- 1Department of Medical Parasitology and Mycology, Arak University of Medical Sciences, Arak, Iran
- 2Department of Microbiology, School of Medicine, Hamadan University of Medical Sciences, Hamadan, Iran
- 3Molecular and Medicine Research Center, Khomein University of Medical Sciences, Khomein, Iran
- 4Department of Medical Laboratory Sciences, Khomein University of Medical Sciences, Khomein, Iran
The high occurrence and mortality rates related to candidiasis emphasize the urgent need to introduce new therapeutic approaches to treat this infection. Eugenol, the main phenolic component of Clove and Cinnamomum essential oil, has been used to inhibit growth and different virulence factors of Candida, including strains with decreased susceptibility to antifungals, particularly fluconazole. The results showed that this compound could bind to Candida membrane and decrease ergosterol biosynthesis, consequently leading to cell wall and membrane damage. Additionally, eugenol not only reduced germ tube formation, which reduces nutrient absorption from host tissues, but it also increased the levels of lipid peroxidation and reactive oxygen species, which induces oxidative stress and causes high permeability in the fungal cell membrane. Eugenol inhibited Candida cells’ adhesion capacity; additionally, this compound inhibited the formation of biofilms and eliminated established Candida biofilms on a variety of surfaces. Furthermore, by disrupting fungal cell integrity, eugenol could boost the entry of the antifungal drugs into the Candida cell, improving treatment efficacy. Therefore, eugenol could be used in the clinical management of various presentations of candidiasis, especially mucocutaneous presentations such as oral and vulvovaginal infections. However, further investigations, including in vivo and animal studies, toxicology studies and clinical trials, as well as molecular analysis, are needed to improve formulations and develop novel antifungal agents based on eugenol.
Introduction
Candidiasis is emerging as a significant challenge in the healthcare environment due to its high medical costs and high mortality rates (Amirrajab et al., 2016; Pristov and Ghannoum, 2019; Shariati et al., 2020). Recent studies reported systemic infections caused by Candida species (Candida) as the fourth leading cause of nosocomial bloodstream infections. Opportunistic, these fungi are responsible for 90% of all invasive infections (Spampinato and Leonardi, 2013; Marak and Dhanashree, 2018; Sakagami et al., 2019). Although this family contains at least 15 distinct species, five pathogens could lead to invasive infections with a high mortality rate in humans: Candida albicans, Candida tropicalis, Candida parapsilosis, Candida glabrata, and Candida krusei (Pappas et al., 2018).
C. albicans is the most frequent etiology of candidiasis in clinical settings that could lead to a range of harmless superficial mycosis to life-threatening invasive infections. Nevertheless, non-C. albicans species collectively could represent >50% of the bloodstream isolates in certain regions (Wisplinghoff et al., 2004; Kullberg and Arendrup, 2015). In this regard, Candida auris, a previously rare fungus, has been reported as a major pathogen in certain parts of the world (Chowdhary et al., 2017). Overall, Candida species reside in healthy hosts as commensal yeasts in different parts of the human body, such as the gut and skin. Moreover, they are detectable in up to 60% of healthy individuals. However, immunocompromised status could lead to these fungi invading the host tissues and disseminating to internal organs (Pristov and Ghannoum, 2019).
Candida pathogenicity depends on various virulence factors such as yeast-hyphal formation and secretion of proteolytic as well as lipolytic enzymes, along with biofilm formation. Among the mentioned virulence factors, biofilm community of Candida has a potential role in pathogenicity. This biofilm community has a three-dimensional structure comprised of hyphal and yeast cells within a self-secreted matrix of extracellular polymeric substances (EPS), consisting of proteins, polysaccharides, and nucleic acids (Abirami et al., 2020). Biofilms are easily formed on the surface of medical devices such as intravascular and urinary catheters, artificial valves, intrauterine devices, contact lenses, and any foreign objects or host surfaces. Accordingly, the biofilm community of Candida leads to persistent infections and reduced susceptibility to host immune responses as well as antifungal drug therapy (Lohse et al., 2018; Abirami et al., 2020). Furthermore, the surface-associated virulence factors, such as adhesins and degradative virulence enzymes, including phospholipases and proteases have distinguishable roles in attachment and invasion into the host cells, while also strengthening C. albicans pathogenicity by destroying the vital proteins of the skin and cell membrane lipids (Mayer et al., 2013).
The specific type and dose of antifungal medication used to treat invasive candidiasis usually depends on the patient’s age, location and severity of the infection, as well as immune status. Accordingly, various antifungals such as echinocandins (caspofungin, micafungin, or anidulafungin) and azoles (fluconazole) are recommended to manage and treat invasive candidiasis (Fisher et al., 2011; Arendrup and Patterson, 2017). However, azoles-resistance and cross-resistances to azoles and echinocandins in fungi are now considered one of the main challenges in treating fungal infections. In this regard, a higher prevalence of drug resistance in Candida has increased the candidiasis-associated mortality rate (Zore et al., 2011). Furthermore, other drawbacks such as high cost, side effects and toxicity, and lack of fungicidal efficacy limit the usage of antifungals (Kauffman and Carver, 1997). These limitations justify the development of new therapeutic approaches or the discovery of novel antifungals to inhibit Candida-associated infections. Bearing this in mind, plant-derived substances due to high efficacy, few adverse effects, and low cost have shown potential capacity to inhibit these fungi, even azole-resistant strains (Ahmad et al., 2010a; Abrahão et al., 2013; Nisar et al., 2021).
Eugenol or 2-methoxy-4-[2-propenyl] phenol, a phenolic aromatic compound mainly derived from Cinnamomum and Clove essential oil, is one of these natural compounds belonging to a novel class of microbiocidal phenylpropanoids and has been used for a long time as an analgesic in dentistry (Hassan et al., 2018; Wijesinghe et al., 2021). Eugenol can be synthesized by guaiacol allylation with allyl chloride or produced through a biotransformation process that involves microorganisms such as Bacillus cereus, Corynebacterium species, and Escherichia coli (Abrahão et al., 2013). Eugenol has also indicated anesthetic, neuroprotective, antidiabetic, insecticidal, analgesic, anti-inflammatory, and antifungal properties that make this compound a versatile natural ingredient that helps prevent and cure various disorders (Kozam, 1977; Reddy and Lokesh, 1994; Hassan et al., 2018; Nisar et al., 2021).
Eugenol leads to a better penetration of different drugs in the skin. This compound has also been used as a pesticide and fumigant to protect foods from microbial invasion such as Lactobacillus and Listeria monocytogenes during storage. Additionally, the United States Food and Drug Administration approved the use of eugenol and clove oil as a natural antiseptic and analgesic in dentistry and as a fragrance in soaps and cosmetics. Furthermore, this compound has been used as a flavoring substance in food and pharmaceutical products (Kamatou et al., 2012; Mohammadi Nejad et al., 2017).
Eugenol exhibits significant antifungal activity against various fungal species, including dermatophytes, Aspergillus, and Candida, which is primarily due to damage to the fungal cell envelope, biofilm community, and various virulence factors (Chami et al., 2005; Hassan et al., 2018). As a result, researchers are interested in using eugenol and synthetic analogues, resorting to various drug delivery systems to inhibit Candida, biofilm communities, and several cellular pathways associated with these fungi.
This review focuses on the interaction of eugenol and various plant species with a high eugenol content with different cellular pathways in Candida cells. Additionally, we discuss the synergistic antimicrobial activity of eugenol when combined with antifungal drugs to facilitate its widespread use in clinical practice. Notably, the present study undertook a Medline (via PubMed) search using the following search keywords obtained from the National Library of Medicine’s Medical Subject Heading (MeSH) terms, titles, or abstracts using Boolean Operators (and, or): “Eugenol” and “Candida” or “Candidiasis” or “C. albicans”.
Inhibitory effect of eugenol against Candida species
In vitro antifungal studies
Recent in vitro studies used eugenol to inhibit Candida. In this regard, Marcos-Arias et al. evaluated the antifungal effects of this substance on a collection of oral Candida isolates from denture-wearers. The results showed that eugenol inhibited Candida growth at the Minimum inhibitory concentration (MIC) range 0.03%–0.25%. Furthermore, this compound showed potential inhibitory effects at very low concentrations against the susceptible-dose-dependent C. glabrata and the fluconazole-resistant C. krusei isolates (Marcos-Arias et al., 2011).
Another investigation was also performed using Clove essential oil (EO), collected from Syzygium aromaticum, against a collection of Candida strains isolated from patients with recurrent oral candidiasis. Gas Chromatography/Mass Spectrometry (GC/MS) analysis indicated eugenol (85.3%) as the main component of the EO. This compound showed inhibitory effects against all the tested Candida strains (MIC 0.08–0.64 μl/ml), including fungi with decreased susceptibility to fluconazole. Notably, inhibition of ergosterol synthesis and flow cytometry analysis revealed that this EO could cause extensive lesion of the Candida cell membrane via a significant decrease in ergosterol quantity and germ tube formation (Pinto et al., 2009). Noteworthy, ergosterol is a specific fungal cell membrane component, and germ tube formation is generally considered one of the most important C. albicans pathogenicity mechanisms that enables the absorption of nutrients from host tissues by invading them (Pinto et al., 2008). Therefore, the interaction of eugenol with ergosterol and germ tube formation could inhibit Candida-associated infections.
Additionally, recent research has examined the interaction of eugenol with C. albicans (CA04) using various techniques. Electron microscopy showed multiple sites of action in C. albicans, such as injuries to cytoplasmic contents, cell membranes, and cell walls after treatment with 200 μl/ml of eugenol. Furthermore, C. albicans cells exposure to eugenol led to 50% and 76% of dead cells and reduction in ergosterol biosynthesis, respectively. Thus, eugenol’s ability to bind on the C. albicans membrane and decrease the ergosterol biosynthesis may be associated with the cell wall and cell membrane damage (Kerekes et al., 2013).
These data support the findings by Ahmad et al.(2010a) who explored the antifungal effects of eugenol and methyleugenol against various Candida isolates. The authors reported that these components blocked ergosterol synthesis at their MIC values. Methyleugenol, based on the concentrations required to suppress Candida growth, showed higher antifungal activity than eugenol (Ahmad et al., 2010b). Moreover, another study also indicated that eugenol changed the morphogenesis of C. albicans envelope (Braga et al., 2007). In this respect, the authors proposed that eugenol could be a promising anti-Candida agent because it interferes with the morphology of the envelope of C. albicans and prevents morphological transition to hyphal form and adhesion, consequently decreasing C. albicans ability to colonize host tissues and its pathogenesis (Braga et al., 2007).
Furthermore, Khan et al. (2011) indicated that eugenol and methyleugenol, in addition to the inhibition of ergosterol biosynthesis, induced oxidative stress and caused high permeability in the cell membrane. It is noteworthy to mention that, besides being essential to maintain functional integrity and structure of the membrane, ergosterol also inhibits lipid peroxidation (LPO). LPO is one of the most important expressions of oxidative stress induced by reactive oxygen species (ROS). In fact, ROS interact with unsaturated lipids and manufacture polar lipid hydroperoxides that may increase membrane fluidity by disorganizing hydrophobic phospholipids. Thus, eugenol and methyleugenol may increase the level of LPO and ROS, thereby inducing elevated levels of oxidative stress in C. albicans (Khan et al., 2011).
These results were in line with Gupta et al. (2018) findings, who reported that eugenol eliminated around 80% of C. glabrata clinical isolates biofilm from biomaterials and increased ROS generation, cell lysis, and ergosterol content in the plasma membrane. Nonetheless, this compound inhibited catalase, proteinase, and phospholipase activity in C. glabrata cells. Interestingly, different genes such as FKS, KRE1, and AUS1 were downregulated in response to eugenol treatment, where these genes were associated with 1,3-β-glucan synthase, GPI-anchored protein, and sterol importer, respectively. Contrary to previous studies, eugenol increased ergosterol content in C. glabrata cells. The differences in the ergosterol content detected in this study compared to other studies could be attributed to the divergent structure of C. glabrata cell membrane in comparison to C. albicans. Furthermore, the difference in eugenol concentrations may lead to this differentiation; however, further studies are needed to clarify this (Gupta et al., 2018).
Finally, the findings of Ahmad et al. (2010c) study showed that eugenol significantly inhibited H + -ATPase activity and glucose-stimulated H + -extrusion in various clinical isolates of Candida. Notably, H + -ATPase inhibition causes intracellular acidification and cell death. Suppression of cell growth and H + -efflux by eugenol suggests that its antifungal characteristics are also due to its prohibiting activity on H + -ATPase. Therefore, the authors suggested that it would be useful to further study eugenol’s interaction with the purified PM-ATPase enzyme and evaluate its function in both pre-steady and steady-state (Ahmad et al., 2010c). To this end, other studies also used eugenol to inhibit Candida growth, as reported in Table 1. These studies also reported acceptable inhibitory effect for eugenol against Candida species.
Collectively, eugenol may inhibit Candida growth and pathogenesis via the following mechanisms: suppression of plasma membrane ATPase, which may play a role in hydrolytic enzyme secretion, ROS production, and apoptosis; disruption of Candida cell structure by binding to and removing membrane ergosterol; and disruption of gene function that may play a role in membrane biosynthesis (Figure 1) (Gupta et al., 2018). However, more in vitro and molecular studies are needed to understand the exact interaction of eugenol with various Candida cellular pathways.
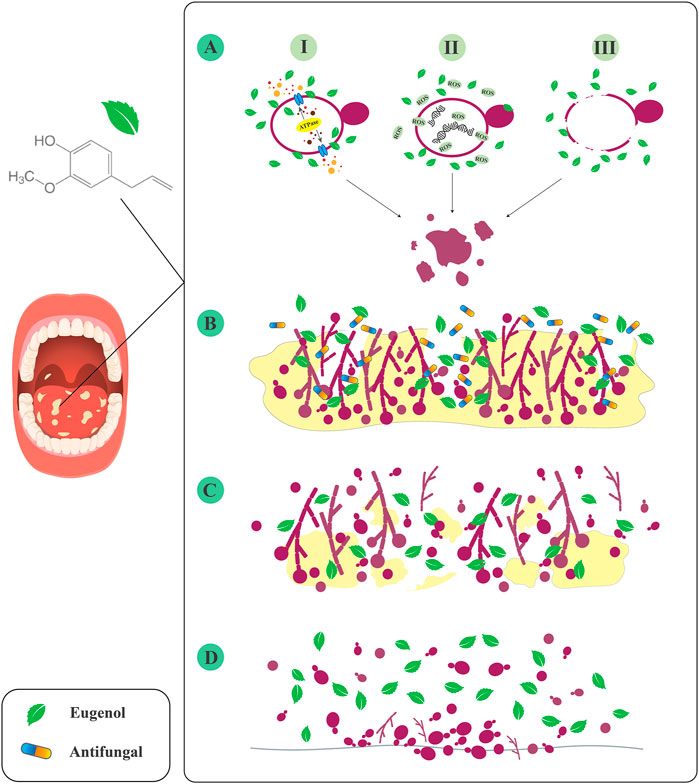
FIGURE 1. Inhibitory effects of eugenol against Candida species. (A) I: inhibition of plasma membrane ATPase. II: ROS production. III: disruption of cell structure. (B) Increase the penetration of antifungals to the deeper layers of biofilm. (C) Destruction of mature biofilm. (D) Inhibition of Candida attachment to the various surfaces.
Anti-biofilm effects
The biofilm community of Candida is resistant to various antifungal treatments and environmental conditions. In this regard, Candida’s ability to form biofilm on abiotic and biotic surfaces is considered one of its most important virulence factors. Extracellular DNA and Exopolysaccharides (EPS) decrease antifungal penetration to the deeper layers of the biofilm, which is a serious concern that is aggravated by the emergence of azole-resistant isolates and the selection of Candida with decreased antifungal susceptibility (Williams et al., 2012; Cirasola et al., 2013). Since the biofilms’ resistance to common antifungal drugs has become more widespread in recent years, more investigations should be performed to produce novel, inexpensive, non-toxic, and effective treatment approaches by mainly controlling biofilm-associated infections. Accordingly, recent studies have focused on eugenol’s ability to inhibit Candida biofilm formation.
The results of He et al. (2007) study showed that eugenol could suppress the adhesion capacity and biofilm community of C. albicans. Additionally, the treatment of this fungus with this compound led to scant biofilms with inhibited filamentous growth. Notably, C. albicans is a dimorphic fungus that can switch from the yeast to filamentous phase. The filamentous phenotype of this fungus is essential for pathogenicity and could have a main role in producing the spatially organized architecture seen in mature, highly structured C. albicans biofilms (López-Ribot, 2005). Thus, these results proposed that eugenol could affect C. albicans cells’ morphogenesis and inhibit biofilm formation, thus attenuating this fungus invasive capacity (He et al., 2007).
Furthermore, molecular docking in another study showed the interaction of eugenol with Als3 (El-Baz et al., 2021). The Als adhesive proteins are one of the most extensively studied virulence characteristics of C. albicans, with the elimination of Als3 resulting in a considerable decrease in fungal adhesion capacity. The binding capacity of other natural compounds such as cinnamaldehyde was lower in comparison to that of eugenol (Hoyer and Cota, 2016). Thus, the interaction of eugenol and Als3 could be a promising finding for using this compound to inhibit C. albicans adhesion and biofilm formation (El-Baz et al., 2021).
In addition, eugenol, as a significant constituent of Cinnamomum, has indicated encouraging antibiofilm effects against Candida. In a study performed in 2018, the authors used Cinnamomum zeylanicum EO to inhibit Candida biofilm. The results showed that C. zeylanicum EO (500 μg/ml) remarkably suppressed the formation of Candida biofilm and inhibited monospecies (C. tropicalis) as well as multispecies biofilm community formed by these fungi. On the other hand, this EO at 1,000 μg/ml did not reduce human red blood cells viability. According to phytochemical evaluations, eugenol was found as the main component (68.96%) of the EO extracted from C. zeylanicum Blume leaves (Rangel et al., 2018). In another study, eugenol was also reported as the main compound (77.22%) of Cinnamomum verum EO. This compound suppressed initial adhesion, germ tube formation, and biofilm progression of Candida. Furthermore, microscopy evaluation demonstrated cell wall damages, diminished hyphal formation, and cellular shrinkages in Candida cells after treatment with this EO. On the other hand, no lethal effect of C. verum EO was observed using the Galleria mellonella experiment model at the various concentrations tested (Wijesinghe et al., 2020).
Wijesinghe et al. (2021) also reported eugenol as the main compound of C. verum EO. This EO destroyed the established biofilm community as well as hyphal production in Candida, and also led to cell wall damages and cellular shrinkages in these fungi. Nevertheless, C. verum EO did not show any effects against HaCaT (aneuploid immortal keratinocyte cell line from adult human skin) cells (Wijesinghe et al., 2021). Therefore, eugenol would destroy the cell wall integrity of Candida cell and suppress the biofilm community of this fungus. Furthermore, recent studies have reported a low cytotoxicity effect for this compound against different human cells.
Additionally, eugenol could also be used for the treatment of oral disorders that are associated with Candida biofilm. In this regard, in a study conducted by Jafri et al.(2019) this compound was used to suppress single and mixed biofilms of C. albicans [resistant to ketoconazole and itraconazole, as well as amphotericin B (AMB)] and Streptococcus mutans. The interaction of these two significant oral pathogens may result in recalcitrant and resistant infections in the oral cavity, thereby increasing the complexity of oral infection management. Microscopy evaluation indicated that eugenol caused cell shape alteration, less cell aggregation, as well as disarrangement of the single and mixed biofilms. Thus, at sub-MICs (100 μg/ml), eugenol significantly suppressed single and mixed biofilms formed by the drug-resistant strains of two oral pathogens (Jafri et al., 2019). Another investigation also reported inhibitory effects for eugenol against the planktonic and biofilm community of C. tropicalis and C. dubliniensis (dose-dependent and fluconazole-resistant strains) isolated from the oral cavity of HIV positive patients. No metabolic activity was detected in the biofilm after 24 h of treatment with eugenol (500 μg/ml); further, this compound markedly reduced biofilm cells on denture material surfaces. Also, eugenol significantly avoided the adhesion of Candida to the polystyrene and HEp-2 cells; thus, the authors proposed that eugenol may have an additional beneficial effect in the treatment of local candidiasis (de Paula et al., 2014).
Accordingly, the results reported in the mentioned studies showed that eugenol not only could potentially suppress the adhesion capacity of planktonic cells of Candida, but also could have a fungicidal activity against this fungus. Furthermore, this compound inhibited biofilm formation and destroyed the established biofilm of these fungi formed on various surfaces. Thus, eugenol is a natural compound with potential for non-toxic therapeutic application in the treatment of candidiasis by interfering with the most important virulence factors of Candida species, such as germ tube formation, adhesion to various host surfaces, and biofilm formation. However, the exact mechanism of antifungal and antibiofilm effects of eugenol against Candida was not clearly understood; therefore, further research is required.
Combination therapy
Recent studies showed that new antifungal agents are needed to decrease the toxicity of conventional antifungals. Additionally, using combination therapy could improve the efficacy of various antifungals such as fluconazole (Pinto et al., 2009; Ahmad et al., 2010a). In this concept, the combined eugenol and different antifungal drugs were considered to inhibit Candida and their biofilm community. Indeed, a recently published study reported that eugenol, the main component of Ocimum campechianum Mill., could increase the efficacy of fluconazole against clinical Candia strains (Tacchini et al., 2021). Furthermore, Ahmad et al. (2010a) reported that eugenol and methyleugenol were active against fluconazole-resistant clinical Candida isolates. The interaction between methyleugenol and eugenol with fluconazole was synergistic in 92% and 90% of the fluconazole-susceptible strains, respectively. Additionally, 85% and 91% of the isolates resistant to fluconazole indicated synergistic effects of eugenol plus fluconazole and methyleugenol plus fluconazole, respectively. Notably, antagonistic interaction between the mentioned compounds was not detected in the strains tested (Ahmad et al., 2010a).
Moreover, previous studies considered the combination therapy of eugenol and fluconazole against Candida biofilms. An experiment conducted in 2014 reported a synergistic effect for combinations of fluconazole and eugenol against a planktonic community of C. albicans. The established biofilm of this fungus was highly resistant to fluconazole; while, sensitization of fungal cells by eugenol (sub-inhibitory concentrations) led to the prevention of biofilm formation at low fluconazole concentrations. The authors hypothesized that eugenol destabilizes the membrane and specific signal intervention. Hence, this compound caused sensitization of C. albicans biofilms, boosting fluconazole penetration and leading to the inhibition of biofilm formation (Doke et al., 2014).
Additionally, another study reported that pre-formed C. albicans biofilms showed ≥1,024× increased resistance to fluconazole, while biofilm formation did not cause tolerance to eugenol. This compound inhibited the biofilm formation of C. albicans and showed a synergistic interaction with fluconazole against biofilms formed by the test strains. The microscopy evaluation also showed eugenol interference with cell membrane integrity, as evidenced by shrinkage of the cell surface in biofilm cells. Therefore, the authors proposed that when eugenol has cidal activity against biofilm cells if combined with fluconazole, the drug’s fungistatic nature is converted to fungicidal (Khan and Ahmad, 2012).
In line with these observations, another investigation performed in 2020 in India reported that eugenol suppressed S. mutans and C. albicans mixed biofilms. The sessile MIC of fluconazole was increased up to 1000-fold over planktonic MIC. Notably, eugenol was highly synergistic with fluconazole against C. albicans single and mixed biofilms. The microscopy analysis also confirmed these findings and showed distorted cell structure, decreased matrix production, and elimination of single and mixed biofilm cells of S. mutans and C. albicans in treated samples with eugenol compared to untreated samples. Therefore, eugenol may disrupt cell membrane integrity and boost the drug entry into the microbial cell. This phenomenon increases the antimicrobial drug availability in the deeper layers and target sites of biofilm, consequently improving treatment efficacy (Jafri et al., 2020).
In addition to fluconazole, eugenol and other antifungals combined also showed promising results for inhibiting C. albicans growth. In this regard, Khan et al. (2012) reported that eugenol has a potential antifungal capability to inhibit fluconazole, itraconazole, and ketoconazole-resistant isolates of C. albicans. Furthermore, this compound showed remarkable synergy with fluconazole and AMB against the test isolates (Khan et al., 2012). The results of a recently published study also revealed that the combination of eugenol and sub-MIC (0.05 mg/ml) of AMB indicated many-fold higher anti-fungal effect against C. albicans compared to single component therapy. Scanning electron microscopy (SEM) showed the following characteristics for C. albicans cells after combination therapy: completely ruptured and shrank cells that aggregated as irregularly shaped material. Moreover, the authors reported that combination therapy induced ROS potentiation in C. albicans cells and with cellular damages, decreased mitochondria levels, and enhanced cytosol cytochrome C levels. Besides, the combination of eugenol and AMB resulted in an intense decline of intracellular Ca2+ concentration in C. albicans cells compared to the single treatments (Khan et al., 2019).
Noteworthy, the expansion of the inner mitochondrial membrane upon matrix swelling could damage the outer membrane, release the cytochrome C to the cytosol, and induce cell death (Wu et al., 2009). Therefore, these findings suggested that eugenol, in addition to C. albicans growth inhibition, could act synergistically with AMB (at less toxic doses) by interfering in the different cellular pathways of this fungus (Khan et al., 2019).
Another study reported a synergistic effect of eugenol in combination with voriconazole against voriconazole-resistant C. tropicalis and C. krusei isolated from the genital tract of mares (Sharifzadeh and Shokri, 2021). To this end, Dąbrowska et al. (2021) reported that eugenol indicated additive and synergistic activities with econazole and miconazole against clinical isolates of C. albicans, respectively.
Therefore, combining eugenol with various antifungal drugs could lead to several benefits such as decreased dose of drugs needed, boosted potency, and minimized toxicity, which ultimately helps suppress or eliminate biofilms and overcome fungal infections caused by drug-resistant Candida strains. However, the precise mechanism by which eugenol synergizes with various antifungals was not investigated in the studies mentioned above. Thus, additional molecular and in vivo studies are required to establish the practical utility of these combinations.
Animal studies
Unfortunately, most in vitro observations of eugenol’s interaction with Candida cells have not been confirmed in animal models. However, some studies have evaluated this compound’s anti-Candida activity in in vivo, and this section discusses those studies.
Established murine models have been used for studying most important clinical forms of candidiasis such as vaginal, disseminated, cutaneous, and oropharyngeal. To this end, to evaluate Candida pathogenies and host responses to the treatment, mice treated with depleting antibodies and genetically modified mice (transgenics and knockouts) are generally used. Additionally, different strains of Candida including genetically modified laboratory strains and clinical isolates are used for analysis of Candida pathogenesis in animal models. C. albicans does not normally colonize the mice mucosa, though under different conditions of immunosuppression, disease can be induced (Solis and Filler, 2012; Conti et al., 2014).
Chami et al. (2004b) used eugenol (topical usage) to treat oral candidiasis induced by C. albicans in immunocompromised rats, where nystatin was used as a positive control treatment. Treatment with eugenol for eight consecutive days remarkably decreased the number of colony-forming units (CFU) sampled from the treated rats’ oral cavity compared to the control rats. Histopathologic examination (HE) revealed that the epithelium of the dorsal surface of the tongue of the untreated control rats was colonized with many fungal hyphae. Nevertheless, only a few focalized zones of the dorsal surface of the tongue were occupied by hyphae in eugenol-treated rats. Furthermore, no acute cytotoxicity effects were detected in eugenol-treated rats. Notably, HE revealed numerous hyphae in the tongue folds of rats treated with nystatin; however, this evaluation revealed no fungal hyphae in the fold following eugenol treatment. The authors suggested that this could be related to eugenol’s volatility characteristics, which allowed its penetration into unreachable zones, such as folds of the tongue (Chami et al., 2004b).
These data aligned with another investigation conducted in 2005 where the authors used eugenol to inhibit oral candidiasis in immunocompromised rats. The findings of this study also showed that eugenol had fungicidal effects against C. albicans. Moreover, treating rats’ oral candidiasis with this compound (eight consecutive days) led to a significant reduction of C. albicans colony count compared to the untreated control rats (Chami et al., 2005).
In another investigation, the authors also used eugenol to prevent and treat vaginal candidiasis caused by C. albicans in the immunosuppressed rat model. In this manner, to permit maximum adhesion of eugenol to the vaginal mucosa, a gelatinous suspension of 0.8% agar (as excipient) was used to treat rats through the intravaginal route. Ten days after infection, prophylactic treatment with this compound reduced C. albicans CFU in the vaginas of infected rats by 98.9%. Additionally, treatment with eugenol for seven consecutive days completely inhibited infection in 23% (2/9) of infected rats, whereas an 84% reduction in C. albicans colony count was detected in the vaginas of the other rats. HE of the lumina of the vagina also showed no C. albicans hyphae in all treated rats (Chami et al., 2004a). It should be noted that all of the above-mentioned candidiasis models in rats required an immunosuppressive host. Thus, the authors proposed eugenol as a potentially useful antifungal agent for preventing and treating candidiasis, particularly in patients with immunosuppressive conditions such as AIDS.
Finally, a recently published study evaluated the treatment effects of eugenol against fluconazole-resistant C. albicans-induced keratitis in the rabbit model. The results showed that 4 mg/ml of eugenol was the highest dose with non-toxic effects in the rabbit’s corneas. High-performance liquid chromatography (HPLC) analysis showed that the mentioned dose of eugenol is detectable in the corneal tissue; hence, this compound could penetrate through corneal epithelium that is an important obstacle for drugs penetration into the cornea. In addition, the clinical markers and HE showed significant lower levels of corneal neovascularization and clouding and conjunctival hyperemia in most rabbits treated with eugenol than the control group. Therefore, because most antifungal drugs are not applicable for the treatment of keratitis and have various side effects, the authors proposed eugenol as a safe and inexpensive antifungal agent that can be used topically for the treatment of keratitis caused by C. albicans (Hassan et al., 2018).
Therefore, as mentioned in the above studies, eugenol could be considered a potential antifungal agent with promising efficacy for treating different candidiasis presentations such as vaginitis, keratitis, and oral candidiasis. However, further molecular and toxicologic studies and clinical trials are required to determine the exact interaction of eugenol with eukaryote cells after prolonged exposure. Because non-albicans Candida species are becoming increasingly common, the antifungal effects of eugenol against these fungi should also be evaluated in future animal studies.
Studies regarding genotoxicity and cytotoxicity of eugenol are very controversial and limited. A dose of 2.5 mg/kg body weight of eugenol is regarded as safe by the Food and Agriculture Organization, while high concentrations of this compound could be harmful and pro-oxidative (Mohammadi Nejad et al., 2017; Ulanowska and Olas, 2021). For instance, 5–10 ml ingestion of clove oil led to acidosis and deep coma in a 2-year old boy (Hartnoll et al., 1993). Moreover, eugenol intravenous infusion (at 4 and 8 µl) caused acute respiratory distress with hemorrhagic pulmonary edema in rats (Wright et al., 1995). Also, eugenol could lead to allergies such as allergic contact dermatitis in some cases, particularly in dental workers (Mohammadi Nejad et al., 2017; Ulanowska and Olas, 2021). In this regard, research on eugenol and clove oil is still ongoing and extensive toxicity evaluations should be conducted to approve whether eugenol is safe for general public health.
Drug delivery systems
Previous studies reported that eugenol is sensitive to degradation processes when exposed to oxygen, light, high temperatures, and humidity. Furthermore, this compound is highly volatile, slightly soluble in water, and unstable; as a result, the disadvantages listed above may reduce eugenol’s efficacy, biological activity, and stability (Karmakar et al., 2012; Shao et al., 2018). Therefore, considering the disadvantages of eugenol concerning solubility, instability, and volatilization, the use of different drug-delivery systems could be an alternative to solve these challenges and provide a stable, safe, and effective antifungal agent for clinical usage.
In this regard, Jacumazo et al. (2020) used nanocapsules and microcapsules containing eugenol coated with chitosan and carboxymethylcellulose to manage eugenol’s release enabling its use as an antifungal agent. This drug-delivery system indicated an inhibitory effect against C. albicans, and the MIC of eugenol was lower than those of the free agent. Thus, the authors suggested that nanocapsules with successive polysaccharide layers protect eugenol and improve its release (Jacumazo et al., 2020). Another investigation also reported that eugenol loaded with water-soluble βCD-grafted chitosan derivatives (QCD-g-CS) showed higher antimicrobial activities against C. albicans in comparison to the free compound. This enhanced antimicrobial activity could be attributed to the increased solubility of eugenol in the aqueous phase caused by the presence of QCD-g-CS, resulting in modified eugenol-microorganism interactions. Furthermore, both eugenol and chitosan have antimicrobial properties. Accordingly, the presence of both of them as inclusion complexes may lead to the synergistic effect on antimicrobial activity (Sajomsang et al., 2012).
Additionally, eugenol-loaded electrospun Polyacrylonitrile (PAN) nanofiber mats’ antifungal effects were evaluated against C. albicans. The findings demonstrated that eugenol release from nanofibers was gradual and continued slowly for 150 h. The PAN nanofiber lacked antifungal activity as a control sample, but these samples exhibited antifungal properties when loaded with eugenol. Hence, these data suggested that PAN nanofibrous mats containing eugenol could be considered an alternative therapeutic agent for localized drug delivery of eugenol due to its acceptable tensile properties, suitable drug release, uniform morphology, and antifungal properties (Semnani et al., 2018). Labib et al. (2015) also introduced another drug-delivery platform for treating local candidiasis in line with these results. The authors used Orabase, bioadhesive bases that have been used for pain relief in the handling of oral aphthous stomatitis, loaded with eugenol. This compound indicated significant antifungal function, acceptable physical features, slow-release pattern, and sensible mucoadhesion. Therefore, the incorporation of eugenol in Orabase, which has demonstrated efficacy in inhibiting local treatment of oral candidiasis, may aid in the design of future drug delivery systems (Labib and Aldawsari, 2015).
As mentioned in the above studies, diverse drug-delivery platforms with eugenol could provide novel agents to inhibit Candida-associated infection. Nonetheless, the precise mechanisms of these platforms interacting with candidal physiology remain unknown. Thus, additional research, including molecular evaluations, in vivo toxicity analysis, and clinical trials, are required before the widespread use of eugenol-drug delivery systems.
Finally, it is noteworthy that recent studies used eugenol-based derivatives to inhibit different cellular pathways in Candida. These studies have been reported in full in Table 2.
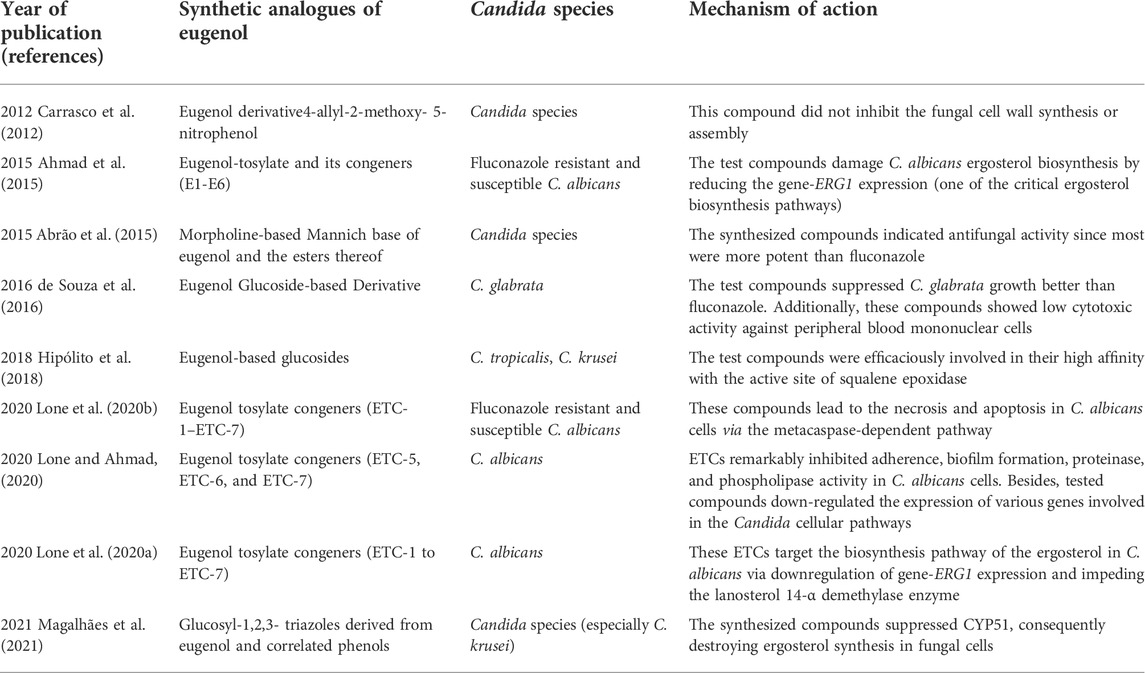
TABLE 2. Studies reporting synthetic analogues of eugenol to inhibit growth and Candida cells’ cellular pathways.
Conclusion
Eugenol may be beneficial in the clinical management of candidiasis, particularly localized forms such as vulvovaginal and oral candidiasis, due to its fungicidal activity and inhibitory effect on germ tube formation. Due to the potent anti-biofilm capacity of eugenol against Candida, covering medical implant devices with this compound could be practical in preventing implant-associated Candida infections. The combined use of eugenol with various antifungals, especially fluconazole, could also be very helpful in combating infections caused by drug-resistant Candida. Nevertheless, although some studies have reported the molecular interactions of eugenol with the different Candida cellular pathways, further research is required to substantiate these findings. Additionally, more in vitro, animal models, and clinical trials should be designed for the exact evaluation of cell cytotoxicity due to long-term exposure to eugenol. Finally, it is proposed that various drug delivery platforms containing eugenol and other eugenol derivatives should be evaluated to develop effective and novel antifungal agents against Candida species.
Author contributions
AS and MD conceived and designed the study. AS and ZC contributed in comprehensive research. ZC, AS, and MD wrote the paper. Notably, all authors have read and approved the manuscript.
Conflict of interest
The authors declare that the research was conducted in the absence of any commercial or financial relationships that could be construed as a potential conflict of interest.
Publisher’s note
All claims expressed in this article are solely those of the authors and do not necessarily represent those of their affiliated organizations, or those of the publisher, the editors and the reviewers. Any product that may be evaluated in this article, or claim that may be made by its manufacturer, is not guaranteed or endorsed by the publisher.
Abbreviations
AMB, amphotericin B; CFU, colony-forming units; EO, essential oil; EPS, Exopolysaccharides; GC/MS, Gas Chromatography/Mass Spectrometry; HE, Histopathologic examination; HPLC, High-performance liquid chromatography; LPO, lipid peroxidation; MIC, minimum inhibitory concentration; PAN, Polyacrylonitrile; ROS, reactive oxygen species; SEM, Scanning electron microscopy.
References
Abirami, G., Alexpandi, R., Durgadevi, R., Kannappan, A., and Veera Ravi, A. (2020). Inhibitory effect of morin against Candida albicans pathogenicity and virulence factor production: An in vitro and in vivo approaches. Front. Microbiol. 11, 561298. doi:10.3389/fmicb.2020.561298
Abrahão, M. R., Molina, G., and Pastore, G. M. (2013). Endophytes: Recent developments in biotechnology and the potential for flavor production. Food Res. Int. 52 (1), 367–372. doi:10.1016/j.foodres.2013.03.007
Abrão, P. H. O., Pizi, R. B., De Souza, T. B., Silva, N. C., Fregnan, A. M., Silva, F. N., et al. (2015). Synthesis and biological evaluation of new eugenol mannich bases as promising antifungal agents. Chem. Biol. Drug Des. 86 (4), 459–465. doi:10.1111/cbdd.12504
Ahmad, A., Khan, A., Khan, L. A., and Manzoor, N. (2010a). In vitro synergy of eugenol and methyleugenol with fluconazole against clinical Candida isolates. J. Med. Microbiol. 59 (10), 1178–1184. doi:10.1099/jmm.0.020693-0
Ahmad, A., Khan, A., Manzoor, N., and Khan, L. A. (2010b). Evolution of ergosterol biosynthesis inhibitors as fungicidal against Candida. Microb. Pathog. 48 (1), 35–41. doi:10.1016/j.micpath.2009.10.001
Ahmad, A., Khan, A., Yousuf, S., Khan, L. A., and Manzoor, N. (2010c). Proton translocating ATPase mediated fungicidal activity of eugenol and thymol. Fitoterapia 81 (8), 1157–1162. doi:10.1016/j.fitote.2010.07.020
Ahmad, A., Wani, M. Y., Khan, A., Manzoor, N., and Molepo, J. (2015). Synergistic interactions of eugenol-tosylate and its congeners with fluconazole against Candida albicans. Plos one 10 (12), e0145053. doi:10.1371/journal.pone.0145053
Amirrajab, N., Badali, H., Didehdar, M., Afsarian, M. H., Mohammadi, R., Lotfi, N., et al. (2016). In vitro activities of six antifungal drugs against Candida glabrata isolates: An emerging pathogen. Jundishapur J. Microbiol. 9 (5), e36638. doi:10.5812/jjm.36638
Arendrup, M. C., and Patterson, T. F. (2017). Multidrug-resistant Candida: Epidemiology, molecular mechanisms, and treatment. J. Infect. Dis. 216 (3), S445–S451. doi:10.1093/infdis/jix131
Boonchird, C., and Flegel, T. (1982). In vitro antifungal activity of eugenol and vanillin against Candida albicans and Cryptococcus neoformans. Can. J. Microbiol. 28 (11), 1235–1241. doi:10.1139/m82-184
Braga, P., Dal Sasso, M., and Culic, Cryptococcus neoformans i, M., and Alfieri, M. (2007). Eugenol and thymol, alone or in combination, induce morphological alterations in the envelope of Candida albicans. Fitoterapia 78 (6), 396–400. doi:10.1016/j.fitote.2007.02.022
Carrasco, H., Raimondi, M., Svetaz, L., Liberto, M. D., Rodriguez, M. V., Espinoza, L., et al. (2012). Antifungal activity of eugenol analogues. Influence of different substituents and studies on mechanism of action. Molecules 17 (1), 1002–1024. doi:10.3390/molecules17011002
Cecchini, C., Silvi, S., Cresci, A., Piciotti, A., Caprioli, G., Papa, F., et al. (2012). Antimicrobial efficacy of Achillea ligustica All.(Asteraceae) essential oils against reference and isolated oral microorganisms. Chem. Biodivers. 9 (1), 12–24. doi:10.1002/cbdv.201100249
Chaieb, K., Zmantar, T., Ksouri, R., Hajlaoui, H., Mahdouani, K., Abdelly, C., et al. (2007). Antioxidant properties of the essential oil of Eugenia caryophyllata and its antifungal activity against a large number of clinical Candida species. Mycoses 50 (5), 403–406. doi:10.1111/j.1439-0507.2007.01391.x
Chami, F., Chami, N., Bennis, S., Trouillas, J., and Remmal, A. (2004a). Evaluation of carvacrol and eugenol as prophylaxis and treatment of vaginal candidiasis in an immunosuppressed rat model. J. Antimicrob. Chemother. 54 (5), 909–914. doi:10.1093/jac/dkh436
Chami, N., Bennis, S., Chami, F., Aboussekhra, A., and Remmal, A. (2005). Study of anticandidal activity of carvacrol and eugenol in vitro and in vivo. Oral Microbiol. Immunol. 20 (2), 106–111. doi:10.1111/j.1399-302X.2004.00202.x
Chami, N., Chami, F., Bennis, S., Trouillas, J., and Remmal, A. (2004b). Antifungal treatment with carvacrol and eugenol of oral candidiasis in immunosuppressed rats. Braz. J. Infect. Dis. 8 (3), 217–226. doi:10.1590/s1413-86702004000300005
Chowdhary, A., Sharma, C., and Meis, J. F. (2017). Candida auris: A rapidly emerging cause of hospital-acquired multidrug-resistant fungal infections globally. PLoS Pathog. 13 (5), e1006290. doi:10.1371/journal.ppat.1006290
Cirasola, D., Sciota, R., Vizzini, L., Ricucci, V., Morace, G., Borghi, E., et al. (2013). Experimental biofilm-related Candida infections. Future Microbiol. 8 (6), 799–805. doi:10.2217/fmb.13.36
Conti, H. R., Huppler, A. R., Whibley, N., and Gaffen, S. L. (2014). Animal models for candidiasis. Curr. Protoc. Immunol. 105, 1. 16.11-19.16.17. doi:10.1002/0471142735.im1906s105
Dąbrowska, M., Zielińska‐Bliźniewska, H., Kwiatkowski, P., Łopusiewicz, Ł., Pruss, A., Kostek, M., et al. (2021). Inhibitory effect of eugenol and trans‐anethole alone and in combination with antifungal medicines on Candida albicans clinical isolates. Chem. Biodivers. 18 (5), e2000843. doi:10.1002/cbdv.202000843
De Paula, S. B., Bartelli, T. F., Di Raimo, V., Santos, J. P., Morey, A. T., Bosini, M. A., et al. (2014). Effect of eugenol on cell surface hydrophobicity, adhesion, and biofilm of Candida tropicalis and Candida dubliniensis isolated from oral cavity of HIV-infected patients. Evid. Based. Complement. Altern. Med. 2014 (5), 505204. doi:10.1155/2014/505204
De Souza, T. B., De Oliveira Brito, K. M., Silva, N. C., Rocha, R. P., De Sousa, G. F., Duarte, L. P., et al. (2016). New eugenol glucoside‐based derivative shows fungistatic and fungicidal activity against opportunistic Candida glabrata. Chem. Biol. Drug Des. 87 (1), 83–90. doi:10.1111/cbdd.12625
Doke, S. K., Raut, J. S., Dhawale, S., and Karuppayil, S. M. (2014). Sensitization of Candida albicans biofilms to fluconazole by terpenoids of plant origin. J. Gen. Appl. Microbiol. 60 (5), 163–168. doi:10.2323/jgam.60.163
El-Baz, A. M., Mosbah, R. A., Goda, R. M., Mansour, B., Sultana, T., Dahms, T. E., et al. (2021). Back to nature: Combating Candida albicans biofilm, phospholipase and hemolysin using plant essential oils. Antibiotics 10 (1), 81. doi:10.3390/antibiotics10010081
Fabri, R. L., Coimbra, E. S., Almeida, A. C., Siqueira, E. P., Alves, T., Zani, C. L., et al. (2012). Essential oil of Mitracarpus frigidus as a potent source of bioactive compounds. An. Acad. Bras. Cienc. 84 (4), 1073–1080. doi:10.1590/S0001-37652012000400021
Fisher, J. F., Sobel, J. D., Kauffman, C. A., and Newman, C. A. (2011). Candida urinary tract infections—treatment. Clin. Infect. Dis. 52 (6), S457–S466. doi:10.1093/cid/cir112
Fontenelle, R. O., Morais, S. M., Brito, E. H., Brilhante, R. S., Cordeiro, R. A., Lima, Y. C., et al. (2011). Alkylphenol activity against Candida spp. and microsporum canis: A focus on the antifungal activity of thymol, eugenol and O-methyl derivatives. Molecules 16 (8), 6422–6431. doi:10.3390/molecules16086422
Gupta, P., Gupta, S., Sharma, M., Kumar, N., Pruthi, V., Poluri, K. M., et al. (2018). Effectiveness of phytoactive molecules on transcriptional expression, biofilm matrix, and cell wall components of Candida glabrata and its clinical isolates. ACS omega 3 (9), 12201–12214. doi:10.1021/acsomega.8b01856
Hartnoll, G., Moore, D., and Douek, D. (1993). Near fatal ingestion of oil of cloves. Arch. Dis. Child. 69 (3), 392–393. doi:10.1136/adc.69.3.392
Hassan, H. A., Geniady, M. M., Abdelwahab, S. F., Abd-Elghany, M. I., Sarhan, H. A., Abdelghany, A. A., et al. (2018). Topical eugenol successfully treats experimental Candida albicans-induced keratitis. Ophthalmic Res. 60 (2), 69–79. doi:10.1159/000488907
He, M., Du, M., Fan, M., and Bian, Z. (2007). In vitro activity of eugenol against Candida albicans biofilms. Mycopathologia 163 (3), 137–143. doi:10.1007/s11046-007-0097-2
Hipólito, T. M. M., Bastos, G. T. L., Barbosa, T. W. L., De Souza, T. B., Coelho, L. F. L., Dias, A. L. T., et al. (2018). Synthesis, activity, and docking studies of eugenol‐based glucosides as new agents against Candida sp. Chem. Biol. Drug Des. 92 (2), 1514–1524. doi:10.1111/cbdd.13318
Hoyer, L. L., and Cota, E. (2016). Candida albicans agglutinin-like sequence (als) family vignettes: A review of als protein structure and function. Front. Microbiol. 7, 280. doi:10.3389/fmicb.2016.00280
Jacumazo, J., De Carvalho, M. M., Parchen, G. P., Campos, I. M., Garcia, M. J. B., Brugnari, T., et al. (2020). Development, characterization and antimicrobial activity of sodium dodecyl sulfate-polysaccharides capsules containing eugenol. Carbohydr. Polym. 230, 115562. doi:10.1016/j.carbpol.2019.115562
Jafri, H., Banerjee, G., Khan, M. S. A., Ahmad, I., Abulreesh, H. H., Althubiani, A. S., et al. (2020). Synergistic interaction of eugenol and antimicrobial drugs in eradication of single and mixed biofilms of Candida albicans and Streptococcus mutans. Amb. Express 10 (1), 185. doi:10.1186/s13568-020-01123-2
Jafri, H., Khan, M. S. A., and Ahmad, I. (2019). In vitro efficacy of eugenol in inhibiting single and mixed-biofilms of drug-resistant strains of Candida albicans and Streptococcus mutans. Phytomedicine 54, 206–213. doi:10.1016/j.phymed.2018.10.005
Kamatou, G. P., Vermaak, I., and Viljoen, A. M. (2012). Eugenol--from the remote maluku islands to the international market place: A review of a remarkable and versatile molecule. Molecules 17 (6), 6953–6981. doi:10.3390/molecules17066953
Karmakar, S., Choudhury, M., Das, A. S., Maiti, A., Majumdar, S., Mitra, C., et al. (2012). Clove (Syzygium aromaticum Linn) extract rich in eugenol and eugenol derivatives shows bone-preserving efficacy. Nat. Prod. Res. 26 (6), 500–509. doi:10.1080/14786419.2010.511216
Kauffman, C. A., and Carver, P. L. (1997). Antifungal agents in the 1990s. Current status and future developments. Drugs 53 (4), 539–549. doi:10.2165/00003495-199753040-00001
Kerekes, E. B., Deák, É., Takó, M., Tserennadmid, R., Petkovits, T., Vágvölgyi, C., et al. (2013). Anti‐biofilm forming and anti‐quorum sensing activity of selected essential oils and their main components on food‐related micro‐organisms. J. Appl. Microbiol. 115 (4), 933–942. doi:10.1111/jam.12289
Khan, A., Ahmad, A., Akhtar, F., Yousuf, S., Xess, I., Khan, L. A., et al. (2011). Induction of oxidative stress as a possible mechanism of the antifungal action of three phenylpropanoids. FEMS Yeast Res. 11 (1), 114–122. doi:10.1111/j.1567-1364.2010.00697.x
Khan, M. S. A., and Ahmad, I. (2012). Antibiofilm activity of certain phytocompounds and their synergy with fluconazole against Candida albicans biofilms. J. Antimicrob. Chemother. 67 (3), 618–621. doi:10.1093/jac/dkr512
Khan, M. S. A., Malik, A., and Ahmad, I. (2012). Anti-candidal activity of essential oils alone and in combination with amphotericin B or fluconazole against multi-drug resistant isolates of Candida albicans. Med. Mycol. 50 (1), 33–42. doi:10.3109/13693786.2011.582890
Khan, S. N., Khan, S., Misba, L., Sharief, M., Hashmi, A., Khan, A. U., et al. (2019). Synergistic fungicidal activity with low doses of eugenol and amphotericin B against Candida albicans. Biochem. Biophys. Res. Commun. 518 (3), 459–464. doi:10.1016/j.bbrc.2019.08.053
Kozam, G. (1977). The effect of eugenol on nerve transmission. Oral Surg. Oral Med. Oral Pathol. 44 (5), 799–805. doi:10.1016/0030-4220(77)90390-5
Kullberg, B. J., and Arendrup, M. C. (2015). Invasive candidiasis. N. Engl. J. Med. 373 (15), 1445–1456. doi:10.1056/NEJMra1315399
Labib, G. S., and Aldawsari, H. (2015). Innovation of natural essential oil-loaded Orabase for local treatment of oral candidiasis. Drug Des. devel. Ther. 9, 3349–3359. doi:10.2147/DDDT.S85356
Lohse, M. B., Gulati, M., Johnson, A. D., and Nobile, C. J. (2018). Development and regulation of single- and multi-species Candida albicans biofilms. Nat. Rev. Microbiol. 16 (1), 19–31. doi:10.1038/nrmicro.2017.107
Lone, S. A., and Ahmad, A. (2020). Inhibitory effect of novel Eugenol Tosylate Congeners on pathogenicity of Candida albicans. BMC Complement. Med. Ther. 20 (1), 131. doi:10.1186/s12906-020-02929-0
Lone, S. A., Khan, S., and Ahmad, A. (2020a). Inhibition of ergosterol synthesis in Candida albicans by novel eugenol tosylate congeners targeting sterol 14α-demethylase (CYP51) enzyme. Arch. Microbiol. 202 (4), 711–726. doi:10.1007/s00203-019-01781-2
Lone, S. A., Wani, M. Y., Fru, P., and Ahmad, A. (2020b). Cellular apoptosis and necrosis as therapeutic targets for novel eugenol tosylate congeners against Candida albicans. Sci. Rep. 10 (1), 1191. doi:10.1038/s41598-020-58256-4
Lopes-Lutz, D., Alviano, D. S., Alviano, C. S., and Kolodziejczyk, P. P. (2008). Screening of chemical composition, antimicrobial and antioxidant activities of Artemisia essential oils. Phytochemistry 69 (8), 1732–1738. doi:10.1016/j.phytochem.2008.02.014
López-Ribot, J. L. (2005). Candida albicans biofilms: More than filamentation. Curr. Biol. 15 (12), R453–R455. doi:10.1016/j.cub.2005.06.020
Magalhães, L. S. D., Reis, A. C. C., Nakao, I. A., Péret, V. a. C., Reis, R. C. F. M., Silva, N. C., et al. (2021). Glucosyl‐1, 2, 3‐triazoles derived from eugenol and analogues: Synthesis, anti‐Candida activity, and molecular modeling studies in CYP‐51. Chem. Biol. Drug Des. 98 (5), 903–913. doi:10.1111/cbdd.13948
Marak, M. B., and Dhanashree, B. (2018). Antifungal susceptibility and biofilm production of Candida spp. isolated from clinical samples. Int. J. Microbiol. 2018, 7495218. doi:10.1155/2018/7495218
Marcos-Arias, C., Eraso, E., Madariaga, L., and Quindós, G. (2011). In vitro activities of natural products against oral Candida isolates from denture wearers. BMC Complement. Altern. Med. 11 (1), 119. doi:10.1186/1472-6882-11-119
Matasyoh, L. G., Matasyoh, J. C., Wachira, F. N., Kinyua, M. G., Muigai, A. W. T., Mukiama, T. K., et al. (2008). Antimicrobial activity of essential oils of Ocimum gratissimum L. from different populations of Kenya. Afr. J. Tradit. Complement. Altern. Med. 5 (2), 187–193. doi:10.4314/ajtcam.v5i2.31272
Mayer, F. L., Wilson, D., and Hube, B. (2013). Candida albicans pathogenicity mechanisms. Virulence 4 (2), 119–128. doi:10.4161/viru.22913
Mohammadi Nejad, S., Özgüneş, H., and Başaran, N. (2017). Pharmacological and toxicological properties of eugenol. Turk. J. Pharm. Sci. 14 (2), 201–206. doi:10.4274/tjps.62207
Nisar, M. F., Khadim, M., Rafiq, M., Chen, J., Yang, Y., Wan, C. C., et al. (2021). Pharmacological properties and health benefits of eugenol: A comprehensive review. Oxid. Med. Cell. Longev. 2021, 2497354. doi:10.1155/2021/2497354
Pappas, P. G., Lionakis, M. S., Arendrup, M. C., Ostrosky-Zeichner, L., and Kullberg, B. J. (2018). Invasive candidiasis. Nat. Rev. Dis. Prim. 4, 18026. doi:10.1038/nrdp.2018.26
Pinto, E., Ribeiro, I. C., Ferreira, N. J., Fortes, C. E., Fonseca, P. A., Figueiral, M. H., et al. (2008). Correlation between enzyme production, germ tube formation and susceptibility to fluconazole in Candida species isolated from patients with denture‐related stomatitis and control individuals. J. Oral Pathol. Med. 37 (10), 587–592. doi:10.1111/j.1600-0714.2008.00687.x
Pinto, E., Vale-Silva, L., Cavaleiro, C., and Salgueiro, L. (2009). Antifungal activity of the clove essential oil from Syzygium aromaticum on Candida, Aspergillus and dermatophyte species. J. Med. Microbiol. 58 (11), 1454–1462. doi:10.1099/jmm.0.010538-0
Pristov, K., and Ghannoum, M. (2019). Resistance of Candida to azoles and echinocandins worldwide. Clin. Microbiol. Infect. 25 (7), 792–798. doi:10.1016/j.cmi.2019.03.028
Rangel, M. D. L., Aquino, S. G. D., Lima, J. M. D., Castellano, L. R., and Castro, R. D. D. (2018). In VitroEffect ofCinnamomum zeylanicumBlume essential oil onCandidaspp. involved in oral infections. Evidence-Based Complementary Altern. Med. 2018, 1–13. doi:10.1155/2018/4045013
Reddy, P., and Lokesh, B. R. (1994). Studies on anti-inflammatory activity of spice principles and dietary n-3 polyunsaturated fatty acids on carrageenan-induced inflammation in rats. Ann. Nutr. Metab. 38 (6), 349–358. doi:10.1159/000177833
Sajomsang, W., Nuchuchua, O., Gonil, P., Saesoo, S., Sramala, I., Soottitantawat, A., et al. (2012). Water-soluble β-cyclodextrin grafted with chitosan and its inclusion complex as a mucoadhesive eugenol carrier. Carbohydr. Polym. 89 (2), 623–631. doi:10.1016/j.carbpol.2012.03.060
Sakagami, T., Kawano, T., Yamashita, K., Yamada, E., Fujino, N., Kaeriyama, M., et al. (2019). Antifungal susceptibility trend and analysis of resistance mechanism for Candida species isolated from bloodstream at a Japanese University hospital. J. Infect. Chemother. 25 (1), 34–40. doi:10.1016/j.jiac.2018.10.007
Semnani, K., Shams-Ghahfarokhi, M., Afrashi, M., Fakhrali, A., and Semnani, D. (2018). Antifungal activity of eugenol loaded electrospun PAN nanofiber mats against Candida albicans. Curr. Drug Deliv. 15 (6), 860–866. doi:10.2174/1567201815666180226120436
Shao, Y., Wu, C., Wu, T., Li, Y., Chen, S., Yuan, C., et al. (2018). Eugenol-chitosan nanoemulsions by ultrasound-mediated emulsification: Formulation, characterization and antimicrobial activity. Carbohydr. Polym. 193, 144–152. doi:10.1016/j.carbpol.2018.03.101
Shariati, A., Moradabadi, A., Chegini, Z., Khoshbayan, A., and Didehdar, M. (2020). An overview of the management of the most important invasive fungal infections in patients with blood malignancies. Infect. Drug Resist. 13, 2329–2354. doi:10.2147/Idr.S254478
Sharifzadeh, A., and Shokri, H. (2021). In vitro synergy of eugenol on the antifungal effects of voriconazole against Candida tropicalis and Candida krusei strains isolated from the genital tract of mares. Equine Vet. J. 53 (1), 94–101. doi:10.1111/evj.13268
Solis, N. V., and Filler, S. G. (2012). Mouse model of oropharyngeal candidiasis. Nat. Protoc. 7 (4), 637–642. doi:10.1038/nprot.2012.011
Spampinato, C., and Leonardi, D. (2013). Candida infections, causes, targets, and resistance mechanisms: Traditional and alternative antifungal agents. Biomed. Res. Int. 2013, 204237. doi:10.1155/2013/204237
Tacchini, M., Echeverria Guevara, M. P., Grandini, A., Maresca, I., Radice, M., Angiolella, L., et al. (2021). Ocimum campechianum mill. from Amazonian Ecuador: Chemical Composition and biological activities of extracts and their main constituents (eugenol and rosmarinic acid). Molecules 26 (1), 84. doi:10.3390/molecules26010084
Thosar, N., Basak, S., Bahadure, R. N., and Rajurkar, M. (2013). Antimicrobial efficacy of five essential oils against oral pathogens: An in vitro study. Eur. J. Dent. 7 (S 01), S071–S077. doi:10.4103/1305-7456.119078
Ulanowska, M., and Olas, B. (2021). Biological properties and prospects for the application of eugenol-A review. Int. J. Mol. Sci. 22 (7), 3671. doi:10.3390/ijms22073671
Wijesinghe, G. K., De Oliveira, T. R., Maia, F. C., De Feiria, S. B., Barbosa, J. P., Joia, F., et al. (2021). Efficacy of true cinnamon (Cinnamomum verum) leaf essential oil as a therapeutic alternative for Candida biofilm infections. Iran. J. Basic Med. Sci. 24 (6), 787–795. doi:10.22038/ijbms.2021.53981.12138
Wijesinghe, G. K., Maia, F. C., De Oliveira, T. R., De Feiria, S. N. B., Joia, F., Barbosa, J. P., et al. (2020). Effect of Cinnamomum verum leaf essential oil on virulence factors of Candida species and determination of the in-vivo toxicity with Galleria mellonella model. Mem. Inst. Oswaldo Cruz 115, e200349. doi:10.1590/0074-02760200349
Williams, D., Silva, S. C., Malic, S., Kuriyama, T., and Lewis, M. A. (2012). Candida biofilms and oral candidosis: Treatment and prevention. Periodontol. 2000 55 (1), 250–265. doi:10.1111/j.1600-0757.2009.00338.x
Wisplinghoff, H., Bischoff, T., Tallent, S. M., Seifert, H., Wenzel, R. P., Edmond, M. B., et al. (2004). Nosocomial bloodstream infections in US hospitals: Analysis of 24, 179 cases from a prospective nationwide surveillance study. Clin. Infect. Dis. 39 (3), 309–317. doi:10.1086/421946
Wright, S. E., Baron, D. A., and Heffner, J. E. (1995). Intravenous eugenol causes hemorrhagic lung edema in rats: Proposed oxidant mechanisms. J. Lab. Clin. Med. 125 (2), 257–264.
Wu, X.-Z., Cheng, A.-X., Sun, L.-M., Sun, S.-J., and Lou, H.-X. (2009). Plagiochin E, an antifungal bis (bibenzyl), exerts its antifungal activity through mitochondrial dysfunction-induced reactive oxygen species accumulation in Candida albicans. Biochim. Biophys. Acta 1790 (8), 770–777. doi:10.1016/j.bbagen.2009.05.002
Yassin, M. T., Mostafa, A. a.-F., and Al-Askar, A. A. (2020). In vitro anticandidal potency of Syzygium aromaticum (clove) extracts against vaginal candidiasis. BMC Complement. Med. Ther. 20 (1), 25. doi:10.1186/s12906-020-2818-8
Keywords: eugenol, Candida species, new antifungal agent, biofilm, combination therapy
Citation: Didehdar M, Chegini Z and Shariati A (2022) Eugenol: A novel therapeutic agent for the inhibition of Candida species infection. Front. Pharmacol. 13:872127. doi: 10.3389/fphar.2022.872127
Received: 09 February 2022; Accepted: 13 July 2022;
Published: 09 August 2022.
Edited by:
Mustofa Mustofa, Gadjah Mada University, IndonesiaReviewed by:
Letizia Angiolella, Sapienza University of Rome, ItalyMonica Zuzarte, University of Coimbra, Portugal
Copyright © 2022 Didehdar, Chegini and Shariati. This is an open-access article distributed under the terms of the Creative Commons Attribution License (CC BY). The use, distribution or reproduction in other forums is permitted, provided the original author(s) and the copyright owner(s) are credited and that the original publication in this journal is cited, in accordance with accepted academic practice. No use, distribution or reproduction is permitted which does not comply with these terms.
*Correspondence: Aref Shariati, YXJlZnNoYXJpYXRpMDExMUBzYm11LmFjLmly