- 1Department of General Surgery (Gastrointestinal Surgery), The Affiliated Hospital of Southwest Medical University, Luzhou, China
- 2School of Clinical Medicine, Southwest Medical University, Luzhou, China
- 3Faculty Affairs and Human Resources Management Department, Southwest Medical University, Luzhou, China
Background and purpose: Radioresistance remains a significant challenge in tumor therapy. This systematic review aims to demonstrate the role of long non-coding RNA (lncRNA) in cancer radioresistance/radiosensitivity.
Material and methods: The electronic databases Pubmed, Embase, and Google Scholar were searched from January 2000 to December 2021 to identify studies addressing the mechanisms of lncRNAs in tumor radioresistance/sensitivity, each of which required both in vivo and in vitro experiments.
Results: Among the 87 studies identified, lncRNAs were implicated in tumor radioresistance/sensitivity mainly in three paradigms. 1) lncRNAs act on microRNA (miRNA) by means of a sponge, and their downstream signals include some specific molecular biological processes (DNA repair and chromosome stabilization, mRNA or protein stabilization, cell cycle and proliferation, apoptosis-related pathways, autophagy-related pathways, epithelial-mesenchymal transition (EMT), cellular energy metabolism) and some signaling mediators (transcription factors, kinases, some important signal transduction pathways) that regulate various biological processes. 2) lncRNAs directly interact with proteins, affecting the cell cycle and autophagy to contribute to tumor radioresistance. 3) lncRNAs act like transcription factors to initiate downstream signaling pathways and participate in tumor radioresistance.
Conclusion: lncRNAs are important regulators involved in tumor radioresistance\sensitivity. Different lncRNAs may participate in the radioresistance with the same regulatory paradigm, and the same lncRNAs may also participate in the radioresistance in different ways. Future research should focus more on comprehensively characterizing the mechanisms of lncRNAs in tumor radioresistance to help us identify corresponding novel biomarkers and develop new lncRNA-based methods to improve radioresistance.
Introduction
Radiotherapy is a standard treatment for many malignant tumors. About half of tumor patients receive this treatment, including radical, adjuvant, and palliative radiotherapy (Delaney et al., 2005; Schaue and McBride, 2015). Some tumors are sensitive to radiotherapy, which can achieve a radical cure. Some malignant tumors can be cured by a combination of radiotherapy, surgery, and chemotherapy. Besides, radiotherapy can also improve the quality of life and prolong the survival of patients with advanced tumors. The impact of radiotherapy on tumors is a complex process encompassing multiple factors and mechanisms. During radiotherapy, radiation can directly act on biologically active macromolecules, such as DNA and enzymes, causing abnormalities in their structure and function. It can also cause ionization and excitation of water molecules, producing free radicals and resulting in secondary damage to biological macromolecules (Nascimento and Bradshaw, 2016). In addition, radiation may have a secondary effect by affecting neurohumoral disorders, changing the permeability of cell membranes and blood vessel walls, and causing toxemia (Sharma et al., 2001; Wang et al., 2018).
While radiotherapy improves the prognosis of tumor patients, tumor cells exhibit varying degrees of resistance to it. The cancer radioresistance directly affects the effectiveness of radiation treatment on tumors, which is closely related to the poor prognosis in patients. Accordingly, tumor cells may possess mechanisms of radiotherapy resistance. However, the molecular mechanisms of radiation resistance of tumor cells remain poorly understood. Obviously, these mechanisms are complex and require more extensive characterization.
The long non-coding RNA (lncRNA) is a type of non-coding RNA with a length greater than 200 nucleotides. They participate in various cellular processes and are involved in the development of diseases (Mercer et al., 2009). It has been estimated that the human genome encodes more than 28,000 different lncRNAs (Tragante et al., 2014). Generally, the expression of lncRNA is typically lower than that of protein-coding genes and is highly tissue and time specific (Derrien et al., 2012). Evidence suggests that lncRNA can participate in multiple biological processes of tumor cells through various mechanisms, such as signal molecules, decoy molecules, guide molecules, scaffold molecules, and RNA sponges (Yang et al., 2014). More recent evidence indicates that lncRNA can modulate radiotherapy response by regulating key signal pathways, including DNA damage repair, cell apoptosis, cell metabolism, and autophagy (Podralska et al., 2020). However, to the best of our knowledge, no systematic review has been published to summarize the mechanisms of lncRNA in cancer radiotherapy resistance.
This study aimed to systematically review the literature and summarize the mechanism by which lncRNA contributes to cancer radioresistance/radiosensitivity. These findings may provide new insights for improving the efficiency of tumor radiotherapy, discovering new therapeutic targets, and translational medicine in the future.
Material and Methods
This research strictly followed the PRISMA (Preferred Reporting Items for Systematic review and Meta-analyses) (Moher et al., 2009). This study was a systematic review and did not directly involve the issue of humans, so the review of the Institutional Review Board (IRB) was exempted.
Search Strategy
The databases Pubmed, Embase, and Google Scholar were used for literature search. The search period for the literature was set from 1 January 2000 to 31 December 2021, and the language was restricted to English.
This study adopted the strategy of combining Pubmed mesh term and free words to determine the search terms, such as “neoplasms”, “cancer”, “tumor”, “RNA, long noncoding”, “long non-coding RNA, “lncRNA”, “radiotherapy”, “ionizing radiation”, “ionizing”, “radiation”, “radioresistance”, and “radiosensitivity”. The search strategy based on Pubmed was shown in Supplementary Table S1.
Exclusion and Inclusion Criteria
Two researchers (Wenhan Wu and Shijian Zhang) independently searched and screened the literature using inclusion and exclusion criteria. We excluded irrelevant records based on the titles and abstracts and carefully evaluated the full text of the remaining documents. If there was a dispute between the two researchers, a third researcher (Jia He) would resolve the matter independently, and a consensus would be reached. Where possible, the original authors were contacted for more detailed data.
Inclusion Criteria
1) Human tumor subjects; 2) the expression of lncRNA and cancer; 3) availability of data for both in vitro cell lines and animal studies; 4) lncRNAs involvement in cancer radioresistance/radiosensitivity and their specific mechanism.
Exclusion Criteria
1) Only including vitro cell lines or animal studies; 2) review, editorial, and case reports; 3) incomplete data or uncertain mechanism.
Data Extraction and Data Items
The literature included in this study has analyzed the relationship between lncRNA and cancer radioresistance/radiosensitivity in specific tumors, and determined their corresponding mechanism. We extracted the following data from each article: author, publication date, title, type of tumor and radiotherapy, involved lncRNAs and their expression levels, type of cell line, and corresponding mechanism of lncRNA.
Results
Study Search and Characteristics
A diagram illustrating the literature search and selection process was shown in Figure 1. In the initial search, we identified a total of 5,653 potentially relevant documents. After removing duplicate publications, 1,668 studies remained. Based on the inclusion and exclusion criteria, we then carefully reviewed the abstracts of these studies and excluded 1,098 records, including reviews, meta-analyses, case reports, and other unrelated studies. We further evaluated the full text of the remaining 570 studies and excluded 483 studies. Because these excluded studies lacked in vivo or in vitro data, or their data was unclear. Finally, A total of 87 articles were included in this study.
Supplementary Table S2 listed the lncRNAs involved in cancer radioresistance/radiosensitivity and their concise mechanisms. The sources of cancer included bladder cancer (Tan et al., 2015; Jiang et al., 2017), breast cancer (Liu et al., 2019a; Zhang et al., 2019a; Wang et al., 2019; Qian et al., 2020; Zhang et al., 2020a), cardiac cancer (Jia et al., 2019), gastric cancer (Jiang et al., 2020; Lu et al., 2020; Xiao et al., 2020), cervical cancer (Jing et al., 2015; Li et al., 2018a; Han et al., 2018; Gao et al., 2019; Zhao et al., 2019; Wang et al., 2020a; Ge et al., 2020), colorectal cancer (Liu et al., 2020a; Liu et al., 2020b; Li et al., 2021; Liang et al., 2021), esophageal cancer (Li et al., 2017; Chen et al., 2018a; Zhang et al., 2019b; Wang et al., 2020b; Cheng et al., 2020; Liu et al., 2021a; Sun et al., 2021; Yang et al., 2021), glioblastoma (Li et al., 2018b; Ahmadov et al., 2021; Li et al., 2022), glioma (Yang et al., 2016; Zheng et al., 2016; Wang et al., 2020c; Wang et al., 2020d; Tang et al., 2020; Zheng et al., 2020; Gao et al., 2021; Tian et al., 2021), head and neck squamous cell cancer (Li et al., 2020a), laryngeal cancer (Cui et al., 2019; Tang et al., 2019; Tang and Shan, 2019), nasopharyngeal cancer (Jin et al., 2016; Wang et al., 2017; Ma et al., 2018; Yi et al., 2019; Han et al., 2020a; Han et al., 2020b; Wang et al., 2020e; Zhong et al., 2020; Wang et al., 2021a; Liu et al., 2021b; Guo et al., 2021), hepatocellular cancer (Chen et al., 2018b; Song et al., 2019; Yang et al., 2020a; Jin et al., 2021; Yu et al., 2021), lung cancer (Chen et al., 2015; Wu et al., 2017; Xue et al., 2017; Liu et al., 2019b; Wang and Hu, 2019; Yang et al., 2019; Brownmiller et al., 2020; Han et al., 2020c; He et al., 2020; Hou et al., 2020; Yu et al., 2020; Wang et al., 2021b; Liu et al., 2021c; Jiang et al., 2021; Zhang et al., 2021), medulloblastoma (Zhu et al., 2021), melanoma (Cui et al., 2021; Liu et al., 2021d), neuroblastoma (Yang et al., 2020b; Mou et al., 2021), prostate cancer (Chen et al., 2018c; Ma et al., 2020; Xiu et al., 2020), renal cell cancer (Zhou et al., 2021), and thyroid cancer (Li et al., 2020b; Chen et al., 2021). Out of the 87 studies, a total of 11 types of lncRNA have been independently reported in at least two articles to participate in cancer radioresistance/radiosensitivity, including lncRNA HOTAIR (n = 7), lncRNA GAS5 (n = 5), lncRNA PVT1 (n = 4), lncRNA TUG1 (n = 4), lncRNA NEAT1 (n = 3), lncRNA DGCR5 (n = 2), lncRNA FAM201A (n = 2), lncRNA KCNQ1OT1 (n = 2), lncRNA LINC00958 (n = 2), lncRNA MALAT1 (n = 2), and lncRNA XIST (n = 2).
Mechanism of lncRNA Contributing to Cancer Radioresistance/Radiosensitivity
Acting on miRNA by Acting as a Sponge to Regulate Downstream Signals
Among the lncRNAs identified in this study, the majority acted as competitive endogenous RNA (ceRNA) in regulating cancer radioresistance/radiosensitivity. The fundamental mechanism was that lncRNA acted on microRNA (miRNA) by acting as a sponge to regulate downstream signals. In our study, the downstream signals that were regulated by the lncRNA/miRNA paradigm and mediated cancer radioresistance/radiosensitivity mainly included some specific molecular biological processes and some signaling mediators that regulate various biological processes (Figure 2). These specific molecular biological processes mainly included DNA repair and chromosome stabilization, mRNA or protein stabilization, cell cycle and proliferation, apoptosis-related pathways, autophagy-related pathways, epithelial-mesenchymal transition (EMT), and cellular energy metabolism. Those signaling mediators primarily included transcription factors, kinases, or some important signal transduction pathways, which often promoted tumor radioresistance\sensitivity through various mechanisms. It is worth noting that a total of six lncRNA\miRNA downstream mechanisms were not fully elucidated, representing the direction of further research (Wu et al., 2017; Xue et al., 2017; Jia et al., 2019; Tang et al., 2019; Jiang et al., 2020; Jin et al., 2021).
Molecular Biological Process 1: DNA Repair and Chromosome Stabilization
DNA double-strand break (DSB) is the most common cellular damage induced by ionizing radiation. If it is not repaired correctly, it may cause chromosomal abnormalities and even cell death. There is growing evidence of the role of lncRNA in DNA repair and chromosome stabilization via miRNA. In gastric cancer, lncRNA LINC01436 was reported to upregulate radioresistance through miR-513a-5p/APE1 axis (Lu et al., 2020). APE1 (DNA-(apurinic or apyrimidinic site) endonuclease) is a protein with multiple functions. It usually participates in DNA damage repair through the DNA base excision repair (BER) pathway (Wierstra, 2013). There was evidence that the lncRNA LINC00958 enhanced radioresistance via miR-5095/RRM2 in cervical cancer (Zhao et al., 2019). RRM2 (ribonucleotide reductase regulatory subunit M2) catalyzes the conversion of ribonucleotides to deoxyribonucleotides, which is the rate-limiting enzyme for DNA synthesis or repair, and plays a crucial role in tumor cell DNA synthesis and proliferation (Zhong et al., 2016). In colorectal cancer, lncRNA lnc-RI enhanced radioresistance through miR-4727-5p/LIG4 (Liu et al., 2020b). LIG4 (DNA ligase 4) is a DNA ligase, which is essential for V(D)J recombination and DNA double-strand break (DSB) repair through non-homologous end joining (NHEJ) (Gu et al., 2007). In esophageal squamous cell cancer, lncRNA FAM201A was reported to upregulate radioresistance via miR-101/ATM axis (Chen et al., 2018a). ATM (ATM serine/threonine kinase) is an essential component of the response to DNA damage and the maintenance genome stability, which is the main repair protein involved in double-strand break (DSB) homologous recombination repair (HRR) induced by ionizing radiation (Qin et al., 2019). Besides, lncRNA NORAD was reported to upregulate esophageal squamous cell cancer radioresistance via miR-199-a1/EEPD1 (Sun et al., 2021). EEPD1 (endonuclease/exonuclease/phosphatase family domain containing 1) participates in DNA repair and maintains genome stability by promoting end excision and homologous recombination repair (Wu et al., 2015). In hepatocellular cancer, lncRNA ROR upregulated radioresistance via miR-145/RAD18 axis (Chen et al., 2018b). RAD18 (RAD18 E3 ubiquitin-protein ligase) participates in the post-replication repair of UV-damaged DNA. It plays a role in the duplication of damaged DNA in filling the gaps of the daughter strands (Cotta-Ramusino et al., 2011). In prostate cancer, lncRNA TUG1 enhanced radioresistance through miR-139-5p/SMC1A axis (Xiu et al., 2020). SMC1A (structural maintenance of chromosomes 1A) is an important part of the functional kinetochore, which helps correctly separate chromosomes during cell division. It is also considered to have potential DNA repair functions (Yazdi et al., 2002). In renal cell cancer, lncRNA LINC02532 upregulated radioresistance via miR-654-5p/YY1 axis (Zhou et al., 2021). YY1 (transcriptional repressor protein YY1) is a multifunctional transcription factor and a core component of the chromatin remodeling INO80 complex. It is involved in transcription regulation, DNA replication, and DNA repair (Wu et al., 2007).
Molecular Biological Process 2: mRNA or Protein Stabilization
During radiation-induced cellular stress, maintaining mRNA and protein stability helps minimize cell damage and facilitate cell survival. A study reported that the lncRNA HOTAIR enhanced radioresistance in breast cancer through the miR-449b-5p/HSPA1A axis (Zhang et al., 2020a). HSPA1A (heat shock protein family A member 1A) can stabilize existing proteins and mediate the correct folding of proteins in the cytoplasm and organelles, thereby protecting tumor cells and enhancing their recovery (Santos et al., 2017). lncRNA LINC00511 also upregulated breast cancer radioresistance via miR-185/STXBP4 axis (Liu et al., 2019a). STXBP4 (syntaxin binding protein 4) plays a role in translocating vesicles from the cytoplasm to the cell membrane, which has been shown to contribute to protein stability (Li et al., 2009). In non-small cell lung cancer, lncRNA PVT1 induced radioresistance through miR-424-5p/CARM1 (Wang and Hu, 2019), while CARM1 (coactivator associated arginine methyltransferase 1) is involved in DNA packaging, transcription regulation, pre-mRNA splicing, and mRNA stability (Yang and Bedford, 2013). Moreover, the lncRNA GAS5 was shown to enhance radiosensitivity through miR-362-5p/SMG1 axis in thyroid cancer (Li et al., 2020b). SMG1 (serine/threonine-protein kinase SMG1) is involved in both mRNA surveillance and genotoxic stress response pathways. Its consumption leads to spontaneous DNA damage and an increasing in sensitivity to ionizing radiation (IR) (Yamashita et al., 2001).
Molecular Biological Process 3: Cell Cycle and Proliferation
The dysfunctional regulation of the cell cycle and proliferation is also associated with cancer radioresistance/radiosensitivity. A study reported that lncRNA SNHG12 enhanced radioresistance in cervical cancer cells via miR-148a/CDK1 (Wang et al., 2020a). CDK1 (cyclin-dependent kinase 1) promotes the transition from the G2 to M phase of the cell cycle, thereby promoting the proliferation of tumor cells (Hirai et al., 1995). lncRNA NEAT1 was also reported to enhance cervical cancer radioresistance via miR-193b-3p/CCND1 axis (Han et al., 2018). CCND1 (cyclin D1) is a highly conserved cyclin, which is mainly involved in the transition of the G1/S phase of the cell cycle by regulating CDK (Jares et al., 2007). In glioblastoma, lncRNA RBPMS-AS1 downregulate radioresistance via miR-301a-3p/CAMTA1 axis (Li et al., 2022). CAMTA1 (calmodulin-binding transcription activator 1) is a transcription factor, which induces the expression of natriuretic peptide A (NPPA), an anti-proliferative cardiac hormone (Schraivogel et al., 2011). Besides, in nasopharyngeal cancer, lncRNA PTPRG-AS1 enhanced radioresistance via miR-194-3p/PRC1 (Yi et al., 2019). PRC1 (protein regulator of cytokinesis 1) is a protein involved in cytokinesis. This protein is present at high levels during the S and G2/M phases of mitosis, but when the cell exits mitosis and enters the G1 phase, its level drops sharply (Zhan et al., 2017a).
Molecular Biological Process 4: Apoptosis-Related Pathways
Radiation-induced DNA damage may activate apoptosis-related signaling pathways, and the anti-apoptotic mechanisms of tumor cells are directly involved in radioresistance. It has been reported that lncRNA GAS5 decreased radioresistance through miR-106b/IER3 in cervical cancer (Gao et al., 2019). IER3 (immediate early response 3) is controlled by many stimuli and cellular conditions. It plays a dual role in tumor cell growth control and apoptosis, depending on the cell type and related conditions (Jin et al., 2015). In glioma, lncRNA NCK1-AS1 upregulated radioresistance via miR-22-3p/IGF1R axis (Wang et al., 2020c). IGF1R (insulin-like growth factor 1 receptor) binds insulin-like growth factors with high affinity. It has tyrosine kinase activity. It is highly overexpressed in many malignant tissues and acts as an anti-apoptotic agent (Yuan et al., 2018). In laryngeal cancer, lncRNA HOTAIR enhanced radioresistance via miR-454-3p/E2F2 axis (Cui et al., 2019). E2F2 (E2F transcription factor 2) is a member of the E2F transcription factor family and plays an inhibitory role in p53-independent apoptosis induced by ionizing radiation (IR) (Wichmann et al., 2010). In non-small cell lung cancer, lncRNA CYTOR upregulated radioresistance via miR-206/PTMA axis (Jiang et al., 2021), and PTMA (prothymosin alpha) involved in inhibiting apoptosis (Malicet et al., 2006).
Molecular Biological Process 5: Autophagy-Related Pathways
The autophagy pathway mediates the degradation of dysfunctional organelles and promotes protein turnover, thereby promoting radioresistance as a means of survival and adaptation in the presence of ionizing radiation. In colorectal cancer, lncRNA HOTAIR enhanced radioresistance via miR-93/ATG12 axis (Liu et al., 2020a). ATG12 (autophagy-related 12) is mainly involved in the formation of autophagic vesicles, and plays a vital role in tumor maintenance and treatment resistance (Yun and Lee, 2018). In lung adenocarcinoma, lncRNA KCNQ1OT1 was also reported to upregulated radioresistance via miR-372-3p/ATG5 and ATG12 axis (He et al., 2020).
Molecular Biological Process 6: Epithelial-Mesenchymal Transition
EMT is the process that epithelial cells transform into mesenchymal cells and acquire the ability to migrate. The acquisition of EMT in tumor cells is associated with radioresistance and poor prognosis. lncRNA TUG1 has been found to enhance radioresistance in bladder cancer via miR-145/ZEB2 axis (Tan et al., 2015). ZEB2 (zinc finger E-box binding homeobox 2) usually functions as a repressor of DNA transcription in the nucleus and plays a crucial role in the EMT of tumor cells (Fardi et al., 2019). In nasopharyngeal cancer, lncRNA MINCR upregulated radioresistance via miR-223/ZEB1 (Zhong et al., 2020), and ZEB1 (zinc finger E-box binding homeobox 1) drives the induction of EMT by activating stem cell characteristics, immune evasion, and epigenetic reprogramming (Zhang et al., 2019c). In neuroblastoma, lncRNA XIST was reported to enhance radioresistance through miR-375/L1CAM axis (Yang et al., 2020b). L1CAM (L1 cell adhesion molecule) is a glycoprotein involved in cancer development, which plays a role in EMT primarily through interactions with other cell adhesion molecules, integrins, or growth factor receptors (Maten et al., 2019).
Molecular Biological Process 7: Cellular Energy Metabolism
Changes in the metabolic pathways of tumor cells are considered to be a hallmark of tumors, and these changes can lead to radioresistance. In neuroblastoma, lncRNA LINC01410 was found to upregulate radioresistance via miR-545-3p/HK2 axis (Mou et al., 2021). HK2 (hexokinase 2) is located in the outer membrane of mitochondria, participates in most glucose metabolism pathways, and is thought to be involved in the supply of tumor cells (Liu et al., 2019c). In melanoma, lncRNA LINC01224 was reported to upregulate radioresistance via miR-193a-5p/NR1D2 axis (Cui et al., 2021). NR1D2 (nuclear receptor subfamily 1 group D member 2) acts as a transcription inhibitor and may affect cancer carbohydrate and lipid metabolism (Yu et al., 2018).
Signaling Mediator 1: Transcription Factor
Besides, the downstream targets of lncRNAs mediated by miRNA and involved in tumor radioresistance/radiosensitivity also included some transcription factors, kinases, or some important signal transduction pathways. These regulated downstream targets often have multiple biological functions in tumor development. In gastric cancer, lncRNA TRPM2-AS enhanced radioresistance via miR-612/FOXM1 axis (Xiao et al., 2020). FOXM1 (Forkhead box protein M1) is a transcription factor that controls the cell cycle and is involved in repairing DNA breaks. FOXM1 stimulates cell proliferation by promoting cells to enter the S and M phases. It also contributes to angiogenesis, invasion, metastasis, and EMT in tumors (Wierstra, 2013). In head and neck squamous cell cancer, lncRNA LINC00520 enhanced radioresistance via miR-195/HOXA10 (Li et al., 2020a), while In lung cancer, lncRNA LINC00483 upregulated radioresistance via miR-144/HOXA10 (Yang et al., 2019). Also, in lung cancer, lncRNA LINC00461 enhanced radioresistance via miR-195/HOXA10 (Hou et al., 2020). HOXA10 (homeobox A10) is a DNA-binding transcription factor that may regulate fertility, embryo vitality, and hematopoietic lineage commitment. It is thought to be related to tumor cell proliferation, migration, and invasion (Carrera et al., 2015).
Signaling Mediator 2: Kinase
In breast cancer, lncRNA LINC00963 upregulated radioresistance via miR-324-3p/ACK1 axis (Zhang et al., 2019a). ACK1 (activated CDC42 kinase 1) is a serine/threonine-protein kinase that contributes to cancer migration, survival, and proliferation via regulating WWOX and AKT1 (Mahajan and Mahajan, 2013). In rectal cancer, lncRNA EGOT enhanced radioresistance via miR-211-5p/ErbB4 axis (Li et al., 2021). ErbB4 (erb-b2 receptor tyrosine kinase 4) is a single-pass type I membrane protein with multiple cysteine rich domains, a transmembrane domain, and a tyrosine kinase domain. It is related to cell proliferation and differentiation in tumors (Segers et al., 2020). In colorectal cancer, lncRNA LINC00958 upregulated radioresistance via miR-422a/MAPK1 axis (Liang et al., 2021). While in glioma, lncRNA TPTEP1 downregulated radioresistance through miR-106a-5p/P38 MAPK (Tang et al., 2020). Moreover, in nasopharyngeal cancer, lncRNA LINC00114 enhanced radioresistance via miR-203/ERK/JNK signaling pathway (Han et al., 2020a). There are three well-defined MAPK subfamilies in mammals: extracellular signal-regulated kinase (ERK), c-Jun N-terminal kinase (JNK), and p38 kinase. The activation of each MAPK signal follows a three-layer kinase module, in which MAP3K phosphorylates and activates MAP2K, and MAP2K phosphorylates and activates MAPK. Once activated, MAPK controls a variety of cellular responses, such as tumor proliferation, differentiation, apoptosis, angiogenesis, and metastasis (Cargnello and Roux, 2011; Guo et al., 2020).
Signaling Mediator 3: Signal Transduction Pathway
In laryngeal cancer, lncRNA DGCR5 upregulated radioresistance through miR-506/Wnt pathway (Tang and Shan, 2019), and Wnt pathway is one of the key cascades that regulate caner development and stemness (Zhan et al., 2017b). Besides, in lung cancer, lncRNA AGAP2-AS1 enhanced radioresistance through miR-296/NOTCH2 axis (Zhang et al., 2021). NOTCH2 (notch receptor 2) is a member of the Notch family. The continuous Notch2 signal promotes tumor cell EMT while avoiding apoptosis, and the increase of Notch2 expression is related to the poor clinical prognosis of patients (Xiu and Liu, 2019).
Acting on Protein to Regulate Downstream Signals
In addition to regulating miRNAs, lncRNAs can also directly interact with specific proteins to participate in cancer radioresistance/radiosensitivity. In breast cancer, lncRNA LINC02582 directly interacted with a ubiquitinase USP7, which reduced the level of CHK1 protein, resulting in radioresistance (Wang et al., 2019). In glioma, Linc-RA1 can combine with H2B to stabilize the level of H2B K120 monoubiquitination and inhibit the interaction between H2Bub1 and ubiquitin-specific protease 44 (USP44), thus regulating autophagy and enhanced radioresistance (Zheng et al., 2020). In non-small cell lung cancer, lncRNA linc-SPRY3 could bind to IGF2BP3 (Insulin Like Growth Factor 2 MRNA Binding Protein 3), which leads to the destabilization of c-Myc and HMGA2, and improves the radiosensitivity of tumors (Brownmiller et al., 2020).
Acting Like Transcription Factor to Regulate Downstream Signals
Besides, lncRNAs can also act like transcription factors to initiate downstream signaling pathways and participate in tumor radioresistance/radiosensitivity. In esophageal cancer, lncRNA MAGI2-AS3 can recruit the histone methyltransferase EZH2 to the HOXB7 promoter region to initiate H3K27me3 and repress HOXB7 expression, resulting in enhanced tumor radiosensitivity (Cheng et al., 2020). In nasopharyngeal cancer, lncRNA PVT1 can act as a scaffold for the chromatin modifier KAT2A, recruiting the nuclear receptor-binding protein TIF1β to activate NF90 transcription, thereby increasing HIF-1α and upregulating radioresistance (Wang et al., 2020e). HIF-1α activates the transcription of many genes that encode proteins involved in cancer angiogenesis, glucose metabolism, cell proliferation/survival, and invasion/metastasis (Semenza, 2003). In lung adenocarcinoma, down-regulated lncRNA LINC00857 inhibited the expression of BIRC5 by inhibiting the enrichment of NF-κB1 in the promoter region of BIRC5, thereby enhancing radiosensitivity (Han et al., 2020c).
In addition, some studies have reported that lncRNAs could be involved in tumor radioresistance/sensitivity through DNA repair (Jiang et al., 2017; Li et al., 2018b; Zhang et al., 2019b; Qian et al., 2020), cell cycle regulation (Jing et al., 2015; Li et al., 2017), and EMT (Yang et al., 2016). However, the definite biological behaviors of lncRNAs remain to be further explored. Finally, we summarized the mechanism of lncRNA-induced tumor radiosensitivity/resistance according to molecular behavior of lncRNA, biological process/signaling mediator, and downstream key molecule in Table 1.
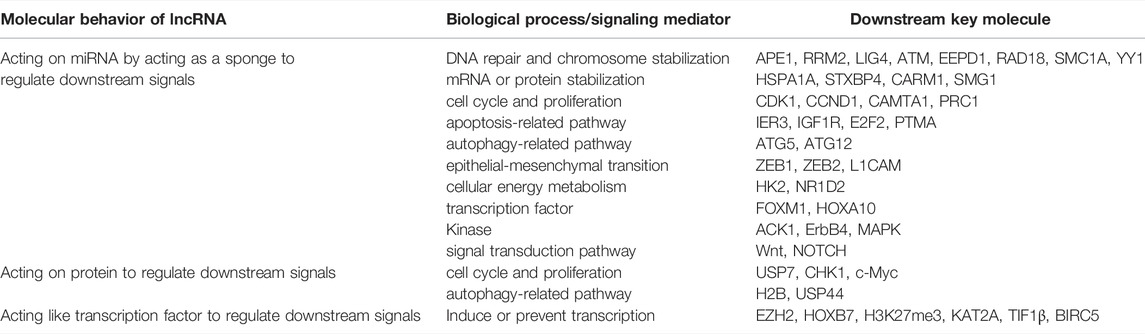
TABLE 1. The summary of mechanisms by which lncRNAs contribute to cancer radioresistance/radiosensitivity.
Discussion
Radiation therapy is one of the core methods of cancer treatment. However, cancer radiation resistance often limits the effectiveness of this treatment, and the mechanisms of radioresistance remain largely unknown. With the development of biotechnologies such as high-throughput sequencing, bioinformatics analysis, and animal modeling, lncRNAs have been shown to play critical regulatory roles in tumorigenesis and progression (Yang et al., 2014). Their role in tumor therapy resistance provided new insights for identifying appropriate treatments for specific populations, improving treatment resistance, and developing novel therapeutic targets (Zhang et al., 2020b). Therefore, exploring the detailed function of lncRNAs in tumor radioresistance/sensitivity will allow us to identify corresponding novel biomarkers and develop new lncRNA-based methods to improve radioresistance to achieve precise radiotherapy for patients. To the best of our knowledge, this study is the first of its kind to systematically evaluate the functions of lncRNAs in cancer radioresistance/sensitivity on the basis of high-quality experimental evidence.
The mechanisms by which lncRNAs participate in tumor radioresistance/sensitivity may mainly include three categories. 1) lncRNAs act on microRNA (miRNA) through a sponge, and their downstream signals include some specific molecular biological processes (DNA repair and chromosome stabilization, mRNA or protein stabilization, cell cycle and proliferation, apoptosis-related pathways, autophagy-related pathways, epithelial-mesenchymal transition (EMT), cellular energy metabolism) and some signaling mediators (transcription factors, kinases, some important signal transduction pathways) that regulate various biological processes. 2) lncRNAs directly interact with proteins to participate in tumor radioresistance through affecting the cell cycle and autophagy. 3) lncRNAs act like transcription factors to initiate downstream signaling pathways and participate in tumor radioresistance. Besides, the same lncRNA may be involved in radioresistance\sensitivity by different mechanisms in different tumors, such as lncRNA HOTAIR, lncRNA GAS5, lncRNA PVT1, lncRNA TUG1, lncRNA DGCR5, lncRNA FAM201A, lncRNA KCNQ1OT1, lncRNA LINC00958, lncRNA MALAT1, lncRNA NEAT1, and lncRNA XIST. This also revealed that lncRNAs may have multiple potential mechanisms of action in tumor radioresistance, and may act through multiple mechanisms simultaneously. Some bioinformatics methods, such as RNA-RNA binding, RNA-protein binding prediction algorithms, will provide clues to comprehensively characterize the biological behavior of lncRNAs (Rinn and Chang, 2012). Of course, further experimental verification is still the top priority.
There have been studies on the relationship between radiotherapy efficacy and lncRNAs as biomarkers for radiotherapy patients, such as in non-small cell lung cancer and glioma (Lin et al., 2020; Song et al., 2021). However, the clinical application of lncRNAs as biomarkers of radioresistance still faces huge challenges. First, in addition to collecting lncRNA data from patients who have already received radiation therapy, it is often necessary to collect lncRNA data from the control (normal) population, which is often difficult to accomplish. Organoids provide new insight into this dilemma, a method that can closely mimic the physiology of humans in vitro (Drost and Clevers, 2018). The expression levels of lncRNAs change dynamically, and the sample obtained represents a snapshot of the patient’s current state. However, radiotherapy is often time- and dose-dependent; therefore, it is imperative to investigate the time- and dose-dependent effects of lncRNAs on tumor radiation resistance. In addition, lncRNAs are highly tissue-specific, and it is also necessary to compare lncRNA-specific changes in different organs after irradiation. Therefore, a comprehensive understanding of the regulatory paradigm of lncRNAs in tumor radioresistance will help us to better screen verifiable, detectable, highly sensitive, and highly specific lncRNA biomarkers using novel biotechnologies.
Moreover, some lncRNA-based precision medicine clinical trials have been carried out or are underway, such as lncRNA MALAT1 (Amodio et al., 2018), lncRNA HOTAIR (Di et al., 2017). Although there is currently no study of lncRNAs used in clinical patients to improve radiotherapy resistance, studies have shown that nanoparticles-meditated LncRNA AFAP1-AS1 silencing to block the wnt/β-catenin signaling pathway can effectively improve the radioresistance of triple-negative breast cancer (Bi et al., 2020). Challenges remain until these techniques can be applied to improve tumor radiation resistance. First, due to the complex mode of function of lncRNAs, this requires further comprehensive understanding and assessment of the specific functions of lncRNAs involved in cancer radioresistance. Second, the design of the lncRNA delivery system still needs to be further optimized to improve transfection efficiency, reduce off-target effects, and prolong the half-life of lncRNA degradation. Mastering the mechanism of lncRNA in tumor radioresistance/sensitivity will help us to screen more suitable biomarkers and therapeutic targets. This systematic review provides convincing evidence for the mechanism by which lncRNAs are involved in tumor radioresistance/sensitivity. More fundamental and clinical research is needed in the future to investigate how lncRNAs affect various aspects of radioresistance/radiosensitivity, and to study the application value of lncRNAs in radiotherapy.
Conclusion
In conclusion, this systematic review studied the mechanism of lncRNA in cancer radioresistance/radiosensitivity. The mechanisms by which lncRNAs participate in tumor radioresistance/sensitivity may mainly include three categories. 1) lncRNAs act on microRNA (miRNA) through a sponge, and their downstream signals include some specific molecular biological processes (DNA repair and chromosome stabilization, mRNA or protein stabilization, cell cycle and proliferation, apoptosis-related pathways, autophagy-related pathways, epithelial-mesenchymal transition (EMT), cellular energy metabolism) and some signaling mediators (transcription factors, kinases, some important signal transduction pathways) that regulate various biological processes. 2) lncRNAs directly interact with proteins to participate in tumor radioresistance through affecting the cell cycle and autophagy. 3) lncRNAs act like transcription factors to initiate downstream signaling pathways and participate in tumor radioresistance. Different lncRNAs may participate in the radioresistance with the same regulatory paradigm, and the same lncRNAs may also participate in the radioresistance through different mechanisms. More detailed studies on how lncRNAs are involved in tumor radioresistance are urgently needed to help us screen more suitable biomarkers and therapeutic targets. This will provide a rationale for large-scale clinical validation and may ultimately improve tumor radioresistance and patient prognosis.
Author Contributions
The authors confirm contribution to the paper as follows: study conception and design: WW data collection: WW, SZ, JH analysis and interpretation of results: WW, JH draft manuscript preparation: WW, SZ, JH. All authors reviewed the results and approved the final version of the manuscript.
Funding
This study was supported by the Doctoral Research Initiation Fund of Affiliated Hospital of Southwest Medical University (No. 21041). Besides, thanks to BioRender.com (https://app.biorender.com/biorender-templates) for supporting the drawings in this article.
Conflict of Interest
The authors declare that the research was conducted in the absence of any commercial or financial relationships that could be construed as a potential conflict of interest.
Publisher’s Note
All claims expressed in this article are solely those of the authors and do not necessarily represent those of their affiliated organizations, or those of the publisher, the editors and the reviewers. Any product that may be evaluated in this article, or claim that may be made by its manufacturer, is not guaranteed or endorsed by the publisher.
Supplementary Material
The Supplementary Material for this article can be found online at: https://www.frontiersin.org/articles/10.3389/fphar.2022.879704/full#supplementary-material
References
Ahmadov, U., Picard, D., and Bartl, J. (2021). The Long Non-coding RNA HOTAIRM1 Promotes Tumor Aggressiveness and Radiotherapy Resistance in Glioblastoma. Cel Death Dis. 12 (10), 1–11. doi:10.1038/s41419-021-04146-0
Amodio, N., Raimondi, L., Juli, G., Stamato, M. A., Caracciolo, D., Tagliaferri, P., et al. (2018). MALAT1: a Druggable Long Non-coding RNA for Targeted Anti-cancer Approaches. J. Hematol. Oncol. 11 (1), 63–19. doi:10.1186/s13045-018-0606-4
Bi, Z., Li, Q., Dinglin, X., Xu, Y., You, K., Hong, H., et al. (2020). Nanoparticles (NPs)-Meditated LncRNA AFAP1-AS1 Silencing to Block Wnt/β-Catenin Signaling Pathway for Synergistic Reversal of Radioresistance and Effective Cancer Radiotherapy. Adv. Sci. (Weinh) 7 (18), 2000915. doi:10.1002/advs.202000915
Brownmiller, T., Juric, J. A., Ivey, A. D., Harvey, B. M., Westemeier, E. S., Winters, M. T., et al. (2020). Y Chromosome LncRNA Are Involved in Radiation Response of Male Non-small Cell Lung Cancer Cells. Cancer Res. 80 (19), 4046–4057. doi:10.1158/0008-5472.CAN-19-4032
Cargnello, M., and Roux, P. P. (2011). Activation and Function of the MAPKs and Their Substrates, the MAPK-Activated Protein Kinases. Microbiol. Mol. Biol. Rev. 75 (1), 50–83. doi:10.1128/MMBR.00031-10
Carrera, M., Bitu, C. C., de Oliveira, C. E., Cervigne, N. K., Graner, E., Manninen, A., et al. (2015). HOXA10 Controls Proliferation, Migration and Invasion in Oral Squamous Cell Carcinoma. Int. J. Clin. Exp. Pathol. 8 (4), 3613–3623.
Chen, C., Wang, K., Wang, Q., and Wang, X. (2018). LncRNA HULC Mediates Radioresistance via Autophagy in Prostate Cancer Cells. Braz. J. Med. Biol. Res. 51, e7080. doi:10.1590/1414-431x20187080
Chen, J., Shen, Z., Zheng, Y., Wang, S., and Mao, W. (2015). Radiotherapy Induced Lewis Lung Cancer Cell Apoptosis via Inactivating β-catenin Mediated by Upregulated HOTAIR. Int. J. Clin. Exp. Pathol. 8 (7), 7878–7886.
Chen, M., Liu, P., Chen, Y., Chen, Z., Shen, M., Liu, X., et al. (2018). Long Noncoding RNA FAM201A Mediates the Radiosensitivity of Esophageal Squamous Cell Cancer by Regulating ATM and mTOR Expression via miR-101. Front. Genet. 9, 611. doi:10.3389/fgene.2018.00611
Chen, W., Yu, J., and Xie, R. (2021). Roles of the SNHG7/microRNA-9-5p/DPP4 ceRNA Network in the Growth and 131 I Resistance of Thyroid Carcinoma Cells through PI3K/Akt Activation. Oncol. Rep. 45 (4), 1. doi:10.3892/or.2021.7954
Chen, Y., Shen, Z., Zhi, Y., Zhou, H., Zhang, K., Wang, T., et al. (2018). Long Non-coding RNA ROR Promotes Radioresistance in Hepatocelluar Carcinoma Cells by Acting as a ceRNA for microRNA-145 to Regulate RAD18 Expression. Arch. Biochem. Biophys. 645, 117–125. doi:10.1016/j.abb.2018.03.018
Cheng, W., Shi, X., Lin, M., Yao, Q., Ma, J., and Li, J. (2020). LncRNA MAGI2-AS3 Overexpression Sensitizes Esophageal Cancer Cells to Irradiation through Down-Regulation of HOXB7 via EZH2. Front. Cel Develop. Biol. 8. doi:10.3389/fcell.2020.552822
Cotta-Ramusino, C., McDonald, E. R., Hurov, K., Sowa, M. E., Harper, J. W., and Elledge, S. J. (2011). A DNA Damage Response Screen Identifies RHINO, a 9-1-1 and TopBP1 Interacting Protein Required for ATR Signaling. Science 332 (6035), 1313–1317. doi:10.1126/science.1203430
Cui, X., Xiao, D., Cui, Y., and Wang, X. (2019). Exosomes-derived Long Non-coding RNA HOTAIR Reduces Laryngeal Cancer Radiosensitivity by Regulating microRNA-454-3p/E2F2 axis. Onco Targets Ther. 12, 10827–10839. doi:10.2147/OTT.S224881
Cui, Y., Zheng, Y., Lu, Y., Zhang, M., Yang, L., and Li, W. (2021). LINC01224 Facilitates the Proliferation and Inhibits the Radiosensitivity of Melanoma Cells through the miR‐193a‐5p/NR1D2 axis. Kaohsiung J. Med. Sci. 38 (3), 196–206. doi:10.1002/kjm2.12467
Delaney, G., Jacob, S., Featherstone, C., and Barton, M. (2005). The Role of Radiotherapy in Cancer Treatment: Estimating Optimal Utilization from a Review of Evidence-Based Clinical Guidelines. Cancer 104 (6), 1129–1137. doi:10.1002/cncr.21324
Derrien, T., Johnson, R., Bussotti, G., Tanzer, A., Djebali, S., Tilgner, H., et al. (2012). The GENCODE V7 Catalog of Human Long Noncoding RNAs: Analysis of Their Gene Structure, Evolution, and Expression. Genome Res. 22 (9), 1775–1789. doi:10.1101/gr.132159.111
Di, W., Li, Q., Shen, W., Guo, H., and Zhao, S. (2017). The Long Non-coding RNA HOTAIR Promotes Thyroid Cancer Cell Growth, Invasion and Migration through the miR-1-CCND2 axis. Am. J. Cancer Res. 7 (6), 1298–1309.
Drost, J., and Clevers, H. (2018). Organoids in Cancer Research. Nat. Rev. Cancer 18 (7), 407–418. doi:10.1038/s41568-018-0007-6
Fardi, M., Alivand, M., Baradaran, B., Farshdousti Hagh, M., and Solali, S. (2019). The Crucial Role of ZEB2: From Development to Epithelial-To-Mesenchymal Transition and Cancer Complexity. J. Cel Physiol 234 (9), 14783–14799. doi:10.1002/jcp.28277
Gao, J., Liu, L., Li, G., Cai, M., Tan, C., Han, X., et al. (2019). LncRNA GAS5 Confers the Radio Sensitivity of Cervical Cancer Cells via Regulating miR-106b/IER3 axis. Int. J. Biol. Macromol 126, 994–1001. doi:10.1016/j.ijbiomac.2018.12.176
Gao, W., Qiao, M., and Luo, K. (2021). Long Noncoding RNA TP53TG1 Contributes to Radioresistance of Glioma Cells via miR-524-5p/RAB5A Axis. Cancer Biother. Radiopharm. 36 (7), 600–612. doi:10.1089/cbr.2020.3567
Ge, X., Gu, Y., Li, D., Jiang, M., Zhao, S., Li, Z., et al. (2020). Knockdown of lncRNA PCAT1 Enhances Radiosensitivity of Cervical Cancer by Regulating miR-128/GOLM1 Axis. Onco Targets Ther. 13, 10373–10385. doi:10.2147/OTT.S263728
Gu, J., Lu, H., Tippin, B., Shimazaki, N., Goodman, M. F., and Lieber, M. R. (2007). XRCC4:DNA Ligase IV Can Ligate Incompatible DNA Ends and Can Ligate across Gaps. EMBO J. 26 (4), 1010–1023. doi:10.1038/sj.emboj.7601559
Guo, Y. J., Pan, W. W., Liu, S. B., Shen, Z. F., Xu, Y., and Hu, L. L. (2020). ERK/MAPK Signalling Pathway and Tumorigenesis. Exp. Ther. Med. 19 (3), 1997–2007. doi:10.3892/etm.2020.8454
Guo, Z., Wang, Y-H., and Xu, H. (2021). LncRNA Linc00312 Suppresses Radiotherapy Resistance by Targeting DNA-PKcs and Impairing DNA Damage Repair in Nasopharyngeal Carcinoma. Cel Death Dis. 12 (1), 1–15. doi:10.1038/s41419-020-03302-2
Han, D., Wang, J., and Cheng, G. (2018). LncRNA NEAT1 Enhances the Radio-Resistance of Cervical Cancer via miR-193b-3p/CCND1 axis. Oncotarget 9 (2), 2395–2409. doi:10.18632/oncotarget.23416
Han, F., Yang, S., Wang, W., Huang, X., Huang, D., and Chen, S. (2020). Silencing of lncRNA LINC00857 Enhances BIRC5-dependent Radio-Sensitivity of Lung Adenocarcinoma Cells by Recruiting NF-Κb1. Mol. Ther. Nucleic Acids 22, 981–993. doi:10.1016/j.omtn.2020.09.020
Han, Y., Li, F., Xie, J., Wang, Y., and Zhang, H. (2020). PVT1 Mediates Cell Proliferation, Apoptosis and Radioresistance in Nasopharyngeal Carcinoma through Regulating miR-515-5p/PIK3CA axis. Cancer Manag. Res. 12, 10077–10090. doi:10.2147/CMAR.S257583
Han, Y. Y., Liu, K., Xie, J., Li, F., Wang, Y., and Yan, B. (2020). LINC00114 Promoted Nasopharyngeal Carcinoma Progression and Radioresistance In Vitro and In Vivo through Regulating ERK/JNK Signaling Pathway via Targeting miR-203. Eur. Rev. Med. Pharmacol. Sci. 24 (5), 2491–2504. doi:10.26355/eurrev_202003_20517
He, H., Song, X., Yang, Z., Mao, Y., Zhang, K., Wang, Y., et al. (2020). Upregulation of KCNQ1OT1 Promotes Resistance to Stereotactic Body Radiotherapy in Lung Adenocarcinoma by Inducing ATG5/ATG12-Mediated Autophagy via miR-372-3p. Cell Death Dis 11 (10), 883–914. doi:10.1038/s41419-020-03083-8
Hirai, H., Roussel, M. F., Kato, J. Y., Ashmun, R. A., and Sherr, C. J. (1995). Novel INK4 Proteins, P19 and P18, Are Specific Inhibitors of the Cyclin D-dependent Kinases CDK4 and CDK6. Mol. Cel Biol 15 (5), 2672–2681. doi:10.1128/mcb.15.5.2672
Hou, J., Wang, Y., Zhang, H., Hu, Y., Xin, X., and Li, X. (2020). Silencing of LINC00461 Enhances Radiosensitivity of Lung Adenocarcinoma Cells by Down-Regulating HOXA10 via microRNA-195. J. Cel Mol Med 24 (5), 2879–2890. doi:10.1111/jcmm.14859
Jares, P., Colomer, D., and Campo, E. (2007). Genetic and Molecular Pathogenesis of Mantle Cell Lymphoma: Perspectives for New Targeted Therapeutics. Nat. Rev. Cancer 7 (10), 750–762. doi:10.1038/nrc2230
Jia, J., Zhang, X., Zhan, D., Li, J., Li, Z., Li, H., et al. (2019). LncRNA H19 Interacted with miR‐130a‐3p and miR‐17‐5p to Modify Radio‐resistance and Chemo‐sensitivity of Cardiac Carcinoma Cells. Cancer Med. 8 (4), 1604–1618. doi:10.1002/cam4.1860
Jiang, G., Yu, H., Li, Z., and Zhang, F. (2021). lncRNA Cytoskeleton Regulator Reduces Non-small Cell Lung Cancer Radiosensitivity by Downregulating miRNA-206 and Activating Prothymosin α. Int. J. Oncol. 59 (5), 1–15. doi:10.3892/ijo.2021.5268
Jiang, H., Hu, X., Zhang, H., and Li, W. (2017). Down-regulation of LncRNA TUG1 Enhances Radiosensitivity in Bladder Cancer via Suppressing HMGB1 Expression. Radiat. Oncol. 12 (1), 65–10. doi:10.1186/s13014-017-0802-3
Jiang, Y., Jin, S., Tan, S., Xue, Y., and Cao, X. (2020). Long Noncoding RNA NEAT1 Regulates Radio-Sensitivity via microRNA-27b-3p in Gastric Cancer. Cancer Cel Int 20 (1), 581–612. doi:10.1186/s12935-020-01655-4
Jin, C., Yan, B., Lu, Q., Lin, Y., and Ma, L. (2016). The Role of MALAT1/miR-1/slug axis on Radioresistance in Nasopharyngeal Carcinoma. Tumour Biol. 37 (3), 4025–4033. doi:10.1007/s13277-015-4227-z
Jin, H., Suh, D. S., Kim, T. H., Yeom, J. H., Lee, K., and Bae, J. (2015). IER3 Is a Crucial Mediator of TAp73β-Induced Apoptosis in Cervical Cancer and Confers Etoposide Sensitivity. Sci. Rep. 5 (1), 8367–8411. doi:10.1038/srep08367
Jin, Q., Hu, H., and Yan, S. (2021). lncRNA MIR22HG-Derived miR-22-5p Enhances the Radiosensitivity of Hepatocellular Carcinoma by Increasing Histone Acetylation through the Inhibition of HDAC2 Activity. Front. Oncol. 11, 56. doi:10.3389/fonc.2021.572585
Jing, L., Yuan, W., Ruofan, D., Jinjin, Y., and Haifeng, Q. (2015). HOTAIR Enhanced Aggressive Biological Behaviors and Induced Radio-Resistance via Inhibiting P21 in Cervical Cancer. Tumour Biol. 36 (5), 3611–3619. doi:10.1007/s13277-014-2998-2
Li, C., Liu, H., Wei, R., Liu, Z., Chen, H., Guan, X., et al. (2021). LncRNA EGOT/miR-211-5p Affected Radiosensitivity of Rectal Cancer by Competitively Regulating ErbB4. Onco Targets Ther. 14, 2867–2878. doi:10.2147/OTT.S256989
Li, J., Ji, X., and Wang, H. (2018). Targeting Long Noncoding RNA HMMR-AS1 Suppresses and Radiosensitizes Glioblastoma. Neoplasia 20 (5), 456–466. doi:10.1016/j.neo.2018.02.010
Li, J., Liu, X., Nan, S., and Xu, C. (2020). Silencing of Long Non-coding RNA LINC00520 Promotes Radiosensitivity of Head and Neck Squamous Cell Carcinoma Cells. Free Radic. Res. 54 (4), 254–270. doi:10.1080/10715762.2020.1752373
Li, L., Lin, X., Xu, P., Jiao, Y., and Fu, P. (2020). LncRNA GAS5 Sponges miR-362-5p to Promote Sensitivity of Thyroid Cancer Cells to 131 I by Upregulating SMG1. IUBMB life 72 (11), 2420–2431. doi:10.1002/iub.2365
Li, N., Meng, D. D., Gao, L., Xu, Y., Liu, P. J., Tian, Y. W., et al. (2018). Overexpression of HOTAIR Leads to Radioresistance of Human Cervical Cancer via Promoting HIF-1α Expression. Radiat. Oncol. 13 (1), 210–219. doi:10.1186/s13014-018-1153-4
Li, W., Cui, Y., Ma, W., Wang, M., Cai, Y., and Jiang, Y. (2022). LncRNA RBPMS-AS1 Promotes NRGN Transcription to Enhance the Radiosensitivity of Glioblastoma through the microRNA-301a-3p/CAMTA1 axis. Transl Oncol. 15 (1), 101282. doi:10.1016/j.tranon.2021.101282
Li, Y., Peart, M. J., and Prives, C. (2009). Stxbp4 Regulates DeltaNp63 Stability by Suppression of RACK1-dependent Degradation. Mol. Cel Biol 29 (14), 3953–3963. doi:10.1128/MCB.00449-09
Li, Z., Zhou, Y., Tu, B., Bu, Y., Liu, A., and Kong, J. (2017). Long Noncoding RNA MALAT1 Affects the Efficacy of Radiotherapy for Esophageal Squamous Cell Carcinoma by Regulating Cks1 Expression. J. Oral Pathol. Med. 46 (8), 583–590. doi:10.1111/jop.12538
Liang, H., Zhao, Q., Zhu, Z., Zhang, C., and Zhang, H. (2021). Long Noncoding RNA LINC00958 Suppresses Apoptosis and Radiosensitivity of Colorectal Cancer through Targeting miR-422a.
Lin, W., Huang, Z., Xu, Y., Chen, X., Chen, T., Ye, Y., et al. (2020). A Three-lncRNA Signature Predicts Clinical Outcomes in Low-Grade Glioma Patients after Radiotherapy. Aging (Albany NY) 12 (10), 9188–9204. doi:10.18632/aging.103189
Liu, A. M., Zhu, Y., Huang, Z. W., Lei, L., Fu, S. Z., and Chen, Y. (2019). Long Noncoding RNA FAM201A Involves in Radioresistance of Non-small-cell Lung Cancer by Enhancing EGFR Expression via miR-370. Eur. Rev. Med. Pharmacol. Sci. 23 (13), 5802–5814. doi:10.26355/eurrev_201907_18319
Liu, C., Wang, X., and Zhang, Y. (2019). The Roles of HK2 on Tumorigenesis of Cervical Cancer. Technol. Cancer Res. Treat. 18, 1533033819871306. doi:10.1177/1533033819871306
Liu, H., Zheng, W., Chen, Q., Zhou, Y., Pan, Y., Zhang, J., et al. (2021). lncRNA CASC19 Contributes to Radioresistance of Nasopharyngeal Carcinoma by Promoting Autophagy via AMPK-mTOR Pathway. Int. J. Mol. Sci. 22 (3), 1407. doi:10.3390/ijms22031407
Liu, J., Zhou, R., Deng, M., Xue, N., Li, T., Guo, Y., et al. (2021). Long Non-coding RNA DIO3OS Binds to microRNA-130b to Restore Radiosensitivity in Esophageal Squamous Cell Carcinoma by Upregulating PAX9. Cancer Gene Ther., 1–12. doi:10.1038/s41417-021-00344-2
Liu, L., Zhu, Y., Liu, A. M., Feng, Y., and Chen, Y. (2019). Long Noncoding RNA LINC00511 Involves in Breast Cancer Recurrence and Radioresistance by Regulating STXBP4 Expression via miR-185. Eur. Rev. Med. Pharmacol. Sci. 23 (17), 7457–7468. doi:10.26355/eurrev_201909_18855
Liu, R., Zhang, Q., Shen, L., Chen, S., He, J., Wang, D., et al. (2020). Long Noncoding RNA Lnc-RI Regulates DNA Damage Repair and Radiation Sensitivity of CRC Cells through NHEJ Pathway. Cell Biol Toxicol 36 (5), 493–507. doi:10.1007/s10565-020-09524-6
Liu, S., Zhan, N., and Gao, C. (2021). Long Noncoding RNA CBR3-AS1 Mediates Tumorigenesis and Radiosensitivity of Non-small Cell Lung Cancer through Redox and DNA Repair by CBR3-AS1/miR-409-3p/SOD1 axis. Cancer letters.
Liu, Y., Chen, X., Chen, X., Liu, J., Gu, H., Fan, R., et al. (2020). Long Non-coding RNA HOTAIR Knockdown Enhances Radiosensitivity through Regulating microRNA-93/ATG12 axis in Colorectal Cancer. Cel Death Dis 11 (3), 175–214. doi:10.1038/s41419-020-2268-8
Liu, Y., He, D., Xiao, M., Zhu, Y., Zhou, J., and Cao, K. (2021). Long Noncoding RNA LINC00518 Induces Radioresistance by Regulating Glycolysis through an miR-33a-3p/HIF-1α Negative Feedback Loop in Melanoma. Cel Death Dis. 12 (3), 1–19. doi:10.1038/s41419-021-03523-z
Lu, M. D., Liu, D., and Li, Y. X. (2020). LINC01436 Promotes the Progression of Gastric Cancer via Regulating miR-513a-5p/APE1 Axis. Onco Targets Ther. 13, 10607–10619. doi:10.2147/OTT.S257747
Ma, X., Wang, Z., Ren, H., Bao, X., Zhang, Y., Wang, B., et al. (2020). Long Non-coding RNA GAS5 Suppresses Tumor Progression and Enhances the Radiosensitivity of Prostate Cancer through the miR-320a/RAB21 Axis. Cancer Manag. Res. 12, 8833–8845. doi:10.2147/CMAR.S244123
Ma, X., Zhou, J., Liu, J., Wu, G., Yu, Y., Zhu, H., et al. (2018). LncRNA ANCR Promotes Proliferation and Radiation Resistance of Nasopharyngeal Carcinoma by Inhibiting PTEN Expression. Onco Targets Ther. 11, 8399–8408. doi:10.2147/OTT.S182573
Mahajan, K., and Mahajan, N. P. (2013). ACK1 Tyrosine Kinase: Targeted Inhibition to Block Cancer Cell Proliferation. Cancer Lett. 338 (2), 185–192. doi:10.1016/j.canlet.2013.04.004
Malicet, C., Giroux, V., Vasseur, S., Dagorn, J. C., Neira, J. L., and Iovanna, J. L. (2006). Regulation of Apoptosis by the P8/prothymosin Alpha Complex. Proc. Natl. Acad. Sci. U S A. 103 (8), 2671–2676. doi:10.1073/pnas.0508955103
Maten, M. V., Reijnen, C., Pijnenborg, J. M. A., and Zegers, M. M. (2019). L1 Cell Adhesion Molecule in Cancer, a Systematic Review on Domain-specific Functions. Int. J. Mol. Sci. 20 (17), 4180. doi:10.3390/ijms20174180
Mercer, T. R., Dinger, M. E., and Mattick, J. S. (2009). Long Non-coding RNAs: Insights into Functions. Nat. Rev. Genet. 10 (3), 155–159. doi:10.1038/nrg2521
Moher, D., Liberati, A., Tetzlaff, J., Altman, D. G., and Group, P. (2009). Preferred Reporting Items for Systematic Reviews and Meta-Analyses: the PRISMA Statement. BMJ 339 (7), b2535. doi:10.1136/bmj.b2535
Mou, L., Wang, L., Zhang, S., and Wang, Q. (2021). Long Noncoding RNA LINC01410 Suppresses Tumorigenesis and Enhances Radiosensitivity in Neuroblastoma Cells through Regulating miR-545-3p/HK2 Axis. Onco Targets Ther. 14, 3225–3238. doi:10.2147/OTT.S297969
Nascimento, F. J. A., and Bradshaw, C. (2016). Direct and Indirect Effects of Ionizing Radiation on Grazer-Phytoplankton Interactions. J. Environ. Radioact 155-156, 63–70. doi:10.1016/j.jenvrad.2016.02.007
Podralska, M., Ciesielska, S., Kluiver, J., van den Berg, A., Dzikiewicz-Krawczyk, A., and Slezak-Prochazka, I. (2020). Non-coding RNAs in Cancer Radiosensitivity: MicroRNAs and Lncrnas as Regulators of Radiation-Induced Signaling Pathways. Cancers (Basel) 12 (6), 1662. doi:10.3390/cancers12061662
Qian, L., Fei, Q., Zhang, H., Qiu, M., Zhang, B., Wang, Q., et al. (2020). lncRNA HOTAIR Promotes DNA Repair and Radioresistance of Breast Cancer via EZH2. DNA Cel Biol 39 (12), 2166–2173. doi:10.1089/dna.2020.5771
Qin, B., Yu, J., Nowsheen, S., Wang, M., Tu, X., Liu, T., et al. (2019). UFL1 Promotes Histone H4 Ufmylation and ATM Activation. Nat. Commun. 10 (1), 1242–1313. doi:10.1038/s41467-019-09175-0
Rinn, J. L., and Chang, H. Y. (2012). Genome Regulation by Long Noncoding RNAs. Annu. Rev. Biochem. 81, 145–166. doi:10.1146/annurev-biochem-051410-092902
Santos, T. G., Martins, V. R., and Hajj, G. N. M. (2017). Unconventional Secretion of Heat Shock Proteins in Cancer. Int. J. Mol. Sci. 18 (5), 946. doi:10.3390/ijms18050946
Schaue, D., and McBride, W. H. (2015). Opportunities and Challenges of Radiotherapy for Treating Cancer. Nat. Rev. Clin. Oncol. 12 (9), 527–540. doi:10.1038/nrclinonc.2015.120
Schraivogel, D., Weinmann, L., Beier, D., Tabatabai, G., Eichner, A., Zhu, J. Y., et al. (2011). CAMTA1 Is a Novel Tumour Suppressor Regulated by miR-9/9* in Glioblastoma Stem Cells. EMBO J. 30 (20), 4309–4322. doi:10.1038/emboj.2011.301
Segers, V. F. M., Dugaucquier, L., Feyen, E., Shakeri, H., and De Keulenaer, G. W. (2020). The Role of ErbB4 in Cancer. Cel Oncol (Dordr) 43 (3), 335–352. doi:10.1007/s13402-020-00499-4
Semenza, G. L. (2003). Targeting HIF-1 for Cancer Therapy. Nat. Rev. Cancer 3 (10), 721–732. doi:10.1038/nrc1187
Sharma, M., Sharma, R., Ge, X. L., Fish, B. L., McCarthy, E. T., Savin, V. J., et al. (2001). Early Detection of Radiation-Induced Glomerular Injury by Albumin Permeability Assay. Radiat. Res. 155 (3), 474–480. doi:10.1667/0033-7587(2001)155[0474:edorig]2.0.co;2
Song, J., Zhang, S., Sun, Y., Gu, J., Ye, Z., Sun, X., et al. (2021). A Radioresponse-Related lncRNA Biomarker Signature for Risk Classification and Prognosis Prediction in Non-small-cell Lung Cancer. J. Oncol. 2021, 4338838. doi:10.1155/2021/4338838
Song, W., Zhang, J., Xia, Q., and Sun, M. (2019). Down-regulated lncRNA TP73-AS1 Reduces Radioresistance in Hepatocellular Carcinoma via the PTEN/Akt Signaling Pathway. Cell Cycle 18 (22), 3177–3188. doi:10.1080/15384101.2019.1671089
Sun, Y., Wang, J., and Ma, Y. (2021). Radiation Induces NORAD Expression to Promote ESCC Radiotherapy Resistance via EEPD1/ATR/Chk1 Signalling and by Inhibiting Pri-miR-199a1 Processing and the Exosomal Transfer of miR-199a-5p. J. Exp. Clin. Cancer Res. 40 (1), 1–22. doi:10.1186/s13046-021-02084-5
Tan, J., Qiu, K., Li, M., and Liang, Y. (2015). Double-negative Feedback Loop between Long Non-coding RNA TUG1 and miR-145 Promotes Epithelial to Mesenchymal Transition and Radioresistance in Human Bladder Cancer Cells. FEBS Lett. 589 (20), 3175–3181. doi:10.1016/j.febslet.2015.08.020
Tang, T., and Shan, G. (2019). DGCR5 Promotes Cancer Stem Cell-like Properties of Radioresistant Laryngeal Carcinoma Cells by Sponging miR-506 via Wnt Pathway. J. Cel Physiol 234 (10), 18423–18431. doi:10.1002/jcp.28478
Tang, T., Shan, G., and Zeng, F. (2019). Knockdown of DGCR5 Enhances the Radiosensitivity of Human Laryngeal Carcinoma Cells via Inducing miR-195. J. Cel Physiol 234 (8), 12918–12925. doi:10.1002/jcp.27958
Tang, T., Wang, L. X., Yang, M. L., and Zhang, R. M. (2020). lncRNA TPTEP1 Inhibits Stemness and Radioresistance of Glioma through miR-106a-5p-mediated P38 MAPK S-ignaling. Mol. Med. Rep. 22 (6), 4857–4867. doi:10.3892/mmr.2020.11542
Tian, W., Zhang, Y., Liu, H., Jin, H., and Sun, T. (2021). LINC01123 Potentially Correlates with Radioresistance in Glioma through the miR‐151a/CENPB axis. Neuropathology.
Tragante, V., Moore, J. H., and Asselbergs, F. W. (2014). The ENCODE Project and Perspectives on Pathways. Genet. Epidemiol. 38 (4), 275–280. doi:10.1002/gepi.21802
Wang, B., Wang, K., Jin, T., Xu, Q., He, Y., Cui, B., et al. (2020). NCK1-AS1 Enhances Glioma Cell Proliferation, Radioresistance and Chemoresistance via miR-22-3p/IGF1R ceRNA Pathway. Biomed. Pharmacother. 129, 110395. doi:10.1016/j.biopha.2020.110395
Wang, B., Zheng, J., Li, R., Tian, Y., Lin, J., Liang, Y., et al. (2019). Long Noncoding RNA LINC02582 Acts Downstream of miR-200c to Promote Radioresistance through CHK1 in Breast Cancer Cells. Cel Death Dis 10 (10), 764–815. doi:10.1038/s41419-019-1996-0
Wang, C., Shao, S., Deng, L., Wang, S., and Zhang, Y. (2020). LncRNA SNHG12 Regulates the Radiosensitivity of Cervical Cancer through the miR-148a/CDK1 Pathway. Cancer Cel Int 20 (1), 554–612. doi:10.1186/s12935-020-01654-5
Wang, D., and Hu, Y. (2019). Long Non-coding RNA PVT1 Competitively Binds microRNA-424-5p to Regulate CARM1 in Radiosensitivity of Non-small-cell Lung Cancer. Mol. Ther. Nucleic Acids 16, 130–140. doi:10.1016/j.omtn.2018.12.006
Wang, J. S., Wang, H. J., and Qian, H. L. (2018). Biological Effects of Radiation on Cancer Cells. Mil. Med. Res. 5 (1), 20–10. doi:10.1186/s40779-018-0167-4
Wang, P., Yang, Z., Ye, T., Shao, F., Li, J., Sun, N., et al. (2020). lncTUG1/miR-144-3p Affect the Radiosensitivity of Esophageal Squamous Cell Carcinoma by Competitively Regulating C-MET. J. Exp. Clin. Cancer Res. 39 (1), 7–12. doi:10.1186/s13046-019-1519-y
Wang, Y., Chen, W., Lian, J., Zhang, H., Yu, B., Zhang, M., et al. (2020). The lncRNA PVT1 Regulates Nasopharyngeal Carcinoma Cell Proliferation via Activating the KAT2A Acetyltransferase and Stabilizing HIF-1α. Cell Death Differ 27 (2), 695–710. doi:10.1038/s41418-019-0381-y
Wang, Y., Wang, C., Chen, C., Wu, F., Shen, P., Zhang, P., et al. (2017). Long Non-coding RNA NEAT1 Regulates Epithelial Membrane Protein 2 Expression to Repress Nasopharyngeal Carcinoma Migration and Irradiation-Resistance through miR-101-3p as a Competing Endogenous RNA Mechanism. Oncotarget 8 (41), 70156–70171. doi:10.18632/oncotarget.19596
Wang, Y-h., Guo, Z., and An, L. (2021). LINC-PINT Impedes DNA Repair and Enhances Radiotherapeutic Response by Targeting DNA-PKcs in Nasopharyngeal Cancer. Cel Death Dis. 12 (5), 1–16. doi:10.1038/s41419-021-03728-2
Wang, Y. P., Li, H. Q., Chen, J. X., Kong, F. G., Mo, Z. H., Wang, J. Z., et al. (2020). Overexpression of XIST Facilitates Cell Proliferation, Invasion and Suppresses Cell Apoptosis by Reducing Radio-Sensitivity of Glioma Cells via miR-329-3p/CREB1 axis. Eur. Rev. Med. Pharmacol. Sci. 24 (6), 3190–3203. doi:10.26355/eurrev_202003_20686
Wang, Z., Liu, L., Du, Y., Mi, Y., and Wang, L. (2021). The HNF1A-AS1/miR-92a-3p axis Affects the Radiosensitivity of Non-small Cell Lung Cancer by Competitively Regulating the JNK Pathway. Cel Biol. Toxicol., 1–15. doi:10.1007/s10565-021-09595-z
Wichmann, A., Uyetake, L., and Su, T. T. (2010). E2F1 and E2F2 Have Opposite Effects on Radiation-Induced P53-independent Apoptosis in Drosophila. Dev. Biol. 346 (1), 80–89. doi:10.1016/j.ydbio.2010.07.023
Wierstra, I. (2013). FOXM1 (Forkhead Box M1) in Tumorigenesis: Overexpression in Human Cancer, Implication in Tumorigenesis, Oncogenic Functions, Tumor-Suppressive Properties, and Target of Anticancer Therapy. Adv. Cancer Res. 119, 191–419. doi:10.1016/B978-0-12-407190-2.00016-2
Wu, D., Li, Y., Zhang, H., and Hu, X. (2017). Knockdown of Lncrna PVT1 Enhances Radiosensitivity in Non-small Cell Lung Cancer by Sponging Mir-195. Cell Physiol Biochem 42 (6), 2453–2466. doi:10.1159/000480209
Wu, S., Shi, Y., Mulligan, P., Gay, F., Landry, J., Liu, H., et al. (2007). A YY1-INO80 Complex Regulates Genomic Stability through Homologous Recombination-Based Repair. Nat. Struct. Mol. Biol. 14 (12), 1165–1172. doi:10.1038/nsmb1332
Wu, Y., Lee, S. H., Williamson, E. A., Reinert, B. L., Cho, J. H., Xia, F., et al. (2015). EEPD1 Rescues Stressed Replication forks and Maintains Genome Stability by Promoting End Resection and Homologous Recombination Repair. Plos Genet. 11 (12), e1005675. doi:10.1371/journal.pgen.1005675
Xiao, J., Lin, L., Luo, D., Shi, L., Chen, W., Fan, H., et al. (2020). Long Noncoding RNA TRPM2-AS Acts as a microRNA Sponge of miR-612 to Promote Gastric Cancer Progression and Radioresistance. Oncogenesis 9 (3), 29–15. doi:10.1038/s41389-020-0215-2
Xiu, D., Liu, L., Cheng, M., Sun, X., and Ma, X. (2020). Knockdown of lncRNA TUG1 Enhances Radiosensitivity of Prostate Cancer via the TUG1/miR-139-5p/SMC1A axis. Onco Targets Ther. 13, 2319–2331. doi:10.2147/OTT.S236860
Xiu, M. X., and Liu, Y. M. (2019). The Role of Oncogenic Notch2 Signaling in Cancer: a Novel Therapeutic Target. Am. J. Cancer Res. 9 (5), 837–854.
Xue, Y., Ni, T., Jiang, Y., and Li, Y. (2017). Long Noncoding RNA GAS5 Inhibits Tumorigenesis and Enhances Radiosensitivity by Suppressing miR-135b Expression in Non-small Cell Lung Cancer. Oncol. Res. 25 (8), 1305–1316. doi:10.3727/096504017X14850182723737
Yamashita, A., Ohnishi, T., Kashima, I., Taya, Y., and Ohno, S. (2001). Human SMG-1, a Novel Phosphatidylinositol 3-Kinase-Related Protein Kinase, Associates with Components of the mRNA Surveillance Complex and Is Involved in the Regulation of Nonsense-Mediated mRNA Decay. Genes Dev. 15 (17), 2215–2228. doi:10.1101/gad.913001
Yang, B., Ma, H., and Bian, Y. (2021). LINC00261 Inhibits Esophageal Cancer Radioresistance by Down-Regulating microRNA-552-3p and Promoting DIRAS1. Cancer Manag. Res. 13, 8559–8573. doi:10.2147/CMAR.S332640
Yang, G., Lu, X., and Yuan, L. (2014). LncRNA: a Link between RNA and Cancer. Biochim. Biophys. Acta 1839 (11), 1097–1109. doi:10.1016/j.bbagrm.2014.08.012
Yang, G., Zhou, L., Xu, Q., Meng, F., Wan, Y., Meng, X., et al. (2020). LncRNA KCNQ1OT1 Inhibits the Radiosensitivity and Promotes the Tumorigenesis of Hepatocellular Carcinoma via the miR-146a-5p/ACER3 axis. Cell Cycle 19 (19), 2519–2529. doi:10.1080/15384101.2020.1809259
Yang, H., Zhang, X., Zhao, Y., Sun, G., Zhang, J., Gao, Y., et al. (2020). Downregulation of lncRNA XIST Represses Tumor Growth and Boosts Radiosensitivity of Neuroblastoma via Modulation of the miR-375/L1CAM axis. Neurochem. Res. 45 (11), 2679–2690. doi:10.1007/s11064-020-03117-9
Yang, Q. S., Li, B., Xu, G., Yang, S. Q., Wang, P., Tang, H. H., et al. (2019). Long Noncoding RNA LINC00483/microRNA-144 Regulates Radiosensitivity and Epithelial-Mesenchymal Transition in Lung Adenocarcinoma by Interacting with HOXA10. J. Cel Physiol 234 (7), 11805–11821. doi:10.1002/jcp.27886
Yang, W., Yu, H., Shen, Y., Liu, Y., Yang, Z., and Sun, T. (2016). MiR-146b-5p Overexpression Attenuates Stemness and Radioresistance of Glioma Stem Cells by Targeting HuR/lincRNA-P21/β-Catenin Pathway. Oncotarget 7 (27), 41505–41526. doi:10.18632/oncotarget.9214
Yang, Y., and Bedford, M. T. (2013). Protein Arginine Methyltransferases and Cancer. Nat. Rev. Cancer 13 (1), 37–50. doi:10.1038/nrc3409
Yazdi, P. T., Wang, Y., Zhao, S., Patel, N., Lee, E. Y., and Qin, J. (2002). SMC1 Is a Downstream Effector in the ATM/NBS1 branch of the Human S-phase Checkpoint. Genes Dev. 16 (5), 571–582. doi:10.1101/gad.970702
Yi, L., Ouyang, L., Wang, S., Li, S. S., and Yang, X. M. (2019). Long Noncoding RNA PTPRG-AS1 Acts as a microRNA-194-3p Sponge to Regulate Radiosensitivity and Metastasis of Nasopharyngeal Carcinoma Cells via PRC1. J. Cel Physiol 234 (10), 19088–19102. doi:10.1002/jcp.28547
Yu, C., Liang, Y., Jin, Y., and Li, Q. (2021). LncRNA GAS5 Enhances Radiosensitivity of Hepatocellular Carcinoma and Restricts Tumor Growth and Metastasis by miR-144-5p/ATF2. Am. J. Transl Res. 13 (9), 10896–10907.
Yu, M., Li, W., Wang, Q., Wang, Y., and Lu, F. (2018). Circadian Regulator NR1D2 Regulates Glioblastoma Cell Proliferation and Motility. Oncogene 37 (35), 4838–4853. doi:10.1038/s41388-018-0319-8
Yu, Z., Wang, G., Zhang, C., Liu, Y., Chen, W., Wang, H., et al. (2020). LncRNA SBF2-AS1 Affects the Radiosensitivity of Non-small Cell Lung Cancer via Modulating microRNA-302a/MBNL3 axis. Cell cycle 19 (3), 300–316. doi:10.1080/15384101.2019.1708016
Yuan, J., Yin, Z., Tao, K., Wang, G., and Gao, J. (2018). Function of Insulin-like Growth Factor 1 Receptor in Cancer Resistance to Chemotherapy. Oncol. Lett. 15 (1), 41–47. doi:10.3892/ol.2017.7276
Yun, C., and Lee, S. (2018). The Roles of Autophagy in Cancer. Ijms 19 (11), 3466. doi:10.3390/ijms19113466
Zhan, P., Zhang, B., Xi, G. M., Wu, Y., Liu, H. B., Liu, Y. F., et al. (2017). PRC1 Contributes to Tumorigenesis of Lung Adenocarcinoma in Association with the Wnt/β-Catenin Signaling Pathway. Mol. Cancer 16 (1), 108–115. doi:10.1186/s12943-017-0682-z
Zhan, T., Rindtorff, N., and Boutros, M. (2017). Wnt Signaling in Cancer. Oncogene 36 (11), 1461–1473. doi:10.1038/onc.2016.304
Zhang, F., Sang, Y., and Chen, D. (2021). M2 Macrophage-Derived Exosomal Long Non-coding RNA AGAP2-AS1 Enhances Radiotherapy Immunity in Lung Cancer by Reducing microRNA-296 and Elevating NOTCH2. Cel Death Dis. 12 (5), 1–15. doi:10.1038/s41419-021-03700-0
Zhang, H., Hua, Y., Jiang, Z., Yue, J., Shi, M., Zhen, X., et al. (2019). Cancer-associated Fibroblast-Promoted LncRNA DNM3OS Confers Radioresistance by Regulating DNA Damage Response in Esophageal Squamous Cell Carcinoma. Clin. Cancer Res. 25 (6), 1989–2000. doi:10.1158/1078-0432.CCR-18-0773
Zhang, N., Zeng, X., Sun, C., Guo, H., Wang, T., Wei, L., et al. (2019). LncRNA LINC00963 Promotes Tumorigenesis and Radioresistance in Breast Cancer by Sponging miR-324-3p and Inducing ACK1 Expression. Mol. Ther. Nucleic Acids 18, 871–881. doi:10.1016/j.omtn.2019.09.033
Zhang, S., Wang, B., Xiao, H., Dong, J., Li, Y., Zhu, C., et al. (2020). LncRNA HOTAIR Enhances Breast Cancer Radioresistance through Facilitating HSPA1A Expression via Sequestering miR-449b-5p. Thorac. Cancer 11 (7), 1801–1816. doi:10.1111/1759-7714.13450
Zhang, X., Xie, K., Zhou, H., Wu, Y., Li, C., Liu, Y., et al. (2020). Role of Non-coding RNAs and RNA Modifiers in Cancer Therapy Resistance. Mol. Cancer 19 (1), 47–26. doi:10.1186/s12943-020-01171-z
Zhang, Y., Xu, L., Li, A., and Han, X. (2019). The Roles of ZEB1 in Tumorigenic Progression and Epigenetic Modifications. Biomed. Pharmacother. 110, 400–408. doi:10.1016/j.biopha.2018.11.112
Zhao, H., Zheng, G. H., Li, G. C., Xin, L., Wang, Y. S., Chen, Y., et al. (2019). Long Noncoding RNA LINC00958 Regulates Cell Sensitivity to Radiotherapy through RRM2 by Binding to microRNA-5095 in Cervical Cancer. J. Cel Physiol 234 (12), 23349–23359. doi:10.1002/jcp.28902
Zheng, J., Wang, B., Zheng, R., Zhang, J., Huang, C., Zheng, R., et al. (2020). Linc-RA1 Inhibits Autophagy and Promotes Radioresistance by Preventing H2Bub1/USP44 Combination in Glioma Cells. Cel Death Dis 11 (9), 758–814. doi:10.1038/s41419-020-02977-x
Zheng, R., Yao, Q., Ren, C., Liu, Y., Yang, H., Xie, G., et al. (2016). Upregulation of Long Noncoding RNA Small Nucleolar RNA Host Gene 18 Promotes Radioresistance of Glioma by Repressing Semaphorin 5A. Int. J. Radiat. Oncol. Biol. Phys. 96 (4), 877–887. doi:10.1016/j.ijrobp.2016.07.036
Zhong, Q., Chen, Y., and Chen, Z. (2020). LncRNA MINCR Regulates Irradiation Resistance in Nasopharyngeal Carcinoma Cells via the microRNA-223/ZEB1 axis. Cell Cycle 19 (1), 53–66. doi:10.1080/15384101.2019.1692176
Zhong, Z., Cao, Y., Yang, S., and Zhang, S. (2016). Overexpression of RRM2 in Gastric Cancer Cell Promotes Their Invasiveness via AKT/NF-κB Signaling Pathway. Pharmazie 71 (5), 280–284.
Zhou, X., Zeng, B., Li, Y., Wang, H., and Zhang, X. (2021). LINC02532 Contributes to Radiosensitivity in Clear Cell Renal Cell Carcinoma through the miR-654-5p/YY1 Axis. Molecules 26 (22), 7040. doi:10.3390/molecules26227040
Keywords: long non-coding RNA, cancer, radioresistance, radiosensitivity, systematic review
Citation: Wu W, Zhang S and He J (2022) The Mechanism of Long Non-coding RNA in Cancer Radioresistance/Radiosensitivity: A Systematic Review. Front. Pharmacol. 13:879704. doi: 10.3389/fphar.2022.879704
Received: 20 February 2022; Accepted: 04 April 2022;
Published: 05 May 2022.
Edited by:
Lei Wang, Queen’s University Belfast, United KingdomReviewed by:
Yanyong Yang, Second Military Medical University, ChinaCopyright © 2022 Wu, Zhang and He. This is an open-access article distributed under the terms of the Creative Commons Attribution License (CC BY). The use, distribution or reproduction in other forums is permitted, provided the original author(s) and the copyright owner(s) are credited and that the original publication in this journal is cited, in accordance with accepted academic practice. No use, distribution or reproduction is permitted which does not comply with these terms.
*Correspondence: Wenhan Wu, wwh@wuwenhan.tech