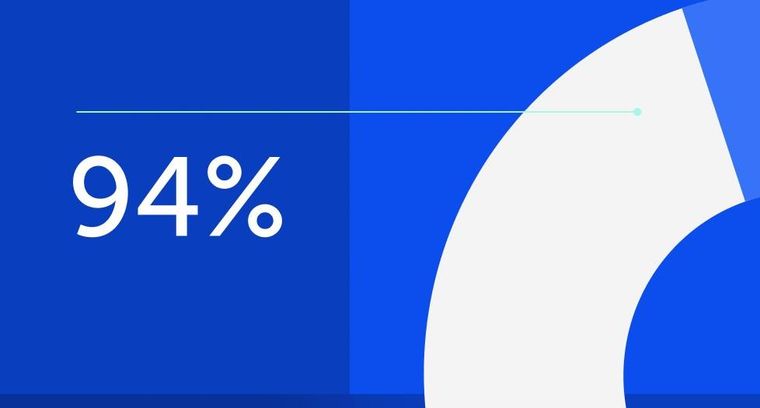
94% of researchers rate our articles as excellent or good
Learn more about the work of our research integrity team to safeguard the quality of each article we publish.
Find out more
REVIEW article
Front. Pharmacol., 13 June 2022
Sec. Pharmacology of Infectious Diseases
Volume 13 - 2022 | https://doi.org/10.3389/fphar.2022.840323
The role of bacterial biofilms in chronic and recalcitrant diseases is widely appreciated, and the treatment of biofilm infection is an increasingly important area of research. Chronic rhinosinusitis (CRS) is a complex disease associated with sinonasal dysbiosis and the presence of bacterial biofilms. While most biofilm-related diseases are associated with highly persistent but relatively less severe inflammation, the presence of biofilms in CRS is associated with greater severity of inflammation and recalcitrance despite appropriate treatment. Oral antibiotics are commonly used to treat CRS but they are often ineffective, due to poor penetration of the sinonasal mucosa and the inherently antibiotic resistant nature of bacteria in biofilms. Topical non-antibiotic antibiofilm agents may prove more effective, but few such agents are available for sinonasal application. We review compounds with antibiofilm activity that may be useful for treating biofilm-associated CRS, including halogen-based compounds, quaternary ammonium compounds and derivatives, biguanides, antimicrobial peptides, chelating agents and natural products. These include preparations that are currently available and those still in development. For each compound, antibiofilm efficacy, mechanism of action, and toxicity as it relates to sinonasal application are summarised. We highlight the antibiofilm agents that we believe hold the greatest promise for the treatment of biofilm-associated CRS in order to inform future research on the management of this difficult condition.
Chronic rhinosinusitis (CRS) is a condition characterised by inflammation of the paranasal sinus mucosa. The aetiology is multifactorial and is likely to reflect complex interactions between anatomical factors, regional microbial community composition and the host immune response (Hoggard et al., 2017a; Fokkens et al., 2020; Orlandi et al., 2021). Sinus irrigation and topical corticosteroids are currently the first-line of treatment, with the addition of systemic antibiotic and corticosteroid therapy as indicated. When appropriate medical therapy does not yield satisfactory symptom improvement, patients may be recommended functional endoscopic sinus surgery (FESS). Even with optimal medical and surgical management, some CRS cases are recalcitrant (Fokkens et al., 2020; Orlandi et al., 2021).
The sinonasal microbiota consists of bacteria (Wagner Mackenzie et al., 2017), fungi (Hoggard et al., 2019), viruses (Hoggard et al., 2017b) and archaea (Wagner Mackenzie et al., 2020). Of these, bacteria form the largest proportion of the microbial community. Perturbation in the composition of the bacterial community (dysbiosis), increased bacterial load (Hoggard et al., 2017a) and increased prevalence of bacterial biofilms are all associated with CRS (Chen et al., 2012). However, no currently available treatment modality optimally and reliably modulates the sinonasal microbiota.
There is significant evidence that suggests bacterial biofilms play a pathogenic role in CRS (Vickery et al., 2019) (Figure 1). In contrast to planktonic bacteria, biofilm communities exist in a framework of extracellular DNA, polysaccharides and proteins (Verderosa et al., 2019). This extracellular matrix (ECM) creates a niche that protects the biofilm from the external environment. The physical conditions within the biofilm (hypoxia and low pH) may inhibit antibiotic action. Biofilms are therefore innately antibiotic resistant, and this has been demonstrated in vitro by survival after exposure to as much as one thousand times the minimum inhibitory concentration (MIC) of antibiotics for planktonic forms of the same strain (Stewart and Costerton, 2001).
FIGURE 1. The role of the biofilm in CRS. (A) A coronal section of a maxillary sinus showing inflamed, oedematous epithelium and a polyp. Mucopus and crusting is seen in the sinus lumen. (B) Magnified view of pseudostratified ciliated columnar (respiratory) epithelium shown in (A), with ciliary destruction in the region of the biofilm. (C) Magnified schematic view of a bacterial biofilm from (B) on the surface of the sinus epithelium. Planktonic bacteria are being released from the surface of the mature biofilm. Fungi and viruses are also present as members of the sinonasal microbiota and the immune cells and cytokines of type I and type II CRS endotypes are depicted. Mac: Macrophage, Eo: Eosinophil, Th1: T helper 1 cell, Th2: T helper 2 cell, IL-4: Interleukin 4, IL-5: Interleukin 5, IL-13: Interleukin 13, IFN-γ: Interferon-γ.
Biofilms attached to the surface of the sinonasal epithelium are associated with ciliary destruction and mucus stasis (Foreman et al., 2011). Within the biofilm, a reservoir of persister cells exist (Berditsch et al., 2019), which may repopulate the biofilm to enable ongoing infection and immune provocation even after bacterial cells near the surface are compromised. The prevalence of sinonasal biofilms in those with CRS may be as high as around 75% although estimates vary (Singhal et al., 2011; Fokkens et al., 2020). Several biofilm-forming bacterial species have been identified in this setting including Staphylococcus aureus, Pseudomonas aeruginosa and Haemophilus influenzae, in single-organism and polymicrobial biofilms (Singhal et al., 2011). S. aureus biofilms in particular are associated with more severe disease and recalcitrance following FESS (Singhal et al., 2011; Vickery et al., 2019). This may be due to the release of superantigens and direct activation of TLR-2 receptors, both of which favour a type 2 immune response (Vickery et al., 2019; Orlandi et al., 2021).
Therapies that modulate the immune response component of CRS, including monoclonal antibodies such as dupilumab, omalizumab, benralizumab and mepolizumab, have been found to be effective in the treatment of some CRS phenotypes. Although effective, these treatments are expensive and are currently recommended for severely recalcitrant cases (Fokkens et al., 2020). However, few therapies that specifically target the microbial imbalance observed in CRS have been proven to be beneficial. Only a small number of randomised controlled trials (RCTs) have shown that macrolide antibiotics may have some clinical efficacy, at least for some subgroups of CRS patients. It may be significant that macrolide antibiotics also have some anti-inflammatory action (Orlandi et al., 2021). The level of antibiotic concentration in nasal mucus is substantially lower than in serum, and this may also reduce their effectiveness in CRS (Siu et al., 2020). Current international guidelines do not recommend the use of topical antibiotics as they have not been found to confer clinical benefit (Fokkens et al., 2020; Orlandi et al., 2021). The increased local concentration that can be achieved with the topical administration of antibiotics may be offset by short residency times in the sinonasal mucus.
There is a clear unmet need to develop low risk, topical treatments targeting the bacterial biofilms involved in CRS. The ideal antibiofilm agent would effectively eradicate biofilms without local or systemic toxicity, and its topical application would be simple and tolerable for the patient (Foreman et al., 2011).
In this review, agents with antibiofilm activity that may be useful in the treatment of CRS when applied topically will be evaluated. Those that hold the most promise for clinical use will be highlighted, and their mechanisms of action, efficacy, and toxicity outlined. Many agents have been found to be safe to apply to other mucosal surfaces, and so could potentially be adopted for CRS treatment. In this review, the topical agents have been grouped by class into halogen based products, quaternary ammonium compounds and their derivatives, biguanides, antimicrobial peptides, chelating agents, and natural products. These compounds are summarised by sites of action in Figure 2, and by methods of testing in Table 1.
FIGURE 2. Sites of action of the antibiofilm agents reviewed. (A) a biofilm, comprised of bacterial cells and extracellular matrix (B) agents active on the biofilm matrix (C) agents active on bacterial cells, with specific sites of action noted *: Secondary effect, by inducing oxidative damage $: By infection, leading to cell lysis.
TABLE 1. Summary of antibiofilm agents. The mechanisms of action, methods used to quantify the effect of treatment on microbial species and methods of toxicity testing are outlined.
Examples of halogen based compounds include povidone-iodine and sodium hypochlorite/hypochlorous acid. These are redox-active substances that cause the denaturation of multiple bacterial components and lead to rapid cell death.
Povidone-iodine (PVP-I) was developed in the mid-20th century when iodine was bound to the polymer polyvinylpyrrolidone (povidone) (Shelanski et al., 1956). Iodine had been used for antisepsis for over a century in Lugol’s solution, but this was painful when applied to wounds or mucosa and caused tissue staining. Iodophores such as povidone largely mitigate these issues, as well as stabilising iodine in solution and acting as a reservoir to maintain the concentration of available iodine as it is consumed during antimicrobial action (Shelanski et al., 1956; Cooper, 2007; Bigliardi et al., 2017).
Iodine can rapidly penetrate microbial cell membranes and acts by the oxidation of proteins, nucleotides, and membrane constituents (Schreier et al., 1997). To date, no instances of bacterial resistance have been identified, owing in part to the multiplicity of cellular structures that it targets (Barreto et al., 2020). It is highly effective in vitro against S. aureus and P. aeruginosa biofilms. Multiple studies have demonstrated complete or near-complete eradication (generally representing around 6 log10 reductions in viable cell counts) with exposures of between 3 min and 24 h and concentrations as low as 0.25%, although a longer time to eradication is associated with lower concentrations (Lefebvre et al., 2016; Hoekstra et al., 2017; Capriotti et al., 2018; Johani et al., 2018; Herruzo and Herruzo, 2020; Premkumar et al., 2021; Lux et al., 2022). Povidone iodine is inactivated by organic material, however even when mixed in equal parts with nasal secretions it retains much of its antimicrobial activity (Hill and Casewell, 2000).
PVP-I is irritating and has a strong odour and taste when applied nasally (Jeronimo et al., 2020), limiting the concentrations with which it can be used in this setting. There is evidence of ciliotoxicity in vitro; when exposed to 5 and 10% PVP-I for 1 minute, cilia cease beating in cultured nasal epithelial cells (Kim et al., 2015). This result was corroborated at these concentrations in an ex vivo study using nasal epithelial cells from healthy volunteers, although no effect on ciliary beat frequency was seen at concentrations of 1.25% or lower (Reimer et al., 2002). Likewise, a prospective cohort study using 0.08% PVP-I in sinus rinses every second day for 7 weeks found no change in saccharin transit time compared to control. Further, in these patients with recalcitrant CRS, significant improvements in modified Lund-Kennedy endoscopic and SNOT-22 symptom scores were seen (Panchmatia et al., 2019). A subsequent RCT using 0.1% PVP-I rinses twice daily for 3 months following primary FESS demonstrated non-superiority of PVP-I over saline for the same outcomes (Wu et al., 2021). In both studies, PVP-I rinses were relatively dilute and the frequency of application allowed only brief contact time with the nasal mucosa. Further studies with more frequent application of more concentrated PVP-I may be beneficial.
Nasodine® (Firebrick Pharma, Melbourne, VIC, Australia) is a new product containing 0.5% PVP-I that has not yet been released to the market. It has been formulated specifically to ameliorate the problems experienced after the intranasal application of PVP-I and has an excellent toxicity profile in vitro (Ramezanpour et al., 2020). No increase in paracellular protein permeability nor decrease in cell viability or ciliary beat frequency was observed in cultures of nasal epithelial cells when exposed for 30 min. However, trans-epithelial electrical resistance increased suggesting some degree of epithelial barrier compromise. Another group has developed a nasal preparation of PVP-I, sodium chloride and a perfume (Jeronimo et al., 2020), and found no evidence of cytotoxicity after a 24 h incubation on cultured human nasal epithelial cells. However, this was tested at very low concentrations (0.009 and 0.0045%). These products represent promising new potential treatments for sinonasal biofilms.
Hypochlorous acid is an endogenous agent produced by neutrophils for killing bacteria. Sodium hypochlorite and hypochlorous acid are used in commercially available topical antiseptics (Severing et al., 2019). Electrochemically activated solutions, also known as superoxidised solutions or electrolysed water, are a heterogeneous group of solutions that contain sodium hypochlorite and hypochlorous acid, among other chemical compounds, as their main active constituents (Thorn et al., 2012; Severing et al., 2019). These solutions are available in nasal sprays such as Nasocyn (Te Arai BioFarma, Auckland, NZ). Once applied, they cause oxidative denaturation of proteins, the cell wall and membranes and DNA (Severing et al., 2019; Herruzo and Herruzo, 2020).
The evidence supporting their antibiofilm efficacy is mixed. Tests of commercial electrolysed water preparations have variably demonstrated complete biofilm eradication in vitro after exposures between 15 min and 24 h, or no effect even after 24 h (Johani et al., 2018; Krasowski et al., 2021; Lux et al., 2022). Tests of sodium hypochlorite and hypochlorous acid solutions have yielded more promising results. Eradication of S. aureus biofilms after exposures as short as 2 min, and P. aeruginosa in as short as 5 min, has been observed using 0.08% sodium hypochlorite (Röhner et al., 2020). Likewise, 0.15% hypochlorous acid has been seen to cause a 6 log10 reduction in viable cells after 5 min exposure (Herruzo and Herruzo, 2020). Preparations with antimicrobial efficacy are also more likely to exhibit cytotoxicity. Sodium hypochlorite at this concentration is toxic to cultured human fibroblasts and keratinocytes and ex-vivo human chondrocytes (Severing et al., 2019; Röhner et al., 2020).
These cationic surfactants have been used as biocides for almost a century. Quaternary ammonium compounds comprise a positively charged nitrogen at their head and a non-polar n-alkyl tail of variable length. Their structure results in attraction toward the negatively charged outer surface of the bacterium and integration into the cell membrane, leading to destabilization and leakage of cytoplasmic contents and subsequently cell death (Gilbert and Moore, 2005).
Benzalkonium chloride (BAC) is a commonly used preservative in nasal and ophthalmic preparations. It is effective against both planktonic bacteria and biofilms (Campanac et al., 2002). The time taken for biofilm eradication reflects differences in extracellular matrix composition, which influences the rate at which BAC can penetrate the biofilm (Bridier et al., 2011). The concentration required for biofilm eradication also depends on the duration of exposure. Like PVP-I, BAC can eradicate biofilms relatively quickly at high concentrations while lower concentrations require longer to exert their effect. S. aureus and P. aeruginosa biofilms, for example, show 4.6 and 6 log10 reductions, respectively, in viable cells after 5 min exposure to around 0.2% BAC (Campanac et al., 2002). However, with an exposure time of 16 h, biofilms are eradicated at around 0.007%, a concentration more consistent with current clinical use (Jennings et al., 2014).
Ciliotoxicity has been observed ex vivo, which is a finding particularly relevant for sinonasal application. In nasal epithelia taken from healthy volunteers, the application of BAC caused a marked dose-dependent reduction in ciliary beat frequency (Riechelmann et al., 2004). The same effect is not seen in vivo. In a double-blind crossover trial, a four-times-daily dosing of 0.007% BAC (applied as a spray) caused no change in saccharin transit time or inflammatory cytokines in nasal mucus compared to placebo. However, participants were more likely to report nasal irritation and discomfort with BAC (Riechelmann et al., 2004). One way to mitigate this may be to exploit its synergy with Cu2+ ions. For example, one study found that BAC eradicates P. aeruginosa biofilm at 0.00016% in the presence of 1 mmol/L Cu2+ with 24 h exposure, whereas viable cells persisted after exposure to 0.04% BAC alone (Harrison et al., 2008). However, copper solutions may themselves produce toxicity, so the clinical potential of this synergy may be limited.
Bacterial susceptibility to BAC is reduced in some strains by membrane-bound efflux pumps, however MICs remain below the concentrations used clinically (Heir et al., 1999).
Quaternary ammonium derivatives may have antibiofilm efficacy and be tolerated by the nasal mucosa. Novel quaternary ammonium products have shown efficacy against planktonic bacteria (Zoono®), and mixed-species biofilms (Daood et al., 2020). Novel quaternary ammoniumyl chitosan derivatives likewise have potent antibiofilm efficacy, with some such compounds eradicating S. aureus biofilms at 0.013% (128 μg/ml) after 24 h exposure (Sahariah et al., 2018). However, these novel compounds are yet to be fully assessed for their safety and efficacy after sinonasal application.
This group of compounds shares structural and functional similarities with QACs. The lipophilic portions of these molecules are shorter than that of the QACs, making them less able to enter the hydrophobic centre of the cell membrane. Instead, they bridge between phospholipid heads displacing cations that stabilise the membrane and altering membrane fluidity. In the same manner as QACs, this leads to leakage of cellular contents and results in cell death (Gilbert and Moore, 2005).
This bisbiguanide is widely used in surgical hand-washes and antiseptic skin preparation (Gilbert and Moore, 2005). Biofilm eradication is reported at concentrations as low as 0.005% for S. aureus and 0.01% for P. aeruginosa after 24 h exposure (Sivaranjani et al., 2021), though this is not consistently observed (Lefebvre et al., 2016). At higher concentrations (0.05–0.1%), S. aureus biofilms were eradicated in some studies in as little as 5 min (Hoekstra et al., 2017; Röhner et al., 2020). In contrast, other studies have reported that the same concentration of chlorhexidine failed to produce even a 3 log10 reduction in viable S. aureus biofilms after a 3 min exposure (Premkumar et al., 2021), or achieved an intermediate result (Ferran et al., 2016).
Chlorhexidine is not without potential toxicity and irreversible ciliostasis has been observed in an embryonic chicken tracheal model at a concentration of 0.01% (van de Donk et al., 1980). Furthermore, increased bacterial tolerance to chlorhexidine has been reported, attributable to some of the same efflux pumps responsible for QAC tolerance (Horner et al., 2012). At present, increased tolerance is defined as an MIC greater than 4 μg/ml (0.004%), which is less than typically used concentrations (Horner et al., 2012).
This polymeric biguanide was synthesized before the bisbiguanides and is generally thought to be more potent (Gilbert and Moore, 2005). The antibiofilm efficacy of polyhexanide is generally equivalent if not superior to that of chlorhexidine across multiple species (Hoekstra et al., 2017; Machuca et al., 2019; Günther et al., 2021). Polyhexanide is often used as a combination product with betaine, a surfactant which may enhance the action of the polyhexanide (Prontosan®, 0.1% polyhexanide and 0.1% betaine, B. Braun Medical, Melsungen, Germany) (Zheng et al., 2021).
The efficacy of this agent against bacterial species is variable with short exposures; one study demonstrated eradication of P. aeruginosa biofilm after 15 min exposure to Prontosan® but minimal effect seen on S. aureus after brief exposure. However, biofilms of both species were eradicated at 24 h (Johani et al., 2018). In another study, Prontosan® diluted to ∼20 and ∼30% of commercial concentration eradicated S. aureus and P. aeruginosa biofilm, respectively, at 24 h. However, full commercial strength resulted in a one third reduction in viable cells at 1 h (Krasowski et al., 2021). Polyhexanide is also able to kill intracellular planktonic S. aureus with mammalian cell uptake by endocytosis in a bovine mammary epithelial model, at concentrations below those causing significant cytotoxicity (Kamaruzzaman et al., 2017).
Cytotoxicity is seen in vitro with concentrations similar to those in clinical use (Müller and Kramer, 2008) or less (Kamaruzzaman et al., 2017). However, this has not prevented clinical use suggesting that these laboratory findings are less relevant in vivo. Polyhexanide drops have been used to treat ophthalmic infections, and one phase I trial has demonstrated no dose-limiting adverse effects when used at a concentration of 0.04% (Papa et al., 2020). Nasal epithelial ciliary beating is markedly slowed on exposure to concentrations as low as 0.01%, however, with complete and relatively swift ciliostasis seen at higher concentrations within the clinical dosage range (Birk et al., 2015).
This large and heterogeneous class of compounds comprises bacterial products and host defence molecules or their derivatives that have antimicrobial action. It includes polymyxins, which have been used clinically for several decades, and some newer compounds that are under ongoing investigation.
Polymyxin proteins are produced by Paenibacillus polymyxa and include polymyxin B and colistin (polymyxin E) (Roy et al., 2018). These proteins act by displacing the divalent cations that stabilise negatively charged lipopolysaccharides on the surfaces of Gram-negative organisms, disrupting the outer membrane (Yin et al., 2020). Polymyxin B has also been shown to induce an oxidative burst in P. aeruginosa, both in biofilm and as planktonic forms, suggesting that reactive oxygen species may also play a role in its bactericidal action (Lima et al., 2019). Some activity against Gram-positive bacteria has been reported but at far higher concentrations; in these bacteria the mechanism of action is thought to be an interaction with teichoic acids in the cell wall and by oxidative damage (Yin et al., 2020). Polymyxin B is not used systemically due to nephro- and neurotoxicity (Klinger-Strobel et al., 2017; Rudilla et al., 2018; Yin et al., 2020). Polymyxin B is found in combination with other antibiotics in well-tolerated topical preparations such as Maxitrol (Novartis Pharma AG, Basel, Switzerland).
The antibiofilm activity of polymyxin B has been variable across a number of in vitro studies. One study demonstrated a ∼60–70% reduction in Klebsiella pneumoniae biofilm biomass with polymyxin B at 8 μg/ml (fourfold greater than MIC and 24 h exposure). Higher concentrations did not confer a greater bactericidal effect (Herrera et al., 2019). In contrast, studies testing polymyxin B against P. aeruginosa biofilms have shown little to no effect at similar concentrations, despite similar planktonic MICs (Berditsch et al., 2015; Gaurav et al., 2020). Interestingly, synergy has been noted with other antibiotics. In one study, a 7.5 log10 decrease in viable P. aeruginosa was seen with combination treatment using doxycycline (Gaurav et al., 2020). In Gram-positive bacteria, bactericidal activity of polymyxin B has been confirmed in vitro against planktonic S. aureus at 1 mg/ml, a concentration achievable with topical application (Yoshida and Hiramatsu, 1993). However, studies testing polymyxin B against Gram-positive biofilms have not been published.
Colistin has been demonstrated to reduce the density of preformed biofilm in S. aureus clinical isolates, and in Escherichia coli biofilms a near 4 log10 reduction in viable cells was seen after application of this antibiotic (Klinger-Strobel et al., 2017). In P. aeruginosa, one study demonstrated a 1–2 log10 reduction on average with 32 μg/ml colistin at 6 h exposure in vitro (Jorge et al., 2017) although in another study the same concentration caused a more than 5 log10 reduction at 3 h and an even greater effect in anaerobic conditions (Kolpen et al., 2016). Against Gram-positive biofilms, colistin is less effective as expected; one of these studies also showed a 1 log10 reduction in viable S. aureus with treatment at 64 μg/ml (Jorge et al., 2017).
Novel polymyxins have been synthesised in order to broaden the spectrum and increase the efficacy of these agents, as well as to reduce nephro- and neurotoxicity. These new compounds show promise against planktonic bacteria of both Gram-positive and Gram-negative species, with MICs between 4 and 6.25 μg/ml against S. aureus, E. coli and P. aeruginosa. Eradication of S. aureus biofilm (irrespective of methicillin status) has been observed between 32 and 256 μg/ml (Rudilla et al., 2018; Su et al., 2019). Dose-dependent toxicity to human hepatocytes was seen in vitro in this concentration range (Rudilla et al., 2018).
Polymyxin resistance is an emerging phenomenon (Khondker and Rheinstädter, 2020), and monotherapy has been associated with emergence of less susceptible subpopulations (Gaurav et al., 2020). It is hoped that novel polymyxins will mitigate this issue and enhance the efficacy of this class (Rudilla et al., 2018).
Gramicidin S is a protein produced by Aneurinibacillus migulanus. In contrast to the polymyxins, gramicidin S acts on the inner membrane, conferring potency against Gram-positive species. In treating Gram-negative bacteria, polymyxins and gramicidin S may act synergistically, as the former makes the outer membrane more permeable to facilitate access of the latter to the inner membrane, enhancing its efficacy (Berditsch et al., 2015).
S. aureus biofilms treated for 18 h with 400 μg/ml gramicidin S showed no regrowth in the planktonic phase upon re-incubation post-treatment (Berditsch et al., 2019). Against P. aeruginosa, gramicidin S has no effect on preformed biofilm alone but is synergistic with polymyxin B by the above mentioned mechanism, giving a greater than 50% reduction in respiratory activity in biofilm cells (8 μg/ml polymyxin B, 32 μg/ml gramicidin S) (Berditsch et al., 2015).
Gramicidin S is haemolytic and is used topically for this reason. It is hoped that novel analogues currently in development may be less toxic. Resistance has not been reported (Berditsch et al., 2015). The clinical safety and efficacy of gramicidin S against sinonasal biofilms is unknown.
Several potential agents have been derived from host defence proteins. Examples include LL-37, which is cleaved from human cathelicidin hCAP18 (Chennupati et al., 2009), and lactoferrin, which is found in both human and cow’s milk (Ramamourthy and Vogel, 2021) as well as in the nasal mucosa (Psaltis et al., 2008).
LL-37 is a helical peptide that acts on the cell membranes of both Gram-positive and negative bacteria (Dean et al., 2011). A recent study has demonstrated a 4.3 log10 reduction in S. aureus biofilm in vitro after 60 min exposure to 10 μmol/L LL-37, with the majority of killing occurring in the first 5 min. However, some cytotoxicity has been observed in this concentration range in vitro (Kang et al., 2019). SAAP-148, a synthetic LL-37 inspired peptide, has achieved eradication of S. aureus biofilms at similar concentrations (Scheper et al., 2021). Other LL-37 derivatives have shown dose-dependent inflammation and deciliation in a rabbit model of sinusitis at concentrations required to fully eradicate P. aeruginosa biofilm (Chennupati et al., 2009). Further work is required to reduce the toxicity while maintaining the antibiofilm activity of these cathelicidin-derived peptides.
Lactoferrin is a protein that sequesters Fe3+, making this element unavailable for its essential role in bacterial metabolism and biofilm formation (Ammons and Copié, 2013). The N-terminal of lactoferrin also has a lytic effect on the bacterial cell membrane (Jain et al., 2015). Lactoferrin is produced by the nasal epithelium as a factor in innate mucosal immunity. Interestingly, the expression of lactoferrin has been shown to be reduced in CRS patients who have sinonasal biofilms (Psaltis et al., 2008). The naturally occurring bovine lactoferricin B can eradicate preformed P. aeruginosa biofilm at 128 μmol/L after 24 h of exposure. At lower concentrations, it significantly reduced the amount of biofilm. In this study, some synthetic lactoferrin derivatives were also found to be effective (Ramamourthy and Vogel, 2021). Lactoferrin was also tested in a rabbit model of sinus mucosal injury and it did not delay wound healing, suggesting safety for use in the postoperative setting (Jain et al., 2015).
Chelating agents destabilise the extracellular matrix by sequestering the cations required to maintain the structure of the biofilm. The weakened biofilm is rendered more likely to disperse and more permeable to other antimicrobials (Banin et al., 2006; Cavaliere et al., 2014).
This chelating agent is used widely as a pharmaceutical excipient and as an anticoagulant for laboratory haematological tests. EDTA salts are administered intravenously to treat severe hypercalcaemia and heavy metal poisoning (Sultan et al., 2017). It also has an antimicrobial effect that has led to its licensing as a lock solution for central venous lines in some countries (Liu et al., 2018).
EDTA inhibits formation of biofilms of pathogens at concentrations permitted as an excipient in other drug preparations (Cherian et al., 2019). EDTA is proven effective against single-species biofilms in vitro including S. aureus (irrespective of methicillin resistance status), P. aeruginosa, E. coli, K. pneumoniae and Serratia marcescens, as well as Candida albicans and Candida glabratus; full eradication is seen after a 24 h exposure to concentrations up to 4% (Liu et al., 2018; Sivaranjani et al., 2021). Indeed, some S. aureus strains were nearly eradicated at 6 h and P. aeruginosa at 1–3 h at this concentration. Other authors have found a weaker antibiofilm effect using different in vitro assays (Hogan et al., 2016; Percival and Salisbury, 2017).
More consistently reported is the potentiating effect of EDTA on other antimicrobials, including polyhexanide and PVP-I (4000-fold and 200-fold increases in activity, respectively) (Lefebvre et al., 2016). Even antibiotics such as gentamicin, ciprofloxacin and ampicillin have improved efficacy with significant reductions in biomass and approximately 2–3 log10 increases in bacterial killing when co-administered with EDTA (Cavaliere et al., 2014; Liu et al., 2017).
Systemic toxicity of EDTA is minimal when given appropriately (Sultan et al., 2017). Ciliotoxicity is again minimal; in an embryonic chicken model, tracheal ciliary beating was inhibited by approximately 50% with 0.1% EDTA and this fully reversed when exposure ceased (van de Donk et al., 1980). In a live sheep model of S. aureus sinusitis, 0.0075% EDTA was seen to have little effect on ciliary structure and distribution while effectively reducing the amount of biofilm present (Drilling et al., 2014).
EDTA is a safe compound that is in wide clinical use for non-antibiofilm indications. Ciliotoxicity testing has examined lower concentrations than those effective against biofilms and further research is warranted to ensure mucosal tolerability at higher concentrations. EDTA may prove to be a clinically useful potentiator of other antibiofilm agents in the treatment of sinonasal biofilms.
Deferiprone is an iron chelating agent used for the treatment of thalassaemia major. Gallium protoporphyrin (GaPP) is a haem analogue that disrupts bacterial iron metabolism. These two compounds have been found effective when given in succession: deferiprone’s action leads to the induction of iron uptake pathways in bacterial cells, allowing GaPP to be readily taken up (Richter et al., 2016). These two molecules have been combined with a chitosan-dextran gel (Chitogel™, Chitogel Ltd., Wellington, New Zealand) which has been developed for use in FESS. Chitogel™ releases deferiprone more quickly than GaPP, causing bacteria to be exposed to the compounds in the optimal order (Richter et al., 2017b).
In vitro, Def-GaPP (20 mmol/L deferiprone, 200 μg/ml GaPP, 2 h of each treatment in succession) gives up to a 95% reduction in viable S. aureus biofilm cells (Richter et al., 2016). Combined with Chitogel™, Def-GaPP at near equivalent doses and 5 days exposure gives a 3.8 log10 reduction in viable S. aureus in biofilm, 3.9 and 3.3 log10 reductions in two different P. aeruginosa strains, and 4.3 log10 reduction in S. epidermidis. Poorer performance is seen against methicillin resistant S. aureus, with a 1.4 log10 reduction, and similar findings are noted with Acinetobacter johnsonii (Richter et al., 2017b). In an ovine sinusitis model, treatment with Chitogel™-Def-GaPP led to an 82% reduction in S. aureus biofilm biomass after 7 days (Ooi et al., 2018b).
No toxicity is seen from either deferiprone or GaPP in human nasal epithelial cells in culture at these concentrations (Richter et al., 2016), and no electron micrographic evidence of ciliary or mucosal injury was seen in treated sheep sinuses (Ooi et al., 2018b). Def-GaPP appears to be safe and effective, and combined with Chitogel™ as a delivery vehicle it may prove beneficial for improving surgical outcomes.
Xylitol is a naturally occurring sugar alcohol used in food preparation as a sugar substitute (Jain et al., 2016). When applied to the nasal mucosa, it acts by osmosis to reduce the chloride concentration of the airway surface liquid (ASL). The ASL chloride concentration is increased in inflammatory states such as CRS, and this impairs the action of the naturally occurring antimicrobial components. Restoring this balance may enhance innate immunity, a theory borne out by in vitro data (Weissman et al., 2011).
Xylitol has direct antimicrobial effects probably due to inhibition of bacterial glucose metabolism. One hour treatment with 5% xylitol causes reductions of biofilm biomass of approximately one third for S. epidermidis, but not for S. aureus or P. aeruginosa. Biofilm formation for the latter two species is, however, significantly impaired in the presence of xylitol (Jain et al., 2016). Clinical trials of xylitol nasal irrigation in postoperative patients have demonstrated improvement in subjective symptom scores compared to saline without any adverse effects seen (Weissman et al., 2011; Lin et al., 2017). Xylitol may have in vivo benefits despite its limited potency as an antibiofilm agent in vitro.
Xylitol has been demonstrated to facilitate the breaking down of sinonasal crusts (Hardcastle et al., 2017). It has also been shown to lead to an increase in inducible nitric oxide synthase expression in the sinonasal mucosa, which leads to an increase in nitric oxide within the sinuses. Nitric oxide is a product of the innate immune system and key modulator of ciliary beating (Lin et al., 2017). It is also known to induce the dispersal of preformed biofilm (Verderosa et al., 2019).
Mānuka honey’s antibacterial effect is conferred by the phenol methylglyoxal, present in far higher concentrations in mānuka honey than that from other flowers (Kilty et al., 2011). The precise mechanisms of action of methylglyoxal are yet to be fully established (Lee et al., 2017). However, in Gram-positive organisms it causes a perturbation of cell division and in Gram-negatives it destabilises the cell membrane (Kilty et al., 2011; Camplin and Maddocks, 2014). Interestingly, pure methylglyoxal offers only around half the antimicrobial effect of intact honey and adding methylglyoxal to non-mānuka honey confers an antimicrobial effect, suggesting that there are other factors required (Jervis-Bardy et al., 2011).
At 33% concentration (equivalent to 0.26 mg/ml methylglyoxal), mānuka honey eradicates preformed S. aureus biofilm in vitro after 24 h exposure. Lower honey concentrations may be used if exogenous methylglyoxal is added to enhance its antibiofilm activity (Jervis-Bardy et al., 2011). In an in vivo sheep model of S. aureus sinusitis, 16.5% mānuka honey supplemented to a total concentration of 1.8 mg/ml methylglyoxal reduced biofilm biomass by around 80% when applied twice daily for 5 days (Paramasivan et al., 2014). These findings have not yet translated to clinical benefit, however. In a randomised controlled trial, participants with recalcitrant CRS post-FESS were treated twice daily with mānuka honey rinses for 2 weeks (16.5% mānuka honey supplemented to 1.3 mg/ml total methylglyoxal). The control group was treated with saline rinses and culture-directed antibiotics. These authors demonstrated no difference between groups in SNOT-22 and Lund-Kennedy scores. This phase I trial was likely underpowered to detect clinical improvement (Ooi et al., 2019b). Another similar RCT demonstrated equivalent outcomes with 10% Medihoney® (a mānuka honey preparation by Derma Sciences, Princeton, NJ, United States) compared to saline rinses twice daily for 30 days (Lee et al., 2017).
Mānuka honey has not caused ciliary or epithelial changes on histological and electron microscopic examination of sheep or rabbit sinuses (Kilty et al., 2010). Methylglyoxal concentrations above 1.8 mg/ml, however, cause ciliary destruction in sheep, and at 7.2 mg/ml gross squamous metaplasia and cellular detachment is seen (Paramasivan et al., 2014).
Cannabis extracts have been known to possess broad antibacterial properties since the 1950s (Karas et al., 2020). More recent studies have confirmed the antimicrobial activity of specific compounds isolated from Cannabis sativa, including cannabidiol, cannabigerol and the psychoactive ∆9-tetrahydrocannabinol (van Klingeren and ten Ham, 1976; Farha et al., 2020), leading to the hypothesis that C. sativa evolved these compounds to defend itself against bacterial pathogens (Farha et al., 2020).
Cannabinoids act by disruption of the bacterial inner cell membrane. They are therefore highly effective against Gram-positives, however activity against Gram-negatives varies by species. For example, cannabigerol and cannabidiol eradicate S. aureus biofilm at concentrations of 4 μg/ml (Farha et al., 2020; Blaskovich et al., 2021), though P. aeruginosa is largely unaffected by these products when applied in isolation. Interestingly, there is a very low rate of spontaneous resistance to cannabinoids among Gram-positive species and attempts to induce resistance in vitro have yielded little to no change in MIC values, making this a particularly promising avenue of future research (Farha et al., 2020; Blaskovich et al., 2021). Cannabinoids are inactivated by serum, so they are better suited to topical administration (van Klingeren and ten Ham, 1976).
The development of cannabis-based products are hampered by issues of legality. However, as medicinal cannabis becomes more widely available, it is likely that the antimicrobial and potential antibiofilm properties of these compounds will be increasingly explored.
Surfactants are commonly used as detergents in household goods such as shampoo. They have previously been used in sinus rinses with the goal of disrupting sinonasal biofilm, but they also have significant associated deleterious effects. Johnson & Johnson Baby Shampoo (Johnson & Johnson, New Brunswick, NJ, United States) is observed to inhibit biofilm formation, but it has only a negligible effect on preformed biofilm in vitro (Chiu et al., 2008). It causes slowing of mucociliary clearance as determined by saccharin transit time (Isaacs et al., 2011). A citric acid/zwitterionic surfactant product has demonstrated early reduction in biofilm burden in a sheep model after a single application, but ciliary destruction and biofilm regrowth occur by day seven (Valentine et al., 2011). In healthy volunteers, SinuSurf (NeilMed Pharmaceuticals, Santa Rosa, CA, United States), a proprietary surfactant designed for sinonasal application, caused reversible hyposmia in 18% and moderate or severe congestion in 29% of participants when used twice daily for 7 days (Turner et al., 2017). It has since been withdrawn from the market. Beyond this, some commonly used surfactants loosen epithelial barriers, an effect that has been implicated in the pathogenesis of multiple allergic and autoimmune diseases including CRS (Akdis, 2021). These findings have led to concerns about the safety of such products in sinonasal application.
Silver nanoparticles and colloidal silver preparations have been found to be effective against biofilms in vitro and in a nematode model (Müller and Kramer, 2008; Richter et al., 2017a). Unfortunately, in a subsequent human trial with patients with CRS, this did not translate to clinical benefit. With twice-daily colloidal silver sinus rinses for 10 days, there were at best non-significant trends towards improvement in a visual-analogue scale and Lund Kennedy and SNOT-22 scores. No adverse effects were identified, though four of eleven patients in the colloidal silver arm had elevated serum silver which normalised upon cessation of treatment (Ooi et al., 2018a).
Simvastatin is an HMG-CoA reductase inhibitor prescribed widely for management of dyslipidaemia (Thangamani et al., 2015). The drug is also an effective antibiotic, but as this effect is seen at systemically toxic concentrations, it holds promise only as a topical agent (Graziano et al., 2015; Ko et al., 2018). Simvastatin acts by inhibiting synthesis of proteins, lipids, and DNA in prokaryotes, but not mammalian cells (Thangamani et al., 2015).
Simvastatin has MICs of 16–64 μg/ml for planktonic S. aureus (Graziano et al., 2015; Ko et al., 2018), but no effect is seen against Gram-negatives except when the outer membrane is permeabilised by another compound (Thangamani et al., 2015). While simvastatin can effectively prevent biofilm formation at twice MIC (Graziano et al., 2015), it has only a modest effect against preformed biofilms (Graziano et al., 2015; Thangamani et al., 2015). In a murine model of S. aureus infection, simvastatin reduced the release of TNF⍺, IL-6 and IL-1β, consistent with the known immune-modulating effects of its class, and a reduction in the expression of Staphylococcal exotoxins was also observed (Thangamani et al., 2015).
Bacteriophages are viruses that infect bacterial cells, replicate within them and cause cell death by lysis. Most phages are species specific in their activity (Fong et al., 2019). The viral life cycle typically leads to increases in viral titres over time, in contrast to most other topical agents which dissipate quickly (Fong et al., 2017).
The use of phages was described in the mid-20th century for the treatment of sinonasal disease (Mills, 1956), and interest has renewed in recent years as the search for effective non-antibiotic treatments becomes more pressing as rates of resistance to established antibiotics increase. Phages specific to both S. aureus and P. aeruginosa have been studied in a sheep sinusitis model; when delivered in regular sinus rinses for 5–7 days, these caused reductions in biofilm biomass (Drilling et al., 2014; Fong et al., 2019). Against S. aureus biofilms, bacteriophages were as effective as EDTA (Drilling et al., 2014). No phages eradicated biofilms, however, and no effect was seen against P. aeruginosa biofilms when other bacteria were identified on sinus culture prior to bacterial inoculation. This finding is consistent with studies that have shown that bacteria in mixed species biofilms are less susceptible to single-species phages in vitro (Fong et al., 2019). In humans, a phase 1 trial has demonstrated the safety and tolerability of sinonasal phage therapy for recalcitrant CRS, though further trials are necessary to determine efficacy (Ooi et al., 2019a).
Bacterial biofilms are associated with greater disease severity, recalcitrance and poorer postoperative outcomes in CRS patients (Singhal et al., 2011; Vickery et al., 2019). Despite these negative associations, there are few antibiofilm agents currently available to target this problem and more effective and well-tolerated agents are required. This review discussed a number of compounds that may have therapeutic potential. Some of these are already widely available and could be adapted for intranasal use, whereas others represent promising avenues of future development that have not yet been fully realised.
The most promising compounds are those with the most potent antibiofilm action combined with the least toxicity and potential for mucosal irritation. On this basis, we propose that of the compounds reviewed, iodine-based products and quaternary ammonium compounds are the most likely to be useful in the treatment of biofilm-associated CRS. Chelating agents such as EDTA are promising as adjuncts, as these agents may act to break up biofilms, allowing better penetration of the primary agent. Other therapies, such as the host defence peptides and their derivatives, novel polymyxin analogues and bacteriophages require considerably more research and development before introduction into clinical use. Large clinical trials in patients with biofilm-associated CRS, with verification of biofilm status pre- and post-treatment, are required to establish the clinical utility of these agents. Indeed, a recent systematic review examining human trials of antibiofilm agents in CRS identified only 13 studies appropriate for inclusion, illustrating the need for further research (Taylor et al., 2021).
The common theme among the most effective compounds reviewed is their mechanism of action: denaturation of cellular structures and/or membrane lysis. This non-reliance on the metabolic activity of the cell differentiates them from most conventional antimicrobials and permits bactericidal activity despite the innate antibiotic resistance of the biofilm. However, biofilm killing tends still to be less efficient than for planktonic bacteria, probably because the biofilm is difficult for the agents to penetrate (Bridier et al., 2011; Scheper et al., 2021). This may be ameliorated by combination treatment with compounds that disrupt the biofilm matrix, including EDTA and surfactants. Other adjuncts have been described, such as the enzymes DNase I (Cavaliere et al., 2014) and Dispersin B (Gawande et al., 2014) that break down the DNA and polysaccharide components of the matrix but which do not themselves lead to biofilm killing. The use of nitric oxide releasing compounds to disperse biofilms is also described, in order to enhance the antimicrobial effect of other agents (Verderosa et al., 2019). Further research is required to determine the role these adjuncts may play in the treatment of biofilm-associated disease.
There is significant methodological heterogeneity in the included studies (Table 1). Biofilms were variously grown, for example, in well plates (e.g., 24 or 96 well plates), MBEC Assay (Innovotech Inc. Edmonton, Alberta, Canada, previously called the Calgary Biofilm Device), and Centre for Disease Control (CDC) Biofilm Reactors (BioSurface Technologies Corporation, Bozeman, Montana, United States); on varying materials such as polystyrene, polycarbonate, or other substrates including glass (Lefebvre et al., 2016), titanium (Premkumar et al., 2021), cloth (Herruzo and Herruzo, 2020), and dentine (Daood et al., 2020) (Figure 3); and for varying lengths of time prior to treatment. The biofilms produced are therefore not equally robust. It has been shown that biofilms grown in the CDC reactor are less susceptible to the effects of EDTA than those grown in the MBEC Assay (Percival and Salisbury, 2017). In a similar vein, the methods used for biofilm quantification varied greatly. In the studies reviewed, viable bacteria and/or biofilm biomass were quantified after treatment by culture and colony counting, crystal violet staining, microscopy and metabolic assays. Furthermore, there is heterogeneity in the nomenclature surrounding antimicrobial testing against biofilms. Minimum biofilm eradication and inhibitory concentrations (MBEC and MBIC) are commonly used parameters, but the specific definitions differ between papers (Thieme et al., 2019). The lack of standardisation of methods and nomenclature makes it difficult to compare studies and hampers the interpretation of in vitro studies more broadly. For clarity, we have described the concentration and duration of exposure required for complete biofilm eradication, or degree of reduction in the biofilm after exposure when eradication was not achieved.
FIGURE 3. Methods of growing bacterial biofilms. (A) a 96 well plate. Wells are inoculated and biofilms form on the inner surfaces of the wells during incubation. (B) The Innovotech MBEC Assay®, previously known as the Calgary Biofilm Device (Innovotech Inc. Edmonton, Alberta, Canada). This system comprises a 96 well plate, or a trough, and a lid with 96 corresponding pegs. Wells are inoculated and biofilms grow on the pegs during incubation. After biofilm formation, biofilms are easily transferred to a new 96 well plate containing treatment solutions for testing. (C) The CDC Biofilm Reactor (BioSurface Technologies Corporation, Bozeman, Montana, United States). Eight reactor rods are suspended in culture broth, with each rod containing three 12.7 mm diameter coupons of a given material. Fresh culture medium may be pumped through the system with effluent collecting in a separate tank. Temperature and rotatory motion is maintained by a hot plate and magnetic stirring apparatus. After biofilm growth, coupons are removed from the rods for testing. (D) Culture using other methodologies on other substrates such as glass, titanium, cloth, and dentine.
The antibiofilm agents presented here are not an exhaustive list of those available. For the purposes of this review, we present and evaluate those classes that are currently available or are the subject of research and development, and which we consider to be promising for the treatment of biofilm-associated CRS. These compounds should form the basis of ongoing research on the treatment of this difficult condition.
SH performed literature searches, critical appraisal, data collection, and wrote the manuscript. BWM, CL, KB, RK, and RD undertook manuscript review and editing.
SH is the recipient of an Academic Surgeon-Scientist Research Scholarship, grant number 3723406, and RK is the recipient of an Academic Surgeon-Scientist Fellowship, grant number 3719672, both generously funded by The Garnett Passe and Rodney Williams Memorial Foundation. BWM is generously supported by the Auckland Medical Research Foundation, grant number 3721397.
The authors declare that the research was conducted in the absence of any commercial or financial relationships that could be construed as a potential conflict of interest.
All claims expressed in this article are solely those of the authors and do not necessarily represent those of their affiliated organizations, or those of the publisher, the editors and the reviewers. Any product that may be evaluated in this article, or claim that may be made by its manufacturer, is not guaranteed or endorsed by the publisher.
Akdis, C. A. (2021). Does the Epithelial Barrier Hypothesis Explain the Increase in Allergy, Autoimmunity and Other Chronic Conditions? Nat. Rev. Immunol. 21, 739–751. doi:10.1038/s41577-021-00538-7
Al-Bakri, A. G., Othman, G., and Bustanji, Y. (2009). The Assessment of the Antibacterial and Antifungal Activities of Aspirin, EDTA and Aspirin-EDTA Combination and Their Effectiveness as Antibiofilm Agents. J. Appl. Microbiol. 107, 280–286. doi:10.1111/j.1365-2672.2009.04205.x
Alandejani, T., Marsan, J., Ferris, W., Slinger, R., and Chan, F. (2009). Effectiveness of Honey on Staphylococcus aureus and Pseudomonas aeruginosa Biofilms. Otolaryngol. Head Neck Surg. 141, 114–118. doi:10.1016/j.otohns.2009.01.005
Ammons, M. C., and Copié, V. (2013). Mini-review: Lactoferrin: a Bioinspired, Anti-biofilm Therapeutic. Biofouling 29, 443–455. doi:10.1080/08927014.2013.773317
Banin, E., Brady, K. M., and Greenberg, E. P. (2006). Chelator-induced Dispersal and Killing of Pseudomonas aeruginosa Cells in a Biofilm. Appl. Environ. Microbiol. 72, 2064–2069. doi:10.1128/aem.72.3.2064-2069.2006
Barreto, R., Barrois, B., Lambert, J., Malhotra-Kumar, S., Santos-Fernandes, V., and Monstrey, S. (2020). Addressing the Challenges in Antisepsis: Focus on Povidone Iodine. Int. J. Antimicrob. Agents 56, 106064. doi:10.1016/j.ijantimicag.2020.106064
Berditsch, M., Afonin, S., Reuster, J., Lux, H., Schkolin, K., and Babii, O. (2019). Supreme Activity of Gramicidin S against Resistant, Persistent and Biofilm Cells of Staphylococci and Enterococci. Sci. Rep. 9, 17938. doi:10.1038/s41598-019-54212-z
Berditsch, M., Jäger, T., Strempel, N., Schwartz, T., Overhage, J., and Ulrich, A. S. (2015). Synergistic Effect of Membrane-Active Peptides Polymyxin B and Gramicidin S on Multidrug-Resistant Strains and Biofilms of Pseudomonas aeruginosa. Antimicrob. Agents Chemother. 59, 5288–5296. doi:10.1128/aac.00682-15
Bigliardi, P. L., Alsagoff, S. A. L., El-Kafrawi, H. Y., Pyon, J.-K., Wa, C. T. C., and Villa, M. A. (2017). Povidone Iodine in Wound Healing: a Review of Current Concepts and Practices. Int. J. Surg. 44, 260–268. doi:10.1016/j.ijsu.2017.06.073
Birk, R., Aderhold, C., Stern-Sträter, J., Hörmann, K., Stuck, B. A., and Sommer, J. U. (2015). Polyhexanide-containing Solution Reduces Ciliary Beat Frequency of Human Nasal Epithelial Cells In Vitro. Eur. Arch. Otorhinolaryngol. 272, 377–383. doi:10.1007/s00405-014-3112-5
Blaskovich, M. A. T., Kavanagh, A. M., Elliott, A. G., Zhang, B., Ramu, S., Amado, M., et al. (2021). The Antimicrobial Potential of Cannabidiol. Commun. Biol. 4, 7. doi:10.1038/s42003-020-01530-y
Bridier, A., Dubois-Brissonnet, F., Greub, G., Thomas, V., and Briandet, R. (2011). Dynamics of the Action of Biocides in Pseudomonas aeruginosa Biofilms. Antimicrob. Agents Chemother. 55, 2648–2654. doi:10.1128/aac.01760-10
Campanac, C., Pineau, L., Payard, A., Baziard-Mouysset, G., and Roques, C. (2002). Interactions between Biocide Cationic Agents and Bacterial Biofilms. Antimicrob. Agents Chemother. 46, 1469–1474. doi:10.1128/aac.46.5.1469-1474.2002
Camplin, A. L., and Maddocks, S. E. (2014). Manuka Honey Treatment of Biofilms of Pseudomonas aeruginosa Results in the Emergence of Isolates with Increased Honey Resistance. Ann. Clin. Microbiol. Antimicrob. 13, 19. doi:10.1186/1476-0711-13-19
Capriotti, K., Pelletier, J., Barone, S., and Capriotti, J. (2018). Efficacy of Dilute Povidone-Iodine against Multi-Drug Resistant Bacterial Biofilms, Fungal Biofilms and Fungal Spores. Clin. Res. Dermatol. Open Access 5, 1–5. doi:10.15226/2378-1726/5/1/00174
Cavaliere, R., Ball, J. L., Turnbull, L., and Whitchurch, C. B. (2014). The Biofilm Matrix Destabilizers, EDTA and DNaseI, Enhance the Susceptibility of Nontypeable Hemophilus Influenzae Biofilms to Treatment with Ampicillin and Ciprofloxacin. MicrobiologyOpen 3, 557–567. doi:10.1002/mbo3.187
Chen, H.-H., Liu, X., Ni, C., Lu, Y.-P., Xiong, G.-Y., Lu, Y.-Y., et al. (2012). Bacterial Biofilms in Chronic Rhinosinusitis and Their Relationship with Inflammation Severity. Auris Nasus Larynx 39, 169–174. doi:10.1016/j.anl.2011.04.014
Chennupati, S. K., Chiu, A. G., Tamashiro, E., Banks, C. A., Cohen, M. B., Bleier, B. S., et al. (2009). Effects of an LL-37–Derived Antimicrobial Peptide in an Animal Model of Biofilm Pseudomonas Sinusitis. Am. J. Rhinol Allergy 23, 46–51. doi:10.2500/ajra.2009.23.3261
Cherian, L. M., Cooksley, C., Richter, K., Ramezanpour, M., Paramasivan, S., Wormald, P.-J., et al. (2019). Effect of Commercial Nasal Steroid Preparation on Bacterial Growth. Int. Forum Allergy Rhinol 9, 766–775. doi:10.1002/alr.22312
Chiu, A. G., Palmer, J. N., Woodworth, B. A., Doghramji, L., Cohen, M. B., Prince, A., et al. (2008). Baby Shampoo Nasal Irrigations for the Symptomatic post–functional Endoscopic Sinus Surgery Patient. Am. J. Rhinol 22, 34–37. doi:10.2500/ajr.2008.22.3122
Cooper, R. A. (2007). Iodine Revisited. Int. Wound J. 4, 124–137. doi:10.1111/j.1742-481x.2007.00314.x
Damour, O., Hua, S. Z., Lasne, F., Villain, M., Rousselle, P., and Collombel, C. (1992). Cytotoxicity Evaluation of Antiseptics and Antibiotics on Cultured Human Fibroblasts and Keratinocytes. Burns 18, 479–485. doi:10.1016/0305-4179(92)90180-3
Daood, U., Matinlinna, J. P., Pichika, M. R., Mak, K.-K., Nagendrababu, V., and Fawzy, A. S. (2020). A Quaternary Ammonium Silane Antimicrobial Triggers Bacterial Membrane and Biofilm Destruction. Sci. Rep. 10, 10970. doi:10.1038/s41598-020-67616-z
Dean, S. N., Bishop, B. M., and van Hoek, M. L. (2011). Susceptibility of Pseudomonas aeruginosa Biofilm to Alpha-Helical Peptides: D-Enantiomer of LL-37. Front. Microbiol. 2, 128. doi:10.3389/fmicb.2011.00128
Drilling, A., Morales, S., Boase, S., Jervis‐Bardy, J., James, C., Jardeleza, C., et al. (2014). Safety and Efficacy of Topical Bacteriophage and Ethylenediaminetetraacetic Acid Treatment of Staphylococcus aureus Infection in a Sheep Model of Sinusitis. Int. Forum Allergy Rhinol 4, 176–186. doi:10.1002/alr.21270
Farha, M. A., El-Halfawy, O. M., Gale, R. T., MacNair, C. R., Carfrae, L. A., Zhang, X., et al. (2020). Uncovering the Hidden Antibiotic Potential of Cannabis. ACS Infect. Dis. 6, 338–346. doi:10.1021/acsinfecdis.9b00419
Ferran, A. A., Liu, J. J., Toutain, P.-L., and Bousquet-Mélou, A. (2016). Comparison of the In Vitro Activity of Five Antimicrobial Drugs against Staphylococcus Pseudintermedius and Staphylococcus Aureus Biofilms. Front. Microbiol. 7, 1187. doi:10.3389/fmicb.2016.01187
Fokkens, W. J., Lund, V. J., Hopkins, C., Hellings, P. W., Kern, R., Reitsma, S., et al. (2020). European Position Paper on Rhinosinusitis and Nasal Polyps 2020. Rhinology Suppl. 29, 1–464. doi:10.4193/rhin20.600
Fong, S. A., Drilling, A. J., Ooi, M. L., Paramasivan, S., Finnie, J. W., Morales, S., et al. (2019). Safety and Efficacy of a Bacteriophage Cocktail in an In Vivo Model of Pseudomonas aeruginosa Sinusitis. Transl Res. 206, 41–56. doi:10.1016/j.trsl.2018.12.002
Fong, S. A., Drilling, A., Morales, S., Cornet, M. E., Woodworth, B. A., Fokkens, W. J., et al. (2017). Activity of Bacteriophages in Removing Biofilms of Pseudomonas aeruginosa Isolates from Chronic Rhinosinusitis Patients. Front Cel Infect Microbiol 7, 418. doi:10.3389/fcimb.2017.00418
Foreman, A., Jervis‐Bardy, J., and Wormald, P.-J. (2011). Do biofilms Contribute to the Initiation and Recalcitrance of Chronic Rhinosinusitis? Laryngoscope 121, 1085–1091. doi:10.1002/lary.21438
Gaurav, A., Kothari, A., Omar, B. J., and Pathania, R. (2020). Assessment of Polymyxin B–Doxycycline in Combination against Pseudomonas aeruginosa In Vitro and in a Mouse Model of Acute Pneumonia. Int. J. Antimicrob. Agents 56, 106022. doi:10.1016/j.ijantimicag.2020.106022
Gawande, P. V., Clinton, A. P., LoVetri, K., Yakandawala, N., Rumbaugh, K. P., and Madhyastha, S. (2014). Antibiofilm Efficacy of DispersinB® Wound spray Used in Combination with a Silver Wound Dressing. Microbiol. Insights 7, 9–13. doi:10.4137/mbi.s13914
Gilbert, P., and Moore, L. E. (2005). Cationic Antiseptics: Diversity of Action under a Common Epithet. J. Appl. Microbiol. 99, 703–715. doi:10.1111/j.1365-2672.2005.02664.x
Graziano, T. S., Cuzzullin, M. C., Franco, G. C., Schwartz-Filho, H. O., de Andrade, E. D., Groppo, F. C., et al. (2015). Statins and Antimicrobial Effects: Simvastatin as a Potential Drug against Staphylococcus aureus Biofilm. PLoS ONE 10, e0128098. doi:10.1371/journal.pone.0128098
Günther, F., Blessing, B., Dapunt, U., Mischnik, A., and Mutters, N. T. (2021). Ability of Chlorhexidine, Octenidine, Polyhexanide and Chloroxylenol to Inhibit Metabolism of Biofilm-Forming Clinical Multidrug-Resistant Organisms. J. Infect. Prev. 22, 12–18. doi:10.1177/1757177420963829
Hardcastle, T., Jain, R., Radcliff, F., Waldvogel‐Thurlow, S., Zoing, M., Biswas, K., et al. (2017). The In Vitro Mucolytic Effect of Xylitol and Dornase Alfa on Chronic Rhinosinusitis Mucus. Int. Forum Allergy Rhinol 7, 889–896. doi:10.1002/alr.21970
Harrison, J. J., Turner, R. J., Joo, D. A., Stan, M. A., Chan, C. S., Allan, N. D., et al. (2008). Copper and Quaternary Ammonium Cations Exert Synergistic Bactericidal and Antibiofilm Activity against Pseudomonas aeruginosa. Antimicrob. Agents Chemother. 52, 2870–2881. doi:10.1128/aac.00203-08
Heir, E., Sundheim, G., and Holck, A. L. (1999). The qacG Gene on Plasmid pST94 Confers Resistance to Quaternary Ammonium Compounds in Staphylococci Isolated from the Food Industry. J. Appl. Microbiol. 86, 378–388. doi:10.1046/j.1365-2672.1999.00672.x
Herrera, K. M. S., da Silva, F. K., de Oliveira, M. E., de Paiva, M. C., Soares, A. C., and Ferreira, J. M. S. (2019). First Report of Polymyxin B Activity in Klebsiella pneumoniae Biofilm. J. Chemother. 31, 127–131. doi:10.1080/1120009x.2018.1558751
Herruzo, R., and Herruzo, I. (2020). Antimicrobial Efficacy of a Very Stable Hypochlorous Acid Formula Compared with Other Antiseptics Used in Treating Wounds: In-Vitro Study on Micro-organisms with or without Biofilm. J. Hosp. Infect. 105, 289–294. doi:10.1016/j.jhin.2020.01.013
Hill, R. L. R., and Casewell, M. W. (2000). The In-Vitro Activity of Povidone–Iodine Cream against Staphylococcus aureus and its Bioavailability in Nasal Secretions. J. Hosp. Infect. 45, 198–205. doi:10.1053/jhin.2000.0733
Hoekstra, M. J., Westgate, S. J., and Mueller, S. (2017). Povidone‐iodine Ointment Demonstrates In Vitro Efficacy against Biofilm Formation. Int. Wound J. 14, 172–179. doi:10.1111/iwj.12578
Hogan, S., Zapotoczna, M., Stevens, N. T., Humphreys, H., O’Gara, J. P., and O’Neill, E. (2016). In Vitro approach for Identification of the Most Effective Agents for Antimicrobial Lock Therapy in the Treatment of Intravascular Catheter-Related Infections Caused by Staphylococcus aureus. Antimicrob. Agents Chemother. 60, 2923–2931. doi:10.1128/aac.02885-15
Hoggard, M., Biswas, K., Zoing, M., Wagner Mackenzie, B., Taylor, M. W., and Douglas, R. G. (2017a). Evidence of Microbiota Dysbiosis in Chronic Rhinosinusitis. Int. Forum Allergy Rhinol 7, 230–239. doi:10.1002/alr.21871
Hoggard, M., Wagner Mackenzie, B., Jain, R., Taylor, M. W., Biswas, K., and Douglas, R. G. (2017b). Chronic Rhinosinusitis and the Evolving Understanding of Microbial Ecology in Chronic Inflammatory Mucosal Disease. Clin. Microbiol. Rev. 30, 321–348. doi:10.1128/cmr.00060-16
Hoggard, M., Zoing, M., Biswas, K., Taylor, M. W., and Douglas, R. G. (2019). The Sinonasal Mycobiota in Chronic Rhinosinusitis and Control Patients. Rhinology 57, 190–199. doi:10.4193/rhin18.256
Horner, C., Mawer, D., and Wilcox, M. (2012). Reduced Susceptibility to Chlorhexidine in Staphylococci: Is it Increasing and Does it Matter? J. Antimicrob. Chemother. 67, 2547–2559. doi:10.1093/jac/dks284
Isaacs, S., Fakhri, S., Luong, A., Whited, C., and Citardi, M. J. (2011). The Effect of Dilute Baby Shampoo on Nasal Mucociliary Clearance in Healthy Subjects. Am. J. Rhinol Allergy 25, e27–e29. doi:10.2500/ajra.2011.25.3583
Jain, R., Kim, R., Waldvogel‐Thurlow, S., Hwang, P., Cornish, J., and Douglas, R. (2015). The Effects of Topical Agents on Paranasal Sinus Mucosa Healing: a Rabbit Study. Int. Forum Allergy Rhinol 5, 310–317. doi:10.1002/alr.21470
Jain, R., Lee, T., Hardcastle, T., Biswas, K., Radcliff, F., and Douglas, R. (2016). The In Vitro Effect of Xylitol on Chronic Rhinosinusitis Biofilms. Rhinology 54, 323–328. doi:10.4193/rhin15.380
Jennings, M. C., Ator, L. E., Paniak, T. J., Minbiole, K. P. C., and Wuest, W. M. (2014). Biofilm‐eradicating Properties of Quaternary Ammonium Amphiphiles: Simple Mimics of Antimicrobial Peptides. ChemBioChem 15, 2211–2215. doi:10.1002/cbic.201402254
Jeronimo, L. P., Choi, M.-R., Yeon, S. H., Park, S. K., Yoon, Y. H., Choi, S. H., et al. (2020). Effects of Povidone‐iodine Composite on the Elimination of Bacterial Biofilm. Int. Forum Allergy Rhinol 10, 884–892. doi:10.1002/alr.22568
Jervis‐Bardy, J., Foreman, A., Bray, S., Tan, L., and Wormald, P.-J. (2011). Methylglyoxal‐infused Honey Mimics the Anti‐ Staphylococcus aureus Biofilm Activity of Manuka Honey: Potential Implication in Chronic Rhinosinusitis. Laryngoscope 121, 1104–1107. doi:10.1002/lary.21717
Johani, K., Malone, M., Jensen, S. O., Dickson, H. G., Gosbell, I. B., Hu, H., et al. (2018). Evaluation of Short Exposure Times of Antimicrobial Wound Solutions against Microbial Biofilms: from In Vitro to In Vivo. J. Antimicrob. Chemother. 73, 494–502. doi:10.1093/jac/dkx391
Jorge, P., Grzywacz, D., Kamysz, W., Lourenço, A., and Pereira, M. O. (2017). Searching for New Strategies against Biofilm Infections: Colistin-AMP Combinations against Pseudomonas aeruginosa and Staphylococcus aureus Single- and Double-Species Biofilms. PLoS ONE 12, e0174654. doi:10.1371/journal.pone.0174654
Kamaruzzaman, N. F., Chong, S. Q. Y., Edmondson-Brown, K. M., Ntow-Boahene, W., Bardiau, M., and Good, L. (2017). Bactericidal and Anti-biofilm Effects of Polyhexamethylene Biguanide in Models of Intracellular and Biofilm of Staphylococcus aureus Isolated from Bovine Mastitis. Front. Microbiol. 8, 1518. doi:10.3389/fmicb.2017.01518
Kang, J., Dietz, M. J., and Li, B. (2019). Antimicrobial Peptide LL-37 Is Bactericidal against Staphylococcus aureus Biofilms. PLoS ONE 14, e0216676. doi:10.1371/journal.pone.0216676
Karas, J. A., Wong, L. J. M., Paulin, O. K. A., Mazeh, A. C., Hussein, M. H., Li, J., et al. (2020). The Antimicrobial Activity of Cannabinoids. Antibiotics 9, 406. doi:10.3390/antibiotics9070406
Khondker, A., and Rheinstädter, M. C. (2020). How Do Bacterial Membranes Resist Polymyxin Antibiotics? Commun. Biol. 3, 77. doi:10.1038/s42003-020-0803-x
Kilty, S. J., AlMutari, D., Duval, M., Groleau, M. A., Nanassy, J. D., and Gomes, M. M. (2010). Manuka Honey: Histological Effect on Respiratory Mucosa. Am. J. Rhinol Allergy 24, e63–e66. doi:10.2500/ajra.2010.24.345310.2500/194589210791496519
Kilty, S. J., Duval, M., Chan, F. T., Ferris, W., and Slinger, R. (2011). Methylglyoxal: (Active Agent of Manuka Honey) In Vitro Activity against Bacterial Biofilms. Int. Forum Allergy Rhinol 1, 348–350. doi:10.1002/alr.20073
Kim, J. H., Rimmer, J., Mrad, N., Ahmadzada, S., and Harvey, R. J. (2015). Betadine Has a Ciliotoxic Effect on Ciliated Human Respiratory Cells. J. Laryngol. Otol 129 (Suppl. S1), S45–S50. doi:10.1017/s0022215114002746
Klinger-Strobel, M., Stein, C., Forstner, C., Makarewicz, O., and Pletz, M. W. (2017). Effects of Colistin on Biofilm Matrices of Escherichia coli and Staphylococcus aureus. Int. J. Antimicrob. Agents 49, 472–479. doi:10.1016/j.ijantimicag.2017.01.005
Ko, H. H. T., Lareu, R. R., Dix, B. R., and Hughes, J. D. (2018). In Vitro antibacterial Effects of Statins against Bacterial Pathogens Causing Skin Infections. Eur. J. Clin. Microbiol. Infect. Dis. 37, 1125–1135. doi:10.1007/s10096-018-3227-5
Kolpen, M., Appeldorff, C. F., Brandt, S., Mousavi, N., Kragh, K. N., Aydogan, S., et al. (2016). Increased Bactericidal Activity of Colistin on Pseudomonas aeruginosa Biofilms in Anaerobic Conditions. Pathog. Dis. 74, ftv086. doi:10.1093/femspd/ftv086
Krasowski, G., Junka, A., Paleczny, J., Czajkowska, J., Makomaska-Szaroszyk, E., Chodaczek, G., et al. (2021). In Vitro evaluation of Polihexanide, Octenidine and NaClO/HClO-Based Antiseptics against Biofilm Formed by Wound Pathogens. Membranes 11, 62. doi:10.3390/membranes11010062
Lee, V. S., Humphreys, I. M., Purcell, P. L., and Davis, G. E. (2017). Manuka Honey Sinus Irrigation for the Treatment of Chronic Rhinosinusitis: a Randomized Controlled Trial. Int. Forum Allergy Rhinol 7, 365–372. doi:10.1002/alr.21898
Lefebvre, E., Vighetto, C., Di Martino, P., Garde, V. L., and Seyer, D. (2016). Synergistic Antibiofilm Efficacy of Various Commercial Antiseptics, Enzymes and EDTA: a Study of Pseudomonas aeruginosa and Staphylococcus aureus Biofilms. Int. J. Antimicrob. Agents 48, 181–188. doi:10.1016/j.ijantimicag.2016.05.008
Lima, M. R., Ferreira, G. F., Neto, W. R. N., de Melo Monteiro, J., Santos, Á. R. C., Tavares, P. B., et al. (2019). Evaluation of the Interaction between Polymyxin B and Pseudomonas aeruginosa Biofilm and Planktonic Cells: Reactive Oxygen Species Induction and Zeta Potential. BMC Microbiol. 19, 115. doi:10.1186/s12866-019-1485-8
Lin, L., Tang, X., Wei, J., Dai, F., and Sun, G. (2017). Xylitol Nasal Irrigation in the Treatment of Chronic Rhinosinusitis. Am. J. Otolaryngol. 38, 383–389. doi:10.1016/j.amjoto.2017.03.006
Liu, F., Hansra, S., Crockford, G., Köster, W., Allan, B. J., Blondeau, J. M., et al. (2018). Tetrasodium EDTA Is Effective at Eradicating Biolms Formed by Clinically Relevant Microorganisms from Patients’ central Venous Catheters. mSphere 3, e00525–18. doi:10.1128/msphere.00525-18fi
Liu, Z., Lin, Y., Lu, Q., Li, F., Yu, J., Wang, Z., et al. (2017). In Vitro and In Vivo Activity of EDTA and Antibacterial Agents against the Biofilm of Mucoid Pseudomonas aeruginosa. Infection 45, 23–31. doi:10.1007/s15010-016-0905-z
Lux, C. A., Biswas, K., Taylor, M. W., and Douglas, R. G. (2022). The In Vitro Efficacy of Neutral Electrolysed Water and Povidone-Iodine against CRS-Associated Biofilms. Rhinology 60 (1), 73–80. doi:10.4193/rhin21.301
Machuca, J., Lopez-Rojas, R., Fernandez-Cuenca, F., and Pascual, Á. (2019). Comparative Activity of a Polyhexanide–Betaine Solution against Biofilms Produced by Multidrug-Resistant Bacteria Belonging to High-Risk Clones. J. Hosp. Infect. 103, e92–e96. doi:10.1016/j.jhin.2019.04.008
Mills, A. E. (1956). Staphylococcus Bacteriophage Lysate Aerosol Therapy of Sinusitis. Laryngoscope 66, 846–858. doi:10.1288/00005537-195607000-00004
Müller, G., and Kramer, A. (2008). Biocompatibility index of Antiseptic Agents by Parallel Assessment of Antimicrobial Activity and Cellular Cytotoxicity. J. Antimicrob. Chemother. 61, 1281–1287. doi:10.1093/jac/dkn125
Ooi, M. L., Drilling, A. J., Morales, S., Fong, S., Moraitis, S., Macias-Valle, L., et al. (2019a). Safety and Tolerability of Bacteriophage Therapy for Chronic Rhinosinusitis Due to Staphylococcus aureus. JAMA Otolaryngol. Head Neck Surg. 145, 723–729. doi:10.1001/jamaoto.2019.1191
Ooi, M. L., Jothin, A., Bennett, C., Ooi, E. H., Vreugde, S., Psaltis, A. J., et al. (2019b). Manuka Honey Sinus Irrigations in Recalcitrant Chronic Rhinosinusitis: Phase 1 Randomized, Single‐blinded, Placebo‐controlled Trial. Int. Forum Allergy Rhinol 9, 1470–1477. doi:10.1002/alr.22423
Ooi, M. L., Richter, K., Bennett, C., Macias-Valle, L., Vreugde, S., Psaltis, A. J., et al. (2018a). Topical Colloidal Silver for the Treatment of Recalcitrant Chronic Rhinosinusitis. Front. Microbiol. 9, 720. doi:10.3389/fmicb.2018.00720
Ooi, M. L., Richter, K., Drilling, A. J., Thomas, N., Prestidge, C. A., James, C., et al. (2018b). Safety and Efficacy of Topical Chitogel- Deferiprone-Gallium Protoporphyrin in Sheep Model. Front. Microbiol. 9, 917. doi:10.3389/fmicb.2018.00917
Orlandi, R. R., Kingdom, T. T., Smith, T. L., Bleier, B., DeConde, A., Luong, A. U., et al. (2021). International Consensus Statement on Allergy and Rhinology: Rhinosinusitis 2021. Int. Forum Allergy Rhinol 11, 213–739. doi:10.1002/alr.22741
Panchmatia, R., Payandeh, J., Al-Salman, R., Kakande, E., Habib, A.-R., Mullings, W., et al. (2019). The Efficacy of Diluted Topical Povidone-Iodine Rinses in the Management of Recalcitrant Chronic Rhinosinusitis: a Prospective Cohort Study. Eur. Arch. Otorhinolaryngol. 276, 3373–3381. doi:10.1007/s00405-019-05628-w
Papa, V., Meulen, I. V. D., Rottey, S., Sallet, G., Overweel, J., Asero, N., et al. (2020). Safety and Tolerability of Topical Polyhexamethylene Biguanide: a Randomised Clinical Trial in Healthy Adult Volunteers. Br. J. Ophthalmol. 106 (2), 190–196. doi:10.1136/bjophthalmol-2020-317848
Paramasivan, S., Drilling, A. J., Jardeleza, C., Jervis‐Bardy, J., Vreugde, S., and Wormald, P. J. (2014). Methylglyoxal‐augmented Manuka Honey as a Topical Anti– Staphylococcus aureus Biofilm Agent: Safety and Efficacy in an In Vivo Model. Int. Forum Allergy Rhinol 4, 187–195. doi:10.1002/alr.21264
Percival, S. L., and Salisbury, A.-M. (2017). “The Efficacy of Tetrasodium EDTA on Biofilms,” in Advances In Microbiology, Infectious Diseases And Public Health. Advances In Experimental Medicine And Biology Advances in Experimental Medicine and Biology. Editor G. Donelli (Cham: Springer), 101–110. doi:10.1007/5584_2017_134
Premkumar, A., Nishtala, S. N., Nguyen, J. T., Bostrom, M. P. G., and Carli, A. V. (2021). Comparing the Efficacy of Irrigation Solutions on Staphylococcal Biofilm Formed on Arthroplasty Surfaces. J. Arthroplasty 36, S26–S32. doi:10.1016/j.arth.2021.02.033
Psaltis, A. J., Wormald, P.-J., Ha, K. R., and Tan, L. W. (2008). Reduced Levels of Lactoferrin in Biofilm-Associated Chronic Rhinosinusitis. Laryngoscope 118, 895–901. doi:10.1097/mlg.0b013e31816381d4
Ramamourthy, G., and Vogel, H. J. (2021). Antibiofilm Activity of Lactoferrin-Derived Synthetic Peptides against Pseudomonas aeruginosa PAO1. Biochem. Cel Biol 99, 138–148. doi:10.1139/bcb-2020-0253
Ramezanpour, M., Smith, J. L. P., Psaltis, A. J., Wormald, P. J., and Vreugde, S. (2020). In Vitro safety Evaluation of a Povidone‐iodine Solution Applied to Human Nasal Epithelial Cells. Int. Forum Allergy Rhinol 10, 1141–1148. doi:10.1002/alr.22575
Reimer, K., Wichelhaus, T. A., Schäfer, V., Rudolph, P., Kramer, A., Wutzler, P., et al. (2002). Antimicrobial Effectiveness of Povidone-Iodine and Consequences for New Application Areas. Dermatology 204 (Suppl. 1), 114–120. doi:10.1159/000057738
Richter, K., Facal, P., Thomas, N., Vandecandelaere, I., Ramezanpour, M., Cooksley, C., et al. (2017a). Taking the Silver Bullet Colloidal Silver Particles for the Topical Treatment of Biofilm-Related Infections. ACS Appl. Mater. Inter. 9, 21631–21638. doi:10.1021/acsami.7b03672
Richter, K., Ramezanpour, M., Thomas, N., Prestidge, C. A., Wormald, P. J., and Vreugde, S. (2016). Mind “De GaPP”: In Vitro Efficacy of Deferiprone and Gallium‐protoporphyrin against Staphylococcus aureus Biofilms. Int. Forum Allergy Rhinol 6, 737–743. doi:10.1002/alr.21735
Richter, K., Thomas, N., Claeys, J., McGuane, J., Prestidge, C. A., Coenye, T., et al. (2017b). A Topical Hydrogel with Deferiprone and Gallium-Protoporphyrin Targets Bacterial Iron Metabolism and Has Antibiofilm Activity. Antimicrob. Agents Chemother. 61, e00481–17. doi:10.1128/aac.00481-17
Riechelmann, H., Deutschle, T., Stuhlmiller, A., Gronau, S., and Bürner, H. (2004). Nasal Toxicity of Benzalkonium Chloride. Am. J. Rhinol 18, 291–299. doi:10.1177/194589240401800506
Röhner, E., Jacob, B., Böhle, S., Rohe, S., Löffler, B., Matziolis, G., et al. (2020). Sodium Hypochlorite Is More Effective Than Chlorhexidine for Eradication of Bacterial Biofilm of Staphylococci and Pseudomonas aeruginosa. Knee Surg. Sports Traumatol. Arthrosc. 28, 3912–3918. doi:10.1007/s00167-020-05887-9
Roy, R., Tiwari, M., Donelli, G., and Tiwari, V. (2018). Strategies for Combating Bacterial Biofilms: a Focus on Anti-biofilm Agents and Their Mechanisms of Action. Virulence 9, 522–554. doi:10.1080/21505594.2017.1313372
Rudilla, H., Pérez-Guillén, I., Rabanal, F., Sierra, J. M., Vinuesa, T., and Viñas, M. (2018). Novel Synthetic Polymyxins Kill Gram-Positive Bacteria. J. Antimicrob. Chemother. 73, 3385–3390. doi:10.1093/jac/dky366
Sahariah, P., Másson, M., and Meyer, R. L. (2018). Quaternary Ammoniumyl Chitosan Derivatives for Eradication of Staphylococcus aureus Biofilms. Biomacromolecules 19, 3649–3658. doi:10.1021/acs.biomac.8b00718
Scheper, H., Wubbolts, J. M., Verhagen, J. A. M., de Visser, A. W., van der Wal, R. J. P., Visser, L. G., et al. (2021). SAAP-148 Eradicates MRSA Persisters within Mature Biofilm Models Simulating Prosthetic Joint Infection. Front. Microbiol. 12, 625952. doi:10.3389/fmicb.2021.625952
Schreier, H., Erdos, G., Reimer, K., König, B., König, W., and Fleischer, W. (1997). Molecular Effects of Povidone-Iodine on Relevant Microorganisms: an Electron-Microscopic and Biochemical Study. Dermatology 195 (Suppl. 2), 111–116. doi:10.1159/000246043
Severing, A.-L., Rembe, J.-D., Koester, V., and Stuermer, E. K. (2019). Safety and Efficacy Profiles of Different Commercial Sodium Hypochlorite/hypochlorous Acid Solutions (NaClO/HClO): Antimicrobial Efficacy, Cytotoxic Impact and Physicochemical Parameters In Vitro. J. Antimicrob. Chemother. 74, 365–372. doi:10.1093/jac/dky432
Shelanski, H. A., and Shelanski, M. V. (1956). PVP-iodine: History, Toxicity and Therapeutic Uses. J. Int. Coll. Surg. 25, 727–734.
Singhal, D., Foreman, A., Jervis-Bardy, J., and Wormald, P.-J. (2011). Staphylococcus aureus Biofilms: Nemesis of Endoscopic Sinus Surgery. Laryngoscope 121, 1578–1583. doi:10.1002/lary.21805
Siu, J., Klingler, L., Wang, Y., Hung, C.-T., Jeong, S. H., Smith, S., et al. (2020). Oral Antibiotics Used in the Treatment of Chronic Rhinosinusitis Have Limited Penetration into the Sinonasal Mucosa: a Randomized Trial. Xenobiotica 50, 1443–1450. doi:10.1080/00498254.2020.1814973
Sivaranjani, M., Liu, F., and White, A. P. (2021). Synergistic Activity of Tetrasodium EDTA, Ethanol and Chlorhexidine Hydrochloride against Planktonic and Biofilm Cells of Clinically Relevant Pathogens. J. Glob. Antimicrob. Resist. 24, 148–157. doi:10.1016/j.jgar.2020.12.002
Stewart, P. S., and Costerton, J. W. (2001). Antibiotic Resistance of Bacteria in Biofilms. Lancet 358, 135–138. doi:10.1016/s0140-6736(01)05321-1
Su, M., Wang, M., Hong, Y., Nimmagadda, A., Shen, N., Shi, Y., et al. (2019). Polymyxin Derivatives as Broad-Spectrum Antibiotic Agents. Chem. Commun. 55, 13104–13107. doi:10.1039/c9cc06908a
Sultan, S., Murarka, S., Jahangir, A., Mookadam, F., Tajik, A. J., and Jahangir, A. (2017). Chelation Therapy in Cardiovascular Disease: an Update. Expert Rev. Clin. Pharmacol. 10, 843–854. doi:10.1080/17512433.2017.1339601
Taylor, A., Fuzi, J., Sideris, A., Banks, C., and Havas, T. E. (2021). Non-steroid, Non-antibiotic Anti-biofilm Therapy for the Treatment of Chronic Rhinosinusitis: a Systematic Review. J. Laryngol. Otol 135, 196–205. doi:10.1017/s0022215121000542
Thangamani, S., Mohammad, H., Abushahba, M. F. N., Hamed, M. I., Sobreira, T. J. P., Hedrick, V. E., et al. (2015). Exploring Simvastatin, an Antihyperlipidemic Drug, as a Potential Topical Antibacterial Agent. Sci. Rep. 5, 16407. doi:10.1038/srep16407
Thieme, L., Hartung, A., Tramm, K., Klinger-Strobel, M., Jandt, K. D., Makarewicz, O., et al. (2019). MBEC versus MBIC: the Lack of Differentiation between Biofilm Reducing and Inhibitory Effects as a Current Problem in Biofilm Methodology. Biol. Proced. Online 21, 18. doi:10.1186/s12575-019-0106-0
Thorn, R. M. S., Lee, S. W. H., Robinson, G. M., Greenman, J., and Reynolds, D. M. (2012). Electrochemically Activated Solutions: Evidence for Antimicrobial Efficacy and Applications in Healthcare Environments. Eur. J. Clin. Microbiol. Infect. Dis. 31, 641–653. doi:10.1007/s10096-011-1369-9
Turner, J. H., Wu, J., Dorminy, C. A., and Chandra, R. K. (2017). Safety and Tolerability of Surfactant Nasal Irrigation. Int. Forum Allergy Rhinol 7, 809–812. doi:10.1002/alr.21959
Valentine, R., Jervis-Bardy, J., Psaltis, A., Tan, L. W., and Wormald, P.-J. (2011). Efficacy of Using a Hydrodebrider and of Citric Acid/zwitterionic Surfactant on a Staphylococcus aureus Bacterial Biofilm in the Sheep Model of Rhinosinusitis. Am. J. Rhinol Allergy 25, 323–326. doi:10.2500/ajra.2011.25.3659
van de Donk, H. J. M., Muller-Plantema, I. P., Zuidema, J., and Merkus, F. W. H. M. (1980). The Effects of Preservatives on the Ciliary Beat Frequency of Chicken Embryo Tracheas. Rhinology 18, 119–133.
van Klingeren, B., and ten Ham, M. (1976). Antibacterial Activity of Δ9-tetrahydrocannabinol and Cannabidiol. Antonie van Leeuwenhoek 42, 9–12. doi:10.1007/BF00399444
Verderosa, A. D., Totsika, M., and Fairfull-Smith, K. E. (2019). Bacterial Biofilm Eradication Agents: a Current Review. Front. Chem. 7, 824. doi:10.3389/fchem.2019.00824
Vickery, T. W., Ramakrishnan, V. R., and Suh, J. D. (2019). The Role of Staphylococcus aureus in Patients with Chronic Sinusitis and Nasal Polyposis. Curr. Allergy Asthma Rep. 19, 21. doi:10.1007/s11882-019-0853-7
Wagner Mackenzie, B., Waite, D. W., Hoggard, M., Douglas, R. G., Taylor, M. W., and Biswas, K. (2017). Bacterial Community Collapse: a Meta‐analysis of the Sinonasal Microbiota in Chronic Rhinosinusitis. Environ. Microbiol. 19, 381–392. doi:10.1111/1462-2920.13632
Wagner Mackenzie, B., West, A. G., Waite, D. W., Lux, C. A., Douglas, R. G., Taylor, M. W., et al. (2020). A Novel Description of the Human Sinus Archaeome during Health and Chronic Rhinosinusitis. Front. Cel Infect Microbiol 10, 398. doi:10.3389/fcimb.2020.00398
Weissman, J. D., Fernandez, F., and Hwang, P. H. (2011). Xylitol Nasal Irrigation in the Management of Chronic Rhinosinusitis: a Pilot Study. Laryngoscope 121, 2468–2472. doi:10.1002/lary.22176
Wu, P.-H., Cheng, P.-C., Chang, C.-M., Lo, W.-C., and Cheng, P.-W. (2021). Efficacy of Povidone‐iodine Nasal Irrigation Solution after Sinonasal Surgery: a Randomized Controlled Study. Laryngoscope 1, 1. doi:10.1002/lary.29818
Yin, J., Meng, Q., Cheng, D., Fu, J., Luo, Q., Liu, Y., et al. (2020). Mechanisms of Bactericidal Action and Resistance of Polymyxins for Gram-Positive Bacteria. Appl. Microbiol. Biotechnol. 104, 3771–3780. doi:10.1007/s00253-020-10525-y
Yoshida, T., and Hiramatsu, K. (1993). Potent In Vitro Bactericidal Activity of Polymyxin B against Methicillin‐resistant Staphylococcus aureus (MRSA). Microbiol. Immunol. 37, 853–859. doi:10.1111/j.1348-0421.1993.tb01716.x
Keywords: antibiofilm agents, biofilms, chronic rhinosinusitis (CRS), dysbiosis, iodine, topical therapies, Pseudomonas aeruginosa, Staphyloccocus aureus
Citation: Hale SJM, Wagner Mackenzie B, Lux CA, Biswas K, Kim R and Douglas RG (2022) Topical Antibiofilm Agents With Potential Utility in the Treatment of Chronic Rhinosinusitis: A Narrative Review. Front. Pharmacol. 13:840323. doi: 10.3389/fphar.2022.840323
Received: 21 December 2021; Accepted: 24 March 2022;
Published: 13 June 2022.
Edited by:
Hong Zhou, Zunyi Medical University, ChinaCopyright © 2022 Hale, Wagner Mackenzie, Lux, Biswas, Kim and Douglas. This is an open-access article distributed under the terms of the Creative Commons Attribution License (CC BY). The use, distribution or reproduction in other forums is permitted, provided the original author(s) and the copyright owner(s) are credited and that the original publication in this journal is cited, in accordance with accepted academic practice. No use, distribution or reproduction is permitted which does not comply with these terms.
*Correspondence: Richard G. Douglas, cmljaGFyZC5kb3VnbGFzQGF1Y2tsYW5kLmFjLm56
Disclaimer: All claims expressed in this article are solely those of the authors and do not necessarily represent those of their affiliated organizations, or those of the publisher, the editors and the reviewers. Any product that may be evaluated in this article or claim that may be made by its manufacturer is not guaranteed or endorsed by the publisher.
Research integrity at Frontiers
Learn more about the work of our research integrity team to safeguard the quality of each article we publish.