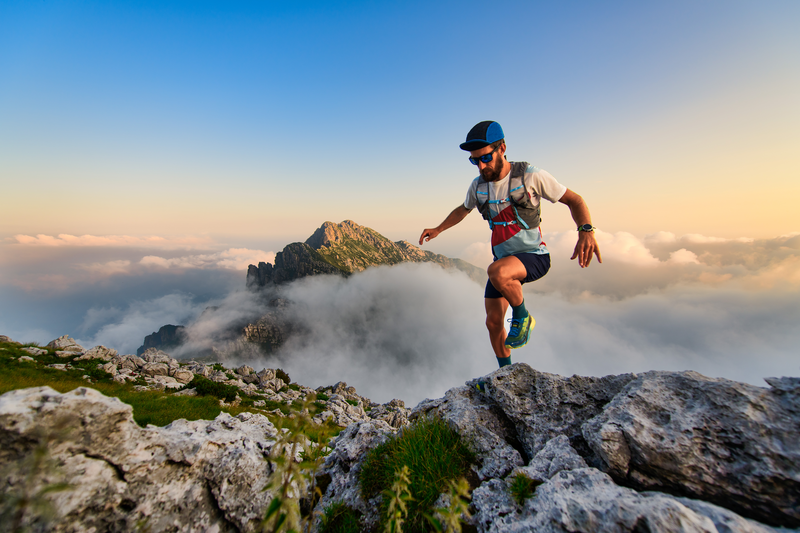
95% of researchers rate our articles as excellent or good
Learn more about the work of our research integrity team to safeguard the quality of each article we publish.
Find out more
SYSTEMATIC REVIEW article
Front. Pharmacol. , 23 March 2022
Sec. Pharmacology of Anti-Cancer Drugs
Volume 13 - 2022 | https://doi.org/10.3389/fphar.2022.878540
This article is part of the Research Topic Novel Biomarkers for Anticancer Therapy View all 33 articles
Background: Immune checkpoint inhibitors (ICIs) have become an effective treatment option for cancer. KRAS, EGFR and TP53 are common mutated oncogenes in cancer whose single gene status may predict the therapeutic effect of clinical ICIs. In this efficacy evaluation, we aimed to clarify whether the single gene mutation status of KRAS, EGFR or TP53 affects the survival benefits of ICIs in cancer patients.
Methods: We used PubMed, Cochrane Library, web of science, and clinical trials Gov database to retrieve qualified documents, the time was up to January 2022. Hazard ratios (HRS) and 95% confidence intervals (CIs) were used to determine the single gene mutation status and no progression of KRAS, EGFR or TP53.
Results: A total of 19 studies included 7029 cancer patients treated with ICIs. The results showed that KRAS, EGFR or TP53 single gene mutation could significantly improve PFS and OS in patients receiving ICIs, but the degree of improvement was different. The risk of prolongation of PFS (HR = 1.48, 95% CI = 1.19-1.85, p = 0.0004) and OS (HR = 1.68, 95% CI = 1.36-2.07, p < 0.00001) caused by TP53 single gene mutation was relatively high, the risk ratio of prolongation of PFS (HR = 1.38, 95% CI = 1.21-1.57, p < 0.00001) and OS (HR = 1.56, 95% CI = 1.20-2.04, p = 0.001) caused by EGFR single gene mutation was the second, the risk ratio of prolongation of PFS (HR = 1.33, 95% CI = 1.12-1.57, p = 0.001) and OS (HR = 1.39, 95% CI = 1.18-1.63, p < 0.00001) caused by KRAS single gene mutation was relatively low, and the results were significantly different.
Conclusion: In cancer patients, KRAS, EGFR or TP53 single gene status is correlated with the benefits of immunotherapy PFS and OS, which suggests that gene sequencing should be carried out in time in the process of clinical treatment to determine the gene mutation of patients and better predict the clinical treatment effect of ICIs.
With the advent of the era of tumor immunity, significant progress has been made in the treatment of cancers. In recent years, immune checkpoint inhibitors have become an effective treatment option and means for the treatment of cancer (Ferlay et al., 2015). Programmed death ligand 1 (PD-L1) is an immune checkpoint protein expressed on cancer cells or cancer infiltrating immune cells. PD-L1 binds to the programmed death 1 (PD-1) receptor on activated T cells and induces tumor immune escape by down regulating the function of antitumor T cells (Alexandrov et al., 2013; Brahmer et al., 2015). Therefore, inhibition of PD-1/PD-L1 pathway can induce immune response to cancer by restoring T cell activity (Moore et al., 2020).
Immune checkpoint inhibitors (ICIs) refer to antibodies against PD-1/PD-L1, which block the inhibition signal mediated by PD-1/PD-L1 (Dong et al., 2017). Many clinical trials involving patients with cancer tumors have shown that ICIs can achieve better survival outcomes than standard chemotherapy (Ozaki et al., 2020).
Tumor mutation load has been considered as a potential marker of cancer response to ICIs. High mutation load may be related to the increase of new antigens recognized by T cells to increase antitumor T cell response (Dong et al., 2017). KRAS, EGFR or TP53 are the most common mutated oncogenes in cancer (Dong et al., 2017). However, it is unclear whether the efficacy of ICIs in cancer patients is related to KRAS, EGFR or TP53 single gene mutations. We conducted this meta-analysis to investigate whether KRAS, EGFR or TP53 single gene mutation status affects the survival benefits of ICIs in cancer patients.
Systematically search the domestic and foreign literature on the efficacy of ICIs in cancer patients and KRAS, EGFR or TP53 single gene mutations, and evaluate whether KRAS, EGFR or TP53 single gene mutations affect the survival benefits of ICIs in cancer patients.
We performed multiple retrieval tools: 1) Computer literature database search: 1) Retrieval of computer literature database: ① Chinese search terms include immune checkpoint inhibitors, cancer, gene mutation, etc; ② English search terms include ICIS, cancer, KRAS, EGFR, TP53, etc; ③ PubMed, Cochrane Library, EMBASE and EBSCO evidence-based medicine databases are searched in different combinations. The search items include title, abstract and keywords. The search time limit is from the establishment of the database to January 2022. 2) Manual retrieval of ASCO conference related literature as a supplement to computer retrieval.
Immunotherapy is used more and more in many cancer patients. Some research results show that KRAS, EGFR or TP53 single gene mutation leads to the reduction of PFS and OS in cancer patients using ICIs. In order to further systematically evaluate the correlation between gene mutation and survival benefit of ICIs patients, We used inclusion and exclusion criteria for this regimen: inclusion criteria were: 1) confirmed solid tumor; 2) Overall survival (OS) or progressionfree survival (PFS) data can be used to evaluate the efficacy of immune checkpoint inhibitors; 3) KRAS EGFR or TP53 single gene mutation status; 4) Literature type: prospective or retrospective studies, randomized controlled trials, whether blind or lacking, also included animal studies reviews editorial reviews or case reports were excluded duplicative studies, unbalanced matching procedures, or incomplete data were excluded.
We used the Newcastle Ottawa scale (NOS) to evaluate the quality of the included studies (Lazarus et al., 2019). The inclusion studies were evaluated according to the following criteria: 1) whether they were representative; 2) determination of blind method; 3) whether the random method is determined; 4) Completeness of outcome events; 5) Comparability of included studies; 6) Assessment of outcome events; 7) Whether there is follow-up; 8) Completeness of follow-up. High quality literature is rated 7-9, medium quality literature is rated 4-6, and low quality literature is rated 3 or lower. Data were extracted independently by two reviewers according to specified selection criteria. Differences of opinion are resolved by discussion between authors or by obtaining input from a third evaluator.
We extracted key data from the preliminary study and analyzed it using Review Manager 5.4. The results are expressed as odd risk with 95% confidence interval (CI), and the continuous results are expressed as weighted mean difference. In the absence of statistical heterogeneity, fixed effect models were used to aggregate data. If there is statistical heterogeneity (p < 0.05, I2 ≥ 50%), the random effect model is used.
125 relevant literatures were obtained through database retrieval, excluding 90 duplicates, case reports, reviews and irrelevant contents. 36 literatures were screened in strict accordance with the above screening process. Finally, 19 (Facchinetti et al., 2017; Fujimoto et al., 2018; Garassino et al., 2018; Lin et al., 2018; Ahn et al., 2019; Assoun et al., 2019; Cho et al., 2019; Guibert et al., 2019; Jeanson et al., 2019; Ng et al., 2019; Gianoncelli et al., 2020; Lyu et al., 2020; Marinelli et al., 2020; Morita et al., 2020; Motzer et al., 2020; Schoenfeld et al., 2020; Xiang et al., 2020; An et al., 2021; Kartolo et al., 2021) studies were included, which met the quantitative analysis, involving 7029 cancer patients, as shown in Figure 1.
A total of 7029 cancer patients who met the requirements were included in 19 literatures, including 3058 patients with KRAS, EGFR or TP53 single gene mutation and 3971 patients without this gene mutation. All 19 literatures are high-quality literatures, as shown in Tables 1, 2, 3.
Eight studies can obtain KRAS single gene mutation. For the PFS data of cancer patients using ICIs, I2 = 43%, p = 0.09. There is no statistical heterogeneity among the studies. The fixed effect model is used for analysis. The results showed that HR = 1.33 (95% CI = 1.12-1.57, p = 0.001), suggesting that KRAS single gene mutation can significantly improve PFS in cancer patients using ICIs, as shown in Figure 2.
FIGURE 2. Meta-analysis of objective responses according to KRAS status of PFS in cancer patients treated with ICIs.
Six studies can obtain EGFR single gene mutation. For PFS data of cancer patients using ICIs, I2 = 0%, p = 0.42. There is no statistical heterogeneity among studies. Fixed effect model is used for analysis. The results showed that HR = 1.38 (95% CI = 1.21-1.57, p < 0.00001), suggesting that EGFR single gene mutation can significantly improve PFS in cancer patients using ICIs, as shown in Figure 3.
FIGURE 3. Meta-analysis of objective responses according to EGFR status of PFS in cancer patients treated with ICIs.
Six studies can obtain TP53 single gene mutation. The heterogeneity of PFS data of cancer patients using ICIs was analyzed, with I2 = 0%, p = 0.58. There was no statistical heterogeneity among the studies. The fixed effect model was used for analysis. The results showed that HR = 1.48 (95% CI = 1.19-1.85, p = 0.0004), suggesting that TP53 single gene mutation can significantly improve PFS in cancer patients using ICIs, as shown in Figure 4.
FIGURE 4. Meta-analysis of objective responses according to TP53 status of PFS in cancer patients treated with ICIs.
The above univariate analysis results showed that KRAS, EGFR or TP53 single gene mutation can improve PFS, but the extended HRS is different. Compared with no mutation, the risk ratio of KRAS single gene mutation prolonging PFS is 1.33, EGFR single gene mutation prolonging PFS is 1.38, and TP53 single gene mutation prolonging PFS is 1.48. The results suggest that TP53 single gene mutation leads to more significant prolongation of PFS in cancer patients using ICIs.
Seven studies can obtain KRAS single gene mutation. For OS data of cancer patients using ICIs, I2 = 0%, p = 0.47. There is no statistical heterogeneity among studies. Fixed effect model is used for analysis. The results showed that HR = 1.39 (95% CI = 1.18-1.63, p < 0.00001), suggesting that KRAS single gene mutation can significantly improve the OS of cancer patients using ICIs, as shown in Figure 5.
FIGURE 5. Meta-analysis of objective responses according to KRAS status of OS in cancer patients treated with ICIs.
Six studies can obtain EGFR single gene mutation. For OS data of cancer patients using ICIs, I2 = 58%, p = 0.04. There is statistical heterogeneity among studies, which is analyzed by random effect model. The results showed that HR = 1.56 (95% CI = 1.20-2.04, p = 0.001), suggesting that EGFR single gene mutation can significantly improve the OS of cancer patients using ICIs, as shown in Figure 6.
FIGURE 6. Meta-analysis of objective responses according to EGFR status of OS in cancer patients treated with ICIs.
Five studies can obtain TP53 single gene mutation. For OS data of cancer patients using ICIs, I2 = 0%, p = 0.68. There is no statistical heterogeneity among studies. Fixed effect model is used for analysis. The results showed that HR = 1.68 (95% CI = 1.36-2.07, p < 0.00001), suggesting that TP53 single gene mutation can significantly improve the OS of cancer patients using ICIs, as shown in Figure 7.
FIGURE 7. Meta-analysis of objective responses according to TP53 status of OS in cancer patients treated with ICIs.
The above univariate analysis results show that KRAS, EGFR or TP53 single gene mutation can significantly improve OS, but the extended HRS is different. Compared with no mutation, the risk ratio of KRAS single gene mutation prolonging OS is 1.39, the risk ratio of EGFR single gene mutation prolonging OS is 1.56, and the risk ratio of TP53 single gene mutation prolonging OS is 1.68. The results suggest that TP53 single gene mutation leads to more significant OS prolongation in cancer patients using ICIs.
At the same time of meta-analysis and comparison of PFS and OS data indicators, the inverted funnel diagram was drawn for the included studies. The results show that OS with EGFR single gene mutation has small publication bias. Individual studies may have less rigorous design and poor research methods, which lead to the asymmetry of inverted funnel diagram and small bias. Other PFS and OS funnel patterns are symmetrical, as shown in Figure 8.
FIGURE 8. Funnel Plot of objective responses according to KRAS/EGFR/TP53 status of PFS and OS in cancer patients treated with ICIs.
In the sensitivity analysis, the sensitivity analysis of meta-analysis results was carried out by excluding one study at a time and then making statistics again. The combined hrs of PFS and OS showed no difference. There was no significant difference in the analysis results before and after elimination, suggesting that all meta-analysis results were stable.
A large number of research data show that the emergence of immune checkpoint inhibitors has significantly affected the clinical treatment strategies of most cancer subtypes, especially non-small cell lung cancer, renal cell carcinoma, melanoma and so on. Programmed cell death-1 (PD-1) inhibitors, such as neruzumab or pemilizumab, and programmed cell death ligand-1 (PD-L1) inhibitors, such as atezolizumab, significantly improved progression free survival (PFS) and overall survival (OS) in cancer patients. Compared with platinum based standard first-line chemotherapy or docetaxel based second-line chemotherapy, excellent disease control time is achieved (Borghaei et al., 2015; Le et al., 2017).
Some studies have shown that tumor mutation burden (TMB) can predict the potential activity of immunotherapy in a variety of tumor types, including NSCLC, RCC, etc. (Song et al., 2020). By producing damaged cell proteins recognized as new antigens by immune cells, the resulting genetic instability can enhance the immunogenicity of tumors, so as to optimize the antitumor cytotoxicity of T lymphocytes recovered by ICIs treatment. For example, in colorectal cancer, mismatch repair status predicts the clinical benefit of anti-PD-1 antibody pamumab (Van Allen et al., 2015). In non-small cell lung cancer, a mutation feature associated with smoking predicts anti-PD-1 efficacy (Hollern et al., 2019). In melanoma, TMB and neoantigen load predict patients’ response to CTLA-4 treatment (Pauken et al., 2016). The presence of CD8 + T cells and the expression of immune checkpoint genes such as PD-1 ligand (PD-L1) and CTLA-4 can also predict the efficacy of ICIs (Wang et al., 2019). These data show that TMB has a significant effect on predicting the response of ICIs, and it is clinically needed.
KRAS is a guanine nucleotide binding protein that regulates the mitogen activated protein kinase (MAPK) pathway. When it is activated, it promotes downstream signaling pathways, leading to cell growth and proliferation (Nagasaka et al., 2020). KRAS mutation rate in patients with non squamous NSCLC is 20–30% (Nagasaka et al., 2020). So far, there is no therapy for KRAS mutation. Although some targeted drugs (such as G12C inhibitors) are being evaluated in clinical trials, the effective drug targeting of KRAS mutation is also an unprecedented challenge. By studying the correlation between KRAS mutation status and TMB, it was found that TMB was associated with tumor immunogenicity and greater survival benefit of ICIs treatment (Adderley et al., 2019). Recent studies reported that compared with chemotherapy patients, OS and PFS were improved in patients with KRAS mutant NSCLC after ICIs treatment, and KRAS mutant tumors showed stronger PD-L1 expression and T cell infiltration (Adderley et al., 2019). Epidermal growth factor receptor (EGFR) mutation is a good predictor of the efficacy of EGFR tyrosine kinase inhibitors. EGFR mutation is common in cancer, especially in non-small cell lung cancer. EGFR mutations are found in 32% of non-small cell lung cancer cases worldwide, of which 39% of tumors occur in Asian ethnic groups and 17% in Caucasian ethnic groups (Zhang et al., 2016). The prevalence of EGFR mutation in lung adenocarcinoma is high; 51% of East Asian lung adenocarcinoma patients have EGFR mutation (Shi et al., 2014). The high prevalence of EGFR mutations makes it more important to evaluate whether EGFR mutations are really negative predictors of PD-1/PD-L1 efficacy. The prognostic role of tumor suppressor gene TP53 mutation in predicting the efficacy of ICIs is highly controversial. An early study (Xiao et al., 2018) showed that TP53 mutation was associated with relatively short PFS and shorter OS in 110 patients receiving CTLA-4 blocking treatment. However, inconsistent results were observed in another group of patients with non-small cell lung cancer treated with PD-1 + CTLA-4 block. The study found that TP53 mutation was enriched in responders, suggesting that TP53 may be related to the enhanced response of combination therapy. In addition, Dong et al. (Ozaki et al., 2020) confirmed that patients with lung adenocarcinoma had a relatively good response to TP53 mutation when treated with PD-1 inhibitors, suggesting that TP53 may help to guide the decision of clinical use of ICIs.
In addition, many cancers show different patterns of genomic changes. In the development of cancer, KRAS, as a driving oncogene, has a high mutation frequency. However, it has recently been found that cancers with KRAS mutations also express TP53 mutations. TP53, as a core cancer suppressor gene, encodes p53 protein in humans and mice to prevent mutations in a stable state. Notably, TP53 mutations drive lung cancer, with a higher frequency of TP53 mutations than EGFR or KRAS mutations in LUAD. Studies have shown that tumors with co-mutations of KRAS/TP53 usually show significant upregulation of PD-L1 expression and accumulation of tumor-killing T cells. In this regard, in addition to PD-L1 expression, TP53 mutations will hopefully guide the clinical application of immune checkpoint blocking therapy for KRAS mutated lung adenocarcinoma. However, the prognostic effect of TP53 mutation on EGFR mutant lung cancer is controversial. TP53/EGFR co-mutations may be associated with treatment resistance and shorter survival in lung cancer patients, the study showed. On the other hand, Labbe et al. (Zhang et al., 2019) studied 105 egFR-mutant NSCLC patients, and among the patients undergoing surgery, progressionfree survival (HR 0.99, 95%CI: 0.56-1.75) and overall survival (OS, HR 1.39, 95%CI: 0.70-2.77) was unrelated to TP53 status. Therefore, considering these controversial findings and evaluating the prognostic value of TP53/EGFR co-mutations in lung cancer will also be the focus of our subsequent studies.
In this meta-analysis, we investigated whether the survival benefits of ICIs in cancer patients vary according to KRAS/EGFR/TP53 single gene mutation status. We found that the PFS and OS of patients with KRAS/EGFR/TP5 single gene mutant tumors were significantly improved and the degree of improvement was different when treated with ICIs. TP53 mutant showed a better effect than KRAS mutation and EGFR mutation. These results suggest that KRAS/EGFR/TP53 single gene mutation status may be a potential biomarker of the survival benefits of ICIs. In the future, ICIs may play an important role in the treatment of early cancer. After radiotherapy and chemotherapy, immunotherapy has become the standard treatment of cancer, regardless of its mutation status. Even in the early stages of the disease, molecular characteristics will become the basis to determine the optimal treatment strategy and its combination with local regional treatment, especially in oncogene addiction diseases.
Considering the limited amount of data based on this meta-analysis, further research is needed to evaluate the effect of KRAS/EGFR/TP53 single gene mutation on the efficacy of ICIs in cancer patients. We also expect that the latest progress of next-generation sequencing technology will help to identify more accurate biomarkers and bring clinical benefits to ICIs immunotherapy.
The original contributions presented in the study are included in the article/Supplementary Material, further inquiries can be directed to the corresponding author.
SL, SG, and NS searched the database and analysed the data. LZ, WX, and YL selected the study and extracted the data. SL and SG wrote the manuscript. The manuscript was reviewed by KJ.
The authors declare that the research was conducted in the absence of any commercial or financial relationships that could be construed as a potential conflict of interest.
All claims expressed in this article are solely those of the authors and do not necessarily represent those of their affiliated organizations, or those of the publisher, the editors and the reviewers. Any product that may be evaluated in this article, or claim that may be made by its manufacturer, is not guaranteed or endorsed by the publisher.
Adderley, H., Blackhall, F. H., and Lindsay, C. R. (2019). KRAS-mutant Non-small Cell Lung Cancer: Converging Small Molecules and Immune Checkpoint Inhibition. EBioMedicine 41, 711–716. doi:10.1016/j.ebiom.2019.02.049
Ahn, B. C., Pyo, K. H., Xin, C. F., Jung, D., Shim, H. S., Lee, C. Y., et al. (2019). Comprehensive Analysis of the Characteristics and Treatment Outcomes of Patients with Non-small Cell Lung Cancer Treated with Anti-PD-1 Therapy in Real-World Practice. J. Cancer Res. Clin. Oncol. 145, 1613–1623. doi:10.1007/s00432-019-02899-y
Alexandrov, L. B., Nik-Zainal, S., Wedge, D. C., Aparicio, S. A., Behjati, S., Biankin, A. V., et al. (2013). Signatures of Mutational Processes in Human Cancer. Nature 500, 415–421. doi:10.1038/nature12477
An, J., Yan, M., Yu, N., Chennamadhavuni, A., Furqan, M., Mott, S. L., et al. (2021). Outcomes of Patients with Stage III Non-small Cell Lung Cancer (NSCLC) that Harbor a STK11 Mutation. Transl Lung Cancer Res. 10, 3608–3615. doi:10.21037/tlcr-21-177
Assoun, S., Theou-Anton, N., Nguenang, M., Cazes, A., Danel, C., Abbar, B., et al. (2019). Association of TP53 Mutations with Response and Longer Survival under Immune Checkpoint Inhibitors in Advanced Non-small-cell Lung Cancer. Lung Cancer 132, 65–71. doi:10.1016/j.lungcan.2019.04.005
Borghaei, H., Paz-Ares, L., Horn, L., Spigel, D. R., Steins, M., Ready, N. E., et al. (2015). Nivolumab versus Docetaxel in Advanced Nonsquamous Non-small-cell Lung Cancer. N. Engl. J. Med. 373, 1627–1639. doi:10.1056/NEJMoa1507643
Brahmer, J., Reckamp, K. L., Baas, P., Crinò, L., Eberhardt, W. E., Poddubskaya, E., et al. (2015). Nivolumab versus Docetaxel in Advanced Squamous-Cell Non-small-cell Lung Cancer. N. Engl. J. Med. 373, 123–135. doi:10.1056/NEJMoa1504627
Cho, J. H., Jung, H. A., Lee, S. H., Ahn, J. S., Ahn, M. J., Park, K., et al. (2019). Impact of EGFR Mutation on the Clinical Efficacy of PD-1 Inhibitors in Patients with Pulmonary Adenocarcinoma. J. Cancer Res. Clin. Oncol. 145, 1341–1349. doi:10.1007/s00432-019-02889-0
Dong, Z. Y., Zhong, W. Z., Zhang, X. C., Su, J., Xie, Z., Liu, S. Y., et al. (2017). Potential Predictive Value of TP53 and KRAS Mutation Status for Response to PD-1 Blockade Immunotherapy in Lung Adenocarcinoma. Clin. Cancer Res. 23, 3012–3024. doi:10.1158/1078-0432.CCR-16-2554
Facchinetti, F., Bluthgen, M. V., Tergemina-Clain, G., Faivre, L., Pignon, J. P., Planchard, D., et al. (2017). LKB1/STK11 Mutations in Non-small Cell Lung Cancer Patients: Descriptive Analysis and Prognostic Value. Lung Cancer 112, 62–68. doi:10.1016/j.lungcan.2017.08.002
Ferlay, J., Soerjomataram, I., Dikshit, R., Eser, S., Mathers, C., Rebelo, M., et al. (2015). Cancer Incidence and Mortality Worldwide: Sources, Methods and Major Patterns in GLOBOCAN 2012. Int. J. Cancer 136, E359–E386. doi:10.1002/ijc.29210
Fujimoto, D., Yoshioka, H., Kataoka, Y., Morimoto, T., Kim, Y. H., Tomii, K., et al. (2018). Efficacy and Safety of Nivolumab in Previously Treated Patients with Non-small Cell Lung Cancer: A Multicenter Retrospective Cohort Study. Lung Cancer 119, 14–20. doi:10.1016/j.lungcan.2018.02.017
Garassino, M. C., Gelibter, A. J., Grossi, F., Chiari, R., Soto Parra, H., Cascinu, S., et al. (2018). Italian Nivolumab Expanded Access Program in Nonsquamous Non-small Cell Lung Cancer Patients: Results in Never-Smokers and EGFR-Mutant Patients. J. Thorac. Oncol. 13, 1146–1155. doi:10.1016/j.jtho.2018.04.025
Gianoncelli, L., Spitaleri, G., Passaro, A., Radice, D., Fumagalli, C., Del Signore, E., et al. (2020). Efficacy of Anti-pd1/pd-L1 Therapy (IO) in KRAS Mutant Non-small Cell Lung Cancer Patients: A Retrospective Analysis. Anticancer Res. 40, 427–433. doi:10.21873/anticanres.13970
Guibert, N., Jones, G., Beeler, J. F., Plagnol, V., Morris, C., Mourlanette, J., et al. (2019). Targeted Sequencing of Plasma Cell-free DNA to Predict Response to PD1 Inhibitors in Advanced Non-small Cell Lung Cancer. Lung Cancer 137, 1–6. doi:10.1016/j.lungcan.2019.09.005
Hollern, D. P., Xu, N., Thennavan, A., Glodowski, C., Garcia-Recio, S., Mott, K. R., et al. (2019). B Cells and T Follicular Helper Cells Mediate Response to Checkpoint Inhibitors in High Mutation Burden Mouse Models of Breast Cancer. Cell 179, 1191–1206. doi:10.1016/j.cell.2019.10.028
Jeanson, A., Tomasini, P., Souquet-Bressand, M., Brandone, N., Boucekine, M., Grangeon, M., et al. (2019). Efficacy of Immune Checkpoint Inhibitors in KRAS-Mutant Non-small Cell Lung Cancer (NSCLC). J. Thorac. Oncol. 14, 1095–1101. doi:10.1016/j.jtho.2019.01.011
Kartolo, A., Feilotter, H., Hopman, W., Fung, A. S., and Robinson, A. (2021). A Single Institution Study Evaluating Outcomes of PD-L1 High KRAS-Mutant Advanced Non-small Cell Lung Cancer (NSCLC) Patients Treated with First Line Immune Checkpoint Inhibitors. Cancer Treat. Res. Commun. 27, 100330. doi:10.1016/j.ctarc.2021.100330
Lazarus, G., Audrey, J., and Iskandar, A. W. B. (2019). Efficacy and Safety Profiles of Programmed Cell Death-1/programmed Cell Death Ligand-1 Inhibitors in the Treatment of Triple-Negative Breast Cancer: A Comprehensive Systematic Review. Oncol. Rev. 13, 425. doi:10.4081/oncol.2019.425
Le, D. T., Durham, J. N., Smith, K. N., Wang, H., Bartlett, B. R., Aulakh, L. K., et al. (2017). Mismatch Repair Deficiency Predicts Response of Solid Tumors to PD-1 Blockade. Science 357, 409–413. doi:10.1126/science.aan6733
Lin, S. Y., Yang, C. Y., Liao, B. C., Ho, C. C., Liao, W. Y., Chen, K. Y., et al. (2018). Tumor PD-L1 Expression and Clinical Outcomes in Advanced-Stage Non-small Cell Lung Cancer Patients Treated with Nivolumab or Pembrolizumab: Real-World Data in Taiwan. J. Cancer 9, 1813–1820. doi:10.7150/jca.24985
Lyu, Q., Lin, A., Cao, M., Xu, A., Luo, P., and Zhang, J. (2020). Alterations in TP53 Are a Potential Biomarker of Bladder Cancer Patients Who Benefit from Immune Checkpoint Inhibition. Cancer Control 27, 1073274820976665. doi:10.1177/1073274820976665
Marinelli, D., Mazzotta, M., Scalera, S., Terrenato, I., Sperati, F., D'Ambrosio, L., et al. (2020). KEAP1-driven Co-mutations in Lung Adenocarcinoma Unresponsive to Immunotherapy Despite High Tumor Mutational burden. Ann. Oncol. 31, 1746–1754. doi:10.1016/j.annonc.2020.08.2105
Moore, A. R., Rosenberg, S. C., McCormick, F., and Malek, S. (2020). RAS-targeted Therapies: Is the Undruggable Drugged? Nat. Rev. Drug Discov. 19, 533–552. doi:10.1038/s41573-020-0068-6
Morita, R., Okishio, K., Shimizu, J., Saito, H., Sakai, H., Kim, Y. H., et al. (2020). Real-world Effectiveness and Safety of Nivolumab in Patients with Non-small Cell Lung Cancer: A Multicenter Retrospective Observational Study in Japan. Lung Cancer 140, 8–18. doi:10.1016/j.lungcan.2019.11.014
Motzer, R. J., Robbins, P. B., Powles, T., Albiges, L., Haanen, J. B., Larkin, J., et al. (2020). Avelumab Plus Axitinib versus Sunitinib in Advanced Renal Cell Carcinoma: Biomarker Analysis of the Phase 3 JAVELIN Renal 101 Trial. Nat. Med. 26, 1733–1741. doi:10.1038/s41591-020-1044-8
Nagasaka, M., Li, Y., Sukari, A., Ou, S. I., Al-Hallak, M. N., and Azmi, A. S. (2020). KRAS G12C Game of Thrones, Which Direct KRAS Inhibitor Will Claim the Iron Throne? Cancer Treat. Rev. 84, 101974. doi:10.1016/j.ctrv.2020.101974
Ng, T. L., Liu, Y., Dimou, A., Patil, T., Aisner, D. L., Dong, Z., et al. (2019). Predictive Value of Oncogenic Driver Subtype, Programmed Death-1 Ligand (PD-L1) Score, and Smoking Status on the Efficacy of PD-1/pd-L1 Inhibitors in Patients with Oncogene-Driven Non-small Cell Lung Cancer. Cancer 125, 1038–1049. doi:10.1002/cncr.31871
Ozaki, Y., Muto, S., Takagi, H., Watanabe, M., Inoue, T., Fukuhara, M., et al. (2020). Tumor Mutation burden and Immunological, Genomic, and Clinicopathological Factors as Biomarkers for Checkpoint Inhibitor Treatment of Patients with Non-small-cell Lung Cancer. Cancer Immunol. Immunother. 69, 127–134. doi:10.1007/s00262-019-02446-1
Pauken, K. E., Sammons, M. A., Odorizzi, P. M., Manne, S., Godec, J., Khan, O., et al. (2016). Epigenetic Stability of Exhausted T Cells Limits Durability of Reinvigoration by PD-1 Blockade. Science 354, 1160–1165. doi:10.1126/science.aaf2807
Schoenfeld, A. J., Rizvi, H., Bandlamudi, C., Sauter, J. L., Travis, W. D., Rekhtman, N., et al. (2020). Clinical and Molecular Correlates of PD-L1 Expression in Patients with Lung Adenocarcinomas. Ann. Oncol. 31, 599–608. doi:10.1016/j.annonc.2020.01.065
Shi, Y., Au, J. S., Thongprasert, S., Srinivasan, S., Tsai, C. M., Khoa, M. T., et al. (2014). A Prospective, Molecular Epidemiology Study of EGFR Mutations in Asian Patients with Advanced Non-small-cell Lung Cancer of Adenocarcinoma Histology (PIONEER). J. Thorac. Oncol. 9, 154–162. doi:10.1097/JTO.0000000000000033
Song, P., Yang, D., Wang, H., Cui, X., Si, X., Zhang, X., et al. (2020). Relationship between the Efficacy of Immunotherapy and Characteristics of Specific Tumor Mutation Genes in Non-small Cell Lung Cancer Patients. Thorac. Cancer 11, 1647–1654. doi:10.1111/1759-7714.13447
Van Allen, E. M., Miao, D., Schilling, B., Shukla, S. A., Blank, C., Zimmer, L., et al. (2015). Genomic Correlates of Response to CTLA-4 Blockade in Metastatic Melanoma. Science 350, 207–211. doi:10.1126/science.aad8366
Wang, X., Wang, G., Wang, Z., Liu, B., Han, N., Li, J., et al. (2019). PD-1-expressing B Cells Suppress CD4(+) and CD8(+) T Cells via PD-1/pd-L1-dependent Pathway. Mol. Immunol. 109, 20–26. doi:10.1016/j.molimm.2019.02.009
Xiang, L., Fu, X., Wang, X., Li, W., Zheng, X., Nan, K., et al. (2020). A Potential Biomarker of Combination of Tumor Mutation Burden and Copy Number Alteration for Efficacy of Immunotherapy in KRAS-Mutant Advanced Lung Adenocarcinoma. Front. Oncol. 10, 559896. doi:10.3389/fonc.2020.559896
Xiao, W., Du, N., Huang, T., Guo, J., Mo, X., Yuan, T., et al. (2018). TP53 Mutation as Potential Negative Predictor for Response of Anti-CTLA-4 Therapy in Metastatic Melanoma. EBioMedicine 32, 119–124. doi:10.1016/j.ebiom.2018.05.019
Zhang, R., Tian, P., Chen, B., Wang, T., and Li, W. (2019). The Prognostic Impact of TP53 Comutation in EGFR Mutant Lung Cancer Patients: a Systematic Review and Meta-Analysis. Postgrad. Med. 131 (3), 199–206. doi:10.1080/00325481.2019.1585690
Keywords: immune checkpoint inhibitors, cancer, gene mutation, progression free survival, overall survival, survival benefit
Citation: Liu S, Geng S, Shi N, Zhang L, Xue W, Li Y and Jiang K (2022) Survival Prediction of Patients Treated With Immune Checkpoint Inhibitors via KRAS/TP53/EGFR-Single Gene Mutation. Front. Pharmacol. 13:878540. doi: 10.3389/fphar.2022.878540
Received: 18 February 2022; Accepted: 08 March 2022;
Published: 23 March 2022.
Edited by:
Ting Wang, Sichuan Cancer Hospital, ChinaReviewed by:
Mingming Wu, Harbin Medical University, ChinaCopyright © 2022 Liu, Geng, Shi, Zhang, Xue, Li and Jiang. This is an open-access article distributed under the terms of the Creative Commons Attribution License (CC BY). The use, distribution or reproduction in other forums is permitted, provided the original author(s) and the copyright owner(s) are credited and that the original publication in this journal is cited, in accordance with accepted academic practice. No use, distribution or reproduction is permitted which does not comply with these terms.
*Correspondence: Kai Jiang, empqaWFuZ2thaUAxNjMuY29t
†These authors have contributed equally to this work
Disclaimer: All claims expressed in this article are solely those of the authors and do not necessarily represent those of their affiliated organizations, or those of the publisher, the editors and the reviewers. Any product that may be evaluated in this article or claim that may be made by its manufacturer is not guaranteed or endorsed by the publisher.
Research integrity at Frontiers
Learn more about the work of our research integrity team to safeguard the quality of each article we publish.