- 1Graduate School, Beijing University of Chinese Medicine, Beijing, China
- 2Department of Cardiovascular, Dongfang Hospital, Beijing University of Chinese Medicine, Beijing, China
- 3Department of Cardiovascular, Guang’anmen Hospital, China Academy of Chinese Medical Sciences, Beijing, China
Background: Phytoestrogens are a class of natural compounds that have structural similarities to estrogens. They have been identified to confer potent cardioprotective effects in experimental myocardial ischemia-reperfusion injury (MIRI) animal models. We aimed to investigate the effect of PE on MIRI and its intrinsic mechanisms.
Methods: A systematic search was conducted to identify PEs that have been validated in animal studies or clinical studies as effective against MIRI. Then, we collected studies that met inclusion and exclusion criteria from January 2016 to September 2021. The SYRCLE’s RoB tool was used to evaluate the quality. Data were analyzed by STATA 16.0 software.
Results: The search yielded 18 phytoestrogens effective against heart disease. They are genistein, quercetin, biochanin A, formononetin, daidzein, kaempferol, icariin, puerarin, rutin, notoginsenoside R1, tanshinone IIA, ginsenoside Rb1, ginsenoside Rb3, ginsenoside Rg1, ginsenoside Re, resveratrol, polydatin, and bakuchiol. Then, a total of 20 studies from 17 articles with a total of 355 animals were included in this meta-analysis. The results show that PE significantly reduced the myocardial infarct size in MIRI animals compared with the control group (p < 0.001). PE treatment significantly reduced the creatine kinase level (p < 0.001) and cTnI level (p < 0.001), increased left ventricular ejection fraction (p < 0.001) and left ventricular fractional shortening (p < 0.001) in MIRI animals. In addition, PE also exerts a significant heart rate lowering effect (p < 0.001).
Conclusion: Preclinical evidence suggests that PE can be multi-targeted for cardioprotective effects in MIRI. More large animal studies and clinical research are still needed in the future to further confirm its role in MIRI.
1 Introduction
Ischemic heart diseases, such as coronary artery disease (CHD), represent the most the leading cause of death worldwide (Ibáñez et al., 2015; Hausenloy et al., 2017), which has crucial socio-economical implications. Percutaneous coronary intervention (PCI) is the primary means of revascularization in patients with coronary artery disease (O'Gara et al., 20132013). Timely and effective PCI treatment can recanalize the occluded coronary artery, reestablish blood perfusion in the infarcted area, and salvage ischemic myocardial tissue, which improves the survival rate of patients with coronary heart disease (Hausenloy and Yellon, 2013). However, it can also paradoxically cause further myocardial ischemia-reperfusion injury (MIRI), which can manifest clinically as an increase in infarct size, cardiac insufficiency, myocardial stunning, arrhythmias, and even sudden death (Piper et al., 1998).
Although, several therapies have been approved to give cardioprotection in experimental models of MIRI (Mokhtari-Zaer et al., 2018; Sawashita et al., 2020; Sun et al., 2021; Wu et al., 2021). MIRI is currently not treated in a clinically effective manner. Estrogen is known to perform well in cardioprotection (Knowlton and Lee, 2012; Crescioli, 2021). But this cardiovascular protection declines after menopause, with myocardial infarction being the primary cause of mortality in older women (Wenger, 2016). Estrogen deficiency has been shown to play an important role in the development of cardiovascular diseases such as MIRI (Deschamps et al., 2010; Sivasinprasasn et al., 2016), atherosclerosis (Kassi et al., 2015; Hajializadeh and Khaksari, 2021), heart failure ((Hajializadeh and Khaksari, 2021), (Adekunle et al., 2021)), atrial fibrillation (Bretler et al., 2012; Odening et al., 2019), hypertension (Colafella and Denton, 2018; Srivaratharajah and Abramson, 2019), myocardial fibrosis (Medzikovic et al., 2019), cardiac hypertrophy (Hajializadeh and Khaksari, 2021), and Takotsubo syndrome (Pelliccia et al., 2017). However, the use of estrogen or estrogen replacement therapy for an extended period can raise the risk of gynecological cancers (Stevenson et al., 2009; Narod, 2011).
Phytoestrogens (PEs) are a class of natural non-steroidal compounds widely found in many plants and herbs (Sirotkin and Harrath, 2014; Rietjens et al., 2017). It can bind to estrogen receptors (ER) to exert estrogen-like effects with few side effects (Kurzer and Xu, 1997; Sirotkin and Harrath, 2014; Li et al., 2016a; Basu and Maier, 2018). PE and PE-containing drugs have been shown to help prevent and treat menopausal symptoms (Franco et al., 2016), osteoporosis (Thangavel et al., 2019), metabolic diseases (Mukund et al., 2017), and especially cardiovascular diseases (Frankenfeld, 2017; Kirichenko et al., 2017; Sathyapalan et al., 2018). Notably, PEs have been identified that confer robust cardioprotection in experimental MIRI animal and cellular models through multiple molecular pathway modalities, such as inflammatory pathways, mitochondrial energy metabolic pathways, oxidative stress pathways, autophagic pathways, etc (Ji et al., 2004; Guo et al., 2018; Huang et al., 2018; Bai et al., 2019; Colareda et al., 2020).
PEs have become a promising cardioprotective candidate for MIRI due to their advantages of multiple therapeutic targets, lower side effects, and higher safety compared to estrogen replacement therapy (Sirtori, 2001; Bloedon et al., 2002; Michael McClain et al., 2006; Douglas et al., 2013; Czuczwar et al., 2017; Bolton et al., 2019).
Systematic preclinical study review aids in the elucidation of pharmacological mechanisms, the integration of therapeutic evidence, the improvement of experimental methods, and, eventually, the translation and transformation from animal studies to clinical trials (Roberts et al., 2002; Sandercock and Roberts, 2002). This study aims to elucidate the treatment mechanism of PE on MIRI and provide credible preclinical evidence by conducting a systematic review.
2 Methods
2.1 Identification of PEs
A systematic search of four databases (Pubmed, Web of Science, Embase, and Cochrane Library) was conducted to identify PEs that have been validated in animal studies or clinical studies for interventions in MIRI.
2.2 Search Strategy
Four databases (Pubmed, Web of Science, Embase, and Cochrane Library) were searched using “myocardial ischemia-reperfusion injury”, “myocardial reperfusion injury”, and the PE retrieved in 2.1 as keywords. Then manually search and add any literature that may have been missed. Timespan: January 2016 - September 2021. Only published articles written in the English language were considered in the current meta-analysis. The specific search process was shown in Figure 1.
2.3 Inclusion and Exclusion Criteria
The following criteria were set in advance. Inclusion criteria: 1) Rats or mice were used as research subjects; 2) methods for establishing animal models of MIRI: ligation and loosening surgery of the left anterior descending branch of coronary artery (in vivo), or Langendroff perfusion (ex vivo); 3) the treatment group received any dose of PE, while the control group received vehicle or no treatment; 4) The myocardial infarction size was taken as the main outcome index, with or without other indexes such as myocardial enzymes, heart rate (HR), left ventricular ejection fraction (LVEF), and left ventricular fractional shortening (LVFS); 5) the size of the myocardial infarction is reported as a percentage (i.e. TTC staining or Evan’s blue/TTC staining). Exclusion criteria: 1) animals with other cardiovascular comorbidities (e.g. diabetes, hyperlipidemia); 2) animals are treated with PE analogs or given additional drugs. 3) Studies with incomplete and inaccessible data; 4) duplicate publications.
2.4 Study Characteristics Extraction
Two authors (YM W and XT S) extracted the study characteristics independently, and discrepancies were resolved by the corresponding author. Information extracted included: first author, year of publication, anesthetics, animal information (animal species, number, sex, and weight), I/R duration, interventions (PE type, dose, method of administration) for the treatment and control groups, outcome indicators, and staining method of infarct size. The data were pooled using the formula as follows when different doses of pharmacological interventions were employed in the treatment group (Zhang and Chen, 2016). When data are represented graphically, every effort will be made to contact the author for more information or to measure from the graph.
2.5 Quality Appraisal
The SYRCLE’s RoB tool (Hooijmans et al., 2014) was used to evaluate the quality of the studies by two authors independently. The SYRCLE’s RoB tool contains 10 entries: sequence generation, baseline characteristics, allocation concealment, random housing, blinding, random outcome assessment, blinding, incomplete outcome data, selective outcome reporting, and other sources of bias. A third person ruled in case of disagreement.
2.6 Outcome Measures and Statistical Analyses
Data were analyzed by STATA 16.0 software. Outcomes were expressed as standardized mean difference (SMD) with a 95% confidence interval (95%CI). In the forest plot, the dark squares represent the standardized mean difference (SMD) for each study, the diamonds represent the pooled SMD, and 95% of the CIs are indicated by lines. p values ≤0.05 were considered statistically significant. Statistics were analyzed using a fixed-effects model (I2 ≤ 50%, p ≥ 0.10) or a random-effects model (I2 > 50%, p < 0.10). Sensitivity analysis, stratification analysis, or univariable meta-regression should be performed to deal with high heterogeneity if necessary. We performed sensitivity analysis by removing each study in turn to assess the impact of this study. We conducted a stratified analysis and meta-regression of myocardial infarction size by study type, route of administration, staining method, PE type, animal species, and anesthetics type. The Egger’s test and Begg’s test for publication bias were performed using STATA 16.0.
3 Results
3.1 Identification of PEs
The following PEs were retrieved that have been experimentally proven to be effective against MIRI. They are divided into four main categories: 1) Isoflavones: genistein, quercetin, biochanin A, formononetin, daidzein, kaempferol, icariin, puerarin, rutin, et al.; 2) Terpenoids: notoginsenoside R1, tanshinone IIA, ginsenoside Rb1, ginsenoside Rb3, ginsenoside Rg1, ginsenoside Re, et al.; 3) stilbenoids: resveratrol, polydatin, et al.; 4) miscellaneous classes: bakuchiol. We collected the chemical structure of these PEs and the representative herbs. The specific information is shown in Table 1.
3.2 Literature Retrieval Results
A total of 337 articles were obtained from various databases. After removing duplicate articles, we reviewed the abstracts and full text sequentially according to strict inclusion and exclusion criteria. Finally, a total of 20 studies from 17 articles (Li et al., 2016b; Li et al., 2016c; Gu et al., 2016; Ling et al., 2016; Liu et al., 2016; Yang et al., 2016; Cui et al., 2017; Wang et al., 2017; Li et al., 2018; Lin et al., 2018; Wu et al., 2018; Bai et al., 2019; Li et al., 2020a; Wang et al., 2020a; Wang et al., 2020b; Kazemirad and Kazerani, 2020; Jiang et al., 2021) were included in our analysis (Figure 1).
3.3 Study Characteristics
The characteristics of included 20 studies are provided in Table 2. The main PEs involved are genistein (n = 1), quercetin (n = 1), biochanin A (n = 1), formononetin (n = 1), kaempferol (n = 1), icariin (n = 2), puerarin (n = 1), rutin (n = 1), tanshinone IIA (n = 1), ginsenoside Rb1 (n = 6), ginsenoside Rg1 (n = 1), resveratrol (n = 2), and polydatin (n = 1). A total of 17 studies were conducted in vivo using ligation followed by the release of LAD to simulate MIRI, and the remaining three studies were conducted ex vivo using Langendroff perfusion. The author Wu B conducted both in vivo and ex vivo studies, which we labeled and distinguished with “Wu B 2018 (1)” and “Wu B 2018 (2).” All studies used infarct size as the primary efficacy index, with 12 studies using TTC single staining and 8 using Evan’s blue/TTC double staining. A total of six kinds of anesthetics were used in the studies included in this analysis: pentobarbital sodium (n = 12), thiopental sodium (n = 1), urethane (n = 1), chloral hydrate (n = 2), isoflurane (n = 3), and a mixture of xylazine and ketamine (n = 1). A total of 355 animals were enrolled for our study, including SD rats, Wistar rats, and C57BL/6J mice. Several studies have included markers of myocardial injury such as levels of cardiac troponin I (cTnI) (n = 5) and creatine kinase (CK) (n = 2) as outcome indicators in their analyses. Seven studies performed echocardiography on animals and reported LVEF and LVFS. All articles were written in English. Only one study is from Iran, the rest were conducted in China.
3.4 Quality Appraisal
We used the SYRCLE’s RoB tool to score the quality of each study. Table 3 shows the information on methodological quality. As shown in Table 3, six studies scored five points, and four studies scored only two points, which indicate reliable data but lower quality of studies. Ten studies mentioned randomized groupings, but all were silent on the specific randomization method. All studies did not describe how allocation concealment is performed.
3.5 Outcome Measures
3.5.1 Infarct Size
Studies that reported infarct size were analyzed using a random-effects model. Figure 2 showed that PE significantly reduced the myocardial infarct size in MIRI animals compared with the control group (SMD = −3.92, 95%CI: −5.19 to −2.66, p < 0.001). I2>50%, which suggests high heterogeneity. We first performed sensitivity analysis by removing each study in turn to assess the impact of this study. Then we performed the stratified analysis (Table 4) and meta-regression (Table 5) with study type, reperfusion time, route of administration, animal species, staining method, or PE type as covariates. Sensitivity analysis and stratified analysis failed to find significant sources of heterogeneity, while meta-regression confirms study type as a heterogeneity source (p = 0.046 < 0.05). Egger’s (p = 0.00) (Supplementary Figure S1) and Begg’s (p = 1.999) (Supplementary Figure S2) tests confirmed the possible existence of publication bias. After additional adjustment for potential missing studies by nonparametric trim-and-fill analysis (Supplementary Figures S3, S4), the statistical results support the robust effect of PE treatment (SMD = -2.634, 95%CI: -4.204 to -1.065, p < 0.001). This result implies that PE can effectively reduce the size of myocardial infarct area in MIRI animals, which may be related to the type of animal experiment involved.
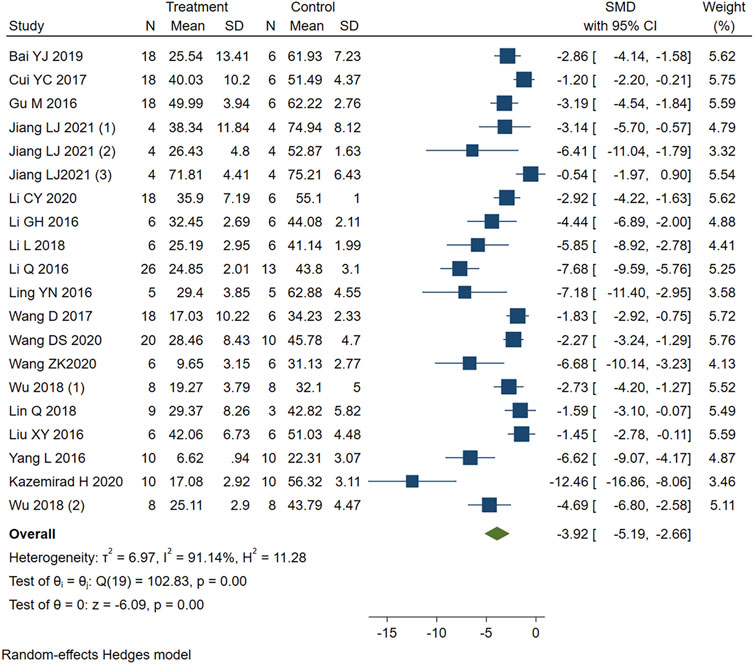
FIGURE 2. Forest plot to study the effect of PE on infarct size. PE reduced the myocardial infarct size in MIRI animals compared with the control group (SMD = −3.92, 95%CI: −5.19 to −2.66, p < 0.001). The dark squares represent the standardized mean difference (SMD) for each study. The diamonds represent the pooled SMD. 95% of the CIs are indicated by lines. The analysis was conducted using a fixed-effects model.
3.5.2 Markers of Myocardial Injury
Five studies that reported serum CK levels were analyzed using a fixed-effects model. Figure 3 shows that PE treatment was associated with significantly lower CK levels in MIRI animals (SMD = −2.61, 95% CI: −3.19 to −2.03, p < 0.001).
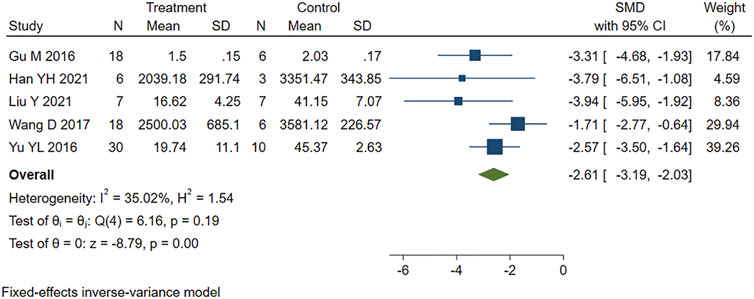
FIGURE 3. Forest plot to study the effect of PE on creatine kinase (CK). PE reduced serum CK levels in MIRI animals compared to control group (SMD = −2.61, 95% CI: −3.19 to −2.03, p < 0.001). The dark squares represent the standardized mean difference (SMD) for each study. The diamonds represent the pooled SMD. 95% of the CIs are indicated by lines. The analysis was conducted using a fixed-effects model.
The five studies that reported cTnI levels showed high heterogeneity (I2 = 82.24%, p = 0.00). We performed a sensitivity analysis by systematically excluding each study. One study (Kazemirad and Kazerani, 2020) was identified as a source of heterogeneity and was therefore removed. The other four studies were analyzed using fixed-effects models. The results showed that PE treatment significantly reduced the cTnI level in MIRI animals compared to the control group (SMD = −3.66, 95% CI: −4.54 to −2.79, p < 0.001) (Figure 4).
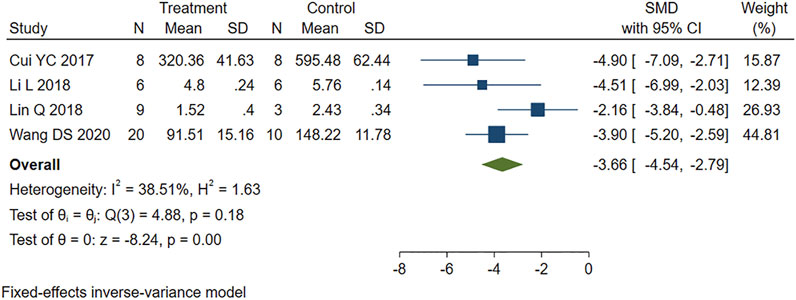
FIGURE 4. Forest plot to study the effect of PE on cardiac troponin I (cTnI). PE reduced serum cTnI levels in MIRI animals compared to control group (SMD = −3.66, 95% CI: −4.54 to −2.79, p < 0.001). The dark squares represent the standardized mean difference (SMD) for each study. The diamonds represent the pooled SMD. 95% of the CIs are indicated by lines. The analysis was conducted using a fixed-effects model.
The statistical results suggest that the serum CK and cTnI levels in the PE group were significantly lower than those in the control group, indicating that PE treatment can effectively alleviate the myocardial damage caused by MIRI.
3.5.3 Indicators of Cardiac Function
Seven studies appropriately reported the effect of PE on LVEF in MIRI animals. Analysis using a fixed-effects model showed that PE significantly increased LVEF (SMD = 2.54, 95% CI: 2.04 to 3.03, p < 0.001) (Figure 5).
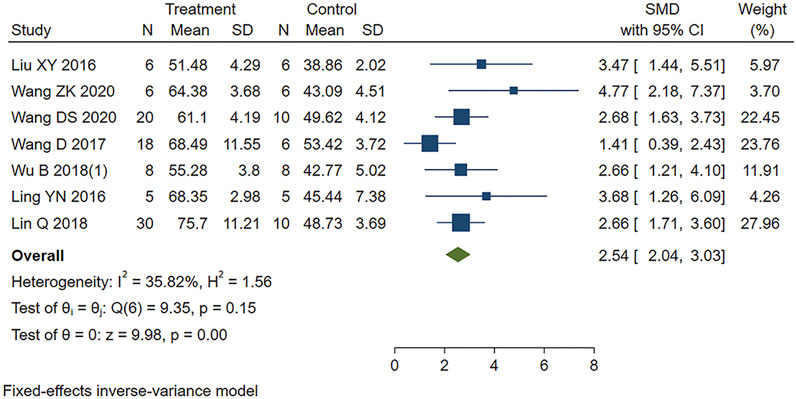
FIGURE 5. Forest plot to study the effect of PE on left ventricular ejection fraction (LVEF). PE improved LVEF in MIRI animals compared to control group (SMD = 2.54, 95% CI: 2.04 to 3.03, p < 0.001). The dark squares represent the standardized mean difference (SMD) for each study. The diamonds represent the pooled SMD. 95% of the CIs are indicated by lines. The analysis was conducted using a fixed-effects model.
Similarly, moderate heterogeneity (I2 = 48.38%, p < 0.001) was found in the eight studies reporting LVFS in our study. We removed one study identified (Wang et al., 2017) by sensitivity analysis as the heterogeneous source. The remaining seven studies were included in the analysis using a fixed-effects model. Figure 6 showed that PE treatment led to higher LVFS compared to the control group (SMD = 2.66, 95% CI: 2.20 to 3.11, p < 0.001).
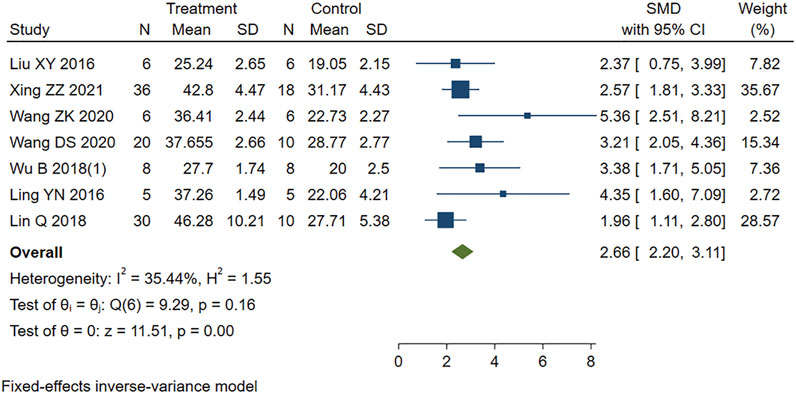
FIGURE 6. Forest plot to study the effect of PE on left ventricular fractional shortening (LVFS). PE improved LVFS in MIRI animals compared to control group (SMD = 2.66, 95% CI: 2.20 to 3.11, p < 0.001). The dark squares represent the standardized mean difference (SMD) for each study. The diamonds represent the pooled SMD. 95% of the CIs are indicated by lines. The analysis was conducted using a fixed-effects model.
The statistical results showed that the difference between PE group and control group was statistically significant. PE treatment can improve cardiac function in MIRI animals.
3.5.4 Heart Rate
Four other studies reported the effect of PE on heart rate variability in MIRI animals. Sensitivity analysis identified and removed one study (Kazemirad and Kazerani, 2020) that was considered a source of heterogeneity. The remaining studies were included in the analysis using a fixed-effects model. The new combined effect size determined the HR lowering effect of PE treatment on MIRI animals (SMD = 4.08 95% CI: 2.98 to 5.18, p < 0.001) (Figure 7), as did the total combined effect size. This indicates that the PE treatment group can reduce the heart rate of MIRI animals compared to the control group, and the difference is statistically significant.
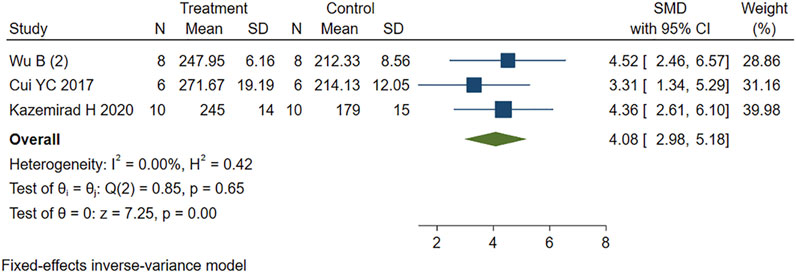
FIGURE 7. Forest plot to study the effect of PE on heart rate. PE reduced heart rate in MIRI animals compared to control group (SMD = 4.08 95% CI: 2.98 to 5.18, p < 0.001). The dark squares represent the standardized mean difference (SMD) for each study. The diamonds represent the pooled SMD. 95% of the CIs are indicated by lines. The analysis was conducted using a fixed-effects model.
4 Discussion
The incidence of CHD increases rapidly in postmenopausal women, with declining estrogen levels being the main cause (Barrett-Connor, 2013). Myocardial infarction (MI) is milder and occurs later in women than in men of the same age (Regitz-Zagrosek and Kararigas, 2017). These sex differences disappear after natural or surgical menopause (ovariectomy) (McSweeney et al., 2016), or under conditions impairing ovarian function and thus estrogen production (Barton, 2013). The effects of estrogen on MIRI are partly attributed to the potent anti-inflammatory (Wang et al., 2006), antioxidant (Kim et al., 1996), and mitochondrial protective properties (Zhai et al., 2000; Wang et al., 2019) of estrogen. There have been some studies demonstrating that elevated estrogen levels are likely to be the cause of PE’s efficacy for MIRI in ovariectomized rats (Zhai et al., 2001; Hao et al., 2011; Tang et al., 2016). PEs are found in many Chinese herbs such as ginseng (Renshen in Chinese), salvia (Danshen in Chinese), geranium (Gegen in Chinese), and safflower (Honghua in Chinese), which are often included in prescriptions for the Chinese medicine treatment of CHD (Li and Zhao, 2009; Chiu et al., 2011; Huang et al., 2016; Li et al., 2020b; Zhang et al., 2021a; Zhang et al., 2021b). In recent years, researchers have made significant progress in the treatment of MIRI with PEs and have published a large number of research results. However, these results have not been systematically analyzed.
4.1 Summary of Evidence
A total of 355 animals were included in our study, 222 of which were treated with PE. As a class of cardioprotective agent, PEs exerted significant anti-MIRI effects in animal models, mainly in reducing infarct size (p < 0.001), mitigating myocardial injury (p < 0.001), improving cardiac function (p < 0.001) and lowering heart rate (p < 0.001). Moreover, this cardioprotective effect was not limited by factors such as phytoestrogen species, rodent species, methods of I/R, I/R duration, or anesthetics, which were found by stratified analysis, sensitivity analysis, and regression analysis of different outcome indicators.
4.2 Molecular Mechanisms
Prior to conducting a clinical trial, animal models can be used to determine the effectiveness of a drug or procedure and to explore its mechanisms. Through a comprehensive search of various databases, the specific mechanisms of PE against MIRI can be summarized as follows.
1. Mitochondrial pathway. The primary mechanism we found is a disturbance in mitochondrial structure, function, and quantity. Mitochondrial structure and function are impaired by ischemia and aggravated by reperfusion (Giedt et al., 2012; Consolini et al., 2017; Ham and Raju, 2017; Anzell et al., 2018). MIRI causes impairment of the mitochondrial respiratory chain leading to abnormal energy metabolism (Leist et al., 1997; Tahrir et al., 2019), and also causes excessive accumulation of reactive oxygen species (ROS) producing oxidative stress (Li and Jackson, 2002; Lesnefsky et al., 2017). PE can suppress mitochondrial swelling and reduce the number of fragmented mitochondria in terms of retaining mitochondrial structure (Zhai et al., 2001). As for the involvement in energy metabolism, PEs has been shown to inhibit the activation RhoA/ROCK signaling (He et al., 2014; Cui et al., 2017; Yan et al., 2021) as well as activate the mKATP channels ((Colareda et al., 2020), (Colareda et al., 2021)), thus promote ATP production. PE also directly or indirectly regulates the activity of mitochondrial complex. For example, PE has been found not only to reduce NADH dehydrogenase activity and inhibit mitochondrial complex I activity, but also to maintain mitochondrial complex V activity. (Jiang et al., 2021; Yan et al., 2021). Meanwhile, PE can further ameliorate oxidative stress injury in cardiomyocytes by regulating silent information regulator 1 (SIRT1) and thus downstream targets such as peroxlsome proliferator-activated receptor-γ coactlvator-1α (PGC-1α) (Feng et al., 2016; Zhong et al., 2019) and forkhead box O 1 (FOXO1) (Wu et al., 2018). Furthermore, it has been suggested that PE’s effect on mitochondrial function is dosage-dependent (Barton, 2013). It works as an antioxidant and enhances mitochondrial biogenesis at low dosages, but it also functions as a pro-oxidant and impairs mitochondrial function at high dosages (Roca et al., 2014). For example, Genistein has been reported to promote cell proliferation at low concentrations (0.1–10M) and inhibit cell proliferation at high concentrations (above 10M) (Matsumura et al., 2005; Seo et al., 2006).
2. Inflammation and immunity. According to our results and analysis, inflammation and immunity have been perceived as significant markers of MIRI injury. The occurrence of I/R promotes the production of inflammatory mediators and chemokines, thus promoting the adhesion and accumulation of leukocytes in the vascular endothelium (Phillipson and Kubes, 2011); at the same time, cardiomyocytes produce large amounts of inflammatory factors (IL6, TNF-α, IL-1β) (Poynter et al., 2011), which further amplify the inflammatory response and eventually induce apoptosis in cardiomyocytes (Slegtenhorst et al., 2014). PEs can inhibit the activation of nuclear factor-κ-gene binding (NF-κB) by regulating its upstream pathway proteins toll-like receptor 4 (TLR4), JunNterminal kinase (JNK), or SIRT1((Bai et al., 2019), (Wang et al., 2020b), (Kim et al., 2009; Ma et al., 2014)). It has additionally been shown that PEs can restrain the activation of NLRP3 inflammasome through multiple pathways while inhibiting the maturation and secretion of inflammatory factors and decreasing their levels in tissues and serum (Wang et al., 2020a; Wang et al., 2020b).
3. Estrogen receptors. There are three subtypes of estrogen receptors: ERα, Erβ, and GPR30 (Deschamps and Murphy, 2009; Jia et al., 2015). Studies have shown that all ER subtypes confer cardioprotection against I/R injury both via genomic and non-genomic mechanisms ((Deschamps et al., 2010), (Deschamps and Murphy, 2009), (Zhu et al., 2020)). By interacting with ER, PEs are shown to sustain NO level by increasing the activity and expression of NO synthase (Zhai et al., 2001). Activation of ER can protect mitochondrial structural integrity and function and reduce mitochondrial autophagy ((Wang et al., 2019), (Feng et al., 2017)). Study has shown that PE significantly improved mitochondrial swelling and reduced the number of mitochondrial fragments by binding to estrogen receptors, thus maintaining the structural integrity of mitochondria. (Zhai et al., 2001).
4. Other mechanisms. In addition, our study found that the cardioprotective effects conferred by PE are associated with calcium homeostasis, ferroptosis, and endoplasmic reticulum stress. There is a concrete example that PE reduces intracellular Ca2+ level and maintains calcium homeostasis by manipulating stromal interacting molecule 1 (STIM1)-mediated store-operated calcium entry (SOCE) (Xu et al., 2019). It has been shown that PE regulates USP19/Beclin1-induced autophagy to suppress ferroptosis (Li et al., 2022). It has also been shown that multiple PEs significantly regulate the unfolded protein response (complex adaptive or pro-apoptotic signaling triggered by endoplasmic reticulum stress) associated proteins glucose-regulated protein (GRP)78, X-box binding protein (XBP)-1, cleaved activating transcription factor (ATF)-6, inositol-requiring protein-1α (IRE1α), and C/EBP-homologous protein (CHOP), in the setting of myocardial I/R injury (Kim et al., 2008; Kim et al., 2010).
In summary, in addition to their estrogen-like cardioprotective effects as hormone replacement therapy, PEs also act through other pathways unrelated to estrogen. PE can maintain mitochondrial function and structure, alleviate oxidative stress, improve Inflammation and immune responses, and mitigate calcium overload by regulating multiple signaling pathways. The signaling pathways involved are engaged in a variety of cross-talk. The mechanisms involved are equally interactive and causal (Figure8).
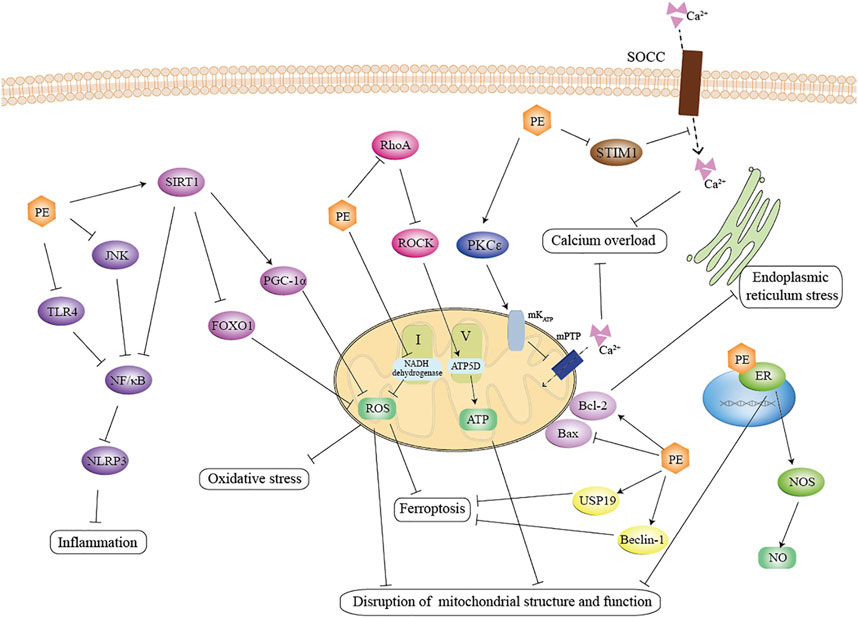
FIGURE 8. Schematic representation of the anti-MIRI effect of PE. PE suppress the inflammation by inhibiting the TLR4-NF/κB and JNK- NF/κB signaling pathways, inhibit mitochondrial oxidative stress by activating the SIRT1 pathway, and promoted the activity of the mitochondrial complex by inhibiting the RohA pathway. In addition, PE attenuated calcium overload via STIM1-mediated SOCE. Notably, PE not only maintained mitochondrial homeostasis through interaction with estrogen receptors, but also promoted NO production, which effectively exerted cardioprotective effects. TLR4: toll-like receptor four; JNK: JunNterminal kinase; NF/κB: nuclear factor-κ-gene binding; SIRT1: silent information regulator 1; FOXO1: forkhead box O; PGC-1α: proliferator-activated receptor-γ coactlvator-1α; ROS: reactive oxygen species; ROCK: RhoA/Rho-associated coiled-coil containing protein kinase; PKCε: protein kinase C; STIM1: stromal interacting molecule 1; SOCC: store-operated calcium channels; ER: estrogen receptor; NOS: nitric oxide synthase; USP19: ubiquity specific peptidase 19.
5 Strengths and Limitations
To our knowledge, this is the first preclinical systematic review to study the cardioprotective effects of PE in MIRI animals. Not only the efficacy of PE on MIRI but also the specific mechanisms were explored in depth. There are still limitations. Systematic evaluation of animal studies is more likely to be affected by significant heterogeneity than clinical research. Phytoestrogens may lead to moderate heterogeneity depending on their form, type, dose, and route of administration, which is difficult to avoid. In addition, most of the phytoestrogens in our included studies were pre-administered and only their preventive effects and immediate efficacy were evaluated. Therefore, there is no additional evidence to further explore the long-term effects of PE and their therapeutic effects on MIRI. More large animal studies and clinical research are still needed in the future to further confirm its role in MIRI.
6 Conclusion
PE can play a beneficial role in MIRI by improving mitochondrial function, reducing inflammation, regulating ER, improving endoplasmic reticulum stress, and reducing ferroptosis. They are expected to be a class of cardioprotective drugs with promising development and application prospects due to their broad pharmacological effects, low toxic side effects, and high safety.
Data Availability Statement
The original contributions presented in the study are included in the article/Supplementary Material, further inquiries can be directed to the corresponding author.
Author Contributions
YuW and XS: study conception and design. ZF and JC: acquisition, analysis, and interpretation of data. DX: creation of charts. YuW and YW: article revision, final approval and overall responsibility for this published work. All authors contributed to the article and approved the submitted version.
Funding
This study was supported by the National Natural Science Foundation of China (81573900).
Conflict of Interest
The authors declare that the research was conducted in the absence of any commercial or financial relationships that could be construed as a potential conflict of interest.
Publisher’s Note
All claims expressed in this article are solely those of the authors and do not necessarily represent those of their affiliated organizations, or those of the publisher, the editors and the reviewers. Any product that may be evaluated in this article, or claim that may be made by its manufacturer, is not guaranteed or endorsed by the publisher.
Supplementary Material
The Supplementary Material for this article can be found online at: https://www.frontiersin.org/articles/10.3389/fphar.2022.847748/full#supplementary-material.
References
Adekunle, A. O., Adzika, G. K., Mprah, R., Ndzie Noah, M. L., Adu-Amankwaah, J., Rizvi, R., et al. (2021). Predominance of Heart Failure with Preserved Ejection Fraction in Postmenopausal Women: Intra- and Extra-cardiomyocyte Maladaptive Alterations Scaffolded by Estrogen Deficiency. Front. Cell Dev. Biol. 9, 685996. doi:10.3389/fcell.2021.685996
Anzell, A. R., Maizy, R., Przyklenk, K., and Sanderson, T. H. (2018). Mitochondrial Quality Control and Disease: Insights into Ischemia-Reperfusion Injury. Mol. Neurobiol. 55 (3), 2547–2564. doi:10.1007/s12035-017-0503-9
Bai, Y., Li, Z., Liu, W., Gao, D., Liu, M., and Zhang, P. (2019). Biochanin A Attenuates Myocardial Ischemia/reperfusion Injury through the TLR4/NF-Κb/nlrp3 Signaling Pathway. Acta Cir. Bras. 34 (11), e201901104. doi:10.1590/s0102-865020190110000004
Barrett-Connor, E. (2013). Menopause, Atherosclerosis, and Coronary Artery Disease. Curr. Opin. Pharmacol. 13 (2), 186–191. doi:10.1016/j.coph.2013.01.005
Barton, M. (2013). Cholesterol and Atherosclerosis: Modulation by Oestrogen. Curr. Opin. Lipidol. 24 (3), 214–220. doi:10.1097/MOL.0b013e3283613a94
Basu, P., and Maier, C. (2018). Phytoestrogens and Breast Cancer: In Vitro Anticancer Activities of Isoflavones, Lignans, Coumestans, Stilbenes and Their Analogs and Derivatives. Biomed. Pharmacother. 107, 1648–1666. doi:10.1016/j.biopha.2018.08.100
Bloedon, L. T., Jeffcoat, A. R., Lopaczynski, W., Schell, M. J., Black, T. M., Dix, K. J., et al. (2002). Safety and Pharmacokinetics of Purified Soy Isoflavones: Single-Dose Administration to Postmenopausal Women. Am. J. Clin. Nutr. 76 (5), 1126–1137. doi:10.1093/ajcn/76.5.1126
Bolton, J. L., Dunlap, T. L., Hajirahimkhan, A., Mbachu, O., Chen, S. N., Chadwick, L., et al. (2019). The Multiple Biological Targets of Hops and Bioactive Compounds. Chem. Res. Toxicol. 32 (2), 222–233. doi:10.1021/acs.chemrestox.8b00345
Bretler, D. M., Hansen, P. R., Lindhardsen, J., Ahlehoff, O., Andersson, C., Jensen, T. B., et al. (2012). Hormone Replacement Therapy and Risk of New-Onset Atrial Fibrillation after Myocardial Infarction-Aa Nationwide Cohort Study. PLoS One 7 (12), e51580. doi:10.1371/journal.pone.0051580
Chiu, P. Y., Wong, S. M., Leung, H. Y., Leong, P. K., Chen, N., Zhou, L., et al. (2011). Acute Treatment with Danshen-Gegen Decoction Protects the Myocardium against Ischemia/reperfusion Injury via the Redox-Sensitive PKCɛ/mK(ATP) Pathway in Rats. Phytomedicine 18 (11), 916–925. doi:10.1016/j.phymed.2011.03.006
Colafella, K. M. M., and Denton, K. M. (2018). Sex-specific Differences in Hypertension and Associated Cardiovascular Disease. Nat. Rev. Nephrol. 14 (3), 185–201. doi:10.1038/nrneph.2017.189
Colareda, G. A., Matera, S. I., Bayley, M., Ragone, M. I., Flores, M. L., Córdoba, O. L., et al. (2021). Lepidium Meyenii (Maca) and Soy Isoflavones Reduce Cardiac Stunning of Ischemia-Reperfusion in Rats by Mitochondrial Mechanisms. J. Tradit. Complement. Med. 11 (6), 471–480. doi:10.1016/j.jtcme.2021.03.004
Colareda, G. A., Ragone, M. I., Bonazzola, P., and Consolini, A. E. (2020). The mKATP Channels and Protein-Kinase C Are Involved in the Cardioprotective Effects of Genistein on Estrogen-Deficient Rat Hearts Exposed to Ischemia/Reperfusion: Energetic Study. J. Cardiovasc Pharmacol. 75 (5), 460–474. doi:10.1097/FJC.0000000000000816
Consolini, A. E., Ragone, M. I., Bonazzola, P., and Colareda, G. A. (2017). Mitochondrial Bioenergetics during Ischemia and Reperfusion. Adv. Exp. Med. Biol. 982, 141–167. doi:10.1007/978-3-319-55330-6_8
Crescioli, C. (2021). The Role of Estrogens and Vitamin D in Cardiomyocyte Protection: A Female Perspective. Biomolecules 11 (12). doi:10.3390/biom11121815
Cui, Y. C., Pan, C. S., Yan, L., Li, L., Hu, B. H., Chang, X., et al. (2017). Ginsenoside Rb1 Protects against Ischemia/reperfusion-Induced Myocardial Injury via Energy Metabolism Regulation Mediated by RhoA Signaling Pathway. Sci. Rep. 7, 44579. doi:10.1038/srep44579
Czuczwar, P., Paszkowski, T., Lisiecki, M., Woźniak, S., and Stępniak, A. (2017). The Safety and Tolerance of Phytotherapies in Menopausal Medicine - a Review of the Literature. Prz. Menopauzalny 16 (1), 8–11. doi:10.5114/pm.2017.67365
Deschamps, A. M., and Murphy, E. (2009). Activation of a Novel Estrogen Receptor, GPER, Is Cardioprotective in Male and Female Rats. Am. J. Physiol. Heart Circ. Physiol. 297 (5), H1806–H1813. doi:10.1152/ajpheart.00283
Deschamps, A. M., Murphy, E., and Sun, J. (2010). Estrogen Receptor Activation and Cardioprotection in Ischemia Reperfusion Injury. Trends Cardiovasc Med. 20 (3), 73–78. doi:10.1016/j.tcm.2010.05.001
Douglas, C. C., Johnson, S. A., and Arjmandi, B. H. (2013). Soy and its Isoflavones: the Truth behind the Science in Breast Cancer. Anticancer Agents Med. Chem. 13 (8), 1178–1187. doi:10.2174/18715206113139990320
Feng, J., Yang, Y., Zhou, Y., Wang, B., Xiong, H., Fan, C., et al. (2016). Bakuchiol Attenuates Myocardial Ischemia Reperfusion Injury by Maintaining Mitochondrial Function: the Role of Silent Information Regulator 1. Apoptosis 21 (5), 532–545. doi:10.1007/s10495-016-1225-6
Feng, Y., Madungwe, N. B., da Cruz Junho, C. V., and Bopassa, J. C. (2017). Activation of G Protein-Coupled Oestrogen Receptor 1 at the Onset of Reperfusion Protects the Myocardium against Ischemia/reperfusion Injury by Reducing Mitochondrial Dysfunction and Mitophagy. Br. J. Pharmacol. 174 (23), 4329–4344. doi:10.1111/bph.14033
Franco, O. H., Chowdhury, R., Troup, J., Voortman, T., Kunutsor, S., Kavousi, M., et al. (2016). Use of Plant-Based Therapies and Menopausal Symptoms: A Systematic Review and Meta-Analysis. JAMA 315 (23), 2554–2563. doi:10.1001/jama.2016.8012
Frankenfeld, C. L. (2017). Cardiometabolic Risk and Gut Microbial Phytoestrogen Metabolite Phenotypes. Mol. Nutr. Food Res. 61 (1). doi:10.1002/mnfr.201500900
Giedt, R. J., Yang, C., Zweier, J. L., Matzavinos, A., and Alevriadou, B. R. (2012). Mitochondrial Fission in Endothelial Cells after Simulated Ischemia/reperfusion: Role of Nitric Oxide and Reactive Oxygen Species. Free Radic. Biol. Med. 52 (2), 348–356. doi:10.1016/j.freeradbiomed.2011.10.491
Gu, M., Zheng, A. B., Jin, J., Cui, Y., Zhang, N., Che, Z. P., et al. (2016). Cardioprotective Effects of Genistin in Rat Myocardial Ischemia-Reperfusion Injury Studies by Regulation of P2X7/NF-Κb Pathway. Evid. Based Complement. Altern. Med. 2016, 5381290. doi:10.1155/2016/5381290
Guo, B. Q., Xu, J. B., Xiao, M., Ding, M., and Duan, L. J. (2018). Puerarin Reduces Ischemia/reperfusion-Induced Myocardial Injury in Diabetic Rats via Upregulation of Vascular Endothelial Growth Factor A/angiotensin-1 and Suppression of Apoptosis. Mol. Med. Rep. 17 (5), 7421–7427. doi:10.3892/mmr.2018.8754
Hajializadeh, Z., and Khaksari, M. (2021). The Protective Effects of 17-β Estradiol and SIRT1 against Cardiac Hypertrophy: a Review. Heart Fail Rev. 27, 725–738. doi:10.1007/s10741-021-10171-0
Ham, P. B., and Raju, R. (2017). Mitochondrial Function in Hypoxic Ischemic Injury and Influence of Aging. Prog. Neurobiol. 157, 92–116. doi:10.1016/j.pneurobio.2016.06.006
Hao, K., Gong, P., Sun, S. Q., Hao, H. P., Wang, G. J., Dai, Y., et al. (2011). Mechanism-based Pharmacokinetic-Pharmacodynamic Modeling of the Estrogen-like Effect of Ginsenoside Rb1 on Neural 5-HT in Ovariectomized Mice. Eur. J. Pharm. Sci. 44 (1-2), 117–126. doi:10.1016/j.ejps.2011.06.014
Hausenloy, D. J., Garcia-Dorado, D., Bøtker, H. E., Davidson, S. M., Downey, J., Engel, F. B., et al. (2017). Novel Targets and Future Strategies for Acute Cardioprotection: Position Paper of the European Society of Cardiology Working Group on Cellular Biology of the Heart. Cardiovasc Res. 113 (6), 564–585. doi:10.1093/cvr/cvx049
Hausenloy, D. J., and Yellon, D. M. (2013). Myocardial Ischemia-Reperfusion Injury: a Neglected Therapeutic Target. J. Clin. Invest. 123 (1), 92–100. doi:10.1172/JCI62874
He, K., Yan, L., Pan, C. S., Liu, Y. Y., Cui, Y. C., Hu, B. H., et al. (2014). ROCK-dependent ATP5D Modulation Contributes to the Protection of Notoginsenoside NR1 against Ischemia-Reperfusion-Induced Myocardial Injury. Am. J. Physiol. Heart Circ. Physiol. 307 (12), H1764–H1776. doi:10.1152/ajpheart.00259.2014
Hooijmans, C. R., Rovers, M. M., de Vries, R. B., Leenaars, M., Ritskes-Hoitinga, M., and Langendam, M. W. (2014). SYRCLE's Risk of Bias Tool for Animal Studies. BMC Med. Res. Methodol. 14, 43. doi:10.1186/1471-2288-14-43
Huang, J., Tang, X., Ye, F., He, J., and Kong, X. (2016). Clinical Therapeutic Effects of Aspirin in Combination with Fufang Danshen Diwan, a Traditional Chinese Medicine Formula, on Coronary Heart Disease: A Systematic Review and Meta-Analysis. Cell Physiol. Biochem. 39 (5), 1955–1963. doi:10.1159/000447892
Huang, Z., Liu, Y., and Huang, X. (2018). Formononetin May Protect Aged Hearts from Ischemia/reperfusion Damage by Enhancing Autophagic Degradation. Mol. Med. Rep. 18 (6), 4821–4830. doi:10.3892/mmr.2018.9544
Ibáñez, B., Heusch, G., Ovize, M., and Van de Werf, F. (2015). Evolving Therapies for Myocardial Ischemia/reperfusion Injury. J. Am. Coll. Cardiol. 65 (14), 1454–1471. doi:10.1016/j.jacc.2015.02.032
Ji, E. S., Yue, H., Wu, Y. M., and He, R. R. (2004). Effects of Phytoestrogen Genistein on Myocardial Ischemia/reperfusion Injury and Apoptosis in Rabbits. Acta Pharmacol. Sin. 25 (3), 306–312.
Jia, M., Dahlman-Wright, K., and Gustafsson, J. Å. (2015). Estrogen Receptor Alpha and Beta in Health and Disease. Best. Pract. Res. Clin. Endocrinol. Metab. 29 (4), 557–568. doi:10.1016/j.beem.2015.04.008
Jiang, L., Yin, X., Chen, Y. H., Chen, Y., Jiang, W., Zheng, H., et al. (2021). Proteomic Analysis Reveals Ginsenoside Rb1 Attenuates Myocardial Ischemia/reperfusion Injury through Inhibiting ROS Production from Mitochondrial Complex I. Theranostics 11 (4), 1703–1720. doi:10.7150/thno.43895
Kassi, E., Spilioti, E., Nasiri-Ansari, N., Adamopoulos, C., Moutsatsou, P., Papapanagiotou, A., et al. (2015). Vascular Inflammation and Atherosclerosis: The Role of Estrogen Receptors. Curr. Med. Chem. 22 (22), 2651–2665. doi:10.2174/0929867322666150608093607
Kazemirad, H., and Kazerani, H. R. (2020). Cardioprotective Effects of Resveratrol Following Myocardial Ischemia and Reperfusion. Mol. Biol. Rep. 47 (8), 5843–5850. doi:10.1007/s11033-020-05653-7
Kim, D. S., Ha, K. C., Kwon, D. Y., Kim, M. S., Kim, H. R., Chae, S. W., et al. (2008). Kaempferol Protects Ischemia/reperfusion-Induced Cardiac Damage through the Regulation of Endoplasmic Reticulum Stress. Immunopharmacol. Immunotoxicol. 30 (2), 257–270. doi:10.1080/08923970701812530
Kim, D. S., Kwon, D. Y., Kim, M. S., Kim, H. K., Lee, Y. C., Park, S. J., et al. (2010). The Involvement of Endoplasmic Reticulum Stress in Flavonoid-Induced Protection on Cardiac Cell Death Caused by Ischaemia/reperfusion. J. Pharm. Pharmacol. 62 (2), 197–204. doi:10.1211/jpp.62.02.0007
Kim, J. W., Jin, Y. C., Kim, Y. M., Rhie, S., Kim, H. J., Seo, H. G., et al. (2009). Daidzein Administration In Vivo Reduces Myocardial Injury in a Rat Ischemia/reperfusion Model by Inhibiting NF-kappaB Activation. Life Sci. 84 (7-8), 227–234. doi:10.1016/j.lfs.2008.12.005
Kim, Y. D., Chen, B., Beauregard, J., Kouretas, P., Thomas, G., Farhat, M. Y., et al. (1996). 17 Beta-Estradiol Prevents Dysfunction of Canine Coronary Endothelium and Myocardium and Reperfusion Arrhythmias after Brief Ischemia/reperfusion. Circulation 94 (11), 2901–2908. doi:10.1161/01.cir.94.11.2901
Kirichenko, T. V., Myasoedova, V. A., Orekhova, V. A., Ravani, A. L., Nikitina, N. A., Grechko, A. V., et al. (2017). Phytoestrogen-Rich Natural Preparation for Treatment of Climacteric Syndrome and Atherosclerosis Prevention in Perimenopausal Women. Phytother. Res. 31 (8), 1209–1214. doi:10.1002/ptr.5841
Knowlton, A. A., and Lee, A. R. (2012). Estrogen and the Cardiovascular System. Pharmacol. Ther. 135 (1), 54–70. doi:10.1016/j.pharmthera.2012.03.007
Kurzer, M. S., and Xu, X. (1997). Dietary Phytoestrogens. Annu. Rev. Nutr. 17, 353–381. doi:10.1146/annurev.nutr.17.1.353
Leist, M., Single, B., Castoldi, A. F., Kühnle, S., and Nicotera, P. (1997). Intracellular Adenosine Triphosphate (ATP) Concentration: a Switch in the Decision between Apoptosis and Necrosis. J. Exp. Med. 185 (8), 1481–1486. doi:10.1084/jem.185.8.1481
Lesnefsky, E. J., Chen, Q., Tandler, B., and Hoppel, C. L. (2017). Mitochondrial Dysfunction and Myocardial Ischemia-Reperfusion: Implications for Novel Therapies. Annu. Rev. Pharmacol. Toxicol. 57, 535–565. doi:10.1146/annurev-pharmtox-010715-103335
Li, A. M., and Zhao, J. (2009). Effect of Renshen Jianxin Capsule for Alleviating Insulin Resistance in Patients with Coronary Heart Disease and Glucose Tolerance Impairment. Zhongguo Zhong Xi Yi Jie He Za Zhi 29 (9), 830–833.
Li, C., and Jackson, R. M. (2002). Reactive Species Mechanisms of Cellular Hypoxia-Reoxygenation Injury. Am. J. Physiol. Cell Physiol. 282 (2), C227–C241. doi:10.1152/ajpcell.00112.2001
Li, C. Y., Yang, P., Jiang, Y. L., Lin, Z., Pu, Y. W., Xie, L. Q., et al. (2020). Ginsenoside Rb1 Attenuates Cardiomyocyte Apoptosis Induced by Myocardial Ischemia Reperfusion Injury through mTOR Signal Pathway. Biomed. Pharmacother. 125, 109913. doi:10.1016/j.biopha.2020.109913
Li, G., Qian, W., and Zhao, C. (2016). Analyzing the Anti-ischemia-reperfusion Injury Effects of Ginsenoside Rb1 Mediated through the Inhibition of P38α MAPK. Can. J. Physiol. Pharmacol. 94 (1), 97–103. doi:10.1139/cjpp-2014-0164
Li, J., Cao, G. Y., Zhang, X. F., Meng, Z. Q., Gan, L., Li, J. X., et al. (2020). Chinese Medicine She-Xiang-Xin-Tong-Ning, Containing Moschus, Corydalis and Ginseng, Protects from Myocardial Ischemia Injury via Angiogenesis. Am. J. Chin. Med. 48 (1), 107–126. doi:10.1142/S0192415X20500068
Li, L., Chen, X., Liu, C. C., Lee, L. S., Man, C., and Cheng, S. H. (2016). Phytoestrogen Bakuchiol Exhibits In Vitro and In Vivo Anti-breast Cancer Effects by Inducing S Phase Arrest and Apoptosis. Front. Pharmacol. 7, 128. doi:10.3389/fphar.2016.00128
Li, L., Pan, C. S., Yan, L., Cui, Y. C., Liu, Y. Y., Mu, H. N., et al. (2018). Ginsenoside Rg1 Ameliorates Rat Myocardial Ischemia-Reperfusion Injury by Modulating Energy Metabolism Pathways. Front. Physiol. 9, 78. doi:10.3389/fphys.2018.00078
Li, Q., Shen, L., Wang, Z., Jiang, H. P., and Liu, L. X. (2016). Tanshinone IIA Protects against Myocardial Ischemia Reperfusion Injury by Activating the PI3K/Akt/mTOR Signaling Pathway. Biomed. Pharmacother. 84, 106–114. doi:10.1016/j.biopha.2016.09.014
Li, T., Tan, Y., Ouyang, S., He, J., and Liu, L. (2022). Resveratrol Protects against Myocardial Ischemia-Reperfusion Injury via Attenuating Ferroptosis. Gene 808, 145968. doi:10.1016/j.gene.2021.145968
Lin, Q., Chen, X.-Y., Zhang, J., Yuan, Y.-L., Zhao, W., and Wei, B. (2018). Upregulation of SIRT1 Contributes to the Cardioprotective Effect of Rutin against Myocardial Ischemia-Reperfusion Injury in Rats. J. Funct. Foods 46, 227–236. doi:10.1016/j.jff.2018.05.007
Ling, Y., Chen, G., Deng, Y., Tang, H., Ling, L., Zhou, X., et al. (2016). Polydatin Post-treatment Alleviates Myocardial Ischaemia/reperfusion Injury by Promoting Autophagic Flux. Clin. Sci. (Lond) 130 (18), 1641–1653. doi:10.1042/cs20160082
Liu, X., Yu, Z., Huang, X., Gao, Y., Wang, X., Gu, J., et al. (2016). Peroxisome Proliferator-Activated Receptor Gamma (PPARgamma) Mediates the Protective Effect of Quercetin against Myocardial Ischemia-Reperfusion Injury via Suppressing the NF-kappaB Pathway. Am. J. Transl. Res. 8 (12), 5169–5186.
Ma, L., Liu, H., Xie, Z., Yang, S., Xu, W., Hou, J., et al. (2014). Ginsenoside Rb3 Protects Cardiomyocytes against Ischemia-Reperfusion Injury via the Inhibition of JNK-Mediated NF-Κb Pathway: a Mouse Cardiomyocyte Model. PLoS One 9 (8), e103628. doi:10.1371/journal.pone.0103628
Matsumura, A., Ghosh, A., Pope, G. S., and Darbre, P. D. (2005). Comparative Study of Oestrogenic Properties of Eight Phytoestrogens in MCF7 Human Breast Cancer Cells. J. Steroid Biochem. Mol. Biol. 94 (5), 431–443. doi:10.1016/j.jsbmb.2004.12.041
McSweeney, J. C., Rosenfeld, A. G., Abel, W. M., Braun, L. T., Burke, L. E., Daugherty, S. L., et al. (2016). Preventing and Experiencing Ischemic Heart Disease as a Woman: State of the Science: A Scientific Statement from the American Heart Association. Circulation 133 (13), 1302–1331. doi:10.1161/CIR.0000000000000381
Medzikovic, L., Aryan, L., and Eghbali, M. (2019). Connecting Sex Differences, Estrogen Signaling, and microRNAs in Cardiac Fibrosis. J. Mol. Med. Berl. 97 (10), 1385–1398. doi:10.1007/s00109-019-01833-6
Michael McClain, R., Wolz, E., Davidovich, A., and Bausch, J. (2006). Genetic Toxicity Studies with Genistein. Food Chem. Toxicol. 44 (1), 42–55. doi:10.1016/j.fct.2005.06.004
Mokhtari-Zaer, A., Marefati, N., Atkin, S. L., Butler, A. E., and Sahebkar, A. (2018). The Protective Role of Curcumin in Myocardial Ischemia-Reperfusion Injury. J. Cell Physiol. 234 (1), 214–222. doi:10.1002/jcp.26848
Mukund, V., Mukund, D., Sharma, V., Mannarapu, M., and Alam, A. (2017). Genistein: Its Role in Metabolic Diseases and Cancer. Crit. Rev. Oncol. Hematol. 119, 13–22. doi:10.1016/j.critrevonc.2017.09.004
Narod, S. A. (2011). Hormone Replacement Therapy and the Risk of Breast Cancer. Nat. Rev. Clin. Oncol. 8 (11), 669–676. doi:10.1038/nrclinonc.2011.110
O'Gara, P. T., Kushner, F. G., Ascheim, D. D., Casey, D. E., Chung, M. K., de Lemos, J. A., et al. (2013). 2013 ACCF/AHA Guideline for the Management of ST-Elevation Myocardial Infarction: Executive Summary: a Report of the American College of Cardiology Foundation/American Heart Association Task Force on Practice Guidelines. Circulation 127 (4), 529–555. doi:10.1016/j.jacc.2012.11.019
Odening, K. E., Deiss, S., Dilling-Boer, D., Didenko, M., Eriksson, U., Nedios, S., et al. (2019). Mechanisms of Sex Differences in Atrial Fibrillation: Role of Hormones and Differences in Electrophysiology, Structure, Function, and Remodelling. Europace 21 (3), 366–376. doi:10.1093/europace/euy215
Pelliccia, F., Kaski, J. C., Crea, F., and Camici, P. G. (2017). Pathophysiology of Takotsubo Syndrome. Circulation 135 (24), 2426–2441. doi:10.1161/CIRCULATIONAHA.116.027121
Phillipson, M., and Kubes, P. (2011). The Neutrophil in Vascular Inflammation. Nat. Med. 17 (11), 1381–1390. doi:10.1038/nm.2514
Piper, H. M., García-Dorado, D., and Ovize, M. (1998). A Fresh Look at Reperfusion Injury. Cardiovasc Res. 38 (2), 291–300. doi:10.1016/s0008-6363(98)00033-9
Poynter, J. A., Herrmann, J. L., Manukyan, M. C., Wang, Y., Abarbanell, A. M., Weil, B. R., et al. (2011). Intracoronary Mesenchymal Stem Cells Promote Postischemic Myocardial Functional Recovery, Decrease Inflammation, and Reduce Apoptosis via a Signal Transducer and Activator of Transcription 3 Mechanism. J. Am. Coll. Surg. 213 (2), 253–260. doi:10.1016/j.jamcollsurg.2011.04.005
Regitz-Zagrosek, V., and Kararigas, G. (2017). Mechanistic Pathways of Sex Differences in Cardiovascular Disease. Physiol. Rev. 97 (1), 1–37. doi:10.1152/physrev.00021.2015
Rietjens, I. M. C. M., Louisse, J., and Beekmann, K. (2017). The Potential Health Effects of Dietary Phytoestrogens. Br. J. Pharmacol. 174 (11), 1263–1280. doi:10.1111/bph.13622
Roberts, I., Kwan, I., Evans, P., and Haig, S. (2002). Does Animal Experimentation Inform Human Healthcare? Observations from a Systematic Review of International Animal Experiments on Fluid Resuscitation. BMJ 324 (7335), 474–476. doi:10.1136/bmj.324.7335.474
Roca, P., Sastre-Serra, J., Nadal-Serrano, M., Pons, D. G., Ma del Mar, B.-R., and Oliver, J. (2014). Phytoestrogens and Mitochondrial Biogenesis in Breast Cancer. Influence of Estrogen Receptors Ratio. Curr. Pharm. Des. 20 (35), 5594–5618. doi:10.2174/1381612820666140306100709
Sandercock, P., and Roberts, I. (2002). Systematic Reviews of Animal Experiments. Lancet 360 (9333), 586. doi:10.1016/S0140-6736(02)09812-4
Sathyapalan, T., Aye, M., Rigby, A. S., Thatcher, N. J., Dargham, S. R., Kilpatrick, E. S., et al. (2018). Soy Isoflavones Improve Cardiovascular Disease Risk Markers in Women during the Early Menopause. Nutr. Metab. Cardiovasc Dis. 28 (7), 691–697. doi:10.1016/j.numecd.2018.03.007
Sawashita, Y., Hirata, N., Yoshikawa, Y., Terada, H., Tokinaga, Y., and Yamakage, M. (2020). Remote Ischemic Preconditioning Reduces Myocardial Ischemia-Reperfusion Injury through Unacylated Ghrelin-Induced Activation of the JAK/STAT Pathway. Basic Res. Cardiol. 115 (4), 50. doi:10.1007/s00395-020-0809-z
Seo, H. S., DeNardo, D. G., Jacquot, Y., Laïos, I., Vidal, D. S., Zambrana, C. R., et al. (2006). Stimulatory Effect of Genistein and Apigenin on the Growth of Breast Cancer Cells Correlates with Their Ability to Activate ER Alpha. Breast Cancer Res. Treat. 99 (2), 121–134. doi:10.1007/s10549-006-9191-2
Sirotkin, A. V., and Harrath, A. H. (2014). Phytoestrogens and Their Effects. Eur. J. Pharmacol. 741, 230–236. doi:10.1016/j.ejphar.2014.07.057
Sirtori, C. R. (2001). Risks and Benefits of Soy Phytoestrogens in Cardiovascular Diseases, Cancer, Climacteric Symptoms and Osteoporosis. Drug Saf. 24 (9), 665–682. doi:10.2165/00002018-200124090-00003
Sivasinprasasn, S., Shinlapawittayatorn, K., Chattipakorn, S. C., and Chattipakorn, N. (2016). Estrogenic Impact on Cardiac Ischemic/Reperfusion Injury. J. Cardiovasc Transl. Res. 9 (1), 23–39. doi:10.1007/s12265-016-9675-3
Slegtenhorst, B. R., Dor, F. J., Rodriguez, H., Voskuil, F. J., and Tullius, S. G. (2014). Ischemia/reperfusion Injury and its Consequences on Immunity and Inflammation. Curr. Transpl. Rep. 1 (3), 147–154. doi:10.1007/s40472-014-0017-6
Srivaratharajah, K., and Abramson, B. L. (2019). Hypertension in Menopausal Women: the Effect and Role of Estrogen. Menopause 26 (4), 428–430. doi:10.1097/GME.0000000000001304
Stevenson, J. C., Hodis, H. N., Pickar, J. H., and Lobo, R. A. (2009). Coronary Heart Disease and Menopause Management: the Swinging Pendulum of HRT. Atherosclerosis 207 (2), 336–340. doi:10.1016/j.atherosclerosis.2009.05.033
Sun, X., Gao, R., Li, W., Zhao, Y., Yang, H., Chen, H., et al. (2021). Alda-1 Treatment Promotes the Therapeutic Effect of Mitochondrial Transplantation for Myocardial Ischemia-Reperfusion Injury. Bioact. Mater 6 (7), 2058–2069. doi:10.1016/j.bioactmat.2020.12.024
Tahrir, F. G., Langford, D., Amini, S., Mohseni Ahooyi, T., and Khalili, K. (2019). Mitochondrial Quality Control in Cardiac Cells: Mechanisms and Role in Cardiac Cell Injury and Disease. J. Cell Physiol. 234 (6), 8122–8133. doi:10.1002/jcp.27597
Tang, Y., Li, S., Zhang, P., Zhu, J., Meng, G., Xie, L., et al. (2016). Soy Isoflavone Protects Myocardial Ischemia/Reperfusion Injury through Increasing Endothelial Nitric Oxide Synthase and Decreasing Oxidative Stress in Ovariectomized Rats. Oxid. Med. Cell Longev. 2016, 5057405. doi:10.1155/2016/5057405
Thangavel, P., Puga-Olguín, A., Rodríguez-Landa, J. F., and Zepeda, R. C. (2019). Genistein as Potential Therapeutic Candidate for Menopausal Symptoms and Other Related Diseases. Molecules 24 (21). doi:10.3390/molecules24213892
Wang, D. S., Yan, L. Y., Yang, D. Z., Lyu, Y., Fang, L. H., Wang, S. B., et al. (2020). Formononetin Ameliorates Myocardial Ischemia/reperfusion Injury in Rats by Suppressing the ROS-TXNIP-NLRP3 Pathway. Biochem. Biophys. Res. Commun. 525 (3), 759–766. doi:10.1016/j.bbrc.2020.02.147
Wang, D., Zhang, X., Li, D., Hao, W., Meng, F., Wang, B., et al. (2017). Kaempferide Protects against Myocardial Ischemia/Reperfusion Injury through Activation of the PI3K/Akt/GSK-3β Pathway. Mediat. Inflamm. 2017, 5278218. doi:10.1155/2017/5278218
Wang, M., Smith, K., Yu, Q., Miller, C., Singh, K., and Sen, C. K. (2019). Mitochondrial Connexin 43 in Sex-dependent Myocardial Responses and Estrogen-Mediated Cardiac Protection Following Acute Ischemia/reperfusion Injury. Basic Res. Cardiol. 115 (1), 1. doi:10.1007/s00395-019-0759-5
Wang, M., Tsai, B. M., Reiger, K. M., Brown, J. W., and Meldrum, D. R. (2006). 17-beta-Estradiol Decreases P38 MAPK-Mediated Myocardial Inflammation and Dysfunction Following Acute Ischemia. J. Mol. Cell Cardiol. 40 (2), 205–212. doi:10.1016/j.yjmcc.2005.06.019
Wang, Z. K., Chen, R. R., Li, J. H., Chen, J. Y., Li, W., Niu, X. L., et al. (2020). Puerarin Protects against Myocardial Ischemia/reperfusion Injury by Inhibiting Inflammation and the NLRP3 Inflammasome: The Role of the SIRT1/NF-Κb Pathway. Int. Immunopharmacol. 89 (Pt B), 107086. doi:10.1016/j.intimp.2020.107086
Wenger, N. K. (2016). Clinical Presentation of CAD and Myocardial Ischemia in Women. J. Nucl. Cardiol. 23 (5), 976–985. doi:10.1007/s12350-016-0593-1
Wu, B., Feng, J. Y., Yu, L. M., Wang, Y. C., Chen, Y. Q., Wei, Y., et al. (2018). Icariin Protects Cardiomyocytes against Ischaemia/reperfusion Injury by Attenuating Sirtuin 1-dependent Mitochondrial Oxidative Damage. Br. J. Pharmacol. 175 (21), 4137–4153. doi:10.1111/bph.14457
Wu, Q., Shang, Y., Bai, Y., Wu, Y., Wang, H., and Shen, T. (2021). Sufentanil Preconditioning Protects against Myocardial Ischemia/reperfusion Injury via miR-125a/DRAM2 axis. Cell Cycle 20 (4), 383–391. doi:10.1080/15384101.2021.1875668
Xu, H., Cheng, J., Wang, X., Liu, H., Wang, S., Wu, J., et al. (2019). Resveratrol Pretreatment Alleviates Myocardial Ischemia/reperfusion Injury by Inhibiting STIM1-Mediated Intracellular Calcium Accumulation. J. Physiol. Biochem. 75 (4), 607–618. doi:10.1007/s13105-019-00704-5
Yan, L., Pan, C. S., Liu, Y. Y., Cui, Y. C., Hu, B. H., Chang, X., et al. (2021). The Composite of 3, 4-Dihydroxyl-Phenyl Lactic Acid and Notoginsenoside R1 Attenuates Myocardial Ischemia and Reperfusion Injury through Regulating Mitochondrial Respiratory Chain. Front. Physiol. 12, 538962. doi:10.3389/fphys.2021.538962
Yang, L., Zhang, Y., Zhu, M., Zhang, Q., Wang, X., Wang, Y., et al. (2016). Resveratrol Attenuates Myocardial Ischemia/reperfusion Injury through Up-Regulation of Vascular Endothelial Growth Factor B. Free Radic. Biol. Med. 101, 1–9. doi:10.1016/j.freeradbiomed.2016.09.016
Zhai, P., Eurell, T. E., Cotthaus, R., Jeffery, E. H., Bahr, J. M., and Gross, D. R. (2000). Effect of Estrogen on Global Myocardial Ischemia-Reperfusion Injury in Female Rats. Am. J. Physiol. Heart Circ. Physiol. 279 (6), H2766–H2775. doi:10.1152/ajpheart.2000.279.6.H2766
Zhai, P., Eurell, T. E., Cotthaus, R. P., Jeffery, E. H., Bahr, J. M., and Gross, D. R. (2001). Effects of Dietary Phytoestrogen on Global Myocardial Ischemia-Reperfusion Injury in Isolated Female Rat Hearts. Am. J. Physiol. Heart Circ. Physiol. 281 (3), H1223–H1232. doi:10.1152/ajpheart.2001.281.3.H1223
Zhang, B. K. J., and Chen, X. M. (2016). Methods to Combine Standard Deviations of Different Subgruops in Meta-Analysis. Chin. J. Evidence-Based Med. 16 (07), 851–854.
Zhang, S., Chen, Z. L., Tang, Y. P., Duan, J. L., and Yao, K. W. (2021). Efficacy and Safety of Xue-Fu-Zhu-Yu Decoction for Patients with Coronary Heart Disease: A Systematic Review and Meta-Analysis. Evid. Based Complement. Altern. Med. 2021, 9931826. doi:10.1155/2021/9931826
Zhang, Y., Wang, J., Liu, Y. M., Chen, Y. Y., Yang, X. C., and Duan, L. (2021). The Synergistic Effects of Astragalus Mongholicus and Salvia Miltiorrhiza on Coronary Heart Disease Identified by Network Pharmacology and Experiment. Drug Des. Devel Ther. 15, 4053–4069. doi:10.2147/DDDT.S326024
Zhong, J., Ouyang, H., Sun, M., Lu, J., Zhong, Y., Tan, Y., et al. (2019). Tanshinone IIA Attenuates Cardiac Microvascular Ischemia-Reperfusion Injury via Regulating the SIRT1-Pgc1α-Mitochondrial Apoptosis Pathway. Cell Stress Chaperones 24 (5), 991–1003. doi:10.1007/s12192-019-01027-6
Keywords: phytoestrogen, isoflavones, myocardial ischemia reperfusion injury, molecular mechanisms, preclinical, meta-analysis, systematic review
Citation: Wang Y, Shou X, Fan Z, Cui J, Xue D and Wu Y (2022) A Systematic Review and Meta-Analysis of Phytoestrogen Protects Against Myocardial Ischemia/Reperfusion Injury: Pre-Clinical Evidence From Small Animal Studies. Front. Pharmacol. 13:847748. doi: 10.3389/fphar.2022.847748
Received: 03 January 2022; Accepted: 26 April 2022;
Published: 20 May 2022.
Edited by:
Fiorentina Roviezzo, University of Naples Federico II, ItalyReviewed by:
Wanpitak Pongkan, Chiang Mai University, ThailandAlicia Consolini, National University of La Plata, Argentina
Copyright © 2022 Wang, Shou, Fan, Cui, Xue and Wu. This is an open-access article distributed under the terms of the Creative Commons Attribution License (CC BY). The use, distribution or reproduction in other forums is permitted, provided the original author(s) and the copyright owner(s) are credited and that the original publication in this journal is cited, in accordance with accepted academic practice. No use, distribution or reproduction is permitted which does not comply with these terms.
*Correspondence: Yang Wu, drwuyang@163.com
†These authors have contributed equally to this work and share first authorship