- 1Pharmacognosy Department, Faculty of Pharmacy, Fayoum University, Fayoum, Egypt
- 2Department of Medicinal Chemistry, Faculty of Pharmacy, King Salman International University (KSIU), South Sinai, Egypt
- 3Department of Pharmaceutics, Faculty of Pharmacy, Umm Al-Qura University, Makkah, Saudi Arabia
- 4Department of Pharmaceutical Sciences, College of Pharmacy, AlMaarefa University, Riyadh, Saudi Arabia
- 5School of Biotechnology, Badr University in Cairo, Badr City, Egypt
- 6Department of Pharmaceutical Chemistry, Faculty of Pharmacy, Kafrelsheikh University, Kafrelsheikh, Egypt
Beginning from December 2019, widespread COVID-19 has caused huge financial misfortunes and exceptional wellbeing emergencies across the globe. Discovering an effective and safe drug candidate for the treatment of COVID-19 and its associated symptoms became an urgent global demand, especially due to restricted information that has been discharged with respect to vaccine efficacy and safety in humans. Reviewing the recent research, olive leaves were selected as a potential co-therapy supplement for the treatment and improvement of clinical manifestations in COVID-19 patients. Olive leaves were reported to be rich in phenolic compounds such as oleuropein, hydroxytyrosol, verbascoside, apigenin-7-O-glucoside, and luteolin-7-O-glucoside and also triterpenoids such as maslinic, ursolic, and oleanolic acids that have been reported as anti–SARS-CoV-2 metabolites in recent computational and in vitro studies. In addition, olive leaf extract was previously reported in several in vivo studies for its anti-inflammatory, analgesic, antipyretic, immunomodulatory, and antithrombotic activities which are of great benefit in the control of associated inflammatory cytokine storm and disseminated intravascular coagulation in COVID-19 patients. In conclusion, the described biological activities of olive leaves alongside their biosafety, availability, and low price make them a potential candidate drug or supplement to control COVID-19 infection and are recommended for clinical investigation.
1 Introduction
Since December 2019, the COVID-19 pandemic resulted in huge economic deterioration and exceptional uncontrolled health crisis throughout the globe. The total number of COVID-19 cases worldwide until now is about 416,614,051cases and 5,844,097 deaths, as estimated by the WHO, on 17 February 2022 (https://covid19.who.int/). Although several vaccine candidates are now available, only few data have been released regarding the efficacy and safety of vaccines in humans, not to mention that the long-term adequacy of those vaccines still remain as an open address. Nowadays, there is a global trend toward the invention of drug leads that act against COVID-19 infection through different techniques such as in silico, in vitro, in vivo, and clinical studies of the drug candidates. However, until now, only one drug (Paxlovid®) has been approved recently, in December 2022, by the FDA for the treatment and prevention of COVID-19 infection (Parums, 2022); hence, there is a crucial requirement to develop antiviral agents capable of controlling the infection.
WHO reports revealed that COVID-19 disease is most spread in Europe (58%), Americas (23%), Southeast Asia (8%), Western Pacific (7%), Eastern Mediterranean (4%), and then Africa (1%) (O (2022). Weekly epidem, 2022). Additionally, according to the WHO, around 80% of the people in many third-world countries rely on conventional plant sources for their health issues (Ekor, 2014; Ganjhu et al., 2015). Natural products have been historically used for acute respiratory infections, and currently, different natural plant products are being investigated as antiviral agents. (Lin et al., 2014; Ganjhu et al., 2015).
The olive (Olea europaea L., family Oleaceae) is a small tree native to Asia, whose cultivation spreads to all the Mediterranean countries, Europe, Iran, and northern Africa (Cimato and Attilio, 2011; Hashmi et al., 2015; Özcan and Matthäus, 2017). Olive trees are abundant and ethnomedically used in the countries where COVID-19 infection is widespread (Hashmi et al., 2015). Olive leaves were reported to exhibit several biological activities such as antioxidant (Benavente-Garcıa et al., 2000; Soni et al., 2006), antihypertensive (Susalit et al., 2011), antihypercholesterolemic (Jemai et al., 2009), cardioprotective (Wang et al., 2008), anti-inflammatory (Khalatbary and Zarrinjoei, 2012), and anti-obesity (Santiago-Mora et al., 2011) activities.
Olive leaf extract was reported to be rich in phenolic compounds such as oleuropein, hydroxytyrosol, verbascoside, apigenin-7-O-glucoside, and luteolin-7-O-glucoside (Benavente-Garcıa et al., 2000; Goldsmith et al., 2014).
2 Phytochemistry Review
Several secondary metabolites with different chemical classification were reported and isolated from olive leaves, such as secoiridoids, flavonoids, triterpenoids, steroids, and lignans.
Oleuropein (1) has been reported from the methanolic and aqueous extracts of leaves of Olea europaea (Wichers et al., 2000; Goulas et al., 2009; Hashmi et al., 2015; Nassir et al., 2019). Oleuropein and other secoiridoids such as ligstroside (2) (Gariboldi et al., 1986; Hashmi et al., 2015), oleuricine A (3), oleuricine B (4) (Wang et al., 2009; Hashmi et al., 2015), oleuroside (5) (Movsumov, 1994; Duquesnoy et al., 2007; Hashmi et al., 2015), secologanoside, 6′-E-p-coumaroyl-secologanoside (comselogoside) (6) (Duquesnoy et al., 2007; Hashmi et al., 2015), 6′-O-[(2E)-2,6-dimethyl-8-hydroxy-2-octenoyloxy]-secologanoside (7) (Duquesnoy et al., 2007; Hashmi et al., 2015), oleoside (8) (Movsumov, 1994; Duquesnoy et al., 2007; Hashmi et al., 2015), secologanoside (9), elenolic acid methyl ester (10), hydroxytyrosol-elenolate (11) (Gariboldi et al., 1986; Hashmi et al., 2015), and 3,4-DHPEA-EDA (oleacein) (12) (Movsumov, 1994; Duquesnoy et al., 2007; Hashmi et al., 2015) were isolated from the extract of leaves of Olea europaea. The phenylethanoid precursors of oleuropein; hydroxytyrosol (13) and hydroxytyrosol acetate (14), were reported in high amount in olive leaves (Goulas et al., 2009) (Figure 1).
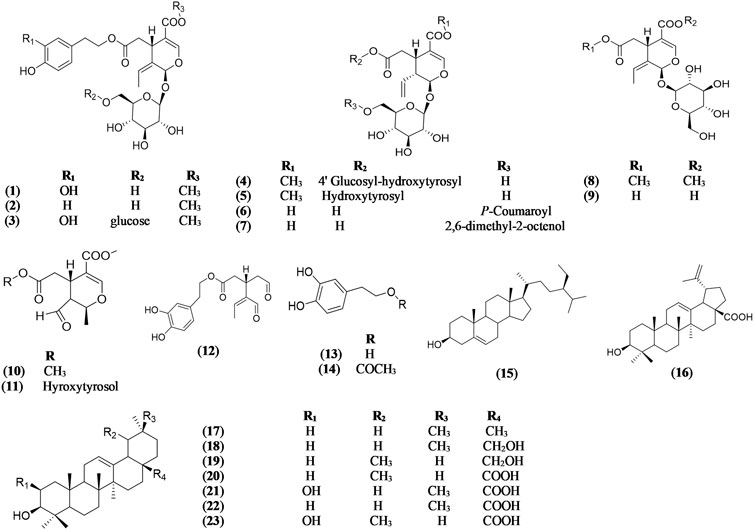
FIGURE 1. Chemical structures of secoiridoids, triterpenoids, and steroids reported in Olea europaea leaves.
The ethyl acetate fraction of O. europaea leaves resulted in the isolation of different steroids and triterpenoids such as β-sitosterol (15) (Mussini et al., 1975; Hashmi et al., 2015), betulinic acid (16) (Bianchi et al., 1992; Hashmi et al., 2015), β-amyrin (17) (Mussini et al., 1975; Wang et al., 2009; Hashmi et al., 2015), erythrodiol (18) (Duquesnoy et al., 2007; Hashmi et al., 2015), uvaol (19) (Bianchi et al., 1992; Hashmi et al., 2015), ursolic acid (20) (Bianchi et al., 1992; Hashmi et al., 2015), maslinic acid (21) (Bianchi et al., 1992; Hashmi et al., 2015), oleanolic acid (22) (Movsumov, 1994; Duquesnoy et al., 2007; Hashmi et al., 2015), and corosolic acid (23) (Bianchi et al., 1992; Hashmi et al., 2015) (Figure 1).
Flavonoids are also important phytochemical constituents reported to be isolated and detected in the extracts of Olea europaea leaves. Different types of flavone and flavonols such as aglycones and glycosides were reported in olive leaves such as kaempferol (24) (Özcan and Matthäus, 2017), quercetin (25) (Özcan and Matthäus, 2017), luteolin (26) (Goulas et al., 2009), diosmetin (27) (Savournin et al., 2001; Meirinhos et al., 2005; Hashmi et al., 2015), apigenin-7-O-glucoside (28) (Savournin et al., 2001; Meirinhos et al., 2005; Hashmi et al., 2015), apigenin-7-O-rutinoside (29) (Bouaziz et al., 2005; Meirinhos et al., 2005; Hashmi et al., 2015), rutin (30) (Bouaziz et al., 2005; Meirinhos et al., 2005; Hashmi et al., 2015), luteolin-4′-O-glucoside (31) (Goulas et al., 2009), luteolin-7-O-glucoside (32) (Bouaziz et al., 2005; Meirinhos et al., 2005; Goulas et al., 2009; Hashmi et al., 2015), luteolin-7,4′-O-diglucoside (33) (Savournin et al., 2001; Meirinhos et al., 2005; Hashmi et al., 2015), and quercitrin (34) (Özcan and Matthäus, 2017) (Figure 2).
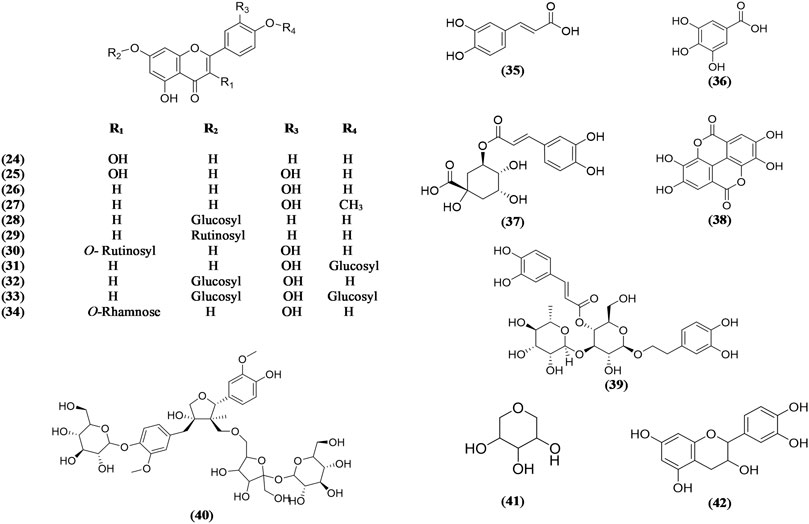
FIGURE 2. Chemical structures of flavonoids and phenolic compounds reported in Olea europaea leaves.
Several phenolic acids were also isolated from Olea europaea leaves, such as caffeic acid (35), gallic acid (36), chlorogenic acid (37), ellagic acid (38), and verbascoside (39) (Özcan and Matthäus, 2017) (Figure 2).
Other miscellaneous compounds were reported from the Olea europaea leaves such as 4′-O-β-D-glucosyl-9-O-(6″-deoxysaccharosyl) olivil (40) (Schumacher et al., 2002), 1,5 anhydroxylitol (41) (Campeol et al., 2004), and epicatechin (42) (Özcan and Matthäus, 2017) (Figure 2).
Several fatty constituents were reported in the hexane extract of Olea europaea leaves, including hydrocarbons, tocopherols, triglycerides, waxes, esters, esterols, terpenic alcohols, terpenic dialcohols, and lineal (Guinda et al., 2002).
3 Biology Review
3.1 Therapeutic Potential of Bioactive Compounds in the Olive Leaves Extract for the Management of COVID-19 Disease
Unlike other coronaviruses that caused several respiratory diseases, SARS-CoV-2 infection is not only a respiratory but also a systematic infection that exhibited severe immune response and resulted in multiorgan dysfunction and finally death (da Rosa Mesquita et al., 2021). The main symptoms of COVID-19 disease are fever, headache, fatigue, malaise, dry cough, and dyspnea with progression to pneumonia, diarrhea, back pain, and loss of smell and taste (da Rosa Mesquita et al., 2021; Zhou et al., 2020; Kabrah et al., 2021; Tawakul et al., 2021). The symptoms vary among patients depending on the viral load and virus strain (da Rosa Mesquita et al., 2021). The recent epidemiological studies detected certain people who are more vulnerable to COVID-19 infection, including older adults and people with serious health problems such as chronic lung disease, asthma, serious heart illness, and immuno-compromised patients (C (2020). Coronavirus D, 2020; Alyami et al., 2020).
The primary reason for most of the deaths occurring in COVID-19 patients is hyper-inflammation due to the associated cytokine storm, especially IL-6, which resulted in acute respiratory distress syndrome (Cron et al., 2021; Zawawi et al., 2021). Moreover, one of the most dangerous complications of COVID-19 is the associated disseminated intravascular coagulation. Numerous reports showed that COVID-19 is related to the increased rate of thrombotic occasions (Goodman, 2020; Klok et al., 2020; Willyard, 2020). Currently, the therapies used for treatment of COVID-19 patients include different categories such as anti–SARS-CoV-2 monoclonal antibodies, antiviral products, immunomodulators, antithrombotic therapy, and supplements (H (2021a). Therapies. A, 2021a). Several medications are prescribed for the management of hospitalized COVID-19 patients such as remdesivir, dexamethasone, tofacitinib, baricitinib, tocilizumab, and sarilumab (H (2021b). Therapeutic, 2021b). Until now, several treatments have been prescribed for COVID-19 patients to relieve symptoms and manage complications, but no single treatment affords antiviral activity and symptomatic treatment.
The total extract of olive leaves and their compounds were reported in several studies for their antiviral, anti-inflammatory, immunomodulatory, and antithrombotic activities as described in this article. In addition, several products in the market that contain standardized olive leaf powder or extract are available for consumers, and most are standardized to 20% or 50% oleuropein. The aim of this study is to emphasize the potential benefit of the natural supplement (olive leaves) to undergo further in vivo or clinical investigations.
3.2 Antiviral (anti-SARS-CoV-2) Activity of Olive Leaf Compounds
3.2.1 In Silico and Computational Antiviral Studies
The antiviral activity of olive leaf metabolites against SARS-CoV-2 was reported in several in silico computational studies. Several viral targets were tested, such as viral proteases (Mpro/3CLpro, PLpro), TLRs, ACE2, RBD, NSP15, HSPA5 SBDβ, TMPRSS2, S protein, and furin (Yu et al., 2020a; Yu et al., 2020b; Derosa et al., 2020; Elfiky, 2020; Hashem, 2020; Hu et al., 2020; Jena et al., 2020; Khaerunnisa et al., 2020; Sampangi-Ramaiah et al., 2020; Shawky et al., 2020; Suručić et al., 2020; Vardhan and Sahoo, 2020; Vijayan and Gourinath, 2020; Khan et al., 2021) (Table 1).
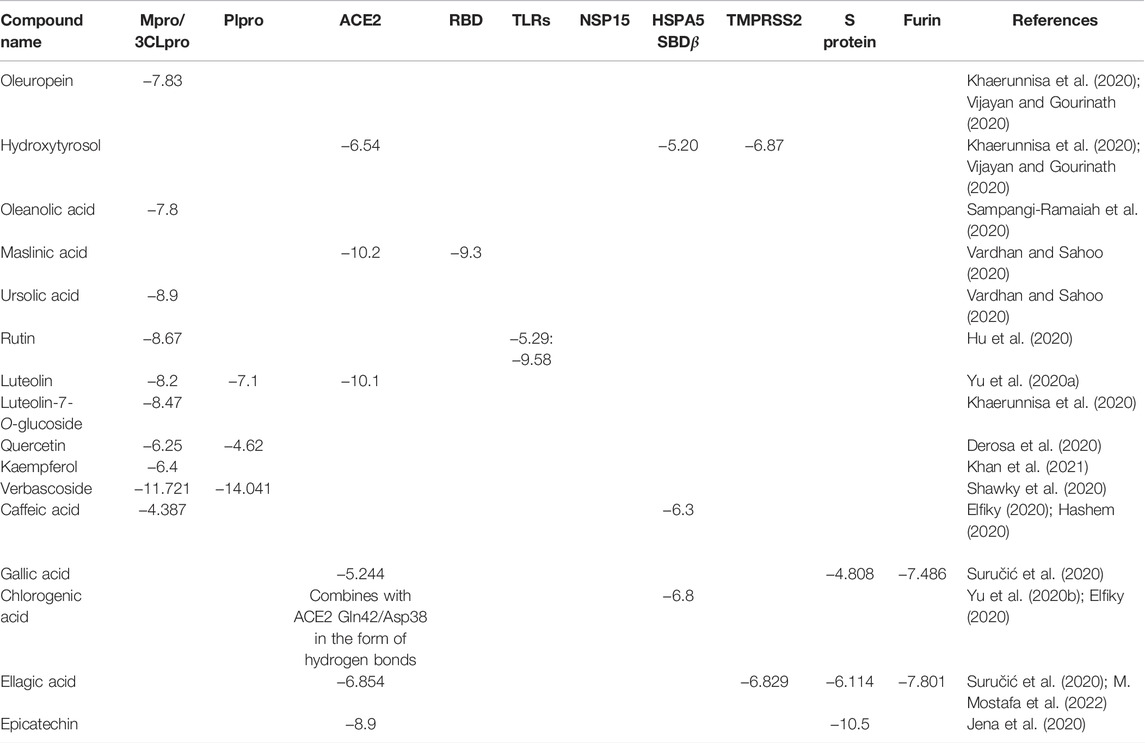
TABLE 1. Binding affinity (Kcal/mole) scores of olive leaf compounds against several targets in SARS-CoV-2.
3.2.2 In Vitro Antiviral Studies
Several studies reported the antiviral activities of compounds present in olive leaves against SARS-CoV-2.
A hydroxytyrosol-rich cream (HIDROX®) showed virucidal activity against SARS-CoV-2 through structural changes in SARS-CoV-2, which is attributed to changing the molecular weight of the spike proteins and disrupting the viral genome (Takeda et al., 2021). Another study showed that the infection of Vero E6 cells by SARS-CoV-2 was decreased by luteolin with an EC50 value of 10.6 μM (CC50 = 155 μM) (Yi et al., 2004; Russo et al., 2020). In addition, kaempferol inhibited SARS-CoV-2 replication in vitro with % of inhibition equaling 88.33, 93.33, and 40.00% at a concentration of 125.00, 62.50, and 31.25 μM, respectively (Khan et al., 2021).
3.2.3 Clinical Antiviral Studies
A spray containing 3.80% hydroxytyrosol was proven for its activity as protection against SARS-CoV-2 infection in 50 volunteers, and it showed decrease in the viral load and cure in six patients within ten days (Ergoren et al., 2020).
3.3 Anti-Inflammatory, Antipyretic, and Analgesic Activities
Olive leaf extract significantly decreased the secreted protein levels of IL-6 and IL-8, and also, mRNA expression of E-selectin in serum amyloid A (SAA)–stimulated human coronary artery endothelial cells (HCAECs) and reduced matrix metalloproteinase (MMP2) levels in unstimulated cells (Burja et al., 2019) (Table 2). In addition, oleuropein was reported as a potential anti-inflammatory molecule for treating asthma and chronic obstructive pulmonary disease (COPD) when administered orally at a dose of 10–20 mg/kg in the experimental BALB/c mice model. It inhibited pulmonary inflammation and subsequent asthmatic fibrosis and alveolar emphysema in vivo of asthma induced by exposure to interleukin IL-4, ovalbumin (OVA), or cigarette smoke (CS). The mechanism of action of oleuropein was by reducing the influx of eosinophils and lymphocytes in the airway and diminishing IL-4 secretion in the lung, suppressing the infiltration of macrophages and neutrophils by blocking the induction of intercellular adhesion molecule 1 (ICAM-1), F4/80, CD68, and CD11b in airways (Kim et al., 2018).
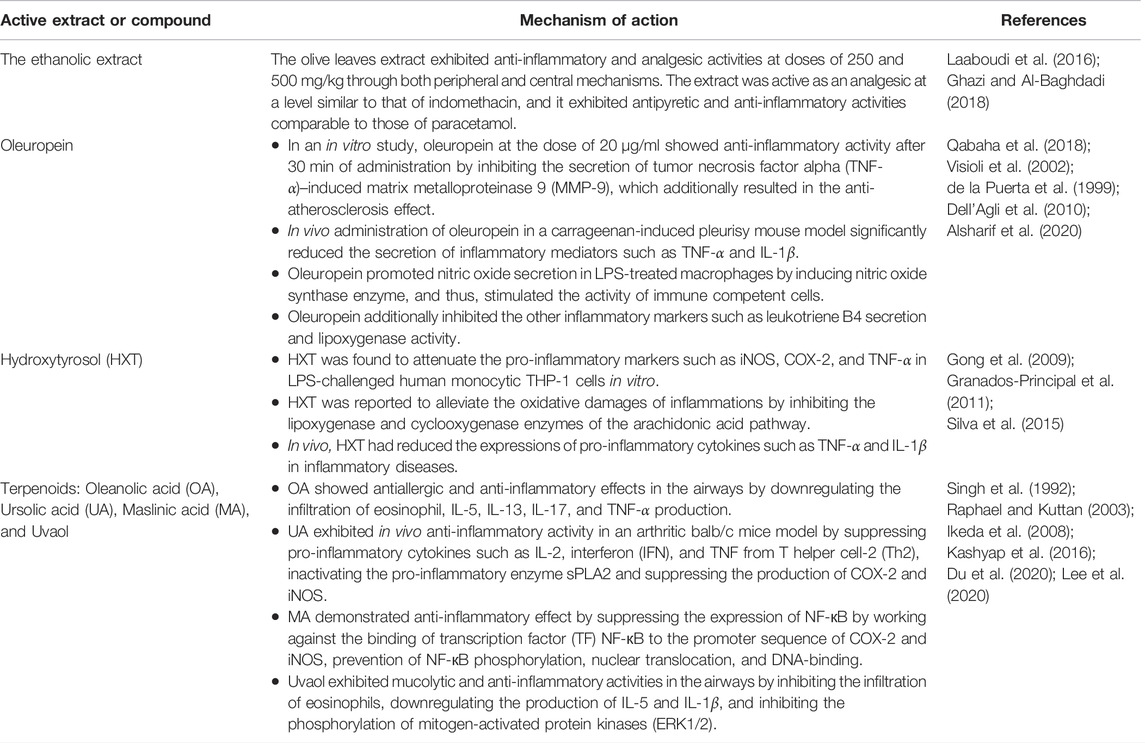
TABLE 2. Anti-inflammatory, antipyretic, and analgesic activities of olive leaf compounds against SARS-CoV-2.
3.4 Immunomodulatory Activity
Olive leaf extract and its phytoconstituents exhibited immune-modulatory effects by reducing the expression of pro-inflammatory mediators (IL-1β, IL-6, IL-8, TNF-α, and iNOS) that also resulted in its anti-inflammatory effects (Randon and Attard, 2007; Sánchez-Tena et al., 2013; Vezza et al., 2017; Harun et al., 2020). In an in vivo study using the mucosal explant cultures of Crohn’s disease patients and healthy volunteers, the ethanolic extract of olive leaves (0.1–100 μg/ml) reduced the expression of pro-inflammatory mediators such as IL-1β, IL-6, IL-8, TNF-α, and iNOS and improved the integrity of the epithelial barrier and restored the expression of ZO-1, MUC-2, and TFF-3 (Vezza et al., 2017). Oleuropein was reported to stimulate the proliferation and aggregation of lymphocytes and induce blastogenesis in vitro (Randon and Attard, 2007). Erythrodiol strongly inhibited the production of IL-6 (Harun et al., 2020). In addition, both uvaol and oleanolic acid significantly inhibited the production of TNF-α at a concentration of 100 μmol/L (Harun et al., 2020). Maslinic acid suppressed the chronic inflammation and exhibited antimodulatory activity comparable with that of dexamethasone through the development and sustainability of intestinal adenomatous polyps in ApcMin/+ (Sánchez-Tena et al., 2013).
3.5 Antithrombotic Activity
The ethanolic extract of olive leaves and its phytoconstituents such as hydroxytyrosol, hydroxytyrosol acetate, and maslinic acid reduced the platelet aggregation and exhibited antithrombotic activity by different mechanisms (Elzagallaai et al., 2000; Dub and Dugani, 2013; Vilaplana-Pérez et al., 2014; Kim et al., 2020). In a thromboplastin-induced thrombosis rabbit model, the pretreatment with the olive leaf ethanolic extract (100 or 200 mg/kg per day) for eight weeks modified the extrinsic coagulation pathway and drastically prolonged the prothrombin time (PT) in contrast to the control group. The extract also changed the thrombus morphology; the thrombus was filament-like in the treatment group, while it was thick in the control group and completely occluded the vein (Dub and Dugani, 2013).
Hydroxytyrosol reduced human platelet aggregation through a reduction in thromboxane B2 level, a platelet-aggregating and vasoconstrictor agent, which is the chemically stable and inactive form of thromboxane A2 (Vilaplana-Pérez et al., 2014).
Oral administration of hydroxytyrosol and hydroxytyrosol acetate for seven days in rats inhibited platelet aggregation with similar effectiveness of acetylsalicylic acid by decreasing thromboxane synthesis and increasing vascular nitric oxide (NO) production (Vilaplana-Pérez et al., 2014).
Maslinic acid was reported to regulate platelet aggregation and exhibited antithrombotic activity by different mechanisms. It inhibited protein kinase C (PKC) activation by activating the phosphorylation of myristoylated alanine-rich C kinase substrate (MARCKS), which is a phosphorylated substrate of PKC. Maslinic acid inhibited the enzymatic activity of coagulation factor Xa (FXa) and platelet aggregation induced by adenosine diphosphate (ADP) and a thromboxane A2 (TXA2) analog (Elzagallaai et al., 2000; Kim et al., 2020).
4 Conclusion
Olive leaves are rich in bioactive secondary metabolites. The major secoiridoid (oleuropein and its metabolite hydroxytyrosol), triterpenes (such as oleanolic, ursolic, and maslinic acids), and flavonoids (luteolin and kaempferol) exhibited in silico, in vitro, or in vivo antiviral activities against SARS-CoV-2.
Apart from the antiviral properties, these bioactive compounds significantly modulated several signaling pathways and demonstrated various activities such as anti-inflammatory, antipyretic, analgesic, immunomodulatory, and antithrombotic properties. These compounds provide a potential natural source to control the cytokine storms observed during COVID-19 infection, manage the symptoms, and protect against complications.
The potential antiviral activity of olive leaves and their other described benefits such as biosafety, availability, and low cost, along with the absence of an effective treatment for COVID-19 infection, makes olive leaves a potential natural remedy for the treatment and control of COVID-19. Clinical studies should be conducted with this plant and its metabolites to prove its efficacy in the treatment of COVID-19 infection.
Author Contributions
All the authors listed have made a substantial, direct, and intellectual contribution to the work and approved it for publication.
Conflict of Interest
The authors declare that the research was conducted in the absence of any commercial or financial relationships that could be construed as a potential conflict of interest.
Publisher’s Note
All claims expressed in this article are solely those of the authors and do not necessarily represent those of their affiliated organizations, or those of the publisher, the editors, and the reviewers. Any product that may be evaluated in this article, or claim that may be made by its manufacturer, is not guaranteed or endorsed by the publisher.
Acknowledgments
The authors extend their appreciation to the Research Center at AlMaarefa University for funding this work. In addition, the authors would like to thank the Deanship of Scientific Research at Umm Al-Qura University for supporting this work by Grant Code: (22UQU4290565DSR08).
Abbreviations
SAA, serum amyloid A; HCAEC, human coronary artery endothelial cells; MMP2, matrix metalloproteinases; COPD, chronic obstructive pulmonary disease; OVA, ovalbumin; CS, cigarette smoke; ICAM-1, intercellular adhesion molecule 1; TNF-α, tumor necrosis factor alpha; MMP-9, matrix metalloproteinase 9; NO, nitric oxide; HXT, hydroxytyrosol; OA, oleanolic acid; UA, ursolic acid; MA, maslinic acid; COX, cyclooxygenase; IFN, interferon; Th2, T helper cell-2; ERK1/2, mitogen-activated protein kinases; PT, prothrombin time; PKC, protein kinase C; MARCKS, myristoylated alanine-rich C kinase substrate; FXa, coagulation factor Xa
References
Alsharif, K. F., Almalki, A. A., Al-Amer, O., Mufti, A. H., Theyab, A., Lokman, M. S., et al. (2020). Oleuropein Protects against Lipopolysaccharide-Induced Sepsis and Alleviates Inflammatory Responses in Mice. IUBMB life 72 (10), 2121–2132. doi:10.1002/iub.2347
Alyami, M. H., Naser, A. Y., Orabi, M. A. A., Alwafi, H., and Alyami, H. S. (2020). Epidemiology of COVID-19 in the Kingdom of Saudi Arabia: An Ecological Study. Front. Public Health 8, 506. doi:10.3389/fpubh.2020.00506
Benavente-Garcıa, O., Castillo, J., Lorente, J., Ortuño, A., and Del Rio, J. (2000). Antioxidant Activity of Phenolics Extracted from Olea Europaea L. Leaves. Food Chem. 68 (4), 457–462. doi:10.1016/S0308-8146(99)00221-6
Bianchi, G., Murelli, C., and Vlahov, G. (1992). Surface Waxes from Olive Fruits. Phytochemistry 31 (10), 3503–3506. doi:10.1016/0031-9422(92)83716-c
Bouaziz, M., Grayer, R. J., Simmonds, M. S., Damak, M., and Sayadi, S. (2005). Identification and Antioxidant Potential of Flavonoids and Low Molecular Weight Phenols in Olive Cultivar Chemlali Growing in Tunisia. J. Agric. Food Chem. 53 (2), 236–241. doi:10.1021/jf048859d
Burja, B., Kuret, T., Janko, T., Topalović, D., Živković, L., Mrak-Poljšak, K., et al. (2019). Olive Leaf Extract Attenuates Inflammatory Activation and DNA Damage in Human Arterial Endothelial Cells. Front. Cardiovasc. Med. 6, 56. doi:10.3389/fcvm.2019.00056
Campeol, E., Flamini, G., Cioni, P. L., Morelli, I., D'Andrea, F., and Cremonini, R. (2004). 1,5-Anhydroxylitol from Leaves of Olea Europaea. Carbohydr. Res. 339 (16), 2731–2732. doi:10.1016/j.carres.2004.09.001
CDC (2020). Coronavirus Disease 2019 (COVID-19). Available at: https://www.cdc.gov/coronavirus/2019-ncov/need-extra-precautions/people-at-higher-risk.html.
Cimato, A., and Attilio, C. (2011). “Worldwide Diffusion and Relevance of Olive Culture,” in Olive Diseases and Disorders (Trivandrum, Kerala, India: Transworld Research NetWork), 1–21.
Cron, R. Q., Caricchio, R., and Chatham, W. W. (2021). Calming the Cytokine Storm in COVID-19. Nat. Med. 27 (10), 1674–1675. doi:10.1038/s41591-021-01500-9
da Rosa Mesquita, R., Francelino Silva Junior, L. C., Santos Santana, F. M., Farias de Oliveira, T., Campos Alcântara, R., Monteiro Arnozo, G., et al. (2021). Clinical Manifestations of COVID-19 in the General Population: Systematic Review. Wien Klin Wochenschr 133 (7-8), 377–382. doi:10.1007/s00508-020-01760-4
de la Puerta, R., Ruiz Gutierrez, V., and Hoult, J. R. (1999). Inhibition of Leukocyte 5-lipoxygenase by Phenolics from virgin Olive Oil. Biochem. Pharmacol. 57 (4), 445–449. doi:10.1016/s0006-2952(98)00320-7
Dell’Agli, M., Fagnani, R., Galli, G. V., Maschi, O., Gilardi, F., Bellosta, S., et al. (2010). Olive Oil Phenols Modulate the Expression of Metalloproteinase 9 in THP-1 Cells by Acting on Nuclear Factor-Κb Signaling. J. Agric. Food Chem. 58 (4), 2246–2252. doi:10.1021/jf9042503
Derosa, G., Maffioli, P., D'Angelo, A., and Di Pierro, F. (2020). A Role for Quercetin in Coronavirus Disease 2019 (COVID‐19). Phytotherapy Res. 35, 1230–1236. doi:10.1002/ptr.6887
Du, S. Y., Huang, H. F., Li, X. Q., Zhai, L. X., Zhu, Q. C., Zheng, K., et al. (2020). Anti-inflammatory Properties of Uvaol on DSS-Induced Colitis and LPS-Stimulated Macrophages. Chin. Med. 15, 43–13. doi:10.1186/s13020-020-00322-0
Dub, A. M., and Dugani, A. M. (2013). Antithrombotic Effect of Repeated Doses of the Ethanolic Extract of Local Olive (Olea Europaea L.) Leaves in Rabbits. Libyan J. Med. 8 (1), 20947. doi:10.3402/ljm.v8i0.20947
Duquesnoy, E., Castola, V., and Casanova, J. (2007). Triterpenes in the Hexane Extract of Leaves of Olea Europaea L.: Analysis Using 13C-NMR Spectroscopy. Phytochem. Anal. 18 (4), 347–353. doi:10.1002/pca.989
Ekor, M. (2014). The Growing Use of Herbal Medicines: Issues Relating to Adverse Reactions and Challenges in Monitoring Safety. Front. Pharmacol. 4, 177. doi:10.3389/fphar.2013.00177
Elfiky, A. A. (2020). Natural Products May Interfere with SARS-CoV-2 Attachment to the Host Cell. J. Biomol. Struct. Dyn. 39 (9), 1–10. doi:10.1080/07391102.2020.1761881
Elzagallaai, A., Rosé, S. D., and Trifaró, J. M. (2000). Platelet Secretion Induced by Phorbol Esters Stimulation Is Mediated through Phosphorylation of MARCKS: A MARCKS-Derived Peptide Blocks MARCKS Phosphorylation and Serotonin Release without Affecting Pleckstrin Phosphorylation. Blood 95 (3), 894–902. doi:10.1182/blood.v95.3.894.003k15_894_902
Ergoren, M. C., Paolacci, S., Manara, E., Dautaj, A., Dhuli, K., Anpilogov, K., et al. (2020). A Pilot Study on the Preventative Potential of Alpha-Cyclodextrin and Hydroxytyrosol against SARS-CoV-2 Transmission. Acta Biomed. 91, e2020022. doi:10.23750/abm.v91i13-S.10817
Ganjhu, R. K., Mudgal, P. P., Maity, H., Dowarha, D., Devadiga, S., Nag, S., et al. (2015). Herbal Plants and Plant Preparations as Remedial Approach for Viral Diseases. Virusdisease 26 (4), 225–236. doi:10.1007/s13337-015-0276-6
Gariboldi, P., Jommi, G., and Verotta, L. (1986). Secoiridoids from Olea Europaea. Phytochemistry 25 (4), 865–869. doi:10.1016/0031-9422(86)80018-8
Ghazi, A. M., and Al-Baghdadi, R. J. T. (2018). Evaluation of the Antinociceptive and Antipyretic Effects of the Iraqi Olive Leaf Extract in Murine Animals. J. Pharm. Sci. Res. 10 (11), 2916–2920.
Goldsmith, C. D., Vuong, Q. V., Stathopoulos, C. E., Roach, P. D., and Scarlett, C. J. (2014). Optimization of the Aqueous Extraction of Phenolic Compounds from Olive Leaves. Antioxidants (Basel) 3 (4), 700–712. doi:10.3390/antiox3040700
Gong, D., Geng, C., Jiang, L., Cao, J., Yoshimura, H., and Zhong, L. (2009). Effects of Hydroxytyrosol-20 on Carrageenan-Induced Acute Inflammation and Hyperalgesia in Rats. Phytother Res. 23 (5), 646–650. doi:10.1002/ptr.2686
Goulas, V., Exarchou, V., Troganis, A. N., Psomiadou, E., Fotsis, T., Briasoulis, E., et al. (2009). Phytochemicals in Olive-Leaf Extracts and Their Antiproliferative Activity against Cancer and Endothelial Cells. Mol. Nutr. Food Res. 53 (5), 600–608. doi:10.1002/mnfr.200800204
Granados-Principal, S., Quiles, J. L., Ramirez‐Tortosa, C., Camacho‐Corencia, P., Sanchez‐Rovira, P., Vera‐Ramirez, L., et al. (2011). Hydroxytyrosol Inhibits Growth and Cell Proliferation and Promotes High Expression of Sfrp4 in Rat Mammary Tumours. Mol. Nutr. Food Res. 55 (S1), S117–S126. doi:10.1002/mnfr.201000220
Guinda, A., Lanzón, A., Rios, J., and Albi, T. (2002). The Isolation and Quantification of the Components from Olive Leaf: Hexane Extract. Grasas y Aceites 53 (4), 419–422. doi:10.3989/gya.2002.v53.i4.340
Harun, N. H., Septama, A. W., Ahmad, W. A. N. W., and Suppian, R. (2020). Immunomodulatory Effects and Structure-Activity Relationship of Botanical Pentacyclic Triterpenes: A Review. Chin. Herbal Medicines 12 (2), 118–124. doi:10.1016/j.chmed.2019.11.007
Hashem, H. E. (2020). In Silico approach of Some Selected Honey Constituents as SARS-CoV-2 Main Protease (COVID-19) Inhibitors. Eurasian J. Med. Oncol. 4, 196–200. doi:10.14744/ejmo.2020.36102
Hashmi, M. A., Khan, A., Hanif, M., Farooq, U., and Perveen, S. (2015). Traditional Uses, Phytochemistry, and Pharmacology of Olea Europaea (Olive). Evidence-Based Complement. Altern. Med. 2015, 541591. doi:10.1155/2015/541591
Hu, X., Cai, X., Song, X., Li, C., Zhao, J., Luo, W., et al. (2020). Possible SARS-Coronavirus 2 Inhibitor Revealed by Simulated Molecular Docking to Viral Main Protease and Host Toll-like Receptor. Future Virol. 15, 359–368. doi:10.2217/fvl-2020-0099
Ikeda, Y., Murakami, A., and Ohigashi, H. (2008). Ursolic Acid: An Anti- and Pro-inflammatory Triterpenoid. Mol. Nutr. Food Res. 52 (1), 26–42. doi:10.1002/mnfr.200700389
Jemai, H., El Feki, A., and Sayadi, S. (2009). Antidiabetic and Antioxidant Effects of Hydroxytyrosol and Oleuropein from Olive Leaves in Alloxan-Diabetic Rats. J. Agric. Food Chem. 57 (19), 8798–8804. doi:10.1021/jf901280r
Jena, A. B., Kanungo, N., Nayak, V., Chainy, G., and Dandapat, J. (2020). Catechin and Curcumin Interact with corona (2019-nCoV/SARS-CoV2) Viral S Protein and ACE2 of Human Cell Membrane: Insights from Computational Study and Implication for Intervention. Sci. Rep. 11 (1), 2043. doi:10.1038/s41598-021-81462-7
Kabrah, S. M., Kabrah, A. M., Flemban, A. F., and Abuzerr, S. (2021). Systematic Review and Meta-Analysis of the Susceptibility of ABO Blood Group to COVID-19 Infection. Transfus. Apher. Sci. 60 (4), 103169. doi:10.1016/j.transci.2021.103169
Kashyap, D., Tuli, H. S., and Sharma, A. K. (2016). Ursolic Acid (UA): A Metabolite with Promising Therapeutic Potential. Life Sci. 146, 201–213. doi:10.1016/j.lfs.2016.01.017
Khaerunnisa, S., Kurniawan, H., Awaluddin, R., Suhartati, S., and Soetjipto, S. (2020). Potential Inhibitor of COVID-19 Main Protease (Mpro) from Several Medicinal Plant Compounds by Molecular Docking Study. Pharmacol. Toxicol.. [Preprint] doi:10.20944/preprints202003.0226.v1
Khalatbary, A. R., and Zarrinjoei, G. R. (2012). Anti-inflammatory Effect of Oleuropein in Experimental Rat Spinal Cord Trauma. Iran Red Crescent Med. J. 14 (4), 229–234.
Khan, A., Heng, W., Wang, Y., Qiu, J., Wei, X., Peng, S., et al. (2021). In Silico and In Vitro Evaluation of Kaempferol as a Potential Inhibitor of the SARS-CoV-2 Main Protease (3CLpro). Phytotherapy Res. 35 (6), 2841–2845. doi:10.1002/ptr.6998
Kim, K. M., Kim, J., Baek, M. C., and Bae, J. S. (2020). Novel Factor Xa Inhibitor, Maslinic Acid, with Antiplatelet Aggregation Activity. J. Cel Physiol 235 (12), 9445–9456. doi:10.1002/jcp.29749
Kim, Y. H., Choi, Y. J., Kang, M. K., Lee, E. J., Kim, D. Y., Oh, H., et al. (2018). Oleuropein Curtails Pulmonary Inflammation and Tissue Destruction in Models of Experimental Asthma and Emphysema. J. Agric. Food Chem. 66 (29), 7643–7654. doi:10.1021/acs.jafc.8b01808
Klok, F. A., Kruip, M., Van der Meer, N., Arbous, M., Gommers, D., Kant, K., et al. (2020). Confirmation of the High Cumulative Incidence of Thrombotic Complications in Critically Ill ICU Patients with COVID-19: An Updated Analysis. Thromb. Res. 191, 148–150. doi:10.1016/j.thromres.2020.04.041
Laaboudi, W., Ghanam, J., Aissam, H., Merzouki, M., and Benlemlih, M. (2016). Anti-inflammatory and Analgesic Activities of Olive Tree Extract. Int. J Pharm. Pharm. Sci. 8 (7), 414–419. doi:10.22159/ijpps.2016v8i12.14077
Lee, W., Kim, J., Park, E. K., and Bae, J. S. (2020). Maslinic Acid Ameliorates Inflammation via the Downregulation of NF-Κb and STAT-1. Antioxidants (Basel) 9 (2), 106. doi:10.3390/antiox9020106
Lin, L. T., Hsu, W. C., and Lin, C. C. (2014). Antiviral Natural Products and Herbal Medicines. J. Tradit Complement. Med. 4 (1), 24–35. doi:10.4103/2225-4110.124335
Meirinhos, J., Silva, B. M., Valentão, P., Seabra, R. M., Pereira, J. A., Dias, A., et al. (2005). Analysis and Quantification of Flavonoidic Compounds from Portuguese Olive (Olea Europaea L.) Leaf Cultivars. Nat. Prod. Res. 19 (2), 189–195. doi:10.1080/14786410410001704886
M. Mostafa, N., I. Ismail, M., M. El-Araby, A., M. Bahgat, D., M. Elissawy, A., M. Mostafa, A., et al. (2022). Investigation of SARS-CoV-2 Main Protease Potential Inhibitory Activities of Some Natural Antiviral Compounds via Molecular Docking and Dynamics Approaches. Phyton-International J. Exp. Bot. 91 (5), 1089–1104. doi:10.32604/phyton.2022.018239
Movsumov, I. S. (1994). Components of the Leaves ofOlea Verrucosa. Chem. Nat. Compd. 30 (5), 626. doi:10.1007/bf00629879
Mussini, P., Orsini, F., and Pelizzoni, F. (1975). Triterpenes in Leaves of Olea Europaea. Phytochemistry 14 (4), 1135. doi:10.1016/0031-9422(75)85210-1
Nassir, A. M., Ibrahim, I. A. A., Afify, M. A., ElSawy, N. A., Imam, M. T., Shaheen, M. H., et al. (2019). Olea Europaea Subsp. Cuspidata and Juniperus Procera Hydroalcoholic Leaves' Extracts Modulate Stress Hormones in Stress-Induced Cystitis in Rats. Urol. Sci. 30 (4), 151.
NIH (2021b). Therapeutic Management of Hospitalized Adults with COVID-19. Available at: https://www.covid19treatmentguidelines.nih.gov/management/clinical-management/hospitalized-adults--therapeutic-management/.
NIH (2021a). Therapies. Available at: https://www.covid19treatmentguidelines.nih.gov/therapies/.
Özcan, M. M., and Matthäus, B. (2017). A Review: Benefit and Bioactive Properties of Olive (Olea Europaea L.) Leaves. Eur. Food Res. Technol. 243 (1), 89–99. doi:10.1007/s00217-016-2726-9
Parums, D. V. (2022). Editorial: Current Status of Oral Antiviral Drug Treatments for SARS-CoV-2 Infection in Non-hospitalized Patients. Med. Sci. Monit. 28, e935952. doi:10.12659/MSM.935952
Qabaha, K., Al-Rimawi, F., Qasem, A., and Naser, S. A. (2018). Oleuropein Is Responsible for the Major Anti-inflammatory Effects of Olive Leaf Extract. J. Med. Food 21 (3), 302–305. doi:10.1089/jmf.2017.0070
Randon, A. M., and Attard, E. (2007). The In Vitro Immunomodulatory Activity of Oleuropein, a Secoiridoid Glycoside from Olea Europaea L. Nat. Product. Commun. 2 (5), 1934578X0700200501. doi:10.1177/1934578x0700200501
Raphael, T. J., and Kuttan, G. (2003). Effect of Naturally Occurring Triterpenoids Glycyrrhizic Acid, Ursolic Acid, Oleanolic Acid and Nomilin on the Immune System. Phytomedicine 10 (6-7), 483–489. doi:10.1078/094471103322331421
Russo, M., Moccia, S., Spagnuolo, C., Tedesco, I., and Russo, G. L. (2020). Roles of Flavonoids against Coronavirus Infection. Chem. Biol. Interact 328, 109211. doi:10.1016/j.cbi.2020.109211
Sampangi-Ramaiah, M. H., Vishwakarma, R., and Uma Shaanker, R. (2020). Molecular Docking Analysis of Selected Natural Products from Plants for Inhibition of SARS-CoV-2 Main Protease. Curr. Sci. 118 (7), 1087–1092. doi:10.18520/cs/v118/i7/1087-1092
Sánchez-Tena, S., Reyes-Zurita, F. J., Díaz-Moralli, S., Vinardell, M. P., Reed, M., García-García, F., et al. (2013). Maslinic Acid-Enriched Diet Decreases Intestinal Tumorigenesis in Apc Min/+ Mice through Transcriptomic and Metabolomic Reprogramming. PloS one 8 (3), e59392.
Santiago-Mora, R., Casado-Díaz, A., De Castro, M. D., and Quesada-Gómez, J. M. (2011). Oleuropein Enhances Osteoblastogenesis and Inhibits Adipogenesis: The Effect on Differentiation in Stem Cells Derived from Bone Marrow. Osteoporos. Int. 22 (2), 675–684. doi:10.1007/s00198-010-1270-x
Savournin, C., Baghdikian, B., Elias, R., Dargouth-Kesraoui, F., Boukef, K., and Balansard, G. (2001). Rapid High-Performance Liquid Chromatography Analysis for the Quantitative Determination of Oleuropein in Olea Europaea Leaves. J. Agric. Food Chem. 49 (2), 618–621. doi:10.1021/jf000596+
Schumacher, B., Scholle, S., Hölzl, J., Khudeir, N., Hess, S., and Müller, C. E. (2002). Lignans Isolated from Valerian: Identification and Characterization of a New Olivil Derivative with Partial Agonistic Activity at A(1) Adenosine Receptors. J. Nat. Prod. 65 (10), 1479–1485. doi:10.1021/np010464q
Shawky, E., Nada, A. A., and Ibrahim, R. S. (2020). Potential Role of Medicinal Plants and Their Constituents in the Mitigation of SARS-CoV-2: Identifying Related Therapeutic Targets Using Network Pharmacology and Molecular Docking Analyses. RSC Adv. 10 (47), 27961–27983. doi:10.1039/d0ra05126h
Silva, S., Sepodes, B., Rocha, J., Direito, R., Fernandes, A., Brites, D., et al. (2015). Protective Effects of Hydroxytyrosol-Supplemented Refined Olive Oil in Animal Models of Acute Inflammation and Rheumatoid Arthritis. J. Nutr. Biochem. 26 (4), 360–368. doi:10.1016/j.jnutbio.2014.11.011
Singh, G. B., Singh, S., Bani, S., Gupta, B. D., and Banerjee, S. K. (1992). Anti-inflammatory Activity of Oleanolic Acid in Rats and Mice. J. Pharm. Pharmacol. 44 (5), 456–458. doi:10.1111/j.2042-7158.1992.tb03646.x
Soni, M. G., Burdock, G. A., Christian, M. S., Bitler, C. M., and Crea, R. (2006). Safety Assessment of Aqueous Olive Pulp Extract as an Antioxidant or Antimicrobial Agent in Foods. Food Chem. Toxicol. 44 (7), 903–915. doi:10.1016/j.fct.2006.01.008
Suručić, R., Tubić, B., Stojiljković, M. P., Djuric, D. M., Travar, M., Grabež, M., et al. (2020). Computational Study of Pomegranate Peel Extract Polyphenols as Potential Inhibitors of SARS-CoV-2 Virus Internalization. Mol. Cell. Biochem. 476 (2), 1179–1193. doi:10.1007/s11010-020-03981-7
Susalit, E., Agus, N., Effendi, I., Tjandrawinata, R. R., Nofiarny, D., Perrinjaquet-Moccetti, T., et al. (2011). Olive (Olea Europaea) Leaf Extract Effective in Patients with Stage-1 Hypertension: Comparison with Captopril. Phytomedicine 18 (4), 251–258. doi:10.1016/j.phymed.2010.08.016
Takeda, Y., Jamsransuren, D., Matsuda, S., Crea, R., and Ogawa, H. (2021). The SARS-CoV-2-Inactivating Activity of Hydroxytyrosol-Rich Aqueous Olive Pulp Extract (HIDROX) and its Use as a Virucidal Cream for Topical Application. Viruses 13 (2), 232. doi:10.3390/v13020232
Tawakul, A. A., Alharbi, A. H., Basahal, A. M., Almalki, A. M., Alharbi, B., Almaghrabi, M., et al. (2021). Neurological Symptoms and Complications of COVID-19 Among Patients in a Tertiary Hospital in Saudi Arabia. Cureus 13 (11), 19200. doi:10.7759/cureus.19200
Vardhan, S., and Sahoo, S. K. (2020). In Silico ADMET and Molecular Docking Study on Searching Potential Inhibitors from Limonoids and Triterpenoids for COVID-19. Comput. Biol. Med. 124, 103936. doi:10.1016/j.compbiomed.2020.103936
Vezza, T., Algieri, F., Rodríguez-Nogales, A., Garrido-Mesa, J., Utrilla, M. P., Talhaoui, N., et al. (2017). Immunomodulatory Properties of Olea Europaea Leaf Extract in Intestinal Inflammation. Mol. Nutr. Food Res. 61 (10), 1601066. doi:10.1002/mnfr.201601066
Vijayan, R., and Gourinath, S. (2020). Structure-based Inhibitor Screening of Natural Products against NSP15 of SARS-CoV-2 Revealed Thymopentin and Oleuropein as Potent Inhibitors. J. Proteins Proteom. 12 (2), 71–80. doi:10.1007/s42485-021-00059-w
Vilaplana-Pérez, C., Auñón, D., García-Flores, L. A., and Gil-Izquierdo, A. (2014). Hydroxytyrosol and Potential Uses in Cardiovascular Diseases, Cancer, and AIDS. Front. Nutr. 1, 18. doi:10.3389/fnut.2014.00018
Visioli, F., Poli, A., and Gall, C. (2002). Antioxidant and Other Biological Activities of Phenols from Olives and Olive Oil. Med. Res. Rev. 22 (1), 65–75. doi:10.1002/med.1028
Wang, L., Geng, C., Jiang, L., Gong, D., Liu, D., Yoshimura, H., et al. (2008). The Anti-atherosclerotic Effect of Olive Leaf Extract Is Related to Suppressed Inflammatory Response in Rabbits with Experimental Atherosclerosis. Eur. J. Nutr. 47 (5), 235–243. doi:10.1007/s00394-008-0717-8
Wang, X. F., Li, C., Shi, Y. P., and Di, D. L. (2009). Two New Secoiridoid Glycosides from the Leaves of Olea Europaea L. J. Asian Nat. Prod. Res. 11 (11), 940–944. doi:10.1080/10286020903310979
Wichers, H. J., Soler-rivas, C., and Espı, J. C. (2000). Oleuropein and Related Compounds. J. Sci. Food Agric. 80 (7), 1013–1023. doi:10.1002/(SICI)1097-0010(20000515)80:7%3C1013::AID-JSFA571%3E3.0.CO;2-C
Willyard, C. (2020). Coronavirus Blood-Clot Mystery Intensifies. Nature 581 (7808), 250. doi:10.1038/d41586-020-01403-8
Yi, L., Li, Z., Yuan, K., Qu, X., Chen, J., Wang, G., et al. (2004). Small Molecules Blocking the Entry of Severe Acute Respiratory Syndrome Coronavirus into Host Cells. J. Virol. 78 (20), 11334–11339. doi:10.1128/JVI.78.20.11334-11339.2004
Yu, J. W., Wang, L., and Bao, L. D. (2020). Exploring the Active Compounds of Traditional Mongolian Medicine in Intervention of Novel Coronavirus (COVID-19) Based on Molecular Docking Method. J. Funct. Foods 71, 104016. doi:10.1016/j.jff.2020.104016
Yu, R., Chen, L., Lan, R., Shen, R., and Li, P. (2020). Computational Screening of Antagonists against the SARS-CoV-2 (COVID-19) Coronavirus by Molecular Docking. Int. J. Antimicrob. Agents 56 (2), 106012. doi:10.1016/j.ijantimicag.2020.106012
Zawawi, A., Naser, A. Y., Alwafi, H., and Minshawi, F. (2021). Profile of Circulatory Cytokines and Chemokines in Human Coronaviruses: A Systematic Review and Meta-Analysis. Front. Immunol. 12, 1453. doi:10.3389/fimmu.2021.666223
Keywords: olive leaves, phytoconstituents, antiviral, SARS-CoV-2, anti-inflammatory, anti-thrombotic
Citation: Abdelgawad SM, Hassab MAE, Abourehab MAS, Elkaeed EB and Eldehna WM (2022) Olive Leaves as a Potential Phytotherapy in the Treatment of COVID-19 Disease; A Mini-Review. Front. Pharmacol. 13:879118. doi: 10.3389/fphar.2022.879118
Received: 18 February 2022; Accepted: 09 March 2022;
Published: 13 April 2022.
Edited by:
Nada Mostafa, Ain Shams University, EgyptCopyright © 2022 Abdelgawad, Hassab, Abourehab, Elkaeed and Eldehna. This is an open-access article distributed under the terms of the Creative Commons Attribution License (CC BY). The use, distribution or reproduction in other forums is permitted, provided the original author(s) and the copyright owner(s) are credited and that the original publication in this journal is cited, in accordance with accepted academic practice. No use, distribution or reproduction is permitted which does not comply with these terms.
*Correspondence: Shimaa M. Abdelgawad, smm13@fayoum.edu.eg; Mohammed A. S. Abourehab, maabourehab@uqu.edu.sa; Wagdy M. Eldehna, wagdy2000@gmail.com