- 1First Department of Neurology, School of Medicine, Eginition Hospital, National and Kapodistrian University of Athens, Athens, Greece
- 2Department of Physiology, School of Medicine, National and Kapodistrian University of Athens, Athens, Greece
- 3Fourth Department of Internal Medicine, School of Medicine, University Hospital Attikon, National and Kapodistrian University of Athens, Haidari, Greece
- 4Joint Academic Rheumatology Program, School of Medicine, National and Kapodistrian University of Athens, Athens, Greece
Objective: While multiple sclerosis (MS) is considered the cornerstone of autoimmune demyelinating CNS disorders, systemic autoimmune diseases (SADs) are important MS mimickers. We sought to explore whether distinct clinical, laboratory, and imaging characteristics along with quantitation of peripheral blood type I interferon (IFN) activity could aid in differentiating between them.
Methods: A total of 193 consecutive patients with imaging features suggesting the presence of CNS demyelinating disease with or without relevant clinical manifestations underwent full clinical, laboratory, and imaging evaluation, including testing for specific antibodies against 15 cellular antigens. Expression analysis of type I IFN-inducible genes (MX-1, IFIT-1, and IFI44) was performed by real-time PCR, and a type I IFN score, reflecting type I IFN peripheral activity, was calculated. After joint neurological/rheumatological evaluation and 1 year of follow-up, patients were classified into MS spectrum and CNS autoimmune disorders.
Results: While 66.3% (n = 128) of the patients were diagnosed with MS spectrum disorders (predominantly relapsing–remitting MS), 24.9% (n = 48) were included in the CNS autoimmune group, and out of those, one-fourth met the criteria for SAD (6.7% of the cohort, n = 13); the rest (18.1% of the cohort, n = 35), despite showing evidence of systemic autoimmunity, did not fulfill SAD criteria and comprised the “demyelinating disease with autoimmune features” (DAF) subgroup. Compared to the MS spectrum, CNS autoimmune patients were older, more frequently females, with increased rates of hypertension/hyperlipidemia, family history of autoimmunity, cortical dysfunction, anti-nuclear antibody titers ≥1/320, anticardiolipin IgM positivity, and atypical for MS magnetic resonance imaging lesions. Conversely, lower rates of infratentorial and callosal MRI lesions, CSF T2 oligoclonal bands, and IgG-index positivity were observed in CNS autoimmune patients. Patients fulfilling SAD criteria, but not the DAF group, had significantly higher peripheral blood type I IFN scores at baseline compared to MS spectrum [median (IQR)]: 50.18 (152.50) vs. −0.64 (6.75), p-value: 0.0001.
Conclusion: Our study suggests that underlying systemic autoimmunity is not uncommon in patients evaluated for possible CNS demyelination. Distinct clinical, imaging and laboratory characteristics can aid in early differentiation between MS and CNS-involving systemic autoimmunity allowing for optimal therapeutic strategies. Activated type I IFN pathway could represent a key mediator among MS-like-presenting SADs and therefore a potential therapeutic target.
Introduction
Though multiple sclerosis (MS) is considered the cornerstone of the central nervous system (CNS) demyelinating disorders, several clinical entities are important MS mimickers and need to be taken into consideration in the differential diagnosis (Brownlee et al., 2017; Thompson et al., 2018a; Thompson et al., 2018b; Solomon et al., 2019). Among those, systemic autoimmune diseases (SADs), neuromyelitis optica spectrum disease (NMOSD), and myelin oligodendrocyte glycoprotein antibody-associated disease (MOGAD) are the most common. Less frequently, neurosarcoidosis, neuro-Behçet disease, and chronic lymphocytic inflammation with pontine perivascular enhancement responsive to steroids (CLIPPERS) may present with MS reminiscent manifestations. Despite a distinct pathogenetic background, atherosclerotic small vessel disease or even cerebral autosomal dominant arteriopathy with subcortical infarcts and leukoencephalopathy (CADASIL), Fabry disease, and Susac’s syndrome can also present with MS-resembling features (Thompson et al., 2018b).
SADs, mainly systemic lupus erythematosus (SLE), Sjögren’s syndrome (SS), and antiphospholipid syndrome (APS) have been previously shown to invariably affect the CNS, with transverse myelitis, optic neuritis, and white matter hyper-intensities (WMH) in CNS magnetic resonance imaging (MRI) being the main MS-like manifestations (Delalande et al., 2004; Ferreira et al., 2005; Pelidou et al., 2007; Theodoridou and Settas, 2008; Massara et al., 2010; Magro Checa et al., 2013; Piga et al., 2017; Hughes, 2018; Schwartz et al., 2019). In previous studies, higher rates of homozygous methylenetetrahydrofolate reductase (MTHFR) mutations have been found among patients with autoimmune features and MS-like or vasculitic CNS disease, and a link has been identified between anti-thyroid antibodies with nonspecific WMH and CNS involvement in the setting of APS (Mavragani et al., 2007; Mavragani et al., 2009).
While usually helpful, brain MRI (Stosic et al., 2010; Akasbi et al., 2012; Magro Checa et al., 2013; Magro-Checa et al., 2018) and cerebrospinal fluid (CSF) analysis (Govoni et al., 2016; Venhoff et al., 2019) cannot consistently differentiate between MS and CNS involvement in the setting of SADs. Though the detection of serum autoantibodies has a central role in the diagnosis of SAD, they are reported to precede disease onset by many years (Arbuckle et al., 2003). Additionally, low-titer antinuclear (ANA) and antiphospholipid antibodies are frequently found in the context of MS at variable frequencies ranging between 3%-–63% and 0.9%–8.5%, respectively (Spadaro et al., 1999; Szmyrka-Kaczmarek et al., 2012; Malyavantham et al., 2015; Merashli et al., 2017). Of interest, MRZ reaction [detection of CSF IgG antibody indices to measles (M), rubella (R), and varicella-zoster (Z)] was found to be prevalent in patients with MS and ANA positivity compared to those with rheumatological disease with CNS involvement, providing a potential differentiating tool between the two entities (Venhoff et al., 2019).
Despite the potential phenotypic similarities, distinct underlying pathogenetic mechanisms and therapeutic approaches characterize MS and SAD with CNS involvement. For instance, activation of type I interferon (IFN) pathway has been considered a central pathogenetic event in SADs in association with antibodies against ribonucleoproteins, disease activity indices, and renal involvement (Baechler et al., 2003; Kirou et al., 2005; Weckerle et al., 2011; Tsokos et al., 2016; Ronnblom and Leonard, 2019; Postal et al., 2020; Idborg and Oke, 2021), with type I IFN receptor blockade being recently approved for lupus patients (Morand et al., 2020; Deeks, 2021).
Compared to lupus, MS patients display 30-fold lower type I IFN serum activity (Feng et al., 2012), while type I IFN-induced gene expression has been shown to vary among relapsing–remitting MS (RRMS) patients (van Baarsen et al., 2006). Moreover, a subgroup of MS patients with high peripheral blood type I IFN signature, was found to be refractory to exogenous administration of recombinant IFN-β (Comabella et al., 2009), a well-established MS treatment (Author anonymous, 1993; Kieseier, 2011). Along the same lines, the latter has been shown to exacerbate lupus or other SADs (Borg and Isenberg, 2007; Airo et al., 2008; Bahri et al., 2012).
In view of these diagnostic and therapeutic challenges, in the current study, we sought to explore whether clinical, laboratory, and imaging characteristics as well as type I IFN inducible gene expression in peripheral blood could differentiate MS from systemic autoimmune diseases presenting with CNS involvement. Furthermore, we sought to investigate potential associations between activation of type I IFN pathway with distinct clinical, serological, and imaging features.
Patients and methods
Patients
This was a prospective study, evaluating consecutive patients presenting with imaging features suggesting the presence of demyelinating disease with or without relevant clinical manifestations. After initial neurological assessment and the exclusion of ischemic/hemorrhagic cerebrovascular events, space-occupying lesions, or acute CNS infections, patients with MRI findings raising the suspicion of CNS demyelinating disease were referred to the Demyelinating Diseases Unit of the First Department of Neurology of the National and Kapodistrian University of Athens (NKUA) Medical School at Eginition Hospital for further diagnostic evaluation. Thus, we enrolled 205 consecutive patients from 1 January 2019 to 31 March 2021. The study was approved by the Eginition Hospital Ethical Committee and prior to study enrollment, all patients provided written informed consent, in accord with the requirements of the declaration of Helsinki (Author anonymous, 2013).
Exclusion criteria included the previous diagnosis of MS or SAD, concurrent active infection, and treatment with immunosuppressive or corticosteroids over the last 3 months. Therefore, our final study sample consisted of 193 patients, which underwent full clinical, laboratory, and imaging evaluation. Demographics (sex and age), clinically relevant co-morbidities, and family history of MS or systemic/organ-specific autoimmune disease (AD) were recorded. Neurological manifestations were classified according to the CNS region affected (cortex, optic nerve, brainstem, pyramidal/sensory tracts, cerebellum, and bowel/bladder function) by an experienced neurologist (M.E.E). Bilateral optic neuritis, other visual disturbances, headache, transient loss of consciousness, tinnitus, speech disturbance, and isolated fatigue were classified as features nontypical for MS (Brownlee et al., 2017; Thompson et al., 2018a; Solomon et al., 2019). Evaluation for systemic autoimmune features in the presence of autoantibody positivity was performed by an experienced rheumatologist (C.P.M) and included assessment of constitutional, musculoskeletal and mucocutaneous symptoms, sicca complaints, Raynaud’s phenomenon, cardiorespiratory manifestations, and gastrointestinal/renal abnormalities.
Laboratory Evaluation
Standard laboratory evaluation at baseline included complete blood count, coagulation tests [including prothrombin time (PT) and activated partial thromboplastin time (aPTT)], chemistry panel, B12, and 25-OH-D3 vitamin levels, thyroid function tests, protein electrophoresis, and urinalysis, as well as testing for chronic viral infections [human immunodeficiency virus (HIV) and hepatitis B/C]. Immunology profile testing was also performed including rheumatoid factor (RF), complement levels, antinuclear antibodies (ANA), anti-double strand DNA (anti-dsDNA), and antiphospholipid antibodies, as well as antibodies against cellular antigens. Anti-MOG and anti-aquaporin-4 (AQP4) antibodies were tested upon clinical indication. CSF was obtained by a lumbar puncture at baseline and tested for cell count, glucose and protein levels, oligoclonal bands (OCBs), and IgG index (cutoff of ≥0.65 for positive values, as set by the Laboratory Department of Eginition Hospital). The presence of OCBs was detected with isoelectric focusing (IEF) on agarose gels followed by immunofixation, one of the two most sensitive techniques for this purpose (Freedman et al., 2005). The procedure included isoelectrofocusing on an agarose gel to fractionate the proteins in the CSF and serum samples, performed on the semi-automatic HYDRASYS system (SEBIA, France) followed by immunofixation with anti-IgG antiserum. CSF findings were compared directly with findings from serum samples run simultaneously in the same assay in an adjacent track. Finally, the IgG immunofixation patterns of CSF and serum from the same patient were visually compared and categorized into five OCB constellation types (1–5), according to the European consensus study (Andersson et al., 1994). The evaluation was performed by one experienced clinical pathologist. The presence of ANA was assessed by indirect immunofluorescence (IIF) on the substrate from human Hep-2 larynx cancer cell culture, while antiphospholipid and anti-double strand DNA antibodies were measured by an enzyme-linked immunosorbent assay (ELISA). In isolated sera from all enrolled patients, we tested for auto-antibodies against 15 cellular antigens (RNP/Sm, Sm, SS-A (Ro-60), Ro-52, SS-B, Scl-70, PM-Scl-100, Jo-1, centromere protein B, PCNA, dsDNA, nucleosomes, histones, ribosomal P-proteins, and AMA M), using the EUROLINE ANA Profile 3 commercial immunoblot kit (EUROIMMUN Medizinische Labordiagnostika AG, Germany), as part of a wholesome diagnostic approach. According to the manufacturer’s instructions for signal intensity, results for each of the above autoantibodies were either: negative (signal <6), borderline (6–10), medium (11–25), or strong (>25) positive. Borderline results were classified as negative.
Imaging Evaluation
MRI studies of the CNS were performed in all enrolled patients (in 68.1% of patients within 30 days from clinical evaluation; for the rest, the median timespan was 3 months). Magnetic fields of 1.5 or 3 T, including at least T1-weighted, T2-weighted, fluid-attenuated inversion recovery (FLAIR), and T1 post-gadolinium sequences for brain studies were implemented. Based on clinical criteria, at least one spinal cord (cervical or thoracic) MRI study was performed in the majority of patients, including at least short-tau inversion recovery (STIR), T2-weighted and T1-weighted pre- and post-gadolinium sequences. MRI data were analyzed blindly to both the clinical and laboratory features of the patients. White matter lesions were classified according to CNS localization and imaging characteristics (Filippi et al., 2016; Filippi et al., 2019). More specifically, classification according to localization was based on lesion distribution in periventricular, juxtacortical/cortical/deep white matter (essentially all non-periventricular, supratentorial lesions), infratentorial, corpus callosum, optic nerve, and spinal cord (cervical and/or thoracic) areas. On the basis of the imaging characteristics, the presence of gadolinium enhancement and characteristic morphology (size>3mm, ovoid shape, long axis vertical orientation toward the corpus callosum/ventricles) points toward lesions “typical” for MS (Filippi et al., 2016; Filippi et al., 2019; Solomon et al., 2019). Tumefactive and Balo lesions were also considered typical MS lesions. Nonspecific WMH and small vessel disease were classified as atypical for MS radiographic lesions (Supplementary Table S1).
Final diagnosis
Upon thorough clinical and laboratory evaluation at 1 year of follow-up patients received a final diagnosis, as follows:
a. MS spectrum disorders [RRMS, primary progressive MS (PPMS), secondary progressive MS (SPMS), clinically isolated syndromes (CIS), radiologically isolated syndromes (RIS), and tumefactive MS and MOGAD](Thompson et al., 2018a).
b. CNS autoimmune group, including patients with clinical and/or laboratory features suggestive of a systemic autoimmune disease either fulfilling previously published classification criteria (SAD with CNS involvement) (Miyakis et al., 2006; Shiboski et al., 2017; Antunes et al., 2018; Aringer et al., 2019a; Aringer et al., 2019b) or not [demyelinating disease with autoimmune features (DAF)], as previously suggested (Nikolopoulos et al., 2021).
c. Non autoimmune small vessel disease (MRI WMH of presumed vascular origin, not typical for MS, in the absence of clinical and serological systemic autoimmune features) (Wardlaw et al., 2019).
d. Other (no evidence of MS, CNS autoimmune disorder, or small vessel disease).
Quantitation of peripheral blood type I IFN score
Total RNA was extracted from freshly isolated, available, whole peripheral blood of 144 patients by TRIzol Reagent (ThermoFisher Scientific, United States). The quantity and quality of RNA samples were spectrophotometrically tested (Biospec Nano, Japan). One microgram of total RNA was reverse-transcribed into cDNA with PrimeScript™ RT Reagent Kit (Perfect Real Time) (TAKARA, Japan). Samples were 1:10 diluted in nuclease-free water and stored at −20°C. Quantitative real-time polymerase chain reaction (qRT-PCR) was performed in order to quantify mRNA expression of the following genes, preferentially induced by type I IFN: myxovirus (influenza virus) resistance 1 (MX-1), IFN-induced protein with tetratricopeptide repeats 1 (IFIT-1), and IFN-induced protein 44 (IFI44). As an internal control and normalization gene, glyceraldehyde phosphate dehydrogenase (GAPDH) was used. All reactions were performed in duplicate. A reference sample was included in each PCR plate, to ensure normalization across experiments. Peripheral blood type I IFN score was calculated as previously described (Kirou et al., 2004; Nezos et al., 2015). In detail, mean and standard deviation (SD) levels of each IFN inducible gene (IFIG) in 15 healthy controls (HC) consistently used as standards in our lab were used to standardize expression levels of each gene for each study subject using the following formula (RE IFIG subject—Mean HC)/SD HC. The standardized expression levels were subsequently summed for each patient to provide a type I IFN score as the sum of each study subject’s relative expression for each of the three genes preferentially induced by type I IFN. According to a cut-off value defined as the mean plus three SD of the corresponding IFN scores in HC (8.50), patients were further divided into high and low IFN groups.
Statistical Analysis
Continuous and categorical variables were assessed by Mann–Whitney and chi-square tests, respectively. Continuous variables are presented as mean ± SD or median and interquartile range (IQR). Statistical analysis was performed using the IBM® SPSS® software platform and GraphPad Prism® software. Differences were considered significant when p-value was <0.05.
Results
Demographics, clinical, laboratory, and imaging characteristics of the study cohort
Table 1 summarizes the demographical, clinical, laboratory, and imaging characteristics of the study cohort. Among 193 patients included in the final study sample, 139 (72.0%) were females and their age was (mean ± SD): 40.2 ± 12.7 years, with sensory, pyramidal tracts, and optic nerve being the main CNS affected areas (35.5%, 26.9%, and 23.1%, respectively). According to MRI, demyelinating brain or spine lesions were detected in 68.6% of patients, while 26.2% had nonspecific (nontypical for MS) WMH. In the entire study cohort, the prevalence of type 2 OCBs in CSF was 49.7%, with the corresponding figure being 2.5% for both 3 and 4 types. Half of the patients with type 3 OCBs were eventually diagnosed with MS spectrum disorders with the other half being classified in the CNS autoimmune group (Thompson and Freedman, 2006; Tumani et al., 2020). Furthermore, 41.3% of study participants had a CSF IgG Index ≥0.65. As for their immune profile, 8.5% of the patients enrolled had ANA titers ≥1/320 and 17.0% were positive for antiphospholipid antibodies. Antibodies against MOG and AQP4 were detected in 2.3% and 1.6% of those tested, respectively.
Final diagnosis at 1 year of follow-up
As shown in Table 2, after 1 year of follow-up, 66.3% (n = 128) of all study participants were diagnosed with MS spectrum disorders, with RRMS being the most prevalent (43.0%). Furthermore, 24.9% (n = 48) had evidence of CNS involvement in a background of systemic autoimmunity (CNS autoimmune group) and they were further divided into those fulfilling SAD classification criteria (6.7%, n = 13) and those classified as DAF (18.1%, n = 35). The SAD diagnoses were: SLE: 2.1% (n = 4), SS: 3.6% (n = 7), and APS: 0.5% (n = 1) and undifferentiated connective tissue disease (UCTD): 0.5% (n = 1). Of interest, one patient with SS also had anti-AQP4 antibodies. Finally, 14 out of 193 patients (7.3%) were classified as non-autoimmune small vessel disease, while 1.6% (n = 3) had no evidence of MS, CNS autoimmune disease, or small vessel disease and were classified as “other.”
Comparative analysis of demographical, clinical, laboratory, and imaging characteristics between MS spectrum and CNS autoimmune groups
A comparative analysis between MS spectrum and CNS autoimmune groups, regarding demographics, family history, and co-morbidities, as well as clinical, imaging, and laboratory characteristics are presented in Table 3. Compared to MS spectrum disorders, patients with autoimmune features, fulfilling or not criteria for SAD diagnosis (CNS autoimmune group), had higher rates of female sex (85.4% vs. 66.4%, p-value: 0.013), as well as of positive family history for SAD and organ-specific AD (22% vs. 5.4%, p-value: 0.002; 24.4% vs. 10.9%, p-value: 0.037, respectively). At the same time, MS spectrum patients were younger both at the time of evaluation [median age (IQR): 37.0 (16.8) vs. 46.1 (26.5), p-value: <0.001] and onset of neurological symptoms [median age (IQR): 34.8 (16.6) vs. 43.0 (20.6), p-value 0.003]. Moreover, both hypertension and hyperlipidemia were more prevalent in the CNS autoimmune group (17.1% vs. 5.3%, p-value: 0.019 and 28.6% vs. 6.1%, p-value: <0.001, respectively). In terms of first clinical manifestation, a statistically significant predominance of cortical dysfunction was observed in CNS autoimmune patients versus the MS spectrum group (8.9% vs. 0%, p-value: 0.001).
Regarding imaging findings, demyelinating brain or spine lesions characterized predominantly the MS spectrum patients (85.7% vs. 63.9%, p-value: 0.003), whereas atypical for MS brain lesions (nonspecific WMH) prevail in the CNS autoimmune group (44.7% vs. 10.2%, p-value: <0.001). As for the affected CNS areas, only the infratentorial and corpus callosum lesions were significantly more frequent in MS spectrum disease (58.1% vs. 37.8%, and 51.0% vs. 28.9%, respectively, with p-value: 0.02 for both comparisons). CSF analysis revealed a higher prevalence of T2 OCBs in MS spectrum compared to the CNS autoimmune group (60.7% vs. 37.1%, p-value: 0.036), with similar results for high IgG index (47.4% vs. 27.3%, p-value: 0.04). Among the blood and urine laboratory parameters, ANA titers equal to or greater than 1/320, contrary to those equal to 1/160, were significantly more prevalent in the CNS autoimmune group compared to the MS spectrum group (27.3% vs. 2.6%, p-value: 0.0001 and 18.2% vs. 8.5%, p-value: 0.08, respectively). Moreover, CNS autoimmune patients had higher rates of anti-cardiolipin IgM and anti-beta-2-glycoprotein-I (β2GPI) IgG antibodies (27.9% vs. 12.9%, p-value: 0.03 and 2.4% vs. 1.7%, p-value: 0.05, respectively). Another worth-mentioning trend was that of lower levels of B12 vitamin in MS spectrum patients [median (IQR): 368.5 (183.3) vs. 480.0 (298.5), p-value: 0.05].
The SAD and DAF groups were very similar in all of the above characteristics, with no statistically significant differences between them (data not shown). Notably, anti-Ro/SSA (Ro52 and Ro60) were the most frequently detected specific autoantibodies among SAD patients (data not shown).
Type I IFN Score
As displayed in Figure 1, peripheral blood type I IFN activity at baseline, as reflected by type I IFN score, was found to be significantly higher in patients, who were ultimately classified as SAD with CNS involvement [median (IQR): 50.18 (152.50)] compared to those within the MS spectrum group [median (IQR): 0.64 (6.75), p-value: 0.0001], DAF [median (IQR): 0.23 (4.40), p-value: 0.0001], and small vessel disease [median (IQR): 0.68 (12.56), p-value: 0.03]. No other significant differences were detected between groups.
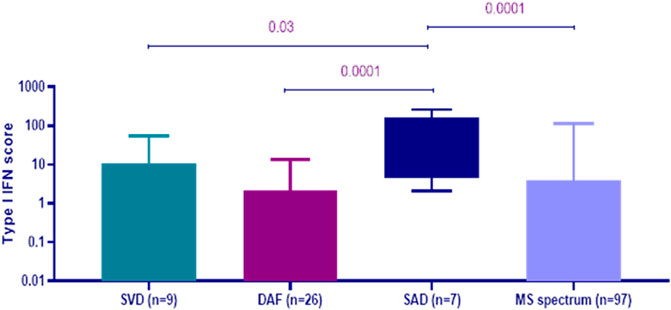
FIGURE 1. Peripheral blood type I interferon (IFN) scores at first evaluation according to the final diagnosis at 1 year of follow-up. Patients with systemic autoimmune disease (SAD) with central nervous system (CNS) involvement (n = 7) had higher type I IFN score at baseline [median (IQR): 50.18 (152.50)] compared to those with small vessel disease (SVD, n = 9) [median (IQR): 0.68 (12.56), p-value: 0.03], demyelinating disease with autoimmune features (DAF, n = 26) [median (IQR): 0.23 (4.40), p-value: 0.0001] and multiple sclerosis (MS) spectrum disorders (n = 97) [median (IQR): 0.64 (6.75), p-value: 0.0001]. Error bars represent the minimum and maximum of data distribution.
Clinical, laboratory, and imaging features in high vs. low type I IFN groups
To further explore whether type I IFN peripheral blood activity may influence demographical, clinical, imaging, and laboratory characteristics, a comparative analysis between high and low type I IFN groups was performed (Table 4).
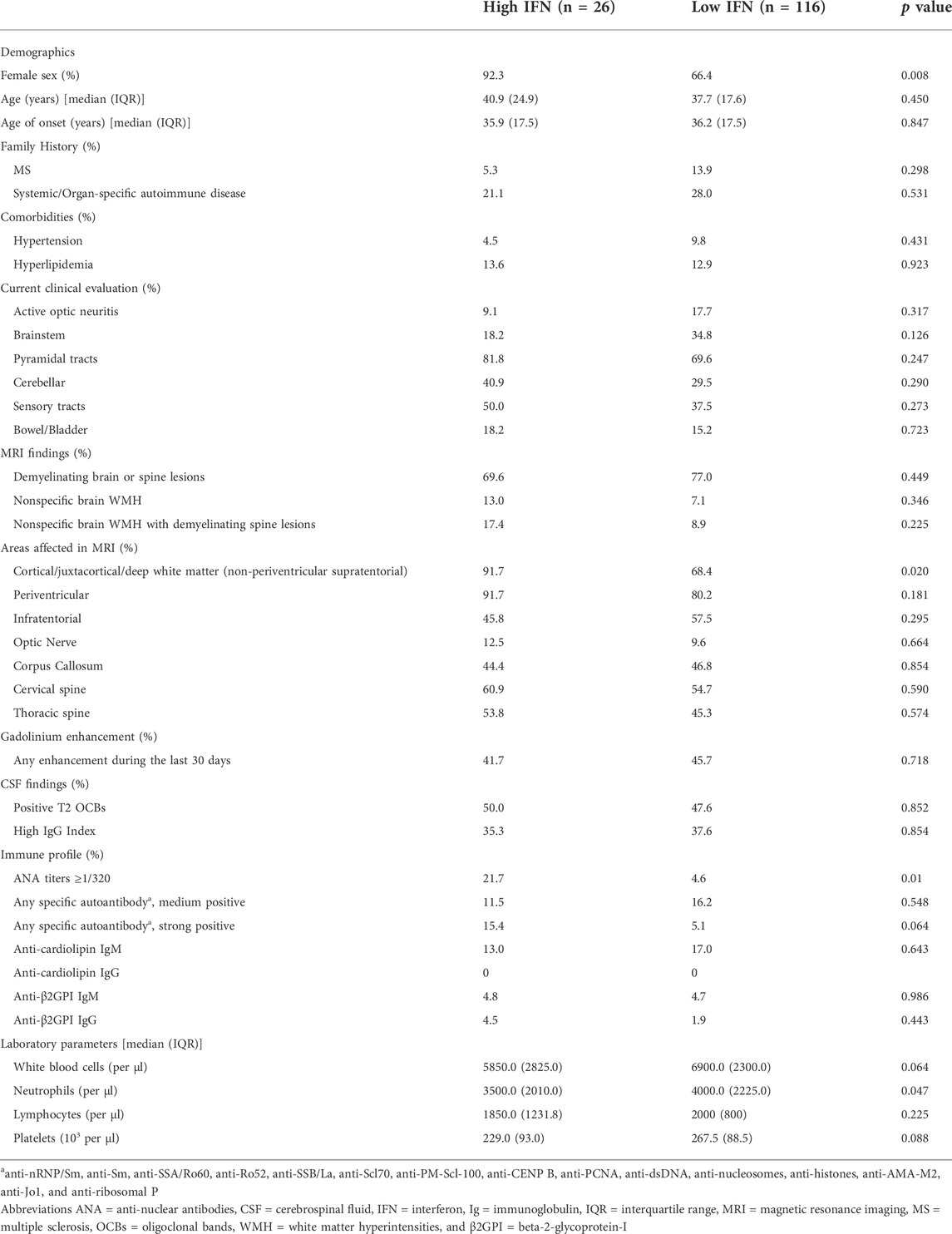
TABLE 4. Clinical, imaging and laboratory features in high vs. low type I IFN groups (See Methods for classification).
Female sex was more prevalent in 92.3% of high IFN patients versus 66.4% of low IFN subjects (p-value: 0.008). Except for cortical/juxtacortical/deep white matter (non-periventricular) lesions, which were more frequently detected in patients with high type I IFN scores (91.7% vs. 68.4%, p-value: 0.02), no other significant association was detected between any other imaging findings or neurological symptom with type I IFN scores. Moreover, high type I IFN scores were associated with ANA titers ≥1/320 (21.7% in high-IFN group vs. 4.6% in the low-IFN group, p-value: 0.01), as well as with lower neutrophil levels [median (IQR): 3500.0 (2010.0) vs. 4000.0 (2225.0) per μl, p-value: 0.047)]. Of note, three out of five patients with high IFN scores within the SAD group had positive serum titres of anti-Ro/SSA antibodies (data not shown).
Discussion
In the present study, we enrolled consecutive, undiagnosed, and treatment-naive patients with MS-like disease presentation, with the aim to explore whether distinct clinical, serological, CSF, and imaging features, as well as baseline peripheral blood type I IFN activity could aid in the formulation of a final diagnosis after 1 year of follow-up. Out of the 193 patients, included in our cohort, two-thirds were classified as MS spectrum disease and one-fourth as CNS autoimmune group. Among the latter, one-fourth met the criteria for SAD (predominantly SS and SLE), while the rest did not fulfill SAD criteria, constituting the DAF subgroup. In comparison to MS spectrum, CNS autoimmune patients displayed distinctive characteristics; they were older, more frequently females, more prone to suffer from hypertension and/or hyperlipidemia, and more likely to report a positive family history of autoimmunity. Cortical dysfunction as the first neurological presentation, atypical for MS MRI lesions, as well as less frequent T2 OCBs and IgG index positivity along with higher rates of ANA titers ≥1/320 and anti-cardiolipin IgM and anti-β2GPI IgG positivity also characterized the CNS autoimmune group. Moreover, peripheral blood type I IFN score at baseline was shown to be significantly elevated only in patients fulfilling classification criteria for SAD compared to all other groups. Of note, patients with high type I IFN scores were mainly females, and had increased rates of cortical/juxtacortical/deep white matter (non-periventricular) supratentorial lesions and ANA titers ≥1/320, together with lower neutrophil counts.
Our study suggests that underlying systemic autoimmunity is not infrequent in undiagnosed patients undergoing diagnostic evaluation for possible demyelinating CNS disease. In line with previous studies, stronger female predominance, frequent sparing of the corpus callosum, and preferential involvement of cortical/juxtacortical/deep white matter (non-periventricular) regions compared to the periventricular area have been well described in the context of neuropsychiatric SLE versus MS, with the exact opposite being true for the cerebellum, brainstem, and basal ganglia (Magro Checa et al., 2013). Moreover, hypertension and dyslipidemia were more prevalent in the CNS autoimmune group in accordance with previous comparative studies reporting a pronounced risk for cardiovascular events in lupus and rheumatoid arthritis compared to MS patients (Setyawan et al., 2021). Though lower ANA titers and antiphospholipid antibody positivity have been extensively reported in MS and MS-like populations (Spadaro et al., 1999; Szmyrka-Kaczmarek et al., 2012; Malyavantham et al., 2015; Merashli et al., 2017), in the current report, we emphasize the role of higher ANA titers (more than 1/320) pointing toward an underlying systemic autoimmune process, in support of previous findings (Magro Checa et al., 2013). Moreover, in agreement with previous works, a higher CSF IgG index and the presence of OCBs reinforce the diagnosis of MS without however ruling out a diagnosis of SAD (Magro Checa et al., 2013; Govoni et al., 2016; Venhoff et al., 2019). Of interest, MS spectrum patients displayed lower B12 vitamin levels in comparison to their CNS autoimmune counterparts, possibly due to malabsorption mediated by autoimmune reactivity toward gastrointestinal antigens commonly found in MS populations (Banati et al., 2013).
Of note, lupus and primary SS were the prevailing SAD diagnoses detected in our cohort, compatible with previous observations (Delalande et al., 2004; Bertsias and Boumpas, 2010; Massara et al., 2010; Unterman et al., 2011; Borowoy et al., 2012; Schwartz et al., 2019) and anti-Ro/SSA were the predominant reactivity among patients with SAD. This is in accord with previous studies on lupus and primary SS also highlighting the role of anti-Ro/SSA as a marker of neuropsychiatric involvement (Alexander et al., 1994; Mikdashi and Handwerger, 2004; Morreale et al., 2014; Fan et al., 2021; Tetsuka et al., 2021) and disease activity in the setting of NMOSD (Lin et al., 2022).
It is also intriguing that peripheral blood type I IFN activity, consistently found to be associated with anti-Ro/SSA antibodies in both lupus and SS patients (Kirou et al., 2005; Weckerle et al., 2011; Mathian et al., 2019), was a strong predictor for SAD diagnosis among patients with presentation suspicious of CNS demyelinating disease included in the current cohort. This is consistent with the work by Yusof et al. suggesting that an IFN score based on quantitation of type I and II IFN inducible gene expression in peripheral blood along with the family history of autoimmunity was able to predict the conversion to SAD in ANA-positive individuals (El-Sherbiny et al., 2018; Md Yusof et al., 2018).
Furthermore, the demyelinating disease with autoimmune features (DAF) group, displaying a low type I IFN score in peripheral blood, may represent a distinct clinical entity deserving close follow-up and tailored diagnostic and therapeutic approaches (Mavragani et al., 2007; Nikolopoulos et al., 2021). Interestingly, a recent study, described that almost half of the patients presenting with demyelinating lesions and coexisting clinical and/or serological evidence of autoimmunity, not fulfilling criteria for either MS or SAD at baseline, will also continue to lack a well-defined diagnosis after a median follow-up period of 3 years (Nikolopoulos et al., 2021). As previously reported, specific autoantibodies such as anti-Ro/SSA can be present years prior to disease diagnosis (Arbuckle et al., 2003), while the emergence of a well-defined autoimmune disease is in parallel with an elevation of type I IFN activity in peripheral blood (Munroe et al., 2016). Therefore, the possibility of DAF switching to SAD in the future is thought to be substantial.
While nonspecific brain WMH, as well as hypertension and dyslipidemia, were significantly higher in the CNS autoimmune group with respect to MS, none of these characteristics could differentiate patients with high versus low IFN activity. Although WMH is the most frequently observed MRI lesion in patients with systemic autoimmune diseases and suspected CNS involvement (Magro Checa et al., 2013; Magro-Checa et al., 2018), our CNS autoimmune group mainly includes the MS-like disease patients with autoimmune features (DAF group), in which type I IFN was not upregulated in contrast to individuals meeting classification criteria for SAD. Since IFN signature surfaces in parallel with clinically overt disease (Munroe et al., 2016), one could hypothesize that WMH represents an early sign of systemic autoimmunity prior to type I IFN pathway activation and full-blown disease manifestation.
Though activation of type I IFN pathway has been postulated as a major effector mechanism for several clinical manifestations in patients with lupus and SS (Mavragani, 2017; Postal et al., 2020), there is limited data supporting a role in the pathogenesis of neuropsychiatric manifestations in both serum (Fragoso-Loyo et al., 2012) and gene expression studies (Baechler et al., 2003). Of interest, variations of three prime repair exonuclease 1 (TREX1) genes–an exonuclease involved in the clearance of endogenous nucleic acids (Stetson et al., 2008) have been previously detected in European SLE cases with neurological involvement (Namjou et al., 2011). Mutations of the TREX1 gene are a hallmark of Aicardi–Goutières syndrome (AGS) (Crow et al., 2015), characterized by a lupus reminiscent picture with high IFN-α in peripheral blood and CSF, white matter lesions, autoantibody positivity and cytopenia (Ramantani et al., 2010; Crow and Manel, 2015; Cuadrado et al., 2015; Cattalini et al., 2016). Besides, previous studies in SS and lupus patients, as well as in TREX1-deficient human neural cells revealed a role of L1 (long interspersed nuclear element-1) retroelements as major triggers of type I IFN production in both target tissues (Mavragani et al., 2018) and astrocytes, respectively (Thomas et al., 2017). Moreover, ubiquitin-specific peptidase 18 (USP18) deletion in young adult mouse models [normally downregulating signal transducer and activator of transcription 1 (STAT1) signaling], led to increased activation of downstream type I IFN signaling in white matter microglial cells causing microgliopathy (Goldmann et al., 2015). Of interest, abrogation of type I IFN signaling in the Rnaset2−/− mice characterized by AGS-resembling leukoencephalopathy syndrome, led to complete resolution of CNS features (Kettwig et al., 2021).
In line with previous reports, associations of type I IFN signature with female sex (Panchanathan et al., 2010; Gabriele et al., 2021), neutropenia (Hall et al., 2015; Mathian et al., 2019; Chasset et al., 2020) or high ANA titers (Wither et al., 2017) were detected. Moreover, the link between non-periventricular lesions and high IFN scores might reflect the presence of an immune-mediated vascular injury as the underlying potential mechanism, as formerly suggested (Skeoch and Bruce, 2015; Spinelli et al., 2018; Filippi et al., 2019; Wardlaw et al., 2019; Sereti et al., 2020). In support of these findings, it has been previously shown that type I IFN inhibition in SLE patients blocked NET formation and restored cholesterol efflux capacity impairment, already shown to contribute to lupus-related atherosclerotic disease (Giannelou and Mavragani, 2017; Casey et al., 2021; O'Neil et al., 2019; Carlucci et al., 2018). Of note, among MS patients, IFN-β therapy was associated with dysregulated metabolic profiles and higher blood pressure (Sternberg et al., 2014).
The main strength of our study was the large number and extensive evaluation of undiagnosed, treatment-naïve individuals, presenting with an episode described as potentially demyelinating. As for limitations, quantitation of type I IFN score was performed in a subset of recruited patients and the final number of SAD patients was rather small to draw definite conclusions. Moreover, our patients were not tested for lupus anticoagulant (LAC); however, the initial aPTT measurement did not reveal time prolongation in any case (Tripodi and Chantarangkul, 2017).
Taken together, the identification of a prominent type I IFN signature among SAD patients with evidence of CNS involvement as the cardinal manifestation might imply a potentially significant therapeutic role of type I IFN receptor blockade, already licensed for the treatment of lupus (Morand et al., 2020; Deeks, 2021). Conversely, treatment with IFN-β in patients with a systemic autoimmune background might lead to disease exacerbation and therefore alternative therapeutic strategies such as B cell depletion should be considered (Evangelopoulos et al., 2017; Sabatino et al., 2019).
In conclusion, our study indicates that underlying systemic autoimmunity is a considerable possibility in patients undergoing evaluation for possible demyelinating CNS disease and distinct clinical, imaging and laboratory features can reliably differentiate CNS autoimmune from MS spectrum individuals in an everyday clinical practice setting. Moreover, activation of type I IFN pathway seems to be a key mediator among SAD patients presenting with MS-like disease and therefore a potential therapeutic target. Finally, a close follow-up is recommended for CNS autoimmune patients not yet fulfilling well-defined SAD classification criteria, given the heightened risk for future development of full-blown systemic autoimmune disease. Thorough studies exploring the molecular pathways governing the generation of diverse aspects of multifaceted CNS demyelinating disease would allow the design and institution of tailored and most appropriate therapeutic strategies.
Data availability statement
The original contributions presented in the study are included in the article/Supplementary Materials, further inquiries can be directed to the corresponding author.
Ethics statement
The studies involving human participants were reviewed and approved by Aeginition Hospital Ethics Committee. The patients/participants provided their written informed consent to participate in this study.
Author contributions
DKK and AR collected the data and contributed to the design of the study, the analysis and interpretation of the data, and the writing of the initial draft. AN and CS contributed to the experimental procedures, analysis, and interpretation of the data. CK contributed to clinical data collection and interpretation. MME and CPM contributed to the study conception, design of the study, collection, analysis, and data interpretation, and the revision of the manuscript. All authors approved the final manuscript version.
Funding
The study was supported by funds from the Department of Physiology, Applied and Molecular Physiology Unit, Medical School of Athens, National and Kapodistrian University of Athens, Athens, Greece.
Acknowledgments
The authors would like to thank HM Moutsopoulos for the astute comments and fruitful suggestions.
Conflict of interest
The authors declare that the research was conducted in the absence of any commercial or financial relationships that could be construed as a potential conflict of interest.
Publisher’s note
All claims expressed in this article are solely those of the authors and do not necessarily represent those of their affiliated organizations, or those of the publisher, the editors, and the reviewers. Any product that may be evaluated in this article, or claim that may be made by its manufacturer, is not guaranteed or endorsed by the publisher.
Supplementary material
The Supplementary Material for this article can be found online at: https://www.frontiersin.org/articles/10.3389/fphar.2022.898049/full#supplementary-material
References
Airo, P., Scarsi, M., Rossi, M., and Mondini, M. (2008). Onset and enhancement of systemic sclerosis after treatments for multiple sclerosis. Rheumatol. Int. 28, 703–707. doi:10.1007/s00296-007-0507-2
Akasbi, M., Berenguer, J., Saiz, A., Brito-Zerón, P., Pérez-de-Lis, M., Bové, A., et al. (2012). White matter abnormalities in primary Sjögren syndrome. Qjm 105, 433–443. doi:10.1093/qjmed/hcr218
Alexander, E. L., Ranzenbach, M. R., Kumar, A. J., Kozachuk, W. E., Rosenbaum, A. E., Patronas, N., et al. (1994). Anti-Ro(SS-A) autoantibodies in central nervous system disease associated with sjögren's syndrome (CNS-SS): Clinical, neuroimaging, and angiographic correlates. Neurology 44, 899–908. doi:10.1212/wnl.44.5.899
Andersson, M., Alvarez-Cermeño, J., Bernardi, G., Cogato, I., Fredman, P., Frederiksen, J., et al. (1994). Cerebrospinal fluid in the diagnosis of multiple sclerosis: A consensus report. J. Neurol. Neurosurg. Psychiatry 57, 897–902. doi:10.1136/jnnp.57.8.897
Antunes, M., Scirè, C. A., Talarico, R., Alexander, T., Avcin, T., Belocchi, C., et al. (2018). Undifferentiated connective tissue disease: State of the art on clinical practice guidelines. RMD Open 4, e000786. doi:10.1136/rmdopen-2018-000786
Arbuckle, M. R., Mcclain, M. T., Rubertone, M. V., Scofield, R. H., Dennis, G. J., James, J. A., et al. (2003). Development of autoantibodies before the clinical onset of systemic lupus erythematosus. N. Engl. J. Med. 349, 1526–1533. doi:10.1056/NEJMoa021933
Aringer, M., Costenbader, K., Daikh, D., Brinks, R., Mosca, M., Ramsey-Goldman, R., et al. (2019b). 2019 European League against Rheumatism/American College of Rheumatology classification criteria for systemic lupus erythematosus. Ann. Rheum. Dis. 78, 1151–1159. doi:10.1136/annrheumdis-2018-214819
Author anonymous (1993). Interferon beta-lb is effective in relapsing-remitting multiple sclerosis. I. Clinical results of a multicenter, randomized, double-blind, placebo-controlled trial. Neurology 57, S3–S9. [classical article].
Author anonymous (2013). World Medical Association Declaration of Helsinki: ethical principles for medical research involving human subjects. Jama 310, 2191–2194.
Baechler, E. C., Batliwalla, F. M., Karypis, G., Gaffney, P. M., Ortmann, W. A., Espe, K. J., et al. (2003). Interferon-inducible gene expression signature in peripheral blood cells of patients with severe lupus. Proc. Natl. Acad. Sci. U. S. A. 100, 2610–2615. doi:10.1073/pnas.0337679100
Bahri, D. M., Khiari, H., Essouri, A., Laadhar, L., Zaraa, I., Mrabet, A., et al. (2012). Systemic lupus erythematosus induced by interferon β1 therapy in a patient with multiple sclerosis. Fundam. Clin. Pharmacol. 26, 210–211. doi:10.1111/j.1472-8206.2011.00929.x
Banati, M., Csecsei, P., Koszegi, E., Nielsen, H. H., Suto, G., Bors, L., et al. (2013). Antibody response against gastrointestinal antigens in demyelinating diseases of the central nervous system. Eur. J. Neurol. 20, 1492–1495. doi:10.1111/ene.12072
Bertsias, G. K., and Boumpas, D. T. (2010). Pathogenesis, diagnosis and management of neuropsychiatric SLE manifestations. Nat. Rev. Rheumatol. 6, 358–367. doi:10.1038/nrrheum.2010.62
Borg, F. A., and Isenberg, D. A. (2007). Syndromes and complications of interferon therapy. Curr. Opin. Rheumatol. 19, 61–66. doi:10.1097/BOR.0b013e328010c547
Borowoy, A. M., Pope, J. E., Silverman, E., Fortin, P. R., Pineau, C., Smith, C. D., et al. (2012). Neuropsychiatric lupus: The prevalence and autoantibody associations depend on the definition: Results from the 1000 faces of lupus cohort. Semin. Arthritis Rheum. 42, 179–185. doi:10.1016/j.semarthrit.2012.03.011
Brownlee, W. J., Hardy, T. A., Fazekas, F., and Miller, D. H. (2017). Diagnosis of multiple sclerosis: Progress and challenges. Lancet 389, 1336–1346. doi:10.1016/S0140-6736(16)30959-X
Carlucci, P. M., Purmalek, M. M., Dey, A. K., Temesgen-Oyelakin, Y., Sakhardande, S., Joshi, A. A., et al. (2018). Neutrophil subsets and their gene signature associate with vascular inflammation and coronary atherosclerosis in lupus. JCI Insight 3, 99276. doi:10.1172/jci.insight.99276
Casey, K. A., Smith, M. A., Sinibaldi, D., Seto, N. L., Playford, M. P., Wang, X., et al. (2021). Modulation of cardiometabolic disease markers by type I interferon inhibition in systemic lupus erythematosus. Arthritis Rheumatol. 73, 459–471. doi:10.1002/art.41518
Cattalini, M., Galli, J., Andreoli, L., Olivieri, I., Ariaudo, G., Fredi, M., et al. (2016). Exploring autoimmunity in a cohort of children with genetically confirmed aicardi-goutières syndrome. J. Clin. Immunol. 36, 693–699. doi:10.1007/s10875-016-0325-y
Chasset, F., Ribi, C., Trendelenburg, M., Huynh-Do, U., Roux-Lombard, P., Courvoisier, D. S., et al. (2020). Identification of highly active systemic lupus erythematosus by combined type I interferon and neutrophil gene scores vs classical serologic markers. Rheumatol. Oxf. 59, 3468–3478. doi:10.1093/rheumatology/keaa167
Comabella, M., Lunemann, J. D., Rio, J., Sanchez, A., Lopez, C., Julia, E., et al. (2009). A type I interferon signature in monocytes is associated with poor response to interferon-beta in multiple sclerosis. Brain 132, 3353–3365. doi:10.1093/brain/awp228
Crow, Y. J., Chase, D. S., Lowenstein Schmidt, J., Szynkiewicz, M., Forte, G. M., Gornall, H. L., et al. (2015). Characterization of human disease phenotypes associated with mutations in TREX1, RNASEH2A, RNASEH2B, RNASEH2C, SAMHD1, ADAR, and IFIH1. Am. J. Med. Genet. A 167a, 296–312. doi:10.1002/ajmg.a.36887
Crow, Y. J., and Manel, N. (2015). Aicardi-Goutières syndrome and the type I interferonopathies. Nat. Rev. Immunol. 15, 429–440. doi:10.1038/nri3850
Cuadrado, E., Vanderver, A., Brown, K. J., Sandza, A., Takanohashi, A., Jansen, M. H., et al. (2015). Aicardi-Goutières syndrome harbours abundant systemic and brain-reactive autoantibodies. Ann. Rheum. Dis. 74, 1931–1939. doi:10.1136/annrheumdis-2014-205396
Deeks, E. D. (2021). Anifrolumab: First approval. Drugs 81, 1795–1802. doi:10.1007/s40265-021-01604-z
Delalande, S., de Seze, J., Fauchais, A. L., Hachulla, E., Stojkovic, T., Ferriby, D., et al. (2004). Neurologic manifestations in primary sjogren syndrome: A study of 82 patients. Med. Baltim. 83, 280–291. doi:10.1097/01.md.0000141099.53742.16
el-sherbiny, Y. M., Psarras, A., Md Yusof, M. Y., Hensor, E. M. A., Tooze, R., Doody, G., et al. (2018). A novel two-score system for interferon status segregates autoimmune diseases and correlates with clinical features. Sci. Rep. 8, 5793. doi:10.1038/s41598-018-24198-1
Evangelopoulos, M. E., Andreadou, E., Koutsis, G., Koutoulidis, V., Anagnostouli, M., Katsika, P., et al. (2017). Treatment of neuromyelitis optica and neuromyelitis optica spectrum disorders with rituximab using a maintenance treatment regimen and close CD19 B cell monitoring. A six-year follow-up. J. Neurol. Sci. 372, 92–96. doi:10.1016/j.jns.2016.11.016
Fan, G., Dai, F., Chen, S., Sun, Y., Qian, H., Yang, G., et al. (2021). Neurological involvement in patients with primary sjogren's syndrome. J. Clin. Rheumatol. 27, 50–55. doi:10.1097/RHU.0000000000001128
Feng, X., Reder, N. P., Yanamandala, M., Hill, A., Franek, B. S., Niewold, T. B., et al. (2012). Type I interferon signature is high in lupus and neuromyelitis optica but low in multiple sclerosis. J. Neurol. Sci. 313, 48–53. doi:10.1016/j.jns.2011.09.032
Ferreira, S., D'Cruz, D. P., and Hughes, G. R. (2005). Multiple sclerosis, neuropsychiatric lupus and antiphospholipid syndrome: Where do we stand? Rheumatol. Oxf. 44, 434–442. doi:10.1093/rheumatology/keh532
Filippi, M., Preziosa, P., Banwell, B. L., Barkhof, F., Ciccarelli, O., de Stefano, N., et al. (2019). Assessment of lesions on magnetic resonance imaging in multiple sclerosis: Practical guidelines. Brain 142, 1858–1875. doi:10.1093/brain/awz144
Filippi, M., Rocca, M. A., Ciccarelli, O., de Stefano, N., Evangelou, N., Kappos, L., et al. (2016). MRI criteria for the diagnosis of multiple sclerosis: MAGNIMS consensus guidelines. Lancet. Neurol. 15, 292–303. doi:10.1016/S1474-4422(15)00393-2
Fragoso-loyo, H., Atisha-Fregoso, Y., Núñez-Alvarez, C. A., Llorente, L., and Sánchez-Guerrero, J. (2012). Utility of interferon-α as a biomarker in central neuropsychiatric involvement in systemic lupus erythematosus. J. Rheumatol. 39, 504–509. doi:10.3899/jrheum.110983
Freedman, M. S., Thompson, E. J., Deisenhammer, F., Giovannoni, G., Grimsley, G., Keir, G., et al. (2005). Recommended standard of cerebrospinal fluid analysis in the diagnosis of multiple sclerosis: A consensus statement. Arch. Neurol. 62, 865–870. doi:10.1001/archneur.62.6.865
Gabriele, L., Fragale, A., Romagnoli, G., Parlato, S., Lapenta, C., Santini, S. M., et al. (2021). Type I IFN-dependent antibody response at the basis of sex dimorphism in the outcome of COVID-19. Cytokine Growth Factor Rev. 58, 66–74. doi:10.1016/j.cytogfr.2020.10.001
Giannelou, M., and Mavragani, C. P. (2017). Cardiovascular disease in systemic lupus erythematosus: A comprehensive update. J. Autoimmun. 82, 1–12. doi:10.1016/j.jaut.2017.05.008
Goldmann, T., Zeller, N., Raasch, J., Kierdorf, K., Frenzel, K., Ketscher, L., et al. (2015). USP18 lack in microglia causes destructive interferonopathy of the mouse brain. Embo J. 34, 1612–1629. doi:10.15252/embj.201490791
Govoni, M., Bortoluzzi, A., Padovan, M., Silvagni, E., Borrelli, M., Donelli, F., et al. (2016). The diagnosis and clinical management of the neuropsychiatric manifestations of lupus. J. Autoimmun. 74, 41–72. doi:10.1016/j.jaut.2016.06.013
Hall, J. C., Baer, A. N., Shah, A. A., Criswell, L. A., Shiboski, C. H., Rosen, A., et al. (2015). Molecular subsetting of interferon pathways in sjögren's syndrome. Arthritis Rheumatol. 67, 2437–2446. doi:10.1002/art.39204
Hughes, G. (2018). Hughes syndrome (antiphospholipid syndrome) and the nervous system. Lupus 27, 15–17. doi:10.1177/0961203318801671
Idborg, H., and Oke, V. (2021). Cytokines as biomarkers in systemic lupus erythematosus: Value for diagnosis and drug therapy. Int. J. Mol. Sci. 22, 11327. doi:10.3390/ijms222111327
Kettwig, M., Ternka, K., Wendland, K., Kr¼Ger, D. M., Zampar, S., Schob, C., et al. (2021). Interferon-driven brain phenotype in a mouse model of RNaseT2 deficient leukoencephalopathy. Nat. Commun. 12, 6530. doi:10.1038/s41467-021-26880-x
Kieseier, B. C. (2011). The mechanism of action of interferon-β in relapsing multiple sclerosis. CNS Drugs 25, 491–502. doi:10.2165/11591110-000000000-00000
Kirou, K. A., Lee, C., George, S., Louca, K., Papagiannis, I. G., Peterson, M. G., et al. (2004). Coordinate overexpression of interferon-alpha-induced genes in systemic lupus erythematosus. Arthritis Rheum. 50, 3958–3967. doi:10.1002/art.20798
Kirou, K. A., Lee, C., George, S., Louca, K., Peterson, M. G., Crow, M. K., et al. (2005). Activation of the interferon-alpha pathway identifies a subgroup of systemic lupus erythematosus patients with distinct serologic features and active disease. Arthritis Rheum. 52, 1491–1503. doi:10.1002/art.21031
Lin, L., Hang, H., Zhang, J., Lu, J., Chen, D., Shi, J., et al. (2022). Clinical significance of anti-SSA/Ro antibody in Neuromyelitis optica spectrum disorders. Mult. Scler. Relat. Disord. 58, 103494. doi:10.1016/j.msard.2022.103494
Magro checa, C., Cohen, D., Bollen, E. L., van Buchem, M. A., Huizinga, T. W., Steup-Beekman, G. M., et al. (2013). Demyelinating disease in SLE: Is it multiple sclerosis or lupus? Best. Pract. Res. Clin. Rheumatol. 27, 405–424. doi:10.1016/j.berh.2013.07.010
Magro-checa, C., Steup-Beekman, G. M., Huizinga, T. W., van Buchem, M. A., and Ronen, I. (2018). Laboratory and neuroimaging biomarkers in neuropsychiatric systemic lupus erythematosus: Where do we stand, where to go? Front. Med. 5, 340. doi:10.3389/fmed.2018.00340
Malyavantham, K., Weinstock-Guttman, B., Suresh, L., Zivadinov, R., Shanahan, T., Badgett, D., et al. (2015). Humoral responses to diverse autoimmune disease-associated antigens in multiple sclerosis. PLoS One 10, e0129503. doi:10.1371/journal.pone.0129503
Massara, A., Bonazza, S., Castellino, G., Caniatti, L., Trotta, F., Borrelli, M., et al. (2010). Central nervous system involvement in sjogren's syndrome: Unusual, but not unremarkable--clinical, serological characteristics and outcomes in a large cohort of Italian patients. Rheumatol. Oxf. 49, 1540–1549. doi:10.1093/rheumatology/keq111
Mathian, A., Mouries-Martin, S., Dorgham, K., Devilliers, H., Yssel, H., Garrido Castillo, L., et al. (2019). Ultrasensitive serum interferon-α quantification during SLE remission identifies patients at risk for relapse. Ann. Rheum. Dis. 78, 1669–1676. doi:10.1136/annrheumdis-2019-215571
Mavragani, C. P., Danielides, S., Zintzaras, E., Vlachoyiannopoulos, P. G., and Moutsopoulos, H. M. (2009). Antithyroid antibodies in antiphospholipid syndrome: Prevalence and clinical associations. Lupus 18, 1096–1099. doi:10.1177/0961203309106763
Mavragani, C. P. (2017). Mechanisms and new strategies for primary sjögren's syndrome. Annu. Rev. Med. 68, 331–343. doi:10.1146/annurev-med-043015-123313
Mavragani, C. P., Nezos, A., Sagalovskiy, I., Seshan, S., Kirou, K. A., Crow, M. K., et al. (2018). Defective regulation of L1 endogenous retroelements in primary Sjogren's syndrome and systemic lupus erythematosus: Role of methylating enzymes. J. Autoimmun. 88, 75–82. doi:10.1016/j.jaut.2017.10.004
Mavragani, C. P., Patronas, N., Dalakas, M., and Moutsopoulos, H. M. (2007). Ill-defined neurological syndromes with autoimmune background: A diagnostic challenge. J. Rheumatol. 34, 341–345.
Md yusof, M. Y., Psarras, A., el-Sherbiny, Y. M., Hensor, E. M. A., Dutton, K., Ul-Hassan, S., et al. (2018). Prediction of autoimmune connective tissue disease in an at-risk cohort: Prognostic value of a novel two-score system for interferon status. Ann. Rheum. Dis. 77, 1432–1439. doi:10.1136/annrheumdis-2018-213386
Merashli, M., Alves, J. D., Gentile, F., and Ames, P. R. J. (2017). Relevance of antiphospholipid antibodies in multiple sclerosis: A systematic review and meta analysis. Semin. Arthritis Rheum. 46, 810–818. doi:10.1016/j.semarthrit.2016.09.010
Mikdashi, J., and Handwerger, B. (2004). Predictors of neuropsychiatric damage in systemic lupus erythematosus: Data from the Maryland lupus cohort. Rheumatol. Oxf. 43, 1555–1560. doi:10.1093/rheumatology/keh384
Miyakis, S., Lockshin, M. D., Atsumi, T., Branch, D. W., Brey, R. L., Cervera, R., et al. (2006). International consensus statement on an update of the classification criteria for definite antiphospholipid syndrome (APS). J. Thromb. Haemost. 4, 295–306. doi:10.1111/j.1538-7836.2006.01753.x
Morand, E. F., Furie, R., Tanaka, Y., Bruce, I. N., Askanase, A. D., Richez, C., et al. (2020). Trial of anifrolumab in active systemic lupus erythematosus. N. Engl. J. Med. 382, 211–221. doi:10.1056/NEJMoa1912196
Morreale, M., Marchione, P., Giacomini, P., Pontecorvo, S., Marianetti, M., Vento, C., et al. (2014). Neurological involvement in primary sjogren syndrome: A focus on central nervous system. PLoS One 9, e84605. doi:10.1371/journal.pone.0084605
Munroe, M. E., Lu, R., Zhao, Y. D., Fife, D. A., Robertson, J. M., Guthridge, J. M., et al. (2016). Altered type II interferon precedes autoantibody accrual and elevated type I interferon activity prior to systemic lupus erythematosus classification. Ann. Rheum. Dis. 75, 2014–2021. doi:10.1136/annrheumdis-2015-208140
Namjou, B., Kothari, P. H., Kelly, J. A., Glenn, S. B., Ojwang, J. O., Adler, A., et al. (2011). Evaluation of the TREX1 gene in a large multi-ancestral lupus cohort. Genes. Immun. 12, 270–279. doi:10.1038/gene.2010.73
Nezos, A., Gravani, F., Tassidou, A., Kapsogeorgou, E. K., Voulgarelis, M., Koutsilieris, M., et al. (2015). Type I and II interferon signatures in Sjogren's syndrome pathogenesis: Contributions in distinct clinical phenotypes and Sjogren's related lymphomagenesis. J. Autoimmun. 63, 47–58. doi:10.1016/j.jaut.2015.07.002
Nikolopoulos, D., Kitsos, D., Papathanasiou, M., Chondrogianni, M., Theodorou, A., Garantziotis, P., et al. (2021). Demyelination with autoimmune features: A distinct clinical entity? Results from a longitudinal cohort. Rheumatol. Oxf. 60, 4166–4174. doi:10.1093/rheumatology/keaa902
O'neil, L. J., Kaplan, M. J., and Carmona-Rivera, C. (2019). The role of neutrophils and neutrophil extracellular traps in vascular damage in systemic lupus erythematosus. J. Clin. Med. 8, E1325. doi:10.3390/jcm8091325
Panchanathan, R., Shen, H., Zhang, X., Ho, S. M., and Choubey, D. (2010). Mutually positive regulatory feedback loop between interferons and estrogen receptor-alpha in mice: Implications for sex bias in autoimmunity. PLoS One 5, e10868. doi:10.1371/journal.pone.0010868
Pelidou, S. H., Giannopoulos, S., Tzavidi, S., Tsifetaki, N., Kitsos, G., Stefanou, D., et al. (2007). Neurological manifestations of connective tissue diseases mimicking multiple sclerosis. Rheumatol. Int. 28, 15–20. doi:10.1007/s00296-007-0384-8
Piga, M., Chessa, E., Peltz, M. T., Floris, A., Mathieu, A., Cauli, A., et al. (2017). Demyelinating syndrome in SLE encompasses different subtypes: Do we need new classification criteria? Pooled results from systematic literature review and monocentric cohort analysis. Autoimmun. Rev. 16, 244–252. doi:10.1016/j.autrev.2017.01.011
Postal, M., Vivaldo, J. F., Fernandez-Ruiz, R., Paredes, J. L., Appenzeller, S., Niewold, T. B., et al. (2020). Type I interferon in the pathogenesis of systemic lupus erythematosus. Curr. Opin. Immunol. 67, 87–94. doi:10.1016/j.coi.2020.10.014
Ramantani, G., Kohlhase, J., Hertzberg, C., Innes, A. M., Engel, K., Hunger, S., et al. (2010). Expanding the phenotypic spectrum of lupus erythematosus in Aicardi-Goutières syndrome. Arthritis Rheum. 62, 1469–1477. doi:10.1002/art.27367
Ronnblom, L., and Leonard, D. (2019). Interferon pathway in SLE: One key to unlocking the mystery of the disease. Lupus Sci. Med. 6, e000270. doi:10.1136/lupus-2018-000270
Sabatino, J. J., Pröbstel, A. K., and Zamvil, S. S. (2019). B cells in autoimmune and neurodegenerative central nervous system diseases. Nat. Rev. Neurosci. 20, 728–745. doi:10.1038/s41583-019-0233-2
Schwartz, N., Stock, A. D., and Putterman, C. (2019). Neuropsychiatric lupus: New mechanistic insights and future treatment directions. Nat. Rev. Rheumatol. 15, 137–152. doi:10.1038/s41584-018-0156-8
Sereti, E., Stamatelopoulos, K. S., Zakopoulos, N. A., Evangelopoulou, A., Mavragani, C. P., Evangelopoulos, M. E., et al. (2020). Hypertension: An immune related disorder? Clin. Immunol. 212, 108247. doi:10.1016/j.clim.2019.108247
Setyawan, J., Mu, F., Yarur, A., Zichlin, M. L., Yang, H., Fernan, C., et al. (2021). Risk of thromboembolic events and associated risk factors, including treatments, in patients with immune-mediated diseases. Clin. Ther. 43, 1392–1407.e1. doi:10.1016/j.clinthera.2021.06.008
Shiboski, C. H., Shiboski, S. C., Seror, R., Criswell, L. A., Labetoulle, M., Lietman, T. M., et al. (2017). 2016 American college of Rheumatology/European league against rheumatism classification criteria for primary sjogren's syndrome: A consensus and data-driven methodology involving three international patient cohorts. Ann. Rheum. Dis. 76, 9–16. doi:10.1136/annrheumdis-2016-210571
Skeoch, S., and Bruce, I. N. (2015). Atherosclerosis in rheumatoid arthritis: Is it all about inflammation? Nat. Rev. Rheumatol. 11, 390–400. doi:10.1038/nrrheum.2015.40
Solomon, A. J., Naismith, R. T., and Cross, A. H. (2019). Misdiagnosis of multiple sclerosis: Impact of the 2017 McDonald criteria on clinical practice. Neurology 92, 26–33. doi:10.1212/WNL.0000000000006583
Spadaro, M., Amendolea, M. A., Mazzucconi, M. G., Fantozzi, R., di Lello, R., Zangari, P., et al. (1999). Autoimmunity in multiple sclerosis: Study of a wide spectrum of autoantibodies. Mult. Scler. 5, 121–125. doi:10.1177/135245859900500209
Spinelli, F. R., Barone, F., Cacciapaglia, F., Pecani, A., and Piga, M. (2018). Atherosclerosis and autoimmunity. Mediat. Inflamm. 2018, 6730421. doi:10.1155/2018/6730421
Sternberg, Z., Leung, C., Sternberg, D., Yu, J., and Hojnacki, D. (2014). Disease modifying therapies modulate cardiovascular risk factors in patients with multiple sclerosis. Cardiovasc. Ther. 32, 33–39. doi:10.1111/1755-5922.12049
Stetson, D. B., Ko, J. S., Heidmann, T., and Medzhitov, R. (2008). Trex1 prevents cell-intrinsic initiation of autoimmunity. Cell. 134, 587–598. doi:10.1016/j.cell.2008.06.032
Stosic, M., Ambrus, J., Garg, N., Weinstock-Guttman, B., Ramanathan, M., Kalman, B., et al. (2010). MRI characteristics of patients with antiphospholipid syndrome and multiple sclerosis. J. Neurol. 257, 63–71. doi:10.1007/s00415-009-5264-6
Szmyrka-kaczmarek, M., Pokryszko-Dragan, A., Pawlik, B., Gruszka, E., Korman, L., Podemski, R., et al. (2012). Antinuclear and antiphospholipid antibodies in patients with multiple sclerosis. Lupus 21, 412–420. doi:10.1177/0961203311427550
Tetsuka, S., Suzuki, T., Ogawa, T., Hashimoto, R., and Kato, H. (2021). Anti-Ro/SSA antibodies may Be responsible for cerebellar degeneration in sjogren's syndrome. J. Clin. Med. Res. 13, 113–120. doi:10.14740/jocmr4429
Theodoridou, A., and Settas, L. (2008). Demyelination in rheumatic diseases. Postgrad. Med. J. 84, 127–132. doi:10.1136/jnnp.2005.075861
Thomas, C. A., Tejwani, L., Trujillo, C. A., Negraes, P. D., Herai, R. H., Mesci, P., et al. (2017). Modeling of TREX1-dependent autoimmune disease using human stem cells highlights L1 accumulation as a source of neuroinflammation. Cell. Stem Cell. 21, 319–331. e8. doi:10.1016/j.stem.2017.07.009
Thompson, A. J., Banwell, B. L., Barkhof, F., Carroll, W. M., Coetzee, T., Comi, G., et al. (2018a). Diagnosis of multiple sclerosis: 2017 revisions of the McDonald criteria. Lancet. Neurol. 17, 162–173. doi:10.1016/S1474-4422(17)30470-2
Thompson, A. J., Baranzini, S. E., Geurts, J., Hemmer, B., and Ciccarelli, O. (2018b). Multiple sclerosis. Lancet 391, 1622–1636. doi:10.1016/S0140-6736(18)30481-1
Thompson, E. J., and Freedman, M. S. (2006). Cerebrospinal fluid analysis in the diagnosis of multiple sclerosis. Adv. Neurol. 98, 147–160.
Tripodi, A., and Chantarangkul, V. (2017). Lupus anticoagulant testing: Activated partial thromboplastin time (APTT) and silica clotting time (SCT). Methods Mol. Biol. 1646, 177–183. doi:10.1007/978-1-4939-7196-1_15
Tsokos, G. C., Lo, M. S., Costa Reis, P., and Sullivan, K. E. (2016). New insights into the immunopathogenesis of systemic lupus erythematosus. Nat. Rev. Rheumatol. 12, 716–730. doi:10.1038/nrrheum.2016.186
Tumani, H., Petereit, H. F., Gerritzen, A., Gross, C. C., Huss, A., Isenmann, S., et al. (2020). S1 guidelines "lumbar puncture and cerebrospinal fluid analysis" (abridged and translated version). Neurol. Res. Pract. 2, 8. doi:10.1186/s42466-020-0051-z
Unterman, A., Nolte, J. E., Boaz, M., Abady, M., Shoenfeld, Y., Zandman-Goddard, G., et al. (2011). Neuropsychiatric syndromes in systemic lupus erythematosus: A meta-analysis. Semin. Arthritis Rheum. 41, 1–11. doi:10.1016/j.semarthrit.2010.08.001
Van baarsen, L. G., van der Pouw, K. R. A. A. N. T. C., Kragt, J. J., Baggen, J. M., Rustenburg, F., et al. (2006). A subtype of multiple sclerosis defined by an activated immune defense program. Genes. Immun. 7, 522–531. doi:10.1038/sj.gene.6364324
Venhoff, N., Thiel, J., Rizzi, M., Venhoff, A., Rauer, S., Endres, D., et al. (2019). The MRZ-reaction and specific autoantibody detection for differentiation of ANA-positive multiple sclerosis from rheumatic diseases with cerebral involvement. Front. Immunol. 10, 514. doi:10.3389/fimmu.2019.00514
Wardlaw, J. M., Smith, C., and Dichgans, M. (2019). Small vessel disease: Mechanisms and clinical implications. Lancet. Neurol. 18, 684–696. doi:10.1016/S1474-4422(19)30079-1
Weckerle, C. E., Franek, B. S., Kelly, J. A., Kumabe, M., Mikolaitis, R. A., Green, S. L., et al. (2011). Network analysis of associations between serum interferon-α activity, autoantibodies, and clinical features in systemic lupus erythematosus. Arthritis Rheum. 63, 1044–1053. doi:10.1002/art.30187
Wither, J., Johnson, S. R., Liu, T., Noamani, B., Bonilla, D., Lisnevskaia, L., et al. (2017). Presence of an interferon signature in individuals who are anti-nuclear antibody positive lacking a systemic autoimmune rheumatic disease diagnosis. Arthritis Res. Ther. 19, 41. doi:10.1186/s13075-017-1243-y
Keywords: type I interferon score, multiple sclerosis, systemic autoimmune disease, MS-like, demyelination, neuropsychiatric lupus, sjögren’s syndrome, systemic lupus erythematosus
Citation: Karathanasis DK, Rapti A, Nezos A, Skarlis C, Kilidireas C, Mavragani CP and Evangelopoulos ME (2022) Differentiating central nervous system demyelinating disorders: The role of clinical, laboratory, imaging characteristics and peripheral blood type I interferon activity. Front. Pharmacol. 13:898049. doi: 10.3389/fphar.2022.898049
Received: 16 March 2022; Accepted: 30 June 2022;
Published: 12 August 2022.
Edited by:
Anna Lisa Giuliani, University of Ferrara, ItalyReviewed by:
Massimiliano Castellazzi, University of Ferrara, ItalyEttore Silvagni, University of Ferrara, Italy
Copyright © 2022 Karathanasis, Rapti, Nezos, Skarlis, Kilidireas, Mavragani and Evangelopoulos. This is an open-access article distributed under the terms of the Creative Commons Attribution License (CC BY). The use, distribution or reproduction in other forums is permitted, provided the original author(s) and the copyright owner(s) are credited and that the original publication in this journal is cited, in accordance with accepted academic practice. No use, distribution or reproduction is permitted which does not comply with these terms.
*Correspondence: Maria Eleftheria Evangelopoulos, ZXZhbmdlbG9wbWVAbWVkLnVvYS5ncg==
†These authors have contributed equally to this work and share first authorship
‡These authors have contributed equally to this work and share last authorship