- Antibiotics Research and Re-Evaluation Key Laboratory of Sichuan Province, School of Pharmacy, Chengdu University, Chengdu, China
The emergence of antibiotic resistant Gram-negative bacteria such as Klebsiella pneumoniae (KP) is becoming a major public health threat and imposing a financial burden worldwide. A serious lack of new drugs under development is undermining efforts to fight them. In this study, we report a potent combination of linezolid and polymyxin B nonapeptide PBNP (LP) against KP infection in vitro and in vivo. The checkerboard test and the time-kill assay were performed to detect the antibacterial activity of LP against KP in vitro. And the Caenorhabditis elegans (C. elegans) was used as infection model to evaluate the protective effect of LP against KP infection in vivo. The LP combination showed significantly synergistic activity and antibacterial effects against KP, while linezolid and PBNP as monotherapies revealed no dramatically antibacterial activity against the KP strains. Additionally, we found that the LP treatment altered the biofilm production and morphology of KP. Furthermore, the LP treatments significantly protected C. elegans from KP infection. In conclusion, this study indicated that the LP combination exhibited significantly synergistic activity against KP and PBNP can be used as a potential activity enhancer. More importantly, this strategy provided the improvement of antibacterial activity spectrum of agents like linezolid and represented a potent alternative to overcome antibiotic resistance in the future.
Introduction
Gram-negative bacteria, such as Klebsiella pneumoniae (KP) is redoubtable threats to public health, leading to huge costs of healthcare worldwide (Li et al., 2016c). KP is a gram-negative, encapsulated, and rod-shaped bacterium and is an extremely important clinical pathogens of hospital infection, causing severe pneumonia, meningitis, and sepsis (Bachman et al., 2015). It is broadly distributed in the environment and mainly settles in human respiratory tract, gastrointestinal tract and urinary tract (Tan et al., 2020). Carbapenem is one of the mainstay of treatment for severe hospital infection caused by KP, while, the drastic increase in the prevalence of carbapenem-resistant KP (CRKP) have caused high morbidity and mortality rates, and leading to a global epidemic in hospitals (Chapelle et al., 2021).
Up to now, the infections with KP or CRKP are resistant to the most antibiotics except polymyxin in worldwide (Doi and van Duin, 2020). Polymyxins were a group of polycationic amphipathic peptide antibiotics and were mainly bactericidal to Gram-negative bacteria by binding its lipid A component of lipopolysaccharide (LPS), permeabilizing the outer membrane, and subsequently inducing the death of cell (Velkov et al., 2013). The polymyxins showed effective activity against a great majority of KP strains, while only two polymyxins (polymyxin B and colistin) are available for clinical application, and are used as the last-line therapy (Li et al., 2006). Moreover, the nephrotoxicity and neurotoxicity of polymyxins are the main concerns preventing their widespread clinical application. Therefore, it is necessary to develop the novel and effective antibacterial agents for the treatment and control of KP infection. To fight the current antibiotic-resistant bacteria, one of the alternative strategies is to combine existing antibiotics with polymyxins by enhancing the efficacy of antibiotics. The ability of polymyxins to exert synergistic effects in combination with lots of antibiotics, including anti-Gram-positive bacterial agents against Gram-negative pathogens or CRKP in vitro, has been recently reported (Liu et al., 2014; Di et al., 2015; Wistrand-Yuen et al., 2020). Linezolid is the first member of the synthetic oxazolidinone class antibiotics and has great antimicrobial activity against a wide range of Gram-positive bacteria including staphylococci, streptococci, enterococci (Stalker and Jungbluth, 2003). Linezolid can impede the initiation of bacterial protein synthesis by binding to the 23S RNA peptidyl transferase of the 50S subunit in the bacterial ribosome (Leach et al., 2007). Recently, combined with other antibiotics showed excellent synergistic and antibacterial activity against multidrug-resistant (MDR) pathogens infection (Zhou et al., 2018; Zhuang et al., 2020), suggesting the potential of linezolid as candidate for developing novel combination therapeutic agents. However, combinations of linezolid and polymyxin derivatives for the treatment of KP infections remains an open question.
In the current study, we examined the antibacterial activity of linezolid alone, and in combination with polymyxin B nonapeptide PBNP (LP) against KP in vitro. In addition, protective potential of LP was investigated by challenging with KP based on infection model with the murine alveolar macrophages and nematode Caenorhabditis elegans (C. elegans). The present study provided a novel and effective strategy to control KP infection and facilitated the development of potent therapeutic agents against KP-related diseases.
Materials and Methods
Antibacterial Agents
The 11 antibiotics were obtained from the Yuanye (Shanghai, China): including erythromycin, linezolid, lincomycin, Nisin, vancomycin, chlortetracycline, penicillin G, ampicillin, ciprofloxacin, ofloxacin, tigecycline. The polymyxin B heptapeptide (PBHP) was obtained from Nanjing Peptide Biotech (Nanjing, China) and polymyxin B octapeptide (PBOP) was obtained from Dayang Chem (Hangzhou, China). The polymyxin B nonapeptide (PBNP) were purchased from MedChem Express (NJ, United States).
Bacterial Strains, Cell Lines, Caenorhabditis elegans Strain and Growth Conditions
The Klebsiella pneumoniae type strain ATCC 13883 (KP13883) was obtained from the American Type Culture Collection and maintained as the manufacturer’s instructions described. The clinical strain carbapenem-resistant K. pneumonia 25826 (KP25826) was kindly provided by Dr. Zongxin Fang (The First People’s Hospital of Hefei). The clinical strain hypervirulent K. pneumonia (hvKP) NTUH-K2044 (KP 2044) and K. pneumonia WT strain ATCC 43816 (KPWT) were kindly provided by Dr. Min Wu (University of North Dakota) and Dr. V. Miller (University of North Carolina) and were cultured in LB (Luria-Bertani) broth/agar at 37°C as described previously (Lawlor et al., 2005; Li et al., 2016a; Li et al., 2016b). The murine alveolar macrophage cell line MH-S cell was preserved in the laboratory and cultured as previously described (Zhao et al., 2019). The C. elegans strain N2 was preserved in the laboratory (Li et al., 2021) and cultured at 20°C on the nematode growth medium by feeding with Escherichia coli (E. coli) OP50 stain as a regular diet.
Susceptibility Tests
The MICs (minimal inhibitory concentration) were detected for the K. pneumonia strains by dilution method, with a final concentration of 1 × 105 CFU/ml on LB medium for 18–24 h, as previously described by the Clinical and Laboratory Standards Institute (CLSI, 2014). All the experiments were carried out in triplicate.
Antibiotic Synergy Test
The synergy of three polymyxin B derivatives (PBHP, PBOP, PBNP) combined with five antibiotics were tested by the checkerboard method as previously described (Magi et al., 2015). All the experiments were performed in triplicate. Briefly, a two-dimensional array was performed to prepare the serial concentrations of test compounds according to the MIC of the two compounds. The output of checkerboard test was carried out and to obtain the Fractional Inhibitory Concentration Index (FIC) based on the formulas: FIC Index = FICA + FICB, FICA = MICA+B/MICA, FICB = MICB+A/MICB. The value of MICA+B is the MIC value of compound A with compound B, and likewise for MICB+A. The FIC Index values were indicated as shown below: synergy (FIC Index ≤0.5), antagonism (FIC Index >4.0), and no interaction (FIC Index >0.5–4.0).
Biofilm Production
The production of biofilm was detected by crystal violet staining as previously described (Wu et al., 2012). Briefly, 1.0 × 106 CFU of KPWT were cultured in 24-well plate or glass tubes with appropriate LB broth and treated with phosphate-buffered saline (PBS, pH 7.4), linezolid (16 μg/ml), PBNP (8 μg/ml) or combination of linezolid and PBNP (LP) at 37°C for 12 h, respectively. The unattached bacteria were removed and the plates or tubes were washed three times with PBS. The biofilms were stained with 0.2% (wt/vol) crystal violet for 30 min and detected at OD595 after dissolution by 95% ethanol. All the experiments were carried out in triplicate.
Scanning Electron Microscopy
The 1.0 × 106 CFU of KPWT were cultured in 12-well plate with LB broth and treated with PBS, linezolid, PBNP or LP at 37°C for 12 h, respectively as described above. The morphological and surface characteristics were detected by scanning electron microscopy (SEM) as previously described (Rajeshwari et al., 2009).
Infection Experiments in MH-S Cell Model
The KPWT strain was grown for 14 h in LB broth at 37°C. The KPWT pellet was prepared by centrifugation at 5,000 g and then was resuspended in sterile PBS for infection. The MH-S cells were grown in RPMI-1640 medium containing 10% fetal bovine serum (FBS) at 37°C with 5% CO2. The infection experiments in MH-S cells were performed according to a previously report (Ye et al., 2015). Briefly, the MH-S cells were changed to an antibiotic-free medium and infected by KPWT at an MOI of 10:1 (bacteria-cell ratio) for 1 h, and treated with PBS, linezolid, PBNP or LP for 1, 6, and 12 h respectively as described above. The bacterial burdens in the MH-S cells were detected at different time point as previously described (Huang et al., 2020).
Infection Experiments in Caenorhabditis elegans Model
To further investigate the protective efficacy of LP, the C. elegans were infected with KPWT or KP25826 (10 worms/group) as previously described (Kamaladevi and Balamurugan, 2017). The infected worms were transferred to the 24-well culture plate containing sterile M9 buffer and then treated with PBS, linezolid (16 μg/ml), PBNP (8 μg/ml) or LP, respectively. The mortality of the challenged C. elegans was monitored for the subsequent 7 days. The nematodes that did not show obviously pharyngeal pumping and response to touch were indicated as dead. The bacterial burdens of KPWT or KP25826 in the C. elegans were detected at 12 or 24 h post infection. All the experiments were performed in triplicate.
Statistical Analysis
The Data and statistical tests were analyzed by using GraphPad Prism 6.0. A Mantel-Cox log rank test was applied to compare the survival rates between the control group and monotherapy-treated or LP-treated C. elegans (Huang et al., 2019). Means were compared by using a one-way analysis of variance (ANOVA), followed by a Tukey–Kramer post hoc test using a 95% confidence interval.
Results
Headings Antibiotic Sensitivity and Resistance Patterns
As shown in Table 1, all the tested KP strains were highly resistant the conventional antibiotics which were used to kill Gram-positive bacteria (including erythromycin, lincomycin, linezolid, nisin, vancomycin). In addition, all the strains were susceptible to the conventional antibiotics against Gram-negative bacteria in a low concentration pattern (penicillin G, ampicillin, ciprofloxacin, ofloxacin, tigecycline, chlortetracycline). However, all the tested strains were highly insusceptible with the 3 different polymyxin B derivatives (PBHP, PBOP, PBNP).
Strong Antibacterial Synergy of Combined Polymyxin B Derivatives and Antibiotics
In the checkerboard assay, 15 different combinations of three polymyxin B derivatives and five conventional anti-Gram-positive agents were tested for K. pneumoniae (Table 2). FIC Index values were calculated by considering all combinations of three polymyxin B derivatives and five conventional antibiotics in which there was no visible growth. The lowest FIC Index value was shown in each combination group as indicated in Table 2, which were ranged from 0.063 to 0.500 in KP plates. The synergy (FIC Index ≤0.5) was detected in all strains and antagonism was not observed. Consideration that combinations of linezolid (16 μg/ml) and PBNP (8 μg/ml) (LP) had the lowest FIC index in all the tested group, we focused on LP as a novel combination and further investigated their antibacterial activity against KP throughout the present study.
LP is Highly Antibacterial in Culture Media
To investigate the antibacterial activity of LP in the K. pneumoniae in vitro, KPWT strains were seeded in 96-well plates and treated with PBS, linezolid, PBNP or LP for 1, 6, 12, and 24 h, respectively. The results showed that bacterial CFU was decreased significantly in the LP treated group compared to the PBS-treated group at different time point (Figures 1A1–A4). To validate these data, we further tested the antimicrobial effects of LP on the hypervirulent clinical strain KP 2044 (Figures 1B1–B4) and clinical CRKP strain KP25826 (Figures 1C1–C4). Treatment with LP significantly decreased the CFU of KP at the indicated time points compared the linezolid-treated or PBNP-treated group (Figures 1B1–B4,C1–C4).
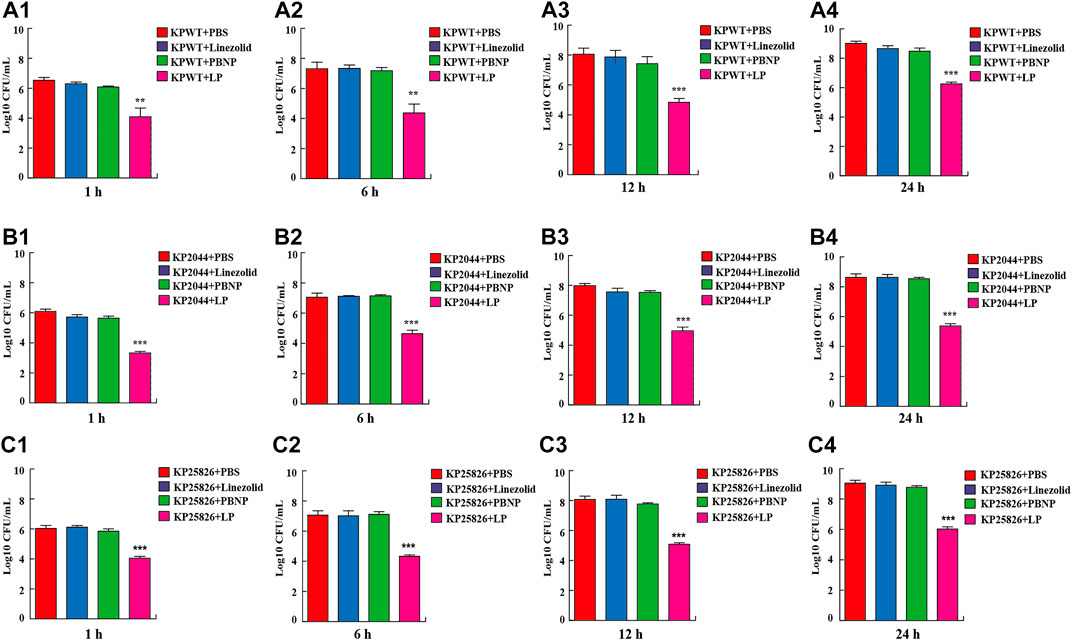
FIGURE 1. Treatment of LP reduced K. pneumoniae viability (A1–A4) 1 × 105 CFU of KPWT were seeded in 96-well plates and treated with PBS, linezolid (16 μg/ml), PBNP (8 μg/ml) or combination of linezolid and PBNP (LP) for 1 h (A1), 6 h (A2), 12 h (A3), and 24 h (A4), respectively. The bacteria on the LB agar plates were counted. (B1–B4) 1 × 105 CFU of KP2044 were seeded in 96-well plates and treated with PBS, linezolid (16 μg/ml), PBNP (8 μg/ml) or LP for 1 h (B1), 6 h (B2), 12 h (B3), and 24 h (B4). (C1–C4) 1 × 105 CFU of KP25826 were seeded in 96-well plates and treated with PBS, linezolid (16 μg/ml), PBNP (8 μg/ml) or LP for 1 h (C1), 6 h (C2), 12 h (C3), and 24 h (C4). The bacteria were determined as described above. Data are shown as the mean ± SEM of three independent experiments. ***p < 0.001, **p < 0.01.
LP Altered Biofilm Production and the Morphology of Klebsiella pneumoniae
K. pneumoniae predominantly form biofilms, which are extremely hard to eradicate with currently commercial antimicrobial agents. To explore whether LP treatment could alter the biofilm production of K. pneumoniae, KPWT were seeded in 24-well plates or glass tubes and treated with PBS, linezolid, PBNP or LP for 12 h. As shown in Figures 2A,B, the LP treatment could significantly reduce the biofilm production of KPWT. Next, to investigate whether LP could affect the morphology of K. pneumoniae, KPWT were cultured in 12-well plates and treated with PBS, linezolid, PBNP or LP for 12 h. Our results showed that the LP significantly affected the morphology of KPWT such as cell shrinkage or cell lysis by SEM (Figure 3D), while the morphology of KPWT in the control group or monotherapy-treated groups did not show obviously changed (Figures 3A–C).
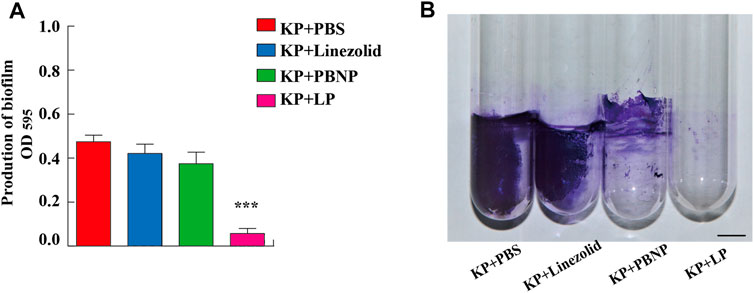
FIGURE 2. The biofilm production of K. pneumoniae treated by LP. (A,B) 1 × 106 CFU of KPWT or were seeded in 24-well plates (A) or glass tubes (B) and treated with PBS, linezolid (16 μg/ml), PBNP (8 μg/ml) or LP for 12 h. The biofilm production was detected at OD595 by microplate reader. Scale bar, 1 cm. Data are shown as the mean ± SEM of three independent experiments. ***p < 0.001.
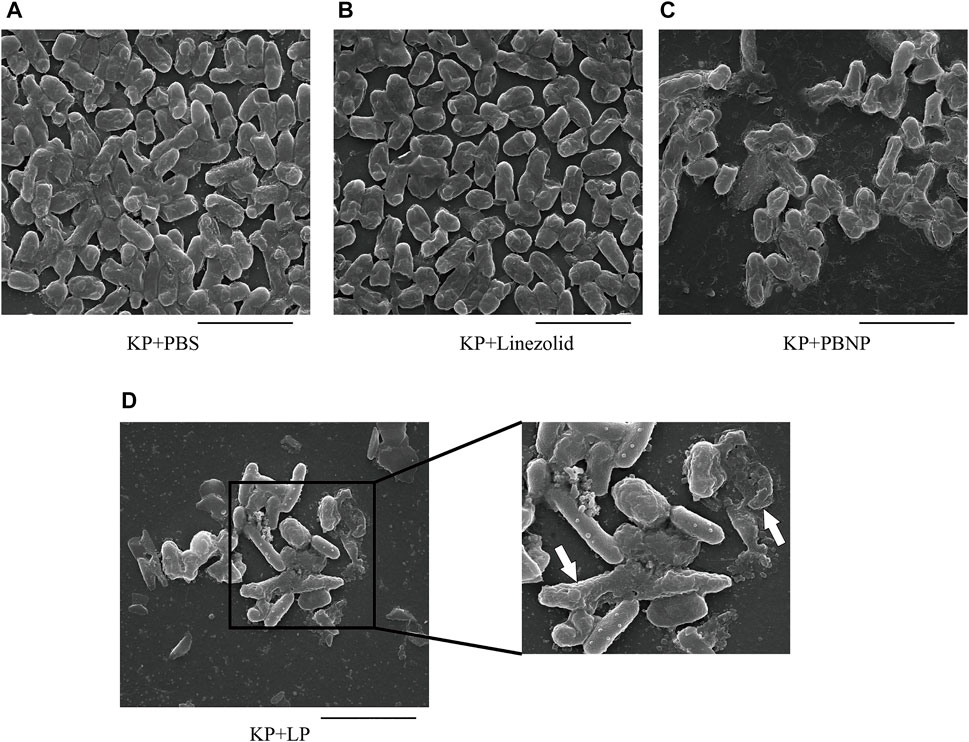
FIGURE 3. The morphology of K. pneumoniae treated by LP (A–D) 1 × 105 CFU of KPWT were seeded in 24-well plates and treated with PBS (A), linezolid (16 μg/ml) (B), PBNP(8 μg/ml) (C) or LP (D) for 12 h. The morphology of KPWT was detected by scanning electron microscopy (SEM). The destroyed KPWT is indicated by the arrow. Scale bar, 5 μm. All the experiments were performed in triplicate.
LP Reduced Bacterial Burdens in MH-S Cell Model In Vitro
As one of the most important cell types in the antibacterial immunity, macrophages are the predominant cells against bacterial infection. To define the collective role of LP against KPWT infection, we next examined the antibacterial effects of LP on KPWT in a MH-S cell model. MH-S cells were infected with KPWT for 1 h, and then treated with PBS, linezolid, PBNP or LP for 1, 6, and 12 h respectively. The results showed that the viability of KPWT in the monotherapy-treated groups were no statistically significant differences compared to the PBS group at indicated time point (Figures 4A–C). However, LP significantly increased elimination of KPWT in MH-S cells from 1 to 12 h post infection, suggesting that LP protected cells from K. pneumoniae infection.
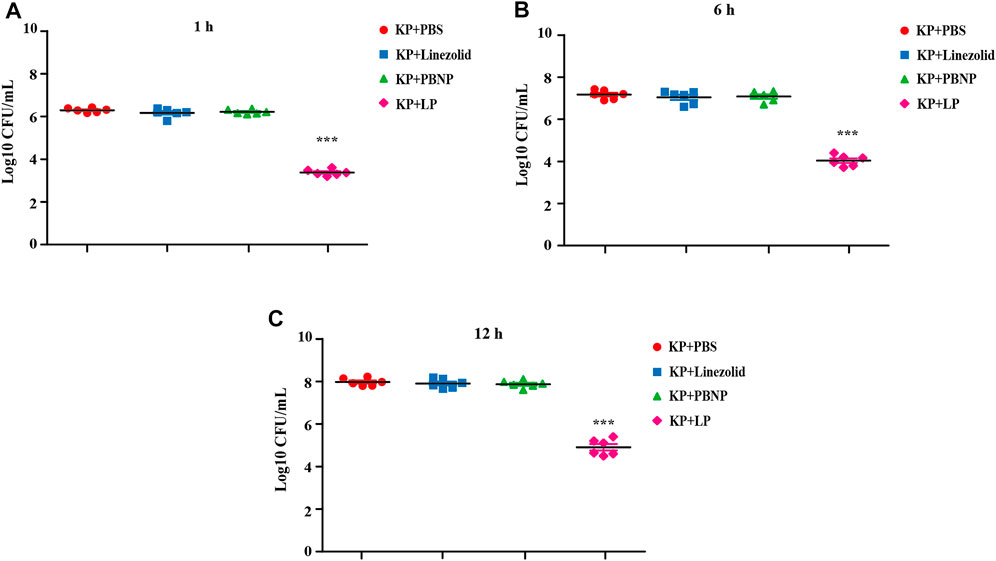
FIGURE 4. Bacterial burdens in MH-S cell infected with K. pneumoniae (A–C) The MH-S cells were infected with KPWT at an MOI of 10 for 1 h. And then treated with PBS, linezolid (16 μg/ml), PBNP (8 μg/ml) or LP for 1 h (A), 6 h (B), and 12 h (C). The bacteria burdens were determined as described above. Data are shown as the mean ± SEM of three independent experiments. ***p < 0.001.
LP Protected Caenorhabditis elegans From Klebsiella pneumoniae Infection
The nematode C. elegans is a simple and efficient model host for high-throughput screening of anti-infective agents in KP. To further investigate the antibacterial effect of LP in vivo, the C. elegans were infected with KPWT or clinical CRKP strain KP25826 and then treated different drugs. We found that large amount of bacteria from the C. elegans were recovered in the PBS, linezolid or PBNP-treated groups (Figure 5A). While, the C. elegans treated with LP displayed few bacteria inside the C. elegans at 12 and 24 h post infection (Figure 5A). As shown in Figure 5B, the PBS, linezolid or PBNP treated-C. elegans died at day 3 or day 4. However, most C. elegans survived the KP challenge at days 7 post infection by treated with LP. Importantly, most C. elegans from LP group without infection survived, suggesting LP has no obvious drug toxicity in C. elegans. To further validate the data, we also tested the antimicrobial effects of LP on clinical CRKP strain KP25826 (Figures 5C,D). Treatment with LP significantly decreased the bacterial CFU at the indicated time points compared the control group (Figure 5C) and protected C. elegans against K. pneumoniae infection (Figure 5D).
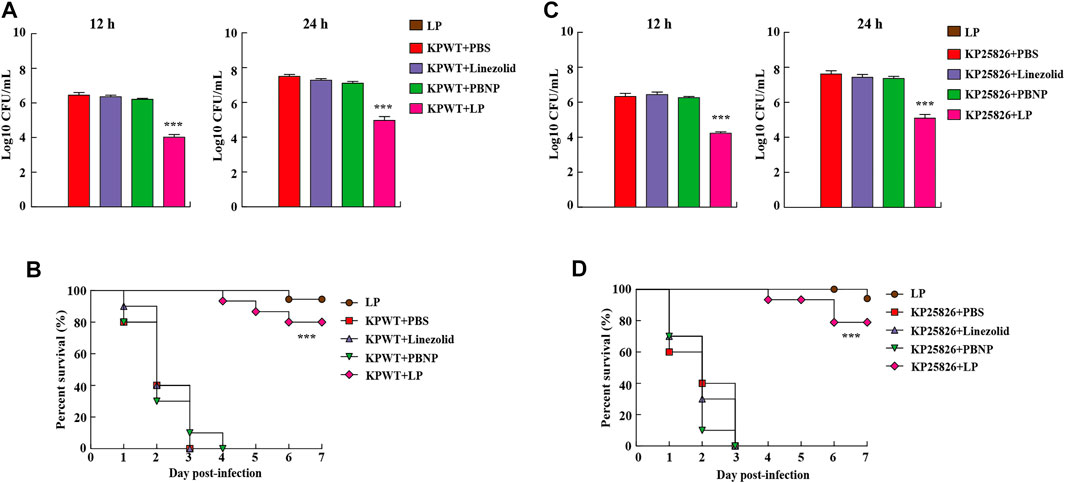
FIGURE 5. Residual CFUs and survival rate of C. elegans infected with K. pneumoniae. The C. elegans were infected at day 0 with KPWT or KP25826 and treated with PBS, linezolid (16 μg/ml), PBNP (8 μg/ml) or LP for determining bacterial burdens at post treatment 12 and 24 h (A,C). The survival rate of C. elegans was monitored for the subsequent 7 days (B,D). PBS was used as a control. ***p < 0.001.
Discussion
The MDR gram negative bacteria are becoming increasingly predominant, for which there is lack of antibacterial agents to fight them in the current antibiotic pipeline (de la Fuente-Núñez et al., 2015). For example, to treat the infections caused by the MDR-KP is difficult because of the adaptive resistance of this pathogen to most commercial antibiotics (Chapelle et al., 2021). Antibiotic combination therapy is currently applied for some severe infections caused by K. pneumoniae (Tan et al., 2020; Wistrand-Yuen et al., 2020), while useful data regarding which combinations are most potent are still limited. In the current study, we investigated the synergistic effects of polymyxin B derivatives and 5 other antibiotics against K. pneumoniae and reported the significantly positive interactions in combination of linezolid and polymyxin B nonapeptide PBNP (LP). In addition, the LP combination treatments showed excellent antibacterial activities in vitro and had protective effects against KP infections in vivo. Thus, this targeted combination provided a promising therapy strategy against KP infections such as improving spectrum of antibiotics or the treatment outcomes.
The membrane-disrupting activity of the polymyxin B, which enables the entry of a second antibacterial agent and subsequently increasing both permeability and antibacterial activity, is the critical synergism for the antibiotic combinations (Olsson et al., 2020). The absence of cross-resistance between linezolid and other protein synthesis inhibitors such as clindamycin against many MDR strains makes linezolid an attractive candidate to apply for combination treatments (Wasserman et al., 2016). To the best of our current knowledge, few studies have demonstrated the synergistic and antibacterial activities of LP against the KP in vitro and in vivo. A recent study has investigated the in vitro efficacy of linezolid in combination with polymyxin B against clinical CRKP (Wistrand-Yuen et al., 2020), while the potential toxicity of polymyxin B in vivo was probably remained. To reduce the toxicity of polymyxin B and retain its membrane-permeabilizing activity, we obtained three different derivatives of polymyxin B (PBHP, PBOP, PBNP). Additionally, we investigated the susceptibility of conventional anti-Gram-positive agents and different derivatives of polymyxin B against type strain KP or clinical CRKP in order to explore the synergistic activities of promising antibiotic combinations. Our results showed that the KP strains were resistant to the traditional anti-Gram-positive agents and polymyxin B derivatives, respectively. Nevertheless, the tested KP isolates were highly susceptible to linezolid combination of PBNP compared to other groups by checkerboard assay, suggesting that this drug combination was a potent candidate for treating KP infections. Since the combinations of LP had the lowest FIC index in all the tested group, we further investigated their antibacterial activity in the whole study.
In the current study, a significant difference was detected between monotherapy treatment and LP combination treatment against type strain KP or clinical CRKP by the time-kill assay. Although the bacteriostatic activity of PBNP was not sustained, linezolid in combination with PBNP showed the excellent antibacterial activity. These results were similar with several previous reports, in which the MDR-KP isolates were treated with polymyxin-based therapeutic combination (Pagès et al., 2015; Wistrand-Yuen et al., 2020). Although linezolid is a potent antimicrobial compound against lots of gram-positive pathogens, a recent follow-up study suggested that linezolid also exhibited the antibacterial activities against Gram-negative bacteria (Guzel Kaya et al., 2020). KP predominantly produce biofilms and can encapsulate bacteria layer by layer assembly and increase the bacterial tolerance to the current antibacterial agents (Tan et al., 2020). Interestingly, the LP combination treatment not only enhanced antibacterial activity, but also altered the biofilm production and morphology of KP, which might be effectively to prevent the colonization or spread of KP. Although the KP strains were highly insusceptible with PBNP alone, the morphology of KP was slightly altered after the PBNP alone treatment, suggesting that the bacteriostatic activity of PBNP was significantly reduced and the membrane-permeabilizing activity of PBNP was sustained. Altogether, these findings indicate that PBNP is the hopeful candidate of antibiotic potentiator and LP is probably excellent combination of antibacterial agents against KP.
To further investigate the protective effects of the LP against K. pneumoniae infection in vivo, the C. elegans were infected by KP or clinical CRKP and then treated with linezolid, PBNP alone or LP. Our results showed that the effective antibacterial activity against KP in vivo was detected by the LP combination treatment, since the linezolid and PBNP worked synergistically and the protective effects were improved as early as the second day of treatment. A very large number of bacteria were detected from the C. elegans in the monotherapy group or the control group, demonstrating that linezolid or PBNP alone could not provide the antibacterial activity in vivo. By contrast, the C. elegans treated with LP showed few bacteria, suggesting that most of bacteria were eliminated and the survival rate of the host was improved. Interestingly, treatment with LP also significantly decreased the CRKP burdens compared the control group and protected C. elegans against CRKP infection. Altogether, these data further confirmed the antibacterial activity of LP as we observed in vitro and indicated that linezolid could be the good alternative for the treatment of KP infections by combined with PBNP. Previous study showed that linezolid has activity against Gram-negative anaerobic bacteria (Yagi and Zurenko, 2003), while this study demonstrated that linezolid also has antibacterial activity against Gram-negative aerobic bacteria, which reinforced the interest in linezolid combined with polymyxin B derivatives against the other MDR Gram-negative bacteria. Thus, our data here provide evidential support that the PBNP could be an excellent potentiator in conjunction with linezolid to improve antibacterial activity and to protect the host against KP infections.
In conclusion, the combination of linezolid and PBNP showed significantly synergistic and antibacterial activities against the KP infection in vitro and in vivo. The presence of PBNP enhanced the antibacterial effects of linezolid, suggesting that PBNP could widen the therapeutic range of linezolid. Moreover, combination of the potent antibacterial agents in novel synergic formulations will provide a promising approach to inhibit the increase of bacterial resistance to the current antibiotics. Nevertheless, further studies to understand the impact of PBNP resistance are still in demand for detecting the ability of PBNP to work synergistically in combination with other available antibiotics. More importantly, we acknowledge that the low number of clinical strains examined or lack of dose-dependent investigation in LP combination treatment are the constraints of this study. Therefore, further more comprehensive or systematic study in rodents by evaluating the clinical practice of these findings may contribute to prevent the spread of K. pneumoniae infection in the long run.
Data Availability Statement
The original contributions presented in the study are included in the article/Supplementary Material, further inquiries can be directed to the corresponding authors.
Author Contributions
TH, XW, and YC designed the experiments. TH, ZL, and LZ performed the experiments. TH and KZ analyzed the data. XW, JL, and YC contributed new reagents or analytic tools. TH, XW, and YC contributed to drafting and revising the manuscript. All authors read and approved the final manuscript.
Funding
This work was supported by the Talent Introduction of Project of Chengdu University (2081918021), National Natural Science Foundation of China (No. 31900120), the National Major Scientific and Technological Special Project for “Significant New Drugs Development” (2019ZX09721001-006-002), the Sichuan Science and Technology Program (2018HH0007), the Science and Technology Bureau Program of Chengdu Municipal Government (2016-XT00-00023-GX).
Conflict of Interest
The authors declare that the research was conducted in the absence of any commercial or financial relationships that could be construed as a potential conflict of interest.
Publisher’s Note
All claims expressed in this article are solely those of the authors and do not necessarily represent those of their affiliated organizations, or those of the publisher, the editors and the reviewers. Any product that may be evaluated in this article, or claim that may be made by its manufacturer, is not guaranteed or endorsed by the publisher.
Acknowledgments
We thank Jing Zhan, Xuan Zhang, Fuxin Liu, and Mao Zeng for their technical help. We also would like to thank Dr. Zongxin Fang for providing KP25826 strain (The First People’s Hospital of Hefei, China).
References
Bachman, M. A., Breen, P., Deornellas, V., Mu, Q., Zhao, L., Wu, W., et al. (2015). Genome-Wide Identification of Klebsiella pneumoniae Fitness Genes during Lung Infection. mBio 6 (3), e00775. doi:10.1128/mBio.00775-15
Chapelle, C., Gaborit, B., Dumont, R., Dinh, A., and Vallée, M. (2021). Treatment of UTIs Due to Klebsiella pneumoniae Carbapenemase-Producers: How to Use New Antibiotic Drugs? A Narrative Review. Antibiotics 10 (11), 1332. doi:10.3390/antibiotics10111332
CLSI (2014). Performance Standards for Antimicrobial Susceptibility Testing : Twenty-Fourth Informational Supplement. CLSI Document M100-S24. Wayne, PA: Clinical and Laboratory Standards Institute.
de la Fuente-Núñez, C., Reffuveille, F., Mansour, S. C., Reckseidler-Zenteno, S. L., Hernández, D., Brackman, G., et al. (2015). D-enantiomeric Peptides that Eradicate Wild-type and Multidrug-Resistant Biofilms and Protect against Lethal Pseudomonas aeruginosa Infections. Chem. Biol. 22 (2), 196–205. doi:10.1016/j.chembiol.2015.01.002
Di, X., Wang, R., Liu, B., Zhang, X., Ni, W., Wang, J., et al. (2015). In Vitro activity of Fosfomycin in Combination with Colistin against Clinical Isolates of Carbapenem-Resistant Pseudomas Aeruginosa. J. Antibiot. (Tokyo) 68 (9), 551–555. doi:10.1038/ja.2015.27
Doi, Y., and van Duin, D. (2020). Polymyxin Resistance in Klebsiella pneumoniae: Complexity at Every Level. Clin. Infect. Dis. 70 (10), 2092–2094. doi:10.1093/cid/ciz627
Guzel Kaya, G., Medaglia, S., Candela-Noguera, V., Tormo-Mas, M. Á., Marcos, M. D., Aznar, E., et al. (2020). Antibacterial Activity of Linezolid against Gram-Negative Bacteria: Utilization of ε-Poly-l-Lysine Capped Silica Xerogel as an Activating Carrier. Pharmaceutics 12 (11), 1126. doi:10.3390/pharmaceutics12111126
Huang, T., Cui, K., Song, X., Jing, J., Lin, J., Wang, X., et al. (2019). MTOR Involved in Bacterial Elimination against Trueperella Pyogenes Infection Based on Mice Model by Transcriptome and Biochemical Analysis. Vet. Microbiol. 235, 199–208. doi:10.1016/j.vetmic.2019.06.021
Huang, T., Pu, Q., Zhou, C., Lin, P., Gao, P., Zhang, X., et al. (2020). MicroRNA-302/367 Cluster Impacts Host Antimicrobial Defense via Regulation of Mitophagic Response against Pseudomonas aeruginosa Infection. Front. Immunol. 11, 569173. doi:10.3389/fimmu.2020.569173
Kamaladevi, A., and Balamurugan, K. (2017). Global Proteomics Revealed Klebsiella pneumoniae Induced Autophagy and Oxidative Stress in Caenorhabditis elegans by Inhibiting PI3K/AKT/mTOR Pathway during Infection. Front. Cel. Infect. Microbiol. 7, 393. doi:10.3389/fcimb.2017.00393
Lawlor, M. S., Hsu, J., Rick, P. D., and Miller, V. L. (2005). Identification of Klebsiella pneumoniae Virulence Determinants Using an Intranasal Infection Model. Mol. Microbiol. 58 (4), 1054–1073. doi:10.1111/j.1365-2958.2005.04918.x
Leach, K. L., Swaney, S. M., Colca, J. R., McDonald, W. G., Blinn, J. R., Thomasco, L. M., et al. (2007). The Site of Action of Oxazolidinone Antibiotics in Living Bacteria and in Human Mitochondria. Mol. Cel. 26 (3), 393–402. doi:10.1016/j.molcel.2007.04.005
Li, J., Chen, X., Lin, J., Yuan, Y., Huang, T., Du, L., et al. (2021). Antibiotic Intervention Redisposes Bacterial Interspecific Interacting Dynamics in Competitive Environments. Environ. Microbiol. 23 (12), 7432–7444. doi:10.1111/1462-2920.15461
Li, J., Nation, R. L., Turnidge, J. D., Milne, R. W., Coulthard, K., Rayner, C. R., et al. (2006). Colistin: the Re-emerging Antibiotic for Multidrug-Resistant Gram-Negative Bacterial Infections. Lancet Infect. Dis. 6 (9), 589–601. doi:10.1016/s1473-3099(06)70580-1
Li, R., Fang, L., Tan, S., Yu, M., Li, X., He, S., et al. (2016a). Type I CRISPR-Cas Targets Endogenous Genes and Regulates Virulence to Evade Mammalian Host Immunity. Cell Res 26 (12), 1273–1287. doi:10.1038/cr.2016.135
Li, X., He, S., Li, R., Zhou, X., Zhang, S., Yu, M., et al. (2016b). Pseudomonas aeruginosa Infection Augments Inflammation through miR-301b Repression of C-Myb-Mediated Immune Activation and Infiltration. Nat. Microbiol. 1 (10), 16132. doi:10.1038/nmicrobiol.2016.132
Li, X., He, S., Zhou, X., Ye, Y., Tan, S., Zhang, S., et al. (2016c). Lyn Delivers Bacteria to Lysosomes for Eradication through TLR2-Initiated Autophagy Related Phagocytosis. Plos Pathog. 12 (1), e1005363. doi:10.1371/journal.ppat.1005363
Liu, B., Liu, Y., Di, X., Zhang, X., Wang, R., Bai, Y., et al. (2014). Colistin and Anti-gram-positive Bacterial Agents against Acinetobacter Baumannii. Rev. Soc. Bras. Med. Trop. 47 (4), 451–456. doi:10.1590/0037-8682-0081-2014
Magi, G., Marini, E., and Facinelli, B. (2015). Antimicrobial Activity of Essential Oils and Carvacrol, and Synergy of Carvacrol and Erythromycin, against Clinical, Erythromycin-Resistant Group A Streptococci. Front. Microbiol. 6, 165. doi:10.3389/fmicb.2015.00165
Olsson, A., Wistrand-Yuen, P., Nielsen, E. I., Friberg, L. E., Sandegren, L., Lagerbäck, P., et al. (2020). Efficacy of Antibiotic Combinations against Multidrug-Resistant Pseudomonas aeruginosa in Automated Time-Lapse Microscopy and Static Time-Kill Experiments. Antimicrob. Agents Chemother. 64 (6), e02111. doi:10.1128/aac.02111-19
Pagès, J. M., Peslier, S., Keating, T. A., Lavigne, J. P., and Nichols, W. W. (2015). Role of the Outer Membrane and Porins in Susceptibility of β-Lactamase-Producing Enterobacteriaceae to Ceftazidime-Avibactam. Antimicrob. Agents Chemother. 60 (3), 1349–1359. doi:10.1128/aac.01585-15
Rajeshwari, H., Nagveni, S., Oli, A., Parashar, D., and Chandrakanth, K. R. (2009). Morphological Changes of Klebsiella pneumoniae in Response to Cefotaxime: a Scanning Electron Microscope Study. World J. Microbiol. Biotechnol. 25 (12), 2263–2266. doi:10.1007/s11274-009-0126-z
Stalker, D. J., and Jungbluth, G. L. (2003). Clinical Pharmacokinetics of Linezolid, a Novel Oxazolidinone Antibacterial. Clin. Pharmacokinet. 42 (13), 1129–1140. doi:10.2165/00003088-200342130-00004
Tan, S., Gao, J., Li, Q., Guo, T., Dong, X., Bai, X., et al. (2020). Synergistic Effect of Chlorogenic Acid and Levofloxacin against Klebsiella Pneumonia Infection In Vitro and In Vivo. Sci. Rep. 10 (1), 20013. doi:10.1038/s41598-020-76895-5
Velkov, T., Roberts, K. D., Nation, R. L., Thompson, P. E., and Li, J. (2013). Pharmacology of Polymyxins: New Insights into an 'old' Class of Antibiotics. Future Microbiol. 8 (6), 711–724. doi:10.2217/fmb.13.39
Wasserman, S., Meintjes, G., and Maartens, G. (2016). Linezolid in the Treatment of Drug-Resistant Tuberculosis: the challenge of its Narrow Therapeutic index. Expert Rev. Anti Infect. Ther. 14 (10), 901–915. doi:10.1080/14787210.2016.1225498
Wistrand-Yuen, P., Olsson, A., Skarp, K. P., Friberg, L. E., Nielsen, E. I., Lagerbäck, P., et al. (2020). Evaluation of Polymyxin B in Combination with 13 Other Antibiotics against Carbapenemase-Producing Klebsiella pneumoniae in Time-Lapse Microscopy and Time-Kill Experiments. Clin. Microbiol. Infect. 26 (9), 1214–1221. doi:10.1016/j.cmi.2020.03.007
Wu, M. C., Chen, Y. C., Lin, T. L., Hsieh, P. F., and Wang, J. T. (2012). Cellobiose-specific Phosphotransferase System of Klebsiella pneumoniae and its Importance in Biofilm Formation and Virulence. Infect. Immun. 80 (7), 2464–2472. doi:10.1128/iai.06247-11
Yagi, B. H., and Zurenko, G. E. (2003). An In Vitro Time-Kill Assessment of Linezolid and Anaerobic Bacteria. Anaerobe 9 (1), 1–3. doi:10.1016/s1075-9964(03)00004-0
Ye, Y., Tan, S., Zhou, X., Li, X., Jundt, M. C., Lichter, N., et al. (2015). Inhibition of P-Iκbα Ubiquitylation by Autophagy-Related Gene 7 to Regulate Inflammatory Responses to Bacterial Infection. J. Infect. Dis. 212 (11), 1816–1826. doi:10.1093/infdis/jiv301
Zhao, K., Li, W., Li, J., Ma, T., Wang, K., Yuan, Y., et al. (2019). TesG Is a Type I Secretion Effector of Pseudomonas aeruginosa that Suppresses the Host Immune Response during Chronic Infection. Nat. Microbiol. 4 (3), 459–469. doi:10.1038/s41564-018-0322-4
Zhou, Y. F., Xiong, Y. Q., Tao, M. T., Li, L., Bu, M. X., Sun, J., et al. (2018). Increased Activity of Linezolid in Combination with Rifampicin in a Murine Pneumonia Model Due to MRSA. J. Antimicrob. Chemother. 73 (7), 1899–1907. doi:10.1093/jac/dky129
Keywords: polymyxin B nonapeptide, linezolid, synergism, Klebsiella pneumoniae, antibacterial activity
Citation: Huang T, Lv Z, Lin J, Zhao K, Zhai L, Wang X and Chu Y (2022) A Potent Antibiotic Combination of Linezolid and Polymycin B Nonapeptide Against Klebsiella pneumoniae Infection In Vitro and In Vivo. Front. Pharmacol. 13:887941. doi: 10.3389/fphar.2022.887941
Received: 02 March 2022; Accepted: 30 March 2022;
Published: 26 April 2022.
Edited by:
Mingyue Li, Wistar Institute, United StatesReviewed by:
Mingkai Li, Fourth Military Medical University, ChinaXiaozhe Xiong, Boston Children’s Hospital and Harvard Medical School, United States
Pyung-Gang Lee, Boston Children’s Hospital and Harvard Medical School, United States
Ratnakar Deole, Oklahoma State University, United States
Copyright © 2022 Huang, Lv, Lin, Zhao, Zhai, Wang and Chu. This is an open-access article distributed under the terms of the Creative Commons Attribution License (CC BY). The use, distribution or reproduction in other forums is permitted, provided the original author(s) and the copyright owner(s) are credited and that the original publication in this journal is cited, in accordance with accepted academic practice. No use, distribution or reproduction is permitted which does not comply with these terms.
*Correspondence: Ting Huang, aHVhbmd0aW5nbG92ZXNvZmlhQDE2My5jb20=; Xinrong Wang, d2FuZ3hpbnJvbmcyMDIyQDE2My5jb20=; Yiwen Chu, Y2h1eWl3ZW5AY2R1LmVkdS5jbg==