- 1Center for Infusions and Specialized Medicines of Bahia, Bahia State Health Department, Salvador, Brazil
- 2SENAI Institute of Innovation in Health Advanced Systems (ISI SAS), University Center SENAI/CIMATEC, Salvador, Brazil
- 3Gonçalo Moniz Institute, Oswaldo Cruz Foundation (FIOCRUZ), Salvador, Brazil
Inflammatory diseases have a high prevalence and has become of great interest due to the increase in life expectancy and the costs to the health care system worldwide. Chronic diseases require long-term treatment frequently using corticosteroids and non-steroidal anti-inflammatory drugs, which are associated with diverse side effects and risk of toxicity. Betulinic acid, a lupane-type pentacyclic triterpene, is a potential lead compound for the development of new anti-inflammatory treatments, and a large number of derivatives have been produced and tested. The potential of betulinic acid and its derivatives has been shown in a number of pre-clinical studies using different experimental models. Moreover, several molecular mechanisms of action have also been described. Here we reviewed the potential use of betulinic acid as a promissory lead compound with anti-inflammatory activity and the perspectives for its use in the treatment of inflammatory conditions.
Introduction
Terpenes are secondary metabolites produced naturally in plants, as a result of interactions with the environment. They are also found in mosses, algae, and lichen, and some can be also found in mammals (Zhou and Pichersky, 2020). In general, terpenes have been a valuable source of medical discoveries. A promising representative of the class is betulinic acid (BA) (Figure 1), a lupane type pentacyclic triterpene, described for the first time in 1917 (Traubenberg et al., 1917).
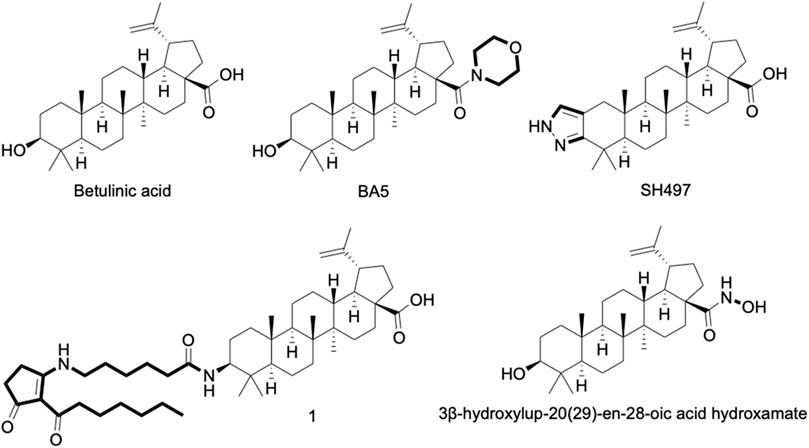
FIGURE 1. Chemical structure of betulinic acid (BA) and its derivatives with anti-inflammatory activity well-known. 1 = 3-Deoxy-3β-((6-(2-heptanoyl-3-oxocyclopent-1-en-1-yl)amino)hexanamido))betulinic acid.
BA is normally obtained from plant sources, mainly from Betula species (Hajati et al., 2018). However, the purification of BA from natural sources is a time-consuming and not environmentally friendly process that results in a low yield, making extraction methods unfeasible for a large-scale production (Eckerman and Ekman, 1985). To solve this problem, alternatively routes to obtain BA were developed, such as chemical synthesis, biotransformation using fungus cultures, and metabolic engineering biosynthetic pathways in microorganisms (Dubey and Goel, 2013; Czarnotta et al., 2017).
BA has several biological activities already described, such as diuretic, antimicrobial, antiviral, antidiabetic, antiparasitic, immunomodulatory, and anticancer activities (de-Sá et al., 2009; Oliveira-Costa et al., 2014; Jiang et al., 2021). The anticancer activity of BA is considered to be promising, since it was shown to be cytotoxic against various types of cancer cells and caused the inhibition of tumor growth in xenograft mouse models (Jiang et al., 2021).
The immunomodulatory activity of betulinic acid is also considered promising, due to its capacity to modulate several cell types of the immune system, such as macrophages and lymphocytes, and its anti-inflammatory activity was shown in different models of inflammation (Oliveira-Costa et al., 2014; Meira et al., 2017; Ou et al., 2019). In this context, this review aims to describe the main findings and mechanisms of action related to the anti-inflammatory activity of BA.
Inflammation and Natural Products
Inflammation plays an important role in resolving imbalances in the body’s homeostasis and is essential for the repair, remodeling, and renewal of different tissues under a variety of harmful conditions (Grivennikov et al., 2010). It is considered the first line of defense that protects the host against infections caused by a number of pathogens, such as bacteria, fungi, and viruses (Rock et al., 2010). In addition, other non-infectious stimuli can also trigger inflammation, such as damaged cells, chemical agents, physical injury, burns, radiation, frostbite, ischemia, and reperfusion (Chen and Nuñez, 2010; Rock et al., 2010). However, inflammation can become uncontrolled, potentially causing a range of dysfunctions, including autoimmune diseases, asthma, inflammatory bowel diseases, cardiovascular complications, among others (Sultana and Saify, 2012; Oyebanji et al., 2014; Arulselvan et al., 2016).
The inflammatory process is complex, involving cellular events, such as the migration of leukocytes (neutrophils, monocytes, basophils and eosinophils) (Huether and Mccange, 2015), the extravasation of plasma and fluids to the site of inflammation, and release of specific mediators and other signaling molecules (including eicosanoids, leukotrienes, histamine, cytokines, chemokines, platelet activating factor, free radicals derived from oxygen and nitrogen, and serotonin) by endothelial cells, resident leukocytes such as macrophages, mast cells, and dendritic cells, or by newly recruited cells (Anwikar and Bhitre, 2010).
Classically, treatment of inflammation is done with non-steroidal anti-inflammatory drugs and steroids, in an attempt to improve the patient’s symptoms (Gupta and Dubois, 2002). Although effective in treating inflammation to varying degrees, both drug classes have a range of undesirable side effects, such as gastrointestinal disturbances, cardiac changes, renal toxicity, hypertension, type 2 diabetes, visceral obesity, and atherosclerosis (Chapmann et al., 2013; Harirforoosh et al., 2013; Sostres et al., 2013).
Thus, the development of new substances with anti-inflammatory activity is of great importance for clinical use, in order to obtain alternative treatments with efficacy and fewer adverse effects. A widely used strategy has been the prospection of molecules with anti-inflammatory potential in medicinal plants, given that plants have a vast diversity of molecules that are still largely unknown (Newman and Cragg, 2016). The pharmacological activities of natural products have been widely reported in the literature, including anti-inflammatory activity, since the discovery of salicylic acid by Stone in 1763. Subsequently, a large number of molecules with anti-inflammatory activity has been identified, many of them from plants used in folk medicine, and others due to random bioprospection.
Among the natural compounds with anti-inflammatory activity, betulinic acid was reported for the first time by Recio et al., in 1995. Subsequently, its pharmacological properties continued to be widely reported in the scientific literature due to its diverse biological activities, in addition to anti-inflammatory actions in different models (Nader, 2012; Oliveira-Costa et al, 2014).
In Vitro Anti-Inflammatory Activity of BA
BA has been shown to modulate the activity of several cell types and molecules involved in the inflammatory response (Table 1; Figure 2). A critical inflammatory mediator is nitric oxide (NO), produced from l-arginine by the action of the enzyme inducible nitric oxide synthase (iNOS) (Förstermann and Sessa, 2012). If produced in excess, NO leads to the development of various inflammatory diseases, such as arthritis, inflammatory bowel disease, and multiple sclerosis (Sharma et al., 2007). Thus, inhibition of iNOS and/or NO production can be assessed to evaluate anti-inflammatory properties. Interestingly, several in vitro studies have shown that BA can inhibit the production of NO, mainly in macrophages cultures stimulated with bacterial lipopolysaccharide (LPS) and/or interferon gamma (IFN-ɣ) (Yun et al., 2003; Blaževski et al., 2013; Oliveira-Costa et al., 2014; Jingbo et al., 2015; Kim et al., 2016; Meira et al., 2017; Ryu et al., 2020).
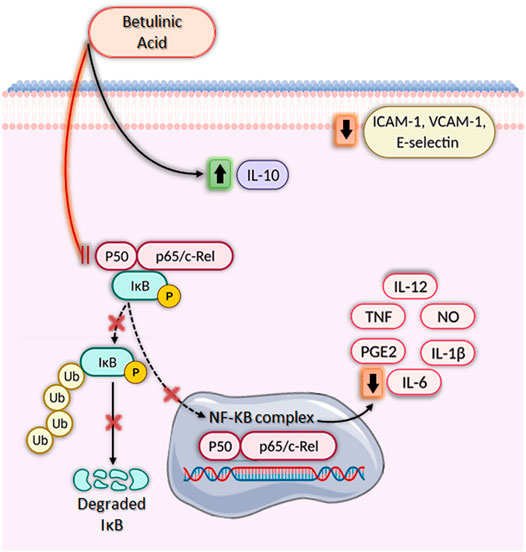
FIGURE 2. Immunomodulatory activities of betulinic acid in vitro. Betulinic acid (BA) has a broad-spectrum anti-inflammatory activity, significantly increasing IL-10 production, decreasing ICAM-1, VCAM-1, and E-selectin expression and inhibiting nuclear factor kappa-light-chain-enhancer of activated B cells (NF-κB), leading to downregulation of several pro-inflammatory genes. BA blocks the NF-κB signaling pathway by inhibiting IκB phosphorylation and degradation through ubiquitination via the proteasome degradation machinery. As a result, NF-κB is not activated and does not translocate from the cytoplasm to the nucleus, impeding the transcription of pro-inflammatory mediators such as IL-1β, IL-6, IL-12, NO, PGE2, and TNF. Created with BioRender.com.
Furthermore, BA also inhibits cyclooxygenase-2 (COX-2) activity and, therefore, decrease prostaglandin E2 (PGE2) synthesis (Dustan et al., 1998; Jalil et al., 2015; Jingbo et al., 2015; Kim et al., 2016). PGE2 is responsible for inflammatory symptoms, such as fever, pain, and platelet aggregation, and thus measuring the reduction of PGE2 production is an attractive strategy for the discovery of anti-inflammatory drugs (Park et al., 2006). The production of critical pro-inflammatory cytokines, such as IL-1β, IL-6, IL-8, IL-12, and TNF, is also decreased by BA treatment (Oliveira-Costa et al., 2014; Kim et al., 2016; Meira et al., 2017; Li et al., 2019). Most of these effects are related to the inhibition of nuclear factor kappa-light-chain-enhancer of activated B cells (NF-κB), a transcription factor involved in the regulation of several pro-inflammatory genes (Figure 1) (Yoon et al., 2010; Jingbo et al., 2015; Kim et al., 2016; Meira et al., 2017; Li et al., 2019). Inhibition of NF-κB activation by BA also decreases the expression of adhesion molecules, such as ICAM-1, VCAM-1, and E-selectin, in endothelial cells, which may have implications for the treatment of vascular inflammation (Yoon et al., 2010). Interestingly, the effects of BA on NF-κB pathway is potentiated by the presence of inhibitors of mitogen activated protein kinases (MAPK), such as SB203580 (p38 inhibitor) and PD98059 (extracellular signal-regulated kinase inhibitor) (Viji et al., 2011). Kim et al. (2016) also demonstrated that induction of HO-1 enzyme activity is associated with the anti-inflammatory effect of BA, since SnPP, an inhibitor of HO-1, promoted a partial reversal of BA’s effect on NF-κB activity, as well as on IL-1β, IL-6, NO, PGE2, TNF, IL-1β, and IL-6 production. In addition to the reduced production of pro-inflammatory molecules, BA also increased the amount of IL-10, a well-known anti-inflammatory cytokine (Oliveira-Costa et al., 2014; Meira et al., 2017). Lastly, BA also modulated lymphocyte function through the inhibition of lymphoproliferation, decreasing the production of pro-inflammatory cytokines, such as IL-2, IL-6, IL-17, and IFN-γ (Blaževski et al., 2013; Meira et al., 2017).
Anti-Inflammatory Activity of BA in Vivo
The anti-inflammatory effects of BA have also been validated in various animal models (Table 2). The initial work by Recio et al. (1995) tested BA, isolated from the leaves of Diospyros leucomelas, in three mouse model of inflammation: 12-O-tetradecanoylphorbol acetate (TPA)-induced ear edema, carrageenan-induced paw edema and ethyl phenylpropiolate (EPP)-induced ear edema, all models using Swiss mice. BA, administered topically at 0.5 mg/kg, induced a reduction in TPA edema and EEP edema. In addition, BA administered orally at 100 mg/kg, alsos promoted a reduction in paw edema induced by carrageenan (Recio et al., 1995). Further studies confirmed the anti-inflammatory activity of BA in carrageenan-induced edema, in doses ranging from 10 to 100 mg/kg, mainly by oral route (Mukherjee et al., 1997; Tsai et al., 2011; Oyebanji et al., 2014; Armah et al., 2015; Ou et al., 2019). Importantly, when administered by intraperitoneal route, BA also reduced carrageenan-induced paw edema in Wistar rats (Oyebanji et al., 2014). In addition, Oyebanji et al. (2014) observed a reduction in carrageenan-induced-pulmonary edema in Wistar rats treated intraperitoneally with BA at 10, 20 or 40 mg/kg.
The main mechanism associated with carrageenan-induced inflammation is well characterized and involves the reduction of inflammatory mediators such as COX-2, Il-1β, NO, PGE2, and TNF (Salvemini et al., 1996; Tsai et al., 2011). In addition, the inhibition of antioxidant enzymes, lipid peroxidation, and production of free radicals, such as hydrogen peroxide, superoxide, and hydroxyl radical in the liver, are common features in carrageenan-induced inflammation related to cell injury (Cuzzocrea et al., 1999). Interestingly, treatment with BA decreased the production of the inflammatory mediators described above at the inflammation site and increased enzyme activity of superoxide dismutase (SOD), glutathione peroxidase (GPx), and glutathione reductase (GRd) in the liver (Tsai et al., 2011; Ou et al., 2019). Moreover, BA decreased malondialdehyde (MDA) levels, a key mediator of oxidative stress and widely used as a marker of free radical mediated lipid peroxidation injury, at the inflammation site (Tsai et al., 2011). Lastly, Ou et al. (2019) provided evidence that BA downregulates MAPK signaling pathways (ERK1/2, JNK, and p38) in the paw edema tissue, which, in part, explains the inhibition of cytokine production (IL-1β and TNF), COX-2 expression, and PGE2 production (Figure 3).
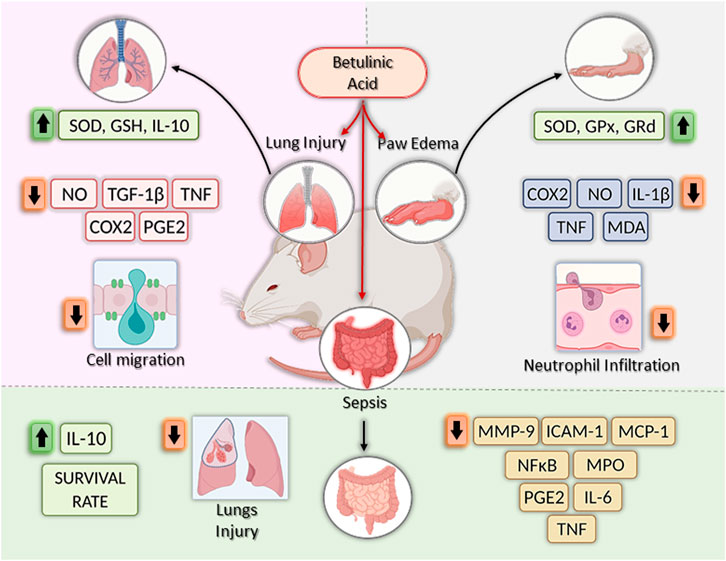
FIGURE 3. Effects of betulinic acid in vivo models. Betulinic acid (BA) reduced lung inflammation by increasing levels of GSH, SOD and IL-10, by inhibiting the expression of proinflammatory mediators, such as NO, TGF-β1, TNF, COX-2, and PGE2, and by reducing cell migration to the lesion site. In the paw edema models, BA treatment increased the activities of antioxidant enzymes (SOD, GPx and GRd), reduced COX-2, IL-1β, NO, TNF, MDA, and TNF, as well as neutrophil infiltration, leading to the reduction of swelling in the paw. Finally, in sepsis models, BA treatment increase survival rate by decreasing levels of MMP-9, ICAM-1, MCP-1, PGE2, IL-6, and TNF, reducing lung injury and decreasing MPO and NF-κB activity. In addition, increase IL-10 production. Created with BioRender.com.
Another model in which BA was well explored is sepsis, which can be induced by LPS injection or cecal ligation and puncture (CLP), both models useful in preclinical trials to screen new anti-inflammatory agents (Viji et al., 2010; Oliveira-Costa et al, 2014; Lingaraju et al., 2015a; Lingaraju et al., 2015b). Initially, BA (20 mg/kg) was tested by intraperitoneal route, in a model of endotoxic shock-induced by LPS in Swiss mice (Viji et al., 2010). BA increased the survival rate of animals, and 40% of the mice pretreated with BA remained alive after the observation time (7 days). In addition, BA reduced PGE2 production and myeloperoxidase (MPO) activity, an index of neutrophil infiltration, in liver and lung tissue (Viji et al., 2010). Using a similar model, Oliveira-Costa et al. (2014) using a higher dose of BA (67 mg/kg) observed a more pronounced protective effect (100% survival rate) in BALB/c mice treated intraperitoneally with BA and challenged with a lethal dose of LPS. Moreover, the protective effect was accompanied by a reduction of the pro-inflammatory cytokine TNF and an increase in the anti-inflammatory cytokine IL-10 in the sera of BA-treated mice, as well as in cultures of peritoneal macrophages obtained from animals treated with BA (67 mg/kg). Using IL-10 deficient C57BL/6 mice, the role of IL-10 in the BA-induced protection against LPS challenge was confirmed, since BA did not protect mice in the absence of IL-10 (Costa et al., 2014). Since IL-10 was shown to be produced by activation of the NF-kB pathway (Saraiva and O´Garra, 2010), and due to the effect of BA in reducing NF-kB activation, the mechanisms leading to the increase in IL-10 production induced by BA still remains to be determined. BA also promoted the increase in survival rate in a sepsis model induced by CLP, in mice pretreated intraperitoneally with BA (Lingaraju et al., 2015a).
Importantly, BA prevented lung injury by decreasing the production of IL-6, MCP-1, MMP-9, TNF, and MPO activity, all these effects related to inhibition of NF-κB activation (Lingaraju et al., 2015a). Interestingly, using the same model, Lingaraju et al. (2015b) also demonstrated that BA reduced lung injury induced by sepsis, at least in part, through its ability to balance oxidant-antioxidant status and to inhibit neutrophil infiltration (Lingaraju et al., 2015b). In accordance with these data, oral administration of BA at 25 mg/kg also reduced lung inflammation induced by LPS in Sprague-Dawley rats (Nader et al., 2012).
In a model of ulcerative colitis (UC) caused by dextran sulfate sodium (DSS) (Matos et al., 2013), BA decreased oxidative stress, production of some inflammatory factors, and visceral pain, fundamental aspect to intestinal bowel diseases (IBD) therapy (Kalra et al., 2018). Thus, while control group had loss in body weight, loose stool consistency, and gross rectal bleeding at end of experiment, the BA oral administration showed improvement of symptoms. BA (at 10 and 30 mg/kg) was able to decrease the bleeding score and augment the stool consistency (at 30 mg/kg), when compared to the control. The disease activity index (DAI) was significantly lower in BA-treated group (10 and 30 mg/kg), if compared to DSS group. BA also reduced colon shortening, observed in the DSS control group. If considered oxidative stress markers, BA was able to reduce nitrite and serum lipid hydroperoxide levels. While the DSS group had colon malondialdehyde (MDA), nitrite levels and serum lipid hydroperoxide increased, to the BA group, this oxidative stress markers were reduced. While in the DSS group was observed an anti-oxidants like superoxide dismutase (SOD), catalase and reduced glutathione (GSH) reduction, BA treatment normalized these parameters, showing protection. BA treatment (30 mg/kg) also inhibited MPO, MMP-9, and PGE2. Moreover, histopathological analyses showed that BA ameliorates mucosa destruction and inflammatory changes, with improving of histological aspects (Kalra et al., 2018).
BA acts on acetic acid-induced writhing (Mohammad et al., 2012; Qu et al., 2015) and mustard oil-induced visceral nociception (Laird et al., 2001), producing an effect comparable to that of etoricoxib, if administered 1 hour before challenge, inhibiting the writhing response and suppressing pain response, in a dose-dependent way (Kalra et al., 2018). BA at 10 and 30 mg/kg was able to protect mice against MO-induced plasma extravasation in colon and death, showing to be an effective molecule in this model of inflammation and analgesia. BA have protective effects in DSS-induced colitis and antinociceptive capacity in an experimental visceral pain model, being a promising agent to the IBD treatment (Kalra et al., 2018).
BA showed a toxicity at 30 mg/kg dose, inducing the increase in ALT levels, indicating the use of lower doses for in vivo experiments, as performed by Zhou (2021), which tested BA in a model using cerulein-induced acute pancreatitis (AP). Pre-treatment with BA is able to decrease pancreatic damage, in analyses observing reduction in acinar cell death, pancreatic edema, inflammatory cell infiltration and pancreatic myeloperoxidase (MPO) activity, reflecting reduction of neutrophil sequestration. Prior administration of BA reduced the levels of amylase and lipase, increased in acute pancreatitis. Lung injury, a common manifestation in acute pancreatitis, was reduced by pretreatment with BA in the acute pancreatitis model. Additionally, BA administration reduced the expression of IL-1, IL-6, and TNF mRNA and proteins in the pancreas, increased in animals with AP. COX-2 mRNA expression, increased in AP, was reduced by BA pretreatment during cerulein-induced pancreatitis. Moreover, prevention of cell death and production of proinflammatory cytokines by pancreatic acinar cells (PAC’s) is observed in BA-treated animals, in a dose-dependent manner. Macrophage and neutrophil pancreas infiltration were also reverted by BA treatment. Finally, in the acute pancreatitis model, BA was also shown to modulate NF-κB activation and mitogen activated protein kinase (MAPK), inhibiting Iκ-Bα degradation, NF-κB p65 translocation into the nucleus. and NF-κB binding activity, without inhibiting the phosphorylation of P38, c-JUN N-terminal kinase (JNK), and extracellular signal-regulated kinases (ERK) (Zhou et al., 2021).
Rheumatoid arthritis (RA) is an important joint disease, immune-mediated condition, with a chronic inflammation associated to progressive synovial hyperplasia and destruction of bone cartilage. Strong evidence indicates the participation of fibroblast-like synoviocytes (FLS’s) in the synovial inflammation and joint erosion as an important player in the process. Activated FLS in RA possess a tumor-like property and different biological characteristics, such as anchorage-independent growth, aggressive migration and invasion, and overexpression of pro-inflammatory cytokines. Although BA at 10 μM did not affect the cell viability of RA FLS, it was not only able to inhibit the migration of RA FLS but also suppressed its invasive ability. Moreover, BA reduced the organization of actin stress fibers and cytoskeleton score, and reduced mRNA expression of IL-1β, IL-6, IL-8, IL-17A, as well as NF-κB nuclear accumulation. Treatment in vivo with BA suppressed the clinical manifestations of RA, characterized by reduction of synovitis, synovial hyperplasia, and invasion into calcified cartilages and bone. These observations reinforce the potential of BA to inhibit the progress of RA (Li et al., 2019).
Finally, Huimin et al. (2019), studying the pharmacological activity of BA on adjuvant-induced arthritis model in rats, showed that this compound has protective effects. BA treatment reduced the arthritis index, improved joint pathology, reduced toe swelling, improved blood rheology, decreased synovial cell apoptosis, and normalized the production of inflammatory cytokines, also acting through the modulation of ROCK/NF-kB pathways.
BA as a Prototype for New Anti-Inflammatory Agents
In addition to having its anti-inflammatory activity described in several experimental models, BA is also considered a promising prototype for the development of more active anti-inflammatory agents (Figure 1) (Meira et al., 2017). Structural changes in the substituents of C-3, C-20 and C-28 of BA were shown (Kim et al., 1998). Modifications in BA structure carried out in C-28 have already contributed to the optimization of compounds with anti-HIV, antitumor, anti-influenza A, and anti-herpes activities (Sun et al., 1998; Jeong et al., 1999; Baltina et al., 2003; Pavlova et al., 2003).
BA5, a semi-synthetic amide derivative of BA, showed a promising anti-inflammatory activity. Activated macrophage cultures produced less NO, TNF and NF-κB activity when incubated in the presence of BA5. Similarly, this compound showed a potent inhibitory effect in activated lymphocyte cultures, inhibiting their proliferation and IL-2 secretion in a concentration-dependent manner. In addition, BA5 showed a protective effect against a lethal dose of LPS in a mouse model of endotoxic shock and decreased edema formation in a delayed-type hypersensitivity model induced by bovine serum albumin (Meira et al., 2017). Interestingly, BA5 (1 and 10 mg/kg) given by oral administration decreased heart inflammation and fibrosis in a C57BL/6 model of chronic cardiomyopathy caused by Chagas disease (Meira et al., 2019). These effects were accompanied by a reduction of pro-inflammatory cytokines, such as IFN-γ and TNF, as well as by the increase in IL-10 production. Importantly, BA5 promoted polarization to anti-inflammatory/M2 macrophage phenotype evidenced by the increase in M2 markers, such as arginase one and chitinase-3-like protein one and a decrease in the expression of nitric oxide synthase two and proinflammatory cytokines, in Chagasic mice treated with BA5 (Meira et al., 2019).
Another promising compound is the heterocyclic ring-fused BA derivative, SH479, which showed potent anti-inflammatory effect in a model of collagen-induced arthritis, acting by inducing a shift in pathogenic Th17/Th1 response to a Th2/Treg phenotype. Moreover, an additional articular bone protection effect was seen in SH479-treated animals (Chen et al., 2017). A series of betulinic acid-azaprostanoid hybrids also showed anti-inflammatory activity in a mouse model of paw edema-induced by concanavalin A (Khlebnicova et al., 2019).
Lastly, a hydroxamate of betulinic acid prevented colon inflammation and fibrosis in TNBS- and DSS-induced inflammatory bowel disease models (Prados et al., 2020). In addition, this compound promoted a significant reduction of fibrosis markers, such as tenascin C, collagen type I alpha two chain, collagen type III alpha one chain, TIMP metallopeptidase inhibitor one and alpha smooth muscle actin, as well as inflammatory markers (F4/80+, CD3+, Il-1β, Ccl3), in colon tissue samples (Padros et al., 2021).
Concluding Remarks
Betulinic acid proved to be a versatile molecule, able to modulate a number of key mediators in the inflammatory process, including COX-2, ICAM-1, IL-1β, IL-6, IL-12, MCP-1, PGE2, and TNF, both in vitro and in vivo, in different disease models (Figures 2, 3). Most of these effects related to inhibition of NF-kB and MAPK pathways. Importantly, BA promotes the production of IL-10, a critical anti-inflammatory mediator able to modulate several immune cell types (Saraiva and O’Garra, 2010). Moreover, BA can be produced by synthetic routes and its structural changes have generated more potent and selective derivatives, making its use as a prototype for the generation of new classes of anti-inflammatory drugs promising. In order to develop BA-based treatments, there is a need for toxicological, as well as clinical studies that will demonstrate the safety and efficacy of this compound in inflammatory and immune-mediated diseases.
Author Contributions
JO-C and CM designed the study and wrote the manuscript. BR and MN conceived the artwork and performed the bibliographical research. MS supervised the writing. All the authors revised and approved the final version of the manuscript.
Funding
This work was supported by grants from PRONEX (grant number 0002/2014).
Conflict of Interest
The authors declare that the research was conducted in the absence of any commercial or financial relationships that could be construed as a potential conflict of interest.
Publisher’s Note
All claims expressed in this article are solely those of the authors and do not necessarily represent those of their affiliated organizations, or those of the publisher, the editors and the reviewers. Any product that may be evaluated in this article, or claim that may be made by its manufacturer, is not guaranteed or endorsed by the publisher.
References
Anwikar, S., and Bhitre, M. (2010). Study of the Synergistic Anti-inflammatory Activity of Solanum Xanthocarpum Schrad and Wendl and Cassia Fistula LinnSolanum Xanthocarpum Schrader and Wendl and Cassia Fistula Linn. International Journal. Int J Ayurveda ResAyurveda Res. 1, 167–171. doi:10.4103/0974-7788.72489
Armah, F. A., Annan, K., Mensah, A. Y., Amponsah, I. K., Tocher, D. A., and Habtemariam, S. (2015). Erythroivorensin: A Novel Anti-inflammatory Diterpene from the Root-Bark of Erythrophleum Ivorense (A Chev.). Fitoterapia 105, 37–42. doi:10.1016/j.fitote.2015.06.001
Arulselvan, P., Fard, M. T., Tan, W. S., Gothai, S., Fakurazi, S., Norhaizan, M. E., et al. (2016). Roles of Antioxidants and Natural Products in Inflammation. Oxid Med Cell Longev. 2016, 5276130. doi:10.1155/2016/5276130
Baltina, L. A., Flekhter, O. B., Nigmatullina, L. R., Boreko, E. I., Pavlova, N. I., Nikolaeva, S. N., et al. (2003). Lupane Triterpenes and Derivatives with Antiviral Activity. Bioorg Med. Chem. Lett. 13, 3549–3552. doi:10.1016/s0960-894x(03)00714-5
Blaževski, J., Petković, F., Momčilović, M., Paschke, R., Kaluđerović, G. N., Mostarica Stojković, M., et al. (2013). Betulinic Acid Regulates Generation of Neuroinflammatory Mediators Responsible for Tissue Destruction in Multiple Sclerosis In Vitro. Acta Pharmacol. Sin. 34, 424–431. doi:10.1038/aps.2012.181
Chapman, K. E., Coutinho, A. E., Zhang, Z., Kipari, T., Savill, J. S., and Seckl, J. R. (2013). Changing Glucocorticoid Action: 11β-Hydroxysteroid Dehydrogenase Type 1 in Acute and Chronic Inflammation. J. Steroid Biochem. Mol. Biol. 137, 82–92. doi:10.1016/j.jsbmb.2013.02.002
Chen, G. Y., and Nuñez, G. (2010). Sterile Inflammation: Sensing and Reacting to Damage. Nat. Rev. Immunol. 10, 826–837. doi:10.1038/nri2873
Chen, S., Bai, Y., Li, Z., Jia, K., Jin, Y., He, B., et al. (2017). A Betulinic Acid Derivative SH479 Inhibits Collagen-Induced Arthritis by Modulating T Cell Differentiation and Cytokine Balance. Biochem. Pharmacol. 126, 69–78. doi:10.1016/j.bcp.2016.12.006
Cuzzocrea, S., Costantino, G., Zingarelli, B., Mazzon, E., Micali, A., and Caputi, A. P. (1999). The Protective Role of Endogenous Glutathione in Carrageenan-Induced Pleurisy in the Rat. Eur. J. Pharmacol. 372, 187–197. doi:10.1016/s0014-2999(99)00200-9
Czarnotta, E., Dianat, M., Korf, M., Granica, F., Merz, J., Maury, J., et al. (2017). Fermentation and Purification Strategies for the Production of Betulinic Acid and its Lupane-type Precursors in Saccharomyces cerevisiae. Biotechnol. Bioeng. 114, 2528–2538. doi:10.1002/bit.26377
de Sá, M. S., Costa, J. F., Krettli, A. U., Zalis, M. G., Maia, G. L., Sette, I. M., et al. (2009). Antimalarial Activity of Betulinic Acid and Derivatives In Vitro against Plasmodium Falciparum and In Vivo in P. Berghei-Infected Mice. Parasitol. Res. 105, 275–279. doi:10.1007/s00436-009-1394-0
Dubey, K. K., and Goel, N. (2013). Evaluation and Optimization of Downstream Process Parameters for Extraction of Betulinic Acid from the Bark of Ziziphus Jujubae L. ScientificWorldJournal 2013, 469674. doi:10.1155/2013/469674
Dunstan, C., Liu, B., Welch, C. J., Perera, P., and Bohlin, L. (1998). Alphitol, a Phenolic Substance from Alphitonia Zizyphoides Which Inhibits Prostaglandin Biosynthesis In Vitro. Phytochemistry 48, 495–497. doi:10.1016/s0031-9422(97)00827-3
Eckerman, C., and Ekman, R. (1985). Comparison of Solvents for Extraction and Crystallization of Betulinol from Birch Bark Waste. Pap. ja Puu 67, 100
Forstermann, U., and Sessa, W. C. (2012). Nitric Oxide Synthases: Regulation and Function. Eur. Heart J. 33, 829–837. doi:10.1093/eurheartj/ehr304
Grivennikov, S. I., Greten, F. R., and Karin, M. (2010). Immunity, Inflammation, and Cancer. Cell 140, 883–899. doi:10.1016/j.cell.2010.01.025
Gupta, R. A., and Dubois, R. N. (2001). Colorectal Cancer Prevention and Treatment by Inhibition of Cyclooxygenase-2. Nat. Rev. Cancer 1, 11–21. doi:10.1038/35094017
Harirforoosh, S., Asghar, W., and Jamali, F. (2013). Adverse Effects of Nonsteroidal Antiinflammatory Drugs: an Update of Gastrointestinal, Cardiovascular and Renal Complications. J. Pharm. Pharm. Sci. 16, 821–847. doi:10.18433/j3vw2f
Huether, S. E., and Mccance, K. L. (2015). Understanding Pathophysiology. Förlag: Elsevier Health Sciences.
Huguet, A., del Carmen Recio, M., Máñez, S., Giner, R., and Ríos, J. (2000). Effect of Triterpenoids on the Inflammation Induced by Protein Kinase C Activators, Neuronally Acting Irritants and Other Agents. Eur. J. Pharmacol. 410, 69–81. doi:10.1016/s0014-2999(00)00860-8
Huimin, D., Hui, C., Guowei, S., Shouyun, X., Junyang, P., and Juncheng, W. (2019). Protective Effect of Betulinic Acid on Freund's Complete Adjuvant-Induced Arthritis in Rats. J. Biochem. Mol. Toxicol. 33, e22373. doi:10.1002/jbt.22373
Jafari Hajati, R., Payamnoor, V., Ahmadian Chashmi, N., and Ghasemi Bezdi, K. (2018). Improved Accumulation of Betulin and Betulinic Acid in Cell Suspension Culture of Betula Pendula Roth by Abiotic and Biotic Elicitors. Prep. Biochem. Biotechnol. 48, 867–876. doi:10.1080/10826068.2018.1514514
Jalil, J., Sabandar, C. W., Ahmat, N., Jamal, J. A., Jantan, I., Aladdin, N. A., et al. (2015). Inhibitory effect of triterpenoids from Dillenia serrata (Dilleniaceae) on prostaglandin e2 production and quantitative HPLC analysis of its koetjapic acid and betulinic acid contents. Molecules 20, 3206–3220. doi:10.3390/molecules20023206
Jeong, H. J., Chai, H. B., Park, S. Y., and Kim, D. S. (1999). Preparation of Amino Acid Conjugates of Betulinic Acid with Activity against Human Melanoma. Bioorg Med. Chem. Lett. 9, 1201–1204. doi:10.1016/s0960-894x(99)00165-1
Jiang, W., Li, X., Dong, S., and Zhou, W. (2021). Betulinic Acid in the Treatment of Tumour Diseases: Application and Research Progress. Biomed. Pharmacother. 142, 111990. doi:10.1016/j.biopha.2021.111990
Jingbo, W., Aimin, C., Qi, W., Xin, L., and Huaining, L. (2015). Betulinic Acid Inhibits IL-1β-induced Inflammation by Activating PPAR-γ in Human Osteoarthritis Chondrocytes. Int. Immunopharmacol. 29, 687–692. doi:10.1016/j.intimp.2015.09.009
Kalra, J., Lingaraju, M. C., Mathesh, K., Kumar, D., Parida, S., Singh, T. U., et al. (2018). Betulinic Acid Alleviates Dextran Sulfate Sodium-Induced Colitis and Visceral Pain in Mice. Naunyn Schmiedeb. Arch. Pharmacol. 391, 285–297. doi:10.1007/s00210-017-1455-3
Khlebnicova, T. S., Piven, Y. A., Lakhvich, F. A., Sorokina, I. V., Frolova, T. S., Baev, D. S., et al. (2019). Betulinic Acid-Azaprostanoid Hybrids: Synthesis and Pharmacological Evaluation as Anti-inflammatory Agents. Antiinflamm. Antiallergy Agents Med. Chem. 19, 254–267. doi:10.2174/1871523018666190426152049
Kim, D. S., Pezzuto, J. M., and Pisha, E. (1998). Synthesis of Betulinic Acid Derivatives with Activity against Human Melanoma. Bioorg Med. Chem. Lett. 8, 1707–1712. doi:10.1016/s0960-894x(98)00295-9
Kim, K. S., Lee, D. S., Kim, D. C., Yoon, C. S., Ko, W., Oh, H., et al. (2016). Anti-inflammatory Effects and Mechanisms of Action of Coussaric and Betulinic Acids Isolated from diospyros Kaki in Lipopolysaccharide-Stimulated RAW 264.7 Macrophages. Molecules 21, 1206. doi:10.3390/molecules21091206
Laird, J. M., Martinez-Caro, L., Garcia-Nicas, E., and Cervero, F. (2001). A New Model of Visceral Pain and Referred Hyperalgesia in the Mouse. Pain 92, 335–342. doi:10.1016/S0304-3959(01)00275-5
Li, N., Gong, Z., Li, X., Ma, Q., Wu, M., Liu, D., et al. (2019). Betulinic Acid Inhibits the Migration and Invasion of Fibroblast-like Synoviocytes from Patients with Rheumatoid Arthritis. Int. Immunopharmacol. 67, 186–193. doi:10.1016/j.intimp.2018.11.042
Lingaraju, M. C., Pathak, N. N., Begum, J., Balaganur, V., Bhat, R. A., Ram, M., et al. (2015a). Betulinic Acid Negates Oxidative Lung Injury in Surgical Sepsis Model. J. Surg. Res. 193, 856–867. doi:10.1016/j.jss.2014.09.008
Lingaraju, M. C., Pathak, N. N., Begum, J., Balaganur, V., Bhat, R. A., Ramachandra, H. D., et al. (2015b). Betulinic Acid Attenuates Lung Injury by Modulation of Inflammatory Cytokine Response in Experimentally-Induced Polymicrobial Sepsis in Mice. Cytokine 71, 101–108. doi:10.1016/j.cyto.2014.09.004
Máñez, S., Recio, M. C., Giner, R. M., and Ríos, J. L. (1997). Effect of Selected Triterpenoids on Chronic Dermal Inflammation. Eur. J. Pharmacol. 334, 103–105. doi:10.1016/s0014-2999(97)01187-4
Matos, I., Bento, A. F., Marcon, R., Claudino, R. F., and Calixto, J. B. (2013). Preventive and Therapeutic Oral Administration of the Pentacyclic Triterpene α,β-amyrin Ameliorates Dextran Sulfate Sodium-Induced Colitis in Mice: the Relevance of Cannabinoid System. Mol. Immunol. 54, 482–492. doi:10.1016/j.molimm.2013.01.018
Meira, C. S., Espírito Santo, R. F. D., Dos Santos, T. B., Orge, I. D., Silva, D. K. C., Guimarães, E. T., et al. (2017). Betulinic Acid Derivative BA5, a Dual NF-kB/calcineurin Inhibitor, Alleviates Experimental Shock and Delayed Hypersensitivity. Eur. J. Pharmacol. 815, 156–165. doi:10.1016/j.ejphar.2017.09.008
Meira, C. S., Santos, E. S., Santo, R. F. D. E., Vasconcelos, J. F., Orge, I. D., Nonaka, C. K. V., et al. (2019). Betulinic Acid Derivative BA5, Attenuates Inflammation and Fibrosis in Experimental Chronic Chagas Disease Cardiomyopathy by Inducing IL-10 and M2 Polarization. Front. Immunol. 10, 1257. doi:10.3389/fimmu.2019.01257
Mohammad, F. K., Al-Baggou, B. Kh., and Naser, A. S. (2012). Antinociception by Metoclopramide, Ketamine and Their Combinations in Mice. Pharmacol. Rep. 64, 299–304. doi:10.1016/s1734-1140(12)70768-5
Mukherjee, P. K., Saha, K., Das, J., Pal, M., and Saha, B. P. (1997). Studies on the Anti-inflammatory Activity of Rhizomes of Nelumbo nucifera. Planta Med. 63 (4), 367–369. doi:10.1055/s-2006-957705
Nader, M. A., and Baraka, H. N. (2012). Effect of Betulinic Acid on Neutrophil Recruitment and Inflammatory Mediator Expression in Lipopolysaccharide-Induced Lung Inflammation in Rats. Eur. J. Pharm. Sci. 46, 106–113. doi:10.1016/j.ejps.2012.02.015
Newman, D. J., and Cragg, G. M. (2016). Natural Products as Sources of New Drugs from 1981 to 2014. J. Nat. Prod. 79, 629–661. doi:10.1021/acs.jnatprod.5b01055
Oliveira-Costa, J. F., Barbosa-Filho, J. M., Maia, G. L., Guimarães, E. T., Meira, C. S., Ribeiro-Dos-Santos, R., et al. (2014). Potent Anti-inflammatory Activity of Betulinic Acid Treatment in a Model of Lethal Endotoxemia. Int. Immunopharmacol. 23, 469–474. doi:10.1016/j.intimp.2014.09.021
Ou, Z., Zhao, J., Zhu, L., Huang, L., Ma, Y., Ma, C., et al. (2019). Anti-inflammatory Effect and Potential Mechanism of Betulinic Acid on λ-carrageenan-induced Paw Edema in Mice. Biomed. Pharmacother. 118, 109347. doi:10.1016/j.biopha.2019.109347
Oyebanji, B. O., Saba, A. B., and Oridupa, O. A. (2014). Studies on the Anti-inflammatory, Analgesic and Antipyrexic Activities of Betulinic Acid Derived from Tetracera Potatoria. Afr. J. Tradit. Complement. Altern. Med. 11, 30–33. doi:10.4314/ajtcam.v11i1.5
Park, J. Y., Pillinger, M. H., and Abramson, S. B. (2006). Prostaglandin E2 Synthesis and Secretion: The Role of PGE2 Synthases. Clin. Immunol. 119, 229–240. doi:10.1016/j.clim.2006.01.016
Pavlova, N. I., Savinova, O. V., Nikolaeva, S. N., Boreko, E. I., and Flekhter, O. B. (2003). Antiviral Activity of Betulin, Betulinic and Betulonic Acids against Some Enveloped and Non-enveloped Viruses. Fitoterapia 74, 489–492. doi:10.1016/s0367-326x(03)00123-0
Prados, M. E., García-Martín, A., Unciti-Broceta, J. D., Palomares, B., Collado, J. A., Minassi, A., et al. (2021). Betulinic Acid Hydroxamate Prevents Colonic Inflammation and Fibrosis in Murine Models of Inflammatory Bowel Disease. Acta Pharmacol. Sin. 42, 1124–1138. doi:10.1038/s41401-020-0497-0
Qu, Z. W., Liu, T. T., Ren, C., Gan, X., Qiu, C. Y., Ren, P., et al. (2015). 17β-Estradiol Enhances ASIC Activity in Primary Sensory Neurons to Produce Sex Difference in Acidosis-Induced Nociception. Endocrinology 156, 4660–4671. doi:10.1210/en.2015-1557
Recio, M. C., Giner, R. M., Máñez, S., Gueho, J., Julien, H. R., Hostettmann, K., et al. (1995). Investigations on the Steroidal Anti-inflammatory Activity of Triterpenoids from Diospyros Leucomelas. Planta Med. 61, 9–12. doi:10.1055/s-2006-957988
Rock, K. L., Latz, E., Ontiveros, F., and Kono, H. (2010). The Sterile Inflammatory Response. Annu. Rev. Immunol. 28, 321–342. doi:10.1146/annurev-immunol-030409-101311
Ryu, S. Y., Oak, M. H., Yoon, S. K., Cho, D. I., Yoo, G. S., Kim, T. S., et al. (2000). Anti-Allergic and Anti-inflammatory Triterpenes from the Herb of Prunella Vulgaris. Planta Med. 66, 358–360. doi:10.1055/s-2000-8531
Salvemini, D., Wang, Z. Q., Wyatt, P. S., Bourdon, D. M., Marino, M. H., Manning, P. T., et al. (1996). Nitric Oxide: a Key Mediator in the Early and Late Phase of Carrageenan-Induced Rat Paw Inflammation. Br. J. Pharmacol. 118, 829–838. doi:10.1111/j.1476-5381.1996.tb15475.x
Saraiva, M., and O'Garra, A. (2010). The Regulation of IL-10 Production by Immune Cells. Nat. Rev. ImmunolImmunology 10, 170–181. doi:10.1038/nri2711
Sharma, J. N., Al-Omran, A., and Parvathy, S. S. (2007). Role of Nitric Oxide in Inflammatory Diseases. Inflammopharmacology 15, 252–259. doi:10.1007/s10787-007-0013-x
Sostres, C., Gargallo, C. J., and Lanas, A. (2013). Nonsteroidal Anti-inflammatory Drugs and Upper and Lower Gastrointestinal Mucosal Damage. Arthritis Res. Ther. 15 Suppl 3, S3. doi:10.1186/ar4175
Sultana, N., and Saify, Z. S. (2012). Naturally Occurring and Synthetic Agents as Potential Anti-inflammatory and Immunomodulants. Antiinflamm. Antiallergy Agents Med. Chem. 11, 3–19. doi:10.2174/187152312803476264
Sun, I. C., Wang, H. K., Kashiwada, Y., Shen, J. K., Cosentino, L. M., Chen, C. H., et al. (1998). Anti-AIDS Agents. 34. Synthesis and Structure-Activity Relationships of Betulin Derivatives as Anti-HIV Agents. J. Med. Chem. 41, 4648–4657. doi:10.1021/jm980391g
Tsai, J. C., Peng, W. H., Chiu, T. H., Lai, S. C., and Lee, C. Y. (2011a). Anti-inflammatory Effects of Scoparia Dulcis L. And Betulinic Acid. Am. J. Chin. Med. 39, 943–956. doi:10.1142/S0192415X11009329
Viji, V., Helen, A., and Luxmi, V. R. (2011). Betulinic Acid Inhibits Endotoxin-Stimulated Phosphorylation Cascade and Pro-inflammatory Prostaglandin E(2) Production in Human Peripheral Blood Mononuclear Cells. Br. J. Pharmacol. 162, 1291–1303. doi:10.1111/j.1476-5381.2010.01112.x
Yoon, J. J., Lee, Y. J., Kim, J. S., Kang, D. G., and Lee, H. S. (2010). Protective Role of Betulinic Acid on TNF-Alpha-Induced Cell Adhesion Molecules in Vascular Endothelial Cells. Biochem. Biophys. Res. Commun. 391, 96–101. doi:10.1016/j.bbrc.2009.11.009
Yue, Q., Deng, X., Li, Y., and Zhang, Y. (2021). Effects of Betulinic Acid Derivative on Lung Inflammation in a Mouse Model of Chronic Obstructive Pulmonary Disease Induced by Particulate Matter 2.5. Med. Sci. Monit. 27, e928954. doi:10.12659/MSM.928954
Yun, Y., Han, S., Park, E., Yim, D., Lee, S., Lee, C. K., et al. (2003). Immunomodulatory Activity of Betulinic Acid by Producing Pro-inflammatory Cytokines and Activation of Macrophages. Arch. Pharm. Res. 26, 1087–1095. doi:10.1007/BF02994763
Zhou, F., and Pichersky, E. (2020). More Is Better: the Diversity of Terpene Metabolism in Plants. Curr. Opin. Plant Biol. 55, 1–10. doi:10.1016/j.pbi.2020.01.005
Keywords: betulinic acid, anti-inflammatory activity, terpenoids, immunomodulation, inflammation
Citation: Oliveira-Costa JF, Meira CS, Neves MVGd, Dos Reis BPZC and Soares MBP (2022) Anti-Inflammatory Activities of Betulinic Acid: A Review. Front. Pharmacol. 13:883857. doi: 10.3389/fphar.2022.883857
Received: 25 February 2022; Accepted: 02 May 2022;
Published: 23 May 2022.
Edited by:
Jaime Ribeiro-Filho, Oswaldo Cruz Foundation (FIOCRUZ), BrazilReviewed by:
Newman Osafo, Kwame Nkrumah University of Science and Technology, GhanaJacqueline Leite, Universidade Federal de Goiás, Brazil
Aracelio Colares, Centro Universitário Leão Sampaio, Brazil
Copyright © 2022 Oliveira-Costa, Meira, Neves, Dos Reis and Soares. This is an open-access article distributed under the terms of the Creative Commons Attribution License (CC BY). The use, distribution or reproduction in other forums is permitted, provided the original author(s) and the copyright owner(s) are credited and that the original publication in this journal is cited, in accordance with accepted academic practice. No use, distribution or reproduction is permitted which does not comply with these terms.
*Correspondence: Milena Botelho Pereira Soares, bWlsZW5hQGJhaGlhLmZpb2NydXouYnI=