- 1Department of Radiation Oncology, The Breast Cancer Institute, The Third Hospital of Nanchang, Nanchang, China
- 2Department of Radiation Oncology, The Fourth Affiliated Hospital of Nanchang University, Nanchang, China
Cancer has become a main public health issue globally. The conventional treatment measures for cancer include surgery, radiotherapy and chemotherapy. Among the various available treatment measures, chemotherapy is still one of the most important treatments for most cancer patients. However, chemotherapy for most cancers still faces many problems associated with a lot of adverse effects, which limit its therapeutic potency, low survival quality and discount cancer prognosis. In order to decrease these side effects and improve treatment effectiveness and patient’s compliance, more targeted treatments are needed. Sustainable and controlled deliveries of drugs with controllable toxicities are expected to address these hurdles. Chitosan is the second most abundant natural polysaccharide, which has excellent biocompatibility and notable antitumor activity. Its biodegradability, biocompatibility, biodistribution, nontoxicity and immunogenicity free have made chitosan become a widely used polymer in the pharmacology, especially in oncotherapy. Here, we make a brief review of the main achievements in chitosan and its derivatives in pharmacology with a special focus on their agents delivery applications, immunomodulation, signal pathway modulation and antitumor activity to highlight their role in cancer treatment. Despite a large number of successful studies, the commercialization of chitosan copolymers is still a big challenge. The further development of polymerization technology may satisfy the unmet medical needs.
1 Introduction
Cancer has been becoming a life-threatening disease and a major public health problem all over the world, which is one of the leading causes of death. It is reported that the probability of people with invasive cancer is about 40 and 25% in the United States and China, respectively (Chen et al., 2016; Pan et al., 2017; Cao et al., 2020; Siegel et al., 2021; Sung et al., 2021). Cancer treatment depends on the type of cancer, the stage at diagnosis and the patient’s tolerance. The available anticancer therapies include surgery, chemotherapy, radiotherapy, and immunotherapy and so on (Miller et al., 2019). Among the various available therapies, chemotherapy is still one of the most important treatment measures for most cancer patients, which is regarded as one of the main treatment approaches to prevent cancer cell proliferation. However, the active chemicals used to treat cancer usually do not distinguish cancer cells from healthy cells because both are exposed to cytotoxic chemotherapeutic drugs, which results in a high rate of severe adverse reactions and limits its therapeutic effect (Grosso and De-Paz, 2021). To improve therapeutic potency and minimize the side effects, more targeted therapies are highly needed (Perez-Herrero and Fernandez-Medarde, 2015). Under this circumstance, researchers around the world have explored a variety of new nanocarriers and targeted modification systems to overcome these shortcomings and improve the therapeutic outcomes (Ghaz-Jahanian et al., 2015; Bai et al., 2021; Shakeran et al., 2021; Dubey et al., 2022). Because nanotechnology provides a suitable means for the targeted and time-controlled delivery of drugs and other bioactive agents, it has been widely studied in drug delivery and has potential application prospects in cancer treatment (Sabra et al., 2017; George et al., 2019; Ion et al., 2021; Maspes et al., 2021; Sohrabi and Packirisamy, 2021; Li et al., 2022). Drug delivery systems (DDSs) refer to the methods of delivering drugs to the targeted tissues, organs, cells or subcellular organs through various drug carriers for controlling drug release and absorption, so as to improve the pharmacological activity, overcome the limited solubility, low bioavailability, poor biological distribution and lack of selectivity, or to minimize the adverse effects (Li et al., 2019). Polymer based DDSs may potentially improve current disease treatment because they can pass through a variety of biological barriers to overcome the shortcomings of insoluble drugs, increase half-life and suppress the side effects of toxic drugs. Among them, chitosan-based nanocarriers were probably the most interesting, flexible and bio-compatible systems (Lin et al., 2017; Zhao et al., 2017; Naskar et al., 2019; Monteiro et al., 2021).
As a natural polysaccharide, chitosan can be found in some types of seafood, such as shrimp, crab and crayfish, and the content is particularly high in their shell. The main properties of chitosan are biocompatibility, biodegradability and non-toxicity (Herdiana et al., 2021; Zhu et al., 2021; Zivarpour et al., 2021). Chitosan has a high concentration of reactive free protonable amino groups along the chitosan backbone, and due to the protonation of amino groups, it presents higher solubility in acid environment. These groups can be chemically modified in a variety of chemical reactions to enhance its solubility, biocompatibility and targeting activity (de Sousa Victor et al., 2020; Ding et al., 2020). Consequently, chitosan has the potential to use in a variety of fields (Aranaz et al., 2021). The positive charge of chitosan allowing for non-covalent interactions with biological tissues has been used in drug delivery, which may assist to overcome the inadequacy of the existing chemotherapy. Some kinds of therapeutic agents conjugated with chitin or chitosan derivatives have displayed wonderful anticancer potency with less adverse effects than the original drugs due to targeted distribution into the cancer and sustainable release (Jia et al., 2014; López-Barrera et al., 2021; Tang et al., 2021). Normally, there are two traits in cancer tissue: acidic pH and the reductive environment, which have been heavily studied as internal trigger for the drug release in smart DDSs (Friedl and Alexander, 2011).
Additionally, chitosan may accumulate in the tumor site, initiate the polarization of M1 macrophages and transform the immunosuppressive tumor microenvironment to immunosupportive state, thus exerting an antitumor effect and promoting the efficacy of cancer immunotherapy (Ding et al., 2020; Zeng et al., 2021). What’s more, chitosan can also activate innate immune responses to exert its anticancer effect (Chakrabarti et al., 2014; Li et al., 2018; Yang et al., 2019a; Wu et al., 2019; Chen et al., 2020; Van Hees et al., 2020; Liang et al., 2021; Lima et al., 2021).
Last but not least, Chitosan itself may also inhibit tumor cell growth, tumor induced angiogenesis and tumor metastasis. Consequently, chitosan and its derivatives may have high performances in cancer treatment fields. There are varieties of publications in this quite fantastic field over last decade. Herein, in this review, based on the current tumor epidemiology and treatment dilemma, we gathered the state-of-the-art publications on chitosan to show its application in anticancer drug-delivery, innate immune stimulation, cancer cell inhibition and signal modulation, providing more information about its characteristics, chemical modifications, and applications in cancer treatment. Finally, we briefly prospected the future trends and challenges of chitosan nanoparticles in cancer treatment.
2 Cancer and its Epidemiology
Cancer is not only the main cause of death, but also a vital obstacle to longevity all over the world. Based on estimates from the World Health Organization (WHO) in 2019, cancer has become the most common cause of death before the age of 70 years in most countries (Sung et al., 2021). From here we see that cancer has become a major public health issue to be solved urgently worldwide. WHO newly updates the 10 most common causes of disease burden by cause-specific disability adjusted life years (DALYs), which is shown in Figure 1.
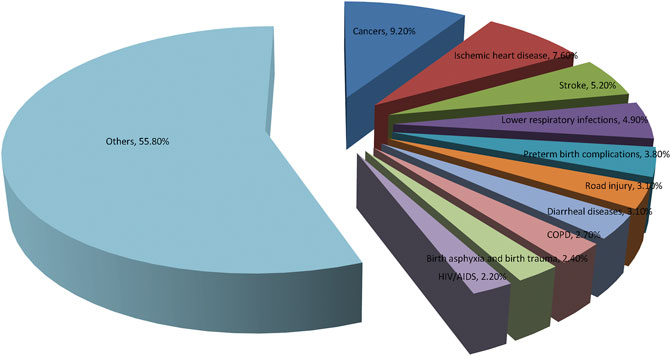
FIGURE 1. World Health Organization (WHO) Global Health Estimates 2019 on the 10 leading causes of disease burden, estimated as cause-specific Disability Adjusted Life Years (DALYs). Cancer has become the leading causes of disease burden by cause-specific disability adjusted life years.
In general, cancer has become the highest clinical, social, and economic burden among all human diseases. The economic burden associated with cancer has a profound impact on the health and non-health outcomes of cancer survivors. American Cancer Society and International Agency for Research on Cancer provide recent information on 10 most frequent types of cancers worldwide in Figure 2.
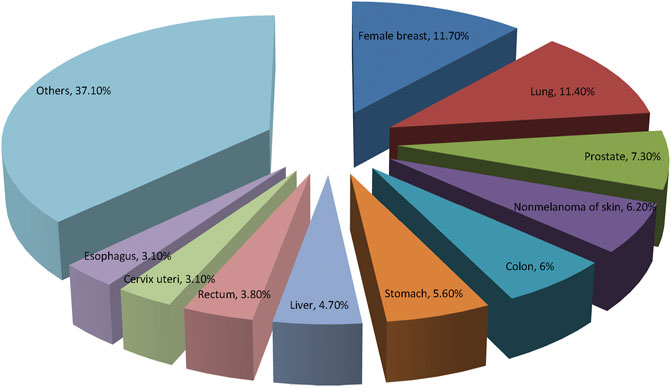
FIGURE 2. The prevalence of the top 10 most common cancers according to the GLOBOCAN 2020. Breast cancer has replaced lung cancer as the most common cancer worldwide.
GLOBOCAN 2020 has summarized the most recent changes of cancer burden all over the world. It is reported that there were nearly 20 million new cases of cancer and about 10 million deaths from cancer in 2020 globally. The top three common cancers were female breast cancer, lung cancer and prostate cancer worldwide. While, lung cancer, liver cancer and stomach cancer are the three of cancer death in general population, lung and breast cancers are most common causes of cancer related-mortality in men and women, respectively (Sung et al., 2021). Figure 3 illustrates the top 10 mortality cancer worldwide. Relatively, China has a lower cancer incidence but higher cancer mortality compared with the developed countries, such as United States and United Kingdom. The high mortality rate in China may be due to the different cancer spectrum, the low diagnosis rate of early cancer and the inconsistent clinical cancer treatment strategies implemented among different regions. Overcoming cancer has become a big global challenge.
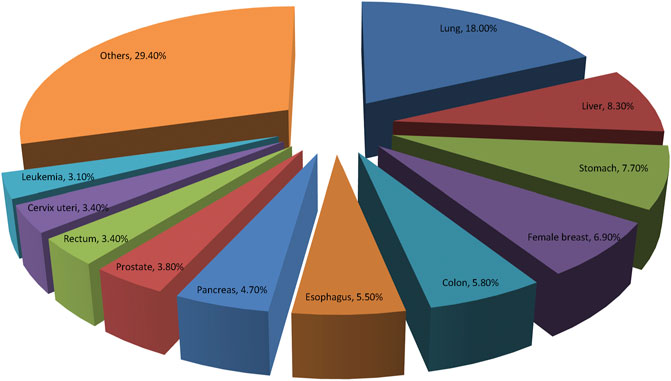
FIGURE 3. Distribution of top 10 deaths of Cancers according to the GLOBOCAN 2020. Lung cancer remains the most common cause of cancer related-mortality all over the world.
3 Cancer Treatment: Prospects and Dilemmas
Cancer is one of the most deadly and life-threatening diseases in the world, causing about 13% of deaths every year. Traditional cancer treatment strategies, such as surgery, chemotherapy, radiotherapy, hyperthermia and immunotherapy, have been developed for cancer therapy. However, these treatment measures often inevitably have their shortcomings, such as low inhibition efficiency and no targeting to cancer cells, which eventually lead to treatment failure. For example, surgical procedures may affect various pathophysiological processes, thereby promoting cancer cell proliferation and tumor recurrence. What’s more, surgical manipulation may increase the dissemination of cancer cells to the circulation and induce local or systemic immunosuppressive responses, which finally increase the chance of colonization in target organs (Narmani and Jafari, 2021). Chemotherapy is the most commonly used measure in the existing cancer treatments. However, chemotherapeutic drugs may lead to serious problems, such as detrimental side effects, drug resistance and heavy financial burden. Similarly, both chemotherapy and radiotherapy cannot differentiate cancer cells from normal healthy cells in patients, and thus both are damaged by treatment. Although significant progress has been made in cancer treatment, the therapeutic effects and overall survival for patients with cancer are still unsatisfactory, especially for patients with advanced cancer. In order to reduce the rate of adverse events, improve therapeutic efficacy and patient’s compliance, more targeted therapies are urgently needed.
Cancer immunotherapy is under the spotlight recently, which is completely different from traditional cancer treatment. It is now considered to be a potentially effective way to conquer cancer. Cancer immunotherapy regulates the immune system rather than focusing on the cancer cells (Esfahani et al., 2020; Mu et al., 2020). However, immunotherapy has encountered a number of challenges in some kinds of cancer, including resistance of immune checkpoint inhibitors, weak immunogenicity of therapeutic vaccines, serious immune related side effects, and off-target effects and so on. Chemoimmunotherapy is a combination of chemotherapy and immunotherapy, which provides synergistic effect for improving anticancer potency. It is a promising cancer treatment method, with the advantages of cooperating two kinds of treatment mechanism (Nowak and Lesterhuis, 2014). The very recent studies demonstrated that nanoparticles could remodel immunosuppressive tumor microenvironment, holding great promise for chemoimmunotherapy (Xu et al., 2022). Moreover, nanobased delivery systems have exhibited excellent properties, such as targeted drug delivery, tumor microenvironment modulation, targeted and time-controlled release of conjugate drugs, so as to improve the pharmacokinetics and stability of drugs in vivo.
In the journey of exploring new cancer treatment measures, the development of nanobased drugs is expected to become a promising method to overcome the current shortcomings of traditional chemotherapy drugs (Nazir et al., 2014). So far, many nanobased anticancer drugs have been developed and extensively studied both in vitro and in vivo, some of which have been under clinical trials in the past few decades. The antitumor effect of nanocarriers is achieved by delivering drug selectively to the tumor cells. Based on the source, drug carriers can be classified into two types: chemosynthetic and natural. Natural biopolymers such as chitosan, collagen, cellulose, and fibrin, are vastly investigated in the field of pharmacology owing to their unique characteristics. In particular, chitosan based nanoparticles have drawn considerable attention as chemotherapeutic drug delivery carriers because of their easy accessibility, terrific stability, toxicity free, and modification friendly (Zhang et al., 2019).
4 Chitosan: Source, Structure, Physicochemical Properties and Application in Oncotherapies
4.1 Source, Structures and Physicochemical Properties
4.1.1 Source of Chitosan
Chitosan, a unique alkaline polysaccharide in nature, is found in the invertebrate shelves (Farhadihosseinabadi et al., 2019). Shrimp, crabs, lobster, crayfish and oyster are the most common origins for chitosan preparation (Marsili et al., 2021). However, chitosan for industrial scale application is normally derived from chitin by a process of deacetylation. Chitin, one of the richest natural polymers, is a polymer of N-acetyl-D-glucosamine. When deacetylation is carried out, the repeating units in the chitin normally do not contain β-1,4-D-glucosamine functional groups, and this chitin is called chitosan. The mole fraction of the N-acetylated repeating units is regarded as the degree of acetylation (DA), on the contrary, the percentage of the repeating units of β-1,4-D-glucosamine in the polysaccharides is defined as the degree of deacetylation (DD). Although there are no universal agreement on the cut-off values for DD between chitin and chitosan, the DD values of most commercial chitosan are between 70 and 90% (Younes and Rinaudo, 2015; Shahbaz, 2020; Kou et al., 2021).
4.1.2 Structures of Chitosan
The molecular structure of chitosan was first resolved comprising of β-1,4-D-glucosamine and N-acetyl-D-glucosamine units in 1950 (Zhang et al., 2019). The molecular weight (MW), DD, degree and site of substitutions carry heavy weight on physiochemical properties of chitosan and its derivatives as well as the antitumor potency of chitosan-based nanoparticles. The average MW of commercially produced chitosan varies from 3,800 to more than 300,000 Da (Bhavsar et al., 2017; Adhikari and Yadav, 2018). Chitosan can be classified as low MW chitosan, medium MW, and high MW chitosan depending on the DD. Low MW chitosan has been proved to be more effective in size reduction, solubility enhancement and nanocrystal formulations’ stability, thereby being considered as a promising nanocarrier to formulate oral sustainable release drugs to improve the bioavailability (Naqvi and Moerschbacher, 2017).
Generally, the chitosan with MW up to 10 kDa is known as chitosan oligosaccharide, which obtains from degradation of chitosan, and exhibits a lot of exciting molecular weight-dependent biological traits, especially antitumor activities (Bonin et al., 2020). Therefore, extensive studies have been conducted to convert chitosan to chitosan oligosaccharides with specific molecular weight in order to find more effective nanocarriers with both economy and environment friendly properties. Tremendous efforts have been made to produce chitosan oligosaccharides in the past decades. Generally, all of these methods come from chemical or enzymatic approaches. Based on chemical approach, acidic hydrolysis has been widely applied for chitosan oligosaccharides production (Salehinik et al., 2021).
4.1.3 Physicochemical Properties of Chitosan
Chitosan is generally considered as a safe and biocompatible polymer material. Based on most studies, chitosan has no toxicity or little toxicity, so it is widely considered to be a safe biomaterial. Chitosan shows several biomedical properties, such as antimicrobial, antitumor and hemostatic activities, which is dependent on the chitosan MW and DD (Park et al., 2011). Molecular weight is a vital factor influencing the physical and chemical properties of chitosan based nanoparticles. Normally, high MW increases both the stability of chitosan-based complexes and circulation time of nanoparticles in blood stream but delays their dissociation and subsequent effect in cells, which subsequently guarantees the high tumor selectivity, while lower MW has the opposite effects.
The DD represents free amino groups in the chitosan structure which can be determined by different methods, also carries out a vital role in physical, chemical and medical properties (Rodrigues et al., 2021). It determines the positive charge density and the ability to bind with DNA/siRNA. Higher DD helps to improve the efficiency of transfection by escaping from the endolysosomal compartment. According to the percentage of DD, chitosan can be categorized into low DD of chitosan, medium DD of chitosan, high DD of chitosan and ultra-high DD of chitosan four different forms, which with DD values between 55 and 70%, 70 and 85%, 85 and 95% and between 95 and 100%. Generally, high DD will make nanoparticles with high surface charge density, thus enhancing cell uptake and antitumor effect. In traditional method, the DD of chitosan obtained is nearly 80%, while ultra-high DD chitosan is still difficult to obtain for medical application scale.
What’s more, the degree and site of substitutions grafted onto chitosan also affects the physical, biochemical traits and the antitumor potency. The abundance of deoxycholic acid and hydrophobic group affects nanoparticle size, entrapment efficiency and drug loading content significantly. The site of carboxymethylation on chitosan can influence the stability and deformability of nanoparticles, which is also strongly correlated with the antitumor activity and cellular uptake of carboxymethyl chitosan conjugates. Furthermore, the abundance of protonated-NH2 groups on the chitosan structure determines its solubility in acid medium, since its pKa value is about 6.5. However, the solubility window of chitosan can be changed by use of hydrogen bond disruptors such as urea or guanidine hydrochloride. In fact, a wide range of solubility can be achieved through the chemical or physical destruction of hydrogen bonds. Though the characteristics of chitosan and its derivatives have great influence on the biochemical effect of chitosan particles, it should be noted that these factors do not work alone, but have a comprehensive impact on the chitosan conjugates. Thus, to ensure the superior in vivo tumor targeting efficiency, solubility, stability and deformability of nanoparticles should be balanced. Selecting appropriate chitosan as nanocarriers needs to comprehensively consider the relationship between the above factors and payload properties, preparation methods of nanoparticles, targeted diseases and so on (Narayanan et al., 2014).
In addition, from a technical point of view, the viscosity of polymer is another very important parameter because high viscosity solutions are difficult to manage. Viscometry is a powerful tool for determining the molecular weight of chitosan because it is a simple and rapid method, although it is not the only method (Wang and Xu, 1994). Glycosidic bonds and acetylamine groups can also be regarded as functional groups, which allow a large number of modifications to produce polymers with new properties and behaviors. One of the most important properties of chitosan is its cationic property. Because electrostatic interaction enhances the adhesion to the negatively charged mucosal surface, chitosan plays an advantage as an ideal drug carrier, which improves the internalization of drugs into target cells (Kurakula et al., 2015). On the other hand, the cationic nature of chitosan also makes it possible to prevent anionic rich nucleic acids from degradation by nucleases in serum, improving the efficiency of gene therapy.
In order to improve the properties of chitosan or introduce new functions or properties to chitosan, many chitosan derivatives have been produced, among which hydrophobically modified ethylene glycol chitosan is one of the most commonly used derivatives for the preparation of self-assembled nanoparticles. The abundant amino and hydroxyl groups on the chitosan skeleton represent more target parts of chemical modification, which improves water solubility and endow chitosan with some new functions, such as targeted and environmentally sensitive drug release, and enhances therapeutic effect and minimize side effects. The most common chitosan derivatives used as drug carriers include thiochitosan, trimethyl chitosan, carboxymethyl chitosan, ethylene glycol chitosan and so on (Hu et al., 2020; Grosso and De-Paz, 2021; Li et al., 2021).
4.2 Applications of Chitosan in Oncotherapies
4.2.1 Chitosan-Based Delivery System
Tremendous efforts have been made to maintain long-term therapeutic levels of drug concentration in the targeted site, administration time span, and decrease side effects for disease treatment. The search for novel controlled drug release systems is closely related to the establishment of more effective treatment approaches that can be administered more safely and with few side effects. Chitosan with good biocompatibility and biodegradability is one of the most functional natural biopolymers widely used in the pharmaceutical field. The properties of chitosan make it a promising nanocarrier, which has been used to deliver variously therapeutic agents such as chemotherapeutic drugs, peptides/proteins, vaccines, DNA/siRNA, and so on (Shikhi-Abadi and Irani, 2021).
4.2.1.1 Chemotherapeutic Drugs Delivery
Various nanocarriers have been used to deliver chemotherapeutic drugs to tumor sites. These nanocarriers normally can evade the immune surveillance system, achieve target selectivity, gain access into the interior of cancerous cells, evade endosomal entrapment and release the drugs in a sustainable manner (Wang et al., 2021). Chitosan has attracted the attention as a promising candidate for the drug carrier and has been vastly exploited in the last decade because of its innate favorable properties (Dousti et al., 2021; Du Q et al., 2021). Basically, there are three categories of chitosan nanoparticles namely self-assembled nanoparticle, ionic cross-linked nanoparticle and polyelectrolyte complex, depending on different preparing methods (Zhang et al., 2019).
Classical chemotherapeutic drugs, such as doxorubicin, paclitaxel, cisplatin and so on, have made tremendous contributions to cancer treatment in last nearly half a century. However, these drugs also bring too many severe side effects, which restrict the intensively clinical use to cure cancer. A large number of studies have been conducted to solve these severe side effects of chemotherapeutic drugs. DDSs are introduced to targeted and sustained release of drugs in a controllable manner to minimize the adverse effects. Chitosan-based nanoparticle is a useful DDS, which can encapsulate and deliver various antitumor drugs to specific tumor tissues (Fathi et al., 2018; Pornpitchanarong et al., 2020; Ryu et al., 2020).
In addition to being encapsulated, chemotherapeutic drugs can also be covalently bound to hydrophilic polymers and self-assembled to form nanoparticles, which are called polymer drug conjugates. The conjugates of some anticancer drugs with chitosan and its derivatives selectively accumulate in tumors and prolong retention time in the blood circulation, thus show excellent anticancer effects with much milder adverse effects than that of the original drug due to cancer site-specific distribution and sustained release characteristics. Based on the cationic properties of chitosan, ionic cross-linked chitosan nanoparticles were prepared by electrostatic interaction, in which the amino group on the main chain interacts with polyanionic cross-linking agents such as tripolyphosphate (TPP), CaCl2, Na2SO4 and so on (Babu and Ramesh, 2017; Nemati et al., 2021). The process of ionically cross-linking requires more simple and mild preparation conditions with no toxic reagents, which is completely different from the chemical cross-linking. Furthermore, the biochemical properties of ionically cross-linking nanoparticles, such as size and surface charge, can be easily modified by adjustment of processing parameters, which changes drug encapsulation potency and time controlled release profile. However, the ionic cross-linked chitosan based particles are weak. It is reported that ionic cross-linked chitosan nanoparticles can improve tumor targeting of some ligands, such as folic acid, herceptin antibody and glycyrrhetinic acid (Tian et al., 2010; Arya et al., 2011; Hefnawy et al., 2020). Table 1 lists a few examples of chitosan based chemotherapeutic agents nanoparticles and their application in oncotherapies.
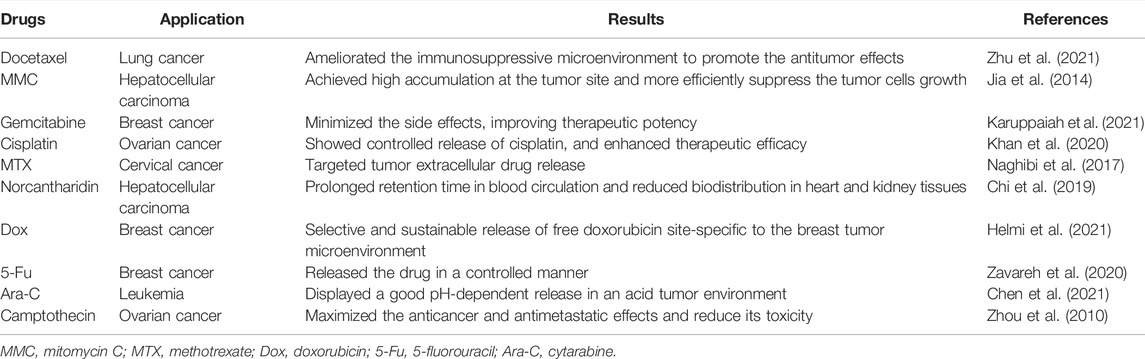
TABLE 1. A few examples of chitosan based chemotherapeutic drugs nanoparticles and their application in oncotherapies.
4.2.1.2 Chitosan-Based Systems as Therapeutic Genes Carriers
Gene-based therapy has become an indispensable part of comprehensive tumor therapy, which is achieved by delivering exogenous nucleic acids (DNA/siRNA) that can regulate gene expression of tumor cells (Senapati et al., 2019). Small interfering RNA (siRNA) system has been used for down-regulation of targeted gene and subsequent inhibition of cancer progression, which is a promising strategy in cancer treatment (Ashrafizadeh et al., 2021; Ferdows et al., 2022). SiRNA is a category of double stranded oligonucleotides containing 20–25 pairs of nucleotides, which is used to knock down targeted messenger RNA (mRNA) to induce gene silencing. However, its off-targeting property and degradation by enzymes in serum and the extracellular matrix prevent it application in cancer therapy. What’s more, therapeutic nucleic acids can be degraded by endonucleases in serum and extracellular matrix easily. Therapeutic nucleic acids are easily degraded by endonucleases in serum and extracellular matrix. Therefore, the development of effective vectors is the vital to successful gene therapy. Chitosan has attracted large attention as a promising polymer vector for delivery gene products because of its minimal toxicity, low immunogenicity, terrific biodegradability and its cationic property. The negatively charged gene products can bind to protonated cationic amino groups on chitosan backbone via electrostatic interaction, which shield them from being degraded by nucleases. Hence, the delivery of siRNA in chitosan-based nanocarriers is an important way to enhance its efficacy in gene silencing. However, due to the instability in vivo and insufficient cell release of natural chitosan, its transfection efficiency is relatively low. Therefore, it is most necessary to chemically modify natural chitosan to develop a more powerful chitosan based delivery system (Ragelle et al., 2013). Moreover, chitosan can also provide a platform for the co-delivery of siRNA and antitumor agents. In the past several years, a large number of studies have reported a lot of different chitosan-based delivery systems with terrific antitumor effects for a variety of therapeutic gene silencing nucleic acids. These systems achieved successful in vivo delivery of therapeutic genes siRNA with desirable tumor specificity and transfection efficiency, which suggested that adjusting the binding strength between vector and siRNA is very important to improve both transfection efficiency and antitumor activity. For instance, applying stimulation responsive chitosan based nanoparticle to co-deliver sgVEGFR2/Cas9 plasmid and Ca2+ channel siRNA demonstrated markedly increasing tumor targeting and therapeutic efficacy both in vitro and in vivo (Zhao et al., 2020; Li et al., 2021). Table 2 lists a few examples of chitosan based gene delivery nanoparticles and their application in oncotherapies.
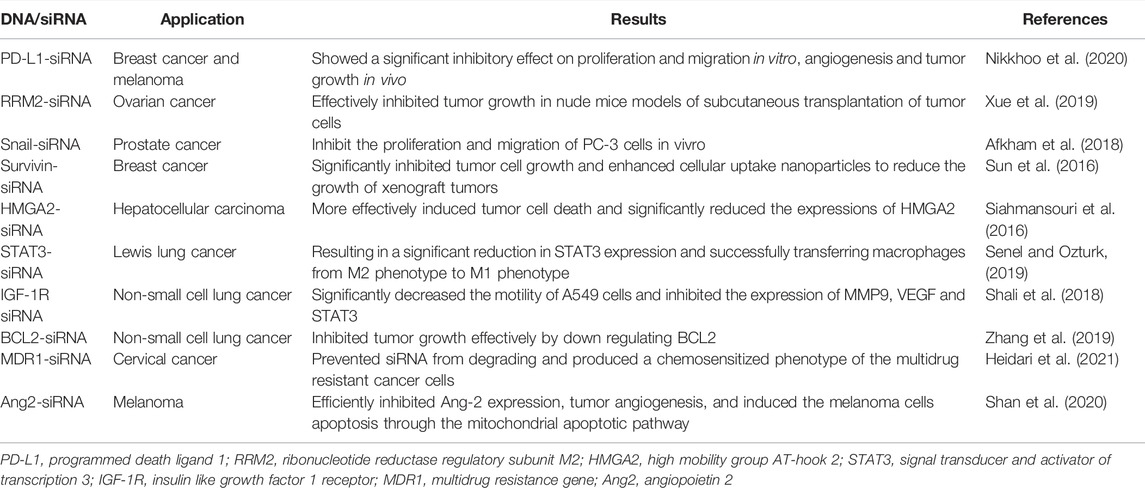
TABLE 2. A few examples of chitosan based therapeutic genes nanoparticles and their application in oncotherapies over the last decades.
4.2.1.3 Chitosan-Based Nanosystems as Carriers of Other Therapeutic Agents
Photodynamic therapy (PDT) is one of the most promising research fields in oncotherapy, which combines a light source with a photosensitizing agent preferably located within the tumor to destroy cancer. Photosensitizers are activated by light and transfer energy to molecular oxygen to produce reactive oxygen species (ROS), resulting in the death of targeted cells (Sun et al., 2022). PDT rapidly increases in cancer treatment due to its minimal toxicity. However, selectively deliver water-insoluble photosensitizers into target tissues is still a big challenge, more intelligent chitosan-based nanoparticles are urgently needed. It is reported that the pH-dependent conjugates exhibited photo toxicity against tumor cells. Furthermore, chlorin e6 (Ce6) and glycol chitosan self-assembled into nanoparticles attenuated its cytotoxicity but improved its therapeutic efficacy by preventing the nanoparticles from serum absorption and prolonged the blood circulation time (Ding et al., 2018). These chitosan-based nanosystems have been successfully applied to photodynamic therapy and photothermal therapy for breast cancer with minimal side effects (Liu et al., 2018).
Chitosan and its derivatives as drug carriers are also applied for cancer immunotherapy (Yang et al., 2021). Interleukin-12 (IL-12) is a heterodimeric pleiotropic cytokine with immunomodulatory activities, which has been intensively studied either as an antitumor agent or a vaccine adjuvant. However, the severe systematic toxicities hinder its clinical applications. Nevertheless, IL-12 conjugates with chitosan nanoparticles have marked effect on inhibiting growth of liver metastatic tumors of colorectal cancer with no obvious toxicity (Xu et al., 2012).
Moreover, it is reported that a chitosan coated copper sulfide (CuS) nanoparticles incorporated with immunoadjuvants which exerted antitumor activity. The chitosan coated nanoparticles are decomposed after laser excitation and then reassemble into chitosan CpG complex, which activates host anti-tumor immunity eventually. The chitosan coated copper sulfide (CuS) nanoparticles therapy shows a more effective effect than immunotherapy or photothermal therapy alone, resulting in synergistic effects against both primary treated and distant untreated tumors (Guo et al., 2014; Niu et al., 2021). Table 3 lists a few examples of chitosan based other therapeutic agents nanoparticles and their application in cancer treatment.
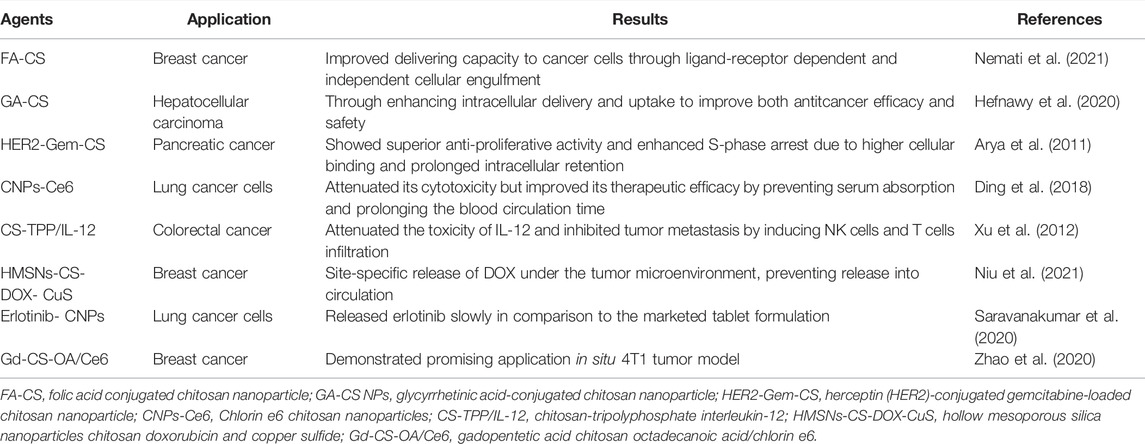
TABLE 3. A few examples of chitosan based other therapeutic agents nanoparticles and their application in oncotherapies over the last decades.
4.2.2 Effects of Chitosan and its Derivatives on Innate Immune Response in Cancer Therapy
Chitosan may activate dendritic cells (DCs) to enhance the antitumor activity of natural killer (NK) cells by upregulating IFN-γ production. Moreover, it is reported that chitosan enhances NK cell activity to kill leukemia cells (Li et al., 2018). Chitosan has also been used as a vaccine adjuvant for regulating tumor microenvironment (TME). The TME plays a vital role in cancer control and elimination (Katsuta et al., 2020). Currently, immune cell becomes a promising candidate for drug delivery and has very broad spectrum tumor-targeting properties. However, the off-target problems and effective release strategies stand in front of us when using immune cells as drug carrier. It is reported that co-delivery of chitosan and IL-12 resulted in high inhibition of tumor growth (Yang and Zaharoff, 2013; Eslahi et al., 2021). Additionally, chitosan based nanoparticle is a potential delivery system for DNA vaccine and IL-12 is an effective gene adjuvant, which can induce strong antitumor immune response. It is reported that chitosan suspension or nanoparticles have immunostimulatory activities and inhibit tumor growth by inducing polarization of macrophage to enhance antitumor activity (Nandgude and Pagar, 2021). Taking chitosan solution as an adjuvant intervention for patients with lung cancer can improve immunity during radiotherapy (Ma et al., 2015). Chitosan also shows a biological activity for activating macrophages for tumoricidal activity and for production of interleukin-2 (Yang et al., 2019b; Lima et al., 2021).
4.2.3 The Directed Effects of Chitosan and its Derivatives on Cancer Progression
Chitosan is a relatively low toxic and compatible polysaccharide. It is reported that administration of chitosan or oligochitosans can shield body from cancer induced oxidative stress. While, their anti-metastatic activity is mainly due to enhancing penetration properties (Kadry et al., 2018; Amirani et al., 2020). The migration of MDA-MB-231 human breast carcinoma cells weakened with elevated concentration of chitosan (Nam and Shon, 2009). Increasing the biodistribution of drugs is another mechanism for anticancer functionality of chitosan. The accumulation of the drug in tumor cells is due to chitosan improving cell permeability and drug retention time to low toxicity (Adhikari and Yadav, 2018).
Self-assembled microparticles from chitosan (SAMC) shows anticancer effect in human breast cancer cell lines and exhibits tumor growth inhibition in Ehrlich ascites tumor (EAT) bearing mice model. What’s more, SAMC decreases VEGF secretion in ascites, which is accompanied with reduction in neovessel formation. Consequently, SAMC may be another potential antitumor dietary supplement (Punarvasu and Prashanth, 2022). Modification of chitosan through its amino, acetamido, and hydroxy groups can give a veriety of derivatives with enhancing solubility and remarkable anticancer activity. Both chitosan and its various derivatives have been reported to show anticancer activity involving different cellular apoptotic pathways (Jiang et al., 2011; Jiang et al., 2015).
4.2.4 Effects of Chitosan and its Derivatives on the Signal Pathway in Cancer Therapy
Phosphatidylinositol 3-kinase (PI3K)-AKT pathway is an important kinase signaling networks in carcinogenesis. The abnormal activation of PI3K-AKT signaling pathway has been implicated in numerous malignancies including endometrial, hepatocellular, breast, colorectal, prostate and cervical cancer (Franke, 2008; Amirani et al., 2020). Thus regulation and blockage of this kinase and its key molecules may be a potential approach in cancer therapy, and tremendous efforts have been made to achieve this goal. It is reported that chitosan and its derivatives down-regulate AKT phosphorylation in a dose-dependent manner and being used to block AKT activities in different cancer types (Liu et al., 2011; Xiong et al., 2018; Amirani et al., 2020; Fang et al., 2021).
Additionally, chitosan could induce apoptosis through increasing the concentration of calcium ion, level of ROS and mitochondrial membrane potential. Too many Fas/FasL pathway associated proteins were expressed abnormally in cancer (Gao et al., 2020). Chitosan may regulate the expression of apoptosis associated proteins, subsequently activated the cleavage of caspase-9 and caspase-3, which finally induced apoptosis in mitochondrial pathway (Wu et al., 2018; Wu et al., 2020).
Furthermore, chitosan oligosaccharide may inhibit the abnormally up-regulated programmed cell death ligand 1 (PD-L1) after chemotherapy in various tumors via the MAPK activation and STAT1 inhibition to improve efficacy of T cell mediated immune killing in tumors, which indicates that chitosan oligosaccharide may be used to improve the efficacy of existing chemotherapies (Chen et al., 2022).
5 Conclusion and Perspectives
Cancer is a major contributor to the global disease burden and is likely to continue in the next 20 years. Though great efforts have been made on cancer treatment in the past decades, cancer associated motality is still the leading cause of death. Intensive studies on chitosan have made it to be one of the most important and potential polymers for cancer treatment. Chitosan-based nanoparticles have exhibited exciting antitumor efficacy both in vitro and in vivo, which indicates that there is vast scope of clinical application. The favorable properties of chitosan have made it an ideal nanocarrier for drug controlled-release. Chitosan-based vehicle can be used for the encapsulation or conjugation of chemotherpeutic drugs, therapeutic gene nucleic acids, photosensitizers and cytokines and so on, achieving a more reliable targeted therapy for cancer. What’s more, chitosan itself also exhibits inhibitory effects on tumor cells through different signal pathways.
However, the clinical use of chitosan still confronts with some major obstacles. First, few studies have tested the safety of chitosan in vivo. Secondly, the chemical versatility of chitosan makes it more difficult to identify each entity. Last but not the least, it is unclear whether the nanoparticle targeting mechanism shown in rodent tumor models works the same in cancer patients. Consequently, the clinical application of antitumor chitosan nanoparticles should still be done with much caution, and an in-depth further clinical translation studies are urgently needed.
Author Contributions
JD and YG conceived the study; JD and YG collected, analyzed, and interpreted the relevant literatures; JD and YG drew all the figures and tables; JD wrote the manuscript; YG supervised the study and revised the manuscript. The final version of the manuscript was read and approved by all authors.
Funding
This research was funded by the Natural Science Foundation of Jiangxi province, grant number 20171BAB205057.
Conflict of Interest
The authors declare that the research was conducted in the absence of any commercial or financial relationships that could be construed as a potential conflict of interest.
Publisher’s Note
All claims expressed in this article are solely those of the authors and do not necessarily represent those of their affiliated organizations, or those of the publisher, the editors and the reviewers. Any product that may be evaluated in this article, or claim that may be made by its manufacturer, is not guaranteed or endorsed by the publisher.
References
Adhikari, H. S., and Yadav, P. N. (2018). Anticancer Activity of Chitosan, Chitosan Derivatives, and Their Mechanism of Action. Int. J. Biomater. 2018, 2952085. doi:10.1155/2018/2952085
Afkham, A., Aghebati-Maleki, L., Siahmansouri, H., Sadreddini, S., Ahmadi, M., Dolati, S., et al. (2018). Chitosan (CMD)-mediated Co-delivery of SN38 and Snail-specific siRNA as a Useful Anticancer Approach against Prostate Cancer. Pharmacol. Rep. 70 (3), 418–425. doi:10.1016/j.pharep.2017.11.005
Amirani, E., Hallajzadeh, J., Asemi, Z., Mansournia, M. A., and Yousefi, B. (2020). Effects of Chitosan and Oligochitosans on the Phosphatidylinositol 3-Kinase-AKT Pathway in Cancer Therapy. Int. J. Biol. Macromol. 164, 456–467. doi:10.1016/j.ijbiomac.2020.07.137
Aranaz, I., Alcántara, A. R., Civera, M. C., Arias, C., Elorza, B., Heras Caballero, A., et al. (2021). Chitosan: An Overview of its Properties and Applications. Polymers (Basel) 13 (19), 3256. doi:10.3390/polym13193256
Arya, G., Vandana, M., Acharya, S., and Sahoo, S. K. (2011). Enhanced Antiproliferative Activity of Herceptin (HER2)-Conjugated Gemcitabine-Loaded Chitosan Nanoparticle in Pancreatic Cancer Therapy. Nanomedicine 7 (6), 859–870. doi:10.1016/j.nano.2011.03.009
Ashrafizadeh, M., Delfi, M., Hashemi, F., Zabolian, A., Saleki, H., Bagherian, M., et al. (2021). Biomedical Application of Chitosan-Based Nanoscale Delivery Systems: Potential Usefulness in siRNA Delivery for Cancer Therapy. Carbohydr. Polym. 260, 117809. doi:10.1016/j.carbpol.2021.117809
Babu, A., and Ramesh, R. (2017). Multifaceted Applications of Chitosan in Cancer Drug Delivery and Therapy. Mar. Drugs 15 (4). 96. doi:10.3390/md15040096
Bai, M., Yang, M., Gong, J., Xu, H., and Wei, Z. (2021). Progress and Principle of Drug Nanocrystals for Tumor Targeted Delivery. AAPS Pharmscitech 23 (1), 41. doi:10.1208/s12249-021-02200-w
Bhavsar, C., Momin, M., Gharat, S., and Omri, A. (2017). Functionalized and Graft Copolymers of Chitosan and its Pharmaceutical Applications. Expert Opin. Drug Deliv. 14 (10), 1189–1204. doi:10.1080/17425247.2017.1241230
Bonin, M., Sreekumar, S., Cord-Landwehr, S., and Moerschbacher, B. M. (2020). Preparation of Defined Chitosan Oligosaccharides Using Chitin Deacetylases. Int. J. Mol. Sci. 21 (21). 7835. doi:10.3390/ijms21217835
Cao, M., Li, H., Sun, D., and Chen, W. (2020). Cancer burden of Major Cancers in China: A Need for Sustainable Actions. Cancer Commun. (Lond) 40 (5), 205–210. doi:10.1002/cac2.12025
Chakrabarti, A., Talukdar, D., Pal, A., and Ray, M. (2014). Immunomodulation of Macrophages by Methylglyoxal Conjugated with Chitosan Nanoparticles against Sarcoma-180 Tumor in Mice. Cell. Immunol. 287 (1), 27–35. doi:10.1016/j.cellimm.2013.11.006
Chen, W., Zheng, R., Baade, P. D., Zhang, S., Zeng, H., Bray, F., et al. (2016). Cancer Statistics in China, 2015. CA Cancer J. Clin. 66 (2), 115–132. doi:10.3322/caac.21338
Chen, J., Dou, Y., Tang, Y., and Zhang, X. (2020). Folate Receptor-Targeted RNAi Nanoparticles for Silencing STAT3 in Tumor-Associated Macrophages and Tumor Cells. Nanomedicine 25, 102173. doi:10.1016/j.nano.2020.102173
Chen, K., Qian, Y., Wang, C., Yang, D., Qiu, X., and Binks, B. P. (2021). Tumor Microenvironment-Responsive, High Internal Phase Pickering Emulsions Stabilized by Lignin/chitosan Oligosaccharide Particles for Synergistic Cancer Therapy. J. Colloid Interf. Sci 591, 352–362. doi:10.1016/j.jcis.2021.02.012
Chen, J., Zhou, Z., Zheng, C., Liu, Y., Hao, R., Ji, X., et al. (2022). Chitosan Oligosaccharide Regulates AMPK and STAT1 Pathways Synergistically to Mediate PD-L1 Expression for Cancer Chemoimmunotherapy. Carbohydr. Polym. 277, 118869. doi:10.1016/j.carbpol.2021.118869
Chi, J., Jiang, Z., Chen, X., Peng, Y., Liu, W., Han, B., et al. (2019). Studies on Anti-hepatocarcinoma Effect, Pharmacokinetics and Tissue Distribution of Carboxymethyl Chitosan Based Norcantharidin Conjugates. Carbohydr. Polym. 226, 115297. doi:10.1016/j.carbpol.2019.115297
de Sousa Victor, R., Marcelo Da Cunha Santos, A., Viana De Sousa, B., de Araújo Neves, G., Navarro De Lima Santana, L., and Rodrigues Menezes, R. (2020). A Review on Chitosan's Uses as Biomaterial: Tissue Engineering, Drug Delivery Systems and Cancer Treatment. Materials (Basel) 13 (21), 4995. doi:10.3390/ma13214995
Ding, Y. F., Li, S., Liang, L., Huang, Q., Yuwen, L., Yang, W., et al. (2018). Highly Biocompatible Chlorin e6-Loaded Chitosan Nanoparticles for Improved Photodynamic Cancer Therapy. ACS Appl. Mater. Inter. 10 (12), 9980–9987. doi:10.1021/acsami.8b01522
Ding, F., Fu, J., Tao, C., Yu, Y., He, X., Gao, Y., et al. (2020). Recent Advances of Chitosan and its Derivatives in Biomedical Applications. Curr. Med. Chem. 27 (18), 3023–3045. doi:10.2174/0929867326666190405151538
Ding, J., Guo, Y., Jiang, X., Li, Q., Li, K., Liu, M., et al. (2020). Polysaccharides Derived from Saposhnikovia Divaricata May Suppress Breast Cancer through Activating Macrophages. Onco Targets Ther. 13, 10749–10757. doi:10.2147/OTT.S267984
Dousti, F., Soleimanbeigi, M., Mirian, M., Varshosaz, J., Hassanzadeh, F., Kasesaz, Y., et al. (2021). Boron Phenyl Alanine Targeted Ionic Liquid Decorated Chitosan Nanoparticles for Mitoxantrone Delivery to Glioma Cell Line. Pharm. Dev. Technol. 26 (8), 899–909. doi:10.1080/10837450.2021.1955927
Du, Q., Lv, F., Huang, J., Tang, X., Zhao, Z., and Chen, J. (2021). A Multiple Environment-Sensitive Prodrug Nanomicelle Strategy Based on Chitosan Graftomer for Enhanced Tumor Therapy of Gambogic Acid. Carbohydr. Polym. 267, 118229. doi:10.1016/j.carbpol.2021.118229
Dubey, S. K., Bhatt, T., Agrawal, M., Saha, R. N., Saraf, S., Saraf, S., et al. (2022). Application of Chitosan Modified Nanocarriers in Breast Cancer. Int. J. Biol. Macromol. 194, 521–538. doi:10.1016/j.ijbiomac.2021.11.095
Esfahani, K., Roudaia, L., Buhlaiga, N., Del Rincon, S. V., Papneja, N., and Miller, W. H. (2020). A Review of Cancer Immunotherapy: from the Past, to the Present, to the Future. Curr. Oncol. 27 (Suppl. 2), S87–S97. doi:10.3747/co.27.5223
Eslahi, M., Dana, P. M., Asemi, Z., Hallajzadeh, J., Mansournia, M. A., and Yousefi, B. (2021). The Effects of Chitosan-Based Materials on Glioma: Recent Advances in its Applications for Diagnosis and Treatment. Int. J. Biol. Macromol. 168, 124–129. doi:10.1016/j.ijbiomac.2020.11.180
Fang, T., Yao, Y., Tian, G., Chen, D., Wu, A., He, J., et al. (2021). Chitosan Oligosaccharide Attenuates Endoplasmic Reticulum Stress-Associated Intestinal Apoptosis via the Akt/mTOR Pathway. Food Funct. 12 (18), 8647–8658. doi:10.1039/d1fo01234g
Farhadihosseinabadi, B., Zarebkohan, A., Eftekhary, M., Heiat, M., Moosazadeh Moghaddam, M., and Gholipourmalekabadi, M. (2019). Crosstalk between Chitosan and Cell Signaling Pathways. Cell. Mol. Life Sci. 76 (14), 2697–2718. doi:10.1007/s00018-019-03107-3
Fathi, M., Majidi, S., Zangabad, P. S., Barar, J., Erfan-Niya, H., and Omidi, Y. (2018). Chitosan-based Multifunctional Nanomedicines and Theranostics for Targeted Therapy of Cancer. Med. Res. Rev. 38 (6), 2110–2136. doi:10.1002/med.21506
Ferdows, B. E., Patel, D. N., Chen, W., Huang, X., Kong, N., and Tao, W. (2022). RNA Cancer Nanomedicine: Nanotechnology-Mediated RNA Therapy. Nanoscale 14, 4448–4455. doi:10.1039/d1nr06991h
Franke, T. F. (2008). PI3K/Akt: Getting it Right Matters. Oncogene 27 (50), 6473–6488. doi:10.1038/onc.2008.313
Friedl, P., and Alexander, S. (2011). Cancer Invasion and the Microenvironment: Plasticity and Reciprocity. Cell 147 (5), 992–1009. doi:10.1016/j.cell.2011.11.016
Gao, J., Zhao, Y., Wang, C., Ji, H., Yu, J., Liu, C., et al. (2020). A Novel Synthetic Chitosan Selenate (CS) Induces Apoptosis in A549 Lung Cancer Cells via the Fas/FasL Pathway. Int. J. Biol. Macromol. 158, 689–697. doi:10.1016/j.ijbiomac.2020.05.016
George, A., Shah, P. A., and Shrivastav, P. S. (2019). Natural Biodegradable Polymers Based Nano-Formulations for Drug Delivery: A Review. Int. J. Pharm. 561, 244–264. doi:10.1016/j.ijpharm.2019.03.011
Ghaz-Jahanian, M. A., Abbaspour-Aghdam, F., Anarjan, N., Berenjian, A., and Jafarizadeh-Malmiri, H. (2015). Application of Chitosan-Based Nanocarriers in Tumor-Targeted Drug Delivery. Mol. Biotechnol. 57 (3), 201–218. doi:10.1007/s12033-014-9816-3
Grosso, R., and De-Paz, M. V. (2021). Thiolated-Polymer-Based Nanoparticles as an Avant-Garde Approach for Anticancer Therapies-Reviewing Thiomers from Chitosan and Hyaluronic Acid. Pharmaceutics 13 (6), 854. doi:10.3390/pharmaceutics13060854
Guo, L., Yan, D. D., Yang, D., Li, Y., Wang, X., Zalewski, O., et al. (2014). Combinatorial Photothermal and Immuno Cancer Therapy Using Chitosan-Coated Hollow Copper Sulfide Nanoparticles. ACS Nano 8 (6), 5670–5681. doi:10.1021/nn5002112
Hefnawy, A., Khalil, I. H., Arafa, K., Emara, M., and El-Sherbiny, I. M. (2020). Dual-Ligand Functionalized Core-Shell Chitosan-Based Nanocarrier for Hepatocellular Carcinoma-Targeted Drug Delivery. Int. J. Nanomedicine 15, 821–837. doi:10.2147/IJN.S240359
Heidari, R., Khosravian, P., Mirzaei, S. A., and Elahian, F. (2021). siRNA Delivery Using Intelligent Chitosan-Capped Mesoporous Silica Nanoparticles for Overcoming Multidrug Resistance in Malignant Carcinoma Cells. Sci. Rep. 11 (1), 20531. doi:10.1038/s41598-021-00085-0
Helmi, O., Elshishiny, F., and Mamdouh, W. (2021). Targeted Doxorubicin Delivery and Release within Breast Cancer Environment Using PEGylated Chitosan Nanoparticles Labeled with Monoclonal Antibodies. Int. J. Biol. Macromol. 184, 325–338. doi:10.1016/j.ijbiomac.2021.06.014
Herdiana, Y., Wathoni, N., Shamsuddin, S., Joni, I. M., and Muchtaridi, M. (2021). Chitosan-Based Nanoparticles of Targeted Drug Delivery System in Breast Cancer Treatment. Polymers (Basel) 13 (11), 1717. doi:10.3390/polym13111717
Hu, H., Qi, Q., Dong, Z., Yu, X., Mo, Y., Luo, J., et al. (2020). Albumin Coated Trimethyl Chitosan-Based Targeting Delivery Platform for Photothermal/chemo-Synergistic Cancer Therapy. Carbohydr. Polym. 241, 116335. doi:10.1016/j.carbpol.2020.116335
Ion, D., Niculescu, A. G., Păduraru, D. N., Andronic, O., Mușat, F., Grumezescu, A. M., et al. (2021). An Up-To-Date Review of Natural Nanoparticles for Cancer Management. Pharmaceutics 14 (1). 18. doi:10.3390/pharmaceutics14010018
Jia, M., Li, Y., Yang, X., Huang, Y., Wu, H., Huang, Y., et al. (2014). Development of Both Methotrexate and Mitomycin C Loaded PEGylated Chitosan Nanoparticles for Targeted Drug Codelivery and Synergistic Anticancer Effect. ACS Appl. Mater. Inter. 6 (14), 11413–11423. doi:10.1021/am501932s
Jiang, M., Ouyang, H., Ruan, P., Zhao, H., Pi, Z., Huang, S., et al. (2011). Chitosan Derivatives Inhibit Cell Proliferation and Induce Apoptosis in Breast Cancer Cells. Anticancer Res. 31 (4), 1321–1328.
Jiang, Z., Han, B., Li, H., Yang, Y., and Liu, W. (2015). Carboxymethyl Chitosan Represses Tumor Angiogenesis In Vitro and In Vivo. Carbohydr. Polym. 129, 1–8. doi:10.1016/j.carbpol.2015.04.040
Kadry, M. O., Abdel-Megeed, R. M., El-Meliegy, E., and Abdel-Hamid, A. Z. (2018). Crosstalk between GSK-3, C-Fos, NFκB and TNF-α Signaling Pathways Play an Ambitious Role in Chitosan Nanoparticles Cancer Therapy. Toxicol. Rep. 5, 723–727. doi:10.1016/j.toxrep.2018.06.002
Karuppaiah, A., Babu, D., Selvaraj, D., Natrajan, T., Rajan, R., Gautam, M., et al. (2021). Building and Behavior of a pH-Stimuli Responsive Chitosan Nanoparticles Loaded with Folic Acid Conjugated Gemcitabine Silver Colloids in MDA-MB-453 Metastatic Breast Cancer Cell Line and Pharmacokinetics in Rats. Eur. J. Pharm. Sci. 165, 105938. doi:10.1016/j.ejps.2021.105938
Katsuta, E., Rashid, O. M., and Takabe, K. (2020). Clinical Relevance of Tumor Microenvironment: Immune Cells, Vessels, and Mouse Models. Hum. Cel. 33 (4), 930–937. doi:10.1007/s13577-020-00380-4
Khan, M. M., Madni, A., Tahir, N., Parveen, F., Khan, S., Jan, N., et al. (2020). Co-Delivery of Curcumin and Cisplatin to Enhance Cytotoxicity of Cisplatin Using Lipid-Chitosan Hybrid Nanoparticles. Int. J. Nanomedicine 15, 2207–2217. doi:10.2147/IJN.S247893
Kou, S. G., Peters, L. M., and Mucalo, M. R. (2021). Chitosan: A Review of Sources and Preparation Methods. Int. J. Biol. Macromol. 169, 85–94. doi:10.1016/j.ijbiomac.2020.12.005
Kurakula, M., El-Helw, A. M., Sobahi, T. R., and Abdelaal, M. Y. (2015). Chitosan Based Atorvastatin Nanocrystals: Effect of Cationic Charge on Particle Size, Formulation Stability, and In-Vivo Efficacy. Int. J. Nanomedicine 10, 321–334. doi:10.2147/IJN.S77731
Li, X., Dong, W., Nalin, A. P., Wang, Y., Pan, P., Xu, B., et al. (2018). The Natural Product Chitosan Enhances the Anti-tumor Activity of Natural Killer Cells by Activating Dendritic Cells. Oncoimmunology 7 (6), e1431085. doi:10.1080/2162402X.2018.1431085
Li, C., Wang, J., Wang, Y., Gao, H., Wei, G., Huang, Y., et al. (2019). Recent Progress in Drug Delivery. Acta Pharm. Sin B 9 (6), 1145–1162. doi:10.1016/j.apsb.2019.08.003
Li, M., Zhao, Y., Zhang, W., Zhang, S., and Zhang, S. (2021). Multiple-therapy Strategies via Polysaccharides-Based Nano-Systems in Fighting Cancer. Carbohydr. Polym. 269, 118323. doi:10.1016/j.carbpol.2021.118323
Li, Y., Jiang, Y., Zheng, Z., Du, N., Guan, S., Guo, W., et al. (202221104). Co‐Delivery of Precisely Prescribed Multi‐Prodrug Combination by an Engineered Nanocarrier Enables Efficient Individualized Cancer Chemotherapy. Adv. Mater. 34, 2110490. doi:10.1002/adma.202110490
Liang, X., Li, L., Li, X., He, T., Gong, S., Zhu, S., et al. (2021). A Spontaneous Multifunctional Hydrogel Vaccine Amplifies the Innate Immune Response to Launch a Powerful Antitumor Adaptive Immune Response. Theranostics 11 (14), 6936–6949. doi:10.7150/thno.58173
Lima, B. V., Oliveira, M. J., Barbosa, M. A., Gonçalves, R. M., and Castro, F. (2021). Immunomodulatory Potential of Chitosan-Based Materials for Cancer Therapy: a Systematic Review of In Vitro, In Vivo and Clinical Studies. Biomater. Sci. 9 (9), 3209–3227. doi:10.1039/d0bm01984d
Lin, J. T., Liu, Z. K., Zhu, Q. L., Rong, X. H., Liang, C. L., Wang, J., et al. (2017). Redox-responsive Nanocarriers for Drug and Gene Co-delivery Based on Chitosan Derivatives Modified Mesoporous Silica Nanoparticles. Colloids Surf. B Biointerfaces 155, 41–50. doi:10.1016/j.colsurfb.2017.04.002
Liu, H. T., Huang, P., Ma, P., Liu, Q. S., Yu, C., and Du, Y. G. (2011). Chitosan Oligosaccharides Suppress LPS-Induced IL-8 Expression in Human Umbilical Vein Endothelial Cells through Blockade of P38 and Akt Protein Kinases. Acta Pharmacol. Sin. 32 (4), 478–486. doi:10.1038/aps.2011.10
Liu, J., Liang, H., Li, M., Luo, Z., Zhang, J., Guo, X., et al. (2018). Tumor Acidity Activating Multifunctional Nanoplatform for NIR-Mediated Multiple Enhanced Photodynamic and Photothermal Tumor Therapy. Biomaterials 157, 107–124. doi:10.1016/j.biomaterials.2017.12.003
López-Barrera, L. D., Díaz-Torres, R., Martínez-Rosas, J. R., Salazar, A. M., Rosales, C., and Ramírez-Noguera, P. (2021). Modification of Proliferation and Apoptosis in Breast Cancer Cells by Exposure of Antioxidant Nanoparticles Due to Modulation of the Cellular Redox State Induced by Doxorubicin Exposure. Pharmaceutics 13 (8), 1251. doi:10.3390/pharmaceutics13081251
Ma, J. X., Qian, L., and Zhou, Y. (2015). Stimulation Effect of Chitosan on the Immunity of Radiotherapy Patients Suffered from Lung Cancer. Int. J. Biol. Macromol. 72, 195–198. doi:10.1016/j.ijbiomac.2014.08.027
Marsili, L., Dal Bo, M., Berti, F., and Toffoli, G. (2021). Chitosan-Based Biocompatible Copolymers for Thermoresponsive Drug Delivery Systems: On the Development of a Standardization System. Pharmaceutics 13 (11). 1876. doi:10.3390/pharmaceutics13111876
Maspes, A., Pizzetti, F., Rossetti, A., Makvandi, P., Sitia, G., and Rossi, F. (2021). Advances in Bio-Based Polymers for Colorectal CancerTreatment: Hydrogels and Nanoplatforms. Gels 7 (1). 6. doi:10.3390/gels7010006
Miller, K. D., Nogueira, L., Mariotto, A. B., Rowland, J. H., Yabroff, K. R., Alfano, C. M., et al. (2019). Cancer Treatment and Survivorship Statistics, 2019. CA Cancer J. Clin. 69 (5), 363–385. doi:10.3322/caac.21565
Monteiro, P. F., Travanut, A., Conte, C., and Alexander, C. (2021). Reduction-responsive Polymers for Drug Delivery in Cancer Therapy-Is There Anything New to Discover? Wiley Interdiscip. Rev. Nanomed Nanobiotechnol 13 (2), e1678. doi:10.1002/wnan.1678
Mu, W., Chu, Q., Liu, Y., and Zhang, N. (2020). A Review on Nano-Based Drug Delivery System for Cancer Chemoimmunotherapy. Nanomicro Lett. 12 (1), 142. doi:10.1007/s40820-020-00482-6
Naghibi Beidokhti, H. R., Ghaffarzadegan, R., Mirzakhanlouei, S., Ghazizadeh, L., and Dorkoosh, F. A. (2017). Preparation, Characterization, and Optimization of Folic Acid-Chitosan-Methotrexate Core-Shell Nanoparticles by Box-Behnken Design for Tumor-Targeted Drug Delivery. AAPS Pharmscitech 18 (1), 115–129. doi:10.1208/s12249-015-0445-3
Nam, K. S., and Shon, Y. H. (2009). Suppression of Metastasis of Human Breast Cancer Cells by Chitosan Oligosaccharides. J. Microbiol. Biotechnol. 19 (6), 629–633. doi:10.4014/jmb.0811.603
Nandgude, T., and Pagar, R. (2021). Plausible Role of Chitosan in Drug and Gene Delivery against Resistant Breast Cancer Cells. Carbohydr. Res. 506, 108357. doi:10.1016/j.carres.2021.108357
Naqvi, S., and Moerschbacher, B. M. (2017). The Cell Factory Approach toward Biotechnological Production of High-Value Chitosan Oligomers and Their Derivatives: an Update. Crit. Rev. Biotechnol. 37 (1), 11–25. doi:10.3109/07388551.2015.1104289
Narayanan, D., Jayakumar, R., and Chennazhi, K. P. (2014). Versatile Carboxymethyl Chitin and Chitosan Nanomaterials: a Review. Wiley Interdiscip. Rev. Nanomed Nanobiotechnol 6 (6), 574–598. doi:10.1002/wnan.1301
Narmani, A., and Jafari, S. M. (2021). Chitosan-based Nanodelivery Systems for Cancer Therapy: Recent Advances. Carbohydr. Polym. 272, 118464. doi:10.1016/j.carbpol.2021.118464
Naskar, S., Koutsu, K., and Sharma, S. (2019). Chitosan-based Nanoparticles as Drug Delivery Systems: a Review on Two Decades of Research. J. Drug Target. 27 (4), 379–393. doi:10.1080/1061186X.2018.1512112
Nazir, S., Hussain, T., Ayub, A., Rashid, U., and Macrobert, A. J. (2014). Nanomaterials in Combating Cancer: Therapeutic Applications and Developments. Nanomedicine 10 (1), 19–34. doi:10.1016/j.nano.2013.07.001
Nemati, M., Bani, F., Sepasi, T., Zamiri, R. E., Rasmi, Y., Kahroba, H., et al. (2021). Unraveling the Effect of Breast Cancer Patients' Plasma on the Targeting Ability of Folic Acid-Modified Chitosan Nanoparticles. Mol. Pharm. 18 (12), 4341–4353. doi:10.1021/acs.molpharmaceut.1c00525
Nikkhoo, A., Rostami, N., Farhadi, S., Esmaily, M., Moghadaszadeh Ardebili, S., Atyabi, F., et al. (2020). Codelivery of STAT3 siRNA and BV6 by Carboxymethyl Dextran Trimethyl Chitosan Nanoparticles Suppresses Cancer Cell Progression. Int. J. Pharm. 581, 119236. doi:10.1016/j.ijpharm.2020.119236
Niu, S., Zhang, X., Williams, G. R., Wu, J., Gao, F., Fu, Z., et al. (2021). Hollow Mesoporous Silica Nanoparticles Gated by Chitosan-Copper Sulfide Composites as Theranostic Agents for the Treatment of Breast Cancer. Acta Biomater. 126, 408–420. doi:10.1016/j.actbio.2021.03.024
Nowak, A. K., and Lesterhuis, W. J. (2014). Chemoimmunotherapy: Still Waiting for the Magic to Happen. Lancet Oncol. 15 (8), 780–781. doi:10.1016/S1470-2045(14)70267-0
Pan, R., Zhu, M., Yu, C., Lv, J., Guo, Y., Bian, Z., et al. (2017). Cancer Incidence and Mortality: A Cohort Study in China, 2008-2013. Int. J. Cancer 141 (7), 1315–1323. doi:10.1002/ijc.30825
Park, J. K., Chung, M. J., Choi, H. N., and Park, Y. I. (2011). Effects of the Molecular Weight and the Degree of Deacetylation of Chitosan Oligosaccharides on Antitumor Activity. Int. J. Mol. Sci. 12 (1), 266–277. doi:10.3390/ijms12010266
Pérez-Herrero, E., and Fernández-Medarde, A. (2015). Advanced Targeted Therapies in Cancer: Drug Nanocarriers, the Future of Chemotherapy. Eur. J. Pharm. Biopharm. 93, 52–79. doi:10.1016/j.ejpb.2015.03.018
Pornpitchanarong, C., Rojanarata, T., Opanasopit, P., Ngawhirunpat, T., and Patrojanasophon, P. (2020). Catechol-modified Chitosan/hyaluronic Acid Nanoparticles as a New Avenue for Local Delivery of Doxorubicin to Oral Cancer Cells. Colloids Surf. B Biointerfaces 196, 111279. doi:10.1016/j.colsurfb.2020.111279
Punarvasu, T. P., and Prashanth, K. V. H. (2022). Self-assembled Chitosan Derived Microparticles Inhibit Tumor Angiogenesis and Induce Apoptosis in Ehrlich-Ascites-Tumor Bearing Mice. Carbohydr. Polym. 278, 118941. doi:10.1016/j.carbpol.2021.118941
Ragelle, H., Vandermeulen, G., and Préat, V. (2013). Chitosan-based siRNA Delivery Systems. J. Control Release. 172 (1), 207–218. doi:10.1016/j.jconrel.2013.08.005
Rodrigues, M. Á. V., Marangon, C. A., Martins, V. D. C. A., and Plepis, A. M. G. (2021). Chitosan/gelatin Films with Jatobá Resin: Control of Properties by Vegetal Resin Inclusion and Degree of Acetylation Modification. Int. J. Biol. Macromol. 182, 1737–1745. doi:10.1016/j.ijbiomac.2021.05.160
Ryu, J. H., Yoon, H. Y., Sun, I. C., Kwon, I. C., and Kim, K. (2020). Tumor-Targeting Glycol Chitosan Nanoparticles for Cancer Heterogeneity. Adv. Mater. 32 (51), e2002197. doi:10.1002/adma.202002197
Sabra, S., Abdelmoneem, M., Abdelwakil, M., Mabrouk, M. T., Anwar, D., Mohamed, R., et al. (2017). Self-Assembled Nanocarriers Based on Amphiphilic Natural Polymers for Anti- Cancer Drug Delivery Applications. Curr. Pharm. Des. 23 (35), 5213–5229. doi:10.2174/1381612823666170526111029
Salehinik, F., Behzad, T., Zamani, A., and Bahrami, B. (2021). Extraction and Characterization of Fungal Chitin Nanofibers from Mucor Indicus Cultured in Optimized Medium Conditions. Int. J. Biol. Macromol. 167, 1126–1134. doi:10.1016/j.ijbiomac.2020.11.066
Saravanakumar, K., Sathiyaseelan, A., Mariadoss, A. V. A., Jeevithan, E., Hu, X., Shin, S., et al. (2020). Dual Stimuli-Responsive Release of Aptamer AS1411 Decorated Erlotinib Loaded Chitosan Nanoparticles for Non-small-cell Lung Carcinoma Therapy. Carbohydr. Polym. 245, 116407. doi:10.1016/j.carbpol.2020.116407
Senapati, D., Patra, B. C., Kar, A., Chini, D. S., Ghosh, S., Patra, S., et al. (2019). Promising Approaches of Small Interfering RNAs (siRNAs) Mediated Cancer Gene Therapy. Gene 719, 144071. doi:10.1016/j.gene.2019.144071
Şenel, B., and Öztürk, A. A. (2019). New Approaches to Tumor Therapy with siRNA-Decorated and Chitosan-Modified PLGA Nanoparticles. Drug Develop. Ind. Pharm. 45 (11), 1835–1848. doi:10.1080/03639045.2019.1665061
Shahbaz, U. (2020). Chitin, Characteristic, Sources, and Biomedical Application. Curr. Pharm. Biotechnol. 21 (14), 1433–1443. doi:10.2174/1389201021666200605104939
Shakeran, Z., Keyhanfar, M., Varshosaz, J., and Sutherland, D. S. (2021). Biodegradable Nanocarriers Based on Chitosan-Modified Mesoporous Silica Nanoparticles for Delivery of Methotrexate for Application in Breast Cancer Treatment. Mater. Sci. Eng. C Mater. Biol. Appl. 118, 111526. doi:10.1016/j.msec.2020.111526
Shali, H., Shabani, M., Pourgholi, F., Hajivalili, M., Aghebati-Maleki, L., Jadidi-Niaragh, F., et al. (2018). Co-delivery of Insulin-like Growth Factor 1 Receptor Specific siRNA and Doxorubicin Using Chitosan-Based Nanoparticles Enhanced Anticancer Efficacy in A549 Lung Cancer Cell Line. Artif. Cell Nanomed Biotechnol 46 (2), 293–302. doi:10.1080/21691401.2017.1307212
Shan, X., Yu, W., Ni, X., Xu, T., Lei, C., Liu, Z., et al. (2020). Effect of Chitosan Magnetic Nanoparticles Loaded with Ang2-siRNA Plasmids on the Growth of Melanoma Xenografts in Nude Mice. Cancer Manag. Res. 12, 7475–7485. doi:10.2147/CMAR.S250479
Shikhi-Abadi, P. G., and Irani, M. (2021). A Review on the Applications of Electrospun Chitosan Nanofibers for the Cancer Treatment. Int. J. Biol. Macromol. 183, 790–810. doi:10.1016/j.ijbiomac.2021.05.009
Siahmansouri, H., Somi, M. H., Babaloo, Z., Baradaran, B., Jadidi-Niaragh, F., Atyabi, F., et al. (2016). Effects of HMGA2 siRNA and Doxorubicin Dual Delivery by Chitosan Nanoparticles on Cytotoxicity and Gene Expression of HT-29 Colorectal Cancer Cell Line. J. Pharm. Pharmacol. 68 (9), 1119–1130. doi:10.1111/jphp.12593
Siegel, R. L., Miller, K. D., Fuchs, H. E., and Jemal, A. (2021). Cancer Statistics, 2021. CA A. Cancer J. Clin. 71 (1), 7–33. doi:10.3322/caac.21654
Sohrabi Kashani, A., and Packirisamy, M. (2021). Cancer-Nano-Interaction: From Cellular Uptake to Mechanobiological Responses. Ijms 22 (17), 9587. doi:10.3390/ijms22179587
Sun, P., Huang, W., Jin, M., Wang, Q., Fan, B., Kang, L., et al. (2016). Chitosan-based Nanoparticles for Survivin Targeted siRNA Delivery in Breast Tumor Therapy and Preventing its Metastasis. Int. J. Nanomedicine 11, 4931–4945. doi:10.2147/IJN.S105427
Sun, N., Wen, X., and Zhang, S. (2022). Strategies to Improve Photodynamic Therapy Efficacy of Metal-free Semiconducting Conjugated Polymers. Int. J. Nanomedicine 17, 247–271. doi:10.2147/IJN.S337599
Sung, H., Ferlay, J., Siegel, R. L., Laversanne, M., Soerjomataram, I., Jemal, A., et al. (2021). Global Cancer Statistics 2020: GLOBOCAN Estimates of Incidence and Mortality Worldwide for 36 Cancers in 185 Countries. CA Cancer J. Clin. 71 (3), 209–249. doi:10.3322/caac.21660
Tang, L., Li, J., Zhao, Q., Pan, T., Zhong, H., and Wang, W. (2021). Advanced and Innovative Nano-Systems for Anticancer Targeted Drug Delivery. Pharmaceutics 13 (8), 1151. doi:10.3390/pharmaceutics13081151
Tian, Q., Zhang, C. N., Wang, X. H., Wang, W., Huang, W., Cha, R. T., et al. (2010). Glycyrrhetinic Acid-Modified Chitosan/poly(ethylene Glycol) Nanoparticles for Liver-Targeted Delivery. Biomaterials 31 (17), 4748–4756. doi:10.1016/j.biomaterials.2010.02.042
Van Hees, S., Elbrink, K., De Schryver, M., Delputte, P. L., and Kiekens, F. (2020). Improving Cellular Uptake and Cytotoxicity of Chitosan-Coated Poly(lactic-Co-Glycolic Acid) Nanoparticles in Macrophages. Nanomedicine (Lond) 15 (27), 2671–2688. doi:10.2217/nnm-2020-0317
Wang, W., and Xu, D. (1994). Viscosity and Flow Properties of Concentrated Solutions of Chitosan with Different Degrees of Deacetylation. Int. J. Biol. Macromol. 16 (3), 149–152. doi:10.1016/0141-8130(94)90042-6
Wang, G., Li, R., Parseh, B., and Du, G. (2021). Prospects and Challenges of Anticancer Agents' Delivery via Chitosan-Based Drug Carriers to Combat Breast Cancer: a Review. Carbohydr. Polym. 268, 118192. doi:10.1016/j.carbpol.2021.118192
Wu, D., Zhao, Y., Fu, S., Zhang, J., Wang, W., Yan, Z., et al. (2018). Seleno-short-chain Chitosan Induces Apoptosis in Human Breast Cancer Cells through Mitochondrial Apoptosis Pathway In Vitro. Cell Cycle 17 (13), 1579–1590. doi:10.1080/15384101.2018.1464845
Wu, C., Dai, Y., Yuan, G., Su, J., and Liu, X. (2019). Immunomodulatory Effects and Induction of Apoptosis by Different Molecular Weight Chitosan Oligosaccharides in Head Kidney Macrophages from Blunt Snout Bream (Megalobrama amblycephala). Front. Immunol. 10, 869. doi:10.3389/fimmu.2019.00869
Wu, P., Yu, S. S., Liu, C., and Liu, A. J. (2020). Seleno-Chitosan Induces Apoptosis of Lung Cancer Cell Line SPC-A-1 via Fas/FasL Pathway. Bioorg. Chem. 97, 103701. doi:10.1016/j.bioorg.2020.103701
Xiong, H., Wu, M., Zou, H., Jiang, S., Yi, H., Yi, T., et al. (2018). Chitosan Inhibits Inflammation and Adipogenesis of Orbital Fibroblasts in Graves Ophthalmopathy. Mol. Vis. 24, 509–517.
Xu, Q., Guo, L., Gu, X., Zhang, B., Hu, X., Zhang, J., et al. (2012). Prevention of Colorectal Cancer Liver Metastasis by Exploiting Liver Immunity via Chitosan-TPP/nanoparticles Formulated with IL-12. Biomaterials 33 (15), 3909–3918. doi:10.1016/j.biomaterials.2012.02.014
Xu, J., Ma, Q., Zhang, Y., Fei, Z., Sun, Y., Fan, Q., et al. (2022). Yeast-derived Nanoparticles Remodel the Immunosuppressive Microenvironment in Tumor and Tumor-Draining Lymph Nodes to Suppress Tumor Growth. Nat. Commun. 13 (1), 110. doi:10.1038/s41467-021-27750-2
Xue, T., Wang, L., Li, Y., Song, H., Chu, H., Yang, H., et al. (2019). SiRNA-Mediated RRM2 Gene Silencing Combined with Cisplatin in the Treatment of Epithelial Ovarian Cancer In Vivo: An Experimental Study of Nude Mice. Int. J. Med. Sci. 16 (11), 1510–1516. doi:10.7150/ijms.33979
Yang, L., and Zaharoff, D. A. (2013). Role of Chitosan Co-formulation in Enhancing Interleukin-12 Delivery and Antitumor Activity. Biomaterials 34 (15), 3828–3836. doi:10.1016/j.biomaterials.2013.02.031
Yang, Y., Xing, R., Liu, S., Qin, Y., Li, K., Yu, H., et al. (2019a). Hydroxypropyltrimethyl Ammonium Chloride Chitosan Activates RAW 264.7 Macrophages through the MAPK and JAK-STAT Signaling Pathways. Carbohydr. Polym. 205, 401–409. doi:10.1016/j.carbpol.2018.10.101
Yang, Y., Xing, R., Liu, S., Qin, Y., Li, K., Yu, H., et al. (2019b). Immunostimulatory Effects of Chitooligosaccharides on RAW 264.7 Mouse Macrophages via Regulation of the MAPK and PI3K/Akt Signaling Pathways. Mar. Drugs 17 (1). 36. doi:10.3390/md17010036
Yang, M., Li, J., Gu, P., and Fan, X. (2021). The Application of Nanoparticles in Cancer Immunotherapy: Targeting Tumor Microenvironment. Bioact Mater. 6 (7), 1973–1987. doi:10.1016/j.bioactmat.2020.12.010
Younes, I., and Rinaudo, M. (2015). Chitin and Chitosan Preparation from marine Sources. Structure, Properties and Applications. Mar. Drugs 13 (3), 1133–1174. doi:10.3390/md13031133
Zavareh, H. S., Pourmadadi, M., Moradi, A., Yazdian, F., and Omidi, M. (2020). Chitosan/carbon Quantum Dot/aptamer Complex as a Potential Anticancer Drug Delivery System towards the Release of 5-fluorouracil. Int. J. Biol. Macromol. 165 (Pt A), 1422–1430. doi:10.1016/j.ijbiomac.2020.09.166
Zeng, Z., Liu, Y., Wen, Q., Li, Y., Yu, J., Xu, Q., et al. (2021). Experimental Study on Preparation and Anti-tumor Efficiency of Nanoparticles Targeting M2 Macrophages. Drug Deliv. 28 (1), 943–956. doi:10.1080/10717544.2021.1921076
Zhang, E., Xing, R., Liu, S., Qin, Y., Li, K., and Li, P. (2019). Advances in Chitosan-Based Nanoparticles for Oncotherapy. Carbohydr. Polym. 222, 115004. doi:10.1016/j.carbpol.2019.115004
Zhang, W., Xu, W., Lan, Y., He, X., Liu, K., and Liang, Y. (2019). Antitumor Effect of Hyaluronic-Acid-Modified Chitosan Nanoparticles Loaded with siRNA for Targeted Therapy for Non-small Cell Lung Cancer. Int. J. Nanomedicine 14, 5287–5301. doi:10.2147/IJN.S203113
Zhao, J., Yang, Y., Han, X., Liang, C., Liu, J., Song, X., et al. (2017). Redox-Sensitive Nanoscale Coordination Polymers for Drug Delivery and Cancer Theranostics. ACS Appl. Mater. Inter. 9 (28), 23555–23563. doi:10.1021/acsami.7b07535
Zhao, X., Shen, R., Bao, L., Wang, C., and Yuan, H. (2020). Chitosan Derived Glycolipid Nanoparticles for Magnetic Resonance Imaging Guided Photodynamic Therapy of Cancer. Carbohydr. Polym. 245, 116509. doi:10.1016/j.carbpol.2020.116509
Zhao, Z., Ji, M., Wang, Q., He, N., and Li, Y. (2020). Ca2+ Signaling Modulation Using Cancer Cell Membrane Coated Chitosan Nanoparticles to Combat Multidrug Resistance of Cancer. Carbohydr. Polym. 238, 116073. doi:10.1016/j.carbpol.2020.116073
Zhou, L., Du, L., Chen, X., Li, X., Li, Z., Wen, Y., et al. (2010). The Antitumor and Antimetastatic Effects of N-Trimethyl Chitosan-Encapsulated Camptothecin on Ovarian Cancer with Minimal Side Effects. Oncol. Rep. 24 (4), 941–948. doi:10.3892/or.2010.941
Zhu, X., Yu, Z., Feng, L., Deng, L., Fang, Z., Liu, Z., et al. (2021). Chitosan-based Nanoparticle Co-delivery of Docetaxel and Curcumin Ameliorates Anti-tumor Chemoimmunotherapy in Lung Cancer. Carbohydr. Polym. 268, 118237. doi:10.1016/j.carbpol.2021.118237
Keywords: cancer treatment, anticancer drugs, drug delivery system, chitosan, polysaccharides
Citation: Ding J and Guo Y (2022) Recent Advances in Chitosan and its Derivatives in Cancer Treatment. Front. Pharmacol. 13:888740. doi: 10.3389/fphar.2022.888740
Received: 03 March 2022; Accepted: 13 April 2022;
Published: 28 April 2022.
Edited by:
Christian Celia, University of Studies G d’Annunzio Chieti and Pescara, ItalyReviewed by:
Se-Kwon Kim, Pukyong National University, South KoreaHamed Barabadi, Shahid Beheshti University of Medical Sciences, Iran
Copyright © 2022 Ding and Guo. This is an open-access article distributed under the terms of the Creative Commons Attribution License (CC BY). The use, distribution or reproduction in other forums is permitted, provided the original author(s) and the copyright owner(s) are credited and that the original publication in this journal is cited, in accordance with accepted academic practice. No use, distribution or reproduction is permitted which does not comply with these terms.
*Correspondence: Yonghong Guo, 404717434@qq.com