- Neurobiology of Adolescent Drinking in Adulthood Consortium, Center for Development and Behavioral Neuroscience, Department of Psychology, Binghamton University, Binghamton, NY, United States
Adolescence is a sensitive developmental period during which alcohol use is often initiated and consumed in high quantities, often at binge or even high-intensity drinking levels. Our lab has repeatedly found that adolescent intermittent ethanol (AIE) exposure in rats results in long-lasting social impairments, specifically in males, however our knowledge of the neuronal underpinnings to this sex-specific effect of AIE is limited. The present study was designed to test whether social anxiety-like alterations in AIE-exposed males would be accompanied by alterations of neuronal activation across brain regions associated with social behavior, with AIE females demonstrating no social impairments and alterations in neuronal activation. Adolescent male and female cFos-LacZ transgenic rats on a Sprague-Dawley background were exposed to ethanol (4 g/kg, 25% v/v) or water via intragastric gavage every other day during postnatal days (P) 25–45 for a total of 11 exposures (n = 13 per group). Social behavior of adult rats was assessed on P70 using a modified social interaction test, and neuronal activation in brain regions implicated in social responding was assessed via β-galactosidase (β-gal) expression. We found that AIE exposure in males resulted in a significantly lower social preference coefficient relative to water-exposed controls, with no effect evident in females. Exposure-specific relationships between social behavior and neuronal activation were identified, with AIE eliminating correlations found in water controls related to social interaction, and eliciting negative correlations mainly in limbic regions in a sex-specific manner. AIE exposure in the absence of social testing was also found to differentially affect neural activity in the orbitofrontal cortex and central amygdala in males and females. These data suggest that AIE produces sex-specific social impairments that are potentially driven by differential neuronal activation states in regions important for social behavior, including the medial prefrontal and orbitofrontal cortices, nucleus accumbens, lateral septum, and central amygdala. Future studies should be focused on identification of specific neuronal phenotypes activated by interaction with a social partner in AIE-exposed subjects and their control counterparts.
Introduction
Adolescence is a sensitive developmental period during which the brain undergoes substantial maturation (Larsen and Luna, 2018) and is especially vulnerable to different insults including alcohol. Alcohol use is often initiated in adolescence (Morean et al., 2018), with over 2 million adolescents in the United States between 12 and 17 years of age initiating alcohol in 2019 (Substance Abuse and Mental Health Services -SAMHSA, 2020). During late adolescence alcohol use becomes heavier, often in the forms of binge (SAMHSA, 2020) and high intensity drinking (Patrick and Terry-McElrath, 2019). These more risky drinking patterns are associated with short-term cognitive deficits (Jones et al., 2018), however less is known about the long-term alterations as a result of adolescent alcohol exposure.
Preclinical rodent models have identified several long-lasting behavioral impairments associated with adolescent alcohol (ethanol) exposure (Crews et al., 2019), including, but not limited to, increases in anxiety-like behavior (Kyzar et al., 2019; Sakharkar et al., 2019; Varlinskaya et al., 2020), reduced learning, memory, and cognitive flexibility (Gass et al., 2014; Fernandez et al., 2017; Fernandez and Savage, 2017; Rico-Barrio et al., 2019; Macht et al., 2020; Varlinskaya et al., 2020), and also increased risk-taking (Nasrallah et al., 2011; Clark et al., 2012; Kruse et al., 2017). These behavioral impairments are also associated with numerous neurobiological changes such as decreased acetylcholine markers (Vetreno and Crews, 2018; Vetreno et al., 2020; Kipp et al., 2021), increased neural inflammation (Vetreno and Crews, 2012; Vetreno et al., 2017; Vetreno and Crews, 2018; Macht et al., 2020; Vetreno et al., 2020), and dysregulated epigenetic regulators (Kokare et al., 2017; Kyzar et al., 2017; Kyzar et al., 2019).
Our lab has found that adolescent intermittent ethanol (AIE) induces alterations of social behavior in adulthood (Varlinskaya et al., 2014; Varlinskaya et al., 2017; Dannenhoffer et al., 2018; Varlinskaya et al., 2020). These AIE-induced social impairments are sex-specific and evident only in males. These social deficits are reversible through systemic administration of either an oxytocin (OXT) agonist or vasopressin receptor 1b (AVPR1b) antagonist (Dannenhoffer et al., 2018). Furthermore, AIE leads to decreased hypothalamic OXT receptor and increased AVPR1b protein membrane surface expression (Dannenhoffer et al., 2018), specifically in males, findings likely contributing to the social deficits evident in these animals. However, our understanding of the neural mechanisms underlying AIE-induced social deficits is still limited.
Social behavior is regulated by various brain regions that together form the social brain network (Newman, 1999). The social brain network was originally outlined as being composed of the extended amygdala, lateral septum (LS), hypothalamus (HYPO), preoptic area, and midbrain (Newman, 1999). Although evidence supports the involvement of these regions in the regulation of social behavior, more recent evidence also indicates regions outside of this network that contribute to social behavior (Yizhar, 2012; Allsop et al., 2014; Vanderschuren et al., 2016; Ko, 2017). For example, social interaction (approach and contact with a social partner) in males elicits greater firing of neurons in the prelimbic (PrL) cortex (Minami et al., 2017), with similar findings of increased firing of projections from the orbitofrontal cortex (OFC) to basolateral amygdala (BLA) during social approach in males (Li et al., 2021). Furthermore, chemogenetic activation of PrL to BLA circuitry reduced social preference in females (Huang et al., 2020) whereas optogenetic activation of the PrL to nucleus accumbens (NAc) pathway in both sexes leads to decreased social investigation (Murugan et al., 2017). Lesioning the PrL, thus disrupting all afferent and efferent pathways of the PrL, leads to increased social interaction among males (Shah and Treit, 2003) although baclofen and muscimol mediated inhibition of the PFC, including the PrL, infralimbic (IL), and OFC, reduced social play behavior in adolescent males (Van Kerkhof et al., 2013). Taken together, recent evidence suggests that select PFC neural ensembles and their connections to limbic and amygdalar regions play a large role in the regulation of social behaviors.
Given our previous sex-specific findings of AIE-associated alteration of the OXT and AVP systems, it is also possible that regions with known sex differences in these neuropeptide systems are differentially involved in modulation of social interaction in AIE-exposed males relative to controls as well as differences between control conditions in each sex. For example, the LS receives greater AVP innervation from the bed nucleus of the stria terminalis (BNST) (De Vries et al., 1981; De Vries and Buijs, 1983) and HYPO (Qiao et al., 2014) in male than in female rodents. Sex differences in the expression of OXT and AVP receptors are also evident across multiple brain regions, including the PFC, subregions of the BNST, NAc, medial amygdala (MeA), and ventromedial HYPO (Dumais et al., 2013; Dumais and Veenema, 2016). In addition, AVP synthesis in regions such as the BNST has been shown to be different between sexes (Van Leeuwen, Caffe, and De Vries, 1985). However, it is still unknown whether neuronal activation within regions that show sex differences in the OXT and AVP systems in response to a social stimulus differs as a function of adolescent ethanol exposure and sex, and whether these brain regions contribute to AIE-induced social alterations in males.
We hypothesized that ethanol exposure during adolescence may affect the brain in a sex-specific manner, with brain regions implicated in social behavior regulation, especially those that show sex differences, being sensitive to AIE in males. The current study was designed to assess neuronal activation induced by a social stimulus in “social” brain regions of adult male and female cFos-LacZ rats exposed to AIE. In these transgenic rats, expression of β-galactosidase (β-gal) is under the control of a cfos promoter (Figure 1B), and cFos and β-gal proteins are co-expressed in neurons strongly activated by a certain stimulus (Cruz et al., 2015). Therefore, β-gal expression can be used as a proxy for cFos. While cFos expression requires immuno-histochemical techniques, β-gal is detected by X-gal staining–a rapid and highly reproducible histochemical assay. In this study, β-gal expression was assessed in the following regions of interest (ROIs): prelimbic cortex (PrL), infralimbic cortex (IL), ventral and lateral orbitofrontal cortex (vOFC, lOFC), nucleus accumbens core (NAcC), nucleus accumbens shell (NAcSh), lateral septum (LS), dorsal bed nucleus of the stria terminalis (dBNST), anterior hypothalamus (AH), central amygdala (CeA), and basolateral amygdala (BLA).
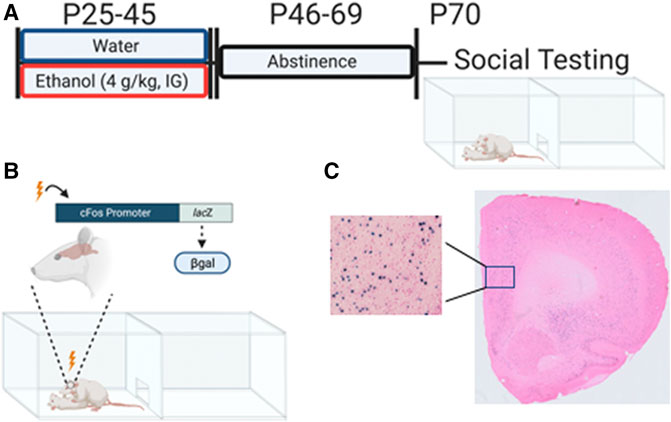
FIGURE 1. Adolescent intermittent ethanol exposure and β-gal expression associated with social interaction in adulthood. (A) Experimental timeline of adolescent intermittent ethanol exposure and social interaction testing in adulthood. (B) cFos-LacZ transgenic model allows for measuring β-galactosidase (β-gal) expression as a proxy for cFos. (C) Representative X-gal enzymatic labeling of active neurons containing (β-gal) following interaction with an unfamiliar social partner. Figure was created using BioRender.com
General Methods
Subjects
Male and female adolescent cFos-LacZ transgenic rats on a Sprague-Dawley background bred and reared in our colony at Binghamton University were used. cFos-LacZ rats have an E. coli derived LacZ gene with expression driven by a cFos promoter allowing for simultaneous transcription of cFos and LacZ. This pairing allows us to use the product of LacZ translation, beta-galactosidase (β-gal), as a proxy for cFos and neuronal activation state (Figure 1B). Litters were culled to 8-10 pups on postnatal day (P)1 and remained with the mother until weaning on P21. Between P21 and 25, offspring were genotyped via tissue punches sent to a commercial source (TransnetYX, Cordova, TN). Offspring were group housed (3-4 per cage) with same-sex littermates with the presence of both wildtype (WT) and LacZ positive (LacZ+) rats. Animals were maintained in a climate controlled (20–22 °C) vivarium on a 12 h on/off light cycle (lights on at 0700), and ad libitum access to food and water. Experiments were conducted in accordance with the National Institutes of Health guidelines for the care and use of animal subjects, and using protocols approved by Binghamton University Institutional Animal Care and Use Committee. All animals in a cage were exposed to water or ethanol, however only LacZ + rats were used in the present study to assess neuronal activation.
Intermittent Ethanol Exposure
Experimental subjects (AIE group) were exposed to ethanol (4 g/kg, 25% v/v in tap water) every other day for a total of 11 exposures between P25 and 45 via intragastric gavage (see Figure 1A). Exposures were conducted by a trained experimenter with intention to limit any possible stress effect of the gavage procedure. Our lab has previously found this exposure results in blood ethanol concentrations ranging from 200 mg/dl at the start (P25) to 125 mg/dl at the end (P45) (Kim et al., 2019). Control rats (Water group) were exposed to an isovolumetric amount of tap water. All exposures occurred between 1,200 and 1,600 h. Following the final exposure, rats were left undisturbed until P70.
Modified Social Interaction
As in our previous studies (Varlinskaya et al., 2014; Dannenhoffer et al., 2018; Kim et al., 2019; Varlinskaya et al., 2020), social behavior was assessed using a modified social interaction test (Figure 1A). Experimental subjects (LacZ +) were weighed, marked for identification, and placed into a plexiglass apparatus (46.0 × 30.3 × 30.5 cm) consisting of two equally sized compartments separated by a wall with an aperture allowing for free movement between the compartments. Pine shavings were placed at the bottom of the apparatus, and 6% hydrogen peroxide was used to clean between testing. Testing took place between 1,200 and 1,600 h in the presence of white noise and under dim lighting (10–15 lux). Rats were placed into the testing apparatus alone for a 30 min habituation period prior to introduction of a non-familiar, same-sex social partner that weighed between 15 and 30 grams less than the experimental animal. Social partners were non-manipulated WT rats. Subjects were left together for 60 min with only the first 10 min video recorded for later scoring by a trained researcher blind to experimental condition.
Two measures sensitive to anxiogenic manipulations, social investigation and social preference, were the two indices of social behavior used in the present study. Social investigation was scored as any instance in which the experimental animal sniffed the social partner. A social preference/avoidance coefficient was calculated using the following formula: (crossovers to the partner—crossovers away from the partner) / (total # of crossovers to and away from the partner) x 100.
Tissue Collection and Enzymatic Histology
At the end of the 60 min social interaction period, experimental rats were injected with sodium pentobarbital and transcardially perfused with 0.1 M phosphate buffered saline (PBS) (200 ml) followed by 4% paraformaldehyde in 0.1 M phosphate buffer (PB) (200 ml). Brains were rapidly extracted and post-fixed in 4% paraformaldehyde for 90 min at 4°C. After postfix, brains were transferred to a 30% sucrose in 0.01 M PBS until saturated, flash-frozen in methyl-butane, and stored at −80°C until processing. In addition to behaviorally tested subjects, a separate cohort of AIE- or water-exposed subjects that did not experience the social context were taken from their home cage environment to obtain control tissue that was processed in a similar fashion. This additional cohort was used to verify whether observed differences in neural activity between water and AIE subjects were due to social interaction or AIE alone.
Using a cryostat (CM 1860, Leica Biosystems, IL, United States), 30 µm coronal slices were collected into an antifreeze solution (30% ethylene glycol, 30% glycerol, 0.05M PBS) and stored at −20 °C. Every 6th non-adjacent section separated by 150 µm of each region of interest was subjected to X-gal enzymatic histology such that at least three bilateral sections per animal were analyzed in accordance with previous literature (Harrison et al., 2008). In short, slices were fixed (0.1 M PB supplemented with 5 mM EGTA) for 15 min followed by two 5-min washes using PB. Slices were then transferred to a staining buffer (0.1 M PB with 2 mM MGCl2, 5 mM potassium ferrocyanide, and 5 mM potassium ferricyanide) containing x-gal stock (5-bromo-4-chloro-3-indolyl-β-d-galactoside in dimethylformamide) in a dilution of 1:50 and placed at 37°C overnight. Slices were then washed twice for 10 min each and mounted on charged microscopy slides (Histobond, VWR, PA, United States). Mounted tissue was dehydrated and counterstained using eosin to aid in visualization of β-gal labeling (for representative image see Figure 1C).
Image Acquisition and Analysis
β-gal stained slices were imaged using an Olympus Research Slide Scanner VS.200 (Olympus, PA, United States) using a 10x magnification objective. Using Olympus ASW software, regions of interest were identified from coronal images and cropped for image analysis. Images were transferred to HALO image analysis platform (Indica Labs, NM, United States) and β-gal containing cells were automatically identified based expression of indigo blue staining and on soma size and roundness. The automated identification of β-gal positive (β-gal+) cells was verified using hand-counted images with over 95% reliability between observation methods.
Regions of Interest
Regions of interest (ROIs) were selected based on the reported contribution of these regions to regulation of social behavior (Newman, 1999; Yizhar, 2012; Allsop et al., 2014; Ko, 2017). We evaluated β-gal expression in 11 ROIs; PrL, IL, vOFC, lOFC, NAcC, NAcSh, LS, dBNST, AH, CeA, and BLA. Coordinates for ROIs can be found in Table 1. Within each region, a 400 μm2 area was extracted, and the number of β-gal + cells were counted and converted to a number/mm2.
Statistical Approach and Analysis
We have previously found AIE-induced sex-specific alterations to social behavior (Varlinskaya et al., 2014; Dannenhoffer et al., 2018; Varlinskaya et al., 2020) therefore all analyses were restricted to within and not between each sex separately. Independent samples t-tests were used to compare social investigation and social preference of water- and AIE-exposed rats within each sex. To explore the relationship of social behavior and neuronal activation (indexed via β-gal + cell count) within a brain region, linear regressions were conducted separately for each adolescent exposure condition in males and females. Correlation analyses were then used to determine the directionality and strength of the relationships. In order to determine whether AIE affected β-gal expression in response to a social stimulus within each ROI, we initially compared the number of β-gal + cells in water- and AIE-exposed socially tested subjects using independent samples t-tests separately in males and females. Once ROIs with significant differences between the two adolescent exposure conditions were identified, we further assessed whether these differences were in fact related to social testing and not an effect of AIE per se. To do so, we used a 2 testing context (home cage, social testing) x 2 adolescent exposure (water, AIE) analyses of variance (ANOVAs). This approach allowed us to identify AIE-associated changes in β-gal expression associated with responding to the social stimulus. Significant main effects and/or interactions were further assessed using Bonferroni’s multiple comparisons tests.
Outliers were identified using values outside 2 standard deviations. One water male, one water female, and one AIE female were removed due to being outliers in behavior and were not included in analyses in β-gal expression. In addition, a low number of outliers were identified within each ROI with final group sizes between 10 - 12.
Results
Social Preference and Investigation
Independent samples t-test of the social preference/avoidance coefficient revealed that social preference of AIE-exposed males was significantly lower than in their water-exposed counterparts (t 23 = 2.90, p < 0.01, Figure 2A). As can be seen in Figure 2C, there was no difference in social preference between females exposed to water and AIE (p > 0.05). However, the independent samples t-tests of social investigation showed no significant difference between adolescent exposure conditions for both males and females (p > 0.05, Figures 2B,D respectively).
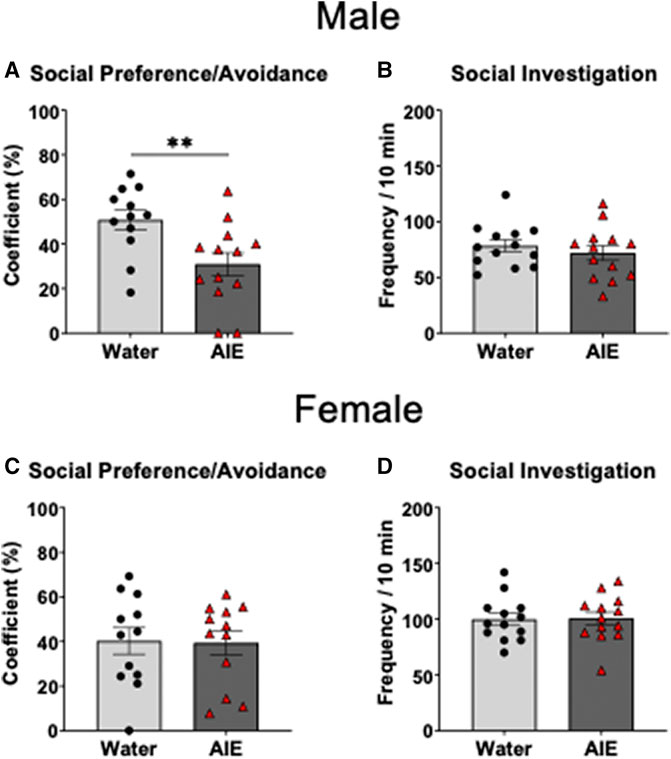
FIGURE 2. Effects of adolescent intermittent ethanol (AIE) exposure on social preference/avoidance and social investigation in adult male (A–B) and female (C–D) cFos-LacZ rats. Social investigation was not altered by AIE, while social preference was decreased in AIE-exposed males relative to water-exposed controls, with no AIE effect evident in females. Significant differences between adolescent exposure conditions within each sex are marked with **p < 0.01.
Relationship Between Social Preference and Neuronal Activation
Linear regressions were performed for social interaction and social preference within each adolescent exposure condition and sex. In water-exposed males, social preference predicted β-gal + cell count in the dBNST (r2 = 0.37, F (1,9) = 5.22, p < 0.05) with a strong positive relationship between these variables, r = 0.61. For AIE-exposed males, linear regressions identified significant relationships between social preference and β-gal + cell count in the dBNST r2 = 0.34, F (1,10) = 5.19, p < 0.05, LS, r2 = 0.35, F (1,10) = 5.32, p < 0.05, and vOFC, r2 = 0.44, F (1,11) = 8.66, p < 0.05. Correlation analyses revealed strong negative relationships between social preference and β-gal expression in all three regions (dBNST, r = −0.58, LS, r = −0.59, vOFC, r = −0.66, see Table 2). No other significant relationships were identified in males (see Table 2).
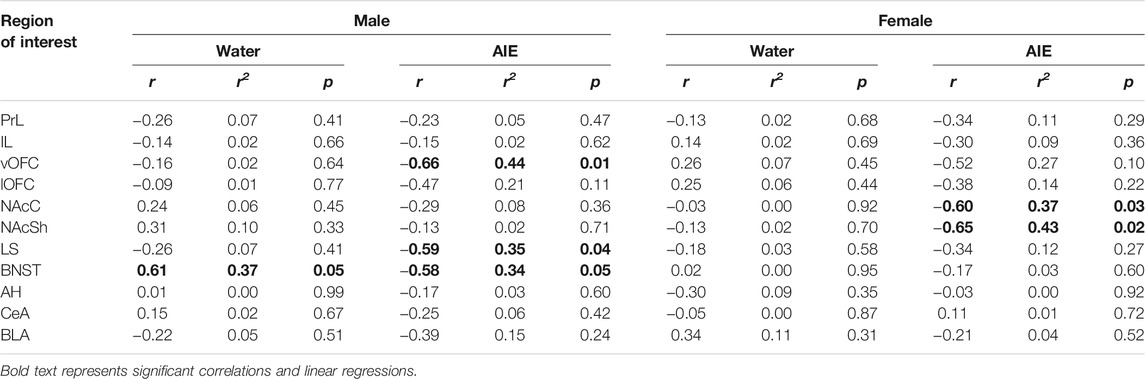
TABLE 2. The relationship between social preference/avoidance coefficient and the number of β-gal positive cells for each region of interest of water and AIE exposed males and females assessed with Pearson correlation and linear regression analyses.
For females, linear regression analyses revealed no significant relationships between social preference and β-gal + cells in the water-exposed females across any of the ROIs analyzed. In AIE-exposed females, regression analyses found relationships between social preference and β-gal expression in both the NAcC (r2 = 0.38, F (1,10) = 5.76, p < 0.05) and NAcSh (r2 = 0.43, F (1,10) = 7.44, p < 0.05), with each being negatively correlated to social preference (NacC, r = −0.60 and NAcSh, r = −0.65). No other relationships were evident across the other ROIs (Table 2).
Relationship Between Social Investigation and Neuronal Activation
For social investigation, linear regression analyses revealed relationships between social investigation and β-gal + neurons for water-exposed males in the PrL (r2 = 0.37, F (1, 10) = 5.76, p < 0.05), IL (r2 = 0.49, F (1,10) = 9.62, p = 0.01), and NAcC (r2 = 0.45, F (1,10) = 8.22, p < 0.05). All three relationships in water-exposed males was positively correlated (PrL r = 0.61, IL r = 0.70, NAcC r = 0.67). For water exposed females, linear regressions revealed a relationship only between investigation and β-gal expression in the vOFC (r2 = 0.50, F (1,9) = 9.18, p = 0.01), with a strong negative correlation between these variables (r = -0.71). In AIE-exposed males and females, no significant linear regressions or correlations between social investigation and β-gal expression in any of the ROI analyzed were identified (see Table 3).
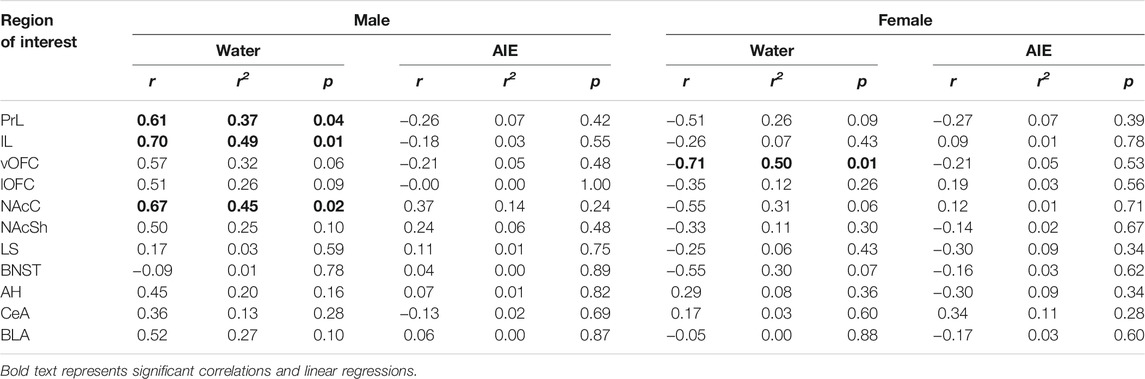
TABLE 3. The relationship between social investigation and the number of β-gal positive cells for each region of interest of water and AIE exposed males and females assessed with Pearson correlation and linear regression analyses.
Neuronal Activation Following Social Stimulus and Home Cage Conditions
To determine ROIs that were different between exposure conditions following social testing, we conducted independent samples t-tests for each ROI within each sex (see Table 4). In males, only the CeA differed, whereas in females both subregions of the OFC (vOFC and lOFC) were affected by AIE. No other ROI for either sex was identified as having differential β-gal labeling between conditions following social interaction (Table 4).
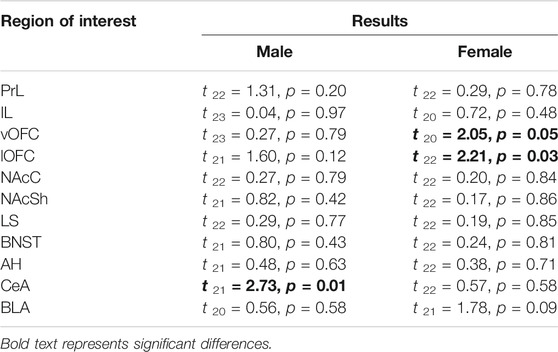
TABLE 4. Independent samples t-test results for social stimulus associated β-gal labeling between adolescent exposure conditions (water; AIE) within each region of interest.
To assess whether our significant findings in β-gal labeling were the result of a social stimulus, we compared home cage subjects and socially tested subjects using two-way ANOVAs. In male CeA β-gal expression, analysis revealed a main effect of adolescent exposure condition (F (1,41) = 11.31, p < 0.01), but not for behavioral testing condition or interaction. As seen in Figure 3A, AIE-exposed males had lower β-gal expression in the CeA in comparison to water-exposed counterparts, regardless of testing condition. Analysis of male OFC β-gal expression revealed a main effect of testing condition in both the vOFC (F (1,42) = 178.00, p < 0.0001) and lOFC (F (1,41) = 89.90, p < 0.0001). Regardless of adolescent exposure condition, socially tested males had greater β-gal labeling in the OFC compared to home-cage subjects (Figures 3B,C).
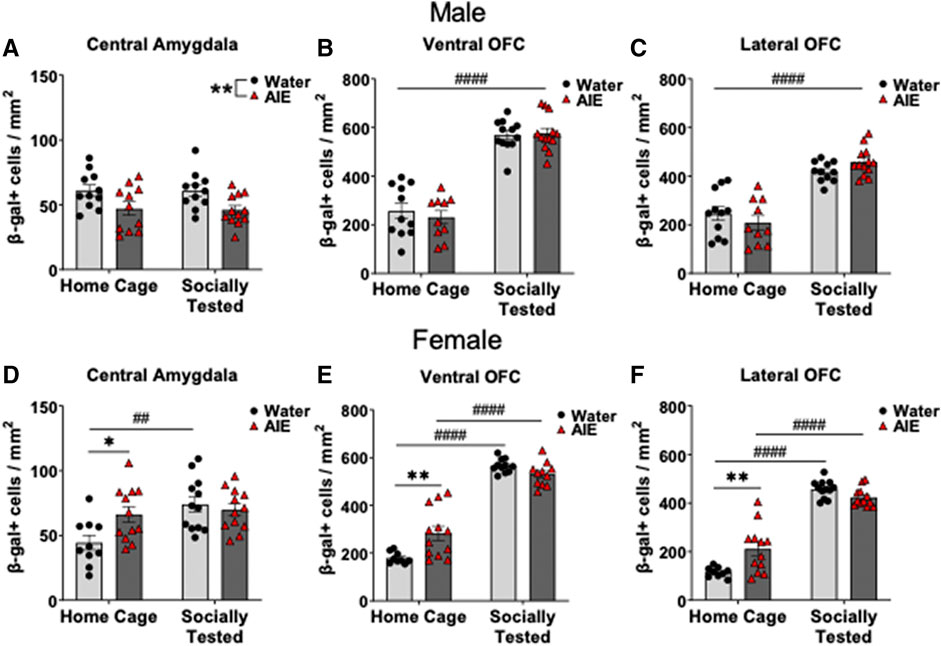
FIGURE 3. Effects of adolescent intermittent ethanol (AIE) exposure on neuronal activation of (A,D) the central amygdala (CeA), (B,E) ventral orbitofrontal cortex (vOFC), and (C,F) lateral orbitofrontal cortex (lOFC) in home cage and socially tested adult males and females. Number of β-gal positive cells in the CeA was significantly lower in AIE-exposed males than in water-exposed controls, with no difference between social testing conditions. In females, CeA β-gal expression was greater for AIE-exposed home cage subjects compared water-exposed controls. Socially tested, water-exposed females had increased CeA β-gal expression compared to home cage water-exposed females. β-gal + cells were greater in both subregions of the OFC in socially tested males, regardless of adolescent exposure. In females, the number of β-gal + cells in the vOFC and lOFC was higher in home cage AIE-exposed females than in their water-exposed counterparts. Additionally, social testing in females led to increases β-gal expression in both OFC subregions, regardless of adolescent exposure. Asterisks denote significant differences between adolescent exposure conditions, *p ≤ 0.05, **p = 0.01 whereas # denote significant differences between testing conditions, ##p < 0.01, ####p < 0.0001.
In females, analysis of CeA β-gal + cells revealed a main effect of testing context (F (1,42) = 8.73, p < 0.01), as well as a testing context by adolescent exposure interaction (F (1,42) = 5.40, p < 0.05). Follow up comparisons of this interaction revealed a difference between adolescent exposure conditions. In home cage controls, AIE-exposed females had more β-gal + cells than water-exposed females (p < 0.05, Figure 3D), whereas socially tested water-exposed females having more β-gal expression than home-cage control females (p < 0.01, Figure 3D).
For the vOFC in females, analysis revealed a main effect of testing context (F (1,39) = 269.90, p < 0.0001), and a testing context by adolescent exposure interaction (F (1,39) = 12.77, p = 0.001). Follow up comparisons identified a significant difference between water- and AIE-exposed females in the home cage condition (p < 0.01), with AIE-exposed females having more β-gal + neurons than their water-exposed counterparts (see Figure 3E). In addition, differences were found between home cage and socially tested subjects’ conditions in both exposure conditions (p < 0.0001), with greater expression of β-gal in socially tested females than in home cage controls (Figure 3E).
Finally, analysis of β-gal labeling in the lOFC also revealed a main effect of testing context (F (1,41) = 249.10, p < 0.0001), and a testing context by adolescent exposure interaction, (F (1,41) = 13.60, p < 0.001). Similar to the vOFC, follow up comparisons in the lOFC revealed a difference between adolescent exposures in the home cage condition (p = 0.001), with AIE-exposed females having a greater number of β-gal + cells than their water-exposed counterparts. Socially tested females in both exposure conditions also had greater β-gal expression relative to home cage subjects (p < 0.0001, see Figure 3F).
Discussion
In this study, we aimed to determine brain regions involved in modulation of social behavior that might contribute to AIE-induced social alterations. Similar to our previous findings in non-transgenic Sprague Dawley rats (Varlinskaya et al., 2014; Varlinskaya et al., 2017; Dannenhoffer et al., 2018; Varlinskaya et al., 2020), AIE led to a sex-specific social impairment in transgenic cFos-LacZ animals, with only males displaying a decreased social preference following adolescent ethanol exposure.
The analyses of relationships between social behaviors and neuronal activation of the ROIs revealed that these relationships are altered by AIE, with these alterations evident in both males and females. Specifically, in water-exposed control males, frequency of social investigation was found to be positively correlated with the number of β-gal + neurons in the PrL, IL, and NAcC, suggesting an involvement of these brain regions in regulation of male social investigation. In contrast, no relationship between activation of these regions and social investigation was evident in AIE-exposed males, suggesting AIE-induced alterations of the neural circuitry involved in modulation of social investigation. Alterations of the mPFC and NAc induced by AIE exposure may limit the ability of these regions to contribute to social investigation. Identified AIE-induced changes in mPFC inhibitory signaling include reduced interneuron intrinsic excitability (Trantham-Davidson et al., 2017), increased inhibitory signaling onto layer V pyramidal neurons (Centanni et al., 2017), and decreases in the number of parvalbumin expressing interneurons (Rice et al., 2019), with these changes to inhibitory signaling in this region potentially contributing to a dysregulated activation associated with social investigation. Additionally, AIE has been found to blunt stimulus evoked dopamine signaling in the NAc (Shnitko et al., 2016) and potentiate kappa opioid receptor function in mediating dopamine release (Spodnick et al., 2020). All these alterations might also contribute to male-specific AIE-associated changes observed in the present study.
Activation of mPFC and NAcC of females did not contribute to social investigation in both adolescent exposure conditions, suggesting sex-specific involvement of these regions in social investigation and AIE-induced deficits. In contrast to males, social investigation in water-exposed females was negatively correlated with vOFC neuronal activation, with no such relationship evident in AIE females, suggesting AIE-associated neuronal alterations in modulation of social behavior in females. As mentioned above, the OFC modulates various social behaviors in males, however the involvement of this region in female social investigation has not been thoroughly studied. It was surprising to find a negative relationship between OFC activity and female social investigation in the current study, given that OFC lesions reduced social play (Van Kerkhof et al., 2013) and increased aggression (Rudebeck et al., 2007). However, the involvement of the OFC in social behaviors has not been evaluated in females, therefore it is likely that regulation of social investigation by the OFC differs between females and males. Sex differences in the OFC are also evident, with females having more myelin basic protein (Bayless and Daniel, 2015; Darling and Daniel, 2019) and greater OFC volume than males (Goldstein et al., 2001; Gur et al., 2002), although the contribution of these sex-differences to social behavior remain unknown.
The relationship between social preference and neuronal activation differed from that of social investigation. In water-exposed control males, β-gal expression in the dBNST was positively correlated with social preference, while AIE reversed this relationship, suggesting AIE-associated alterations in the role of the dBNST in social preference. Indeed, the BNST has been shown to assess social contexts in order to select an appropriate behavioral response, while alterations of socially relevant BNST systems by different insults result in social dysfunctions (Flanigan and Kash, 2020). In addition, social preference was negatively correlated with activation of the vOFC and LS in AIE-exposed males, whereas it was negatively correlated with activation of the NAcC and NAcSh in AIE-exposed females. These findings suggest that AIE produces sex- and region-specific dysfunctions in brain regions implicated in social preference/avoidance. In other words, activation of brain regions that are normally implicated in social behavior (Vanderschuren et al., 2016; Menon et al., 2021) is associated with lower social preference and/or social avoidance following AIE, potentially due to AIE-induced changes in either expression of certain cell types expressing β-gal or alterations to connectivity, and/or neurotransmitter release within these regions compared to control animals. Clearly, more work is needed to understand neural mechanisms of dysfunctions in brain regions implicated in social preference/avoidance sex-specifically affected by AIE. Furthermore, the results of the present study suggest that social investigation and social preference of males have different underlying circuitry, with IL, PrL, and NAcC implicated in regulation of social investigation and BNST contributing to social preference. This finding was not surprising, given that social investigation is a form of exploratory behavior that provides needed information about a novel conspecific, whereas the coefficient of social preference/avoidance reflects approach/avoidance behavior under social circumstances.
When assessing neuronal activation following an interaction with a social partner and under home cage condition in cFos-LacZ rats using β-gal as the proxy for cFos, we found that AIE and water-exposed males had differential activation of the CeA regardless of testing condition, with AIE-exposed males having less β-gal + cells in the CeA than water-exposed controls. It is likely that in males, AIE produces a long-lasting reduction in CeA activity indexed through β-gal expression. This finding is in accordance with others that have shown AIE-associated alterations in the CeA, including decreases expression the activity-regulated cytoskeletal (Arc) gene (Pandey et al., 2015; Kyzar et al., 2019), another early gene marker used to assess neuronal activation (Korb and Finkbeiner, 2011). We cannot preclude, however, that the attenuated activation state of the CeA in AIE males plays a role in AIE-induced social impairments by predisposing these males to avoidance behavior. Future studies are needed to further assess the role of the CeA in social deficits associated with AIE. Although no difference in CeA neuronal activation between exposure conditions was evident in socially tested females, we found that β-gal expression in the CeA under home cage conditions was also affected by AIE. However, in contrast to males in this study and others (Pandey et al., 2015; Kyzar et al., 2019), AIE-exposed females had more β-gal + cells in the CeA than their water-exposed counterparts. Interestingly, social behavior led to a recruitment of neurons in the CeA of control females, however this effect was absent in AIE-exposed females. Although no behavioral difference was observed between female exposure conditions, it is possible that social behavior in each of these exposure groups relies on differential activation of brain regions such as the CeA. These findings add to the growing literature suggesting that AIE leads to sex-specific alterations (Robinson et al., 2021), including opposite effects of AIE on CeA in males and females.
In females, social investigation and social preference did not differ as a function of adolescent exposure, however, the OFC was differentially activated in water- and AIE-exposed females. In, ventral and lateral subregions of the OFC, AIE-exposed females had lower β-gal expression than their water-exposed counterparts, with these differences evident in both home cage controls and socially tested subject, indicating AIE-associated alterations of the OFC in females. Alterations of the OFC following AIE have been recently identified. For example, AIE leads to reduced cholinergic markers and lower acetylcholine release in the OFC during a spontaneous alternation task, although these results were similar between sexes (Kipp et al., 2021). AIE also results in increased neuroimmune signaling in the OFC of males (Vetreno et al., 2013), however this was not assessed in females. Furthermore, AIE has been shown to increase perineuronal net (PNNs) expression in the OFC (Coleman et al., 2014) and given that these nets mediate inhibitory signaling (Bosiacki et al., 2019; Carceller et al., 2020), it is possible that PNN upregulation contributes to the increased neuronal activity among AIE-exposed females evident in the current study. The increases in neuroinflammation and perineuronal nets following AIE have only been studied in males, and more work is needed to determine whether these changes are evident in females and whether they contribute to differences in basal activity of the OFC. In both sexes, however, exposure to a social stimulus enhanced neuronal activation of the vOFC and lOFC, with greater number of β-gal-containing neurons evident in socially tested animals than home cage controls regardless of adolescent exposure condition. This finding suggest that the OFC contributes to social responding of adult males and females, and that the OFC is still responsive to social stimuli after AIE. Evidence supports a role of the OFC in modulating different social behaviors, including social play (Van Kerkhof et al., 2013), aggression (Rudebeck et al., 2007), and dominance (Kolb et al., 2004), whereas no information is available regarding possible involvement of the OFC in regulation of social investigation and/or social preference in males and females.
The present study has certain limitations. Our initial analyses using independent samples t-tests revealed significant differences between socially tested adolescent exposure conditions in the vOFC and lOFC however when using two-way ANOVAs these comparisons failed to reach significance. It is likely that our effect was small in these analyses and our power was too low for identification of these differences with additional analyses. Future work will determine whether these differences in OFC activation between socially tested females can be replicated. Moreover, a lack of differences in activation of a certain brain region does not imply that the same types of neurons were activated by a social stimulus in water-exposed controls and AIE animals. Therefore, our future studies will focus on identification of subtypes of neurons activated by exposure to a social partner in ROIs activation of which was correlated either with social preference or social investigation. In addition, the use of a single immediate early gene limits our understanding of the timeframe in which a neuron was active. The use of either different immediate early genes or different timepoints may be appropriate to further assess the activation of the ROIs in relation to social behavior. Furthermore, we cannot rule out that changes associated with social interaction relative to home cage levels of β-gal expression can be detected in other ROIs examined, since baseline neuronal activation was only assessed in regions with significant differences in activation between socially tested water- and AIE-exposed males and females. Future studies will determine whether AIE induces alterations in basal β-gal expression and whether exposure to a social stimulus produces changes relative to baseline condition differentially in AIE animals and water-exposed controls.
Overall, in males, AIE reduced social preference and affected the relationship between social behaviors’ and neuronal activation of IL, PrL, vOFC, NAcC, BNST, and LS. Importantly, although no behavioral alterations were observed in AIE-exposed females, our findings suggest that the relationship between social behaviors and neuronal activation of vOFC, nAcC, and NAcSh were affected by AIE. Further studies need to be conducted to determine neuronal phenotypes are activated in response to a social stimulus and to test whether these phenotypes differ as a result of AIE exposure in males and females.
Data Availability Statement
The raw data supporting the conclusion of this article will be made available by the authors, without undue reservation.
Ethics Statement
The animal study was reviewed and approved by Binghamton University IACUC.
Author Contributions
TT contributed to the data collection, analysis, and writing of the manuscript. DA contributed to data collection and analysis. EV and DW contributed to the conception and design of the study, data analysis as well as interpretation and writing of the manuscript. All authors assisted in editing and revising the manuscript to its final form, and all provide approval for publication of content.
Funding
Supported by NIH grants U01 AA019972 (Neurobiology of adolescent drinking in adulthood, NADIA), T32 AA025606 (Development and Neuroadaptation in Alcohol and Addictions, DNAA) and F31 AA029300.
Conflict of Interest
The authors declare that the research was conducted in the absence of any commercial or financial relationships that could be construed as a potential conflict of interest.
Publisher’s Note
All claims expressed in this article are solely those of the authors and do not necessarily represent those of their affiliated organizations, or those of the publisher, the editors, and the reviewers. Any product that may be evaluated in this article, or claim that may be made by its manufacturer, is not guaranteed or endorsed by the publisher.
References
Allsop, S. A., Vander Weele, C. M., Wichmann, R., and Tye, K. M. (2014). Optogenetic Insights on the Relationship between Anxiety-Related Behaviors and Social Deficits. Front. Behav. Neurosci. 8, 241. doi:10.3389/fnbeh.2014.00241
Bayless, D. W., and Daniel, J. M. (2015). Sex Differences in Myelin-Associated Protein Levels within and Density of Projections between the Orbital Frontal Cortex and Dorsal Striatum of Adult Rats: Implications for Inhibitory Control. Neuroscience 300, 286–296. doi:10.1016/j.neuroscience.2015.05.029
Bosiacki, M., Gąssowska-Dobrowolska, M., Kojder, K., Fabiańska, M., Jeżewski, D., Gutowska, I., et al. (2019). Perineuronal Nets and Their Role in Synaptic Homeostasis. Int. J. Mol. Sci. 20, 1–22. doi:10.3390/ijms20174108
Carceller, H., Guirado, R., Ripolles-Campos, E., Teruel-Marti, V., and Nacher, J. (2020). Perineuronal Nets Regulate the Inhibitory Perisomatic Input onto Parvalbumin Interneurons and γ Activity in the Prefrontal Cortex. J. Neurosci. 40, 5008–5018. doi:10.1523/jneurosci.0291-20.2020
Centanni, S. W., Burnett, E. J., Trantham-Davidson, H., and Chandler, L. J. (2017). Loss of δ-GABAA Receptor-Mediated Tonic Currents in the Adult Prelimbic Cortex Following Adolescent Alcohol Exposure. Addict. Biol. 22, 616–628. doi:10.1111/adb.12353
Clark, J. J., Nasrallah, N. A., Hart, A. S., Collins, A. L., Bernstein, I. L., and Phillips, P. E. (2012). Altered Risk-Based Decision Making Following Adolescent Alcohol Use Results from an Imbalance in Reinforcement Learning in Rats. PLoS ONE 7, e37357–10. doi:10.1371/journal.pone.0037357
Coleman, L. G., Liu, W., Oguz, I., Styner, M., and Crews, F. T. (2014). Adolescent Binge Ethanol Treatment Alters Adult Brain Regional Volumes, Cortical Extracellular Matrix Protein and Behavioral Flexibility. Pharmacol. Biochem. Behav. 116, 142–151. doi:10.1016/j.pbb.2013.11.021
Crews, F. T., Robinson, D. L., Chandler, L. J., Ehlers, C. L., Mulholland, P. J., Pandey, S. C., et al. (2019). Mechanisms of Persistent Neurobiological Changes Following Adolescent Alcohol Exposure: NADIA Consortium Findings. Alcohol. Clin. Exp. Res. 43, 1806–1822. doi:10.1111/acer.14154
Cruz, F. C., Javier Rubio, F., and Hope, B. T. (2015). Using C-Fos to Study Neuronal Ensembles in Corticostriatal Circuitry of Addiction. Brain Res. 1628 (Pt A), 157–173. doi:10.1016/j.brainres.2014.11.005
Dannenhoffer, C. A., Kim, E. U., Saalfield, J., Werner, D. F., Varlinskaya, E. I., and Spear, L. P. (2018). Oxytocin and Vasopressin Modulation of Social Anxiety Following Adolescent Intermittent Ethanol Exposure. Psychopharmacology (Berl) 235, 3065–3077. doi:10.1007/s00213-018-5003-8
Darling, J. S., and Daniel, J. M. (2019). Pubertal Hormones Mediate Sex Differences in Levels of Myelin Basic Protein in the Orbitofrontal Cortex of Adult Rats. Neuroscience 406, 487–495. doi:10.1016/j.neuroscience.2019.03.041
De Vries, G. J., Buijs, R. M., and Swaab, D. F. (1981). Ontogeny of the Vasopressinergic Neurons of the Suprachiasmatic Nucleus and Their Extrahypothalamic Projections in the Rat Brain-Ppresence of a Sex Difference in the Lateral Septum. Brain Res. 218, 67–78. doi:10.1016/0006-8993(81)90989-6
De Vries, G. J., and Buijs, R. M. (1983). The Origin of the Vasopressinergic and Oxytocinergic Innervation of the Rat Brain with Special Reference to the Lateral Septum. Brain Res. 273, 307–317. doi:10.1016/0006-8993(83)90855-7
Dumais, K. M., Bredewold, R., Mayer, T. E., and Veenema, A. H. (2013). Sex Differences in Oxytocin Receptor Binding in Forebrain Regions: Correlations with Social Interest in Brain Region- and Sex- Specific Ways. Horm. Behav. 64 (4), 693–701. doi:10.1016/j.yhbeh.2013.08.012
Dumais, K. M., and Veenema, A. H. (2016). Vasopressin and Oxytocin Receptor Systems in the Brain: Sex Differences and Sex-specific Regulation of Social Behavior. Front. Neuroendocrinol 40, 1–23. doi:10.1016/j.yfrne.2015.04.003
Fernandez, G. M., Lew, B. J., Vedder, L. C., and Savage, L. M. (2017). Chronic Intermittent Ethanol Exposure Leads to Alterations in Brain-Derived Neurotrophic Factor within the Frontal Cortex and Impaired Behavioral Flexibility in Both Adolescent and Adult Rats. Neuroscience 348, 324–334. doi:10.1016/j.neuroscience.2017.02.045
Fernandez, G. M., and Savage, L. M. (2017). Adolescent Binge Ethanol Exposure Alters Specific Forebrain Cholinergic Cell Populations and Leads to Selective Functional Deficits in the Prefrontal Cortex. Neuroscience 361, 129–143. doi:10.1016/j.neuroscience.2017.08.013
Flanigan, M. E., and Kash, T. L. (2020). Coordination of Social Behaviors by the Bed Nucleus of the Stria Terminalis. Eur. J. Neurosci. 2020. doi:10.1111/ejn.14991
Gass, J. T., Glen, W. B., Mcgonigal, J. T., Trantham-Davidson, H., Lopez, M. F., Randall, P. K., et al. (2014). Adolescent Alcohol Exposure Reduces Behavioral Flexibility, Promotes Disinhibition, and Increases Resistance to Extinction of Ethanol Self-Administration in Adulthood. Neuropsychopharmacology 39, 2570–2583. doi:10.1038/npp.2014.109
Goldstein, J. M., Seidman, L. J., Horton, N. J., Makris, N., Kennedy, D. N., Caviness, V. S., et al. (2001). Normal Sexual Dimorphism of the Adult Human Brain Assessed by In Vivo Magnetic Resonance Imaging. Cereb. Cortex 11, 490–497. doi:10.1093/cercor/11.6.490
Gur, R. C., Gunning-Dixon, F., Bilker, W. B., and Gur, R. E. (2002). Sex Differences in Temporo-Limbic and Frontal Brain Volumes of Healthy Adults. Cereb. Cortex 12, 998–1003. doi:10.1093/cercor/12.9.998
Huang, W. C., Zucca, A., Levy, J., and Page, D. T. (2020). Social Behavior Is Modulated by Valence-Encoding mPFC-Amygdala Sub-circuitry. Cell Rep 32 (2), 107899. doi:10.1016/j.celrep.2020.107899
Jones, S. A., Lueras, J. M., and Nagel, B. J. (2018). Effects of Binge Drinking on the Developing Brain. Alcohol. Res. 39, 87–96.
Kim, E. U., Varlinskaya, E. I., Dannenhoffer, C. A., and Spear, L. P. (2019). Adolescent Intermittent Ethanol Exposure: Effects on Pubertal Development, novelty Seeking, and Social Interaction in Adulthood. Alcohol 75, 19–29. doi:10.1016/j.alcohol.2018.05.002
Kipp, B. T., Nunes, P. T., Galaj, E., Hitchcock, B., Nasra, T., Poynor, K. R., et al. (2021). Adolescent Ethanol Exposure Alters Cholinergic Function and Apical Dendritic Branching within the Orbital Frontal Cortex. Neuroscience 473, 52–65. doi:10.1016/j.neuroscience.2021.08.014
Ko, J. (2017). Neuroanatomical Substrates of Rodent Social Behavior: The Medial Prefrontal Cortex and its Projection Patterns. Front. Neural Circuits 11, 41–16. doi:10.3389/fncir.2017.00041
Kokare, D. M., Kyzar, E. J., Zhang, H., Sakharkar, A. J., and Pandey, S. C. (2017). Adolescent Alcohol Exposure-Induced Changes in Alpha-Melanocyte Stimulating Hormone and Neuropeptide Y Pathways via Histone Acetylation in the Brain during Adulthood. Int. J. Neuropsychopharmacol. 20, 758–768. doi:10.1093/ijnp/pyx041
Kolb, B., Pellis, S., and Robinson, T. E. (2004). Plasticity and Functions of the Orbital Frontal Cortex. Brain Cogn. 55 (1), 104–115. doi:10.1016/s0278-2626(03)00278-1
Korb, E., and Finkbeiner, S. (2011). Arc in Synaptic Plasticity: from Gene to Behavior. Trends Neurosci. 34 (11), 591–598. doi:10.1016/j.tins.2011.08.007
Kruse, L. C., Schindler, A. G., Williams, R. G., Weber, S. J., and Clark, J. J. (2017). Maladaptive Decision Making in Adults with a History of Adolescent Alcohol Use, in a Preclinical Model, Is Attributable to the Compromised Assignment of Incentive Value during Stimulus-Reward Learning. Front. Behav. Neurosci. 11, 134. doi:10.3389/fnbeh.2017.00134
Kyzar, E. J., Zhang, H., and Pandey, S. C. (2019). Adolescent Alcohol Exposure Epigenetically Suppresses Amygdala Arc Enhancer RNA Expression to Confer Adult Anxiety Susceptibility. Biol. Psychiatry 85, 904–914. doi:10.1016/j.biopsych.2018.12.021
Kyzar, E. J., Zhang, H., Sakharkar, A. J., and Pandey, S. C. (2017). Adolescent Alcohol Exposure Alters Lysine Demethylase 1 (LSD1) Expression and Histone Methylation in the Amygdala during Adulthood. Addict. Biol. 22, 1191–1204. doi:10.1111/adb.12404
Larsen, B., and Luna, B. (2018). Adolescence as a Neurobiological Critical Period for the Development of Higher-Order Cognition. Neurosci. Biobehav Rev. 94, 179–195. doi:10.1016/j.neubiorev.2018.09.005
Li, A., Jing, D., Dellarco, D. V., Hall, B. S., Yang, R., Heilberg, R. T., et al. (2021). Role of BDNF in the Development of an OFC-Amygdala Circuit Regulating Sociability in Mouse and Human. Mol. Psychiatry 26 (3), 955–973. doi:10.1038/s41380-019-0422-4
Macht, V., Elchert, N., and Crews, F. (2020). Adolescent Alcohol Exposure Produces Protracted Cognitive-Behavioral Impairments in Adult Male and Female Rats. Brain Sci. 10 (11). doi:10.3390/brainsci10110785
Menon, R., Süß, T., Oliveira, V. E. d. M., Neumann, I. D., and Bludau, A. (2022). Neurobiology of the Lateral Septum: Regulation of Social Behavior. Trends Neurosciences 45, 27–40. doi:10.1016/j.tins.2021.10.010
Minami, C., Shimizu, T., and Mitani, A. (2017). Neural Activity in the Prelimbic and Infralimbic Cortices of Freely Moving Rats during Social Interaction: Effect of Isolation Rearing. PLoS ONE 12, e0176740–22. doi:10.1371/journal.pone.0176740
Morean, M. E., L'Insalata, A., Butler, E. R., McKee, A., and Krishnan-Sarin, S. (2018). Age at Drinking Onset, Age at First Intoxication, and Delay to First Intoxication: Assessing the Concurrent Validity of Measures of Drinking Initiation with Alcohol Use and Related Problems. Addict. Behav. 79, 195–200. doi:10.1016/j.addbeh.2017.12.017
Murugan, M., Jang, H. J., Park, M., Miller, E. M., Cox, J., Taliaferro, J. P., et al. (2017). Combined Social and Spatial Coding in a Descending Projection from the Prefrontal Cortex. Cell 171, 1663–e16. e1616. doi:10.1016/j.cell.2017.11.002
Nasrallah, N. A., Clark, J. J., Collins, A. L., Akers, C. A., Phillips, P. E., and Bernstein, I. L. (2011). Risk Preference Following Adolescent Alcohol Use Is Associated with Corrupted Encoding of Costs but Not Rewards by Mesolimbic Dopamine. Proc. Natl. Acad. Sci. U S A. 108, 5466–5471. doi:10.1073/pnas.1017732108
Newman, S. W. (1999). The Medial Extended Amygdala in Male Reproductive Behavior. A Node in the Mammalian Social Behavior Network. Ann. N. Y Acad. Sci. 877, 242–257. doi:10.1111/j.1749-6632.1999.tb09271.x
Pandey, S. C., Sakharkar, A. J., Tang, L., and Zhang, H. (2015). Potential Role of Adolescent Alcohol Exposure-Induced Amygdaloid Histone Modifications in Anxiety and Alcohol Intake during Adulthood. Neurobiol. Dis. 82, 607–619. doi:10.1016/j.nbd.2015.03.019
Patrick, M. E., and Terry-McElrath, Y. M. (2019). Prevalence of High-Intensity Drinking from Adolescence through Young Adulthood: National Data from 2016-2017. Subst. Abuse 13, 1178221818822976–10. doi:10.1177/1178221818822976
Qiao, X., Yan, Y., Wu, R., Tai, F., Hao, P., Cao, Y., et al. (2014). Sociality and Oxytocin and Vasopressin in the Brain of Male and Female Dominant and Subordinate Mandarin Voles. J. Comp. Physiol. A. Neuroethol Sens Neural Behav. Physiol. 200 (2), 149–59. doi:10.1007/s00359-013-0870-2
Rice, J., Coutellier, L., Weiner, J. L., and Gu, C. (2019). Region-specific Interneuron Demyelination and Heightened Anxiety-like Behavior Induced by Adolescent Binge Alcohol Treatment. Acta Neuropathol. Commun. 7, 173. doi:10.1186/s40478-019-0829-9
Rico-Barrio, I., Peñasco, S., Puente, N., Ramos, A., Fontaine, C. J., Reguero, L., et al. (2019). Cognitive and Neurobehavioral Benefits of an Enriched Environment on Young Adult Mice after Chronic Ethanol Consumption during Adolescence. Addict. Biol. 24, 969–980. doi:10.1111/adb.12667
Robinson, D. L., Amodeo, L. R., Chandler, L. J., Crews, F. T., Ehlers, C. L., Gómez-A, A., et al. (2021). The Role of Sex in the Persistent Effects of Adolescent Alcohol Exposure on Behavior and Neurobiology in Rodents. Int. Rev. Neurobiol. 160, 305–340. doi:10.1016/bs.irn.2021.07.007
Rudebeck, P. H., Walton, M. E., Millette, B. H., Shirley, E., Rushworth, M. F., and Bannerman, D. M. (2007). Distinct Contributions of Frontal Areas to Emotion and Social Behaviour in the Rat. Eur. J. Neurosci. 26 (8), 2315–26. doi:10.1111/j.1460-9568.2007.05844.x
Sakharkar, A. J., Kyzar, E. J., Gavin, D. P., Zhang, H., Chen, Y., Krishnan, H. R., et al. (2019). Altered Amygdala DNA Methylation Mechanisms after Adolescent Alcohol Exposure Contribute to Adult Anxiety and Alcohol Drinking. Neuropharmacology 157, 107679. doi:10.1016/j.neuropharm.2019.107679
Shah, A. A., and Treit, D. (2003). Excitotoxic Lesions of the Medial Prefrontal Cortex Attenuate Fear Responses in the Elevated-Plus Maze, Social Interaction and Shock Probe Burying Tests. Brain Res. 969, 183–94. doi:10.1016/S0006-8993(03)02299-6
Shnitko, T. A., Spear, L. P., and Robinson, D. L. (2016). Adolescent Binge-like Alcohol Alters Sensitivity to Acute Alcohol Effects on Dopamine Release in the Nucleus Accumbens of Adult Rats. Psychopharmacology (Berl) 233 (3), 361–71. doi:10.1007/s00213-015-4106-8
Spodnick, M. B., Amirault, R. T., Towner, T. T., Varlinskaya, E. I., Spear, L. P., and Karkhanis, A. N. (2020). Adolescent Intermittent Ethanol Exposure Effects on Kappa Opioid Receptor Mediated Dopamine Transmission: Sex and Age of Exposure Matter. Brain Sci. 10 (8). doi:10.3390/brainsci10080472
Trantham-Davidson, H., Centanni, S. W., Garr, S. C., New, N. N., Mulholland, P. J., Gass, J. T., et al. (2017). Binge-Like Alcohol Exposure during Adolescence Disrupts Dopaminergic Neurotransmission in the Adult Prelimbic Cortex. Neuropsychopharmacology 42, 1024–1036. doi:10.1038/npp.2016.190
Van Kerkhof, L. W., Damsteegt, R., Trezza, V., Voorn, P., and Vanderschuren, L. J. (2013). Social Play Behavior in Adolescent Rats Is Mediated by Functional Activity in Medial Prefrontal Cortex and Striatum. Neuropsychopharmacology 38, 1899–909. doi:10.1038/npp.2013.83
Vanderschuren, L. J., Achterberg, E. J., and Trezza, V. (2016). The Neurobiology of Social Play and its Rewarding Value in Rats. Neurosci. Biobehav Rev. 70, 86–105. doi:10.1016/j.neubiorev.2016.07.025
Varlinskaya, E. I., Hosová, D., Towner, T., Werner, D. F., and Spear, L. P. (2020). Effects of Chronic Intermittent Ethanol Exposure during Early and Late Adolescence on Anxiety-like Behaviors and Behavioral Flexibility in Adulthood. Behav. Brain Res. 378, 112292. doi:10.1016/j.bbr.2019.112292
Varlinskaya, E. I., Kim, E. U., and Spear, L. P. (2017). Chronic Intermittent Ethanol Exposure during Adolescence: Effects on Stress-Induced Social Alterations and Social Drinking in Adulthood. Brain Res. 1654, 145–156. doi:10.1016/j.brainres.2016.03.050
Varlinskaya, E. I., Truxell, E., and Spear, L. P. (2014). Chronic Intermittent Ethanol Exposure during Adolescence: Effects on Social Behavior and Ethanol Sensitivity in Adulthood. Alcohol 48, 433–44. doi:10.1016/j.alcohol.2014.01.012
Vetreno, R. P., Bohnsack, J. P., Kusumo, H., Liu, W., Pandey, S. C., and Crews, F. T. (2020). Neuroimmune and Epigenetic Involvement in Adolescent Binge Ethanol-Induced Loss of Basal Forebrain Cholinergic Neurons: Restoration with Voluntary Exercise. Addict. Biol. 25, e12731–14. doi:10.1111/adb.12731
Vetreno, R. P., and Crews, F. T. (2012). Adolescent Binge Drinking Increases Expression of the Danger Signal Receptor Agonist HMGB1 and Toll-like Receptors in the Adult Prefrontal Cortex. Neuroscience 226, 475–88. doi:10.1016/j.neuroscience.2012.08.046
Vetreno, R. P., and Crews, F. T. (2018). Adolescent Binge Ethanol-Induced Loss of Basal Forebrain Cholinergic Neurons and Neuroimmune Activation Are Prevented by Exercise and Indomethacin. PLoS ONE 13, e0204500–22. doi:10.1371/journal.pone.0204500
Vetreno, R. P., Patel, Y., Patel, U., Walter, T. J., and Crews, F. T. (2017). Adolescent Intermittent Ethanol Reduces Serotonin Expression in the Adult Raphe Nucleus and Upregulates Innate Immune Expression that Is Prevented by Exercise. Brain Behav. Immun. 60, 333–345. doi:10.1016/j.bbi.2016.09.018
Vetreno, R. P., Qin, L., and Crews, F. T. (2013). Increased Receptor for Advanced Glycation End Product Expression in the Human Alcoholic Prefrontal Cortex Is Linked to Adolescent Drinking. Neurobiol. Dis. 59, 52–62. doi:10.1016/j.nbd.2013.07.002
Keywords: adolescence, ethanol exposure, social behavior, sex differences, cfos, βgalactosidase
Citation: Towner TT, Applegate DT, Varlinskaya EI and Werner DF (2022) Impact of Adolescent Intermittent Ethanol Exposure on Social Investigation, Social Preference, and Neuronal Activation in cFos-LacZ Male and Female Rats. Front. Pharmacol. 13:841657. doi: 10.3389/fphar.2022.841657
Received: 22 December 2021; Accepted: 28 February 2022;
Published: 23 March 2022.
Edited by:
Susan F Tapert, University of California, San Diego, United StatesReviewed by:
Jacqueline M Barker, Drexel University, United StatesLeslie Amodeo, California State University, United States
Copyright © 2022 Towner, Applegate, Varlinskaya and Werner. This is an open-access article distributed under the terms of the Creative Commons Attribution License (CC BY). The use, distribution or reproduction in other forums is permitted, provided the original author(s) and the copyright owner(s) are credited and that the original publication in this journal is cited, in accordance with accepted academic practice. No use, distribution or reproduction is permitted which does not comply with these terms.
*Correspondence: David F. Werner, ZHdlcm5lckBiaW5naGFtdG9uLmVkdQ==