- 1Department of Urology, The Second Affiliated Hospital of Nanjing Medical University, Nanjing, China
- 2Department of Emergency Medicine, The Second Affiliated Hospital, Zhejiang University School of Medicine, Hangzhou, China
- 3Department of Urology, The First Affiliated Hospital of Nanjing Medical University, Nanjing, China
Background: This study aimed to explore the effect and mechanism of iguratimod (IGT) on M1 macrophage polarization and antibody-mediated rejection (ABMR) after renal transplant.
Methods: Bioinformatics analysis was performed using three public databases derived from the GEO database. Sprague–Dawley (SD) rats were pre-sensitized with donors of Wistar rats in skin transplantation and a rat renal transplant ABMR model was established from the donors to skin pre-sensitized recipients. Subsequently, IGT was treated on the ABMR model. Routine staining and immunofluorescence (IF) staining were performed to observe the pathological changes in each group and flow cytometry was performed to detect the changes of DSA titers in peripheral blood. In addition, bone-marrow-derived macrophage (BMDM) was extracted and interfered with IGT to explore the effect of IGT in vivo. PCR, IF staining, and Western blot were used to detect the expression of related genes and proteins.
Results: Bioinformatics analysis revealed that several immune cells were significantly infiltrated in the ABMR allograft, while M1 macrophage was noticed with the most significance. Results of IF staining and PCR proved the findings of the bioinformatics analysis. Based on this, IGT was observed to significantly attenuate the degree of peritubular capillary vasculitis and arteriolitis in the rat renal transplant ABMR model, whereas it decreases the expression of C4d and reduces the titer of DSA. Results in vitro suggested that M1 macrophage-related transcripts and proteins were significantly reduced by the treatment of IGT in a dose- and time-dependent manner. Furthermore, IGT intervention could remarkably decrease the expression of KLF4.
Conclusion: Polarization of M1 macrophages may aggravate ABMR after renal transplant by promoting DSA-mediated endothelial cell injury, and IGT may attenuate the pathogenesis of ABMR by targeting KLF4.
Introduction
Allograft rejection after kidney transplantation is the main factor affecting the long-term survival of renal allograft (Gerlach et al., 2017; Wang et al., 2017; Nunes-Dos-Santos et al., 2020). According to its mechanism, rejection episodes following renal transplant can be classified into T-cell-mediated rejection (TCMR) and humoral rejection (Rodríguez-Perálvarez et al., 2017; Haas, 2018). At present, most immunosuppressive drugs on the market are aimed at T-cell-mediated rejection. However, an increasing number of studies have noticed the profound effect of antibody-mediated rejection (ABMR) on the long-term allograft function, which was considered as one of crucial factors contributing to graft loss (Loupy and Lefaucheur, 2018; Louis et al., 2019).
Previous studies have reported that donor endothelial cells are the initial target of de novo antibodies (DSA) (Piotti et al., 2014; Valenzuela et al., 2014; Cardinal et al., 2017). As antibodies bind to the corresponding antigens of endothelial cells, inflammatory damage to the vascular endothelium of the graft was caused through complement-dependent and independent pathways (Djamali et al., 2014; Horwitz et al., 2019). In the complement-dependent pathway, C4d has been considered a specific diagnostic marker of ABMR by the Banff diagnostic criteria, and complement inhibitors targeting C5 have been used to treat ABMR (Jordan et al., 2020; Patel et al., 2021). In complement-independent pathway studies, various types of immune cells, including NK cells, macrophages, T and B lymphocytes, and dendritic cells, were observed to be involved in chronic ABMR (Koenig et al., 2019). Moreover, recent studies have found that increasing the depletion of M1 macrophages can significantly reduce the occurrence of tissue damage and rejection (Cheng et al., 2015; Dai et al., 2020).
Macrophages can be divided into M1 macrophages (promoting inflammatory response) and M2 macrophages (promoting cell proliferation and mediating tissue repair) (Shapouri-Moghaddam et al., 2018; Orecchioni et al., 2019). Under different conditions of immune microenvironments and stimulating factors, macrophages can polarize into M1 or M2 macrophages, which play an important role in maintaining the homeostasis of immune microenvironments by secreting different cytokines (Mills, 2012). M1 macrophages exert pro-inflammatory and anti-tumor biological effects mainly by secreting cytokines such as tumor necrosis factor-α (TNF-α) and interleukin1-beta (IL-1β) (Jakubzick et al., 2017). A bioinformatics study containing 1,697 biopsy samples found that M1 macrophage-associated gene scores were significantly elevated in ABMR biopsies and were strongly associated with poor prognosis (Azad et al., 2018). In addition, some studies have shown that pro-inflammatory cytokines such as TNF-α and IL-1β, which are significantly increased in the progression of pathological injury, are mainly secreted by macrophages (Riquelme et al., 2013). Therefore, it is strongly believed that the secretion of pro-inflammatory factors after the polarization of macrophages may be related to the progression of ABMR.
The Kruppel-like factor (KLF) family is a class of DNA-bound transcription regulatory factors of zinc-finger proteins, which play an important regulatory role in various physiological functions (Takahashi and Yamanaka, 2006). It has been confirmed that KLF4 deficiency can lead to the activation of NF-κB transcriptional activity in macrophages, promoting the polarization of M1 macrophages and significantly enhancing the inflammatory function of M1 macrophages (Liao et al., 2011a). Recently, it was found that the lack of KLF4 can significantly increase the infiltration of M1 macrophages in kidney tissue and accelerate the progression of chronic nephritis in the mouse model of chronic kidney injury, ultimately resulting in end-stage renal disease and renal fibrosis (Wen et al., 2019). These results suggest that KLF4 may be involved in the M1 polarization in ABMR after kidney transplantation.
Iguratimod (IGT) is a small molecule anti-inflammatory drug, which has been widely used in the treatment of rheumatoid arthritis because of its efficient and safe anti-cytokine-mediated inflammatory response (Hara et al., 2014; Ishikawa and Ishikawa, 2019). Previous studies have shown that IGT can play an important role in anti-inflammatory and immune regulation by inhibiting the synthesis of prostaglandin E2, TNF-α, and interleukin-17 (IL-17) (Liu et al., 2021). Studies have shown that IGT can delay kidney injury in mouse models of lupus nephropathy by inhibiting antibody-mediated immune response (Yan et al., 2014; Zhao et al., 2019). More importantly, our previous clinical study on the application of IGT in transplantation reported that the combination of IGU and conventional immunosuppressants can reduce the probability of rejection after transplantation (Tao et al., 2021). Therefore, whether IGT could attenuate the progression of ABMR still remains unknown.
From what has been discussed previously, we hypothesized that IGT may inhibit the polarization of M1-type macrophages by upregulating the expression of KLF4, reduce the secretion of TNF-α cytokines, and consequently alleviate DSA-mediated allograft endothelial injury and the progression of ABMR after renal transplantation.
Methods and Materials
Ethnic Statement
The research protocol meets the ethical standards of the Declaration of Helsinki and Istanbul. The research protocol involving human kidney tissues was approved by the local ethics committee of the First Affiliated Hospital of Nanjing Medical University (2016-SR-029). Written informed consent was obtained from all transplant recipients and nephrectomy patients.
Bioinformatics Analysis
The series of matrix files of GSE36059, GSE98320, and GSE124203 were downloaded from the GEO database (https://www.ncbi.nlm.nih.gov/geo) and merged into a metadata cohort after removing the batch effect by the “SVA” package of R software. The “limma” package was used to identify differentially expressed genes. To quantify the relative proportions of infiltrating immune cells, a bioinformatics algorithm was used to calculate immune cell infiltrations by the CIBERSORT R script. The putative abundance of immune cells was estimated using a reference set with 22 types of immune cell subtypes (LM22) with 1,000 permutations (Ali et al., 2016). We used Spearman’s rank correlation analysis performed by the R package “corrplot” and “ggplot2” when exploring the association.
Animals
Adult male Sprague–Dawley (SD) and Wistar rats were procured from Charles River Laboratories (Beijing, China). Adult male Balb/c mice were procured from the Animal Core Facility of Nanjing Medical University. The animal center provided the animals with clean tap water and standard feedstuff. All animal handling procedures followed guidelines issued by the US National Institutes of Health and animal ethics established by Nanjing Medical University.
Establishment of Rat Renal Transplant ABMR Model
We pre-sensitized recipient SD rats with donor Wistar rats by skin transplantation and constructed an ABMR model by abdominal ectopic kidney transplantation from donors to skin pre-sensitized recipients. Based on ABMR models, we gave a gavage of IGT (15 mg/kg) every morning and evening after surgery to explore the effect of IGT on ABMR. Detailed procedures are described in previous studies (Cheng, 2019).
Isolation, Culture, and Treatment of Bone-Marrow-Derived Macrophage
Bone marrow suspension was obtained by rinsing the bone marrow cavity of the femur and tibia of Balb/c mice. A monocyte separator (LTS1092PK-200, tbdscience) was used to isolate monocytes from the bone marrow suspension. Isolated monocytes were cultured with DMEM (01-05201ACS, Biological Industries) containing 10% FBS (04-001-1ACS, Biological Industries), 1% penicillin-streptomycin, and 30 ng/ml M-CSF (CB34, novoprotein). The new medium was replaced 3 days later. The cells matured 7 days after the extraction and could be used for downstream experiments. BMDMs were stimulated with 50 ng/ml LPS (00-4976-93, Invitrogen) and 10 ng/ml IFN-γ (CM41, Selleck) to promote M1 polarization. To explore the effect of IGT in vitro, cells were treated for 24 h with IGT (10 ng/ml, S5648, Selleck).
Cell Counting Kit-8 Analysis
The cell suspension was prepared and the cell number was counted. Planking cells (about 1–2 × 104) were inoculated into a 96-well plate. After incubation at 37 °C for 4 h, 10uL of CCK8 (Beyotime, Shanghai, China) was added and cells were cultured for 0.5–4 h. It is recommended to use dual wavelengths to determine the absorbance, with a detection wavelength of 450–490 nm and a reference wavelength of 600–650 nm (Hou et al., 2007).
RNA Isolation and Quantitative Real-Time PCR
RNA was isolated from cells or tissues using the commercial RNA Isolation Kit (RC112-01, Vazyme). cDNA was synthesized with a reverse transcription kit (R333-01, Vazyme). Quantitative real-time PCR was performed with a SYBR Green PCR kit (Q341-AA, Vazyme). The specific primers used were as follows: CD68: 5′‐TGTCTGATCTTGCTAGGACCG‐3′ (F),5′-GAGAGTAACGGCCTTTTTGTGA‐3′ (R); CD86: 5′‐TGTTTCCGTGGAGACGCAAG‐3′(F),5′-TTGAGCCTTTGTAAATGGGCA‐3′ (R); NOS2: 5′‐GTTCTCAGCCCAACAATACAAGA‐3′ (F),5′- GTGGACGGGTCGATGTCAC‐3′ (R); CD206: 5′‐CTCTGTTCAGCTATTGGACGC‐3′ (F),5′- CGGAATTTCTGGGATTCAGCTTC‐3′ (R); IL-1β: 5′‐TTCAGGCAGGCAGTATCACTC‐3′ (F),5′-GAAGGTCCACGGGAAAGACAC.‐3′ (R); IL-6: 5′‐TAGTCCTTCCTACCCCAATTTCC‐3′ (F),5′- TTGGTCCTTAGCCACTCCTTC‐3′ (R); KLF4: 5′‐GTGCCCCGACTAACCGTTG‐3′ (F),5′- GTCGTTGAACTCCTCGGTCT‐3′ (R); and GAPDH: 5′‐GAAGGTCGGTGTGAACGGAT‐3′ (F),5′-CCCATTTGATGTTAGCGGGAT‐3′ (R). The primers were bought from Tsingke Biotechnology (Nanjing, China).
Histology and Immunofluorescence Microscopy
Kidney tissue sections were stained with HE and PAS for the histopathological examination. Immunofluorescence microscopy was performed as previously described, using antibodies against C4d (HP8034, Hycult Biotec), CD68 (ab955, Abcam), iNOS (ab178945, Abcam), iNOS (ab49999, Abcam), F4/80 (28463-1-AP, proteintech), and KLF4 (1180-1-AP, proteintech) (Wang et al., 2017). Images from each stained slide were captured, and quantitative analyses of positive staining were carried out independently by two authors (Z Hang and M Zheng) under a light microscope equipped with a digital camera (Nikon, ECLIPSE 80i, Japan). The mean fluorescence intensity (MFI) of each image was calculated and mean relative abundance was obtained from MFIs of five representative images.
Flow Cytometry
Flow cytometry was performed to identify macrophage polarization. We use fluorescein isothiocyanate (FITC)-conjugated anti-mouse iNOS (5201004082, Miltenyi Biotec) to identify M1 macrophages and phycoerythrin (PE)-conjugated anti-mouse F4/80 (5200809116, Miltenyi Biotec) to identify M0 macrophages according to the instructions.
DSAs Examination
We co-cultured the spleen lymphocytes of normal Wistar rats with the serum of the rats to be tested. The cells were incubated with PE-conjugated anti-rat IgG (GB21302, Servicebio) after cleaning. The mean fluorescence intensity (MFI) of the cell suspension was measured by flow cytometry and the level of serum DSA was evaluated (Chandran et al., 2018; Zhao et al., 2018).
Statistical Analysis
All analyses were independently repeated at least three times. All data were expressed as the mean ± SD value determined by three independent experiments. One-way analysis of variance (ANOVA) was used for intergroup comparison. Multiple methods were compared using the Tukey test. The difference between the two groups was determined by a non-parametric test with regard to the limited sample size. p < 0.05 was considered statistically significant.
Results
M1 Macrophage Infiltration Increased Significantly in ABMR Allograft
The CIBERPORT algorithm was used to explore the immune infiltration in renal allograft specimens from three GEO databases (GSE124203, GSE36059, and GSE98320). Figures 1A,B show that macrophage polarization was significantly activated during the pathogenesis of ABMR in the pooled results. Moreover, it was found that macrophage infiltration, including macrophage M0, M1, and M2 types in allograft tissue with ABMR increased significantly (Figure 1C), while M1 macrophages were suggested as the most significant cells infiltrated in the progression of ABMR (Figure 1D), suggesting that M1 macrophages may play an important role in the pathogenesis of ABMR.
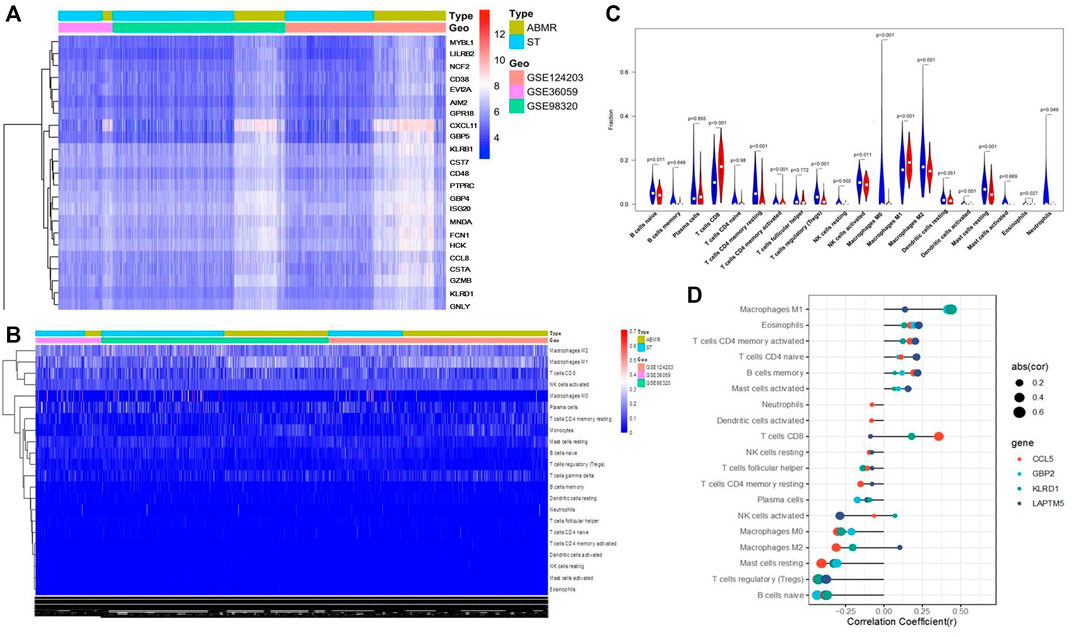
FIGURE 1. Bioinformatics analysis results of three public databases from the GEO database. (A) Heat map after the joint analysis of the three databases. (B) Cell distribution after the combined analysis of the three databases. (C) Analysis of immune cell infiltration in ABMR transplanted kidney tissue. (D) Correlation between immune cell infiltration and ABMR in renal transplantation.
Significant renal injury and more C4d deposition in peritubular capillaries and glomeruli in the ABMR group when compared with the STA group were observed by histological studies and immunofluorescence microscopy (Figures 2A–C). Moreover, infiltration of M1 macrophages was increased significantly in ABMR allografts (Figure 2D), which was mainly observed around the area of glomerular and peritubular capillaries. However, no significant difference of M2 macrophages was observed between the two groups (Figure 2E).
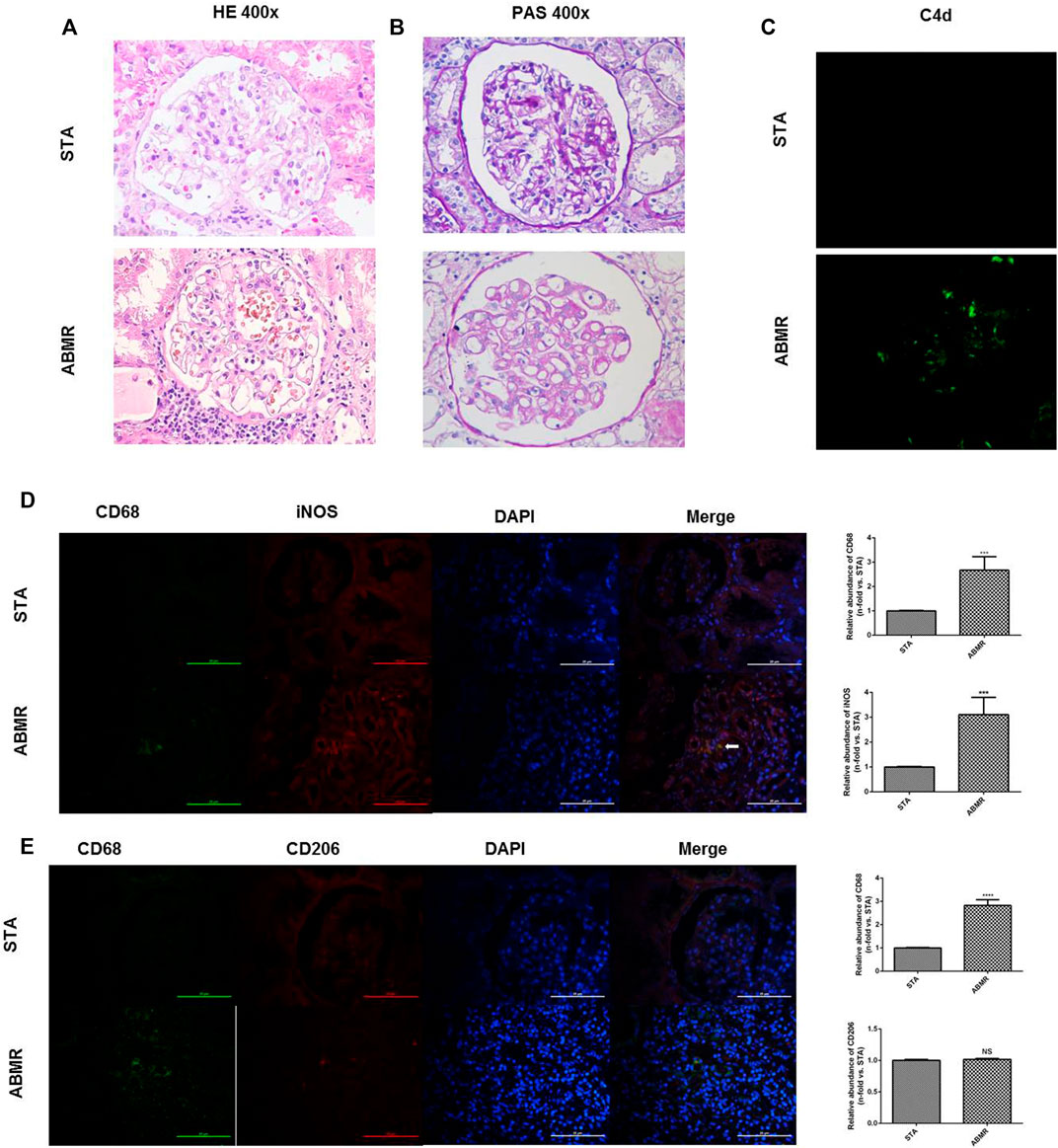
FIGURE 2. Determination of macrophage infiltration in human renal allograft samples. (A–B) Renal pathology in STA and ABMR patients (400×). (C) Representative images of C4d immunostaining in kidney sections from STA and ABMR patients (400×). (D) Double indirect fluorescence staining of CD68 and iNOS in ABMR and STA groups (400×). (E) Double indirect fluorescence staining of CD68 and CD206 in ABMR and STA groups (400×). ***p < 0.001 compared with the STA group; ****p < 0.0001 compared with the STA group; NS: no significant difference compared with the STA group; a non-parametric test was used for statistical analysis in this figure.
Polarization of M1 Macrophages Increased in Rat Renal Transplant ABMR Model
We established a rat renal transplant ABMR model by skin pre-sensitization. Immunofluorescence microscopy revealed that iNOS, the specific transcription factor of M1 macrophages, was highly expressed in the ABMR group compared with other groups (Figure 3A). The expression of CD206 in the ABMR group was also increased (Figure 3B). Furthermore, compared with the non-pre-sensitized group, CD206, CD68, CD86, and iNOS increased in the ABMR group, but CD86 and iNOS were more significant (Figures 3C–F).
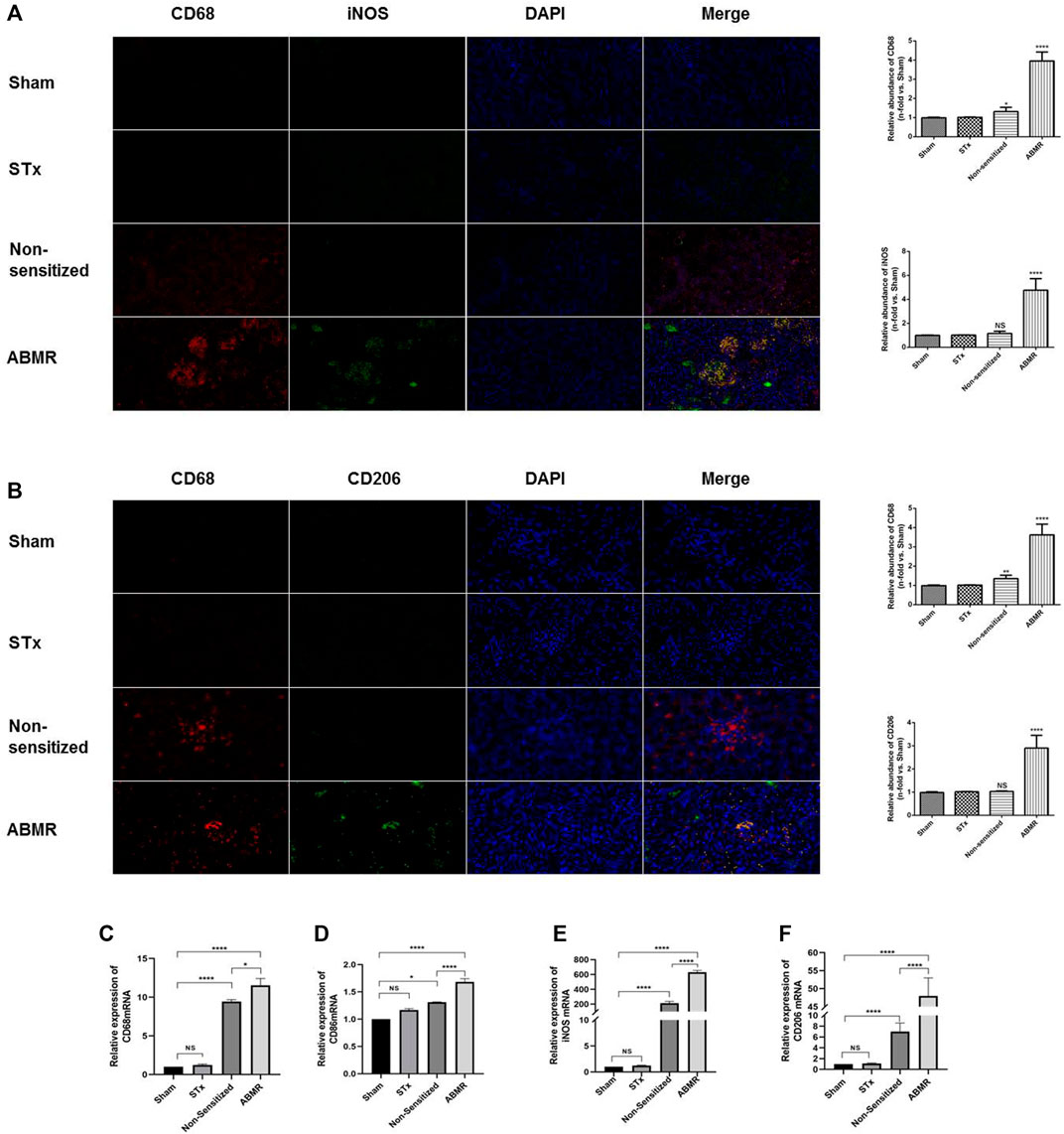
FIGURE 3. Determination of macrophage infiltration in renal allograft samples derived from the rat renal transplant ABMR model. (A) Double indirect fluorescence staining of CD68 and iNOS in the sham operation group (sham), skin transplant group (STx), non-sensitive kidney transplant group (non-sensitive), and ABMR group (ABMR) (200×). (B) Double indirect fluorescence staining of CD68 and iNOS in the sham group, STx group, non-sensitive group, and ABMR group (200×). (C–F) Renal mRNA expression of (C) CD68 (D) CD86 (E) iNOS, and (F) CD206 in the sham group, STx group, non-sensitive group, and ABMR group. *p < 0.05 compared with the sham group; **p < 0.01 compared with the sham group; ***p < 0.001 compared with the sham group; ****p < 0.0001 compared with the sham group; NS: no significant difference compared with the sham group; a non-parametric test was used for the statistical analysis in this figure.
IGT Attenuated the Progression of ABMR and M1 Macrophage Polarization in Rat Model
HE and PAS staining showed significant peritubular capillary inflammation and glomerulitis in the ABMR group. Compared with the ABMR group, the inflammation in ABMR + IGT group was significantly relieved (Figures 4A,B). IGT can reduce the deposition of C4d and DSA which further confirms the effect of IGT (Figures 4C,D).
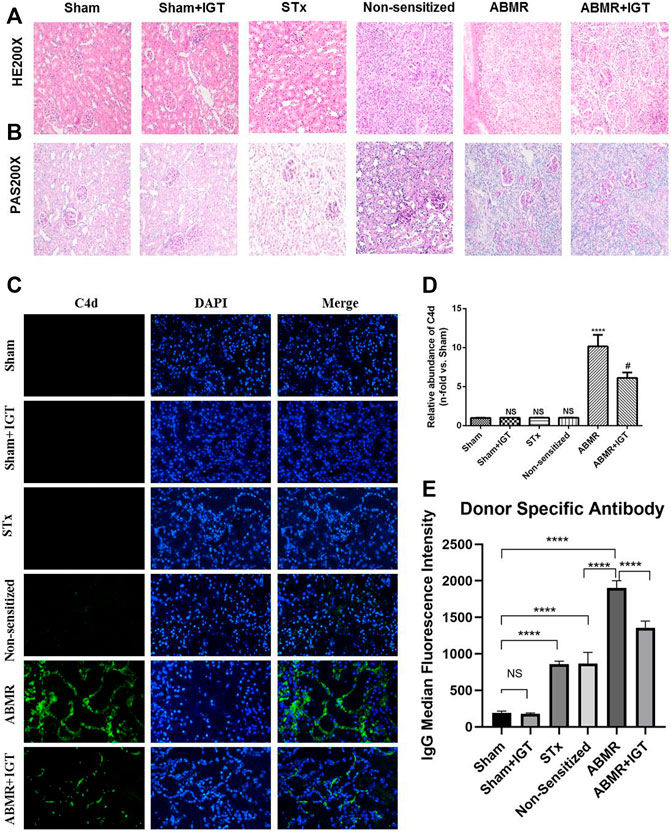
FIGURE 4. Effect of iguratimod intervention in the rat renal transplant ABMR model. (A–B) Representative images of renal pathology in sham, sham + IGT, STx, non-sensitive, ABMR, and ABMR + IGT groups (400×). (C) Indirect fluorescence staining of C4d in sham, sham + IGT, STx, non-sensitive, ABMR, and ABMR + IGT groups (400×). (D) Quantitative analysis of C4d immunostaining in each group. (E) DSA examination of sham, sham + IGT, STx, non-sensitive, ABMR, and ABMR + IGT groups. ****p < 0.0001 compared with the sham group; #p < 0.001 compared with the ABMR group; the non-parametric test was used for the statistical analysis in this figure.
Immunofluorescence microscopy revealed remarkably high expression of iNOS in the ABMR group (Figure 5A). After the treatment of IGT, the positive area of iNOS in the allograft was dramatically decreased (Figure 5A). Results of the quantitative real-time PCR also indicated that IGT could downregulate the expression of CD86 and iNOS in the rat allograft kidney (Figures 5B–D). Therefore, the remission of ABMR progression by IGT may be correlated with the inhibition of M1 macrophage polarization.
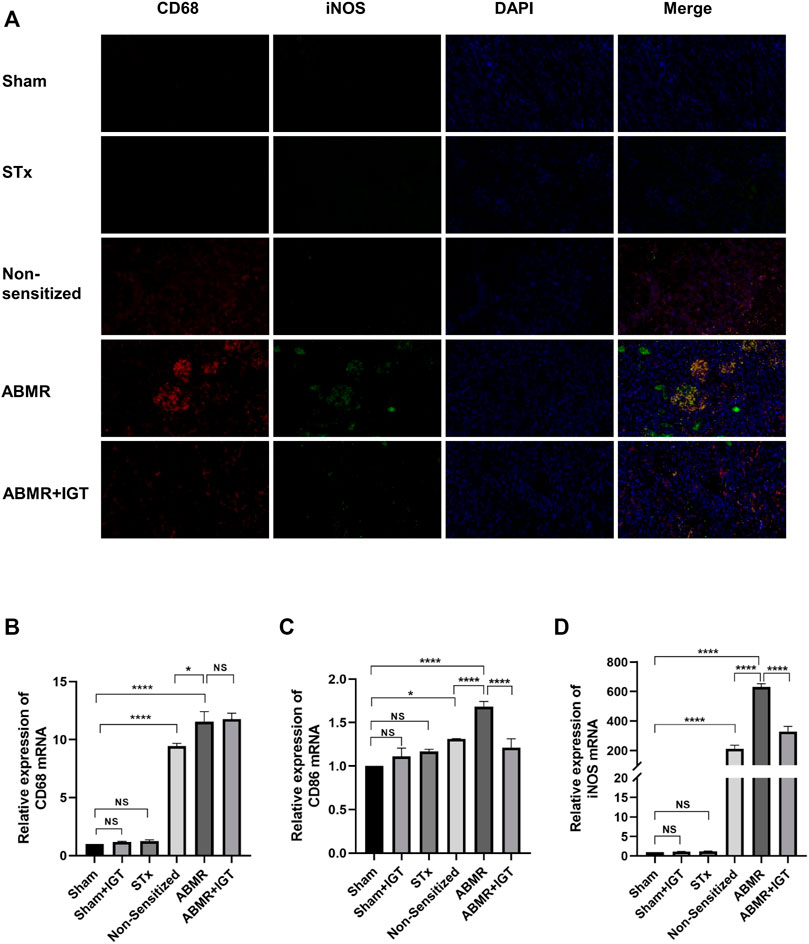
FIGURE 5. Determination of macrophage infiltration after intervention of IGT in the rat model. (A) Double indirect fluorescence staining of CD68 and iNOS in sham, sham + IGT, STx, non-sensitive, ABMR, and ABMR + IGT groups (400×). (B–D) Renal mRNA expression of (B) CD68 (C) CD86, and (D) iNOS in the sham, STx, non-sensitive, and ABMR groups. The non-parametric test was used for statistical analysis in this figure.
IGT Inhibited M1 Macrophage Polarization in Vitro
We used CCK8 to detect the toxicity of IGT and little effect has been noticed in the dose-dependent manner of IGT on cell proliferation (Figure 6A). Under a light microscope, the morphology of macrophages becomes thin during M1 polarization and IGT intervention can partially reverse the morphology of macrophages (Figure 6B). With the extension of IGT intervention, the change of iNOS at the transcription level reached the peak at 12 h and lost its effect after 48 h (Figure 6C). IGT of different concentrations (5, 10, 20, and 40 μg/ml) can inhibit macrophage polarization and reduce the secretion of pro-inflammatory factors, such as IL1-β and IL-6, at the transcription level (Figures 6D–G). This result was further confirmed by flow cytometry and immunofluorescence (Figures 6H,I). To further explore the effect of IGT on M2 macrophages, IGT intervention (10 μg/ml) was performed on M2 macrophages for 12 h. As shown in Supplementary Figure S1, no significant difference was observed in YM1, Fizz1, Arg-1, and MR mRNA between M2 macrophage with or without IGT (Supplementary Figure S1).
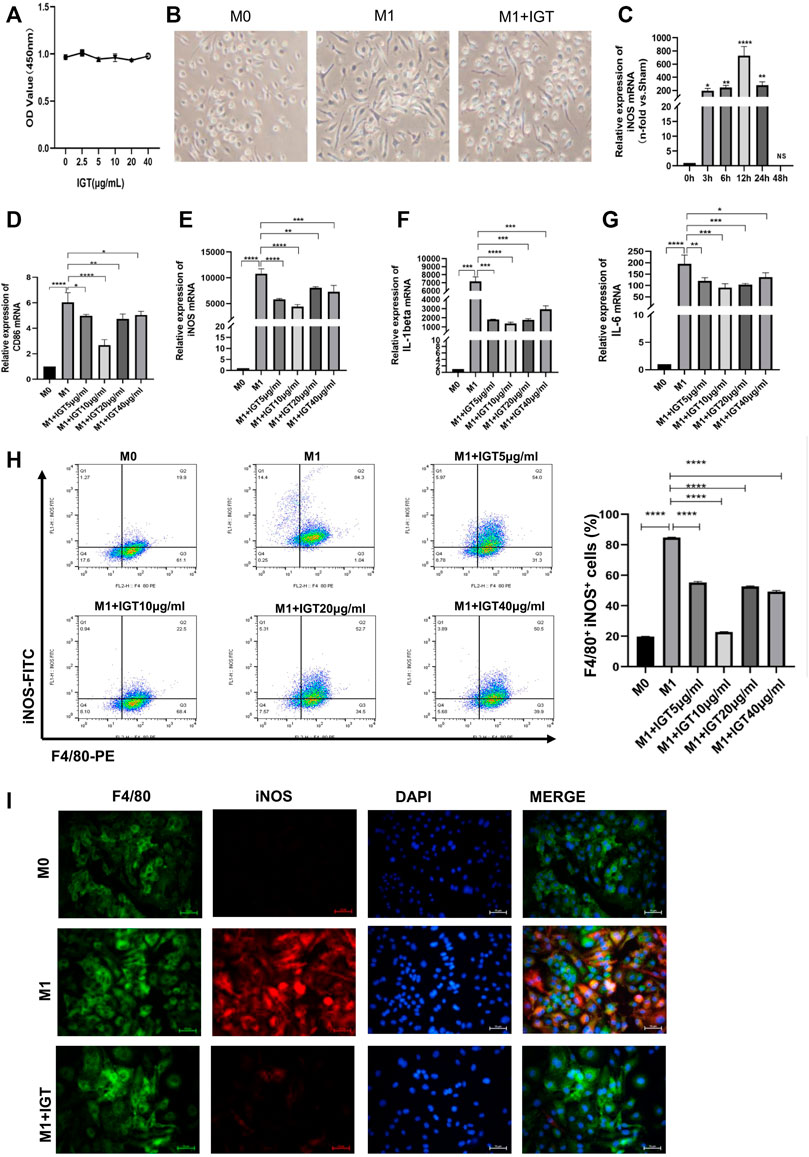
FIGURE 6. IGT-inhibited M1 macrophage polarization in vitro. (A) CCK8 assay of IGT intervention with BMDM. (B) Representative images of different macrophages under a light microscope (400×). (C) mRNA expression of iNOS in BMDM after the time-dependent intervention of IGT. (D–G) mRNA expression of CD86, iNOS, IL1-β, and IL6 in BMDM after concentration-dependent intervention of IGT. (H) Flow cytometry for F4/80 and iNOS in BMDM after concentration-dependent intervention of IGT. (I) Double indirect fluorescence staining of F4/80 and iNOS in M0 (M0), M1 (M1), and M1 macrophages after IGT intervention (M1+IGT). The non-parametric test was used for statistical analysis in this figure.
KLF4 Was Involved in Macrophage Polarization Regulation in ABMR
Previous studies have noticed that KLF4 may be involved in the polarization of M1 macrophages, which could be served as a critical target for the modulation of macrophage polarization (Almatroodi et al., 2020; Wang et al., 2020; Qiu et al., 2021). Allograft from ABMR rats at different time points (3, 5, and 7 days) post-transplant were stained by indirect immunofluorescence. The degree of ABMR gradually increased, macrophage infiltration increased, and the expression of KLF4 in macrophages decreased in a time-dependent manner (Figure 7A). We also found that the expression of KLF4 mRNA and protein decreased significantly during the polarization of M1 macrophages. The expression of KLF4 mRNA and protein also increased significantly after the intervention of 10 μg/ml IGT (Figures 7B–G), suggesting that KLF4 may be involved in the process of IGT inhibiting the polarization of M1 macrophages.
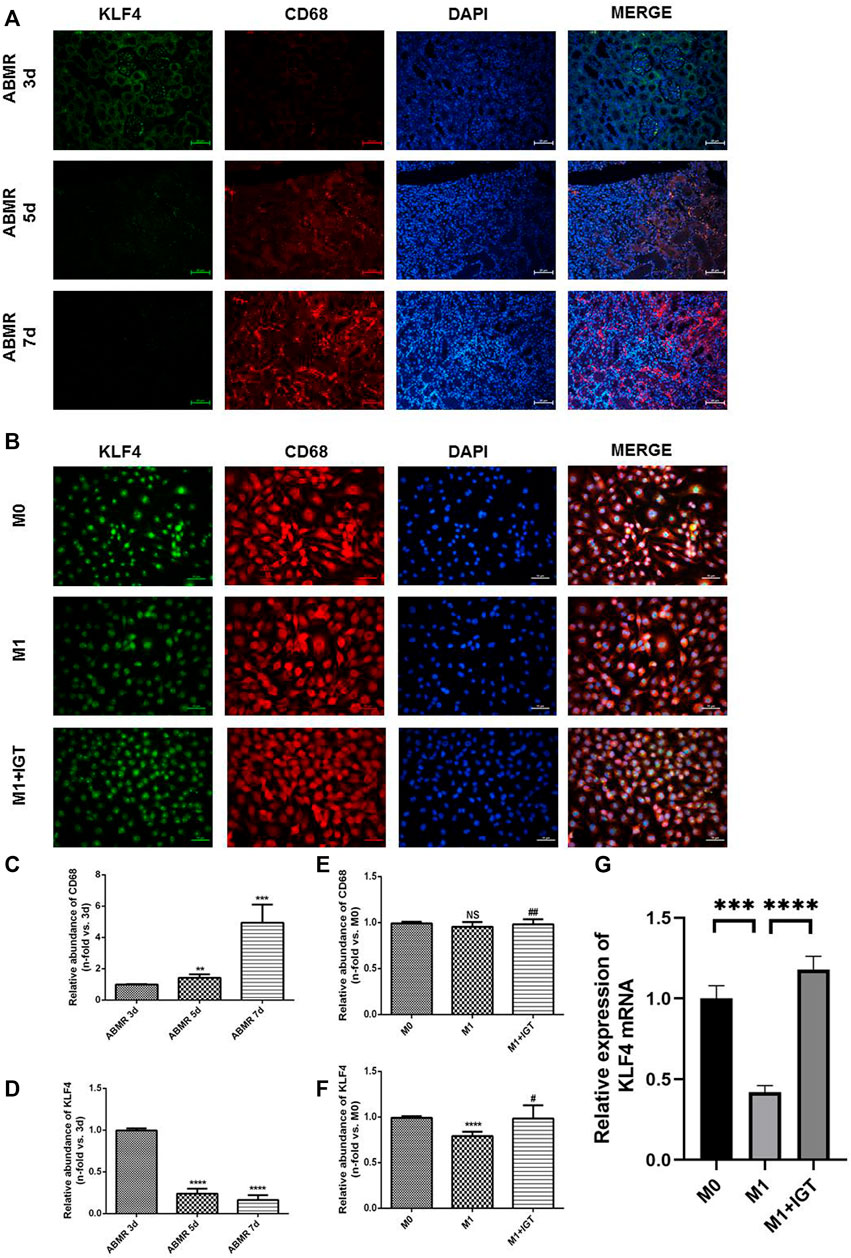
FIGURE 7. KLF4 was involved in macrophage polarization regulation in ABMR. (A) Double indirect fluorescence staining of CD68 and KLF4 in ABMR rat models. (B) Double indirect fluorescence staining of CD68 and KLF4 in M0, M1, and M1+IGT. (C–D) Quantitative analysis of CD68 (C) and KLF4 (D) in ABMR rat models. (E–F) Quantitative analysis of CD68 (E) and KLF4 (F) in cell culture. (G) mRNA expression of KLF4 in macrophages. **p < 0.01 compared with the control group; ***p < 0.001 compared with the control group; ****p < 0.0001 compared with the control group; #p < 0.05 compared with the M1 group; ##p > 0.05 compared with the M1 group; non-parametric test was used for statistical analysis in this figure.
Discussion
In the present study, we reported that IGT could attenuate ABMR after kidney transplantation via regulating M1 macrophage polarization induced by the deficiency of the transcription factor KLF4. The roles of IGT are not only to block M1 macrophage polarization but to also largely reduce the manufacture of proinflammatory cytokines such as TNF-α and IL6 which are closely related to vascular endothelial injury. In the scope of our knowledge, this is the first time that IGT has been applied to the field of transplantation.
Macrophage polarization plays an important role in maintaining the homeostasis of the immune microenvironment. In the present study, we found that M1 macrophages infiltrated significantly in ABMR transplanted kidney tissues, both in rat models and humans. These experiments elucidate that the occurrence and development of ABMR are related to M1 macrophage infiltration and that the mechanism may be related to the secretion of pro-inflammatory cytokines and exosomes. In further experiments, we found that M1 secreted a large number of inflammatory factors during polarization. Previous studies have reported that TNF-α and other pro-inflammatory factors are closely related to endothelial injury. We can reasonably suspect that pro-inflammatory factors secreted by M1 cause endothelial cell damage through potential pathways, and then result in ABMR progression. Currently, studies on macrophage polarization in the field of transplantation mainly focus on ischemia-reperfusion injury, and research on rejection, especially ABMR, is still lacking (Zhang et al., 2018; Ye et al., 2020). More importantly, recent studies have pointed out that monocytes and macrophages could be considered as secondary effectors and capable of direct allorecognition, indicating the potential therapeutic target for ABMR treatment (Callemeyn et al., 2022; Lebraud et al., 2022). The concrete molecular mechanism needs to be studied further.
Our in vitro experiments indicate that KLF4 had low expression in M1 macrophages while IGT can reverse this trend. We speculated that KLF4 in macrophages could play a protective role by suppressing their M1 polarization. Sharma et al demonstrated that KLF4 reduced the secretion of pro-inflammatory cytokines in myeloid cells and thereby constrains atherogenesis (Sharma et al., 2012). This finding is consistent with our research. Xudong Liao et al believed that KLF4 could inhibit M1 polarization via the intercept of co-activators required for NF-κB activation (Liao et al., 2011b). These studies indicated that KLF4, as an important regulatory factor, could regulate the polarization process of macrophages.
In recent years, our team has conducted a clinical study in the world for the first time in combination with traditional immunosuppression regimens in kidney transplant recipients. The results showed that the incidence of biopsy-proven acute rejection in the IGT group was significantly lower than that in the control group and no obvious toxic side effects were observed. However, its mechanism of immunosuppression is still unclear, which restricts its further clinical application. In this study, we found that IGT significantly slowed the occurrence of ABMR after surgery, and this process was associated with the targeted inhibition of M1-type macrophages. This result explained part of the mechanism of the effect of IGT, which is conducive to further promoting the clinical application of IGT after kidney transplantation.
Obviously, there are still certain limitations in this study. The mechanism of KLF4 may be further explored. We still need to explore the specific mechanism of the interaction between macrophages and endothelial cells. Furthermore, the LM22 matrix used for the bioinformatics analysis in this study was to explore the immune cell infiltrations, which were originally designed for tumor biology. With regard to the difference in the microenvironment in various pathogenesis, the immune infiltration in renal organs, especially in the renal allograft, should be interpreted with caution.
In conclusion, studies on the rat ABMR model and in vitro suggest that IGT may attenuate the progression of ABMR after kidney transplantation by inhibiting KLF4-regulated M1 macrophage polarization. Our study of IGT provided a novel insight into the further exploration of treatment for ABMR.
Data Availability Statement
The original contributions presented in the study are included in the article/Supplementary Material, further inquiries can be directed to the corresponding authors.
Ethics Statement
The studies involving human participants were reviewed and approved by the First Affiliated Hospital of Nanjing Medical University. The patients/participants provided their written informed consent to participate in this study. The animal study was reviewed and approved by the Institutional Animal Care and Use Committee.
Author Contributions
ZW, MG, and RT contributed to the conception and design of the study. ZH, MZ, JW, ZG, ZH, and JT collected experimental data. ZH, MZ, JW, LS, SF, and HC performed the statistical analysis. ZH wrote the first draft of the manuscript. ZW and HC wrote sections of the manuscript. MG, RT, and ZW raised funds for this study. All authors contributed to manuscript revision, read, and approved the submitted version.
Funding
This work was supported by the National Natural Science Foundation of China (grant numbers 82170769, 82070769, 81900684, 81870512, and 81770751), the “333 High Level Talents Project” in Jiangsu Province (grant numbers BRA2017532, BRA2016514), and the Jiangsu Province Natural Science Foundation Program (grant number BK20191063).
Conflict of Interest
The authors declare that the research was conducted in the absence of any commercial or financial relationships that could be construed as a potential conflict of interest.
Publisher’s Note
All claims expressed in this article are solely those of the authors and do not necessarily represent those of their affiliated organizations, or those of the publisher, the editors, and the reviewers. Any product that may be evaluated in this article, or claim that may be made by its manufacturer, is not guaranteed or endorsed by the publisher.
Supplementary Material
The Supplementary Material for this article can be found online at: https://www.frontiersin.org/articles/10.3389/fphar.2022.865363/full#supplementary-material
Supplementary Figure S1 | IGT showed no effect on M2 macrophage polarization in vitro. (A–D) mRNA expression of Arg-1 (A), YM1 (B), Fizz1 (C), and MR (D) in BMDM after the intervention of IGT (10 μg/ml) for 12 h. A non-parametric test was used for the statistical analysis in this figure.
References
Ali, H. R., Chlon, L., Pharoah, P. D., Markowetz, F., and Caldas, C. (2016). Patterns of Immune Infiltration in Breast Cancer and Their Clinical Implications: A Gene-Expression-Based Retrospective Study. Plos Med. 13 (12), e1002194. doi:10.1371/journal.pmed.1002194
Almatroodi, S. A., Almatroudi, A., Alsahli, M. A., Aljasir, M. A., Syed, M. A., and Rahmani, A. H. (2020). Epigallocatechin-3-Gallate (EGCG), an Active Compound of Green Tea Attenuates Acute Lung Injury Regulating Macrophage Polarization and Krüpple-Like-Factor 4 (KLF4) Expression. Molecules 25 (12). doi:10.3390/molecules25122853
Azad, T. D., Donato, M., Heylen, L., Liu, A. B., Shen-Orr, S. S., Sweeney, T. E., et al. (2018). Inflammatory Macrophage-Associated 3-gene Signature Predicts Subclinical Allograft Injury and Graft Survival. JCI Insight 3 (2). doi:10.1172/jci.insight.95659
Callemeyn, J., Lamarthée, B., Koenig, A., Koshy, P., Thaunat, O., and Naesens, M. (2022). Allorecognition and the Spectrum of Kidney Transplant Rejection. Kidney Int. 101 (4), 692–710. doi:10.1016/j.kint.2021.11.029
Cardinal, H., Dieudé, M., and Hébert, M. J. (2017). The Emerging Importance of Non-HLA Autoantibodies in Kidney Transplant Complications. J. Am. Soc. Nephrol. 28 (2), 400–406. doi:10.1681/ASN.2016070756
Chandran, S. R., Mulley, W. R., Kanellis, J., Nikolic-Paterson, D. J., and Ma, F. Y. (2018). A Model of Acute Antibody-Mediated Renal Allograft Rejection in the Sensitized Rata. Exp. Clin. Transpl. 16 (3), 294–300. doi:10.6002/ect.2016.0316
Cheng, H. (2019). Construction and Identification of an Antibody-Mediated Rejection Model after Kidney Transplantation. J. Nanjing Med. Univ. Nat. Sci. Edition 39 (5), 677–681.
Cheng, L., Xing, H., Mao, X., Li, L., Li, X., and Li, Q. (2015). Lipocalin-2 Promotes M1 Macrophages Polarization in a Mouse Cardiac Ischaemia-Reperfusion Injury Model. Scand. J. Immunol. 81 (1), 31–38. doi:10.1111/sji.12245
Dai, H., Lan, P., Zhao, D., Abou-Daya, K., Liu, W., Chen, W., et al. (2020). PIRs Mediate Innate Myeloid Cell Memory to Nonself MHC Molecules. Science 368 (6495), 1122–1127. doi:10.1126/science.aax4040
Djamali, A., Kaufman, D. B., Ellis, T. M., Zhong, W., Matas, A., and Samaniego, M. (2014). Diagnosis and Management of Antibody-Mediated Rejection: Current Status and Novel Approaches. Am. J. Transpl. 14 (2), 255–271. doi:10.1111/ajt.12589
Gerlach, U. A., Klöpfel, M., Atanasov, G., Polenz, D., Vogt, K., Ahrlich, S., et al. (2017). Intragraft and Systemic Immune Parameters Discriminating between Rejection and Long-Term Graft Function in a Preclinical Model of Intestinal Transplantation. Transplantation 101 (5), 1036–1045. doi:10.1097/TP.0000000000001469
Haas, M. (2018). The Relationship between Pathologic Lesions of Active and Chronic Antibody-Mediated Rejection in Renal Allografts. Am. J. Transpl. 18 (12), 2849–2856. doi:10.1111/ajt.15088
Hara, M., Ishiguro, N., Katayama, K., Kondo, M., Sumida, T., Mimori, T., et al. (2014). Safety and Efficacy of Combination Therapy of Iguratimod with Methotrexate for Patients with Active Rheumatoid Arthritis with an Inadequate Response to Methotrexate: an Open-Label Extension of a Randomized, Double-Blind, Placebo-Controlled Trial. Mod. Rheumatol. 24 (3), 410–418. doi:10.3109/14397595.2013.843756
Horwitz, J. K., Chun, N. H., and Heeger, P. S. (2019). Complement and Transplantation: From New Mechanisms to Potential Biomarkers and Novel Treatment Strategies. Clin. Lab. Med. 39 (1), 31–43. doi:10.1016/j.cll.2018.10.004
Hou, G., Xue, L., Lu, Z., Fan, T., Tian, F., and Xue, Y. (2007). An Activated mTOR/p70S6K Signaling Pathway in Esophageal Squamous Cell Carcinoma Cell Lines and Inhibition of the Pathway by Rapamycin and siRNA against mTOR. Cancer Lett. 253 (2), 236–248. doi:10.1016/j.canlet.2007.01.026
Ishikawa, K., and Ishikawa, J. (2019). Iguratimod, a Synthetic Disease Modifying Anti-rheumatic Drug Inhibiting the Activation of NF-Κb and Production of RANKL: Its Efficacy, Radiographic Changes, Safety and Predictors over Two Years' Treatment for Japanese Rheumatoid Arthritis Patients. Mod. Rheumatol. 29 (3), 418–429. doi:10.1080/14397595.2018.1481565
Jakubzick, C. V., Randolph, G. J., and Henson, P. M. (2017). Monocyte Differentiation and Antigen-Presenting Functions. Nat. Rev. Immunol. 17 (6), 349–362. doi:10.1038/nri.2017.28
Jordan, S. C., Kucher, K., Bagger, M., Hockey, H. U., Wagner, K., Ammerman, N., et al. (2020). Intravenous Immunoglobulin Significantly Reduces Exposure of Concomitantly Administered Anti-C5 Monoclonal Antibody Tesidolumab. Am. J. Transpl. 20 (9), 2581–2588. doi:10.1111/ajt.15922
Koenig, A., Chen, C. C., Marçais, A., Barba, T., Mathias, V., Sicard, A., et al. (2019). Missing Self Triggers NK Cell-Mediated Chronic Vascular Rejection of Solid Organ Transplants. Nat. Commun. 10 (1), 5350. doi:10.1038/s41467-019-13113-5
Lebraud, E., Eloudzeri, M., Rabant, M., Lamarthée, B., and Anglicheau, D. (2022). Microvascular Inflammation of the Renal Allograft: A Reappraisal of the Underlying Mechanisms. Front. Immunol. 13, 864730. doi:10.3389/fimmu.2022.864730
Liao, X., Sharma, N., Kapadia, F., Zhou, G., Lu, Y., Hong, H., et al. (2011). Krüppel-like Factor 4 Regulates Macrophage Polarization. J. Clin. Invest. 121 (7), 2736–2749. doi:10.1172/JCI45444
Liao, X., Sharma, N., Kapadia, F., Zhou, G., Lu, Y., Hong, H., et al. (2011). Krüppel-like Factor 4 Regulates Macrophage Polarization. J. Clin. Invest. 121 (7), 2736–2749. doi:10.1172/JCI45444
Liu, S., Cui, Y., and Zhang, X. (2021). Molecular Mechanisms and Clinical Studies of Iguratimod for the Treatment of Ankylosing Spondylitis. Clin. Rheumatol. 40 (1), 25–32. doi:10.1007/s10067-020-05207-z
Louis, K., Hertig, A., Taupin, J. L., Buob, D., Jamme, M., Brocheriou, I., et al. (2019). Markers of Graft Microvascular Endothelial Injury May Identify Harmful Donor-specific Anti-HLA Antibodies and Predict Kidney Allograft Loss. Am. J. Transpl. 19 (9), 2434–2445. doi:10.1111/ajt.15340
Loupy, A., and Lefaucheur, C. (2018). Antibody-Mediated Rejection of Solid-Organ Allografts. N. Engl. J. Med. 379 (12), 1150–1160. doi:10.1056/NEJMra1802677
Mills, C. D. (2012). M1 and M2 Macrophages: Oracles of Health and Disease. Crit. Rev. Immunol. 32 (6), 463–488. doi:10.1615/critrevimmunol.v32.i6.10
Nunes-Dos-Santos, D. L., Gomes, S. V., Rodrigues, V. P., and Pereira, A. L. A. (2020). Periodontal Status and Clinical Outcomes in Kidney Transplant Recipients: A Systematic Review. Oral Dis. 26 (1), 22–34. doi:10.1111/odi.13040
Orecchioni, M., Ghosheh, Y., Pramod, A. B., and Ley, K. (2019). Macrophage Polarization: Different Gene Signatures in M1(LPS+) vs. Classically and M2(LPS-) vs. Alternatively Activated Macrophages. Front. Immunol. 10, 1084. doi:10.3389/fimmu.2019.01084
Patel, J. K., Coutance, G., Loupy, A., Dilibero, D., Hamilton, M., Kittleson, M., et al. (2021). Complement Inhibition for Prevention of Antibody-Mediated Rejection in Immunologically High-Risk Heart Allograft Recipients. Am. J. Transpl. 21 (7), 2479–2488. doi:10.1111/ajt.16420
Piotti, G., Palmisano, A., Maggiore, U., and Buzio, C. (2014). Vascular Endothelium as a Target of Immune Response in Renal Transplant Rejection. Front. Immunol. 5, 505. doi:10.3389/fimmu.2014.00505
Qiu, Y., Xu, J., Yang, L., Zhao, G., Ding, J., Chen, Q., et al. (2021). MiR-375 Silencing Attenuates Pro-inflammatory Macrophage Response and Foam Cell Formation by Targeting KLF4. Exp. Cel Res 400 (1), 112507. doi:10.1016/j.yexcr.2021.112507
Riquelme, P., Tomiuk, S., Kammler, A., Fändrich, F., Schlitt, H. J., Geissler, E. K., et al. (2013). IFN-γ-induced iNOS Expression in Mouse Regulatory Macrophages Prolongs Allograft Survival in Fully Immunocompetent Recipients. Mol. Ther. 21 (2), 409–422. doi:10.1038/mt.2012.168
Rodríguez-Perálvarez, M., De Luca, L., Crespo, G., Rubin, Á., Marín, S., Benlloch, S., et al. (2017). An Objective Definition for Clinical Suspicion of T-Cell-Mediated Rejection after Liver Transplantation. Clin. Transpl. 31 (7). doi:10.1111/ctr.13005
Shapouri-Moghaddam, A., Mohammadian, S., Vazini, H., Taghadosi, M., Esmaeili, S. A., Mardani, F., et al. (2018). Macrophage Plasticity, Polarization, and Function in Health and Disease. J. Cel Physiol 233 (9), 6425–6440. doi:10.1002/jcp.26429
Sharma, N., Lu, Y., Zhou, G., Liao, X., Kapil, P., Anand, P., et al. (2012). Myeloid Krüppel-like Factor 4 Deficiency Augments Atherogenesis in ApoE-/- Mice-Bbrief Report. Arterioscler Thromb. Vasc. Biol. 32 (12), 2836–2838. doi:10.1161/ATVBAHA.112.300471
Takahashi, K., and Yamanaka, S. (2006). Induction of Pluripotent Stem Cells from Mouse Embryonic and Adult Fibroblast Cultures by Defined Factors. Cell 126 (4), 663–676. doi:10.1016/j.cell.2006.07.024
Tao, J., Sun, L., Wang, Z., Chen, H., Han, Z., Zhang, H., et al. (2021). Efficacy and Safety of Iguratimod Supplement to the Standard Immunosuppressive Regimen in Highly Mismatched Renal Transplant Recipients: A Pilot Study. Front. Immunol. 12, 738392. doi:10.3389/fimmu.2021.738392
Valenzuela, N. M., McNamara, J. T., and Reed, E. F. (2014). Antibody-mediated Graft Injury: Complement-dependent and Complement-independent Mechanisms. Curr. Opin. Organ. Transpl. 19 (1), 33–40. doi:10.1097/MOT.0000000000000040
Wang, Z., Han, Z., Tao, J., Wang, J., Liu, X., Zhou, W., et al. (2017). Role of Endothelial-To-Mesenchymal Transition Induced by TGF-Β1 in Transplant Kidney Interstitial Fibrosis. J. Cel Mol Med 21 (10), 2359–2369. doi:10.1111/jcmm.13157
Wang, Z., Li, C., Sun, X., Li, Z., Li, J., Wang, L., et al. (2020). Hypermethylation of miR-181b in Monocytes Is Associated with Coronary Artery Disease and Promotes M1 Polarized Phenotype via PIAS1-KLF4 axis. Cardiovasc. Diagn. Ther. 10 (4), 738–751. doi:10.21037/cdt-20-407
Wen, Y., Lu, X., Ren, J., Privratsky, J. R., Yang, B., Rudemiller, N. P., et al. (2019). KLF4 in Macrophages Attenuates TNFα-Mediated Kidney Injury and Fibrosis. J. Am. Soc. Nephrol. 30 (10), 1925–1938. doi:10.1681/ASN.2019020111
Yan, Q., Du, F., Huang, X., Fu, Q., Chen, S., Dai, D., et al. (2014). Prevention of Immune Nephritis by the Small Molecular Weight Immunomodulator Iguratimod in MRL/lpr Mice. PLoS One 9 (10), e108273. doi:10.1371/journal.pone.0108273
Ye, L., He, S., Mao, X., Zhang, Y., Cai, Y., and Li, S. (2020). Effect of Hepatic Macrophage Polarization and Apoptosis on Liver Ischemia and Reperfusion Injury during Liver Transplantation. Front. Immunol. 11, 1193. doi:10.3389/fimmu.2020.01193
Zhang, M., Nakamura, K., Kageyama, S., Lawal, A. O., Gong, K. W., Bhetraratana, M., et al. (2018). Myeloid HO-1 Modulates Macrophage Polarization and Protects against Ischemia-Reperfusion Injury. JCI Insight 3 (19). doi:10.1172/jci.insight.120596
Zhao, D., Li, S., Liao, T., Wei, Y., Liu, M., Han, F., et al. (2018). Triptolide Inhibits Donor-specific Antibody Production and Attenuates Mixed Antibody-Mediated Renal Allograft Injury. Am. J. Transpl. 18 (5), 1083–1095. doi:10.1111/ajt.14602
Keywords: macrophage polarization, antibody-mediated rejection, KLF4, iguratimod, kidney transplant
Citation: Hang Z, Wei J, Zheng M, Gui Z, Chen H, Sun L, Fei S, Han Z, Tao J, Wang Z, Tan R and Gu M (2022) Iguratimod Attenuates Macrophage Polarization and Antibody-Mediated Rejection After Renal Transplant by Regulating KLF4. Front. Pharmacol. 13:865363. doi: 10.3389/fphar.2022.865363
Received: 29 January 2022; Accepted: 11 April 2022;
Published: 09 May 2022.
Edited by:
Chiara Bolego, University of Padua, ItalyReviewed by:
Quan Zhuang, Central South University, ChinaBaptiste Lamarthée, INSERM U1098 Interactions Hôte-Greffon-Tumeur and Ingénierie Cellulaire et Génique, France
Copyright © 2022 Hang, Wei, Zheng, Gui, Chen, Sun, Fei, Han, Tao, Wang, Tan and Gu. This is an open-access article distributed under the terms of the Creative Commons Attribution License (CC BY). The use, distribution or reproduction in other forums is permitted, provided the original author(s) and the copyright owner(s) are credited and that the original publication in this journal is cited, in accordance with accepted academic practice. No use, distribution or reproduction is permitted which does not comply with these terms.
*Correspondence: Zijie Wang, bmptdXd6akAxMjYuY29t; Min Gu, bGFuY2V0Z3VAYWxpeXVuLmNvbQ==; Ruoyun Tan, dGFucnVveXVuMTEyQHZpcC5zaW5hLmNvbQ==
†These authors have contributed equally to this work and share senior authorship