- 1Laboratorio de Productos Naturales, Departamento de Ecología y Recursos Naturales, Facultad de Ciencias, Universidad Nacional Autónoma de México, Mexico City, Mexico
- 2Flora de Veracruz, Secretaría Académica, Instituto de Ecología, A.C, Xalapa, Mexico
- 3Laboratorio de Neurofarmacología de Productos Naturales, Dirección de Investigaciones en Neurociencias del Instituto Nacional de Psiquiatría Ramón de la Fuente Muñiz, Mexico City, Mexico
- 4Jardin Botánico, Instituto de Biología, Universidad Nacional Autónoma de México, Ciudad Universitaria, Mexico City, Mexico
- 5Departamento de Biología Comparada, Herbario de la Facultad de Ciencias, Facultad de Ciencias, Universidad Nacional Autónoma de México, Mexico City, Mexico
Salvia is the most diverse genus within the mint family (Lamiaceae), many of its species are well-known due to their medicinal and culinary uses. Most of the ethnopharmacological and phytochemical studies on Salvia are centred on species from the European and Asian clades. However, studies about the most diverse clade, the Neotropical sages (Salvia subgenus Calosphace; 587 spp.), are relatively scarce. This review aims to compile the information on the traditional medicinal uses, pharmacological and phytochemistry properties of the Neotropical sages. To do so, we carried out a comprehensive review of the articles available in different online databases published from the past to 2022 (i.e., PubMed, Scopus, and Web of Science, among others) and summarized the information in tables. To uncover phylogenetic patterns in the distribution of four different groups of metabolites (mono-, sesqui-, di-, and triterpenes), we generated presence-absence matrices and plotted the tip states over a dated phylogeny of Salvia. We found several studies involving Mexican species of Salvia, but only a few about taxa from other diversity centres. The main traditional uses of the Mexican species of Calosphace are medicinal and ceremonial. In traditional medicine 56 species are used to treat diseases from 17 categories according to the WHO, plus cultural-bound syndromes. Pharmacological studies reveal a wide range of biological properties (e.g., antinociceptive, anti-inflammatory, anxiolytic, cytotoxic, and antidiabetic, etc.) found in extracts and isolated compounds of 38 Neotropical sages. From extracts of these species, at least 109 compounds have been isolated, identified and evaluated pharmacologically; 73 of these compounds are clerodanes, 21 abietanes, six flavonoids, five sesquiterpenoids, and four triterpenoids. The most characteristic metabolites found in the Neotropical sages are the diterpenes, particularly clerodanes (e.g., Amarisolide A, Tilifodiolide), that are found almost exclusively in this group. The Neotropical sages are a promising resource in the production of herbal medication, but studies that corroborate the properties that have been attributed to them in traditional medicine are scarce. Research of these metabolites guided by the phylogenies is recommended, since closely related species tend to share the presence of similar compounds and thus similar medicinal properties.
1 Introduction
The Lamiaceae family is the sixth most diverse family within the flowering plants, with 241 genera and 7,530 species widely distributed in the world (Christenhusz and Byng, 2016). Many of its species are well-known because of their important uses for human activities (Harley et al., 2004; Mint Evolutionary Genomics Consortium, 2018). They have culinary value, for example Ocimum spp. (Purushothaman et al., 2018); medicinal properties such as Salvia officinalis L. (Ghorbani and Esmaeilizadeh, 2017; Nishino et al., 2021), S. miltiorrhiza Bunge (Su et al., 2015) and Scutellaria baicalensis Georgi (Zhao et al., 2016), and they are used in different industries, such as the cosmetic (e.g., Pogostemon cablin (White) Benth., (Van Beek and Joulain, 2017)), and alimentary industries (e.g., S. hispanica L. (Muñoz et al., 2013; Hernández-Pérez et al., 2020)). Thus, it is of growing interest in the study of the phytochemistry and pharmacological properties of the members of this family.
The Nepetoideae is the most diverse subfamily of Lamiaceae with 33 genera and 3,685 species (Gul et al., 2019). It includes genera of great chemical diversity, particularly of essential oils (Hegnauer, 1989; Lawrence, 1992; Basset, 2000). The most diverse genus of this subfamily and of the entire family is Salvia, which is valued for its medicinal properties, particularly S. officinalis L., a prominent species since ancient times. The name Salvia comes from the Latin “salvare”, which means “to save”, a name given to the genus due to the medicinal properties attributed to it. From an economic point of view, the species of the genus are an important group that is widely known as ornamental, culinary, and medicinal. The species of Salvia are considered valuable due to their antiviral (Tada et al., 1994), anti-Alzheimer (Akhondzadeh et al., 2003), anti-inflammatory (Baricevic et al., 2001), antidiarrheal and antispasmodic (Khan et al., 2011), and antimicrobial (Khalil and Zheng-Guo, 2011) activities, among others. The presence of a wide range of bioactive constituents—terpenoids and phenolic compounds—is considered responsible for their pharmacological activities.
Salvia is a highly diverse genus with ca. 1,000 species (Etminan et al., 2018), that has been considered a natural group for a long time, due to the presence of a peculiar androecium structure, which exhibits two stamens with elongated connectives forming a structure known as a staminal lever (Claßen-Bockhoff et al., 2003; Claßen-Bockhoff et al., 2004; Walker and Sytsma, 2007). This structure, which is involved in the pollination mechanisms of the genus, has been considered a key innovation and synapomorphy for the group (Claßen-Bockhoff et al., 2003; Wester and Claßen-Bockhoff, 2007). However, since the rise of molecular studies, the genus was demonstrated to be a paraphyletic (Walker et al., 2004; Li et al., 2013), thus it was proposed to expand its circumscription to include other five genera that were nested within Salvia: Dorystaechas Boiss and Heldr. Ex Benth., Meriandra Benth., Perovskia Karel., Rosmarinus L., and Zhumeria Rech and Wendelbo (Drew et al., 2017; Hu G. R. et al., 2018). Alternatively, it was proposed to segregate different lineages of Salvia into several genera (Will and Claßen-Bockoff, 2017). However, the expanded circumscription of the genus has been widely accepted due to its practicality.
Currently, Salvia is subdivided into 11 subgenera (Drew et al., 2017; Hu G. R. et al., 2018; Kriebel et al., 2019). Salvia subgenus Calosphace is the most diverse subgenus of Salvia, including about half of the species of the genus (587 spp, González-Gallegos et al., 2020; Figure 1). It is endemic to America (Ramamoorthy and Elliot, 1993; Froissart, 2008; González-Gallegos et al., 2020), and its species are mainly distributed in the Neotropics (Jenks et al., 2013; Fragoso-Martínez et al., 2018). Mexico is the main centre of diversification of Calosphace, harbouring 295 species. Secondary centres are found in the Andean region and the Antilles, and the most outstanding endemism level is found in Mexico (82%), followed by Brazil (72%), Peru (63%), and Colombia (61%) (González-Gallegos et al., 2020). Calosphace is monophyletic and was originally subdivided into several sections by Epling (1939). However, only 12 of these sections have proven to be monophyletic (Jenks et al., 2013; Fragoso-Martínez et al., 2018). Most of the species that have been sampled to date are included in the core Calosphace clade, a group that likely resulted from an evolutionary radiation within the subgenus and that encompasses highly morphological diverse taxa from all the diversity centres of the subgenus (Fragoso-Martínez et al., 2018).
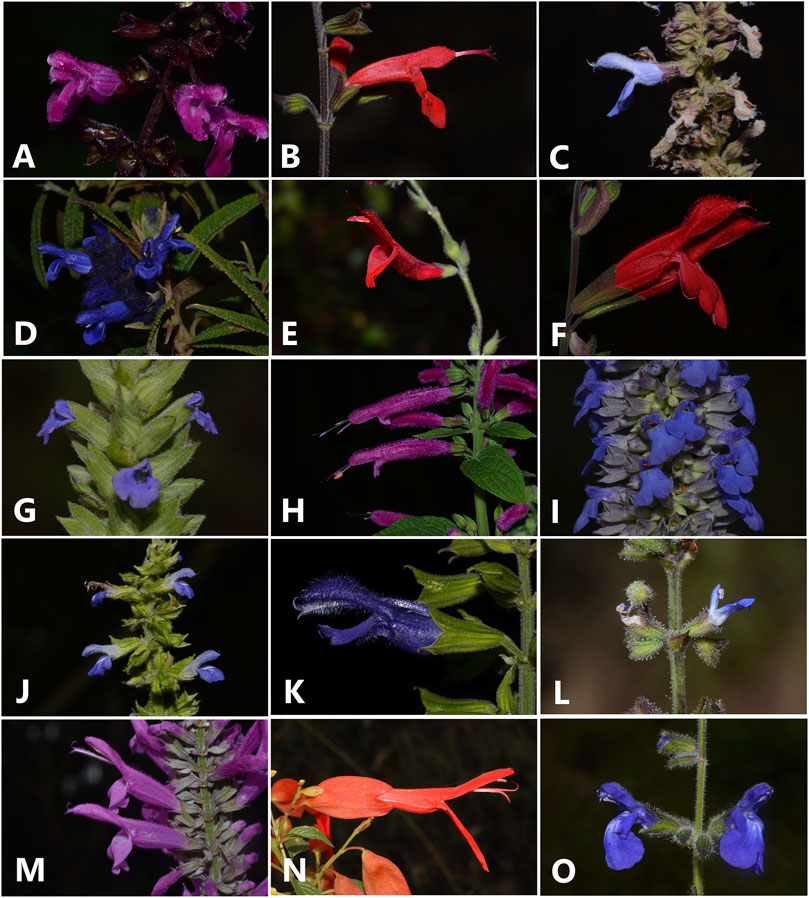
FIGURE 1. Selected species of Neotropical sages (Salvia subgenus Calosphace) that have been studied from phytochemical and/or ethnopharmacological perspectives. (A) Salvia carnea, (B) S. coccinea, (C) S. connivens, (D) S. corrugata, (E) S. elegans, (F) S. gesneriiflora, (G) S. hispanica (chia), (H) S. iodantha, (I) S. lavanduloides, (J) S. longispicata, (K) S. mexicana, (L) S. occidentalis, (M) S. purpurea, (N) S. sessei, (O) S. urica. Photo credits Gerardo Salazar.
The species of Calosphace are generally recognized by the presence of a calyx with an entire or tridentate upper lip, a corolla with the upper lip straight and the lower lip patent, the corolla tube lacking a ring of trichomes and, the androecium with the connective of the two stamens fully fused on the posterior region, and the posterior thecae sterile or absent (Bentham, 1848; Epling, 1939). Some of the Mexican species are well known due to their documented nutritional properties (i.e., S. hispanica L.; Cahill, 2003; Mohd Ali et al., 2012; Ullah et al., 2016) or for their psychoactive properties that are of pharmacological interest due to their antinociceptive effects (i.e., Salvia divinorum Epling and Jativa; Jenks et al., 2011; Tlacomulco-Flores et al., 2020). The most commonly reported bioactive constituents within the subgenus have been characterized as diterpenoids—abietanes and clerodanes—(Bautista et al., 2016; Jiang et al., 2016; Bautista et al., 2017; Esquivel et al., 2017; Campos-Xolalpa et al., 2021).
Despite the high species diversity and economic importance of subgenus Calosphace, the most comprehensive ethnopharmacological and phytochemical studies in Salvia have been conducted on Asian and European species. The aims of this review are the following: 1) to compile the ethnobotanical knowledge of subgenus Calosphace in Mexico, 2) to integrate the pharmacological studies that have been carried out for the species of this subgenus as a promising resource for health resources as antimicrobial, antidiabetic, cytotoxic, anti-inflammatory, and antinociceptive, among other activities, and 3) to summarize the bioactive metabolites characterized within Calosphace, in order to explore if their distribution within the subgenus shows a phylogenetic pattern.
2 Materials and Methods
2.1 Literature Review
The species considered for this review were selected from those correctly identified and reported to belong to subgenus Calosphace according to the checklists of Martínez-Gordillo et al. (2017) and González-Gallegos et al. (2020). Then accordingly, the ethnopharmacology and phytochemistry studies were searched in scientific databases of several platforms and editorials such as Google, Google Scholar, PubMed, Elsevier, Science Direct, Springer, Wiley online library, Taylor and Francis, ACS, and RSC using keywords like Salvia, Calosphace, and more specific terms such as epithets (e.g., Salvia adenophora, S. assurgens, and S. urica, etc.) which were also combined with other words such as pharmacological, ethnobotany, chemistry, terpenoids. Depending on the topic searched, this review includes literature from 1923 to 2022. All the literature found in these systems was classified, systematized, and organized in tables (Supplementary Tables S1, S2). The disease classifications from the ethnobotanical information followed those recommended by the WHO (WHO. ICD-11, 2021). From the information compiled in the tables, data matrices were generated to classify it. To avoid the use of synonyms in chemical nomenclature or the trivial names of molecules we verified the correct names of the chemical compounds using the digital databases of the National Institute of Standards and Technology (NIST) and of the National Center for Biotechnology Information (NCBI).
2.2 Plot of the Differential Distribution of Metabolites in Salvia
To uncover phylogenetic patterns on the metabolite distribution of Salvia subgenus Calosphace, we plotted the presence or absence of the main metabolites found in the subgenus. To do so, we constructed presence-absence matrices that included the 90 species of the Neotropical sages surveyed in this study and 29 species from other Salvia s.l. lineages (Supplementary Table S3). For each species, the presence of the following metabolites was scored: mono-, di-, tri-, and sesquiterpenes. The diterpenes were further classified into abietanes and clerodanes and their presence in the surveyed species was scored too. The dated phylogeny of Salvia s.l. from Kriebel et al. (2019) was used as a phylogenetic framework to plot the metabolite distribution in the Neotropical sages.
We compared and matched the tips of the phylogenetic tree with the species included in the data matrices, the tips belonging to species that lacked data were pruned using the “drop.tip” function in the ape package (Paradis and Schliep, 2019) in R (R Core Team, 2021). The presence-absence data was plotted over a pruned phylogeny containing 102 taxa: 74 species from subgenus Calosphace, and 28 representative species of other clades of Salvia s.l. (i.e., Audibertia, Glutinaria, “Heterosphace”, Rosmarinus, Salvia, Salvia aegyptiaca, and Sclarea). To create the figure that summarizes the states at the tips of the trees of multiple metabolites, we used the function “plotTree.datamatrix” in the phytools package (Revell, 2012) in R.
3 Results
3.1 Traditional Uses of the Neotropical Sages
Salvia is a genus with a long record of traditional use not only in America but also around the world. Among the most widely known and traditionally used species in the Old World are Salvia officinalis, from the Mediterranean, and Salvia miltiorrhiza, from China (Chun-Yan et al., 2015; Ghorbani and Esmaeilizadeh, 2017). In America, the species of subgenus Calosphace have been used since the pre-Columbian era mainly in Mexico, which is their center of diversity. Two Mexican species stand out for their importance for the pre-Hispanic cultures: Salvia hispanica (Figure 1G), known as Chia, whose nutritional, ceremonial, and medicinal properties were recognized long before the arrival of the Spanish and were later described in codexes and the chronicles narrated by the conquerors. The diviner’s sage or S. divinorum is another widely known species with pre-Columbine use. This species was used by the Mazatec in rituals and later became fashionable as a recreational drug throughout the world (Diaz, 2013). Many of the metabolites of the diviner’s sage remain unknown, and therapeutic properties for the treatment of diseases, particularly neurological ones, have begun to be studied (e.g., Simón-Arceo et al., 2017; Tlacomulco-Flores et al., 2020).
From the literature revision, we identified 56 species of Calosphace that have different uses in Mexico. The main use of these species is medicinal, but some of them are also used as substitutes for common household items such as brooms (S. mexicana L., Bello et al., 2015, Figure 1K; S. chamaedryoides Cav., Solano and Blancas, 2018). The flowers of S. cinnabarina M. Martens and Galeotti are used to dye yarns to brown or marron tones (Naranjo, 2012). Salvia hispanica (Chia) has been used as a food source since the pre-Columbian era by the Aztecs (Cahill, 2003). Other species whose fruits are consumed are S. mexicana and S. polystachia Cav. (Bello et al., 2015). Stems and flowers are other organs that are also consumed in certain regions of Mexico (S. fulgens Cav., Cornejo et al., 2008). Despite the beauty and variety of colors of the flowers of the Neotropical sages (Figure 1), only a handful of species are used as ornamentals: S. elegans Cav. (Cornejo et al., 2008, Figure 1E), S. leucantha Cav. (Villavicencio and Pérez, 2002), and S. splendens Sellow ex Wied-Neuw. (Martínez et al., 1995). A less frequent use of these species is cosmetic, for instance, the red flowers of S. coccinea Buc’hoz ex Etl. are used as blush (Jenks and Seung-Chul, 2013, Figure 1B), while infusions of S. lavanduloides Kunth (Figure 1I) and S. polystachia are used to wash the hair, darken it, stimulate growth, and prevent loss (Martínez, 1975; Navarro and Avendaño, 2002). Finally, some species have ceremonial use, like the diviner’s sage for the Mazatec (S. divinorum, Diaz, 2013). In catholic ceremonies S. gesneriflora Lindl. and Paxton (Figure 1F), S. mocinoi Benth., S. purpurea Cav., (Figure 1M) and S. thyrsiflora Benth., are used to decorate religious images and altars (Bello et al., 2015).
Despite the diversity of the subgenus in America, and particularly in Mexico as its main distribution centre, science popularization and scientific information about the properties of the species of Neotropical sages are scarce. There are reports of 56 species of Calosphace with medicinal use; however, only for 48 of these species there is specific information on the ailments they are used for (Supplementary Table S1). These species are used to treat diseases from 17 categories according to the WHO classification (WHO. ICD-11, 2021), and cultural-bound syndromes. The reported species are mainly used to cure diseases of the digestive system (32 spp.), followed by symptoms, signs or clinical findings (24 spp.), pregnancy, childbirth, and puerperium (21 spp.), and culture-bound syndromes (19 spp.; Figure 2A). The species that was used to treat diseases of most of the categories was S. microphylla Kunth (14 categories, Figure 2B), followed by S. coccinea (12), S. lavanduloides, S. elegans, and S. mexicana (9 each).
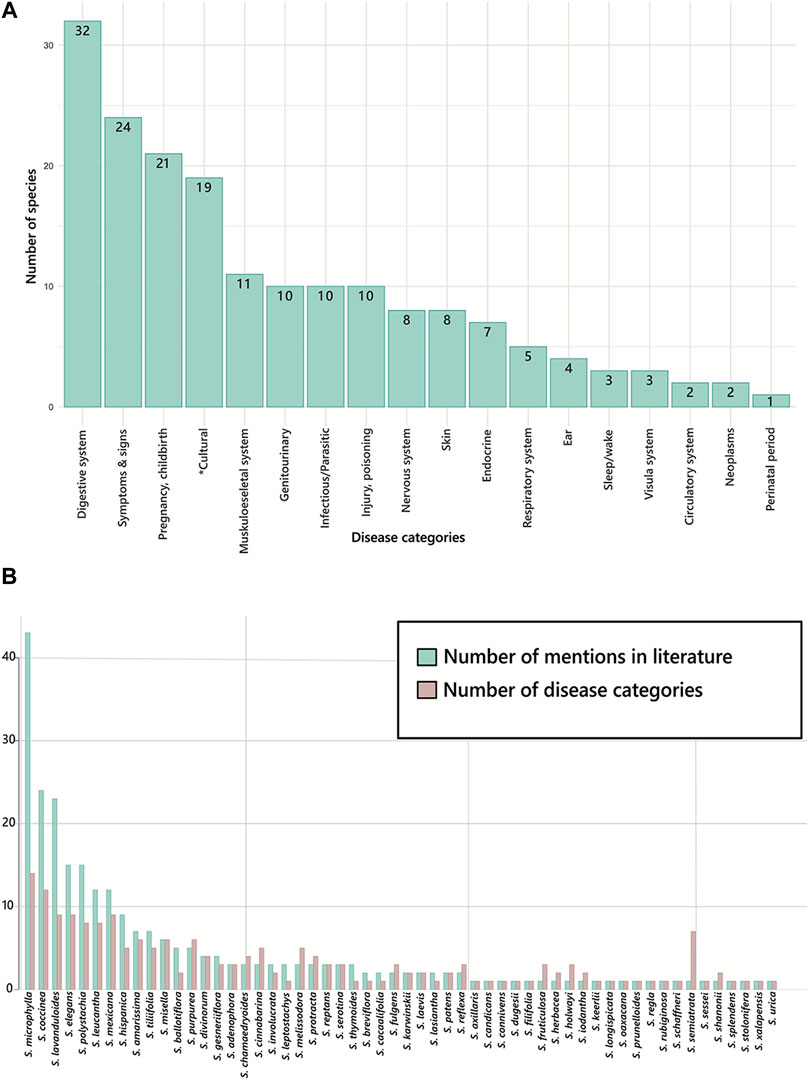
FIGURE 2. Medicinal uses reported for species of Salvia subgenus Calosphace in Mexico. (A) Number of species used to tackle diseases from different categories according to the WHO (WHO. ICD-11, 2021), (B) Number of mentions and disease categories associated with different species of Neotropical sages in Mexican traditional medicine. Abbreviations of the disease categories are as follows: Digestive system (diseases of the digestive system); symptoms and signs (Symptoms, signs or clinical findings, not elsewhere classified); pregnancy, childbirth (Pregnancy, childbirth or the puerperium); *cultural (Cultural-bound syndromes, not included in the WHO classification); musculoskeletal system (Diseases of the musculoskeletal system or connective tissue); infectious/Parasitic (Certain infectious or parasitic diseases); genitourinary (Diseases of the genitourinary system); injury, poisoning (Injury, poisoning or certain other consequences of external causes); nervous system (Diseases of the nervous system); skin (Diseases of the skin); endocrine (Endocrine, nutritional or metabolic diseases); respiratory system (Diseases of the respiratory system); ear (Diseases of the ear or mastoid process); sleep/wake (Sleep-wake disorders); visual system (Diseases of the visual system); neoplasms (Neoplasms); circulatory system (Diseases of the circulatory system); perinatal period (Certain conditions originating in the perinatal period).
3.2 Pharmacological Activities in Salvia Subgenus Calosphace
Different studies reveal that the Neotropical sages exhibit a wide range of pharmacological properties. For instance, S. divinorum and its active compound salvinorin A have been widely evaluated (e.g., Valdés et al., 2001; Bigham et al., 2003; Lee et al., 2005) using experimental models, such as in vitro (lipopolysaccharide-stimulated macrophages, binding affinity opioid receptors, among others), in vivo (antidepressant and anxiolytic-like effects, antinociceptive, and to mention a few), ex vivo (presynaptic modulator, inhibited twitch contractions, and among others) and in the clinic (i.e., elevated blood cortisol levels) (Casselman et al., 2014).
This section describes the main biological activities reported for species of subgenus Calosphace distributed in Mexico (Supplementary Table S2). Table 1 summarizes the biological activities of species that are widely mentioned in Mexican traditional medicine but have been scarcely studied from the pharmacological perspective.
3.2.1 Antibacterial Activity
The large groups of secondary metabolites of plants—mainly phenolics, terpenes, and alkaloids—selectively exert their antibacterial action against different microorganisms through different routes of intervention, such as binding to proteins, for example, adhesins, by enzyme inhibition, substrate deprivation, membrane disruption, metal ion complexation or with cell wall (Cowan, 1999). A few species from Salvia have been investigated for their effective antibacterial activity, using the crude extract or the isolated bioactive compounds to compare with a positive control. Examples found in the literature were S. adenophora Fernald, S. albocaerulea Linden, S. buchananii Hedge, S. chamaedryoides, S. farinacea Benth., S. greggii A. Gray, S. mexicana, S. microphylla, S. reptans Jacq., S. sessei Benth., and S. urica Epling (Pereda-Miranda et al., 1992; Martínez-Vázquez et al., 1998; Kawahara et al., 2004; Romero et al., 2005; Aydoǧmuş et al., 2006; Bisio et al., 2015; Gómez-Rivera et al., 2018; Afonso et al., 2019a). The experiments refer to a specific part of the plant used in traditional medicine, reporting mainly aerial parts or leaves and flowers, and rarely roots (Supplementary Table S2). Antibacterial activity of majority of them has been reported against Staphylococcus aureus and/or S. epidermidis, as well as Escherichia coli and Psedomona aeruginosa. For S. chamaedryoides, several bioactive compounds effective against Enterococcus faecium and E. faecalis were isolated (Bisio et al., 2017). Compounds from S. reptans and S. greggii had antibacterial activity against Bacillus cereus, Micrococus luteus (Martínez-Vázquez et al., 1998), and Bacillus subtilis (Kawahara et al., 2004). Whereas the extracts from different polarities of S. sessei were effective against S. haemolyticus, S. hominis, and E. faecalis (Gómez-Rivera et al., 2018) (Supplementary Table S2).
3.2.2 Anticholinesterase Effect
Anticholinesterase drugs can prolong the presence of released acetylcholine in the synapse producing an increase in the muscarinic and nicotinic receptors activities. Thus, these drugs might have an influence on pharmacological activity in neuromuscular junction; cardiovascular, respiratory, and gastrointestinal systems; secretory glands, and in local application in eyes mediated by a medium duration (reversible) or long-acting (irreversible) activity depending on the anticholinesterase drug. Clinical applications involve Myasthenia gravis (an autoimmune disease), Alzheimer’s disease (dementia associated with deficiency of structural intact cholinergic neurons), paralytic ileus and glaucoma, among others (Nair and Hunter, 2004). In Salvia subgenus Calosphace, the essential oil of S. buchananii has antiacetylcholinesterase and antibutyrylcholinesterase effects (Beladjila et al., 2018a). On the other hand, S. leucantha has been reported with anticholinesterase activity (Villalta et al., 2021), in part due to the presence of the compounds salvileucantholide (IC50 = 50.55 μM) (Table 1), 3β-methoxyisopuberulin (IC50 = 32.20 μM) Dugesin B (IC50 = 22.13 μM) (Jiang et al., 2016), as well as its essential oil (IC50 = 32.60 μg/ml) obtained from aerial parts. (Supplementary Table S2).
3.2.3 Antidepressant and Anxiolytic Effects
Central nervous system (CNS) activity produced by plants is commonly appreciated by people, mainly because of their effects on mood and the belief of them being an efficient and innocuous treatment for diseases. It has been reported greater use of traditional medicine more than 2 fold in individuals affected by a mental disorder (52.9%) compared to people without a mental disorder (22.1%), in depression or anxiety where prevalence is higher in women, being those who use it for self-help more than men. Severity of side effects over the course of treatment with antidepressant or anxiolytic medications is an important factor that causes people to give up pharmacological treatment, mainly those suffering comorbidly, where the time of treatment and response play an important role (Braund et al., 2021). Antidepressant activity is recognized in Salvia species from subgenus Calosphace such as S. elegans (Mora et al., 2006) due to the compound 5-O-(6-rhamnosylglucoside)-7-hydroxy-4′-methoxyflavanone (González-Cortazar et al., 2013) using the forced swimming test. Whereas S. tiliifolia Vahl contains tilifodiolide as bioactive compound responsible for its antidepressant activity evaluated in the tail suspension test (Alba-Betancourt et al., 2019). A relevant compound with several pharmacological activities is Salvinorin A obtained from S. divinorum which not only has antidepressant activity (Calzada et al., 2015), but also anxiolytic-like response was detected in the light-dark test (Herrera-Ruiz et al., 2006). Anxiolytic-like response due to tranquilizing effects has also been reported for S. cinnabarina (Maione et al., 2009), S. elegans (Mora et al., 2006), and S. semiatrata using the elevated plus-maze test. The herbal medication for these two CNS health conditions has not reported severe adverse effects, being recognized as one of the most important advantages of their use (Posadzki et al., 2013). In fact, one of the most common secondary effects in depressant activity of medicinal plants could be the sedative response, which is also useful in CNS diseases such as in the sleep architecture alterations. Salvia cinnabarina might exemplify this property by enhancing the sodium pentobarbital hypnotic effects (Maione et al., 2009) (Supplementary Table S2).
3.2.4 Antifungal and Insecticidal Activities
The most common types of antifungal drugs are systemically administered and target different parts of the fungal cell. An example of this kind of antifungal compound is the heptaene amphotericin B that interacts with the major component of the fungal cell membrane called ergosterol (ergosta-5,7,22-trien-3β-ol), modifying the permeability and fluidity of membrane. The most used antifungal families of drugs azoles (miconazol itraconazole, and clotrimazole) or alilamines (terbinafine) also inhibit the ergosterol synthesis. On the other hand, the most selective drugs for fungal cells are the echinocandins, which inhibit the synthesis of the fungal cell wall. In mammalian cells these drugs have reduced adverse effects, compared to those that have been commonly observed with the 5-Flucytosine (5-FC) (e.g., hepatotoxicity, nephrotoxicity, myelotoxicity, and gastrointestinal problems), that interacts with the nucleus of the fungus, altering the biosynthesis of deoxyribonucleic acid (DNA) alone or combined with cytarabine (Houšť et al., 2020). Medicinal preparations of S. hispanica have been reported to produce not only antifungal effects such as in the case of its essential oil (Elshafie et al., 2018), but also insecticidal properties (Chen et al., 2021). These preparations have been tested against Spodoptera frugiperda, an important and well-known pest in the agricultural field that attacks various crops of economic importance, such as corn and cotton (León-García et al., 2012) (Supplementary Table S2). Regarding insecticidal substances, these have been classified depending on their chemical structure, toxicological effects, and or the mechanism of penetration. For this pharmacological activity, several organic compounds occurring naturally in plants are useful insecticides. Toxic action of them occurs by ingestion when insects inhale or have contact with the poison that penetrates their exoskeleton, or when they bite or chew some parts of the plant. Some species from subgenus Calosphace have been reported with these properties mainly by using the essential oils, organic extracts, or even isolated constituents. The essential oil of S. ballotiflora Benth., has these properties in part due to the presence of β-caryophyllene (Cárdenas-Ortega et al., 2015), S. connivens (Zavala-Sánchez et al., 2013; Figure 1C), S. keerlii Benth., (Zavala-Gómez et al., 2021), and S. microphylla (Romo-Asunción et al., 2016) also possess these activities (Supplementary Table S2).
3.2.5 Antiprotozoal Activity
Protozoans are responsible for a variety of diseases including malaria (caused by species of Plasmodium) and Chagas disease (caused by flagellated protozoan Trypanosoma cruzi). Antiprotozoal drugs are agents that kill or inhibit the growth of these organisms. Many of them can cause DNA breakage or prevent its replication, such as metronidazole used for vaginal infections caused by Trichomonas vaginalis, but also in the treatment of giardiasis by flagellated amoeba. Other drugs can produce enzymatic inhibition, for example trimethoprim-sulfamethoxazole, which is used to inhibit folic acid synthesis in Pneumocystis carinii. However, the chemotherapy for protozoal infections causes adverse effects during therapeutic doses that limit their safety use where combined therapies are considered too (Thurston et al., 2015). At least nine species from Calosphace have been investigated as potential options for antiprotozoal therapy the 5,6-dihydroxy-7,3′,4′-trimethoxyflavone was isolated from acetone extract and ethyl acetate fraction of S. amarissima Ortega and proved to be effective against E. histolytica, IC50 = 0.05 μg/ml and G. lamblia, IC50 =0.13 μg/ml (Calzada and Bautista, 2020). Whereas nuchensin (flavone) from S. connivens produced effects at similar potency with an IC50 = 0.072 µM and IC50 = 0.118 μM, respectively (Bautista et al., 2020). Salvia divinorum contains abundant salvinorin A that had effect on the same protozoans at a reported IC50 = 49.0 µM and IC50 = 64.8 μM, respectively (Calzada et al., 2015) (Supplementary Table S2). Other diterpenoids (clerodanes) with activity against E. histolytica and G. lamblia have been isolated from S. herbacea Benth., S. microphylla, S. shannonii Donn. Sm. (Bautista et al., 2013; Calzada et al., 2015). The diterpenoids (abietanes) Clinopodiolide A-C, 19-O-acetylclinopodiolide A, Triacetylclinopodiolide B from S. clinopodiodies Kunth exhibit the same activity (Bustos-Brito et al., 2019). The linearolactone from S. polystachia had amoebicidal and giardicidal activities at IC50 = 22.9 µM and IC50 = 28.2 µM (Calzada et al., 2015) (Supplementary Table S2). Finally, the salviandulin E from S. leucantha had activity against Trypanosoma brucei at IC50 0.72 μg/ml (Aoyagi et al., 2014).
3.2.6 Neuroprotective Activity
Neurodegenerative diseases cause cellular damage that can affect the structure of neurons and/or their function. This damage is in part due to processes of inflammation, oxidative stress, and neurotransmission or hormone-modified conditions that can be regulated by the presence of extracts and their chemical constituents improving the quality of life of people. According to different studies, Salvia leucantha, S. polystachia, and S. tiliifolia are sources of neuroprotective alternatives (Supplementary Table S2). From these species, leucansalvialin G and J from aerial parts of S. leucantha (Li et al., 2018) (Table 1), and Tiliifolin E produced this activity in PC12 cells differentiation (Fan et al., 2017). Differentiation of PC12 cells is assessed by semi-quantitative or quantitative morphological methods. These methods can include the measurement of the cell size, neurite number, and neurite length (Hu R. et al., 2018).
3.2.7 Antidiabetic and Antioxidant Activities
The anti-hyperglycemic effects of medicinal plants are considered to improve the function of pancreatic tissue by rising insulin secretion or reducing the intestinal absorption of glucose (Kooti et al., 2016). The ether extract and fraction (Solares-Pascasio et al., 2021), as well as the compounds (Salinas-Arellano et al., 2020) of S. amarissima, S. chamaedryoides (Bisio et al., 2017), S. coccinea (Sudaramoorthy et al., 2021), and S. elegans (Pereira et al., 2018) are examples of species from Salvia subgenus Calosphace reported as significant antidiabetic plants. For instance, the aerial parts of S. amarissima contain in abundance the flavonoid pedalitin, which has been reported to possess antidiabetic activity by the recombinant α-glucosidase with maltase-glucoamylase action (Flores-Bocanegra et al., 2017), and the inhibition of the protein tyrosine phosphatase (PTP-1b) (Salinas-Arellano et al., 2020). The dichloromethane extract and the compound (1R,5S,7S,8S,9R,10R,12R)-1,7,8-trihydroxycleroda-3,13 (16),14-triene-17,12; 18,19-diolide from aerial parts of S. chamaedryoides was reported to be inhibitory of a-glucosidase and α-amylase activities (Bisio et al., 2017) (Supplementary Table S2).
Since diabetes is a chronic endocrinological disorder in which the metabolism of proteins, fats, and carbohydrates is altered in the oxidative stress that enhances the reactive oxygen species, the antidiabetic activity of plants could be due to their antioxidant properties. Thus, plants that contain several natural antioxidants from different natures can intervene in the regulation of tissular damage, as it has been corroborated using the essential oil, organic extracts, and/or a few bioactive isolated compounds (Supplementary Table S2). The aqueous and dichloromethane extracts from aerial parts of S. greggii (Pereira et al., 2018) and S. wagneriana Pol. (Giamperi et al., 2012) and 6,7,11,14-tetrahydro-7-oxo-icetexone isolated from the aerial parts of S. ballotiflora (Esquivel et al., 2017), respectively, produced significant antioxidant activity evaluated in DPPH assay. The DPPH assay is complemented by using other tests, such as ferric reducing power (FRAP) and TBARS explored with the hexane, dichloromethane, methanol extracts, and the isolated compounds isosessein and sessein from the aerial parts of S. sessei (Gómez-Rivera et al., 2018), and the aqueous extract of the aerial parts of S. mexicana (Afonso et al., 2019b).
3.2.8 Antidiarrheal and Antispasmodic Activities
Both diarrhea and spasms can be part of symptoms of several unhealth conditions associated with the gastrointestinal system. For these symptoms, the identification of its aetiology to establish a pharmacological treatment is very important. Antidiarrheal drugs are motility inhibitors, adsorbents, and/or fluid and electrolyte transport modifiers. The most common adverse effects produced by these substances are constipation, nausea, dry mouth, and abdominal pain, whereas serious adverse effects of some products are paralytic ileus, toxic megacolon, and angioneurotic edema. Spasmolitic drugs are a group of substances that prevent or interrupt the painful and involuntary contraction of intestinal smooth muscle named spasm, a mechanism referred to as the genesis of pain in gastrointestinal pathologies. Several substances isolated as natural products have been recognized and used as spasmolytic drugs, many of them associated with direct smooth muscle relaxants such as those agents derived from papaverine. Other substances act as anticholinergics, for example, butylhyoscine, hyoscine, hyoscyamine, levocine, dicycloverine, butylscopolamine, trimebutine and cimetropium bromide, as well as calcium channel blocking agents as pinaverium bromide, otilonium bromide, alverine, fenoverine, rociverine, and pirenzepine. At least three species of Neotropical sages have been reported to possess antidiarrheal properties: the methanol extract of the aerial parts of S. connivens (Pérez-Gutiérrez et al., 2014; the isolated compounds 19-deoxyicetexone (Pérez-Gutiérrez et al., 2013) and clinopodiolide A, B, and C, triacetylclinopodiolide B (Bustos-Brito et al., 2019) from S. ballotiflora and S. clinopodiodies, respectively. Finally, tilifodiolide obtained from aerial parts of S. tiliifolia (Alba-Betancourt et al., 2019) and 3,4-secoisopimara-4 (18),7,15-triene-3-oic acid from S. cinnabarina have been investigated as spasmolytic treatment (Romussi et al., 2000) (Supplementary Table S2).
3.2.9 Antihypertensive Activity
Hypertension is one of the highest risk factors for cardiovascular accidents, coronary heart disease, cardiac hypertrophy with heart failure (hypertensive heart disease), aortic dissection, and renal failure being a major cause of morbidity and mortality. Hypertension is a disease that very often is not detected and when diagnosed, is not always adequately treated. Anti-hypertensive drugs act by decreasing the cardiac output, the peripheral vascular resistance, or both. The classes of drugs most often used to treat hypertension include the thiazide diuretics, b-blockers, angiotensin-converting enzyme (ECA) inhibitors, angiotensin II receptors antagonists, calcium channel blockers, a-adrenoceptor blockers, combined a- and b-blockers, direct vasodilators, and some centrally acting drugs such as a2-adrenoceptor agonists (Dphil, 2004). In the Neotropical sages hypotensive and antihypertensive effects have been reported in the 3,4-secoisopimara-4 (18),7,15-triene-3-oic acid isolated from aerial parts of S. cinnabarina (Alfieri et al., 2007), and the hydroalcoholic extract and fractions from aerial parts of S. elegans (Jiménez-Ferrer et al., 2010).
3.2.10 Antinociceptive and Anti-inflammatory Activities
Pain alone or associated with inflammation is a clinical condition present in several pathologies. It could be per se a disease, producing disabling conditions and diminished quality of live-in people. Both analgesic and anti-inflammatory drugs are pharmacological armaments for pain relief. Despite these medications being recognized because of their efficacy, the risk of the presence of several adverse effects are limited in their chronic use. Thus, there is a continuous need to develop new efficacious and safety alternatives. Plants have been the origin of several potent and efficacious analgesic compounds for the most important groups of analgesics drugs, such as opiates and non-steroidal anti-inflammatory drugs. To this respect, Calosphace species have been reported to possess significant antinociceptive and/or anti-inflammatory activities according to the results obtained in several pain models, mainly induced by thermal and chemical stimuli. Non-polar, medium polar, and polar extracts of the aerial parts of S. amarissima (Moreno-Pérez et al., 2019; Fernando et al., 2021) and S. semiatrata (Ortiz-Mendoza et al., 2020), as well as from leaves of S. divinorum (Tlacomulco-Flores et al., 2020) were explored in the plantar, writhing and/or formalin test (Fragoso-Serrano et al., 2019; Moreno-Pérez et al., 2019). Amarisolide A and pedalitin from S. amarissima (Moreno-Pérez et al., 2019; Fernando et al., 2021), salvinorin A from S. divinorum (Tlacomulco-Flores et al., 2020), and tilifodiolide from S. tiliifolia (González-Chávez et al., 2018) were isolated as the most abundant bioactive constituents using the same nociceptive tests. Carrageenan-induced edema assay was also applied to corroborate the anti-inflammatory properties of the tilifodiolide isolated from S. tiliifolia (González-Chávez et al., 2018) and the ethyl acetate extract containing amarisolide A in higher concentration vs. other extracts of the same Salvia species (Calzada et al., 2015; Fernando et al., 2021). To corroborate their anti-inflammatory properties the chloroform and dichloromethane extracts from the aerial parts of S. ballotiflora (Campos-Xolalpa et al., 2021) and S. connivens (González-Chávez et al., 2017), respectively, were explored in the TPA-induced ear edema (Supplementary Table S2).
3.2.11 Cytotoxic Activity
Cytotoxic drugs inhibit or prevent the function of cells, they include the substances used in chemotherapy for cancer treatment. When therapeutic doses are given to patients, cytotoxic drugs produce toxic side effects due to their poor selectivity between the target (i.e., cancerous cells) and normal cells, having mutagenic, teratogenic, and carcinogenic effects in humans, particularly in cancer patients receiving long-term therapy. These adverse effects include neoplasms and leukemias, testicular and ovarian dysfunction—including permanent sterility, cumulative chromosome damage, and other organ damage such as in the cases of the alkylating agents (nitrogen mustards, ethylenimine derivatives, and nitrosoureas). However, not all cytotoxic drugs are carcinogenic. Moreover, the use of combined drugs might be more effective than single agents, reducing the adverse effects. In the case of plants with cytotoxic properties, a synergy among their bioactive constituents is recognized, which makes them a good option for cancer therapy, being effective and safe to use. Several species of Salvia subgenus Calosphace have been reported to possess cytotoxic activity, for example: S. amarissima, S. anastomosans Ramamoorthy, S. ballotiflora, S. buchananii, S. leucantha, and S. semiatrata (Supplementary Table S2). The ethyl acetate fraction obtained from the acetone extract of the arterial parts of S. amarissima produced cytotoxic activity in HeLa cells with an IC50 = 1.50 ± 0.21 μg/ml (Bautista et al., 2016). The isolation of pure compounds showed the same activity but in a reduced potency, as it was noticed for teotihuacanin (IC50 = 13.7 ± 4.9 μg/ml) and amarissinin A (IC50 = 14.0 ± 1.04 μg/ml) (Bautista et al., 2016). Cytotoxic activity was also reported for cariocal isolated from the roots of S. anastomosans (Esquivel et al., 2005). From the chloroform extract of the aerial parts of S. ballotiflora antitumoral and cytotoxicity activities were described (Campos-Xolalpa et al., 2017; Campos-Xolalpa et al., 2021), in part due to the presence of 7,20-dihydroanastomosine, 7α-acetoxy-6,7-dihydroicetexone, and anastomosine (Esquivel et al., 2017), as well as 19-deoxyisoicetexone (Campos-Xolalpa et al., 2017). From the roots of S. buchananii it was isolated hyptadienic and salvibuchanic acids (Beladjila et al., 2018b), and tilifolidione from S. semiatrata (Esquivel et al., 2005). Whereas from the aerial parts of S. leucantha, salvileucantholide were evaluated using MTT assay (Jiang et al., 2016) (Table 1), a colorimetric assay to measure cellular metabolic activity as an indicator of cell viability, proliferation, and cytotoxicity. Finally, the aqueous extract from aerial parts of S. mexicana was evaluated to confirm cytotoxic activity using HepG2 (hepatocellular carcinoma), HeLa (cervical carcinoma), and MCF-7 (breast carcinoma) (Afonso et al., 2019b) (Supplementary Table S2).
3.3 Bioactive Compounds Found in Salvia Subgenus Calosphace
Phytochemical studies have resulted in the isolation and identification of a wide variety of bioactive secondary metabolites—grouped in terpenoids and phenolic compounds—, from ca. 90 species of Neotropical sages (Esquivel, 2008; Wu et al., 2012). In the following section these groups of constituents are described.
3.3.1 Terpenoids
A number of in vitro, in vivo, and ex vivo studies, have demonstrated that the terpenoids are the main compounds responsible for the therapeutic activity of the sages (Aydoǧmuş et al., 2006; Pérez-Gutiérrez et al., 2013; Li et al., 2018; Fernando et al., 2021). The active terpenoids that have been isolated from species of Salvia subgenus Calosphace can be grouped into mono-, sesqui-, di-, and triterpenes. The diterpenes (abietanes and clerodanes) are the most common of these compounds and thus have been more extensively studied, having several pharmacological evaluations and biological activities reported.
3.3.1.1 Monoterpenoids
Monoterpenoids are widely distributed in land plants and are mostly found in essential oils (Kabir et al., 2020). Studies have demonstrated the insecticidal activity of the essential oils of S. ballotiflora, S. elegans, and S. keerlii (Cardenas et al., 2015; Mathew and Thoppil, 2011; Zavala-Gómez et al., 2021), the antioxidant potential of S. microphylla (Lima et al., 2012), and the antifungal activity and inhibition of the butyrylcholinesterase enzyme of S. hispanica and S. leucantha (Elshafie et al., 2018; Villalta et al., 2021). Species such as S. elegans, S. keerlii and S. leucantha have a great diversity of these types of metabolites in their essential oils (De Martino et al., 2010; Rojas et al., 2010; Zavala-Gómez et al., 2021). Some of the most common components of the essential oils from these species belong to the monocyclic type—γ-terpinene (1), limonene (2) and 4-terpineol (3)—, while others are bicyclic (e.g., α-pinene (4), cis-thujone (5), 1,8-cineole (6) and camphor (7) (Figure 3A). The essential oils of S. cinnabarina and S. greggii showed the same diversity of monoterpenoids; however, they lack pharmacological or biological evaluations (Bisio et al., 1998; Giuliani et al., 2017).
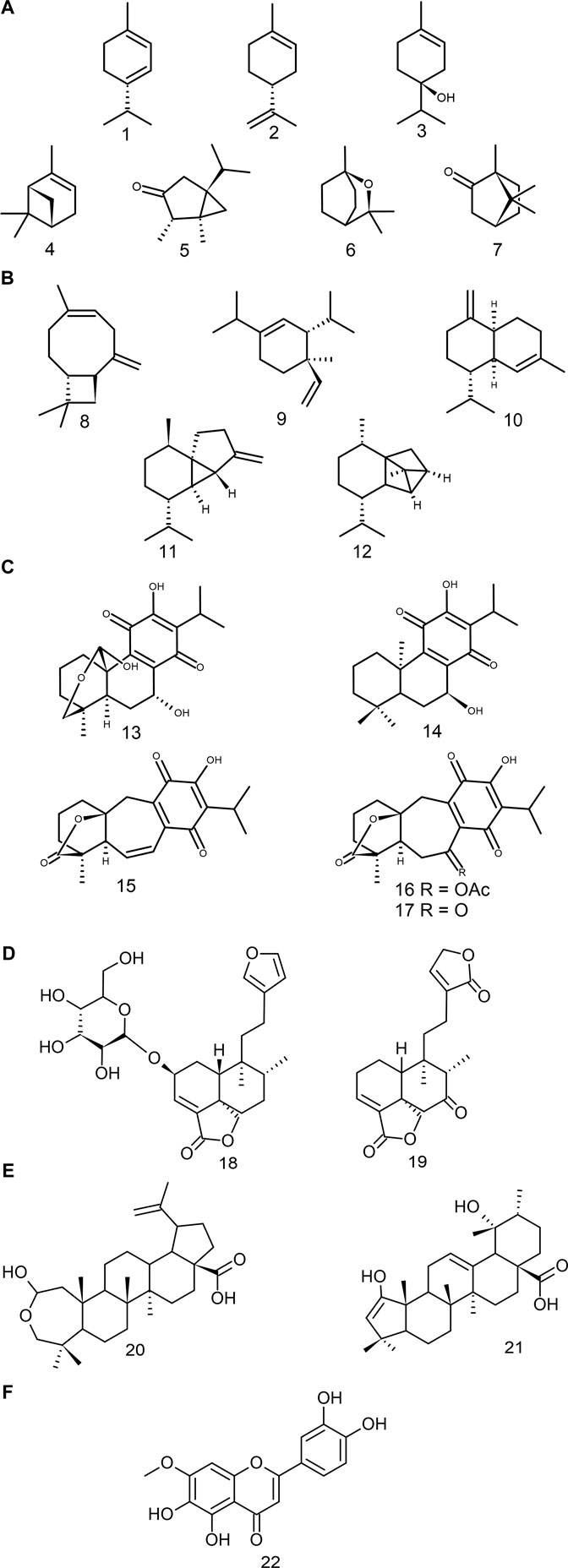
FIGURE 3. Bioactive compounds found in Salvia subgenus Calosphace. (A) Monoterpenoids, (B) Sesquiterpenoids, (C) Abietanes, (D) Clerodanes, (E) Triterpenoids, (F) Polyphenols.
3.3.1.2 Sesquiterpenoids
These compounds, as the monoterpenoids do, form a significant part of the essential oils of sages. They constitute the most diverse groups of terpenoids, having lineal, mono-, bi-, tri-, and tetracyclic structures (Ludwiczuk et al., 2017). However, within the Neotropical sages, the cytotoxic effect has only been demonstrated by the insecticidal effect of the β-caryophyllene 8) (Cárdenas-Ortega et al., 2015). The latter is a bicyclic compound common to the species of the genus Salvia s.l. (Tenore et al., 2011; Ascrizzi et al., 2017; Borges et al., 2019). A wide diversity of these metabolites can be found in different species of the subgenus, for instance, δ-elemene 9), γ-muurolene 10), β-cubebene 11) and cyclosativene 12) (Figure 3B) have been identified in the essential oil of Salvia elegans. Sesquiterpenoids are a potential field of study in pharmacology, due to their anti-inflammatory properties (Da Silveira e Sá Rde et al., 2015).
3.3.1.3 Diterpenoids
These terpenoids are characteristic of Salvia and have been proposed as a quimiotaxonomic marker. A number of these metabolites have been characterised from species of subgenus Calosphace, they can be classified mainly in abietanes and clerodanes (Esquivel, 2008). In several evaluations of pharmacological and biological activities, it has been demonstrated that the most active extracts are those of median polarity, where these compounds are found in the highest concentration (Ortiz-Mendoza et al., 2020; Tlacomulco-Flores et al., 2020; Fernando et al., 2021).
Abietanes
Conacytone (13), horminone (14), and icetexone (15) are all examples of compounds commonly found in species of Neotropical sages (Wu et al., 2012) (Figure 3C). The icetexone and its derivatives (i.e., α-acetoxy-6,7-dihydroicetexone (16) and the 6,7,11,14-tetrahydro-7-oxo-icetexone (17)) have mainly cytotoxic, anti-inflammatory and antioxidant activities (Esquivel et al., 2017). On the other hand, the conacytone and horminone have anti-inflammatory and antibacterial properties (Esquivel et al., 2017; Martínez-Vázquez et al., 1998). Some species that have a wide range of abietanes but lack pharmacological studies are: S. anastomosans, S. ballotiflora, S. candicans M. Martens and Galeotti, S. concolor Lamb. ex Benth., S. corrugata Vahl (Figure 1D), S. pubescens Benth., S. reptans, and S. uliginosa Benth., (Sanchez et al.,. 1989; Cárdenas and Rodríguez-Hahn, 1995; Esquivel et al., 1997; Martínez-Vázquez et al., 1998; Giacomelli et al., 2013; Esquivel et al., 2017; Cezarotto et al., 2019; Díaz-Fernández et al., 2019).
Clerodanes
A number of clerodanes have been characterised in the following species of subgenus Calosphace: Salvia amarissima, S. divinorum, S. dugesii Fernald, S. hispanica, S. leucantha, and S. polystachia (Shirota et al., 2006; Gang et al., 2011; Jiang et al., 2016; Bautista et al., 2017; Calzada et al., 2020; Fan et al., 2020). Most of these clerodanes are classified, according to their absolute stereochemistry, as neo-clerodanes (Li et al., 2016). More than 80 neo-clerodanes isolated from Neotropical sages have been evaluated in pharmacological and biological activity studies, corroborating their several properties (Supplementary Table S2). Recent studies have demonstrated that neo-clerodanes such as, Amarisolide A (18) and 7-keto-neoclerodan-3,13-dien-18,19:15,16-diolide (19) (Figure 3D), have great antinociceptive and anti-inflammatory potentials (Ortiz-Mendoza et al., 2020; Fernando et al., 2021).
3.3.1.4 Triterpenoids
In this group stand out the oleanolic and ursolic acids, two compounds common to sages from all the subgenus (Wu et al., 2012). Both pentacyclic triterpenoids have several biological effects, such as antimicrobial, anti-inflammatory, anti-ulcer, antihyperlipidemic, hepatoprotective, and hypoglycemic effects (Topçu, 2006). Within Calosphace, two compounds that are derived from these acids have been isolated: hyptadienic acid (20) and the salvibuchanic acid (21) (Figure 3E). Both compounds have a cytotoxic effect against Jurkat and HeLa cell lines (Beladjila et al., 2018b).
3.3.1.5 Polyphenols
These metabolites are notorious for their potent antioxidant effect, and recently activity associated with chronic illness prevention has been detected (Ziaullah and Rupasinghe, 2015). This group of compounds is characterised by the presence of hydroxyl groups (-OH) in their structures, which gives them an affinity for high-polarity solvents. In high-polarity extracts from species of subgenus Calosphace antidiabetic, antioxidant, antibacterial, antinociceptive and anxiolytic effects have been reported (Supplementary Table S2). However, pharmacological evaluations of the isolated compounds from this group are scarce, and only six of them have been studied, being the Pedalitine (22) the most cited (Figure 3F) (Flores-Bocanegra et al., 2017; Moreno-Pérez et al., 2019; Salinas-Arellano et al., 2020).
3.4 Differential Distribution of Terpenoids in Salvia Subgenus Calosphace
The most characteristic metabolites found in Salvia are the diterpenoids and there is no clear pattern on their distribution throughout the phylogeny (Figure 4). However, when the diterpenoids are further classified into abietanes and clerodanes, there is a marked difference in their distribution along the lineages of Salvia (Supplementary Figure S1). The abietanes are mostly found in the European, Asian, and North American lineages of Salvia (i.e., subgenus Audibertia, Glutinaria, Rosmarinus, and the clades Salvia, Sclarea and Salvia aegyptiaca). Within Salvia subgenus Calosphace, these metabolites are mainly present in members of the early diverging lineages, and are absent mostly in the members of the most diverse clade, core Calosphace. On the other hand, the clerodanes are restricted to the Neotropical sages, and they are mostly found in the members of the core Calosphace clade (Supplementary Figure S1).
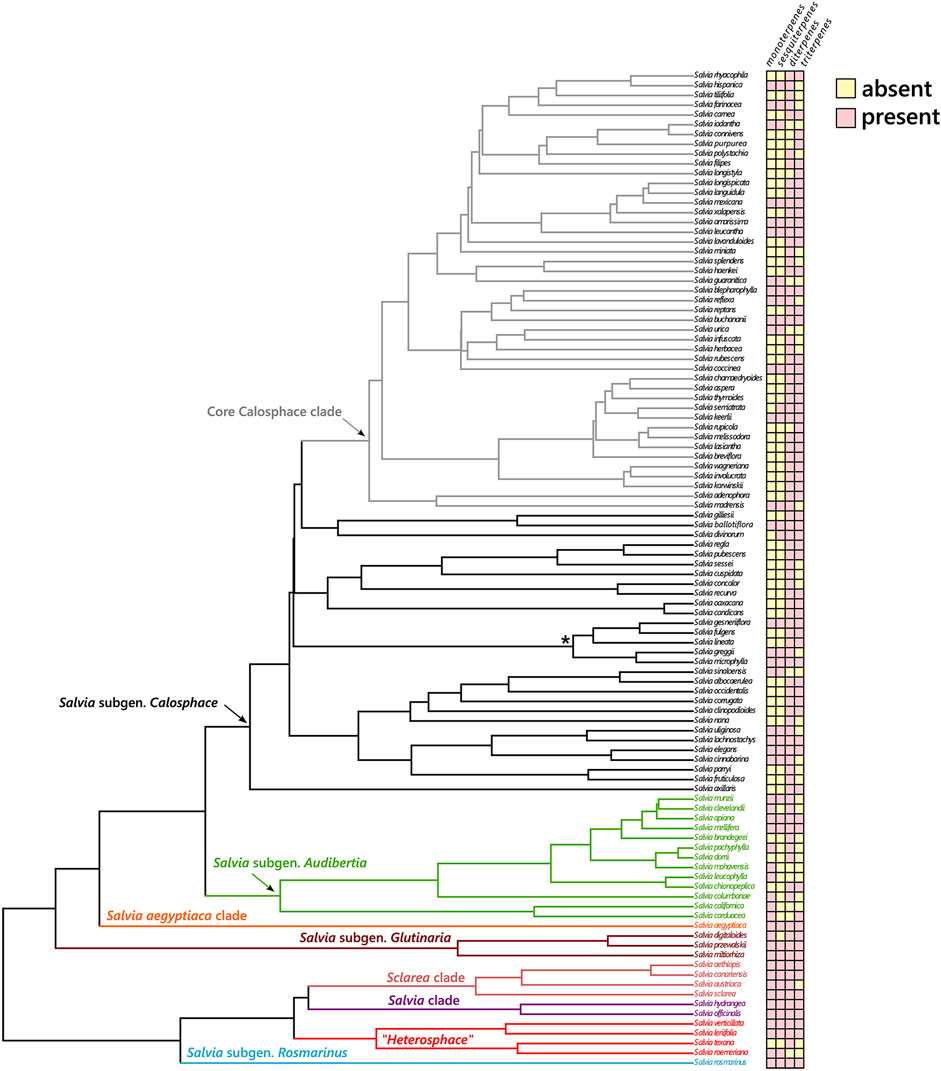
FIGURE 4. Distribution of different types of terpenes in the Neotropical sages (Salvia subgen. Calosphace) and their related lineages. The lineages of Salvia s.l. are color-coded. An asterisk (*) marks the Fulgentes subclade, recovered as part of the core Calosphace clade in other phylogenies (i.e., Jenks et al., 2013; Fragoso-Martínez et al., 2018).
Regarding the distribution of the remaining terpenoids in the subgenus, the second most common metabolites found in Salvia subgenus Calosphace were the triterpenoids. However, they were also common in the remaining lineages of Salvia, although they have been scarcely reported for the Californian sages (Salvia subgenus Audibertia), which are the sister lineage of the Neotropical sages (Figure 4). According to the phytochemical studies reviewed, the sesquiterpenes are the third most abundant metabolite in subgenus Calosphace. They were present in the other lineages of Salvia as well, and they were usually produced by the same species that present monoterpenes (Figure 4). Nevertheless, this pattern was not observed in S. subgenus Audibertia, probably due to the scarcity of phytochemical studies that identified them. Lastly, the monoterpenes seem to be the least abundant metabolites in subgenus Calosphace. Only 26% of the surveyed species have reports of monoterpenoids, being apparently more widely distributed in the other lineages of Salvia (86% of studies from the included species).
4 Discussion
Salvia subgenus Calosphace is the most diverse lineage of the genus, and it is mainly distributed in the Neotropics. In Mexico, Salvia is the most diverse genus of flowering plants, which explains its central paper in traditional medicine, being used by many of the ethnic groups found in the country to treat a wide variety of diseases. The most employed species are S. microphylla, S. coccinea, and S. lavanduloides, all of them native and widely distributed species, but none of them endemic to Mexico. The main uses attributed to the Mexican species are those related to the digestive system, followed by symptoms and signs (e.g., fever, headaches), pregnancy and childbirth, and cultural-bound syndromes. Despite the diversity of traditional uses reported for the different species of Neotropical sages, many are yet to be explored and corroborated by scientific studies. For instance, the properties of S. lavanduloides, which is used to treat diseases from 9 categories, have not been investigated in any pharmacological study. Reviews such as the one presented here can encourage the study of the medicinal properties and pharmacological potential of the native sages.
Regarding the pharmacological studies of species from subgenus Calosphace, literature revision allowed us to notice that the investigation of their potential is scarce. The extracts or isolated compounds of 38 species of subgenus Calosphace have been studied, from these ca. 109 chemical structures have been evaluated, being the diterpenes with clerodane- and abietane-type skeleton the most common with 73 and 21 structures, respectively. The few species that have been explored in preclinical studies have shown a wide spectrum of biological activities. Most of the studied species were referred to as potential antioxidant, anti-inflammatory and cytotoxic, followed by the group of plants used for their activities against microorganisms and insects (antibacterial > insecticidal > antiprotozoal effects). It is worth noting that the extracts of some species produced important effects on the central nervous system, including those species used as anxiolytic > antinociceptive > antidepressant. Finally, few species are reported to be effective for gastrointestinal and cardiovascular systems and some others have influence on metabolic illnesses.
The anti-inflammatory, antioxidant, and/or cytotoxic properties reported for independent species or even a single one, suggests their potential use for difficult conditions such as cancer therapy. It is well-known that free radicals contribute to protein and DNA damage, inflammation, tissue damage, and subsequent cellular apoptosis, which is thought to play a role in the development of cancer and neurodegenerative diseases (Uttara et al., 2009). Thus, antioxidant properties of plants and their natural products can exert protective effects to this and others health conditions, due to its remarkable activity against reactive oxygen species and other free radicals, a property mainly recognized for polyphenols, nitrogen compounds, terpenoids, and vitamins (Uttara et al., 2009; Nani et al., 2021). Anti-inflammatory effects are also an important property of plants, since several alterations in health at peripheral, and central level begin with the presence of inflammation. Chronic inflammation has been associated to be involved in tumorigenesis (i.e., in cellular transformation, promotion, survival, proliferation, invasion, angiogenesis, and metastasis), as well as in degenerative diseases such as Alzheimer, Parkinson, Multiple Sclerosis, and Huntington’s disease (Amor et al., 2010; Singh et al., 2019). According to our literature search, other mental conditions such as anxiety, depression, and sleep alterations, can also be impacted by several extracts of Neotropical sages.
The most characteristic and studied metabolites in the Neotropical sages are the diterpenoids (clerodanes and abietanes). Studies focusing on the pharmacological properties of other metabolites such as monoterpenes, sesquiterpenes, triterpenes, and phenolic compounds should be expanded to provide a more complete picture of the medicinal potential of Salvia subgenus Calosphace.
The members of Salvia subgenus Calosphace are rich in bioactive compounds, but many of them are shared with their sister lineages (e.g., mono-, tri-, and sesquiterpenoids) or even with other groups of land plants (e.g., the oleanolic and ursolic acids). However, the diterpenoids, particularly the clerodanes, are almost exclusive to the Neotropical sages. One of such clerodanes is the Amarisolide A, found in Salvia amarissima, that has been studied for its analgesic, anti-inflammatory, antidiabetic, anxiolytic, and cytotoxic properties. An increased study of members of Calosphace from other centres of diversity (i.e., Andean region, Antilles, and Brazil) is needed to further understand the evolution of the phytochemistry of the Neotropical sages and uncover finer biogeographic, ecological, karyological, phylogenetic, and/or morphological patterns that could potentially be associated with the distribution of the terpenoids in Calosphace.
5 Perspectives
Ethnobotanical, pharmacological, and phytochemical information integrated into this review gives evidence that the Neotropical sages are a source of possible new drugs for acute and chronic diseases that will support chemical weaponry of medicine which always requires novel, effective and safe options for the treatment of diseases. We recommend that future studies targeting specific compounds at finer scales use the phylogenies available for the group to guide their sampling, focusing on sister species or taxa from the same clades that are likely to share some of the compounds of interest.
Author Contributions
EA-H and MM-G conceived the idea of the paper. NO-M reviewed, compiled, and elaborated the tables of the terpenoids in Salvia. IF-M translated text, made figures and plots, adjusted the data matrices and plotted the distribution of terpenoids in Salvia. EA-H and NO-M contributed with the information on phytochemistry; MM-G and FB-P compiled and processed the information on ethnobotany; MG-T, EA-H, and NO-M compiled and processed the information on pharmacology. All the authors contributed equally to the discussion and writing of the paper. This paper was taken in part from the Ph.D of NO-M.
Funding
This work was supported by grants from the UNAM-PAPIIT (No. IN221221). Additional funding to cover publication fees through the National System of Researchers (SNI-Conacyt) grant to EA-H (52151), IF-M (83797), MG-T (25375), and MM-G (55788).
Conflict of Interest
The authors declare that the research was conducted in the absence of any commercial or financial relationships that could be construed as a potential conflict of interest.
Publisher’s Note
All claims expressed in this article are solely those of the authors and do not necessarily represent those of their affiliated organizations, or those of the publisher, the editors and the reviewers. Any product that may be evaluated in this article, or claim that may be made by its manufacturer, is not guaranteed or endorsed by the publisher.
Acknowledgments
The authors thank G. Salazar for providing photographs of species of Neotropical sages; Maria Teresa Jiménez Segura for processing and adjusting the figures to the required specifications of the journal; the editors of the special issue and the two anonymous reviewers for their comments on the manuscript. Gabriel Fernando Moreno-Pérez thanks Postgraduate in Biological Sciences at the National Autonomous University of Mexico for having received Ph.D. academic training and CONACYT fellowship number 631351. NO-M thanks Postgraduate in Biological Sciences at the National Autonomous University of Mexico for having received Ph.D. academic training and CONACYT fellowship number 793655.
Supplementary Material
The Supplementary Material for this article can be found online at: https://www.frontiersin.org/articles/10.3389/fphar.2022.867892/full#supplementary-material
References
Afonso, A. F., Pereira, O. R., Fernandes, Â. S. F., Calhelha, R. C., Silva, A. M. S., Ferreira, I. C. F. R., et al. (2019a). The Health-Benefits and Phytochemical Profile of Salvia Apiana and Salvia Farinacea Var. Victoria Blue Decoctions. Antioxidants (Basel). 8, 1–14. doi:10.3390/antiox8080241
Afonso, A. F., Pereira, O. R., Fernandes, Â., Calhelha, R. C., Silva, A. M. S., Ferreira, I. C. F. R., et al. (2019b). Phytochemical Composition and Bioactive Effects of Salvia Africana, Salvia Officinalis 'Icterina' and Salvia Mexicana Aqueous Extracts. Molecules. 24, 1–13. doi:10.3390/molecules24234327
Akhondzadeh, S., Noroozian, M., Mohammadi, M., Ohadinia, S., Jamshidi, A. H., and Khani, M. (2003). Melissa Officinalis Extract in the Treatment of Patients with Mild to Moderate Alzheimer's Disease: a Double Blind, Randomised, Placebo Controlled Trial. J. Neurol. Neurosurg. Psychiatry. 74, 863–866. doi:10.1136/jnnp.74.7.863
Alba-Betancourt, C., Sánchez-Recillas, A., Alonso-Castro, A. J., Esquivel-Juárez, D., Zapata-Morales, J. R., Yáñez-Pérez, V., et al. (2019). Antidiarrheal, Vasorelaxant, and Neuropharmacological Actions of the Diterpene Tilifodiolide. Drug Dev. Res. 80, 981–991. doi:10.1002/ddr.21578
Alfieri, A., Maione, F., Bisio, A., Romussi, G., Mascolo, N., and Cicala, C. (2007). Effect of a Diterpenoid from Salvia Cinnabarina on Arterial Blood Pressure in Rats. Phytother. Res. 21, 690–692. doi:10.1002/ptr.2128
Amor, S., Puentes, F., Baker, D., and van der Valk, P. (2010). Inflammation in Neurodegenerative Diseases. Immunology. 129 (2), 154–169. doi:10.1111/j.1365-2567.2009.03225.x
Aoyagi, Y., Fujiwara, K., Yamazaki, A., Sugawara, N., Yano, R., Fukaya, H., et al. (2014). Semisynthesis of salviandulin e analogues and their antitrypanosomal activity. Bioorg. Med. Chem. Lett. 24, 442–446. doi:10.1016/j.bmcl.2013.12.052
Aoyagi, Y., Yamazaki, A., Nakatsugawa, C., Fukaya, H., Takeya, K., Kawauchi, S., et al. (2008). Salvileucalin B, A Novel Diterpenoid with an Unprecedented Rearranged Neoclerodane Skeleton from Salvia leucantha Cav. Org. Lett. 10 (20), 4429–4432. doi:10.1021/ol801620u
Ascrizzi, R., Cioni, P. L., Amadei, L., Maccioni, S., and Flamini, G. (2017). Geographical Patterns of In Vivo Spontaneously Emitted Volatile Organic Compounds in S Alvia Species. Microchemical J. 133, 13–21. doi:10.1016/j.microc.2017.03.002
Aydoğmuş, Z., Yeşİlyurt, V., and Topcu, G. (2006). Constituents ofSalvia Microphylla. Nat. Product. Res. 20, 775–781. doi:10.1080/14786410500462843
Ayoub, I. M., George, M. Y., Menze, E. T., Mahmoud, M., Botros, M., Essam, M., et al. (2022). Insights into the Neuroprotective Effects of Salvia Officinalis L. And Salvia Microphylla Kunth in the Memory Impairment Rat Model. Food Funct. 13, 2253–2268. doi:10.1039/d1fo02988f
Baricevic, D., Sosa, S., Della Loggia, R., Tubaro, A., Simonovska, B., Krasna, A., et al. (2001). Topical Anti-inflammatory Activity of Salvia Officinalis L. Leaves: the Relevance of Ursolic Acid. J. Ethnopharmacol. 75 (2–3), 125–132. doi:10.1016/s0378-8741(00)00396-2
Bautista, E., Fragoso-Serrano, M., Ortiz-Pastrana, N., Toscano, R. A., and Ortega, A. (2016). Structural Elucidation and Evaluation of Multidrug-Resistance Modulatory Capability of Amarissinins A-C, Diterpenes Derived from Salvia Amarissima. Fitoterapia. 114, 1–6. doi:10.1016/j.fitote.2016.08.007
Bautista, E., Ortiz-Pastrana, N., Pastor-Palacios, G., Montoya-Contreras, A., Toscano, R. A., Morales-Jiménez, J., et al. (2017). Neo-Clerodane Diterpenoids from Salvia polystachya Stimulate the Expression of Extracellular Matrix Components in Human Dermal Fibroblasts. J. Nat. Prod. 80, 3003–3009. doi:10.1021/acs.jnatprod.7b00591
Bautista, E., Calzada, F., Yépez-Mulia, L., Bedolla-García, B. Y., Fragoso-Serrano, M., Pastor-Palacios, G., et al. (2020). Salvia Connivens, a Source of Bioactive Flavones with Amoebicidal and Giardicidal Activity. Rev. Bras. Farmacogn. 30, 729–732. doi:10.1007/s43450-020-00103-8
Bautista, E., Toscano, A., Calzada, F., Díaz, E., Yépez-Mulia, L., and Ortega, A. (2013). Hydroxyclerodanes from Salvia shannoni. J. Nat. Prod. 76, 1971–1975. doi:10.1021/np400606g
Beladjila, K. A., Berrehal, D., Al-Aboudi, A., Semra, Z., Al-Jaber, H., Bachari, K., et al. (2018a). Composition and Antioxidant, Anticholinesterase, and Antibacterial Activities of the Essential Oil of Salvia Buchananii from Algeria. Chem. Nat. Compd. 54, 581–583. doi:10.1007/s10600-018-2414-z
Beladjila, K. A., Cotugno, R., Berrehal, D., Kabouche, Z., de Tommasi, N., Braca, A., et al. (2018b). Cytotoxic Triterpenes from Salvia Buchananii Roots. Nat. Prod. Res. 32, 2025–2030. doi:10.1080/14786419.2017.1365072
Bello, M. F., Hernández, S., Chávez, B., and Salgado, R. (2015). Plantas útiles de la comunidad indígena Nuevo San Juan Parangaricutiro, Michoacán, México. Polibotánica. 39, 175–215.
Bentham, G. (1948). “Labiatae,” in Prodromus Systematis Naturalis Regni Vegetabilis. Editor A. P. De Candolle (Paris: Victor Masson).
Bigham, A. K., Munro, T. A., Rizzacasa, M. A., and Robins-Browne, R. M. (2003). Divinatorins A-C, New Neoclerodane Diterpenoids from the Controlled Sage Salvia Divinorum. J. Nat. Prod. 66, 1242–1244. doi:10.1021/np030313i
Bisio, A., de Mieri, M., Milella, L., Schito, A. M., Parricchi, A., Russo, D., et al. (2017). Antibacterial and Hypoglycemic Diterpenoids from Salvia Chamaedryoides. J. Nat. Prod. 80, 503–514. doi:10.1021/acs.jnatprod.6b01053
Bisio, A., Schito, A. M., Ebrahimi, S. N., Hamburger, M., Mele, G., Piatti, G., et al. (2015). Antibacterial Compounds from Salvia Adenophora Fernald (Lamiaceae). Phytochemistry. 110, 120–132. doi:10.1016/j.phytochem.2014.10.033
Bisio, A., Ciarallo, G., Romussi, G., Fontana, N., Mascolo, N., Capasso, R., et al. (1998). Chemical Composition of Essential Oils from Some Salvia Species. Phytother. Res. 12, 117–120. doi:10.1002/(sici)1099-1573(1998)12:1+<s117::aid-ptr269>3.0.co;2-2
Borges, R. S., Ortiz, B. L. S., Pereira, A. C. M., Keita, H., and Carvalho, J. C. T. (2019). Rosmarinus Officinalis Essential Oil: A Review of its Phytochemistry, Anti-inflammatory Activity, and Mechanisms of Action Involved. J. Ethnopharmacol. 229, 29–45. doi:10.1016/j.jep.2018.09.038
Braund, T. A., Tillman, G., Palmer, D. M., Gordon, E., Rush, A. J., and Harris, A. W. F. (2021). Antidepressant Side Effects and Their Impact on Treatment Outcome in People with Major Depressive Disorder: an iSPOT-D Report. Transl. Psychiatry. 11, 417. doi:10.1038/s41398-021-01533-1
Bustos-Brito, C., Joseph-Nathan, P., Burgueño-Tapia, E., Martínez-Otero, D., Nieto-Camacho, A., Calzada, F., et al. (2019). Structure and Absolute Configuration of Abietane Diterpenoids from Salvia Clinopodioides: Antioxidant, Antiprotozoal, and Antipropulsive Activities. J. Nat. Prod. 82 (5), 1207–1216. doi:10.1021/acs.jnatprod.8b00952
Cahill, J. P. (2003). Ethnobotany of Chia, Salvia Hispanica L. (Lamiaceae). Econ. Bot. 57, 604–618. doi:10.1663/0013-0001(2003)057[0604:eocshl]2.0.co;2
Calzada, F., and Bautista, E. (2020). Plants Used for the Treatment of Diarrhoea from Mexican flora with Amoebicidal and Giadicidal Activity, and Their Phytochemical Constituents. J. Ethnopharmacol. 253, 112676. doi:10.1016/j.jep.2020.112676
Calzada, F., Bautista, E., Yépez-Mulia, L., García-Hernandez, N., and Ortega, A. (2015). Antiamoebic and Antigiardial Activity of Clerodane Diterpenes from Mexican Salvia Species Used for the Treatment of Diarrhea. Phytother. Res. 29, 1600–1604. doi:10.1002/ptr.5421
Calzada, F., Bautista, E., Barbosa, E., Salazar-Olivo, L. A., Alvidrez-Armendáriz, E., and Yepez-Mulia., L. (2020). Antiprotozoal Activity of Secondary Metabolites from Salvia Circinata. Rev. Bras. Farmacogn. 30, 593–596. doi:10.1007/s43450-020-00077-7/Published
Campos-Xolalpa, N., Alonso-Castro, Á. J., Ortíz-Sanchez, E., Zapata-Morales, J. R., González-Chávez, M. M., and Pérez, S. (2021). Anti-inflammatory and Antitumor Activities of the Chloroform Extract and Anti-inflammatory Effect of the Three Diterpenes Isolated from Salvia Ballotiflora Benth. BMC Complement. Med. Ther. 21, 17–11. doi:10.1186/s12906-020-03179-w
Campos-Xolalpa, N., Alonso-Castro, Á. J., Sánchez-Mendoza, E., Zavala-Sánchez, M. Á., and Pérez-Gutiérrez, S. (2017). Cytotoxic Activity of the Chloroform Extract and Four Diterpenes Isolated from Salvia Ballotiflora. Revista Brasileira de Farmacognosia. 27, 302–305. doi:10.1016/j.bjp.2017.01.007
Cárdenas, J., and Rodríguez-Hahn, L. (1995). Abietane and Icetexane Diterpenoids from Salvia Cadicans. Phytochemistry. 38, 199–204. doi:10.1016/0031-9422(94)00569-F
Cárdenas-Ortega, N., González-Chávez, M., Figueroa-Brito, R., Flores-Macías, A., Romo-Asunción, D., Martínez-González, D., et al. (2015). Composition of the Essential Oil of Salvia Ballotiflora (Lamiaceae) and its Insecticidal Activity. Molecules. 20, 8048–8059. doi:10.3390/molecules20058048
Casselman, I., Nock, C. J., Wohlmuth, H., Weatherby, R. P., and Heinrich, M. (2014). From Local to Global-Fifty Years of Research on Salvia Divinorum. J. Ethnopharmacol. 151, 768–783. doi:10.1016/j.jep.2013.11.032
Cezarotto, C. S., Dorneles, A., Baldissera, F. G., da Silva, M. B., Markoski, M. M., Júnior, L. C. R., et al. (2019). Leishmanicidal and Antichemotactic Activities of Icetexanes from Salvia Uliginosa Benth. Phytomedicine. 58, 152748. doi:10.1016/j.phymed.2018.11.009
Chen, Y., Luo, J., Zhang, N., Yu, W., Jiang, J., and Dai, G. (2021). Insecticidal Activities of Salvia Hispanica L. Essential Oil and Combinations of Their Main Compounds against the Beet Armyworm Spodoptera Exigua. Ind. Crops Prod. 162, 113271. doi:10.1016/j.indcrop.2021.113271
Christenhusz, M. J. M., and Byng, J. W. (2016). The Number of Known Plants Species in the World and its Annual Increase. Phytotaxa. 261 (3), 201–217. doi:10.11646/phytotaxa.261.3.1
Chun-Yan, S., Qian-Liang, M., Khalid, R., Ting, H., and Lu-Ping, Q. (2015). Salvia Miltiorrhiza: Traditional Medicinal Uses, Chemistry, and Pharmacology. Chin. J. Nat. Med. 13, 163–182. doi:10.1016/S1875-5364(15)30002-9
Claßen-Bockhoff, R., Wester, P., and Tweraser, E. (2003). The Staminal Lever Mechanism in Salvia L. (Lamiaceae)-A Review. Plant Biol. 5, 33–41. doi:10.1055/s-2003-37973
Claßen-Bockhoff, R., Crone, M., and Baikova, E. (2004). Stamen Development in Salvia L.: Homology Reinvestigated. Int. J. Plant Soil Sci. 165, 475–498. doi:10.1086/386565
Cornejo, G., Ibarra, G., and México, D. F. (2008). Flora ilustrada de la reserva de la mariposa monarca. CONABIO: UNAM.
Cowan, M. M. (1999). Plant Products as Antimicrobial Agents. Clin. Microbiol. Rev. 12 (4), 564–582. doi:10.1128/CMR.12.4.564
Da Silveira e Sá Rde, C., Andrade, L. N., and Pergentino De Sousa, D. (2015). Sesquiterpenes from Essential Oils and Anti-inflammatory Activity. Nat. Prod. Commun. 10, 1767–1774. doi:10.1177/1934578X1501001033
De Martino, L., Roscigno, G., Mancini, E., de Falco, E., and de Feo, V. (2010). Chemical Composition and Antigerminative Activity of the Essential Oils from Five Salvia Species. Molecules. 15, 735–746. doi:10.3390/molecules15020735
Diaz, J. L. (2013). Salvia Divinorum: A Psychopharmacological Riddle and a Mind-Body prospect. Curr. Drug Abuse Rev. 6, 43–53. doi:10.2174/18744737112059990004
Díaz-Fernández, M., Salazar, M. I., Joseph-Nathan, P., and Burgueño-Tapia, E. (2019). Configurational Study of Diastereoisomeric Royleanone Diterpenoids from Salvia Concolor. Nat. Product. Commun. 14, 1934578X1986265–8. doi:10.1177/1934578X19862650
Dphil, P. F. (2004). Hypertension: Pathophysiology and Treatment. Anaesth. Crit. Care Pain Med. 4, 71–75. doi:10.1093/bjaceaccp/mkh045
Drew, B. T., González-Gallegos, J. G., Xiang, C. L., Walked, J. B., and Sytsma, K. J. (2017). Salvia United: the Greatest Good for the Greatest Number. Taxon. 66, 133–145. doi:10.12705/661.7
Elshafie, H. S., Aliberti, L., Amato, M., de Feo, V., and Camele, I. (2018). Chemical Composition and Antimicrobial Activity of Chia (Salvia Hispanica L.) Essential Oil. Eur. Food Res. Technol. 244, 1675–1682. doi:10.1007/s00217-018-3080-x
Epling, C. (1939). A Revision of Salvia Subgenus Calosphace. Repert Spec. Nov. Regni Veg. 85, 1–341.
Esquivel, B., Bustos-Brito, C., Sánchez-Castellanos, M., Nieto-Camacho, A., Ramírez-Apan, T., Joseph-Nathan, P., et al. (2017). Structure, Absolute Configuration, and Antiproliferative Activity of Abietane and Icetexane Diterpenoids from Salvia Ballotiflora. Molecules. 22 (10), 1690. doi:10.3390/molecules22101690
Esquivel, B., Sánchez, A. A., Vergara, F., Matus, W., Hernandez-Ortega, S., and Ramírez-Apan, M. T. (2005). Abietane Diterpenoids from the Roots of Some Mexican Salvia Species (Labiatae): Chemical Diversity, Phytogeographical Significance, and Cytotoxic Activity. Chem. Biodivers. 2, 738–747. doi:10.1002/cbdv.200590051
Esquivel, B., Calderon, J. S., Flores, E., Chavez, C., and Juarez, M. (1997). Abietane and Icetexane Diterpenoids from Salvia Pubescens. Nat. Product. Lett. 10, 87–93. doi:10.1080/10575639708043720
Esquivel, B. (2008). Rearranged Clerodane and Abietane Derived Diterpenoids from American Salvia Species. Nat. Prod. Commun. 3, 989–1002. doi:10.1177/1934578x0800300628
Etminan, A., Pour-Aboughadareh, A., Noori, A., Ahmadi-Rad, A., Shooshtari, L., Mahdavian, Z., et al. (2018). Genetic Relationships and Diversity Among Wild Salvia Accessions Revealed by ISSR and SCoT Markers. Biotechnol. Biotechnological Equipment. 32 (3), 610–617. doi:10.1080/13102818.2018.1447397
Fan, M., Bao, Y., Zhang, Z. J., Zhang, H. B., and Zhao, Q. S. (2017). New Neo-Clerodane Diterpenoids with Neurotrophic Activity from the Aerial Parts of Salvia Tiliifolia. Fitoterapia. 123, 44–50. doi:10.1016/j.fitote.2017.09.013
Fan, M., Luo, D., Peng, L. Y., Wu, X. D., Ji, X., and Zhao, Q. S. (2020). Rearranged Neoclerodane Diterpenoids from the Aerial Parts of Salvia Hispanica L. Fitoterapia. 146, 104672. doi:10.1016/j.fitote.2020.104672
Fernando, M. P., Alberto, H. L., María Guadalupe, V. D., Agustina, C. M., Fernando, N. G., Eva, A. H., et al. (2021). Neo-clerodane Diterpenic Influence in the Antinociceptive and Anti-inflammatory Properties of Salvia Circinnata Cav. J. Ethnopharmacology. 268, 113550. doi:10.1016/j.jep.2020.113550
Flores-Bocanegra, L., González-Andrade, M., Bye, R., Linares, E., and Mata, R. (2017). α-Glucosidase Inhibitors from Salvia Circinata. J. Nat. Prod. 80, 1584–1593. doi:10.1021/acs.jnatprod.7b00155
Fragoso-Martínez, I., Martínez-Gordillo, M., Salazar, G. A., Jenks, A. A., García-Peña, M. R., Barrera-Aveleida, G., et al. (2018). Phylogeny of the Neotropical Sages (Salvia Subg. Calosphace, Lamiaceae) and Insights into Pollinator and Area Shifts. Plant Syst. Evol. 304, 1–13. doi:10.1007/s00606-017-1445-4
Fragoso-Serrano, M., Ortiz-Pastrana, N., Luna-Cruz, N., Toscano, R. A., Alpuche-Solís, A. G., Ortega, A., et al. (2019). Amarisolide F, an Acylated Diterpenoid Glucoside and Related Terpenoids from Salvia Amarissima. J. Nat. Prod. 82, 631–635. doi:10.1021/acs.jnatprod.8b00565
Gang, X., Fang, Z., Xian-Wen, Y., Juan, Z., Li-Xin, Y., Shen, X.-L., et al. (2011). Neo-Clerodane Diterpenoids from Salvia Dugesii and Their Bioactive Studies. Nat. Prod. Bioprospect. 1, 81–86. doi:10.1007/s13659-011-0016-6
Ghorbani, A., and Esmaeilizadeh, M. (2017). Pharmacological Properties of Salvia Officinalis and its Components. J. Tradit Complement. Med. 7, 433–444. doi:10.1016/j.jtcme.2016.12.014
Giacomelli, E., Bertrand, S., Nievergelt, A., Zwick, V., Simoes-Pires, C., Marcourt, L., et al. (2013). Cancer Chemopreventive Diterpenes from Salvia Corrugata. Phytochemistry. 96, 257–264. doi:10.1016/j.phytochem.2013.09.011
Giamperi, L., Bucchini, A., Bisio, A., Giacomelli, E., Romussi, G., and Ricci, D. (2012). Total Phenolic Content and Antioxidant Activity of Salvia Spp. Exudates. Nat. Prod. Commun. 7 (2), 201–202. doi:10.1177/1934578x1200700221
Giuliani, C., Ascrizzi, R., Corrà, S., Maleci Bini, L., Flamini, G., and Fico, G. (2017). Ultrastructural Insight into Terpene-Producing Trichomes and Essential Oil Profile in Salvia Greggii A. Gray. Flora. 236-237, 107–114. doi:10.1016/j.flora.2017.10.004
Gómez-Rivera, A., González-Cortazar, M., Herrera-Ruíz, M., Zamilpa, A., and Rodríguez-López, V. (2018). Sessein and Isosessein with Anti-inflammatory, Antibacterial and Antioxidant Activity Isolated from Salvia Sessei Benth. J. Ethnopharmacology. 217, 212–219. doi:10.1016/j.jep.2018.02.012
González-Chávez, M. M., Alonso-Castro, A. J., Zapata-Morales, J. R., Arana-Argáez, V., Torres-Romero, J. C., Medina-Rivera, Y. E., et al. (2018). Anti-inflammatory and Antinociceptive Effects of Tilifodiolide, Isolated fromSalvia tiliifoliaVahl (Lamiaceae). Drug Dev. Res. 79, 165–172. doi:10.1002/ddr.21432
González-Chávez, M. M., Ramos-Velázquez, C. S., Serrano-Vega, R., Pérez-González, C., Sánchez-Mendoza, E., and Pérez-Gutiérrez, S. (2017). Anti-inflammatory Activity of Standardized Dichloromethane Extract of Salvia Connivens on Macrophages Stimulated by LPS. Pharm. Biol. 55, 1467–1472. doi:10.1080/13880209.2017.1305423
González-Cortazar, M., Maldonado-Abarca, A., Jiménez-Ferrer, E., Marquina, S., Ventura-Zapata, E., Zamilpa, A., et al. (2013). Isosakuranetin-5-O-rutinoside: A New Flavanone with Antidepressant Activity Isolated from Salvia Elegans Vahl. Molecules. 18, 13260–13270. doi:10.3390/molecules181113260
González-Gallegos, J. G., Bedolla-García, B. Y., Cornejo-Tenorio, G., Fernández-Alonso, J. L., Fragoso-Martínez, I., García-Peña, M. R., et al. (2020). Richness and Distribution of Salvia Subg. Calosphace (Lamiaceae). Int. J. Plant Sci. 181 (8), 831–856. doi:10.1086/709133
Gul, S., Ahmad, M., Zafar, M., Bahadur, S., Sultana, S., Begum, N., et al. (2019). Taxonomic Study of Subfamily Nepetoideae (Lamiaceae) by Polynomorphological Approach. Microsc. Res. Tech. 82 (7), 1021–1031. doi:10.1002/jemt2324910.1002/jemt.23249
Harley, R. M., Atkins, S., Budantsev, A. L., Atkins, S., Conn, B. J., Budantsev, A. L., et al. (2004). Labiatae. The Families and Genera of Vascular Plants. 7, 167–275. doi:10.1007/978-3-642-18617-2_11
Hernández-Pérez, T., Valverde, M. E., Orona-Tamayo, D., and Paredes-Lopez, O. (2020). Chia (Salvia Hispanica): Nutraceutical Properties and Therapeutic Applications. Proceedings. 53 (17), 1–5. doi:10.3390/proceedings2020053017
Herrera-Ruiz, M., García-Beltrán, Y., Mora, S., Díaz-Véliz, G., Viana, G. S., Tortoriello, J., et al. (2006). Antidepressant and Anxiolytic Effects of Hydroalcoholic Extract from Salvia Elegans. J. Ethnopharmacol. 107, 53–58. doi:10.1016/j.jep.2006.02.003
Houšť, J., Spížek, J., and Havlíček, V. (2020). Antifungal Drugs. Metabolites. 10 (3), 106. doi:10.3390/metabo10030106
Hu, G. X., Takano, A., Drew, B. T., Liu, E. D., Soltis, D. E., Soltis, P. S., et al. (2018). Phylogeny and Staminal Evolution of Salvia (Lamiaceae, Nepetoideae) in East Asia. Ann. Bot. 122, 649–668. doi:10.1093/aob/mcy104
Hu, R., Cao, Q., Sun, Z., Chen, J., Zheng, Q., and Xiao, F. (2018). A Novel Method of Neural Differentiation of PC12 Cells by Using Opti-MEM as a Basic Induction Medium. Int. J. Mol. Med. 41, 195–201. doi:10.3892/ijmm.2017.3195
Jenks, A. A., and Kim, S. C. (2013). Medicinal Plant Complexes of Salvia Subgenus Calosphace: An Ethnobotanical Study of New World Sages. J. Ethnopharmacol. 146, 214–224. doi:10.1016/j.jep.2012.12.035
Jenks, A. A., Walker, J. B., and Seung-Chul, K. (2011). Evolution and Origins of the Mazatec Hallucinogenic Sage, Salvia Divinorum (Lamiaceae): a Molecular Phylogenetic Approach. J. Plant Res. 124, 593–600. doi:10.1007/s10265-010-0394-6
Jenks, A. A., Walker, J. B., and Seung-Chul, K. (2013). Phylogeny of New World Salvia Subgenus Calosphace (Lamiaceae) Based on cpDNA (psbA-trnH) and nrDNA (ITS) Sequence Data. J. Plant Res. 126, 483–496. doi:10.1007/s10265-012-0543-1
Jiang, Y.-J., Su, J., Shi, X., Wu, X.-D., Chen, X.-Q., He, J., et al. (2016). Neo -Clerodanes from the Aerial Parts of Salvia Leucantha. Tetrahedron. 72, 5507–5514. doi:10.1016/j.tet.2016.07.037
Jiménez-Ferrer, E., Hernández Badillo, F., González-Cortazar, M., Tortoriello, J., and Herrera-Ruiz, M. (2010). Antihypertensive Activity of Salvia Elegans Vahl. (Lamiaceae): ACE Inhibition and Angiotensin II Antagonism. J. Ethnopharmacology. 130, 340–346. doi:10.1016/j.jep.2010.05.013
Kabir, A., Cacciagrano, F., Tartaglia, A., Lipsi, M., Ulusoy, H. I., and Locatelli, M. (2020). “Analysis of Monoterpenes and Monoterpenoids,” in Recent Advances in Natural Product Analysis. Editor A. S. Silva (Elsevier), 274–286. doi:10.1016/B978-0-12-816455-6.00007-X
Kawahara, N., Tamura, T., Inoue, M., Hosoe, T., Kawai, K., Sekita, S., et al. (2004). Diterpenoid Glucosides from Salvia Greggii. Phytochemistry. 65, 2577–2581. doi:10.1016/j.phytochem.2004.08.012
Khalil, R., and Zheng-Guo, L. (2011). Antimicrobial Activity of Essential Oil of Salvia Officinalis L. Collected in Syria. Afr. J. Biotechnol. 10 (42), 8397–8402. doi:10.5897/AJB10.2615
Khan, A., Rehman, N.-u., AlKharfy, K. M., and Gilani, A.-H. (2011). Antidiarrheal and Antispasmodic Activities of Salvia Officinalis Are Mediated through Activation of K+ Channels. Bangladesh J. Pharmacol. 6 (2), 111–116. doi:10.3329/bjp.v6i2.9156
Kooti, W., Farokhipour, M., Asadzadeh, Z., Ashtary-Larky, D., and Asadi-Samani, M. (2016). The Role of Medicinal Plants in the Treatment of Diabetes: a Systematic Review. Electron. Physician. 8 (1), 1832–1842. doi:10.19082/1832
Kriebel, R., Drew, B. T., and Drummond, C. (2019). Tracking Temporal Shifts in Area, Biomes, and Pollinators in the Radiation of Salvia (Sages) across Continents: Leveraging Anchored Hybrid Enrichment and Targeted Sequence Data. Am. J. Bot. 106, 573–597. doi:10.1002/ajb2.1268
Lawrence, B. M. (1992). “Chemical Components of Labiatae Oils and Their Exploitation,” in Advances in Labiatae Science. Editors R. M. Harley, and T. Reynolds (Kew: Royal Botanic Gardens), 399–436.
Lee, D. Y., Ma, Z., Liu-Chen, L. Y., Wang, Y., Chen, Y., Carlezon, W. A., et al. (2005). New Neoclerodane Diterpenoids Isolated from the Leaves of Salvia Divinorum and Their Binding Affinities for Human Kappa Opioid Receptors. Bioorg. Med. Chem. 13, 5635–5639. doi:10.1016/j.bmc.2005.05.054
León-García, I., Rodríguez-Leyva, E., Ortega-Arenas, L. D., and Solìs-Aguilar, J. F. (2012). Insecticide Susceptibility of Spodoptera Frugiperda (J.E. Smith) (Lepidoptera: Noctuidae) Associated with Turfgrass at Quintana Roo, México. Agrociencia. 46 (3), 279–287.
Li, L. W., Qi, Y. Y., Liu, S. X., Wu, X. D., and Zhao, Q. S. (2018). Neo-clerodane and Abietane Diterpenoids with Neurotrophic Activities from the Aerial Parts of Salvia Leucantha Cav. Fitoterapia. 127, 367–374. doi:10.1016/j.fitote.2018.03.007
Li, Q.-Q., Li, M.-H., Yuan, Q.-J., Cui, Z.-H., Huang, L.-Q., and Xiao, P.-G. (2013). Phylogenetic Relationships ofSalvia(Lamiaceae) in China: Evidence from DNA Sequence Datasets. Jnl Sytematics Evol. 51 (2), 184–195. doi:10.1111/j.1759-6831.2012.00232.x
Li, R., Morris-Natschke, S. L., and Lee, K. H. (2016). Clerodane Diterpenes: Sources, Structures, and Biological Activities. Nat. Prod. Rep. 33, 1166–1226. doi:10.1039/c5np00137d
Lima, R. K., Cardoso, M. D. G., Andrade, M. A., Guimarães, P. L., Batista, L. R., and Nelson, D. L. (2012). Bactericidal and Antioxidant Activity of Essential Oils from Myristica Fragrans Houtt and Salvia Microphylla H.B.K. J. Am. Oil Chem. Soc. 89, 523–528. doi:10.1007/s11746-011-1938-1
Ludwiczuk, A., Skalicka-Woźniak, K., and Georgiev, M. I. (2017). “Terpenoids,” in Pharmacognosy. Editors S. Badal, and R. Delgoda (Boston: Academic Press), 233–266.
Maione, F., Bonito, M. C., Colucci, M., Cozzolino, V., Bisio, A., Romussi, G., et al. (2009). First Evidence for an Anxiolytic Effect of a Diterpenoid from Salvia Cinnabarina. Nat. Prod. Commun. 4, 469–472. doi:10.1177/1934578x0900400404
Martínez, M. A., Evangelista, V., Mendoza, M., Morales, G., Toledo, G., and Wong, A. (1995). Catálogo de Plantas útiles de la Sierra Norte de Puebla, México. Cuadernos. 27, 9–303.
Martínez, M. (1975). Flora medicinal del estado de México. Gobierno del estado de México. México: Toluca.
Martínez-Gordillo, M. J., Bedolla-García, B., Cornejo-Tenorio, G., Fragoso-Martínez, I., Fragoso-Martínez, I., García-Peña, M. d. R., et al. (2017). Lamiaceae de México. Bot. Sci. 95, 780–806. doi:10.17129/botsci.1871
Martínez-Vázquez, M., Miranda, P., Valencia, N. A., Torres, M. L., Miranda, R., Cárdenas, J., et al. (1998). Antimicrobial Diterpenes from Salvia Reptans. Pharm. Biol. 36, 77–80. doi:10.1076/phbi.36.2.77.4611
Mathew, J., and Thoppil, J. E. (2011). Chemical Composition and Mosquito Larvicidal Activities of Salvia Essential Oils. Pharm. Biol. 49, 456–463. doi:10.3109/13880209.2010.523427
Mint Evolutionary Genomics Consortium (2018). Phylogenomic Mining of the Mints Reveals Multiple Mechanisms Contributing to the Evolution of Chemical Diversity in Lamiaceae. Mol. Plant 11, 1084–1096. doi:10.1016/j.mol.2018.06.002
Mohd Ali, N., Yeap, S. K., Ho, W. Y., Beh, B. K., Tan, S. W., and Tan, S. G. (2012). The Promising Future of Chia, Salvia Hispanica L. J. Biomed. Biotechnol. 2012, 171956. doi:10.1155/2012/171956
Mora, S., Millán, R., Lungenstrass, H., Díaz-Véliz, G., Morán, J. A., Herrera-Ruiz, M., et al. (2006). The Hydroalcoholic Extract of Salvia Elegans Induces Anxiolytic- and Antidepressant-like Effects in Rats. J. Ethnopharmacol. 106, 76–81. doi:10.1016/j.jep.2005.12.004
Moreno-Pérez, G. F., González-Trujano, M. E., Martínez-Gordillo, M. J., San Miguel-Chávez, R., Basurto-Peña, F. A., Dorazco-González, A., et al. (2019). Amarisolide A and Pedalitin as Bioactive Compounds in the Antinociceptive Effects of Salvia Circinata. Bot. Sci. 97, 355–365. doi:10.17129/botsci.2187
Muñoz, L. A., Cobos, A., Diaz, O., and Aguilera, J. M. (2013). Chia Seed (Salvia Hispanica): an Ancient Grain and a New Functional Food. Food Rev. Int. 29, 394–408. doi:10.1080/87559129.2013.818014
Nair, V. P., and Hunter, J. M. (2004). Anticholinesterases and Anticholinergic Drugs. Continuing Education Anaesth. Crit. Care Pain. 4 (5), 164–168. doi:10.1093/bjaceaccp/mkh045
Nani, A., Murtaza, B., Sayed Khan, A., Khan, N. A., and Hichami, A. (2021). Antioxidant and Anti-inflammatory Potential of Polyphenols Contained in Mediterranean Diet in Obesity: Molecular Mechanisms. Molecules. 26 (4), 985. doi:10.3390/molecules26040985
Naranjo, M. (2012). Etnobotánica de las Plantas vasculares de San Andrés Chicahuaxtla, Putla, Oaxaca. [Tesis profesional]. México, D. F.: FES Zaragoza, UNAM.
Navarro, L., and Avendaño, S. (2002). Flora útil del municipio de Astacinga, Veracruz, México. Polibotánica 14, 67–84.
Nishino, K., Someya, K., Ksouri, R., Ishikawa, T., Isoda, H., Irie, K., et al. (2021). Abietane Diterpenoids from Salvia Officinalis Leaves as Aryl Hydrocarbon Receptor Ligands. Phytochemistry Lett. 41, 78–82. doi:10.1016/j.phytol.2020.11.006
Ortiz-Mendoza, N., Zavala-Ocampo, L. M., Martínez-Gordillo, M. J., González-Trujano, M. E., Peña, F. A. B., Rodríguez, I. J. B., et al. (2020). Antinociceptive and Anxiolytic-like Effects of a Neo-Clerodane Diterpene from Salvia Semiatrata Aerial Parts. Pharm. Biol. 58, 620–629. doi:10.1080/13880209.2020.1784235
Paradis, E., and Schliep, K. (2019). Ape 5.0: an Environment for Modern Phylogenetics and Evolutionary Analyses in R. Bioinformatics. 35, 526–528. doi:10.1093/bioinformatics/bty633
Pereda-Miranda, R., Hernández, L., and López, R. (1992). A Novel Antimicrobial Abietane-type Diterpene from Salvia Albocaerulea. Planta Med. 58 (2), 223–224. doi:10.1055/s-2006-961436
Pereira, O. R., Catarino, M. D., Afonso, A. F., Silva, A. M. S., and Cardoso, S. M. (2018). Salvia Elegans, Salvia Greggii and Salvia Officinalis Decoctions: Antioxidant Activities and Inhibition of Carbohydrate and Lipid Metabolic Enzymes. Molecules. 23 (12), 3169. doi:10.3390/molecules23123169
Pérez Gutiérrez, S., Zavala Mendoza, D., Soto Peredo, C., Sánchez Sánchez, O., and Zavala Sánchez, M. A. (2014). Evaluation of the Anti-diarrheal Activity ofSalvia Connivens. Pharm. Biol. 52, 1467–1470. doi:10.3109/13880209.2014.898076
Pérez-Gutiérrez, S., Zavala-Mendoza, D., Hernández-Munive, A., Mendoza-Martínez, Á., Pérez-González, C., and Sánchez-Mendoza, E. (2013). Antidiarrheal Activity of 19-deoxyicetexone Isolated from Salvia Ballotiflora Benth in Mice and Rats. Molecules 18, 8895–8905. doi:10.3390/molecules18088895
Pineda-Ramírez, N., Calzada, F., Alquisiras-Burgos, I., Medina-Campos, O. N., Pedraza-Chaverri, J., Ortiz-Plata, A., et al. (2020). Antioxidant Properties and Protective Effects of Some Species of the Annonaceae, Lamiaceae, and Geraniaceae Families against Neuronal Damage Induced by Excitotoxicity and Cerebral Ischemia. Antioxidants (Basel) 9, 253. doi:10.3390/antiox9030253
Posadzki, P., Watson, L. K., and Ernst, E. (2013). Adverse Effects of Herbal Medicines: an Overview of Systematic Reviews. Clin. Med. (Lond). 13 (1), 7–12. doi:10.7861/clinmedicine.13-1-7
Purushothaman, B., Srinivasan, R. P., Suganthi, P., Ranganathan, B., Gimbun, J., and Shanmugam1, K. (2018). A Comprehensive Review on Ocimum Basilicum. J. Nat. Remedies. 18 (3), 71–85. doi:10.18311/jnr/2018/21324
R Core Team (2021). R: A Language and Environment for Statistical Computing. Vienna: R Foundation for Statistical Computing. Available at: https://www.R-project.org/.
Ramamoorthy, T. P., and Elliott, M. (1993). “Mexican Lamiaceae: Diversity, Distribution, Endemism and Evolution,” in Biological Diversity of Mexico Origins and Distribution. Editors T. P. Ramamoorthy, R. Bye, A. Lot, and J. Fa (New York: Oxford University Press).
Revell, L. J. (2012). Phytools: an R Package for Phylogenetic Comparative Biology (And Other Things). Methods Ecol. Evol. 3, 217–223. doi:10.1111/j.2041-210X.2011.00169.x
Rojas, L. B., Visbal, T., Morillo, M., de Rojas, Y. C., Arzola, J. C., and Usubillaga, A. (2010). The Volatile Constituents of Salvia Leucantha. Nat. Prod. Commun. 5, 937–938. doi:10.1177/1934578X1000500627
Romero, C. D., Chopin, S. F., Buck, G., Martinez, E., Garcia, M., and Bixby, L. (2005). Antibacterial Properties of Common Herbal Remedies of the Southwest. J. Ethnopharmacol. 99, 253–257. doi:10.1016/j.jep.2005.02.028
Romo-Asunción, D., Antonio Ávila-Calderón, M., Ramos-López, M. A., Barranco-Florido, J. E., Rodríguez-Navarro, S., Romero-Gomez, S., et al. (2016). Juvenomimetic and Insecticidal Activities of Senecio Salignus (Asteraceae) and Salvia Microphylla (Lamiaceae) on Spodoptera Frugiperda (Lepidoptera: Noctuidae). Fla. Entomol. 99, 345–351. doi:10.1653/024.099.0301
Romussi, G., Ciarallo, G., Bisio, A., Fontana, N., de Simone, F., de Tommasi, N., et al. (2000). A New Diterpenoid with Antispasmodic Activity from Salvia Cinnabarina. Planta Med. 67, 153–155. doi:10.1055/s-2001-11511
Salinas-Arellano, E., Pérez-Vásquez, A., Rivero-Cruz, I., Torres-Colin, R., González-Andrade, M., Rangel-Grimaldo, M., et al. (2020). Flavonoids and Terpenoids with PTP-1B Inhibitory Properties from the Infusion of Salvia Amarissima Ortega. Molecules. 25 (15), 3530. doi:10.3390/molecules25153530
Sánchez, C., Cárdenas, J., Rodríguez-Hahn, L., and Ramamoorthy, T. P. (1989). Abietane Diterpenoids of Salvia Anastomosans. Phytochemistry. 28, 1681–1684. doi:10.1016/s0031-9422(00)97824-5
Shirota, O., Nagamatsu, K., and Sekita, S. (2006). Neo-Clerodane Diterpenes from the Hallucinogenic Sage Salvia Divinorum. J. Nat. Prod. 69, 1782–1786. doi:10.1021/np060456f
Simón-Arceo, K., González-Trujano, M. E., Coffeen, U., Fernández-Mas, R., Mercado, F., Almanza, A., et al. (2017). Neuropathic and Inflammatory Antinociceptive Effects and Electrocortical Changes Produced by Salvia Divinorum in Rats. J. Ethnopharmacology. 206, 115–124. doi:10.1016/j.jep.2017.05.016
Singh, N., Baby, D., Rajguru, J. P., Patil, P. B., Thakkannavar, S. S., and Pujari, V. B. (2019). Inflammation and Cancer. Ann. Afr. Med. 18 (3), 121–126. doi:10.4103/aam.aam_56_18
Solano, C., and Blancas, J. (2018). Etnobotánica de wirikuta: uso de recursos vegetales silvestres en el desierto de San Luís Potosí, México. Etnobiología. 16 (3), 54–77.
Solares-Pascasio, J. I., Ceballos, G., Calzada, F., Barbosa, E., and Velazquez, C. (2021). Antihyperglycemic and Lipid Profile Effects of Salvia Amarissima Ortega on Streptozocin-Induced Type 2 Diabetic Mice. Molecules. 26, 947. doi:10.3390/molecules26040947
Sudaramoorthy, A., Shanmugam, G., and Shanmugam, N. (2021). Inhibitory Effect of Salvia Coccinea on Inflammatory Responses through NF-Κb Signaling Pathways in THP-1 Cells and Acute Rat Diabetes Mellitus. Acta Histochem. 123, 151735. doi:10.1016/j.acthis.2021.151735
Tada, M., Okuno, K., Chiba, K., Ohnishi, E., and Yoshii, T. (1994). Antiviral Diterpenes from Salvia Officinalis. Phytochemistry. 35 (2), 359–541. doi:10.1016/S0031-9422(00)94798-8
Tenore, G. C., Ciampaglia, R., Arnold, N. A., Piozzi, F., Napolitano, F., Rigano, D., et al. (2011). Antimicrobial and Antioxidant Properties of the Essential Oil of Salvia Lanigera from Cyprus. Food Chem. Toxicol. 49, 238–243. doi:10.1016/j.fct.2010.10.022
Thurston, S., Hite, G. L., Petry, A. N., and Ray, S. D. (2015). Antiprotozoal Drugs. Side Effects Drugs Annu. 37, 321–327. doi:10.1016/bs.seda.2015.08.008
Tlacomulco-Flores, L. L., Déciga-Campos, M., González-Trujano, M. E., Carballo-Villalobos, A. I., and Pellicer, F. (2020). Antinociceptive Effects of Salvia Divinorum and Bioactive Salvinorins in Experimental Pain Models in Mice. J. Ethnopharmacol. 248, 112276. doi:10.1016/j.jep.2019.112276
Topçu, G. (2006). Bioactive Triterpenoids from Salvia Species. J. Nat. Prod. 69, 482–487. doi:10.1021/np0600402
Ullah, R., Nadeem, M., Khalique, A., Imran, M., Mehmood, S., Javid, A., et al. (2016). Nutritional and Therapeutic Perspectives of Chia (Salvia Hispanica L.): a Review. J. Food Sci. Technol. 53 (4), 1750–1758. doi:10.1007/s13197-015-1967-0
Uttara, B., Singh, A. V., Zamboni, P., and Mahajan, R. T. (2009). Oxidative Stress and Neurodegenerative Diseases: a Review of Upstream and Downstream Antioxidant Therapeutic Options. Curr. Neuropharmacol. 7, 65–74. doi:10.2174/157015909787602823
Valdés, L. J., Chang, H. M., Visger, D. C., and Koreeda, M. (2001). Salvinorin C, a New Neoclerodane Diterpene from a Bioactive Fraction of the Hallucinogenic Mexican Mint Salvia Divinorum. Org. Lett. 3, 3935–3937. doi:10.1021/ol016820d
Van Beek, T. A., and Joulain, D. (2017). The Essential Oil of patchouli,Pogostemon Cablin: A Review. Flavour Fragr J. 33 (6), 6–51. doi:10.1002/ffj.3418
Villalta, G., Salinas, M., Calva, J., Bec, N., Larroque, C., Vidari, G., et al. (2021). Selective Buche Inhibitory Activity, Chemical Composition, and Enantiomeric Content of the Essential Oil from Salvia Leucantha Cav. Collected in Ecuador. Plants (Basel). 10, 1169. doi:10.3390/plants10061169
Villavicencio, M. A., and Pérez, B. (2002). Plantas útiles del estado de Hidalgo II. Hidalgo: PachucaUAEH.
Walker, J. B., and Sytsma, K. J. (2007). Staminal Evolution in the Genus Salvia (Lamiaceae): Molecular Phylogenetic Evidence for Multiple Origins of the Staminal Lever. Ann. Bot. 100, 375–391. doi:10.1093/aob/mcl176
Walker, J. B., Sytsma, K. J., Treutlein, J., and Wink, M. (2004). Salvia (Lamiaceae) Is Not Monophyletic: Implications for the Systematics, Radiation, and Ecological Specializations of Salvia and Tribe Mentheae. Am. J. Bot. 91, 1115–1125. doi:10.3732/ajb.91.7.1115
Wester, P., and Classen-Bockhoff, R. (2007). Floral Diversity and Pollen Transfer Mechanisms in Bird-Pollinated Salvia Species. Ann. Bot. 100, 401–421. doi:10.1093/aob/mcm036
WHO. ICD-11. International Classification of Diseases 11th Revision (2021). The Global Standard for Diagnostic Health Information. Available at: https://icd.who.int/en.
Will, M., and Claßen-Bockhoff, R. (2017). Time to Split Salvia s.L. (Lamiaceae) - New Insights from Old World Salvia Phylogeny. Mol. Phylogenet. Evol. 109, 33–58. doi:10.1016/j.ympev.2016.12.041
Wu, Y. B., Ni, Z. Y., Shi, Q. W., Dong, M., Kiyota, H., Gu, Y. C., et al. (2012). Constituents from Salvia Species and Their Biological Activities. Chem. Rev. 112, 5967–6026. doi:10.1021/cr200058f
Zavala-Gómez, C. E., Zamora-Avella, D., Luis Rodríguez-Chávez, J., Zavala-Sánchez, M. Á., Campos-Guillén, J., Moustapha Bah, M., et al. (2021). Bioactivity of 1,8-Cineole and Essential Oil of Salvia Keerlii (Lamiaceae) against Spodoptera Frugiperda. Southwest. Entomol. 46, 385–396. doi:10.3958/059.046.0209
Zavala-Sánchez, M. A., Gutiérrez, S. P., Romo-Asunción, D., Cárdenas-Ortega, N. C., and Ramos-López, M. A. (2013). Activity of FourSalviaSpecies AgainstSpodoptera frugiperda(J.E. Smith) (Lepidoptera: Noctuidae). Southwest. Entomologist. 38, 67–73. doi:10.3958/059.038.0107
Zhao, Q., Chen, X. Y., and Martin, C. (2016). Scutellaria Baicalensis, the golden Herb from the Garden of Chinese Medicinal Plants. Sci. Bull. (Beijing). 61 (18), 1391–1398. doi:10.1007/s11434-016-1136-5
Keywords: abietane, bioactive compounds, clerodane, diterpene, mexican traditional medicine
Citation: Ortiz-Mendoza N, Aguirre-Hernández E, Fragoso-Martínez I, González-Trujano ME, Basurto-Peña FA and Martínez-Gordillo MJ (2022) A Review on the Ethnopharmacology and Phytochemistry of the Neotropical Sages (Salvia Subgenus Calosphace; Lamiaceae) Emphasizing Mexican Species. Front. Pharmacol. 13:867892. doi: 10.3389/fphar.2022.867892
Received: 01 February 2022; Accepted: 28 March 2022;
Published: 19 April 2022.
Edited by:
Marie Jeanne Mukazayire, University of Rwanda, RwandaReviewed by:
Concepción Obon, Miguel Hernández University of Elche, SpainSamad Nejad Ebrahimi, Shahid Beheshti University, Iran
Copyright © 2022 Ortiz-Mendoza, Aguirre-Hernández, Fragoso-Martínez, González-Trujano, Basurto-Peña and Martínez-Gordillo. This is an open-access article distributed under the terms of the Creative Commons Attribution License (CC BY). The use, distribution or reproduction in other forums is permitted, provided the original author(s) and the copyright owner(s) are credited and that the original publication in this journal is cited, in accordance with accepted academic practice. No use, distribution or reproduction is permitted which does not comply with these terms.
*Correspondence: Eva Aguirre-Hernández, ZXZhX2FndWlycmVAY2llbmNpYXMudW5hbS5teA==; Itzi Fragoso-Martínez, aXR6aS5mcmFnb3NvQGluZWNvbC5teA==