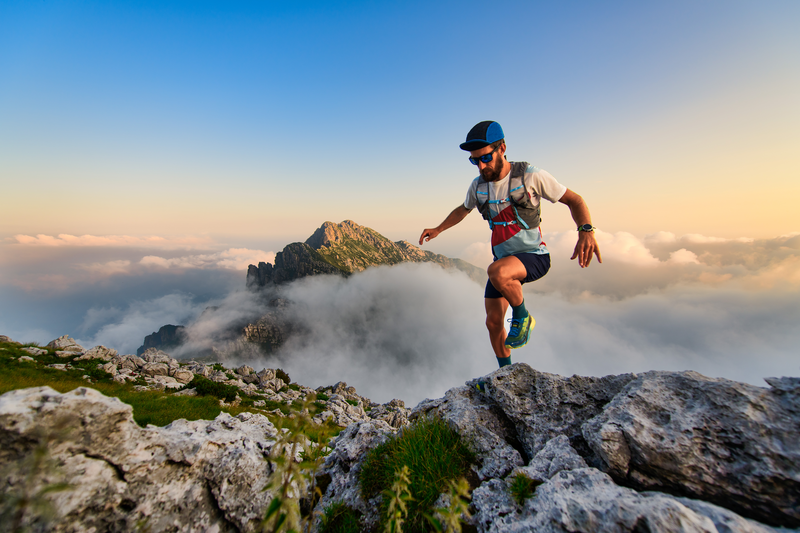
94% of researchers rate our articles as excellent or good
Learn more about the work of our research integrity team to safeguard the quality of each article we publish.
Find out more
ORIGINAL RESEARCH article
Front. Pharmacol. , 15 July 2022
Sec. Neuropharmacology
Volume 13 - 2022 | https://doi.org/10.3389/fphar.2022.898360
This article is part of the Research Topic Gut Microbiota as a Therapeutic Target in Neuropsychiatric Disorders: Current status and future directions View all 13 articles
Background: Cognitive dysfunction is a critical complication of diabetes mellitus, and there are still no clinically approved drugs. Zi Shen Wan Fang (ZSWF) is an optimized prescription composed of Anemarrhenae Rhizoma, Phellodendri Chinensis Cortex, and Cistanches Herba. The purpose of this study is to investigate the effect of ZSWF on DCI and explore its mechanism from the perspective of maintaining intestinal microbial homeostasis in order to find an effective prescription for treating DCI.
Methods: The diabetes model was established by a high-fat diet combined with intraperitoneal injections of streptozotocin (STZ, 120 mg/kg) and the DCI model was screened by Morris water maze (MWM) after 8 weeks of continuous hyperglycemic stimulation. The DCI mice were randomly divided into the model group (DCI), the low- and high-ZSWF–dose groups (9.63 g/kg, 18.72 g/kg), the mixed antibiotic group (ABs), and the ZSWF combined with mixed antibiotic group (ZSWF + ABs). ZSWF was administered orally once a day for 8 weeks. Then, cognitive function was assessed using MWM, neuroinflammation and systemic inflammation were analyzed by enzyme-linked immunosorbent assay kits, intestinal barrier integrity was assessed by hematoxylin-eosin (HE) staining and Western blot and high performance liquid chromatography tandem mass spectrometry (UPLC-MS/MS). Furthermore, the alteration to intestinal flora was monitored by 16S rDNA sequencing.
Results: ZSWF restored cognitive function in DCI mice and reduced levels of proinflammatory cytokines such as IL-1β, IL-6, and TNF-α. Moreover, ZSWF protected the integrity of the intestinal barrier by increasing intestinal ZO-1 and occludin protein expression and decreasing urinary lactulose to mannitol ratio. In addition, ZSWF reshaped the imbalanced gut microbiota in DCI mice by reversing the abundance changes of a wide range of intestinal bacteria at the phyla and genus levels. In contrast, removing gut microbiota with antibiotics partially eliminated the effects of ZSWF on improving cognitive function and reducing inflammation, confirming the essential role of gut microbiota in the improvement of DCI by ZSWF.
Conclusion: ZSWF can reverse cognitive impairment in DCI mice by remolding the structure of destructed gut microbiota community, which is a potential Chinese medicine prescription for DCI treatment.
Diabetes mellitus (DM) is a common metabolic disease with increasing incidence. Cumulative studies confirm that DM is a key risk factor for cognitive impairment (Zilliox et al., 2016; Biessels and Despa, 2018; Biessels and Whitmer, 2020). Although many excellent studies of DCI exist, its potential mechanisms remain elusive and effective drugs are lacking. Several studies suggested that DCI might be attributed to cerebrovascular dysfunction (Prakash et al., 2012), neuroinflammation (Rom et al., 2019), and metabolic disorders (Zhang et al., 2020a). In recent years, emerging studies noticed that intestinal microbiota structure and function disorders are closely related to brain function. Gut-brain crosstalk is a complex network system, which maintains the stability of gastrointestinal tract on the one hand and affects the brain homeostasis on the other hand (Rhee et al., 2009). Intestinal microbes regulate brain homeostasis through multiple pathways, among which intestinal barrier-inflammation is one of the main pathways (Rogers et al., 2016). Next, the intestinal barrier plays a crucial role in maintaining of intestinal microbiome and peripheral homeostasis. In contrast, intestinal microbiome dysbiosis can provoke disruption of the intestinal barrier. Increased intestinal permeability can cause excessive translocation of bacterial lipopolysaccharide into the bloodstream, which can trigger systemic inflammation (Zhang et al., 2019). Microbiota-derived inflammatory response ultimately leads to neuroinflammation and neuronal damage (Bairamian et al., 2022).
Increasing evidence indicates that the structural changes of intestinal microbiota are associated with the development of DCI (Xu et al., 2017). Clinical studies found significant differences in gut microbiome composition between diabetic patients with and without cognitive impairment (Zhang et al., 2021a). Also, preclinical studies suggested that DCI was associated with alterations of the gut microbiome (Gao et al., 2019). Furthermore, Yu et al. (2019) transplanted fecal bacteria from DCI and non-DCI mice into the gut of pseudo-germ-free mice, respectively, and they found that the escape latency was significantly longer in pseudo-germ-free mice receiving DCI mouse fecal bacteria than those receiving non-DCI mouse fecal bacteria. These studies suggest that maintaining intestinal microbial homeostasis is an effective strategy for the prevention and treatment of DCI.
Traditional Chinese medicine (TCM) believes that “poison damage brain collateral” and “deficiency of kidney essence” are the main pathogenesis of DCI, and “clearing heat-fire and detoxifying” and “invigorating kidney for protecting semen” are the key therapeutic principles. Anemarrhenae Rhizoma and Phellodendri Chinensis Cortex are the most commonly used heat-clearing drug pair in clinical practice, and they were originally derived from “Tong Guan Wan.” Previous modern pharmacological evidence suggests that Anemarrhenae Rhizoma and Phellodendri Chinensis Cortex drug pair exhibit an effect on improving diabetes-related complications (Zhang et al., 2014), and their active ingredients demonstrate a clear protective effect on cognitive function (Liu et al., 2012; Xian et al., 2013; Liang et al., 2017; Piwowar et al., 2020). However, studies also found that the bioavailability of saponins and alkaloids (the main components of Anemarrhenae Rhizoma and Phellodendri Chinensis Cortex, respectively) is low (Singh and Chaudhuri, 2018; Baldim et al., 2020), suggesting that regulation of intestinal microbial homeostasis may be the key pathway for them to improve diabetes-related complications. Furthermore, considering that the treatment of diabetes-related complications is a long process and that Anemarrhenae Rhizoma and Phellodendri Chinensis Cortex are severe cold medicines, the compatibility of warm drugs to neutralize cold is the basic principle of the compatibility of TCM prescriptions. Cistanches Herba (Cistanche deserticola Y. C. Ma), a classic Chinese medicine with the effect of tonifying kidney and nourishing essence, is warm in property. Also, modern pharmacological studies suggest that Cistanches Herba demonstrates the function of maintaining intestinal flora homeostasis and neuroprotection (Li et al., 2017; Wang et al., 2017). Therefore, under the guidance of basic theories of TCM, we formed ZSWF by the compatibility of the Cistanches Herba (C. deserticola Y. C. Ma) and Anemarrhenae Rhizoma (Anemarrhena asphodeloides Bge)-Phellodendri Chinensis Cortex (Phellodendron chinense Schneid) herb pair, hoping to find a new prescription to treat DCI.
In this study, we investigated the effects of ZSWF on the cognitive function of DCI mice, and we explored the possible mechanism by regulating intestinal flora. Cognitive function, neuroinflammation and systemic inflammation, intestinal barrier integrity, and gut microbiome diversity were examined. Furthermore, an antibiotic intervention was used to assess the possibility of a causal relation between ZSWF improving cognitive and gut microbiota.
ZSWF is composed of Anemarrhenae Rhizoma (dried rhizome of A. asphodeloides Bge.), Phellodendri Chinensis Cortex (dried bark of P. chinense Schneid.), and Cistanches Herba (dried fleshy stem of C. deserticola Y. C.Ma) with a ratio of 1:1:1 in weight. The drug materials of Anemarrhenae Rhizoma and Phellodendri Chinensis Cortex were purchased from Hebei anguo Chinese Herbal Medicine Co., Ltd., and Cistanches Herba was purchased from Inner Mongolia Mandera Biological Technology Co., Ltd. These herbs were identified by professor Tianxiang Li (TianJin University of Traditional Chinese Medicine) and were extracted according to our previous method (Zheng et al., 2018). In simple terms, Anemarrhenae Rhizoma and Phellodendri Chinensis Cortex were mixed at the ratio of 1:1 by weight, then extracted by reflux for 3 times with 80% ethanol of 8 times (2 h each time), and filtrate was collected. The same weight Cistanches Herba was first extracted by reflux for 3 times with 8 times the amount of 80% ethanol (2 h each time), and filtrate was collected and then extracted with 10 times the amount of distilled water for 3 times (2 h each time). Next, the volume of liquid required for ingastric administration of mice during the treatment cycle was calculated, and all filtrate was mixed and concentrated to 0.94 g/ml ZSWF crude extract by rotary evaporator, which was separated and stored in a refrigerator at −80°C for use. Moreover, our previous study systematically investigated the main chemical components of ZSWF extract (Zheng et al., 2018), including in vitro components (e.g., Neomangiferin, Berberine, TimosaponinBⅡ, and Cistansinenside A), absorbed components (e.g., Mangiferin, Timosaponin C, and 3/5-O-Feruloylquinic acid), as well as prototypical (e.g., Phellodenrine, Tetrahydropalmatine, and Oxoberberine) and metabolic components [e.g., 3,4-dihydroxybenzenepropionic acid and (20R, 25S)-timosaponin AI] in feces.
Two hundred male C57BL/6J mice (8 weeks-old) were purchased from Beijing Vital River Laboratory Animal Technology Co., Ltd., [SCXK (Jing) 2016-006]. The animals were housed in the Laboratory Animal Center of Tianjin University of Traditional Chinese Medicine under a standard laboratory condition (temperature 22 ± 2°C, humidity at 50 ± 15%, 12-h-light/12-h-dark cycle) and were given ad libitum access to water and food. All experimental procedures were approved by the Animal Ethics Committee of Tianjin University of Traditional Chinese Medicine (TCM-LAEC2019083, Tianjin, China).
After 1 week of adaptive feeding, the diabetic mouse model was replicated as previously reported (Kusakabe et al., 2009). In simple terms, mice were intraperitoneally injected with 120 mg/kg streptozotocin (STZ, Sigma, United States) after being fed a high-fat diet with 60% energy from fat for 3 weeks (Beijing Vital River Laboratory Animal Technology Co., Ltd.). One week after injection, fasting blood glucose (FBG) of mice after 12 h of fasting was detected, and mice with FBG > 11.1 mmol/L were selected to continue high-fat diet feeding. After 8 weeks of continuous hyperglycemic stimulation, mice with cognitive impairment were screened with MWM (detailed in 2.3) and used in follow-up studies.
To investigate the effect of ZSWF on the cognitive function of DCI mice and its potential mechanism, the mice with cognitive impairment were randomly divided into five groups: vehicle treated group (DCI), ZSWF low dose (9.36 g/kg, clinical equivalent dose) treated group (ZSWFL), ZSWF high dose (18.72 g/kg, 2 times the clinical equivalent dose) treated group (ZSWFH), mixed antibiotic (natamycin 3 mg/ml, neomycin 2 mg/ml and bacitracin 2 mg/ml) treated group (ABs), and ZSWF combined with mixed antibiotic treated group (ZSWF + ABs). Mice in the treatment group were orally gavaged with crude extract of ZSWF for 8 weeks, and mice in Con group and DCI group were gavaged with equal volume of distilled water. The control group was fed the normal diet, and the other groups were kept on HFD during drug administration. Weight was measured every 2 weeks, and FBG was measured weekly during continuous treatment.
To screen mice with abnormal cognitive function after 8 weeks of hyperglycemia and investigate the effect of ZSWF treatment on cognitive function, the MWM experiment was performed as previously described (Morris., 1984). In short, to acclimate to the maze environment, all the mice were allowed to swim freely in the maze without a platform for 1 min the day before the experiment began. Next, the positioning navigation experiment was conducted for five consecutive days, during which the mice were allowed to swim freely for 60 s. If they did not reach the platform within 60 s, the mice were slowly guided to the platform and held there for 10 s. In the space exploration experiment, the mice were allowed to swim freely for 60 s in a maze where the platform was removed. During this process, the time to first reach the original platform, the duration in the platform quadrant, and the frequency of crossing the platform were recorded. During the whole test, data acquisition is completed by the automatic image surveillance and processing system.
To investigate the effect of ZSWF on hippocampal neurons of DCI mice, cresyl violet staining was performed. At the end of the treatment, the mice were deeply anesthetized by inhalation with 4% isoflurane, and blood samples were collected. Apical perfusion with PBS was then performed to remove residual blood from the brain tissue, and whole brain tissue was collected. After fixation with 4% paraformaldehyde, the brain tissue was dehydrated with gradient sucrose solution (10%, 20%, and 30%, respectively), and the coronal sections with thickness of 10 μm were obtained using a frozen slicer. Sections were stained with 0.1% cresol violet solution, dehydrated with ascending grades of alcohol, cleared with xylene, mounted with neutral resin, and images were taken using Leica DM4000B biological microscope (Beijing, China).
To investigate the effects of ZSWF on neuroinflammation in DCI mice, the mice were sacrificed by cervical dislocation after deep anesthesia. Then, the skull was cut along the midline of the skull to expose the whole brain tissue, the cortex was separated to expose the hippocampus, and the hippocampus was collected with bamboo sticks and stored at −80°C for detection. Also, commercially available enzyme-linked immunosorbent assay (ELISA) kits were used to measure the hippocampus IL-6 (JYM0012Mo, Colorful-Gene, 2–150 pg/ml), IL-1β (JYM0531Mo, Colorful-Gene, 1.5–100 pg/ml), TNF-α (JYM0218Mo, Colorful-Gene, 8–500 pg/ml), and monocyte chemotaxis protein-1 (MCP-1, JYM0099Mo, Colorful-Gene, 6–450 pg/m) according to manufacturer’s instructions. To investigate the effects of ZSWF on proinflammatory cytokines in peripheral circulation in DCI mice, blood samples from each group were collected and centrifuged at 3,000 g for 15 min. The levels of IL-6, IL-1β, TNF-α, and INF-γ (abs552811, Absin Bioscience Inc., 7.81–500 pg/ml) in the serum were also detected using ELISA kits. Furthermore, to investigate the effect of ZSWF on the integrity of intestinal mucosal barrier in DCI mice, colon tissue approximately 5 cm connected to the cecum of mice in each group was taken, colon contents were scraped, and then, the colon and colon contents were stored in EP tubes, respectively. Next, colon tissue was lysed with lysate, and the content of mucin-2 (MUC2) in colon was determined by the ELISA kit (SEA705Mu, Cloud Clone Biotechnology Co., LTD., 0.78–50 ng/ml). The colon contents were diluted with 0.9% Nacl, homogenized and centrifuged (3,000 g, 15 min), and the content of secretory immunoglobulin A (SlgA) in the colon contents was determined by the ELISA kit (SEA641Mu, Cloud Clone Biotechnology Co., LTD., 0.156–10 ng/ml). All experimental procedures were performed according to the manufacturer’s instructions. The optical density at 450 nm was obtained with an ELISA microplate reader (Infinite® 200 PRO, Tecan, Swit). There were six biological repetitions in each group for proinflammatory cytokines and five biological repetitions in each group for Muc2 and SlgA.
To evaluate the effect of ZSWF on intestinal barrier integrity in DCI mice, the colon was fixed in 4% paraformaldehyde for 24 h, and then, the paraffin section was performed. Sections with a thickness of 0.5 μm were stained with hematoxylin (5 min) and 0.5% eosin (3 min), and the histopathological images of the colon were taken with a Leica DM4000B biologic microscope.
To investigate the effect of ZSWF on intestinal permeability of DCI mice, Western blot analysis was used to detect the protein expressions of occludin and ZO-1. Proteins from colon tissues were extracted and quantified using the BCA protein quantification kit. Total proteins were separated by SDS polyacrylamide gel electrophoresis (SDS-PAGE) and transferred onto a polyvinylidene fluoride (PVDF) membrane by wet transfer apparatus. Tight junction protein associated antibodies ZO-1 (ab216880, Abcam, United Kingdom) and occludin (ab216327, Abcam, United Kingdom) were used to incubate the bands overnight at 4°C, where β-actin (13E5, CST, United States) was used as a reference protein, and then, goat anti-rabbit secondary antibodies (ab205718, Abcam, United Kingdom) were used to incubate the bands at room temperature for 2 h. In conclusion, the immunoreactive bands were visualized using enhanced chemiluminescence reagents, and the luminescence intensity was quantitatively analyzed using Image-pro Plus6.0.
To observe the effect of ZSWF on intestinal barrier integrity in DCI mice, the ratio of lactulose to mannitol in urine was determined by HPLC-MS/MS according to the previous method (Kubica et al., 2012). At the end of treatment, mice were given a 2:1 lactulose-mannitol solution, and urine was collected in a metabolic cage for 6 h. The supernatant was obtained by centrifugation and stored at −80°C. A Waters ACQUITY UPLC BEH Amide (2.1 mm × 50 mm, 1.7 μm, VK) was used for separation. The mobile phase consisted of Methanol (A) and acetonitrile-water (B), and the flow rate was set at 0.25 ml/min. The gradient profile was: 0–1 min (A: 90%; B: 10%), 1–8 min (A: 60%; B: 40%), 8–15 min (A: 90%; B: 10%). The sample injection volume was 2 μL. The ESI source operates in negative mode, with a capillary voltage of 2.0 kV and a desolvation temperature of 550°C. The source of the gas was set as follows: desolvation at 200 L/h and cone at 0 L/h. The collision cell pressure was 4.5 × 10−3 mbar. Dates were processed using MassLynx™4.1 software (Waters Corp, Milford, MA, United States). Lactulose and mannitol contents were calculated as well as the standard curve and corresponding peak area.
To investigate the effect of ZSWF on intestinal microflora of DCI mice, feces of each group were collected by individual metabolic chamber for approximately 24 h after treatment, and 16S rDNA sequencing was performed. The metagenomic DNA from each feces sample was extracted using a QIAamp DNA Stool Mini Kit (Qiagen, Hamburg, Germany). Thereafter, the DNA concentration was determined, and the V3-V4 regions of bacterial 16S rDNA was amplified by PCR (95°C for 3 min, followed by 27 cycles at 95°C for 30 s, 55°C for 30 s, and 72°C for 45 s and a final extension at 72°C for 10 min, 10°C until halted by user) using 10 ng DNA as a template. The 16S primers 338F-ACTCCTACGGGAGGCAGCAG and 806R-GGACTACHVGGGTWTCTAAT were used as fusion primers containing Ion Torrent sequencing adapters. PCR reactions were performed in triplicate 20 μl reaction mixtures containing 4 μl of 5 × FastPfu Buffer (1 μM), 2 μl of 2.5 mM dNTPs, 0.8 μl of Forward Primer (5 μM), and 0.8 μl of Reverse Primer (5 μM), 0.4 μl of FastPfu Polymerase, 2 μl of Microbial DNA (5 ng/μl), and add ddH2O to 20 μl. Afterwards PCR products were gel-purified, and the amplicon DNA concentration was determined. Sequencing of pooled amplicons was performed with an Illumina MiSeq platform (Illumina Inc., San Diego, United States), and the resulting analysis using the MiSeq Reporter software (MSR) and the classification is based on the Greengenes database. Subsequent cord diversity analysis applied to determine α (within sample) and β (between samples) diversity. To demonstrate the clustering of different groups, the Nonmetric multidimensional scaling and weighted unifrac principal coordinate analysis (PCoA) were conducted.
Data were processed and analyzed using the statistical package SPSS (version 17.0), and the results were expressed as mean ± standard deviation (SD). Data were tested for normality before difference analysis, escape latency data were analyzed by repeated measures ANOVA, the remaining data were analyzed by one-way ANOVA, followed by a post hoc Tukey’s Honest Significant Difference test for multiple comparisons among the groups. A p value of less than 0.05 was considered to indicate statistical significance. For 16S rRNA gene sequence analysis, all reads were deposited and grouped into operational taxonomic units (OTU) at a sequence identity of 97%, and the taxonomic affiliation of the OTUs was determined with quantitative insights into microbial ecology (version 1.8.0) against the Greengenes database (version 13.5). Principal component analysis was performed using SIMCA 14.0, and metastats was used to analyze differences between groups.
To investigate the effect of ZSWF on the body weight of DCI mice, we analyzed the changes of body weight of each group of mice every 2 weeks during the treatment of ZSWF, and we found that ZSWF tended to increase the body weight of DCI mice, but no statistical difference (p > 0.05) was found, indicating that ZSWF demonstrated no effect on the body weight of DCI mice. Considering that hyperglycemia is the primary cause of cognitive dysfunction, we first investigated the effect of ZSWF on FBG in DCI mice. The results showed that no significant difference in FBG was found between the ZSWF group and the DCI group during continuous treatment (p > 0.05), whether the ZSWFL or ZSWFH group (Supplementary Figure S1). Next, to investigate the effect of ZSWF on cognitive impairment in DCI mice, the MWM experiment was performed. The results of the positioning navigation experiment showed that the escape latency of DCI group was significantly increased compared with the Con group (p < 0.05), while the escape latency of mice in the ZSWF group was significantly decreased compared with the DCI group (p < 0.05) (Figure 1A). Next, the results of the space exploration experiment showed that ZSWF significantly increased the frequency of crossing the platform (p < 0.01, F = 6.590) (Figure 1B) and the duration of swimming in the platform quadrant (Figure 1C), and it reduced the time for DCI mice to reach the platform for the first time (p < 0.05, F = 6.434) (Figure 1D). Moreover, the swimming trajectory of the space exploration experiment showed that the swimming trajectory of the ZSWF group is oriented, while that of the DCI group is disordered (Figure 1E).
FIGURE 1. ZSWF ameliorated cognitive impairment and prevented neuron damage in DCI mice. (A) escape latency, (B) the frequency of crossing the platform, (C) duration in the platform quadrant, (D) time of first arrival at platform. These data are expressed as the mean ± SD, (n = 15). Escape latency data were analyzed by repeated measurement ANOVA, other data were analyzed by one-way ANOVA. **p < 0.01, *p < 0.05 vs. Con group, ##p < 0.01, #p < 0.05 vs. DCI group. (E) representative swimming tracks of each group of mice in the space exploration experiment. (F) representative images of cresol violet staining in hippocampus of each group of mice.
The hippocampus is the main brain region regulating learning and memory, and the damage of hippocampus neurons is the final pathological change of cognitive dysfunction. Therefore, we investigated the protective effect of ZSWF on DCI hippocampal neurons by cresol violet staining the neuronal Nissl bodies. As described in Figure 1F, the DCI animals neuron layer is thin, irregularly and loosely arranged, intercellular spaces are widened, and the cellular structure is incomplete, even with the loss of large amounts of cells. Meanwhile, this phenomenon can be reversed by the ZSWF administration, whether ZSWFL or ZSWFH.
Cumulative studies reported that neuroinflammation is an important pathological mechanism of DCI (Rom et al., 2019), and chronic systemic inflammation is one of the important characteristics of DM (Esser et al., 2014). Thus, we measured the levels of classic proinflammatory cytokines IL-1β, IL-6, TNFα, MCP-1, and INF-γ in the hippocampus and serum. Our results indicated that the hippocampus levels of IL-1β (F = 8.909, p < 0.01) (Figure 2A), IL-6 (F = 20.64, p < 0.01) (Figure 2B), TNF-α (F = 6.935, p > 0.05) (Figure 2C), and MCP-1 (F = 7.033, p < 0.05) (Figure 2D) in DCI mice were significantly increased, and the levels of IL-1β (F = 10.12, p < 0.01) (Figure 2E), IL-6 (F = 4.719, p < 0.05) (Figure 2F), TNF-α (F = 6.767, p < 0.01) (Figure 2G), and INF-γ (F = 4.727, p < 0.05) (Figure 2H) in serum were also significantly increased. In contrast, these proinflammatory cytokines were significantly reduced in the ZSWF group compared with the DCI group (p < 0.05), although no significant dose dependence occurred.
FIGURE 2. ZSWF suppressed hippocampal and peripheral inflammation in DCI mice. (A) IL-1β contents in the hippocampus, (B) IL-6 contents in the hippocampus, (C) TNF-α contents in the hippocampus, (D) MCP-1 contents in the hippocampus, (E) IL-1β levels in the serum, (F) IL-6 levels in the serum, (G) TNF-α levels in the serum, (H) INF-γ levels in the serum. All data were expressed as mean ± SD (n = 6). One-way ANOVA was used to compare statistical differences between groups. **p < 0.01, *p < 0.05 vs. Con group, ##p < 0.01, #p < 0.05 vs. DCI group.
Given that intestinal dysbiosis in diabetes animals may affect gut integrity and subsequently lead to release of bacterial proinflammatory cytokines into the circulation, we examined the effect of ZSWF on intestinal barrier integrity in DCI mice. HE staining results showed that compared with the Con group, the colonic mucosa of mice in the DCI group was damaged, the number of goblet cells was reduced, and mucosal muscle layer was thinned, while ZSWF protected the colonic injury of DCI mice (Figure 3A). Furthermore, intestinal SIgA plays a key role in the intestinal immune system. Our data showed that compared with the Con group, the SIgA level of colon contents was significantly reduced in the DCI group (F = 8.092, p < 0.01), while ZSWF treatment significantly reversed the decreased SIgA level in DCI mice (p < 0.05) (Figure 3B). Next, we examined the level of Muc2 secreted by goblet cells and found that ZSWF treatment significantly increased the content of Muc2 in the DCI mice colon (F = 33.39, p < 0.01) (Figure 3C). Moreover, the urinary lactulose/mannitol (L/M) ratio was used to determine intestinal permeability and found that the urinary L/M ratio of the ZSWF group was significantly lower than that of the DCI group (F = 12.06, p < 0.01) (Figure 3D). In addition, we also investigated the effect of ZSWF on tight junction protein expression in the colon of DCI mice and found that compared with the Con group, the expression of tight junction proteins ZO-1 and occludin in the colon of DCI mice was significantly decreased, while ZSWF treatment significantly increased the expression of ZO-1(F = 326.8, p < 0.01) and occludin (F = 181.2, p < 0.01) in the colon of DCI mice (Figures 3E,F).
FIGURE 3. ZSWF maintains intestinal integrity in DCI mice. (A) representative images of colon HE staining, the goblet cells marked by the red arrow, scale bar 50 μm (n = 3). (B,C) SlgA and Muc2 contents in the colon, (D) lactulose to mannitol ratio, these data were expressed as mean ± SD (n = 5). (E,F) occludin and ZO-1 represented Western blotting bands and relative protein expression levels in the colon, data were expressed as mean ± SD (n = 3). All data were analyzed using one-way ANOVA, **p < 0.01 vs. Con group, ##p < 0.01, #p < 0.05 vs. DCI group.
Gut microbiota may be an ideal target for understanding and treating DCI. To reveal the effect of ZSWF in regulating gut microbiota in DCI mice, the feces were sampled and sequenced by performing a pyrosequencing-based analysis of bacterial 16S rDNA (V3-V4 region). Weighted UniFrac distance-based PCoA revealed a distinct clustering of microbiota composition for each group (Figure 4A). At the phylum level, the DCI mice displayed an increased the relative abundance of Firmicutes (54.27% vs. 27.53%) and Proteoobacteria (30.08% vs. 3.24%) and decreased abundance of Bacteroidetes (15.21% vs. 64.25%) compared with Con mice. In contrast, the microbiota imbalance was ameliorated by ZSWF administration as it decreased the abundance of Firmicutes (32.95% vs. 54.27%) and Proteoobacteria (14.71% vs. 30.18%) and increased Bacteroidetes (50.34% vs. 15.2%) (Figure 4B). Furthermore, treatment with ZSWF reduced the ratio of Firmicutes to Bacteroidetes in DCI mice (Figure 4C). At the genus level, the relative abundance accounted for the top 30 was analyzed, and after ZSWF treatment, several important modifications of the gut microbiota composition were found. The relative abundance of Bacteroides (F = 14.48, p < 0.01) and Alistipes (F = 4.559, p < 0.05) were markedly increased, and Dorea (F = 5.396, p < 0.01), Intestinimonas (F = 11.79, p > 0.05), Desuifouibrio (F = 12.45, p < 0.05), and Allobaculum (F = 10.32, p < 0.05) were significantly decreased in the ZSWF administration group compared to the DCI group (Figures 4D–J). These results confirmed that the homeostasis of the gut microbiota in DCI individuals was destroyed, and ZSWF could play an important role in modulating the composition of gut microbiota on DCI mice. Furthermore, considering that short-chain fatty acids (SCFAs) are major metabolites of intestinal microbiota and play an important role in gut-brain axis communication, we examined the effect of ZSWF on fecal SCFAs concentration and found that ZSWF could increase the concentration of SCFAs in feces of DCI mice (Supplementary Figure S3).
FIGURE 4. ZSWF reversed gut microbiota dysbiosis in DCI mice. (A) principal coordinates analysis (PcoA), (B) relative abundances at phylum levels, (C) ratio of Firmicutes to Bacteroidetes, (D) relative abundance of bacteria at the genus level, (E–J) relative abundance of Dorea, Bacteroides, Intestinimonas, Desulfourbrio, Allobaculum, and Alistipes. All data were expressed as mean ± SD (n = 6), and one-way ANOVA was used to compare statistical differences between groups, **p < 0.01, *p < 0.05 vs. Con group, ##p < 0.01, #p < 0.05 vs. DCI group.
The above results suggest that the gut microbiota-brain axis plays an important role in ZSWF in improving cognition impairment of DCI mice. To further investigate the relationship between the improvement of cognitive function and the regulation of intestinal microbiota by ZSWF, a cocktail of oral antibiotics was used to eliminate the gut microbiota. The mice were administrated with antibiotics in the drinking water starting 7 days before ZSWF treatment and throughout the experiment. First, we found that antibiotics demonstrated no effect on FBG in DCI mice (Supplementary Figure S4). Furthermore, antibiotic treatment significantly reduced the amount of OTU in the feces of DCI mice (Figure 5A), suggesting that most intestinal microbiota were eliminated. Antibiotics alone did not significantly alter cognitive function and inflammation in the DCI group, suggesting antibiotics did not further impair cognitive function and increase inflammation induced diabetes (Figures 5B–E). Of note, the antibiotic treatment abrogated the beneficial effects of ZSWF treatment in DCI mice. We found that the cognition improvement with ZSWF was abolished by antibiotics treatment, with longer escape times (Figure 5B), longer times of first to arrival platform (Figure 5C), lower times in the target quadrant (Figure 5D), and lower frequency to cross platform (Figure 5E). Moreover, we also observed that the effect of ZSWF on reducing hippocampus and serum proinflammatory cytokines was eliminated by antibiotic intervention (Figures 5F–M). Meanwhile, the effect of ZSWF intervention on increasing fecal SCFAs in DCI mice was eliminated (Supplementary Figure S5). These results suggest that maintaining intestinal microflora homeostasis is a critical pathway for ZSWF to reverse cognitive function and reduce inflammation in DCI mice.
FIGURE 5. Microbiota ablation with antibiotics eliminated the effects of ZSWF on improving cognitive function and reducing inflammation in DCI mice. (A) effects of antibiotic treatment on relative abundance of intestinal bacteria at genus level in DCI mice. The heat map represents the relative sequence abundance of Top50 OTU of genus level bacteria. The redder color represents more bacteria composition or the higher relative abundance of genus level bacteria. The horizontal axis represents groups, D1–D6 are six samples from the DCI group, and A1–A6 are six samples from the antibiotic group. (B) escape latency, (C) time of first arrival at platform, (D) duration in platform quadrant, (E) the frequency of crossing the platform. These data were expressed as mean ± SD (n = 15). Escape latency data were analyzed by repeated measurement ANOVA, and other data were analyzed by one-way, ##p < 0.01, #p < 0.05 vs. DCI group, $p < 0.05 vs. ZSWF group. (F) MCP-1 contents in the hippocampus, (G) TNF-α contents in the hippocampus, (H) IL-1β contents in the hippocampus, (I) IL-6 contents in the hippocampus, (J) INF-γ levels in the serum, (K) TNF-α levels in the serum, (L) IL-1β levels in the serum, (M) IL-6 levels in the serum. These data were expressed as mean ± SD (n = 6), one-way ANOVA was used to compare statistical differences between groups, ##p < 0.01, #p < 0.05 vs. DCI group, $$p < 0.01, $p < 0.05 vs. ZSWF group.
Cognitive dysfunction is one of the complications of diabetes, and no clinically approved treatment drug exists. Previous cumulative studies showed that DCI is closely associated with intestinal microbiome dysregulation (Xu et al., 2017; Zhang et al., 2021a), suggesting that maintaining intestinal microbiome homeostasis may be an effective strategy to prevent and treat DCI. In this study, we investigated the effects of ZSWF on the cognitive function of DCI mice, and we explored the mechanism from the perspective of gut-microbial-brain axis. Next, we present evidence that suggests that ZSWF supplementation ameliorated the diabetes-induced cognitive dysfunction and reduced neuroinflammation and systemic inflammation. Furthermore, ZSWF treatment protected intestinal barrier integrity and maintained intestinal microbiota homeostasis in DCI mice. In particular, the effects of ZSWF treatment on improving diabetes-induced cognitive impairment and inflammation were eliminated after antibiotic treatment deleted intestinal bacteria, highlighting the essential role of gut microbiota in improving cognitive function of ZSWF.
In this study, we established a diabetic mouse model according to the method previously reported (Kusakabe et al., 2009). Given that our previous studies confirmed a certain incidence of diabetes-induced cognitive impairment (Song et al., 2017), mice with cognitive impairment were screened by MWM after 8 weeks of hyperglycemic stimulation. Our screening results revealed that diabetic mice demonstrated an approximately 80% chance of developing cognitive impairment (Unpublished). Then, we treated DCI mice with ZSWF extract for 8 weeks. FBG was measured weekly during treatment, and cognitive function was assessed after treatment. Likewise, we found that ZSWF significantly improved cognitive function and protected hippocampal neuron damage in DCI mice. However, this study found that ZSWF could not improve FBG, the initial pathological factor of DCI mice, suggesting that improving the subsequent complex pathological links mediated by hyperglycemia may be the mechanism by which ZSWF improves DCI.
Accumulated evidence suggests that neuroinflammation is an important pathological mechanism of DCI (Muriach et al., 2014; Jeong et al., 2021). IL-6, IL-1β, and TNF-α are common proinflammatory cytokines, which are significantly altered in a variety of acute and chronic inflammatory diseases. IFN-γ is secreted mainly by natural killer cells and natural killer T cells and plays a role in innate immunity. A large number of excellent previous studies confirmed that the diabetic hyperglycemic environment mediates changes in innate immune system function and significantly increases IFN-γ levels. MCP-1 is a major chemokine that recruits monocyte/macrophage to the site of tissue injury and plays a critical role in microvascular complications of diabetes. Therefore, in order to investigate the effects of ZSWF on the system and neuroinflammation of DCI mice, we used the ELISA kit to detect the changes of the above cytokines, and we found that the levels of these proinflammatory factors in the hippocampus of DCI mice were significantly increased, which was consistent with previous reports (Wang et al., 2019; Zeinivand et al., 2020). Likewise, our current study also revealed that ZSWF reduced hippocampal proinflammatory cytokines levels in DCI mice. Also, we found that ZSWF inhibited the activation of brain-resident immune cells (microglia and astrocytes) in DCI mice (unpublished). In addition to brain-resident immune cells releasing proinflammatory cytokines, peripheral circulating proinflammatory cytokines crossing the blood-brain barrier is also a key pathologic pathway of neuroinflammation. In a similar manner, our results showed that ZSWF significantly reduced peripheral circulating proinflammatory cytokines in DCI mice. These results suggest that the reduction of peripheral circulation and neuroinflammation is the mechanism of ZSWF to improve DCI. However, studies showed that the bioavailability of main chemical components of Anemarrhenae Rhizoma and Phellodendri Chinensis Cortex is low (Singh and Chaudhuri, 2018; Baldim et al., 2020), and our previous in vivo chemical analysis of ZSWF also showed that most components in Cistanches Herba were concentrated in feces (Zheng et al., 2018), suggesting that regulation of gastrointestinal function or intestinal flora may play a crucial role in the reversal of DCI inflammation by ZSWF.
Increasing evidence also indicates that diabetes-induced systemic inflammation is mainly caused by impaired intestinal barrier integrity and dysbiosis of intestinal flora (Cani et al., 2008; Cani et al., 2009; Li et al., 2019). Our detection results of intestinal barrier integrity related parameters showed that the intestinal barrier integrity was damaged in DCI mice, which was consistent with previous reports (Visser et al., 2010; Li et al., 2022; Xiao et al., 2022). Likewise, our results show that ZSWF exhibits a protective effect on intestinal barrier integrity in DCI mice by increasing colon tight junction protein expression (ZO-1 and occludin) and mucin (Muc2) content, as well as colon content immunoglobulin A (SlgA) levels. These results systematically confirmed that ZSWF maintained intestinal barrier integrity in DCI mice.
Intestinal microbiome is a key component of the intestinal barrier, and its homeostasis can lead to an increase in the permeability of the intestinal barrier. The results of our 16S rDNA sequencing revealed that the intestinal microbiome composition of DCI mice was significantly altered, and ZSWF demonstrated a significant reversal effect on the microbiome disorder of DCI mice. The relative abundance results of phylum level bacteria showed that DCI mice increased the relative abundance of Firmicutes and Proteoobacteria, and they significantly decreased the abundance of Bacteroidetes, which was consistent with previous reports (Zhu et al., 2016). More notably, ZSWF improved the imbalance of intestinal bacteria, as demonstrated by a decreased ratio of Firmicutes to Bacteroides. At the genus levels, our results showed that ZSWF treatment significantly increased the abundance of Bacteroides and Alistipes, and it decreased the abundance of Desulfouibrio, Dorea, and Allobaculum. Clinical studies linked changes in Bacteroides to cognitive and neurodegenerative diseases (Cattaneo et al., 2017; Saji et al., 2019). Also, previous studies found that Bacteroides can reduce inflammation (Tan et al., 2019) and protect intestinal mucosal permeability (Hooper et al., 2001). Studies found that the abundance of Alistipes is significantly reduced in patients with mild cognitive impairment, suggesting that Alistipes is negatively correlated with cognitive function (Zhang et al., 2021b). Desulfovibrio is a proinflammatory bacterium (Simpson et al., 2021) and can produce hydrogen sulfide, which demonstrates a cytotoxic effect (Gibson, 1990). The above evidence suggested that ZSWF could maintain intestinal microbiome homeostasis in DCI mice, which was reflected in that ZSWF increased the relative abundance of beneficial bacteria in DCI mice and decreased the abundance of bacteria that produced cytotoxic substances. Antibiotic cocktail treatment is an appropriate method to explore the effects of intestinal bacteria on physiology and disease in animal (Kennedy et al., 2018). Data from ZSWF combined with antibiotic therapy revealed that oral antibiotics did not improve or worsen FBG, cognitive function, as well as inflammation in DCI mice. However, oral antibiotics treatment eliminated the effect of ZSWF on cognitive function and inflammation in DCI mice, suggesting that gut microbes are necessary to ZSWF to improve cognitive function and inflammation in DCI mice.
The regulation of intestinal flora by ZSWF in DCI mice may be bidirectional. On the one hand, the chemical composition of ZSWF maintained the composition of intestinal microbes in DCI mice, and on the other hand, intestinal microbes in DCI mice metabolized the components with low bioavailability of ZSWF. The potential active components of ZSWF regulating intestinal flora of DCI mice may be polysaccharides in Cistanches Herba and alkaloids in Phellodendri Chinensis Cortex. Previous studies confirmed that Cistanche polysaccharides demonstrate a clear regulatory effect on intestinal flora (Fu et al., 2020; Fan et al., 2021; Gao et al., 2021). Although accumulative studies confirmed that berberine, the main alkaloid component of Phellodendri Chinensis Cortex, can protect cognitive function (Aski et al., 2018; Shinjyo et al., 2020; Yi et al., 2021), subsequent studies gradually recognized that regulating intestinal flora may be the initial link of berberine improving cognitive function (Zhang et al., 2020b; Habtemariam, 2020). The ZSWF components metabolized by intestinal flora of DCI mice may be saponins in Anemarrhenae Rhizoma (Tian et al., 2016; Dong et al., 2021). Furthermore, besides the immune signaling pathway investigated in this study, the mechanism of ZSWF regulating intestinal flora to improve DCI may also include the following aspects: first, regulating the tryptophan-kynurenine metabolic pathway. Previous cumulative studies confirmed that gut microbiota plays an important role in regulating tryptophan-kynurenine metabolism (Kennedy et al., 2017; Agus et al., 2018; Deng et al., 2021), and several studies also found that tryptophan-kynurenine metabolism is involved in the regulation of glutamate neurotransmitters and synaptic excitability, which is crucial for the protection of cognitive function (Oxenkrug, 2007; Forrest et al., 2015; Tanaka et al., 2020; Bakker et al., 2021). More notably, our previous study found abnormal kynurenine metabolism in DCI mice, and ZSWF treatment could improve kynurenine metabolism in DCI mice (Yin et al., 2022). These studies suggest that regulation of tryptophan-kynurenine metabolic pathway may be the mechanism by which ZSWF improves cognitive function by maintaining intestinal microbiome homeostasis in DCI mice. Second, the increase the level of SCFAs derived from gut microbiota. As the main metabolite of gut microbiota, SCFAs have been reported to ameliorate cognitive impairment mediated by a variety of etiologies, including DCI (Lee et al., 2020; Enry et al., 2021; Qian et al., 2021; Zheng et al., 2021). Our previous study also found that ZSWF increased fecal SCFAs levels in DCI mice (unpublished). These results suggest that increasing the level of SCFAs derived from gut microbiota may also be the mechanism by which ZSWF regulates intestinal microflora to improve cognitive function in DCI mice. However, this study still demonstrates some limitations. For example, although our previous studies identified the main chemical components of ZSWF extract in vivo and in vitro, including the main archetypes and metabolic components in feces (Zheng et al., 2018), which components are involved in maintaining intestinal microbiota homeostasis and their mechanisms remain unclear. Moreover, since no drug with a definite therapeutic effect for DCI exists in clinical practice at present, this study did not select a suitable positive control.
ZSWF can improve cognitive dysfunction and neuroinflammation in DCI mice, while oral antibiotics can partially eliminate these effects, suggesting that maintaining intestinal microbial homeostasis may be the underlying mechanism of ZSWF to improve cognitive function in DCI mice.
The datasets presented in this study can be found in online repositories. The names of the repository/repositories and accession number(s) can be found below: https://www.ncbi.nlm.nih.gov/bioproject/PRJNA836714/.
The animal study was reviewed and approved by Animal Ethics Committee of Tianjin University of Traditional Chinese Medicine.
YZ and PZ designed research; JS, QY, LZ, YW, PY, MG, LY, and ZW performed research; JS, QY, LZ, YW, PY, MG, LY, and ZW analyzed data; JS, QY, LZ, and HL wrote the paper. All authors read and approved the final manuscript.
This work was supported by the National Natural Science Foundation of China (Nos. 82174112 and Nos. 81904146).
The authors declare that the research was conducted in the absence of any commercial or financial relationships that could be construed as a potential conflict of interest.
All claims expressed in this article are solely those of the authors and do not necessarily represent those of their affiliated organizations, or those of the publisher, the editors, and the reviewers. Any product that may be evaluated in this article, or claim that may be made by its manufacturer, is not guaranteed or endorsed by the publisher.
The Supplementary Material for this article can be found online at: https://www.frontiersin.org/articles/10.3389/fphar.2022.898360/full#supplementary-material
ZSWF, Zi Shen Wan Fang; DCI, diabetic cognitive impairment; STZ, streptozotocin; ABs, antibiotic; MWM, morris water maze; HE, hematoxylin-eosin; UPLC-MS/MS, high performance liquid chromatography tandem mass spectrometry; DM, diabetes mellitus; SCFAs, short-chain fatty acids; HFD, high-fat diet; Muc2, Mucin-2; SIgA, secretory immunoglobulin A; PCoA, weighted unifrac principal coordinate analysis; FBG, fasting bood glucose.
Agus, A., Planchais, J., and Sokol, H. (2018). Gut Microbiota Regulation of Tryptophan Metabolism in Health and Disease. Cell. Host Microbe 23 (6), 716–724. doi:10.1016/j.chom.2018.05.003
Aski, M. L., Rezvani, M. E., Khaksari, M., Hafizi, Z., Pirmoradi, Z., Niknazar, S., et al. (2018). Neuroprotective Effect of Berberine Chloride on Cognitive Impairment and Hippocampal Damage in Experimental Model of Vascular Dementia. Iran. J. Basic Med. Sci. 21 (1), 53–58. doi:10.22038/IJBMS.2017.23195.5865
Bairamian, D., Sha, S., Rolhion, N., Sokol, H., Dorothée, G., Lemere, C. A., et al. (2022). Microbiota in Neuroinflammation and Synaptic Dysfunction: a Focus on Alzheimer's Disease. Mol. Neurodegener. 17 (1), 19. doi:10.1186/s13024-022-00522-2
Bakker, L., Ramakers, I. H. G. B., van Boxtel, M. P. J., Schram, M. T., Stehouwer, C. D. A., van der Kallen, C. J. H., et al. (2021). Associations between Plasma Kynurenines and Cognitive Function in Individuals with Normal Glucose Metabolism, Prediabetes and Type 2 Diabetes: the Maastricht Study. Diabetologia 64 (11), 2445–2457. doi:10.1007/s00125-021-05521-4
Baldim, I., Oliveira, W. P., Kadian, V., Rao, R., Yadav, N., Mahant, S., et al. (2020). Natural Ergot Alkaloids in Ocular Pharmacotherapy: Known Molecules for Novel Nanoparticle-Based Delivery Systems. Biomolecules 10 (7), 980. doi:10.3390/biom10070980
Biessels, G. J., and Despa, F. (2018). Cognitive Decline and Dementia in Diabetes Mellitus: Mechanisms and Clinical Implications. Nat. Rev. Endocrinol. 14 (10), 591–604. doi:10.1038/s41574-018-0048-7
Biessels, G. J., and Whitmer, R. A. (2020). Cognitive Dysfunction in Diabetes: How to Implement Emerging Guidelines. Diabetologia 63, 3–9. doi:10.1007/s00125-019-04977-9
Cai, T. T., Ye, X. L., Li, R. R., Chen, H., Wang, Y. Y., Yong, H. J., et al. (2020). Resveratrol Modulates the Gut Microbiota and Inflammation to Protect against Diabetic Nephropathy in Mice. Front. Pharmacol. 11, 1249. doi:10.3389/fphar.2020.01249
Cani, P. D., Bibiloni, R., Knauf, C., Waget, A., Neyrinck, A. M., Delzenne, N. M., et al. (2008). Changes in Gut Microbiota Control Metabolic Endotoxemia-Induced Inflammation in High-Fat Diet-Induced Obesity and Diabetes in Mice. Diabetes 57 (6), 1470–1481. doi:10.2337/db07-1403
Cani, P. D., Possemiers, S., Van de Wiele, T., Guiot, Y., Everard, A., Rottier, O., et al. (2009). Changes in Gut Microbiota Control Inflammation in Obese Mice through a Mechanism Involving GLP-2-Driven Improvement of Gut Permeability. Gut 58 (8), 1091–1103. doi:10.1136/gut.2008.165886
Cattaneo, A., Cattane, N., Galluzzi, S., Provasi, S., Lopizzo, N., Festari, C., et al. (2017). Association of Brain Amyloidosis with Pro-inflammatory Gut Bacterial Taxa and Peripheral Inflammation Markers in Cognitively Impaired Elderly. Neurobiol. Aging. 49, 60–68. doi:10.1016/j.neurobiolaging.2016.08.019
Daniel, H., Gholami, A. M., Berry, D., Desmarchelier, C., Hahne, H., Loh, G., et al. (2014). High-fat Diet Alters Gut Microbiota Physiology in Mice. ISME J. 8 (2), 295–308. doi:10.1038/ismej.2013.155
Deng, Y., Zhou, M., Wang, J., Yao, J., Yu, J., Liu, W., et al. (2021). Involvement of the Microbiota-Gut-Brain axis in Chronic Restraint Stress: Disturbances of the Kynurenine Metabolic Pathway in Both the Gut and Brain. Gut Microbes 13, 1–16. doi:10.1080/19490976.2020.1869501
Dong, G. M., Yu, H., Pan, L. B., Ma, S. R., Xu, H., Zhang, Z. W., et al. (2021). Biotransformation of Timosaponin BII into Seven Characteristic Metabolites by the Gut Microbiota. Molecules 26 (13), 3861. doi:10.3390/molecules26133861
Erny, D., Dokalis, N., Mezö, C., Castoldi, A., Mossad, O., Staszewski, O., et al. (2021). Microbiota-derived Acetate Enables the Metabolic Fitness of the Brain Innate Immune System during Health and Disease. Cell. Metab. 33 (11), 2260–2276.e7. doi:10.1016/j.cmet.2021.10.010
Esser, N., Legrand-Poels, S., Piette, J., Scheen, A. J., and Paquot, N. (2014). Inflammation as a Link between Obesity, Metabolic Syndrome and Type 2 Diabetes. Diabetes Res. Clin. Pract. 105 (2), 141–150. doi:10.1016/j.diabres.2014.04.006
Fan, L., Peng, Y., Wang, J., Ma, P., Zhao, L., and Li, X. (2021). Total Glycosides from Stems of Cistanche Tubulosa Alleviate Depression-like Behaviors: Bidirectional Interaction of the Phytochemicals and Gut Microbiota. Phytomedicine 83, 153471. doi:10.1016/j.phymed.2021.153471
Forrest, C. M., McNair, K., Pisar, M., Khalil, O. S., Darlington, L. G., and Stone, T. W. (2015). Altered Hippocampal Plasticity by Prenatal Kynurenine Administration, Kynurenine-3-Monoxygenase (KMO) Deletion or Galantamine. Neuroscience 310, 91–105. doi:10.1016/j.neuroscience.2015.09.022
Fu, Z., Han, L., Zhang, P., Mao, H., Zhang, H., Wang, Y., et al. (2020). Cistanche Polysaccharides Enhance Echinacoside Absorption In Vivo and Affect the Gut Microbiota. Int. J. Biol. Macromol. 149, 732–740. doi:10.1016/j.ijbiomac.2020.01.216
Gao, H., Jiang, Q., Ji, H., Ning, J., Li, C., and Zheng, H. (2019). Type 1 Diabetes Induces Cognitive Dysfunction in Rats Associated with Alterations of the Gut Microbiome and Metabolomes in Serum and hippocampus. Biochim. Biophys. Acta Mol. Basis Dis. 1865, 165541. doi:10.1016/j.bbadis.2019.165541
Gao, Y., Li, B., Liu, H., Tian, Y., Gu, C., Du, X., et al. (2021). Cistanche Deserticola Polysaccharides Alleviate Cognitive Decline in Aging Model Mice by Restoring the Gut Microbiota-Brain axis. Aging (Albany NY) 13 (11), 15320–15335. doi:10.18632/aging.203090
Gibson, G. R. (1990). Physiology and Ecology of the Sulphate-Reducing Bacteria. J. Appl. Bacteriol. 69 (6), 769–797. doi:10.1111/j.1365-2672.1990.tb01575.x
Habtemariam, S. (2020). Berberine Pharmacology and the Gut Microbiota: A Hidden Therapeutic Link. Pharmacol. Res. 155, 104722. doi:10.1016/j.phrs.2020.104722
Hooper, L. V., Wong, M. H., Thelin, A., Hansson, L., Falk, P. G., and Gordon, J. I. (2001). Molecular Analysis of Commensal Host-Microbial Relationships in the Intestine. Science 291 (5505), 881–884. doi:10.1126/science.291.5505.881
Jeong, E. A., Lee, J., Shin, H. J., Lee, J. Y., Kim, K. E., An, H. S., et al. (2021). Tonicity-responsive Enhancer-Binding Protein Promotes Diabetic Neuroinflammation and Cognitive Impairment via Upregulation of Lipocalin-2. J. Neuroinflammation 18 (1), 278. doi:10.1186/s12974-021-02331-8
Kennedy, E. A., King, K. Y., and Baldridge, M. T. (2018). Mouse Microbiota Models: Comparing Germ-free Mice and Antibiotics Treatment as Tools for Modifying Gut Bacteria. Front. Physiol. 9, 1534. doi:10.3389/fphys.2018.01534
Kennedy, P. J., Cryan, J. F., Dinan, T. G., and Clarke, G. (2017). Kynurenine Pathway Metabolism and the Microbiota-Gut-Brain axis. Neuropharmacology 112 (Pt B), 399–412. doi:10.1016/j.neuropharm.2016.07.002
Kubica, P., Kot-Wasik, A., Wasik, A., Namieśnik, J., and Landowski, P. (2012). Modern Approach for Determination of Lactulose, Mannitol and Sucrose in Human Urine Using HPLC-MS/MS for the Studies of Intestinal and Upper Digestive Tract Permeability. J Chromatogr B Analyt Technol Biomed Life Sci. 907, 34–40. doi:10.1016/j.jchromb.2012.08.031
Kusakabe, T., Tanioka, H., Ebihara, K., Hirata, M., Miyamoto, L., Miyanaga, F., et al. (2009). Beneficial Effects of Leptin on Glycaemic and Lipid Control in a Mouse Model of Type 2 Diabetes with Increased Adiposity Induced by Streptozotocin and a High-Fat Diet. Diabetologia 52 (4), 675–683. doi:10.1007/s00125-009-1258-2
Lee, J., d'Aigle, J., Atadja, L., Quaicoe, V., Honarpisheh, P., Ganesh, B. P., et al. (2020). Gut Microbiota-Derived Short-Chain Fatty Acids Promote Poststroke Recovery in Aged Mice. Circ. Res. 127 (4), 453–465. doi:10.1161/CIRCRESAHA.119.316448
Li, K., Zhang, L., Xue, J., Yang, X., Dong, X., Sha, L., et al. (2019). Dietary Inulin Alleviates Diverse Stages of Type 2 Diabetes Mellitus via Anti-inflammation and Modulating Gut Microbiota in Db/db Mice. Food Funct. 10 (4), 1915–1927. doi:10.1039/c8fo02265h
Li, X., Zhao, T., Gu, J., Wang, Z., Lin, J., Wang, R., et al. (2022). Intake of Flavonoids from Astragalus Membranaceus Ameliorated Brain Impairment in Diabetic Mice via Modulating Brain-Gut axis. Chin. Med. 17 (1), 22. doi:10.1186/s13020-022-00578-8
Li, Y., Peng, Y., Wang, M., Tu, P., and Li, X. (2017). Human Gastrointestinal Metabolism of the Cistanches Herba Water Extract In Vitro: Elucidation of the Metabolic Profile Based on Comprehensive Metabolite Identification in Gastric Juice, Intestinal Juice, Human Intestinal Bacteria, and Intestinal Microsomes. J. Agric. Food Chem. 65, 7447–7456. doi:10.1021/acs.jafc.7b02829
Liang, Y., Huang, M., Jiang, X., Liu, Q., Chang, X., and Guo, Y. (2017). The Neuroprotective Effects of Berberine against Amyloid β-protein-induced Apoptosis in Primary Cultured Hippocampal Neurons via Mitochondria-Related Caspase Pathway. Neurosci. Lett. 655, 46–53. doi:10.1016/j.neulet.2017.06.048
Liu, C. S., Liang, X., Wei, X. H., Jin, Z., Chen, F. L., Tang, Q. F., et al. (2019). Gegen Qinlian Decoction Treats Diarrhea in Piglets by Modulating Gut Microbiota and Short-Chain Fatty Acids. Front. Microbiol. 10, 825. doi:10.3389/fmicb.2019.00825
Liu, Y. W., Zhu, X., Lu, Q., Wang, J. Y., Li, W., Wei, Y. Q., et al. (2012). Total Saponins from Rhizoma Anemarrhenae Ameliorate Diabetes-Associated Cognitive Decline in Rats: Involvement of Amyloid-Beta Decrease in Brain. J. Ethnopharmacol. 139, 194–200. doi:10.1016/j.jep.2011.11.004
Mills, D. J., Tuohy, K. M., Booth, J., Buck, M., Crabbe, M. J., Gibson, G. R., et al. (2008). Dietary Glycated Protein Modulates the Colonic Microbiota towards a More Detrimental Composition in Ulcerative Colitis Patients and Non-ulcerative Colitis Subjects. J. Appl. Microbiol. 105 (3), 706–714. doi:10.1111/j.1365-2672.2008.03783.x
Morris, R. (1984). Developments of a Water-Maze Procedure for Studying Spatial Learning in the Rat. J. Neurosci. Methods 11 (1), 47–60. doi:10.1016/0165-0270(84)90007-4
Muriach, M., Flores-Bellver, M., Romero, F. J., and Barcia, J. M. (2014). Diabetes and the Brain: Oxidative Stress, Inflammation, and Autophagy. Oxidative Med. Cell. Longev. 2014 (9), 1. doi:10.1155/2014/102158
Nathavitharana, K. A., Lloyd, D. R., Raafat, F., Brown, G. A., and McNeish, A. S. (1988). Urinary Mannitol: Lactulose Excretion Ratios and Jejunal Mucosal Structure. Arch. Dis. Child. 63 (9), 1054–1059. doi:10.1136/adc.63.9.1054
Oxenkrug, G. F. (2007). Genetic and Hormonal Regulation of Tryptophan Kynurenine Metabolism: Implications for Vascular Cognitive Impairment, Major Depressive Disorder, and Aging. Ann. N. Y. Acad. Sci. 1122, 35–49. doi:10.1196/annals.1403.003
Piwowar, A., Rembiałkowska, N., Rorbach-Dolata, A., Garbiec, A., Ślusarczyk, S., Dobosz, A., et al. (2020). Anemarrhenae Asphodeloides Rhizoma Extract Enriched in Mangiferin Protects PC12 Cells against a Neurotoxic Agent-3-Nitropropionic Acid. Int. J. Mol. Sci. 21 (7), 2510. doi:10.3390/ijms21072510
Prakash, R., Somanath, P. R., El-Remessy, A. B., Kelly-Cobbs, A., Stern, J. E., Dore-Duffy, P., et al. (2012). Enhanced Cerebral but Not Peripheral Angiogenesis in the Goto-Kakizaki Model of Type 2 Diabetes Involves VEGF and Peroxynitrite Signaling. Diabetes 61, 1533–1542. doi:10.2337/db11-1528
Qian, X., Xie, R., Liu, X., Chen, S., and Tang, H. (2021). Mechanisms of Short-Chain Fatty Acids Derived from Gut Microbiota in Alzheimer's Disease. Aging Dis. doi:10.14336/AD.2021.1215
Rhee, S. H., Pothoulakis, C., and Mayer, E. A. (2009). Principles and Clinical Implications of the Brain-Gut-Enteric Microbiota axis. Nat. Rev. Gastroenterol. Hepatol. 6, 306–314. doi:10.1038/nrgastro.2009.35
Rogers, G. B., Keating, D. J., Young, R. L., Wong, M. L., Licinio, J., and Wesselingh, S. (2016). From Gut Dysbiosis to Altered Brain Function and Mental Illness: Mechanisms and Pathways. Mol. Psychiatry. 21, 738–748. doi:10.1038/mp.2016.50
Rom, S., Zuluaga-Ramirez, V., Gajghate, S., Seliga, A., Winfield, M., Heldt, N. A., et al. (2019). Hyperglycemia-Driven Neuroinflammation Compromises BBB Leading to Memory Loss in Both Diabetes Mellitus (DM) Type 1 and Type 2 Mouse Models. Mol. Neurobiol. 56 (3), 1883–1896. doi:10.1007/s12035-018-1195-5
Saji, N., Niida, S., Murotani, K., Hisada, T., Tsuduki, T., Sugimoto, T., et al. (2019). Analysis of the Relationship between the Gut Microbiome and Dementia: a Cross-Sectional Study Conducted in Japan. Sci. Rep. 9, 1008–1009. doi:10.1038/s41598-018-38218-7
Shinjyo, N., Parkinson, J., Bell, J., Katsuno, T., and Bligh, A. (2020). Berberine for Prevention of Dementia Associated with Diabetes and its Comorbidities: A Systematic Review. J. Integr. Med. 18 (2), 125–151. doi:10.1016/j.joim.2020.01.004
Simpson, C. A., Diaz-Arteche, C., Eliby, D., Schwartz, O. S., Simmons, J. G., and Cowan, C. S. M. (2021). The Gut Microbiota in Anxiety and Depression - A Systematic Review. Clin. Psychol. Rev. 83, 101943. doi:10.1016/j.cpr.2020.101943
Singh, D., and Chaudhuri, P. K. (2018). Structural Characteristics, Bioavailability and Cardioprotective Potential of Saponins. Integr. Med. Res. 7 (1), 33–43. doi:10.1016/j.imr.2018.01.003
Song, L., Zhuang, P., Lin, M., Kang, M., Liu, H., Zhang, Y., et al. (2017). Urine Metabonomics Reveals Early Biomarkers in Diabetic Cognitive Dysfunction. J. Proteome Res. 16, 3180–3189. doi:10.1021/acs.jproteome.7b00168
Tan, H., Zhao, J., Zhang, H., Zhai, Q., and Chen, W. (2019). Novel Strains of Bacteroides Fragilis and Bacteroides Ovatus Alleviate the LPS-Induced Inflammation in Mice. Appl. Microbiol. Biotechnol. 103 (5), 2353–2365. doi:10.1007/s00253-019-09617-1
Tanaka, M., Bohár, Z., and Vécsei, L. (2020). Are Kynurenines Accomplices or Principal Villains in Dementia? Maintenance of Kynurenine Metabolism. Molecules 25 (3), 564. doi:10.3390/molecules25030564
Tian, X., Xu, Z., Li, Z., Ma, Y., Lian, S., Guo, X., et al. (2016). Pharmacokinetics of Mangiferin and its Metabolite-Norathyriol, Part 2: Influence of UGT, CYP450, P-Gp, and Enterobacteria and the Potential Interaction in Rhizoma Anemarrhenae Decoction with Timosaponin B2 as the Major Contributor. Biofactors 42 (5), 545–555. doi:10.1002/biof.1290
Visser, J. T., Lammers, K., Hoogendijk, A., Boer, M. W., Brugman, S., Beijer-Liefers, S., et al. (2010). Restoration of Impaired Intestinal Barrier Function by the Hydrolysed Casein Diet Contributes to the Prevention of Type 1 Diabetes in the Diabetes-Prone BioBreeding Rat. Diabetologia 53 (12), 2621–2628. doi:10.1007/s00125-010-1903-9
Wang, D., Wang, H., and Gu, L. (2017). The Antidepressant and Cognitive Improvement Activities of the Traditional Chinese HerbCistanche. Evidence-Based Complementary Altern. Med. 2017, 1–9. doi:10.1155/2017/3925903
Wang, K., Song, F., Xu, K., Liu, Z., Han, S., Li, F., et al. (2019). Irisin Attenuates Neuroinflammation and Prevents the Memory and Cognitive Deterioration in Streptozotocin-Induced Diabetic Mice. Mediat. Inflamm. 2019, 1–8. doi:10.1155/2019/1567179
Wenzel, T. J., Gates, E. J., Ranger, A. L., and Klegeris, A. (2020). Short-chain Fatty Acids (SCFAs) Alone or in Combination Regulate Select Immune Functions of Microglia-like Cells. Mol. Cell. Neurosci. 105, 103493. doi:10.1016/j.mcn.2020.103493
Xian, Y. F., Lin, Z. X., Ip, S. P., Su, Z. R., Chen, J. N., and Lai, X. P. (2013). Comparison the Neuropreotective Effect of Cortex Phellodendri Chinensis and Cortex Phellodendri Amurensis against Beta-Amyloid-Induced Neurotoxicity in PC12 Cells. Phytomedicine 20, 187–193. doi:10.1016/j.phymed.2012.09.028
Xiao, Y., Li, K., Bian, J., Liu, H., Zhai, X., El‐Omar, E., et al. (2022). Urolithin A Attenuates Diabetes‐Associated Cognitive Impairment by Ameliorating Intestinal Barrier Dysfunction via N‐glycan Biosynthesis Pathway. Mol. Nutr. Food Res. 66, 2100863. doi:10.1002/mnfr.202100863
Xu, Y., Zhou, H., and Zhu, Q. (2017). The Impact of Microbiota-Gut-Brain axis on Diabetic Cognition Impairment. Front. Aging Neurosci. 9, 106–118. doi:10.3389/fnagi.2017.00106
Xu, Y. H., Gao, C. L., Guo, H. L., Zhang, W. Q., Huang, W., Tang, S. S., et al. (2018). Sodium Butyrate Supplementation Ameliorates Diabetic Inflammation in Db/db Mice. J. Endocrinol. 238 (3), 231–244. doi:10.1530/JOE-18-0137
Yi, L.-t., Zhu, J.-x., Dong, S.-q., Chen, M., and Li, C.-f. (2021). Berberine Exerts Antidepressant-like Effects via Regulating miR-34a-synaptotagmin1/Bcl-2 axis. Chin. Herb. Med. 13, 116–123. doi:10.1016/j.chmed.2020.11.001
Yin, Q., Zhang, L., Han, X., Zhang, H., Wang, F., Qin, X., et al. (2022). Zi Shen Wan Fang Regulates Kynurenine Metabolism to Alleviate Diabetes-Associated Cognitive Impairment via Activating the Skeletal Muscle PGC1α-Pparα Signaling. Phytomedicine 99, 154000. doi:10.1016/j.phymed.2022.154000
Yu, F., Han, W., Zhan, G., Li, S., Xiang, S., Zhu, B., et al. (2019). Abnormal Gut Microbiota Composition Contributes to Cognitive Dysfunction in Streptozotocin-Induced Diabetic Mice. Aging (Albany NY) 11, 3262–3279. doi:10.18632/aging.101978
Zeinivand, M., Nahavandi, A., and Zare, M. (2020). Deferoxamine Regulates Neuroinflammation and Oxidative Stress in Rats with Diabetes-Induced Cognitive Dysfunction. Inflammopharmacology 28 (2), 575–583. doi:10.1007/s10787-019-00665-7
Zhang, J., Zhuang, P., Wang, Y., Song, L., Zhang, M., Lu, Z., et al. (2014). Reversal of Muscle Atrophy by Zhimu-Huangbai Herb-Pair via Akt/mTOR/FoxO3 Signal Pathway in Streptozotocin-Induced Diabetic Mice. PLoS One 9 (6), e100918. doi:10.1371/journal.pone.0100918
Zhang, P., Yu, Y., Qin, Y., Zhou, Y., Tang, R., Wang, Q., et al. (2019). Alterations to the Microbiota-Colon-Brain axis in High-Fat-Diet-Induced Obese Mice Compared to Diet-Resistant Mice. J. Nutr. Biochem. 65, 54–65. doi:10.1016/j.jnutbio.2018.08.016
Zhang, T., Zheng, H., Fan, K., Xia, N., Li, J., Yang, C., et al. (2020a). NMR-based Metabolomics Characterizes Metabolic Changes in Different Brain Regions of Streptozotocin-Induced Diabetic Mice with Cognitive Decline. Metab. Brain Dis. 35, 1165–1173. doi:10.1007/s11011-020-00598-z
Zhang, X., Wang, Y., Liu, W., Wang, T., Wang, L., Hao, L., et al. (2021b). Diet Quality, Gut Microbiota, and microRNAs Associated with Mild Cognitive Impairment in Middle-Aged and Elderly Chinese Population. Am. J. Clin. Nutr. 114, 429–440. doi:10.1093/ajcn/nqab078
Zhang, Y., Gu, Y., Ren, H., Wang, S., Zhong, H., Zhao, X., et al. (2020b). Gut Microbiome-Related Effects of Berberine and Probiotics on Type 2 Diabetes (The PREMOTE Study). Nat. Commun. 11 (1), 5015. doi:10.1038/s41467-020-18414-8
Zhang, Y., Lu, S., Yang, Y., Wang, Z., Wang, B., Zhang, B., et al. (2021a). The Diversity of Gut Microbiota in Type 2 Diabetes with or without Cognitive Impairment. Aging Clin. Exp. Res. 33, 589–601. doi:10.1007/s40520-020-01553-9
Zheng, H., Xu, P., Jiang, Q., Xu, Q., Zheng, Y., Yan, J., et al. (2021). Depletion of Acetate-Producing Bacteria from the Gut Microbiota Facilitates Cognitive Impairment through the Gut-Brain Neural Mechanism in Diabetic Mice. Microbiome 9 (1), 145. doi:10.1186/s40168-021-01088-9
Zheng, Y., Zhang, Y., Geng, S., Xu, M., Yin, Q., Song, L., et al. (2018). Identification of the Constituents and Metabolites in Rats after Oral Administration of Zi Shen Formula by UPLC-Q-TOF/MS Combined Pattern Recognition Analysis. Biomed. Chromatogr. 32. doi:10.1002/bmc.4060
Zhu, Y., Bai, J., Zhang, Y., Xiao, X., and Dong, Y. (2016). Effects of Bitter Melon (Momordica Charantia L.) on the Gut Microbiota in High Fat Diet and Low Dose Streptozocin-Induced Rats. Int. J. Food Sci. Nutr. 67 (6), 686–695. doi:10.1080/09637486.2016.1197185
Keywords: ZSWF, DCI, inflammation, intestinal barrier, gut microbiome
Citation: Shi J, Yin Q, Zhang L, Wu Y, Yi P, Guo M, Li H, Yuan L, Wang Z, Zhuang P and Zhang Y (2022) Zi Shen Wan Fang Attenuates Neuroinflammation and Cognitive Function Via Remodeling the Gut Microbiota in Diabetes-Induced Cognitive Impairment Mice. Front. Pharmacol. 13:898360. doi: 10.3389/fphar.2022.898360
Received: 17 March 2022; Accepted: 02 June 2022;
Published: 15 July 2022.
Edited by:
Aurelijus Burokas, Vilnius University, LithuaniaReviewed by:
Ruihua Xin, Chinese Academy of Agricultural Sciences, ChinaCopyright © 2022 Shi, Yin, Zhang, Wu, Yi, Guo, Li, Yuan, Wang, Zhuang and Zhang. This is an open-access article distributed under the terms of the Creative Commons Attribution License (CC BY). The use, distribution or reproduction in other forums is permitted, provided the original author(s) and the copyright owner(s) are credited and that the original publication in this journal is cited, in accordance with accepted academic practice. No use, distribution or reproduction is permitted which does not comply with these terms.
*Correspondence: Pengwei Zhuang, emh1YW5ncGVuZ3dlaUAxNjMuY29t; Yanjun Zhang, enlqc3VueWVAMTYzLmNvbQ==
†These authors have contributed equally to this work
Disclaimer: All claims expressed in this article are solely those of the authors and do not necessarily represent those of their affiliated organizations, or those of the publisher, the editors and the reviewers. Any product that may be evaluated in this article or claim that may be made by its manufacturer is not guaranteed or endorsed by the publisher.
Research integrity at Frontiers
Learn more about the work of our research integrity team to safeguard the quality of each article we publish.