- Department of Pharmaceutical Biology, Institute of Pharmacy, Kiel University, Kiel, Germany
Momordica charantia L. (Cucurbitaceae), commonly known as bitter gourd or bitter melon, is widely cultivated in many tropical and subtropical regions of the world, where its unripe fruits are eaten as a vegetable. Apart from its culinary use, M. charantia has a long history in traditional medicine, serving as stomachic, laxative or anthelmintic, and, most notably, for the treatment of diabetes and its complications. Its antidiabetic properties and its beneficial effects on blood glucose and lipid concentrations have been reported in numerous in vitro and in vivo studies, but the compounds responsible for the observed effects have not yet been adequately described. Early reports were made for charantin, a mixture of two sterol glucosides, and the polypeptide p-insulin, but their low concentrations in the fruits or their limited bioavailability cannot explain the observed therapeutic effects. Still, for many decades the search for more reasonable active principles was omitted. However, in the last years, research more and more focused on the particular cucurbitane-type triterpenoids abundant in the fruits and other parts of the plant. This mini review deals with compounds isolated from the bitter gourd and discusses their bioactivities in conjunction with eventual antidiabetic or adverse effects. Furthermore, methods for the quality control of bitter gourd fruits and preparations will be evaluated for their meaningfulness and their potential use in the standardization of commercial preparations.
Introduction
Diabetes is one of the major health challenges of the 21st century. Currently an estimated 537 million adults suffer from diabetes, a number that is expected to rise by another 100 million until the end of this decade (International Diabetes Federation, 2021). With three out of four adults with diabetes living in low- or middle-income countries, their access to conventional antidiabetic drugs is restricted (International Diabetes Federation, 2021; Liu et al., 2021). Therefore, alternative remedies for the treatment of diabetes are of great importance.
One such remedy is Momordica charantia L. (Cucurbitaceae), an herbaceous vine cultivated throughout the tropics, where it is referred to as balsam pear, cundeamor (South America), karela (India), or goo-fah (Jamaica) (Walters and Decker-Walters, 1988; Raman and Lau, 1996). However, the two most common names are bitter gourd and bitter melon, indicating the consumption of its (unripe) fruits as a vegetable (Tan et al., 2016). Moreover, M. charantia has been used since ancient times in Traditional Chinese Medicine for treating high blood sugar and early signs of diabetes (Yin et al., 2008). The most popular ethnomedicinal preparations of the bitter gourd are karela juice, which is obtained by crushing and straining the unripe fruits, and cerasee, a decoction of the aerial parts of the plant (Bailey et al., 1986). Nowadays, capsules and tablets containing powdered drug or extracts are marketed as dietary supplements and can be purchased over-the-counter and from internet suppliers (Ma et al., 2012).
Due to its long traditional usage, M. charantia was subjected to several studies in humans, of which only few fulfilled the criteria of a randomized controlled trial (Leung et al., 2009). This shortcoming and the high bias associated with these studies as well as their overall low quality was emphasized in two meta-analysis of four RCTs, which revealed no significant difference in the glycemic control of bitter gourd treatment compared to placebo or untreated group, respectively (Ooi et al., 2012; Yin et al., 2014). In contrast, a systematic review of ten studies (including also non-randomized clinical trials) found a significant reduction of fasting blood glucose, post prandial glucose and HBA1c in type 2 diabetic patients, however, with low quality evidence (Peter et al., 2019). All reviews conclude that further studies are warranted before bitter gourd preparations can be recommended for clinical practice.
Apart from clinical trials, the hypoglycemic effect of bitter gourd juice, fresh and dried fruit, or extracts was evaluated in repeated animal studies, reporting hypoglycemic effects for the juice as well as for water, ethanol, and acetone extracts (Singh et al., 1989; Basch et al., 2003; Krawinkel and Keding, 2006; Shetty et al., 2005). Additionally, bitter gourd was found less effective on blood glucose levels when applied as dried fruit powder (Ahmed et al., 2004), which is of interest because most clinical studies were conducted with capsules or tablets containing fruit powder rather than extracts (Efird et al., 2014). This might explain the lower outcome compared to animal studies as well as the missing standardization of the used preparations, with only two studies reporting contents of charantin (Fuangchan et al., 2011; Zänker et al., 2012). The lack of standardization was also noticed in the abovementioned meta-analysis, which demanded an increased focus on the quality control of bitter gourd preparations (Ooi et al., 2012; Peter et al., 2019).
The standardization of extracts depends on suitable marker compounds, which ideally represent the bioactive ingredient(s). In the case of M. charantia, early reports mentioned three active principles, namely charantin (Lotlikar and Rajarama Rao, 1966), p-insulin (Khanna et al., 1981), and vicine (Handa et al., 1990), which meanwhile are regarded controversially (Raman and Lau, 1996). Still, even recent studies and articles consider these compounds important antidiabetic agents (Desai et al., 2021; Pahlavani et al., 2019) though in the last years there has been growing evidence that the particular compound class of cucurbitanoids are responsible for the potential antidiabetic effects (Jia et al., 2017; Saeed et al., 2018; Sun et al., 2021).
In the following, potentially antidiabetic compounds isolated from M. charantia are summarized and discussed for their role in treating diabetes and for their use in the standardization of commercial preparations.
Compounds of the Bitter Gourd and Diabetes-Related Activities
Compounds With Unspecified Hypoglycemic Effects
Like many other plant species of the Cucurbitaceae family, the bitter gourd contains various cucurbitane-type triterpenoids (Figure 1), with about 200 reported structures so far (Sun et al., 2021). These compounds are generally referred to as momordicosides, but also other compound names can be found, such as karavilosides, kuguacins, or goyaglycosides.
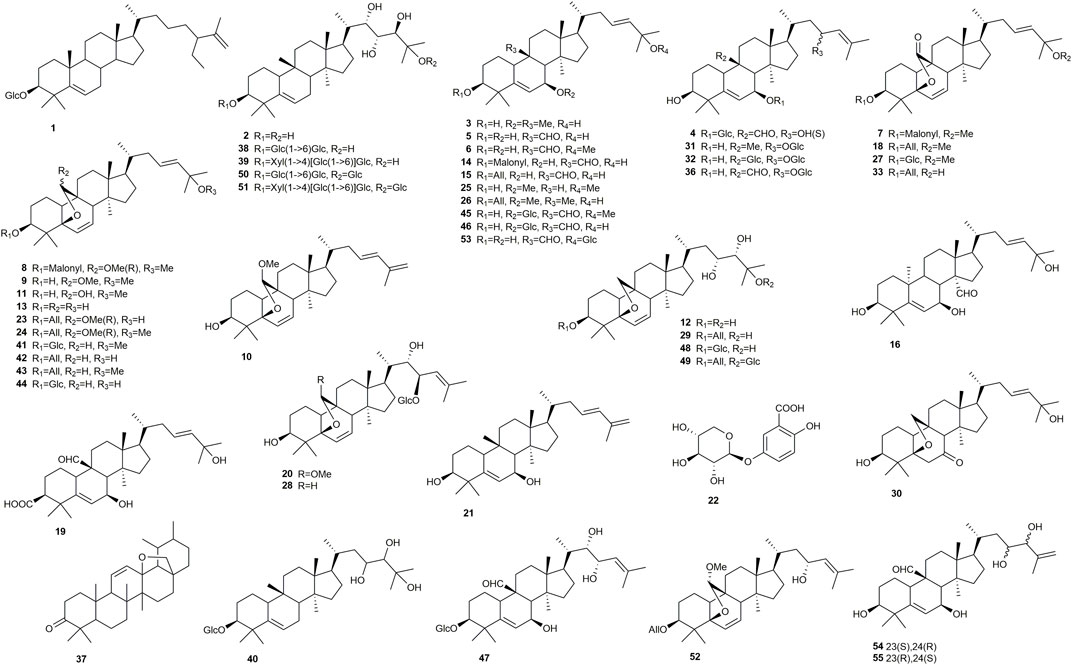
FIGURE 1. Chemical structures of compounds isolated from Momordica charantia with activity on targets relevant for diabetes. All means β-D-allopyranoside, Glc means β-D-glucopyranoside.
Nevertheless, the first report of a possible antidiabetic principle mentioned a mixture of the two phytosterol glycosides β-sitosterol 3-O-β-D-glucoside and 5,22-stigmasterol 3-O-β-D-glucoside, which was named charantin (Lotlikar and Rajarama Rao, 1966). Charantin elicited a hypoglycemic response in rabbits, however, at doses equivalent to 180–315 g of fruit. The same accounts for the pyrimidine nucleoside vicine, which was isolated from the seeds (Dutta et al., 1981; Barron et al., 1982). Here, administration of a dose equivalent to 16 g of seeds per kg body weight caused hypoglycemia in rats (Handa et al., 1990).
Khanna et al. (1981) described p-insulin, an 11 kDa polypeptide similar to bovine insulin, as possible antidiabetic principle. p-Insulin showed blood glucose lowering effects in patients with juvenile and maturity onset diabetes after subcutaneous administration. Another large molecule with reported antidiabetic activity is M. charantia polysaccharide IIa (MCPIIa, 35) (Zhang et al., 2018). The 13 kDa polysaccharide, which is mainly composed of arabinofuranose, glucuronic acid, and xylopyranosyl residues, lowered blood glucose at doses from 100 mg/kg in STZ-induced diabetic mice (Table 1).
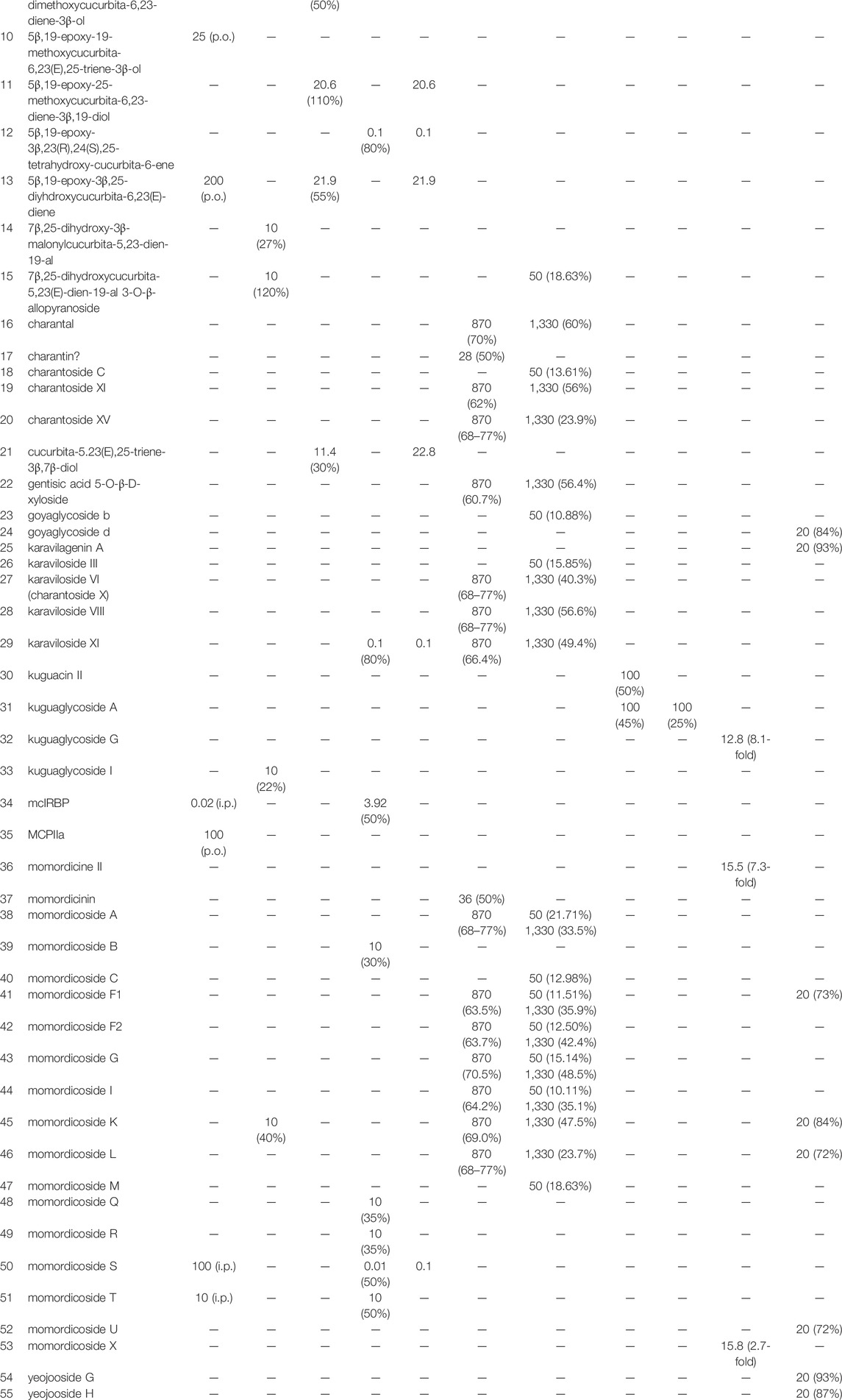
TABLE 1. Summary of reported in vitro and in vivo effects. All concentrations are given in µM, except for the measurements of blood glucose, where the administered dose is given in mg/kg body weight. Amount of stimulation or inhibition, respectively, is given in parenthesis.
It was 40 years after the first report of charantin, when Harinantenaina et al. (2006) reported blood glucose-lowering effects for two cucurbitane-type triterpenoids (5, 13) in alloxan-induced diabetic mice. Both compounds were obtained from the ether-soluble fraction of a methanol extract and were significantly active upon oral administration of 200 mg/kg. In the same study β-sitosterol and 5,22-stigmasterol, the two aglycones of charantin, exhibited no effect. Jiang et al. (2016) demonstrated blood glucose and triglyceride lowering effects for another cucurbitane-type triterpenoid (10), which was administered at a dose of 25 mg/kg by oral gavage over a period of 4 weeks.
Compounds Enhancing Glucose Uptake
Tan et al. (2008) reported eight cucurbitane-type triterpenoids (2, 12, 29, 39, 48–51) with enhanced GLUT4-translocation in L6 myotubes and 3T3-L1 adipocyte cells. Karaviloside XI (29) and momordicoside S (50) as well as their agylcones (2, 12) were the most active components, with the observed effects starting at a concentration of 0.1 nM and reaching their maximum between 10 and 100 nM. The study furthermore revealed that GLUT4-translocation was mediated via AMPK phosphorylation and not via the PI3K/Akt pathway. Momordicoside S (50) and momordicoside T (51) were further studied for their hypoglycemic effects in vivo. At doses of 100 mg/kg (50) and 10 mg/kg 51) both compounds showed a significantly higher glucose clearance than the traditional AMPK agonist AICAR. In addition, momordicoside T (51) lowered blood glucose levels in insulin-resistant high-fat-fed mice.
Cheng et al. (2008) investigated the effect of extracts and fractions from the stems of M. charantia in hepatic FL83B cells leading to the isolation of three triterpenoids (3, 5, 21). At a dose of 5 μg/ml, all three compounds enhanced glucose uptake comparably to insulin-treated cells. The study, moreover, showed that the effect was mediated via AMPK and that the compounds reduced insulin resistance in cells. AMPK was also the target of a study by Chang et al. (2011), who identified three effective triterpenoids (9, 11, 13). While the activity of compounds 9 and 13 was close to that of the control troglitazone, the effect of 11 was even greater.
Hsiao et al. (2013) investigated 15 cucurbitane type triterpenoids from bitter gourd fruits in C2C12 myoblasts. At a concentration of 10 μM, two components (15 and 45) enhanced glucose uptake by 50% and more than 100%, respectively. The latter compound was even more effective than the positive control insulin. Han et al. (2018) investigated another four triterpenoids (7, 8, 14, 33) in C2C12 cells. At the highest concentrations, all four compounds increased glucose uptake from 22 to 27% compared to control. The effect was even more pronounced in insulin-stimulated cells, with values of 29% (7, 14), 48% (8), and 39% (33).
The most recent study was done by Lo et al. (2013), who isolated M. charantia insulin receptor-binding protein (mcIRBP, 34). The polypeptide stimulated glucose uptake into 3T3-L1 cells and significantly decreased blood glucose levels upon intraperitoneal injection (Lo et al., 2014). In order to eventually increase oral bioavailability of the newly identified peptide, mcIRBP (34) was digested with pepsin in a follow-up study (Lo et al., 2016). Thereby, a 19 amino-acid spanning residue (mcIRBP-19) was identified as blood glucose-lowering motif with even increased stimulation of glucose uptake. In a recent RCT, the effect of an aqueous bitter gourd preparation containing 0.17% mcIRBP-19 on fasting blood glucose and HbA1c levels was investigated (Yang et al., 2022). The results were of only borderline significance, however, a subgroup not responding to antidiabetic medication was affected more significantly.
Compounds Targeting Glucose Absorption
Nhiem et al. (2010) tested 14 cucurbitane glycosides for their inhibition of α-glucosidase. Eight of the isolated compounds (15, 18, 23, 26, 40, 41, 44) showed weak activity at a concentration of 50 μM, whereas momordicosides A (38) and M (47) exhibited moderate inhibitory effects. The latter two compounds were the most polar components, each possessing two glycoside moieties as well as four hydroxyl groups in position C-22, C-23, C-24, and C25. Kulkarni et al. (2021) investigated the effect of charantin (17) and momordicinin (37) on the enzyme α-amylase with IC50 values of 28 and 36 μM, respectively. However, with regard to charantin (17) the results have to be regarded with caution as the structure given in the publication shows a different molecule and no details on the identification of the compound are given.
α-Glucosidase and α-amylase were also the target of three recent studies done by the same group (Shivanadoudra et al., 2019a and, 2019b; Perera et al., 2021). Thereby, a total of 15 triterpenoids (1, 5, 16, 19, 20, 27–29, 38, 41–46) and one phenolic compound (22) were tested at (relatively high) concentrations of 1.33 and 0.87 mM, respectively. The inhibitory effects against α-glucosidase were ranging from 24 to 71% while the activity against α-amylase was determined from 61 to 79%. In both assay, compound 1 showed the strongest inhibition.
Compounds Targeting Glucose Production
Chen et al. (2015) investigated 21 cucurbitane-type triterpenoids for their effect on glucose production in hepatic H4IIE cells. At a concentration of 100 μM, four triterpenoids (5, 6, 30, 31) were inhibiting gluconeogenesis by approximately 50%. At the same concentration, 6 and 31 reduced PEPCK activity, however, by only about 25% compared to untreated control.
Compounds Stimulating Insulin Secretion
Ma et al. (2010) investigated momordicoside X (53) for its effect on insulin secretion in MIN6 β-cells and found the compound to be active at a concentration of 15.8 µM. Of note, the compound was named momordicoside U in the original publication though this compound name was used before by Nhiem et al. (2010) for another structure (52). This fact was later clarified in the respective Erratum (Planta Medica, 2012). In a follow-up study, another five cucurbitane-type triterpenoids, two aglycones and three glycosides, were evaluated in the same assay (Keller et al., 2011). The monodesmoside momordicine II (36) and the bisdesmoside kuguaglycoside G (32) were both effective at concentrations of 10 and 25 μM, respectively, while the third glycoside and the two tested aglycones did not enhance insulin secretion. Interestingly, one of the inactive components was the aglycone of momordicoside X (53).
Compounds Targeting Insulin Resistance
In a recent study, Lee et al. (2021) tested 22 cucurbitanoids for their inhibition of PTPN2, an enzyme associated with insulin resistance. At a concentration of 20 μM, nine compounds (4, 24, 25, 41, 45, 46, 52, 54, 55) showed inhibitory activity ranging from 72 to 93%. Additionally, all of the isolates were tested for their inhibition of α-glucosidase, however, with no effect compared to acarbose.
Quality Control and Standardization of Extracts
Several methods for the quality control of M. charantia fruits and preparations have been reported. Wang et al. (2008) quantified the amount of five cucurbitane-type triterpenoids (5, 38, 42, 45, 46) in three different populations by HPLC-ELSD, while Ma et al. (2012) determined compounds 5, 38, 45, 46, and momordicine I in ten commercial products by HPLC-MS/MS. Other methods were dealing with the quantitation of charantin by HPTLC (Ahamad et al., 2015) and HPLC-UV (Desai et al., 2021) or with the determination of total fatty acid, saponin, and/or phenolic content (Habicht et al., 2011; Tan et al., 2014). Mishra et al. (2022) presented an NMR-based metabolomic approach for the differentiation of pericarp and seeds from the fruit skin by the abundance of phenolic compounds, charantin, and specific amino acids. However, pericarp and seed profiles were not clearly separated. Moreover, mature fruits were used in this study and no attention was paid to cucurbitane glycosides.
As explained above, the evidence for charantin as active principle is very low, wherefore its use for the standardization of extracts must be questioned. Apart from that, there is not one or a few single components that explain the bioactivity, but a plethora of cucurbitanoids acting on different targets. Thus, the HPLC methods reported by Wang et al. (2008) and Ma et al. (2012) seem most appropriate for the quality control of bitter gourd fruits and preparations. Each of the two methods determines one bidesmoside, three monodesmosides, and one aglycone, a ratio that quite well represents the glycosylation pattern in bitter gourd fruits. At the same time, the quantitation of five components is not too complex but indeed seems necessary with regard to the varying amounts in both, plant populations and commercial products. Especially, the comparison of ten different market products revealed distinct differences in the estimated daily intake of triterpenoids, with values ranging from 17 to 6,927 µg/day (Ma et al., 2012). The calculation of the estimated daily intake might also be a valuable tool for the standardization of extracts. Here, a daily intake of e.g., 3 mg cucurbitane-type triterpenoids may be a reasonable amount, which was both the mean and median of the investigated commercial preparations.
Toxicological Properties and Safety Considerations
Early animal studies on the reproductive effects of M. charantia found a reduced fertility rate of female mice upon administration of fresh leave juice (Stepka et al., 1974). Furthermore, reduced spermatogenesis was reported in male rats and dogs after feeding of seed and fruit extracts at doses of 250 and 97–167 mg/kg, respectively (Dixit et al., 1978; Naseem et al., 1998). Additionally, uterine bleeding in pregnant rats and rabbits was induced with 6 ml/kg fresh fruit juice (Raman et Lau, 1993). Hsu et al. (2011) investigated a potential estrogenic effect for the reduced fertility rate by testing six isolated triterpenoids in an estradiol transactivation assay. Four of the tested compounds showed partial agonistic and/or antagonistic activity via α- and β-estrogen receptors. A more recent study investigated the effect of M. charantia fruit and seed extracts on developmental toxicity in zebra fish embryos (Khan et al., 2019). Thereby, a crude seed extract was lethal with LD50 values of 50 μg/ml, whereas the fruit extract did not result in any lethality up to 200 μg/ml. However, at a concentration of 50 μg/ml, cardiac toxicity for the embryo was observed, which was also much more pronounced for the seed extract (5 μg/ml).
Studies on the toxicity in humans did not results in serious adverse effects. However, two reports observed hypoglycemic coma of two children aged three and four after drinking a tee of the leaves and vines before food consumption (Raman and Lau, 1996). With regard to toxic constituents, a mildly toxic lectine was reported in the seeds and outer rind or the fruits (Marles and Farnsworth, 1997). Moreover, the alkaloid vicine in the seeds causes favism in people suffering from glucose-6-phosphate dehydrogenase deficiency (Mager et al., 1965). Therefore, decoctions of the seeds and other parts of the plant should be regarded with caution. The most common traditional mode of application is the juice of the unripe fruits, for which no serious adverse events have been reported. Moreover, the fruits are regularly consumed in Asian cuisine. Nevertheless, some studies reported uterine bleeding in pregnant animals and the estrogenic activity of four bitter gourd triterpenoids was confirmed. Therefore, like most other drugs, also bitter gourd preparations should be omitted during pregnancy.
Conclusions and Outlook
The long traditional use and the mainly positive results from in vivo studies suggest beneficial effects for bitter gourd preparations in the treatment of diabetes. Continuous studies on cucurbitane-type triterpenoids point towards this compound class as active principle, with several of the investigated compounds exhibiting pronounced effects in glucose uptake assays at nanomolar (2, 12, 29, 39) to low micromolar (15, 45) concentrations. Furthermore, some of the tested compounds (3, 5, 9, 11, 13, 21) showed insulin-sensitizing effects comparable to thiazolidinediones as well as enhanced insulin secretion (32, 36, 53). Moreover, a recent study found several compounds to target insulin resistance via protein tyrosine phosphatase inhibitory activity. Apart from their abundance in bitter gourd fruits, triterpenoids (and especially their glycosylated forms) tend to dissolve in many solvents commonly used for the preparation of extracts, e.g., acetone, ethanol, and water, or mixtures thereof. This may explain the activity of different types of extracts and furthermore corroborates the cucurbitane-type triterpenoids most significantly contributing to the antidiabetic effect. Still, most clinical trials did not reflect the antidiabetic effect, which may be due to the use of fruit powder rather than extracts in these studies and the varying cucurbitanoid contents of commercial products. In addition, missing standardization of the used preparations or standardization with an inadequate maker might be an explanation for the inconsistent pharmacological results. Thus, future studies should apply formulations (preferably made of extracts) with a defined amount of daily intake. Considering the safety of bitter gourd preparations, the use of fruits free from seeds might reduce the risk of adverse events.
Author Contributions
The author confirms being the sole contributor of this work and has approved it for publication.
Funding
The author acknowledges financial support by the DFG within the funding program “Open Access-Publikationskosten”.
Conflict of Interest
The author declares that the research was conducted in the absence of any commercial or financial relationships that could be construed as a potential conflict of interest.
Publisher’s Note
All claims expressed in this article are solely those of the authors and do not necessarily represent those of their affiliated organizations, or those of the publisher, the editors and the reviewers. Any product that may be evaluated in this article, or claim that may be made by its manufacturer, is not guaranteed or endorsed by the publisher.
References
Ahamad, J., Amin, S., and Mir, S. R. (2015). Simultaneous Quantification of Gymnemic Acid as Gymnemagenin and Charantin as β-Sitosterol Using Validated HPTLC Densitometric Method. J. Chromatogr. Sci. 53, 1203–1209. doi:10.1093/chromsci/bmu166
Ahmed, I., Adeghate, E., Cummings, E., Sharma, A. K., and Singh, J. (2004). Beneficial Effects and Mechanism of Action of Momordica Charantia Juice in the Treatment of Streptozotocin-Induced Diabetes Mellitus in Rat. Mol. Cell. Biochem. 261, 63–70. doi:10.1023/b:mcbi.0000028738.95518.90
Bailey, C. J., Day, C., and Leatherdale, B. A. (1986). Traditional Treatments for Diabetes from Asia and the West Indies. Pract. Diab. Int. 3, 190–192. doi:10.1002/pdi.1960030406
Barron, D., Kaouadji, M., and Mariotte, A. (1982). Etude Comparative de Deux Cucurbitacees a Usage Medicinal. Planta Med. 46, 184–186. doi:10.1055/s-2007-971211
Basch, E., Gabardi, S., and Ulbricht, C. (2003). Bitter Melon (Momordica Charantia): A Review of Efficacy and Safety. Am. J. Health Syst. Pharm. 60, 356–359. doi:10.1093/ajhp/60.4.356
Chang, C.-I., Tseng, H.-I., Liao, Y.-W., Yen, C.-H., Chen, T.-M., Lin, C.-C., et al. (2011). In Vivo and In Vitro Studies to Identify the Hypoglycaemic Constituents of Momordica Charantia Wild Variant WB24. Food Chem. 125, 521–528. doi:10.1016/j.foodchem.2010.09.043
Chen, J. C., Lau, C. B., Chan, J. Y., Fung, K. P., Leung, P. C., Liu, J. Q., et al. (2015). The Antigluconeogenic Activity of Cucurbitacins from Momordica Charantia. Planta Med. 81, 327–332. doi:10.1055/s-0035-1545695
Cheng, H. L., Huang, H. K., Chang, C. I., Tsai, C. P., and Chou, C. H. (2008). A Cell-Based Screening Identifies Compounds from the Stem of Momordica Charantia that Overcome Insulin Resistance and Activate AMP-Activated Protein Kinase. J. Agric. Food Chem. 56, 6835–6843. doi:10.1021/jf800801k
Desai, S., Tatke, P., Mane, T., and Gabhe, S. (2021). Isolation, Characterization and Quantitative HPLC-DAD Analysis of Components of Charantin from Fruits of Momordica Charantia. Food Chem. 345, 128717. doi:10.1016/j.foodchem.2020.128717
Dixit, V. P., Khanna, P., and Bhargava, S. K. (1978). Effects of Momordica Charantia L. Fruit Extract on the Testicular Function of Dog. Planta Med. 34, 280–286. doi:10.1055/s-0028-1097451
Dutta, P. K., Chakravarty, A. K., Chaudhury, U. S., and Pakrashi, S. C. (1981). Vicine, a Favism-Inducing Toxin from Momordica Charantia Linn Seeds. Indian J. Pharm. 20B, 669–671.
Efird, J. T., Choi, Y. M., Davies, S. W., Mehra, S., Anderson, E. J., and Katunga, L. A. (2014). Potential for Improved Glycemic Control with Dietary Momordica Charantia in Patients with Insulin Resistance and Pre-diabetes. Int. J. Environ. Res. Public Health 11, 2328–2345. doi:10.3390/ijerph110202328
Fuangchan, A., Sonthisombat, P., Seubnukarn, T., Chanouan, R., Chotchaisuwat, P., Sirigulsatien, V., et al. (2011). Hypoglycemic Effect of Bitter Melon Compared with Metformin in Newly Diagnosed Type 2 Diabetes Patients. J. Ethnopharmacol. 134, 422–428. doi:10.1016/j.jep.2010.12.045
Habicht, S. D., Kind, V., Rudloff, S., Borsch, C., Mueller, A. S., Pallauf, J., et al. (2011). Quantification of Antidiabetic Extracts and Compounds in Bitter Gourd Varieties. Food Chem. 126, 172–176. doi:10.1016/j.foodchem.2010.10.094
Han, J. H., Tuan, N. Q., Park, M. H., Quan, K. T., Oh, J., Heo, K. S., et al. (2018). Cucurbitane Triterpenoids from the Fruits of Momordica Charantia Improve Insulin Sensitivity and Glucose Homeostasis in Streptozotocin-Induced Diabetic Mice. Mol. Nutr. Food Res. 62, e1700769. doi:10.1002/mnfr.201700769
Handa, G., Singh, J., Sharma, M. I., Kaul, A., and Zafar, R. (1990). Hypoglycaemic Principle of Momordica Charantia Seeds. Indian J. Nat. Prod. 6, 16–19.
Harinantenaina, L., Tanaka, M., Takaoka, S., Oda, M., Mogami, O., Uchida, M., et al. (2006). Momordica Charantia Constituents and Antidiabetic Screening of the Isolated Major Compounds. Chem. Pharm. Bull. (Tokyo) 54, 1017–1021. doi:10.1248/cpb.54.1017
Hsiao, P. C., Liaw, C. C., Hwang, S. Y., Cheng, H. L., Zhang, L. J., Shen, C. C., et al. (2013). Antiproliferative and Hypoglycemic Cucurbitane-type Glycosides from the Fruits of Momordica Charantia. J. Agric. Food Chem. 61, 2979–2986. doi:10.1021/jf3041116
Hsu, C., Hsieh, C. L., Kuo, Y. H., and Huang, C. J. (2011). Isolation and Identification of Cucurbitane-type Triterpenoids with Partial Agonist/antagonist Potential for Estrogen Receptors from Momordica Charantia. J. Agric. Food Chem. 59, 4553–4561. doi:10.1021/jf200418g
International Diabetes Federation, . (2021). International Diabetes Federation. IDF Diabetes Atlas. 10th edition. Available at: www.diabetesatlas.org.
Jia, S., Shen, M., Zhang, F., and Xie, J. (2017). Recent Advances in Momordica Charantia: Functional Components and Biological Activities. Int. J. Mol. Sci. 18, 2555. doi:10.3390/ijms18122555
Jiang, B., Ji, M., Liu, W., Chen, L., Cai, Z., Zhao, Y., et al. (2016). Antidiabetic Activities of a Cucurbitane-type Triterpenoid Compound from Momordica charantia in A-lloxan-induced D-iabetic M-ice. Mol. Med. Rep. 14, 4865–4872. doi:10.3892/mmr.2016.5800
Keller, A. C., Ma, J., Kavalier, A., He, K., Brillantes, A. M., and Kennelly, E. J. (2011). Saponins from the Traditional Medicinal Plant Momordica Charantia Stimulate Insulin Secretion In Vitro. Phytomedicine 19, 32–37. doi:10.1016/j.phymed.2011.06.019
Khan, M. F., Abutaha, N., Nasr, F. A., Alqahtani, A. S., Noman, O. M., and Wadaan, M. A. M. (2019). Bitter Gourd (Momordica Charantia) Possess Developmental Toxicity as Revealed by Screening the Seeds and Fruit Extracts in Zebrafish Embryos. BMC Complement. Altern. Med. 19, 184. doi:10.1186/s12906-019-2599-0
Khanna, P., Jain, S. C., Panagariya, A., and Dixit, V. P. (1981). Hypoglycemic Activity of Polypeptide-P from a Plant Source. J. Nat. Prod. 44, 648–655. doi:10.1021/np50018a002
Krawinkel, M. B., and Keding, G. B. (2006). Bitter Gourd (Momordica Charantia): a Dietary Approach to Hyperglycemia. Nutr. Rev. 64, 331–337. doi:10.1301/nr.2006.jul.331-337
Kulkarni, P., Lohidasan, S., and Mahadik, K. (2021). Isolation, Characterisation and Investigation of In Vitro Antidiabetic and Antioxidant Activity of Phytoconstituents from Fruit of Momordica Charantia Linn. Nat. Prod. Res. 35, 1035–1037. doi:10.1080/14786419.2019.1613400
Lee, Y. H., Yoon, S. Y., Baek, J., Kim, S. J., Yu, J. S., Kang, H., et al. (2021). Metabolite Profile of Cucurbitane-type Triterpenoids of Bitter Melon (Fruit of Momordica Charantia) and Their Inhibitory Activity against Protein Tyrosine Phosphatases Relevant to Insulin Resistance. J. Agric. Food Chem. 69, 1816–1830. doi:10.1021/acs.jafc.0c06085
Leung, L., Birtwhistle, R., Kotecha, J., Hannah, S., and Cuthbertson, S. (2009). Anti-diabetic and Hypoglycaemic Effects of Momordica Charantia (Bitter Melon): a Mini Review. Br. J. Nutr. 102, 1703–1708. doi:10.1017/S0007114509992054
Liu, Z., Gong, J., Huang, W., Lu, F., and Dong, H. (2021). The Effect of Momordica Charantia in the Treatment of Diabetes Mellitus: a Review. Evid. Based Complement. Altern. Med. 2021, e3796265. doi:10.1155/2021/3796265
Lo, H. Y., Ho, T. Y., Li, C. C., Chen, J. C., Liu, J. J., and Hsiang, C. Y. (2014). A Novel Insulin Receptor-Binding Protein from Momordica Charantia Enhances Glucose Uptake and Glucose Clearance In Vitro and In Vivo through Triggering Insulin Receptor Signaling Pathway. J. Agric. Food Chem. 62, 8952–8961. doi:10.1021/jf5002099
Lo, H. Y., Ho, T. Y., Lin, C., Li, C. C., and Hsiang, C. Y. (2013). Momordica Charantia and its Novel Polypeptide Regulate Glucose Homeostasis in Mice via Binding to Insulin Receptor. J. Agric. Food Chem. 61, 2461–2468. doi:10.1021/jf3042402
Lo, H. Y., Li, C. C., Ho, T. Y., and Hsiang, C. Y. (2016). Identification of the Bioactive and Consensus Peptide Motif from Momordica Charantia Insulin Receptor-Binding Protein. Food Chem. 204, 298–305. doi:10.1016/j.foodchem.2016.02.135
Lotlikar, M. M., and Rajarama Rao, M. R. (1966). Pharmacology of a Hypoglycaemic Principle Isolated from the Fruits of Momordica Charantia Linn. Indian J. Pharm. 28, 129–133.
Ma, J., Krynitsky, A. J., Grundel, E., and Rader, J. I. (2012). Quantitative Determination of Cucurbitane-type Triterpenes and Triterpene Glycosides in Dietary Supplements Containing Bitter Melon (Momordica Charantia) by HPLC-MS/MS. J. AOAC Int. 95, 1597–1608. doi:10.5740/jaoacint.11-511
Ma, J., Whittaker, P., Keller, A. C., Mazzola, E. P., Pawar, R. S., White, K. D., et al. (2010). Cucurbitane-type Triterpenoids from Momordica Charantia. Planta Med. 76, 1758–1761. doi:10.1055/s-0030-1249807
Mager, J., Razin, A., and Hershko, A. (1969). “Favism,” in Toxic Constituents of Plant Foodstuffs. Editor I. E. Liener (Academic Press), 293–318. doi:10.1016/b978-0-12-395739-9.50015-5
Marles, R. J., and Farnsworth, N. R. (1995). Antidiabetic Plants and Their Active Constituents. Phytomedicine 2, 137–189. doi:10.1016/S0944-7113(11)80059-0
Medica, P. (2012). Erratum: Cucurbitane-type Triterpenoids from Momordica Charantia. Planta Med. 78, 102.
Mishra, S., AnkitSharma, R., Sharma, R., Gogna, N., and Dorai, K. (2022). NMR-based Metabolomic Profiling of the Differential Concentration of Phytomedicinal Compounds in Pericarp, Skin and Seeds of Momordica Charantia (Bitter Melon). Nat. Prod. Res. 36, 390–395. doi:10.1080/14786419.2020.1762190
Naseem, M. Z., Patil, S. R., Patil, S. R., Ravindra, , , and Patil, R. S. (1998). Antispermatogenic and Androgenic Activities of Momordica Charantia (Karela) in Albino Rats. J. Ethnopharmacol. 61, 9–16. doi:10.1016/s0378-8741(98)00006-3
Nhiem, N. X., Kiem, P. V., Minh, C. V., Ban, N. K., Cuong, N. X., Tung, N. H., et al. (2010). Alpha-Glucosidase Inhibition Properties of Cucurbitane-type Triterpene Glycosides from the Fruits of Momordica Charantia. Chem. Pharm. Bull. (Tokyo) 58, 720–724. doi:10.1248/cpb.58.720
Ooi, C. P., Yassin, Z., and Hamid, T. A. (2012). Momordica Charantia for Type 2 Diabetes Mellitus. Cochrane Database Syst. Rev. 8, CD007845. doi:10.1002/14651858.CD007845.pub3
Pahlavani, N., Roudi, F., Zakerian, M., Ferns, G. A., Navashenaq, J. G., Mashkouri, A., et al. (2019). Possible Molecular Mechanisms of Glucose-Lowering Activities of Momordica Charantia (Karela) in Diabetes. J. Cell. Biochem. 120, 10921–10929. doi:10.1002/jcb.28483
Perera, W. H., Shivanagoudra, S. R., Pérez, J. L., Kim, D. M., Sun, Y., Guddadarangavvanahally, K J., et al. (2021). Anti-inflammatory, Antidiabetic Properties and In Silico Modeling of Cucurbitane-type Triterpene Glycosides from Fruits of an Indian Cultivar of Momordica Charantia L. Molecules 26, 1038. doi:10.3390/molecules26041038
Peter, E. L., Kasali, F. M., Deyno, S., Mtewa, A., Nagendrappa, P. B., Tolo, C. U., et al. (2019). Momordica Charantia L. Lowers Elevated Glycaemia in Type 2 Diabetes Mellitus Patients: Systematic Review and Meta-Analysis. J. Ethnopharmacol. 231, 311–324. doi:10.1016/j.jep.2018.10.033
Raman, A., and Lau, C. (1996). Anti-diabetic Properties and Phytochemistry of Momordica Charantia L. (Cucurbitaceae). Phytomedicine 2, 349–362. doi:10.1016/S0944-7113(96)80080-8
Saeed, F., Afzaal, M., Niaz, B., Arshad, M. U., Tufail, T., Hussain, M. B., et al. (2018). Bitter Melon (Momordica Charantia): a Natural Healthy Vegetable. Int. J. Food Prop. 21, 1270–1290. doi:10.1080/10942912.2018.1446023
Shetty, A. K., Suresh Kumar, G., Sambaiah, K., and Salimath, P. V. (2005). Effect of Bitter Gourd (Momordica charantia) on Glycaemic Status in Streptozotocin Induced Diabetic Rats. Plant Foods Hum. Nutr. 60, 109–112. doi:10.1007/s11130-005-6837-x
Shivanagoudra, S. R., Perera, W. H., Perez, J. L., Athrey, G., Sun, Y., Jayaprakasha, G. K., et al. (2019a). Cucurbitane-type Compounds from Momordica Charantia: Isolation, In Vitro Antidiabetic, Anti-inflammatory Activities and In Silico Modeling Approaches. Bioorg. Chem. 87, 31–42. doi:10.1016/j.bioorg.2019.02.040
Shivanagoudra, S. R., Perera, W. H., Perez, J. L., Athrey, G., Sun, Y., Wu, C. S., et al. (2019b). In Vitro and In Silico Elucidation of Antidiabetic and Anti-inflammatory Activities of Bioactive Compounds from Momordica Charantia L. Bioorg. Med. Chem. 27, 3097–3109. doi:10.1016/j.bmc.2019.05.035
Singh, N., Tyagi, S. D., and Agarwal, S. C. (1989). Effects of Long Term Feeding of Acetone Extract of Momordica Charantia (Whole Fruit Powder) on Alloxan Diabetic Albino Rats. Indian J. Physiol. Pharmacol. 33, 97–100.
Stepka, W., Wilson, K. E., and Madge, G. E. (1974). Antifertility Investigation on Momordica. Lloydia 37, 645.
Sun, L., Zhang, X., Dong, L., Zhang, C., Guo, P., and Wu, C. (2021). The Triterpenoids of the Bitter Gourd (Momordica charantia) and their Pharmacological Activities: A Review. J. Food Compost. Anal. 96, 103726. doi:10.1016/j.jfca.2020.103726
Tan, M. J., Ye, J. M., Turner, N., Hohnen-Behrens, C., Ke, C. Q., Tang, C. P., et al. (2008). Antidiabetic Activities of Triterpenoids Isolated from Bitter Melon Associated with Activation of the AMPK Pathway. Chem. Biol. 15, 263–273. doi:10.1016/j.chembiol.2008.01.013
Tan, S. P., Parks, S. E., Stathopoulos, C. E., and Roach, P. D. (2014). Greenhouse-grown Bitter Melon: Production and Quality Characteristics. J. Sci. Food Agric. 94, 1896–1903. doi:10.1002/jsfa.6509
Tan, S. P., Kha, T. C., Parks, S. E., and Roach, P. D. (2016). Bitter Melon (Momordica charantiaL.) Bioactive Composition and Health Benefits: A Review. Food Rev. Int. 32, 181–202. doi:10.1080/87559129.2015.1057843
Walters, T. W., and Decker-Walters, D. S. (1988). Balsam-pear (Momordica Charantia, Cucurbitaceae). Econ. Bot. 42, 286–288.
Wang, Y. H., Avula, B., Liu, Y., and Khan, I. A. (2008). Determination and Quantitation of Five Cucurbitane Triterpenoids in Momordica Charantia by Reversed-phase High-Performance Liquid Chromatography with Evaporative Light Scattering Detection. J. Chromatogr. Sci. 46, 133–136. doi:10.1093/chromsci/46.2.133
Yang, Y. S., Wu, N. Y., Kornelius, E., Huang, C. N., and Yang, N. C. (2022). A Randomized, Double-Blind, Placebo-Controlled Trial to Evaluate the Hypoglycemic Efficacy of the mcIRBP-19-Containing Momordica Charantia L. Fruit Extracts in the Type 2 Diabetic Subjects. Food Nutr. Res. 66, 3685. doi:10.29219/fnr.v66.3685
Yin, J., Zhang, H., and Ye, J. (2008). Traditional Chinese Medicine in Treatment of Metabolic Syndrome. Endocr. Metab. Immune. Disord. Drug Targets 8, 99–111. doi:10.2174/187153008784534330
Yin, R. V., Lee, N. C., Hirpara, H., and Phung, O. J. (2014). The Effect of Bitter Melon (Mormordica Charantia) in Patients with Diabetes Mellitus: a Systematic Review and Meta-Analysis. Nutr. Diabetes 4, e145. doi:10.1038/nutd.2014.42
Zänker, K., Mang, B., Wolters, M., and Hahn, A. (2012). Personalized Diabetes and Cancer Medicine: a Rationale for Anti-diabetic Nutrition (Bitter Melon) in a Supportive Setting. Curr. Cancer Ther. Rev. 8, 66–77.
Keywords: diabetes mellitus, anti-diabetic activity, triterpene saponins, momordicosides, cucurbitanoids, GLUT4, momordica charantia, bitter melon
Citation: Çiçek SS (2022) Momordica charantia L.—Diabetes-Related Bioactivities, Quality Control, and Safety Considerations. Front. Pharmacol. 13:904643. doi: 10.3389/fphar.2022.904643
Received: 25 March 2022; Accepted: 28 April 2022;
Published: 17 May 2022.
Edited by:
Ulrike Grienke, University of Vienna, AustriaReviewed by:
Olivier Potterat, University of Basel, SwitzerlandCica Vissiennon, Leipzig University, Germany
Copyright © 2022 Çiçek. This is an open-access article distributed under the terms of the Creative Commons Attribution License (CC BY). The use, distribution or reproduction in other forums is permitted, provided the original author(s) and the copyright owner(s) are credited and that the original publication in this journal is cited, in accordance with accepted academic practice. No use, distribution or reproduction is permitted which does not comply with these terms.
*Correspondence: Serhat S. Çiçek, scicek@pharmazie.uni-kiel.de