- 1Institute of Functional Genomics (IGF), University of Montpellier, CNRS, INSERM, Montpellier, France
- 2School of Medicine, Zhejiang University City College, Hangzhou, China
- 3Center for Laser Microscopy, Institute of Physiology and Biochemistry “Ivan Djaja”, University of Belgrade, Faculty of Biology, Belgrade, Serbia
- 4Department of Life Sciences, University of Belgrade, Institute for Multidisciplinary Research, Belgrade, Serbia
- 5Department of Neurophysiology, University of Belgrade, Institute for Biological Research Siniša Stanković, National Institute of Republic of Serbia, Belgrade, Serbia
Epilepsy and multiple sclerosis (MS), two of the most common neurological diseases, are characterized by the establishment of inflammatory environment in the central nervous system that drives disease progression and impacts on neurodegeneration. Current therapeutic approaches in the treatments of epilepsy and MS are targeting neuronal activity and immune cell response, respectively. However, the lack of fully efficient responses to the available treatments obviously shows the need to search for novel therapeutic candidates that will not exclusively target neurons or immune cells. Accumulating knowledge on epilepsy and MS in humans and analysis of relevant animal models, reveals that astrocytes are promising therapeutic candidates to target as they participate in the modulation of the neuroinflammatory response in both diseases from the initial stages and may play an important role in their development. Indeed, astrocytes respond to reactive immune cells and contribute to the neuronal hyperactivity in the inflamed brain. Mechanistically, these astrocytic cell to cell interactions are fundamentally mediated by the purinergic signalling and involve metabotropic P2Y1 receptors in case of astrocyte interactions with neurons, while ionotropic P2X7 receptors are mainly involved in astrocyte interactions with autoreactive immune cells. Herein, we review the potential of targeting astrocytic purinergic signalling mediated by P2Y1 and P2X7 receptors to develop novel approaches for treatments of epilepsy and MS at very early stages.
Introduction
Neuroinflammation, an inflammatory response within the central nervous system (CNS), orchestrated by the cross-talk between CNS and immune system is now considered a key player in different neurological disorders, including epilepsy and multiple sclerosis (MS). It is characterized by the recruitment of immune cells to the CNS and also by a transformation of astrocytes and microglia into reactive profiles (Ransohoff, 2016; Yang and Zhou, 2019). These events have the primary role to maintain homeostasis by inducing the production and release of cytokines and chemokines that favour cell growth and survival. However, sustainment of inflammatory pathways leads to cellular dysfunctions actively contributing to the development and progression of chronic CNS disease (Vezzani et al., 2011; Xanthos and Sandkuhler, 2014; Yang and Zhou, 2019).
Epilepsy, with its incidence of ∼1%, represents one of the most common neurological disorders (Thijs et al., 2019). Evidence from both human patients and animal models demonstrated that neuroinflammation is not only a consequence but is actively involved in epileptogenesis and in seizure generation (ictogenesis) (Ravizza et al., 2008; Prabowo et al., 2013). For a long time, the study of epilepsy has been characterized by a neuro-centric view focused on the imbalance between excitatory and inhibitory transmissions that leads to neuronal hyperexcitability (Naylor, 2010; Bonansco and Fuenzalida, 2016). For this reason, current therapeutic strategies target mostly neuronal receptors and voltage-gated ion channels (Bialer et al., 2018). Epilepsy treatment however, remains still a challenge considering that ∼30% of patients do not respond to current antiseizure drugs (Janmohamed et al., 2020).
MS is a chronic inflammatory disease of the CNS, affecting 2.8 million people worldwide (Baecher-Allan et al., 2018). Understanding of MS pathology is characterized by the immuno-centric view focused on the immune cell infiltration into the CNS leading to the neuroinflammation and development of the multifocal inflammation, oligodendrocyte loss, demyelination, and axonal degeneration (Baecher-Allan et al., 2018; Dobson and Giovannoni, 2019). Current therapeutic strategies for MS treatment thus mostly focus on preventing and limiting immune cells trafficking into the CNS and modifying the immune cells responses (De Jager and Hafler, 2007; Rommer et al., 2019). However, immune cell-mediated inflammatory mechanisms alone, cannot explain neuronal degeneration without the CNS-resident cells component.
Great part in maintaining an optimal milieu for proper neuronal functioning rests with astrocytes. Indeed, astrocytes are positioned between blood vessels and synapses which allows them to fulfil metabolic and homeostatic maintenance functions (Parpura and Verkhratsky, 2012). This strategic location engages astrocytes in crosstalk with neurons, microglia, oligodendrocytes, other astrocytes, immune cells and blood vessels. Several studies have shown that neuroinflammation promoted astrogliosis changes the interaction between astrocytes and other cell types and alters the excitability of neuronal networks (Araque et al., 2014; Colombo and Farina, 2016; Vezzani et al., 2019; Sanz and Garcia-Gimeno, 2020; Audinat and Rassendren, 2021). Purinergic signalling, mediated by ATP and its breakdown products, is crucial in maintaining this astrocytic cell-to-cell communication (Franke et al., 2012) and increasing evidence have drawn considerable attention to an important role of astrocytic purinergic receptors (PRs) in epilepsy and MS from the initial stages of diseases (Narcisse et al., 2005; Dona et al., 2009; Franke and Illes, 2014; Rassendren and Audinat, 2016; Amadio et al., 2017; Brambilla, 2019; Domercq and Matute, 2019; Illes, 2020; Nikolic et al., 2020; Sidoryk-Wegrzynowicz and Struzynska, 2021). This opens possibility to target astrocytic purinergic signalling to develop non-neuronal and non-immune cell based therapeutic strategies for treatment of epilepsy and MS, respectively. These strategies would aim at better control of epilepsy and MS progression and even serve as a diseases-modifying entry points.
Considering the possibility to identify novel purine-based astrocyte-targeting treatments for epilepsy and MS, we here discuss the impact of astrocyte intercellular communication mediated by purinergic signalling on the pathogenic mechanisms underlying these two diseases. We focus on specific P2Rs, namely P2Y1R and P2X7R, which have been recently identified as key astrocyte receptors involved in epilepsy and MS, respectively.
At the Interface of Astrocyte Purinergic Signalling and Neuroinflammation Properties of Astrocyte Purinergic Signalling
ATP is a predominant extracellular signalling molecule that mediates signalling among astrocytes, and between astrocytes and other cell types. In response to various physiological and pathological stimuli, astrocytes can release ATP through different ways, including exocytosis and large conductance channels, such as hemichannels, pannexin channels, volume-regulated anion channels and P2X7Rs (Coco et al., 2003; Franke et al., 2012; Bijelic et al., 2020). Furthermore, released ATP is rapidly converted into adenosine by a set of ectonucleotidases. Extracellular levels of adenosine are also controlled by astrocytes through the action of nucleoside membrane transporters and of the enzyme adenosine kinase (ADK) (Boison, 2012). Purine and its metabolites activate PRs, classified as adenosine P1Rs, and ATP/ADP P2Rs that are expressed in astrocytes and in a variety of cell types (Franke et al., 2012; Burnstock, 2020). P2Rs are divided into the cationic ion channel P2XRs and the G-protein-coupled P2YRs. Activation by purines (ATP, ADP, UDP) induces increase in intracellular Ca2+ in astrocytes: from extracellular sources for P2XRs and from intracellular sites for P2YRs (Burnstock, 2016).
Astrocyte Purinergic Signalling Regulates Neuronal Activity
We and others have shown that selective photo-stimulation of hippocampal astrocytes expressing the light-sensitive Channelrhodopsin 2 (ChR2), increases Ca2+ signalling in astrocytes and subsequently triggers ATP release. This ATP excites inhibitory interneurons through the activation of P2Y1Rs but also activates P2Y1Rs expressed by astrocytes (Shen et al., 2017; Tan et al., 2017). This leads to the release of Ca2+ from intracellular stores and triggers glutamate release from astrocytes (Shen et al., 2017). In turn, this glutamate activates postsynaptic NMDA receptors on principal neurons and presynaptic metabotropic glutamate receptors (mGluR) in CA1 or presynaptic NMDA receptors in the dentate gyrus (Shen et al., 2017; Nikolic et al., 2018). Eventually, the degradation of ATP into adenosine leads to a delayed inhibition of principal neurons through the activation of adenosine A1Rs (Shen et al., 2017; Tan et al., 2017).
ATP as an Inflammatory Signal and Astrogliosis
Besides its role in astrocyte intercellular communication, ATP may be an important signal contributing to astrogliosis, a common hallmark of neuropathological conditions that affects the physiological functions of astrocytes and leads to the appearance of new ones (Sofroniew, 2014; Escartin et al., 2019). Reactive astrocytes undergo morphological, molecular and functional changes (Sofroniew, 2020; Escartin et al., 2021) in diseases whereby context (pathogenic trigger, age, brain area, etc.) is important in determining reactive astrocyte phenotype and its consequences on the outcome of a disease (Anderson et al., 2016; Bonansco and Fuenzalida, 2016; Rothhammer et al., 2016; John Lin et al., 2017; Liddelow et al., 2017; Hartmann et al., 2019; Guttenplan et al., 2020; Borggrewe et al., 2021; Diaz-Castro et al., 2021; Escartin et al., 2021). Astrogliosis in vivo is observed after direct infusion of ATP or ADP (Hindley et al., 1994) or its structural analogue 2-methylthio ATP (2-MeSATP) in the brain (Franke et al., 1999), and in vitro after astrocyte challenge by ATP (Brambilla and Abbracchio, 2001) as measured by the hypertrophy of astrocytes and an increase in the glial fibrillary acidic protein (GFAP) immunoreactivity. These studies also showed that in vivo administration of P2Rs antagonists, suramine, reactive blue 2 and pyridoxal-phosphate-6-azophenyl-2, 4-disulphonic acid (PPADS) counteracted astrogliosis. Moreover, activation of P2Rs is linked to the transduction pathways that involve astrocyte production of various chemokines and cytokines, inflammatory mediators that all stimulate neuroinflammation (Franke et al., 2012). In context of epilepsy and MS, purine-mediated signalling could therefore be implicated in the appearance of reactive astrocytes and deregulation of their main homeostatic functions. Indeed, persistent astrogliosis and release of pro-inflammatory factors by reactive astrocytes can contribute to the BBB disruption that commonly occurs in epilepsy, and alterations in the two main astrocyte functions, such as potassium and glutamate buffering, leading to hyperexcitability (Abbott, 2000; Friedman et al., 2009; Marchi et al., 2014; Iori et al., 2016; Smith et al., 2018). Likewise, in MS purine-mediated signalling could be involved in appearance of reactive astrocytes with neurotoxic activities that release numerous inflammatory mediators that are responsible for the recruitment of peripheral inflammatory cells, activation of microglia and promotion of oligodendrocyte loss and demyelination (Schonrock et al., 2000; Stadelmann et al., 2002; Linker et al., 2010; Argaw et al., 2012; Prins et al., 2014; Rothhammer and Quintana, 2015; Brambilla, 2019; Wheeler and Quintana, 2019; Linnerbauer et al., 2020; Colombo et al., 2021).
Astrocyte Purinergic Signalling Alterations and Their Impact on Epilepsy and MS
An enhancement in purinergic signalling is a common feature of different neuropathological conditions including epilepsy and MS (Franke et al., 2012; Rassendren and Audinat, 2016; Domercq and Matute, 2019). Considering that purinergic signalling is at the basis of astrocyte intercellular communication and associated with a reactive astrocyte profile, alterations in this purine-based signalling can impact on the neuroinflammatory response that occurs in both diseases. For this reason, strategies targeting astrocyte purinergic signalling are considered as promising new therapeutic treatments to better control the development and progression of both, epilepsy and MS.
ATP and its Degradation Product Adenosine in Epilepsy
Increasing evidence indicates that astroglial-mediated purinergic signalling modulates seizure threshold and epileptogenesis (Cieslak et al., 2017). Adenosine has a well-known anticonvulsant action. However increased expression of the astroglial enzyme ADK, the major metabolic clearance route for adenosine, during epileptogenesis reduces the inhibitory tone of adenosine and consequently reduces the threshold for seizure generation (Boison and Steinhauser, 2018). Regarding ATP, Dossi et al. (Dossi et al., 2018) recently demonstrated an 80% increase of extracellular ATP during high potassium induced ictal discharges on slices obtained from resected tissues of TLE patients. This increase was pharmacologically blocked by inhibiting pannexin-1 channels and had anticonvulsive effects also in a mouse model of kainic acid (KA)-induced seizures.
Ionotropic P2X7R in Epilepsy
Among ionotropic P2XRs, P2X7R is showed to be upregulated in epileptic mice and TLE patients, and P2X7R antagonism was able to reduce seizure severity and seizure-induced neuronal death (Jimenez-Pacheco et al., 2013), supporting the targeting of P2X7R as a potential therapeutic strategy for epilepsy. However, although P2X7R increased expression was well documented in neurons and microglia, its expression in hippocampal astrocytes remains highly controversial (Rassendren and Audinat, 2016). Even though, P2X7Rs may have a role in microglia-astrocyte communication in epilepsy. Activation of P2X7Rs on microglia induces their production and release of inflammatory signals (Campagno and Mitchell, 2021) that can promote astrogliosis. This can be a powerful mechanism of increasing a magnitude of inflammatory response in the CNS in epilepsy.
Evidence Supporting Astrocytic P2Y1R as a Key Modulator of Epileptic Activity
Metabotropic P2Y1R is a promising astrocytic candidate to target in epilepsy as its downstream signalling is permanently active in the sclerotic hippocampus and its blockade restores normal neuronal activity in epilepsy (Alvarez-Ferradas et al., 2015; Nikolic et al., 2018; Wellmann et al., 2018). An increased P2Y1R expression was observed at the hippocampal level in both epilepsy animal models and TLE patients (Alves et al., 2017) and moreover, the activation of P2Y1R by means of ADP administration was sufficient to worsen seizure activity. Furthermore, it has been shown that P2Y1R is responsible for either proconvulsive or anticonvulsive effects depending if the receptor activation was occurring before or after SE induction (Alves et al., 2019a). There are some disagreements regarding the P2Y1R cell type specific expression. For example, P2Y1R expression is observed in microglia at a relatively weak level (Hidetoshi et al., 2012), and also in a cortical microglia in the epileptic brain (Alves et al., 2019b). Literature data, however, show that expression of P2Y1Rs is mainly detected in astrocytes (Franke et al., 1999; Jourdain et al., 2007; Kuboyama et al., 2011; Pascual et al., 2012; Delekate et al., 2014). Moreover, activation of P2Y1Rs mediates aberrant astrocytic Ca2+ activity and glutamate release that drives abnormal synaptic activity in epilepsy (Alvarez-Ferradas et al., 2015; Nikolic et al., 2018; Wellmann et al., 2018; Martorell et al., 2020). These data on expression and function support an argument on crucial role of astrocytic P2Y1Rs in mediating epileptic activity (Table 1).
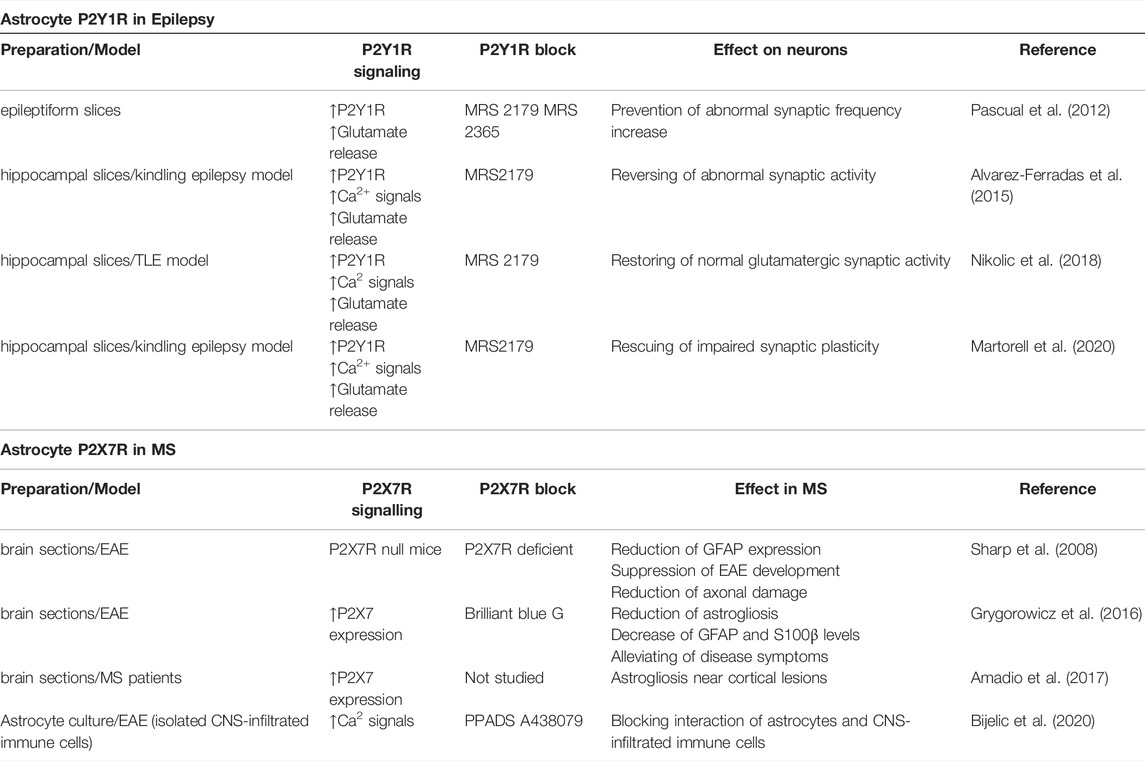
TABLE 1. Examples of astrocyte P2Y1R- and P2X7R-mediated signalling in epilepsy and MS, respectively. Properties of astrocyte P2Rs-mediated signalling and effects of their blocking on pathophysiological events are showed for both diseases.
Astrocyte P2Y1R and Neuronal Activity in Epilepsy
We recently showed that the pro-inflammatory cytokine tumor necrosis factor-α (TNFα), which is increased during seizure activity, is able to trigger a Ca2+-dependent glutamate release from astrocytes that boosts excitatory synaptic activity in the hippocampus of a TLE mouse model (Figure 1). We demonstrated that this mechanism involves an autocrine activation of P2Y1Rs by astrocyte-derived ATP/ADP. Indeed, reducing extracellular ATP/ADP with apyrase or blocking P2Y1Rs normalize glutamate release from astrocytes and restore normal synaptic activity during epileptogenesis (Nikolic et al., 2018). Furthermore, ATP can evoke a Ca2+-dependent glutamate release from astrocytes and trigger slow inward current (SIC) in neurons (Perea and Araque, 2005) leading to the synchronization of neuronal activity in the hippocampus (Angulo et al., 2004; Fellin et al., 2004). Activation of astrocytic P2X7Rs and P2Y1Rs do not mediate SIC appearance in physiological conditions (Fellin et al., 2006; Shigetomi et al., 2008). However, in epilepsy P2Y1-dependent glutamate release from astrocytes increases SIC frequency in CA1 neurons that can promote neuronal synchronisation (Alvarez-Ferradas et al., 2015), (Figure 1). Likewise, endogenously released ATP acting through astrocytic P2Y1Rs contributes to Ca2+ wave propagation of these glial cells (Bowser and Khakh, 2007) and it can play a role in promoting synchronized neuronal activity in epilepsy. These data support the importance of astroglial P2Y1R-mediated signalling in the epileptogenic process and suggest that purinergic therapeutic strategies could be useful in suppressing or ameliorating epileptic activity.
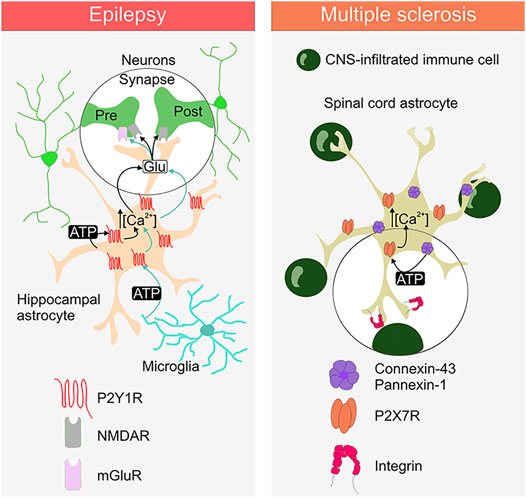
FIGURE 1. Purinergic signalling between astrocytes, neurons, microglia and CNS-infiltrated immune cell in epilepsy and multiple sclerosis. The ATP release and the involvement of the specific astrocytic purinergic receptor (PR) type is depicted for the diseases. Activation of astrocytic P2Y1R and P2X7R induce the rise in intracellular Ca2+. Activation of P2Y1R promotes the release of glutamate, which acts on the presynaptic and postsynaptic glutamate receptors (NMDAR and mGluR). Activation of P2X7Rs is mediated by the release of ATP through connexin-43 hemichannels and/or pannexin-1 channels. Integrins are depicted as a putative link to the astrocytic P2X7R activation via connexin-43/pannexin-1-derived ATP.
P2Y1R-Mediated Astrocyte-Microglia Crosstalk in Epilepsy
TNFα can trigger the astrocyte ATP-P2Y1R loop (see above) in the dentate gyrus in the context of epilepsy (Nikolic et al., 2018) and maybe also in the context of experimental autoimmune encephalitis (EAE) (Santello et al., 2011;Habbas et al., 2015). Whereas the source of TNFα in these inflammatory conditions is probably microglia (Zhang et al., 2014), microglial cells can also activate astrocyte P2Y1R-mediated signalling directly by releasing ATP. Alain Bessis and colleagues found that LPS application triggers, within few minutes in acute hippocampal slices, ATP release from microglia that activates astrocytic P2Y1Rs. This leads to the glutamate release from astrocytes that increases excitatory postsynaptic current (EPSC) frequency in CA1 pyramidal neurons through presynaptic mGluR activation. Moreover, activation of this microglia-astrocyte purinergic signalling promotes the generation of epileptiform activities (Figure 1) (Pascual et al., 2012). These observations reinforce the idea that purinergic signaling is critical in astrocyte-microglia crosstalk under various pathological conditions (Franke et al., 2012; Agostinho et al., 2020; Illes et al., 2020; Matejuk and Ransohoff, 2020; Pietrowski et al., 2021).
Astrocyte Purinergic Signalling in MS
Although less examined compared to epilepsy, astrocyte purinergic signalling can also be a critical contributor to the pathology of MS from its early stages and can also affect the course of the disease. Indeed, screening of selected P1R, P2XR and P2YR genes revealed that changes in their expression occur along with the different phases of the EAE development (Jakovljevic et al., 2017). The same study showed a decrease in the expression of ectonucleoside triphosphate diphosphohydrolase 2 (NTPDase2) in EAE, an ATP hydrolysing enzyme preferentially expressed in astrocytes. Furthermore, purine-based signalling mediates communication between astrocytes and oligodendrocytes (Fields and Burnstock, 2006; Ishibashi et al., 2006; Welsh and Kucenas, 2018; Agostinho et al., 2020) which may be critical in neuroinflammatory processes in MS. ATP release during neuronal activity induces astrocytes to release the promyelinating cytokine leukemia inhibitory factor (LIF), which promotes oligodendrocyte development (Ishibashi et al., 2006). However, others demonstrated that mechanical stimulation of astrocytes increases ATP release and ATP evokes Ca2+ signals in oligodendrocyte progenitor cells (OPCs) by a mechanism involving the activation of P2Y1Rs and P2X7Rs (Agresti et al., 2005; Hamilton et al., 2010). Moreover, over-activation of P2X7Rs in oligodendrocytes causes cell death due to the high cytosol Ca2+ levels, which contributes to demyelinating diseases. Considering that neurotoxic astrocytes contribute to the oligodendrocyte death in MS (Liddelow et al., 2017), targeting purine-based astrocyte-oligodendrocyte communication, could be a promising approach to prevent demyelination in this disease.
Evidence Supporting P2X7R as a Key Astrocytic Target in MS
Ionotropic P2X7R could be a promising astroglial candidate to target in MS as this receptor is upregulated in astrocytes, its blockade reduces astrogliosis (Narcisse et al., 2005; Grygorowicz et al., 2016; Amadio et al., 2017), and it is involved in the communication between astrocytes and components of the immune system in this disease (Table 1). Although characterized as dominantly expressed by microglia, P2X7R is also expressed and functional in astrocytes in specific areas (Oliveira et al., 2011; Illes, 2020). Within CNS lesions of patients with MS, P2X7R expression is upregulated in reactive astrocytes surrounding infiltrated immune cells. The same study demonstrated that a treatment with the proinflammatory cytokine interleukin 1β increases mRNA expression of P2X7R, and also increases P2X7R-dependent Ca2+ response of human fetal astrocytes (Narcisse et al., 2005).
P2X7R and Astrogliosis in MS
Previous research showed alterations in P2X7R expression in reactive astrocytes in the inflamed CNS. For example, P2X7Rs are up-regulated in reactive astrocytes in the frontal cortex of patients with MS (Amadio et al., 2017), while in EAE reactive astrocytes show increase in P2X7Rs immunoreactivity (Grygorowicz et al., 2016). In fact, blockade of P2X7Rs by Brilliant blue G administration in rats with EAE, decreases astrogliosis and alleviates disease symptoms (Grygorowicz et al., 2016). Furthermore, P2X7R deficiency supresses EAE development in P2X7R KO mice, reduces astroglial activation and axonal damage, but does not influence immune cell infiltration into the CNS (Sharp et al., 2008). Interestingly, P2X7Rs co-immunoprecipitate with pannexin-1 channel in astrocytes (Silverman et al., 2009), and pannexin-1 KO mice display a delay in the onset of the EAE clinical signs (Lutz et al., 2013). In this modality, pannexins-1 release ATP that activates P2X7Rs and further recruit components of the inflammasome.
Astrocyte P2X7R and Interaction With CNS-Infiltrated Immune Cells
In addition to signalling through the release of ATP to communicate with other types of neural cells (Franke et al., 2012), astrocytes utilize this purine-based signalling also to interact with CNS-infiltrated immune cells (Bijelic et al., 2020). Our recent study showed that astrocyte interaction with a nearby CNS-infiltrated immune cells requires astrocytic P2X7R- and not P2Y1R-dependent signalling (Bijelic et al., 2020). Furthermore, the presence of CD4+ autoreactive immune T cells promotes astroglial hemichannel and or/pannexin channel-controlled ATP release that activates P2X7Rs and causes increase in the cytosolic Ca2+ in these glial cells (Bijelic et al., 2020). Initial step promoting P2X7Rs activation in astrocytes upon encountering autoreactive CD4+ T cells, can involve integrins (Figure 1), as integrin engagement has been linked to the astrocyte P2X7R activation via connexin-43 hemichannel and pannexin-1 channel release of ATP (Henriquez et al., 2011; Alvarez et al., 2016). Integrins are adhesion molecules that mediate cell-to-cell interaction, they are expressed on neural and immune cells and implicated in pathology of MS (Archelos et al., 1999). Moreover, integrin-inhibiting therapies such as natalizumab are currently in use for treatment of MS to reduce relapses in patients (Slack et al., 2022). This consideration linking astrocytic P2X7Rs with integrins pertains to the potential role of this PR as an astroglia-targeting therapeutic candidate for treatment of MS, to control interaction between astrocytes and CNS-inflitrated immune cells to reduce neuroiflammation in this disease (Figure 1).
Conclusion
Deciphering astrocytic purinergic signalling and neuroinflammation in epilepsy and MS is a challenging task considering the cell types involved and the communication pathways operating in the inflamed CNS in these diseases. ATP release and astroglial-mediated purinergic signalling are crucial mechanisms through which astrocytes communicate between themselves, with neurons in epilepsy and with autoreactive immune cells in EAE (Figure 1), making them suitable targets for designing innovative disease-modifying strategies. In case of epilepsy, this would mean developing effective therapies that will not just target neurons, but also astrocytes, which by themselves provide conditions for proper neuronal functioning. Similarly in MS, demyelination and neuronal loss, could be rescued by effective controlling and targeting astrocyte interaction with autoreactive immune cells. Quite possibly, saving astrocyte protective functions would be a far more effective strategy to save neurons from damage in both, epilepsy and MS as virtually every aspect of brain function involves a neuron-astroglial partnership. We pointed toward P2Y1R and P2X7R as two potential astrocytic therapeutic candidates for treatment of epilepsy and MS, respectively. Common for both astrocytic receptor types is their involvement in autocrine loops, which further confirms that astrocyte targeting can be exploited to design effective therapeutic strategy. Further understanding of the purine-based signalling in mediating astrocyte cell to cell interactions holds a promise to develop new strategies for treatment of these neurological disorders.
Author Contributions
All authors wrote the initial draft, contributed to conception of the manuscript, interpretation of the literature, revised the manuscript, read, and approved the submitted version.
Funding
This work was supported by the Ministry of Education, Science and Technological Development of Republic of Serbia (Contract no. 451-03-68/2022-14/200007, 451-03-68/2022-14/200178, 451-03-68/2022-14/200053), Grants from European Commission (H2020 MSCA-ITN EU-GliaPhD No. 72205), Agence Nationale de la Recherche (contract numbers: ANR-19-CE16-0018-03 and ANR-20CE16-0003-02), Grants from Science and Technology Department of Zhejiang Province (2018C37131), and Scientific Research Foundation for Returned Scholars of Hangzhou City (2019).
Conflict of Interest
The authors declare that the research was conducted in the absence of any commercial or financial relationships that could be construed as a potential conflict of interest.
Publisher’s Note
All claims expressed in this article are solely those of the authors and do not necessarily represent those of their affiliated organizations, or those of the publisher, the editors and the reviewers. Any product that may be evaluated in this article, or claim that may be made by its manufacturer, is not guaranteed or endorsed by the publisher.
References
Abbott, N. J. (2000). Inflammatory Mediators and Modulation of Blood-Brain Barrier Permeability. Cell Mol Neurobiol 20, 131–147. doi:10.1023/a:1007074420772
Agostinho, P., Madeira, D., Dias, L., Simões, A. P., Cunha, R. A., and Canas, P. M. (2020). Purinergic Signaling Orchestrating Neuron-Glia Communication. Pharmacol. Res. 162, 105253. doi:10.1016/j.phrs.2020.105253
Agresti, C., Meomartini, M. E., Amadio, S., Ambrosini, E., Serafini, B., Franchini, L., et al. (2005). Metabotropic P2 Receptor Activation Regulates Oligodendrocyte Progenitor Migration and Development. Glia 50, 132–144. doi:10.1002/glia.20160
Alvarez, A., Lagos-Cabré, R., Kong, M., Cárdenas, A., Burgos-Bravo, F., Schneider, P., et al. (2016). Integrin-mediated Transactivation of P2X7R via Hemichannel-dependent ATP Release Stimulates Astrocyte Migration. Biochim. Biophys. Acta 1863, 2175–2188. doi:10.1016/j.bbamcr.2016.05.018
Álvarez-Ferradas, C., Morales, J. C., Wellmann, M., Nualart, F., Roncagliolo, M., Fuenzalida, M., et al. (2015). Enhanced Astroglial Ca2+ Signaling Increases Excitatory Synaptic Strength in the Epileptic Brain. Glia 63, 1507–1521. doi:10.1002/glia.22817
Alves, M., De Diego Garcia, L., Conte, G., Jimenez-Mateos, E. M., D'orsi, B., Sanz-Rodriguez, A., et al. (2019a). Context-Specific Switch from Anti- to Pro-epileptogenic Function of the P2Y1 Receptor in Experimental Epilepsy. J. Neurosci. 39, 5377–5392. doi:10.1523/JNEUROSCI.0089-19.2019
Alves, M., Gomez-Villafuertes, R., Delanty, N., Farrell, M. A., O'brien, D. F., Miras-Portugal, M. T., et al. (2017). Expression and Function of the Metabotropic Purinergic P2Y Receptor Family in Experimental Seizure Models and Patients with Drug-Refractory Epilepsy. Epilepsia 58, 1603–1614. doi:10.1111/epi.13850
Alves, M., Smith, J., and Engel, T. (2019b). Differential Expression of the Metabotropic P2Y Receptor Family in the Cortex Following Status Epilepticus and Neuroprotection via P2Y1 Antagonism in Mice. Front. Pharmacol. 10, 1558. doi:10.3389/fphar.2019.01558
Amadio, S., Parisi, C., Piras, E., Fabbrizio, P., Apolloni, S., Montilli, C., et al. (2017). Modulation of P2X7 Receptor during Inflammation in Multiple Sclerosis. Front. Immunol. 8, 1529. doi:10.3389/fimmu.2017.01529
Anderson, M. A., Burda, J. E., Ren, Y., Ao, Y., O'shea, T. M., Kawaguchi, R., et al. (2016). Astrocyte Scar Formation Aids central Nervous System Axon Regeneration. Nature 532, 195–200. doi:10.1038/nature17623
Angulo, M. C., Kozlov, A. S., Charpak, S., and Audinat, E. (2004). Glutamate Released from Glial Cells Synchronizes Neuronal Activity in the hippocampus. J. Neurosci. 24, 6920–6927. doi:10.1523/JNEUROSCI.0473-04.2004
Araque, A., Carmignoto, G., Haydon, P. G., Oliet, S. H., Robitaille, R., and Volterra, A. (2014). Gliotransmitters Travel in Time and Space. Neuron 81, 728–739. doi:10.1016/j.neuron.2014.02.007
Archelos, J. J., Previtali, S. C., and Hartung, H. P. (1999). The Role of Integrins in Immune-Mediated Diseases of the Nervous System. Trends Neurosci. 22, 30–38. doi:10.1016/s0166-2236(98)01287-9
Argaw, A. T., Asp, L., Zhang, J., Navrazhina, K., Pham, T., Mariani, J. N., et al. (2012). Astrocyte-derived VEGF-A Drives Blood-Brain Barrier Disruption in CNS Inflammatory Disease. J. Clin. Invest. 122, 2454–2468. doi:10.1172/JCI60842
Audinat, E., and Rassendren, F. (2021). “Glial Mechanisms of Inflammation during Seizures,”. Editors D. Janigro, A. Nehlig, and N. Marchi (Cham: Springer International Publishing), 45–70. doi:10.1007/978-3-030-67403-8_3Inflammation and Epilepsy: New Vistas
Baecher-Allan, C., Kaskow, B. J., and Weiner, H. L. (2018). Multiple Sclerosis: Mechanisms and Immunotherapy. Neuron 97, 742–768. doi:10.1016/j.neuron.2018.01.021
Bialer, M., Johannessen, S. I., Koepp, M. J., Levy, R. H., Perucca, E., Tomson, T., et al. (2018). Progress Report on New Antiepileptic Drugs: A Summary of the Fourteenth Eilat Conference on New Antiepileptic Drugs and Devices (EILAT XIV). I. Drugs in Preclinical and Early Clinical Development. Epilepsia 59, 1811–1841. doi:10.1111/epi.14557
Bijelic, D. D., Milicevic, K. D., Lazarevic, M. N., Miljkovic, D. M., Bogdanovic Pristov, J. J., Savic, D. Z., et al. (2020). Central Nervous System-Infiltrated Immune Cells Induce Calcium Increase in Astrocytes via Astroglial Purinergic Signaling. J. Neurosci. Res. 98, 2317–2332.
Boison, D., and Steinhäuser, C. (2018). Epilepsy and Astrocyte Energy Metabolism. Glia 66, 1235–1243. doi:10.1002/glia.23247
Bonansco, C., and Fuenzalida, M. (2016). Plasticity of Hippocampal Excitatory-Inhibitory Balance: Missing the Synaptic Control in the Epileptic Brain. Neural Plast. 2016, 8607038. doi:10.1155/2016/8607038
Borggrewe, M., Grit, C., Vainchtein, I. D., Brouwer, N., Wesseling, E. M., Laman, J. D., et al. (2021). Regionally Diverse Astrocyte Subtypes and Their Heterogeneous Response to EAE. Glia 69, 1140–1154. doi:10.1002/glia.23954
Bowser, D. N., and Khakh, B. S. (2007). Vesicular ATP Is the Predominant Cause of Intercellular Calcium Waves in Astrocytes. J. Gen. Physiol. 129, 485–491. doi:10.1085/jgp.200709780
Brambilla, R., and Abbracchio, M. P. (2001). Modulation of Cyclooxygenase-2 and Brain Reactive Astrogliosis by Purinergic P2 Receptors. Ann. N. Y Acad. Sci. 939, 54–62. doi:10.1111/j.1749-6632.2001.tb03612.x
Brambilla, R. (2019). The Contribution of Astrocytes to the Neuroinflammatory Response in Multiple Sclerosis and Experimental Autoimmune Encephalomyelitis. Acta Neuropathol. 137, 757–783. doi:10.1007/s00401-019-01980-7
Burnstock, G. (2020). Introduction to Purinergic Signaling. Methods Mol. Biol. 2041, 1–15. doi:10.1007/978-1-4939-9717-6_1
Burnstock, G. (2016). Short- and Long-Term (Trophic) Purinergic Signalling. Philos. Trans. R. Soc. Lond. B Biol. Sci. 371 (1700), 20150422. doi:10.1098/rstb.2015.0422
Campagno, K. E., and Mitchell, C. H. (2021). The P2X7 Receptor in Microglial Cells Modulates the Endolysosomal Axis, Autophagy, and Phagocytosis. Front Cel Neurosci 15, 645244. doi:10.3389/fncel.2021.645244
Cieslak, M., Wojtczak, A., and Komoszynski, M. (2017). Role of the Purinergic Signaling in Epilepsy. Pharmacol. Rep. 69, 130–138.
Coco, S., Calegari, F., Pravettoni, E., Pozzi, D., Taverna, E., Rosa, P., et al. (2003). Storage and Release of ATP from Astrocytes in Culture. J. Biol. Chem. 278, 1354–1362. doi:10.1074/jbc.M209454200
Colombo, E., and Farina, C. (2016). Astrocytes: Key Regulators of Neuroinflammation. Trends Immunol. 37, 608–620. doi:10.1016/j.it.2016.06.006
Colombo, E., Triolo, D., Bassani, C., Bedogni, F., Di Dario, M., Dina, G., et al. (2021). Dysregulated Copper Transport in Multiple Sclerosis May Cause Demyelination via Astrocytes. Proc. Natl. Acad. Sci. U S A. 118 (27), e2025804118. doi:10.1073/pnas.2025804118
De Jager, P. L., and Hafler, D. A. (2007). New Therapeutic Approaches for Multiple Sclerosis. Annu. Rev. Med. 58, 417–432. doi:10.1146/annurev.med.58.071105.111552
Delekate, A., Füchtemeier, M., Schumacher, T., Ulbrich, C., Foddis, M., and Petzold, G. C. (2014). Metabotropic P2Y1 Receptor Signalling Mediates Astrocytic Hyperactivity In Vivo in an Alzheimer's Disease Mouse Model. Nat. Commun. 5, 5422. doi:10.1038/ncomms6422
Diaz-Castro, B., Bernstein, A. M., Coppola, G., Sofroniew, M. V., and Khakh, B. S. (2021). Molecular and Functional Properties of Cortical Astrocytes during Peripherally Induced Neuroinflammation. Cell Rep 36, 109508. doi:10.1016/j.celrep.2021.109508
Dobson, R., and Giovannoni, G. (2019). Multiple Sclerosis - a Review. Eur. J. Neurol. 26, 27–40. doi:10.1111/ene.13819
Domercq, M., and Matute, C. (2019). Targeting P2X4 and P2X7 Receptors in Multiple Sclerosis. Curr. Opin. Pharmacol. 47, 119–125. doi:10.1016/j.coph.2019.03.010
Doná, F., Ulrich, H., Persike, D. S., Conceição, I. M., Blini, J. P., Cavalheiro, E. A., et al. (2009). Alteration of Purinergic P2X4 and P2X7 Receptor Expression in Rats with Temporal-Lobe Epilepsy Induced by Pilocarpine. Epilepsy Res. 83, 157–167. doi:10.1016/j.eplepsyres.2008.10.008
Dossi, E., Blauwblomme, T., Moulard, J., Chever, O., Vasile, F., Guinard, E., et al. (2018). Pannexin-1 Channels Contribute to Seizure Generation in Human Epileptic Brain Tissue and in a Mouse Model of Epilepsy. Sci. Transl Med. 10 (443), eaar3796. doi:10.1126/scitranslmed.aar3796
Escartin, C., Galea, E., Lakatos, A., O'callaghan, J. P., Petzold, G. C., Serrano-Pozo, A., et al. (2021). Reactive Astrocyte Nomenclature, Definitions, and Future Directions. Nat. Neurosci. 24, 312–325. doi:10.1038/s41593-020-00783-4
Escartin, C., Guillemaud, O., and Carrillo-De Sauvage, M. A. (2019). Questions and (Some) Answers on Reactive Astrocytes. Glia 67, 2221–2247. doi:10.1002/glia.23687
Fellin, T., Pascual, O., Gobbo, S., Pozzan, T., Haydon, P. G., and Carmignoto, G. (2004). Neuronal Synchrony Mediated by Astrocytic Glutamate through Activation of Extrasynaptic NMDA Receptors. Neuron 43, 729–743. doi:10.1016/j.neuron.2004.08.011
Fellin, T., Pozzan, T., and Carmignoto, G. (2006). Purinergic Receptors Mediate Two Distinct Glutamate Release Pathways in Hippocampal Astrocytes. J. Biol. Chem. 281, 4274–4284. doi:10.1074/jbc.M510679200
Fields, R. D., and Burnstock, G. (2006). Purinergic Signalling in Neuron-Glia Interactions. Nat. Rev. Neurosci. 7, 423–436. doi:10.1038/nrn1928
Franke, H., and Illes, P. (2014). Pathological Potential of Astroglial Purinergic Receptors. Adv. Neurobiol. 11, 213–256. doi:10.1007/978-3-319-08894-5_11
Franke, H., Krügel, U., and Illes, P. (1999). P2 Receptor-Mediated Proliferative Effects on Astrocytes In Vivo. Glia 28, 190–200. doi:10.1002/(sici)1098-1136(199912)28:3<190::aid-glia3>3.0.co;2-0
Franke, H., Verkhratsky, A., Burnstock, G., and Illes, P. (2012). Pathophysiology of Astroglial Purinergic Signalling. Purinergic Signal. 8, 629–657. doi:10.1007/s11302-012-9300-0
Friedman, A., Kaufer, D., and Heinemann, U. (2009). Blood-brain Barrier Breakdown-Inducing Astrocytic Transformation: Novel Targets for the Prevention of Epilepsy. Epilepsy Res. 85, 142–149. doi:10.1016/j.eplepsyres.2009.03.005
Grygorowicz, T., Wełniak-Kamińska, M., and Strużyńska, L. (2016). Early P2X7R-Related Astrogliosis in Autoimmune Encephalomyelitis. Mol. Cel Neurosci 74, 1–9. doi:10.1016/j.mcn.2016.02.003
Guttenplan, K. A., Stafford, B. K., El-Danaf, R. N., Adler, D. I., Münch, A. E., Weigel, M. K., et al. (2020). Neurotoxic Reactive Astrocytes Drive Neuronal Death after Retinal Injury. Cel Rep 31, 107776. doi:10.1016/j.celrep.2020.107776
Habbas, S., Santello, M., Becker, D., Stubbe, H., Zappia, G., Liaudet, N., et al. (2015). Neuroinflammatory TNFα Impairs Memory via Astrocyte Signaling. Cell 163, 1730–1741. doi:10.1016/j.cell.2015.11.023
Hamilton, N., Vayro, S., Wigley, R., and Butt, A. M. (2010). Axons and Astrocytes Release ATP and Glutamate to Evoke Calcium Signals in NG2-Glia. Glia 58, 66–79. doi:10.1002/glia.20902
Hartmann, K., Sepulveda-Falla, D., Rose, I. V. L., Madore, C., Muth, C., Matschke, J., et al. (2019). Complement 3+-astrocytes Are Highly Abundant in Prion Diseases, but Their Abolishment Led to an Accelerated Disease Course and Early Dysregulation of Microglia. Acta Neuropathol. Commun. 7, 83. doi:10.1186/s40478-019-0735-1
Henríquez, M., Herrera-Molina, R., Valdivia, A., Alvarez, A., Kong, M., Muñoz, N., et al. (2011). ATP Release Due to Thy-1-Integrin Binding Induces P2X7-Mediated Calcium Entry Required for Focal Adhesion Formation. J. Cel Sci 124, 1581–1588. doi:10.1242/jcs.073171
Hidetoshi, T.-S., Makoto, T., and Inoue, K. (2012). P2Y Receptors in Microglia and Neuroinflammation. Wires Membr. Transp Signal. 1, 493–501. doi:10.1002/wmts.46
Hindley, S., Herman, M. A., and Rathbone, M. P. (1994). Stimulation of Reactive Astrogliosis In Vivo by Extracellular Adenosine Diphosphate or an Adenosine A2 Receptor Agonist. J. Neurosci. Res. 38, 399–406. doi:10.1002/jnr.490380405
Illes, P. (2020). P2X7 Receptors Amplify CNS Damage in Neurodegenerative Diseases. Int. J. Mol. Sci. 21 (17), 5996. doi:10.3390/ijms21175996
Illes, P., Rubini, P., Ulrich, H., Zhao, Y., and Tang, Y. (2020). Regulation of Microglial Functions by Purinergic Mechanisms in the Healthy and Diseased CNS. Cells 9 (5), 1108. doi:10.3390/cells9051108
Iori, V., Frigerio, F., and Vezzani, A. (2016). Modulation of Neuronal Excitability by Immune Mediators in Epilepsy. Curr. Opin. Pharmacol. 26, 118–123. doi:10.1016/j.coph.2015.11.002
Ishibashi, T., Dakin, K. A., Stevens, B., Lee, P. R., Kozlov, S. V., Stewart, C. L., et al. (2006). Astrocytes Promote Myelination in Response to Electrical Impulses. Neuron 49, 823–832. doi:10.1016/j.neuron.2006.02.006
Jakovljevic, M., Lavrnja, I., Bozic, I., Savic, D., Bjelobaba, I., Pekovic, S., et al. (2017). Down-regulation of NTPDase2 and ADP-Sensitive P2 Purinoceptors Correlate with Severity of Symptoms during Experimental Autoimmune Encephalomyelitis. Front. Cel Neurosci 11, 333. doi:10.3389/fncel.2017.00333
Janmohamed, M., Brodie, M. J., and Kwan, P. (2020). Pharmacoresistance - Epidemiology, Mechanisms, and Impact on Epilepsy Treatment. Neuropharmacology 168, 107790. doi:10.1016/j.neuropharm.2019.107790
Jimenez-Pacheco, A., Mesuret, G., Sanz-Rodriguez, A., Tanaka, K., Mooney, C., Conroy, R., et al. (2013). Increased Neocortical Expression of the P2X7 Receptor after Status Epilepticus and Anticonvulsant Effect of P2X7 Receptor Antagonist A-438079. Epilepsia 54, 1551–1561. doi:10.1111/epi.12257
John Lin, C. C., Yu, K., Hatcher, A., Huang, T. W., Lee, H. K., Carlson, J., et al. (2017). Identification of Diverse Astrocyte Populations and Their Malignant Analogs. Nat. Neurosci. 20, 396–405. doi:10.1038/nn.4493
Jourdain, P., Bergersen, L. H., Bhaukaurally, K., Bezzi, P., Santello, M., Domercq, M., et al. (2007). Glutamate Exocytosis from Astrocytes Controls Synaptic Strength. Nat. Neurosci. 10, 331–339. doi:10.1038/nn1849
Kuboyama, K., Harada, H., Tozaki-Saitoh, H., Tsuda, M., Ushijima, K., and Inoue, K. (2011). Astrocytic P2Y(1) Receptor Is Involved in the Regulation of Cytokine/chemokine Transcription and Cerebral Damage in a Rat Model of Cerebral Ischemia. J. Cereb. Blood Flow Metab. 31, 1930–1941. doi:10.1038/jcbfm.2011.49
Liddelow, S. A., Guttenplan, K. A., Clarke, L. E., Bennett, F. C., Bohlen, C. J., Schirmer, L., et al. (2017). Neurotoxic Reactive Astrocytes Are Induced by Activated Microglia. Nature 541, 481–487. doi:10.1038/nature21029
Linker, R. A., Lee, D. H., Demir, S., Wiese, S., Kruse, N., Siglienti, I., et al. (2010). Functional Role of Brain-Derived Neurotrophic Factor in Neuroprotective Autoimmunity: Therapeutic Implications in a Model of Multiple Sclerosis. Brain 133, 2248–2263. doi:10.1093/brain/awq179
Linnerbauer, M., Wheeler, M. A., and Quintana, F. J. (2020). Astrocyte Crosstalk in CNS Inflammation. Neuron 108, 608–622. doi:10.1016/j.neuron.2020.08.012
Lutz, S. E., González-Fernández, E., Ventura, J. C., Pérez-Samartín, A., Tarassishin, L., Negoro, H., et al. (2013). Contribution of Pannexin1 to Experimental Autoimmune Encephalomyelitis. PLoS One 8 (6), e66657. doi:10.1371/journal.pone.0066657
Marchi, N., Granata, T., and Janigro, D. (2014). Inflammatory Pathways of Seizure Disorders. Trends Neurosci. 37 (2), 55–65. doi:10.1016/j.tins.2013.11.002
Martorell, A., Wellmann, M., Guiffa, F., Fuenzalida, M., and Bonansco, C. (2020). P2Y1 Receptor Inhibition Rescues Impaired Synaptic Plasticity and Astroglial Ca2+-dependent Activity in the Epileptic hippocampus. Neurobiol. Dis. 146, 105132. doi:10.1016/j.nbd.2020.105132
Matejuk, A., and Ransohoff, R. M. (2020). Crosstalk between Astrocytes and Microglia: An Overview. Front. Immunol. 11, 1416. doi:10.3389/fimmu.2020.01416
Narcisse, L., Scemes, E., Zhao, Y., Lee, S. C., and Brosnan, C. F. (2005). The Cytokine IL-1beta Transiently Enhances P2X7 Receptor Expression and Function in Human Astrocytes. Glia 49 (2), 245–258. doi:10.1002/glia.20110
Naylor, D. E. (2010). Glutamate and GABA in the Balance: Convergent Pathways Sustain Seizures during Status Epilepticus. Epilepsia 51 (Suppl. 3), 106–109. doi:10.1111/j.1528-1167.2010.02622.x
Nikolic, L., Nobili, P., Shen, W., and Audinat, E. (2020). Role of Astrocyte Purinergic Signaling in Epilepsy. Glia 68 (9), 1677–1691. doi:10.1002/glia.23747
Nikolic, L., Shen, W., Nobili, P., Virenque, A., Ulmann, L., and Audinat, E. (2018). Blocking TNFα-Driven Astrocyte Purinergic Signaling Restores normal Synaptic Activity during Epileptogenesis. Glia 66 (12), 2673–2683. doi:10.1002/glia.23519
Oliveira, J. F., Riedel, T., Leichsenring, A., Heine, C., Franke, H., Krügel, U., et al. (2011). Rodent Cortical Astroglia Express In Situ Functional P2X7 Receptors Sensing Pathologically High ATP Concentrations. Cereb. Cortex 21, 806–820. doi:10.1093/cercor/bhq154
Parpura, V., and Verkhratsky, A. (2012). Astrocytes Revisited: Concise Historic Outlook on Glutamate Homeostasis and Signaling. Croat. Med. J. 53, 518–528. doi:10.3325/cmj.2012.53.518
Pascual, O., Ben Achour, S., Rostaing, P., Triller, A., and Bessis, A. (2012). Microglia Activation Triggers Astrocyte-Mediated Modulation of Excitatory Neurotransmission. Proc. Natl. Acad. Sci. U S A. 109, E197–E205. doi:10.1073/pnas.1111098109
Perea, G., and Araque, A. (2005). Properties of Synaptically Evoked Astrocyte Calcium Signal Reveal Synaptic Information Processing by Astrocytes. J. Neurosci. 25, 2192–2203. doi:10.1523/JNEUROSCI.3965-04.2005
Pietrowski, M. J., Gabr, A. A., Kozlov, S., Blum, D., Halle, A., and Carvalho, K. (2021). Glial Purinergic Signaling in Neurodegeneration. Front. Neurol. 12, 654850. doi:10.3389/fneur.2021.654850
Prabowo, A. S., Anink, J. J., Lammens, M., Nellist, M., Van Den Ouweland, A. M., Adle-Biassette, H., et al. (2013). Fetal Brain Lesions in Tuberous Sclerosis Complex: TORC1 Activation and Inflammation. Brain Pathol. 23, 45–59. doi:10.1111/j.1750-3639.2012.00616.x
Prins, M., Dutta, R., Baselmans, B., Brevé, J. J., Bol, J. G., Deckard, S. A., et al. (2014). Discrepancy in CCL2 and CCR2 Expression in white versus Grey Matter Hippocampal Lesions of Multiple Sclerosis Patients. Acta Neuropathol. Commun. 2, 98. doi:10.1186/s40478-014-0098-6
Ransohoff, R. M. (2016). How Neuroinflammation Contributes to Neurodegeneration. Science 353, 777–783. doi:10.1126/science.aag2590
Rassendren, F., and Audinat, E. (2016). Purinergic Signaling in Epilepsy. J. Neurosci. Res. 94, 781–793. doi:10.1002/jnr.23770
Ravizza, T., Gagliardi, B., Noé, F., Boer, K., Aronica, E., and Vezzani, A. (2008). Innate and Adaptive Immunity during Epileptogenesis and Spontaneous Seizures: Evidence from Experimental Models and Human Temporal Lobe Epilepsy. Neurobiol. Dis. 29, 142–160. doi:10.1016/j.nbd.2007.08.012
Rommer, P. S., Milo, R., Han, M. H., Satyanarayan, S., Sellner, J., Hauer, L., et al. (2019). Immunological Aspects of Approved MS Therapeutics. Front. Immunol. 10, 1564. doi:10.3389/fimmu.2019.01564
Rothhammer, V., Mascanfroni, I. D., Bunse, L., Takenaka, M. C., Kenison, J. E., Mayo, L., et al. (2016). Type I Interferons and Microbial Metabolites of Tryptophan Modulate Astrocyte Activity and central Nervous System Inflammation via the Aryl Hydrocarbon Receptor. Nat. Med. 22, 586–597. doi:10.1038/nm.4106
Rothhammer, V., and Quintana, F. J. (2015). Control of Autoimmune CNS Inflammation by Astrocytes. Semin. Immunopathol 37, 625–638. doi:10.1007/s00281-015-0515-3
Santello, M., Bezzi, P., and Volterra, A. (2011). TNFα Controls Glutamatergic Gliotransmission in the Hippocampal Dentate Gyrus. Neuron 69, 988–1001. doi:10.1016/j.neuron.2011.02.003
Sanz, P., and Garcia-Gimeno, M. A. (2020). Reactive Glia Inflammatory Signaling Pathways and Epilepsy. Int. J. Mol. Sci. 21 (11), 4096. doi:10.3390/ijms21114096
Schönrock, L. M., Gawlowski, G., and Brück, W. (2000). Interleukin-6 Expression in Human Multiple Sclerosis Lesions. Neurosci. Lett. 294, 45–48. doi:10.1016/s0304-3940(00)01543-3
Sharp, A. J., Polak, P. E., Simonini, V., Lin, S. X., Richardson, J. C., Bongarzone, E. R., et al. (2008). P2x7 Deficiency Suppresses Development of Experimental Autoimmune Encephalomyelitis. J. Neuroinflammation 5, 33. doi:10.1186/1742-2094-5-33
Shen, W., Nikolic, L., Meunier, C., Pfrieger, F., and Audinat, E. (2017). An Autocrine Purinergic Signaling Controls Astrocyte-Induced Neuronal Excitation. Sci. Rep. 7, 11280. doi:10.1038/s41598-017-11793-x
Shigetomi, E., Bowser, D. N., Sofroniew, M. V., and Khakh, B. S. (2008). Two Forms of Astrocyte Calcium Excitability Have Distinct Effects on NMDA Receptor-Mediated Slow Inward Currents in Pyramidal Neurons. J. Neurosci. 28, 6659–6663. doi:10.1523/JNEUROSCI.1717-08.2008
Sidoryk-Wegrzynowicz, M., and Struzynska, L. (2021). Astroglial and Microglial Purinergic P2X7 Receptor as a Major Contributor to Neuroinflammation during the Course of Multiple Sclerosis. Int. J. Mol. Sci. 22 (16), 8404.
Silverman, W. R., De Rivero Vaccari, J. P., Locovei, S., Qiu, F., Carlsson, S. K., Scemes, E., et al. (2009). The Pannexin 1 Channel Activates the Inflammasome in Neurons and Astrocytes. J. Biol. Chem. 284, 18143–18151. doi:10.1074/jbc.M109.004804
Slack, R. J., Macdonald, S. J. F., Roper, J. A., Jenkins, R. G., and Hatley, R. J. D. (2022). Emerging Therapeutic Opportunities for Integrin Inhibitors. Nat. Rev. Drug Discov. 21, 60–78. doi:10.1038/s41573-021-00284-4
Smith, N. M., Giacci, M. K., Gough, A., Bailey, C., Mcgonigle, T., Black, A. M. B., et al. (2018). Inflammation and Blood-Brain Barrier Breach Remote from the Primary Injury Following Neurotrauma. J. Neuroinflammation 15, 201. doi:10.1186/s12974-018-1227-0
Sofroniew, M. V. (2020). Astrocyte Reactivity: Subtypes, States, and Functions in CNS Innate Immunity. Trends Immunol. 41, 758–770. doi:10.1016/j.it.2020.07.004
Sofroniew, M. V. (2014). Astrogliosis. Cold Spring Harb Perspect. Biol. 7, a020420. doi:10.1101/cshperspect.a020420
Stadelmann, C., Kerschensteiner, M., Misgeld, T., Brück, W., Hohlfeld, R., and Lassmann, H. (2002). BDNF and gp145trkB in Multiple Sclerosis Brain Lesions: Neuroprotective Interactions between Immune and Neuronal Cells? Brain 125, 75–85. doi:10.1093/brain/awf015
Tan, Z., Liu, Y., Xi, W., Lou, H. F., Zhu, L., Guo, Z., et al. (2017). Glia-derived ATP Inversely Regulates Excitability of Pyramidal and CCK-Positive Neurons. Nat. Commun. 8, 13772. doi:10.1038/ncomms13772
Thijs, R. D., Surges, R., O'brien, T. J., and Sander, J. W. (2019). Epilepsy in Adults. Lancet 393, 689–701. doi:10.1016/S0140-6736(18)32596-0
Vezzani, A., Balosso, S., and Ravizza, T. (2019). Neuroinflammatory Pathways as Treatment Targets and Biomarkers in Epilepsy. Nat. Rev. Neurol. 15, 459–472. doi:10.1038/s41582-019-0217-x
Vezzani, A., French, J., Bartfai, T., and Baram, T. Z. (2011). The Role of Inflammation in Epilepsy. Nat. Rev. Neurol. 7, 31–40. doi:10.1038/nrneurol.2010.178
Wellmann, M., Álvarez-Ferradas, C., Maturana, C. J., Sáez, J. C., and Bonansco, C. (2018). Astroglial Ca2+-dependent Hyperexcitability Requires P2Y1 Purinergic Receptors and Pannexin-1 Channel Activation in a Chronic Model of Epilepsy. Front. Cel Neurosci 12, 446. doi:10.3389/fncel.2018.00446
Welsh, T. G., and Kucenas, S. (2018). Purinergic Signaling in Oligodendrocyte Development and Function. J. Neurochem. 145, 6–18. doi:10.1111/jnc.14315
Wheeler, M. A., and Quintana, F. J. (2019). Regulation of Astrocyte Functions in Multiple Sclerosis. Cold Spring Harb Perspect. Med. 9 (1), a029009. doi:10.1101/cshperspect.a029009
Xanthos, D. N., and Sandkühler, J. (2014). Neurogenic Neuroinflammation: Inflammatory CNS Reactions in Response to Neuronal Activity. Nat. Rev. Neurosci. 15, 43–53. doi:10.1038/nrn3617
Yang, Q. Q., and Zhou, J. W. (2019). Neuroinflammation in the central Nervous System: Symphony of Glial Cells. Glia 67, 1017–1035. doi:10.1002/glia.23571
Keywords: astroglia, disease, intercellular interaction, neuroinflammation, P2X7, P2Y1
Citation: Nobili P, Shen W, Milicevic K, Bogdanovic Pristov J, Audinat E and Nikolic L (2022) Therapeutic Potential of Astrocyte Purinergic Signalling in Epilepsy and Multiple Sclerosis. Front. Pharmacol. 13:900337. doi: 10.3389/fphar.2022.900337
Received: 20 March 2022; Accepted: 19 April 2022;
Published: 02 May 2022.
Edited by:
Savina Apolloni, University of Rome Tor Vergata, ItalyReviewed by:
Tobias Engel, Royal College of Surgeons in Ireland, IrelandSusanna Amadio, Santa Lucia Foundation (IRCCS), Italy
Peter Bedner, University Hospital Bonn, Germany
Copyright © 2022 Nobili, Shen, Milicevic, Bogdanovic Pristov, Audinat and Nikolic. This is an open-access article distributed under the terms of the Creative Commons Attribution License (CC BY). The use, distribution or reproduction in other forums is permitted, provided the original author(s) and the copyright owner(s) are credited and that the original publication in this journal is cited, in accordance with accepted academic practice. No use, distribution or reproduction is permitted which does not comply with these terms.
*Correspondence: Etienne Audinat, ZXRpZW5uZS5hdWRpbmF0QGlnZi5jbnJzLmZyJiN4MDIwMGE7; Ljiljana Nikolic, bGpuaWtvbGljQGliaXNzLmJnLmFjLnJz, bmlrb2xpYzEzQGdtYWlsLmNvbQ==
†These authors share first authorship