H., Kazi, A., Kuhn, D. J., Chow, L. M., Chan, A. S., Dou, Q. P., et al. (2004). A Potential Prodrug for a Green Tea Polyphenol Proteasome Inhibitor: Evaluation of the Peracetate Ester of (-)-epigallocatechin Gallate [(-)-EGCG]. Bioorg Med. Chem. 12, 5587–5593. doi:10.1016/j.bmc.2004.08.002
Landis-Piwowar, K. R., Huo, C., Chen, D., Milacic, V., Shi, G., Chan, T. H., et al. (2007). A Novel Prodrug of the Green Tea Polyphenol (-)-Epigallocatechin-3-Gallate as a Potential Anticancer Agent. Cancer Res. 67, 4303–4310. doi:10.1158/0008-5472.CAN-06-4699
Landis-Piwowar, K. R., Kuhn, D. J., Wan, S. B., Chen, D., Chan, T. H., and Dou, Q. P. (2005). Evaluation of Proteasome-Inhibitory and Apoptosis-Inducing Potencies of Novel (-)-EGCG Analogs and Their Prodrugs. Int. J. Mol. Med. 15, 735–742. doi:10.3892/ijmm.15.4.735
Lassed, S., Deus, C. M., Djebbari, R., Zama, D., Oliveira, P. J., Rizvanov, A. A., et al. (2017). Protective Effect of Green Tea (Camellia Sinensis (L.) Kuntze) against Prostate Cancer: From In Vitro Data to Algerian Patients. Evid. Based Complement. Altern. Med. 2017, 1691568. doi:10.1155/2017/1691568
Lassed, S., Deus, C. M., Lourenco, N., Dahdouh, A., Rizvanov, A. A., Oliveira, P. J., et al. (2016). Diet, Lifestyles, Family History, and Prostate Cancer Incidence in an East Algerian Patient Group. Biomed. Res. Int. 2016, 5730569. doi:10.1155/2016/5730569
Lee, A. H., Su, D., Pasalich, M., and Binns, C. W. (2013). Tea Consumption Reduces Ovarian Cancer Risk. Cancer Epidemiol. 37, 54–59. doi:10.1016/j.canep.2012.10.003
Lee, J. J., Jain, V., and Amaravadi, R. K. (2021). Clinical Translation of Combined MAPK and Autophagy Inhibition in RAS Mutant Cancer. Int. J. Mol. Sci. 22, 12402. doi:10.3390/ijms222212402
Lee, M. S., Cherla, R. P., Jenson, M. H., Leyva-Illades, D., Martinez-Moczygemba, M., and Tesh, V. L. (2011). Shiga Toxins Induce Autophagy Leading to Differential Signalling Pathways in Toxin-Sensitive and Toxin-Resistant Human Cells. Cell Microbiol. 13, 1479–1496. doi:10.1111/j.1462-5822.2011.01634.x
Lee, P. M. Y., Ng, C. F., Liu, Z. M., Ho, W. M., Lee, M. K., Wang, F., et al. (2017). Reduced Prostate Cancer Risk with Green Tea and Epigallocatechin 3-gallate Intake Among Hong Kong Chinese Men. Prostate Cancer Prostatic Dis. 20, 318–322. doi:10.1038/pcan.2017.18
Lee, S. C., Chan, W. K., Lee, T. W., Lam, W. H., Wang, X., Chan, T. H., et al. (2008). Effect of a Prodrug of the Green Tea Polyphenol (-)-epigallocatechin-3- Gallate on the Growth of Androgen-independent Prostate Cancer In Vivo. Nutr. Cancer 60, 483–491. doi:10.1080/01635580801947674
Levine, B., and Kroemer, G. (2008). Autophagy in the Pathogenesis of Disease. Cell 132, 27–42. doi:10.1016/j.cell.2007.12.018
Levine, B., and Yuan, J. (2005). Autophagy in Cell Death: an Innocent Convict? J. Clin. Invest 115, 2679–2688. doi:10.1172/JCI26390
Levy, J. M. M., Towers, C. G., and Thorburn, A. (2017). Targeting Autophagy in Cancer. Nat. Rev. Cancer 17, 528–542. doi:10.1038/nrc.2017.53
Li, L. Y., Guan, Y. D., Chen, X. S., Yang, J. M., and Cheng, Y. (2020). DNA Repair Pathways in Cancer Therapy and Resistance. Front. Pharmacol. 11, 629266. doi:10.3389/fphar.2020.629266
Li, M., Tse, L. A., Chan, W. C., Kwok, C. H., Leung, S. L., Wu, C., et al. (2016a). Evaluation of Breast Cancer Risk Associated with Tea Consumption by Menopausal and Estrogen Receptor Status Among Chinese Women in Hong Kong. Cancer Epidemiol. 40, 73–78. doi:10.1016/j.canep.2015.11.013
Li, M., Wang, J., Jing, J., Hua, H., Luo, T., Xu, L., et al. (2009). Synergistic Promotion of Breast Cancer Cells Death by Targeting Molecular Chaperone GRP78 and Heat Shock Protein 70. J. Cell. Mol. Med. 13, 4540–4550. doi:10.1111/j.1582-4934.2008.00575.x
Li, M., Zhao, X., Yong, H., Shang, B., Lou, W., Wang, Y., et al. (2021). FBXO22 Promotes Growth and Metastasis and Inhibits Autophagy in Epithelial Ovarian Cancers via the MAPK/ERK Pathway. Front. Pharmacol. 12, 778698. doi:10.3389/fphar.2021.778698
Li, W., He, N., Tian, L., Shi, X., and Yang, X. (2016b). Inhibitory Effects of Polyphenol-Enriched Extract from Ziyang Tea against Human Breast Cancer MCF-7 Cells through Reactive Oxygen Species-dependent Mitochondria Molecular Mechanism. J. Food Drug Analysis 24, 527–538. doi:10.1016/j.jfda.2016.01.005
Li, W., Yang, J., Luo, L., Jiang, M., Qin, B., Yin, H., et al. (2019). Targeting Photodynamic and Photothermal Therapy to the Endoplasmic Reticulum Enhances Immunogenic Cancer Cell Death. Nat. Commun. 10, 3349. doi:10.1038/s41467-019-11269-8
Li, W., Zhu, S., Li, J., Assa, A., Jundoria, A., Xu, J., et al. (2011). EGCG Stimulates Autophagy and Reduces Cytoplasmic HMGB1 Levels in Endotoxin-Stimulated Macrophages. Biochem. Pharmacol. 81, 1152–1163. doi:10.1016/j.bcp.2011.02.015
Li, Y., Buckhaults, P., Cui, X., and Tollefsbol, T. O. (2016c). Combinatorial Epigenetic Mechanisms and Efficacy of Early Breast Cancer Inhibition by Nutritive Botanicals. Epigenomics 8, 1019–1037. doi:10.2217/epi-2016-0024
Li, Y., Meeran, S. M., and Tollefsbol, T. O. (2017). Combinatorial Bioactive Botanicals Re-sensitize Tamoxifen Treatment in ER-Negative Breast Cancer via Epigenetic Reactivation of ERα Expression. Sci. Rep. 7, 1. doi:10.1038/s41598-017-09764-3
Li, Y., Yuan, Y. Y., Meeran, S. M., and Tollefsbol, T. O. (2010). Synergistic Epigenetic Reactivation of Estrogen Receptor-α (ERα) by Combined Green Tea Polyphenol and Histone Deacetylase Inhibitor in ERα-Negative Breast Cancer Cells. Mol. Cancer 9, 1. doi:10.1186/1476-4598-9-274
Li, Z., Ha, J., Zou, T., and Gu, L. (2014). Fabrication of Coated Bovine Serum Albumin (BSA)-epigallocatechin Gallate (EGCG) Nanoparticles and Their Transport across Monolayers of Human Intestinal Epithelial Caco-2 Cells. Food Funct. 5, 1278–1285. doi:10.1039/c3fo60500k
Liang, X. H., Jackson, S., Seaman, M., Brown, K., Kempkes, B., Hibshoosh, H., et al. (1999). Induction of Autophagy and Inhibition of Tumorigenesis by Beclin 1. Nature 402, 672–676. doi:10.1038/45257
Lim, J., and Murthy, A. (2020). Targeting Autophagy to Treat Cancer: Challenges and Opportunities. Front. Pharmacol. 11, 590344. doi:10.3389/fphar.2020.590344
Lim, V., Zhu, H., Diao, S., Hu, L., and Hu, J. (2019). PKP3 Interactions with MAPK-JNK-Erk1/2-mTOR Pathway Regulates Autophagy and Invasion in Ovarian Cancer. Biochem. Biophys. Res. Commun. 508, 646–653. doi:10.1016/j.bbrc.2018.11.163
Lin, Q., Chen, H., Zhang, M., Xiong, H., and Jiang, Q. (2019). Knocking Down FAM83B Inhibits Endometrial Cancer Cell Proliferation and Metastasis by Silencing the PI3K/AKT/mTOR Pathway. Biomed. Pharmacother. 115, 108939. doi:10.1016/j.biopha.2019.108939
Liu, F., Chang, L., and Hu, J. (2020). Activating Transcription Factor 6 Regulated Cell Growth, Migration and Inhibiteds Cell Apoptosis and Autophagy via MAPK Pathway in Cervical Cancer. J. Reprod. Immunol. 139, 103120. doi:10.1016/j.jri.2020.103120
Liu, S. M., Ou, S. Y., and Huang, H. H. (2017). Green Tea Polyphenols Induce Cell Death in Breast Cancer MCF-7 Cells through Induction of Cell Cycle Arrest and Mitochondrial-Mediated Apoptosis. J. Zhejiang Univ. Sci. B 18, 89–98. doi:10.1631/jzus.B1600022
Liu, S., Wang, X. J., Liu, Y., and Cui, Y. F. (2013). PI3K/AKT/mTOR Signaling Is Involved in (-)-Epigallocatechin-3-Gallate-Induced Apoptosis of Human Pancreatic Carcinoma Cells. Am. J. Chin. Med. 41, 629–642. doi:10.1142/S0192415X13500444
Liu, S., Xu, Z. L., Sun, L., Liu, Y., Li, C. C., Li, H. M., et al. (2016). Epigallocatechin3gallate Induces Apoptosis in Human Pancreatic Cancer Cells via PTEN. Mol. Med. Rep. 14, 599–605. doi:10.3892/mmr.2016.5277
Lu, C., Li, X., Ren, Y., and Zhang, X. (2021a). Disulfiram: a Novel Repurposed Drug for Cancer Therapy. Cancer Chemother. Pharmacol. 87, 159–172. doi:10.1007/s00280-020-04216-8
Lu, G., Wu, Z., Shang, J., Xie, Z., Chen, C., and Zhang, C. (2021b). The Effects of Metformin on Autophagy. Biomed. Pharmacother. 137, 111286. doi:10.1016/j.biopha.2021.111286
Lu, K. H., and Broaddus, R. R. (2020). Endometrial Cancer. N. Engl. J. Med. 383, 2053–2064. doi:10.1056/NEJMra1514010
Luo, T., Wang, J., Yin, Y., Hua, H., Jing, J., Sun, X., et al. (2010). (-)-Epigallocatechin Gallate Sensitizes Breast Cancer Cells to Paclitaxel in a Murine Model of Breast Carcinoma. Breast Cancer Res. 12, R8. doi:10.1186/bcr2473
Maeda-Yamamoto, M., Suzuki, N., Sawai, Y., Miyase, T., Sano, M., Hashimoto-Ohta, A., et al. (2003). Association of Suppression of Extracellular Signal-Regulated Kinase Phosphorylation by Epigallocatechin Gallate with the Reduction of Matrix Metalloproteinase Activities in Human Fibrosarcoma HT1080 Cells. J. Agric. Food Chem. 51, 1858–1863. doi:10.1021/jf021039l
Maiti, T. K., Chatterjee, J., and Dasgupta, S. (2003). Effect of Green Tea Polyphenols on Angiogenesis Induced by an Angiogenin-like Protein. Biochem. Biophys. Res. Commun. 308, 64–67. doi:10.1016/s0006-291x(03)01338-x
Man, G. C. W., Wang, J., Song, Y., Wong, J. H., Zhao, Y., Lau, T. S., et al. (2020). Therapeutic Potential of a Novel Prodrug of Green Tea Extract in Induction of Apoptosis via ERK/JNK and Akt Signaling Pathway in Human Endometrial Cancer. BMC Cancer 20, 964. doi:10.1186/s12885-020-07455-3
Mathew, R., Karp, C. M., Beaudoin, B., Vuong, N., Chen, G., Chen, H. Y., et al. (2009). Autophagy Suppresses Tumorigenesis through Elimination of P62. Cell 137, 1062–1075. doi:10.1016/j.cell.2009.03.048
Maycotte, P., and Thorburn, A. (2014). Targeting Autophagy in Breast Cancer. World J. Clin. Oncol. 5, 224–240. doi:10.5306/wjco.v5.i3.224
Mazumder, M. E. H., Beale, P., Chan, C., Yu, J. Q., and Huq, F. (2012). Epigallocatechin Gallate Acts Synergistically in Combination with Cisplatin and Designed Trans -palladiums in Ovarian Cancer Cells. Anticancer Res. 32, 4851–4860.
Mbuthia, K. S., Mireji, P. O., Ngure, R. M., Stomeo, F., Kyallo, M., Muoki, C., et al. (2017). Tea (Camellia Sinensis) Infusions Ameliorate Cancer in 4TI Metastatic Breast Cancer Model. BMC Complementary Altern. Med. 17, 202. doi:10.1186/s12906-017-1683-6
Mccarthy, S., Caporali, A., Enkemann, S., Scaltriti, M., Eschrich, S., Davalli, P., et al. (2007). Green Tea Catechins Suppress the DNA Synthesis Marker MCM7 in the TRAMP Model of Prostate Cancer. Mol. Oncol. 1, 196–204. doi:10.1016/j.molonc.2007.05.007
Mcdonnell, A. M., Pyles, H. M., Diaz-Cruz, E. S., and Barton, C. E. (2019). Enoxacin and Epigallocatechin Gallate (EGCG) Act Synergistically to Inhibit the Growth of Cervical Cancer Cells in Culture. Molecules 24, 1580. doi:10.3390/molecules24081580
Mclarty, J., Bigelow, R. L. H., Smith, M., Elmajian, D., Ankem, M., and Cardelli, J. A. (2009). Tea Polyphenols Decrease Serum Levels of Prostate-specific Antigen, Hepatocyte Growth Factor, and Vascular Endothelial Growth Factor in Prostate Cancer Patients and Inhibit Production of Hepatocyte Growth Factor and Vascular Endothelial Growth Factor in V. Cancer Prev. Res. 2, 673–682. doi:10.1158/1940-6207.CAPR-08-0167
Mineva, N. D., Paulson, K. E., Naber, S. P., Yee, A. S., and Sonenshein, G. E. (2013). Epigallocatechin-3-gallate Inhibits Stem-like Inflammatory Breast Cancer Cells. PloS one 8, e73464. doi:10.1371/journal.pone.0073464
Mizushima, N., and Levine, B. (2020). Autophagy in Human Diseases. N. Engl. J. Med. 383, 1564–1576. doi:10.1056/NEJMra2022774
Mizushima, N., Levine, B., Cuervo, A. M., and Klionsky, D. J. (2008). Autophagy Fights Disease through Cellular Self-Digestion. Nature 451, 1069–1075. doi:10.1038/nature06639
Mizushima, N., and Yoshimori, T. (2007). How to Interpret LC3 Immunoblotting. Autophagy 3, 542–545. doi:10.4161/auto.4600
Modernelli, A., Naponelli, V., Giovanna Troglio, M., Bonacini, M., Ramazzina, I., Bettuzzi, S., et al. (2015). EGCG Antagonizes Bortezomib Cytotoxicity in Prostate Cancer Cells by an Autophagic Mechanism. Sci. Rep. 5, 15270. doi:10.1038/srep15270
Moradzadeh, M., Hosseini, A., Erfanian, S., and Rezaei, H. (2017). Epigallocatechin-3-gallate Promotes Apoptosis in Human Breast Cancer T47D Cells through Down-Regulation of PI3K/AKT and Telomerase. Pharmacol. Rep. 69, 924–928. doi:10.1016/j.pharep.2017.04.008
Morice, P., Leary, A., Creutzberg, C., Abu-Rustum, N., and Darai, E. (2016). Endometrial Cancer. Lancet 387, 1094–1108. doi:10.1016/S0140-6736(15)00130-0
Mowers, E. E., Sharifi, M. N., and Macleod, K. F. (2017). Autophagy in Cancer Metastasis. Oncogene 36, 1619–1630. doi:10.1038/onc.2016.333
Mukherjee, S., Siddiqui, M. A., Dayal, S., Ayoub, Y. Z., and Malathi, K. (2014). Epigallocatechin-3-gallate Suppresses Proinflammatory Cytokines and Chemokines Induced by Toll-like Receptor 9 Agonists in Prostate Cancer Cells. J. Inflamm. Res. 7, 89–101. doi:10.2147/JIR.S61365
Muthusami, S., Prabakaran, D. S., An, Z., Yu, J. R., and Park, W. Y. (2013). EGCG Suppresses Fused Toes Homolog Protein through P53 in Cervical Cancer Cells. Mol. Biol. Rep. 40, 5587–5596. doi:10.1007/s11033-013-2660-x
Nair, S., Barve, A., Khor, T. O., Shen, G. X., Lin, W., Chan, J. Y., et al. (2010). Regulation of Nrf2-And AP-1-Mediated Gene Expression by Epigallocatechin-3-Gallate and Sulforaphane in Prostate of Nrf2-Knockout or C57BL/6J Mice and PC-3 AP-1 Human Prostate Cancer Cells. Acta Pharmacol. Sin. 31, 1223–1240. doi:10.1038/aps.2010.147
Nakachi, K., Suemasu, K., Suga, K., Takeo, T., Imai, K., and Higashi, Y. (1998). Influence of Drinking Green Tea on Breast Cancer Malignancy Among Japanese Patients. Jpn. J. Cancer Res. 89, 254–261. doi:10.1111/j.1349-7006.1998.tb00556.x
Nakkas, H., Ocal, B. G., Kipel, S., Akcan, G., Sahin, C., Ardicoglu, A., et al. (2021). Ubiquitin Proteasome System and Autophagy Associated Proteins in Human Testicular Tumors. Tissue Cell 71, 101513. doi:10.1016/j.tice.2021.101513
Naponelli, V., Modernelli, A., Bettuzzi, S., and Rizzi, F. (2015). Roles of Autophagy Induced by Natural Compounds in Prostate Cancer. Biomed. Res. Int. 2015, 121826. doi:10.1155/2015/121826
Neufeld, T. P. (2012). Autophagy and Cell Growth-Tthe Yin and Yang of Nutrient Responses. J. Cell Sci. 125, 2359–2368. doi:10.1242/jcs.103333
Nguyen, M. M., Ahmann, F. R., Nagle, R. B., Hsu, C. H., Tangrea, J. A., Parnes, H. L., et al. (2012). Randomized, Double-Blind, Placebo-Controlled Trial of Polyphenon E in Prostate Cancer Patients before Prostatectomy: Evaluation of Potential Chemopreventive Activities. Cancer Prev. Res. (Phila) 5, 290–298. doi:10.1158/1940-6207.CAPR-11-0306
Noguchi, M., Hirata, N., Tanaka, T., Suizu, F., Nakajima, H., and Chiorini, J. A. (2020). Autophagy as a Modulator of Cell Death Machinery. Cell Death Dis. 11, 517. doi:10.1038/s41419-020-2724-5
Nunez-Olvera, S. I., Gallardo-Rincon, D., Puente-Rivera, J., Salinas-Vera, Y. M., Marchat, L. A., Morales-Villegas, R., et al. (2019). Autophagy Machinery as a Promising Therapeutic Target in Endometrial Cancer. Front. Oncol. 9, 1326. doi:10.3389/fonc.2019.01326
Opuwari, C., and Monsees, T. (2020). Green Tea Consumption Increases Sperm Concentration and Viability in Male Rats and Is Safe for Reproductive, Liver and Kidney Health. Sci. Rep. 10, 15269. doi:10.1038/s41598-020-72319-6
Ozpolat, B., and Benbrook, D. M. (2015). Targeting Autophagy in Cancer Management - Strategies and Developments. Cancer Manag. Res. 7, 291–299. doi:10.2147/CMAR.S34859
Pal, D., Sur, S., Roy, R., Mandal, S., and Kumar Panda, C. (2018). Epigallocatechin Gallate in Combination with Eugenol or Amarogentin Shows Synergistic Chemotherapeutic Potential in Cervical Cancer Cell Line. J. Cell. Physiology 234, 825–836. doi:10.1002/jcp.26900
Pan, M. H., Lin, C. C., Lin, J. K., and Chen, W. J. (2007). Tea Polyphenol (-)-epigallocatechin 3-gallate Suppresses Heregulin-Β1-Induced Fatty Acid Synthase Expression in Human Breast Cancer Cells by Inhibiting Phosphatidylinositol 3-kinase/Akt and Mitogen-Activated Protein Kinase Cascade Signaling. J. Agric. Food Chem. 55, 5030–5037. doi:10.1021/jf070316r
Panji, M., Behmard, V., Zare, Z., Malekpour, M., Nejadbiglari, H., Yavari, S., et al. (2021a). Synergistic Effects of Green Tea Extract and Paclitaxel in the Induction of Mitochondrial Apoptosis in Ovarian Cancer Cell Lines. Gene 787, 145638. doi:10.1016/j.gene.2021.145638
Panji, M., Behmard, V., Zare, Z., Malekpour, M., Nejadbiglari, H., Yavari, S., et al. (2021b). Suppressing Effects of Green Tea Extract and Epigallocatechin-3-Gallate (EGCG) on TGF-β- Induced Epithelial-To-Mesenchymal Transition via ROS/Smad Signaling in Human Cervical Cancer Cells. Gene 794, 145774. doi:10.1016/j.gene.2021.145774
Papandreou, C. N., and Logothetis, C. J. (2004). Bortezomib as a Potential Treatment for Prostate Cancer. Cancer Res. 64, 5036–5043. doi:10.1158/0008-5472.CAN-03-2707
Park, H., Cho, M., Do, Y., Park, J. K., Bae, S. J., Joo, J., et al. (2021). Autophagy as a Therapeutic Target of Natural Products Enhancing Embryo Implantation. Pharm. (Basel) 15, 53. doi:10.3390/ph15010053
Paul, P., Koh, W. P., Jin, A., Michel, A., Waterboer, T., Pawlita, M., et al. (2019). Soy and Tea Intake on Cervical Cancer Risk: the Singapore Chinese Health Study. Cancer Causes Control 30, 847–857. doi:10.1007/s10552-019-01173-3
Pianetti, S., Guo, S., Kavanagh, K. T., and Sonenshein, G. E. (2002). Green Tea Polyphenol Epigallocatechin-3 Gallate Inhibits Her-2/neu Signaling, Proliferation, and Transformed Phenotype of Breast Cancer Cells. Cancer Res. 62, 652–655.
Pilleron, S., Soto-Perez-De-Celis, E., Vignat, J., Ferlay, J., Soerjomataram, I., Bray, F., et al. (2021). Estimated Global Cancer Incidence in the Oldest Adults in 2018 and Projections to 2050. Int. J. Cancer 148, 601–608. doi:10.1002/ijc.33232
Poorvu, P. D., Frazier, A. L., Feraco, A. M., Manley, P. E., Ginsburg, E. S., Laufer, M. R., et al. (2019). Cancer Treatment-Related Infertility: A Critical Review of the Evidence. JNCI Cancer Spectr. 3, pkz008. doi:10.1093/jncics/pkz008
Qin, G., Li, P., and Xue, Z. (2018). Triptolide Induces Protective Autophagy and Apoptosis in Human Cervical Cancer Cells by Downregulating Akt/mTOR Activation. Oncol. Lett. 16, 3929–3934. doi:10.3892/ol.2018.9074
Qin, J., Fu, M., Wang, J., Huang, F., Liu, H., Huangfu, M., et al. (2020). PTEN/AKT/mTOR Signaling Mediates Anticancer Effects of Epigallocatechin-3-Gallate in Ovarian Cancer. Oncol. Rep. 43, 1885–1896. doi:10.3892/or.2020.7571
Qu, X., Yu, J., Bhagat, G., Furuya, N., Hibshoosh, H., Troxel, A., et al. (2003). Promotion of Tumorigenesis by Heterozygous Disruption of the Beclin 1 Autophagy Gene. J. Clin. Invest 112, 1809–1820. doi:10.1172/JCI20039
Rahman, M. A., Saha, S. K., Rahman, M. S., Uddin, M. J., Uddin, M. S., Pang, M. G., et al. (2020). Molecular Insights into Therapeutic Potential of Autophagy Modulation by Natural Products for Cancer Stem Cells. Front. Cell Dev. Biol. 8, 283. doi:10.3389/fcell.2020.00283
Rahman, S. U., Huang, Y., Zhu, L., Feng, S., Khan, I. M., Wu, J., et al. (2018). Therapeutic Role of Green Tea Polyphenols in Improving Fertility: A Review. Nutrients 10, 834. doi:10.3390/nu10070834
Ram Kumar, R. M., and Schor, N. F. (2018). Methylation of DNA and Chromatin as a Mechanism of Oncogenesis and Therapeutic Target in Neuroblastoma. Oncotarget 9, 22184–22193. doi:10.18632/oncotarget.25084
Rao, S. D., and Pagidas, K. (2010). Epigallocatechin-3-gallate, a Natural Polyphenol, Inhibits Cell Proliferation and Induces Apoptosis in Human Ovarian Cancer Cells. Anticancer Res. 30, 2519–2523.
Raufi, A. G., Liguori, N. R., Carlsen, L., Parker, C., Hernandez Borrero, L., Zhang, S., et al. (2021). Therapeutic Targeting of Autophagy in Pancreatic Ductal Adenocarcinoma. Front. Pharmacol. 12, 751568. doi:10.3389/fphar.2021.751568
Reddy, D., Kumavath, R., Tan, T. Z., Ampasala, D. R., and Kumar, A. P. (2020). Peruvoside Targets Apoptosis and Autophagy through MAPK Wnt/beta-Catenin and PI3K/AKT/mTOR Signaling Pathways in Human Cancers. Life Sci. 241, 117147. doi:10.1016/j.lfs.2019.117147
Reuter, V. E. (2005). Origins and Molecular Biology of Testicular Germ Cell Tumors. Mod. Pathol. 18 (Suppl. 2), S51–S60. doi:10.1038/modpathol.3800309
Rizzi, F., Naponelli, V., Silva, A., Modernelli, A., Ramazzina, I., Bonacini, M., et al. (2014). Polyphenon E(R), a Standardized Green Tea Extract, Induces Endoplasmic Reticulum Stress, Leading to Death of Immortalized PNT1a Cells by Anoikis and Tumorigenic PC3 by Necroptosis. Carcinogenesis 35, 828–839. doi:10.1093/carcin/bgt481
Roomi, M. W., Cha, J., Kalinovsky, T., Roomi, N., Niedzwiecki, A., and Rath, M. (2015a). Effect of a Nutrient Mixture on the Localization of Extracellular Matrix Proteins in HeLa Human Cervical Cancer Xenografts in Female Nude Mice. Exp. Ther. Med. 10, 901–906. doi:10.3892/etm.2015.2591
Roomi, M. W., Ivanov, V., Kalinovsky, T., Niedzwiecki, A., and Rath, M. (2005a). In Vitro and In Vivo Antitumorigenic Activity of a Mixture of Lysine, Proline, Ascorbic Acid, and Green Tea Extract on Human Breast Cancer Lines MDA-MB-231 and MCF-7. Med. Oncol. 22, 129–138. doi:10.1385/MO:22:2:129
Roomi, M. W., Ivanov, V., Kalinovsky, T., Niedzwiecki, A., and Rath, M. (2005b). In Vivo antitumor Effect of Ascorbic Acid, Lysine, Proline and Green Tea Extract on Human Prostate Cancer PC-3 Xenografts in Nude Mice: Evaluation of Tumor Growth and Immunohistochemistry. Vivo 19, 179–184.
Roomi, M. W., Ivanov, V., Kalinovsky, T., Niedzwiecki, A., and Rath, M. (2007). Inhibitory Effects of a Nutrient Mixture on Human Testicular Cancer Cell Line NT 2/DT Matrigel Invasion and MMP Activity. Med. Oncol. 24, 183–188. doi:10.1007/BF02698038
Roomi, M. W., Kalinovsky, T., Cha, J., Roomi, N. W., Niedzwiecki, A., and Rath, M. (2015b). Effects of a Nutrient Mixture on Immunohistochemical Localization of Cancer Markers in Human Cervical Cancer Hela Cell Tumor Xenografts in Female Nude Mice. Exp. Ther. Med. 9, 294–302. doi:10.3892/etm.2014.2127
Roomi, M. W., Kalinovsky, T., Roomi, N. M., Cha, J., Rath, M., and Niedzwiecki, A. (2014). In Vitro and In Vivo Effects of a Nutrient Mixture on Breast Cancer Progression. Int. J. Oncol. 45, 1933–1944. doi:10.3892/ijo.2014.2379
Roth, Z., Aroyo, A., Yavin, S., and Arav, A. (2008). The Antioxidant Epigallocatechin Gallate (EGCG) Moderates the Deleterious Effects of Maternal Hyperthermia on Follicle-Enclosed Oocytes in Mice. Theriogenology 70, 887–897. doi:10.1016/j.theriogenology.2008.05.053
Roychoudhury, S., Agarwal, A., Virk, G., and Cho, C. L. (2017). Potential Role of Green Tea Catechins in the Management of Oxidative Stress-Associated Infertility. Reprod. Biomed. Online 34, 487–498. doi:10.1016/j.rbmo.2017.02.006
Saedmocheshi, S., Saghebjoo, M., Vahabzadeh, Z., and Sheikholeslami-Vatani, D. (2019). Aerobic Training and Green Tea Extract Protect against N-Methyl-N-Nitrosourea-Induced Prostate Cancer. Med. Sci. Sports Exerc 51, 2210–2216. doi:10.1249/MSS.0000000000002054
Sah, J. F., Balasubramanian, S., Eckert, R. L., and Rorke, E. A. (2004). Epigallocatechin-3-gallate Inhibits Epidermal Growth Factor Receptor Signaling Pathway. Evidence for Direct Inhibition of ERK1/2 and AKT Kinases. J. Biol. Chem. 279, 12755–12762. doi:10.1074/jbc.M312333200
Saleem, M., Adhami, V. M., Ahmad, N., Gupta, S., and Mukhtar, H. (2005). Prognostic Significance of Metastasis-Associated Protein S100A4 (Mts1) in Prostate Cancer Progression and Chemoprevention Regimens in an Autochthonous Mouse Model. Clin. Cancer Res. 11, 147–153.
Salminen, A., Hyttinen, J. M., Kauppinen, A., and Kaarniranta, K. (2012). Context-Dependent Regulation of Autophagy by IKK-NF-kappaB Signaling: Impact on the Aging Process. Int. J. Cell Biol. 2012, 849541. doi:10.1155/2012/849541
Samavat, H., Ursin, G., Emory, T. H., Lee, E., Wang, R., Torkelson, C. J., et al. (2017). A Randomized Controlled Trial of Green Tea Extract Supplementation and Mammographic Density in Postmenopausal Women at Increased Risk of Breast Cancer. Cancer Prev. Res. 10, 710–718. doi:10.1158/1940-6207.CAPR-17-0187
Samavat, H., Wu, A. H., Ursin, G., Torkelson, C. J., Wang, R., Yu, M. C., et al. (2019). Green Tea Catechin Extract Supplementation Does Not Influence Circulating Sex Hormones and Insulin-like Growth Factor axis Proteins in a Randomized Controlled Trial of Postmenopausal Women at High Risk of Breast Cancer. J. Nutr. 149, 619–627. doi:10.1093/jn/nxy316
Santos, R. A., Andrade, E. D. S., Monteiro, M., Fialho, E., Silva, J. L., Daleprane, J. B., et al. (2021). Green Tea (Camellia Sinensis) Extract Induces P53-Mediated Cytotoxicity and Inhibits Migration of Breast Cancer Cells. Foods 10, 3154. doi:10.3390/foods10123154
Sartippour, M. R., Heber, D., Ma, J., Lu, Q., Go, V. L., and Nguyen, M. (2001). Green Tea and its Catechins Inhibit Breast Cancer Xenografts. Nutr. Cancer 40, 149–156. doi:10.1207/S15327914NC402_11
Sartippour, M. R., Pietras, R., Marquez-Garban, D. C., Chen, H. W., Heber, D., Henning, S. M., et al. (2006). The Combination of Green Tea and Tamoxifen Is Effective against Breast Cancer. Carcinogenesis 27, 2424–2433. doi:10.1093/carcin/bgl066
Sartippour, M. R., Shao, Z. M., Heber, D., Beatty, P., Zhang, L., Liu, C., et al. (2002). Green Tea Inhibits Vascular Endothelial Growth Factor (VEGF) Induction in Human Breast Cancer Cells. J. Nutr. 132, 2307–2311. doi:10.1093/jn/132.8.2307
Sawada, N. (2017). Risk and Preventive Factors for Prostate Cancer in Japan: The Japan Public Health Center-based Prospective (JPHC) Study. J. Epidemiol. 27, 2–7. doi:10.1016/j.je.2016.09.001
Scaltriti, M., Belloni, L., Caporali, A., Davalli, P., Remondini, D., Rizzi, F., et al. (2006). Molecular Classification of Green Tea Catechin-Sensitive and Green Tea Catechin-Resistant Prostate Cancer in the TRAMP Mice Model by Quantitative Real-Time PCR Gene Profiling. Carcinogenesis 27, 1047–1053. doi:10.1093/carcin/bgi287
Schramm, L. (2013). Going Green: The Role of the Green Tea Component EGCG in Chemoprevention. J. Carcinog. Mutagen 4, 1000142. doi:10.4172/2157-2518.1000142
Semenza, G. L. (2010). HIF-1: Upstream and Downstream of Cancer Metabolism. Curr. Opin. Genet. Dev. 20, 51–56. doi:10.1016/j.gde.2009.10.009
Shan, L., Gao, G., Wang, W., Tang, W., Wang, Z., Yang, Z., et al. (2019). Self-assembled Green Tea Polyphenol-Based Coordination Nanomaterials to Improve Chemotherapy Efficacy by Inhibition of Carbonyl Reductase 1. Biomaterials 210, 62–69. doi:10.1016/j.biomaterials.2019.04.032
Shimizu, M., Deguchi, A., Lim, J. T., Moriwaki, H., Kopelovich, L., and Weinstein, I. B. (2005). (-)-Epigallocatechin Gallate and Polyphenon E Inhibit Growth and Activation of the Epidermal Growth Factor Receptor and Human Epidermal Growth Factor Receptor-2 Signaling Pathways in Human Colon Cancer Cells. Clin. Cancer Res. 11, 2735–2746. doi:10.1158/1078-0432.CCR-04-2014
Shirakami, Y., and Shimizu, M. (2018). Possible Mechanisms of Green Tea and its Constituents against Cancer. Molecules 23, 2284. doi:10.3390/molecules23092284
Siddiqui, I. A., Adhami, V. M., Afaq, F., Ahmad, N., and Mukhtar, H. (2004). Modulation of Phosphatidylinositol-3-Kinase/protein Kinase B- and Mitogen-Activated Protein Kinase-Pathways by Tea Polyphenols in Human Prostate Cancer Cells. J. Cell. Biochem. 91, 232–242. doi:10.1002/jcb.10737
Siddiqui, I. A., Asim, M., Hafeez, B. B., Adhami, V. M., Tarapore, R. S., and Mukhtar, H. (2011). Green Tea Polyphenol EGCG Blunts Androgen Receptor Function in Prostate Cancer. FASEB J. 25, 1198–1207. doi:10.1096/fj.10-167924
Siddiqui, I. A., Shukla, Y., Adhami, V. M., Sarfaraz, S., Asim, M., Hafeez, B. B., et al. (2008). Suppression of NFκB and its Regulated Gene Products by Oral Administration of Green Tea Polyphenols in an Autochthonous Mouse Prostate Cancer Model. Pharm. Res. 25, 2135–2142. doi:10.1007/s11095-008-9553-z
Singh, M., Singh, R., Bhui, K., Tyagi, S., Mahmood, Z., and Shukla, Y. (2011). Tea Polyphenols Induce Apoptosis through Mitochondrial Pathway and by Inhibiting Nuclear Factor-Κb and Akt Activation in Human Cervical Cancer Cells. Oncol. Res. 19, 245–257. doi:10.3727/096504011x13021877989711
Solomon, V. R., and Lee, H. (2009). Chloroquine and its Analogs: a New Promise of an Old Drug for Effective and Safe Cancer Therapies. Eur. J. Pharmacol. 625, 220–233. doi:10.1016/j.ejphar.2009.06.063
Spinaci, M., Volpe, S., De Ambrogi, M., Tamanini, C., and Galeati, G. (2008). Effects of Epigallocatechin-3-Gallate (EGCG) on In Vitro Maturation and Fertilization of Porcine Oocytes. Theriogenology 69, 877–885. doi:10.1016/j.theriogenology.2008.01.005
Stanislawska, I. J., Granica, S., Piwowarski, J. P., Szawkalo, J., Wiazecki, K., Czarnocki, Z., et al. (2019). The Activity of Urolithin A and M4 Valerolactone, Colonic Microbiota Metabolites of Polyphenols, in a Prostate Cancer In Vitro Model. Planta Med. 85, 118–125. doi:10.1055/a-0755-7715
Su, M., Mei, Y., and Sinha, S. (2013). Role of the Crosstalk between Autophagy and Apoptosis in Cancer. J. Oncol. 2013, 102735. doi:10.1155/2013/102735
Suganuma, M., Saha, A., and Fujiki, H. (2011). New Cancer Treatment Strategy Using Combination of Green Tea Catechins and Anticancer Drugs. Cancer Sci. 102, 317–323. doi:10.1111/j.1349-7006.2010.01805.x
Sun, Y., Zou, M., Hu, C., Qin, Y., Song, X., Lu, N., et al. (2013). Wogonoside Induces Autophagy in MDA-MB-231 Cells by Regulating MAPK-mTOR Pathway. Food Chem. Toxicol. 51, 53–60. doi:10.1016/j.fct.2012.09.012
Sung, H., Ferlay, J., Siegel, R. L., Laversanne, M., Soerjomataram, I., Jemal, A., et al. (2021). Global Cancer Statistics 2020: GLOBOCAN Estimates of Incidence and Mortality Worldwide for 36 Cancers in 185 Countries. CA Cancer J. Clin. 71, 209–249. doi:10.3322/caac.21660
Takenokuchi, M., Miyamoto, K., Saigo, K., and Taniguchi, T. (2015). Bortezomib Causes ER Stress-Related Death of Acute Promyelocytic Leukemia Cells through Excessive Accumulation of PML-RARA. Anticancer Res. 35, 3307–3316.
Tam, C., Rao, S., Waye, M. M. Y., Ng, T. B., and Wang, C. C. (2021). Autophagy Signals Orchestrate Chemoresistance of Gynecological Cancers. Biochim. Biophys. Acta Rev. Cancer 1875, 188525. doi:10.1016/j.bbcan.2021.188525
Tang, D., Kang, R., Livesey, K. M., Cheh, C. W., Farkas, A., Loughran, P., et al. (2010). Endogenous HMGB1 Regulates Autophagy. J. Cell Biol. 190, 881–892. doi:10.1083/jcb.200911078
Teichert, F., Verschoyle, R. D., Greaves, P., Jones, D. J. L., Wilson, I. D., Farmer, P. B., et al. (2010). Plasma Metabolic Profiling Reveals Age-Dependency of Systemic Effects of Green Tea Polyphenols in Mice with and without Prostate Cancer. Mol. Biosyst. 6, 1911–1916. doi:10.1039/c004702c
Terzic, M., Aimagambetova, G., Kunz, J., Bapayeva, G., Aitbayeva, B., Terzic, S., et al. (2021). Molecular Basis of Endometriosis and Endometrial Cancer: Current Knowledge and Future Perspectives. Int. J. Mol. Sci. 22, 9274. doi:10.3390/ijms22179274
Testa, U., Castelli, G., and Pelosi, E. (2019). Cellular and Molecular Mechanisms Underlying Prostate Cancer Development: Therapeutic Implications. Med. (Basel) 6, 82. doi:10.3390/medicines6030082
Thangapazham, R. L., Singh, A. K., Sharma, A., Warren, J., Gaddipati, J. P., and Maheshwari, R. K. (2007). Green Tea Polyphenols and its Constituent Epigallocatechin Gallate Inhibits Proliferation of Human Breast Cancer Cells In Vitro and In Vivo. Cancer Lett. 245, 232–241. doi:10.1016/j.canlet.2006.01.027
Thomas, R., and Kim, M. H. (2005). Epigallocatechin Gallate Inhibits HIF-1α Degradation in Prostate Cancer Cells. Biochem. Biophysical Res. Commun. 334, 543–548. doi:10.1016/j.bbrc.2005.06.114
Tong, D. (2021). Unravelling the Molecular Mechanisms of Prostate Cancer Evolution from Genotype to Phenotype. Crit. Rev. Oncol. Hematol. 163, 103370. doi:10.1016/j.critrevonc.2021.103370
Torgovnick, A., and Schumacher, B. (2015). DNA Repair Mechanisms in Cancer Development and Therapy. Front. Genet. 6, 157. doi:10.3389/fgene.2015.00157
Trocoli, A., and Djavaheri-Mergny, M. (2011). The Complex Interplay between Autophagy and NF-kappaB Signaling Pathways in Cancer Cells. Am. J. Cancer Res. 1, 629–649.
Trudel, D., Labbé, D. P., Araya-Farias, M., Doyen, A., Bazinet, L., Duchesne, T., et al. (2013). A Two-Stage, Single-Arm, Phase II Study of EGCG-Enriched Green Tea Drink as a Maintenance Therapy in Women with Advanced Stage Ovarian Cancer. Gynecol. Oncol. 131, 357–361. doi:10.1016/j.ygyno.2013.08.019
Tsai, C. Y., Chen, C. Y., Chiou, Y. H., Shyu, H. W., Lin, K. H., Chou, M. C., et al. (2017). Epigallocatechin-3-Gallate Suppresses Human Herpesvirus 8 Replication and Induces ROS Leading to Apoptosis and Autophagy in Primary Effusion Lymphoma Cells. Int. J. Mol. Sci. 19, 16. doi:10.3390/ijms19010016
Tuntiteerawit, P., Jarukamjorn, K., and Porasuphatana, S. (2020). The Effect of Green Tea Catechins on Breast Cancer Resistance Protein Activity and Intestinal Efflux of Aflatoxin B1 via Breast Cancer Resistance Protein in Caco-2 Cells. Toxicol. Res. 36, 293–300. doi:10.1007/s43188-019-00032-2
Tyutyunyk-Massey, L., and Gewirtz, D. A. (2020). Roles of Autophagy in Breast Cancer Treatment: Target, Bystander or Benefactor. Semin. Cancer Biol. 66, 155–162. doi:10.1016/j.semcancer.2019.11.008
Vahabzadeh, Z., Molodi, M., Nikkho, B., Saghebjoo, M., Saedmocheshi, S., Zamani, F., et al. (2020). Aerobic Training and Hydroalcoholic Extracts of Green Tea Improve Pro-oxidant-antioxidant Balance and Histopathological Score in the N-Methyl-N-Nitrosourea-Induced Prostate Cancer Model of Rat. EXCLI J. 19, 762–772. doi:10.17179/excli2019-2069
Vequaud, E., Seveno, C., Loussouarn, D., Engelhart, L., Campone, M., Juin, P., et al. (2015). YM155 Potently Triggers Cell Death in Breast Cancer Cells through an Autophagy-NF-kB Network. Oncotarget 6, 13476–13486. doi:10.18632/oncotarget.3638
Verzella, D., Pescatore, A., Capece, D., Vecchiotti, D., Ursini, M. V., Franzoso, G., et al. (2020). Life, Death, and Autophagy in Cancer: NF-kappaB Turns up Everywhere. Cell Death Dis. 11, 210. doi:10.1038/s41419-020-2399-y
Walboomers, J. M., Jacobs, M. V., Manos, M. M., Bosch, F. X., Kummer, J. A., Shah, K. V., et al. (1999). Human Papillomavirus Is a Necessary Cause of Invasive Cervical Cancer Worldwide. J. Pathol. 189, 12–19. doi:10.1002/(SICI)1096-9896(199909)189:1<12::AID-PATH431>3.0.CO;2-F
Wang, H., Deng, L., Cai, M., Zhuang, H., Zhu, L., Hao, Y., et al. (2017). Annexin A4 Fucosylation Enhances its Interaction with the NF-kB P50 and Promotes Tumor Progression of Ovarian Clear Cell Carcinoma. Oncotarget 8, 108093–108107. doi:10.18632/oncotarget.10226
Wang, J., Man, G. C. W., Chan, T. H., Kwong, J., and Wang, C. C. (2018a). A Prodrug of Green Tea Polyphenol (-)-Epigallocatechin-3-Gallate (Pro-EGCG) Serves as a Novel Angiogenesis Inhibitor in Endometrial Cancer. Cancer Lett. 412, 10–20. doi:10.1016/j.canlet.2017.09.054
Wang, P., Heber, D., and Henning, S. M. (2012). Quercetin Increased the Antiproliferative Activity of Green Tea Polyphenol (-)-epigallocatechin Gallate in Prostate Cancer Cells. Nutr. Cancer 64, 580–587. doi:10.1080/01635581.2012.661514
Wang, Q., He, W. Y., Zeng, Y. Z., Hossain, A., and Gou, X. (2018b). Inhibiting Autophagy Overcomes Docetaxel Resistance in Castration-Resistant Prostate Cancer Cells. Int. Urol. Nephrol. 50, 675–686. doi:10.1007/s11255-018-1801-5
Wang, Y., Wu, N., and Jiang, N. (2021). Autophagy Provides a Conceptual Therapeutic Framework for Bone Metastasis from Prostate Cancer. Cell Death Dis. 12, 909. doi:10.1038/s41419-021-04181-x
Wei, R., Mao, L., Xu, P., Zheng, X., Hackman, R. M., Mackenzie, G. G., et al. (2018). Suppressing Glucose Metabolism with Epigallocatechin-3-Gallate (EGCG) Reduces Breast Cancer Cell Growth in Preclinical Models. Food Funct. 9, 5682–5696. doi:10.1039/c8fo01397g
White, E., and Dipaola, R. S. (2009). The Double-Edged Sword of Autophagy Modulation in Cancer. Clin. Cancer Res. 15, 5308–5316. doi:10.1158/1078-0432.CCR-07-5023
WHO, (2021). Breast Cancer [Online]. Geneva: World Health Organization. Available at: https://www.who.int/news-room/fact-sheets/detail/breast-cancer (Accessed 03, 2022).
WHO, (2022). Cervical Cancer [Online]. Geneva: World Health Organization. Available: https://www.who.int/news-room/fact-sheets/detail/cervical-cancer (Accessed 03, 2022).
Xi, B., Huang, Y., Reilly, K. H., Li, S., Zheng, R., Barrio-Lopez, M. T., et al. (2015). Sugar-sweetened Beverages and Risk of Hypertension and CVD: a Dose-Response Meta-Analysis. Br. J. Nutr. 113, 709–717. doi:10.1017/S0007114514004383
Xinqiang, S., Mu, Z., Lei, C., and Mun, L. Y. (2017). Bioinformatics Analysis on Molecular Mechanism of Green Tea Compound Epigallocatechin-3-Gallate against Ovarian Cancer. Clin. Transl. Sci. 10, 302–307. doi:10.1111/cts.12470
Xu, L., Zhang, X., Li, Y., Lu, S., Lu, S., Li, J., et al. (2016). Neferine Induces Autophagy of Human Ovarian Cancer Cells via P38 MAPK/JNK Activation. Tumour Biol. 37, 8721–8729. doi:10.1007/s13277-015-4737-8
Xu, P., Yan, F., Zhao, Y., Chen, X., Sun, S., Wang, Y., et al. (2020). Green Tea Polyphenol EGCG Attenuates MDSCs-Mediated Immunosuppression through Canonical and Non-canonical Pathways in a 4T1 Murine Breast Cancer Model. Nutrients 12, 1042. doi:10.3390/nu12041042
Xuan, F., and Jian, J. (2016). Epigallocatechin Gallate Exerts Protective Effects against Myocardial Ischemia/reperfusion Injury through the PI3K/Akt Pathway-Mediated Inhibition of Apoptosis and the Restoration of the Autophagic Flux. Int. J. Mol. Med. 38, 328–336. doi:10.3892/ijmm.2016.2615
Yang, K., Gao, Z. Y., Li, T. Q., Song, W., Xiao, W., Zheng, J., et al. (2019). Anti-tumor Activity and the Mechanism of a Green Tea (Camellia Sinensis) Polysaccharide on Prostate Cancer. Int. J. Biol. Macromol. 122, 95–103. doi:10.1016/j.ijbiomac.2018.10.101
Yang, S., Shao, S., Huang, B., Yang, D., Zeng, L., Gan, Y., et al. (2020). Tea Polyphenols Alleviate Tri-ortho-cresyl Phosphate-Induced Autophagy of Mouse Ovarian Granulosa Cells. Environ. Toxicol. 35, 478–486. doi:10.1002/tox.22883
Yeung, T. L., Leung, C. S., Yip, K. P., Au Yeung, C. L., Wong, S. T., and Mok, S. C. (2015). Cellular and Molecular Processes in Ovarian Cancer Metastasis. A Review in the Theme: Cell and Molecular Processes in Cancer Metastasis. Am. J. Physiol. Cell Physiol. 309, C444–C456. doi:10.1152/ajpcell.00188.2015
Yoon, J. S., Kristal, A. R., Wicklund, K. G., Cushing-Haugen, K. L., and Rossing, M. A. (2008). Coffee, Tea, Colas, and Risk of Epithelial Ovarian Cancer. Cancer Epidemiol. Biomarkers Prev. 17, 712–716. doi:10.1158/1055-9965.EPI-07-2511
Yousefi, S., Perozzo, R., Schmid, I., Ziemiecki, A., Schaffner, T., Scapozza, L., et al. (2006). Calpain-mediated Cleavage of Atg5 Switches Autophagy to Apoptosis. Nat. Cell Biol. 8, 1124–1132. doi:10.1038/ncb1482
Yuan, C. H., Horng, C. T., Lee, C. F., Chiang, N. N., Tsai, F. J., Lu, C. C., et al. (2017). Epigallocatechin Gallate Sensitizes Cisplatin-Resistant Oral Cancer CAR Cell Apoptosis and Autophagy through Stimulating AKT/STAT3 Pathway and Suppressing Multidrug Resistance 1 Signaling. Environ. Toxicol. 32, 845–855. doi:10.1002/tox.22284
Yugawa, T., and Kiyono, T. (2009). Molecular Mechanisms of Cervical Carcinogenesis by High-Risk Human Papillomaviruses: Novel Functions of E6 and E7 Oncoproteins. Rev. Med. Virol. 19, 97–113. doi:10.1002/rmv.605
Zeng, F., and Harris, R. C. (2014). Epidermal Growth Factor, from Gene Organization to Bedside. Semin. Cell Dev. Biol. 28, 2–11. doi:10.1016/j.semcdb.2014.01.011
Zeng, L., Yan, J., Luo, L., Ma, M., and Zhu, H. (2017). Preparation and Characterization of (-)-Epigallocatechin-3-Gallate (EGCG)-loaded Nanoparticles and Their Inhibitory Effects on Human Breast Cancer MCF-7 Cells. Sci. Rep. 7, 45521. doi:10.1038/srep45521
Zhang, C., Qin, Y. Y., Wei, X., Yu, F. F., Zhou, Y. H., and He, J. (2015). Tea Consumption and Risk of Cardiovascular Outcomes and Total Mortality: a Systematic Review and Meta-Analysis of Prospective Observational Studies. Eur. J. Epidemiol. 30, 103–113. doi:10.1007/s10654-014-9960-x
Zhang, M., Binns, C. W., and Lee, A. H. (2002). Tea Consumption and Ovarian Cancer Risk: A Case-Control Study in China. Cancer Epidemiol. Biomarkers Prev. 11, 713–718.
Zhang, M., Lee, A. H., Binns, C. W., and Xie, X. (2004). Green Tea Consumption Enhances Survival of Epithelial Ovarian Cancer. Int. J. Cancer 112, 465–469. doi:10.1002/ijc.20456
Zhang, Q., Tang, X., Lu, Q., Zhang, Z., Rao, J., and Le, A. D. (2006). Green Tea Extract and (-)-Epigallocatechin-3-Gallate Inhibit Hypoxia- and Serum-Induced HIF-1alpha Protein Accumulation and VEGF Expression in Human Cervical Carcinoma and Hepatoma Cells. Mol. Cancer Ther. 5, 1227–1238. doi:10.1158/1535-7163.MCT-05-0490
Zhang, Q. Y., Wang, F. X., Jia, K. K., and Kong, L. D. (2018a). Natural Product Interventions for Chemotherapy and Radiotherapy-Induced Side Effects. Front. Pharmacol. 9, 1253. doi:10.3389/fphar.2018.01253
Zhang, S., Cao, M., and Fang, F. (2020a). The Role of Epigallocatechin-3-Gallate in Autophagy and Endoplasmic Reticulum Stress (ERS)-Induced Apoptosis of Human Diseases. Med. Sci. Monit. 26, e924558. doi:10.12659/MSM.924558
Zhang, Y., Han, G., Fan, B., Zhou, Y., Zhou, X., Wei, L., et al. (2009). Green Tea (-)-Epigallocatechin-3-Gallate Down-Regulates VASP Expression and Inhibits Breast Cancer Cell Migration and Invasion by Attenuating Rac1 Activity. Eur. J. Pharmacol. 606, 172–179. doi:10.1016/j.ejphar.2008.12.033
Zhang, Y., Lin, H., Liu, C., Huang, J., and Liu, Z. (2020b). A Review for Physiological Activities of EGCG and the Role in Improving Fertility in Humans/mammals. Biomed. Pharmacother. 127, 110186. doi:10.1016/j.biopha.2020.110186
Zhang, Y., Sha, R., Zhang, L., Zhang, W., Jin, P., Xu, W., et al. (2018b). Harnessing Copper-Palladium Alloy Tetrapod Nanoparticle-Induced Pro-survival Autophagy for Optimized Photothermal Therapy of Drug-Resistant Cancer. Nat. Commun. 9, 4236. doi:10.1038/s41467-018-06529-y
Zhang, Y., Yang, N. D., Zhou, F., Shen, T., Duan, T., Zhou, J., et al. (2012). (-)-Epigallocatechin-3-gallate Induces Non-apoptotic Cell Death in Human Cancer Cells via ROS-Mediated Lysosomal Membrane Permeabilization. PLoS One 7, e46749. doi:10.1371/journal.pone.0046749
Zhao, L., Liu, S., Xu, J., Li, W., Duan, G., Wang, H., et al. (2017). A New Molecular Mechanism Underlying the EGCG-Mediated Autophagic Modulation of AFP in HepG2 Cells. Cell Death Dis. 8, e3160. doi:10.1038/cddis.2017.563
Zhao, X., Fang, Y., Yang, Y., Qin, Y., Wu, P., Wang, T., et al. (2015). Elaiophylin, a Novel Autophagy Inhibitor, Exerts Antitumor Activity as a Single Agent in Ovarian Cancer Cells. Autophagy 11, 1849–1863. doi:10.1080/15548627.2015.1017185
Zhen, M. C., Huang, X. H., Wang, Q., Sun, K., Liu, Y. J., Li, W., et al. (2006). Green Tea Polyphenol Epigallocatechin-3-Gallate Suppresses Rat Hepatic Stellate Cell Invasion by Inhibition of MMP-2 Expression and its Activation. Acta Pharmacol. Sin. 27, 1600–1607. doi:10.1111/j.1745-7254.2006.00439.x
Zheng, T., Wang, A., Hu, D., and Wang, Y. (2017). Molecular Mechanisms of Breast Cancer Metastasis by Gene Expression Profile Analysis. Mol. Med. Rep. 16, 4671–4677. doi:10.3892/mmr.2017.7157
Zhou, Z. W., Li, X. X., He, Z. X., Pan, S. T., Yang, Y., Zhang, X., et al. (2015). Induction of Apoptosis and Autophagy via Sirtuin1- and PI3K/Akt/mTOR-Mediated Pathways by Plumbagin in Human Prostate Cancer Cells. Drug Des. Devel Ther. 9, 1511–1554. doi:10.2147/DDDT.S75976
Zhu, K., Dunner, K., and Mcconkey, D. J. (2010). Proteasome Inhibitors Activate Autophagy as a Cytoprotective Response in Human Prostate Cancer Cells. Oncogene 29, 451–462. doi:10.1038/onc.2009.343
Keywords: green tea, EGCG, autophagy, reproductive cancers, anticancer
Citation: Hung SW, Li Y, Chen X, Chu KO, Zhao Y, Liu Y, Guo X, Man GC-W and Wang CC (2022) Green Tea Epigallocatechin-3-Gallate Regulates Autophagy in Male and Female Reproductive Cancer. Front. Pharmacol. 13:906746. doi: 10.3389/fphar.2022.906746
Received: 29 March 2022; Accepted: 17 May 2022;
Published: 04 July 2022.
Edited by:
Yubo Zhang, Jinan University, ChinaReviewed by:
Loh Teng Hern Tan, Monash University Malaysia, MalaysiaAyse Kocak, Kutahya Health Sciences University, Turkey
Copyright © 2022 Hung, Li, Chen, Chu, Zhao, Liu, Guo, Man and Wang. This is an open-access article distributed under the terms of the Creative Commons Attribution License (CC BY). The use, distribution or reproduction in other forums is permitted, provided the original author(s) and the copyright owner(s) are credited and that the original publication in this journal is cited, in accordance with accepted academic practice. No use, distribution or reproduction is permitted which does not comply with these terms.
*Correspondence: Gene Chi-Wai Man, geneman@cuhk.edu.hk; Chi Chiu Wang, ccwang@cuhk.edu.hk
†These authors have contributed equally to this work and share the first authorship