- 1Xi’an Hospital of Traditional Chinese Medicine, Xi’an, China
- 2Shaanxi University of Chinese Medicine, Xianyang, China
- 3Guang’anmen Hospital, Chinese Academy of Chinese Medical Sciences, Beijing, China
Ischemic stroke (IS) is characterized by high recurrence and disability; however, its therapies are very limited. As one of the effective methods of treating acute attacks of IS, intravenous thrombolysis has a clear time window. Quercetin, a flavonoid widely found in vegetables and fruits, inhibits immune cells from secreting inflammatory cytokines, thereby reducing platelet aggregation and limiting inflammatory thrombosis. In pre-clinical studies, it has been shown to exhibit neuroprotective effects in patients with ischemic brain injury. However, its specific mechanism of action remains unknown. Therefore, this review aims to use published data to elucidate the potential value of quercetin in patients with ischemic brain injury. This article also reviews the plant sources, pharmacological effects, and metabolic processes of quercetin in vivo, thus focusing on its mechanism in inhibiting immune cell activation and inflammatory thrombosis as well as promoting neuroprotection against ischemic brain injury.
1 Introduction
Quercetin, which is present in many plants, has become a nutraceutical because of its significant antioxidant and anti-inflammatory activities (Shen et al., 2021; Zou et al., 2021), especially with its ability to scavenge free radicals (Anand David et al., 2016). Clinical studies have shown that quercetin has certain therapeutic effects on cardiovascular diseases (Dehghani et al., 2021), metabolic syndrome (Leyva-Soto et al., 2021), COVID-19 (Di Pierro et al., 2021), and central nervous system diseases.
Ischemic stroke (IS) is caused by hypoxic necrosis of brain tissue due to impaired blood supply to the brain, thereby leading to ischemia. It is the third leading cause of death worldwide (GBD, 2021). In 2017, there were 80.5 (UI78.9-82.6) deaths per 100,000 people, of which 45% were related to IS (Avan et al., 2019); IS accounted for 62.4% of stroke events in 2019 (GBD, 2021). Various risk factors, such as hypertension, diabetes, high body mass index, and smoking, determine the prevalence of IS and its complications; the antioxidant and inflammatory balance mechanisms in the body are severely damaged, thus causing an increase in neuronal reactive oxygen species (ROS), dysfunction, calcium excess, and oxidative stress (Lo et al., 2003).
Vitamins, carotenoids, and quercetin, which are natural antioxidants, are effectively used to prevent IS. Their mechanism may be related to the synergistic effects of vitamins and antioxidants (Ratnam et al., 2006). The molecular structure of quercetin is C15H10O7 (Magar and Sohng, 2020), which means that there is one -OH at each of the 3, 3′, 5, 7, and 4′ positions (Rice-Evans et al., 1997), and is present in a variety of plants and fruits (Figure 1). Quercetin is a polyphenol belonging to the flavonoid family (Brüll et al., 2015). Inflammatory thrombus formation exacerbates nerve damage in IS (De Meyer et al., 2022). Clinical studies have found that oral quercetin can reduce collagen-stimulated platelet tyrosine phosphorylation and thus inhibit platelet aggregation (Hubbard et al., 2004). Quercetin pre-treatment also reduces lipopolysaccharide-induced neutrophil IL-6 secretion (Liu J. et al., 2005). This process slows the formation of inflammatory thrombi, thus reducing the occurrence of IS.
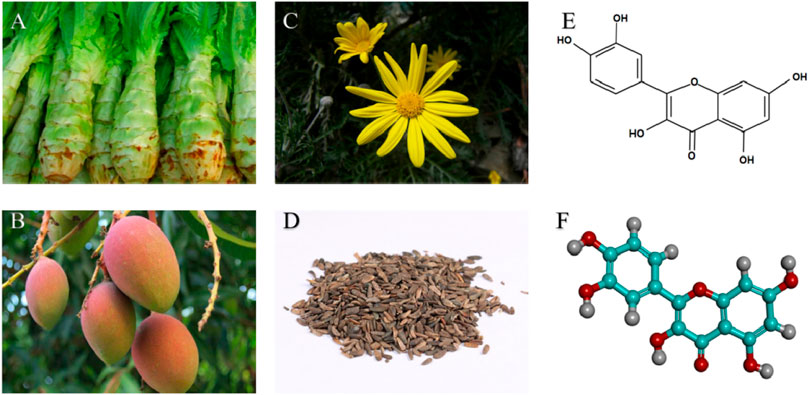
FIGURE 1. Natural sources and structures of quercetin: (A) Lettuce Rootstock; (B) Mango fruit; (C) Arnica flowering plants; (D) Dried ripe fruit of burdock; (E) Chemical structure of quercetin; (F) 3D structure of quercetin (Visualized by DiscoveryStudio 2016).
Recent studies have demonstrated the neuroprotective properties of quercetin in in vivo and in vitro IS models (Yang et al., 2021). Therefore, this article is the first to describe the source and physicochemical properties of quercetin as well as the pathogenesis of ischemic brain injury. The therapeutic potential of quercetin in ischemic brain injury has been highlighted, including its role in limiting the secretion of inflammatory factors by various immune cells, thereby inhibiting inflammatory thrombosis, oxidative stress, apoptosis, autophagy.
2 Source and Physicochemical Properties of Quercetin
The term, “quercetin,” has been used since the mid-18th century and is derived from the Latin word, “quercetum” (Jaimand et al., 2012). Quercetin is highly lipophilic and has poor water solubility, rapid metabolism, short half-life, and low bioavailability (Mukhopadhyay and Prajapati, 2015). Meanwhile, it is a unique polyphenol found in large quantities in various leafy vegetables, fruits, and herbs, such as apples, berries, long-leaf berry cilantro, cumin, lingonberry, lingonberry, wild grapes, and onions (Yang D. et al., 2020; Sharifi-Rad et al., 2021). According to previous research, quercetin has more than seven biological features, including neuroprotection, anti-allergy, anti-oxidation, anti-inflammation, immune regulation, anti-microbial, and anti-tumor properties (Bjeldanes and Chang, 1977; Dajas, 2012; Oboh et al., 2016; Darband et al., 2018; Dhiman et al., 2019; Huang et al., 2020; Shabbir et al., 2021). However, some studies have indicated that quercetin can induce mutations and promote mutagenesis (Bjeldanes and Chang, 1977). Conversely, Sumi et al. (2013) found that quercetin glucoside promoted angiogenesis after ischemia, but did not promote tumor growth.
In addition, the effectiveness of quercetin depends mainly on the plant source, dose, and chemical properties after processing (Najda et al., 2019). It can also be combined with salivary proteins to form soluble protein-quercetin binary aggregates. It is generated in the small intestines and is directly absorbed by the sodium-dependent glucose transporter-1 in the cecum and colon (Manach et al., 2004). Quercetin is also absorbed by intestinal epithelial cells, thus entering the liver through lipophilic dispersion and undergoing metabolism (Shen et al., 2021). In humans, quercetin has very low bioavailability and is highly unstable (0–50%), with a half-life of 1–2 h in the body after ingesting quercetin-rich foods or supplements (Graefe et al., 1999). Furthermore, quercetin poorly crosses the blood-brain barrier (BBB) (Oliveira et al., 2021). After dietary absorption, quercetin is digested and metabolized extremely quickly; therefore, its pharmacological effects are concentrated on in vitro studies rather than in vivo (Williams et al., 2004; Barnes et al., 2011). Therefore, various approaches have been attempted to improve the bioavailability of quercetin in the brain, such as enzyme modification or nano-encapsulation (Pateiro et al., 2021). Simultaneously, nanotechnology and targeted vectors are solutions to overcome the shortcomings of quercetin, such as low bioavailability and poor BBB passage (Naseri et al., 2015). The bioavailability of quercetin is 50 times higher than that of standard quercetin products after being packaged into nanocapsules (Riva et al., 2019). Alternatively, it changes the basic structure of quercetin to improve its pharmacokinetic and neuroprotective abilities (Chen et al., 2005). Table 1 summarises the sources of quercetin.
3 Pathogenesis of Ischemic Stroke
3.1 Inflammatory Thrombus
In the pathophysiological process of IS, inflammatory thrombi lead to cerebral vascular occlusion, inflammatory response, and severe nerve damage after ischemic events (De Meyer et al., 2022). Early platelet adhesion and activation are key factors for the development of IS inflammatory thrombosis. The main receptors that mediate platelet adhesion are glycoprotein (GP) VI and integrin α2β1, both of which bind to the GPIbα subunit of collagen and the GPIB-IX-V complex, which interact with the von Willebrand factor (vWF) (Poulter et al., 2017; Constantinescu-Bercu et al., 2022; Feitsma et al., 2022). After endothelial injury, vWF interacts with GPIbα, thus causing platelets to decelerate on the fixed vWF (Constantinescu-Bercu et al., 2022; Kanaji et al., 2022) and thereby contributing to platelet aggregation. The use of GPIbα-vWF inhibitors restores vascular patency by specifically breaking down the outer layer of the occlusive thrombus (Le Behot et al., 2014). Subsequently, platelet activation induces a conformational change in the GPIIb/IIIa surface receptor and its affinity to fibrinogen and vWF, thus promoting platelet aggregation (O'Brien and Salmon, 1990).
In addition, vWF was found in different samples of thrombus extracted from IS patients; the thrombus contained 20.3% ± 10.1% vWF on average (Denorme et al., 2016). In a middle cerebral artery occlusion (MCAO) rat model, cerebral infarct size and fibrinogen deposition were significantly increased in platelet-only vWF chimeric rats (Verhenne et al., 2015). Interestingly, vWF can be cleaved by metalloprotease ADAMTS13, a disintegrin and metalloproteinase with a thrombospondin type 1 motif member 13. ADAMTS13 effectively dissolves anti-tissue-plasminogen activator (t-PA) thrombus within 5–60 min of MCAO occlusion (Denorme et al., 2016). Furthermore, caADAMTS13, a ADAMTS13 variant, significantly reduced residual vWF, fibrin, and platelet aggregation as well as neutrophil recruitment in the middle cerebral artery (MCA) (South et al., 2022).
However, thrombosis not only involves simple platelet aggregation, but also includes leukocyte-platelet complexation (Li et al., 2015; Pircher et al., 2019; Schrottmaier et al., 2020). This may be because basic diseases, such as hyperlipidemia and hyperglycemia, stimulate hematopoietic cells in the bone marrow to produce a large number of white blood cells in the circulating blood (Stumvoll et al., 2005; Zhou et al., 2016; Vekic et al., 2019). Neutrophils are closely related to thrombosis in IS patients with COVID-19 (Genchi et al., 2022). Neutrophils account for the majority of leukocytes in IS thrombi, followed by macrophages and T cells (Heo et al., 2020). This difference is partly due to their proportion in the circulating blood under physiological conditions; however, IS is also related to the level of activation of various white blood cells. Thrombus formation is a series of complex events that occur sequentially in the vascular system, including endothelial activation, neutrophil extracellular trap (NET) formation, vWF secretion, blood cell adhesion, aggregation, and activation (Yang J. et al., 2020). Genetic deletion of PKM2 in bone marrow hematopoietic cells reduces NET after cerebral ischemia/reperfusion, which further reduces fibrinogen, platelet deposition, and inflammatory cytokines in the brain (Dhanesha et al., 2022). Similarly, rats lacking CD84 on their platelets or T cells showed reduced cerebral thrombosis and milder nerve damage after MCAO (Schuhmann et al., 2020). In contrast, endothelial CD69 deficiency increases fibrinogen and vWF levels in ischemic tissue and exacerbates nerve injury (Brait et al., 2019). Thus, inhibition of inflammatory thrombi formation is one of the goals of IS prevention.
3.2 Immune Activation
Activation of immune cells, including neutrophils, T cells, and microglia, is involved in brain tissue repair after IS (Ma et al., 2017). Subsequently, neutrophils are attracted along a concentration gradient of chemokines in areas of ischemia to release pro-inflammatory factors, ROS, proteases, and matrix metalloproteinases (MMPs) (Wang et al., 2007), thus leading to the disruption of the BBB and exacerbation of neurological damage (Rosell et al., 2008). Similarly, in the acute phase of IS, Th1 and Th17 cells degrade tight junction proteins (TJ) by secreting IFN-γ, IL-17, and IL-21, thereby further disrupting the integrity of the BBB (Gelderblom et al., 2012; Clarkson et al., 2014). T cells and their isoforms have also been associated with repair and functional improvement in late brain injury (Liesz et al., 2009). During ongoing inflammation, activated M1 microglia phagocytose astrocyte ends and disrupt the integrity of the BBB by secreting various vascular proteins (Wang et al., 2018; Haruwaka et al., 2019).
3.3 Oxidative Stress
Oxidative stress and mitochondrial dysfunction are important factors for the development of cerebral ischemic injury (Lo et al., 2003). Mitochondria are central to ROS production and cell death (Lo et al., 2003). Cerebral ischemia induces a cascade of excessive ROS production. Excess ROS leads to lipid peroxidation (LPO), exacerbates oxidative damage to proteins and nucleic acids, and contributes to neuronal apoptosis and BBB destruction (Allen and Bayraktutan, 2009; Kleinschnitz et al., 2010; Casas et al., 2017; Sun et al., 2018). Immune activation and oxidative stress also contribute to programmed neuronal death in the ischemic zone.
3.4 Procedural Death
Both hypoxia and ischemia induce autophagy. Shortly thereafter, autophagic vesicles accumulate extensively in the brain tissue (Tuo et al., 2021). Mitochondrial autophagy facilitates the maintenance of cellular homeostasis under mild ischemia or hypoxia. In contrast, sustained ischemia-reperfusion (I/R) results in prolonged autophagy, thus promoting neuronal cell damage or even death (Zhang et al., 2013). Similarly, neuronal apoptosis is the main mechanism through which I/R injury induces cell death. The balance between anti-apoptotic Bcl-2 and pro-apoptotic Bax protein expression is critical for the regulation of apoptosis (Culmsee and Plesnila, 2006). ROS production and mitochondria-dependent apoptosis play an important role in neuronal death following I/R injury (Jordan et al., 2011; Wang et al., 2014). After IS, a series of molecular events induced by oxidative stress overlap with iron sagging/oxidation processes; these have common molecular targets, such as LPO and glutathione (GSH) depletion (Seiler et al., 2008; Choi et al., 2013; Yang et al., 2014). Iron death is dependent on excessive iron accumulation, with the core process being LPO (Cao and Dixon, 2016). In a rat model of MCAO, GSH inhibited iron death by driving glutathione peroxidase 4 (GPx4) expression, thereby protecting neurons and reducing core ischemic areas (Karuppagounder et al., 2016; Alim et al., 2019).
4 Pharmacological Effects of Quercetin on Ischemic Stroke
4.1 Inhibition of Immune Cell Recruitment
The activation of peripheral immune cells promotes platelet aggregation. Pre-treatment of activated T cells with quercetin blocks IL-12-induced JAK-STAT tyrosine phosphorylation, thereby reducing T cell proliferation and Th1 differentiation (Muthian and Bright, 2004). Quercetin has a similar effect on neutrophils. NETs are closely associated with inflammatory thromboses. Quercetin does not directly affect NET formation, but inhibits it in peripheral blood polykaryotic cells by downregulating TNF-α production in lipopolysaccharide (LPS) peripheral blood monocytes (Yuan et al., 2020; Jo et al., 2021). During inflammation, LPS delays the spontaneous apoptosis of neutrophils, while quercetin accelerates this process (Liu J. J. et al., 2005; Yuan et al., 2020). This is associated with a reduction in the expression of inflammatory cytokines, activation of PKCa, and enhancement of CD95-mediated apoptosis in neutrophils (Russo et al., 2003). Quercetin effectively protects LDL from neutrophil-mediated modification at physiological concentrations (1 µM) and inhibits myeloperoxidase (MPO) oxidative damage (Loke et al., 2008) (Figure 2). Subsequently, quercetin downregulates the TLR-NF-κB signaling pathway, reduces the activities of COX, 5-LOx, NOS, MPO, and CRP, inhibits ldL-induced adhesion molecule expression, and ameliorates endothelial dysfunction in atherosclerosis (Bhaskar et al., 2016). Quercetin also reduces the activity of neutrophil MPO and inhibits the production of HOCl, a powerful oxidant, to protect endothelial cells from oxidative damage (Lu et al., 2018). In contrast, Suri et al. (2008) found that quercetin did not exert excessive influence on neutrophils, but only reduced the calcium response induced by N-formyl-methionyl-leucyl-phenylalanine (fMLP).
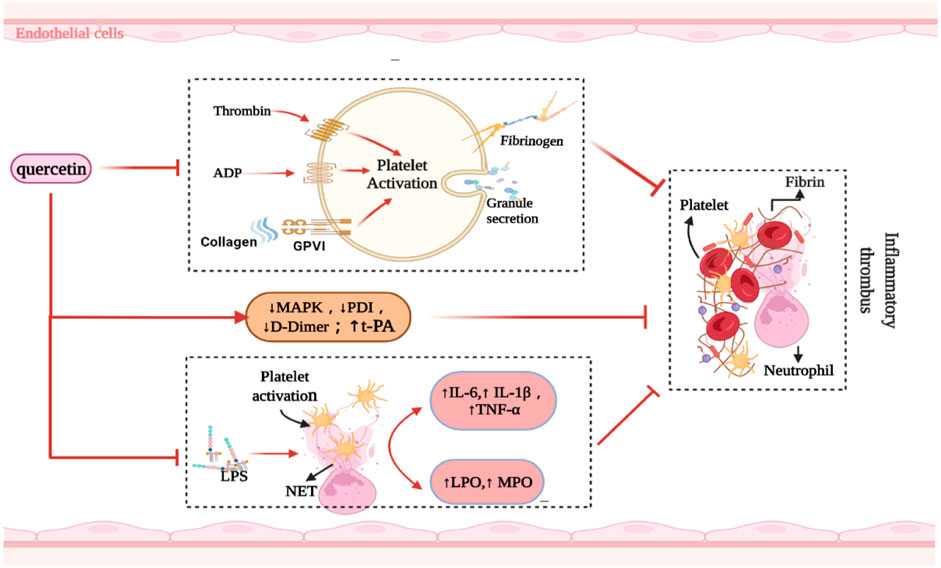
FIGURE 2. Summarized the mechanism of quercetin inhibiting inflammatory thrombosis. Abbreviations: ↑, increase; ↓, decrease; ADP, Adenosine diphosphate; PDI, Protein disulfide isomerase; NET, neutrophil extracellular trap; LPO, lipid peroxidation; MPO, myeloperoxidase; t-PA, anti-tissue-plasminogen activator; MAPK, mitogen-activated protein kinase; LPS, lipopolysaccharide; GPVI, glycoprotein VI; IL, interleukin; TNF-α, tumor necrosis factor-alfa.
4.2 Inhibition of Thrombosis
The key factor for the occurrence of IS is the formation of an inflammatory thrombus; thrombolysis significantly alleviates brain injury. Quercetin, a natural flavonol compound, can significantly reduce diabetes-induced platelet aggregation (Mosawy et al., 2014), which may be related to the inhibition of compact platelet granule exocytosis (Mosawy et al., 2013b). Similarly, quercetin inhibits agonists (ADP, collagen, and thrombin) as well as induces platelet aggregation and granule secretion (Liang et al., 2015). Quercetin also binds to the GPIIb/IIIa platelet receptor, thus inhibiting the aggregation-promoting properties of calcium ion carriers and avoiding an increase in platelet-derived particles; these improve hemorheology (Zaragozá et al., 2021) and reduce thrombosis after carotid artery injury induced by FeCl3 in C57BL/6 rats (Mosawy et al., 2013a). Quercetin also effectively blocked in vivo FeCl3-induced arterial thrombosis and reduced IS infarct volume by inhibiting glycoprotein VI (GPVI)-mediated platelet signal transduction (Oh et al., 2021).
The binding of collagen to GPVI leads to receptor aggregation, which stimulates tyrosine phosphorylation and thus causes platelet aggregation (Gibbins et al., 1996; Poole et al., 1997). Quercetin inhibits platelet activation by inhibiting various components of the GPVI signaling pathway (e.g., collagenous tyrosine phosphorylation) (Hubbard et al., 2003; Hubbard et al., 2006; Wright et al., 2010), which may be a key factor for improving nerve injury in IS. In clinical trials, platelet aggregation was inhibited 30 and 120 min after oral quercetin administration, with a corresponding reduction in collagen-stimulated platelet tyrosine phosphorylation (Hubbard et al., 2004). Quercetin also inhibited platelet aggregation when collagen was stimulated at concentrations between 0.5 and 1.0 μg/ml, with IC50 values below 3 μM (Hubbard et al., 2003). Thus, these results support the clinical transformation of quercetin.
In contrast, Lee et al. (2013) found that quercetin did not reduce PT, aPTT, or platelet aggregation in experimental rats. They revealed that its downregulation of mitogen-activated protein kinase (MAPK) activation restricted tissue factor expression, thereby prolonging the time for atherothrombosis development. In endothelial cells, quercetin transcription induces the human t-PA gene by requiring a specific Sp1 (b) element within the proximal promoter region, which is mediated by the P38 MAPK-dependent signaling pathway (Pan et al., 2008).
Protein disulfide isomerase (PDI), a thiol isomerase secreted by vascular cells, is required for thrombosis. Quercetin-3-rutinoside prevents thrombosis in a PDI-dependent manner in experimental rats (Jasuja et al., 2012). Oral administration of 1,000 mg isoquercetin can reduce the plasma concentration of D-dimer by 21.9% and inhibit the activity of PDI in the plasma, thereby exerting an antithrombotic effect (Zwicker et al., 2019). This was related to the reduction of platelet-dependent thrombin content by blocking the production of platelet factor Va (Stopa et al., 2017). Intravenous administration of quercetin significantly attenuated TNF-α levels and prothrombin activity in a rabbit model of LPS-induced DIC (Yu et al., 2013) (Figure 2). Nonetheless, oral quercetin did not prevent thrombo-embolic stroke in an earlier Dutch cohort study (Knekt et al., 2000). This finding may be related to the use of dietary quercetin content as an intervening factor. Table 2 summarizes the role of quercetin and its derivatives in limiting thrombosis.
4.3 The Role of Histomorphology
In an in vitro ischemia model, quercetin-treated cells showed improved tolerance to oxygen-glucose deprivation (OGD) or oxygen glucose recovery (ROG) (Lee et al., 2016). Quercetin administration reduced the corrected total infarct volume and edema percentage by 43.6% and 48.5%, respectively, along with a significant behavioral recovery effect (Lee et al., 2015). Quercetin and Kolaviron pre-treatment significantly improved the I/R-induced changes in brain water content. Significant remission of cerebral infarction was observed in the Kolaviron and quercetin treatment groups (Akinmoladun et al., 2015), which might be linked to the role of quercetin in the Sirt1/nuclear factor-erythroid 2-related factor 2 (Nrf2)/heme oxygenase-1 (HO-1) signaling cascade (Yang et al., 2021). Compared to free quercetin or quercetin-carrying exosome (quercetin-EXO) therapy, treatment with quercetin/mAb gAP43-EXO dramatically reduced infarct size and improved neurological recovery in MCAO reperfusion-induced rats (Guo et al., 2021) (Figure 3). Simultaneously, quercetin improved the IS-associated motor and sensory deficits in the dorsal striatum, which may be related to the upregulation of MC4R-mRNA expression (Ulya et al., 2021). More intuitively, quercetin showed 6.79 2 ± 0.41 right turn in rats in the permanent MCAO model and 9.31 ± 0.33 right turn in the control group. Most rats in the treatment group showed mild to moderate neuromotor deficits (p < 0.0001) (Ahn and Jeon, 2015). In addition, the infarct volume of rats in the control and treatment groups was 26.35 ± 2.25% and 14.87 ± 1.75%, respectively (Park et al., 2020). Quercetin can improve cognitive function in rats with ischemic injury. In the Morris water maze (MWM) test, quercetin therapy restored spatial learning deficits by increasing the time and amount of access to the central region (Le et al., 2020). By boosting the number of new Olig2+ oligodendrocyte progenitors in the subventricular zone, quercetin alleviated hypoxia/ischemia (HI)-induced cognitive impairment (Qu et al., 2014). Compared with the control group, I/R rats pre-treated with quercetin (20 mg/kg) for 7 days showed a significant reduction in cognitive impairment as well as improvement in motor capacity, cerebral edema, and infarct volume (p < 0.001) (Viswanatha et al., 2018; Viswanatha et al., 2019). Table 3 summarizes the neuroprotective effects of quercetin on IS.
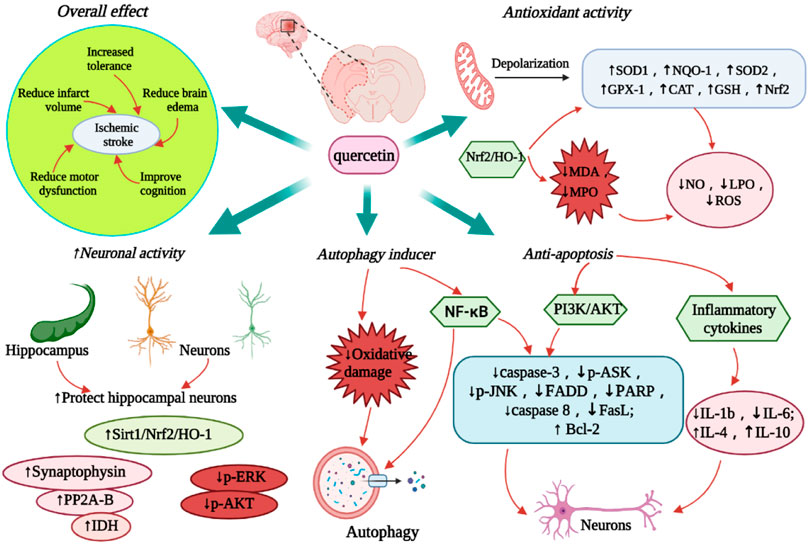
FIGURE 3. Diagram of the neuroprotective effect of quercetin on IS. The figure highlights the overall therapeutic effect of quercetin, anti-oxidative stress, anti-apoptosis, promoting autophagy and neuroprotective activity. Abbreviations: ↑, increase; ↓, decrease; SOD, Superoxide dismutase; NQO-1, NAD(P)H dehydrogenase quinone-1; GPX-1, glutathione peroxidase-1; CAT, catalase; NOS1, nitric oxide synthase 1; GSH, glutathione; Nrf2, nuclear factor 2-related factor 2; HO-1, Heme oxygenase-1; NO, nitric oxide; LPO, lipid peroxidation; ROS, reactive oxygen species; IL, interleukin; Sirt1, sirtuin 1; NF-kB, nuclear factor kappa B; IDH, isocitrate dehydrogenase; PP2A, Protein phosphatase 2A; FADD, Fas-associated death domain; PARP, poly (ADP-ribose) polymerase; PI3K, phosphoinositide 3-kinase; AKT, protein kinase B; ASK, apoptosis signal-regulating kinase; JNK, c-Jun N-terminal protein kinases; ERK, extracellular-signal-regulated kinase; FasL, factor associated suicide ligand.
4.4 Prevention of Oxidative Stress
4.4.1 Quercetin
The mitochondria are the main source of oxidative stress. Quercetin can activate mitochondrial large-conductance Ca2+ to regulate potassium (mitoBKCa) channels, participate in mitochondrial depolarization, and protect brain tissue from HI damage (Kampa et al., 2021). Quercetin synergistically enhances mitochondrial spare respiration, maintains neuronal mitochondrial function, and increases the expression of CREB target genes (PGC-1a), which promote neuronal survival and mitochondrial biogenesis in an OGD model (Nichols et al., 2015). Furthermore, quercetin can control the Sirt1/Nrf2/HO-1 pathway, thus drastically reducing ROS formation following IS (Yang et al., 2021). In several IS models, quercetin revealed a dose-dependent reversal of OGD-induced declines in superoxide dismutase-1 (SOD1), SOD2, glutathione peroxidase-1 (GPX-1), and catalase (CAT) levels (Le et al., 2020), which may be achieved by its LPO-reducing capability (Shalavadi et al., 2020). Another study pointed out that quercetin also regulates the expression of oxidase and other antioxidant enzyme genes, thereby preventing IS-associated oxidative stress (Yamagata, 2019). Further studies showed that quercetin induced the expression of Nrf2 in erythrocytes, thus strongly inhibiting the production of adhesion molecules; this action may be related to the antioxidant effect of HO-1 (Li C. et al., 2016). Lee et al. (2016) discovered that quercetin increased the expression of Nrf2, HO-1, and nitric oxide synthase 1 (NOS1) in SHSY5Y cells, thus indicating its antioxidative stress impact.
4.4.2 Quercetin and Other Herbs
Quercetin and other herbal pre-treatments inhibit I/R-induced decreases in catalase and SOD enzyme activity, prevent LPO production, and increase GSH levels (Viswanatha et al., 2018; Viswanatha et al., 2019). Additionally, reduced NO and hippocampal lactate dehydrogenase (LDH) levels were observed in the cortex, striatum, and hippocampus of I/R rats (Ojo et al., 2019). Furthermore, intragastric injection of quercetin and rutin 10 min before reperfusion significantly reduced malondialdehyde (MDA) and myeloperoxidase (MPO) levels, increased endogenous antioxidant enzyme SOD and CAT levels, and improved I/R-induced inflammatory response (Annapurna et al., 2013).
4.4.3 Optimization of Quercetin
Optimization of quercetin can significantly improve its efficiency and pharmacological effects across the BBB. Quercetin liposome preparations slow down the decline of GSH levels in the ipsilateral striatum and cortex after ischemia; it also maintains GSH levels in the ischemic areas and increases GSH concentration in neuronal and glial cells (Rivera et al., 2008). During cerebral I/R, intracellular GSH levels significantly increased in young and old rats receiving nano-quercetin (27 mg/kg) (Ghosh et al., 2013). Quercetin/mAb GAP43-Exo targets neurons by mediating mAb GAP43, thus enhancing the accumulation of quercetin in the ischemic areas as well as inhibiting ROS production by activating the Nrf2/HO-1 pathway to increase LDH levels (Guo et al., 2021). Quercetin/mAb GAP43-Exo decreased oxidative stress-induced I/R damage by boosting the nuclear translocation of Nrf2 and upregulating the transcription of NAD(P)H dehydrogenase quinone-1 (NQO-1), HO-1, SOD1 and GPx1 (Guo et al., 2021) (Figure 3).
4.5 Protection of Hippocampal Neurons
IS significantly induced endogenous neurogenesis in the dentate gyrus of the hippocampus. However, newborn neurons are difficult to differentiate into mature neurons (Arvidsson et al., 2002; Doeppner et al., 2011). Quercetin maintains isocitrate dehydrogenase levels in MCAO animal models and helps to preserve neuronal cell energy production, thereby reducing IS-induced neuronal cell damage (Shah et al., 2018). Moreover, quercetin attenuates the decrease in PP2A subunit B expression caused by glutamate treatment, thus further reducing neuronal cell death (Park et al., 2019). Through the Sirt1/Nrf2/HO-1 signaling pathway, quercetin restores the normal structure of hippocampal neurons in I/R mice with severe neuronal injury (Yang et al., 2021). Quercetin also reduces the activity and pathophysiology of the following processes: protein tyrosine and serine/threonine phosphatase in rat cortical tissue, oxygen-glucose deprivation/reoxygenation (OGD/R) in hippocampal slices and neuronal/glial cell lines, phosphorylation of ERK and Akt, and I/R-induced hindbrain damage (Wang et al., 2020). Quercetin treatment can also significantly increase the activity of SHSY5Y cells and E18 mouse cortical neurons (Lee et al., 2016), enhance the expression of synaptophysin in PC12 cells in the OGD model, and promote neurite growth in PC12 cells (Orbán-Gyapai et al., 2014). Three days after reperfusion, oral administration of nano-encapsulated quercetin reduced the activity of iNOS and caspase-3, expanded the number of neurons in the hippocampus, and prevented neuronal cell damage (Ghosh et al., 2013) (Figure 3).
4.6 Promotion of Autophagy
The mechanism of quercetin-induced autophagy in cell survival is complex because of the large number of biomolecules involved in this process. Based on the scope of the damage caused by HI, autophagy is used as a pro-apoptotic signal, wherein quercetin can be used as its inducer (Costa et al., 2016). In models of oxidative damage and ischemia, studies have revealed that the protective impact of quercetin is directly linked to the induction of autophagy (Wu et al., 2017). As a result, autophagy is linked to the pro-survival mechanism of quercetin in IS-induced brain injury and other related events (Wang et al., 2011; Zhi et al., 2016; Granato et al., 2017; Liu et al., 2017) (Figure 3). Quercetin has a protective effect against MCAO-induced neuronal cell apoptosis and likewise induces autophagy-mediated neuronal PC12 cell survival (Ahn and Jeon, 2015; Park et al., 2018). In IS, if myeloid cells lack an autophagy response, inflammatory glial cells would thus play a significant role in neuronal cell apoptosis by increasing ischemia; this happens in compensation for the reduced activity of myeloid cells (Kotoda et al., 2018). In this case, autophagy protected the neurons from ischemia-induced cell death. Surprisingly, quercetin, like many other polyphenols, induces autophagy (Pallauf and Rimbach, 2013). Quercetin plays a role in cellular survival by activating autophagy in brain myeloid cells (Chang et al., 2017). Furthermore, in MCAO-induced ischemia, quercetin altered the apoptosis/autophagy interaction and its linkage with the nuclear factor kappa B (NF-κB) signaling pathway by upregulating ubiquitin carboxy-terminal hydrolase L1, which is a related gene enzyme, at almost double the rate (Chirumbolo et al., 2019).
4.7 Inhibition of Apoptosis
Quercetin upregulates the intracellular Ca2+ concentration in the cerebral cortical and hippocampal neurons of MCAO rats. It also regulates the gene expression of Bcl-2, Bax, and caspase-3, thereby preventing apoptosis (Park et al., 2020). Another study found that quercetin reduced HI-induced cortical cell death by blocking the neuro-inflammatory response mediated by the toll-like receptor 4 (TLR4)/nuclear factor-kappa B (NF-κB) signaling pathway (Wu et al., 2019). The anti-apoptotic activity of quercetin may be due to its ability to suppress inflammatory genes in BV2 microglia (Mrvová et al., 2015). In addition, quercetin has also been reported to improve I/R-induced cognitive deficits as well as inhibit neuronal apoptosis by increasing p-Akt and decreasing p-ASK1, P-JNK3, cleaved caspase-3, and FADD protein expressions (Pei et al., 2016). Furthermore, quercetin not only inhibits acid toxicity mediated by acid-sensing ion channels, but also improves neuronal apoptosis in focal cerebral ischemia by reducing caspase-3 and PARP expression through the PI3K/Akt pathway (Park et al., 2018). After local cerebral ischemia, human umbilical cord mesenchymal stem cells (HUMSCs) transplanted with quercetin can reduce pro-inflammatory cytokines IL-1B and IL-6, increase anti-inflammatory cytokines IL-4 and IL-10, inhibit the expression of apoptosis factor caspase-3, and promote the recovery of nerve function (Zhang et al., 2016). Furthermore, isorhamnetin (30-methoxy-3,40,5, 7-tetrahydroxy flavanone), a quercetin metabolite, has been demonstrated to lower blood pressure and endothelial dysfunction in spontaneously hypertensive rats (Sánchez et al., 2006; Sanchez et al., 2007). In the methylglyoxal-binding OGD model, isorhamnetin inhibited caspase 8 activation and decreased Fas and FasL expression, thereby lowering the activation and ability of NF-κB to perform an anti-mitochondrial-dependent apoptotic role (Li W. et al., 2016) (Figure 3).
5 Conclusion and Perspectives
Quercetin has a unique chemical structure and is widely found in our daily diet (e.g., vegetables and fruits), thus making it easy to obtain. Quercetin has showed respectable therapeutic effects on IS-induced models. It inhibits inflammatory thrombosis, reduces cerebral edema, infarct size, and oxidative stress, promotes autophagy and anti-apoptosis, and can be used as an adjuvant agent in the treatment of IS. Importantly, quercetin has been found to inhibit platelet activation and limit inflammatory thrombosis in both animal and clinical studies. The anti-inflammatory properties of quercetin are mediated by the regulation of the expression of various inflammatory factors. It also prevents neuronal death by stimulating the NF-κB signaling pathway, which suppresses caspase-3 and Bax, and promotes Bcl-2 expression.
To date, a number of studies have suggested that quercetin can be used as a neuroprotective drug in the treatment of IS. However, owing to its poor bioavailability and ability to cross the BBB, its application in the clinical setting is limited. Therefore, future research should focus on optimizing the conformation of quercetin or developing a quercetin nano-drug delivery system to improve its bioavailability and BBB passing rate. Meanwhile, we should pay attention to the effects of quercetin on neurogenesis and synaptic plasticity after IS. More clinical trials should also be designed to clarify the effective dose of quercetin in the treatment of IS. Quercetin also has a variety of metabolic components in the body; only a few studies have focused on the pharmacological effects of its metabolites, thus warranting further research on its biochemical and metabolic properties.
Author Contributions
LZ, LY, JM, and FY were involved in literature search, manuscript writing, draft preparation, graphic production, and content production. XC, WM, and SL were involved in conception, drafting, and editing of the manuscript.
Conflict of Interest
The authors declare that the research was conducted in the absence of any commercial or financial relationships that could be construed as a potential conflict of interest.
Publisher’s Note
All claims expressed in this article are solely those of the authors and do not necessarily represent those of their affiliated organizations, or those of the publisher, the editors and the reviewers. Any product that may be evaluated in this article, or claim that may be made by its manufacturer, is not guaranteed or endorsed by the publisher.
References
Abdelkhalek, A., Al-Askar, A. A., Alsubaie, M. M., and Behiry, S. I. (2021). First Report of Protective Activity of Paronychia Argentea Extract against Tobacco Mosaic Virus Infection. Plants 10 (11), 2435. doi:10.3390/plants10112435
Ahmad, A., Khan, M. M., Hoda, M. N., Raza, S. S., Khan, M. B., Javed, H., et al. (2011). Quercetin Protects against Oxidative Stress Associated Damages in a Rat Model of Transient Focal Cerebral Ischemia and Reperfusion. Neurochem. Res. 36 (8), 1360–1371. doi:10.1007/s11064-011-0458-6
Ahn, T. B., and Jeon, B. S. (2015). The Role of Quercetin on the Survival of Neuron-like PC12 Cells and the Expression of α-synuclein. Neural Regen. Res. 10 (7), 1113–1119. doi:10.4103/1673-5374.160106
Akinmoladun, A. C., Akinrinola, B. L., Olaleye, M. T., and Farombi, E. O. (2015). Kolaviron, a Garcinia Kola Biflavonoid Complex, Protects against Ischemia/reperfusion Injury: Pertinent Mechanistic Insights from Biochemical and Physical Evaluations in Rat Brain. Neurochem. Res. 40 (4), 777–787. doi:10.1007/s11064-015-1527-z
Alim, I., Caulfield, J. T., Chen, Y., Swarup, V., Geschwind, D. H., Ivanova, E., et al. (2019). Selenium Drives a Transcriptional Adaptive Program to Block Ferroptosis and Treat Stroke. Cell 177 (5), 1262–e25. e1225. doi:10.1016/j.cell.2019.03.032
Allen, C. L., and Bayraktutan, U. (2009). Oxidative Stress and its Role in the Pathogenesis of Ischaemic Stroke. Int. J. Stroke 4 (6), 461–470. doi:10.1111/j.1747-4949.2009.00387.x
Anand David, A. V., Arulmoli, R., and Parasuraman, S. (2016). Overviews of Biological Importance of Quercetin: A Bioactive Flavonoid. Pharmacogn. Rev. 10 (20), 84–89. doi:10.4103/0973-7847.194044
Annapurna, A., Ansari, M. A., and Manjunath, P. M. (2013). Partial Role of Multiple Pathways in Infarct Size Limiting Effect of Quercetin and Rutin against Cerebral Ischemia-Reperfusion Injury in Rats. Eur. Rev. Med. Pharmacol. Sci. 17 (4), 491–500.
Arvidsson, A., Collin, T., Kirik, D., Kokaia, Z., and Lindvall, O. (2002). Neuronal Replacement from Endogenous Precursors in the Adult Brain after Stroke. Nat. Med. 8 (9), 963–970. doi:10.1038/nm747
Assefa, A. D., Hur, O.-S., Hahn, B.-S., Kim, B., Ro, N.-Y., and Rhee, J.-H. (2021). Nutritional Metabolites of Red Pigmented Lettuce (Lactuca sativa) Germplasm and Correlations with Selected Phenotypic Characters. Foods 10 (10), 2504. doi:10.3390/foods10102504
Avan, A., Digaleh, H., Di Napoli, M., Stranges, S., Behrouz, R., and Shojaeianbabaei, G. (2019). Socioeconomic Status and Stroke Incidence, Prevalence, Mortality, and Worldwide Burden: An Ecological Analysis From the Global Burden of Disease Study 2017. BMC Medicine 17 (1), 191. doi:10.1186/s12916-019-1397-3
Barnes, S., Prasain, J., D'Alessandro, T., Arabshahi, A., Botting, N., Lila, M. A., et al. (2011). The Metabolism and Analysis of Isoflavones and Other Dietary Polyphenols in Foods and Biological Systems. Food Funct. 2 (5), 235–244. doi:10.1039/c1fo10025d
Bashmil, Y. M., Ali, A., Bk, A., Dunshea, F. R., and Suleria, H. A. R. (2021). Screening and Characterization of Phenolic Compounds from Australian Grown Bananas and Their Antioxidant Capacity. Antioxidants 10 (10), 1521. doi:10.3390/antiox10101521
Bhaskar, S., Sudhakaran, P. R., and Helen, A. (2016). Quercetin Attenuates Atherosclerotic Inflammation and Adhesion Molecule Expression by Modulating TLR-NF-Κb Signaling Pathway. Cell Immunol. 310, 131–140. doi:10.1016/j.cellimm.2016.08.011
Bjeldanes, L. F., and Chang, G. W. (1977). Mutagenic Activity of Quercetin and Related Compounds. Science 197 (4303), 577–578. doi:10.1126/science.327550
Brait, V. H., Miró-Mur, F., Pérez-de-Puig, I., Notario, L., Hurtado, B., Pedragosa, J., et al. (2019). CD69 Plays a Beneficial Role in Ischemic Stroke by Dampening Endothelial Activation. Circ. Res. 124 (2), 279–291. doi:10.1161/circresaha.118.313818
Brüll, V., Burak, C., Stoffel-Wagner, B., Wolffram, S., Nickenig, G., Müller, C., et al. (2015). Effects of a Quercetin-Rich Onion Skin Extract on 24 H Ambulatory Blood Pressure and Endothelial Function in Overweight-To-Obese Patients with (Pre-)hypertension: a Randomised Double-Blinded Placebo-Controlled Cross-Over Trial. Br. J. Nutr. 114 (8), 1263–1277. doi:10.1017/s0007114515002950
Cao, J. Y., and Dixon, S. J. (2016). Mechanisms of Ferroptosis. Cell Mol. Life Sci. 73, 2195–2209. doi:10.1007/s00018-016-2194-1
Casas, A. I., Geuss, E., Kleikers, P. W. M., Mencl, S., Herrmann, A. M., Buendia, I., et al. (2017). NOX4-dependent Neuronal Autotoxicity and BBB Breakdown Explain the Superior Sensitivity of the Brain to Ischemic Damage. Proc. Natl. Acad. Sci. U. S. A. 114 (46), 12315–12320. doi:10.1073/pnas.1705034114
Chang, J. L., Chow, J. M., Chang, J. H., Wen, Y. C., Lin, Y. W., Yang, S. F., et al. (2017). Quercetin Simultaneously Induces G0/G1 -phase Arrest and Caspase-Mediated Crosstalk between Apoptosis and Autophagy in Human Leukemia HL-60 Cells. Environ. Toxicol. 32 (7), 1857–1868. doi:10.1002/tox.22408
Chen, X., Yin, O. Q., Zuo, Z., and Chow, M. S. (2005). Pharmacokinetics and Modeling of Quercetin and Metabolites. Pharm. Res. 22 (6), 892–901. doi:10.1007/s11095-005-4584-1
Chirumbolo, S., Vella, A., and Bjørklund, G. (2019). Quercetin Might Promote Autophagy in a Middle Cerebral Artery Occlusion-Mediated Ischemia Model: Comments on Fawad-Ali Shah et al. Neurochem. Res. 44 (2), 297–300. doi:10.1007/s11064-018-2692-7
Choi, I. Y., Lim, J. H., Kim, C., Song, H. Y., Ju, C., and Kim, W. K. (2013). 4-hydroxy-2(E)-Nonenal Facilitates NMDA-Induced Neurotoxicity via Triggering Mitochondrial Permeability Transition Pore Opening and Mitochondrial Calcium Overload. Exp. Neurobiol. 22 (3), 200–207. doi:10.5607/en.2013.22.3.200
Clarkson, B. D., Ling, C., Shi, Y., Harris, M. G., Rayasam, A., Sun, D., et al. (2014). T Cell-Derived Interleukin (IL)-21 Promotes Brain Injury Following Stroke in Mice. J. Exp. Med. 211 (4), 595–604. doi:10.1084/jem.20131377
Constantinescu-Bercu, A., Wang, Y. A., Woollard, K. J., Mangin, P., Vanhoorelbeke, K., Crawley, J. T. B., et al. (2022). The GPIbα Intracellular Tail - Role in Transducing VWF- and collagen/GPVI-Mediated Signaling. Haematologica 107 (4), 933–946. doi:10.3324/haematol.2020.278242
Costa, L. G., Garrick, J. M., Roquè, P. J., and Pellacani, C. (2016). Mechanisms of Neuroprotection by Quercetin: Counteracting Oxidative Stress and More. Oxid. Med. Cell Longev. 2016, 2986796. doi:10.1155/2016/2986796
Culmsee, C., and Plesnila, N. (2006). Targeting Bid to Prevent Programmed Cell Death in Neurons. Biochem. Soc. Trans. 34, 1334–1340. doi:10.1042/bst0341334
Dajas, F. (2012). Life or Death: Neuroprotective and Anticancer Effects of Quercetin. J. Ethnopharmacol. 143 (2), 383–396. doi:10.1016/j.jep.2012.07.005
Darband, S. G., Kaviani, M., Yousefi, B., Sadighparvar, S., Pakdel, F. G., Attari, J. A., et al. (2018). Quercetin: A Functional Dietary Flavonoid with Potential Chemo-Preventive Properties in Colorectal Cancer. J. Cell Physiol. 233 (9), 6544–6560. doi:10.1002/jcp.26595
De Meyer, S. F., Langhauser, F., Haupeltshofer, S., Kleinschnitz, C., and Casas, A. I. (2022). Thromboinflammation in Brain Ischemia: Recent Updates and Future Perspectives. Stroke. 53(5), 101161strokeaha122038733. doi:10.1161/strokeaha.122.038733
Dehghani, F., Sezavar Seyedi Jandaghi, S. H., Janani, L., Sarebanhassanabadi, M., Emamat, H., and Vafa, M. (2021). Effects of Quercetin Supplementation on Inflammatory Factors and Quality of Life in Post-myocardial Infarction Patients: A Double Blind, Placebo-Controlled, Randomized Clinical Trial. Phytother. Res. 35 (4), 2085–2098. doi:10.1002/ptr.6955
Denorme, F., Langhauser, F., Desender, L., Vandenbulcke, A., Rottensteiner, H., Plaimauer, B., et al. (2016). ADAMTS13-mediated Thrombolysis of t-PA-resistant Occlusions in Ischemic Stroke in Mice. Blood 127 (19), 2337–2345. doi:10.1182/blood-2015-08-662650
Dhanesha, N., Patel, R. B., Doddapattar, P., Ghatge, M., Flora, G. D., Jain, M., et al. (2022). PKM2 Promotes Neutrophil Activation and Cerebral Thromboinflammation: Therapeutic Implications for Ischemic Stroke. Blood 139 (8), 1234–1245. doi:10.1182/blood.2021012322
Dhiman, P., Malik, N., Sobarzo-Sánchez, E., Uriarte, E., and Khatkar, A. (2019). Quercetin and Related Chromenone Derivatives as Monoamine Oxidase Inhibitors: Targeting Neurological and Mental Disorders. Molecules 24 (3). doi:10.3390/molecules24030418
Di Pierro, F., Iqtadar, S., Khan, A., Ullah Mumtaz, S., Masud Chaudhry, M., Bertuccioli, A., et al. (2021). Potential Clinical Benefits of Quercetin in the Early Stage of COVID-19: Results of a Second, Pilot, Randomized, Controlled and Open-Label Clinical Trial. Int. J. Gen. Med. 14, 2807–2816. doi:10.2147/ijgm.S318949
Doeppner, T. R., Kaltwasser, B., ElAli, A., Zechariah, A., Hermann, D. M., and Bähr, M. (2011). Acute Hepatocyte Growth Factor Treatment Induces Long-Term Neuroprotection and Stroke Recovery via Mechanisms Involving Neural Precursor Cell Proliferation and Differentiation. J. Cereb. Blood Flow. Metab. 31 (5), 1251–1262. doi:10.1038/jcbfm.2010.211
Feitsma, L. J., Brondijk, H. C., Jarvis, G. E., Hagemans, D., Bihan, D. G., Jerah, N. E., et al. (2022). Structural Insights into Collagen-Binding by Platelet Receptor Glycoprotein VI. Blood. doi:10.1182/blood.2021013614
Fredotović, Ž., Puizina, J., Nazlić, M., Maravić, A., Ljubenkov, I., Soldo, B., et al. (2021). Phytochemical Characterization and Screening of Antioxidant, Antimicrobial and Antiproliferative Properties of Allium × Cornutum Clementi and Two Varieties of Allium cepa L. Peel Extracts. Plants 10 (5), 832. doi:10.3390/plants10050832
GBD (2021). Global, Regional, and National Burden of Stroke and its Risk Factors, 1990-2019: a Systematic Analysis for the Global Burden of Disease Study 2019. LancetNeurology. 20(10). doi:10.1016/s1474-4422(21)00252-0
Gelderblom, M., Weymar, A., Bernreuther, C., Velden, J., Arunachalam, P., Steinbach, K., et al. (2012). Neutralization of the IL-17 axis Diminishes Neutrophil Invasion and Protects from Ischemic Stroke. Blood 120 (18), 3793–3802. doi:10.1182/blood-2012-02-412726
Genchi, A., Semerano, A., Schwarz, G., Dell'Acqua, B., Gullotta, G. S., Sampaolo, M., et al. (2022). Neutrophils Predominate the Immune Signature of Cerebral Thrombi in COVID-19 Stroke Patients. Acta Neuropathol. Commun. 10 (1), 14. doi:10.1186/s40478-022-01313-y
Ghosh, A., Sarkar, S., Mandal, A. K., and Das, N. (2013). Neuroprotective Role of Nanoencapsulated Quercetin in Combating Ischemia-Reperfusion Induced Neuronal Damage in Young and Aged Rats. PloS one 8 (4), e57735. doi:10.1371/journal.pone.0057735
Gibbins, J., Asselin, J., Farndale, R., Barnes, M., Law, C. L., and Watson, S. P. (1996). Tyrosine Phosphorylation of the Fc Receptor Gamma-Chain in Collagen-Stimulated Platelets. J. Biol. Chem. 271 (30), 18095–18099. doi:10.1074/jbc.271.30.18095
Girardelo, J. R., Munari, E. L., Dallorsoleta, J. C. S., Cechinel, G., Goetten, A. L. F., Sales, L. R., et al. (202010961). Bioactive Compounds, Antioxidant Capacity and Antitumoral Activity of Ethanolic Extracts from Fruits and Seeds of Eugenia Involucrata DC. Food Res. Int. 137, 109615. doi:10.1016/j.foodres.2020.109615
Graefe, E. U., Derendorf, H., and Veit, M. (1999). Pharmacokinetics and Bioavailability of the Flavonol Quercetin in Humans. Int. J. Clin. Pharmacol. Ther. 37 (5), 219–233.
Granato, M., Rizzello, C., Gilardini Montani, M. S., Cuomo, L., Vitillo, M., Santarelli, R., et al. (2017). Quercetin Induces Apoptosis and Autophagy in Primary Effusion Lymphoma Cells by Inhibiting PI3K/AKT/mTOR and STAT3 Signaling Pathways. J. Nutr. Biochem. 41, 124–136. doi:10.1016/j.jnutbio.2016.12.011
Guevara, L., Domínguez-Anaya, M. Á., Ortigosa, A., González-Gordo, S., Díaz, C., Vicente, F., et al. (2021). Identification of Compounds with Potential Therapeutic Uses from Sweet Pepper (Capsicum Annuum L.) Fruits and Their Modulation by Nitric Oxide (NO). Int. J. Mol. Sci. 22 (9). doi:10.3390/ijms22094476
Guo, L., Huang, Z., Huang, L., Liang, J., Wang, P., Zhao, L., et al. (2021). Surface-modified Engineered Exosomes Attenuated Cerebral Ischemia/reperfusion Injury by Targeting the Delivery of Quercetin towards Impaired Neurons. J. Nanobiotechnol 19 (1), 141. doi:10.1186/s12951-021-00879-4
Hanganu, D., Niculae, M., Ielciu, I., Olah, N.-K., Muntean, M., Burtescu, R., et al. (2021). Chemical Profile, Cytotoxic Activity and Oxidative Stress Reduction of Different Syringa Vulgaris L. Extracts. Molecules 26 (11), 3104. doi:10.3390/molecules26113104
Haruwaka, K., Ikegami, A., Tachibana, Y., Ohno, N., Konishi, H., Hashimoto, A., et al. (2019). Dual Microglia Effects on Blood Brain Barrier Permeability Induced by Systemic Inflammation. Nat. Commun. 10 (1), 5816. doi:10.1038/s41467-019-13812-z
Heo, J. H., Nam, H. S., Kim, Y. D., Choi, J. K., Kim, B. M., Kim, D. J., et al. (2020). Pathophysiologic and Therapeutic Perspectives Based on Thrombus Histology in Stroke. J. Stroke 22 (1), 64–75. doi:10.5853/jos.2019.03440
Huang, Y. Y., Wang, Z. H., Deng, L. H., Wang, H., and Zheng, Q. (2020). Oral Administration of Quercetin or its Derivatives Inhibit Bone Loss in Animal Model of Osteoporosis. Oxid. Med. Cell Longev. 2020, 6080597. doi:10.1155/2020/6080597
Hubbard, G. P., Stevens, J. M., Cicmil, M., Sage, T., Jordan, P. A., Williams, C. M., et al. (2003). Quercetin Inhibits Collagen-Stimulated Platelet Activation through Inhibition of Multiple Components of the Glycoprotein VI Signaling Pathway. J. Thromb. Haemost. 1 (5), 1079–1088. doi:10.1046/j.1538-7836.2003.00212.x
Hubbard, G. P., Wolffram, S., de Vos, R., Bovy, A., Gibbins, J. M., and Lovegrove, J. A. (2006). Ingestion of Onion Soup High in Quercetin Inhibits Platelet Aggregation and Essential Components of the Collagen-Stimulated Platelet Activation Pathway in Man: a Pilot Study. Br. J. Nutr. 96 (3), 482–488.
Hubbard, G. P., Wolffram, S., Lovegrove, J. A., and Gibbins, J. M. (2004). Ingestion of Quercetin Inhibits Platelet Aggregation and Essential Components of the Collagen-Stimulated Platelet Activation Pathway in Humans. J. Thromb. Haemost. 2 (12), 2138–2145. doi:10.1111/j.1538-7836.2004.01067.x
Jaimand, K., Rezaee, M. B., Behrad, Z., and Najafy-Ashtiany, A. (2012). Comparison of Extraction and Measurement of Quercetin from Stigma, Style, Sepals, Petals and Stamen of Crocus Sativus by HPLC in Combination with Heat and Ultrasonic. J. Med. Plants Prod. 2, 167–170.
Jasuja, R., Passam, F. H., Kennedy, D. R., Kim, S. H., van Hessem, L., Lin, L., et al. (2012). Protein Disulfide Isomerase Inhibitors Constitute a New Class of Antithrombotic Agents. J. Clin. Invest. 122 (6), 2104–2113. doi:10.1172/jci61228
Jo, H. M., Ahn, C., Kim, H., Kang, B. T., Jeung, E. B., and Yang, M. P. (2021). Effect of Quercetin on Formation of Porcine Neutrophil Extracellular Trap. Vet. Immunol. Immunopathol. 241, 110335. doi:10.1016/j.vetimm.2021.110335
Jordan, J., de Groot, P. W., and Galindo, M. F. (2011). Mitochondria: the Headquarters in Ischemia-Induced Neuronal Death. Cent. Nerv. Syst. Agents Med. Chem. 11 (2), 98–106. doi:10.2174/187152411796011358
Kalogiouri, N. P., and Samanidou, V. F. (2021). A Validated Ultrasound-Assisted Extraction Coupled with SPE-HPLC-DAD for the Determination of Flavonoids in By-Products of Plant Origin: An Application Study for the Valorization of the Walnut Septum Membrane. Molecules 26 (21), 6418. doi:10.3390/molecules26216418
Kampa, R. P., Sęk, A., Szewczyk, A., and Bednarczyk, P. (2021). Cytoprotective Effects of the Flavonoid Quercetin by Activating Mitochondrial BKCa Channels in Endothelial Cells. Biomed. Pharmacother. 142, 112039. doi:10.1016/j.biopha.2021.112039
Kanaji, S., Morodomi, Y., Weiler, H., Zarpellon, A., Montgomery, R. R., Ruggeri, Z. M., et al. (2022). The impact of aberrant von Willebrand Factor-GPIbα Interaction on Megakaryopoiesis and Platelets in Humanized Type 2B von Willebrand Disease Model Mouse. Haematologica. doi:10.3324/haematol.2021.280561
Karuppagounder, S. S., Alim, I., Khim, S. J., Bourassa, M. W., Sleiman, S. F., John, R., et al. (2016). Therapeutic Targeting of Oxygen-Sensing Prolyl Hydroxylases Abrogates ATF4-dependent Neuronal Death and Improves Outcomes after Brain Hemorrhage in Several Rodent Models. Sci. Transl. Med. 8 (328), 328ra29. 328ra329. doi:10.1126/scitranslmed.aac6008
Kleinschnitz, C., Grund, H., Wingler, K., Armitage, M. E., Jones, E., Mittal, M., et al. (2010). Post-stroke Inhibition of Induced NADPH Oxidase Type 4 Prevents Oxidative Stress and Neurodegeneration. PLoS Biol. 8 (9). doi:10.1371/journal.pbio.1000479
Knekt, P., Isotupa, S., Rissanen, H., Heliövaara, M., Järvinen, R., Häkkinen, S., et al. (2000). Quercetin Intake and the Incidence of Cerebrovascular Disease. Eur. J. Clin. Nutr. 54 (5), 415–417. doi:10.1038/sj.ejcn.1600974
Kotoda, M., Furukawa, H., Miyamoto, T., Korai, M., Shikata, F., Kuwabara, A., et al. (2018). Role of Myeloid Lineage Cell Autophagy in Ischemic Brain Injury. Stroke 49 (6), 1488–1495. doi:10.1161/strokeaha.117.018637
Le Behot, A., Gauberti, M., Martinez De Lizarrondo, S., Montagne, A., Lemarchand, E., Repesse, Y., et al. (2014). GpIbα-VWF Blockade Restores Vessel Patency by Dissolving Platelet Aggregates Formed under Very High Shear Rate in Mice. Blood 123 (21), 3354–3363. doi:10.1182/blood-2013-12-543074
Le, K., Song, Z., Deng, J., Peng, X., Zhang, J., Wang, L., et al. (2020). Quercetin Alleviates Neonatal Hypoxic-Ischemic Brain Injury by Inhibiting Microglia-Derived Oxidative Stress and TLR4-Mediated Inflammation. Inflamm. Res. 69 (12), 1201–1213. doi:10.1007/s00011-020-01402-5
Lee, J. K., Kwak, H. J., Piao, M. S., Jang, J. W., Kim, S. H., and Kim, H. S. (2011). Quercetin Reduces the Elevated Matrix Metalloproteinases-9 Level and Improves Functional Outcome after Cerebral Focal Ischemia in Rats. Acta Neurochir. (Wien) 153 (6), 1321–1329. discussion 1329. doi:10.1007/s00701-010-0889-x
Lee, S. M., Moon, J., Chung, J. H., Cha, Y. J., and Shin, M. J. (2013). Effect of Quercetin-Rich Onion Peel Extracts on Arterial Thrombosis in Rats. Food Chem. Toxicol. 57, 99–105. doi:10.1016/j.fct.2013.03.008
Lee, Y. H., Kim, H. J., Yoo, H., Jung, S. Y., Kwon, B. J., Kim, N. J., et al. (2015). Synthesis of (2-amino)ethyl Derivatives of Quercetin 3-O-Methyl Ether and Their Antioxidant and Neuroprotective Effects. Bioorg Med. Chem. 23 (15), 4970–4979. doi:10.1016/j.bmc.2015.05.023
Lee, Y. J., Bernstock, J. D., Nagaraja, N., Ko, B., and Hallenbeck, J. M. (2016). Global SUMOylation Facilitates the Multimodal Neuroprotection Afforded by Quercetin against the Deleterious Effects of Oxygen/glucose Deprivation and the Restoration of Oxygen/glucose. J. Neurochem. 138 (1), 101–116. doi:10.1111/jnc.13643
Leyva-Soto, A., Alejandra Chavez-Santoscoy, R., Porras, O., Hidalgo-Ledesma, M., Serrano-Medina, A., Alejandra Ramírez-Rodríguez, A., et al. (2021). Epicatechin and Quercetin Exhibit In Vitro Antioxidant Effect, Improve Biochemical Parameters Related to Metabolic Syndrome, and Decrease Cellular Genotoxicity in Humans. Food Res. Int. 142, 110101. doi:10.1016/j.foodres.2020.110101
Li, C., Zhang, W. J., and Frei, B. (2016a). Quercetin Inhibits LPS-Induced Adhesion Molecule Expression and Oxidant Production in Human Aortic Endothelial Cells by P38-Mediated Nrf2 Activation and Antioxidant Enzyme Induction. Redox Biol. 9, 104–113. doi:10.1016/j.redox.2016.06.006
Li, J., Kim, K., Barazia, A., Tseng, A., and Cho, J. (2015). Platelet-neutrophil Interactions under Thromboinflammatory Conditions. Cell Mol. Life Sci. 72 (14), 2627–2643. doi:10.1007/s00018-015-1845-y
Li, W., Chen, Z., Yan, M., He, P., Chen, Z., and Dai, H. (2016b). The Protective Role of Isorhamnetin on Human Brain Microvascular Endothelial Cells from Cytotoxicity Induced by Methylglyoxal and Oxygen-Glucose Deprivation. J. Neurochem. 136 (3), 651–659. doi:10.1111/jnc.13436
Liang, M. L., Da, X. W., He, A. D., Yao, G. Q., Xie, W., Liu, G., et al. (2015). Pentamethylquercetin (PMQ) Reduces Thrombus Formation by Inhibiting Platelet Function. Sci. Rep. 5, 11142. doi:10.1038/srep11142
Liesz, A., Suri-Payer, E., Veltkamp, C., Doerr, H., Sommer, C., Rivest, S., et al. (2009). Regulatory T Cells Are Key Cerebroprotective Immunomodulators in Acute Experimental Stroke. Nat. Med. 15 (2), 192–199. doi:10.1038/nm.1927
Liu, J. J., Song, C. W., Yue, Y., Duan, C. G., Yang, J., He, T., et al. (2005b). Quercetin Inhibits LPS-Induced Delay in Spontaneous Apoptosis and Activation of Neutrophils. Inflamm. Res. 54 (12), 500–507. doi:10.1007/s00011-005-1385-2
Liu, J., Li, X., Yue, Y., Li, J., He, T., and He, Y. (2005a). The Inhibitory Effect of Quercetin on IL-6 Production by LPS-Stimulated Neutrophils. Cell Mol. Immunol. 2 (6), 455–460.
Liu, Y., Gong, W., Yang, Z. Y., Zhou, X. S., Gong, C., Zhang, T. R., et al. (2017). Quercetin Induces Protective Autophagy and Apoptosis through ER Stress via the P-STAT3/Bcl-2 axis in Ovarian Cancer. Apoptosis 22 (4), 544–557. doi:10.1007/s10495-016-1334-2
Lo, E. H., Dalkara, T., and Moskowitz, M. A. (2003). Mechanisms, Challenges and Opportunities in Stroke. Nat. Rev. Neurosci. 4 (5), 399–415. doi:10.1038/nrn1106
Loke, W. M., Proudfoot, J. M., McKinley, A. J., Needs, P. W., Kroon, P. A., Hodgson, J. M., et al. (2008). Quercetin and its In Vivo Metabolites Inhibit Neutrophil-Mediated Low-Density Lipoprotein Oxidation. J. Agric. Food Chem. 56 (10), 3609–3615. doi:10.1021/jf8003042
Lu, N., Sui, Y., Tian, R., and Peng, Y. Y. (2018). Inhibitive Effects of Quercetin on Myeloperoxidase-dependent Hypochlorous Acid Formation and Vascular Endothelial Injury. J. Agric. Food Chem. 66 (19), 4933–4940. doi:10.1021/acs.jafc.8b01537
Ma, Y., Wang, J., Wang, Y., and Yang, G. Y. (2017). The Biphasic Function of Microglia in Ischemic Stroke. Prog. Neurobiol. 157, 247–272. doi:10.1016/j.pneurobio.2016.01.005
Magar, R. T., and Sohng, J. K. (2020). A Review on Structure, Modifications and Structure-Activity Relation of Quercetin and its Derivatives. J. Microbiol. Biotechnol. 30 (1), 11–20. doi:10.4014/jmb.1907.07003
Manach, C., Scalbert, A., Morand, C., Rémésy, C., and Jiménez, L. (2004). Polyphenols: Food Sources and Bioavailability. Am. J. Clin. Nutr. 79 (5), 727–747. doi:10.1093/ajcn/79.5.727
Mar, J. M., da Silva, L. S., Moreira, W. P., Biondo, M. M., Pontes, F. L. D., Campos, F. R., et al. (2021). Edible Flowers from Theobroma Speciosum: Aqueous Extract Rich in Antioxidant Compounds. Food Chem. 356, 129723. doi:10.1016/j.foodchem.2021.129723
Moro, T. M. A., and Clerici, M. T. P. S. (2021). Burdock (Arctium Lappa L) Roots as a Source of Inulin-type Fructans and Other Bioactive Compounds: Current Knowledge and Future Perspectives for Food and Non-food Applications. Food Res. Int. 141, 109889. doi:10.1016/j.foodres.2020.109889
Mosawy, S., Jackson, D. E., Woodman, O. L., and Linden, M. D. (2013a). Inhibition of Platelet-Mediated Arterial Thrombosis and Platelet Granule Exocytosis by 3',4'-dihydroxyflavonol and Quercetin. Platelets 24 (8), 594–604. doi:10.3109/09537104.2012.749396
Mosawy, S., Jackson, D. E., Woodman, O. L., and Linden, M. D. (2014). The Flavonols Quercetin and 3',4'-dihydroxyflavonol Reduce Platelet Function and Delay Thrombus Formation in a Model of Type 1 Diabetes. Diab Vasc. Dis. Res. 11 (3), 174–181. doi:10.1177/1479164114524234
Mosawy, S., Jackson, D. E., Woodman, O. L., and Linden, M. D. (2013b). Treatment with Quercetin and 3',4'-dihydroxyflavonol Inhibits Platelet Function and Reduces Thrombus Formation In Vivo. J. Thromb. Thrombolysis 36 (1), 50–57. doi:10.1007/s11239-012-0827-2
Mrvová, N., Škandík, M., Kuniaková, M., and Račková, L. (2015). Modulation of BV-2 Microglia Functions by Novel Quercetin Pivaloyl Ester. Neurochem. Int. 90, 246–254. doi:10.1016/j.neuint.2015.09.005
Mukhopadhyay, P., and Prajapati, A. K. (2015). Quercetin in Anti-diabetic Research and Strategies for Improved Quercetin Bioavailability Using Polymer-Based Carriers - a Review. RSC Adv. 5 (118), 97547–97562. doi:10.1039/c5ra18896b
Muthian, G., and Bright, J. J. (2004). Quercetin, a Flavonoid Phytoestrogen, Ameliorates Experimental Allergic Encephalomyelitis by Blocking IL-12 Signaling through JAK-STAT Pathway in T Lymphocyte. J. Clin. Immunol. 24 (5), 542–552. doi:10.1023/B:JOCI.0000040925.55682.a5
Mwaurah, P. W., Kumar, S., Kumar, N., Panghal, A., Attkan, A. K., Singh, V. K., et al. (2020). Physicochemical Characteristics, Bioactive Compounds and Industrial Applications of Mango Kernel and its Products: A Review. Compr. Rev. food Sci. food Saf. 19 (5), 2421–2446. doi:10.1111/1541-4337.12598
Najda, A., Klimek, K., Balant, S., Wrzesinska-Jedrusiak, E., and Piekarski, W. (2019). Optimization of the Process of Polyphenol Extraction from Mentha Spicata with Various Solvents. Przemysl Chem. 98 (8), 1286–1289.
Naseri, N., Valizadeh, H., and Zakeri-Milani, P. (2015). Solid Lipid Nanoparticles and Nanostructured Lipid Carriers: Structure, Preparation and Application. Adv. Pharm. Bull. 5 (3), 305–313. doi:10.15171/apb.2015.043
Nichols, M., Zhang, J., Polster, B. M., Elustondo, P. A., Thirumaran, A., Pavlov, E. V., et al. (2015). Synergistic Neuroprotection by Epicatechin and Quercetin: Activation of Convergent Mitochondrial Signaling Pathways. Neuroscience 308, 75–94. doi:10.1016/j.neuroscience.2015.09.012
Nieto-Trujillo, A., Cruz-Sosa, F., Luria-Pérez, R., Gutiérrez-Rebolledo, G. A., Román-Guerrero, A., Burrola-Aguilar, C., et al. (2021). Arnica montana Cell Culture Establishment, and Assessment of its Cytotoxic, Antibacterial, α-Amylase Inhibitor, and Antioxidant In Vitro Bioactivities. Plants 10 (11), 2300. doi:10.3390/plants10112300
O'Brien, J. R., and Salmon, G. P. (1990). An Independent Haemostatic Mechanism: Shear Induced Platelet Aggregation. Adv. Exp. Med. Biol. 281, 287–296. doi:10.1007/978-1-4615-3806-6_30
Oboh, G., Ademosun, A. O., and Ogunsuyi, O. B. (2016). Quercetin and its Role in Chronic Diseases. Adv. Exp. Med. Biol. 929, 377–387. doi:10.1007/978-3-319-41342-6_17
Oh, T. W., Do, H. J., Jeon, J. H., and Kim, K. (2021). Quercitrin Inhibits Platelet Activation in Arterial Thrombosis. Phytomedicine 80, 153363. doi:10.1016/j.phymed.2020.153363
Ojo, O. B., Amoo, Z. A., Saliu, I. O., Olaleye, M. T., Farombi, E. O., and Akinmoladun, A. C. (2019). Neurotherapeutic Potential of Kolaviron on Neurotransmitter Dysregulation, Excitotoxicity, Mitochondrial Electron Transport Chain Dysfunction and Redox Imbalance in 2-VO Brain Ischemia/reperfusion Injury. Biomed. Pharmacother. 111, 859–872. doi:10.1016/j.biopha.2018.12.144
Oliveira, A. I., Pinho, C., Sarmento, B., and Dias, A. C. P. (2021). Quercetin-biapigenin Nanoparticles Are Effective to Penetrate the Blood-Brain Barrier. Drug Deliv. Transl. Res. 12, 267–281. doi:10.1007/s13346-021-00917-6
Orbán-Gyapai, O., Raghavan, A., Vasas, A., Forgo, P., Hohmann, J., and Shah, Z. A. (2014). Flavonoids Isolated from Rumex Aquaticus Exhibit Neuroprotective and Neurorestorative Properties by Enhancing Neurite Outgrowth and Synaptophysin. CNS Neurol. Disord. Drug Targets 13 (8), 1458–1464. doi:10.2174/1871527313666141023154446
Pallauf, K., and Rimbach, G. (2013). Autophagy, Polyphenols and Healthy Ageing. Ageing Res. Rev. 12 (1), 237–252. doi:10.1016/j.arr.2012.03.008
Pan, W., Chang, M. J., Booyse, F. M., Grenett, H. E., Bradley, K. M., Wolkowicz, P. E., et al. (2008). Quercetin Induced Tissue-type Plasminogen Activator Expression Is Mediated through Sp1 and P38 Mitogen-Activated Protein Kinase in Human Endothelial Cells. J. Thromb. Haemost. 6 (6), 976–985. doi:10.1111/j.1538-7836.2008.02977.x
Park, D. J., Jeon, S. J., Kang, J. B., and Koh, P. O. (2020). Quercetin Reduces Ischemic Brain Injury by Preventing Ischemia-Induced Decreases in the Neuronal Calcium Sensor Protein Hippocalcin. Neuroscience 430, 47–62. doi:10.1016/j.neuroscience.2020.01.015
Park, D. J., Kang, J. B., Shah, M. A., and Koh, P. O. (2019). Quercetin Alleviates the Injury-Induced Decrease of Protein Phosphatase 2A Subunit B in Cerebral Ischemic Animal Model and Glutamate-Exposed HT22 Cells. J. Vet. Med. Sci. 81 (7), 1047–1054. doi:10.1292/jvms.19-0094
Park, D. J., Shah, F. A., and Koh, P. O. (2018). Quercetin Attenuates Neuronal Cells Damage in a Middle Cerebral Artery Occlusion Animal Model. J. Vet. Med. Sci. 80 (4), 676–683. doi:10.1292/jvms.17-0693
Pateiro, M., Gómez, B., Munekata, P. E. S., Barba, F. J., Putnik, P., Kovačević, D. B., et al. (2021). Nanoencapsulation of Promising Bioactive Compounds to Improve Their Absorption, Stability, Functionality and the Appearance of the Final Food Products. Molecules 26 (6), 1547. doi:10.3390/molecules26061547
Pei, B., Yang, M., Qi, X., Shen, X., Chen, X., and Zhang, F. (2016). Quercetin Ameliorates Ischemia/reperfusion-Induced Cognitive Deficits by Inhibiting ASK1/JNK3/caspase-3 by Enhancing the Akt Signaling Pathway. Biochem. Biophys. Res. Commun. 478 (1), 199–205. doi:10.1016/j.bbrc.2016.07.068
Pircher, J., Engelmann, B., Massberg, S., and Schulz, C. (2019). Platelet-Neutrophil Crosstalk in Atherothrombosis. Thromb. Haemost. 119 (8), 1274–1282. doi:10.1055/s-0039-1692983
Poole, A., Gibbins, J. M., Turner, M., van Vugt, M. J., van de Winkel, J. G., Saito, T., et al. (1997). The Fc Receptor Gamma-Chain and the Tyrosine Kinase Syk Are Essential for Activation of Mouse Platelets by Collagen. Embo J. 16 (9), 2333–2341. doi:10.1093/emboj/16.9.2333
Poulter, N. S., Pollitt, A. Y., Owen, D. M., Gardiner, E. E., Andrews, R. K., Shimizu, H., et al. (2017). Clustering of Glycoprotein VI (GPVI) Dimers upon Adhesion to Collagen as a Mechanism to Regulate GPVI Signaling in Platelets. J. Thromb. Haemost. 15 (3), 549–564. doi:10.1111/jth.13613
Pourakbar, L., Moghaddam, S. S., Enshasy, H. A. E., and Sayyed, R. Z. (2021). Antifungal Activity of the Extract of a Macroalgae, Gracilariopsis Persica, against Four Plant Pathogenic Fungi. Plants 10 (9), 1781. doi:10.3390/plants10091781
Qu, X., Qi, D., Dong, F., Wang, B., Guo, R., Luo, M., et al. (2014). Quercetin Improves Hypoxia-Ischemia Induced Cognitive Deficits via Promoting Remyelination in Neonatal Rat. Brain Res. 1553, 31–40. doi:10.1016/j.brainres.2014.01.035
Ratnam, D. V., Ankola, D. D., Bhardwaj, V., Sahana, D. K., and Kumar, M. N. (2006). Role of Antioxidants in Prophylaxis and Therapy: A Pharmaceutical Perspective. J. Control Release 113 (3), 189–207. doi:10.1016/j.jconrel.2006.04.015
Rice-Evans, C., Miller, N., and Paganga, G. (1997). Antioxidant Properties of Phenolic Compounds. Trends plant Sci. 2 (4), 152–159. doi:10.1016/s1360-1385(97)01018-2
Riva, A., Ronchi, M., Petrangolini, G., Bosisio, S., and Allegrini, P. (2019). Improved Oral Absorption of Quercetin from Quercetin Phytosome®, a New Delivery System Based on Food Grade Lecithin. Eur. J. Drug Metab. Pharmacokinet. 44 (2), 169–177. doi:10.1007/s13318-018-0517-3
Rivera, F., Costa, G., Abin, A., Urbanavicius, J., Arruti, C., Casanova, G., et al. (2008). Reduction of Ischemic Brain Damage and Increase of Glutathione by a Liposomal Preparation of Quercetin in Permanent Focal Ischemia in Rats. Neurotox. Res. 13 (2), 105–114. doi:10.1007/bf03033562
Rojas-Garbanzo, C., Rodríguez, L., Pérez, A. M., Mayorga-Gross, A. L., Vásquez-Chaves, V., Fuentes, E., et al. (2021). Anti-platelet Activity and Chemical Characterization by UPLC-DAD-ESI-QTOF-MS of the Main Polyphenols in Extracts from Psidium Leaves and Fruits. Food Res. Int. 141, 110070. doi:10.1016/j.foodres.2020.110070
Rosell, A., Cuadrado, E., Ortega-Aznar, A., Hernández-Guillamon, M., Lo, E. H., and Montaner, J. (2008). MMP-9-positive Neutrophil Infiltration Is Associated to Blood-Brain Barrier Breakdown and Basal Lamina Type IV Collagen Degradation during Hemorrhagic Transformation after Human Ischemic Stroke. Stroke 39 (4), 1121–1126. doi:10.1161/strokeaha.107.500868
Russo, M., Palumbo, R., Mupo, A., Tosto, M., Iacomino, G., Scognamiglio, A., et al. (2003). Flavonoid Quercetin Sensitizes a CD95-Resistant Cell Line to Apoptosis by Activating Protein Kinase Calpha. Oncogene 22 (21), 3330–3342. doi:10.1038/sj.onc.1206493
Rutkowska, M., Kolodziejczyk-Czepas, J., Owczarek, A., Zakrzewska, A., Magiera, A., and Olszewska, M. A. (2021). Novel Insight into Biological Activity and Phytochemical Composition of Sorbus Aucuparia L. Fruits: Fractionated Extracts as Inhibitors of Protein Glycation and Oxidative/nitrative Damage of Human Plasma Components. Food Res. Int. 147, 110526. doi:10.1016/j.foodres.2021.110526
Sánchez, M., Galisteo, M., Vera, R., Villar, I. C., Zarzuelo, A., Tamargo, J., et al. (2006). Quercetin Downregulates NADPH Oxidase, Increases eNOS Activity and Prevents Endothelial Dysfunction in Spontaneously Hypertensive Rats. J. Hypertens. 24 (1), 75–84. doi:10.1097/01.hjh.0000198029.22472.d9
Sanchez, M., Lodi, F., Vera, R., Villar, I. C., Cogolludo, A., Jimenez, R., et al. (2007). Quercetin and Isorhamnetin Prevent Endothelial Dysfunction, Superoxide Production, and Overexpression of P47phox Induced by Angiotensin II in Rat Aorta. J. Nutr. 137 (4), 910–915. doi:10.1093/jn/137.4.910
Schrottmaier, W. C., Mussbacher, M., Salzmann, M., and Assinger, A. (2020). Platelet-leukocyte Interplay during Vascular Disease. Atherosclerosis 307, 109–120. doi:10.1016/j.atherosclerosis.2020.04.018
Schuhmann, M. K., Stoll, G., Bieber, M., Vögtle, T., Hofmann, S., Klaus, V., et al. (2020). CD84 Links T Cell and Platelet Activity in Cerebral Thrombo-Inflammation in Acute Stroke. Circ. Res. 127 (8), 1023–1035. doi:10.1161/circresaha.120.316655
Seiler, A., Schneider, M., Förster, H., Roth, S., Wirth, E. K., Culmsee, C., et al. (2008). Glutathione Peroxidase 4 Senses and Translates Oxidative Stress into 12/15-lipoxygenase Dependent- and AIF-Mediated Cell Death. Cell Metab. 8 (3), 237–248. doi:10.1016/j.cmet.2008.07.005
Shabbir, U., Rubab, M., Daliri, E. B.-M., Chelliah, R., Javed, A., and Oh, D.-H. (2021). Curcumin, Quercetin, Catechins and Metabolic Diseases: The Role of Gut Microbiota. Nutrients 13 (1), 206. doi:10.3390/nu13010206
Shah, F. A., Park, D. J., and Koh, P. O. (2018). Identification of Proteins Differentially Expressed by Quercetin Treatment in a Middle Cerebral Artery Occlusion Model: A Proteomics Approach. Neurochem. Res. 43 (8), 1608–1623. doi:10.1007/s11064-018-2576-x
Shalavadi, M. H., Chandrashekhar, V. M., and Muchchandi, I. S. (2020). Neuroprotective Effect of Convolvulus Pluricaulis Choisy in Oxidative Stress Model of Cerebral Ischemia Reperfusion Injury and Assessment of MAP2 in Rats. J. Ethnopharmacol. 249, 112393. doi:10.1016/j.jep.2019.112393
Sharifi-Rad, J., Quispe, C., Shaheen, S., El Haouari, M., Azzini, E., Butnariu, M., et al. (2021). Flavonoids as Potential Anti-Platelet Aggregation Agents: From Biochemistry to Health Promoting Abilities. Crit. Rev. Food Sci. Nutr. [Epub ahead of print], 1–14. doi:10.1080/10408398.2021.1924612
Shen, P., Lin, W., Deng, X., Ba, X., Han, L., Chen, Z., et al. (2021). Potential Implications of Quercetin in Autoimmune Diseases. Front. Immunol. 12, 689044. doi:10.3389/fimmu.2021.689044
South, K., Saleh, O., Lemarchand, E., Coutts, G., Smith, C. J., Schiessl, I., et al. (2022). Robust Thrombolytic and Anti-inflammatory Action of a Constitutively Active ADAMTS13 Variant in Murine Stroke Models. Blood 139 (10), 1575–1587. doi:10.1182/blood.2021012787
Stopa, J. D., Neuberg, D., Puligandla, M., Furie, B., Flaumenhaft, R., and Zwicker, J. I. (2017). Protein Disulfide Isomerase Inhibition Blocks Thrombin Generation in Humans by Interfering with Platelet Factor V Activation. JCI Insight 2 (1), e89373. doi:10.1172/jci.insight.89373
Stumvoll, M., Goldstein, B. J., and van Haeften, T. W. (2005). Type 2 Diabetes: Principles of Pathogenesis and Therapy. Lancet 365 (9467), 1333–1346. doi:10.1016/s0140-6736(05)61032-x
Sumi, M., Tateishi, N., Shibata, H., Ohki, T., and Sata, M. (2013). Quercetin Glucosides Promote Ischemia-Induced Angiogenesis, but Do Not Promote Tumor Growth. Life Sci. 93 (22), 814–819. doi:10.1016/j.lfs.2013.09.005
Sun, M. S., Jin, H., Sun, X., Huang, S., Zhang, F. L., Guo, Z. N., et al. (2018). Free Radical Damage in Ischemia-Reperfusion Injury: An Obstacle in Acute Ischemic Stroke after Revascularization Therapy. Oxid. Med. Cell Longev. 2018, 3804979. doi:10.1155/2018/3804979
Suri, S., Taylor, M. A., Verity, A., Tribolo, S., Needs, P. W., Kroon, P. A., et al. (2008). A Comparative Study of the Effects of Quercetin and its Glucuronide and Sulfate Metabolites on Human Neutrophil Function In Vitro. Biochem. Pharmacol. 76 (5), 645–653. doi:10.1016/j.bcp.2008.06.010
Tuo, Q. z., Zhang, S. t., and Lei, P. (2021). Mechanisms of Neuronal Cell Death in Ischemic Stroke and Their Therapeutic Implications. Med. Res. Rev. 42, 259–305. doi:10.1002/med.21817
Ulya, T., Ardianto, C., Anggreini, P., Budiatin, A. S., Setyawan, D., and Khotib, J. (2021). Quercetin Promotes Behavioral Recovery and Biomolecular Changes of Melanocortin-4 Receptor in Mice with Ischemic Stroke. J. Basic Clin. Physiol. Pharmacol. 32 (4), 349–355. doi:10.1515/jbcpp-2020-0490
Vek, V., Keržič, E., Poljanšek, I., Eklund, P., Humar, M., and Oven, P. (2021). Wood Extractives of Silver Fir and Their Antioxidant and Antifungal Properties. Molecules 26 (21), 6412. doi:10.3390/molecules26216412
Vekic, J., Zeljkovic, A., Stefanovic, A., Jelic-Ivanovic, Z., and Spasojevic-Kalimanovska, V. (2019). Obesity and Dyslipidemia. Metabolism 92, 71–81. doi:10.1016/j.metabol.2018.11.005
Verhenne, S., Denorme, F., Libbrecht, S., Vandenbulcke, A., Pareyn, I., Deckmyn, H., et al. (2015). Platelet-derived VWF Is Not Essential for Normal Thrombosis and Hemostasis but Fosters Ischemic Stroke Injury in Mice. Blood 126 (14), 1715–1722. doi:10.1182/blood-2015-03-632901
Viswanatha, G. L., Venkataranganna, M. V., Prasad, N. B. L., and Hanumanthappa, S. (2018). Chemical Characterization and Cerebroprotective Effect of Methanolic Root Extract of Colebrookea Oppositifolia in Rats. J. Ethnopharmacol. 223, 63–75. doi:10.1016/j.jep.2018.05.009
Viswanatha, G. L., Venkataranganna, M. V., Prasad, N. B. L., and Shylaja, H. (2019). Achyranthes aspera Linn. Alleviates Cerebral Ischemia-Reperfusion-Induced Neurocognitive, Biochemical, Morphological and Histological Alterations in Wistar Rats. J. Ethnopharmacol. 228, 58–69. doi:10.1016/j.jep.2018.09.018
Wang, J., Wang, P., Li, S., Wang, S., Li, Y., Liang, N., et al. (2014). Mdivi-1 Prevents Apoptosis Induced by Ischemia-Reperfusion Injury in Primary Hippocampal Cells via Inhibition of Reactive Oxygen Species-Activated Mitochondrial Pathway. J. Stroke Cerebrovasc. Dis. 23 (6), 1491–1499. doi:10.1016/j.jstrokecerebrovasdis.2013.12.021
Wang, J., Xing, H., Wan, L., Jiang, X., Wang, C., and Wu, Y. (2018). Treatment Targets for M2 Microglia Polarization in Ischemic Stroke. Biomed. Pharmacother. 105, 518–525. doi:10.1016/j.biopha.2018.05.143
Wang, K., Liu, R., Li, J., Mao, J., Lei, Y., Wu, J., et al. (2011). Quercetin Induces Protective Autophagy in Gastric Cancer Cells: Involvement of Akt-mTOR- and Hypoxia-Induced Factor 1α-Mediated Signaling. Autophagy 7 (9), 966–978. doi:10.4161/auto.7.9.15863
Wang, Q., Tang, X. N., and Yenari, M. A. (2007). The Inflammatory Response in Stroke. J. Neuroimmunol. 184, 53–68. doi:10.1016/j.jneuroim.2006.11.014
Wang, Y. Y., Chang, C. Y., Lin, S. Y., Wang, J. D., Wu, C. C., Chen, W. Y., et al. (2020). Quercetin Protects against Cerebral Ischemia/reperfusion and Oxygen Glucose Deprivation/reoxygenation Neurotoxicity. J. Nutr. Biochem. 83, 108436. doi:10.1016/j.jnutbio.2020.108436
Williams, R. J., Spencer, J. P., and Rice-Evans, C. (2004). Flavonoids: Antioxidants or Signalling Molecules? Free Radic. Biol. Med. 36 (7), 838–849. doi:10.1016/j.freeradbiomed.2004.01.001
Wright, B., Moraes, L. A., Kemp, C. F., Mullen, W., Crozier, A., Lovegrove, J. A., et al. (2010). A Structural Basis for the Inhibition of Collagen-Stimulated Platelet Function by Quercetin and Structurally Related Flavonoids. Br. J. Pharmacol. 159 (6), 1312–1325. doi:10.1111/j.1476-5381.2009.00632.x
Wu, L., Zhang, Q., Dai, W., Li, S., Feng, J., Li, J., et al. (20172017). Quercetin Pretreatment Attenuates Hepatic Ischemia Reperfusion-Induced Apoptosis and Autophagy by Inhibiting ERK/NF-κB Pathway. Gastroenterol. Res. Pract. 2017, 9724217. doi:10.1155/2017/9724217
Wu, M., Liu, F., and Guo, Q. (2019). Quercetin Attenuates Hypoxia-Ischemia Induced Brain Injury in Neonatal Rats by Inhibiting TLR4/NF-Κb Signaling Pathway. Int. Immunopharmacol. 74, 105704. doi:10.1016/j.intimp.2019.105704
Yamagata, K. (2019). Polyphenols Regulate Endothelial Functions and Reduce the Risk of Cardiovascular Disease. Curr. Pharm. Des. 25 (22), 2443–2458. doi:10.2174/1381612825666190722100504
Yang, D., Wang, T., Long, M., and Li, P. (2020a). Quercetin: Its Main Pharmacological Activity and Potential Application in Clinical Medicine. Oxid. Med. Cell Longev. 2020, 8825387. doi:10.1155/2020/8825387
Yang, J., Wu, Z., Long, Q., Huang, J., Hong, T., Liu, W., et al. (2020b). Insights Into Immunothrombosis: The Interplay Among Neutrophil Extracellular Trap, von Willebrand Factor, and ADAMTS13. Front. Immunol. 11, 610696. doi:10.3389/fimmu.2020.610696
Yang, R., Shen, Y.-J., Chen, M., Zhao, J.-Y., Chen, S.-H., Zhang, W., et al. (2021). Quercetin Attenuates Ischemia Reperfusion Injury by Protecting the Blood-Brain Barrier through Sirt1 in MCAO Rats. J. Asian Nat. Prod. Res. 24, 278–289. doi:10.1080/10286020.2021.1949302
Yang, W. S., SriRamaratnam, R., Welsch, M. E., Shimada, K., Skouta, R., Viswanathan, V. S., et al. (2014). Regulation of Ferroptotic Cancer Cell Death by GPX4. Cell 156, 317–331. doi:10.1016/j.cell.2013.12.010
Yousefi-Manesh, H., Dehpour, A. R., Nabavi, S. M., Khayatkashani, M., Asgardoon, M. H., Derakhshan, M. H., et al. (2021). Therapeutic Effects of Hydroalcoholic Extracts from the Ancient Apple Mela Rosa dei Monti Sibillini in Transient Global Ischemia in Rats. Pharmaceuticals 14 (11), 1106. doi:10.3390/ph14111106
Yu, P. X., Zhou, Q. J., Zhu, W. W., Wu, Y. H., Wu, L. C., Lin, X., et al. (2013). Effects of Quercetin on LPS-Induced Disseminated Intravascular Coagulation (DIC) in Rabbits. Thromb. Res. 131 (6), e270–3. doi:10.1016/j.thromres.2013.03.002
Yuan, K., Zhu, Q., Lu, Q., Jiang, H., Zhu, M., Li, X., et al. (2020). Quercetin Alleviates Rheumatoid Arthritis by Inhibiting Neutrophil Inflammatory Activities. J. Nutr. Biochem. 84, 108454. doi:10.1016/j.jnutbio.2020.108454
Zaragozá, C., Monserrat, J., Mantecón, C., Villaescusa, L., Álvarez-Mon, M. Á., Zaragozá, F., et al. (2021). Binding and Antiplatelet Activity of Quercetin, Rutin, Diosmetin, and Diosmin Flavonoids. Biomed. Pharmacother. 141, 111867. doi:10.1016/j.biopha.2021.111867
Zhang, L. L., Zhang, H. T., Cai, Y. Q., Han, Y. J., Yao, F., Yuan, Z. H., et al. (2016). Anti-inflammatory Effect of Mesenchymal Stromal Cell Transplantation and Quercetin Treatment in a Rat Model of Experimental Cerebral Ischemia. Cell Mol. Neurobiol. 36 (7), 1023–1034. doi:10.1007/s10571-015-0291-6
Zhang, X., Yan, H., Yuan, Y., Gao, J., Shen, Z., Cheng, Y., et al. (2013). Cerebral Ischemia-Reperfusion-Induced Autophagy Protects against Neuronal Injury by Mitochondrial Clearance. Autophagy 9 (9), 1321–1333. doi:10.4161/auto.25132
Zhi, K., Li, M., Bai, J., Wu, Y., Zhou, S., Zhang, X., et al. (2016). Quercitrin Treatment Protects Endothelial Progenitor Cells from Oxidative Damage via Inducing Autophagy through Extracellular Signal-Regulated Kinase. Angiogenesis 19 (3), 311–324. doi:10.1007/s10456-016-9504-y
Zhou, M., Zhu, L., Cui, X., Feng, L., Zhao, X., He, S., et al. (2016). Influence of Diet on Leukocyte Telomere Length, Markers of Inflammation and Oxidative Stress in Individuals with Varied Glucose Tolerance: a Chinese Population Study. Nutr. J. 15, 39. doi:10.1186/s12937-016-0157-x
Zou, H., Ye, H., Kamaraj, R., Zhang, T., Zhang, J., and Pavek, P. (2021). A Review on Pharmacological Activities and Synergistic Effect of Quercetin with Small Molecule Agents. Phytomedicine 92, 153736. doi:10.1016/j.phymed.2021.153736
Keywords: quercetin, ischemic stroke, inflammatory thrombus, neuroprotection, immune cell activation, mechanism
Citation: Zhang L, Ma J, Yang F, Li S, Ma W, Chang X and Yang L (2022) Neuroprotective Effects of Quercetin on Ischemic Stroke: A Literature Review. Front. Pharmacol. 13:854249. doi: 10.3389/fphar.2022.854249
Received: 13 January 2022; Accepted: 28 April 2022;
Published: 18 May 2022.
Edited by:
Yongjun Sun, Hebei University of Science and Technology, ChinaReviewed by:
Pallavi Shrivastava, Louisiana State University, United StatesPeng Sun, Shandong University of Traditional Chinese Medicine, China
Gollapalle L. Viswanatha, Independent Consultant, Bengaluru, India
Copyright © 2022 Zhang, Ma, Yang, Li, Ma, Chang and Yang. This is an open-access article distributed under the terms of the Creative Commons Attribution License (CC BY). The use, distribution or reproduction in other forums is permitted, provided the original author(s) and the copyright owner(s) are credited and that the original publication in this journal is cited, in accordance with accepted academic practice. No use, distribution or reproduction is permitted which does not comply with these terms.
*Correspondence: Xiang Chang, 3590150795@qq.com; Lin Yang, yang_lin2005@126.com
†These authors have contributed equally to this work