- 1Department of Pediatrics, Institute for Maternal and Child Health—IRCCS Burlo Garofolo, Trieste, Italy
- 2Department of Medical, Surgical and Health Sciences, University of Trieste, Trieste, Italy
- 3Department of Clinical and Experimental Medicine, University of Pisa, Pisa, Italy
- 4Transfusion Medicine Department, Azienda Sanitaria Universitaria “Giuliano Isontina”, Trieste, Italy
- 5Laboratory for Hygiene and Public Health, University Hospital of Trieste, Trieste, Italy
Background: Acyclovir represents the first-line prophylaxis and therapy for herpes virus infections. However, its pharmacokinetics in children exposes them to the risk of ineffective or toxic concentrations. The study was aimed at investigating the population pharmacokinetics (POP/PK) of intravenous (IV) acyclovir in oncologic children.
Methods: Patients (age, 8.6 ± 5.0 years, 73 males and 47 females) received IV acyclovir for prophylaxis (n = 94) and therapy (n = 26) under a therapeutic drug monitoring (i.e., minimum and maximal plasma concentrations, >0.5 and <25 mg/L, respectively). Plasma concentrations were fitted by nonlinear mixed effect modeling and a simulation of dosing regimens was performed. Findings were stratified according to an estimated glomerular filtration rate (eGFR) threshold of 250 ml/min/1.73 m2.
Results: The final 1-compartment POP/PK model showed that eGFR had a significant effect on drug clearance, while allometric body weight influenced both clearance and volume of distribution. The population clearance (14.0 ± 5.5 L/h) was consistent across occasions. Simulation of standard 1-h IV infusion showed that a 10-mg/kg dose every 6 h achieved target concentrations in children with normal eGFR (i.e., ≤250 ml/min/1.73 m2). Increased eGFR values required higher doses that led to an augmented risk of toxic peak concentrations. On the contrary, simulated prolonged (i.e., 2 and 3-h) or continuous IV infusions at lower doses increased the probability of target attainment while reducing the risk of toxicities.
Conclusion: Due to the variable pharmacokinetics of acyclovir, standard dosing regimens may not be effective in some patients. Prospective trials should confirm the therapeutic advantage of prolonged and continuous IV infusions
Introduction
Herpesvirus infections in immunocompromised patients, particularly in hematopoietic stem cell transplant (HSCT) recipients, lead to severe disease with high dissemination rates, complications, and mortality (Beyar-Katz et al., 2020). Herpes simplex virus (HSV) is a ubiquitous virus that results in lifelong infections due to its ability to alternate between lytic replication and latency (Ly et al., 2021). The worldwide prevalence of HSV-1 increases consistently with age, reaching 40% by age 15 and increasing to 60%–90% in older adults (Chayavichitsilp et al., 2009). Up to 80% of adult leukemia patients are HSV seropositive, as well as up to 80% of HSV-seropositive allogeneic HSCT recipients had post-transplant HSV reactivation (Styczynski et al., 2009; Flowers et al., 2013). In the first post-transplant year, symptomatic varicella-zoster virus (VZV) reactivation in adult recipients is described with rates of 13%–55% (Beyar-Katz et al., 2020). Similarly, 30%–33% of pediatric HSCT recipients had VZV reactivation, and 11% of these were disseminated (Fisher et al., 2008). In the current era of antiviral prophylaxis in seropositive HSCT recipients, the infection rate has decreased significantly, besides a significant reduction in mortality (Dadwal, 2019).
In pediatric patients undergoing allogeneic HSCT, surveillance algorithms, antiviral prophylaxis, or pre-emptive treatment are well established for many viruses due to the high incidence of severe systemic complications in this population (Czyzewski et al., 2019; Jaing et al., 2019). In contrast to HSCT recipients, data on systemic viral infections in children receiving chemotherapy for hematological malignancies are very limited (Buus-Gehrig et al., 2020). However, few reports confirm that children with malignant diseases who experience prolonged periods of myelosuppression due to cytotoxic chemotherapy are highly susceptible to invasive viral infections (Feldman and Lott, 1987).
Most viral reactivation in adult cancer patients during neutropenia after myelotoxic chemotherapy is due to HSV (Saral et al., 1984). However, despite the high infection rate, there is not enough evidence from randomized trials on acyclovir prophylaxis in patients with acute leukemia to establish a strong recommendation in adult and pediatric patients undergoing intensive chemotherapy (Styczynski et al., 2009).
Acyclovir (ACV) effectively prevents and treats HSV and VZV infections but demonstrates high interindividual variability in its treatment response. Indeed, ACV prophylaxis is recommended for all HSV-seropositive HSCT recipients from conditioning until engraftment or until mucositis resolves to prevent HSV reactivation during the early post-transplant period. For VZV-seropositive HSCT recipients antiviral prophylaxis is recommended for at least one year, while for VZV-seronegative HSCT recipients passive immunization is preferred (Tomblyn et al., 2009; Carreras et al., 2018). For instance, the prophylactic regimen of ACV for the European Blood and Marrow Transplant Group consists in 250 mg/m2 (or 5 mg/kg) i.v., every 12 h, while the treatment of infections needs an intensified regimen (250 mg/m2 or 5 mg/kg i.v., every 8 h for 7–10 days) (Carreras et al., 2018). Due to the time-dependent killing of ACV, plasma concentrations should be higher than 1 mg/L for at least 50% of time interval between two consecutive doses (Saiag et al., 1999), so that a minimum plasma concentrations >0.5 mg/L could be considered an appropriate target. Moreover, high peak concentrations in plasma (i.e., >50 mg/L) are associated with an increased rick of neurotoxicity (Wade and Monk, 2015), even if the correlation between high plasma concentrations and the risk of nephrotoxicity and bone marrow adverse events remains to be fully investigated.
ACV is eliminated by the kidney’s glomerular filtration and tubular secretion, has low oral bioavailability, approximately 10% in children (Carcao et al., 1998), and a short half-life, hence it requires high and repeated doses to exceed the value of the inhibitory concentration (Abdalla et al., 2020). Furthermore, ACV has high interindividual variability (Blum et al., 1982; O'Brien and Campoli-Richards, 1989), which is particularly evident in the younger patients, and related to changes in renal function during the first months after the birth and body weight across the ages (De Miranda and Blum, 1983; Zeng et al., 2009; Abdalla et al., 2020). In addition to this, the genetic status of the patient may be considered a further cause of variability of pharmacokinetics and clinical outcome, as recently demonstrated for the NUDT15 polymorphism (Nishii et al., 2021).
Therefore, information regarding ACV optimal use in children with malignancies is restricted because pharmacokinetic data are limited in this population (Zeng et al., 2009; Abdalla et al., 2020). This study aims to characterize the pharmacokinetics of ACV following intravenous (IV) administration and to evaluate the adequacy of current dose regimens for children with malignancy undergoing myelosuppressive chemotherapy or HSCT. Additionally, the study aims to explore alternative dosing regimens that could be more effective and tolerable.
Materials and Methods
Study Design and Population
This prospective, single-center, observational study was carried out at the pediatric Onco-Hematology Department and pediatric Bone Marrow Transplant Center of the Institute for Maternal and Child Health—IRCCS “Burlo Garofolo,” Trieste, Italy, from 2011 to 2020. The Institutional Review Board of the IRCCS Burlo Garofolo (reference no. IRB RC 10/20) approved the protocol, and the study was conducted following the Declaration of Helsinki (Clinicaltrials.gov code: NCT05198570). The patients’ parents gave their written consent to collect and use personal data for research purposes. From January 2011 to December 2020, consecutive patients aged 0–18 years, affected by hematological malignancies, undergoing ACV prophylaxis or treatment for HSV-VZV infection during allogeneic HSCT or ACV treatment during high-intensity chemotherapy were included in this study. All patients underwent ACV therapeutic drug monitoring (TDM), and consequent dose adjustment was applied to maintain minimum (Cmin) and maximum (Cmax) plasma concentrations >0.5 mg/L and <25 mg/L, respectively, as per laboratory practice. Data collection included patient demographic characteristics as gender, age, weight, height, body mass index, body surface area, serum creatinine in addition to primary diagnosis, treatment for primary diagnosis, ACV dose, administration interval, concomitant medications, duration of ACV treatment, and cause of treatment interruption. The Schwartz formula determined the estimated glomerular filtration rate (eGFR) for each patient (Schwartz et al., 1976).
ACV Administration Regimens and Blood Sampling
ACV was administered every 6–8 h intravenously (Acyclovir Recordati®, Biologici Italia Laboratories S.r.l., Masate, Milan, Italy) over 60 min infusion, with median (range) starting daily doses equal to 40.7 (15.6–136.7) mg/kg/day. The prescribed doses refer to the local protocols and depend on the age, treatment indication, and renal clearance. These differences in dosage reflect systematic changes in institutional practice over the ten years covered by the study. The first pharmacokinetic assessment (two samples) was performed after at least four days of ACV administration when the steady state was achieved. In particular, blood samples were withdrawn 10 min before (trough levels, Cmin) or 30 min after IV infusion (maximum concentration, Cmax). For some patients, blood samples were collected on several occasions. Blood samples were centrifuged, and plasma concentrations of ACV were measured by a liquid chromatography-tandem mass spectroscopy (LC-MS/MS) performed in multiple reaction monitoring mode (Kanneti et al., 2009). Briefly, after deproteinization, calibration standards, quality controls (both prepared in human blank plasma) and patients’ plasma samples (using fluconazole as internal standard, IS) were prepared by solid phase extraction (Oasis HLB Vac Cartridge, Waters, Milford, CT, United States). Acyclovir and IS were eluted through a C18 column with a mobile phase consisting of 0.1% formic acid solution and methanol (30:70 v/v) at a flow rate of 0.8 ml/min in isocratic conditions. The LC-MS apparatus worked in positive ion detection, and quantification was performed in multiple reactions monitoring (MRM) of transition ions m/z 226.3 > 152.1 and m/z 306.9 > 219.9 for ACV and IS, respectively.
Pharmacokinetic Modeling and Simulation
The population pharmacokinetics (POP/PK) analysis was performed on the available plasma concentrations using a nonlinear mixed effect modeling approach using NONMEM software vers. 7.4 (ICON, Dublin, Ireland)1 and the packages PsN and Xpose (Jonsson and Karlsson, 1999; Lindbom et al., 2004). One- and two-compartment models with first-order elimination were tested, while the residual error was assayed as an additive, proportional and mixed model. Interoccasion variability (IOV) was evaluated for pharmacokinetic parameters. The introduction of covariates within the model was guided by their range of values in the dataset and their possible mechanistic involvement in ACV pharmacokinetics. Overall, a decrease in the objective function value (OFV) greater than 3.84 points (p < 0.05) and 6.63 points (p < 0.01) in the forward inclusion and backward exclusion, respectively, were considered during the model development. The conditional weighted residuals (CWRES) were calculated (Hooker et al., 2007), and the goodness-of-fit (GOF) plots, the precision of parameter estimates, η- and ε-shrinkage values were evaluated for each model. A bootstrap analysis and a prediction-corrected visual predictive check (pcVPC) were used to judge the final model (Bergstrand et al., 2011). The terminal elimination half-life (t1/2) was calculated as t1/2 = ln (2)/kel, where kel is the individual empirical Bayes estimate of the ACV elimination constant.The software NONMEM was used to simulate different drug administration schedules based on the final POP/PK model. The simulation included dosages in the range 15–30 mg/kg administered as standard (i.e., 1 h), prolonged (i.e., 2 and 3 h) and continuous IV infusions every 6 and 8 h in 1,000 individuals for each dose level. Cmin values higher than 0.56 or 1.156 mg/L in at least 50% of patients or Cmax values > 25 mg/L in less than one-fourth of patients were considered as the desired pharmacokinetic targets in patients grouped according to the presence of augmented renal clearance (ARC) or not (i.e., eGFR >250 or ≤250 ml/min/1.73 m2, respectively) (Abdalla et al., 2020).
Statistical Analysis
Data are presented as mean ± standard deviation (SD), median and minimum-maximum range, or 95% confidence interval (95% CI) according to the parameter described. Statistical computations (i.e., unpaired Student’s t-test with Welch’s correction, Mann-Whitney test, ANOVA, Fisher exact test) were performed using Prism 5.0 (GraphPad Software Inc., La Jolla, CA, United States) after checking for normal distribution of values (when appropriate) by the Kolmogorov-Smirnov test, and the significance level was set at p < 0.05.
Results
Patients and Acyclovir Monitoring
The current database included 73 boys and 47 girls (age, mean ± SD, 8.0 ± 5.2 and 9.5 ± 4.6 years, respectively; Table 1). Most patients were affected by hematological malignancies and addressed to allogenic HSCT (Figure 1). Twenty-six patients received ACV to treat a viral infection caused by HSV or VZV, whereas in the remaining 94 children the drug was administered for prophylaxis. Along with the treatment, blood samples were withdrawn to perform ACV monitoring of plasma concentrations in 120, 54, and 13 patients on a first, second, and third occasion, respectively. On the first occasion, measured Cmax accounted for 7.6 ± 5.4 mg/L, while Cmin values were 1.0 ± 1.1 and 0.85 ± 1.3 mg/L for the 6-h and 8-administration schedule of ACV, respectively, without significant differences across occasions (Figure 2). The dose was changed in 47 children (increased in 39) and 8 patients (increased in 7) at the second and third occasions, respectively.
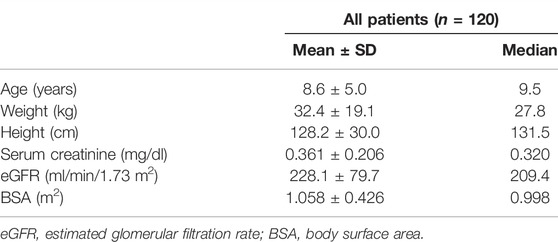
TABLE 1. Characteristics of the 120 patients enrolled in the present study. There were not statistically significant differences between males and females.
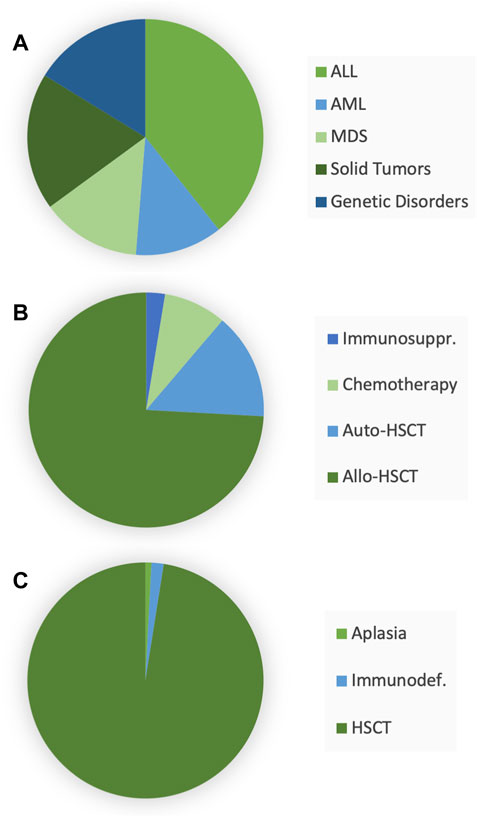
FIGURE 1. Characteristics of the 120 enrolled patients: initial diagnosis (A), first-line therapy (B), and reasons for acyclovir administration (C). Abbreviations: ALL, acute lymphoblastic leukemia; AML, acute myeloblastic leukemia; MDS, myelodysplastic syndrome; HSCT, hematopoietic stem cell transplantation.
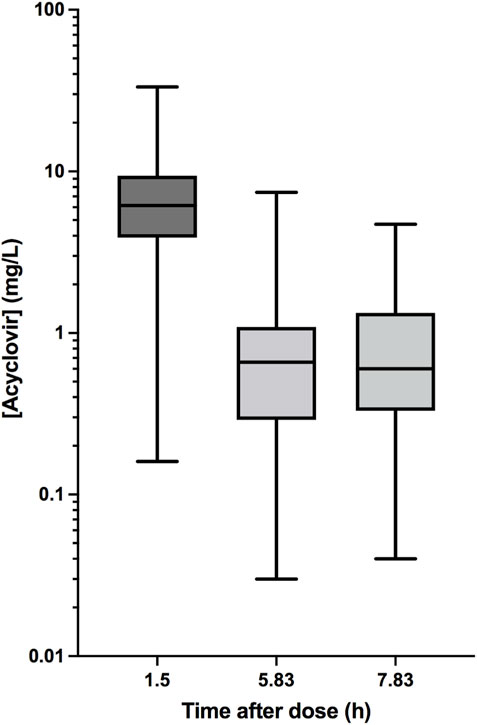
FIGURE 2. Box-and-whiskers plot of single measured plasma concentrations of acyclovir 30 min after the end of the infusion (1.5 h) and 10 min before the next dose in 6-h (5.83 h) and 8-h (7.83 h) regimens.
In 26 patients (21.7%) who received ACV to treat herpetic infections, doses were 381.3 ± 199.2 mg (median, 400 mg) on the first occasion. In 11 and 4 patients, a second and a third occasions were available, with doses of 432.5 ± 150.9 mg (median 400 mg) and 500.0 ± 163.3 mg (median 500 mg), respectively. Among the measured ACV plasma concentrations, 15 and 11 patients had at least one Cmin value > 0.56 and >1.156 mg/L, respectively. ACV administration was followed by a complete recovery, improvement, or infection control in approximately 75% of patients. In comparison, in the remaining individuals, the records showed a worsening of symptoms and signs (14.3% of patients) or the emergence of a further viral infection (i.e., cytomegalovirus, 9.5% of individuals). Measured Cmin values in 3 out of 4 patients with poor response to therapy were greater than 1 mg/L, while in the fourth child, the Cmin value accounted for 0.78 mg/L.
POP/PK Modeling
A total of 187 pairs (120, 54, and 13 for the first, second, and third occasion, respectively) of ACV plasma concentrations for a total of 374 plasma concentration values were available for model development that started with a 1-compartment model with additive residual error (OFV, points 1,372.979). The residual variability was best described by a proportional error model (−141.269 points), while interindividual (IIV) and interoccasion variability (IOV) in systemic clearance (CL) significantly decreased the OFV value (−197.363 and −49.596 points, respectively). Furthermore, the introduction of IIV on the volume of distribution (V) ameliorated the model (−47.866 points), whereas IOV did not likely because changes in body weight across occasions were not significant (i.e., 32.2 ± 19.1, 30.9 ± 19.8, and 32.8 ± 22.7 kg, respectively at the first, second, and third occasion) and it was not considered in the following modeling.
Among covariates with a possible effect on acyclovir pharmacokinetics, only eGFR on CL (−12.386 points) and body weight (with allometric scaling) on both CL and V (−22.811 and −12.664 points, respectively) did significantly decrease OFV; hence they were retained in the final model (Table 2). Among the GOF plots (Figure 3), the CWRES versus time after dose graph revealed an overprediction during the first few hours after the dose, which was likely dependent on the schedule of blood withdrawal, while the pcVPC plot did not entirely fit the lowest Cmin and highest Cmax values (Figure 4). However, the bootstrap analysis (with 1,000 resampled databases) resulted in good performance of the final model (Table 2), with nearly 90% of runs that ended successfully.
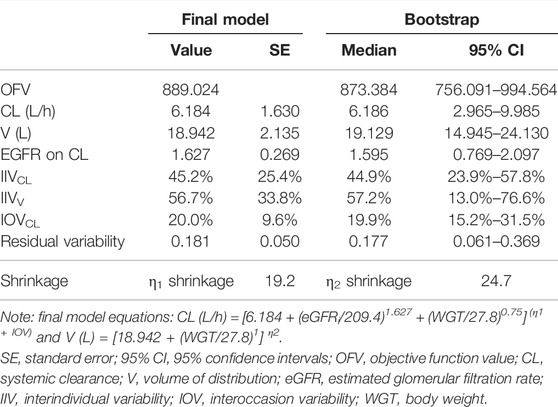
TABLE 2. Parameter values of the final model and bootstrap results obtained in 1,000 resampled databases.
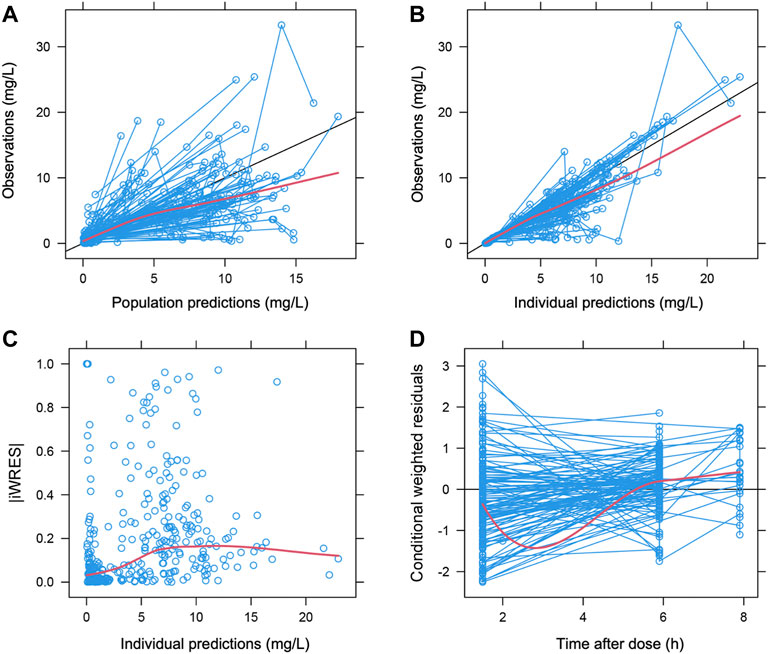
FIGURE 3. Goodness-of-fit plots. Population (A) and individual (B) prediction values vs. observations. (C), absolute values of individual weighted residuals (iWRES) error vs. individual predictions and (D) conditional weighted residuals vs. time. Symbols, individual plasma concentrations of acyclovir. Red lines, regression, and Loess lines. Black line, line of identity.
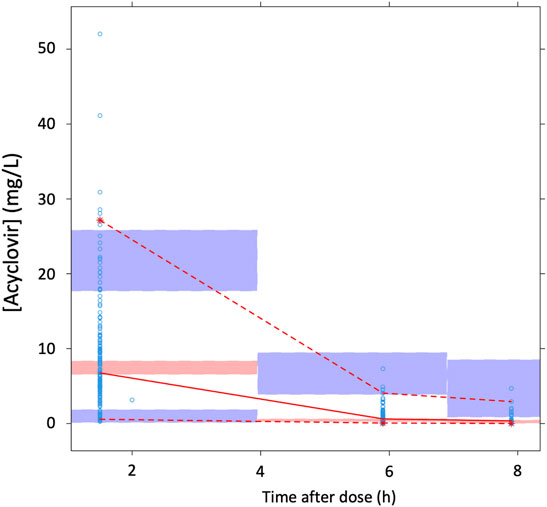
FIGURE 4. The prediction-corrected visual predictive check based on the present data. Open circles, individual plasma concentrations of acyclovir together with their median and 5th-95th percentiles (solid and dashed red lines, respectively). The 95% confidence intervals of the simulated median (pale pink) and 5th-95th percentiles (pale blue) are showed.
The analysis of PK parameters of the final model (showed in Table 3) did not demonstrate significant gender-based differences. The terminal t1/2 accounted for 1.364 ± 0.614 h, while IIVCL and IOVCL values were 46.4% and 20.0%, respectively.
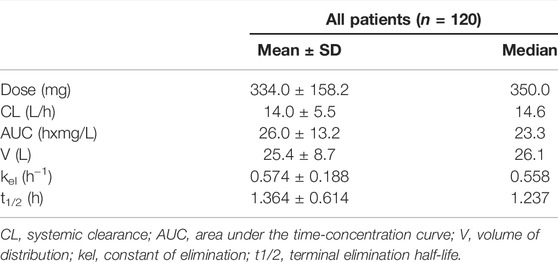
TABLE 3. Values of the pharmacokinetic parameter obtained in the present 120 children by the final POP/PK model. There were not statistically significant differences between males and females.
The present CL and V values for a typical individual do match those previously obtained (Abdalla et al., 2020), despite the higher eGFR values calculated in the present patients (median, 209.4 ml/min/1.73 m2) with respect to previous ones (164 ml/min/1.73 m2).
More interestingly, the analysis of PK parameters between the first and the second occasion did not reveal significant differences for any pharmacokinetic parameter considered (Table 4). In agreement with these results, an additional analysis of ACV PK in 13 patients did not show significant differences across the three occasions (Table 5).

TABLE 4. Pharmacokinetics of ACV across two occasions. Values are presented as mean ± SD (median) values.
POP/PK Simulation
The present simulation was based on the median values of covariates that were included in the final model. In particular, individual values of eGFR were randomly calculated from a distribution similar to that obtained from the present population of patients (i.e., mean and standard deviation values of 228.1 and 79.7 ml/min/1.73 m2, respectively), while body weight was fixed at 27.8 kg.
In agreement with a previous study (Abdalla et al., 2020), an ACV dose of 20 mg/kg every 6 h may ensure a Cmin value > 0.56 and >1.125 mg/L in approximately 62.5% and 50.9% of the patients, respectively, while 28.6% of patients may experience a Cmax value > 25 mg/L when the eGFR was ≤250 ml/min/1.73 m2 (Table 6). On the contrary, at eGFR values > 250 ml/min/1.73 m2, standard dosing regimens had a lower probability of target attainment, especially when the time interval between doses was 8 h (Table 6). The increase in dose to improve target attainment may expose the patients to an augmented risk of high peak plasma concentrations.
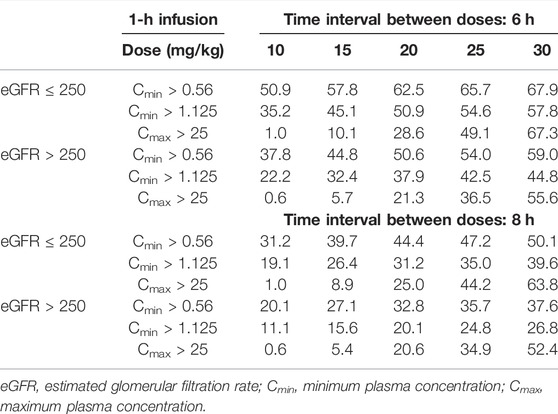
TABLE 6. Results from simulated regimens consisting in ACV dose ranging from 10 up to 30 mg/kg infused in 1 h (standard regimen). The percentages of patients who achieved Cmin values > 0.56 and >1.125 mg/L or Cmax values > 25 mg/L are showed according to an eGFR threshold of 250 ml/min/m2. Each regimen consisted of 1,000 simulated patients.
The following simulation of prolonged 2- and 3-h IV infusions resulted in an increased probability of ACV Cmin values > 0.56 and >1.125 mg/L (Figure 5; Table 7). Of note, the reduced rate of infusion resulted in a lower probability of Cmax values > 25 mg/L. In line with these results, simulated continuous infusions at doses of 10 mg/kg every 8 h were associated with Cmin values > 0.56 mg/L in all patients regardless of the eGFR value. When the desired Cmin threshold was set at 1.125 mg/L, 96.1% and 90.5% of patients achieved the target when the eGFR was ≤250 (Cmin = 3.18 ± 1.69 mg/L) and >250 ml/min/1.73 m2 (Cmin = 2.51 ± 1.26 mg/L), respectively.
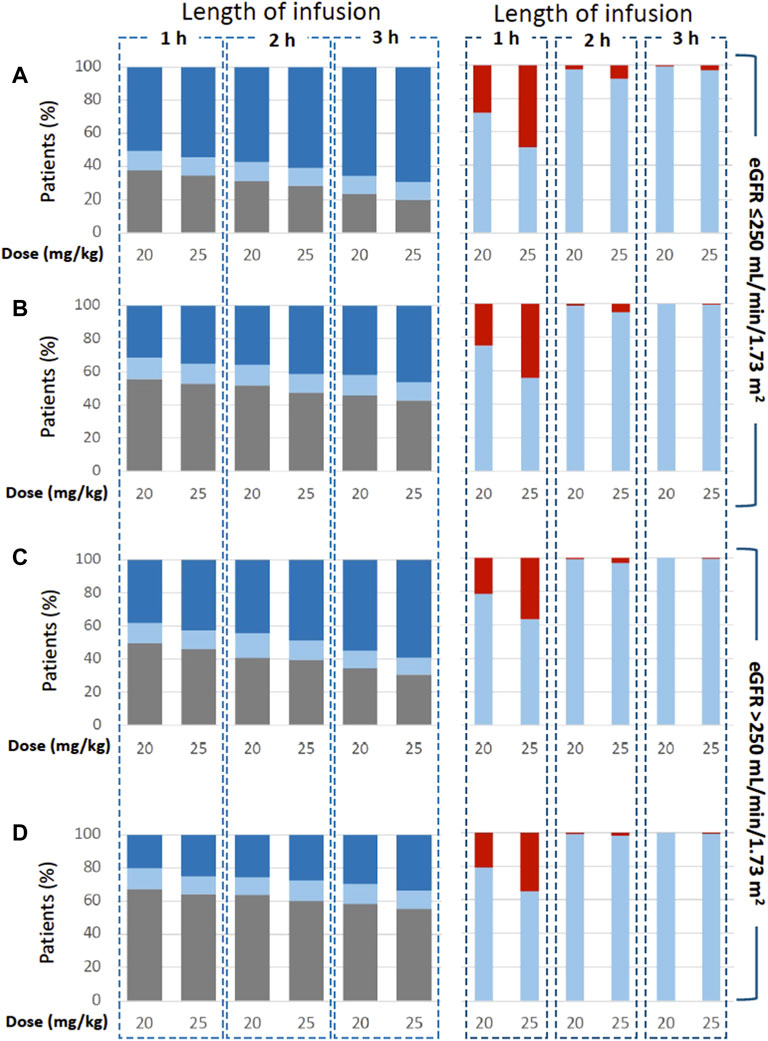
FIGURE 5. Simulated regimens consisting in ACV 20 or 25 mg/kg administered as 1-h, 2-h and 3-h infusion every 6 h [graphs (A,C)] and 8 h [graphs (B,D)]. Plots show the probability of target attainment (on the left) according to Cmin values < 0.56 (grey), ≥0.56-<1.125 (pale blue) and >1.125 mg/L (blue). Moreover, graphs show the probability of achieving Cmax values < 25 (pale blue) and >25 mg/L (red).
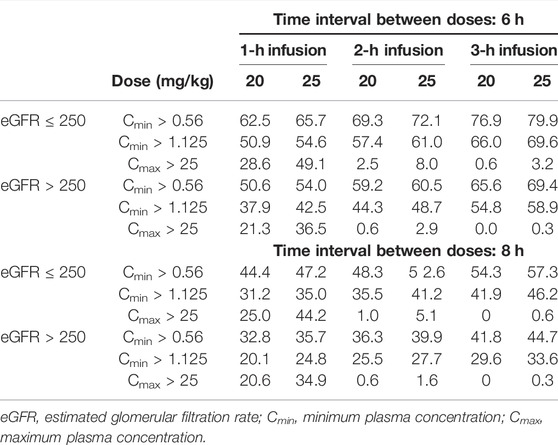
TABLE 7. Results from simulated regimens consisting in ACV doses of 20 and 25 mg/kg infused in 1 h (standard regimen) or in prolonged infusions (2 and 3 h). The percentages of patients who achieved Cmin values > 0.56 and >1.156 mg/L or Cmax values > 25 mg/L are showed according to an eGFR threshold of 250 ml/min/m2. Each regimen consists of 1,000 simulated patients.
Discussion
The present study was performed in a homogeneous population of oncologic paediatric patients receiving IV ACV for prophylaxis or to treat HSV and VZV infections. The final findings of the POP/PK analysis demonstrate a high variability between and within individuals that warrants the adoption of therapeutic drug monitoring. Furthermore, the simulation suggested that prolonged IV infusions could increase concentrations in the therapeutic range while reducing the risk of toxic peak concentrations.
ACV is a fundamental agent for prophylaxis and treatment of herpes virus infections, especially in HSCT patients or those who received high-dose antineoplastic chemotherapy like those enrolled in the present study. However, appropriate use of ACV depends on maintaining effectiveness through plasma concentrations above the threshold of sensitivity of known viral strains while reducing the risk of toxic concentrations identified in plasma levels >25 mg/L (Abdalla et al., 2020). The achievement of those goals may be severely influenced by patients’ characteristics, especially in children. Indeed, previous paediatric studies have clearly demonstrated that the variability in ACV pharmacokinetics is better explained by the renal function rather than dose (De Miranda and Blum, 1983), meaning that including plasma creatinine or eGFR within the model reduced IIVCL and predicted the individual values with respect to observations (Zeng et al., 2009). In addition to this, the variability in both CL and Vd was significantly associated with body weight (Zeng et al., 2009; Abdalla et al., 2020). The present findings do confirm those conclusions in the largest population of enrolled patients published so far, showing a large interpatient and intrapatient variability that requires the adoption of TDM protocols. In the present study the unexplained IIVCL (46.3%) was accompanied by a IOVCL that accounted for 20.0%. Together, these values strengthen the benefit to include IOV in the estimation of individual PK parameters (Karlsson and Sheiner, 1993), and do sustain the monitoring of ACV concentrations during chemotherapy, because the time-varying covariates help to explain the variability across different occasions. Since it is possible that “the magnitude of IOV increases with the time between study occasions” (Karlsson and Sheiner, 1993), the mean/median interval time between two consecutive occasions in the present study was 16.5/8 days (minimum-maximum range, 2–165 days), likely explaining the lower IOVCL with respect to IIVCL.
Some characteristics of the present study have to be pointed out. First of all, the number of enrolled patients did allow the analysis of patients who received IV ACV only, thus overcoming the variability associated with the oral administration of ACV or its prodrug valacyclovir. Second, the database used to develop the POP/PK model included plasma concentrations obtained at fixed time points that corresponded to those adopted in TDM protocols (Di Paolo et al., 2013) instead of dense blood sampling (Zeng et al., 2009), or random time points (Abdalla et al., 2020). The choice of the sampling scheme may depend on several factors, but ultimately the present POP/PK model returned PK estimates very similar to those published by other studies (Zeng et al., 2009; Abdalla et al., 2020). For example, the present mean values of CL (0.54 L/h/kg) and V (0.97 L/kg) were comparable to those previously reported in children with a mean bodyweight of 20 kg (Eksborg et al., 2002; Nadal et al., 2002). Analogous conclusions can be drawn for terminal t1/2 (De Miranda and Blum, 1983; Zeng et al., 2009) and the IOVCL value (20.0%), which was in agreement with the value (19.2%) found in a previous study (Zeng et al., 2009). Even the simulation part of the present study showed a concordance with previous findings. For example, ACV doses of 10–20 mg/kg administered as a conventional 1-h IV infusion every 6 h showed almost identical percentages of toxic concentrations (Abdalla et al., 2020). Moreover, the present findings are suggesting that a 20-mg/kg dose every 6 h may achieve effective concentrations in approximately 50% of patients, while the dose should be increased in children with ARC.
The study’s novelty resides in the simulation of alternative regimens of acyclovir administration. With a short terminal half-life, the maintenance of effective Cmin values is guaranteed by higher doses of acyclovir (i.e., 20 mg/kg) or more frequent dosing (i.e., every 6 h instead of 8 h). However, those high-dose-intensity regimens may result in high Cmax values that could expose the patients to the risk of toxicities, while ineffective through concentrations may be measured especially in patients with ARC (Abdalla et al., 2020). As observed for other antimicrobial drugs that have a short plasma half-life, a prolonged (i.e., 2 or 3 h) or continuous infusion together with an appropriate dose increase may allow the achievement of effective target plasma concentrations. Indeed, the simulation of a prolonged infusion of a 20 mg/kg dose resulted in an increased percentage of patients achieving the predefined PK targets for the standard 1-h IV infusion. The percentage of patients who achieve effective Cmin values sharply increased when the simulation considered a continuous infusion, even with a low dose-intensity regimen, consisting of 10-mg/kg doses every 8 h. Noteworthy, fragile patients affected by severe HSV infections were successfully cured with continuous IV infusions of acyclovir at higher doses (up to 30 mg/kg) (Engel et al., 1990; Kim et al., 2011). Interestingly, in most individuals “clinical response was seen within 72 h of continuous ACV administration” that “was well tolerated, even in patients with renal insufficiency.” Indeed, the authors did not observe any sign of toxicity, including hematological adverse reactions or deterioration in renal function.
Further studies have demonstrated that continuous infusions would prolong the time during which the ACV concentrations exceed the IC50 value for HSV and VZV and therefore may be considered a valid alternative to intermittent IV dosing (Spector et al., 1982; O'Leary et al., 2020). It is worth noting that in some cases, continuous ACV infusion was adopted to treat neonatal HSV encephalitis (Kakisaka et al., 2009; Cies et al., 2015; O’Leary et al., 2020). In particular, plasma concentrations were maintained above 3 mg/L (Cies et al., 2015) or even higher (5.5–8 mg/L) (O’Leary et al., 2020) to ensure cerebrospinal fluid concentrations of at last 1 mg/L.
Interestingly, a former study that enrolled 13 patients included 4 children who received continuous i.v., infusions of acyclovir at doses of 6.1–9.7 mg/kg/h from 7 up to 13 days (Fletcher et al., 1989). Plasma concentrations were ≥4.5 mg/L (up to 22.1 mg/L in some patients) and one child developed neutropenia, whereas none of the patients experienced renal insufficiency. In agreement with those observations, another study did not report signs or laboratory findings of systemic toxicity in 3 adolescents who received continuous i.v., infusions of ACV at doses of 7.2–28.8 mg/kg/day (Spector et al., 1982). Finally, the present simulation showed that a 10 mg/kg dose of ACV administered as a continuous infusion every 8 h allowed the achievement of therapeutic plasma concentrations in more than 90% of patients, regardless the eGFR value.
Those results do sustain the adoption of prolonged infusions (or continuous ones) because the reduced rate of drug infusion may be advantageous to decrease the risk of toxic Cmax values. In particular, the percentage of patients at risk of higher peak plasma concentrations is abated, moving from a standard 1-h infusion to prolonged or continuous infusions. Additionally, a loading dose consisting of a 30-min infusion may precede the continuous infusion to ensure the achievement of therapeutic Cmin values.
Although these premises of greater efficacy and good tolerability, the nephrotoxic effects of acyclovir in children have been associated with a variety of causes, as well as the concomitant administration of nephrotoxic drugs, a reduced eGFR at baseline, hypertension, older age, and obesity (Schreiber et al., 2008; Richelsen et al., 2018; Yalçınkaya et al., 2021). Therefore, these risk factors should be carefully evaluated, especially when dose-intense regimens were adopted (Fletcher et al., 1989).
In conclusion, the present findings confirm the high variability of ACV pharmacokinetics in immunocompromised children undergoing HSCT or myelotoxic chemotherapies, hence TDM protocols are recommended to adjust drug dosing. Indeed, standard dosing regimens seems adequate to achieve effective plasma concentrations of ACV, despite the drug could be ineffective in a variable percentage of some patients. The further increase in dosage may expose patients to an augmented risk of toxicities. Noteworthy, alternative regimens based on prolonged IV infusions (i.e., 20 mg/kg as a 3-h infusion every 6 h) or even continuous infusions (i.e., 10 mg/kg every 8 h) may increase the efficacy of ACV while reducing the risk of highest plasma peaks and sparing patients from severe toxicities. Prospective trials adopting TDM protocols are required to confirm the present findings.
Data Availability Statement
The original contributions presented in the study are included in the article/Supplementary Material, further inquiries can be directed to the corresponding author.
Ethics Statement
The studies involving human participants were reviewed and approved by the Institutional Review Board of the IRCCS Burlo Garofolo (reference no. IRB RC 10/20). Written informed consent to participate in this study was provided by the participants’ legal guardian/next of kin.
Author Contributions
NM, DN, GL, RS, EP, LS, EB and AP developed the concept and designed the study. NM, DN, EP and LS. provided study material or participants. GL, RS and AP verified the data and performed the statistical analyses. NM, EB and AP wrote the initial draft of the manuscript. All authors provided critical comments and editing, contributed to the data interpretation, reviewed the analyses of this manuscript, and approved its final version.
Conflict of Interest
The authors declare that the research was conducted in the absence of any commercial or financial relationships that could be construed as a potential conflict of interest.
Publisher’s Note
All claims expressed in this article are solely those of the authors and do not necessarily represent those of their affiliated organizations, or those of the publisher, the editors and the reviewers. Any product that may be evaluated in this article, or claim that may be made by its manufacturer, is not guaranteed or endorsed by the publisher.
Acknowledgments
The authors thank the children and their parents for participating in the present study. Moreover, a thank goes to the nurse staff and all other caregivers who participate in the study.
Footnotes
1ICON plc. NONMEM®. [Accessed 1 September 2021]. https://www.iconplc.com/innovation/nonmem/.
References
Abdalla, S., Briand, C., Oualha, M., Bendavid, M., Béranger, A., Benaboud, S., et al. (2020). Population Pharmacokinetics of Intravenous and Oral Acyclovir and Oral Valacyclovir in Pediatric Population to Optimize Dosing Regimens. Antimicrob. Agents Chemother. 64, e01426. doi:10.1128/AAC.01426-20
Bergstrand, M., Hooker, A. C., Wallin, J. E., and Karlsson, M. O. (2011). Prediction-corrected Visual Predictive Checks for Diagnosing Nonlinear Mixed-Effects Models. AAPS J. 13, 143–151. doi:10.1208/s12248-011-9255-z
Beyar-Katz, O., Bitterman, R., Zuckerman, T., Ofran, Y., Yahav, D., and Paul, M. (2020). Anti-herpesvirus Prophylaxis, Pre-emptive Treatment or No Treatment in Adults Undergoing Allogeneic Transplant for Haematological Disease: Systematic Review and Meta-Analysis. Clin. Microbiol. Infect. 26, 189–198. doi:10.1016/j.cmi.2019.09.003
Blum, M. R., Liao, S. H., and de Miranda, P. (1982). Overview of Acyclovir Pharmacokinetic Disposition in Adults and Children. Am. J. Med. 73 (1A), 186–192. doi:10.1016/0002-9343(82)90088-2
Buus-Gehrig, C., Bochennek, K., Hennies, M. T., Klingebiel, T., Groll, A. H., and Lehrnbecher, T. (2020). Systemic Viral Infection in Children Receiving Chemotherapy for Acute Leukemia. Pediatr. Blood Cancer 67, e28673. doi:10.1002/pbc.28673
Carcao, M. D., Lau, R. C., Gupta, A., Huerter, H., Koren, G., and King, S. M. (1998). Sequential Use of Intravenous and Oral Acyclovir in the Therapy of Varicella in Immunocompromised Children. Pediatr. Infect. Dis. J. 17, 626–631. doi:10.1097/00006454-199807000-00010
Carreras, E., Dufour, C., Mohty, M., and Kröger, N. (2018). The EBMT Handbook: Hematopoietic Stem Cell Transplantation and Cellular Therapies. Cham: Springer Open.
Chayavichitsilp, P., Buckwalter, J. V., Krakowski, A. C., and Friedlander, S. F. (2009). Herpes Simplex. Pediatr. Rev. 30, 119–130. doi:10.1542/pir.30-4-119
Cies, J. J., Moore, W. S., Miller, K., Small, C., Carella, D., Conley, S., et al. (2015). Therapeutic Drug Monitoring of Continuous-Infusion Acylovir for Disseminated Herpes Simplex Virus Infection in a Neonate Receiving Concurrent Extracorporeal Life Support and Continuous Renal Replacement Therapy. Pharmacotherapy 35, 229–233. doi:10.1002/phar.1526
Czyzewski, K., Dziedzic, M., Salamonowicz, M., Fraczkiewicz, J., Zajac-Spychala, O., Zaucha-Prazmo, A., et al. (2019). Epidemiology, Outcome and Risk Factors Analysis of Viral Infections in Children and Adolescents Undergoing Hematopoietic Cell Transplantation: Antiviral Drugs Do Not Prevent Epstein-Barr Virus Reactivation. Infect. Drug Resist. 12, 3893–3902. doi:10.2147/IDR.S224291
Dadwal, S. S. (2019). Herpes Virus Infections Other Than Cytomegalovirus in the Recipients of Hematopoietic Stem Cell Transplantation. Infect. Dis. Clin. North. Am. 33, 467–484. doi:10.1016/j.idc.2019.02.012
De Miranda, P., and Blum, M. R. (1983). Pharmacokinetics of Acyclovir after Intravenous and Oral Administration. J. Antimicrob. Chemother. 12, 29–37. doi:10.1093/jac/12.suppl_b.29
Di Paolo, A., Tascini, C., Polillo, M., Gemignani, G., Nielsen, E. I., Bocci, G., et al. (2013). Population Pharmacokinetics of Daptomycin in Patients Affected by Severe Gram-Positive Infections. Int. J. Antimicrob. Agents 42, 250–255. doi:10.1016/j.ijantimicag.2013.06.006
Eksborg, S., Pal, N., Kalin, M., Palm, C., and Söderhäll, S. (2002). Pharmacokinetics of Acyclovir in Immunocompromized Children with Leukopenia and Mucositis after Chemotherapy: Can Intravenous Acyclovir Be Substituted by Oral Valacyclovir? Med. Pediatr. Oncol. 38, 240–246. doi:10.1002/mpo.1317
Engel, J. P., Englund, J. A., Fletcher, C. V., and Hill, E. L. (1990). Treatment of Resistant Herpes Simplex Virus with Continuous-Infusion Acyclovir. Jama 263, 1662–1664. Available at: http://www.ncbi.nlm.nih.gov/pubmed/2308204. doi:10.1001/jama.1990.03440120084042
Feldman, S., and Lott, L. (1987). Varicella in Children with Cancer: Impact of Antiviral Therapy and Prophylaxis. Pediatrics 80, 465–472. Available at: http://www.ncbi.nlm.nih.gov/pubmed/2821476. doi:10.1542/peds.80.4.465
Fisher, B., Alexander, S., Dvorak, C., Zaoutis, T., Zerr, M., and Sung, L. (2008). The British Childhood Cancer Survivor Study: Objectives, Methods, Population Structure, Response Rates and Initial Descriptive Information. Pediatr. Blood Cancer 50, 1018–1025. doi:10.1002/pbc
Fletcher, C. V., Englund, J. A., Bean, B., Chinnock, B., Brundage, D. M., and Balfour, H. H. (1989). Continuous Infusion of High-Dose Acyclovir for Serious Herpesvirus Infections. Antimicrob. Agents Chemother. 33, 1375–1378. doi:10.1128/AAC.33.8.1375
Flowers, C. R., Seidenfeld, J., Bow, E. J., Karten, C., Gleason, C., Hawley, D. K., et al. (2013). Antimicrobial Prophylaxis and Outpatient Management of Fever and Neutropenia in Adults Treated for Malignancy: American Society of Clinical Oncology Clinical Practice Guideline. J. Clin. Oncol. 31, 794–810. doi:10.1200/JCO.2012.45.8661
Hooker, A. C., Staatz, C. E., and Karlsson, M. O. (2007). Conditional Weighted Residuals (CWRES): A Model Diagnostic for the FOCE Method. Pharm. Res. 24, 2187–2197. doi:10.1007/s11095-007-9361-x
Jaing, T. H., Chang, T. Y., Chen, S. H., Wen, Y. C., Yu, T. J., Lee, C. F., et al. (2019). Factors Associated with Cytomegalovirus Infection in Children Undergoing Allogeneic Hematopoietic Stem-Cell Transplantation. Medicine (Baltimore) 98, e14172. doi:10.1097/MD.0000000000014172
Jonsson, E. N., and Karlsson, M. O. (1999). Xpose--an S-PLUS Based Population Pharmacokinetic/pharmacodynamic Model Building Aid for NONMEM. Comput. Methods Programs Biomed. 58, 51–64. doi:10.1016/s0169-2607(98)00067-4
Kakisaka, Y., Ishitobi, M., Wakusawa, K., Haginoya, K., Togashi, N., Kitamura, T., et al. (2009). Efficacy of Continuous Acyclovir Infusion in Neonatal Herpes Virus Encephalitis. Neuropediatrics 40, 199–200. doi:10.1055/s-0029-1241187
Kanneti, R., Rajesh, R., Aravinda Raj, J. R., and Bhatt, P. A. (2009). An LC-MS-MS Method for the Simultaneous Quantitation of Acyclovir and Valacyclovir in Human Plasma. Chroma 70, 407–414. doi:10.1365/s10337-009-1171-3
Karlsson, M. O., and Sheiner, L. B. (1993). The Importance of Modeling Interoccasion Variability in Population Pharmacokinetic Analyses. J. Pharmacokinet. Biopharm. 21, 735–750. doi:10.1007/BF01113502
Kim, J. H., Schaenman, J. M., Ho, D. Y., and Brown, J. M. (2011). Treatment of Acyclovir-Resistant Herpes Simplex Virus with Continuous Infusion of High-Dose Acyclovir in Hematopoietic Cell Transplant Patients. Biol. Blood Marrow Transpl. 17, 259–264. doi:10.1016/j.bbmt.2010.06.020
Lindbom, L., Ribbing, J., and Jonsson, E. N. (2004). Perl-speaks-NONMEM (PsN)-Aa Perl Module for NONMEM Related Programming. Comput. Methods Programs Biomed. 75, 85–94. doi:10.1016/j.cmpb.2003.11.003
Ly, C. Y., Yu, C., McDonald, P. R., Roy, A., Johnson, D. K., and Davido, D. J. (2021). Simple and Rapid High-Throughput Assay to Identify HSV-1 ICP0 Transactivation Inhibitors. Antivir. Res 194, 105160. doi:10.1016/j.antiviral.2021.105160
Nadal, D., Leverger, G., Sokal, E. M., Floret, D., Perel, Y., Leibundgut, K., et al. (2002). An Investigation of the Steady-State Pharmacokinetics of Oral Valacyclovir in Immunocompromised Children. J. Infect. Dis. 186, S123–S130. doi:10.1086/342968
Nishii, R., Mizuno, T., Rehling, D., Smith, C., Clark, B. L., Zhao, X., et al. (2021). NUDT15 Polymorphism Influences the Metabolism and Therapeutic Effects of Acyclovir and Ganciclovir. Nat. Commun. 12, 4181. doi:10.1038/s41467-021-24509-7
O'Brien, J. J., and Campoli-Richards, D. M. (1989). Acyclovir. An Updated Review of its Antiviral Activity, Pharmacokinetic Properties and Therapeutic Efficacy. Drugs 37, 233–309. doi:10.2165/00003495-198937030-00002
O'Leary, C. K., Jones, C., Bryant, P. A., Abo, Y. N., Osowicki, J., and Gwee, A. (2020). Feasibility of Continuous Infusions of Acyclovir. Pediatr. Infect. Dis. J. 39, 830–832. doi:10.1097/INF.0000000000002692
Richelsen, R. K. B., Jensen, S. B., and Nielsen, H. (2018). Incidence and Predictors of Intravenous Acyclovir-Induced Nephrotoxicity. Eur. J. Clin. Microbiol. Infect. Dis. 37, 1965–1971. doi:10.1007/s10096-018-3332-5
Saiag, P., Praindhui, D., and Chastang, C. (1999). A Double-Blind, Randomized Study Assessing the Equivalence of Valacyclovir 1000 Mg once Daily versus 500 Mg Twice Daily in the Episodic Treatment of Recurrent Genital Herpes. Genival Study Group. J. Antimicrob. Chemother. 44, 525–531. doi:10.1093/jac/44.4.525
Saral, R., Burns, W. H., and Prentice, H. G. (1984). Herpes Virus Infections: Clinical Manifestations and Therapeutic Strategies in Immunocompromised Patients. Clin. Haematol. 13, 645–660. doi:10.1016/s0308-2261(21)00449-5
Schreiber, R., Wolpin, J., and Koren, G. (2008). Determinants of Aciclovir-Induced Nephrotoxicity in Children. Paediatr. Drugs 10, 135–139. doi:10.2165/00148581-200810020-00008
Schwartz, G. J., Haycock, G. B., Edelmann, C. M., and Spitzer, A. (1976). A Simple Estimate of Glomerular Filtration Rate in Children Derived from Body Length and Plasma Creatinine. Pediatrics 58, 259–263. Available at: http://www.ncbi.nlm.nih.gov/pubmed/951142. doi:10.1542/peds.58.2.259
Spector, S. A., Hintz, M., Wyborny, C., Connor, J. D., Keeney, R. E., and Liao, S. (1982). Treatment of Herpes Virus Infections in Immunocompromised Patients with Acyclovir by Continuous Intravenous Infusion. Am. J. Med. 73, 275–280. doi:10.1016/0002-9343(82)90105-x
Styczynski, J., Reusser, P., Einsele, H., de la Camara, R., Cordonnier, C., Ward, K. N., et al. (2009). Management of HSV, VZV and EBV Infections in Patients with Hematological Malignancies and after SCT: Guidelines from the Second European Conference on Infections in Leukemia. Bone Marrow Transpl. 43, 757–770. doi:10.1038/bmt.2008.386
Tomblyn, M., Chiller, T., Einsele, H., Gress, R., Sepkowitz, K., Storek, J., et al. (2009). Guidelines for Preventing Infectious Complications Among Hematopoietic Cell Transplantation Recipients: A Global Perspective. Biol. Blood Marrow Transpl. 15, 1143–1238. doi:10.1016/j.bbmt.2009.06.019
Wade, K. C., and Monk, H. M. (2015). New Antifungal and Antiviral Dosing. Clin. Perinatol 42, 177. doi:10.1016/j.clp.2014.10.010
Yalçınkaya, R., Öz, F. N., Kaman, A., Aydın Teke, T., Yaşar Durmuş, S., Çelikkaya, E., et al. (2021). Factors Associated with Acyclovir Nephrotoxicity in Children: Data from 472 Pediatric Patients from the Last 10 Years. Eur. J. Pediatr. 180, 2521–2527. doi:10.1007/s00431-021-04093-0
Zeng, L., Nath, C. E., Blair, E. Y., Shaw, P. J., Stephen, K., Earl, J. W., et al. (2009). Population Pharmacokinetics of Acyclovir in Children and Young People with Malignancy after Administration of Intravenous Acyclovir or Oral Valacyclovir. Antimicrob. Agents Chemother. 53, 2918–2927. doi:10.1128/AAC.01138-08
Keywords: acyclovir, pediatric patients, hematopoietic stem cell transplantation, pharmacokinetics, non-linear mixed effect modeling, prolonged infusion acyclovir, children, prolonged infusion
Citation: Maximova N, Nisticò D, Luci G, Simeone R, Piscianz E, Segat L, Barbi E and Di Paolo A (2022) Population Pharmacokinetics of Intravenous Acyclovir in Oncologic Pediatric Patients. Front. Pharmacol. 13:865871. doi: 10.3389/fphar.2022.865871
Received: 30 January 2022; Accepted: 29 March 2022;
Published: 14 April 2022.
Edited by:
Annalisa Capuano, University of Campania Luigi Vanvitelli, ItalyReviewed by:
Georg Hempel, University of Münster, GermanyPascal Le Corre, University of Rennes 1, France
Copyright © 2022 Maximova, Nisticò, Luci, Simeone, Piscianz, Segat, Barbi and Di Paolo. This is an open-access article distributed under the terms of the Creative Commons Attribution License (CC BY). The use, distribution or reproduction in other forums is permitted, provided the original author(s) and the copyright owner(s) are credited and that the original publication in this journal is cited, in accordance with accepted academic practice. No use, distribution or reproduction is permitted which does not comply with these terms.
*Correspondence: Natalia Maximova, bmF0YWxpYS5tYXhpbW92YUBidXJsby50cmllc3RlLml0