- 1Houston Methodist Cancer Center, Houston Methodist Hospital, Houston, TX, United States
- 2Cockrell Center for Advanced Therapeutic Phase I Program, Houston Methodist Research Institute, Houston, TX, United States
- 3Weill Cornell Medical College, Institute of Academic Medicine, Houston, TX, United States
- 4Houston Methodist Research Institute, Houston, TX, United States
- 5Faculty of Medicine and Health Sciences, University of Science and Technology, Sanaa, Yemen
- 6Faculty of Medicine, Xavier University School of Medicine Aruba, Oranjestad, Aruba
- 7Faculty of Medicine, University of Tripoli, Tripoli, Libya
- 8Faculty of Medicine, University of Jordan, Amman, Jordan
- 9Department of Biology and Chemistry, Buffalo State College, Buffalo, NY, United States
- 10Department of Medical Oncology, The University of Texas MD Anderson Cancer Center, Houston, TX, United States
The COVID-19 pandemic has impacted every country in the world. With more than 400 million cases and more than 5.5 million deaths. The FDA either approved or authorized the emergency use for three vaccines against COVID-19. The treatment options of COVID-19 are very limited. Multiple complementary and alternative medicine modalities were suggested to be efficacious in the treatment of COVID-19 such as Thymoquinone. The effects of Thymoquinone have been examined and multiple studies indicate a promising beneficial effect. However, the current body of research is limited in terms of its scope, quality, and quantity. While higher-quality studies are required, physicians do not routinely recommend the use of marketed supplements of natural products, including Thymoquinone for COVID-19. Given the numerous suggested positive effects of Thymoquinone, including anti-inflammatory and antimicrobial properties, additional research is required to confirm or refute these promising benefits. Complementary and alternative medicine is an area that requires additional evidence-based practice and research to confirm effects observed in clinical practice.
Introduction
The COVID-19 pandemic has impacted almost every country in the world. With more than 400 million cases and around 5.5 million deaths, finding a treatment is a priority (World Health Organization, 2021a). However, the necessity of finding a treatment has led to the adoption of non-evidence-based practices. Hydroxychloroquine was one of the first medications to be proposed as a possible treatment for COVID-19. Additionally, multiple complementary and alternative medicine strategies have been suggested as possible treatments of COVID-19 (Ang et al., 2020; Badakhsh et al., 2021).
In addition to the recently discovered COVID-19, six unique strains of human coronaviruses have been identified (Elfiky, 2020; Hui et al., 2020). Coronaviruses are around 30 kb enclosed, positive-sense single-stranded RNA viruses. They infect a wide variety of hosts (Channappanavar et al., 2014). Coronaviruses are classified into four genera based on their genetic structure: α, β, γ, and δ. Only mammals are infected by the α and β coronaviruses (Rabi et al., 2020). The common cold and croup are caused by human coronaviruses such as 229E and NL63, which belong to the alpha coronavirus family. β Coronaviruses, on the other hand, include SARS-CoV, OC43, Middle East respiratory syndrome coronavirus (MERS-CoV), and SARS-CoV-2 are the most dangerous and are responsible for roughly 800 fatalities each year. According to the WHO, SARS HCoV has a 10% fatality rate, whereas MERS HCoV has a 36% mortality rate (World Health Organization, 2021b).
Since the emergence of COVID, physicians and researchers have struggled to effectively treat the novel coronavirus. More recently medications of varying levels of effectivity have been implicated in the treatment of COVID 19. These include steroids, antiviral drug Remdesivir (RDV), and monoclonal antibody (mAb) (Beigel et al., 2020; Deb et al., 2021). The mAbs are thought to help reduce the viral load by blocking virus entrance into cells by binding to viral spikes and therefore preventing virus attachment to cell surface receptors (Deb et al., 2021). The mAbs may potentially target host cell receptors or co-receptors, rendering the host cells’ binding sites inaccessible to SARS-CoV-2. Alternatively, mAbs can act as immunosuppressive agents, limiting immune-mediated damage (Deb et al., 2021). Most recently, Molnupiravir as early treatment has shown a reduced risk of hospitalization or death in at-risk, unvaccinated adults with Covid-19 (Jayk Bernal et al., 2021). furthermore, Pfizer released phase 2/3 results from the PAXLOVID trial, confirming the novel COVID-19 oral antiviral treatment’s robust efficacy in reducing the risk of hospitalization or death by 89% (within 3 days of symptom onset) and 88% (within 5 days of symptom onset) compared to placebo; no deaths in non-hospitalized, high-risk adults with COVID-19 compared to placebo (Archive, 2021), in addition, PAXLOVID has been authorized by the United States Food and Drug Administration (Ashraf et al., 2021) as emergency use authorization and became the first oral antiviral authorized by FDA for treatment of COVID-19 (U.S. Food and Drug Administration, 2021a).
Three COVID-19 vaccines, Pfizer-BioNTech, Moderna, and Johnson & Johnson’s Janssen, were either approved or authorized for emergency use by the FDA (U.S. Food and Drug Administration, 2021b; U.S. Food and Drug Administration, 2021c; U.S. Food and Drug Administration, 2021d). The vaccines were effective in 90% of people regardless of age, gender, and underlying health issues. Furthermore, effectiveness was demonstrated in a subsequent analysis that included people with and without evidence of past SARS-CoV-2 infections (Oliver et al., 2020). Discomfort at the injection site, muscle pain, chills, joint pain, fatigue, headache, and fever were described as common adverse events of the COVID19 vaccinations (Centers for Disease Control and Prevention, 2021a; Abdelrahim and Esmail, 2021). Adverse effects following the second dose included pain at the injection site, muscle pain, chills, joint pain, tiredness, headache, and fever often lasted several days more than the first dose (Abdelrahim and Esmail, 2021; U.S. Food and Drug Administration, 2021e).
Multiple complementary and alternative medicine modalities where there suggested to be efficacious in the treatment of COVID-19. Suggested treatment options in the scientific literature include Thymoquinone and its natural source Nigella sativa (Bouchentouf and Noureddine, 2020). Thymoquinone is a component of many plants, with Nigella sativa being its primary natural source. Nigella sativa is used complementary or alternative medicine for its many proposed effects by different cultures and traditions (Goyal et al., 2017). The molecular formula of Thymoquinone is C10H12O2, whereas it is C18H28ClN3O5S for Hydroxychloroquine sulfate. Thus, it is unlikely for both chemical structures to have similar effects Figure1. Table 1 shows the different plants that contain Thymoquinone. Thymoquinone was found to have possible effects on certain biological functions (Khader and Eckl, 2014) and this led to some interest in studying the anti-microbial properties of Thymoquinone (Forouzanfar et al., 2014). Studies on the anti-viral effects of Thymoquinone are limited in literature; however, multiple in-vitro and in-vivo studies suggest some therapeutic potential. Moreover, Salim and Nour (Bouchentouf and Noureddine, 2020) have recently demonstrated that compounds other than Thymoquinone within the Nigella sativa plant may play a role in targeting COVID-19.
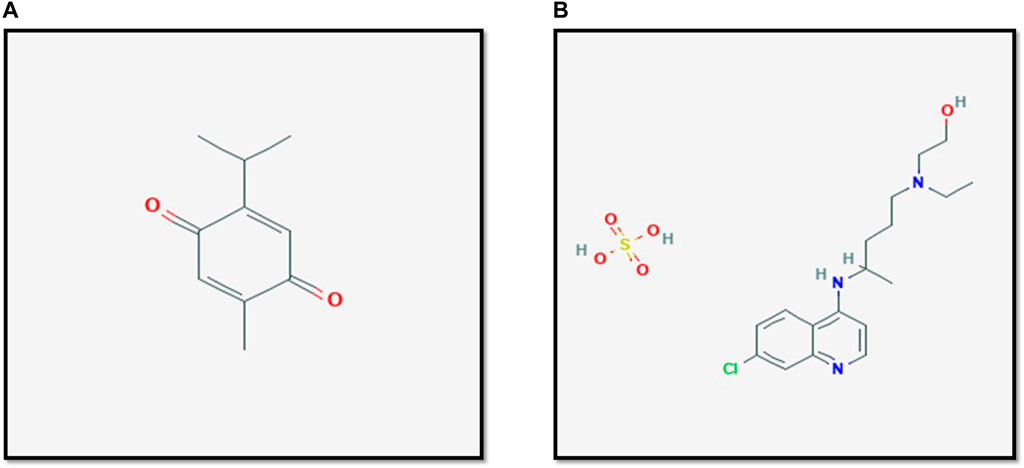
FIGURE 1. The structure of Thymoqunione (A) and Hydroxychloroquine (B). The molecular formula of Hydroxychloroquine sulfate is C18H28ClN3O5S, whereas it is C10H12O2 for Thymoquinone. Thus, it is unlikely for both chemical structures to have similar effects. Figures are adapted from PubChem (National. Center for Biotechnology Information, 2021a; National. Center for Biotechnology Information, 2021b).
This review is a timely review of Thymoquinone’s properties as an anti-viral agent. Similar reports are needed to keep the medical community updated regarding the efficacy of various alternative medicines so that medical professionals can inform and educate patients. This review aims to illustrate the role Thymoquinone effect in the immunological response to COVID-19 and other viral infections. In addition, we hope to shed the light on the potential drug development and the clinical utility of Thymoquinone to treat COVID-19 patients which is an era of unmet need for the time being.
Coronavirus Overview
In the downstream areas of Open Reading Frame 1 (ORF 1), all coronaviruses have particular genes that encode proteins for viral replication, nucleocapsid development, and spike creation (Elfiky, 2020). The glycoprotein spikes on coronaviruses’ outer surface are essential for the virus’s attachment and penetration into host cells. The MERS-coronavirus requires dipeptidyl peptidase 4 (DPP4), whereas the HCoV-NL63 and SARS-coronaviruses require angiotensin-converting enzyme 2 (ACE2) as a major receptor (Gralinski and Menachery, 2020). The cell-surface Heat Shock Protein A5 (HSPA5), also known as GRP78 or BiP, has been found to be identified by the viral spike proteins of SARS-Cov-2 (Datau et al., 2010). SARS-CoV-2 employs the same ACE2 cell receptor and method for entrance into host cells as SARS-CoV, these details were validated in a fluorescence experiments (Gralinski and Menachery, 2020; Shereen et al., 2020; Xu et al., 2020).
Attachment, penetration, biosynthesis, maturity, and release are the five phases in a virus’s life cycle within the host. Viruses enter host cells by endocytosis or membrane fusion after binding to host receptors (attachment) (penetration). The components of the virus are subsequently released into the host cells, and viral RNA is taken into the nucleus for replication. Viral proteins are made from viral mRNA (biosynthesis). Finally, new virus particles (maturation) are produced and discharged. Spike (S), membrane (M), envelop (E), and nucleocapsid (N) are the four structural proteins found in Coronaviruses (N) (Walls et al., 2020). The spike protein is a transmembrane trimetric glycoprotein that protrudes from the viral surface and controls coronavirus diversification and host tropism. Spike proteins are made up of two functional subunits: the S1 subunit is in charge of binding to the host cell receptor, and the S2 subunit is in charge of fusing the viral and cellular membranes. The ACE2 receptor has already been identified as a functioning SARS-CoV receptor (Li et al., 2003). The spike protein for SARS-CoV-2 interacts with ACE2 according to structural and functional investigations (Chen et al., 2020; Letko et al., 2020; Walls et al., 2020). ACE2 is highly expressed in the lungs, heart, ileum, kidneys, and bladder (Zou et al., 2020). ACE2 is a highly expressed epithelial cell of the lungs. Following SARS-attachment CoV-2’s to the host protein, the spike protein is cleaved by proteases. The S1 and S2 subunits remain non-covalently linked after cleavage at the S1/S2 cleavage site, and the distal S1 subunit aids in the prefusion stabilization of the membrane-anchored S2 subunit (Walls et al., 2020). Following cleavage at the S2 site, the spike protein is probably activated for membrane fusion with irreversible conformational changes.
Antigen presentation by dendritic cells (DCs) and macrophages trigger T cell responses against coronaviruses. DCs and macrophages can phagocytize virus-infected apoptotic cells (Fujimoto et al., 2000). DCs and macrophages, for example, can phagocytize virus-infected apoptotic epithelial cells, resulting in antigen presentation to T cells. In addition to ACE2, SARS-CoV may bind to dendritic cell-specific intercellular adhesion molecule-3-grabbing nonintegrin (DC-SIGN) and DC-SIGNR (L-SIGN) and DC-SIGNR-related protein (DC-SIGNR, L-SIGN) (Jeffers et al., 2004; Marzi et al., 2004; Yang et al., 2004). Dendritic cells and macrophages both express high numbers of DC-SIGN. To present viral antigens to T cells, these antigen-presenting cells go to the draining lymph nodes. T lymphocytes, both CD4+ and CD8+, play an important function. CD4+ T cells stimulate B cells to produce virus-specific antibodies, whereas CD8+ T cells destroy virally infected cells. Regarding to coronavirus variants, the scientists track all variants, however, some are classified as variants to be monitored, variants of interest, variants of high consequence, and variants of concern such as Omicron–B.1.1.29 and Delta–B.1.617.2 (Centers for Disease Control and Prevention, 2021b; Karim and Karim, 2021). Some variants spread more easily and quickly than others, for example, the Omicron variant may spread more easily than other variants, including Delta (Centers for Disease Control and Prevention, 2021a). These classifications are based on the ease with which the variant spreads, the severity of the symptoms, how well the variant responds to treatments, and how well immunizations protect against the variant.
Scientific Report
Several synthetic compounds initially thought to have shown promise in COVID-19 therapy, including hydroxychloroquine and chloroquine phosphate (Cortegiani et al., 2020; Gao et al., 2020) and newer antiviral drugs like lopinavir (Yao et al., 2020), have subsequently been shown to have little or no effect on hospitalized COVID- 19 patients, as indicated by overall mortality, initiation of ventilation and duration of hospital stay (Pan et al., 2021). On the other hand, Remdesivir (Holshue et al., 2020; Wang et al., 2020) clinical data suggest efficacy in treating COVID-19 and is the first FDA-approved COVID-19 therapy (Lamb, 2020).
The creation of innovative antiviral medications may be driven by traditional herbal medicines and purified natural ingredients. For example, Emetine an isoquinoline alkaloid isolated from Cephaelis ipecacuanha is an effective amoebicidal drug. Similarly, the drug quinine is derived from Cinchona tree bark. Other common drugs derived from natural compounds include aspirin, morphine, and paclitaxel, an antineoplastic drug (Ganjhu et al., 2015). Between 1981 and 2014, half of all medications approved were derived from or resembled a natural component (Newman and Cragg, 2016).
According to scientific investigations, Nigella sativa (Family Ranunculaceae) is developing as a therapeutic plant with a wide range of pharmacological potential. Nigella sativa, often known as black seed, is native to Southern Europe, North Africa, and Southwest Asia. It is also cultivated in other regions of the world, including the Eastern Mediterranean and India (Khare, 2004). Nigella sativa is a commonly used medicinal herb in several traditional medical systems across the world, including Unani and Tibb, Ayurveda, and Siddha. The seeds and oil of the plant have a long history of use as both medicinal and sustenance (Ahmad et al., 2013). The star of this study, Thymoquinone is one such product derived from Nigella sativa. Thymoquinone has been investigated for its potential anti-inflammatory, anti-microbial, and anti-tumor effects (Beigel et al., 2020) (Bouchentouf and Noureddine, 2020). Most of these studies have been performed in vitro or animal-based models. However, very few studies have been able to establish clear clinical evidence of therapeutic effects.
Experiments demonstrate that Thymoquinone inhibits the growth of a variety of bacteria. Different extracts of Nigella sativa showed possible effects on multiple bacteria, including extracts that contained Thymoquinone alone. Chaieb et al. (2011) showed that Thymoquinone was effective against seven out of sixteen tested bacteria. These bacteria were mainly Gram-positive bacteria. Other studies have confirmed that Thymoquinone has the most potent effect on Gram-positive bacteria (Kokoska et al., 2008). Additionally, in-vivo studies using animal models have also suggested possible positive effects. In an acute pyelonephritis model, treatment with Thymoquinone (a dose of 10 mg/kg) was given before bacterial inoculation of E-Coli and Thymoquinone was also repeated every 24 h. Histological examination exhibited a reduction in oxidative damage and a nephron-protective effect due to Thymoquinone treatment (Evirgen et al., 2011).
The effects of Thymoquinone have also been studied in fungal infections. Both in vitro and in vivo studies have suggested that Thymoquinone may play a possible therapeutic role in the treatment of different fungal infections, such as dermatophytes and candida (Aljabre et al., 2005). Like in anti-bacterial studies, anti-fungal experiments also have inconsistencies in dosing, the type of extracts, as well as a lack of clinical proof.
In direction of antiviral effect, Thymoquinone’s antiviral effectiveness against various viral infections has been supported by several studies, according to its multiple positive benefits, including antioxidant, anti-inflammatory, and immunomodulatory properties, as well as the possibility of direct viral elimination, and these studies reported that the viral loads in the liver and spleen were dramatically reduced, which correlated with increased IFN- production and CD4 (+) T cell response (Forouzanfar et al., 2014; Sommer et al., 2020; Salem and Hossain, 2000). In addition, multiple studies have suggested the same concept of that Thymoquinone has antiviral properties specifically in regards to cytomegalovirus (CMV), human immunodeficiency virus (Archive, 2021), and influenza. In contrast to other anti-microbial studies, these reports included clinical outcomes which highlight the possible efficacy of Thymoquinone as a therapeutic agent for HIV (Onifade et al., 2015). Two patients who were ineligible for the highly active antiretroviral therapy (HAART) achieved seroconversion with Thymoquinone. One of the patients was a 27-year-old pregnant female, who achieved seroconversion and no vertical transmission. In another study, Thymoquinone was shown to decrease hyperinsulinemia associated with HAART therapy (Chandra et al., 2009).
These promising studies support the need for further investigation. Table 2 summarizes some selected examples of the antiviral effects of Thymoquinone.

TABLE 2. Selected examples of the anti-viral effects of Thymoquinone (TQ) and Nigella sativa extracts.
In addition to Thymoquinone, anti-viral effects of compounds found in the oils of the Nigella sativa plant have also been examined, as illustrated by in-vitro and animal studies of the murine CMV (Salem and Hossain, 2000). Thymoquinone treatment with or without curcumin led to reduced symptoms and viral shedding in animals infected by the H9N2 virus, a form of avian influenza that affects poultry and in more rare cases humans (Cortegiani et al., 2020). Other extracts have been shown to have probable effects in the treatment of hepatitis C (Barakat et al., 2013).
Interestingly, one study has demonstrated that extracts from multiple plants or their scientific extraction, including Thymoquinone (Nigella sativa), Anthemis hyalina, and Citrus sinensismay influence the outcomes of coronavirus infections. All three extracts showed possible therapeutic benefit, with Anthemis hyaline, having the most effect (Ulasli et al., 2014). A recent study (under pre-print review) has shown that compounds, other than Thymoquinone, extracted from Nigella sativa regulate molecular docking (Bouchentouf and Noureddine, 2020). Molecular docking is promising in silico method for screening diverse drugs for their antiviral potential by comparing their binding affinities to various viral or host cell receptor proteins. Various viral proteins involved in viral entry, such as spike proteins, and replication, such as viral proteases, are molecular targets of SARS-CoV-2 (Senger et al., 2020). Additionally, a double-blind randomized controlled trial discovered that Nigella sativa extracts reduced inflammatory cytokine response in a patient with rheumatoid arthritis (Hadi et al., 2016). These results are promising in the case of COVID-19 due to the fact that infected patients are in a state of chronic inflammation and at risk of developing cytokine release syndrome. These observations were suggestive of a potential role for Thymoquinone in the treatment of COVID-19 Figure 2.
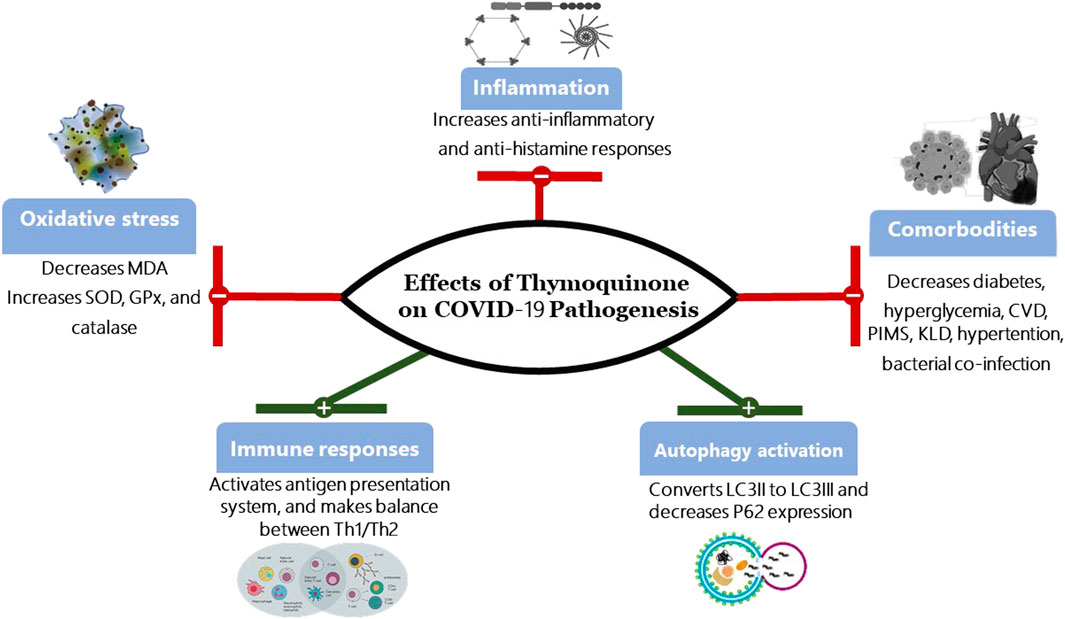
FIGURE 2. Effects of Thymoquinone on COVID-19 Pathogenesis. SOD, Superoxide dismutase; GPx, Glutathione peroxidase; MDA, Malonaldehyde Th1, type I helper T lymphocytes; Th2, type II helper T cells; CVD, Cardiovascular disease; PIMS, Paediatric Inflammatory Multisystem Syndrome; KLD, Kawasaki-like diseases; LC3, Microtubule-associated protein 1A/1B-light chain 3; P62, protein 62.
Abdel-Fattah et al. (2000) found that since Thymoquinone has antinociceptive effects by indirectly activating the supraspinal μ1-and κ-opioid receptor subtypes, it may prevent SARS-CoV-2 entrance into pneumocytes via ACE2. Multiple investigations have found that opioid receptors and ACE have overlapping inhibitory chemicals, for example, Rahman (2020) speculated that Thymoquinone might also block ACE2. Takai et al. (1996) additionally proposed that brain endogenous angiotensin II, by its antagonistic interaction with the endogenous opioid system, was implicated in central nociceptive pathways. Furthermore, Lantz et al. (1991) showed that opioid-active peptides, such as hemorphins, have an inhibitory effect on ACE. The above line of evidence suggests that opioid receptors and ACE share similar inhibitory molecules and as such, in publication, Rahman indicated that Thymoquinone may also block ACE2 (Rahman, 2020).
In a collaborative research project, Codex Bio Labs tested black seed oil and Thymoquinone for their effect on viral entry and viral protein translation using Codex’s Murine Leukemia Virus (MLV) particles pseudotyped (PP) with the SARS-CoV-2 Spike protein (unpublished data). Various combinations/concentrations of black seed oil and Thymoquinone were tested against SARS-CoV-2 MLV pseudovirus particles (pp) by assessing Luciferase activities measured with a Firefly Luciferase Assay Kit (CB- 80552-010, Codex BioSolutions Inc.). It was observed that Thymoquinone seemed to block viral infection. However, at high concentrations Thymoquinone caused cell death indicating cytotoxic effects. To confirm this result, cell growth assays were performed in the presence of Thymoquinone with Codex’s EnerCount cell growth assay kit which measures ATP levels inside the cells.
Similarly, in a seropositive HIV infected patient treated with Thymoquinone (10 ml twice/day for 6 months), Onifade et al. (2013) demonstrated a decrease in viral load to an undetectable level within 3 months, an increase in CD4 count, relief of symptoms, and a sustained sero-reversion following COVID-19 therapy. Another investigation on a seropositive HIV-infected woman who received Thymoquinone (Nigella sativa) and honey treatment (10 ml thrice/day for 1 year) demonstrated prolonged sero-reversion, which the author attributed to Thymoquinone’s possible virucidal effect (Onifade et al., 2015).
Akhtar and Riffat (1991) demonstrated the efficacy of a single oral administration of Thymoquinone (Nigella sativa) as powdered seeds and ethanolic extract (40 mg/kg body weight) in reducing the percentage of fecal eggs per Gram in children who were infected with cestodes.
In a study conducted on Hepatitis C (HCV) patients, Abdel-Moneim et al. were able to demonstrate that extracts of Nigella sativa (Thymoquinone) and Zingiber officinale, alone and together (500 mg of Nigella sativa and/or Zingiber officinale twice daily for 1 month), improved liver function and decreased viral load in the HCV patients (Onifade et al., 2013). Decreased viral load and improved liver function were similarly reported in another study by Barakat et al. (2013) where HCV patients received capsules of Nigella sativa oil (450 mg) three times a day over 3 months. Furthermore, Thymoquinone has been studied for benefits other than anti-inflammatory effects which are beyond the scope of this review (Mostofa et al., 2017; Mohammed and Islam, 2018; Shanmugam et al., 2018; Jehan et al., 2020; Leong et al., 2021; Salehi et al., 2021).
Thymoquinone Studies in Covid-19 Patients
Clinical Studies
In an investigator-initiated, 313 COVID-19 positive patients were divided into two groups: mild to moderate (cough, fever, sore throat, nasal congestion, malaise and/or shortness of breath) and severe (fever and/or cough along with pneumonia, severe dyspnea, respiratory distress, tachypnea (>30 breaths/min or hypoxia (SpO2 <90% on room air) however, this was conducted as open-label-placebo and randomized controlled trial, 210 and 103 patients were allocated to the mild/moderate and severe groups, respectively, using the clinical care criteria for COVID-19 implemented by Pakistan’s Ministry of National Health Services (Ashraf et al., 2020). Within each of the two groups, the patients were randomly allocated to the treatment group (which received honey + Thymoquinone (Nigella sativa) [HNS]) or the control group (which received no therapy) (receiving empty capsules). Honey (1 g) and Nigella sativa seeds (80 mg) per kg body weight were given orally in 2-3 split doses daily for up to 13 days in the HNS group, whereas the control group got a placebo (empty capsules). The primary outcomes were viral elimination (no RT-PCR for SARS-CoV-2 RNA), clinical symptom relief, and a reduction in Clinical Grading Score (CGS) on day 6. Fever decrease (day 4), C-Reactive protein CRP levels (day 6), the intensity of symptoms (day 8), CGS score (day 10), and death on day 30 were all secondary outcomes. HNS aided with symptom relief and viral clearance, as well as lowering mortality in individuals with moderate and severe illness, according to the findings. COVID-19 symptoms were shown to be relieved earlier in the HNS groups than in the control groups: 4 versus 7 days for moderate patients and 6 versus 13 days for severe disease patients. For both moderate and severe cases, viral elimination (being negative for the SARS-CoV-2 RT-PCR test) occurred 4 days sooner in the HNS group. On day 4, there was a considerable decrease in the severity of fever in the severe patients (OR: 0.21; 95% CI: 0.09–0.46; p = 0.0001). On day 6, C- reactive proteins (CRP) levels in both HNS groups reduced dramatically (p 0.0001) when compared to their respective control groups. On day 8, 98.13% of patients in HNS-treated mild cases were asymptomatic, compared to 56.31% in the control group (OR: 0.009; 95% CI: 0.001–0.08; p < 0.0001). More patients in the HNS group were asymptomatic in severe instances, whereas more in the control arm experienced mild symptoms (median) (OR: 0.1; 95% CI: 0.04–0.24). On day 10, 96.26% of moderate case-patients with HNS had fully resumed regular activities, compared to 68.93% in the control group (OR: 0.07; 95% CI: 0.02–0.21). The median CGS at day 10 for the severe group demonstrated that HNS treated patients returned to normal activities, whereas control patients remained hospitalized and required oxygen treatment (OR:0.05; 95% CI: 0.02–0.15). Morality after 30 days was 18.87% in the control group and 4% with HNS treatment (OR: 0.18 95% CI: 0.02–0.92) (Ashraf et al., 2020).
Non-Clinical Studies
A molecular docking and molecular dynamics stimulation study conducted by Elfiky (2021) tested the effect of natural products against the HSPA5 substrate-binding domain. The results showed that active components in cinnamon and seeds of Nigella sativa may tightly bind to cell-surface HSPA5 (one of the host cell receptors recognized by the viral spike protein) and could be successful in hindering SARS-CoV-2 spike recognition and attachment.
In an, in vitro model of rheumatoid arthritis, Vaillancourt et al. (2011) illustrated that Thymoquinone significantly decreases lipopolysaccharide (LPS) -induced proinflammatory cytokines such as interleukin1beta (IL-1β), tumor necrosis factor-alpha (TNF-α), metalloproteinase-13 (MMP-13), COX-2, and prostaglandin E2.
Similarly, Gholamnezhad et al. (2019) reported an anti-inflammatory effect of Thymoquinone in allergic lung inflammation. There was a Thymoquinone-associated decrease in IL-4, IL-5, and IL-13, but an increase in IFN-γ in BALF and lung homogenates.
As part of an in-vitro study, Cobourne-Duval et al. compared LPS/IFNγ-activated BV-2 microglial cells (immortalized murine microglial cell line) with and without Thymoquinone treatment in a quantitative proteomic study. The following inflammatory cytokines had considerably increased protein expression in LPS/IFNγ-activated BV-2 cells compared to controls: IL-2 (127%), IL-4 (151%), IL-6 (670%), IL-10 (133%), and IL-17a (127%). When comparing the protein expression levels of the same inflammatory cytokines in Thymoquinone treated LPS/IFNγ-activated cells to the protein expression levels in activated cells without Thymoquinone treatment, the protein expression levels in Thymoquinone treated LPS/IFN-activated cells were significantly reduced (p < 0.0001). IL-2, IL-4, IL-6, IL-10, and IL-17a levels were reduced by 38 percent, 19 percent, 83 percent, 23 percent, and 29 percent, respectively, when compared to controls (Cobourne-Duval et al., 2018). Additional findings of the study showed that Thymoquinone significantly inhibited the production of various inflammatory cytokines in LPS/IFNγ stimulated BV-2 microglial cells, displaying an inhibitory impact on the expression of several interleukins such as IL-2, IL-4, IL-6, IL-10, and IL-17a (Cobourne-Duval et al., 2018).
Discussion
In pre-clinical studies, Thymoquinone has been shown to possess anti-inflammatory properties as well as anti-corona virus properties by blocking viral entry. The acute and sub-acute toxicity of Thymoquinone has been examined in various in-vitro and in-vivo experiments. Thymoquinone/Nigella sativa has been studied extensively over many years and has been found to be relatively safe, with very few side effects despite the low level of toxicity that the seed extract and its constituent’s exhibit (Abukhader, 2012; Ong et al., 2016). Furthermore, Black seed (Black Cumin or Nigella sativa) has been categorized by the FDA under spices and other natural seasonings/flavorings that are generally recognized as safe for their intended use (409 of the Act Title 21, Chapter I, Subchapter B, Sec. 182.10 Spices and other natural seasonings and flavorings).
A review of the literature on the therapeutic uses of Thymoquinone/Nigella sativa shows some promising results but remains inconclusive. There is a scarcity of studies investigating clinical efficacy, especially at higher doses. Furthermore, any results derived from preclinical studies are confounded by the use of varied extracts thus introducing heterogeneity in the product being tested. More rigorous pre-clinical and clinical research studies need to be conducted before Thymoquinone/Nigella sativa can be routinely used as an effective complementary or alternative treatment.
In addition to efficacy, alternative medicine must also satisfy safety criteria. For instance, it is a misconception that these substances are always healthy, since, in addition to possible intrinsic adverse effects, marketed preparations may also have additives that can increase the risk of negative side effects. For example, Thymoquinone was found to inhibit CYP enzymes, particularly CYP29C, which may lead to possible interactions (Albassam et al., 2018).
Importantly, the regulatory processes governing complementary and alternative medicine preparations are not as strict as for other pharmaceuticals. The devastating health effects of the COVID-19 pandemic have led to the use of a variety of non-evidence-based treatments that are yet to be validated by large, randomized control trials.
Conclusion
Although multiple studies indicate promising beneficial effects of Thymoquinone in the treatment of various diseases, the current body of research is limited in terms of its scope, quality, and quantity. Physicians are discouraged from recommending the use of marketed supplements of natural products, including Thymoquinone, for COVID-19. Given the numerous suggested positive effects of Thymoquinone, including its anti-inflammatory, additional research is required to confirm these promising benefits or refute the suggested benefits.
Author Contributions
AE and MA wrote the first draft of the manuscript. All authors vouch for the accuracy and contents of the manuscript. All authors approved the final version of the draft.
Conflict of Interest
The authors declare that the research was conducted in the absence of any commercial or financial relationships that could be construed as a potential conflict of interest.
Publisher’s Note
All claims expressed in this article are solely those of the authors and do not necessarily represent those of their affiliated organizations or those of the publisher, the editors, and the reviewers. Any product that may be evaluated in this article, or claim that may be made by its manufacturer, is not guaranteed or endorsed by the publisher.
Acknowledgments
The assistance provided by Ibrahim N. Muhsen; MD in editing this manuscript and providing some comments to improve this review was greatly appreciated.
References
Abdel-Fattah, A. M., Matsumoto, K., and Watanabe, H. (2000). Antinociceptive Effects of Nigella Sativa Oil and its Major Component, Thymoquinone, in Mice. Eur. J. Pharmacol. 400 (1), 89–97. doi:10.1016/s0014-2999(00)00340-x
Abdelrahim, M., and Esmail, A. (2021). Regional Lymphadenopathy after COVID-19 Vaccine in a Cancer Patient: A Case Report. J. Surg. Res. 4 (4), 4. doi:10.26502/jsr.10020171
Abukhader, M. M. (2012). The Effect of Route of Administration in Thymoquinone Toxicity in Male and Female Rats. Indian J. Pharm. Sci. 74 (3), 195–200. doi:10.4103/0250-474X.106060
Ahmad, A., Husain, A., Mujeeb, M., Khan, S. A., Najmi, A. K., Siddique, N. A., et al. (2013). A Review on Therapeutic Potential of Nigella Sativa: A Miracle Herb. Asian Pac. J. Trop. Biomed. 3 (5), 337–352. doi:10.1016/S2221-1691(13)60075-1
Akhtar, M. S., and Riffat, S. (1991). Field Trial of Saussurea Lappa Roots against Nematodes and Nigella Sativa Seeds against Cestodes in Children. J. Pak Med. Assoc. 41 (8), 185–187.
Albassam, A. A., Ahad, A., Alsultan, A., and Al-Jenoobi, F. I. (2018). Inhibition of Cytochrome P450 Enzymes by Thymoquinone in Human Liver Microsomes. Saudi Pharm. J. 26 (5), 673–677. doi:10.1016/j.jsps.2018.02.024
Aljabre, S. H., Randhawa, M. A., Akhtar, N., Alakloby, O. M., Alqurashi, A. M., and Aldossary, A. (2005). Antidermatophyte Activity of Ether Extract of Nigella Sativa and its Active Principle, Thymoquinone. J. Ethnopharmacol 101 (1-3), 116–119. doi:10.1016/j.jep.2005.04.002
Ang, L., Song, E., Lee, H. W., and Lee, M. S. (2020). Herbal Medicine for the Treatment of Coronavirus Disease 2019 (COVID-19): A Systematic Review and Meta-Analysis of Randomized Controlled Trials. J. Clin. Med. 9 (5), 4–8. doi:10.3390/jcm9051583
Archive, P. R. (2021). Pfizer Announces Additional Phase 2/3 Study Results Confirming Robust Efficacy of Novel COVID-19 Oral Antiviral Treatment Candidate in Reducing Risk of Hospitalization or Death. Available from: https://www.pfizer.com/news/press-release/press-release-detail/pfizer-announces-additional-phase-23-study-results.
Ashraf, S., Ashraf, S., Akmal, R., Ashraf, M., Kalsoom, L., Maqsood, A., et al. (2021). Prophylactic Potential of Honey and Nigella Sativa L. Against Hospital and Community-Based SARS-CoV-2 Spread: A Structured Summary of a Study Protocol for a Randomised Controlled Trial. Trials 22 (1), 618. doi:10.1186/s13063-021-05510-3
Ashraf, S., Ashraf, S., Ashraf, M., Imran, M. A., Kalsoom, L., Siddiqui, U. N., et al. (2020). Honey and Nigella Sativa against COVID-19 in Pakistan (HNS-COVID-PK): A Multi-center Placebo-Controlled Randomized Clinical Trial. medRxiv 2020, 17–19. doi:10.1101/2020.10.30.20217364
Badakhsh, M., Dastras, M., Sarchahi, Z., Doostkami, M., Mir, A., and Bouya, S. (2021). Complementary and Alternative Medicine Therapies and COVID-19: a Systematic Review. Rev. Environ. Health 36 (3), 443–450. doi:10.1515/reveh-2021-0012
Barakat, E. M., El Wakeel, L. M., and Hagag, R. S. (2013). Effects of Nigella Sativa on Outcome of Hepatitis C in Egypt. World J. Gastroenterol. 19 (16), 2529–2536. doi:10.3748/wjg.v19.i16.2529
Beigel, J. H., Tomashek, K. M., Dodd, L. E., Mehta, A. K., Zingman, B. S., Kalil, A. C., et al. (2020). Remdesivir for the Treatment of Covid-19 - Final Report. N. Engl. J. Med. 383 (19), 1813–1826. doi:10.1056/NEJMoa2007764
Bouchentouf, M., and Noureddine, M. (2020). identification-of-compounds-from-nigella-sativa-as-new-potential-inhibitors-of-2019-novel-coronasvirus-covid-19-molecular-docking. Angiosperms.
Centers for Disease Control and Prevention (2021a). Pfizer-BioNTech COVID-19 Vaccine Overview and Safety 2021. Available from: https://www.cdc.gov/coronavirus/2019-ncov/vaccines/different-vaccines.html.
Centers for Disease Control and Prevention (2021b). Variants of the Virus,Omicron Variant: What You Need to Know. updated 2021. Available from: https://www.cdc.gov/coronavirus/2019-ncov/variants/about-variants.html.
Chaieb, K., Kouidhi, B., Jrah, H., Mahdouani, K., and Bakhrouf, A. (2011). Antibacterial Activity of Thymoquinone, an Active Principle of Nigella Sativa and its Potency to Prevent Bacterial Biofilm Formation. BMC Complement. Altern. Med. 11 (1), 29. doi:10.1186/1472-6882-11-29
Chandra, S., Murthy, S. N., Mondal, D., and Agrawal, K. C. (2009). Therapeutic Effects of Nigella Sativa on Chronic HAART-Induced Hyperinsulinemia in Rats. Can. J. Physiol. Pharmacol. 87 (4), 300–309. doi:10.1139/Y09-014
Channappanavar, R., Zhao, J., and Perlman, S. (2014). T Cell-Mediated Immune Response to Respiratory Coronaviruses. Immunol. Res. 59 (1-3), 118–128. doi:10.1007/s12026-014-8534-z
Chen, Y., Guo, Y., Pan, Y., and Zhao, Z. J. (2020). Structure Analysis of the Receptor Binding of 2019-nCoV. Biochem. Biophys. Res. Commun. 525 (1), 135–140. doi:10.1016/j.bbrc.2020.02.071
Cobourne-Duval, M. K., Taka, E., Mendonca, P., and Soliman, K. F. A. (2018). Thymoquinone Increases the Expression of Neuroprotective Proteins while Decreasing the Expression of Pro-inflammatory Cytokines and the Gene Expression NFκB Pathway Signaling Targets in LPS/IFNγ -activated BV-2 Microglia Cells. J. Neuroimmunol 320, 87–97. doi:10.1016/j.jneuroim.2018.04.018
Cortegiani, A., Ingoglia, G., Ippolito, M., Giarratano, A., and Einav, S. (2020). A Systematic Review on the Efficacy and Safety of Chloroquine for the Treatment of COVID-19. J. Crit. Care 57, 279–283. doi:10.1016/j.jcrc.2020.03.005
Datau, E. A., Wardhana, W., Surachmanto, E. E., Pandelaki, K., Langi, J. A., and Fias, F. (2010). Efficacy of Nigella Sativa on Serum Free Testosterone and Metabolic Disturbances in central Obese Male. Acta Med. Indones 42 (3), 130–134.
Deb, P., Molla, M. M. A., and Saif-Ur-Rahman, K. M. (2021). An Update to Monoclonal Antibody as Therapeutic Option against COVID-19. Biosaf Health 3 (2), 87–91. doi:10.1016/j.bsheal.2021.02.001
Elfiky, A. A. (2020). Anti-HCV, Nucleotide Inhibitors, Repurposing against COVID-19. Life Sci. 248, 117477. doi:10.1016/j.lfs.2020.117477
Elfiky, A. A. (2021). Natural Products May Interfere with SARS-CoV-2 Attachment to the Host Cell. J. Biomol. Struct. Dyn. 39 (9), 3194–3203. doi:10.1080/07391102.2020.1761881
Evirgen, O., Gökçe, A., Ozturk, O. H., Nacar, E., Onlen, Y., Ozer, B., et al. (2011). Effect of Thymoquinone on Oxidative Stress in Escherichia Coli-Induced Pyelonephritis in Rats. Curr. Ther. Res. Clin. Exp. 72 (5), 204–215. doi:10.1016/j.curtheres.2011.09.002
Forouzanfar, F., Bazzaz, B. S., and Hosseinzadeh, H. (2014). Black Cumin (Nigella Sativa) and its Constituent (Thymoquinone): a Review on Antimicrobial Effects. Iran J. Basic Med. Sci. 17 (12), 929–938.
Fujimoto, I., Pan, J., Takizawa, T., and Nakanishi, Y. (2000). Virus Clearance through Apoptosis-dependent Phagocytosis of Influenza A Virus-Infected Cells by Macrophages. J. Virol. 74 (7), 3399–3403. doi:10.1128/jvi.74.7.3399-3403.2000
Ganjhu, R. K., Mudgal, P. P., Maity, H., Dowarha, D., Devadiga, S., Nag, S., et al. (2015). Herbal Plants and Plant Preparations as Remedial Approach for Viral Diseases. Virusdisease 26 (4), 225–236. doi:10.1007/s13337-015-0276-6
Gao, J., Tian, Z., and Yang, X. (2020). Breakthrough: Chloroquine Phosphate Has Shown Apparent Efficacy in Treatment of COVID-19 Associated Pneumonia in Clinical Studies. Biosci. Trends 14 (1), 72–73. doi:10.5582/bst.2020.01047
Gholamnezhad, Z., Shakeri, F., Saadat, S., Ghorani, V., and Boskabady, M. H. (2019). Clinical and Experimental Effects of Nigella Sativa and its Constituents on Respiratory and Allergic Disorders. Avicenna J. Phytomed 9 (3), 195–212.
Goyal, S. N., Prajapati, C. P., Gore, P. R., Patil, C. R., Mahajan, U. B., Sharma, C., et al. (2017). Therapeutic Potential and Pharmaceutical Development of Thymoquinone: A Multitargeted Molecule of Natural Origin. Front. Pharmacol. 8, 656. doi:10.3389/fphar.2017.00656
Gralinski, L. E., and Menachery, V. D. (2020). Return of the Coronavirus: 2019-nCoV. Viruses 12 (2), 1-6. doi:10.3390/v12020135
Hadi, V., Kheirouri, S., Alizadeh, M., Khabbazi, A., and Hosseini, H. (2016). Effects of Nigella Sativa Oil Extract on Inflammatory Cytokine Response and Oxidative Stress Status in Patients with Rheumatoid Arthritis: a Randomized, Double-Blind, Placebo-Controlled Clinical Trial. Avicenna J. Phytomed 6 (1), 34–43.
Holshue, M. L., DeBolt, C., Lindquist, S., Lofy, K. H., Wiesman, J., Bruce, H., et al. (2020). First Case of 2019 Novel Coronavirus in the United States. N. Engl. J. Med. 382 (10), 929–936. doi:10.1056/NEJMoa2001191
Hui, D. S., I Azhar, E., Madani, T. A., Ntoumi, F., Kock, R., Dar, O., et al. (2020). The Continuing 2019-nCoV Epidemic Threat of Novel Coronaviruses to Global Health - the Latest 2019 Novel Coronavirus Outbreak in Wuhan, China. Int. J. Infect. Dis. 91, 264–266. doi:10.1016/j.ijid.2020.01.009
Jayk Bernal, A., Gomes da Silva, M. M., Musungaie, D. B., Kovalchuk, E., Gonzalez, A., Delos Reyes, V., et al. (2021). Molnupiravir for Oral Treatment of Covid-19 in Nonhospitalized Patients. New Engl. J. Med. 386 (6), 4–9. doi:10.1056/NEJMoa2116044
Jeffers, S. A., Tusell, S. M., Gillim-Ross, L., Hemmila, E. M., Achenbach, J. E., Babcock, G. J., et al. (2004). CD209L (L-SIGN) Is a Receptor for Severe Acute Respiratory Syndrome Coronavirus. Proc. Natl. Acad. Sci. U S A. 101 (44), 15748–15753. doi:10.1073/pnas.0403812101
Jehan, S., Zhong, C., Li, G., Zulqarnain Bakhtiar, S., Li, D., and Sui, G. (2020). Thymoquinone Selectively Induces Hepatocellular Carcinoma Cell Apoptosis in Synergism with Clinical Therapeutics and Dependence of P53 Status. Front. Pharmacol. 11, 555283. doi:10.3389/fphar.2020.555283
Karim, S. S. A., and Karim, Q. A. (2021). Omicron SARS-CoV-2 Variant: a New Chapter in the COVID-19 Pandemic. The Lancet 398 (10317), 2126–2128. doi:10.1016/s0140-6736(21)02758-6
Khader, M., and Eckl, P. M. (2014). Thymoquinone: an Emerging Natural Drug with a Wide Range of Medical Applications. Iran J. Basic Med. Sci. 17 (12), 950–957.
Kokoska, L., Havlik, J., Valterova, I., Sovova, H., Sajfrtova, M., and Jankovska, I. (2008). Comparison of Chemical Composition and Antibacterial Activity of Nigella Sativa Seed Essential Oils Obtained by Different Extraction Methods. J. Food Prot. 71 (12), 2475–2480. doi:10.4315/0362-028x-71.12.2475
Lamb, Y. N. (2020). Remdesivir: First Approval. Drugs 80 (13), 1355–1363. doi:10.1007/s40265-020-01378-w
Lantz, I., Glämsta, E. L., Talbäck, L., and Nyberg, F. (1991). Hemorphins Derived from Hemoglobin Have an Inhibitory Action on Angiotensin Converting Enzyme Activity. FEBS Lett. 287 (1-2), 39–41. doi:10.1016/0014-5793(91)80011-q
Leong, X. F., Choy, K. W., and Alias, A. (2021). Anti-Inflammatory Effects of Thymoquinone in Atherosclerosis: A Mini Review. Front. Pharmacol. 12, 758929. doi:10.3389/fphar.2021.758929
Letko, M., Marzi, A., and Munster, V. (2020). Functional Assessment of Cell Entry and Receptor Usage for SARS-CoV-2 and Other Lineage B Betacoronaviruses. Nat. Microbiol. 5 (4), 562–569. doi:10.1038/s41564-020-0688-y
Li, W., Moore, M. J., Vasilieva, N., Sui, J., Wong, S. K., Berne, M. A., et al. (2003). Angiotensin-converting Enzyme 2 Is a Functional Receptor for the SARS Coronavirus. Nature 426 (6965), 450–454. doi:10.1038/nature02145
Marzi, A., Gramberg, T., Simmons, G., Möller, P., Rennekamp, A. J., Krumbiegel, M., et al. (2004). DC-SIGN and DC-SIGNR Interact with the Glycoprotein of Marburg Virus and the S Protein of Severe Acute Respiratory Syndrome Coronavirus. J. Virol. 78 (21), 12090–12095. doi:10.1128/JVI.78.21.12090-12095.2004
Mohammed, A., and Islam, M. S. (2018). Spice-Derived Bioactive Ingredients: Potential Agents or Food Adjuvant in the Management of Diabetes Mellitus. Front. Pharmacol. 9, 893. doi:10.3389/fphar.2018.00893
Mostofa, A. G. M., Hossain, M. K., Basak, D., and Bin Sayeed, M. S. (2017). Thymoquinone as a Potential Adjuvant Therapy for Cancer Treatment: Evidence from Preclinical Studies. Front. Pharmacol. 8, 295. doi:10.3389/fphar.2017.00295
National. Center for Biotechnology Information (2021a). PubChem Compound Summary for CID 10281, Thymoquinone. Available from: https://pubchem.ncbi.nlm.nih.gov/compound/Thymoquinone (Accessed Dec 25, 2021).
National. Center for Biotechnology Information (2021b). PubChem Compound Summary for CID 12947, Hydroxychloroquine Sulfate. Available from: https://pubchem.ncbi.nlm.nih.gov/compound/Hydroxychloroquine-sulfate (Accessed Dec 25, 2021).
Newman, D. J., and Cragg, G. M. (2016). Natural Products as Sources of New Drugs from 1981 to 2014. J. Nat. Prod. 79 (3), 629–661. doi:10.1021/acs.jnatprod.5b01055
Oliver, S. E., Gargano, J. W., Marin, M., Wallace, M., Curran, K. G., Chamberland, M., et al. (2020). The Advisory Committee on Immunization Practices' Interim Recommendation for Use of Pfizer-BioNTech COVID-19 Vaccine - United States, December 2020. MMWR Morb Mortal Wkly Rep. 69 (50), 1922–1924. doi:10.15585/mmwr.mm6950e2
Ong, Y. S., Saiful Yazan, L., Ng, W. K., Noordin, M. M., Sapuan, S., Foo, J. B., et al. (2016). Acute and Subacute Toxicity Profiles of Thymoquinone-Loaded Nanostructured Lipid Carrier in BALB/c Mice. Int. J. Nanomedicine 11, 5905–5915. doi:10.2147/IJN.S114205
Onifade, A. A., Jewell, A. P., and Adedeji, W. A. (2013). Nigella Sativa Concoction Induced Sustained Seroreversion in HIV Patient. Afr. J. Tradit Complement. Altern. Med. 10 (5), 332–335. doi:10.4314/ajtcam.v10i5.18
Onifade, A. A., Jewell, A. P., and Okesina, A. B. (2015). Seronegative Conversion of an HIV Positive Subject Treated with Nigella sativa and Honey. Afr. J. Infect. Dis. 9 (2), 47–50. doi:10.4314/ajid.v9i2.6
Pan, H., Pan, H., Peto, R., Henao-Restrepo, A. M., Preziosi, M. P., Sathiyamoorthy, V., et al. (2021). Repurposed Antiviral Drugs for Covid-19 - Interim WHO Solidarity Trial Results. N. Engl. J. Med. 384 (6), 497–511. doi:10.1056/NEJMoa2023184
Rabi, F. A., Al Zoubi, M. S., Kasasbeh, G. A., Salameh, D. M., and Al-Nasser, A. D. (2020). SARS-CoV-2 and Coronavirus Disease 2019: What We Know So Far. Pathogens 9 (3), 1–8. doi:10.3390/pathogens9030231
Rahman, M. T. (2020). Potential Benefits of Combination of Nigella Sativa and Zn Supplements to Treat COVID-19. J. Herb Med. 23, 100382. doi:10.1016/j.hermed.2020.100382
Salehi, B., Quispe, C., Imran, M., Ul-Haq, I., Živković, J., Abu-Reidah, I. M., et al. (2021). Nigella Plants – Traditional Uses, Bioactive Phytoconstituents, Preclinical and Clinical Studies. Front. Pharmacol. 12, 4-10. doi:10.3389/fphar.2021.625386
Salem, M. L., and Hossain, M. S. (2000). Protective Effect of Black Seed Oil from Nigella Sativa against Murine Cytomegalovirus Infection. Int. J. Immunopharmacol 22 (9), 729–740. doi:10.1016/s0192-0561(00)00036-9
Senger, M. R., Evangelista, T. C. S., Dantas, R. F., Santana, M. V. D. S., Gonçalves, L. C. S., de Souza Neto, L. R., et al. (2020). COVID-19: Molecular Targets, Drug Repurposing and New Avenues for Drug Discovery. Mem. Inst. Oswaldo Cruz 115, e200254. doi:10.1590/0074-02760200254
Shanmugam, M. K., Ahn, K. S., Hsu, A., Woo, C. C., Yuan, Y., Tan, K. H. B., et al. (2018). Thymoquinone Inhibits Bone Metastasis of Breast Cancer Cells through Abrogation of the CXCR4 Signaling Axis. Front. Pharmacol. 9, 1294. doi:10.3389/fphar.2018.01294
Shereen, M. A., Khan, S., Kazmi, A., Bashir, N., and Siddique, R. (2020). COVID-19 Infection: Origin, Transmission, and Characteristics of Human Coronaviruses. J. Adv. Res. 24, 91–98. doi:10.1016/j.jare.2020.03.005
Sommer, A. P., Försterling, H-D., and Naber, K. G. (2020). Thymoquinone: Shield and Sword against SARS-CoV-2. Precision Nanomedicine 3 (3), 541–548. doi:10.33218/001c.12984
Takai, S., Song, K., Tanaka, T., Okunishi, H., and Miyazaki, M. (1996). Antinociceptive Effects of Angiotensin-Converting Enzyme Inhibitors and an Angiotensin II Receptor Antagonist in Mice. Life Sci. 59 (21), PL331–6. doi:10.1016/0024-3205(96)00527-9
Ulasli, M., Gurses, S. A., Bayraktar, R., Yumrutas, O., Oztuzcu, S., Igci, M., et al. (2014). The Effects of Nigella Sativa (Ns), Anthemis Hyalina (Ah) and Citrus Sinensis (Cs) Extracts on the Replication of Coronavirus and the Expression of TRP Genes Family. Mol. Biol. Rep. 41 (3), 1703–1711. doi:10.1007/s11033-014-3019-7
U.S. Food and Drug Administration (2021a). Coronavirus (COVID-19) Update: FDA Authorizes First Oral Antiviral for Treatment of COVID-19 2021. Available from: https://www.fda.gov/news-events/press-announcements/coronavirus-covid-19-update-fda-authorizes-first-oral-antiviral-treatment-covid-19.
U.S. Food and Drug Administration (2021b). Janssen COVID-19 Vaccine. [Available from: https://www.fda.gov/media/146303/download.
U.S. Food and Drug Administration (2021c). Moderna COVID-19 Vaccine. Available from: https://www.fda.gov/media/144636/download.
U.S. Food and Drug Administration (2021d). Pfizer-BioNTech COVID-19 Vaccine. Available from: https://www.fda.gov/media/144412/download.
U.S. Food and Drug Administration (2021e). Pfizer-BioNTech COVID-19 Vaccine;Common Side Effects. Available from: https://www.fda.gov/emergency-preparedness-and-response/coronavirus-disease-2019-covid-19/pfizer-biontech-covid-19-vaccine.
Vaillancourt, F., Silva, P., Shi, Q., Fahmi, H., Fernandes, J. C., and Benderdour, M. (2011). Elucidation of Molecular Mechanisms Underlying the Protective Effects of Thymoquinone against Rheumatoid Arthritis. J. Cel Biochem 112 (1), 107–117. doi:10.1002/jcb.22884
Walls, A. C., Park, Y. J., Tortorici, M. A., Wall, A., McGuire, A. T., and Veesler, D. (2020). Structure, Function, and Antigenicity of the SARS-CoV-2 Spike Glycoprotein. Cell 181 (2), 281–e6. doi:10.1016/j.cell.2020.02.058
Wang, M., Cao, R., Zhang, L., Yang, X., Liu, J., Xu, M., et al. (2020). Remdesivir and Chloroquine Effectively Inhibit the Recently Emerged Novel Coronavirus (2019-nCoV) In Vitro. Cell Res 30 (3), 269–271. doi:10.1038/s41422-020-0282-0
World Health Organization (2021a). WHO Coronavirus (COVID-19) Dashboard. Available from: https://covid19.who.int/.
World Health Organization (2021b). Middle East Respiratory Syndrome Coronavirus (MERS-CoV) 2016. Available from: https://www.who.int/health-topics/middle-east-respiratory-syndrome-coronavirus-mers#tab=tab_1.
Xu, X., Chen, P., Wang, J., Feng, J., Zhou, H., Li, X., et al. (2020). Evolution of the Novel Coronavirus from the Ongoing Wuhan Outbreak and Modeling of its Spike Protein for Risk of Human Transmission. Sci. China Life Sci. 63 (3), 457–460. doi:10.1007/s11427-020-1637-5
Yang, Z. Y., Huang, Y., Ganesh, L., Leung, K., Kong, W. P., Schwartz, O., et al. (2004). pH-Dependent Entry of Severe Acute Respiratory Syndrome Coronavirus Is Mediated by the Spike Glycoprotein and Enhanced by Dendritic Cell Transfer through DC-SIGN. J. Virol. 78 (11), 5642–5650. doi:10.1128/JVI.78.11.5642-5650.2004
Yao, T. T., Qian, J. D., Zhu, W. Y., Wang, Y., and Wang, G. Q. (2020). A Systematic Review of Lopinavir Therapy for SARS Coronavirus and MERS Coronavirus-A Possible Reference for Coronavirus Disease-19 Treatment Option. J. Med. Virol. 92 (6), 556–563. doi:10.1002/jmv.25729
Keywords: COVID-19, pandemic, Coronavirus, Thymoquinone, PAXLOVID, molnupiravir, COVID-19 vaccines and anti-viral agents, Omicron variant
Citation: Abdelrahim M, Esmail A, Al Saadi N, Zsigmond E, Al Najjar E, Bugazia D, Al-Rawi H, Alsaadi A and Kaseb AO (2022) Thymoquinone’s Antiviral Effects: It is Time to be Proven in the Covid-19 Pandemic Era and its Omicron Variant Surge. Front. Pharmacol. 13:848676. doi: 10.3389/fphar.2022.848676
Received: 04 January 2022; Accepted: 17 March 2022;
Published: 05 April 2022.
Edited by:
Carlos L. Cespedes-Acuña, University of Bio-Bio, ChileReviewed by:
Raju Dash, Bangladesh Council of Scientific and Industrial Research (BCSIR), BangladeshJosé Guillermo Avila Acevedo, Universidad Nacional Autónoma de México, Mexico
Copyright © 2022 Abdelrahim, Esmail, Al Saadi, Zsigmond, Al Najjar, Bugazia, Al-Rawi, Alsaadi and Kaseb. This is an open-access article distributed under the terms of the Creative Commons Attribution License (CC BY). The use, distribution or reproduction in other forums is permitted, provided the original author(s) and the copyright owner(s) are credited and that the original publication in this journal is cited, in accordance with accepted academic practice. No use, distribution or reproduction is permitted which does not comply with these terms.
*Correspondence: Maen Abdelrahim, bWFiZGVscmFoaW1AaG91c3Rvbm1ldGhvZGlzdC5vcmc=; Ahmed O. Kaseb, YWthc2ViQG1kYW5kZXJzb24ub3Jn