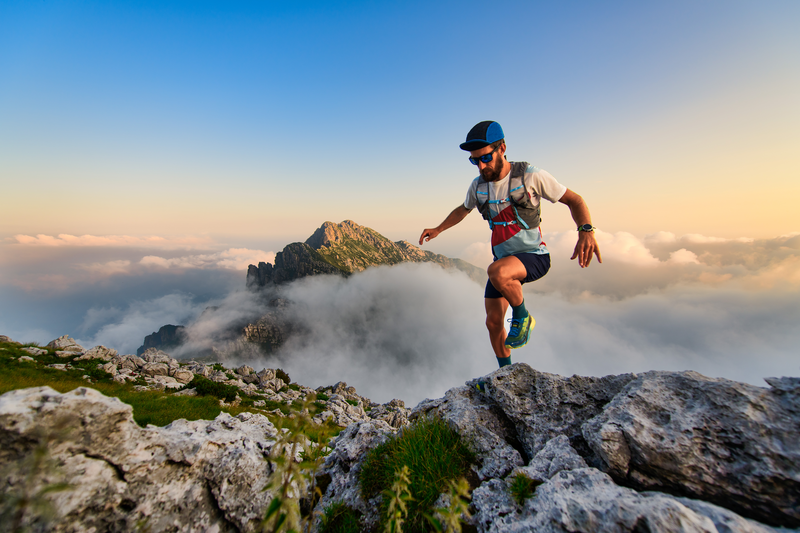
94% of researchers rate our articles as excellent or good
Learn more about the work of our research integrity team to safeguard the quality of each article we publish.
Find out more
REVIEW article
Front. Pharmacol. , 03 May 2022
Sec. Pharmacology of Anti-Cancer Drugs
Volume 13 - 2022 | https://doi.org/10.3389/fphar.2022.903699
This article is part of the Research Topic Combating Cancer with Natural Products: non-coding RNA and RNA modification View all 20 articles
Accumulating evidence indicates that RNA methylation, as the most common modification of mRNA, is of great significance in tumor progression and metastasis. Colorectal cancer is a common malignant tumor of the digestive system that seriously affects the health of middle-aged and elderly people. Although there have been many studies on the biological mechanism of the occurrence and development of colorectal cancer, there are still major deficiencies in the diagnosis and prognosis of colorectal cancer. With the deep study of RNA methylation, it was found that RNA modification is highly related to colorectal cancer tumorigenesis, development and prognosis. Here, we will highlight various RNA chemical modifications including N6-methyladenosine, 5-methylcytosine, N1-methyladenosine, 7-methylguanine, pseudouridine and their modification enzymes followed by summarizing their functions in colorectal cancer.
With the fourth modality rate among all diseases, colorectal cancer (CRC) is one of the most commonly seen tumors of the gastrointestinal tract, and its incidence is the highest in developed countries (Brody, 2015; Bray et al., 2018; Siegel et al., 2020). At present, colorectal cancer is gradually tending to be younger (Akimoto et al., 2021). The pathogenesis of colorectal cancer is complex and diverse, which may be led by an unhealthy diet, obesity, lack of exercise, and microbial infection. Long-term exposure to these risk factors affects the intestinal microbiota and host immunity, resulting in genetic and epigenetic alterations in colorectal epithelial cells, ultimately predisposing them to colorectal cancer (Brody, 2015; Akimoto et al., 2021). The progression of colorectal tumors from adenoma to colorectal cancer is a multi-step pathological process of tumorigenesis that takes about 10 years. Abnormal mutations, accumulation of proto-oncogenes and tumor suppressor genes play a huge part in this process which includes heritable changes in the genome with changes in the basic nucleotides of DNA and stable inheritance of unchanged nucleotide sequences that cause changes in gene expression and function, that is, epigenetics (Sjöblom et al., 2006; Markowitz and Bertagnolli, 2009).
Epigenetic modifications are dynamically reversible and heritable, including DNA methylation, histone modifications, chromatin remodeling, microRNAs (miRNAs) and noncoding RNAs (ncRNAs) which are of great importance in the progression and metastasis of colorectal cancer (Huang et al., 2021a). Recently, major breakthroughs have been made in the research on DNA omics and proteomics in the occurrence and development of tumors, and the epigenetic modification at the RNA level has also attracted the attention of many researchers (Skvortsova et al., 2019; Sivanand and Vander Heiden, 2020; Mobet et al., 2022; Wishart, 2022).
RNA modifications play a key regulatory role in gene expression (Helm and Motorin, 2017; Wu et al., 2021). In recent years, over 170 RNA chemical modifications have been discovered, involving both coding and noncoding RNAs (Boccaletto et al., 2022). In eukaryotic, the most abundant modification found is N6-methyladenosine (m6A), which is considered as eukaryotic characteristic internal modification since its discovery in the 1870s (Gilbert et al., 2016; Davalos et al., 2018). RNA is not only an intermediate or effector molecule in protein synthesis but also has direct functional effects on gene expression through a variety of other noncoding RNAs. Dynamic modification of RNA enables cells to respond rapidly to changes in the external environment, and the ability to adapt to changing microenvironments (such as stimuli and stress) is critical for tumor cell survival. Recent research has demonstrated that RNA modification has become a main emerging regulator in cancer by regulating various RNA metabolic processes (Cui et al., 2017; Barbieri and Kouzarides, 2020; Miano et al., 2021). More and more evidence has shown that the abnormal expression of various m6A regulatory proteins plays a role in promoting or suppressing tumors in human tumors (Nombela et al., 2021). Abnormal changes in RNA modification are often functionally related to cell proliferation, differentiation, stress adaptation, invasion and resistance to chemotherapy. Therefore, targeting abnormal RNA modifications in cancer cells is expected to be an effective way to treat tumors.
In this review, we will summarize the biological characteristics of various RNA modifications, including m6A, m5C, N1-methyladenosine, 7-methylguanine, pseudouridine and their roles in the occurrence and development of colorectal tumors by providing a basis for further search for biomarkers and therapeutic targets.
m6A methylation modification is a common RNA methylation modification on the 6th N of adenine. It is a dynamically reversible post-transcriptional modification that is most abundant in mRNA and noncoding RNA (Figure 1). The modification of m6A occurs mainly in the RRACH sequence motif (where R = A or G, H = A, C, or U) and is significantly enriched in the mRNA 3′UTR as well as CDS regions (Dominissini et al., 2012; Meyer et al., 2012). m6A is involved in multiple processes of RNA metabolism, including post-transcriptional splicing, translation efficiency and stability maintenance, and is essential in normal physiological processes such as growth and development, learning and memory, and is also involved in regulating the response to body heat shock (Zhou et al., 2015; Shi et al., 2018; Wang et al., 2018). It is also crucial for tumor development and tumor immune drug resistance (Han et al., 2019). m6A modification is regulated by methyltransferases, demethylases, and methyl-recognition proteins as a post-translational RNA modification. Among them, methyltransferase catalyzes the modification of adenylate by m6A in RNA, which is composed of various proteins such as METTL3 (methyltransferase like 3), METTL14 (methyltransferase like 14), WTAP (Wilms tumor 1 associating protein) (Frye et al., 2018; Shi et al., 2019) and KIAA1429 (Liu et al., 2014). The core proteins of demethylase include FTO (fat mass and obesity-associated protein) and ALKBH5 (Alkb homolog 5), which can demethylate bases that undergo a modification of m6A, which is also the cause of dynamic reversibility. Methylation recognition proteins can recognize and bind to m6A-modified bases, and regulate biological processes such as RNA degradation and stabilization, nuclear export, and translation efficiency. Given their functional characteristics, these proteins are called “writer,” “eraser,” and “reader” (Zaccara et al., 2019).
FIGURE 1. Schematic diagram of RNA m6A methylation mechanism. m6A modification is a dynamic and reversible process, which could be regulated by writers (WTAP, METTL3/14, KIAA1429, VIRMA and RBM15), erasers (FTO and ALKBH5) and readers (eIF3, YTHDCs, YTDFs and IGF2BPs).
In recent years, m6A modification has gradually become the hot field in studying colorectal tumorigenesis, development and metastasis. m6A modifications have widely altered the expression of certain colorectal tumor-associated genes. Abnormally expressed writer protein, eraser protein, and reader protein, by regulating the level of m6A in different RNAs, affects the function of downstream pathways, such as the classical pathway related to colorectal tumorigenesis and apoptosis, the Wnt pathway and the Hippo pathway, and plays pro- or anti-cancer effects (Chen et al., 2020a; Xu et al., 2020a; Chen et al., 2020b).
It was found that METTL3 expression was increased in tumor tissues of colorectal cancer patients, and the higher its expression, the worse the prognosis of patients (Zhou et al., 2021a; Chen et al., 2021). METTL3 regulates the expression of various oncogenes and tumor suppressor genes at the post-transcriptional level to enhance the progression of colorectal cancer. Overexpression of METTL3 represses SOCS2 and promotes LGR5 promoter activity to maintain cell proliferation in colon cancer cells (Xu et al., 2020b). Knockdown of METTL3 can inhibit the development of colorectal cancer significantly. Research have suggested that METTL3 targets the YPEL5 m6A modification site to inhibit its expression and promote the progression of colorectal cancer (Zhou et al., 2021a). On the other hand, METTL3 stabilizes its expression level by methylating the m6A site of the CCNE1 3′UTR mRNA to increase the expression of the cyclin E1 to promote colorectal cancer cell proliferation (Zhu et al., 2020). Furthermore, a group found that the m6A methyltransferase METTL3 promoted the malignant proliferation of colorectal cancer cells by directly or indirectly upregulating MYC expression (Xiang et al., 2020). In an indirect mechanism, it was found that METTL3 may upregulate MYC expression through the WNT, TGF-β and other signaling pathways. The expression of MYC was also found to be recognized by IGF2BP1 and its stability was enhanced dependent on m6A-IGF2BP1. In addition, METTL3 increases the expression of PTTG3P through an m6A/IGF2BP2-dependent mechanism and regulates the PTTG3P/YAP1 axis to induce colon cancer cell proliferation and promote colon cancer progression (Zheng et al., 2021). METTL3 also regulates the progression of colorectal cancer by affecting the metabolism of tumor cells. Shen et al. found that the m6A methyltransferase METTL3 regulates the expression levels of HK2 and SLC2A1 by regulating m6A expression and stabilizing m6A under the action of the methyl-binding protein IGF2BP, which further promotes the activation of the glycolytic pathway, inducing malignant proliferation of colorectal cancer cells (Shen et al., 2020). Another study has confirmed that METTL3 carries out m6A modification on GLUT1 mRNA to improve its mRNA stability and translation level, and promote glucose metabolism and colorectal cancer occurrence (Chen et al., 2021). Through the regulation of the m6A-GLUT1-mTORC1 axis, METTL3 is actively involved in the proliferation of colorectal cancer, and simultaneous inhibition of mTORC1 and METTL3 has an additive effect on the treatment of colorectal cancer. In addition to the effects on cell proliferation, METTL3 also has relations with the progression and metastasis of colorectal cancer. Peng et al. (2019) demonstrated that the overexpressed m6A methyltransferase METTL3 in colorectal cancer can increase miRNA-1246 expression by upregulating the methylation level of pri-miR-1246, and further lead to tumor metastasis by downregulating the expression of the metastasis-related suppressor gene SPRED2. In addition, it was found that SPRED2 regulates colorectal cancer metastasis through the MAPK signaling pathway. Research has suggested that MAPK can regulate the epithelial-mesenchymal transition (EMT) process, thus impacting tumor metastasis (Huang et al., 2021b). Li et al. (2019) shown that METTL3 regulates the progression and migration of colorectal cancer by increasing the methylation level of SOX2 and enhancing the stability of methylated SOX2 through an m6A-IGF2BP2-dependent mechanism. It was also found that the tumor-suppressing METTL3 had low expression level in colorectal tumor, and it lost its inhibitory effect on p38/ERK in the MAPK signaling pathway and promoted colorectal cancer migration (Deng et al., 2019). In sum, METTL3 modulates the expression and function of various target molecules in a m6A-modified manner to affect the proliferation, apoptosis, metabolism, invasion and metastasis of colorectal cancer cells. Therefore, specific targeting of METTL3 has important application prospects in the treatment of colorectal cancer.
In contrast, another m6A writer protein, METTL14, expressed at a low level in colorectal cancer. The higher the expression of METTL14, the longer the overall survival of the patients, and overexpression of METTL14 can suppress the metastasis and progression of colorectal cancer (Chen et al., 2020b). METTL14 knockout was shown to reduce the level of m6A of its downstream target SOX4, resulting in reduced recognition of the modified SOX4 mRNA by YTHDF2, thus increasing SOX4 gene expression and promoting the SOX4-mediated EMT process and activity of the PI3K/AKT signaling pathway, leading to malignant progression of colorectal cancer (Chen et al., 2020a). This was also confirmed in another study in which the researcher found that the suppression of METTL14 can enhance the progression and metastasis of colorectal cancer (Wang et al., 2021). Tumor-associated macrophages (TAM) can inhibit the antitumor activity of T cells, but the mechanism is not clear. A recent study found that in colorectal cancer patients’ tumor tissue, the expression of METTL14 was negatively correlated with CD8+ T cell infiltration (Dong et al., 2021). Reduced expression of METTL14 in TAM subset C1q + cells can promote colon cancer cell growth and impede CD8+ T cell infiltration. It was further found that the reduction of Ebi3 expression level in C1q + cells can restore the anti-tumor killing ability of CD8+ T cells, while the loss of METTL14 expression can reduce the m6A modification level of Ebi3 mRNA in C1q + cells and promote the increase of its transcription level. Therefore, increasing the expression of METTL14 in TAM subset C1q + cells can promote CD8+ T cell infiltration and antitumor effects (Dong et al., 2021).
The third writer protein WTAP has been shown to have tumor-promoting action in many malignancies, including liver cancer, gastric cancer and osteosarcoma (Chen et al., 2019a; Li et al., 2020a; Chen et al., 2020c; Zhou et al., 2021b; Feng et al., 2021). In colorectal cancer, studies have pointed out WTAP is also oncogenic and can promote the progression of colorectal cancer through the WTAP/WT1/TBL1 axis in the canonical Wnt signaling pathway (Zhang et al., 2016a). Another group also suggested the higher expression of WTAP protein in colorectal cancer (Dong et al., 2022). In sum, both the dual role of METTL3 in colorectal tumors and the pro-oncogenic effect of METTL14 and WTAP in other tumors suggest that the m6A modification sites on different downstream target genes are different, which affect the activation status of various signaling pathways they are involved in, and thus play specific roles in tumors. Therefore, the next step in the investigation of m6A modification in the progression of colorectal tumor disease could be how to preserve the oncogenic role of the regulator by using inhibitors or developing new drugs to block its oncogenic signaling in specific signaling pathways.
However, unlike m6A methyltransferase, eraser and reader proteins have been less studied in colorectal cancer. It has been found that FTO is regulated by miR-1266 in the nucleus and initiates cell signaling molecules STAT3, cyclin D1 and MMPs to promote the development of colorectal cancer (Shen et al., 2018; Roslan et al., 2019; Ge et al., 2021). In addition, one group recently found that FTO is regulated by miR-96, which affects the methylation level of MYC and increase the expression of MYC, thus participating in the pro-proliferative and anti-apoptotic effects of miR-96 in colorectal cancer (Yue et al., 2020). While in the cytoplasm, FTO can dynamically regulate m6A modifications to contribute to the stemness of colorectal cancer cells and influence tumor drug resistance (Relier et al., 2021). Preliminary progress has also been made in the mechanism study of another demethylase, ALKBH5, in mediating colorectal cancer metastasis and immunotherapy resistance. The expression of ALKBH5 is downregulated in colon cancer and is associated with tumor metastasis, and it is pointed out that this molecule can be used as an independent predictor of patient prognosis (Yang et al., 2020). This study further confirmed the tumor suppressor effect of ALKBH5 both in vitro and in vivo (Yang et al., 2020). One study found that ALKBH5 can affect the tumor microenvironment of colorectal cancer, thus mediating the resistance of colorectal cancer patients to the anti-PD-1 therapy response (Li et al., 2020b). Meanwhile, ALKBH5 inhibitors can significantly improve immunotherapy efficacy, providing new possibilities for targeted therapy of colorectal cancer, melanoma, and other malignant tumors. Together, these demonstrated that ALKBH5 can not only be used as a breakthrough target for the clinical treatment of colorectal cancer in the future, but also has the potential to become a new biomarker for the patient population. Furthermore, there is still a gap in the study of the functional mechanisms of other members of the Alkb subfamily in colorectal tumors. The search for new molecules related to m6A modification and the study of their mechanisms is still a promising direction worth studying in the future.
Reader protein reads m6A modification sites of key molecules of colorectal tumor signaling pathways, affecting the expression level and RNA stability of these genes. In addition, the reader protein also forms complexes with noncoding RNAs related to colorectal tumors to improve its stability. Transcriptional and translational levels of YTHDF1 are significantly elevated in colorectal cancer patients. One study found YTHDF1 could enhance tumor cell stemness to promote tumorigenesis by inhibiting the canonical Wnt/β-catenin pathway (Bai et al., 2019). Another member of the YTHDF family, YTHDF3, affects colorectal cancer progression by regulating the negative feedback axis of the lncRNA GAS5-YAP-YTHDF3 in the Hippo pathway (Ni et al., 2019). Downregulation of the YTHDC2 gene can significantly inhibit the translation of tumor metastasis-related genes, such as hypoxia-inducible factor-1alpha (HIF-1α), and the high expression of this molecule is positively correlated with tumor stage and colon cancer metastasis (Tanabe et al., 2016).
circRNA is an RNA molecule with a novel structure. In recent years, modification of m6A has also been found in some circRNAs (Figure 2), with functions in the tumor occurrence and progression being reported (Zhao et al., 2019a; Li et al., 2021; Rao et al., 2021). In colorectal cancer, a study reported that circNSUN2 modified with m6A was frequently upregulated in tumor tissue and serum samples from patients with liver metastases of colorectal cancer (Chen et al., 2019b). The results show that after being recognized by YTHDC1, m6A-modified circNSUN2 will be exported to the cytoplasm, contributing to the formation of the ternary complex of circNSUN2-insulin-like growth factor 2 mRNA binding protein. circNSUN2 enhances the stability of HMGA2 mRNA, thereby promoting colorectal cancer invasion and liver metastasis (Chen et al., 2019b). circNSUN2 may become a potential therapeutic target for liver metastases of colorectal cancer.
FIGURE 2. Regulation of m6A methylation in circRNA. YTHDC1 could promote circRNA nuclear export through binding to m6A methylation sites. The complex of eIF4G2, eIF4, eIF4B and YTHDF3 could initiate the translation of m6A-modified circRNA, and the circRNA could be cleaved by YTHDF2-HRSP12-RNase P/MRP complex.
m5C modifications are widely found in mRNA, rRNA, tRNA, and ncRNA, with the greatest abundance in tRNA and rRNA in eukaryotes (Figure 3). Enzymes responsible for RNA m5C modification include NSUN1 to NSUN7 of the NSUN family and DNA Methyltransferase-2 (DNMT2) (Bohnsack et al., 2019; Kuznetsova et al., 2019). NSUN1 and NSUN5 are involved in regulating 28S rRNA m5C modification, while NSUN3 and NSUN4 regulate mitochondrial tRNA and rRNA m5C modification, respectively. NSUN2 and DNMT2 regulate tRNA m5C modification in the cytoplasm, while NSUN7 targets eRNAs. The m5C regulator also functions in some digestive tumors. Studies have shown that the copy number of the NSUN2 gene is significantly increased in colorectal cancer, and the RNA methyltransferase NSUN2 is associated with the oncogene MYC. When the MYC protein is activated, the expression of NSUN2 is also upregulated (Alboushi et al., 2021). To date, no specific m5C methyltransferase inhibitor has been developed. However, studies have shown that azacytidine, as a novel antitumor drug, can reduce the proliferation ability of cancer cells by inhibiting DNMT2-mediated m5C modification, suggesting that reducing m5C modification of tRNA may be an effective cancer treatment strategy (Esteller and Pandolfi, 2017).
FIGURE 3. Functions of RNA m5C modification in RNA processing and metabolism. DNMT2 and NSUNs could methylate tRNA, rRNA, mRNA and ncRNA; YTHDF1, YBX1 and ALYREF could recognize m5C modification, while TETs could demethelate them.
m1A modification means the attachment of a methyl group to the N1 position of RNA adenosine. The modification of m1A can significantly alter the structure of the RNA and the strength of protein-RNA interactions, thus affecting the protein translation process (Wiener and Schwartz, 2021). TRM10 and the TRM6-TRM61 complex mediate m1A modification of tRNA (Xiong et al., 2018; Zhang and Jia, 2018). The presence of m1A modification was also detected in 28S rRNA (Sloan et al., 2017). The YTH protein family can bind to m1A with low affinity, so it is considered as a potential m1A reader (Dai et al., 2018). ALKBH1 and ALKBH3, as demethylases, are involved in the regulation of m1A modification. Abnormal expression of m1A-related regulatory genes in digestive tumors is closely related to patient prognosis. Overexpression of ALKBH1 in colorectal cancer is one of the causative factors of a lower survival rate, as well as low expression of ALKBH3 (Zhao et al., 2019b).
The epigenetic modification m7G was originally found to exist within eukaryotic mRNA, tRNA, and rRNA. The most typical enzyme characterizing m7G methylation modification is METTL1. In tRNA, METTL1-WDR4 complex-mediated m7G modification maintains its structural integrity (Lin et al., 2018). The m7G in rRNA is mediated by the Williams-Beuren syndrome chromosome region 22 (WBSCR22), but its role is not fully understood. The m7G modification on rRNA may be involved in ribosome maturation, but has little effect on translation (Haag et al., 2015). The m7G modification within mRNA is enriched at the 5′UTR and is dynamically regulated with stress changes, and its role is to promote the translation process (Malbec et al., 2019). As an important regulator of m7G, METTL1 functions as a tumor suppressor in colorectal cancer (Liu et al., 2020). In addition, overexpression of METTL1 also enhance the chemosensitivity of colorectal cancer cells to cisplatin by regulating the miR-149-3p/S100A4/p53 axis (Liu et al., 2019). These results suggest that maintaining high levels of functional tRNA may be crucial for METTL1 executing function in cancer cells. Although the effect of METTL1 on tRNA may be cancer-promoting, there is no direct evidence that m7G modification plays a role in cancer cells. Another study demonstrated that WBSCR22 expression in colorectal cancer tissues is remarkably increased and upregulated WBSCR22 predicts a poor prognosis in patients (Yan et al., 2017). Knockdown of WBSCR22 can significantly improve the sensitivity of colorectal cancer cells to oxaliplatin, while overexpression of WBSCR22 increases cell resistance. Knockdown of WBSCR22 can increase oxaliplatin-induced intracellular ROS production and ROS-induced accumulation of 8-oxoguanine oxidative damage, which makes cancer cells more susceptible to oxaliplatin treatment (Yan et al., 2017).
Pseudouridine is another abundant RNA modification in mRNA, tRNA, rRNA, snRNA, and lncRNA. In human cell lines, the proportion of pseudouridine (ψ) modifications in mRNA and lncRNA is about 30%–84% (Zhang et al., 2019). Pseudouridine is important in regulating the response to environmental stress. The presence of ψ can increase the rigidity of the RNA backbone, affecting its thermodynamic stability and spatial conformation, thus making the structure and function of the RNA more stable. ψ modification is involved in maintaining structural stability in tRNA and in the assembly of ribosomes in rRNA. One of the most studied diseases associated with defective pseudouridine modification is dyskeratosis congenita (DC) caused by inactivating mutations in pseudouridine synthase 1 (DKC1). Deficiency of DKC1 activity results in impaired telomerase activity and impaired mRNA translation, resulting in reduced cell replicative potential and premature senescence. Abnormal mutations in DKC1 are associated with various tumors such as colorectal cancer and hepatocellular carcinoma (McMahon, 2019). In colorectal cancer, reduced ψ modification on rRNA alters translation in cancer cells by affecting its interaction with the ribosomal P site (Babaian et al., 2020).
RNA modification plays an important role as a key post-transcriptional regulator in gene expression, and researchers gradually realize that the functional network of its interaction involves many fields such as metabolism, epigenetics, chromatin remodeling, as well as the immune system. Although research on the epitranscriptome has made great progress, most studies have only focused on the biological functions of m6A modifications in mRNA, and the epitranscriptome includes more than 170 chemical modifications that modify coding and noncoding RNAs. Therefore, the association of hundreds of other RNA modifications with coding and noncoding RNAs remains to be explored. To explore the specific biological functions of various RNA modifications, it is first necessary to develop systematic methods and tools for rapid and quantitative detection of RNA modifications. Most existing sequencing methods are based on second generation sequencing technology, which cannot accurately identify chemical modifications on RNA. When researchers detect specific RNA modifications, they often need to detect RNA modifications using specific antibody-based immunoprecipitation techniques or indirect methods such as chemical labeling and the unique base modification properties of RNA pairings. Although these methods have good feasibility, its reproducibility is still low due to the reasons such as technology and incomplete algorithms. Therefore, future detection technologies and methods for various RNA modifications still need further research and exploration.
To date, treatments for colorectal cancer are still very limited. The rapid development of experimental technologies based on high-throughput sequencing and proteomics provides more possibilities for a deep study of the mechanism of RNA modification in colorectal cancer. In colorectal cancer, the complex of methyltransferase modified by m6A methylation, including METTL3, which plays a major catalytic role, has been thoroughly studied and is involved in the regulation of multiple classical signaling pathways in tumor development and apoptosis. However, there are still few studies on other writer molecules, and the roles of WTAP and METTL16, which can act independently, in colorectal tumors are still unclear. Similarly, eraser and reader proteins have been shown to contribute to the occurrence, development, metastasis and drug resistance of colorectal cancers by regulating classical signaling pathways, such as the Wnt pathway and the PI3K/AKT pathway, as well as changing the m6A modification level of noncoding RNAs, but there is still wide room for improvement in the transformation from basic to clinical. Although m6A methylation modification is abundant at the mRNA level, tumor-associated noncoding RNAs (miRNAs, lncRNAs and circRNAs) are also regulated by m6A methylation and act in the disease progression of colorectal cancer. circRNAs have recently been found to encode polypeptides. Whether m6A modification plays a key role and the specific mechanism has attracted the attention of researchers. Therefore, it is also essential to pay attention to tumor-associated noncoding RNAs. In addition, few eraser proteins have been found and only two more abundantly studied molecules, FTO and ALKBH5. ALKBH3 has been confirmed to have the function of demethylase, but there are still many areas that we have not studied in the Alk homolog family. It is still a research direction to explore whether more members of this family can function as demethylases. There are many types of reader proteins, and it is still unknown that reader proteins specifically read m6A modifications on different RNAs and play different downstream functions.
Various RNA modifications including m6A, m5C and ψ function in the regulation of stem cell function and cell survival under stress. The regulation of these RNA modifications may be of great value in reducing tumor cell chemotherapy resistance and tumor recurrence. The identification of precise epitranscriptomic biomarkers in a specific cell type or tumor microenvironment and the identification of aberrantly modified oncogenic or tumor suppressor effects are of great significance for finding precise molecular targets and developing highly selective and effective therapeutic approaches (Table 1). In the future, precision medicine based on epitranscriptomic signatures may be used for the diagnosis and treatment of colorectal cancer (Figure 4).
WL and JX contributed directly to this review. WL designed and wrote the preliminary version of the manuscript. All authors were involved in the manuscript writing, including discussion of content and writing and editing of the manuscript. All authors approved the final text.
This study was supportive by Hebei Funding Project for High-level Talents (A202101062); Training Project for Excellent Clinical Medical Talents of Hebei Provincial Department of Finance (361009); Hebei Provincial Health Commission Medical Science Research Key Project Program (20210954).
The authors declare that the research was conducted in the absence of any commercial or financial relationships that could be construed as a potential conflict of interest.
All claims expressed in this article are solely those of the authors and do not necessarily represent those of their affiliated organizations, or those of the publisher, the editors and the reviewers. Any product that may be evaluated in this article, or claim that may be made by its manufacturer, is not guaranteed or endorsed by the publisher.
Akimoto, N., Ugai, T., Zhong, R., Hamada, T., Fujiyoshi, K., Giannakis, M., et al. (2021). Rising Incidence of Early-Onset Colorectal Cancer - a Call to Action. Nat. Rev. Clin. Oncol. 18 (4), 230–243. doi:10.1038/s41571-020-00445-1
Alboushi, L., Hackett, A. P., Naeli, P., Bakhti, M., and Jafarnejad, S. M. (2021). Multifaceted Control of mRNA Translation Machinery in Cancer. Cell Signal 84, 110037. doi:10.1016/j.cellsig.2021.110037
Babaian, A., Rothe, K., Girodat, D., Minia, I., Djondovic, S., Milek, M., et al. (2020). Loss of M1acp3Ψ Ribosomal RNA Modification Is a Major Feature of Cancer. Cell Rep 31 (5), 107611. doi:10.1016/j.celrep.2020.107611
Bai, Y., Yang, C., Wu, R., Huang, L., Song, S., Li, W., et al. (2019). YTHDF1 Regulates Tumorigenicity and Cancer Stem Cell-like Activity in Human Colorectal Carcinoma. Front. Oncol. 9, 332. doi:10.3389/fonc.2019.00332
Bantis, A., Giannopoulos, A., Gonidi, M., Liossi, A., Aggelonidou, E., Petrakakou, E., et al. (2004). Expression of P120, Ki-67 and PCNA as Proliferation Biomarkers in Imprint Smears of Prostate Carcinoma and Their Prognostic Value. Cytopathology 15 (1), 25–31. doi:10.1046/j.0956-5507.2003.00090.x
Barbieri, I., and Kouzarides, T. (2020). Role of RNA Modifications in Cancer. Nat. Rev. Cancer 20 (6), 303–322. doi:10.1038/s41568-020-0253-2
Barbieri, I., Tzelepis, K., Pandolfini, L., Shi, J., Millán-Zambrano, G., Robson, S. C., et al. (2017). Promoter-bound METTL3 Maintains Myeloid Leukaemia by m6A-dependent Translation Control. Nature 552 (7683), 126–131. doi:10.1038/nature24678
Boccaletto, P., Stefaniak, F., Ray, A., Cappannini, A., Mukherjee, S., Purta, E., et al. (2022). MODOMICS: a Database of RNA Modification Pathways. 2021 Update. Nucleic Acids Res. 50 (D1), D231–d235. doi:10.1093/nar/gkab1083
Bohnsack, K. E., Höbartner, C., and Bohnsack, M. T. (2019). Eukaryotic 5-methylcytosine (m⁵C) RNA Methyltransferases: Mechanisms, Cellular Functions, and Links to Disease. Genes (Basel) 10 (2), 20102. doi:10.3390/genes10020102
Bray, F., Ferlay, J., Soerjomataram, I., Siegel, R. L., Torre, L. A., and Jemal, A. (2018). Global Cancer Statistics 2018: GLOBOCAN Estimates of Incidence and Mortality Worldwide for 36 Cancers in 185 Countries. CA Cancer J. Clin. 68 (6), 394–424. doi:10.3322/caac.21492
Chen, H., Gao, S., Liu, W., Wong, C. C., Wu, J., Wu, J., et al. (2021). RNA N6-Methyladenosine Methyltransferase METTL3 Facilitates Colorectal Cancer by Activating the m6A-GLUT1-mTORC1 Axis and Is a Therapeutic Target. Gastroenterology 160 (4), 1284–e16. e16. doi:10.1053/j.gastro.2020.11.013
Chen, R. X., Chen, X., Xia, L. P., Zhang, J. X., Pan, Z. Z., Ma, X. D., et al. (2019). N6-methyladenosine Modification of circNSUN2 Facilitates Cytoplasmic export and Stabilizes HMGA2 to Promote Colorectal Liver Metastasis. Nat. Commun. 10 (1), 4695. doi:10.1038/s41467-019-12651-2
Chen, S., Li, Y., Zhi, S., Ding, Z., Wang, W., Peng, Y., et al. (2020). WTAP Promotes Osteosarcoma Tumorigenesis by Repressing HMBOX1 Expression in an m6A-dependent Manner. Cell Death Dis 11 (8), 659. doi:10.1038/s41419-020-02847-6
Chen, X., Li, A., Sun, B. F., Yang, Y., Han, Y. N., Yuan, X., et al. (2019). 5-methylcytosine Promotes Pathogenesis of Bladder Cancer through Stabilizing mRNAs. Nat. Cel Biol 21 (8), 978–990. doi:10.1038/s41556-019-0361-y
Chen, X., Xu, M., Xu, X., Zeng, K., Liu, X., Pan, B., et al. (2020). METTL14-mediated N6-Methyladenosine Modification of SOX4 mRNA Inhibits Tumor Metastasis in Colorectal Cancer. Mol. Cancer 19 (1), 106. doi:10.1186/s12943-020-01220-7
Chen, X., Xu, M., Xu, X., Zeng, K., Liu, X., Sun, L., et al. (2020). METTL14 Suppresses CRC Progression via Regulating N6-methyladenosine-dependent Primary miR-375 Processing. Mol. Ther. 28 (2), 599–612. doi:10.1016/j.ymthe.2019.11.016
Chen, Y., Peng, C., Chen, J., Chen, D., Yang, B., He, B., et al. (2019). WTAP Facilitates Progression of Hepatocellular Carcinoma via m6A-HuR-dependent Epigenetic Silencing of ETS1. Mol. Cancer 18 (1), 127. doi:10.1186/s12943-019-1053-8
Chen, Z., Qi, M., Shen, B., Luo, G., Wu, Y., Li, J., et al. (2019). Transfer RNA Demethylase ALKBH3 Promotes Cancer Progression via Induction of tRNA-Derived Small RNAs. Nucleic Acids Res. 47 (5), 2533–2545. doi:10.1093/nar/gky1250
Cui, Q., Shi, H., Ye, P., Li, L., Qu, Q., Sun, G., et al. (2017). m6A RNA Methylation Regulates the Self-Renewal and Tumorigenesis of Glioblastoma Stem CellsA RNA Methylation Regulates the Self-Renewal and Tumorigenesis of Glioblastoma Stem Cells. Cel Rep 18 (11), 2622–2634. doi:10.1016/j.celrep.2017.02.059
Dai, X., Wang, T., Gonzalez, G., and Wang, Y. (2018). Identification of YTH Domain-Containing Proteins as the Readers for N1-Methyladenosine in RNA. Anal. Chem. 90 (11), 6380–6384. doi:10.1021/acs.analchem.8b01703
Davalos, V., Blanco, S., and Esteller, M. (2018). SnapShot: Messenger RNA Modifications. Cell 174 (2), 498–e1. doi:10.1016/j.cell.2018.06.046
Deng, R., Cheng, Y., Ye, S., Zhang, J., Huang, R., Li, P., et al. (2019). m6A Methyltransferase METTL3 Suppresses Colorectal Cancer Proliferation and Migration through P38/ERK pathwaysA Methyltransferase METTL3 Suppresses Colorectal Cancer Proliferation and Migration through P38/ERK Pathways. Onco Targets Ther. 12, 4391–4402. doi:10.2147/OTT.S201052
Dominissini, D., Moshitch-Moshkovitz, S., Schwartz, S., Salmon-Divon, M., Ungar, L., Osenberg, S., et al. (2012). Topology of the Human and Mouse m6A RNA Methylomes Revealed by m6A-Seq. Nature 485 (7397), 201–206. doi:10.1038/nature11112
Dong, L., Chen, C., Zhang, Y., Guo, P., Wang, Z., Li, J., et al. (2021). The Loss of RNA N6-Adenosine Methyltransferase Mettl14 in Tumor-Associated Macrophages Promotes CD8+ T Cell Dysfunction and Tumor Growth. Cancer Cell 39 (7), 945–e10. e10. doi:10.1016/j.ccell.2021.04.016
Dong, X. F., Wang, Y., Tang, C. H., Huang, B. F., Du, Z., Wang, Q., et al. (2022). Upregulated WTAP Expression in Colorectal Cancer Correlates with Tumor Site and Differentiation. PLoS One 17 (2), e0263749. doi:10.1371/journal.pone.0263749
Esteller, M., and Pandolfi, P. P. (2017). The Epitranscriptome of Noncoding RNAs in Cancer. Cancer Discov. 7 (4), 359–368. doi:10.1158/2159-8290.CD-16-1292
Feng, Z. Y., Wang, T., Su, X., and Guo, S. (2021). Identification of the m6A RNA Methylation Regulators WTAP as a Novel Prognostic Biomarker and Genomic Alterations in Cutaneous Melanoma. Front. Mol. Biosci. 8, 665222. doi:10.3389/fmolb.2021.665222
Frye, M., Harada, B. T., Behm, M., and He, C. (2018). RNA Modifications Modulate Gene Expression during Development. Science 361 (6409), 1346–1349. doi:10.1126/science.aau1646
Ge, Y., Liu, T., Wang, C., Zhang, Y., Xu, S., Ren, Y., et al. (2021). N6-methyladenosine RNA Modification and its Interaction with Regulatory Non-coding RNAs in Colorectal Cancer. RNA Biol. 18 (Suppl. 2), 551–561. doi:10.1080/15476286.2021.1974749
Gilbert, W. V., Bell, T. A., and Schaening, C. (2016). Messenger RNA Modifications: Form, Distribution, and Function. Science 352 (6292), 1408–1412. doi:10.1126/science.aad8711
Haag, S., Kretschmer, J., and Bohnsack, M. T. (2015). WBSCR22/Merm1 Is Required for Late Nuclear Pre-ribosomal RNA Processing and Mediates N7-Methylation of G1639 in Human 18S rRNA. Rna 21 (2), 180–187. doi:10.1261/rna.047910.114
Han, D., Liu, J., Chen, C., Dong, L., Liu, Y., Chang, R., et al. (2019). Anti-tumour Immunity Controlled through mRNA m6A Methylation and YTHDF1 in Dendritic Cells. Nature 566 (7743), 270–274. doi:10.1038/s41586-019-0916-x
Helm, M., and Motorin, Y. (2017). Detecting RNA Modifications in the Epitranscriptome: Predict and Validate. Nat. Rev. Genet. 18 (5), 275–291. doi:10.1038/nrg.2016.169
Huang, Y., Yuan, K., Tang, M., Yue, J., Bao, L., Wu, S., et al. (2021). Melatonin Inhibiting the Survival of Human Gastric Cancer Cells under ER Stress Involving Autophagy and Ras-Raf-MAPK Signalling. J. Cel Mol Med 25 (3), 1480–1492. doi:10.1111/jcmm.16237
Huang, Y., Zhou, Z., Zhang, J., Hao, Z., He, Y., Wu, Z., et al. (2021). lncRNA MALAT1 Participates in Metformin Inhibiting the Proliferation of Breast Cancer Cell. J. Cel Mol Med 25 (15), 7135–7145. doi:10.1111/jcmm.16742
Iles, M. M., Law, M. H., Stacey, S. N., Han, J., Fang, S., Pfeiffer, R., et al. (2013). A Variant in FTO Shows Association with Melanoma Risk Not Due to BMI. Nat. Genet. 45 (4), 428432e1–432e1. doi:10.1038/ng.2571
Jana, S., Hsieh, A. C., and Gupta, R. (2017). Reciprocal Amplification of Caspase-3 Activity by Nuclear export of a Putative Human RNA-Modifying Protein, PUS10 during TRAIL-Induced Apoptosis. Cel Death Dis 8 (10), e3093. doi:10.1038/cddis.2017.476
Konishi, N., Nakamura, M., Ishida, E., Shimada, K., Mitsui, E., Yoshikawa, R., et al. (2005). High Expression of a New Marker PCA-1 in Human Prostate Carcinoma. Clin. Cancer Res. 11 (14), 5090–5097. doi:10.1158/1078-0432.CCR-05-0195
Kuznetsova, S. A., Petrukov, K. S., Pletnev, F. I., Sergiev, P. V., and Dontsova, O. A. (2019). RNA (C5-Cytosine) Methyltransferases. Biochemistry (Mosc) 84 (8), 851–869. doi:10.1134/S0006297919080029
Li, B., Zhu, L., Lu, C., Wang, C., Wang, H., Jin, H., et al. (2021). CircNDUFB2 Inhibits Non-small Cell Lung Cancer Progression via Destabilizing IGF2BPs and Activating Anti-tumor Immunity. Nat. Commun. 12 (1), 295. doi:10.1038/s41467-020-20527-z
Li, H., Su, Q., Li, B., Lan, L., Wang, C., Li, W., et al. (2020). High Expression of WTAP Leads to Poor Prognosis of Gastric Cancer by Influencing Tumour-Associated T Lymphocyte Infiltration. J. Cel Mol Med 24 (8), 4452–4465. doi:10.1111/jcmm.15104
Li, N., Kang, Y., Wang, L., Huff, S., Tang, R., Hui, H., et al. (2020). ALKBH5 Regulates Anti-PD-1 Therapy Response by Modulating Lactate and Suppressive Immune Cell Accumulation in Tumor Microenvironment. Proc. Natl. Acad. Sci. U S A. 117 (33), 20159–20170. doi:10.1073/pnas.1918986117
Li, T., Hu, P. S., Zuo, Z., Lin, J. F., Li, X., Wu, Q. N., et al. (2019). METTL3 Facilitates Tumor Progression via an m6A-igf2bp2-dependent Mechanism in Colorectal Carcinoma. Mol. Cancer 18 (1), 112. doi:10.1186/s12943-019-1038-7
Li, Y., Li, J., Luo, M., Zhou, C., Shi, X., Yang, W., et al. (2018). Novel Long Noncoding RNA NMR Promotes Tumor Progression via NSUN2 and BPTF in Esophageal Squamous Cell Carcinoma. Cancer Lett. 430, 57–66. doi:10.1016/j.canlet.2018.05.013
Li, Z., Weng, H., Su, R., Weng, X., Zuo, Z., Li, C., et al. (2017). FTO Plays an Oncogenic Role in Acute Myeloid Leukemia as a N6-Methyladenosine RNA Demethylase. Cancer Cell 31 (1), 127–141. doi:10.1016/j.ccell.2016.11.017
Lin, S., Choe, J., Du, P., Triboulet, R., and Gregory, R. I. (2016). The M(6)A Methyltransferase METTL3 Promotes Translation in Human Cancer Cells. Mol. Cel 62 (3), 335–345. doi:10.1016/j.molcel.2016.03.021
Lin, S., Liu, Q., Lelyveld, V. S., Choe, J., Szostak, J. W., and Gregory, R. I. (2018). Mettl1/Wdr4-Mediated m7G tRNA Methylome Is Required for Normal mRNA Translation and Embryonic Stem Cell Self-Renewal and Differentiation. Mol. Cel 71 (2), 244–e5. e5. doi:10.1016/j.molcel.2018.06.001
Liu, J., Yue, Y., Han, D., Wang, X., Fu, Y., Zhang, L., et al. (2014). A METTL3-METTL14 Complex Mediates Mammalian Nuclear RNA N6-Adenosine Methylation. Nat. Chem. Biol. 10 (2), 93–95. doi:10.1038/nchembio.1432
Liu, Y., Yang, C., Zhao, Y., Chi, Q., Wang, Z., and Sun, B. (2019). Overexpressed Methyltransferase-like 1 (METTL1) Increased Chemosensitivity of colon Cancer Cells to Cisplatin by Regulating miR-149-3p/S100A4/p53 axis. Aging (Albany NY) 11 (24), 12328–12344. doi:10.18632/aging.102575
Liu, Y., Zhang, Y., Chi, Q., Wang, Z., and Sun, B. (2020). Methyltransferase-like 1 (METTL1) served as a tumor suppressor in colon cancer by activating 7-methyguanosine (m7G) regulated let-7e miRNA/HMGA2 axis. Life Sci. 249, 117480. doi:10.1016/j.lfs.2020.117480
Malbec, L., Zhang, T., Chen, Y. S., Zhang, Y., Sun, B. F., Shi, B. Y., et al. (2019). Dynamic Methylome of Internal mRNA N7-Methylguanosine and its Regulatory Role in Translation. Cell Res 29 (11), 927–941. doi:10.1038/s41422-019-0230-z
Markowitz, S. D., and Bertagnolli, M. M. (2009). Molecular Origins of Cancer: Molecular Basis of Colorectal Cancer. N. Engl. J. Med. 361 (25), 2449–2460. doi:10.1056/NEJMra0804588
McMahon, M. (2019). A Single H/ACA Small Nucleolar RNA Mediates Tumor Suppression Downstream of Oncogenic RAS. Elife 8, 48847. doi:10.7554/elife.48847
Meyer, K. D., Saletore, Y., Zumbo, P., Elemento, O., Mason, C. E., and Jaffrey, S. R. (2012). Comprehensive Analysis of mRNA Methylation Reveals Enrichment in 3' UTRs and Near Stop Codons. Cell 149 (7), 1635–1646. doi:10.1016/j.cell.2012.05.003
Miano, V., Codino, A., Pandolfini, L., and Barbieri, I. (2021). The Non-coding Epitranscriptome in Cancer. Brief. Funct. Genomics 20 (2), 94–105. doi:10.1093/bfgp/elab003
Mobet, Y., Liu, X., Liu, T., Yu, J., and Yi, P. (2022). Interplay between m6A RNA Methylation and Regulation of Metabolism in Cancer. Front Cel Dev Biol 10, 813581. doi:10.3389/fcell.2022.813581
Montanaro, L., Calienni, M., Bertoni, S., Rocchi, L., Sansone, P., Storci, G., et al. (2010). Novel Dyskerin-Mediated Mechanism of P53 Inactivation through Defective mRNA Translation. Cancer Res. 70 (11), 4767–4777. doi:10.1158/0008-5472.CAN-09-4024
Müller, S., (2019). IGF2BP1 Promotes SRF-dependent Transcription in Cancer in a m6A- and miRNA-dependent Manner. Nucleic Acids Res. 47 (1), 375–390. doi:10.1093/nar/gky1012
Ni, W., Yao, S., Zhou, Y., Liu, Y., Huang, P., Zhou, A., et al. (2019). Long Noncoding RNA GAS5 Inhibits Progression of Colorectal Cancer by Interacting with and Triggering YAP Phosphorylation and Degradation and Is Negatively Regulated by the m6A Reader YTHDF3. Mol. Cancer 18 (1), 143. doi:10.1186/s12943-019-1079-y
Nombela, P., Miguel-López, B., and Blanco, S. (2021). The Role of m6A, m5C and Ψ RNA Modifications in Cancer: Novel Therapeutic Opportunities. Mol. Cancer 20 (1), 18. doi:10.1186/s12943-020-01263-w
Pandolfini, L., Barbieri, I., Bannister, A. J., Hendrick, A., Andrews, B., Webster, N., et al. (2019). METTL1 Promotes Let-7 MicroRNA Processing via m7G Methylation. Mol. Cel 74 (6), 1278–e9. e9. doi:10.1016/j.molcel.2019.03.040
Paris, J., Morgan, M., Campos, J., Spencer, G. J., Shmakova, A., Ivanova, I., et al. (2019). Targeting the RNA m6A Reader YTHDF2 Selectively Compromises Cancer Stem Cells in Acute Myeloid Leukemia. Cell Stem Cell 25 (1), 137–e6. e6. doi:10.1016/j.stem.2019.03.021
Peng, W., Li, J., Chen, R., Gu, Q., Yang, P., Qian, W., et al. (2019). Upregulated METTL3 Promotes Metastasis of Colorectal Cancer via miR-1246/SPRED2/MAPK Signaling Pathway. J. Exp. Clin. Cancer Res. 38 (1), 393. doi:10.1186/s13046-019-1408-4
Penzo, M., Ludovini, V., Treré, D., Siggillino, A., Vannucci, J., Bellezza, G., et al. (2015). Dyskerin and TERC Expression May Condition Survival in Lung Cancer Patients. Oncotarget 6 (25), 21755–21760. doi:10.18632/oncotarget.4580
Rao, X., Lai, L., Li, X., Wang, L., Li, A., and Yang, Q. (2021). N6 -methyladenosine Modification of Circular RNA Circ-ARL3 Facilitates Hepatitis B Virus-Associated Hepatocellular Carcinoma via Sponging miR-1305. IUBMB Life 73 (2), 408–417. doi:10.1002/iub.2438
Relier, S., Ripoll, J., Guillorit, H., Amalric, A., Achour, C., Boissière, F., et al. (2021). FTO-mediated Cytoplasmic m6Am Demethylation Adjusts Stem-like Properties in Colorectal Cancer Cell. Nat. Commun. 12 (1), 1716. doi:10.1038/s41467-021-21758-4
Roslan, N. H., Makpol, S., and Mohd Yusof, Y. A. (2019). A Review on Dietary Intervention in Obesity Associated Colon Cancer. Asian Pac. J. Cancer Prev. 20 (5), 1309–1319. doi:10.31557/APJCP.2019.20.5.1309
Ruggero, D., Grisendi, S., Piazza, F., Rego, E., Mari, F., Rao, P. H., et al. (2003). Dyskeratosis Congenita and Cancer in Mice Deficient in Ribosomal RNA Modification. Science 299 (5604), 259–262. doi:10.1126/science.1079447
Saijo, Y., Sato, G., Usui, K., Sato, M., Sagawa, M., Kondo, T., et al. (2001). Expression of Nucleolar Protein P120 Predicts Poor Prognosis in Patients with Stage I Lung Adenocarcinoma. Ann. Oncol. 12 (8), 1121–1125. doi:10.1023/a:1011617707999
Shen, C., Xuan, B., Yan, T., Ma, Y., Xu, P., Tian, X., et al. (2020). m6A-dependent Glycolysis Enhances Colorectal Cancer progressionA-dependent Glycolysis Enhances Colorectal Cancer Progression. Mol. Cancer 19 (1), 72. doi:10.1186/s12943-020-01190-w
Shen, X. P., Ling, X., Lu, H., Zhou, C. X., Zhang, J. K., and Yu, Q. (2018). Low Expression of microRNA-1266 Promotes Colorectal Cancer Progression via Targeting FTO. Eur. Rev. Med. Pharmacol. Sci. 22 (23), 8220–8226. doi:10.26355/eurrev_201812_16516
Shi, H., Wei, J., and He, C. (2019). Where, When, and How: Context-dependent Functions of RNA Methylation Writers, Readers, and Erasers. Mol. Cel 74 (4), 640–650. doi:10.1016/j.molcel.2019.04.025
Shi, H., Zhang, X., Weng, Y. L., Lu, Z., Liu, Y., Lu, Z., et al. (2018). m6A Facilitates Hippocampus-dependent Learning and Memory through YTHDF1A Facilitates Hippocampus-dependent Learning and Memory through YTHDF1. Nature 563 (7730), 249–253. doi:10.1038/s41586-018-0666-1
Siegel, R. L., Miller, K. D., Goding Sauer, A., Fedewa, S. A., Butterly, L. F., Anderson, J. C., et al. (2020). Colorectal Cancer Statistics, 2020. CA Cancer J. Clin. 70 (3), 145–164. doi:10.3322/caac.21601
Sivanand, S., and Vander Heiden, M. G. (2020). Emerging Roles for Branched-Chain Amino Acid Metabolism in Cancer. Cancer Cell 37 (2), 147–156. doi:10.1016/j.ccell.2019.12.011
Sjöblom, T., Jone, S., Wood, L. D., Parsons, D. W., Lin, J., Barber, T. D., et al. (2006). The Consensus Coding Sequences of Human Breast and Colorectal Cancers. Science 314 (5797), 268–274. doi:10.1126/science.1133427
Skvortsova, K., Stirzaker, C., and Taberlay, P. (2019). The DNA Methylation Landscape in Cancer. Essays Biochem. 63 (6), 797–811. doi:10.1042/EBC20190037
Sloan, K. E., Warda, A. S., Sharma, S., Entian, K. D., Lafontaine, D. L. J., and Bohnsack, M. T. (2017). Tuning the Ribosome: The Influence of rRNA Modification on Eukaryotic Ribosome Biogenesis and Function. RNA Biol. 14 (9), 1138–1152. doi:10.1080/15476286.2016.1259781
Tanabe, A., Tanikawa, K., Tsunetomi, M., Takai, K., Ikeda, H., Konno, J., et al. (2016). RNA Helicase YTHDC2 Promotes Cancer Metastasis via the Enhancement of the Efficiency by Which HIF-1α mRNA Is Translated. Cancer Lett. 376 (1), 34–42. doi:10.1016/j.canlet.2016.02.022
Vu, L. P., Pickering, B. F., Cheng, Y., Zaccara, S., Nguyen, D., Minuesa, G., et al. (2017). The N6-Methyladenosine (m6A)-Forming Enzyme METTL3 Controls Myeloid Differentiation of normal Hematopoietic and Leukemia Cells. Nat. Med. 23 (11), 1369–1376. doi:10.1038/nm.4416
Wang, S., Gan, M., Chen, C., Zhang, Y., Kong, J., Zhang, H., et al. (2021). Methyl CpG Binding Protein 2 Promotes Colorectal Cancer Metastasis by Regulating N6 -methyladenosine Methylation through Methyltransferase-like 14. Cancer Sci. 112 (8), 3243–3254. doi:10.1111/cas.15011
Wang, Y., Li, Y., Yue, M., Wang, J., Kumar, S., Wechsler-Reya, R. J., et al. (2018). N6-methyladenosine RNA Modification Regulates Embryonic Neural Stem Cell Self-Renewal through Histone Modifications. Nat. Neurosci. 21 (2), 195–206. doi:10.1038/s41593-017-0057-1
Wang, Y., Lu, J. H., Wu, Q. N., Jin, Y., Wang, D. S., Chen, Y. X., et al. (2019). LncRNA LINRIS Stabilizes IGF2BP2 and Promotes the Aerobic Glycolysis in Colorectal Cancer. Mol. Cancer 18 (1), 174. doi:10.1186/s12943-019-1105-0
Weng, H., Huang, H., Wu, H., Qin, X., Zhao, B. S., Dong, L., et al. (2018). METTL14 Inhibits Hematopoietic Stem/Progenitor Differentiation and Promotes Leukemogenesis via mRNA m6A Modification. Cell Stem Cell 22 (2), 191–e9. e9. doi:10.1016/j.stem.2017.11.016
Wiener, D., and Schwartz, S. (2021). The Epitranscriptome beyond m6A. Nat. Rev. Genet. 22 (2), 119–131. doi:10.1038/s41576-020-00295-8
Wishart, D. (2022). Metabolomics and the Multi-Omics View of Cancer. Metabolites 12 (2), 154. doi:10.3390/metabo12020154
Woo, H. H., and Chambers, S. K. (2019). Human ALKBH3-Induced m1A Demethylation Increases the CSF-1 mRNA Stability in Breast and Ovarian Cancer Cells. Biochim. Biophys. Acta Gene Regul. Mech. 1862 (1), 35–46. doi:10.1016/j.bbagrm.2018.10.008
Wu, S., Li, T., Liu, W., and Huang, Y. (2021). Ferroptosis and Cancer: Complex Relationship and Potential Application of Exosomes. Front. Cel Dev Biol 9, 733751. doi:10.3389/fcell.2021.733751
Xiang, S., Liang, X., Yin, S., Liu, J., and Xiang, Z. (2020). N6-methyladenosine Methyltransferase METTL3 Promotes Colorectal Cancer Cell Proliferation through Enhancing MYC Expression. Am. J. Transl Res. 12 (5), 1789–1806.
Xiong, X., Li, X., and Yi, C. (2018). N1-methyladenosine Methylome in Messenger RNA and Non-coding RNA. Curr. Opin. Chem. Biol. 45, 179–186. doi:10.1016/j.cbpa.2018.06.017
Xu, D., Shao, J., Song, H., and Wang, J. (2020). The YTH Domain Family of N6-Methyladenosine "Readers" in the Diagnosis and Prognosis of Colonic Adenocarcinoma. Biomed. Res. Int. 2020, 9502560. doi:10.1155/2020/9502560
Xu, J., Chen, Q., Tian, K., Liang, R., Chen, T., Gong, A., et al. (2020). m6A Methyltransferase METTL3 Maintains colon Cancer Tumorigenicity by Suppressing SOCS2 to Promote Cell Proliferation. Oncol. Rep. 44 (3), 973–986. doi:10.3892/or.2020.7665
Yan, D., Tu, L., Yuan, H., Fang, J., Cheng, L., Zheng, X., et al. (2017). WBSCR22 Confers Oxaliplatin Resistance in Human Colorectal Cancer. Sci. Rep. 7 (1), 15443. doi:10.1038/s41598-017-15749-z
Yang, P., Wang, Q., Liu, A., Zhu, J., and Feng, J. (2020). ALKBH5 Holds Prognostic Values and Inhibits the Metastasis of Colon Cancer. Pathol. Oncol. Res. 26 (3), 1615–1623. doi:10.1007/s12253-019-00737-7
Yang, S., Wei, J., Cui, Y. H., Park, G., Shah, P., Deng, Y., et al. (2019). m6A mRNA Demethylase FTO Regulates Melanoma Tumorigenicity and Response to Anti-PD-1 blockadeA mRNA Demethylase FTO Regulates Melanoma Tumorigenicity and Response to Anti-PD-1 Blockade. Nat. Commun. 10 (1), 2782. doi:10.1038/s41467-019-10669-0
Yue, C., Chen, J., Li, Z., Li, L., Chen, J., and Guo, Y. (2020). microRNA-96 Promotes Occurrence and Progression of Colorectal Cancer via Regulation of the AMPKα2-FTO-m6A/MYC axis. J. Exp. Clin. Cancer Res. 39 (1), 240. doi:10.1186/s13046-020-01731-7
Zaccara, S., Ries, R. J., and Jaffrey, S. R. (2019). Reading, Writing and Erasing mRNA Methylation. Nat. Rev. Mol. Cel Biol 20 (10), 608–624. doi:10.1038/s41580-019-0168-5
Zhang, C., and Jia, G. (2018). Reversible RNA Modification N1-Methyladenosine (m1A) in mRNA and tRNA. Genomics Proteomics Bioinformatics 16 (3), 155–161. doi:10.1016/j.gpb.2018.03.003
Zhang, C., Samanta, D., Lu, H., Bullen, J. W., Zhang, H., Chen, I., et al. (2016). Hypoxia Induces the Breast Cancer Stem Cell Phenotype by HIF-dependent and ALKBH5-Mediated m⁶A-Demethylation of NANOG mRNA. Proc. Natl. Acad. Sci. U S A. 113 (14), E2047–E2056. doi:10.1073/pnas.1602883113
Zhang, J., Tsoi, H., Li, X., Wang, H., Gao, J., Wang, K., et al. (2016). Carbonic Anhydrase IV Inhibits colon Cancer Development by Inhibiting the Wnt Signalling Pathway through Targeting the WTAP-WT1-TBL1 axis. Gut 65 (9), 1482–1493. doi:10.1136/gutjnl-2014-308614
Zhang, S., Zhao, B. S., Zhou, A., Lin, K., Zheng, S., Lu, Z., et al. (2017). m6A Demethylase ALKBH5 Maintains Tumorigenicity of Glioblastoma Stem-like Cells by Sustaining FOXM1 Expression and Cell Proliferation ProgramA Demethylase ALKBH5 Maintains Tumorigenicity of Glioblastoma Stem-like Cells by Sustaining FOXM1 Expression and Cell Proliferation Program. Cancer Cell 31 (4), 591–e6. doi:10.1016/j.ccell.2017.02.013
Zhang, W., Eckwahl, M. J., Zhou, K. I., and Pan, T. (2019). Sensitive and Quantitative Probing of Pseudouridine Modification in mRNA and Long Noncoding RNA. Rna 25 (9), 1218–1225. doi:10.1261/rna.072124.119
Zhao, J., Lee, E. E., Kim, J., Yang, R., Chamseddin, B., Ni, C., et al. (2019). Transforming Activity of an Oncoprotein-Encoding Circular RNA from Human Papillomavirus. Nat. Commun. 10 (1), 2300. doi:10.1038/s41467-019-10246-5
Zhao, Y., Zhao, Q., Kaboli, P. J., Shen, J., Li, M., Wu, X., et al. (2019). m1A Regulated Genes Modulate PI3K/AKT/mTOR and ErbB Pathways in Gastrointestinal Cancer. Transl Oncol. 12 (10), 1323–1333. doi:10.1016/j.tranon.2019.06.007
Zheng, Y., Wang, Y., Liu, Y., Xie, L., Ge, J., Yu, G., et al. (2021). N6-Methyladenosine Modification of PTTG3P Contributes to Colorectal Cancer Proliferation via YAP1. Front. Oncol. 11, 669731. doi:10.3389/fonc.2021.669731
Zhou, D., Tang, W., Xu, Y., Xu, Y., Xu, B., Fu, S., et al. (2021). METTL3/YTHDF2 m6A axis Accelerates Colorectal Carcinogenesis through Epigenetically Suppressing YPEL5. Mol. Oncol. 15 (8), 2172–2184. doi:10.1002/1878-0261.12898
Zhou, J., Wan, J., Gao, X., Zhang, X., Jaffrey, S. R., and Qian, S. B. (2015). Dynamic M(6)A mRNA Methylation Directs Translational Control of Heat Shock Response. Nature 526 (7574), 591–594. doi:10.1038/nature15377
Zhou, X., Chang, Y., Zhu, L., Shen, C., Qian, J., and Chang, R. (2021). LINC00839/miR-144-3p/WTAP (WT1 Associated Protein) axis Is Involved in Regulating Hepatocellular Carcinoma Progression. Bioengineered 12 (2), 10849–10861. doi:10.1080/21655979.2021.1990578
Keywords: colorectal cancer, RNA modification, RNA methylation, N6-methyladenosine, 5-methylcytosine, N1-methyladenosine, 7-methylguanine, circular RNA
Citation: Liang W, Yi H, Mao C, Meng Q, Wu X, Li S and Xue J (2022) Research Progress of RNA Methylation Modification in Colorectal Cancer. Front. Pharmacol. 13:903699. doi: 10.3389/fphar.2022.903699
Received: 24 March 2022; Accepted: 19 April 2022;
Published: 03 May 2022.
Edited by:
Yongye Huang, Northeastern University, ChinaReviewed by:
Jiangbo Wei, The University of Chicago, United StatesCopyright © 2022 Liang, Yi, Mao, Meng, Wu, Li and Xue. This is an open-access article distributed under the terms of the Creative Commons Attribution License (CC BY). The use, distribution or reproduction in other forums is permitted, provided the original author(s) and the copyright owner(s) are credited and that the original publication in this journal is cited, in accordance with accepted academic practice. No use, distribution or reproduction is permitted which does not comply with these terms.
*Correspondence: Weizheng Liang, am1iYjEyMDNAMTI2LmNvbQ==; Jun Xue, eHVlanVuQGhlYmVpbnUuZWR1LmNu
Disclaimer: All claims expressed in this article are solely those of the authors and do not necessarily represent those of their affiliated organizations, or those of the publisher, the editors and the reviewers. Any product that may be evaluated in this article or claim that may be made by its manufacturer is not guaranteed or endorsed by the publisher.
Research integrity at Frontiers
Learn more about the work of our research integrity team to safeguard the quality of each article we publish.