- 1School of Medicine, Shahid Beheshti University of Medical Sciences, Tehran, Iran
- 2School of Medicine, Tehran University of Medical Sciences, Tehran, Iran
- 3Department of Stem Cells and Developmental Biology, Cell Science Research Center, Royan Institute for Stem Cell Biology and Technology, ACECR, Tehran, Iran
- 4Department of Biology, Faculty of Natural Science, University of Tabriz, Tabriz, Iran
- 5Belfer Center for Applied Cancer Science, Department of Medical Oncology, Dana-Farber Cancer Institute, Harvard Medical School, Boston, MA, United States
- 6Translational Sciences, Xsphera Biosciences Inc., Boston, MA, United States
- 7Department of Microbiology, School of Biology, University College of Science, University of Tehran, Tehran, Iran
- 8Research Center for New Technologies in Life Science Engineering, University of Tehran, Tehran, Iran
Cellular differentiation is pivotal in health and disease. Interfering with the process of differentiation, such as inhibiting the differentiation of adipocytes and inducing the differentiation of cancer cells, is considered a therapeutic approach. Sesquiterpene lactones, primarily found in plants, have been attracted attention as differentiating/dedifferentiating agents tested on various human or animal cells. However, a consensus on sesquiterpene lactones’ effects and their mechanism of action is required. In this sense, through a systematic review, we have investigated the differentiating/dedifferentiating effects of sesquiterpene lactones on human or animal cells. 13 different cell lines originated from humans, mice, and rats, in addition to the effects of a total of 21 sesquiterpene lactones, were evaluated in the included studies. These components had either inducing, inhibiting, or no effect on the cells, mediating their effects through JAK-STAT, PI3K-Akt, mitogen-activated protein kinases, NFκB, PPARγ pathways. Although nearly all inducing and inhibiting effects were attributed to cancerous and normal cells, respectively, this is likely a result of a biased study design. Few studies reported negative results along with others, and no study was found reporting only negative results. As a result, not only are the effects and mechanism of action of sesquiterpene lactones not vivid but our knowledge and decisions are also misconducted. Moreover, there is a significant knowledge gap regarding the type of evaluated cells, other sesquiterpene lactones, and the involved signaling pathways. In conclusion, sesquiterpene lactones possess significant effects on differentiation status, leading to potentially efficient therapy of obesity, osteoporosis, and cancer. However, reporting negative results and further investigations on other cells, sesquiterpene lactones, and signaling pathways are highly suggested to pave the path of sesquiterpene lactones to the clinic more consciously.
Introduction
Cellular differentiation is perhaps one of the most crucial biological processes in almost all living creatures. Cells reorganize their facilities and redirect their functions to adapt to certain needs through differentiation; however, not all these reorganizations and redirections are commonly considered differentiation (Márquez-Zacarías et al., 2021). Changes of preadipocytes to adipocytes, hematopoietic stem cells to erythrocytes, macrophages to osteoclasts, and stem cells to epithelial cells are some instances of cellular differentiation.
Various human diseases are at least partially related to the process of differentiation. The early reports on the involvement of the differentiation process in cancer formation can be traced back to at least 100 years ago (Mallory, 1910). Over the years, the components of the differentiation process were identified, and differentiation therapy of cancer emerged (de Thé, 2018). In contrast, inhibition of differentiation is required to achieve therapeutic goals in other diseases. For instance, suppressing the differentiation of preadipocytes to adipocytes or macrophages to osteoclasts would prevent metabolic disorders (Gu et al., 2018) or osteoporosis (Jing et al., 2018), respectively.
They are currently medications such as all-trans retinoic acid (or known as Tretinoin, see (Wang and Chen, 2008) for clinical trials) for Acute Promyelocytic Leukemia treatment (Warrell et al., 1991) and statins (e.g., Atorvastatin (McCrindle et al., 2003)) for hyperlipidemia treatment (Nakata et al., 2006) that exert their therapeutic function through differentiation. There is a trend toward identifying more compounds for this purpose. Sesquiterpene lactones have been investigated extensively as differentiation-inducing/-inhibiting agents, either in vivo or in vitro. Sesquiterpene lactones can be isolated from plant extracts (Laid et al., 2008); however, semi-synthetic (Perri et al., 2019) and synthetic (Wedge et al., 2000) compounds also exist. Despite various derivations, the lactone ring and C15-terpenoids, built from three isoprene units (i.e., sesquiterpene), are shared in all members of this group (Aliarab et al., 2018). Differentiation and dedifferentiation therapies are a growing field, and sesquiterpene lactones can alter differentiation. Therefore, a comprehensive understanding of how different sesquiterpene lactones affect cell differentiation is necessary.
Two systematic reviews have investigated the effects of sesquiterpene lactones on cancer cells and skin cells (da Silva Castro et al., 2018; da Silva et al., 2020); however, no systematic review has been conducted focusing on the effect of sesquiterpene lactones on cellular differentiation, to the best of our knowledge. Herein, we have conducted a systematic review on the differentiating/dedifferentiating effects of sesquiterpene lactones on various human or animal cells, evaluated in vitro. Both inducing and inhibiting effects of sesquiterpene lactones on differentiation are covered in this survey to scheme a general idea regarding their effects. Moreover, this review briefly discusses the underlying mechanisms of sesquiterpene lactones’ effects on differentiation. The gap in our current knowledge about this field is identified and suggestions for future researches are also discussed. It is worth noting, due to data heterogeneity (i.e., variation in cell and lactone type, and variation in measurement tools), and lack of quantitative measurements in some studies, we were not able to conduct a meta-analysis (as in (da Silva Castro et al., 2018; da Silva et al., 2020)).
Methods
Search Strategy and Databases
This systematic review was conducted according to the PRISMA (Preferred Recording Items for Systematic Reviews and Meta-analyses) statement (www.prisma-statement.org). We have asked how sesquiterpene lactones would affect the differentiation status of human or animal cells in an in vitro experiment. A preliminary search was conducted to obtain keywords for a systematic search. “Sesquiterpene lactone” and “differentiation” were selected as primary keywords; however, the search string was formed based on other derivations of these two keywords, according to MeSH (www.ncbi.nlm.nih.gov/mesh). Scopus, Web of Science, and MEDLINE were searched for the current survey. No limitations were set in terms of date, but the language of the results was limited to English. The detailed search string for each database can be found in supplementary material 1.
Eligibility Criteria
Any in vitro study on animal or human cells (population) which the treatment was purified sesquiterpene lactones and not crude plant extract (exposure) with an untreated or vehicle control group, with or without a positive control group (comparison), assessing the status of cellular differentiation, either induction or inhibition (outcome) were included. The article should clearly state the occurrence of differentiation with any manifestation as the primary outcome (e.g., morphological, biochemical, gene expression profile alternation, and biomarkers expression). Any interpretation by the authors of the current systematic review was prohibited. Non-comparative studies, including abstracts, opinions, reviews, and systematic reviews, were excluded. The studies evaluating the concurrent (synergistic or antagonistic) effect of sesquiterpene lactones and another component on the induction of differentiation were excluded. The studies reporting the duration and concentration of treatment as a range were excluded since the exact condition of induction or inhibition of differentiation and a general conclusion could not be obtained from these studies. All cells with either cancerous or non-cancerous (at any stage of differentiation) were acceptable. In addition, genetic modification of the cells was not acceptable since it imposes an unknown impact on the cellular physiology and the effects sesquiterpene lactones. Moreover, through the last step of screening, the studies were screened in terms of significant inaccuracy in study design and analysis with major impact on the interpretation of results and those with no statistical analysis in the presentation and explanation of results, not specified duration and concentration of treatment, and not dedicated control group.
Study Selection
The extracted studies from the databases were first screened for the key components of the question of the review (population, exposure, comparison, and outcome) by their title and abstract by two authors independently. Similarly, the included studies from the last stage were assessed by their full text by two authors independently. Discrepancies were resolved by discussion or a third reviewer if they remained.
Data Extraction
The extraction of data was conducted by two authors independently. Discrepancies were resolved by discussion or a third reviewer if they remained. The following data were extracted: the name of publication, cell name and type, the origin of the cell (species), Kind of the cell (i.e., cancer cell, differentiated cell, and stem cell), sesquiterpene lactone name, the origin of sesquiterpene lactone (i.e., natural, semi-synthetic, synthetic), the concentration of treatment, duration of treatment, differentiation status after treatment (i.e., induction, inhibition, or no response), control group, detection method, involved genes, and pathways.
Quality Assessment
Since no standard checklist exists for in vitro studies, we have created our criteria based on the most important components of in vitro studies to adapt our requirements. We have assessed studies by nine items. The items are designed to evaluate the selected studies after the study selection process regarding their precise, rigor, and comprehensive description of study design and data presentation. No study, selected according to the eligibility criteria will be eliminated based on the quality assessment. The items were answered by yes or no. Quality assessment was done independently by two authors. Discrepancies were resolved by discussion or a third reviewer if they remained. The traffic light plot is created using robvis tool (McGuinness and Higgins, 2020).
Data Synthesis
The studied cells were collected, categorized into cancer, normal, and stem cells, and their developmental origin was determined. The effects of sesquiterpene lactones on differentiation status regarding the dose range and duration of treatment were investigated. We further evaluated if some sesquiterpene lactones had double responses in single or multiple cell lines. The involved pathways were determined in inhibiting and inducing effect groups and compared to obtain a general scheme of the underlying mechanism in each group. The biological assays used in the studies were collected and analyzed to demonstrate the level of evidence on the status of differentiation.
Protocol Registration
The protocol of this systematic review was registered on 28 September 2021, on Open Science Framework (http://www.osf.io) before the stage of data extraction with a registration DOI of 10.17605/OSF.IO/T7QPJ.
Results and Discussion
Selected Studies
A total of 594 studies were identified from Scopus, MEDLINE, and Web of Science databases regarding the keywords. After eliminating duplicates manually or by Mendeley, 345 studies remained for the title- and abstract-screening. 202 and 105 studies failed to fulfill the inclusion criteria in the title and abstract and full-text screening, respectively. The remaining 38 studies screened for inaccuracy in study design or statistical analysis. Despite meeting the inclusion criteria, 12 studies lacked statistical analysis, 1 study stated no treatment concentration, and two studies did not report the duration of treatment accurately and therefore eliminated. As a result, 23 studies were included in the review. The follow chart of study inclusion and exclusion is demonstrated in Figure 1.
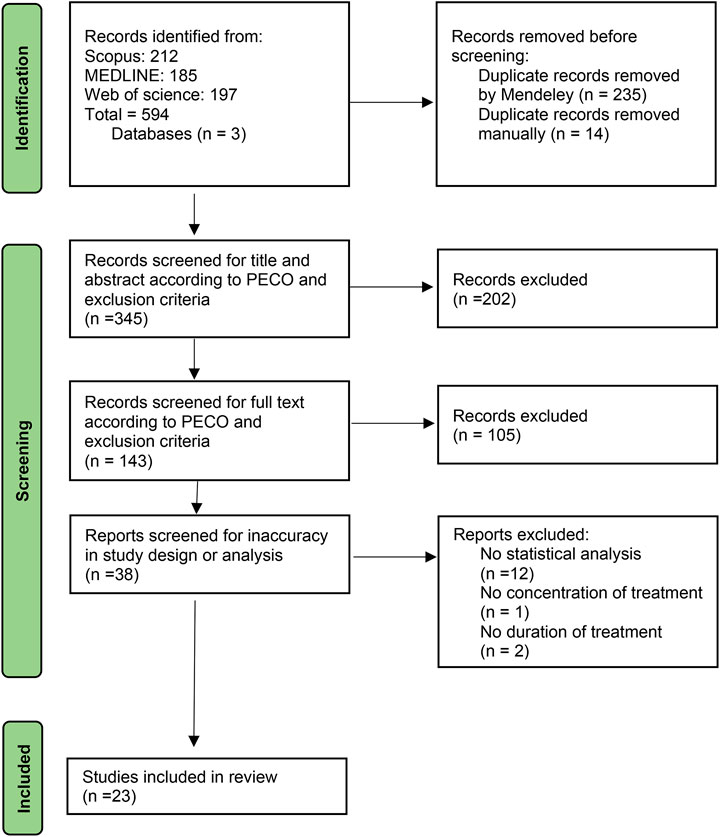
FIGURE 1. Flow chart of study inclusion and exclusion steps and study selection. PECO: Population, Exposure, Comparison, Outcome
Quality Assessment
The quality assessment of included studies is illustrated in the traffic light plot (Figure 2). All studies did not fulfill at least one criteria. Among 23 studies, 17 studies failed to mention all data accurately according to the study design. About 47 and 39% of studies did not fulfill one or two criteria. Moreover, two studies did not fulfill three criteria, and one study did not fulfill four criteria. No studies were removed based on quality assessment for further steps. The studies that fail to meet these criteria are difficult to replicate, interpret, and be used in further studies. Therefore, more strict and rigor guidelines are needed to standardize the study design and results description in the articles.
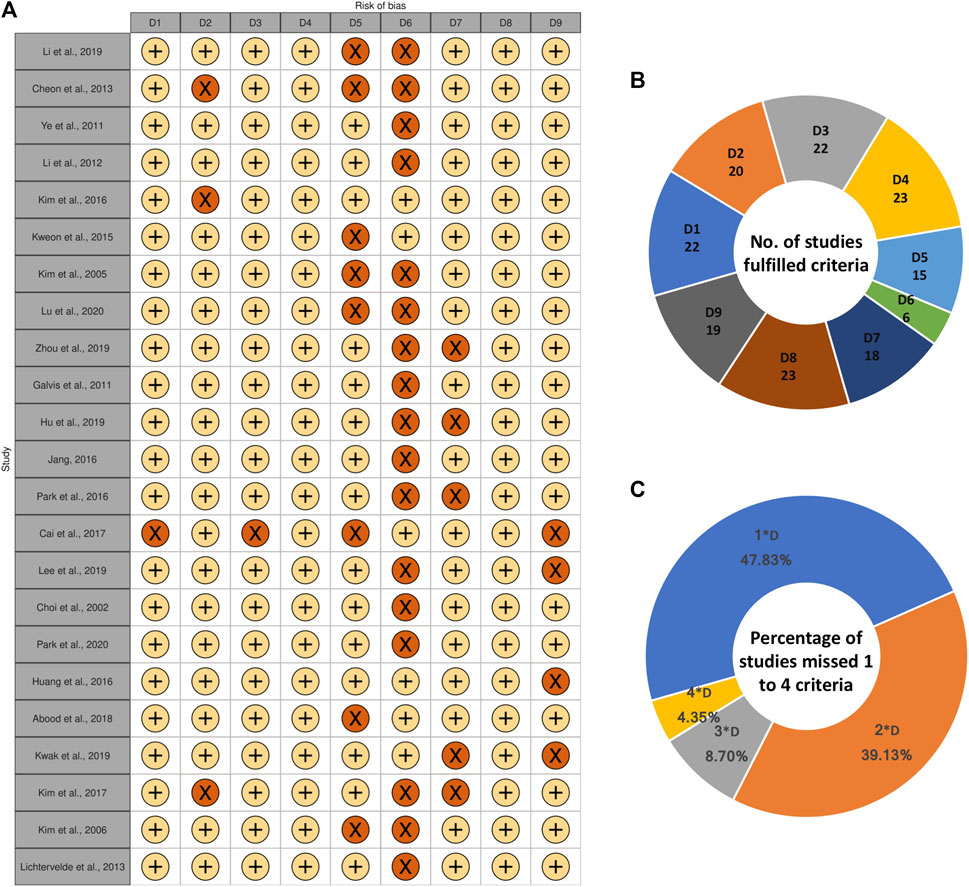
FIGURE 2. (A) Quality assessment of included studies illustrated in traffic light plot. D1: Does the study report a well-defined control group? D2: Can the origin (species and the cell bank obtained from) of the studied cells be confirmed? D3: Does the study mention the duration of treatment clear and accurate (Ranges are not accepted)? D4: Does the study mention the concentration of treatment clear and accurate (Ranges are not accepted)? D5: Does the study mention the condition of cell culture in details (culture medium, additives, atmosphere, temperature)? D6: Does the study mention all data accurately according to the study design? D7: Is the lactone either characterized by authors or bought from identified company? D8: Does the study mention the detection method of differentiation? D9: Does the study mention experimental repetitions? +: YES, ×: NO, (B) Number of studies fulfilled each criterion, (C) Percentage of studies which missed 1, 2, 3, or four criteria among nine criteria.
Evaluated Cells
A total of 13 different cell lines originated from humans, mice, and rats were evaluated in the studies (Figure 3). Among them, four cells were cancer cell lines including, mice melanoma B16 cells (Ye et al., 2011), human HL-60 cell line (Choi et al., 2002; Kim et al., 2005; Kim et al., 2006; Kweon et al., 2015), human CML cell line K562 (Huang et al., 2016; Cai et al., 2017), human AML cell line U937 (Huang et al., 2016), three cells were stem cells including rat mesenchymal stem cell (Li et al., 2012), mice mesenchymal stem cells C3H10T1/2 (Kim et al., 2017), hematopoietic stem and progenitor cells (HSPCs) (de Lichtervelde et al., 2013), and six cells were normal cell lines including, 3T3-L1 cell line (Galvis et al., 2011; Jang, 2016; Kim et al., 2016; Abood et al., 2018; Kwak and Kim, 2019), mice bone marrow macrophage (Cheon et al., 2014; Hu et al., 2019; Lee et al., 2019; Li et al., 2019; Zhou et al., 2019; Lu et al., 2020), CD4+ T cell (Park et al., 2016), human periosteum-derived cells (Park et al., 2020), human preadipocytes (Jang, 2016), mice MC3T3-E1 cell line (Kim et al., 2017). All cell lines except the murine melanoma B16 cell line had a mesodermal origin.
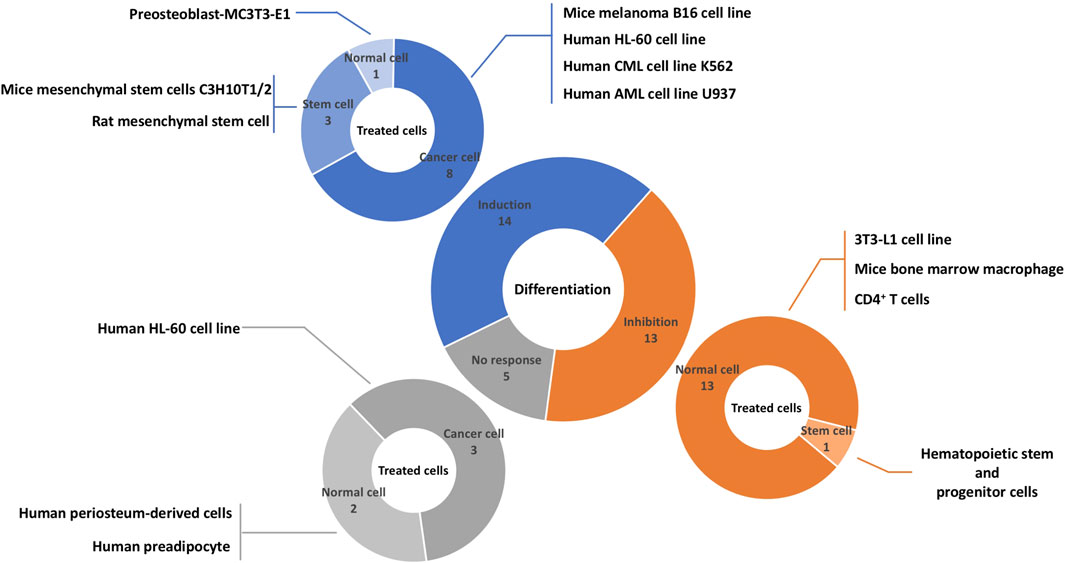
FIGURE 3. Overview of literature on the effects of sesquiterpene lactones on differentiation and affected cells. Sesquiterpene lactones either induced differentiation, inhibit it, or had no effect. The effects of sesquiterpene lactones were evaluated in normal and cancer cell lines and stem cells. The numbers represent the experiments and not papers.
Differentiation of germ layer to endoderm, mesoderm, and ectoderm and differentiation of these lineages to specific cells and tissues involve specific signaling pathways leading to different cellular characteristics (Murry and Keller, 2008; Xie et al., 2013). Therefore, the developmental origin of cells might be influential on the quality of response to differentiating or dedifferentiating agents. There is currently a significant gap of knowledge in the effects of sesquiterpene lactones on other cells with mesodermal origins in addition to the cells with endodermal and ectodermal origins. This is of importance in both basic and clinical research. For instance, dedifferentiation is considered a crucial step in state-of-the-art therapeutic approaches, specifically regenerative medicine (Jopling et al., 2011; Tata et al., 2013). Dedifferentiation of epithelial cells or cardiomyocytes to regenerate the kidney and heart in acute renal failure and heart failure is demonstrated as an effective treatment (Jopling et al., 2010; Allison, 2020). Moreover, differentiation therapy is considered an effective treatment in various cancers (Sell, 2004). Differentiation therapy would also benefit diabetes mellitus, routing potentially from the dedifferentiation of pancreatic beta-cells (Dor and Glaser, 2013). Sesquiterpene lactones might also be found helpful in such applications, and further researches is recommended.
Investigating the effects of sesquiterpene lactones on other cells with different origins may facilitate establishing a general scheme on the mechanism of action of sesquiterpene lactones and their targets. Moreover, the general administration of sesquiterpene lactones to the body may interfere with the differentiation of unwanted targets; hence, expanding the investigations in this field to other cells would increase the ability to predict adverse effects. The effect of sesquiterpene lactones on tissues or multiple physiologically-related cells is also missed in the literature. Sesquiterpene lactones can be metabolized by certain cells, leading to active metabolites potentially interfering with the differentiation status of other cells (Yu et al., 2021).
Sesquiterpene Lactones and Their Effects
Sesquiterpene lactones are a diverse family of bioactive molecules primarily discovered in plants. Sesquiterpene lactones play important roles in plants and are beneficial due to their anti-herbivory effects, anti-microbial activity, and allelopathic function. They may also act as a defense against ozone damage in plants (Chadwick et al., 2013). The health benefits of sesquiterpene lactones for people have been widely investigated. Their diverse bioactivities such as anti-microbial activity, anti-malaria activity, anti-tumor activity, and anti-inflammatory activity have drawn a considerable interest into their therapeutic potentials (Shoaib et al., 2017).
A total of 21 sesquiterpene lactones were evaluated in the selected studies (Figure 4). Sixteen sesquiterpene lactones had a natural origin, and four of them were semi-synthetic (i.e., synthesized from a natural sesquiterpene lactone with further modifications). The sesquiterpene lactones had inducing, inhibiting, or no effects on the differentiation status of the cells. Through investigating the inducing effects, the cells were solely treated with sesquiterpene lactones. On the other hand, for investigating the inhibiting effects, the cells were primarily treated with approved differentiating agents in addition to sesquiterpene lactones. Three sesquiterpene lactones, including artesunate, dehydrocostus lactone, and zaluzanin C, had multiple responses in different cells lines. artesunate inhibited the differentiation of the 3T3-L1 cell line and had no effect on human preadipocytes (Jang, 2016). dehydrocostus lactone inhibited the differentiation of bone marrow-derived macrophages (also referred to as primary murine bone monocyte/macrophage precursors) (Hu et al., 2019; Lee et al., 2019; Li et al., 2019) and induced the differentiation of the K562 CML cell line (Cai et al., 2017). zaluzanin C inhibited the differentiation of the 3T3-L1 cell line (Kwak and Kim, 2019) and induced the differentiation of mesenchymal stem cells C3H10T1/2 and preosteoblast MC3T3-E1 (Kim et al., 2017). The effective dose of sesquiterpene lactones evaluated in studies ranged from 0.5 to 120 μM for inhibiting effects and 2 to 217 μM for inducing effects. The effective duration of treatment evaluated in studies ranged from 2 to 9 days for inhibiting effects and 2–7 days for inducing effects.
Sesquiterpene lactones also impose other effects on human and animal cells. Sesquiterpene lactones interfere with the cell cycle, inhibit proliferation, and induce apoptosis (Guzman et al., 2005; Yami et al., 2020). In some studies, the effective concentration to exert these effects is overlapped with the concentration effective for inducing or inhibiting differentiation [for instance as in (Guzman et al., 2005) and (Li et al., 2020)], which needs to be clarified in future studies. Moreover, the number of studies on each sesquiterpene lactone is extremely low, limiting a robust conclusion on the true effects and mechanism of actions of each molecule. More than thousands of sesquiterpene lactones exist (Acevedo et al., 2018). Investigations on these molecules are also suggested as minor structural variations between molecules would make some of them more effective for certain purposes (Schmidt, 2006; Tang et al., 2018). For instance, addition of morpholino and dimethylamino groups to sesquiterpene lactones would increase the in vitro activity over native sesquiterpene lactones (Zimmermann et al., 2014). In addition, extensive research on other sesquiterpene lactones would lead to a structure-response library suitable for modeling and prediction.
Pathways Aaffected by Ssesquiterpene Lactones
Four main pathways, including JAK-STAT, PI3K-Akt, MAPK, and NFκB, were commonly involved in both inhibiting and inducing effects of sesquiterpene lactones, which the main components are illustrated in Figure 5. PPARγ pathway was only reported in the studies on inhibiting effects of sesquiterpene lactones; however, it was not evaluated in the studies investigating the inducing effects of sesquiterpene lactones, and its role cannot be ruled out.
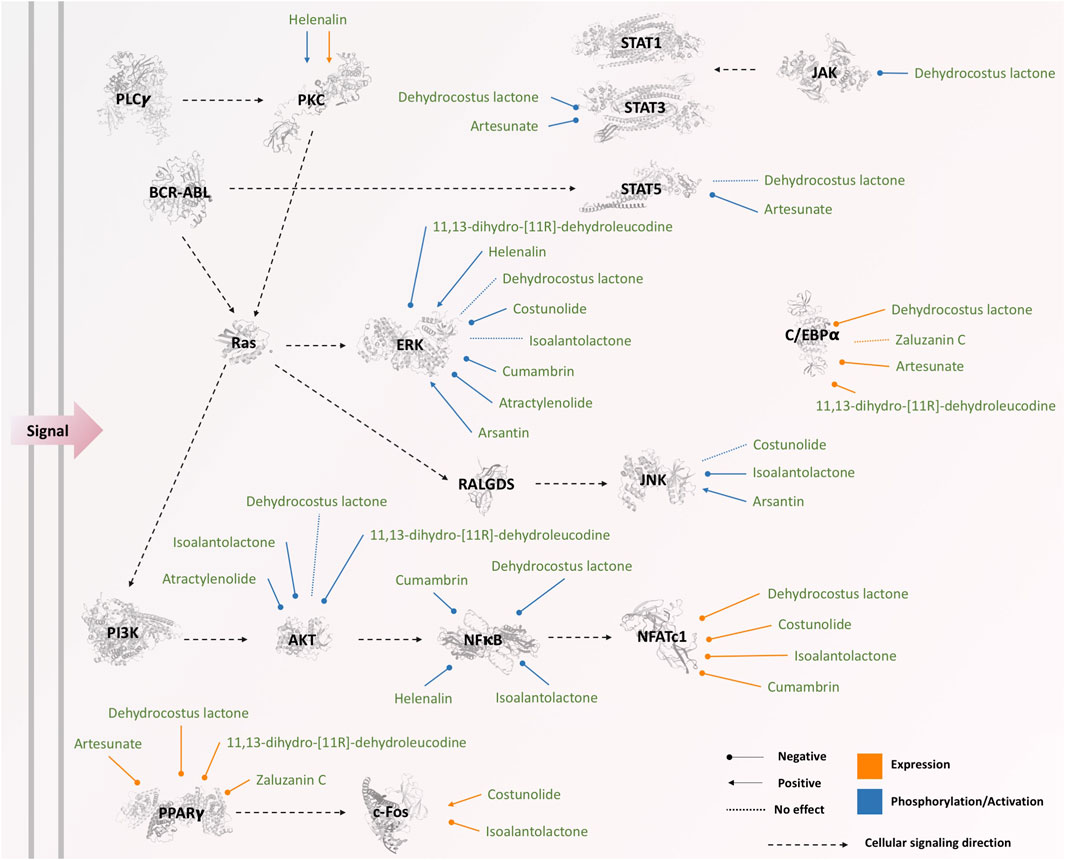
FIGURE 5. The effects of sesquiterpene lactones on different components of signaling pathways. The phosphorylation status of JAK, BCR-ABL, ERK, PKC, STATs, PI3K, AKT, and NFκB was affected by sesquiterpene lactones, while the expression of NFATc1, PKC, c-Fos, PPARγ, and C/EBPα was changed as a result of the treatment. The three-dimensional illustrations of proteins were retrieved from Protein Data Bank in Europe (PDBe) hosted by EMBL-EBI (https://www.ebi.ac.uk/pdbe).
MAPK Pathway
The mammalian mitogen-activated protein kinases (MAPK) family consists of three members, including extracellular signal-reduced kinase (ERK), p38, and c-Jun NH2-terminal kinase (JNK) each exists in several isoforms (Kim and Choi, 2010). In order to activate a MAPK, MAPK-kinase kinase should phosphorylate MAPK kinase, which in turn the latter will phosphorylate and activate MAPK (Wagner and Nebreda, 2009). Activated MAPK plays a pivotal role in cell proliferation, apoptosis, inflammation, and differentiation (Wagner and Nebreda, 2009).
The phosphorylation of some components from the MAPK pathway, such as ERK, p38, and JNK, was evaluated during inducing or inhibiting differentiation via sesquiterpene lactones in some of the studies (n = 10) included in this systematic review (Table 1). The level of phosphorylation of these components was either increased (Kim et al., 2005; Kweon et al., 2015), decreased (Ye et al., 2011; Park et al., 2016; Abood et al., 2018; Zhou et al., 2019; Lu et al., 2020), or unchanged (Galvis et al., 2011; Cheon et al., 2014; Park et al., 2016; Li et al., 2019; Lu et al., 2020); however, there was no association between the status of phosphorylation and induction or inhibition of differentiation. The expression of c-Fos downstream of the MAPK pathway is also affected by sesquiterpene lactones (Cheon et al., 2014; Lu et al., 2020). Two studies (Galvis et al., 2011; Li et al., 2019) reporting inhibitory effects of dehydrocostus lactone on differentiation unanimously stated that dehydrocostus lactone does not affect the phosphorylation of ERK, and two studies (Cheon et al., 2014; Park et al., 2016) reporting inhibitory effects of costunolide unanimously stated that costunolide does not affect the phosphorylation of JNK. Other studies did not report opposite observations; however, further investigation is required to confirm the effects of dehydrocostus lactone and costunolide on differentiation since the current studies are insufficient to obtain a general conclusion.
NFκB Pathway
NFκB is a family of transcription factors including five genes (NFκB1, NFκB2, RelA, c-Rel, and RelB) that encode seven proteins (p50, p105, p52, p100, p65, c-Rel, RelB). Many signaling pathways might activate the NFB pathway through classical or alternative pathways; either way, activated NFκB is translocated to the nucleus and acts as a transcription factor. Cell differentiation, cell survival, and inflammation may be affected by the NFκB pathway (Dolcet et al., 2005).
Included studies in this systematic review reported that sesquiterpene lactones also affect the NFκB pathway (Kim et al., 2005; Zhou et al., 2019; Li et al., 2019; Hu et al., 2019; Lu et al., 2020). The phosphorylation level of NFκB was decreased in response to sesquiterpene lactones with either inhibiting or inducing effects on differentiation (Table 1). Among five studies evaluating NFκB, one reported inducing effects (Kim et al., 2005), and the others reported inhibiting effects (Hu et al., 2019; Li et al., 2019; Zhou et al., 2019; Lu et al., 2020). In the downstream of NFκB, the expression level of NFATc1 has also been shown to be reduced by sesquiterpene lactones unanimously in all studies evaluating their effects (Cheon et al., 2014; Lee et al., 2019; Li et al., 2019; Zhou et al., 2019; Lu et al., 2020). Although the studies evaluating NFκB concluded that this pathway is responsible for the occurrence or blockage of differentiation (as in (Hu et al., 2019)), our observation on these data suggests that NFκB might be affected as a general response to sesquiterpene lactones, not directly related to differentiation. In other words, other pathways might be more effective, or the cumulative effects of a set of pathways might redirect the response. Moreover, the structural differences of sesquiterpene lactones, the physiological condition of cells, and the treatment condition in different studies are other parameters limiting the generalization. It is worth noting that two studies (Hu et al., 2019; Li et al., 2019) reporting inhibitory effects of dehydrocostus lactone on differentiation unanimously reported that dehydrocostus lactone does not affect the phosphorylation of NFκB.
JAK-STAT Pathway
In mammals, JAK and STAT family consists of four (JAK1, JAK2, JAK3, TYK2) (Stark et al., 1998) and seven (STAT1, STAT2, STAT3, STAT4, STAT5a, STAT5b, STAT6) proteins, respectively (Copeland et al., 1995; Darnell, 1997). Different recruitment of JAKs and STATs is based on the cell types and receptors engaged in the signaling pathway (Rawlings et al., 2004). The binding of ligand to the JAK-binding transmembrane receptors allows them to multimerize, leading to JAK activation through trans-phosphorylation. Activated JAKs subsequently phosphorylate STATs. Phosphorylated STATs dimerize and translocate to the nucleus and act as transcription factors (Murray, 2007). Activated STATs have anti-apoptosis activity and also affect Cell-cycle progression, cell-cycle inhibition, Lipid metabolism, and differentiation according to KEGG database (Kanehisa and Goto, 2000; Kanehisa, 2019; Kanehisa et al., 2021). JAK-STAT pathway has been shown to be affected by sesquiterpene lactones in the studies included in this systematic review (Jang, 2016; Cai et al., 2017). The phosphorylation level of STAT3, STAT5, and JAK is reduced through both induction and inhibition of differentiation via sesquiterpene lactones (Table 1).
PI3K-Akt Pathway
Phosphatydilinositol-3-kinase (PI3K) can be stimulated by many growth factors and regulators (Vivanco and Sawyers, 2002). Activated PI3K generates phosphatidylinositol-3,4,5-trisphosphate, which helps AKT to translocate to the plasma membrane where it gets phosphorylated and hence activated (Osaki et al., 2004; Hennessy et al., 2005). Activated PI3K/AKT pathway may play a pivotal role in cell cycle progression, apoptosis, and differentiation (Kanehisa, 2019; Kanehisa and Goto, 2000; Kanehisa et al., 2021; Delgoffe et al., 2011). Also, PI3K/AKT pathway can indirectly affect cellular differentiation by interfacing with other signaling pathways such as the NFκB pathway (through IKK activation) and JAK/STAT pathway (Kanehisa, 2019; Kanehisa and Goto, 2000; Kanehisa et al., 2021). The involvement of the PI3K-AKT pathway in response to three sesquiterpene lactones (i.e., atractylenolide I and II, 11,13-dihydro-[11R]-dehydroleucodine, isoalantolactone) through AKT has been reported (Ye et al., 2011; Abood et al., 2018; Lu et al., 2020). The level of phosphorylation of AKT was decreased in treatment with three lactones with either inhibiting or inducing effects (Table 1). However, one lactone (dehydroleucodine) failed to affect the phosphorylation of AKT (Galvis et al., 2011).
PPARγ and C/EBPα Pathway
The expression of PPARγ and C/EBPα have been decreased in response to sesquiterpene lactones (Table 1). However, in one study, the expression of C/EBPα remained unchanged in response to zalzuzanin (Kwak and Kim, 2019). The involvement of the PPARγ pathway and C/EBPα was only reported in the studies investigating the inhibitory effects of sesquiterpene lactones. This might be because of the experimental designs as the PPARγ pathway and C/EBPα are involved in adipogenesis and lipid metabolism (Farmer, 2005; Lefterova et al., 2008), which were intended to be inhibited by sesquiterpene lactones. Therefore, still, the question remains that how the PPARγ pathway and C/EBPα are affected by sesquiterpene lactones in other cells, especially cancer cells.
Miscellaneous Pathways
In one study, the expression of SHH and GLI was increased by atractrynolide with inducing effects on differentiation, indicating the possible involvement of Sonic-Hedgehog signaling pathway in the differentiation (Li et al., 2012). Sesquiterpene lactones are also found to decrease the expression of Fas involved in apoptosis (Jang, 2016; Abood et al., 2018), increase the phosphorylation and expression of PKC involved in signaling in general (Kim et al., 2005) and increase the expression of p27 and p21 and decrease the expression of c-Myc involved in the cell cycle (Choi et al., 2002; Abood et al., 2018).
Biological Assays for Evaluating Differentiation
Physiological cellular differentiation is routed from comprehensive alteration of various hierarchical molecular mechanisms in the cells. Gene and protein profiles of the cells change during cellular differentiation or dedifferentiation, and miRNA profiles can also be indicative of differentiation status (Letzen et al., 2010; Branco et al., 2015; Frese et al., 2017). More primarily, chromatin remodeling is identified as a pivotal step in this process (Chen and Dent, 2014). Molecular-level changes would lead to functional and morphological changes, measurable by colorimetric and enzymatic assays, microscopy, and flow cytometry. The process of differentiation, as a continuous spectrum of events, as well as the final status of differentiation, can be measured at every molecular, functional, and morphological level (Clark, 1957; Chen et al., 1989; Moyes et al., 1997; Flaim et al., 2005). In this sense, evaluating various molecular, functional, and morphological levels of the cells is required for the most confident assessment of cellular differentiation.
Various types of assays such as morphological and molecular assays, microscopy, colorimetry, flow cytometry, and enzyme activity assay were applied in the selected studies to evaluate the status of differentiation. These assays were used for confirmation of differentiation or studying the underlying mechanism of it. Nineteen studies applied qRT-PCR or western blot techniques to assess the sesquiterpene lactones’ underlying mechanism of action. Only eight studies used flow cytometry to approve the status of differentiation. The assays used for evaluation of differentiation are summarized in the Table 2.
In some cases, the assays were not sufficient to confirm the differentiation status with high certainty. Differentiation and dedifferentiation affect all aspects of cellular physiology initiated from the upstream. Evaluating limited aspects of the cells would not necessarily demonstrate the status of differentiation. As an example, limited morphological studies or alteration in specific markers do not prove the redirection of cellular fate into a new differentiation status (Alt et al., 2011). Flow cytometry, gene expression (e.g., RT-PCR, and RNA-Seq), protein expression (e.g., immunoblotting, enzyme-linked immunosorbent assay, and mass spectrometry), imaging (e.g., electron microscopy, immunocytochemistry), specific staining techniques, cellular function assay, and motility assay can be used to confirm the differentiation status more accurately. Using these techniques together may further increase the accuracy. It is also worth noting that all the studies were biased to use the assays to confirm their primary hypothesis and not to rule out the other possibilities (Pannucci and Wilkins, 2010). The assays, although insufficient, demonstrated that the cells were differentiated or dedifferentiated; however, some questions still are remained: What are the other effects of sesquiterpene lactones on cellular physiology (e.g., metabolism, gene expression, and proliferation)? Will all these other effects be in line with the observed status of differentiation? How does one sure the treated cells do not obtain other characteristics during treatments rather than those assumed primarily?
Overlooking Negative Results and Potential Misconception in Systematic Reviews
Both induction and inhibition of differentiation are considered therapeutic approaches in specific conditions. Not surprisingly, the results of all studies were in line with their objectives, either inhibiting or inducing effects. Only some studies (Kim et al., 2006; Jang, 2016; Park et al., 2020) reported the negative results, which were either evaluating and comparing the effects of other sesquiterpene lactones or non-lactone derivatives, or the primary objective of the study was not evaluating the differentiation. Moreover, the occurrence of reverse effects was not reported in any studies. The observation above might relate the differentiation status to the cells’ condition (i.e., cancerous or normal), leading to a potential misapprehension that sesquiterpene lactones induce differentiation in normal cells and inhibit it in cancer cells. However, this seems unlikely and biased since, in contrast to clinical researches, there is a much less tendency to report negative results, and many journals do not consider them in this field.
It has been a long time that researchers have expressed their concerns regarding the under-reporting of negative results which generally arises form “publication bias” (Earp, 2018). In fact, data from a 2012 study of more than 4,000 published papers show that scientific literature as a whole is trending towards more reports of positive results reached to more than 85% of published studies by 2007 (Mehta, 2019). In this case, a lucky lab with a positive result among many other labs with negative results have a chance to publish their findings, leading to a misconception; however, a cumulative evaluation of both positive and negative results could have unveiled that the positive result was a fluke, a basic statistical issue (Earp, 2018). In the absence of negative results, obtaining true-positive conclusions through systematic reviews and meta-analysis would be devastated. Interestingly, a simulation study confirmed that a higher probability of including positive results would increase the magnitude of the effect of publication bias, leading to an increase of the false-positive rate in meta-analysis (Kicinski, 2014).
The current approach for in vitro studies would slacken the progress in producing knowledge about sesquiterpene lactones’ effects on various cells and their underlying mechanism of action. Therefore, the answer to why sesquiterpene lactones affect differentiation differently remains unclear. Moreover, as a result, the translation of these molecules to in vivo studies and human trials will be limited. Waste of resources on not-reported failed experiments and manipulation of data to get papers published would be other problems rise from under-reporting negative results (Mehta, 2019).
Conclusion
Sesquiterpene lactones can both inhibit and induce differentiation in various cells. Their differentiating/dedifferentiating effects are mediated through JAK-STAT, PI3K-Akt, MAPK, NFκB, PPARγ pathways. Moreover, they interfere with the cell cycle and apoptosis. However, extensively biased study design, under-reporting negative results (publication bias), a significant gap of knowledge regarding the other types of cells, sesquiterpene lactones, and involved pathways hamper a robust conclusion on the effects of sesquiterpene lactones and their mechanism of actions. Future studies should address these issues to prepare the knowledge basis and facilitate the translation of sesquiterpene lactones to the clinic.
Data Availability Statement
The raw data supporting the conclusion of this article will be made available by the authors, without undue reservation.
Author Contributions
SETF and SATF did the systematic search, SETF and SATF wrote the main manuscript, SETF prepared the figures, SATF prepared the tables, AA, FS, and MM edited the manuscript and AA and AS-N supervised the study. All authors reviewed the manuscript.
Conflict of Interest
AA is the vice president at Xsphera Biosciences Inc.
The remaining authors declare that the research was conducted in the absence of any commercial or financial relationships that could be construed as a potential conflict of interest.
Publisher’s Note
All claims expressed in this article are solely those of the authors and do not necessarily represent those of their affiliated organizations, or those of the publisher, the editors, and the reviewers. Any product that may be evaluated in this article, or claim that may be made by its manufacturer, is not guaranteed or endorsed by the publisher.
Supplementary Material
The Supplementary Material for this article can be found online at: https://www.frontiersin.org/articles/10.3389/fphar.2022.862446/full#supplementary-material
References
Abood, S., Veisaga, M. L., López, L. A., and Barbieri, M. A. (2018). Leucodine Blocks Mitotic Clonal Expansion during Preadipoctye Differentiation through Cell Cycle Arrest. Phytochemistry Lett. 24, 79–87. doi:10.1016/j.phytol.2017.12.011
Acevedo, C. H., Scotti, L., and Scotti, M. T. (2018). In Silico Studies Designed to Select Sesquiterpene Lactones with Potential Antichagasic Activity from an In-House Asteraceae Database. ChemMedChem 13 (6), 634–645. doi:10.1002/cmdc.201700743
Aliarab, A., babaei, G., Abroon, S., Rasmi, Y., and Aziz, S. G-G. (2018). Application of Sesquiterpene Lactone: A New Promising Way for Cancer Therapy Based on Anticancer Activity. Biomed. Pharmacother. 106, 239–246. doi:10.1016/j.biopha.2018.06.131
Allison, S. J. (2020). Contribution of Dedifferentiated Proximal Tubule Cells to Repair in Acute Kidney Injury. Nat. Rev. Nephrol. 16 (2), 65. doi:10.1038/s41581-019-0235-3
Alt, E., Yan, Y., Gehmert, S., Song, Y. H., Altman, A., Gehmert, S., et al. (2011). Fibroblasts Share Mesenchymal Phenotypes with Stem Cells, but Lack Their Differentiation and colony-forming Potential. Biol. Cel 103 (4), 197–208. doi:10.1042/BC20100117
Branco, A. F., Pereira, S. P., Gonzalez, S., Gusev, O., Rizvanov, A. A., and Oliveira, P. J. (2015). Gene Expression Profiling of H9c2 Myoblast Differentiation towards a Cardiac-like Phenotype. PloS one 10 (6), e0129303. doi:10.1371/journal.pone.0129303
Cai, H., Qin, X., and Yang, C. (2017). Dehydrocostus Lactone Suppresses Proliferation of Human Chronic Myeloid Leukemia Cells through Bcr/Abl-JAK/STAT Signaling Pathways. J. Cel Biochem 118 (10), 3381–3390. doi:10.1002/jcb.25994
Chadwick, M., Trewin, H., Gawthrop, F., and Wagstaff, C. (2013). Sesquiterpenoids Lactones: Benefits to Plants and People. Int. J. Mol. Sci. 14 (6), 12780–12805. doi:10.3390/ijms140612780
Chen, P. L., Scully, P., Shew, J. Y., Wang, J. Y., and Lee, W. H. (1989). Phosphorylation of the Retinoblastoma Gene Product Is Modulated during the Cell Cycle and Cellular Differentiation. Cell 58 (6), 1193–1198. doi:10.1016/0092-8674(89)90517-5
Chen, T., and Dent, S. Y. (2014). Chromatin Modifiers and Remodellers: Regulators of Cellular Differentiation. Nat. Rev. Genet. 15 (2), 93–106. doi:10.1038/nrg3607
Cheon, Y. H., Song, M. J., Kim, J. Y., Kwak, S. C., Park, J. H., Lee, C. H., et al. (2014). Costunolide Inhibits Osteoclast Differentiation by Suppressing C-Fos Transcriptional Activity. Phytother Res. 28 (4), 586–592. doi:10.1002/ptr.5034
Choi, J. H., Shin, K. M., Kim, N. Y., Hong, J. P., Lee, Y. S., Kim, H. J., et al. (2002). Taraxinic Acid, a Hydrolysate of Sesquiterpene Lactone Glycoside from the Taraxacum Coreanum NAKAI, Induces the Differentiation of Human Acute Promyelocytic Leukemia HL-60 Cells. Biol. Pharm. Bull. 25 (11), 1446–1450. doi:10.1248/bpb.25.1446
Clark, S. L. (1957). Cellular Differentiation in the Kidneys of Newborn Mice Studies with the Electron Microscope. J. Biophys. Biochem. Cytol. 3 (3), 349–362. doi:10.1083/jcb.3.3.349
Copeland, N. G., Gilbert, D. J., Schindler, C., Zhong, Z., Wen, Z., Darnell, J. E., et al. (1995). Distribution of the Mammalian Stat Gene Family in Mouse Chromosomes. Genomics 29 (1), 225–228. doi:10.1006/geno.1995.1235
da Silva Castro, E., Alves Antunes, L. A., Revoredo Lobo, J. F., Ratcliffe, N. A., Borges, R. M., Rocha, L., et al. (2018). Antileukemic Properties of Sesquiterpene Lactones: a Systematic Review. Anti-Cancer Agents Med. Chem. 18 (3), 323–334. doi:10.2174/1871520617666170918130126
da Silva, L. P., Borges, B. A., Veloso, M. P., Chagas-Paula, D. A., Gonçalves, R. V., and Novaes, R. D. (2020). Impact of Sesquiterpene Lactones on the Skin and Skin-Related Cells? A Systematic Review of In Vitro and In Vivo Evidence. Life Sci. 265, 118815. doi:10.1016/j.lfs.2020.118815
Darnell, J. E. (1997). STATs and Gene Regulation. Science 277 (5332), 1630–1635. doi:10.1126/science.277.5332.1630
de Lichtervelde, L., Boitano, A. E., Wang, Y., Krastel, P., Petersen, F., Cooke, M. P., et al. (2013). Eupalinilide E Inhibits Erythropoiesis and Promotes the Expansion of Hematopoietic Progenitor Cells. ACS Chem. Biol. 8 (5), 866–870. doi:10.1021/cb4000234
de Thé, H. (2018). Differentiation Therapy Revisited. Nat. Rev. Cancer 18 (2), 117–127. doi:10.1038/nrc.2017.103
Delgoffe, G. M., Pollizzi, K. N., Waickman, A. T., Heikamp, E., Meyers, D. J., Horton, M. R., et al. (2011). The Kinase mTOR Regulates the Differentiation of Helper T Cells through the Selective Activation of Signaling by mTORC1 and mTORC2. Nat. Immunol. 12 (4), 295–303. doi:10.1038/ni.2005
Dolcet, X., Llobet, D., Pallares, J., and Matias-Guiu, X. (2005). NF-kB in Development and Progression of Human Cancer. Virchows Arch. 446 (5), 475–482. doi:10.1007/s00428-005-1264-9
Dor, Y., and Glaser, B. (2013). β-Cell Dedifferentiation and Type 2 Diabetes. N. Engl. J. Med. 368 (6), 572–573. doi:10.1056/NEJMcibr1214034
Earp, B. D. (2018). The Need for Reporting Negative Results - a 90 Year Update. J. Clin. Transl Res. 3 (Suppl. 2), 344–347. doi:10.18053/jctres.03.2017S2.001
Farmer, S. R. (2005). Regulation of PPARgamma Activity during Adipogenesis. Int. J. Obes. (Lond) 29 (1), S13–S16. doi:10.1038/sj.ijo.0802907
Flaim, C. J., Chien, S., and Bhatia, S. N. (2005). An Extracellular Matrix Microarray for Probing Cellular Differentiation. Nat. Methods 2 (2), 119–125. doi:10.1038/nmeth736
Frese, C. K., Mikhaylova, M., Stucchi, R., Gautier, V., Liu, Q., Mohammed, S., et al. (2017). Quantitative Map of Proteome Dynamics during Neuronal Differentiation. Cell Rep 18 (6), 1527–1542. doi:10.1016/j.celrep.2017.01.025
Galvis, A., Marcano, A., Stefancin, C., Villaverde, N., Priestap, H. A., Tonn, C. E., et al. (2011). The Effect of Dehydroleucodine in Adipocyte Differentiation. Eur. J. Pharmacol. 671 (1-3), 18–25. doi:10.1016/j.ejphar.2011.09.033
Gu, M., Luo, L., and Fang, K. (2018). Crocin Inhibits Obesity via AMPK-dependent Inhibition of Adipocyte Differentiation and Promotion of Lipolysis. Biosci. Trends 12 (6), 587–594. doi:10.5582/bst.2018.01240
Guzman, M. L., Rossi, R. M., Karnischky, L., Li, X., Peterson, D. R., Howard, D. S., et al. (2005). The Sesquiterpene Lactone Parthenolide Induces Apoptosis of Human Acute Myelogenous Leukemia Stem and Progenitor Cells. Blood 105 (11), 4163–4169. doi:10.1182/blood-2004-10-4135
Hennessy, B. T., Smith, D. L., Ram, P. T., Lu, Y., and Mills, G. B. (2005). Exploiting the PI3K/AKT Pathway for Cancer Drug Discovery. Nat. Rev. Drug Discov. 4 (12), 988–1004. doi:10.1038/nrd1902
Hu, B., Wu, F., Shi, Z., He, B., Zhao, X., Wu, H., et al. (2019). Dehydrocostus Lactone Attenuates Osteoclastogenesis and Osteoclast-Induced Bone Loss by Modulating NF-Κb Signalling Pathway. J. Cel Mol Med 23 (8), 5762–5770. doi:10.1111/jcmm.14492
Huang, H. L., Lin, T. W., Huang, Y. L., and Huang, R. L. (2016). Induction of Apoptosis and Differentiation by Atractylenolide-1 Isolated from Atractylodes Macrocephala in Human Leukemia Cells. Bioorg. Med. Chem. Lett. 26 (8), 1905–1909. doi:10.1016/j.bmcl.2016.03.021
Jang, B. C. (2016). Artesunate Inhibits Adipogeneis in 3T3-L1 Preadipocytes by Reducing the Expression And/or Phosphorylation Levels of C/EBP-α, PPAR-γ, FAS, Perilipin A, and STAT-3. Biochem. Biophys. Res. Commun. 474 (1), 220–225. doi:10.1016/j.bbrc.2016.04.109
Jing, H., Su, X., Gao, B., Shuai, Y., Chen, J., Deng, Z., et al. (2018). Epigenetic Inhibition of Wnt Pathway Suppresses Osteogenic Differentiation of BMSCs during Osteoporosis. Cell Death Dis 9, 176. doi:10.1038/s41419-017-0231-0
Jopling, C., Boue, S., and Izpisua Belmonte, J. C. (2011). Dedifferentiation, Transdifferentiation and Reprogramming: Three Routes to Regeneration. Nat. Rev. Mol. Cel Biol 12 (2), 79–89. doi:10.1038/nrm3043
Jopling, C., Sleep, E., Raya, M., Martí, M., Raya, A., and Izpisúa Belmonte, J. C. (2010). Zebrafish Heart Regeneration Occurs by Cardiomyocyte Dedifferentiation and Proliferation. Nature 464 (7288), 606–609. doi:10.1038/nature08899
Kanehisa, M., Furumichi, M., Sato, Y., Ishiguro-Watanabe, M., and Tanabe, M. (2021). KEGG: Integrating Viruses and Cellular Organisms. Nucleic Acids Res. 49 (D1), D545–D551. doi:10.1093/nar/gkaa970
Kanehisa, M., and Goto, S. (2000). KEGG: Kyoto Encyclopedia of Genes and Genomes. Nucleic Acids Res. 28 (1), 27–30. doi:10.1093/nar/28.1.27
Kanehisa, M. (2019). Toward Understanding the Origin and Evolution of Cellular Organisms. Protein Sci. 28 (11), 1947–1951. doi:10.1002/pro.3715
Kicinski, M. (2014). How Does Under-reporting of Negative and Inconclusive Results Affect the False-Positive Rate in Meta-Analysis? A Simulation Study. BMJ open 4 (8), e004831. doi:10.1136/bmjopen-2014-004831
Kim, E. K., and Choi, E. J. (2010). Pathological Roles of MAPK Signaling Pathways in Human Diseases. Biochim. Biophys. Acta 1802 (4), 396–405. doi:10.1016/j.bbadis.2009.12.009
Kim, S. H., Oh, S. M., and Kim, T. S. (2005). Induction of Human Leukemia HL-60 Cell Differentiation via a PKC/ERK Pathway by Helenalin, a Pseudoguainolide Sesquiterpene Lactone. Eur. J. Pharmacol. 511 (2-3), 89–97. doi:10.1016/j.ejphar.2005.02.008
Kim, S. H., Song, J. H., Choi, B. G., Kim, H. J., and Kim, T. S. (2006). Chemical Modification of Santonin into a Diacetoxy Acetal Form Confers the Ability to Induce Differentiation of Human Promyelocytic Leukemia Cells via the Down-Regulation of NF-kappaB DNA Binding Activity. J. Biol. Chem. 281 (19), 13117–13125. doi:10.1074/jbc.M510944200
Kim, T., Jo, C., Kim, H.-S., Park, Y.-M., Wu, Y.-X., Cho, J.-H., et al. (2016). Chemical Constituents from Ainsliaea Acerifolia as Potential Anti-obesity Agents. Phytochemistry Lett. 16, 146–151. doi:10.1016/j.phytol.2016.04.005
Kim, K. M., Jang, W. G., and Zaluzanin, C (Z. C. (2017). Zaluzanin C (ZC) Induces Osteoblast Differentiation through Regulating of Osteogenic Genes Expressions in Early Stage of Differentiation. Bioorg. Med. Chem. Lett. 27 (21), 4789–4793. doi:10.1016/j.bmcl.2017.09.061
Kwak, S. H., and Kim, Y. H. (2019). Zaluzanin C Inhibits Differentiation of 3T3-L1 Preadipocytes into Mature Adipocytes. J. Obes. Metab. Syndr. 28 (2), 105–111. doi:10.7570/jomes.2019.28.2.105
Kweon, S. H., Song, J. H., Kim, H. J., Kim, T. S., and Choi, B. G. (2015). Induction of Human Leukemia Cell Differentiation via PKC/MAPK Pathways by Arsantin, a Sesquiterpene Lactone from Artemisia Santolina. Arch. Pharm. Res. 38 (11), 2020–2028. doi:10.1007/s12272-015-0609-4
Laid, M., Hegazy, M.-E. F., Ahmed, A. A., Ali, K., Belkacemi, D., and Ohta, S. (2008). Sesquiterpene Lactones from Algerian Artemisia Herba-alba. Phytochemistry Lett. 1 (2), 85–88. doi:10.1016/j.phytol.2008.04.002
Lee, H. I., Lee, J., Hwang, D., Lee, G. R., Kim, N., Kwon, M., et al. (2019). Dehydrocostus Lactone Suppresses Osteoclast Differentiation by Regulating NFATc1 and Inhibits Osteoclast Activation through Modulating Migration and Lysosome Function. FASEB J. 33 (8), 9685–9694. doi:10.1096/fj.201900862R
Lefterova, M. I., Zhang, Y., Steger, D. J., Schupp, M., Schug, J., Cristancho, A., et al. (2008). PPARgamma and C/EBP Factors Orchestrate Adipocyte Biology via Adjacent Binding on a Genome-wide Scale. Genes Dev. 22 (21), 2941–2952. doi:10.1101/gad.1709008
Letzen, B. S., Liu, C., Thakor, N. V., Gearhart, J. D., All, A. H., and Kerr, C. L. (2010). MicroRNA Expression Profiling of Oligodendrocyte Differentiation from Human Embryonic Stem Cells. PloS one 5 (5), e10480. doi:10.1371/journal.pone.0010480
Li, X., Wei, G., Wang, X., Liu, D. H., Deng, R. D., Li, H., et al. (2012). Targeting of the Sonic Hedgehog Pathway by Atractylenolides Promotes Chondrogenic Differentiation of Mesenchymal Stem Cells. Biol. Pharm. Bull. 35 (8), 1328–1335. doi:10.1248/bpb.b12-00265
Li, Z., Yuan, G., Lin, X., Liu, Q., Xu, J., Lian, Z., et al. (2019). Dehydrocostus Lactone (DHC) Suppresses Estrogen Deficiency-Induced Osteoporosis. Biochem. Pharmacol. 163, 279–289. doi:10.1016/j.bcp.2019.02.002
Li, Q., Wang, Z., Xie, Y., and Hu, H. (2020). Antitumor Activity and Mechanism of Costunolide and Dehydrocostus Lactone: Two Natural Sesquiterpene Lactones from the Asteraceae Family. Biomed. Pharmacother. 125, 109955. doi:10.1016/j.biopha.2020.109955
Lu, J., Kuang, Z., Chen, T., Ye, C., Hou, W., Tang, L., et al. (2020). Isoalantolactone Inhibits RANKL-Induced Osteoclast Formation via Multiple Signaling Pathways. Int. Immunopharmacol 84, 106550. doi:10.1016/j.intimp.2020.106550
Mallory, F. B. (1910). Recent Progress in the Microscopic Anatomy and Differentiation of Cancer. Jama 55 (18), 1513–1516. doi:10.1001/jama.1910.04330180001001
Márquez-Zacarías, P., Pineau, R. M., Gomez, M., Veliz-Cuba, A., Murrugarra, D., Ratcliff, W. C., et al. (2021). Evolution of Cellular Differentiation: from Hypotheses to Models. Trends Ecol. Evol. 36 (1), 49–60. doi:10.1016/j.tree.2020.07.013
McCrindle, B. W., Ose, L., and Marais, A. D. (2003). Efficacy and Safety of Atorvastatin in Children and Adolescents with Familial Hypercholesterolemia or Severe Hyperlipidemia: a Multicenter, Randomized, Placebo-Controlled Trial. J. Pediatr. 143 (1), 74–80. doi:10.1016/S0022-3476(03)00186-0
McGuinness, L. A., and Higgins, J. P. T. (2020). Risk-of-bias VISualization (Robvis): An R Package and Shiny Web App for Visualizing Risk-Of-Bias Assessments. Res. Synth. Methods 12, 55–61. doi:10.1002/jrsm.1411
Mehta, D. (2019). Highlight Negative Results to Improve Science. Nature. doi:10.1038/d41586-019-02960-3
Moyes, C. D., Mathieu-Costello, O. A., Tsuchiya, N., Filburn, C., and Hansford, R. G. (1997). Mitochondrial Biogenesis during Cellular Differentiation. Am. J. Physiol. 272 (4), C1345–C1351. doi:10.1152/ajpcell.1997.272.4.C1345
Murray, P. J. (2007). The JAK-STAT Signaling Pathway: Input and Output Integration. J. Immunol. 178 (5), 2623–2629. doi:10.4049/jimmunol.178.5.2623
Murry, C. E., and Keller, G. (2008). Differentiation of Embryonic Stem Cells to Clinically Relevant Populations: Lessons from Embryonic Development. Cell 132 (4), 661–680. doi:10.1016/j.cell.2008.02.008
Nakata, M., Nagasaka, S., Kusaka, I., Matsuoka, H., Ishibashi, S., and Yada, T. (2006). Effects of Statins on the Adipocyte Maturation and Expression of Glucose Transporter 4 (SLC2A4): Implications in Glycaemic Control. Diabetologia 49 (8), 1881–1892. doi:10.1007/s00125-006-0269-5
Osaki, M., Oshimura, M., and Ito, H. (2004). PI3K-Akt Pathway: its Functions and Alterations in Human Cancer. Apoptosis 9 (6), 667–676. doi:10.1023/B:APPT.0000045801.15585.dd
Pannucci, C. J., and Wilkins, E. G. (2010). Identifying and Avoiding Bias in Research. Plast. Reconstr. Surg. 126 (2), 619–625. doi:10.1097/PRS.0b013e3181de24bc
Park, E., Song, J. H., Kim, M. S., Park, S. H., and Kim, T. S. (2016). Costunolide, a Sesquiterpene Lactone, Inhibits the Differentiation of Pro-inflammatory CD4+ T Cells through the Modulation of Mitogen-Activated Protein Kinases. Int. Immunopharmacol 40, 508–516. doi:10.1016/j.intimp.2016.10.006
Park, J. H., Kang, Y. H., Hwang, S. C., Oh, S. H., and Byun, J. H. (2020). Parthenolide Has Negative Effects on In Vitro Enhanced Osteogenic Phenotypes by Inflammatory Cytokine TNF-α via Inhibiting JNK Signaling. Int. J. Mol. Sci. 21 (15), 5433. doi:10.3390/ijms21155433
Perri, F., Frattaruolo, L., Haworth, I., Brindisi, M., El-magboub, A., Ferrario, A., et al. (2019). Naturally Occurring Sesquiterpene Lactones and Their Semi-synthetic Derivatives Modulate PGE2 Levels by Decreasing COX2 Activity and Expression. Heliyon 5 (3), e01366. doi:10.1016/j.heliyon.2019.e01366
Rawlings, J. S., Rosler, K. M., and Harrison, D. A. (2004). The JAK/STAT Signaling Pathway. J. Cel Sci 117 (8), 1281–1283. doi:10.1242/jcs.00963
Schmidt, T. J. (2006). “Structure-activity Relationships of Sesquiterpene Lactones,” in Studies in Natural Products Chemistry (Elsevier), 309–392. doi:10.1016/s1572-5995(06)80030-x
Sell, S. (2004). Stem Cell Origin of Cancer and Differentiation Therapy. Crit. Rev. Oncol. Hematol. 51 (1), 1–28. doi:10.1016/j.critrevonc.2004.04.007
Shoaib, M., Shah, I., Ali, N., Adhikari, A., Tahir, M. N., Shah, S. W., et al. (2017). Sesquiterpene Lactone! a Promising Antioxidant, Anticancer and Moderate Antinociceptive Agent from Artemisia Macrocephala Jacquem. BMC Complement. Altern. Med. 17 (1), 27–11. doi:10.1186/s12906-016-1517-y
Stark, G. R., Kerr, I. M., Williams, B. R., Silverman, R. H., and Schreiber, R. D. (1998). How Cells Respond to Interferons. Annu. Rev. Biochem. 67 (1), 227–264. doi:10.1146/annurev.biochem.67.1.227
Tang, J.-J., He, Q-R., Dong, S., Guo, X., Wang, Y-G., Lei, B-L., et al. (2018). Diversity Modification and Structure-Activity Relationships of Two Natural Products 1β-Hydroxy Alantolactone and Ivangustin as Potent Cytotoxic Agents. Scientific Rep. 8 (1), 1–16. doi:10.1038/s41598-018-20192-9
Tata, P. R., Mou, H., Pardo-Saganta, A., Zhao, R., Prabhu, M., Law, B. M., et al. (2013). Dedifferentiation of Committed Epithelial Cells into Stem Cells In Vivo. Nature 503 (7475), 218–223. doi:10.1038/nature12777
Vivanco, I., and Sawyers, C. L. (2002). The Phosphatidylinositol 3-Kinase AKT Pathway in Human Cancer. Nat. Rev. Cancer 2 (7), 489–501. doi:10.1038/nrc839
Wagner, E. F., and Nebreda, A. R. (2009). Signal Integration by JNK and P38 MAPK Pathways in Cancer Development. Nat. Rev. Cancer 9 (8), 537–549. doi:10.1038/nrc2694
Wang, Z. Y., and Chen, Z. (2008). Acute Promyelocytic Leukemia: from Highly Fatal to Highly Curable. Blood 111 (5), 2505–2515. doi:10.1182/blood-2007-07-102798
Warrell, R. P., Frankel, S. R., Miller, W. H., Scheinberg, D. A., Itri, L. M., Hittelman, W. N., et al. (1991). Differentiation Therapy of Acute Promyelocytic Leukemia with Tretinoin (All-trans-retinoic Acid). N. Engl. J. Med. 324 (20), 1385–1393. doi:10.1056/NEJM199105163242002
Wedge, D. E., Galindo, J. C., and Macías, F. A. (2000). Fungicidal Activity of Natural and Synthetic Sesquiterpene Lactone Analogs. Phytochemistry 53 (7), 747–757. doi:10.1016/s0031-9422(00)00008-x
Xie, W., Schultz, M. D., Lister, R., Hou, Z., Rajagopal, N., Ray, P., et al. (2013). Epigenomic Analysis of Multilineage Differentiation of Human Embryonic Stem Cells. Cell 153 (5), 1134–1148. doi:10.1016/j.cell.2013.04.022
Yami, A., Hamzeloo-Moghadam, M., Darbandi, A., Karami, A., Mashati, P., Takhviji, V., et al. (2020). Ergolide, a Potent Sesquiterpene Lactone Induces Cell Cycle Arrest along with ROS-dependent Apoptosis and Potentiates Vincristine Cytotoxicity in ALL Cell Lines. J. Ethnopharmacol 253, 112504. doi:10.1016/j.jep.2019.112504
Ye, Y., Chou, G. X., Hui, W., Chu, J. H., Fong, W. F., and Yu, Z. L. (2011). Effects of Sesquiterpenes Isolated from Largehead Atractylodes Rhizome on Growth, Migration, and Differentiation of B16 Melanoma Cells. Integr. Cancer Ther. 10 (1), 92–100. doi:10.1177/1534735410378660
Yu, Z., Chen, Z., Li, Q., Yang, K., Huang, Z., Wang, W., et al. (2021). What Dominates the Changeable Pharmacokinetics of Natural Sesquiterpene Lactones and Diterpene Lactones: a Review Focusing on Absorption and Metabolism. Drug Metab. Rev. 53 (1), 122–140. doi:10.1080/03602532.2020.1853151
Zhou, L., Liu, Q., Hong, G., Song, F., Zhao, J., Yuan, J., et al. (2019). Cumambrin A Prevents OVX-Induced Osteoporosis via the Inhibition of Osteoclastogenesis, Bone Resorption, and RANKL Signaling Pathways. FASEB J. 33 (6), 6726–6735. doi:10.1096/fj.201800883RRR
Zimmermann, S., Fouché, G., De Mieri, M., Yoshimoto, Y., Usuki, T., Nthambeleni, R., et al. (2014). Structure-activity Relationship Study of Sesquiterpene Lactones and Their Semi-synthetic Amino Derivatives as Potential Antitrypanosomal Products. Molecules 19 (3), 3523–3538. doi:10.3390/molecules19033523
Keywords: sesquiterpene lactone, lactone, differentiation, signaling pathways, cancer, negative results, in-vitro, systematic review
Citation: Fateh ST, Fateh ST, Shekari F, Mahdavi M, Aref AR and Salehi-Najafabadi A (2022) The Effects of Sesquiterpene Lactones on the Differentiation of Human or Animal Cells Cultured In-Vitro: A Critical Systematic Review. Front. Pharmacol. 13:862446. doi: 10.3389/fphar.2022.862446
Received: 25 January 2022; Accepted: 08 March 2022;
Published: 04 April 2022.
Edited by:
Mythily Srinivasan, Indiana University—Purdue University Indianapolis, United StatesReviewed by:
Pranabananda Dutta, Charles R. Drew University of Medicine and Science, United StatesEszter Lajkó, Semmelweis University, Hungary
Copyright © 2022 Fateh, Fateh, Shekari, Mahdavi, Aref and Salehi-Najafabadi. This is an open-access article distributed under the terms of the Creative Commons Attribution License (CC BY). The use, distribution or reproduction in other forums is permitted, provided the original author(s) and the copyright owner(s) are credited and that the original publication in this journal is cited, in accordance with accepted academic practice. No use, distribution or reproduction is permitted which does not comply with these terms.
*Correspondence: Amir Salehi-Najafabadi, amirsalehi@ut.ac.ir