- Department of Cardiology, Tangdu Hospital, Air Force Medical University, Xi’an, China
Myocardial infarction (MI) is a coronary artery-related disease and ranks as the leading cause of sudden death globally. Resveratrol (Res) is a bioactive component and has presented antioxidant, anti-inflammatory and anti-microbial properties. However, the effect of Res on ferroptosis during MI progression remains elusive. Here, we aimed to explore the function of Res in the regulation of ferroptosis and myocardial injury in MI. We observed that the treatment of Res attenuated the MI-related myocardium injury and fibrosis in the rats. The expression of collagen 1 and α-SMA was induced in MI rats, in which the treatment of Res could decrease the expression. Treatment of Res suppressed the levels of IL-6 and IL-1β in MI rats. The GSH levels were inhibited and MDA, lipid ROS, and Fe2+ levels were induced in MI rats, in which the treatment of Res could reverse the phenotypes. Meanwhile, the expression of GPX4 and SLC7A11 was reduced in MI rats, while the treatment of Res could rescue the expression in the model. Meanwhile, Res relieved oxygen-glucose deprivation (OGD)-induced cardiomyocyte injury. Importantly, Res repressed OGD-induced cardiomyocyte ferroptosis in vitro. Mechanically, we identified that Res was able to enhance GPX4 expression by inducing KAT5 expression. We confirmed that KAT5 alleviated OGD-induced cardiomyocyte injury and ferroptosis. The depletion of KAT5 or GPX4 could reverse the effect of Res on OGD-induced cardiomyocyte injury. Thus, we concluded that Res attenuated myocardial injury by inhibiting ferroptosis via inducing KAT5/GPX4 in myocardial infarction. Our finding provides new evidence of the potential therapeutic effect of Res on MI by targeting ferroptosis.
Introduction
Myocardial infarction (Fang et al., 2020) is a coronary artery-related disease that severely threatens human life, and ranks as the leading cause of sudden death globally (Anderson and Morrow, 2017). The common pathological factors of MI include abnormal supplement of myocardial oxygen or coronary atherothrombosis (Gulati et al., 2020). When the atherosclerotic thrombosis is raptured, the released plaque causes accumulation of platelets, which leads to occlusion of coronary artery, and subsequent myocardial ischemia and necrosis (Boateng and Sanborn, 2013). On the other hand, the lack of nutrients and oxygen leads to inflammatory response, and death of cardiomyocytes (Cabello et al., 2016; Reed et al., 2017). It has been well-identified that the inflammatory process is involved in the pathogenesis of MI and the pro-inflammatory cytokines, including interleukin‐1β (IL‐1β) and interleukin‐6 (IL-6), were enhanced (Bezerra et al., 2017; Liu et al., 2019). Meanwhile, MI is associated with left ventricular (Liu et al., 2021) remodeling and is featured by the cardiac fibrosis, leading to the increases of fibrosis markers, such as collagen I and α-smooth muscle actin (α-SMA) (Wang et al., 2020). Although the exploration of pathogenesis of MI has made great progress over the past years, the incidence of MI still steadily increases (Chen et al., 2017). Therefore, it is urgent to develop therapeutic manners for MI.
Integrated Traditional Chinese and Western medicine plays an important role in the treatment of various diseases (Bonnefont-Rousselot, 2016). Noteworthy, the Traditional Chinese medicine has been widely applied in the prevention and treatment of metabolic syndrome (Dong-mei et al., 2013; Lu et al., 2019). Res is a bioactive component first discovered in Veratrum gandiflorum roots, and has been widely studies in diseases treatment, owing to its antioxidant, anti-inflammatory and anti-microbial properties (Chaplin and Mercader, 2018; Huang et al., 2019). Moreover, Res has been discovered to modulate multiple molecular targets, such as AMP-activated protein kinases, nuclear factor erythroid 2-associated factor 2, nuclear factor—(NF-) κB and endothelial nitric oxide synthase (Davies and Roulleau, 2012; Price et al., 2012; Kim et al., 2018; Huang et al., 2019). Meanwhile, the oxidative stress and inflammation are important pathophysiological mechanisms of various cardiovascular diseases.
Ferroptosis is a newly discovered form of non-apoptotic cell death related to metabolic dysfunction, which leads to abnormal decomposition of glutamine during metabolism process, iron-dependent accumulation of reactive oxygen species (ROS), mitochondrial superoxide, as well as decreased mitochondrial membrane potential (Dixon et al., 2012; Koppula et al., 2020). During ferroptosis, the phospholipid glutathione peroxidase 4 (GPX4) catalyzes the reduction of peroxide such as hydrogen peroxide and organic hydroperoxides, and plays critical inhibitory role (Yang et al., 2014). In addition to iron overload, depletion of glutathione (GSH) is another crucial mechanism during ferroptosis, and the accumulation of cellular GSH is regulated by SLC7A11, which transports GSH precursor cystine in the cytosol (Fang et al., 2020). Studies have revealed that ferroptosis contributes to the death of cardiomyocytes and development of MI (Song et al., 2021; Wang and Kang, 2021). Nevertheless, the molecular mechanism of ferroptosis in the pathogenesis of MI, as well as the function of Res on ferroptosis during MI is unclear.
In this work, we aimed to explore the function of Res on MI, determined that the administration of Res alleviated MI and inflammatory response both in vitro and in vivo, and inhibited ferroptosis through recruiting KAT5 to enhance GPX4 expression. Our work presented novel mechanism of Res as a therapeutic agent for MI.
Materials and Methods
Cell Culture and Treatment
Rat cardiomyocytes H9c2, was obtained from the American Type Culture Collection (ATCC, United States), cultured in DMEM (Thermo, United States) supplemented with 10% fetal bovin serum (FBS, Gibco) and 1% penicillin/streptomycin, in a 37°C humidified incubator with 5% CO2. The small interfering RNAs targeting KAT5 (siKAT5) or GPX4 (siGPX4) were synthesized by RiboBio (China), and transfected to H9c2 cells by using lipofectamine 2000 reagent (Invitrogen, United States) in line with manufacturer’s protocol. Res was purchased from Sigma (United States) and H9c2 cells were treated with Res (50 μM) according to the references (Xu et al., 2019a; Hu et al., 2021), and the same volume of PBS was used in the Mock group. Ferrostatin-1 (Fer-1) was purchased from MCE (United States) and H9c2 cells were treated with Fer-1 (2.5 μM) according to the reference (Ning et al., 2021).
Establishment of MI Rat Model
All animal experiments were authorized by the Ethics Committee of the Tangdu Hospital. The SD rats aged 8-weeks old were brought from Beijing Vital River laboratory (China). To establish MI model, the rats were administrated with left anterior descending (LAD) ligation by using 6–0 silk suture in accordance with standard protocol (Huang et al., 2020). The rats in treatment group received injection of Res (20 mg/days) surrounding the infarcted heart area. Rats in the control group received operation without ligation, and were injected with same volume of PBS instead of resveratrol. Transthoracic two-dimensional (2D) echocardiography was performed to evaluate the heart function 7 days after the operation by using a Vevo 2,100 ultrasound system. Left ventricular ejection fraction (LVEF), left ventricular fractional shortening (LVFS), left ventricle anterior wall thickness in diastole (LVAWd), and left ventricular posterior wall end diastole (LVPWd) were evaluated.
Histological Analysis
The heart tissues were collected 7 days after operation, fixed in 4% paraformaldehyde (PFA), and made into paraffin-embedded slices. The hematoxylin and eosin (H&E) staining, Masson’s trichrome staining (Thermo, United States), and Prussian blue staining were determined according to manufacturer’s protocol, followed by observation under microscope (Leica, Germany).
Oxygen-Glucose DeprivationModel
To establish in vitro MI model, H9c2 cells were placed in DMEM deprived of glucose (Thermo) and placed in a 37°C anaerobic atmosphere (1% O2 and 5% CO2) for 24 h (Du et al., 2020; Niu et al., 2020; Wu et al., 2021).
Cell Viability
The viability of H9c2 cells was determined by cell counting kit 8 (CCK-8) following the manufacturer’s description. In brief, cells were placed in 96-well plates at a density of 5,000 cells per well, and treated with Res for 48 h, followed by incubation with CCK-8 reagent for another 1.5 h. The absorbance at 450 nm was checked by a microplate detector (Thermo).
ELISA
The levels of inflammatory factors including IL-6 and IL-1β in heart tissues, serum, and culture medium of H9c2 cells were measured by ELISA assay using commercial IL-6 Rat ELISA Kit (BMS625, Invitrogen, United States) and IL-1β Rat ELISA Kit (BMS630, Invitrogen, United States) in accordance with manufacturer’s protocols.
Western Blotting
The total proteins were extracted from heart tissues, or cells by using ice-cold RIPA lysis buffer (Beyotime, China). A total of 30 μg proteins were separated by SDS-polyacrylamide gel (SDS-PAGE), shifted to polyvinylidene difluoride (PVDF) membranes (Millipore, Germany), and blocked in 5% non-fat milk, followed by incubation with specific primary anti-collagen 1 (ab254113, Abcam, United States, 1:1,000), anti-α-SMA (ab40854, Abcam, United States, 1:1,000), anti-GPX4 (ab252833, Abcam, United States, 1:1,000), anti-SLC7A11 (ab175186, Abcam, United States, 1:1,000) antibodies at 4°C overnight. Next day, the protein bands were incubated with HRP conjugated secondary anti-mouse or anti-rabbit antibodies (ab175775/ab288151, Abcam, United States, 1:1,000) at room temperature for 1 h. The bands were then visualized by using ECL (Thermo) on a gel image system (Bio-Rad, United States). All antibodies were purchased from Abcam.
Determination of Ferroptosis Biomarkers
The occurrence of ferroptosis was evaluated by levels of malondialdehyde (MDA), glutathione (GSH), lipid ROS, and iron. To determine lipid ROS, cells were labeled with C11-BODIPY (581/591) (5 µM) in dark for 30 min at 37°C, then were harvested for flow cytometry on FACs Calibur (BD Biosciences). The level of intracellular iron was measured by an Iron Assay Kit as per the manufacturer’s protocols. The MDA level was assessed by using an MDA assay kit (Sigma). To check GSH activity, cells were collected and lysed in a 5% 5-sulfosalicylic acid (SSA) solution and measured by a Reduced Glutathione Assay kit (BioVision) in line with the manufacturer’s instructions.
Quantitative RT-PCR (qRT-PCR)
The heart tissues and H9c2 cells were homogenized with Trizol reagent (Thermo) to isolate total RNA. The RNA was reverse-transcribed to cDNA by using the PrimeScript First Strand cDNA Synthesis Kit (Takara, China), followed by quantification with SYBR Green Master Mix (Takara) using the 2-ΔΔCt method. The GAPDH was adopted as internal control for normalization of KAT5 and GPX4.
The primer sequences are as follows:
Statistical Analysis
SPSS 20.0 software was adopted for statistical analysis. Data were shown as means ± standard deviation. Student’s t test or one-way analysis of variance with Dunnett’s multiple comparisons test were applied for comparison between two or multiple groups. The experiments were performed at least in triplicate.
Results
Res Relieves Myocardial Injury in MI Rats
In order to assess the impact of Res on MI-induced myocardial dysfunction, the MI rat model was constructed and was injected with Res. The treatment of Res did not affect the body weight of the rats, suggesting that there was no toxicological effect on the rats (data bot shown). We observed that the treatment of Res attenuated the MI-related myocardium injuries in the rats (Figure 1A). The myocardial fibrosis was induced in the MI rats and the treatment of Res relieved the phenotype (Figure 1B). Meanwhile, the expression of collagen one and α-SMA was induced in MI rats, in which the treatment of Res could decrease the expression (Figures 1C–E).
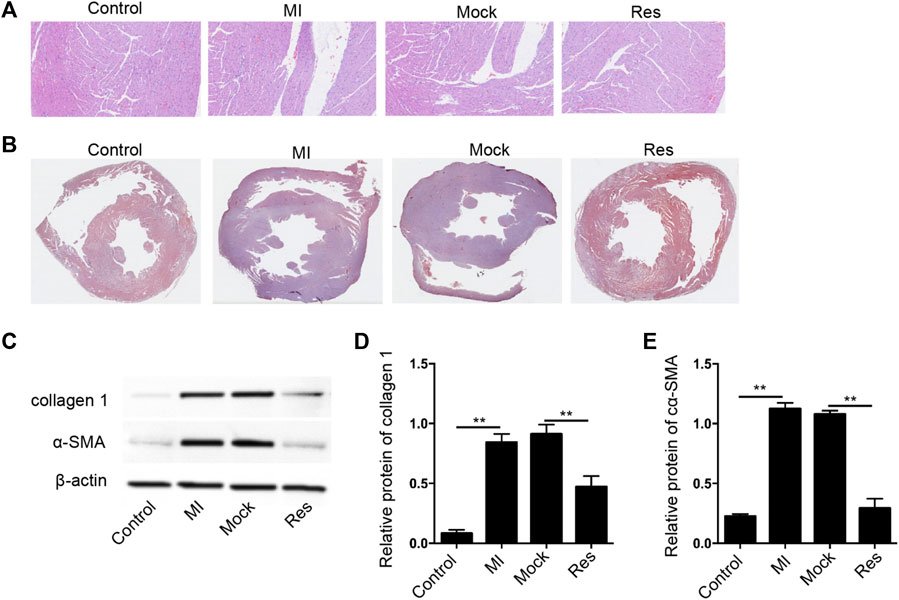
FIGURE 1. Res attenuates myocardial injury in MI rats (A–E) The MI rats were injected with Res (n = 5 for each group). (A) The myocardium injury was analyzed by Hematoxylin & Eosin (H&E) staining (B) The myocardial fibrosis was measured by Masson’s staining. (C–E) The expression of collagen 1 and α-SMA was detected by Western blot analysis and was normalized on the expression of β-actin. Data are presented as mean ± SD. Statistic significant differences were indicated: **p < 0.01.
Res Alleviates Inflammation and Ferroptosis in MI Rats
Next, we further assessed the effect of Res on inflammation and ferroptosis in MI rats. We identified that treatment of Res suppressed the levels of IL-6 and IL-1β in heart tissues and serum samples of the MI rats (Figures 2A–D). Importantly, the GSH levels were inhibited and MDA, lipid ROS, and Fe2+ levels were induced in MI rats in which the treatment of Res could reverse the phenotypes (Figures 2E–H). Meanwhile, the expression of GPX4 and SLC7A11 was reduced in MI rats, while the treatment of Res could rescue the expression in the model (Figure 2I).
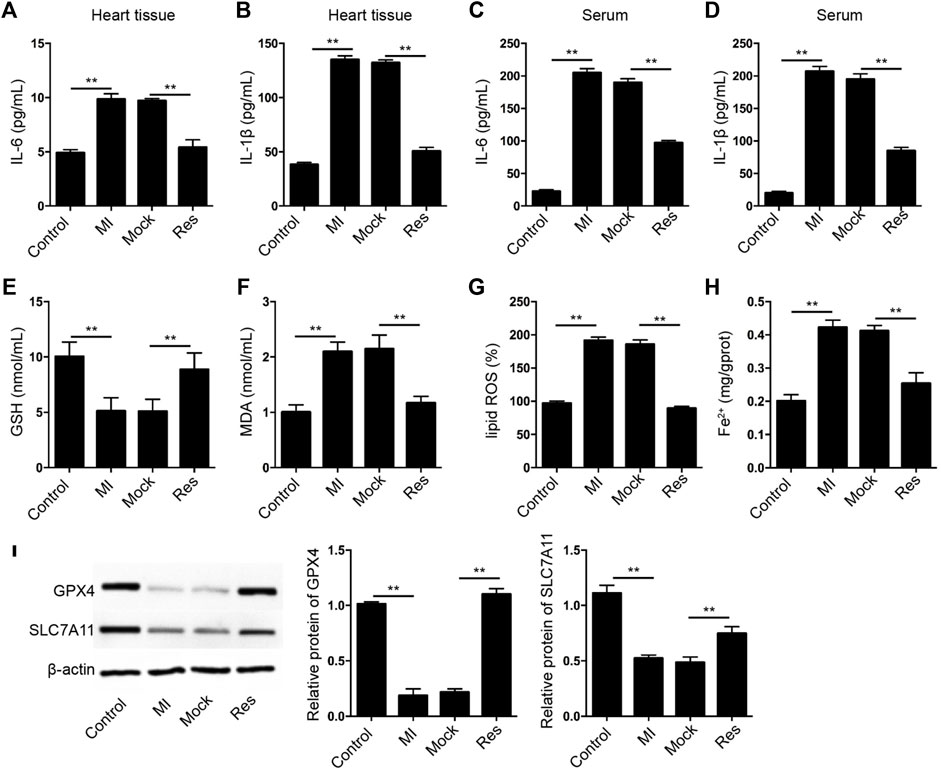
FIGURE 2. Res alleviates inflammation and ferroptosis in MI rats. (A–I) The MI rats were injected with Res (n = 5 for each group). (A–D) The levels of IL-6 and IL-1β were measured by ELISA assay in heart tissues and serum samples of the rats. The levels of GSH (E), MDA (F), lipid ROS (G), and Fe2+ (H) were analyzed. (I) The expression of GPX4 and SLC7A11 was detected by Western blot analysis and was normalized on the expression of β-actin. Data are presented as mean ± SD. Statistic significant differences were indicated: **p < 0.01.
Res Relieves OGD-Induced Cardiomyocyte Injury
Next, we further evaluated the effect of Res on OGD-induced cardiomyocyte injury in vitro. The cell viability was reduced in OGD-treated H9c2 cells but the treatment of Res rescued the phenotype in the cells (Figure 3A). The expression of collagen 1 and α-SMA was enhanced by OGD treatment, while the treatment of Res suppressed the expression in H9c2 cells (Figures 3B–D). In addition, the OGD-induced IL-6 and IL-1β levels were attenuated by the treatment of Res in H9c2 cells (Figures 3E,F).
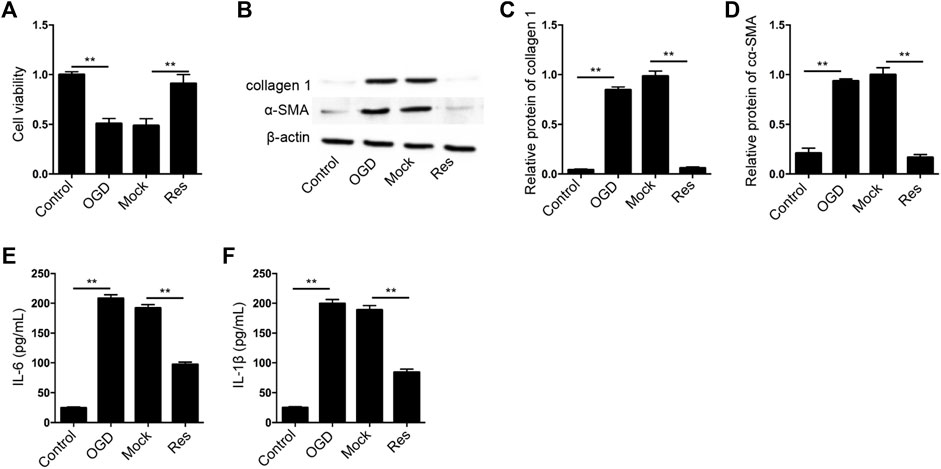
FIGURE 3. Res relieves OGD-induced cardiomyocyte injury. (A–F) The OGD-treated H9c2 cells were treated with Res. (A) The cell viability was measured by CCK-8 assay. (B–D) The expression of collagen 1 and α-SMA was tested by Western blot analysis and was normalized on the expression of β-actin. (E,F) The levels of IL-6 and IL-1β were detected by ELISA. Data are presented as mean ± SD. Statistic significant differences were indicated: **p < 0.01.
Res Represses OGD-Induced Cardiomyocyte Ferroptosis
Then, we further evaluated the effect of Res on the ferroptosis of cardiomyocytes. The GSH levels were decreased and MDA, lipid ROS, and Fe2+ levels were increased in OGD-treated H9c2 cells, in which the treatment of Res was able to reverse the phenotypes (Figures 4A–D). Besides, the expression of GPX4 and SLC7A11 was repressed in OGD-treated H9c2 cells, while the treatment of Res enhanced the expression in the cells (Figure 4E).
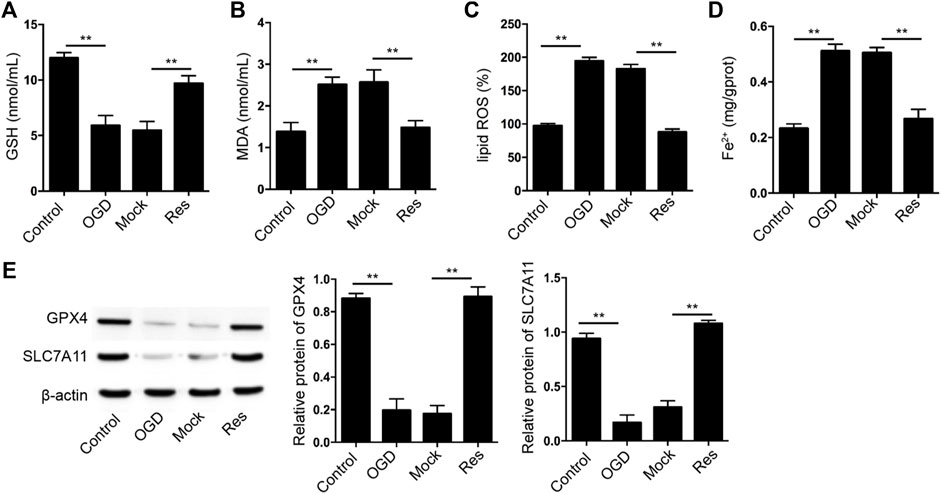
FIGURE 4. Res represses OGD-induced cardiomyocyte ferroptosis. (A–E) The OGD-treated H9c2 cells were treated with Res. The levels of GSH (A), MDA (B), lipid ROS (C), and Fe2+ (D) were analyzed. (E) The expression of GPX4 and SLC7A11 was detected by Western blot analysis and was normalized on the expression of β-actin. Data are presented as mean ± SD. Statistic significant differences were indicated: **p < 0.01.
Res Enhances GPX4 Expression by Inducing KAT5 in Cardiomyocytes
Next, we tried to explore the mechanism of Res protection against cardiomyocyte injury. We identified that the treatment of Res was able to induce KAT5 expression in H9c2 cells (Figure 5A). Meanwhile, the treatment of Res enhanced GPX4 expression, but the depletion of KAT5 could reverse the effect of Res in H9c2 cells (Figure 5B). We then verified the effect of KAT5 on OGD-induced cardiomyocyte injury in vitro. The cell viability was reduced in OGD-treated H9c2 cells but the overexpression of KAT5 rescued the phenotype in the cells (Figure 5C). The expression of collagen 1 and α-SMA was enhanced by OGD treatment, while the overexpression of KAT5 suppressed the expression in H9c2 cells (Figure 5D). Besides, the OGD-induced IL-6 and IL-1β levels were attenuated by the overexpression of KAT5 in H9c2 cells (Figures 5E,F).
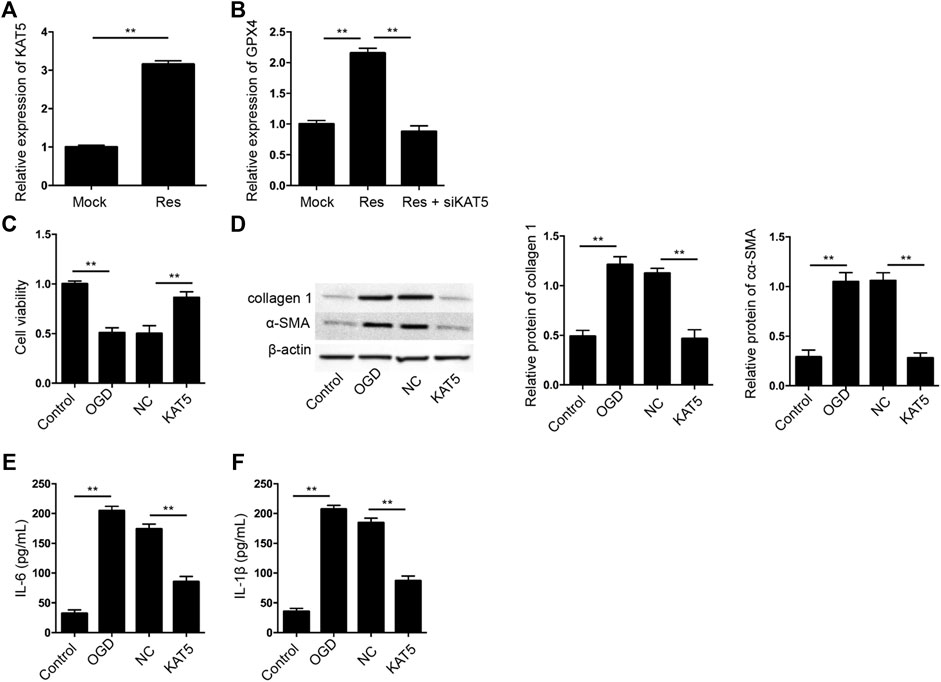
FIGURE 5. Res enhances GPX4 expression by inducing KAT5 in cardiomyocytes. (A) The expression of KAT5 was measured by qPCR in H9c2 cells treated with Res. (B) The expression of GPX4 was determined by qPCR in H9c2 cells treated with Res and KAT5 siRNA. (C–F) The OGD-treated H9c2 cells were treated with KAT5 overexpressing plasmid. (C) The cell viability was measured by CCK-8 assay. (D) The expression of collagen 1 and α-SMA was tested by Western blot analysis and was normalized on the expression of β-actin. (E,F) The levels of IL-6 and IL-1β were detected by ELISA. Control, H9c2 without OGD treatment; NC, empty plasmid. Data are presented as mean ± SD. Statistic significant differences were indicated: **p < 0.01.
KAT5 Reduces OGD-Induced Cardiomyocyte Ferroptosis
Next, we further assessed the effect of KAT5 on the ferroptosis of cardiomyocytes. The GSH levels were reduced and MDA, lipid ROS, and Fe2+ levels were enhanced in OGD-treated H9c2 cells, in which the overexpression of KAT5 was able to reverse the phenotypes (Figures 6A–D). In addition, the expression of GPX4 and SLC7A11 was suppressed in OGD-treated H9c2 cells, while the overexpression of KAT5 rescued the expression in the cells (Figure 6E).
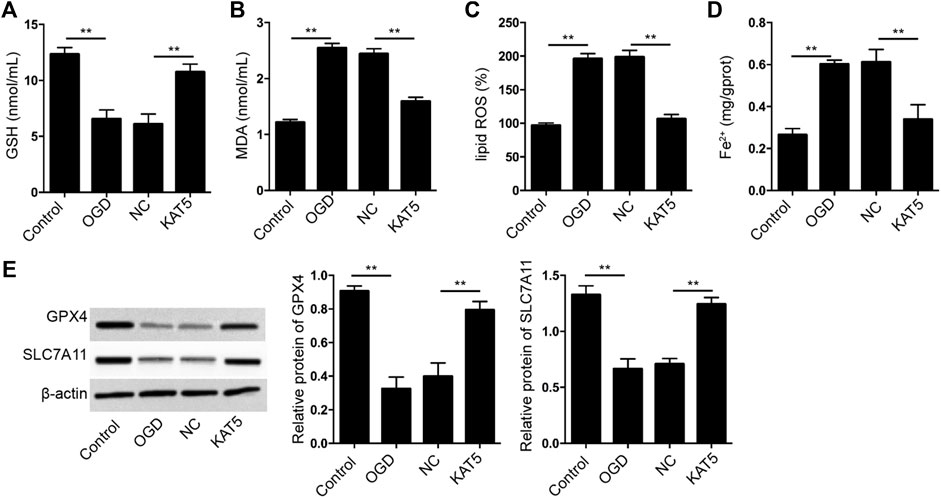
FIGURE 6. KAT5 reduces OGD-induced cardiomyocyte ferroptosis. (A–E) The OGD-treated H9c2 cells were treated with KAT5 overexpressing plasmid. The levels of GSH (A), MDA (B), lipid ROS (C), and Fe2+ (D) were analyzed. (E) The expression of GPX4 and SLC7A11 was detected by Western blot analysis and was normalized on the expression of β-actin. Data are presented as mean ± SD. Statistic significant differences were indicated: **p < 0.01.
Res Relieves OGD-Induced Cardiomyocyte Injury by KAT5/GPX4 Signaling
Next, we analyzed the correlation of Res/KAT5/GPX4 axis with OGD-induced cardiomyocyte injury in vitro. The cell viability was induced by the treatment of Res in OGD-treated H9c2 cells, in which the depletion of KAT5 or GPX4 could attenuate the phenotype (Figure 7A). The expression of collagen 1 and α-SMA was reduced by Res in OGD-treated H9c2 cells, but the knockdown of KAT5 or GPX4 rescued the expression (Figures 7B–D). In addition, the levels of IL-6 and IL-1β were inhibited by Res, while the silencing of KAT5 or GPX4 could reverse the effect in OGD-treated H9c2 cells (Figures 7E,F).
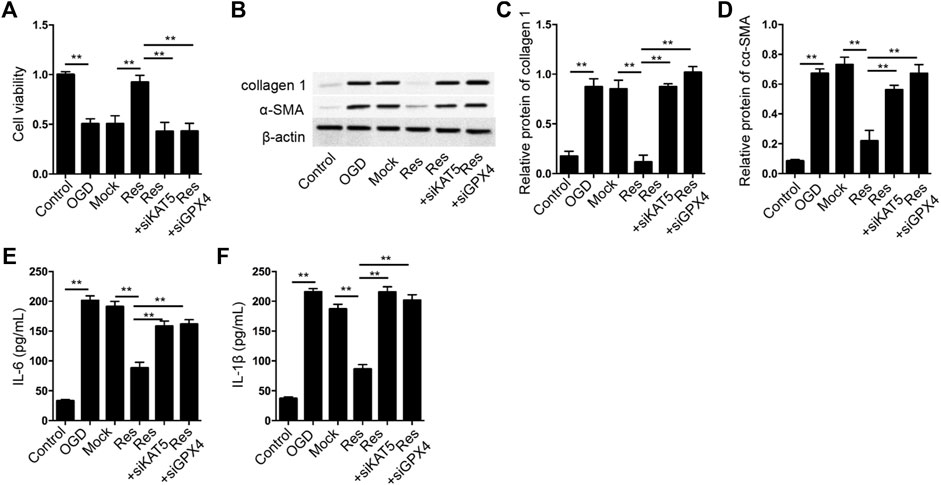
FIGURE 7. Res relieves OGD-induced cardiomyocyte injury by KAT5/GPX4 signaling. (A–F) The OGD-treated H9c2 cells were treated with Res, or co-treated with GPX4 or SLC7A11 siRNA. (A) The cell viability was measured by CCK-8 assay. (B–D) The expression of collagen 1 and α-SMA was tested by Western blot analysis and was normalized on the expression of β-actin. (E,F) The levels of IL-6 and IL-1β were detected by ELISA. Data are presented as mean ± SD. Statistic significant differences were indicated: **p < 0.01.
Moreover, we validated that the ferroptosis inhibitor Fer-1 was able to inhibit OGD-induced H9c2 cell injury, inflammation, and ferroptosis, while the depletion of KAT5 or GPX4 could reverse the effect of Fer-1 in the model (Figure S1).
Discussion
MI serves as a coronary artery-related disease and is a leading cause of sudden death globally. Res is a bioactive component and has presented antioxidant, anti-inflammatory and anti-microbial properties. Nevertheless, the impact of Res on ferroptosis in MI progression is still unclear. In the present study, we discovered the effect of Res on ferroptosis and myocardial injury in MI.
The function of Res in myocardial injury has been reported in several studies. It has been reported that Res attenuates ischemia-reperfusion injury by enhancing autophagy in diabetic myocardium (Qu et al., 2019). Res relieves isoproterenol-induced MI by AMPK/eNOS/NO signaling in rats (Feng et al., 2019). Res attenuates CIH-induced myocardial injury through regulating Nrf2 expression and the activation of NLRP3 inflammasome (Sun et al., 2020). Res induces myocardial Nrf2 expression to attenuate myocardial ischemia/reperfusion injury in type 2 diabetic rats (Xu et al., 2019b). Res suppresses ischemia-induced myocardial senescence by targeting the activation of NLRP3 inflammasome (Feng et al., 2020). Ferroptosis plays crucial role in MI progression and has been identified as a therapeutic target in MI. Icariin represses hypoxia-reoxygenation-regulated ferroptosis of cardiomyocytes by targeting Nrf2/HO-1 signaling (Liu et al., 2021). Human umbilical cord blood-derived MSCs exosomes relieves myocardial injury via repressing ferroptosis in MI mice (Song et al., 2021). MiR-30d suppresses cardiomyocyte autophagy and inducing ferroptosis in MI (Tang et al., 2020). Oxidative stress plays crucial roles in the process of ferroptosis (Lillo- Moya et al., 2021). The antioxidant effects of Res contribute substantially to the health benefits and the biomedical activities of Res (Xia et al., 2017). In this study, we found that the treatment of Res attenuated the MI-related myocardium injury, inflammation, fibrosis in the rats. Meanwhile, the GSH levels were inhibited and MDA, lipid ROS, and Fe2+ levels were induced in MI rats, in which the treatment of Res could reverse the phenotypes. The expression of GPX4 and SLC7A11 was reduced in MI rats, while the treatment of Res could rescue the expression in the model. These data present a novel function of Res in MI-related cardiac dysfunction, providing valuable evidence for the potential therapeutic role of Res in MI. In addition, we administrated Res directly around the infarction, like some previous studies (Li et al., 2021a), and some studies apply the intraperitoneal injection (He et al., 2021), the effectiveness needs to be compared between these two manner in future investigations.
Meanwhile, it has been reported that KAT5 enhances cell-cycle arrest and DNA damage response in neonatal cardiomyocytes (Wang et al., 2021). The Egr-1/miR-15a-5p/GPX4 signaling modulates ferroptosis in acute MI (Fan et al., 2021). The downregulation of GPX4 induces ferroptosis of cardiomyocytes during MI progression (Park et al., 2019). Interestingly, a recent study reported that Res attenuates myocardial ischemia-reperfusion injury by targeting ferroptosis via by the mechanism of ubiquity specific peptidase 19 (USP19)-Beclin1-mediated autophagy (Li et al., 2022). Moreover, it has been reported that Ketamine inhibits proliferation and promotes ferroptosis of by targeting KAT5/GPX4 signaling in breast cancer cells (Li et al., 2021b). In our study, we identified that Res alleviated MI-induced myocardial injury by modulating ferroptosis via the mechanism of KAT5-induced epigenetic regulation of GPX4. We confirmed that KAT5 alleviated OGD-induced cardiomyocyte injury and ferroptosis. The depletion of KAT5 or GPX4 could reverse the effect of Res on OGD-induced cardiomyocyte injury. Our data suggest that Res attenuates cardiomyocyte injury by targeting KAT5/GPX4 signaling.
In summary, we concluded that Res attenuated myocardial injury by inhibiting ferroptosis via inducing KAT5/GPX4 in myocardial infarction. Our finding provides new evidence of the potential therapeutic effect of Res on MI by targeting ferroptosis.
Data Availability Statement
The raw data supporting the conclusion of this article will be made available by the authors, without undue reservation.
Ethics Statement
All animal experiments were authorized by the Ethics Committee of the Tangdu Hospital.
Author Contributions
JL and XN designed the study JL, MZ, CQ, ZW, JC, RW, and JH performed the experiements. JL and XN wrote the manuscript. JL, MZ, and CQ contributed equally to this work.
Funding
This study was supported by the National Nature Science Foundation of China (No. 81570227) and Research Program of Shaanixi Province (No.2022SF-600).
Conflict of Interest
The authors declare that the research was conducted in the absence of any commercial or financial relationships that could be construed as a potential conflict of interest.
Publisher’s Note
All claims expressed in this article are solely those of the authors and do not necessarily represent those of their affiliated organizations, or those of the publisher, the editors and the reviewers. Any product that may be evaluated in this article, or claim that may be made by its manufacturer, is not guaranteed or endorsed by the publisher.
References
Anderson, J. L., and Morrow, D. A. (2017). Acute Myocardial Infarction. N. Engl. J. Med. 376, 2053–2064. doi:10.1056/NEJMra1606915
Bezerra, O. C., França, C. M., Rocha, J. A., Neves, G. A., Souza, P. R. M., Teixeira Gomes, M., et al. (2017). Cholinergic Stimulation Improves Oxidative Stress and Inflammation in Experimental Myocardial Infarction. Sci. Rep. 7, 13687. doi:10.1038/s41598-017-14021-8
Boateng, S., and Sanborn, T. (2013). Acute Myocardial Infarction. Dis. Mon. 59, 83–96. doi:10.1016/j.disamonth.2012.12.004
Bonnefont-Rousselot, D. (2016). Resveratrol and Cardiovascular Diseases. Nutrients 8. doi:10.3390/nu8050250
Cabello, J. B., Burls, A., Emparanza, J. I., Bayliss, S. E., and Quinn, T. (2016). Oxygen Therapy for Acute Myocardial Infarction. Cochrane Database Syst. Rev. 12, CD007160. doi:10.1002/14651858.CD007160.pub4
Chaplin, A., Carpéné, C., and Mercader, J. (2018). Resveratrol, Metabolic Syndrome, and Gut Microbiota. Nutrients 10, 1651. doi:10.3390/nu10111651
Chen, W. W., Gao, R. L., Liu, L. S., Zhu, M. L., Wang, W., and Wang, Y. J. (2017). China Cardiovascular Diseases Report 2015: a Summary. J. Geriatr. Cardiol. 14, 1–10. doi:10.11909/j.issn.1671-5411.2017.01.012
Davies, M., and Roulleau, J. (2012). Statement of Retraction. Cardioprotective Effect of Resveratrol via HO-1 Expression Involves P38 Map Kinase and PI-3-Kinase Signaling, but Does Not Involve NFkB. Free Radic. Res. 46, 359. doi:10.3109/10715762.2012.659892
Dixon, S. J., Lemberg, K. M., Lamprecht, M. R., Skouta, R., Zaitsev, E. M., Gleason, C. E., Patel, D N, Bauer, A J, Yang, W S, and Stockwell, B R (2012). Ferroptosis: an Iron-dependent Form of Nonapoptotic Cell Death. Cell 149, 1060–1072. doi:10.1016/j.cell.2012.03.042
Dong-mei, X., Jun-hua, Z., Li-yuan, K., Ming-jun, Z., and Hong-cai, S. (2013). Traditional Chinese Medicine for Myocardial Infarction: an Overview. Int. J. Clin. Pract. 67, 1254–1260. doi:10.1111/ijcp.12172
Du, J., Lv, W., Yang, S., Liu, J., Zhen, J., and Leng, J. (2020). Glatiramer Acetate Protects against Oxygen-Glucose Deprivation/reperfusion-Induced Injury by Inhibiting Egr-1 in H9c2 Cells. Mol. Immunol. 120, 61–66. doi:10.1016/j.molimm.2020.02.003
Fan, K., Huang, W., Qi, H., Song, C., He, C., Liu, Y., Zhang, Q, Wang, L, and Sun, H (2021). The Egr-1/miR-15a-5p/GPX4 axis Regulates Ferroptosis in Acute Myocardial Infarction. Eur. J. Pharmacol. 909, 174403. doi:10.1016/j.ejphar.2021.174403
Fang, X., Cai, Z., Wang, H., Han, D., Cheng, Q., Zhang, P., Gao, F, Yu, Y, Song, Z, and Wu, Q (2020). Loss of Cardiac Ferritin H Facilitates Cardiomyopathy via Slc7a11-Mediated Ferroptosis. Circ. Res. 127, 486–501. doi:10.1161/CIRCRESAHA.120.316509
Feng, H., Mou, S. Q., Li, W. J., Zhang, N., Zhou, Z. Y., Ding, W., Bian, Z Y, and Liao, H H (2020). Resveratrol Inhibits Ischemia-Induced Myocardial Senescence Signals and NLRP3 Inflammasome Activation. Oxid. Med. Cell Longev. 2020, 2647807–20. doi:10.1155/2020/2647807
Feng, L., Ren, J., Li, Y., Yang, G., Kang, L., Zhang, S., Ma, C, Li, J, Liu, J, Yang, L, and Qi, Z (2019). Resveratrol Protects against Isoproterenol Induced Myocardial Infarction in Rats through VEGF-B/AMPK/eNOS/NO Signalling Pathway. Free Radic. Res. 53, 82–93. doi:10.1080/10715762.2018.1554901
Gulati, R., Behfar, A., Narula, J., Kanwar, A., Lerman, A., Cooper, L., and Singh, M (2020). Acute Myocardial Infarction in Young Individuals. Mayo Clin. Proc. 95, 136–156. doi:10.1016/j.mayocp.2019.05.001
He, Y., Lu, X., Chen, T., Yang, Y., Zheng, J., Chen, C., Zhang, Y, and Lei, W (2021). Resveratrol Protects against Myocardial Ischemic Injury via the Inhibition of NFkappaBdependent Inflammation and the Enhancement of Antioxidant Defenses. Int. J. Mol. Med. 47, 29. doi:10.3892/ijmm.2021.4862
Hu, Y., Pan, H., Peng, J., He, J., Tang, M., Yan, S., Rong, J, Li, J, Zheng, Z, Wang, H, Liu, Y, and Zhong, X (2021). Resveratrol Inhibits Necroptosis by Mediating the TNF-Α/rip1/rip3/MLKL Pathway in Myocardial Hypoxia/reoxygenation Injury. Acta Biochim. Biophys. Sin. (Shanghai) 53, 430–437. doi:10.1093/abbs/gmab012
Huang, P., Wang, L., Li, Q., Tian, X., Xu, J., Xu, J., Xiong, Y, Chen, G, Qian, H, Jin, C, Yu, Y, Cheng, K, Qian, L, and Yang, Y (2020). Atorvastatin Enhances the Therapeutic Efficacy of Mesenchymal Stem Cells-Derived Exosomes in Acute Myocardial Infarction via Up-Regulating Long Non-coding RNA H19. Cardiovasc Res. 116, 353–367. doi:10.1093/cvr/cvz139
Huang, Y., Zhu, X., Chen, K., Lang, H., Zhang, Y., Hou, P., Ran, L, Zhou, M, Zheng, J, Yi, L, Mi, M, and Zhang, Q (2019). Resveratrol Prevents Sarcopenic Obesity by Reversing Mitochondrial Dysfunction and Oxidative Stress via the PKA/LKB1/AMPK Pathway. Aging (Albany NY) 11, 2217–2240. doi:10.18632/aging.101910
Kim, E. N., Lim, J. H., Kim, M. Y., Ban, T. H., Jang, I. A., Yoon, H. E., Park, C W, Chang, Y S, and Choi, B S (2018). Resveratrol, an Nrf2 Activator, Ameliorates Aging-Related Progressive Renal Injury. Aging (Albany NY) 10, 83–99. doi:10.18632/aging.101361
Koppula, P., Zhuang, L., and Gan, B. (2020). Cystine Transporter SLC7A11/xCT in Cancer: Ferroptosis, Nutrient Dependency, and Cancer Therapy. Protein Cell 12, 599. doi:10.1007/s13238-020-00789-5
Li, H., Liu, W., Zhang, X., Wu, F., Sun, D., and Wang, Z. (2021). Ketamine Suppresses Proliferation and Induces Ferroptosis and Apoptosis of Breast Cancer Cells by Targeting KAT5/GPX4 axis. Biochem. Biophys. Res. Commun. 585, 111–116. doi:10.1016/j.bbrc.2021.11.029
Li, T., Chen, Z., Zhou, Y., Li, H., Xie, J., and Li, L. (2021). Resveratrol Pretreatment Inhibits Myocardial Apoptosis in Rats Following Coronary Microembolization via Inducing the PI3K/Akt/GSK-3β Signaling Cascade. Drug Des. Devel Ther. 15, 3821–3834. doi:10.2147/DDDT.S323555
Li, T., Tan, Y., Ouyang, S., He, J., and Liu, L. (2022). Resveratrol Protects against Myocardial Ischemia-Reperfusion Injury via Attenuating Ferroptosis. Gene 808, 145968. doi:10.1016/j.gene.2021.145968
Lillo-Moya, J., Rojas-Sole, C., Munoz-Salamanca, D., Panieri, E., Saso, L., and Rodrigo, R. (2021). Targeting Ferroptosis against Ischemia/Reperfusion Cardiac Injury. Antioxidants (Basel) 10. doi:10.3390/antiox10050667
Liu, M., Li, W., Wang, H., Yin, L., Ye, B., Tang, Y., and Huang, C (2019). CTRP9 Ameliorates Atrial Inflammation, Fibrosis, and Vulnerability to Atrial Fibrillation in Post-Myocardial Infarction Rats. J. Am. Heart Assoc. 8, e013133. doi:10.1161/JAHA.119.013133
Liu, X. J., Lv, Y. F., Cui, W. Z., Li, Y., Liu, Y., Xue, Y. T., and Dong, F (2021). Icariin Inhibits Hypoxia/reoxygenation-Induced Ferroptosis of Cardiomyocytes via Regulation of the Nrf2/HO-1 Signaling Pathway. FEBS Open Bio 11, 2966. doi:10.1002/2211-5463.13276
Lu, C. Y., Lu, P. C., and Chen, P. C. (2019). Utilization Trends in Traditional Chinese Medicine for Acute Myocardial Infarction. J. Ethnopharmacol. 241, 112010. doi:10.1016/j.jep.2019.112010
Ning, D., Yang, X., Wang, T., Jiang, Q., Yu, J., and Wang, D. (2021). Atorvastatin Treatment Ameliorates Cardiac Function and Remodeling Induced by Isoproterenol Attack through Mitigation of Ferroptosis. Biochem. Biophys. Res. Commun. 574, 39–47. doi:10.1016/j.bbrc.2021.08.017
Niu, X., Pu, S., Ling, C., Xu, J., Wang, J., Sun, S., et al. (2020). lncRNA Oip5-As1 Attenuates Myocardial Ischaemia/reperfusion Injury by Sponging miR-29a to Activate the SIRT1/AMPK/PGC1α Pathway. Cell Prolif. 53, e12818. doi:10.1111/cpr.12818
Park, T. J., Park, J. H., Lee, G. S., Lee, J. Y., Shin, J. H., Kim, M. W., et al. (2019). Quantitative Proteomic Analyses Reveal that GPX4 Downregulation during Myocardial Infarction Contributes to Ferroptosis in Cardiomyocytes. Cell Death Dis. 10, 835. doi:10.1038/s41419-019-2061-8
Price, N. L., Gomes, A. P., Ling, A. J., Duarte, F. V., Martin-Montalvo, A., and North, B. J. (2012). SIRT1 Is Required for AMPK Activation and the Beneficial Effects of Resveratrol on Mitochondrial Function. Cell Metab. 15, 675–690. doi:10.1016/j.cmet.2012.04.003
Qu, X., Chen, X., Shi, Q., Wang, X., Wang, D., and Yang, L. (2019). Resveratrol Alleviates Ischemia/reperfusion Injury of Diabetic Myocardium via Inducing Autophagy. Exp. Ther. Med. 18, 2719–2725. doi:10.3892/etm.2019.7846
Reed, G. W., Rossi, J. E., and Cannon, C. P. (2017). Acute Myocardial Infarction. Lancet 389, 197–210. doi:10.1016/S0140-6736(16)30677-8
Song, Y., Wang, B., Zhu, X., Hu, J., Sun, J., Xuan, J., and Ge, Z (2021). Human Umbilical Cord Blood-Derived MSCs Exosome Attenuate Myocardial Injury by Inhibiting Ferroptosis in Acute Myocardial Infarction Mice. Cell Biol. Toxicol. 37, 51–64. doi:10.1007/s10565-020-09530-8
Sun, Z. M., Guan, P., Luo, L. F., Qin, L. Y., Wang, N., Zhao, Y. S., and Ji, E S (2020). Resveratrol Protects against CIH-Induced Myocardial Injury by Targeting Nrf2 and Blocking NLRP3 Inflammasome Activation. Life Sci. 245, 117362. doi:10.1016/j.lfs.2020.117362
Tang, S., Wang, Y., Ma, T., Lu, S., Huang, K., Li, Q., Wu, M, Yang, H, and Zhong, J (2020). MiR-30d Inhibits Cardiomyocytes Autophagy Promoting Ferroptosis after Myocardial Infarction. Panminerva Med. doi:10.23736/s0031-0808.20.03979-8
Wang, G., Wang, R., Ruan, Z., Liu, L., Li, Y., and Zhu, L. (2020). MicroRNA-132 Attenuated Cardiac Fibrosis in Myocardial Infarction-Induced Heart Failure Rats. Biosci. Rep. 40, 40. doi:10.1042/BSR20201696
Wang, X., Lupton, C., Lauth, A., Wan, T. C., Foster, P., Patterson, M., et al. (2021). Evidence that the Acetyltransferase Tip60 Induces the DNA Damage Response and Cell-Cycle Arrest in Neonatal Cardiomyocytes. J. Mol. Cell Cardiol. 155, 88–98. doi:10.1016/j.yjmcc.2021.02.005
Wang, X. D., and Kang, S. (2021). Ferroptosis in Myocardial Infarction: Not a Marker but a Maker. Open Biol. 11, 200367. doi:10.1098/rsob.200367
Wu, S., Zhang, H., Chen, N., Zhang, C., and Guo, X. (2021). Metformin Protects Cardiomyocytes against Oxygen-Glucose Deprivation Injury by Promoting Autophagic Flux through AMPK Pathway. J. Drug Target 29, 551–561. doi:10.1080/1061186X.2020.1868478
Xia, N., Daiber, A., Förstermann, U., and Li, H. (2017). Antioxidant Effects of Resveratrol in the Cardiovascular System. Br. J. Pharmacol. 174, 1633–1646. doi:10.1111/bph.13492
Xu, G., Zhao, X., Fu, J., and Wang, X. (2019). Resveratrol Increase Myocardial Nrf2 Expression in Type 2 Diabetic Rats and Alleviate Myocardial Ischemia/reperfusion Injury (MIRI). Ann. Palliat. Med. 8, 565–575. doi:10.21037/apm.2019.11.25
Xu, R. Y., Xu, X. W., Deng, Y. Z., Ma, Z. X., Li, X. R., Zhao, L., Qiu, L J, Liu, H. Y, and Chen, H. P (2019). Resveratrol Attenuates Myocardial Hypoxia/reoxygenation-Induced Cell Apoptosis through DJ-1-Mediated SIRT1-P53 Pathway. Biochem. Biophys. Res. Commun. 514, 401–406. doi:10.1016/j.bbrc.2019.04.165
Keywords: myocardial infarction, myocardial injury, ferroptosis, resveratrol, KAT5, GPX4
Citation: Liu J, Zhang M, Qin C, Wang Z, Chen J, Wang R, Hu J, Zou Q and Niu X (2022) Resveratrol Attenuate Myocardial Injury by Inhibiting Ferroptosis Via Inducing KAT5/GPX4 in Myocardial Infarction. Front. Pharmacol. 13:906073. doi: 10.3389/fphar.2022.906073
Received: 28 March 2022; Accepted: 27 April 2022;
Published: 24 May 2022.
Edited by:
Vincenzo Calderone, University of Pisa, ItalyReviewed by:
Valentina Citi, University of Pisa, ItalyEleonora Turrini, University of Bologna, Italy
Copyright © 2022 Liu, Zhang, Qin, Wang, Chen, Wang, Hu, Zou and Niu. This is an open-access article distributed under the terms of the Creative Commons Attribution License (CC BY). The use, distribution or reproduction in other forums is permitted, provided the original author(s) and the copyright owner(s) are credited and that the original publication in this journal is cited, in accordance with accepted academic practice. No use, distribution or reproduction is permitted which does not comply with these terms.
*Correspondence: Xiaolin Niu, xiaolinniu151@163.com