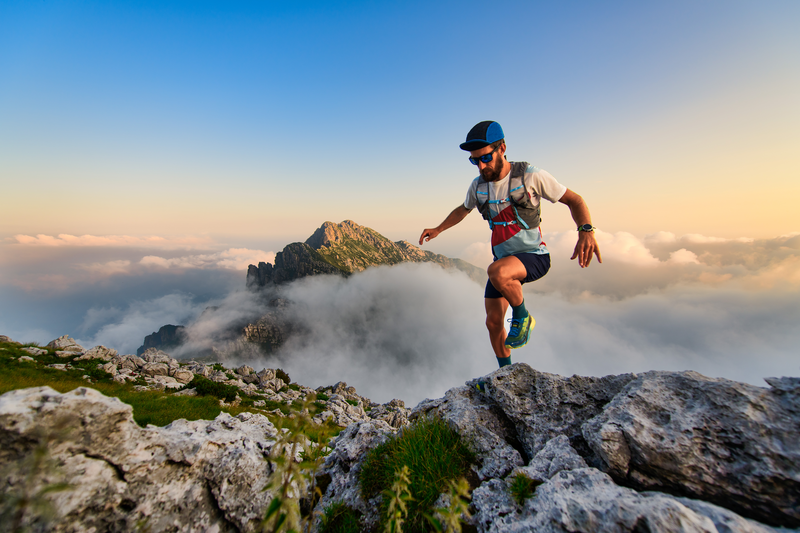
94% of researchers rate our articles as excellent or good
Learn more about the work of our research integrity team to safeguard the quality of each article we publish.
Find out more
REVIEW article
Front. Pharmacol. , 03 May 2022
Sec. Neuropharmacology
Volume 13 - 2022 | https://doi.org/10.3389/fphar.2022.898574
This article is part of the Research Topic Novel Therapeutic Mechanisms Targeting Neuro-Immune Regulation of Neurological Disorders View all 16 articles
Chronic pain is a common, complex and unpleasant sensation following nerve injury, tissue trauma, inflammatory diseases, infection and cancer. It affects up to 25% of adults and is increasingly recognized as the leading cause of distress, disability and disease burden globally. Chronic pain is often refractory to most current analgesics, thus emphasizing the requirement for improved therapeutic medications. It is of great importance to elucidate the specific pathogenesis of chronic pain with different etiologies. Recent progress has advanced our understanding in the contribution of neuroinflammation and glial cells (microglia and astrocyte) activation in the plasticity of excitatory nociceptive synapses and the development of chronic pain phenotypes. Oxidative stress-associated neuronal apoptosis is also identified to be a pivotal step for central pain sensitization. The family of cysteine aspartate specific proteases (Caspases) has been well known to be key signaling molecules for inflammation and apoptosis in several neurological conditions. Recent studies have highlighted the unconventional and emerging role of caspases in microgliosis, astrocytes morphogenesis, chemokines release, cytokines secretion and neuronal apoptosis in initiating and maintaining synaptogenesis, synaptic strength and signal transduction in persistent pain hypersensitivity, suggesting the possibility of targeting caspases pathway for prevention and treatment of chronic pain. In this review, we will discuss and summarize the advances in the distinctive properties of caspases family in the pathophysiology of chronic pain, especially in neuropathic pain, inflammatory pain, cancer pain and musculoskeletal pain, with the aim to find the promising therapeutic candidates for the resolution of chronic pain to better manage patients undergoing chronic pain in clinics.
Pain is officially declared as “The Fifth Vital Sign” (Walid et al., 2008). Chronic pain is characterized by pain that sustains or recurs for longer than 3 months (Klein, 2015; Treede et al., 2019). Chronic pain remains to become a major medical issue which affects at least 25% of the general population and imposes a heavy financial burden to patients and healthcare systems worldwide (Gereau et al., 2014; Mills et al., 2019). It frequently presents spontaneous pain, allodynia and hyperalgesia, as a result of nerve injury, cancer, chemotherapy, tissue trauma and inflammation (Ji et al., 2014; Ji et al., 2016; Baral et al., 2019). Patients with chronic pain also often experience insomnia, depression, anxiety and cognitive impairments, which is known to be associated with worsening pain and a serious threat to their quality of life (Moriarty et al., 2011; Tajerian et al., 2014; Tracey et al., 2019). The etiopathogenesis of chronic pain is still debated and, consequently, are the strategies for treating this condition (Ji et al., 2018; Tracey et al., 2019). Chronic pain is identified to be refractory to most analgesics currently (opioids, non-steroidal anti-inflammatory drugs and anticonvulsants) in use (Chou and Huffman, 2007; Tracey et al., 2019), thus emphasizing the urgent need for investigating the specific molecular mechanism that underlies the generation and persistence of chronic pain with different etiology.
Peripheral nociceptive sensitization (trigeminal ganglion and dorsal root ganglion, DRG) and central nociceptive sensitization (spinal cord and brain) mediated changes of neural plasticity in pain neurocircuits contributes to chronic pain phenotypes (Latremoliere and Woolf, 2009; Luo et al., 2014; Bliss et al., 2016; Han et al., 2016; Ji et al., 2018). While acute pain is an essential defensive response involving inflammation, chronic pain that is critically initiated by continuous neuroinflammation can be pathologic and maladaptive (Ji et al., 2016). Neuroinflammation involves glial cells (microglia and astrocyte) activation, chemokines (CCL1, CCL2, CCL7, CXCL1) release and pro-inflammatory mediators (TNF-α, IL-1β, IL-18, BDNF, PGE2) secretion in pain neural circuitry that, subsequently, mediates excitatory neuronal plasticity and synaptic transmission for producing and sustaining chronic inflammatory pain, chronic neuropathic pain, chronic fracture-associated pain, as well as chronic cancer pain (Zhang et al., 2013; Ji et al., 2014; Ni et al., 2019; Qiang and Yu, 2019; Wang et al., 2020b). Accumulating evidence emphasizes that oxidative stress drive neuronal apoptosis and sensitize nociceptors in the pathogenesis of chronic pain, such as chemotherapy-induced peripheral neuropathy (CIPN) and opioid-induced hyperalgesia (OIH) (Zhang et al., 2014; Shu et al., 2015; Grace et al., 2016a; Yousuf et al., 2020; Squillace and Salvemini, 2022). Nevertheless, the involvement of specific molecular signaling in neuroinflammation and neuronal apoptosis remains controversial.
Caspases are a family of conserved aspartate-specific cysteine proteases, which generally exhibits similar structures and presents in the cytoplasm in an inactive form (pro-caspases) (Graham et al., 2011). When an appropriate stimulus is given, caspases will be activated, dimerized and cleaved to form a heterotetramer, the active form of the enzyme (Van Opdenbosch and Lamkanfi, 2019). Activated caspases represent unique catalytic properties and can specifically recognize certain tetrapeptide motifs and cleave an aspartate residue in their substrates, executing programmed cell death (apoptosis) induced by a variety of injuries, including cytokines, chemokines, inflammatory damage and excitotoxicity (Van Opdenbosch and Lamkanfi, 2019). The human caspases family can be subdivided into three functional groups: apoptosis initiator caspases (Caspase-2, 8, 9, and 10), apoptosis effector caspases (Caspase-3, 6, and 7), and inflammatory caspases (Caspase-1, 4, 5, 11, and 12). Initiator caspases elicit the apoptosis signal while the effector caspases carry out the mass proteolysis that leads to apoptosis. Inflammatory caspases do not function in apoptosis but are rather involved in inflammatory signaling and other types of cell death such as pyroptosis (Julien and Wells, 2017).
The caspases have been gradually recognized as a cardinal contributor in neuroinflammatory responses and neuronal apoptosis in a wide variety of neurological and neuropsychiatric disorders, including Alzheimer’s disease (AD), Parkinson’s disease (PD), Huntington’s disease, multiple sclerosis, amyotrophic lateral sclerosis, tauopathies and age-related macular degeneration (Flores et al., 2018; Kirby et al., 2019). Intriguingly, recent progress has advanced our understanding in the unconventional properties of the caspases in mediating the perception of pain. Of note, caspase-1, caspase-3 and caspase-6 are identified as key signaling molecules for nociception induction and persistence by regulating neuroinflammation, neural apoptosis and synaptic plasticity (Berta et al., 2014; Berta et al., 2017a; Gao et al., 2018) in the spinal dorsal horn (Chen et al., 2018; Ji et al., 2018). In this review, we provide a more comprehensive view on the emerging role of caspases in the mechanisms responsible for various pain states. Apart from nerve injury-induced neuropathic pain, we discuss caspases cascades in chronic inflammatory pain, cancer pain, chemotherapy-induced peripheral neuropathy and opioid-induced hyperalgesia. In particular, we summarize the latest basic and clinical advance in this field, and propose the potential therapeutic targets for the resolution of chronic pain in the clinical setting.
Neuropathic pain is primarily triggered by direct nerve trauma in the neurocircuits of peripheral and central somatosensory nervous system. Caspase-1 is the prototypical member of inflammatory caspases involved in cytokine maturation. Dysregulation of inflammasome is strongly associated with the human inflammatory diseases by the enhancement of Caspase-1 activity (Venero et al., 2013). The NOD-like receptor protein 3 (NLRP3) inflammasome are cytosolic multiprotein complexes, which consists of inactive pro-caspase-1 (Liang et al., 2022). When the recruitment of pro-caspase-1 into NLRP3 inflammasome occurs following the exposure to noxious stimuli, pro-caspase-1 will be auto-cleaved to become mature caspase-1 with high bioactivity (Liang et al., 2022). Then, caspase-1 can cleave the pro-IL-1β and pro-IL-18 to generate the activated forms IL-1β and IL-18, further mediating the extracellular secretion of IL-1β and IL-18, which facilitates the transmission of painful information (Rocha et al., 2020; Chen R. et al., 2021). Xu and his colleagues reported that mice with a chronic constriction injury (CCI) of sciatic nerve experience pain-like behaviors followed by the increase of NLRP3 and activated caspase-1 expression in neurons and astrocytes in the superficial dorsal horn of spinal cord, and that pharmacological inhibition of caspase-1 activation attenuates CCI-induced mechanical allodynia and thermal hyperalgesia (Xu et al., 2019). Moreover, Grace et al. (2018) demonstrated that morphine treatment after CCI-induced peripheral nerve injury results in persistent damage associated molecular patterns (DAMP) release, which is critical for formation/activation of spinal caspase-1-dependent NLRP3 inflammasomes, thus causing the extension of CCI induced neuropathic allodynia, whereas inhibition of caspase-1, or IL-1β in the spinal dorsal horn reversed prolonged allodynia. Additionally, morphine induces the phosphorylation of p38, and up-modulates the expressions of Nuclear Factor-κB (NF-κB) subunit P65, toll-like receptor 4 (TLR4) and ionotropic P2X receptors (P2X4 and P2X7) in spinal microglia in CCI rats (Grace et al., 2016b). These alternations are involved in the activation of caspase-1 and the secretion of downstream cytokine IL-1β, which mediates the long-term increase of activity and excitability in nociceptive sensory neurons, and results in the production and persistence of chronic neuropathic pain. In addition to the CCI model, NLRP3 and activated caspase-1 expression were also significantly elevated in spinal glial cells of mice with partial sciatic nerve ligation (pSNL)-induced neuropathic pain (Pan et al., 2018). Meanwhile, the synthesis of caspase-1 in DRG of SNL rats is also increased (Zhang Y. et al., 2015), suggesting that activation of caspase-1 in peripheral nervous system is the pathophysiological basis for pronociceptive hypersensitivity in chronic neuropathic pain. Furthermore, Qian and his colleagues manifest that spinal suppression of caspase-1 activation can effectively reduce the synthesis of cytokines IL-1β and IL-18 and ameliorate mechanical allodynia phenomena in a model of neuropathic pain with spinal cord injury (SCI) (Qian et al., 2017).
In addition to neuropathic pain induced by nerve injury, many antineoplastic agents are capable of eliciting CIPN, which is characterized by mechanical allodynia, a pain evoked by non-nociceptive stimuli such as light touch (Shim et al., 2019). Repetitive injections of intraperitoneal paclitaxel generate and sustain long-lasting CIPN in rats, which is accompanied by NLRP3 over-expression, caspase-1 activation and IL-1β secretion in DRG and sciatic nerve at 3 weeks after paclitaxel application. Furthermore, inhibition of caspase-1 activity reduces the occurrence of CIPN and alleviates the severity of CIPN (Jia et al., 2017). Simultaneously, up-regulations of caspase-1 and NLRP3 in the spinal dorsal horn were verified in oxaliplatin-associated CIPN in rats (Wahlman et al., 2018). The aforementioned findings identified that inhibition of caspase-1-dependent neuroinflammation may offer a novel therapeutic strategy for neuropathic pain control (Table 1).
Caspase-3 is known as an executioner caspase in apoptosis because of its potent role in coordinating the destruction of cellular structures such as DNA fragmentation or degradation of cytoskeletal proteins (McIlwain et al., 2015). Caspase-3 is one of the key indicators of apoptosis, and studies in the field of chronic pain with caspase-3 are still in its exploratory stage. Wu et al. (2012) elucidated that sciatic nerve injury induced by the CCI model not only initiates chronic neuropathic pain, but also increases the expression of caspase-3 in the spinal cord and caspase-3-dependent apoptosis of dorsal horn neurons, which is associated with up-regulation of growth associated protein 43 (GAP-43) expression. Behavioral results also indicate that inhibition of caspase-3 activity by both pharmacological therapy with caspase-3 inhibitor Z-DEVD-FMK and caspase-3 knockdown attenuates the thermal hyperalgesia in CCI rats (Wu et al., 2012). Li et al. also found that microRNA-212-3p controls peripheral neuropathic allodynia and sodium voltage-gated channel alpha subunit 3 (Nav 1.3) through inhibiting caspase-3 cleavage and B-cell lymphoma 2-associated X apoptosis regulator (BAX) expression in rats with CCI surgery (Li Y. et al., 2019). EphrinB/EphB signaling, the most important subfamily of receptor tyrosine kinases (RTKs) in humans, is one of pivotal cascades in the spinal pain processing and nociceptive synaptic plasticity (Deng et al., 2017; Peng et al., 2019). Recent evidence discloses that caspase-3 activation in the spinal dorsal horn neurons but not microglia and astrocyte is implicated in EphrinB/EphB signaling dependent neuropathic pain formation in a mouse model of CCI (Yang et al., 2018) (Table 2).
Furthermore, spinal caspase-3 cleavage is required for axonal degeneration, mitochondrial dysfunction, oxidative stress and apoptosis in the pathogenesis of CIPN after intraperitoneal vincristine stimulation in mice (Chen et al., 2020). The over-expression of apoptosis-related proteins of BAX, BCL2, and caspase-3 in the sciatic nerve is reported in streptozotocin (STZ)-induced diabetic peripheral neuropathy in rats (Yu et al., 2021). Blocking caspase-3 signaling cascades can reduce spinal neuronal apoptosis and down-regulate nociceptor hyper-responsiveness, which may emerge as a promising strategy for the treatment of neuropathic pain. Conversely, peripheral nerve injury induced by common peroneal nerve (CPN) ligation blocks long-term depression (LTD) induction and caspase-3 expression in the anterior cingulate cortex (ACC). Electrophysiological and behavioral tests found that disrupting the link between caspase-3 and α-amino-3-hydroxy-5-methyl-4-isoxazolepropionic acid (AMPA) receptor in the ACC inhibits LTD expression and induces peripheral pro-nociception phenotypes (Wang YJ. et al., 2020). Restoration of LTD through caspase-3 accumulation in the ACC relieves persistent mechanical allodynia (Wang YJ. et al., 2020), which may be used as a promising therapeutic approach for the management of chronic pain (Table 2). Acetyl-l-carnitine (ALCAR) is a short chain ester of carnitine L-isomer, which is the predominant acyl-carnitine and is involved in the redox reactions to eliminate reactive oxygen species, and finally, can increase acetylcholine levels, thus having neuroprotective action. Di Cesare Mannelli et al. (2007) found the decreased effect of ALCAR on the induction of apoptosis, the release of cytosolic cytochrome C, the activation of caspase-3, and the fragmentation of the genome in CCI rats. And that means ALCAR may be an agent suitable for clinical use in the prevention of nervous tissue cell death after peripheral nerve trauma via the inhibition by caspase-3. Di Cesare Mannelli et al. (2013) also found that antioxidants (such as silibinin and α-tocopherol) can ameliorate the symptoms of neuropathy and protect astrocytes from caspase-3-dependent apoptotic signaling activation in oxaliplatin-treated rats.
Caspase-6 is also tightly associated with the pathophysiology of neuropathic pain. Peripheral nerve injury after CCI intervention is demonstrated to cause the release of activating transcription factor-3 (ATF3) and caspase-6 from axonal terminal, which then acts on microglia to trigger their activation. After microglial activation, p38 will be phosphorylated to further induce TNF-α release, and brain-derived neuro-trophic factor (BDNF) expression, which inducing central sensitization and supporting the transition from acute pain to chronic pain (Berta et al., 2016). Caspase-6 inhibition significantly reverse the development of mechanical allodynia in a rat model of neuropathic pain following spared nerve injury (SNI) of sciatic nerve. Moreover, caspase-6 deletion attenuated behavioral mechanical allodynia in the paclitaxel-induced CIPN, although the mechanisms that produce neuropathic pain after exposure to chemotherapeutics may be fundamentally different from those operating after nerve injury (Berta et al., 2017b). (Table 3)
In recent years, in addition to caspase-1, caspase-3 and caspase-6, other caspases have gained great emphasis on neuropathic pain syndromes. Specifically, increase of caspase-9, apoptosis, mitochondrial reactive free oxygen species (fROS), lipid peroxidation, glutathione (GSH), transient receptor potential vanilloid-1 (TRPV1) current density, and calcium concentrations in the DRG and hippocampus was detected in STZ-induced diabetic peripheral mechanical allodynia and thermal hyperalgesia (Düzova et al., 2021). Application of metabotropic glutamate receptor 1 (mGluR1) antagonist can prevent CCI-induced neuropathic pain by reducing the synthesis of caspase-7 in the spinal dorsal horn and inhibiting the process of caspase-7 dependent neuronal apoptosis (Siniscalco et al., 2008). However, whether caspase-9 and caspase-7 inhibitors can provide definitive relief of chronic pain is a scientific question that requires to be addressed.
Inflammatory pain is evoked by inflammation-associated stimuli and is often established in animal models using wound incision or injection of inflammatory chemical irritants, such as complete Freund’s adjuvant (CFA), carrageenan or lipopolysaccharides (LPS) (Pan et al., 2021). Recent literatures have demonstrated that intraplantar administration of complete Freund’s adjuvant (CFA) or ceramide not only down-regulates paw withdrawal mechanical threshold and paw withdrawal thermal latency, but also up-regulates the expression of the NOD-like receptor protein 2 (NLRP2)/caspase-1/IL-1β in small-sized DRG primary sensory neurons and the generation of NLRP3/caspase-1 in spinal dorsal horn neurons (Matsuoka et al., 2019; Hua et al., 2022). Intrathecal injection of a caspase-1 inhibitor Z-YVAD-FMK impairs CFA-induced inflammatory pain hypersensitivity through inhibiting IL-1β secretion in DRG (Matsuoka et al., 2019). Liang et al. also found, in the mouse model of hind paw incision, that caspase-1 activity was significantly increased in peri-incisional tissues. Caspase-1 inhibitor VRTXSD727 significantly reverses mechanical allodynia and thermal hyperalgesia, and reduces the synthesis and release of macrophage inflammatory protein-1α (MIP-1α), granulocyte-colony stimulating factor (G-CSF), Prostaglandin E2 (PGE2), as well as IL-1β around the wound incision (Liang et al., 2010). Additionally, caspase-1 knockout mice exhibit the impairment in the mechanical allodynia induced by intraplantar exposure to carrageenin, TNF-a and exogenous CXCL1, respectively. Meanwhile, caspase-1 deficiency suppresses carrageenin-induced PGE2 production, IL-1β maturation and cyclooxygenase-2 (COX-2) accumulation (Cunha et al., 2010). These detailed evidences strongly suggest the importance of caspase-1-dependent inflammatory cascades in the pathophysiology of inflammatory hyper-nociception (Table 1).
Caspase-3 plays an important role in inflammatory pain. N-methyl-d-aspartate (NMDA) receptor, an ionotropic glutaminergic receptor, consists of the primary GluN1 subunit and one or more GluN2A-D modulatory subunits (Zhang et al., 2014). Activation of NMDA receptor is a leading determinant in the hyper-excitability of nociceptive neurons and central synaptic plasticity underlying pain-associated syndromes (Chen et al., 2016; Xu et al., 2020). The excitotoxicity of NMDA receptor is GluN2B dependent (Zhang et al., 2021). Notably, CFA injection into hind paw can not only aggravate neuronal apoptosis but also increase the expressions of Bax, caspase-3 and GluN2B-containing NMDA receptors in the ACC. Inhibiting caspase-3-dependent cascades protects neuronal survival, reduces GluN2B-containing NMDA receptor electrophysiological function and attenuates chronic inflammation-induced mechanical allodynia and thermal hyperalgesia (Fan et al., 2018) (Table 2).
In 2014, a preclinical study by Berta et al. showed that caspase-6 is specifically expressed in C-fiber axonal terminals in the superficial spinal cord dorsal horn, and co-localizes with calcitonin-gene-related peptide (CGRP), suggesting the transportation of caspase-6 in peptidergic primary afferents to spinal central terminals, which sustaining nociception-related synaptic potency. Moreover, injections of formalin or bradykinin into the plantar induce the cleavage and activation of caspase-6 in nociceptive neurons of spinal dorsal horn. Spinal application of specific caspase-6 inhibitor Z-VEID-FMK or caspase-6 neutralizing antibody or delivery of caspase-6 siRNA around the sciatic nerve can effectively relieve the inflammatory pain induced by formalin intervention. Similarly, caspase-6 gene knockout reduces bradykinin-induced spontaneous pain, CFA-induced mechanical allodynia, and carrageenan-induced heat hyperalgesia, respectively. In addition, spinal exposure to recombinant caspase-6 not only facilitates microgliosis and microglial activation to result in TNF-α secretion, but also increases glutamate release from primary afferent terminals to enhance excitatory postsynaptic currents (Berta et al., 2014). These detailed results identify that caspase-6 activation may be a predominant feature of neuroinflammation and neuron-microglia interaction, as well as a key driver of synaptic plasticity and central sensitization, thereby mediating persistent inflammatory pain. Thus, targeting the caspase-6/TNF-α cascades may offer a novel choice for treating inflammatory pain states by microglial and synaptic modulation.
In addition, recent report recapitulates the elevated concentration of caspase-11, NOD-like receptor C4 (NLRC4), ASC, and IL-1β in the brain and spinal cord of mice with lipopolysaccharide (LPS)-induced inflammatory heat hyperalgesia (Cagli et al., 2021), suggesting the implication of caspase-11-dependent NLRC4 inflammasome in pain perception.
Cancer pain is also an important category of chronic pain and have the distinctive characteristic of both neuropathic pain and inflammatory pain processing (Wang K. et al., 2020; Wang K. et al., 2021). Mounting evidence reveal that the expression of NLRP3 inflammasome, including NLRP3, apoptosis-associated speck-like protein containing a caspase activation and recruitment domain (ASC), and caspase-1, were significantly increased in a time-dependent manner in bone cancer pain (Chen et al., 2019). Behavioral tests confirmed that both single and repetitive treatment with NLRP3 inhibitor MCC950 markedly attenuated cancer pain behaviors (Chen et al., 2019), suggesting that the activation of NLRP3/ASC/Caspase-1 signaling cascades is an essential step for the initiation and maintenance of central pain sensitization following bone cancer (Table 1).
Bioactivity of endoplasmic reticulum (ER) is vital for life yet toxic if dysfunction of ER occurs. Especially, ER stress plays a positive role in acute pain perception and chronic pain sensitization (Zhang E. et al., 2015; Inceoglu et al., 2015). Bone cancer pain not only induces bone destruction and unbearable mechanical allodynia, but also up-regulates the spinal expression of ER stress markers, including glucose-regulated protein 78 (GRP78), activating transcription factor-6 (ATF6), phosphorylated protein kinase RNA-like endoplasmic reticulum kinase (p-PERK), phosphorylated inositol-requiring enzyme-1 (p-IRE1) and cleaved caspase-3. Intrathecal blockage of ER stress impairs caspase-3 cleavage-dependent apoptosis in dorsal horn neurons and relieves bone cancer pain. More importantly, spinal therapy with a specific caspase-3 inhibitor Z-DEVD-FMK is enough and effective against bone cancer pain (He et al., 2019). Also, the modulation of Bax overload and caspase-3 cleavage in mitochondrial fission and apoptosis in bone cancer pain is confirmed by other research teams (Li MY. et al., 2019; Chen P. et al., 2021). Overall, this suggests that preventing ER stress-induced cellular dysfunction and caspase-3-dependent neuronal apoptosis may be a new approach for treating cancer pain (Table 2).
Musculoskeletal pain refers to pain in the muscles, bones, ligaments, tendons, and nerves. Chronic musculoskeletal pain patients in general show signs of peripheral/central sensitization. Dynamic recruitment of GluA1-containing AMPAR at spinal synapses contributes to central sensitization underlying pain-associated syndromes (Luo et al., 2014; Zhang et al., 2018; Wang Z. et al., 2020; Liu et al., 2020). Leucine-rich repeat transmembrane protein 1 (LRRTM1) is demonstrated to mediate post-synaptic translocation of AMPA receptor and synaptogenesis (Bhouri et al., 2018; Schroeder et al., 2018). But the regulation of LRRTM1 in pain development remains underestimated. We recently revealed that tibial fracture with intramedullary pinning causes long-lasting mechanical allodynia and cold allodynia after orthopedic surgery in mice, along with the upregulated caspase-3 activity (but not caspase-3 protein expression) and LRRTM1 expression in spinal dorsal horn (Zhang et al., 2020). Pharmacological intervention with caspase-3 specific inhibitor Z-DEVD-FMK reduces fracture-associated behavioral pain and LRRTM1-mediated alterations in excitatory synaptic plasticity. Spinal exposure to recombinant caspase-3 evoked acute pain phenotypes and spinal LRRTM1 over-expression is reversed by LRRTM1 deficiency (Zhang et al., 2020). Collectively, this demonstrates the tight interaction between caspase-3 and LRRTM1 in inducing AMPA receptor trafficking and chronic central sensitization, ultimately regulating the formation and maintenance of fracture-associated pain. Sure, it will be of great interest to investigate how fracture trauma regulates caspase-3 activation and thus mediates the onset of chronic pain (Table 2).
Our latest study provides several lines of evidence to confirm the requirement of caspase-6 in musculoskeletal pain induced by tibial fracture with intramedullary pinning (Cui W. et al., 2021). First, behavioral tests showed that tibial fractures after orthopedic operation initiate and persist postsurgical mechanical allodynia and cold allodynia, which is detectable on 3 days, peaks on 7–14 days, and sustains for at least 21 days. Second, biochemical tests found that tibial fracture up-regulates spinal active caspase-6 activity, GluA1-containing AMPA receptor trafficking, spine density in dorsal horn neurons. Third, spinal delivery of specific caspase-6 inhibitor Z-VEID-FMK and caspase-6 neutralizing antibody is sufficient to reduce the recruitment of GluA1-containing AMPA receptor at synapses and the amounts of mushroom spines, thereby attenuating fracture-associated chronic pain. Fourth, electrophysiological tests manifested that pharmacological inhibition of caspase-6 blocks AMPA receptor-mediated excitatory post-synaptic currents in the dorsal horn neurons in fracture animals (Cui W. et al., 2021). These above-mentioned results demonstrate that caspase-6-mediated changes in excitatory synaptic structure and functional plasticity is an important mechanism for the formation and maintenance of spinal nociception sensitization after fracture and orthopedic surgery, which provides a promising approach for chronic fracture pain therapy. However, there are several outstanding questions regarding how caspase-6 mediates AMPA receptor post-synaptic trafficking and ultimately triggers musculoskeletal pain. Simultaneously, in addition to the mechanism of affecting receptor transport, future researches are warranted to explore whether there are epigenetic regulations that interfere with the expression of glutamate receptors.
The requirement of caspase-1 for postoperative pain development has also been clarified. Extension of laparotomy-associated postoperative pain after morphine treatment is attributed to up-regulation of inflammatory genes, including those encoding caspase-1, NLRP3, TLR4, NF-κB, IL-1β, and TNF-α (Grace et al., 2019). Thoracotomy induces persistent postoperative behavioral pain, along with the spinal up-modulation of caspase-1 and TLR4 co-localization in dorsal horn neurons (Hu et al., 2020). The decrease of mechanical withdrawal threshold is attributed to caspase-1-dependent microglial activation and the overload of inflammatory mediators (TNF-α, IL-6, and IL-1β) in spinal dorsal horn. Additionally, the alleviation of postoperative mechanical allodynia by intrathecal therapy of caspase-1 inhibitor (Ac-YVAD-CMK) is reversed after TLR4 agonist treatment (Hu et al., 2020), further indicating the tight interaction between caspase-1 and TLR4 in spinal nociception transduction and central sensitization (Table 1).
Remifentanil is a potent short-acting µ-opioid agonist, regarded as an important component of balanced anesthesia in the clinical setting. Unfortunately, the intraoperative exposure to remifentanil elicits behavioral OIH phenotype in animals and patients (Zhang et al., 2017; Zhang et al., 2018; Li et al., 2021). Population-based studies also found that remifentanil can elevate peripheral mechanical nociceptive sensitivity, elicit hyperalgesia area around the wound incision, and trigger OIH, even leading to chronicity of postoperative pain (Fletcher and Martinez, 2014; Zhang et al., 2016). Chemokines and their receptors-associated neuroinflammation is essential for OIH generation (Yang et al., 2016; Zhu et al., 2017). Wang et al. (2020a) confirmed that remifentanil infusion causes mechanical allodynia and thermal hyperalgesia, along with the spinal increase in the cleavage of caspase-6, the release of CCL21 in neurons and the expression of CXCR3 in microglia. Spinal inhibition of caspase-6 activation ameliorates OIH behavior and spinal CCL21/CXCR3 accumulation. Exogenous caspase-6 also evokes acute mechanical pain and represents microglial activation, which is impaired after spinal CCL21 neutralization. This suggests the contribution of caspase-6 in CCL21 signaling in chronic pain perception. However, the downstream target molecules through which caspase-6 upregulates the synthesis of CCL21 need to be further investigated. As a result, interactions of microglia-neurons are triggered by caspase-6 activation in synaptic plasticity and the formation and maintenance of chronic pain, as the potential pain circuits in the spinal cord dorsal horn is shown in Figure 1.
FIGURE 1. Caspase-6 cascades-mediated neuron-microglia interaction in the spinal cord initiates chronic pain through neuroinflammation and central nociception sensitization. Chronic pain is categorized as inflammatory pain, neuropathic pain, cancer pain, musculoskeletal pain, and drug treatment-induced pain, which is driven by neuroinflammation in the spinal cord dorsal horn. Painful insults, which includes peripheral tissue inflammation, nerve trauma, fracture with orthopedic surgery, chemotherapy and opioids treatment, result in the hyperexcitability of primary sensory neurons and trigger the release of caspase-6 from the central terminals of primary nociceptive afferents, which causing microgliosis and microglia activation, and subsequent microglial TNF-α secretion. Then, the interaction between TNF-α and TNFR at presynaptic sites causes the release of glutamate via ERK and TRPV1 pathway. Activation of TNFR at postsynaptic neurons also facilitates the phosphorylation of ERK, which drives central sensitization via positive modulations of NMDAR and AMPAR and subsequent intracellular calcium influx. Simultaneously, caspase-6 cleavage promotes chemokine CCL21 release from presynaptic neurons, which elicits microglia activation via acting on its specific receptor CXCR3. Microglia activation further increases the secretion of the pro-inflammatory mediators (IL-1β, IL-18, and PGE2). These regulations of excitatory synaptic transmission by microglial mediators at pre-synaptic, post-synaptic, and extra-synaptic sites drive central sensitization in the nociception circuits, leading to the development of chronic pain. Abbreviations: AMPAR, a-amino-3-hydroxy-5-methyl-4-isoxazolepropionic acid receptor; CCL21, chemokine (C-C motif) ligand 21; CXCR3, chemokine (C-X-C motif) receptor 3; ERK, extracellular signal-regulated kinase; IL-1β, interleukin-1β; IL-18, interleukin-18; NMDAR, N-methyl-D-aspartate receptor; p-ERK, ERK phosphorylation; PGE2, prostaglandin E2; TNF-α, tumor necrosis factor-α; TNFR, tumor necrosis factor receptor; TRPV1: transient receptor potential vanilloid-1.
Despite decades of clinical investigation and medical advancement, current approaches for chronic pain-relief are still limited (Tracey et al., 2019). Non-steroidal anti-inflammatory drugs and acetaminophen must be cautiously administered in patients with gastrointestinal diseases, renal dysfunction and hepatic insufficiency (Bindu et al., 2020; Ishitsuka et al., 2020). Tricyclic antidepressants, norepinephrine reuptake inhibitors, NMDA receptor antagonists and α2-δ anticonvulsants are only partially beneficial to neuropathic pain and several dose-limiting adverse-effects including sedation, somnolence and dizziness may block their practical utilization (Staahl et al., 2009; Thompson et al., 2019). Opioids, as 1st-line analgesics, frequently cause constipation, nausea, addiction, tolerance and hyperalgesia (Zhang et al., 2016; Imam et al., 2018; Colvin et al., 2019). Thus, alternative agents for pain control are urgently required. In view of the pivotal role of caspases in the pathogenesis of chronic pain, their targeting agents in the management of chronic pain have been identified as above-mentioned. The summarization and detail on therapeutic value of these drugs are shown in Table 4.
We have summarized the role of the caspases in the development of chronic pain with different etiologies, with a view to providing new ideas for the management of chronic pain. Although significant progress has been made in preclinical study, most of the studies have only been conducted at the behavioral level, and the upstream and downstream molecular mechanisms have been poorly investigated, so there are plenty of issues that still need to be addressed. Future research should include the following directions: 1) high selective inhibitors developed for caspase-1, caspase-3 or caspase-6 can release chronic pain by inhibiting neuroinflammation, altered excitatory synaptic plasticity or neuronal apoptosis in animal models; however, whether these inhibitors can alleviate chronic pain in patients and be safely utilized in clinics remains to be explored. 2) In addition to caspases-1, 3, 6, 7, 9, and 11, it has not been conclusively established whether other members of the caspase family are also involved in the regulation of chronic pain. 3) most studies on the role of caspases in the occurrence and development of chronic pain were conducted in male animals. Considering the sex differences in the formation mechanism of chronic pain (Chen et al., 2018; Luo et al., 2018; Luo et al., 2019a; Luo et al., 2019b; Luo et al., 2021), future research should emphasize whether the caspase signaling is involved in the formation of chronic pain in female animals. 4) Despite recent advances in pain therapy, visceral pain remains poorly understood. Recent study confirms that antibiotic-induced microbial changes resulted in neuro-immune responses and visceral pain attenuation in wild type but not in caspase-1/11 knockout mice (Aguilera et al., 2021), supported the notion of the inflammasome as a promising therapeutic target in the visceral pain. Therefore, further study of caspase family and visceral pain will be a promising field. 5) It is unclear whether there is any interaction between different caspases in specific pain conditions, which needs further investigations. Anyway, the findings of the above will further provide new interventional targets for the management of chronic pain and promote the development of novel drugs related to the caspase cascades.
LZ and HZ designed, collected literatures, and wrote the manuscript; NL, ZL, and YL collected some literatures and created figures and tables; LZ and YY revised the manuscript.
This work was supported by research grants of National Natural Science Foundation of China to LZ (Grant nos. 82171205 and 81801107) and National Natural Science Foundation of China to YL (Grant no. 82071243).
The authors declare that the research was conducted in the absence of any commercial or financial relationships that could be construed as a potential conflict of interest.
All claims expressed in this article are solely those of the authors and do not necessarily represent those of their affiliated organizations, or those of the publisher, the editors and the reviewers. Any product that may be evaluated in this article, or claim that may be made by its manufacturer, is not guaranteed or endorsed by the publisher.
Agnes, J. P., Santos, V. W. D., das Neves, R. N., Gonçalves, R. M., Delgobo, M., Girardi, C. S., et al. (2021). Antioxidants Improve Oxaliplatin-Induced Peripheral Neuropathy in Tumor-Bearing Mice Model: Role of Spinal Cord Oxidative Stress and Inflammation. J. Pain. 22, 996–1013. doi:10.1016/j.jpain.2021.03.142
Aguilera, M., Rossini, V., Hickey, A., Simnica, D., Grady, F., Felice, V. D., et al. (2021). Inflammasome Signaling Regulates the Microbial-Neuroimmune Axis and Visceral Pain in Mice. Int. J. Mol. Sci. 22. doi:10.3390/ijms22158336
Al-Massri, K. F., Ahmed, L. A., and El-Abhar, H. S. (2018). Pregabalin and Lacosamide Ameliorate Paclitaxel-Induced Peripheral Neuropathy via Inhibition of JAK/STAT Signaling Pathway and Notch-1 Receptor. Neurochem. Int. 120, 164–171. doi:10.1016/j.neuint.2018.08.007
Baral, P., Udit, S., and Chiu, I. M. (2019). Pain and Immunity: Implications for Host Defence. Nat. Rev. Immunol. 19, 433–447. doi:10.1038/s41577-019-0147-2
Berta, T., Lee, J. E., and Park, C. K. (2017a). Unconventional Role of Caspase-6 in Spinal Microglia Activation and Chronic Pain. Mediators Inflamm. 2017, 9383184. doi:10.1155/2017/9383184
Berta, T., Park, C. K., Xu, Z. Z., Xie, R. G., Liu, T., Lü, N., et al. (2014). Extracellular Caspase-6 Drives Murine Inflammatory Pain via Microglial TNF-α Secretion. J. Clin. Invest. 124, 1173–1186. doi:10.1172/jci72230
Berta, T., Perrin, F. E., Pertin, M., Tonello, R., Liu, Y. C., Chamessian, A., et al. (2017b). Gene Expression Profiling of Cutaneous Injured and Non-injured Nociceptors in SNI Animal Model of Neuropathic Pain. Sci. Rep. 7, 9367. doi:10.1038/s41598-017-08865-3
Berta, T., Qadri, Y. J., Chen, G., and Ji, R. R. (2016). Microglial Signaling in Chronic Pain with a Special Focus on Caspase 6, P38 MAP Kinase, and Sex Dependence. J. Dent. Res. 95, 1124–1131. doi:10.1177/0022034516653604
Bhouri, M., Morishita, W., Temkin, P., Goswami, D., Kawabe, H., Brose, N., et al. (2018). Deletion of LRRTM1 and LRRTM2 in Adult Mice Impairs Basal AMPA Receptor Transmission and LTP in Hippocampal CA1 Pyramidal Neurons. Proc. Natl. Acad. Sci. U. S. A. 115, E5382–e5389. doi:10.1073/pnas.1803280115
Bindu, S., Mazumder, S., and Bandyopadhyay, U. (2020). Non-steroidal Anti-inflammatory Drugs (NSAIDs) and Organ Damage: A Current Perspective. Biochem. Pharmacol. 180, 114147. doi:10.1016/j.bcp.2020.114147
Bliss, T. V., Collingridge, G. L., Kaang, B. K., and Zhuo, M. (2016). Synaptic Plasticity in the Anterior Cingulate Cortex in Acute and Chronic Pain. Nat. Rev. Neurosci. 17, 485–496. doi:10.1038/nrn.2016.68
Cagli, A., Senol, S. P., Temiz-Resitoglu, M., Guden, D. S., Sari, A. N., Sahan-Firat, S., et al. (2021). Soluble Epoxide Hydrolase Inhibitor Trifluoromethoxyphenyl-3-(1-Propionylpiperidin-4-Yl)urea Prevents Hyperalgesia through Regulating NLRC4 Inflammasome-Related Pro-inflammatory and Anti-inflammatory Signaling Pathways in the Lipopolysaccharide-Induced Pain Mouse Model. Drug Dev. Res. 82, 815–825. doi:10.1002/ddr.21786
Chen, G., Luo, X., Qadri, M. Y., Berta, T., and Ji, R. R. (2018). Sex-Dependent Glial Signaling in Pathological Pain: Distinct Roles of Spinal Microglia and Astrocytes. Neurosci. Bull. 34, 98–108. doi:10.1007/s12264-017-0145-y
Chen, G., Xie, R. G., Gao, Y. J., Xu, Z. Z., Zhao, L. X., Bang, S., et al. (2016). β-arrestin-2 Regulates NMDA Receptor Function in Spinal Lamina II Neurons and Duration of Persistent Pain. Nat. Commun. 7, 12531. doi:10.1038/ncomms12531
Chen, P., Pan, M., Lin, Q. S., Lin, X. Z., and Lin, Z. (2021a). CSF-CN Contributes to Cancer-Induced Bone Pain via the MKP-1-Mediated MAPK Pathway. Biochem. Biophys. Res. Commun. 547, 36–43. doi:10.1016/j.bbrc.2021.02.010
Chen, R., Yin, C., Fang, J., and Liu, B. (2021b). The NLRP3 Inflammasome: an Emerging Therapeutic Target for Chronic Pain. J. Neuroinflammation. 18, 84. doi:10.1186/s12974-021-02131-0
Chen, S. P., Zhou, Y. Q., Wang, X. M., Sun, J., Cao, F., HaiSam, S., et al. (2019). Pharmacological Inhibition of the NLRP3 Inflammasome as a Potential Target for Cancer-Induced Bone Painammasome as a Potential Target for Cancer-Induced Bone Pain. Pharmacol. Res. 147, 104339. doi:10.1016/j.phrs.2019.104339
Chen, X. J., Wang, L., and Song, X. Y. (2020). Mitoquinone Alleviates Vincristine-Induced Neuropathic Pain through Inhibiting Oxidative Stress and Apoptosis via the Improvement of Mitochondrial Dysfunction. Biomed. Pharmacother. 125, 110003. doi:10.1016/j.biopha.2020.110003
Chine, V. B., Au, N. P. B., Kumar, G., and Ma, C. H. E. (2019). Targeting Axon Integrity to Prevent Chemotherapy-Induced Peripheral Neuropathy. Mol. Neurobiol. 56, 3244–3259. doi:10.1007/s12035-018-1301-8
Choi, S. S., Koh, W. U., Nam, J. S., Shin, J. W., Leem, J. G., and Suh, J. H. (2013). Effect of Ethyl Pyruvate on Paclitaxel-Induced Neuropathic Pain in Rats. Korean J. Pain 26, 135–141. doi:10.3344/kjp.2013.26.2.135
Chou, R., and Huffman, L. H. (2007). Medications for Acute and Chronic Low Back Pain: a Review of the Evidence for an American Pain Society/American College of Physicians Clinical Practice Guideline. Ann. Intern. Med. 147, 505–514. doi:10.7326/0003-4819-147-7-200710020-00008
Clark, A. K., D'Aquisto, F., Gentry, C., Marchand, F., McMahon, S. B., and Malcangio, M. (2006). Rapid Co-release of Interleukin 1beta and Caspase 1 in Spinal Cord Inflammation. J. Neurochem. 99, 868–880. doi:10.1111/j.1471-4159.2006.04126.x
Colvin, L. A., Bull, F., and Hales, T. G. (2019). Perioperative Opioid Analgesia-When Is Enough Too Much? A Review of Opioid-Induced Tolerance and Hyperalgesia. Lancet 393, 1558–1568. doi:10.1016/s0140-6736(19)30430-1
Cui, J., Fan, J., Li, H., Zhang, J., and Tong, J. (2021a). Neuroprotective Potential of Fisetin in an Experimental Model of Spinal Cord Injury: via Modulation of NF-Κb/iκbα Pathway. Neuroreport 32, 296–305. doi:10.1097/wnr.0000000000001596
Cui, W., Li, Y., Wang, Z., Song, C., Yu, Y., Wang, G., et al. (2021b). Spinal Caspase-6 Regulates AMPA Receptor Trafficking and Dendritic Spine Plasticity through Netrin-1 in Postoperative Pain after Orthopedic Surgery for Tibial Fracture in Mice. Pain 162, 124–134. doi:10.1097/j.pain.0000000000002021
Cunha, T. M., Talbot, J., Pinto, L. G., Vieira, S. M., Souza, G. R., Guerrero, A. T., et al. (2010). Caspase-1 Is Involved in the Genesis of Inflammatory Hypernociception by Contributing to Peripheral IL-1β Maturation. Mol. Pain 6, 63. doi:10.1186/1744-8069-6-63
Deng, X. T., Wu, M. Z., Xu, N., Ma, P. C., and Song, X. J. (2017). Activation of ephrinB-EphB Receptor Signalling in Rat Spinal Cord Contributes to Maintenance of Diabetic Neuropathic Pain. Eur. J. Pain. 21, 278–288. doi:10.1002/ejp.922
Di Cesare Mannelli, L., Ghelardini, C., Calvani, M., Nicolai, R., Mosconi, L., Vivoli, E., et al. (2007). Protective Effect of Acetyl-L-Carnitine on the Apoptotic Pathway of Peripheral Neuropathy. Eur. J. Neurosci. 26, 820–827. doi:10.1111/j.1460-9568.2007.05722.x
Di Cesare Mannelli, L., Zanardelli, M., Failli, P., and Ghelardini, C. (2013). Oxaliplatin-induced Oxidative Stress in Nervous System-Derived Cellular Models: Could it Correlate with In Vivo Neuropathy? Free Radic. Biol. Med. 61, 143–150. doi:10.1016/j.freeradbiomed.2013.03.019
Düzova, H., Nazıroğlu, M., Çiğ, B., Gürbüz, P., and Akatlı, A. N. (2021). Noopept Attenuates Diabetes-Mediated Neuropathic Pain and Oxidative Hippocampal Neurotoxicity via Inhibition of TRPV1 Channel in Rats. Mol. Neurobiol. 58, 5031–5051. doi:10.1007/s12035-021-02478-8
Fan, Y. F., Guan, S. Y., Luo, L., Li, Y. J., Yang, L., Zhou, X. X., et al. (2018). Tetrahydroxystilbene Glucoside Relieves the Chronic Inflammatory Pain by Inhibiting Neuronal Apoptosis, Microglia Activation, and GluN2B Overexpression in Anterior Cingulate Cortex. Mol. Pain 14, 1744806918814367. doi:10.1177/1744806918814367
Fletcher, D., and Martinez, V. (2014). Opioid-induced Hyperalgesia in Patients after Surgery: a Systematic Review and a Meta-Analysis. Br. J. Anaesth. 112, 991–1004. doi:10.1093/bja/aeu137
Flores, J., Noël, A., Foveau, B., Lynham, J., Lecrux, C., and LeBlanc, A. C. (2018). Caspase-1 Inhibition Alleviates Cognitive Impairment and Neuropathology in an Alzheimer's Disease Mouse Model. Nat. Commun. 9, 3916. doi:10.1038/s41467-018-06449-x
Fu, H., Li, F., Thomas, S., and Yang, Z. (2017). Hyperbaric Oxygenation Alleviates Chronic Constriction Injury (CCI)-induced Neuropathic Pain and Inhibits GABAergic Neuron Apoptosis in the Spinal Cord. Scand. J. Pain 17, 330–338. doi:10.1016/j.sjpain.2017.08.014
Gao, F., Xiang, H. C., Li, H. P., Jia, M., Pan, X. L., Pan, H. L., et al. (2018). Electroacupuncture Inhibits NLRP3 Inflammasome Activation through CB2 Receptors in Inflammatory Pain. Brain Behav. Immun. 67, 91–100. doi:10.1016/j.bbi.2017.08.004
García, G., Martínez-Rojas, V. A., Oviedo, N., and Murbartián, J. (2018). Blockade of Anoctamin-1 in Injured and Uninjured Nerves Reduces Neuropathic Pain. Brain Res. 1696, 38–48. doi:10.1016/j.brainres.2018.06.001
Gereau, R. W., Sluka, K. A., Maixner, W., Savage, S. R., Price, T. J., Murinson, B. B., et al. (2014). A Pain Research Agenda for the 21st century. J. Pain 15, 1203–1214. doi:10.1016/j.jpain.2014.09.004
Grace, P. M., Galer, E. L., Strand, K. A., Corrigan, K., Berkelhammer, D., Maier, S. F., et al. (2019). Repeated Morphine Prolongs Postoperative Pain in Male Rats. Anesth. Analg. 128, 161–167. doi:10.1213/ane.0000000000003345
Grace, P. M., Gaudet, A. D., Staikopoulos, V., Maier, S. F., Hutchinson, M. R., Salvemini, D., et al. (2016a). Nitroxidative Signaling Mechanisms in Pathological Pain. Trends Neurosci. 39, 862–879. doi:10.1016/j.tins.2016.10.003
Grace, P. M., Strand, K. A., Galer, E. L., Rice, K. C., Maier, S. F., and Watkins, L. R. (2018). Protraction of Neuropathic Pain by Morphine Is Mediated by Spinal Damage Associated Molecular Patterns (DAMPs) in Male Rats. Brain Behav. Immun. 72, 45–50. doi:10.1016/j.bbi.2017.08.018
Grace, P. M., Strand, K. A., Galer, E. L., Urban, D. J., Wang, X., Baratta, M. V., et al. (2016b). Morphine Paradoxically Prolongs Neuropathic Pain in Rats by Amplifying Spinal NLRP3 Inflammasome Activation. Proc. Natl. Acad. Sci. U. S. A. 113, E3441–E3450. doi:10.1073/pnas.1602070113
Graham, R. K., Ehrnhoefer, D. E., and Hayden, M. R. (2011). Caspase-6 and Neurodegeneration. Trends Neurosci. 34, 646–656. doi:10.1016/j.tins.2011.09.001
Hajimashhadi, Z., Aboutaleb, N., and Nasirinezhad, F. (2017). Chronic Administration of [Pyr1] Apelin-13 Attenuates Neuropathic Pain after Compression Spinal Cord Injury in Rats. Neuropeptides 61, 15–22. doi:10.1016/j.npep.2016.08.010
Han, Q., Kim, Y. H., Wang, X., Liu, D., Zhang, Z. J., Bey, A. L., et al. (2016). SHANK3 Deficiency Impairs Heat Hyperalgesia and TRPV1 Signaling in Primary Sensory Neurons. Neuron 92, 1279–1293. doi:10.1016/j.neuron.2016.11.007
He, Q., Wang, T., Ni, H., Liu, Q., An, K., Tao, J., et al. (2019). Endoplasmic Reticulum Stress Promoting Caspase Signaling Pathway-dependent Apoptosis Contributes to Bone Cancer Pain in the Spinal Dorsal Horn. Mol. Pain 15, 1744806919876150. doi:10.1177/1744806919876150
Hu, Q., Fang, L., Li, F., Thomas, S., and Yang, Z. (2015). Hyperbaric Oxygenation Treatment Alleviates CCI-Induced Neuropathic Pain and Decreases Spinal Apoptosis. Eur. J. Pain. 19, 920–928. doi:10.1002/ejp.618
Hu, Z., Liao, J., Zhang, F., Qing, W., Yu, R., and Liao, Q. (2020). Mechanism of Toll-like Receptor 4 on Chronic Post-thoracotomy Pain via Caspase-1 Activation. Curr. Neurovasc. Res. 17, 667–675. doi:10.2174/1567202617666201106150620
Hua, T., Wang, H., Fan, X., An, N., Li, J., Song, H., et al. (2022). BRD4 Inhibition Attenuates Inflammatory Pain by Ameliorating NLRP3 Inflammasome-Induced Pyroptosis. Front. Immunol. 13, 837977. doi:10.3389/fimmu.2022.837977
Huang, Z. Z., Li, D., Liu, C. C., Cui, Y., Zhu, H. Q., Zhang, W. W., et al. (2014). CX3CL1-mediated Macrophage Activation Contributed to Paclitaxel-Induced DRG Neuronal Apoptosis and Painful Peripheral Neuropathy. Brain Behav. Immun. 40, 155–165. doi:10.1016/j.bbi.2014.03.014
Imam, M. Z., Kuo, A., Ghassabian, S., and Smith, M. T. (2018). Progress in Understanding Mechanisms of Opioid-Induced Gastrointestinal Adverse Effects and Respiratory Depression. Neuropharmacology 131, 238–255. doi:10.1016/j.neuropharm.2017.12.032
Inceoglu, B., Bettaieb, A., Trindade da Silva, C. A., Lee, K. S., Haj, F. G., and Hammock, B. D. (2015). Endoplasmic Reticulum Stress in the Peripheral Nervous System Is a Significant Driver of Neuropathic Pain. Proc. Natl. Acad. Sci. U. S. A. 112, 9082–9087. doi:10.1073/pnas.1510137112
Ishitsuka, Y., Kondo, Y., and Kadowaki, D. (2020). Toxicological Property of Acetaminophen: The Dark Side of a Safe Antipyretic/Analgesic Drug? Biol. Pharm. Bull. 43, 195–206. doi:10.1248/bpb.b19-00722
Ji, R. R., Chamessian, A., and Zhang, Y. Q. (2016). Pain Regulation by Non-neuronal Cells and Inflammation. Science 354, 572–577. doi:10.1126/science.aaf8924
Ji, R. R., Nackley, A., Huh, Y., Terrando, N., and Maixner, W. (2018). Neuroinflammation and Central Sensitization in Chronic and Widespread Pain. Anesthesiology 129, 343–366. doi:10.1097/aln.0000000000002130
Ji, R. R., Xu, Z. Z., and Gao, Y. J. (2014). Emerging Targets in Neuroinflammation-Driven Chronic Pain. Nat. Rev. Drug Discov. 13, 533–548. doi:10.1038/nrd4334
Ji, Z. H., Liu, Z. J., Liu, Z. T., Zhao, W., Williams, B. A., Zhang, H. F., et al. (2017). Diphenyleneiodonium Mitigates Bupivacaine-Induced Sciatic Nerve Damage in a Diabetic Neuropathy Rat Model by Attenuating Oxidative Stress. Anesth. Analg. 125, 653–661. doi:10.1213/ane.0000000000002186
Jia, M., Wu, C., Gao, F., Xiang, H., Sun, N., Peng, P., et al. (2017). Activation of NLRP3 Inflammasome in Peripheral Nerve Contributes to Paclitaxel-Induced Neuropathic Pain. Mol. Pain 13, 1744806917719804. doi:10.1177/1744806917719804
Julien, O., and Wells, J. A. (2017). Caspases and Their Substrates. Cell Death Differ 24, 1380–1389. doi:10.1038/cdd.2017.44
Jurga, A. M., Piotrowska, A., Makuch, W., Przewlocka, B., and Mika, J. (2017). Blockade of P2X4 Receptors Inhibits Neuropathic Pain-Related Behavior by Preventing MMP-9 Activation and, Consequently, Pronociceptive Interleukin Release in a Rat Model. Front. Pharmacol. 8, 48. doi:10.3389/fphar.2017.00048
Khan, A., Khan, A., Khalid, S., Shal, B., Kang, E., Lee, H., et al. (2021). 7β-(3-Ethyl-cis-crotonoyloxy)-1α-(2-methylbutyryloxy)-3,14-dehydro-Z Notonipetranone Attenuates Neuropathic Pain by Suppressing Oxidative Stress, Inflammatory and Pro-apoptotic Protein Expressions. Molecules 26, 181. doi:10.3390/molecules26010181
Kirby, L., Jin, J., Cardona, J. G., Smith, M. D., Martin, K. A., Wang, J., et al. (2019). Oligodendrocyte Precursor Cells Present Antigen and Are Cytotoxic Targets in Inflammatory Demyelination. Nat. Commun. 10, 3887. doi:10.1038/s41467-019-11638-3
Klein, J. (2015). Chronic Pain, Psychopathology, and DSM-5 Somatic Symptom Disorder. Can. J. Psychiatry 60, 528. doi:10.1177/070674371506001109
Latremoliere, A., and Woolf, C. J. (2009). Central Sensitization: a Generator of Pain Hypersensitivity by central Neural Plasticity. J. Pain 10, 895–926. doi:10.1016/j.jpain.2009.06.012
Li, M. Y., Ding, J. Q., Tang, Q., Hao, M. M., Wang, B. H., Wu, J., et al. (2019a). SIRT1 Activation by SRT1720 Attenuates Bone Cancer Pain via Preventing Drp1-Mediated Mitochondrial Fission. Biochim. Biophys. Acta Mol. Basis Dis. 1865, 587–598. doi:10.1016/j.bbadis.2018.12.017
Li, Q., Tian, Y., Wang, Z. F., Liu, S. B., Mi, W. L., Ma, H. J., et al. (2013). Involvement of the Spinal NALP1 Inflammasome in Neuropathic Pain and Aspirin-Triggered-15-Epi-Lipoxin A4 Induced Analgesia. Neuroscience 254, 230–240. doi:10.1016/j.neuroscience.2013.09.028
Li, Y., Zhang, L., Li, J., Wang, C., Chen, Y., Yuan, Y., et al. (2021). A Role for Transmembrane Protein 16C/Slack Impairment in Excitatory Nociceptive Synaptic Plasticity in the Pathogenesis of Remifentanil-Induced Hyperalgesia in Rats. Neurosci. Bull. 37, 669–683. doi:10.1007/s12264-021-00652-5
Li, Y., Zhang, X., Fu, Z., and Zhou, Q. (2019b). MicroRNA-212-3p Attenuates Neuropathic Pain via Targeting Sodium Voltage-Gated Channel Alpha Subunit 3 (NaV 1.3). Curr. Neurovasc. Res. 16, 465–472. doi:10.2174/1567202616666191111104145
Liang, D. Y., Li, X., Li, W. W., Fiorino, D., Qiao, Y., Sahbaie, P., et al. (2010). Caspase-1 Modulates Incisional Sensitization and Inflammation. Anesthesiology 113, 945–956. doi:10.1097/ALN.0b013e3181ee2f17
Liang, T., Zhang, Y., Wu, S., Chen, Q., and Wang, L. (2022). The Role of NLRP3 Inflammasome in Alzheimer's Disease and Potential Therapeutic Targets. Front. Pharmacol. 13, 845185. doi:10.3389/fphar.2022.845185
Liu, P., Song, C., Wang, C., Li, Y., Su, L., Li, J., et al. (2020). Spinal SNAP-25 Regulates Membrane Trafficking of GluA1-Containing AMPA Receptors in Spinal Injury-Induced Neuropathic Pain in Rats. Neurosci. Lett. 715, 134616. doi:10.1016/j.neulet.2019.134616
Luo, C., Kuner, T., and Kuner, R. (2014). Synaptic Plasticity in Pathological Pain. Trends Neurosci. 37, 343–355. doi:10.1016/j.tins.2014.04.002
Luo, X., Chen, O., Wang, Z., Bang, S., Ji, J., Lee, S. H., et al. (2021). IL-23/IL-17A/TRPV1 axis Produces Mechanical Pain via Macrophage-Sensory Neuron Crosstalk in Female Mice. Neuron 109, 2691–e5. e5. doi:10.1016/j.neuron.2021.06.015
Luo, X., Fitzsimmons, B., Mohan, A., Zhang, L., Terrando, N., Kordasiewicz, H., et al. (2018). Intrathecal Administration of Antisense Oligonucleotide against P38α but Not P38β MAP Kinase Isoform Reduces Neuropathic and Postoperative Pain and TLR4-Induced Pain in Male Mice. Brain Behav. Immun. 72, 34–44. doi:10.1016/j.bbi.2017.11.007
Luo, X., Gu, Y., Tao, X., Serhan, C. N., and Ji, R. R. (2019a). Resolvin D5 Inhibits Neuropathic and Inflammatory Pain in Male but Not Female Mice: Distinct Actions of D-Series Resolvins in Chemotherapy-Induced Peripheral Neuropathy. Front. Pharmacol. 10, 745. doi:10.3389/fphar.2019.00745
Luo, X., Huh, Y., Bang, S., He, Q., Zhang, L., Matsuda, M., et al. (2019b). Macrophage Toll-like Receptor 9 Contributes to Chemotherapy-Induced Neuropathic Pain in Male Mice. J. Neurosci. 39, 6848–6864. doi:10.1523/jneurosci.3257-18.2019
Lv, Y., Zhang, L., Li, N., Mai, N., Zhang, Y., and Pan, S. (2017). Geraniol Promotes Functional Recovery and Attenuates Neuropathic Pain in Rats with Spinal Cord Injury. Can. J. Physiol. Pharmacol. 95, 1389–1395. doi:10.1139/cjpp-2016-0528
Ma, Z., Huang, Z., Zhang, L., Li, X., Xu, B., Xiao, Y., et al. (2020). Vanillic Acid Reduces Pain-Related Behavior in Knee Osteoarthritis Rats through the Inhibition of NLRP3 Inflammasome-Related Synovitis. Front. Pharmacol. 11, 599022. doi:10.3389/fphar.2020.599022
Matsuoka, Y., Yamashita, A., Matsuda, M., Kawai, K., Sawa, T., and Amaya, F. (2019). NLRP2 Inflammasome in Dorsal Root Ganglion as a Novel Molecular Platform that Produces Inflammatory Pain Hypersensitivity. Pain 160, 2149–2160. doi:10.1097/j.pain.0000000000001611
McIlwain, D. R., Berger, T., and Mak, T. W. (2015). Caspase Functions in Cell Death and Disease. Cold Spring Harb. Perspect. Biol. 7. doi:10.1101/cshperspect.a026716
Mills, S. E. E., Nicolson, K. P., and Smith, B. H. (2019). Chronic Pain: a Review of its Epidemiology and Associated Factors in Population-Based Studies. Br. J. Anaesth. 123, e273–e283. doi:10.1016/j.bja.2019.03.023
Mo, C., Xu, M., Wen, C., Chang, R., Huang, C., Zou, W., et al. (2018). Normalizing JMJD6 Expression in Rat Spinal Dorsal Horn Alleviates Hyperalgesia Following Chronic Constriction Injury. Front. Neurosci. 12, 542. doi:10.3389/fnins.2018.00542
Moriarty, O., McGuire, B. E., and Finn, D. P. (2011). The Effect of Pain on Cognitive Function: a Review of Clinical and Preclinical Research. Prog. Neurobiol. 93, 385–404. doi:10.1016/j.pneurobio.2011.01.002
Ni, S., Ling, Z., Wang, X., Cao, Y., Wu, T., Deng, R., et al. (2019). Sensory Innervation in Porous Endplates by Netrin-1 from Osteoclasts Mediates PGE2-Induced Spinal Hypersensitivity in Mice. Nat. Commun. 10, 5643. doi:10.1038/s41467-019-13476-9
Pan, Z., Shan, Q., Gu, P., Wang, X. M., Tai, L. W., Sun, M., et al. (2018). miRNA-23a/CXCR4 Regulates Neuropathic Pain via Directly Targeting TXNIP/NLRP3 Inflammasome axis. J. Neuroinflammation. 15, 29. doi:10.1186/s12974-018-1073-0
Pan, Z., Zhang, Q., Liu, X., Zhou, H., Jin, T., Hao, L. Y., et al. (2021). Methyltransferase-like 3 Contributes to Inflammatory Pain by Targeting TET1 in YTHDF2-dependent Manner. Pain 162, 1960–1976. doi:10.1097/j.pain.0000000000002218
Peng, Y., Zang, T., Zhou, L., Ni, K., and Zhou, X. (2019). COX-2 Contributed to the Remifentanil-Induced Hyperalgesia Related to ephrinB/EphB Signaling. Neurol. Res. 41, 519–527. doi:10.1080/01616412.2019.1580459
Qian, J., Zhu, W., Lu, M., Ni, B., and Yang, J. (2017). D-β-hydroxybutyrate Promotes Functional Recovery and Relieves Pain Hypersensitivity in Mice with Spinal Cord Injury. Br. J. Pharmacol. 174, 1961–1971. doi:10.1111/bph.13788
Qiang, Z., and Yu, W. (2019). Chemokine CCL7 Regulates Spinal Phosphorylation of GluA1-Containing AMPA Receptor via Interleukin-18 in Remifentanil-Induced Hyperalgesia in Rats. Neurosci. Lett. 711, 134440. doi:10.1016/j.neulet.2019.134440
Rasoulian, B., Hajializadeh, Z., Esmaeili-Mahani, S., Rashidipour, M., Fatemi, I., and Kaeidi, A. (2019). Neuroprotective and Antinociceptive Effects of Rosemary (Rosmarinus Officinalis L.) Extract in Rats with Painful Diabetic Neuropathy. J. Physiol. Sci. 69, 57–64. doi:10.1007/s12576-018-0620-x
Rocha, F. R. G., Delitto, A. E., de Souza, J. A. C., González-Maldonado, L. A., Wallet, S. M., and Rossa Junior, C. (2020). Relevance of Caspase-1 and Nlrp3 Inflammasome on Inflammatory Bone Resorption in A Murine Model of Periodontitis. Sci. Rep. 10, 7823. doi:10.1038/s41598-020-64685-y
Schroeder, A., Vanderlinden, J., Vints, K., Ribeiro, L. F., Vennekens, K. M., Gounko, N. V., et al. (2018). A Modular Organization of LRR Protein-Mediated Synaptic Adhesion Defines Synapse Identity. Neuron 99, 329–e7. e7. doi:10.1016/j.neuron.2018.06.026
Shim, H. S., Bae, C., Wang, J., Lee, K. H., Hankerd, K. M., Kim, H. K., et al. (2019). Peripheral and central Oxidative Stress in Chemotherapy-Induced Neuropathic Pain. Mol. Pain 15, 1744806919840098. doi:10.1177/1744806919840098
Shu, R. C., Zhang, L. L., Wang, C. Y., Li, N., Wang, H. Y., Xie, K. L., et al. (2015). Spinal Peroxynitrite Contributes to Remifentanil-Induced Postoperative Hyperalgesia via Enhancement of Divalent Metal Transporter 1 without Iron-Responsive Element-Mediated Iron Accumulation in Rats. Anesthesiology 122, 908–920. doi:10.1097/aln.0000000000000562
Siniscalco, D., Giordano, C., Fuccio, C., Luongo, L., Ferraraccio, F., Rossi, F., et al. (2008). Involvement of Subtype 1 Metabotropic Glutamate Receptors in Apoptosis and Caspase-7 Over-expression in Spinal Cord of Neuropathic Rats. Pharmacol. Res. 57, 223–233. doi:10.1016/j.phrs.2008.01.007
Squillace, S., and Salvemini, D. (2022). Nitroxidative Stress in Pain and Opioid-Induced Adverse Effects: Therapeutic Opportunities. Pain 163, 205–213. doi:10.1097/j.pain.0000000000002347
Staahl, C., Olesen, A. E., Andresen, T., Arendt-Nielsen, L., and Drewes, A. M. (2009). Assessing Efficacy of Non-opioid Analgesics in Experimental Pain Models in Healthy Volunteers: an Updated Review. Br. J. Clin. Pharmacol. 68, 322–341. doi:10.1111/j.1365-2125.2009.03433.x
Tajerian, M., Leu, D., Zou, Y., Sahbaie, P., Li, W., Khan, H., et al. (2014). Brain Neuroplastic Changes Accompany Anxiety and Memory Deficits in a Model of Complex Regional Pain Syndrome. Anesthesiology 121, 852–865. doi:10.1097/aln.0000000000000403
Thompson, T., Whiter, F., Gallop, K., Veronese, N., Solmi, M., Newton, P., et al. (2019). NMDA Receptor Antagonists and Pain Relief: A Meta-Analysis of Experimental Trials. Neurology 92, e1652–e1662. doi:10.1212/wnl.0000000000007238
Tonkin, R. S., Bowles, C., Perera, C. J., Keating, B. A., Makker, P. G. S., Duffy, S. S., et al. (2018). Attenuation of Mechanical Pain Hypersensitivity by Treatment with Peptide5, a Connexin-43 Mimetic Peptide, Involves Inhibition of NLRP3 Inflammasome in Nerve-Injured Mice. Exp. Neurol. 300, 1–12. doi:10.1016/j.expneurol.2017.10.016
Tracey, I., Woolf, C. J., and Andrews, N. A. (2019). Composite Pain Biomarker Signatures for Objective Assessment and Effective Treatment. Neuron 101, 783–800. doi:10.1016/j.neuron.2019.02.019
Treede, R. D., Rief, W., Barke, A., Aziz, Q., Bennett, M. I., Benoliel, R., et al. (2019). Chronic Pain as a Symptom or a Disease: the IASP Classification of Chronic Pain for the International Classification of Diseases (ICD-11). Pain 160, 19–27. doi:10.1097/j.pain.0000000000001384
Turtle, J. D., Strain, M. M., Aceves, M., Huang, Y. J., Reynolds, J. A., Hook, M. A., et al. (2017). Pain Input Impairs Recovery after Spinal Cord Injury: Treatment with Lidocaine. J. Neurotrauma. 34, 1200–1208. doi:10.1089/neu.2016.4778
Van Opdenbosch, N., and Lamkanfi, M. (2019). Caspases in Cell Death, Inflammation, and Disease. Immunity 50, 1352–1364. doi:10.1016/j.immuni.2019.05.020
Venero, J. L., Burguillos, M. A., and Joseph, B. (2013). Caspases Playing in the Field of Neuroinflammation: Old and New Players. Dev. Neurosci. 35, 88–101. doi:10.1159/000346155
Wahlman, C., Doyle, T. M., Little, J. W., Luongo, L., Janes, K., Chen, Z., et al. (2018). Chemotherapy-induced Pain Is Promoted by Enhanced Spinal Adenosine Kinase Levels through Astrocyte-dependent Mechanisms. Pain 159, 1025–1034. doi:10.1097/j.pain.0000000000001177
Walid, M. S., Donahue, S. N., Darmohray, D. M., Hyer, L. A., and Robinson, J. S. (2008). The Fifth Vital Sign-Wwhat Does it Mean? Pain Pract. 8, 417–422. doi:10.1111/j.1533-2500.2008.00222.x
Wang, C., Li, Q., Jia, Z., Zhang, H., Li, Y., Zhao, Q., et al. (2020a). Spinal Caspase-6 Contributes to Remifentanil-Induced Hyperalgesia via Regulating CCL21/CXCR3 Pathway in Rats. Neurosci. Lett. 721, 134802. doi:10.1016/j.neulet.2020.134802
Wang, C., Xu, R., Wang, X., Li, Q., Li, Y., Jiao, Y., et al. (2020b). Spinal CCL1/CCR8 Regulates Phosphorylation of GluA1-Containing AMPA Receptor in Postoperative Pain after Tibial Fracture and Orthopedic Surgery in Mice. Neurosci. Res. 154, 20–26. doi:10.1016/j.neures.2019.05.003
Wang, K., Donnelly, C. R., Jiang, C., Liao, Y., Luo, X., Tao, X., et al. (2021a). STING Suppresses Bone Cancer Pain via Immune and Neuronal Modulation. Nat. Commun. 12, 4558. doi:10.1038/s41467-021-24867-2
Wang, K., Gu, Y., Liao, Y., Bang, S., Donnelly, C. R., Chen, O., et al. (2020c). PD-1 Blockade Inhibits Osteoclast Formation and Murine Bone Cancer Pain. J. Clin. Invest. 130, 3603–3620. doi:10.1172/jci133334
Wang, Y. H., Tang, Y. R., Gao, X., Liu, J., Zhang, N. N., Liang, Z. J., et al. (2021b). The Anti-inflammatory and Analgesic Effects of Intraperitoneal Melatonin after Spinal Nerve Ligation Are Mediated by Inhibition of the NF-Κb/nlrp3 Inflammasome Signaling Pathway. Brain Res. Bull. 169, 156–166. doi:10.1016/j.brainresbull.2021.01.015
Wang, Y. J., Liu, M. G., Wang, J. H., Cao, W., Wu, C., Wang, Z. Y., et al. (2020d). Restoration of Cingulate Long-Term Depression by Enhancing Non-apoptotic Caspase 3 Alleviates Peripheral Pain Hypersensitivity. Cell Rep 33, 108369. doi:10.1016/j.celrep.2020.108369
Wang, Z., Tao, Y., Song, C., Liu, P., Wang, C., Li, Y., et al. (2020e). Spinal Hevin Mediates Membrane Trafficking of GluA1-Containing AMPA Receptors in Remifentanil-Induced Postoperative Hyperalgesia in Mice. Neurosci. Lett. 722, 134855. doi:10.1016/j.neulet.2020.134855
Wu, F., Miao, X., Chen, J., Sun, Y., Liu, Z., Tao, Y., et al. (2012). Down-regulation of GAP-43 by Inhibition of Caspases-3 in a Rat Model of Neuropathic Pain. Int. J. Clin. Exp. Pathol. 5, 948–955.
Xie, X. J., Ma, L. G., Xi, K., Fan, D. M., Li, J. G., Zhang, Q., et al. (2017). Effects of microRNA-223 on Morphine Analgesic Tolerance by Targeting NLRP3 in a Rat Model of Neuropathic Pain. Mol. Pain 13, 1744806917706582. doi:10.1177/1744806917706582
Xu, L., Wang, Q., Jiang, W., Yu, S., and Zhang, S. (2019). MiR-34c Ameliorates Neuropathic Pain by Targeting NLRP3 in a Mouse Model of Chronic Constriction Injury. Neuroscience 399, 125–134. doi:10.1016/j.neuroscience.2018.12.030
Xu, X., Tao, X., Huang, P., Lin, F., Liu, Q., Xu, L., et al. (2020). N-methyl-d-aspartate Receptor Subunit 2B on Keratinocyte Mediates Peripheral and central Sensitization in Chronic post-ischemic Pain in Male Rats. Brain Behav. Immun. 87, 579–590. doi:10.1016/j.bbi.2020.02.003
Yang, L. H., Xu, G. M., and Wang, Y. (2016). Up-regulation of CXCL1 and CXCR2 Contributes to Remifentanil-Induced Hypernociception via Modulating Spinal NMDA Receptor Expression and Phosphorylation in Rats. Neurosci. Lett. 626, 135–141. doi:10.1016/j.neulet.2015.12.044
Yang, M., Chen, W., Zhang, Y., Yang, R., Wang, Y., and Yuan, H. (2018). EphrinB/EphB Signaling Contributes to Spinal Nociceptive Processing via Calpain-1 and C-aspase-3. Mol. Med. Rep. 18, 268–278. doi:10.3892/mmr.2018.8996
Yousuf, M. S., Maguire, A. D., Simmen, T., and Kerr, B. J. (2020). Endoplasmic Reticulum-Mitochondria Interplay in Chronic Pain: The Calcium Connection. Mol. Pain. 16, 1744806920946889. doi:10.1177/1744806920946889
Yu, M. X., Lei, B., Song, X., Huang, Y. M., Ma, X. Q., Hao, C. X., et al. (2021). Compound XiongShao Capsule Ameliorates Streptozotocin-Induced Diabetic Peripheral Neuropathy in Rats via Inhibiting Apoptosis, Oxidative - Nitrosative Stress and Advanced Glycation End Products. J. Ethnopharmacol. 268, 113560. doi:10.1016/j.jep.2020.113560
Zhang, E., Yi, M. H., Shin, N., Baek, H., Kim, S., Kim, E., et al. (2015a). Endoplasmic Reticulum Stress Impairment in the Spinal Dorsal Horn of a Neuropathic Pain Model. Sci. Rep. 5, 11555. doi:10.1038/srep11555
Zhang, L., Guo, S., Zhao, Q., Li, Y., Song, C., Wang, C., et al. (2018). Spinal Protein Kinase Mζ Regulates α-Amino-3-hydroxy-5-methyl-4-isoxazolepropionic Acid Receptor Trafficking and Dendritic Spine Plasticity via Kalirin-7 in the Pathogenesis of Remifentanil-Induced Postincisional Hyperalgesia in Rats. Anesthesiology 129, 173–186. doi:10.1097/aln.0000000000002190
Zhang, L., Li, J., Li, Y., Wang, Z., Wang, G., Yu, Y., et al. (2020). Spinal Caspase-3 Contributes to Tibial Fracture-Associated Postoperative Allodynia via Up-Regulation of LRRTM1 Expression in Mice. Neurosci. Lett. 739, 135429. doi:10.1016/j.neulet.2020.135429
Zhang, L., Shu, R., Wang, H., Yu, Y., Wang, C., Yang, M., et al. (2014). Hydrogen-rich saline Prevents Remifentanil-Induced Hyperalgesia and Inhibits MnSOD Nitration via Regulation of NR2B-Containing NMDA Receptor in Rats. Neuroscience 280, 171–180. doi:10.1016/j.neuroscience.2014.09.024
Zhang, L., Shu, R., Zhao, Q., Li, Y., Wang, C., Wang, H., et al. (2017). Preoperative but Not Postoperative Flurbiprofen Axetil Alleviates Remifentanil-Induced Hyperalgesia after Laparoscopic Gynecological Surgery: A Prospective, Randomized, Double-Blinded, Trial. Clin. J. Pain. 33, 435–442. doi:10.1097/ajp.0000000000000416
Zhang, L., Shu, R., Zhao, Q., Li, Y., Yu, Y., and Wang, G. (2016). Preoperative Butorphanol and Flurbiprofen Axetil Therapy Attenuates Remifentanil-Induced Hyperalgesia after Laparoscopic Gynaecological Surgery: a Randomized Double-Blind Controlled Trial. Br. J. Anaesth. 117, 504–511. doi:10.1093/bja/aew248
Zhang, L., Wang, Z., Song, C., Liu, H., Li, Y., Li, J., et al. (2021). Spinal NR2B Phosphorylation at Tyr1472 Regulates IRE(-)DMT1-mediated Iron Accumulation and Spine Morphogenesis via Kalirin-7 in Tibial Fracture-Associated Postoperative Pain after Orthopedic Surgery in Female Mice. Reg. Anesth. Pain Med. 46, 363–373. doi:10.1136/rapm-2020-101883
Zhang, Y., Laumet, G., Chen, S. R., Hittelman, W. N., and Pan, H. L. (2015b). Pannexin-1 Up-Regulation in the Dorsal Root Ganglion Contributes to Neuropathic Pain Development. J. Biol. Chem. 290, 14647–14655. doi:10.1074/jbc.M115.650218
Zhang, Y. K., Huang, Z. J., Liu, S., Liu, Y. P., Song, A. A., and Song, X. J. (2013). WNT Signaling Underlies the Pathogenesis of Neuropathic Pain in Rodents. J. Clin. Invest. 123, 2268–2286. doi:10.1172/jci65364
Zhu, M., Yuan, S. T., Yu, W. L., Jia, L. L., and Sun, Y. (2017). CXCL13 Regulates the Trafficking of GluN2B-Containing NMDA Receptor via IL-17 in the Development of Remifentanil-Induced Hyperalgesia in Rats. Neurosci. Lett. 648, 26–33. doi:10.1016/j.neulet.2017.03.044
ALCAR Acetyl-l-carnitine
AMPAR alpha-amino-3-hydroxy-5-methyl-4-isoxazolepropionic acid receptor
ACC anterior cingulate cortex
AD Alzheimer’s disease
ASC apoptosis-associated speck-like protein containing a Caspase activation and recruitment domain
ATF3 activating transcription factor-3
BAX B-cell lymphoma 2-associated X apoptosis regulator
BDNF brain-derived neuro-trophic factor
CCI chronic constriction injury
CCL21 C-C Motif Chemokine Ligand 21
CFA complete Freund’s adjuvant
CGRP calcitonin-gene-related peptide
CIBP cancer-induced bone pain
CIPN chemotherapy induced neuropathic pain
COX-2 cyclooxygenase-2
CPN common peroneal nerve
CPTP chronic post-thoracotomy pain
CSF-CN Cerebrospinal fluid-contacting nucleus
CXCL1 Chemokine (C-X-C motif) ligand 1
CXCR3 C-X-C Motif Chemokine Receptor 3
DAMP damage associated molecular patterns
DRG dorsal root ganglion
ER endoplasmic reticulum
ERK extracellular signal-regulated kinase
GAP-43 growth associated protein 43
GFAP Glial fibrillary acidic protein
GSH glutathione
G-CSF granulocyte-colony stimulating factor
IL-1β interleukin-1beta
LPS lipopolysaccharide
LRRTM1 leucine-rich repeat transmembrane protein 1
LTD long-term depression
MDA malondialdehyde
MIP-1α macrophage inflammatory protein-1α
mGluR1 metabotropic glutamate receptor 1
NF-κB Nuclear Factor-κB
NLRP3 NOD-like receptor protein 3
NMDA N-methyl-d-aspartate
NLRC4 NOD-like receptor C4
NP neuropathic pain
3-NT 3-nitrotyrosine
OIH opioid-induced hyperalgesia
PD Parkinson’s disease
p-ERK ERK phosphorylation
PGE2 Prostaglandin E2
p-IRE1 phosphorylated inositol-requiring enzyme-1
p-PERK phosphorylated protein kinase RNA-like endoplasmic reticulum kinase
pSNL partial sciatic nerve ligation
RTKs receptor tyrosine kinases
SCI spinal cord injury model
SNI spared nerve injury
SNL sciatic nerve ligation
STZ streptozotocin
TLR4 toll-like receptor 4
TNF-α Tumor necrosis factor-α
TRPV1 transient receptor potential vanilloid-1.
Keywords: caspase, chronic pain, neuroinflammation, neuronal apoptosis, synaptic plasticity, spinal cord
Citation: Zhang H, Li N, Li Z, Li Y, Yu Y and Zhang L (2022) The Involvement of Caspases in Neuroinflammation and Neuronal Apoptosis in Chronic Pain and Potential Therapeutic Targets. Front. Pharmacol. 13:898574. doi: 10.3389/fphar.2022.898574
Received: 17 March 2022; Accepted: 13 April 2022;
Published: 03 May 2022.
Edited by:
Xin Luo, Guangdong-Hong Kong-Macao Greater Bay Area Center for Brain Science and Brain-Inspired Intelligence, ChinaReviewed by:
Tong Liu, Nantong University, ChinaCopyright © 2022 Zhang, Li, Li, Li, Yu and Zhang. This is an open-access article distributed under the terms of the Creative Commons Attribution License (CC BY). The use, distribution or reproduction in other forums is permitted, provided the original author(s) and the copyright owner(s) are credited and that the original publication in this journal is cited, in accordance with accepted academic practice. No use, distribution or reproduction is permitted which does not comply with these terms.
*Correspondence: Yonghao Yu, eXl1QHRtdS5lZHUuY24=; Linlin Zhang, bGlubGluemhhbmdAdG11LmVkdS5jbg==
†These authors have contributed equally to this work
Disclaimer: All claims expressed in this article are solely those of the authors and do not necessarily represent those of their affiliated organizations, or those of the publisher, the editors and the reviewers. Any product that may be evaluated in this article or claim that may be made by its manufacturer is not guaranteed or endorsed by the publisher.
Research integrity at Frontiers
Learn more about the work of our research integrity team to safeguard the quality of each article we publish.