- 1Department of Pharmacy, The Children’s Hospital, Zhejiang University School of Medicine, National Clinical Research Center for Child Health, Hangzhou, China
- 2Department of Tuina, The Third Affiliated Hospital of Zhejiang Chinese Medical University, Hangzhou, China
- 3Department of Critical Care Medicine, The Third Affiliated Hospital of Zhejiang Chinese Medical University, Hangzhou, China
- 4Department of Critical Care Medicine, Zhejiang Provincial People’s Hospital, People’s Hospital of Hangzhou Medical College, Hangzhou, China
The incidence of cerebral ischemia has increased in the past decades, and the high fatality and disability rates seriously affect human health. Apelin is a bioactive peptide and the ligand of the G protein-coupled receptor APJ. Both are ubiquitously expressed in the peripheral and central nervous systems, and regulate various physiological and pathological process in the cardiovascular, nervous and endocrine systems. Apelin-13 is one of the subtypes of apelin, and the apelin-13/APJ signaling pathway protects against cerebral ischemia by promoting angiogenesis, inhibiting excitotoxicity and stabilizing atherosclerotic plaques. In this review, we have discussed the role of apelin-13 in the regulation of cerebral ischemia and the underlying mechanisms, along with the therapeutic potential of the apelin-13/APJ signaling pathway in cerebral ischemia.
1 Introduction
Cerebral ischemia is a serious threat to human health, and is associated with high morbidity, disability and mortality. The rapidly aging population, as well as significant changes in lifestyle and diet brought about by the socio-economic development in China in recent years, has significantly increased the risk of stroke. It is currently the primary cause of death and disability among adults in China (Wang et al., 2015; Wang et al., 2017a; Guan et al., 2017). Therefore, it is crucial to devise suitable intervention methods in order to improve the prognosis of patients with cerebral ischemia and reduce the burden of disease.
Studies increasingly show that the apelin/apelin receptor (APJ) signaling pathway is involved in the occurrence and development of cerebral ischemia (Tables 1, 2). APJ is an orphan G protein-coupled receptor that was discovered by O'Dowd et al. (1993), and apelin is its endogenous ligand. The apelin/APJ system is ubiquitous in the peripheral and central nervous systems, and regulates blood pressure, myocardial contraction, immune response, angiogenesis, cancer development and other biological processes (Hosoya et al., 2000; Kawamata et al., 2001; Li et al., 2008; Barnes et al., 2010; Yang et al., 2016a). The currently known subtypes of apelin include apelin-12, apelin-13, apelin-17, apelin-28, and apelin-36, of which apelin-13 is the predominant subtype found in the heart, brain and hypothalamus. Previous studies have shown that apelin-13 plays an important role in cerebral ischemia (Duan et al., 2019; Wang et al., 2020) and ischemic stroke, and changes in the expression level of endogenous apelin-13 following ischemia has diagnostic and therapeutic relevance. Exogenous apelin-13 supplementation in ischemic stroke patients can play a neuroprotective role by regulating multiple signaling pathways. In this review, we have summarized the role and mechanism of apelin-13 in cerebral ischemia, in order to offer new insights into its diagnosis and treatment.
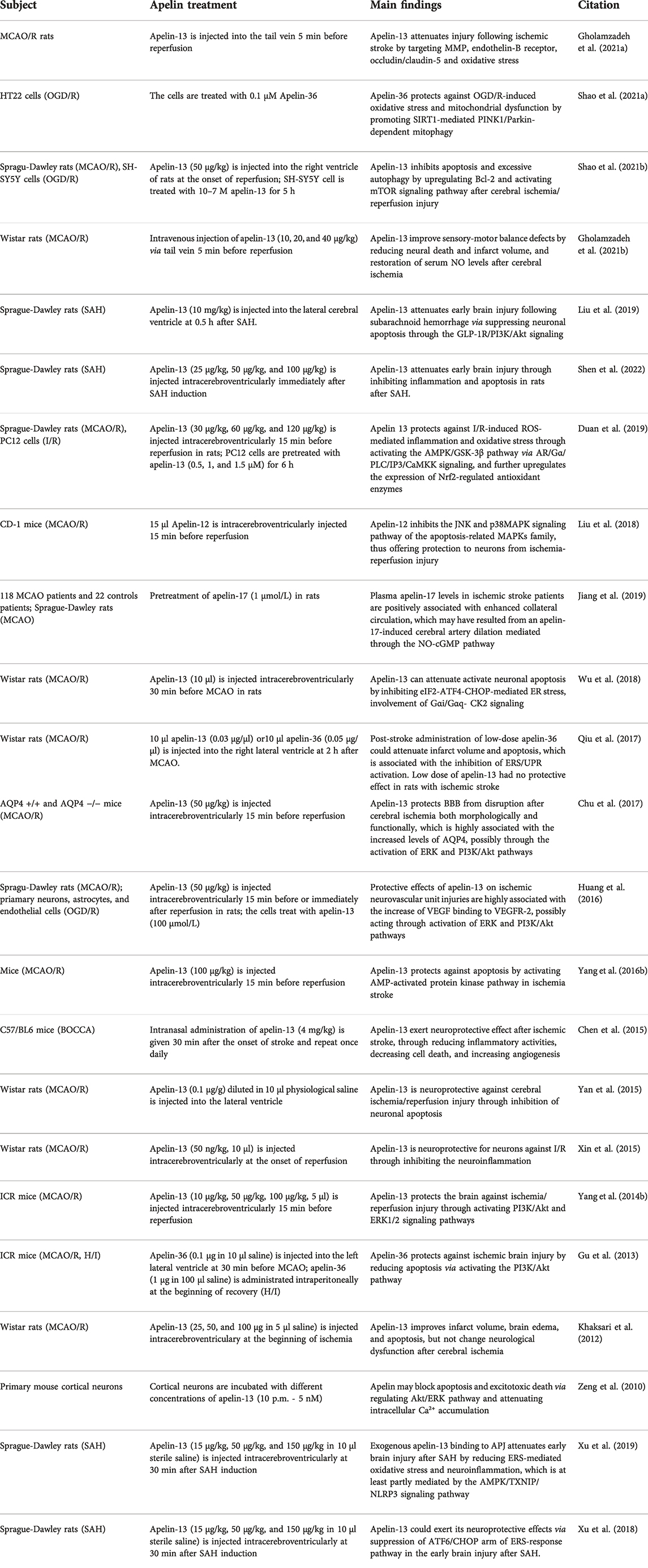
TABLE 2. The evidence from experimental trials demonstrating the role and mechanism of apelin in stroke.
2 Apelin-13
2.1 Biological characteristics of apelin-13
The gene encoding the arginine and lysin-rich apelin precursor peptide is located on chromosome Xg 25–26.1, and consists of three exons and two introns. The precursor peptide contains multiple potential sites of post-translational enzymatic processing, and can therefore generate multiple active apelin peptide fragments. For instance, cleavage of the apelin precursor peptide by angiotensin converting enzyme 2 (ACE2) generates isoforms of varying lengths such as aplein-12, apelin-13, apelin-17, and apelin-36. These isoforms differ in terms of tissue distribution, physiological and pharmacological effects, and binding strength with APJ (Lee et al., 2000; Reaux et al., 2001; De Mota et al., 2004). Furthermore, the biological activity of apelin, especially involving receptor binding and intracellular receptor transport, is greatly influenced by the size of the molecular fragment. Smaller apelin isoforms typically display stronger binding to APJ (Kleinz and Davenport, 2005; Carpéné et al., 2007).
Apelin-13 is a short peptide consisting of 13 amino acids. The N-terminal of apelin-13 binds to the APJ receptor, while the C-terminal is mainly involved in regulating its biological activity (Kawamata et al., 2001; Medhurst et al., 2003). It is degraded into an inactive form in the presence of ACE2 (Vickers et al., 2002), and is also modified into the more stable and active pyroglutamyl apelin-13. Studies show that pyroglutamyl apelin-13 is the most biological relevant subtype of apelin present in healthy human plasma (Mesmin et al., 2010; Zhen et al., 2013). Nevertheless, apelin-13 is ubiquitously expressed in the digestive system, cardiovascular system, central nervous system (CNS), kidneys, adipose tissue and retina, whereas apelin pro-peptide is predominantly present in the heart, lungs, kidneys and endothelial cells of large blood vessels (O'Carroll et al., 2000). Furthermore, apelin mRNA has been detected in the mammary glands, heart, lungs, brain, kidneys and other tissues of rats. Based on these findings, we can surmise that apelin has wide-ranging functions in humans as well as rodents. In particular, the presence of apelinergic neurons in the brain suggests that apelin may regulate food intake and digestion, pituitary hormone release and circadian rhythms (Reaux et al., 2002).
2.2 The APJ receptor
The apelin receptor APJ, also known as angiotensin II (Ang II) receptor-like 1, is a G protein-coupled receptor consisting of 380 amino acids with seven transmembrane structures. It is currently the only known apelin-13 receptor so far, and is highly expressed in neurons and glial cytoplasm in caudate nucleus, corpus callosum and hippocampus (Hosoya et al., 2000; Medhurst et al., 2003). APJ relays the signals through Gα subunit (Gαi or Gαq) of G protein. The structure of APJ is similar to that of the Ang II type I (AT1) receptor, although it cannot bind to Ang II (O'Dowd et al., 1993). In addition, G protein-independent signaling pathways are also involved in the activation of the apelin/APJ system. Upon binding to apelin, APJ is activated and recruits G protein-coupled receptor kinases (GRKs), resulting in APJ phosphorylation. The inhibitor protein (β-arrestin) then rapidly binds to APJ, resulting in receptor desensitization, and activation of the G protein-independent signaling pathways (Chen et al., 2014; Chen et al., 2020).
2.3 The tissue distribution pattern of apelin-13 and APJ
Apelin 13 is widely distributed in the CNS, with high expression levels in neurons and oligodendrocytes, and relatively lower expression in the astrocytes. Apelin 13 mRNA has been detected in the spinal cord, brain stem, cerebral cortex, hypothalamus, cerebellum, striatum, and hippocampus (O'Carroll et al., 2000). The differential expression pattern of apelin 13 and APJ in the CNS is indicative of multiple physiological or pathological functions. Both APJ and apelin are highly expressed in the hypothalamus, the master regulator of the neuroendocrine and humoral balance. The co-localization of apelin and hypothalamic arginine vasopressin (AVP) neurons suggests that apelin may regulate body fluid balance, feeding and drinking behavior and the HPA axis by interacting with AVP (De Mota et al., 2000; Reaux-Le Goazigo et al., 2004). In addition, the distribution of apelin in hypothalamus and pituitary region also indicates that apelin may be involved in the regulation of neurological and adenohypophysial hormones (Brailoiu et al., 2002; Yang et al., 2019).
Several studies have shown that apelin and APJ are highly expressed in the cardiovascular system, and can enhance myocardial contraction, reduce cardiac load, dilate blood vessels, promote angiogenesis, and regulate cardiac electrical conduction (Maguire et al., 2009; Aydin et al., 2014; Yu et al., 2014). Interestingly, the apelin/APJ system is also expressed in the cerebral blood vessels, and regulates vascular function. For instance, some studies have demonstrated that apelin can promote vasodilation in cerebral vessels (Nagano et al., 2019; Mughal et al., 2020). Mughal et al. (2018) found that apelin inhibits nitric oxide (NO)-dependent relaxation of cerebral arteries by activating APJ and inhibiting large-conductance, calcium-activated K channel in cerebral arterial smooth muscle cells, partially via a PI3K-dependent mechanism (Modgil et al., 2013). In addition, apelin promotes development of new blood branches from preexisting cerebral vessels following ischemic stroke (Han et al., 2015; Hiramatsu et al., 2017; Wu et al., 2017). Jiang et al. (2019) correlated the increased levels of plasma apelin-17 in ischemic stroke patients with enhanced collateral circulation, which can be attributed to cerebral artery dilation induced by apelin-17 via regulating the NO-cGMP pathway.
2.4 The neuroprotective effects of apelin 13
There is ample evidence demonstrating the neuroprotective effects of apelin-13. It can protect neuronal cells against apoptosis and excitotoxic injury by inhibiting NMDA-induced intracellular Ca2+ accumulation, oxidative stress, mitochondrial damage, cytochrome C release and caspase-3 activation via the ERK1/2 signaling pathway (Zeng et al., 2010). In addition, one study showed that supraspinal administration of apelin-13 in mice induced antinociception via the opioid receptor (Xu et al., 2009). The same group reported that apelin-13 relieved acetic acid-induced visceral pain in mice when injected into the subarachnoid space, and this analgesic effect was blocked by opioid receptor antagonists (Lv et al., 2012). Similarly, Hajimashhadi et al. (2017) demonstrated that intrathecal injection of apelin-13 increased the autonomic activity and relieved signs of pain in rats with spinal cord injury. However, one study showed that peripheral administration of apelin-13 reduced the latency of painful stimuli and enhanced pain sensitivity in a dose- and time-dependent manner (Canpolat et al., 2016), and intrathecal administration of ML221, an APJ antagonist, transiently reduced chronic constriction injury-induced pain hypersensitivity (Xiong et al., 2017). These findings suggest that the spinal apelin/APJ system may drive neuropathic pain. Thus, the regulatory effects of apelin-13 on pain may depend on the route of administration, as well as the type and degree of pain, and needs further clarification.
Previous studies have shown that apelin-13 can enhance the consolidation of passive avoidance learning and memory in mice, and these protective effects are neutralized by antagonists of α-adrenergic, cholinergic, dopamine, 5-hydroxytryptophan and γ -aminobutyric acid receptors, as well as inhibitors of nitric oxide synthesis (Telegdy et al., 2013). In a mouse model of chronic stress-induced memory deficit, apelin-13 significantly improved the cognition of new objects and memory deficit of Y maze, likely through to the upregulation of BDNF (Shen et al., 2019). In addition, exogenous apelin-13 attenuated cisplatin-induced cognitive dysfunction by activating the BDNF/TrkB signaling pathway and suppressing neuroinflammation. Apelin-13 is also known to relieve the symptoms of anxiety in mice, and these anti-anxiety effects may be related to α, ß adrenergic, dopamine and 5-HT receptors since they were blocked by the administration of phenbenzamine, haloperidol, propranolol, and dimethylergometrine (Telegdy and Jászberényi, 2014). Apelin-13 also reversed depression-like behavior in rats subjected to chronic social defeat stress and chronic water immersion restraint stress by regulating microglial polarization, and ameliorating a dysfunctional HPA axis and hippocampal glucocorticoid receptor (Dai et al., 2018; Tian et al., 2018; Zhou et al., 2020).
A clinical study on 126 patients with severe TBI and 126 healthy controls found that lower serum level of apelin-13 in the patients correlated significantly with increased severity of TBI, and was an independent predictor of short-term mortality, indicating that serum apelin-13 is a promising prognostic biomarker for severe TBI (Zhuang et al., 2021). The protective effects of apelin-13 in TBI are associated with inhibition of autophagy (Bao et al., 2015), suppression of neuronal apoptosis through the GLP-1R/PI3K/Akt signaling (Liu et al., 2019), and mitigation of blood-brain barrier (BBB) destruction and brain edema (Bao et al., 2016a). Early brain injury (EBI) is at present considered to the key determinant of the neurological function and clinical outcomes of subarachnoid hemorrhage (SAH) (Sehba et al., 2012; Fujii et al., 2013). Apelin-13 can attenuate EBI by inhibiting neuronal apoptosis and degeneration, and reducing the release of inflammatory cytokines such as TNF-α and IL-1β in the CSF. These protective effects were neutralized upon administration of the APJ inhibitor ML221 (Shen et al., 2022). The anti-apoptosis effect of apelin-13 in SAH may be related to the activation of the GLP-1R/PI3K/Akt signaling pathway (Liu et al., 2019). Xu et al. (2019) found that exogenous apelin-13 can alleviate EBI by suppressing endoplasmic reticulum (ER) stress-induced NLRP3 inflammasome activation and oxidative stress after SAH. Furthermore, the APJ inhibitor dorsomorphine reversed the neuroprotective effects of apelin-13 in SAH. Another study by Xu et al. (2018) confirmed that apelin-13 reduced neuronal apoptosis and prevented BBB disruption after SAH, and eventually improved EBI by alleviating ER stress partly via the ATF6/CHOP pathway. Intracerebral hemorrhage (ICH) shares certain pathological characteristics with SAH. Intracerebroventricular administration of apelin-13 improved motor function and brain edema after ICH by reducing neuronal death, which demonstrates its therapeutic potential (Bao et al., 2016b).
3 Apelin-13 and cerebral ischemia
The apelin/APJ system is closely associated with the pathogenesis of ischemic stroke, which is currently the most common cerebrovascular disease. Clinical studies suggest that apelin is related to the diagnosis and prognosis of cerebral ischemia, while studies in animal and cellular models indicate that exogenous apelin-13 can effectively reduce infarct volume and cerebral edema, and improve neurological function after cerebral ischemia.
3.1 Clinical studies
In a follow-up cohort study, Wang et al. (2017b) found that the variant rs9943582 of APJ gene was not significantly associated with ischemic stroke in the Chinese Han population. Consistent with this finding, another clinical study reported that the variant rs9943582 was not associated with the age at onset and clinical outcomes of ischemic stroke (Zhang et al., 2017). However, other clinical studies have reported contradictory findings. One study conducted in China on 244 AIS patients recruited within 24 h of stroke onset and 167 healthy controls showed that serum apelin-13 levels were lower in the patients compared to the healthy controls. In addition, patients with NIHSS score ≤3 had higher apelin-13 levels than those with NIHSS score >3. Low apelin-13 level in the patients was associated with death or major disability within 3-months, whereas patients with high apelin-13 levels showed a lower incidence of stroke and combined events after 1-year. These findings indicated that serum apelin-13 is a potential prognostic biomarker for acute ischemic stroke (Wang et al., 2020). Another clinical study demonstrated that higher apelin levels were associated with increased risk of stroke (including ischemic and hemorrhagic stroke) (Yu et al., 2021). Intravenous thrombolytic therapy (ITT) is commonly used to treat acute ischemic stroke, although it can enhance the risk of hemorrhagic transformation (HT). To analyze the predictive significance of apelin on HT in acute ischemic stroke patients after ITT, Zhu et al. (2021) analyzed the data of 109 acute ischemic stroke patients that received ITT, and found that a higher HT grade was associated with lower apelin level and increased levels of interleukin-1β (IL-1β) and IL-6. Moreover, lower apelin was also related with a higher risk of death of patients with both ischemic stroke and HT, indicating that apelin is an independent protective factor in stroke patients. Atrial fibrillation (AF) is associated with a high risk of stroke, and should therefore be detected in a timely manner. Bohm et al. (2021) showed that apelin levels were significantly lower in stroke patients with AF compared to the non-AF group in a multicenter, matched-cohort, and only apelin was identified as an independent predictor of AF. Thus, apelin administration should be considered in patients with high risk of stroke to exclude the possibility of AF. However, another clinical trial shown that apelin level did not differ between stroke patients and healthy individuals, and was not associated with cardiovascular mortality and morbidity during follow-up. This discrepancy can be attributed to differences in sample size and patients selection, and measurement assays for apelin. Further large-scale multicenter clinical trials are needed, along with detailed subgroup analysis, to clarify the therapeutic value of apelin in stroke.
3.2 Mechanistic investigation
3.2.1 Apelin-13 protects against blood-brain barrier disruption after cerebral ischemia
The BBB controls the exchange of substances between blood and brain tissue, allows nutrients to pass and prevents harmful substances from entering, thereby protecting the CNS. Given that the secondary injuries after cerebral ischemia is closely related to the morphological and structural destruction of BBB, protecting the integrity of the latter and alleviating cerebral edema are increasingly being considered as treatment options for ischemic stroke (Huang et al., 2020; Parvez et al., 2022). Apelin-13 can reduce BBB permeability and brain vasogenic edema after ischemia by mitigating oxidative stress, and inhibiting the expression of matrix metalloproteinases (MMP) and endothelin-B receptor (Gholamzadeh et al., 2021a). Furthermore, the protective effect of apelin-13 on BBB post-stroke is significantly associated with the elevated expression of aquaporin-4 (AQP4), which is partly achieved by activating the extracellular signal-regulated kinase (ERK) and PI3K/Akt pathways (Chu et al., 2017). Several factors are involved in the destruction of BBB after ischemic stroke, such as inflammatory cytokines, microvessel and endothelial cell injury, and the degradation of extracellular matrix. It remains to be explored how apeline-13 affects these pathological pathways.
3.2.2 Apelin-13 promotes angiogenesis after cerebral ischemia
The apelin/APJ system plays an important role in embryonic vascular development and adult angiogenesis (Cox et al., 2006). Both APJ and apelin are expressed in retinal vascular endothelial cells, and apelin promotes the proliferation and chemotaxis of these cells, as well as formation of capillary tubes. In addition, the apelin/APJ system may also be involved in endothelial cell proliferation and neovascularization (Tao et al., 2010; Zhang et al., 2013). Apelin-13 can promote proliferation, migration and tube formation in myocardial microvascular endothelial cells, as well as angiogenesis via modulation of AMPK and Akt signaling (Yang et al., 2014a). Knocking out APJ in glioblastoma cells reduced tumor growth and angiogenesis, suggesting that targeting the apelin/APJ system is a promising strategy for preventing angiogenesis in glioblastoma (Amoozgar et al., 2019; Frisch et al., 2020).
Apelin-13 plays an important role in the formation of collateral circulation. A clinical trial demonstrated that apelin-13 was significantly increased in patients with moyamoya disease compared to those with middle cerebral artery occlusion independent of NO and VEGF. Given that moyamoya disease has better collateral circulation compared to ischemic stroke, high plasma levels of apelin may be indicative of good collateral circulation (Wu et al., 2022). Furthermore, intranasal administration of apelin-13 increased the number of new vessels in the area surrounding infarction, restored the local cerebral blood flow, and promoted long-term functional recovery by upregulating vascular endothelial growth factor (VEGF) and MMP-9 (Chen et al., 2015). Apelin-13 can protect neurovascular units from ischemic injury by increasing the expression of VEGF and VEGFR2, and promoting VEGF binding to VEGFR-2 by activating the ERK and PI3K/Akt pathways (Huang et al., 2016). Cerebral blood flow blockade is often accompanied by hypoxia, which activates the apelin/APJ system, and consequently promotes endothelial cell proliferation via the PI3K/Akt and MAPK signaling pathways (Zhang et al., 2015; Zhang et al., 2016).
3.2.3 Apelin-13 inhibits excitotoxicity after cerebral ischemia
Aspartic acid, glutamate and glycine are excitatory neurotransmitters that are mainly distributed in the synaptic terminals of neurons in the CNS. The main excitatory amino acid released after cerebral ischemia is glutamate, which binds to the excitatory amino acid receptors on the postsynaptic membrane, resulting in neurotoxicity and neuronal damage. Nerve cells are rich in NMDA, which mediates the excitotoxicity of glutamate. Glutamate activates NMDA receptors and triggers a massive Ca2+ influx through the specific ion channel, resulting in intracellular calcium overload in the early stage of ischemia, and eventually cell death (Hossmann, 1994; Lai et al., 2014). Apelin-13 can reduce NMDA activity by directly reducing the ion flow potential of the NMDA receptor membrane. In addition, apelin-13 also inhibits NMDA in a dose-dependent manner by activating the pro-survival Ca2+, IP3, PKC, MEK-1/2, Akt, and Raf/ERK-1/2 signaling pathways, thereby antagonizing the excitotoxicity effects of glutamate and alleviating neuron injury (Cook et al., 2011; O'Donnell et al., 2007). Another experimental study established that apelin protects against NMDA-induced retinal neuronal death via APJ receptor by activating Akt and ERK1/2, and downregulating TNF-α (Ishimaru et al., 2017). Zeng et al. (2010) showed that apelin-13 can prevent serum deprivation-induced changes in Akt and ERK1/2 phosphorylation, and attenuate NMDA-induced intracellular Ca2+ accumulation, which in turn inhibits apoptosis and excitotoxic death.
3.2.4 Apelin-13 promotes the stability of atherosclerotic plaques
Apelin-13 has been implicated in atherosclerosis in several studies on account of its immunoreactivity in human aortas and coronary arteries. Furthermore, apelin/APJ expression patterns are inversely correlated to human aortic and coronary atherosclerosis (Kostopoulos et al., 2014). In addition, serum apelin levels are negatively correlated with the severity of arterial stenosis, and positively correlated with the stability of atherosclerotic plaques, indicating its value as a potential biomarker of atherosclerotic plaque stability (Zhou et al., 2014). Consistent with this, a clinical trial conducted on 235 (114 black, 121 white) rheumatoid arthritis patients showed that apelin concentration in the serum was associated with altered levels of plaque stability mediators (MMP-2, MMP-9) and atherosclerosis, in a manner partly dependent on population origin and systemic inflammatory status (Gunter et al., 2017). A recent study showed that the apelin/APJ system is involved in the development of atherosclerosis by influencing vascular smooth muscle cells (Luo et al., 2018). Moreover, apelin is up-regulated in human atherosclerotic coronary artery and localized to the plaque along with macrophages and smooth muscle cells (Pitkin et al., 2010). Another study confirmed that apelin-13 significantly improves plaque stability by increasing collagen content and decreasing MMP-9 expression, reducing inflammatory cell infiltration (neutrophils and macrophages) and intracellular reactive oxygen species (ROS) content (Fraga-Silva et al., 2018). Furthermore, PINK1/Parkin-mediated mitophagy promotes apelin-13-induced vascular smooth muscle cell proliferation by AMPKα and exacerbates atherosclerotic lesions (He et al., 2019).
4 Summary and prospects
The apelin-13/APJ signaling axis is ubiquitous in the peripheral and central nervous systems. Apelin-13 is an endogenous neuroprotective molecule that regulates various physiological and pathological processes in the brain. Following cerebral ischemia, apelin-13 promotes angiogenesis, increases the stability of atherosclerotic plaques and reduces excitatory toxicity, thereby improving prognosis. At present, little is known regarding the function of the apelin-13/APJ pathway, and its mechanisms have not been clarified. To this end, we first need to clarify the biological functions and mechanism of apelin-13/APJ signaling in cerebral ischemia, and the long-term effects of activating this pathway. Secondly, novel APJ receptor agonists or antagonists have to be developed to verify the feasibility and efficacy of the apelin-13/APJ system as an intervention target in ischemic stroke. In addition, the variation loci related to the apelin/APJ system, their relationship with brain structure and function, and their impact on the prognosis of cerebral ischemia also need to be elucidated. Finally, the injection route, injection time and treatment frequency of apelin-13 in pre-clinical studies need to be optimized before clinical studies on ischemic stroke patients.
Author contributions
All the authors participated in discussing the literature. JC and YY supervised the research, JL and ZC wrote and revised the manuscript. All authors read and approved the final manuscript.
Conflict of interest
The authors declare that the research was conducted in the absence of any commercial or financial relationships that could be construed as a potential conflict of interest.
Publisher’s note
All claims expressed in this article are solely those of the authors and do not necessarily represent those of their affiliated organizations, or those of the publisher, the editors and the reviewers. Any product that may be evaluated in this article, or claim that may be made by its manufacturer, is not guaranteed or endorsed by the publisher.
References
Amoozgar, Z., Jain, R. K., and Duda, D. G. (2019). Role of apelin in glioblastoma vascularization and invasion after anti-VEGF therapy: What is the impact on the immune system? Cancer Res. 79 (9), 2104–2106. doi:10.1158/0008-5472.CAN-19-0749
Aydin, S., Eren, M. N., Sahin, I., and Aydin, S. (2014). The role of apelins in the physiology of the heart. Protein Pept. Lett. 21 (1), 2–9. doi:10.2174/09298665113209990083
Bao, H. J., Qiu, H. Y., Kuai, J. X., Song, C. J., Wang, S. X., Wang, C. Q., et al. (2016). Apelin-13 as a novel target for intervention in secondary injury after traumatic brain injury. Neural Regen. Res. 11 (7), 1128–1133. doi:10.4103/1673-5374.187049
Bao, H. J., Zhang, L., Han, W. C., and Dai, D. K. (2015). Apelin-13 attenuates traumatic brain injury-induced damage by suppressing autophagy. Neurochem. Res. 40 (1), 89–97. doi:10.1007/s11064-014-1469-x
Bao, H., Yang, X., Huang, Y., Qiu, H., Huang, G., Xiao, H., et al. (2016). The neuroprotective effect of apelin-13 in a mouse model of intracerebral hemorrhage. Neurosci. Lett. 628, 219–224. doi:10.1016/j.neulet.2016.06.046
Barnes, G., Japp, A. G., and Newby, D. E. (2010). Translational promise of the apelin--APJ system. Heart 96 (13), 1011–1016. doi:10.1136/hrt.2009.191122
Bohm, A., Snopek, P., Tothova, L., Bezak, B., Jajcay, N., Vachalcova, M., et al. (2021). Association between apelin and atrial fibrillation in patients with high risk of ischemic stroke. Front. Cardiovasc. Med. 8, 742601. doi:10.3389/fcvm.2021.742601
Brailoiu, G. C., Dun, S. L., Yang, J., Ohsawa, M., Chang, J. K., and Dun, N. J. (2002). Apelin-immunoreactivity in the rat hypothalamus and pituitary. Neurosci. Lett. 327 (3), 193–197. doi:10.1016/s0304-3940(02)00411-1
Canpolat, S., Ozcan, M., Saral, S., Kalkan, O. F., and Ayar, A. (2016). Effects of apelin-13 in mice model of experimental pain and peripheral nociceptive signaling in rat sensory neurons. J. Recept. Signal Transduct. Res. 36 (3), 243–247. doi:10.3109/10799893.2015.1080274
Carpéné, C., Dray, C., Attané, C., Valet, P., Portillo, M. P., Churruca, I., et al. (2007). Expanding role for the apelin/APJ system in physiopathology. J. Physiol. Biochem. 63 (4), 358–373. doi:10.1007/bf03165767
Chen, D., Lee, J., Gu, X., Wei, L., and Yu, S. P. (2015). Intranasal delivery of apelin-13 is neuroprotective and promotes angiogenesis after ischemic stroke in mice. ASN Neuro 7 (5), 1759091415605114. doi:10.1177/1759091415605114
Chen, J., Chen, X., Li, S., Jiang, Y., Mao, H., Zhang, R., et al. (2020). Individual phosphorylation sites at the C-terminus of the apelin receptor play different roles in signal transduction. Redox Biol. 36, 101629. doi:10.1016/j.redox.2020.101629
Chen, X., Bai, B., Tian, Y., Du, H., and Chen, J. (2014). Identification of serine 348 on the apelin receptor as a novel regulatory phosphorylation site in apelin-13-induced G protein-independent biased signaling. J. Biol. Chem. 289 (45), 31173–31187. doi:10.1074/jbc.M114.574020
Chu, H., Yang, X., Huang, C., Gao, Z., Tang, Y., and Dong, Q. (2017). Apelin-13 protects against ischemic blood-brain barrier damage through the effects of aquaporin-4. Cerebrovasc. Dis. 44 (1-2), 10–25. doi:10.1159/000460261
Cook, D. R., Gleichman, A. J., Cross, S. A., Doshi, S., Ho, W., Jordan-Sciutto, K. L., et al. (2011). NMDA receptor modulation by the neuropeptide apelin: Implications for excitotoxic injury. J. Neurochem. 118 (6), 1113–1123. doi:10.1111/j.1471-4159.2011.07383.x
Cox, C. M., D'Agostino, S. L., Miller, M. K., Heimark, R. L., and Krieg, P. A. (2006). Apelin, the ligand for the endothelial G-protein-coupled receptor, APJ, is a potent angiogenic factor required for normal vascular development of the frog embryo. Dev. Biol. 296 (1), 177–189. doi:10.1016/j.ydbio.2006.04.452
Dai, T. T., Wang, B., Xiao, Z. Y., You, Y., and Tian, S. W. (2018). Apelin-13 upregulates BDNF against chronic stress-induced depression-like phenotypes by ameliorating HPA Axis and hippocampal glucocorticoid receptor dysfunctions. Neuroscience 390, 151–159. doi:10.1016/j.neuroscience.2018.08.018
De Mota, N., Lenkei, Z., and Llorens-Cortès, C. (2000). Cloning, pharmacological characterization and brain distribution of the rat apelin receptor. Neuroendocrinology 72 (6), 400–407. doi:10.1159/000054609
De Mota, N., Reaux-Le Goazigo, A., El Messari, S., Chartrel, N., Roesch, D., Dujardin, C., et al. (2004). Apelin, a potent diuretic neuropeptide counteracting vasopressin actions through inhibition of vasopressin neuron activity and vasopressin release. Proc. Natl. Acad. Sci. U. S. A. 101 (28), 10464–10469. doi:10.1073/pnas.0403518101
Duan, J., Cui, J., Yang, Z., Guo, C., Cao, J., Xi, M., et al. (2019). Neuroprotective effect of Apelin 13 on ischemic stroke by activating AMPK/GSK-3β/Nrf2 signaling. J. Neuroinflammation 16 (1), 24. doi:10.1186/s12974-019-1406-7
Fraga-Silva, R. A., Seeman, H., Montecucco, F., da Silva, A. R., Burger, F., Costa-Fraga, F. P., et al. (2018). Apelin-13 treatment enhances the stability of atherosclerotic plaques. Eur. J. Clin. Invest. 48 (3), e12891. doi:10.1111/eci.12891
Frisch, A., Kälin, S., Monk, R., Radke, J., Heppner, F. L., and Kälin, R. E. (2020). Apelin controls angiogenesis-dependent glioblastoma growth. Int. J. Mol. Sci. 21 (11), E4179. doi:10.3390/ijms21114179
Fujii, M., Yan, J., Rolland, W. B., Soejima, Y., Caner, B., and Zhang, J. H. (2013). Early brain injury, an evolving frontier in subarachnoid hemorrhage research. Transl. Stroke Res. 4 (4), 432–446. doi:10.1007/s12975-013-0257-2
Gholamzadeh, R., Aboutaleb, N., and Nazarinia, D. (2021). Intravenous injection of apelin-13 improves sensory-motor balance deficits caused by cerebral ischemic reperfusion injury in male wistar rats via restoration of nitric oxide. J. Chem. Neuroanat. 112, 101886. doi:10.1016/j.jchemneu.2020.101886
Gholamzadeh, R., Ramezani, F., Tehrani, P. M., and Aboutaleb, N. (2021). Apelin-13 attenuates injury following ischemic stroke by targeting matrix metalloproteinases (MMP), endothelin- B receptor, occludin/claudin-5 and oxidative stress. J. Chem. Neuroanat. 118, 102015. doi:10.1016/j.jchemneu.2021.102015
Gu, Q., Zhai, L., Feng, X., Chen, J., Miao, Z., Ren, L., et al. (2013). Apelin-36, a potent peptide, protects against ischemic brain injury by activating the PI3K/Akt pathway. Neurochem. Int. 63 (6), 535–540. doi:10.1016/j.neuint.2013.09.017
Guan, T., Ma, J., Li, M., Xue, T., Lan, Z., Guo, J., et al. (2017). Rapid transitions in the epidemiology of stroke and its risk factors in China from 2002 to 2013. Neurology 89 (1), 53–61. doi:10.1212/WNL.0000000000004056
Gunter, S., Solomon, A., Tsang, L., Woodiwiss, A. J., Robinson, C., Millen, A. M., et al. (2017). Apelin concentrations are associated with altered atherosclerotic plaque stability mediator levels and atherosclerosis in rheumatoid arthritis. Atherosclerosis 256, 75–81. doi:10.1016/j.atherosclerosis.2016.11.024
Hajimashhadi, Z., Aboutaleb, N., and Nasirinezhad, F. (2017). Chronic administration of [Pyr(1)] apelin-13 attenuates neuropathic pain after compression spinal cord injury in rats. Neuropeptides 61, 15–22. doi:10.1016/j.npep.2016.08.010
Han, L., Li, J., Chen, Y., Zhang, M., Qian, L., Chen, Y., et al. (2015). Human urinary kallidinogenase promotes angiogenesis and cerebral perfusion in experimental stroke. PLoS One 10 (7), e0134543. doi:10.1371/journal.pone.0134543
He, L., Zhou, Q., Huang, Z., Xu, J., Zhou, H., Lv, D., et al. (2019). PINK1/Parkin-mediated mitophagy promotes apelin-13-induced vascular smooth muscle cell proliferation by AMPKα and exacerbates atherosclerotic lesions. J. Cell. Physiol. 234 (6), 8668–8682. doi:10.1002/jcp.27527
Hiramatsu, M., Hishikawa, T., Tokunaga, K., Kidoya, H., Nishihiro, S., Haruma, J., et al. (2017). Combined gene therapy with vascular endothelial growth factor plus apelin in a chronic cerebral hypoperfusion model in rats. J. Neurosurg. 127 (3), 679–686. doi:10.3171/2016.8.JNS16366
Hosoya, M., Kawamata, Y., Fukusumi, S., Fujii, R., Habata, Y., Hinuma, S., et al. (2000). Molecular and functional characteristics of APJ. Tissue distribution of mRNA and interaction with the endogenous ligand apelin. J. Biol. Chem. 275 (28), 21061–21067. doi:10.1074/jbc.M908417199
Hossmann, K. A. (1994). Glutamate-mediated injury in focal cerebral ischemia: The excitotoxin hypothesis revised. Brain Pathol. 4 (1), 23–36. doi:10.1111/j.1750-3639.1994.tb00808.x
Huang, C., Dai, C., Gong, K., Zuo, H., and Chu, H. (2016). Apelin-13 protects neurovascular unit against ischemic injuries through the effects of vascular endothelial growth factor. Neuropeptides 60, 67–74. doi:10.1016/j.npep.2016.08.006
Huang, Q., Wang, X., Lin, X., Zhang, J., You, X., and Shao, A. (2020). The role of transient receptor potential channels in blood-brain barrier dysfunction after ischemic stroke. Biomed. Pharmacother. 131, 110647. doi:10.1016/j.biopha.2020.110647
Ishimaru, Y., Sumino, A., Kajioka, D., Shibagaki, F., Yamamuro, A., Yoshioka, Y., et al. (2017). Apelin protects against NMDA-induced retinal neuronal death via an APJ receptor by activating Akt and ERK1/2, and suppressing TNF-α expression in mice. J. Pharmacol. Sci. 133 (1), 34–41. doi:10.1016/j.jphs.2016.12.002
Jiang, W., Hu, W., Ye, L., Tian, Y., Zhao, R., Du, J., et al. (2019). Contribution of apelin-17 to collateral circulation following cerebral ischemic stroke. Transl. Stroke Res. 10 (3), 298–307. doi:10.1007/s12975-018-0638-7
Kadoglou, N. P., Fotiadis, G., Lambadiari, V., Maratou, E., Dimitriadis, G., and Liapis, C. D. (2014). Serum levels of novel adipokines in patients with acute ischemic stroke: Potential contribution to diagnosis and prognosis. Peptides 57, 12–16. doi:10.1016/j.peptides.2014.04.008
Kawamata, Y., Habata, Y., Fukusumi, S., Hosoya, M., Fujii, R., Hinuma, S., et al. (2001). Molecular properties of apelin: Tissue distribution and receptor binding. Biochim. Biophys. Acta 1538 (2-3), 162–171. doi:10.1016/s0167-4889(00)00143-9
Khaksari, M., Aboutaleb, N., Nasirinezhad, F., Vakili, A., and Madjd, Z. (2012). Apelin-13 protects the brain against ischemic reperfusion injury and cerebral edema in a transient model of focal cerebral ischemia. J. Mol. Neurosci. 48 (1), 201–208. doi:10.1007/s12031-012-9808-3
Kleinz, M. J., and Davenport, A. P. (2005). Emerging roles of apelin in biology and medicine. Pharmacol. Ther. 107 (2), 198–211. doi:10.1016/j.pharmthera.2005.04.001
Kostopoulos, C. G., Spiroglou, S. G., Varakis, J. N., Apostolakis, E., and Papadaki, H. H. (2014). Adiponectin/T-cadherin and apelin/APJ expression in human arteries and periadventitial fat: Implication of local adipokine signaling in atherosclerosis? Cardiovasc. Pathol. 23 (3), 131–138. doi:10.1016/j.carpath.2014.02.003
Lai, T. W., Zhang, S., and Wang, Y. T. (2014). Excitotoxicity and stroke: Identifying novel targets for neuroprotection. Prog. Neurobiol. 115, 157–188. doi:10.1016/j.pneurobio.2013.11.006
Lee, D. K., Cheng, R., Nguyen, T., Fan, T., Kariyawasam, A. P., Liu, Y., et al. (2000). Characterization of apelin, the ligand for the APJ receptor. J. Neurochem. 74 (1), 34–41. doi:10.1046/j.1471-4159.2000.0740034.x
Li, F., Li, L., Qin, X., Pan, W., Feng, F., Chen, F., et al. (2008). Apelin-induced vascular smooth muscle cell proliferation: The regulation of cyclin D1. Front. Biosci. 13, 3786–3792. doi:10.2741/2967
Liu, D. R., Hu, W., and Chen, G. Z. (2018). Apelin-12 exerts neuroprotective effect against ischemia-reperfusion injury by inhibiting JNK and P38MAPK signaling pathway in mouse. Eur. Rev. Med. Pharmacol. Sci. 22 (12), 3888–3895. doi:10.26355/eurrev_201806_15273
Liu, Y., Zhang, T., Wang, Y., Wu, P., Li, Y., Wang, C., et al. (2019). Apelin-13 attenuates early brain injury following subarachnoid hemorrhage via suppressing neuronal apoptosis through the GLP-1R/PI3K/Akt signaling. Biochem. Biophys. Res. Commun. 513 (1), 105–111. doi:10.1016/j.bbrc.2019.03.151
Luo, X., Liu, J., Zhou, H., and Chen, L. (2018). Apelin/APJ system: A critical regulator of vascular smooth muscle cell. J. Cell. Physiol. 233 (7), 5180–5188. doi:10.1002/jcp.26339
Lv, S. Y., Qin, Y. J., Wang, N. B., Yang, Y. J., and Chen, Q. (2012). Supraspinal antinociceptive effect of apelin-13 in a mouse visceral pain model. Peptides 37 (1), 165–170. doi:10.1016/j.peptides.2012.06.007
Maguire, J. J., Kleinz, M. J., Pitkin, S. L., and Davenport, A. P. (2009). [Pyr1]apelin-13 identified as the predominant apelin isoform in the human heart: Vasoactive mechanisms and inotropic action in disease. Hypertension 54 (3), 598–604. doi:10.1161/HYPERTENSIONAHA.109.134619
Medhurst, A. D., Jennings, C. A., Robbins, M. J., Davis, R. P., Ellis, C., Winborn, K. Y., et al. (2003). Pharmacological and immunohistochemical characterization of the APJ receptor and its endogenous ligand apelin. J. Neurochem. 84 (5), 1162–1172. doi:10.1046/j.1471-4159.2003.01587.x
Mesmin, C., Dubois, M., Becher, F., Fenaille, F., and Ezan, E. (2010). Liquid chromatography/tandem mass spectrometry assay for the absolute quantification of the expected circulating apelin peptides in human plasma. Rapid Commun. Mass Spectrom. 24 (19), 2875–2884. doi:10.1002/rcm.4718
Modgil, A., Guo, L., O'Rourke, S. T., and Sun, C. (2013). Apelin-13 inhibits large-conductance Ca2+-activated K+ channels in cerebral artery smooth muscle cells via a PI3-kinase dependent mechanism. PLoS One 8 (12), e83051. doi:10.1371/journal.pone.0083051
Mughal, A., Anto, S., Sun, C., and O'Rourke, S. T. (2020). Apelin inhibits an endothelium-derived hyperpolarizing factor-like pathway in rat cerebral arteries. Peptides 132, 170350. doi:10.1016/j.peptides.2020.170350
Mughal, A., Sun, C., and OʼRourke, S. T. (2018). Apelin reduces nitric oxide-induced relaxation of cerebral arteries by inhibiting activation of large-conductance, calcium-activated K channels. J. Cardiovasc. Pharmacol. 71 (4), 223–232. doi:10.1097/FJC.0000000000000563
Nagano, K., Kwon, C., Ishida, J., Hashimoto, T., Kim, J. D., Kishikawa, N., et al. (2019). Cooperative action of APJ and α1A-adrenergic receptor in vascular smooth muscle cells induces vasoconstriction. J. Biochem. 166 (5), 383–392. doi:10.1093/jb/mvz071
O'Carroll, A. M., Selby, T. L., Palkovits, M., and Lolait, S. J. (2000). Distribution of mRNA encoding B78/apj, the rat homologue of the human APJ receptor, and its endogenous ligand apelin in brain and peripheral tissues. Biochim. Biophys. Acta 1492 (1), 72–80. doi:10.1016/s0167-4781(00)00072-5
O'Donnell, L. A., Agrawal, A., Sabnekar, P., Dichter, M. A., Lynch, D. R., and Kolson, D. L. (2007). Apelin, an endogenous neuronal peptide, protects hippocampal neurons against excitotoxic injury. J. Neurochem. 102 (6), 1905–1917. doi:10.1111/j.1471-4159.2007.04645.x
O'Dowd, B. F., Heiber, M., Chan, A., Heng, H. H., Tsui, L. C., Kennedy, J. L., et al. (1993). A human gene that shows identity with the gene encoding the angiotensin receptor is located on chromosome 11. Gene 136 (1-2), 355–360. doi:10.1016/0378-1119(93)90495-o
Parvez, S., Kaushik, M., Ali, M., Alam, M. M., Ali, J., Tabassum, H., et al. (2022). Dodging blood brain barrier with "nano" warriors: Novel strategy against ischemic stroke. Theranostics 12 (2), 689–719. doi:10.7150/thno.64806
Pitkin, S. L., Maguire, J. J., Kuc, R. E., and Davenport, A. P. (2010). Modulation of the apelin/APJ system in heart failure and atherosclerosis in man. Br. J. Pharmacol. 160 (7), 1785–1795. doi:10.1111/j.1476-5381.2010.00821.x
Qiu, J., Wang, X., Wu, F., Wan, L., Cheng, B., Wu, Y., et al. (2017). Low dose of apelin-36 attenuates ER stress-associated apoptosis in rats with ischemic stroke. Front. Neurol. 8, 556. doi:10.3389/fneur.2017.00556
Reaux, A., De Mota, N., Skultetyova, I., Lenkei, Z., El Messari, S., Gallatz, K., et al. (2001). Physiological role of a novel neuropeptide, apelin, and its receptor in the rat brain. J. Neurochem. 77 (4), 1085–1096. doi:10.1046/j.1471-4159.2001.00320.x
Reaux, A., Gallatz, K., Palkovits, M., and Llorens-Cortes, C. (2002). Distribution of apelin-synthesizing neurons in the adult rat brain. Neuroscience 113 (3), 653–662. doi:10.1016/s0306-4522(02)00192-6
Reaux-Le Goazigo, A., Morinville, A., Burlet, A., Llorens-Cortes, C., and Beaudet, A. (2004). Dehydration-induced cross-regulation of apelin and vasopressin immunoreactivity levels in magnocellular hypothalamic neurons. Endocrinology 145 (9), 4392–4400. doi:10.1210/en.2004-0384
Sehba, F. A., Hou, J., Pluta, R. M., and Zhang, J. H. (2012). The importance of early brain injury after subarachnoid hemorrhage. Prog. Neurobiol. 97 (1), 14–37. doi:10.1016/j.pneurobio.2012.02.003
Shao, Z., Dou, S., Zhu, J., Wang, H., Xu, D., Wang, C., et al. (2021). Apelin-36 protects HT22 cells against oxygen-glucose deprivation/reperfusion-induced oxidative stress and mitochondrial dysfunction by promoting SIRT1-mediated PINK1/parkin-dependent mitophagy. Neurotox. Res. 39 (3), 740–753. doi:10.1007/s12640-021-00338-w
Shao, Z. Q., Dou, S. S., Zhu, J. G., Wang, H. Q., Wang, C. M., Cheng, B. H., et al. (2021). Apelin-13 inhibits apoptosis and excessive autophagy in cerebral ischemia/reperfusion injury. Neural Regen. Res. 16 (6), 1044–1051. doi:10.4103/1673-5374.300725
Shen, P., Yue, Q., Fu, W., Tian, S. W., and You, Y. (2019). Apelin-13 ameliorates chronic water-immersion restraint stress-induced memory performance deficit through upregulation of BDNF in rats. Neurosci. Lett. 696, 151–155. doi:10.1016/j.neulet.2018.11.051
Shen, X., Yuan, G., Li, B., Cao, C., Cao, D., Wu, J., et al. (2022). Apelin-13 attenuates early brain injury through inhibiting inflammation and apoptosis in rats after experimental subarachnoid hemorrhage. Mol. Biol. Rep. 49 (3), 2107–2118. doi:10.1007/s11033-021-07028-y
Tao, Y., Lu, Q., Jiang, Y. R., Qian, J., Wang, J. Y., Gao, L., et al. (2010). Apelin in plasma and vitreous and in fibrovascular retinal membranes of patients with proliferative diabetic retinopathy. Invest. Ophthalmol. Vis. Sci. 51 (8), 4237–4242. doi:10.1167/iovs.09-4466
Telegdy, G., Adamik, A., and Jászberényi, M. (2013). Involvement of neurotransmitters in the action of apelin-13 on passive avoidance learning in mice. Peptides 39, 171–174. doi:10.1016/j.peptides.2012.10.011
Telegdy, G., and Jászberényi, M. (2014). Transmitter mediation of the anxiolytic action of apelin-13 in male mice. Behav. Brain Res. 263, 198–202. doi:10.1016/j.bbr.2014.01.009
Tian, S. W., Xu, F., and Gui, S. J. (2018). Apelin-13 reverses memory impairment and depression-like behavior in chronic social defeat stressed rats. Peptides 108, 1–6. doi:10.1016/j.peptides.2018.08.009
Vickers, C., Hales, P., Kaushik, V., Dick, L., Gavin, J., Tang, J., et al. (2002). Hydrolysis of biological peptides by human angiotensin-converting enzyme-related carboxypeptidase. J. Biol. Chem. 277 (17), 14838–14843. doi:10.1074/jbc.M200581200
Wang, J., An, Z., Li, B., Yang, L., Tu, J., Gu, H., et al. (2015). Increasing stroke incidence and prevalence of risk factors in a low-income Chinese population. Neurology 84 (4), 374–381. doi:10.1212/WNL.0000000000001175
Wang, P., Wang, C., Li, S., Wang, B., Xiong, L., Tu, X., et al. (2017). Lack of association between the APLNR variant rs9943582 with ischemic stroke in the Chinese Han GeneID population. Oncotarget 8 (64), 107678–107684. doi:10.18632/oncotarget.22588
Wang, W., Jiang, B., Sun, H., Ru, X., Sun, D., Wang, L., et al. (2017). Prevalence, incidence, and mortality of stroke in China: Results from a nationwide population-based survey of 480 687 adults. Circulation 135 (8), 759–771. doi:10.1161/CIRCULATIONAHA.116.025250
Wang, X., Tian, X., Pei, L. L., Niu, P. P., Guo, Y., Hu, R., et al. (2020). The association between serum apelin-13 and the prognosis of acute ischemic stroke. Transl. Stroke Res. 11 (4), 700–707. doi:10.1007/s12975-019-00769-w
Wu, F., Qiu, J., Fan, Y., Zhang, Q., Cheng, B., Wu, Y., et al. (2018). Apelin-13 attenuates ER stress-mediated neuronal apoptosis by activating Gα(i)/Gα(q)-CK2 signaling in ischemic stroke. Exp. Neurol. 302, 136–144. doi:10.1016/j.expneurol.2018.01.006
Wu, H., Xia, C., Li, R., Tao, C., Tang, Q., and Hu, W. (2022). Correlation between apelin and collateral circulation in patients with middle cerebral artery occlusion and moyamoya disease. Int. J. Gen. Med. 15, 699–709. doi:10.2147/IJGM.S341015
Wu, L., Chen, L., and Li, L. (2017). Apelin/APJ system: A novel promising therapy target for pathological angiogenesis. Clin. Chim. Acta. 466, 78–84. doi:10.1016/j.cca.2016.12.023
Xin, Q., Cheng, B., Pan, Y., Liu, H., Yang, C., Chen, J., et al. (2015). Neuroprotective effects of apelin-13 on experimental ischemic stroke through suppression of inflammation. Peptides 63, 55–62. doi:10.1016/j.peptides.2014.09.016
Xiong, Q., He, W., Wang, H., Zhou, J., Zhang, Y., He, J., et al. (2017). Effect of the spinal apelin-APJ system on the pathogenesis of chronic constriction injury-induced neuropathic pain in rats. Mol. Med. Rep. 16 (2), 1223–1231. doi:10.3892/mmr.2017.6734
Xu, N., Wang, H., Fan, L., and Chen, Q. (2009). Supraspinal administration of apelin-13 induces antinociception via the opioid receptor in mice. Peptides 30 (6), 1153–1157. doi:10.1016/j.peptides.2009.02.011
Xu, W., Gao, L., Li, T., Zheng, J., Shao, A., and Zhang, J. (2018). Apelin-13 alleviates early brain injury after subarachnoid hemorrhage via suppression of endoplasmic reticulum stress-mediated apoptosis and blood-brain barrier disruption: Possible involvement of ATF6/CHOP pathway. Neuroscience 388, 284–296. doi:10.1016/j.neuroscience.2018.07.023
Xu, W., Li, T., Gao, L., Zheng, J., Yan, J., Zhang, J., et al. (2019). Apelin-13/APJ system attenuates early brain injury via suppression of endoplasmic reticulum stress-associated TXNIP/NLRP3 inflammasome activation and oxidative stress in a AMPK-dependent manner after subarachnoid hemorrhage in rats. J. Neuroinflammation 16 (1), 247. doi:10.1186/s12974-019-1620-3
Yan, X. G., Cheng, B. H., Wang, X., Ding, L. C., Liu, H. Q., Chen, J., et al. (2015). Lateral intracerebroventricular injection of Apelin-13 inhibits apoptosis after cerebral ischemia/reperfusion injury. Neural Regen. Res. 10 (5), 766–771. doi:10.4103/1673-5374.157243
Yang, N., Li, T., Cheng, J., Tuo, Q., and Shen, J. (2019). Role of apelin/APJ system in hypothalamic-pituitary axis. Clin. Chim. Acta. 499, 149–153. doi:10.1016/j.cca.2019.09.011
Yang, X., Zhu, W., Zhang, P., Chen, K., Zhao, L., Li, J., et al. (2014). Apelin-13 stimulates angiogenesis by promoting cross-talk between AMP-activated protein kinase and Akt signaling in myocardial microvascular endothelial cells. Mol. Med. Rep. 9 (5), 1590–1596. doi:10.3892/mmr.2014.1984
Yang, Y., Lv, S. Y., Ye, W., and Zhang, L. (2016). Apelin/APJ system and cancer. Clin. Chim. Acta. 457, 112–116. doi:10.1016/j.cca.2016.04.001
Yang, Y., Zhang, X., Cui, H., Zhang, C., Zhu, C., and Li, L. (2014). Apelin-13 protects the brain against ischemia/reperfusion injury through activating PI3K/Akt and ERK1/2 signaling pathways. Neurosci. Lett. 568, 44–49. doi:10.1016/j.neulet.2014.03.037
Yang, Y., Zhang, X. J., Li, L. T., Cui, H. Y., Zhang, C., Zhu, C. H., et al. (2016). Apelin-13 protects against apoptosis by activating AMP-activated protein kinase pathway in ischemia stroke. Peptides 75, 96–100. doi:10.1016/j.peptides.2015.11.002
Yu, D., Huang, B., Wu, B., and Xiao, J. (2021). Association of serum vaspin, apelin, and visfatin levels and stroke risk in a Chinese case-control study. Med. Baltim. 100 (12), e25184. doi:10.1097/MD.0000000000025184
Yu, X. H., Tang, Z. B., Liu, L. J., Qian, H., Tang, S. L., Zhang, D. W., et al. (2014). Apelin and its receptor APJ in cardiovascular diseases. Clin. Chim. Acta. 428, 1–8. doi:10.1016/j.cca.2013.09.001
Zeng, X. J., Yu, S. P., Zhang, L., and Wei, L. (2010). Neuroprotective effect of the endogenous neural peptide apelin in cultured mouse cortical neurons. Exp. Cell. Res. 316 (11), 1773–1783. doi:10.1016/j.yexcr.2010.02.005
Zhang, H., Sun, L., Wang, H., Cai, H., Niu, G., Bai, Y., et al. (2017). A study of GWAS-supported variants of rs9943582 in a Chinese han population with ischemic stroke: No associations with disease onset and clinical outcomes. J. Stroke Cerebrovasc. Dis. 26 (10), 2294–2299. doi:10.1016/j.jstrokecerebrovasdis.2017.05.013
Zhang, J., Liu, Q., Fang, Z., Hu, X., Huang, F., Tang, L., et al. (2016). Hypoxia induces the proliferation of endothelial progenitor cells via upregulation of Apelin/APLNR/MAPK signaling. Mol. Med. Rep. 13 (2), 1801–1806. doi:10.3892/mmr.2015.4691
Zhang, J., Liu, Q., Hu, X., Fang, Z., Huang, F., Tang, L., et al. (2015). Apelin/APJ signaling promotes hypoxia-induced proliferation of endothelial progenitor cells via phosphoinositide-3 kinase/Akt signaling. Mol. Med. Rep. 12 (3), 3829–3834. doi:10.3892/mmr.2015.3866
Zhang, Y., Jiang, Y. R., Lu, Q., Yin, H., and Tao, Y. (2013). Apelin in epiretinal fibrovascular membranes of patients with retinopathy of prematurity and the changes after intravitreal bevacizumab. Retina 33 (3), 613–620. doi:10.1097/IAE.0b013e31826d3a76
Zhen, E. Y., Higgs, R. E., and Gutierrez, J. A. (2013). Pyroglutamyl apelin-13 identified as the major apelin isoform in human plasma. Anal. Biochem. 442 (1), 1–9. doi:10.1016/j.ab.2013.07.006
Zhou, S., Chen, S., Xie, W., Guo, X., and Zhao, J. (2020). Microglia polarization of hippocampus is involved in the mechanism of Apelin-13 ameliorating chronic water immersion restraint stress-induced depression-like behavior in rats. Neuropeptides 81, 102006. doi:10.1016/j.npep.2020.102006
Zhou, Y., Wang, Y., and Qiao, S. (2014). Apelin: A potential marker of coronary artery stenosis and atherosclerotic plaque stability in ACS patients. Int. Heart J. 55 (3), 204–212. doi:10.1536/ihj.13-234
Zhu, B., Yan, L., Ren, H., Li, Q., and Chen, X. (2021). Predictive value of apelin and vaspin on hemorrhagic transformation in patients with acute ischemic stroke after intravenous thrombolysis and analysis of related factors. Evid. Based. Complement. Altern. Med. 2021, 5020622. doi:10.1155/2021/5020622
Keywords: apelin-13, APJ, cerebral ischemia, pathway, angiogenesis, atherosclerotic plaque, excitotoxicity
Citation: Li J, Chen Z, Chen J and Yu Y (2022) The beneficial roles of apelin-13/APJ system in cerebral ischemia: Pathogenesis and therapeutic strategies. Front. Pharmacol. 13:903151. doi: 10.3389/fphar.2022.903151
Received: 24 March 2022; Accepted: 18 July 2022;
Published: 10 August 2022.
Edited by:
Anwen Shao, Zhejiang University, ChinaReviewed by:
Liang Wu, Wenzhou Medical University, ChinaStephen O’Rourke, North Dakota State University, United States
Copyright © 2022 Li, Chen, Chen and Yu. This is an open-access article distributed under the terms of the Creative Commons Attribution License (CC BY). The use, distribution or reproduction in other forums is permitted, provided the original author(s) and the copyright owner(s) are credited and that the original publication in this journal is cited, in accordance with accepted academic practice. No use, distribution or reproduction is permitted which does not comply with these terms.
*Correspondence: Jingyu Chen, 532688835@qq.com; Yue Yu, 253279940@qq.com
†These authors have contributed equally to this work