- 1Division of Dermatology, Department of Pediatrics, Faculty of Medicine, Chulalongkorn University, Bangkok, Thailand
- 2The Pediatrics-Thai Severe Cutaneous Adverse Drug Reaction (Ped-Thai-SCAR) Research Group, Bangkok, Thailand
- 3Division of Pharmacogenomics and Personalized Medicine, Department of Pathology, Faculty of Medicine Ramathibodi Hospital, Mahidol University, Bangkok, Thailand
- 4Laboratory for Pharmacogenomics, Somdech Phra Debaratana Medical Center (SDMC), Ramathibodi Hospital, Bangkok, Thailand
- 5The Thai Severe Cutaneous Adverse Drug Reaction (Thai-SCAR) Research Group, Bangkok, Thailand
- 6Division of Dermatology, Department of Medicine, Faculty of Medicine, Skin, and Allergy Research Unit, Chulalongkorn University, Bangkok, Thailand
- 7Division of General Pharmacy Practice, Department of Pharmaceutical Care, College of Pharmacy, Rangsit University, Pathum Thani, Thailand
- 8Excellence Pharmacogenomics and Precision Medicine Centre, College of Pharmacy, Rangsit University, Pathum Thani, Thailand
- 9Pharmacogenomics and Precision Medicine, The Preventive Genomics & Family Check-up Services Center, Bumrungrad International Hospital, Bangkok, Thailand
- 10MRC Centre for Drug Safety Science, Department of Pharmacology and Therapeutics, Institute of Systems, Molecular and Integrative Biology, University of Liverpool, Liverpool, United Kingdom
SCARs are rare and life-threatening hypersensitivity reactions. In general, the increased duration of hospital stays and the associated cost burden are common issues, and in the worst-case scenario, they can result in mortality. SCARs are delayed T cell-mediated hypersensitivity reactions. Recovery can take from 2 weeks to many months after dechallenging the culprit drugs. Genetic polymorphism of the HLA genes may change the selection and presentation of antigens, allowing toxic drug metabolites to initiate immunological reactions. However, each SCARs has a different onset latency period, clinical features, or morphological pattern. This explains that, other than HLA mutations, other immuno-pathogenesis may be involved in drug-induced severe cutaneous reactions. This review will discuss the clinical morphology of various SCARs, various immune pathogenesis models, diagnostic criteria, treatments, the association of various drug-induced reactions and susceptible alleles in different populations, and the successful implementation of pharmacogenomics in Thailand for the prevention of SCARs.
Introduction
Cutaneous adverse drug reactions (CADRs) include a wide range of clinical symptoms, from moderate and self-limited cutaneous eruptions such as maculopapular exanthema (MPE) to severe cutaneous adverse drug reactions (SCARs) (Alvestad et al., 2007). CADRs are delayed-type hypersensitivity reactions. Usually, the inflammation sign starts within 12–24 h or sometimes after many days of drug exposure (Patel et al., 2014a). Though these reactions are usually mild and can be treated by reducing the dose of the culprit drug or by simple treatments, SCARs need hospitalization. In very severe cases, intensive care is necessary. Other concerns for patients with SCARs include longer hospital stays if they are already hospitalized, extra-economic load, and death (Campos-Fernández et al., 2005).
SCARs can manifest in a variety of ways, the most common SCAR is Stevens−Johnson syndrome/toxic epidermal necrolysis (SJS/TEN), followed by drug reaction with eosinophilia and systemic symptoms (DRESS) and acute generalized exanthematous pustulosis (AGEP). Antibiotics are the most commonly implicated medications of SCARs, followed by anti-epileptic drugs (AEDs), allopurinol, and non-steroidal anti-inflammatory drugs (NSAIDs). Genetic mutations, in addition to clinical and non-clinical factors, have a significant influence on the development of SCARs (Fowler et al., 2019).
Based on our ThaiSCAR research experience, this review not only provides a pragmatic understanding of SCARs but also recommends pharmacogenetic testing (PGx) before treatment. Our goal is to inform about the prevalence of SCARs in different ethnic groups; how clinical features differ for different SCARs; what pathogenesis mechanisms each SCARs may have; diagnostic challenges and established diagnostic criteria; drug-specific, phenotype-specific, and ethnicity-specific pharmacogenetic variants associated with SCARs; and the importance of PGx testing in predicting and preventing SCARs.
Epidemiology
CADRs affect 1–3% of people in developed nations and 2–5% of people in developing countries (Bigby, 2001; Craig et al., 2001). SCARs are rare reactions, yet they have a significant mortality rate. SCARs have a global frequency of 0.4–1.2 cases per million per year, with a 14–70 percent death rate (Verma et al., 2013). According to a prospective population-based registry in Germany, the annual incidence of SCARs was 1.53–1.89 per million People (Mockenhaupt, 2012a). However, the incidence rate of SCARs is reported to be higher among East Asians. The prevalence of SCARs was reported to be 0.32/1000 hospitalizations in a Beijing study (Li and Ma, 2006). Another prospective study in Singapore found a 5.2% SCAR incidence and a 0.09/1000 hospital death rate due to allergies (Thong et al., 2003). SJS/TEN is the most prevalent SCAR among SCARs, followed by DRESS, AGEP, SJS/TEN accounted for nearly 67% of the 755 SCAR cases documented in the Korean ADRs system, followed by DRESS at 32.7% (Kang et al., 2019).
Many epidemiological studies have found the same type of SCARs occurrence pattern in different populations. These events, however, may vary depending on the drug. According to the same Korean ADRs system study, DRESS was the most prevalent SCARs for allopurinol, with an 11.3% incidence rate compared to SJS/TEN (10.2%) (Kang et al., 2019). In case of antibiotics and phenytoin (PHT), AGEP and DRESS were prevalent SCARs. Among many drug categories, antibiotics are the ones that reported the highest number of SCARs in many studies (Choon and Lai, 2012; Loo et al., 2018). The second most common pharmacological class involved in SCARs is AEDs. Antibiotics (29.5%) and anticonvulsants (24.1%), for example, were the most common causal drug classes in cohort research conducted at Fujian Medical University (Loo et al., 2018). A similar implication pattern on SCARs was discovered in retrospective research conducted in Singapore. Though no gender disparity in the occurrence of SCAR was found in some studies (Su and Aw, 2014), Patients' age ranged from 40.8 to 56 years old (21 years), with a mean age of 40.8 years, are vulnerable to SCARs (Patel et al., 2013; Yang et al., 2018).
Clinical Manifestation
SJS/TEN
In about one-third of cases, SJS/TEN is preceded by non-specific prodromal symptoms lasting for 1–7 days. For the first few days, SJS/TEN is characterized by erythroderma. The erythrodermic rashes then develop into erythematous macules with purpuric centers and blisters in a symmetrical pattern. These severe cutaneous lesions aggressively detach the epidermis and cause mucous membrane erosion. Stinging eyes, photophobia, malaise, fever, headache, anorexia, sore throat, and pseudo-membrane formation of the eyes and genitalia are other pertinent symptoms of SJS/TEN. SJS and TEN can be distinguished by the extent of the epidermal detachment. As a result, the degree of body surface area (BSA) involvement is determined by adding all blisters and skin detachment together (Saha et al., 2012; Tan and Tay, 2012).
In SJS, SJS/TEN overlapping, and TEN, the BSA of involved skin detachment was less than 10%, 10–30%, and more than 30%, respectively. Nikolsky sign is manifested by a dermal-epidermal cleavage when applying tangential pressure to apparently intact skin, even this sign was not pathognomonic but also helpful to diagnose this condition. At least two mucous membrane involvements were found in 95% of SJS/TEN cases. Edematous, erythematous, and flaccid bullae lesions are other clinical features generally followed by painful, burning sensations in the conjunctivae, oral cavity, and genitalia (Figure 1). The most commonly affected systems in SJS/TEN patients are the respiratory system (pneumonitis, bronchial hypersecretion), gastrointestinal system (transaminitis, hematemesis, gastrointestinal hemorrhage, esophageal stricture), and renal system (micro-albuminuria, hematuria, interstitial nephritis, acute renal failure). In SJS/TEN patients, however, septicemia is the leading cause of death, with Staphylococcus aureus and Pseudomonas aeruginosa being the most common pathogens. Multiple organ failure is caused by septicemia, hypovolemia, hypoalbuminemia, and transaminitis (Schwartz et al., 2013).
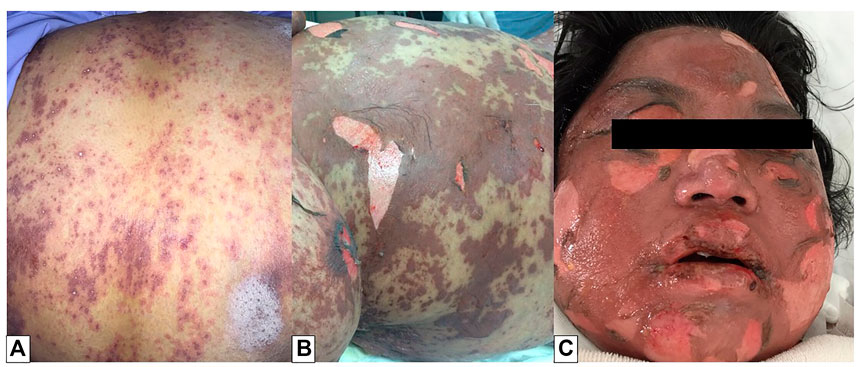
FIGURE 1. Toxic epidermal necrolysis (TEN). Multiple macules with central dusky red (atypical target lesions) were found on the trunk (A). The lesions progressed to flaccid bullae and large sheets of skin necrosis, leading to diffuse erythema. Nikolsky’s sign was also positive (B). Facial, oral, and ocular involvements were noted (C).
DRESS
The most prevalent cutaneous sign of DRESS is a polymorphous maculopapular eruption, which is followed by exfoliation and desquamation. The skin lesion starts on the face, with periorbital and facial edema, and then spreads to the upper torso and lower limbs in 25–76% of cases (Watanabe, 2018). Pustules, purpura, plaques, blisters, target-like lesions, urticarial lesions, and lichenoid lesions are also seen in DRESS patients (Figure 2).
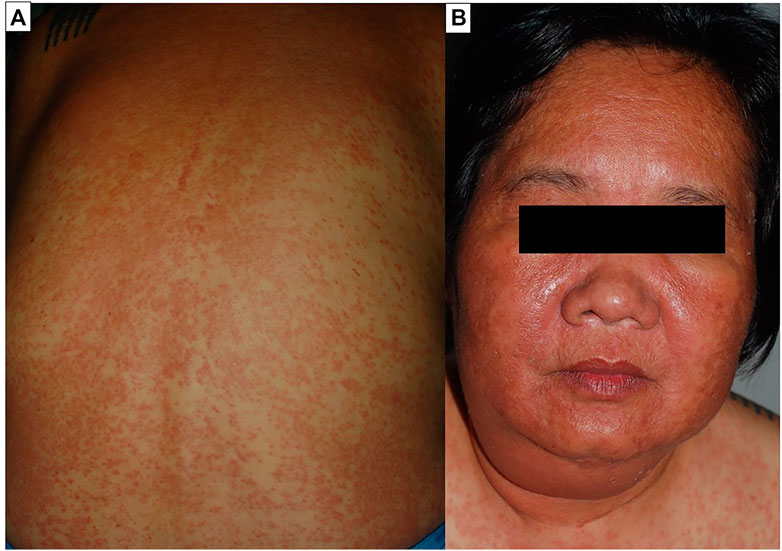
FIGURE 2. Drug reaction with eosinophilia and systemic symptoms (DRESS). Diffuse maculopapular eruption involving more than 50% of body surface area. The plaques were infiltrated and exhibited follicular accentuation (A). Facial edema is a prominent feature of the syndrome (B).
The natural course of dermatologic presentation in DRESS is frequently diverse and recurs for several weeks, with an average duration for clinical improvement of 6–9 weeks (average 21.8 days) (Spriet and Banks, 2015a; Kim et al., 2020a). The recurrence, on the contrary, could have been prompted by exposure to a drug that was unrelated to the offending substances and had weaker symptoms than the earlier manifestation. Mucosal involvement can be found in about 56% of cases at lip and mucosa (Kardaun et al., 2013a). Internal organ involvement, such as lymphadenopathy and hematological disorders (leukocytosis, eosinophilia, atypical lymphocytes, thrombocytopenia), is common in DRESS. The most prevalent systemic manifestation is hepatic involvement, followed by renal, pulmonary, neurological, and cardiac involvement (Cacoub et al., 2011). The most serious sequela is hepatic necrosis, which causes severe liver injury or sudden liver failure, followed by coagulopathy and sepsis, which is the major cause of death (Husain et al., 2013a). Endocrine involvement is uncommon, although it has long-term consequences. Thyroid gland is the most typically impacted gland (Kano et al., 2010; Tempark et al., 2022).
AGEP
AGEP manifests as a fever with rapidly spreading multiple pinhead-sized, sterile, non-follicular pustules on an erythematous and edematous base, particularly in the intertriginous zones of the neck, sub-mammary, inguinal, axillary area, trunk, and upper extremities. Pruritus with or without a burning sensation is reported by some patients. TEN-like AGEP (TEN-AGEP overlap) and AGEP-DRESS overlap are two examples of atypical systemic presentation. Atypical localized AGEP, also known as atypical localized exanthematous pustulosis (ALEP), is a kind of AGEP that affects the face, neck, and chest (Villani et al., 2017) (Figure 3). Fever and leukocytosis are the first symptoms, followed by isolated non-follicular pustules at the site. Ryder ENC proposed the Acute localized exanthematous pustulosis diagnosis criteria recently (Ryder and Perkins, 2018). Leukocytosis (>10,000/ml) and an increased neutrophil count (neutrophilia >7,000/ml) are found in the majority of AGEP patients. Eosinophilia is prevalent in about 30% of patients. Hepatic dysfunction, renal insufficiency, respiratory distress, agranulocytosis, and lymphadenopathy are examples of multi-organ involvement that have been documented in roughly 17% of patients (Szatkowski and Schwartz, 2015a).
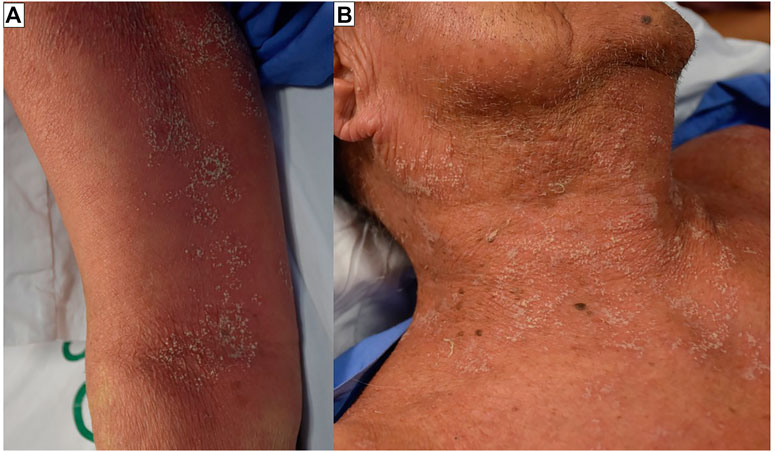
FIGURE 3. Acute generalized exanthematous pustulosis (AGEP). Multiple pin-head sized, non-follicular pustules on edematous, homogenous, poorly demarcated, erythematous background. The lesions were found mainly on the folds of the body (A). The pustules can become confluent (B).
GBFDE is differentiated from FDE by the occurrence of widespread bullae and erosions. The most commonly affected locations are the head, neck, anterior and posterior trunck, upper and lower limbs, and genitalia. The 10% skin detachment is discovered in several cases (Figure 4). The onset of cutaneous symptoms can help distinguish GBFDE from SJS/TEN. FDE symptoms, for example, usually appear within 30 min to 24 h after exposure to the culprit drug, whereas SJS/TEN symptoms appear 1 to 3 weeks. Another noteworthy distinction is the affliction site; because FDE recurs at the same location, more FDE/GBFDE patients have a positive past allergy history compared to SJS/TEN patients (Mitre et al., 2017).
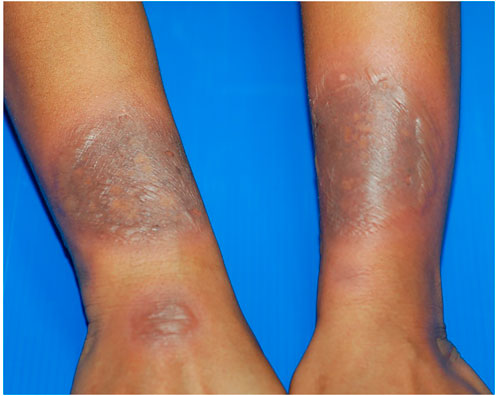
FIGURE 4. Generalized bullous fixed drug eruption (GBFDE). Multiple large and well-demarcated round to oval plaques with blisters formation. The lesions were found at different sites of the body. Fewer than two sites of the mucous membrane were involved. The patient has experienced a similar reaction in the past.
Investigation
Skin patch testing, lymphocyte transformation test (LTT), and ELISpot assay were three effective ways for finding the culprit drug for SCARs (Husain et al., 2013b). Positive patch tests produce a clinically red spots on the skin due to the recruitment of inflammatory cells and can be used to prove the activation of drug-specific T cells. This test should be carried out on the previously afflicted site. However, the type of drug, drug concentration, formulation (intravenous powder, capsule), diluents used, patch testing site, and duration after exposure are different variables that can affect the interpretation of patch test results. According to the recommendation of the International Contact Dermatitis Research Group (ICDRG), a patch test should be performed during the 6 months following DRESS and at least 1 month after cessation of immunosuppressant (Barbaud, 2014).
Multiple factors could affect the sensitivity and specificity of patch testing. A positive patch test is useful to confirm an inflammatory cutaneous hypersensitivity reaction, but a negative test cannot exclude the causative drugs (Husain et al., 2013b). Studies showed a higher positive predictive value for the patch test than the negative predictive value. For example, among AEDs, the patch test of CBZ shows a high positive predictive value of about 80%–90% for DRESS (Shanbhag et al., 2020), but it ranges only 10%–20% for phenobarbitol (Elzagallaai et al., 2009; Ohtoshi et al., 2014; Correa-de-Castro et al., 2016a). According to a multicenter study conducted by Barbaud et al., the comparative value of patch testing for the diagnosis of AGEP, DRESS, and SJS/TEN was 58%, 64%, and 24%, respectively (Barbaud, 2014).
The lymphocyte transformation test (LTT) is an in vitro test for delayed hypersensitivity reaction that measures radioactive thymidine incorporation into the DNA of proliferating cells to detect regulatory T cell activation after pharmacological stimulation. In DRESS and drug-induced hypersensitivity syndrome (DIHS), these tests can be conducted from 5–7 weeks to 1 year after the commencement of the rash (Kano et al., 2007; Fernando, 2014). However, for SJS/TEN, LTT must be performed within the first week of rash development (Kano et al., 2007; Ferrandiz-Pulido and Garcia-Patos, 2013). Although drug identification was not done on a regular basis, the LTT sensitivity was positive in 21%–56% of these patients (Kano et al., 2007; Porebski et al., 2013), and it is much higher for AGEP, DRESS (Hassoun-Kheir et al., 2016; Pinho et al., 2017). ELISpot assay is the in vitro test to access functional Tregs on cytokine production in the acute phase of DRESS by drug-specific interferon-γ releasing cell. In cultured peripheral blood mononuclear cells (PBMCs) with a suspected drug, the IFN-ELISpot assay evaluates IFN produced by DRESS cells. This assay is drug-specific; several studies, for example, beta-lactam-induced maculopapular rash (Suthumchai et al., 2018a) and allopurinol-induced SCARs (Rozieres et al., 2009), have verified the drug-specific release of IFN (Klaewsongkram et al., 2016).
Causative Agents and Risk Factors of SCARs
The most common cause of SJS/TEN is drug administration; however, other reasons may account for 5%–15% (Sassolas et al., 2010a). The prevalence of SCARs varies according to the medications used and the age of the patient. Common culprit drugs in DRESS include AEDs (carbamazepine, lamotrigine, phenobarbital, phenytoin), antibiotics (amoxicillin, ampicillin, azithromycin, levofloxacin, minocycline), anti-tuberculosis agents (ethambutol, isoniazid, rifampicin, pyrazinamide), analgesics (diclofenac, ibuprofen, aspirin, celecoxib), sulfonamides (dapsone, sulfamethoxazole-trimethoprim, sulfasalazine), anti-viral (abacavir, nevirapine), anti-hypertensive (amlodipine, captopril), allopurinol, and amitriptyline. Aminopenicillin, quinolones, hydroxychloroquine, sulfonamides, terbinafine, and diltiazem were the most common causes of AGEP in the EuroSCAR study. On the contrary, corticosteroids, macrolides, oxicam, NSAIDs, and antiepileptic medications have a weak connection with AGEP (Szatkowski and Schwartz, 2015b).
The onset of SCARs varies depending on the type of SCARs. For example, the onset of DRESS patients ranges from 2 to 8 weeks (average 22.2 days, range 0.42–53 days) (Bocquet et al., 1996; Schwartz et al., 2013; Kim et al., 2020b), whereas it ranges from 24 to 48 h (1 hour to 25 days) (Halevy, 2009; Hadavand et al., 2020). Similarly, there were disparities between children and adults in terms of the causative substance, associated causes, and severity. Sulfonamides, aromatic anticonvulsants (carbamazepine, phenytoin, and phenobarbital), penicillin, and NSAIDs were prevalent implicated drugs in children, while allopurinol, oxicam, NSAIDs, and nevirapine induced SCARs were common in adults (Ferrandiz-Pulido and Garcia-Patos, 2013). After being exposed to the probable drugs, the rash usually appears within 8 weeks (Ferrandiz-Pulido and Garcia-Patos, 2013). In the prior literature, carbamazepine has been identified as the most common causal agent in adult and pediatric DRESS patients (Bocquet et al., 1996; Cacoub et al., 2011; Spriet and Banks, 2015b). However, according to Oberlin KE et al., antibiotics (cephalosporin, trimethoprim-sulfamethoxazole, and vancomycin) were the most common cause of pediatric DRESS (Zhou et al., 20161936; Oberlin et al., 2019).
Identifying the risk factors and understanding their pathogenesis can help optimize therapy. The risk factors are generally 1) immune-mediated: HLA allele genetic polymorphisms can cause these reactions; these variants can differ in every population, drug, and phenotype; and they are metabolism independent and 2) non-immune mediated: these reactions can be caused by genetic abnormalities in genes that encode enzymes involved in drug metabolism, as well as patient, disease, and drug-related variables (Galindo et al., 2002).
Genetic Factors
Roujeau et al. were the first to report an association between HLA and SJS in 1986, the year after he confirmed an association between HLA and TEN. The suspected alleles of SCARs could be drug-specific, ethnicity-specific, and phenotypic-specific. Abacavir and HLA-B* 57:01 were the subjects of the first groundbreaking studies on immune-mediated reactions (Mallal et al., 2002; Hughes et al., 2009). Many clinical guideline agencies recommended the pre-prescription genotyping of HLA-B* 57:01 before initiating the abacavir treatment. The second breakthrough research in ADR-pharmacogenetics was reported by Chung et al., showing a strong association between SJS/TEN and HLA-B* 15:02 among the Han Chinese population in Taiwan in 2004 (Chung et al., 2004). Later, this association was confirmed in many other populations, including Chinese (Wang et al., 2011), Thai (Tassaneeyakul et al., 2010a), Malaysians (Chang et al., 2011a), Indian (Mehta et al., 2009), Vietnamese (Nguyen et al., 2015a), and Indonesians (Yuliwulandari et al., 2017). On the contrary, the involvement of HLA-A* 31:01 in CBZ induced SJS/TEN has been demonstrated in Western and Japanese populations (Kashiwagi et al., 2008a; McCormack et al., 2011). As a result, the FDA recommended a pharmacogenetic (PGx) biomarker classification system based on dependability, scientific evidence, and clinical utility (US Department of Health and Human Services, United States Food and Drug Administration, August 2011) (US Department of Health and Human Services, 2011). It provides a valuable framework for evaluating putative biomarkers, although the marker has less positive predictive value. Table 1 shows the most associated HLA alleles with SCARs in various population.
Non-Genetic Factors
Identifying the risk alleles and their predictive positive and negative values for the occurrence of SCARs can be helpful in the optimization of therapy. However, defects in several gene loci cannot be the only reason for any SCARs. Recognizing the other non-genetic factors and the level of their association with each SCARs can help increase specificity (Table 2). Overall, to establish a highly specific treatment strategy, both genetic and non-genetic factors should be studied in depth. For example, the incidence of SJS among children on lamotrigine (LTG) treatment has been estimated to be as high as 1:100 compared to adults 1:1000 (Dreifuss et al., 1987a). Recent Thai research, on the contrary, has found no link between age and the occurrence of AED-induced CADRs (Yampayon et al., 2017a; Sukasem et al., 2020a). However, in gender, women are at high risk of developing CADRs (Tran et al., 1998).
A study conducted by Alvestad et al. reported that a striking difference was seen among males (8%) and females (19%) with p < 0.001 (Alvestad et al., 2007). The explained reasons for this are that the immunological process of rash is influenced by sex hormones. The female sex steroids augment the immune response both pathologically and physiologically, whereas androgens inhibit the inflammatory response more than endogenous glucocorticoid (Talal, 1987; Da Silva, 1999; Osman, 2003).
The history of drug allergy and family history were shown to be associated with SCARs. A study conducted by Asakura et al. (2001) for 5 years reported that 14% of patients had a positive family history for CADRs and Campos-Fernández et al. (2005) reported 23% of family history. The risks for CADRs vary as per the patients’ ethnicity. The incidence rate was usually higher among East Asians, especially Han Chinese. A study from Thailand confirmed that Chinese ethnicity was associated with phenytoin- (PHT-) induced SJS/TEN (Yampayon et al., 2017a). The drug interactions between concurrent drugs may cause CADRs. Concurrent administration of valproic acid (VPA) and LTG increases the risk for CADRs. The reason for it is the inhibiting effect of VPA on uridine diphosphate glucuronyltransferase. According to a 2007 study by Alvestad et al. (2007b), administering VPA with PHT enhances the likelihood of PHT-induced rashes by displacing PHT from protein binding sites and perhaps accumulating reactive PHT metabolites.
Patients comorbid can be a predisposition to CADRs. A systematic review study from India has reported that the commonly reported comorbid conditions were diabetes mellitus (DM) (0.84%), human immunodeficiency virus (HIV) (0.65%), systemic lupus erythematosus (SLE) (0.56%), and hypertension (HTN) (0.28%) in CADRs patients (Patel et al., 2014b).
In the overlap and initiation of SCARs, the underlying illnesses and infection history play a significant role. Infection with Mycoplasma pneumoniae, for example, is the second most prevalent cause of SJS in children. However, an infection-induced rash may be less severe than drug-induced SJS/TEN. Coxsackievirus, influenza, herpes simplex virus, cytomegalovirus, parvovirus, varicella-zoster virus, Epstein–Barr virus, measles virus, human herpesvirus types 6 and 7 (HHV-6, HHV-7), Streptococcus group A, mycobacterium, and rickettsia are among the infection-induced SJS/TEN (Kunimi et al., 2011; Ferrandiz-Pulido and Garcia-Patos, 2013), occurring 1 week before the rash appears. Human herpesvirus- (HHV-) 6, HHV-7, Epstein–Barr virus (EBV), and cytomegalovirus are also thought to be responsible for reactivating the DRESS symptoms. Patients should have their viral load and antibody titers checked, especially if they have severe cases that have not responded to systemic corticosteroids. A spider bite, viral infection (Coxsackie B4, cytomegalovirus, parvovirus B19), bacterial infection (Mycoplasma pneumoniae, Chlamydial pneumoniae, Escherichia coli), and parasitic infestation have all been documented as AGEP trigger factors (Szatkowski and Schwartz, 2015c; Hadavand et al., 2020).
Diagnosis
SCARs have a wide range of clinical characteristics, making diagnosis difficult. Differential diagnoses for SCARs include viral infections, malignancy, hypereosinophilic syndrome, and lymphadenopathy (Fiszenson-Albala et al., 2003). SJS/TEN patients are more likely to have skin detachment and mucosal involvement, whereas DRESS patients are more likely to have organ involvement. Although most reactions are delayed hypersensitivity reactions, the onset latency duration is crucial in SCARs diagnosis. Due to pharmacokinetic features, it may differ depending on the drug or class of drugs. The latency period for phenytoin, for example, might last up to 30 days or more, whereas the latency period of SCARs for CBZ is 7–15 days (John et al., 2021b). When suspecting a substance for SCARs, time and frequency of drug exposure are important factors to examine. AEDs, antibiotics, and allopurinol, for example, are all suspected of being involved with SCARs (Pichler, 2007). As a result, new users of these medications are at risk. As a result, the history and duration of drug exposure, as well as a physical examination, are suspected of diagnosing this disorder.
Because the clinical aspects and duration of each SCARs differ, dermatologists can identify it using a range of established diagnostic criteria. The ALDEN (algorithm of drug causality for TEN) algorithm determines the diagnostic possibility (very likely, probable, possible, unlikely, and extremely unlikely) using six criteria (Sassolas et al., 2010b). As validation criteria for DRESS, the diagnostic criteria, RegiSCAR, Bocquet’s criteria, and J-SCAR were presented (Palmieri et al., 2002). However, there were some differences between these criteria, such as the drug exposure length, evidence of HHV reactivation, and other organ involvement. Clinical signs, histology, and other tests can all be used to diagnose AGEP. Using morphology, clinical history, and histology, the EuroSCAR study group offered four categories of AGEP validation score: no AGEP (score 0), possible (scores 1–4), probable (scores 5–7), and confirmed AGEP (scores 8–12) (Sukasem et al., 2018).
Treatment
In order to reduce morbidity and death in all SCARs, early diagnosis and drug withdrawal of the potentially causative substance are required. The treatment of SJS/TEN necessitates a multidisciplinary approach. The death rate may drop from 51.4 % to 29.8% when patients are referred to a specialized intensive care unit (ICU) or comparable high-dependency unit and/or the burn unit for optimal supportive care within days (Zimmermann et al., 2017). Supportive treatment typically includes skin mucosa and wound management, air-fluidized beds, thermoregulation, airway protection, fluid replacement, and fluid balance assessment. The need for nutritional assistance, eye care, and pain control cannot be overstated. Secondary bacterial skin infection and septic shock should be monitored to decrease mortality.
Multiple treatments are given, including systemic corticosteroid, intravenous immunoglobulin (IVIG), cyclosporine, anti-tumor necrosis factor (TNF), and cyclophosphamide. There was no standard consensus in terms of the type of immunosuppressive agents (systemic corticosteroids, IVIG, and cyclosporine), efficacy, and dosage of these medications (Huang et al., 2012). Glucocorticoid could probably be considered in some cases. Regardless of the risk of infection or sepsis, a systematic review and meta-analysis revealed that glucocorticoid and cyclosporine seem to be the most promising systemic immuno-modulation therapy that decreases the length of stay in the hospital and increases re-epithelization (Zhang et al., 2020). IVIG lowers the mortality rate caused by SJS/TEN in children (Zhang et al., 2020) compared to adults (Chiou et al., 2008). TNF-inhibitors have also been discovered to be a safe and effective treatment for SJS/TEN. However, the evidence is limited (Teyton et al., 1990a).
Systemic corticosteroid therapy is advised in the early stages of the DRESS diagnosis. Prednisolone (1 mg/kg/day) or an equivalent is frequently given to the patients (Funck-Brentano et al., 2015). Because abrupt discontinuation commonly induces DRESS relapse and prolongs the course of this illness, tapering oral prednisolone should be done gradually for 6–8 weeks to 3 months (Huang et al., 2012) (mean length therapy 56.2 days, range 2–185 days) (Hadavand et al., 2020). Fluid replenishment, an antipyretic drug for fever, correction of electrolyte imbalance, skincare with a proper dressing, and prevention of secondary bacterial infection are the mainstays of treatment. Topical corticosteroids, systemic anti-histamines, and emollients for rashes have been shown to reduce relapse and hospitalization in mild cases of DRESS (Schwartz et al., 2013). The vast majority of AGEP cases just required supportive care. For inflammatory or pruritic lesions, topical corticosteroids might be administered to shorten the clinical course. However, because several organs are affected in severe cases, step-wise treatment with systemic corticosteroids (intravenous/oral) or cyclosporine, as well as adjuvant therapy such as infliximab and IVIG, is employed (Olteanu et al., 2018).
Prognosis
The prognosis of SJS/TEN patients can be determined using a score of toxic epidermal necrolysis (SCORTEN), which consists of seven independent factors: age, body surface area of skin detachment, underlying malignancy, tachycardia, blood urea nitrogen, serum glucose, and serum bicarbonate. SCORTEN assessments should be done on days 1 and 3 after admission. To assess pediatric SJS/TEN and estimate a mortality rate, pediatric SCORTEN was proposed (Alvestad et al., 2007) (Sassolas et al., 2010c). A study shows that the mortality rates of SJS, SJS/TEN overlap, and TEN were 1%–5%, 5%–25%, and 25%–30%, respectively (Sassolas et al., 2010c).
The most common chronic sequelae were dermatologic and ocular involvements, which were recorded in 88% of SJS patients (Halevy et al., 2008). Atrophic or hypertrophic scars, dyspigmentation, milia, eruptive melanocytic nevi, hyperhidrosis, and xerosis are some of the other dermatological complications. Hair, nail involvement, and mucosal adhesion had also been represented. Chronic inflammation, sicca syndrome, entropion, sub-conjunctival fibrosis, trichiasis, symblepharon, corneal ulceration, permanent dry eye, photophobia, vision loss or impairment, and blindness are common ocular sequelae resulting from changes in the conjunctival epithelium (Kamada et al., 2006; Spriet and Banks, 2015c). The long-term psychosocial effects of SJS/TEN on survivors and their families should be investigated. As long-term sequelae, autoimmune illnesses such as autoimmune thyroiditis, systemic lupus erythematosus, and the Sjögren syndrome might be detected (Kardaun et al., 2013b). The DRESS syndrome is a medication reaction that can be fatal.
DRESS has a better prognosis and lower mortality in children than in adults (Correa-de-Castro et al., 2016b). The death rate in pediatric and adult patients was around 3% and 10%, respectively (Correa-de-Castro et al., 2016b). The mortality rate in the prospective RegiSCAR study was 1.7 percent (Manuyakorn et al., 2016a). However, extensive systemic involvement can considerably increase morbidity and mortality; fulminant hepatitis, coagulopathy, sepsis, and respiratory distress syndrome were the most prevalent causes of DRESS-induced death (Mockenhaupt, 2012b). According to Chen et al., high absolute eosinophil count (>600/UL), thrombocytopenia, pancytopenia, history of chronic renal insufficiency, multiple organ involvement, and underlying illnesses are all bad prognostic markers for this disorder (Fowler et al., 2019). Autoimmune sequelae including thyroid dysfunction, diabetes mellitus, and autoimmune hemolytic anemia should be monitored for several weeks to years after the onset of this condition (Tempark et al., 2022).
The prognosis for AGEP is usually favorable. According to the Euro SCAR study, AGEP has a mortality rate of around 4% (Teyton et al., 1990b). The atypical and severe presentation had a poor clinical prognosis and necessitated systemic treatment.
Pathogenesis
CADRs can be immediate (mediated by IgE or histamine) or delayed (mediated by histamine) (mediated by T cells). IV drug delivery has been linked to immediate responses such as urticarial rash and angioedema. Because these reactions occur in order with the causative drugs, the diagnosis is rarely suspected. Desensitization should be avoided in this situation due to the high risk-to-benefit ratio. In contrast, the delayed T-cell-mediated effects take anything from 12 h to a week after the first therapeutic dose to manifest. As a result, clinical assessment is critical. SJS/TEN is a serious multi-organ systemic disease with symptoms ranging from a mild skin rash to severe systemic multi-organ disease (Bodmer et al., 1994).
MHC, TCR, CD4+, CD8+, and SCARs
Every individual and tissue has its unique MHC. All nucleated cells have MHC-I, whereas APCs such as dendritic cells, macrophages, and neutrophils have MHC-II. CD4+ T cells are known as helper T cells, and these exclusively engage with MHC-II, whereas CD8+ T cells interact with MHC-I and are known as cytotoxic T cells. MHC I has a full-length alpha chain and a partial beta chain with a presenting site in the middle, whereas MHC-II has both full-length alpha and beta chains with a presenting site in the middle. For successful cell surface antigen presentation, a series of highly coordinated intracellular activities must occur first. The monomorphic protein invariant chain is essential for antigen presentation by MHC class II molecules. From the endoplasmic reticulum, this molecule delivers freshly synthesized class II heterodimers to the endosomal system. As soon as it encounters an acidic environment, this complex will be degraded and dissociate proteolytically, leaving the class II binding site accessible to antigenic peptides produced by external proteins. CLIP (class II-associated invariant chain peptides) requires the assistance of another MHC-encoded protein in order to physically escape the class II binding groove (Neefjes and Ploegh, 1992; Wucherpfennig et al., 2010; Mariuzza et al., 2020).
Cytotoxic T cells and helper T cells are the most influential cells in the immune system. Cytotoxic T cells contain CD8+ glycoprotein, releasing perforin that makes pore on the surface of the cell membrane, and granzyme kills the infected cells in a programmed way, called apoptosis. Upon engagement of the MHC-TCR complex, T cell clonal proliferation and differentiation of memory and effector T cells are formed. Among memory T cells, a set of cells are called effectors memory T cells (TEM) which are expressed with CD4RO and involved in the surveillance of pathogen re-exposure. Because the activation of these cells requires few co-stimulatory signals, they produce swift immune response upon the reentry of pathogen-specific antigen by producing pro-inflammatory cytokines such as TNF alpha, IL-2, and INF gamma and cytotoxic peptides. T cell response is initiated when the TCR and 1 homodimer z and CD3 (1–3) are collectively expressed as a unit on the surface of the T cell (Chung et al., 2008). For example, CDR1 and CDR2 contact with an alpha unit of MHC, the CDR3 contacts with peptides, showing the greatest sequences variability in the TCR gene. Therefore, the polymorphism of the genes that encode these proteins leads to increased expression of diverse MHC protein, and this may increase the diversity of peptides produced T cells, so diverse pathogens will be recognized and elicit an immune response (Su et al., 2017) (Figure 5).
Granulysin is produced and secreted by both CD8+ and natural killer cells (NK cells), which are the key mediators of apoptosis in SJS/TEN. Chung et al. revealed the high concentration of granulysin in TEN blister fluid. These mediators cause extensive epithelial keratinocyte apoptosis and necrosis (Stern and Divito, 2017). According to recent research, granulysin correlates with illness severity and can be used as a diagnostic test and therapeutic target. Serum IL-15 has been found as an SJS/TEN biomarker (Clayberger and Krensky, 2003; Castillo and Schluns, 2012). Cytotoxic lymphocytes (CTLs) and natural killer cells (NK cells) use IL-5 to differentiate and dominate effector function (Hashizume et al., 2016). The IL-5 signal is sent to the JAK-STAT pathway, which is then followed by the PI3K/AKT/mTOR pathways. In contrast, granulysin expression in CTLs and NK cells is dependent on IL-15 (Yuliwulandari et al., 2017) (Tapia et al., 2004). SJS/TEN patients had IL-17 expression cells in the peripheral blood and fluid blisters, as well as CCR6+ Th17 lymphocyte infiltration in the skin (Bocquet et al., 1996). Human keratinocytes may produce more pro-inflammatory cytokines when IL-17 and IFN- are coupled (Bocquet et al., 1996). CCL27 (CTACK), a keratinocyte-specific chemokine, attracts CCR 10 + lymphocytes to the epidermis. CCR 10 + lymphocytes can be found in high numbers in the peripheral blood during the acute phase of SJS/TEN (Husain et al., 2013).
According to current data, DRESS is caused by a combination of genetic predisposing factors, mainly HLA alleles, viral reactions (HHV-6, HHV-7, EBV, CMV) (Descamps et al., 2001; Ogawa et al., 2013; Cho et al., 2017), and immune system changes, including changes in the drug detoxifying enzyme pathway. These viral reactivations contribute to illnesses being more severe and lasting longer (Ogawa et al., 2014; Tsai et al., 2019). CD4+, CD8+, Tregs, and monomyeloid cells make up DRESS drug-specific T cells (plasmacytoid dendritic cells, monocyte). CCL17/TARC is a chemokine produced by dermal dendritic cells (DC), attracting CCR4+ Th2 lymphocytes to the skin (Yang et al., 2017). Th2 T cells and innate type 2 lymphocytes (ILC2) produce large quantities of IL-5, which are eosinophil-specific differentiation factors and contribute to the development of eosinophilia (Yang et al., 2017). CD4+ T cells that produce IL-4 and IL-13 are also involved. Th2 cell frequencies in DRESS patients are closely related to blood TARC levels, which are linked to HHV reactivation (Yang et al., 2017). ILC2 reflects a high serum concentration of soluble ST2 and IL-33 in the blood and skin of DRESS patients (Carr et al., 2016) via expressing the IL-33 receptor ST2 pathway. In the acute stage of DRESS, IL-33 has also been considered a possible biomarker of severity (Carr et al., 2016). DAMP, also known as an alarmin, is an endogenous agonist of pattern-recognition receptors (PRRs) produced by wounded tissue to activate a different immune system (Shear and Spielberg, 1988).
The DAMP/alarmin family includes HMGB1, a non-histone chromatin protein. Transcriptional regulation and extracellular stimulation of inflammation, including recruitment and activation of immunocompetent cells, are two of these dual functions. In the active phase of DRESS, HMGB1 was identified in blood and skin lesions, as well as in the serum and blister fluid of SJS/TEN (Schaerli et al., 2004). Changes in immunological response and metabolic enzymes in the liver, such as the cytochrome P450 (CYP450) enzyme, result in an accumulation of toxin metabolites, causing necrosis and apoptosis (Britschgi and Pichler, 2002).
IL-8/CXCL8 and granulocyte-macrophage colony-stimulating factor (GM-CSF) are produced by drug-specific CD4+ T cells for the AGEP (Kabashima et al., 2011). IFN- and TNF-producing T cell clones released IFN and TNF (Kabashima et al., 2011), and a small proportion secreted IL-4 and IL-5. In the skin, IL-8/CXCL8, a neutrophil chemotactic factor, may cause neutrophil and T-cell recruitment to the epidermis, resulting in the accumulation of numerous sterile pustules, as well as recruiting, activating neutrophil from the bone marrow to the peripheral blood, resulting in leukocyte exocytosis and neutrophilia (Romagnani et al., 2009). These recruited neutrophils may be protected from apoptosis by GM-CSF (Kabashima et al., 2011). The cytokines IL-17 and IL-22 are released during the Th17 immune response. Th17 cells have been observed in the skin of AGEP patients, as well as increased serum IL-22 and circulating Th17 cells in the serum (Hadavand et al., 2020). IFN and TNF, which drive surrounding keratinocytes to create IL-8, are connected to IL-17. Furthermore, Th17 cells are known for expressing the C-C motif chemokine receptor- (CCR-) 6. CCR6+ lymphocytes also invaded the afflicted skin (Meier-Schiesser et al., 2019a). A genetic mutation of AGEP pathogenesis involves the IL-36, IL-36 Ra axis. T cells and macrophages produce IL-36 in the skin, whereas monocytes produce IL-36 in the peripheral blood mononuclear cells (PBMCs). This alteration could increase express of various pro-inflammatory cytokines and chemokines such as IL-1, IL-6, and CXCL8/IL-8 production (Meier-Schiesser et al., 2019b; Hadavand et al., 2020).
HLA and SCARs
The “Hapten concept” or “Haptenization” was first introduced by Landsteiner and Jacobs (1936). As per this model, the hapten is a substance that has <1000 Dalton molecular weight capable of triggering the immune response when it binds with larger proteins or peptides. The electrophilic or reactive chemicals or drugs behave as a hapten and initiate the immune response. According to prohapten theory, the hapten can be generated from metabolites after metabolization. Therefore, the non-reactive drug acquires reactivity by virtue of its metabolites. For instance, sulfamethoxazole is prohapten which is converted into a proactive hydroxylamine metabolite by CYP2C9 at first, then into the unstable and readily protein binding nitroso sulfamethoxazole. This prohapten complex can initiate the immunological response as it is processed by an antigen-presenting cell (APC) and presented to T cell through MHC or HLA (Yun et al., 2016). However, the US FDA label for sulfamethoxazole states that patients with a slow acetylator status are at increased risk of hypersensitivity reactions. The reason could be that acetylation is the major metabolic pathway for sulfamethoxazole, as the acetylation is saturated. Then reactive metabolites are generated by CYP450 2C9 (Ikediobi and Schneider, 2021).
In p-i interactions theory, the drug may bind to TCR itself with high affinity at sites that make contact with pMHC resulting in T-cell activation, even in the presence of different peptides or allogeneic HLA (Zanni et al., 1998; Burkhart et al., 2002; Watkins and Pichler, 2013). For example, lidocaine activates T cells almost instantly in the presence of APCs, as revealed by a rapid and sustained intracellular Ca2+ rise (within 1 min). Thus, the immune response can occur before metabolism (Pichler, 2008).
Repertoire alteration theory proposes that drugs may bind with MHC binding site by non-covalent bond and alter the chemistry of binding cleft and endogenous peptide repertoire. For example, a study by Illing et al. demonstrated that isolated peptides eluted from carbamazepine-treated APCs expressing HLA-B*15:02 were distinct from those obtained in untreated cells. These findings support the altered peptide repertoire model as a feasible explanation for carbamazepine-induced SJS/TEN (Illing et al., 2012). The findings of Norcross et al. (2012) show that abacavir binds non-covalently to HLA-B*57:01 molecule, causing changes in the peptides binding ability of HLA-B*57:01 molecule causing an alteration in the repertoire of endogenous peptides presented to TCR (Figure 6).
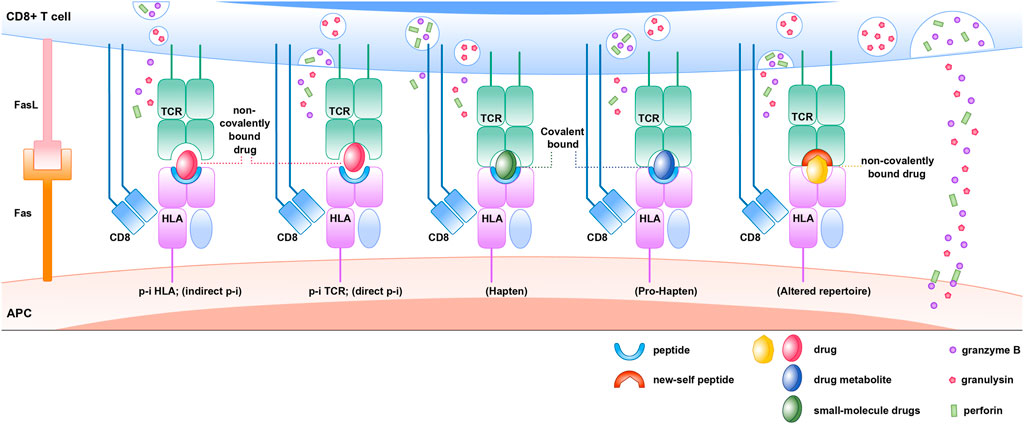
FIGURE 6. Immune mechanisms of HLA, drug, peptide, and TCR associated drug hypersensitivity reactions.
However, some concerns about these T cell-mediated SCARs remain unsolved. Those are like why the differences in clinical features and the clinical onset of each SCARs have been observed, the reason for drug-specific re-call reactions years after drug exposure and withdrawal, and the cause of high negative and low positive predictive values. Hence, understanding T cell plasticity and heterologous immunity are critical. The T cell repertoire interacts with several types of antigen to initiate the robust immunological response. Because the human immune system has evolved to have a larger degree of antigenic diversity, this poly-specific TCR identifies several peptides.
Heterologous immunity refers to the ability of a single TCR to recognize various pathogens and protect against the entire microbial universe. Tissue rejection occurs in organ transplantation when the cross-reactive TCR identifies the antigen presented in non-MHC molecules (Gamadia et al., 2004). For example, TCR LC13 can recognize both the HLA-B* 08:01 (EBV epitope) and the endogenous peptide presented in HLA-B* 44:02 and HLA-B* 44:05. The cross-reactivity in the molecular mimicry paradigm is owing to the contact residue’s structure and chemical characteristics being preserved. The structural flexibility of the TCR itself is another mechanism of T cell cross-reactivity. According to this theory, T cells can modify the peptide-MHC interaction by shifting the orientation of CDRs (1–3) via an induced-fit mechanism (immune surveillance by CD8αα+ skin-resident T cells in human herpesvirus infection (Zhu et al., 2013).
Heterologous immunity can also be seen in T cell-mediated hypersensitivity events. According to this paradigm, pathogen-specific effector memory T cells recognize the neo-antigen generated by drug exposure in cross-reactive T cell responses. As a result, viral reactivation or the presence of the pathogen at the initiation of ADR is not required. There is no pathogen reactivation after the initial ADR because the peptide and drug complex activate the T-cell response. This has been verified that HHV replication is not found by viral DNA PCR at an earlier stage of DRESS but is observed after 2 weeks. This reactivation could be because of immune regulation in general. This explains why, while viral reactivation is not the cause of DRESS, it can exacerbate several symptoms of the disease, such as hepatitis and rash, in the absence of a drug (Zhu et al., 2013) (Figure 7).
Cutaneous Adverse Drug Reaction in Special Populations
HIV
CADRs are a prevalent HIV-related complication that may affect the patients’ care plan. There are several reasons behind this. For example, the ART may be the instigators. Aside from that, different factors could be implicated in the onset of CADRs, such as polypharmacy, slow acetylator status, relative glutathione status, CD+4 T-cell counts, latent cytomegalovirus and Epstein–Barr virus infections, and high CD8+ T cells count of >460 cells/mm3 (Hoosen et al., 2019). The occurrence of cutaneous adverse drug reactions to trimethoprim/sulfamethoxazole (TMP/SMX) is thought to be due to a glutathione deficiency (van der Ven et al., 1991). CADRs are common in HIV patients because opportunistic infections, as well as the use of ART, anti-TB, and NSAIDs, are common. According to a retrospective study conducted in a dermatology ward, 21.8% of CADR cases are anti-TB related, with 92 percent infected with HIV.
Abacavir is a nucleoside nucleotide reverse transcriptase inhibitor (NRTI) available as monotherapy or in fixed-dose combination with lamivudine and lamivudine/zidovudine. Hypersensitivity reaction is the most common skin reaction associated with abacavir. Tipranavir (protease inhibitor), darunavir (protease inhibitor), etravirine (NNRTI)/raltegravir (integrase inhibitor), and maraviroc (chemokine receptor antagonist) are among the ARTs that can produce CADRs (Borras-Blasco et al., 2008). An abacavir hypersensitivity reaction is significantly connected to the presence of the HLA-B*57:01 variant. Likewise, HLA-B* 13:01 is found to be associated with co-trimoxazole-induced CADRs in Thai, Malays, and Taiwanese populations (Kiertiburanakul et al., 2015). As a result, early detection of the causative drug and its correlation with susceptible genetic and non-genetic predictors can aid in the prevention of reactions from progressing.
Autoimmune/Immune Disorders/Systemic Lupus Erythematous
Drug-induced cutaneous reactions are quite common among patients with autoimmune disorders or patients with immune dysregulations. A retrospective study found that drug allergies are more common among patients with SLE than controls (p < 0.01) (Becker, 1973). Another study investigating the imputable drugs of CADRs found 48 patients who suffered from human immunodeficiency virus (HIV) infection (19%), connective tissue disease (10%), and viral or autoimmune hepatitis (12%) (Fiszenson-Albala et al., 2003).
A 10-month prospective cohort study found that immuno-suppressed patients were most frequently affected. Patients with systemic lupus erythematosus (SLE) had a 4.68 greater chance of having a CADRs (CI 95% = 1.794–12.186, p = 0.001). Patients with AIDS had a risk of 8.68% (CI 95% = 2.18–33.19, p = 0.001). Patients with non-Hodgkin lymphoma had a higher probability of having CADRs (Hernández-Salazar et al., 2006). A tertiary center in India evaluated and their correlation with autoimmune disorders and found a strong relationship between CADRs and autoimmune diseases (p-value = 0.004) (Rana et al., 2021). Immune dysregulations present in autoimmune disorders are the major reason for this kind of delayed-type T-cell-mediated hypersensitivity reaction. On the contrary, some studies suggest that autoimmune disorders are the long-term complications of CADRs (Kano* and Shiohara, 2013).
Tumor Lysis Syndrome
TLS is a life-threatening hemato-oncological emergency (Howard et al., 2011) Hyperuricemia, hyperkalemia, hyperphosphatemia, hypocalcaemia, and metabolic acidosis can all result from the fast disintegration of cancer cells. Systemic tumor lysis syndrome and spontaneous (idiopathic) cutaneous tumor lysis syndrome are the two most common types (Cohen et al., 2021). This cutaneous TLS might be idiopathic (unrelated to therapy) or therapy-related. The clinical signs of cutaneous tumor lysis syndrome are ulcers or necrosis inside the tumor mass.
Rapid tumor cell death is the main diagnostic criteria of TLS. According to an earlier study, bevacizumab, as part of a combined anti-neoplastic treatment, was connected to cutaneous tumor lysis syndrome in people with metastatic breast cancer (Cottu et al., 2011; Ono et al., 2013; Sharma and Marcus, 2013; Kijima et al., 2015). By blocking the vascular endothelial growth factor, bevacizumab damages the tumor vascular system that leads to the breakdown of metastatic tumor cells in the dermis.
Cutaneous TLS due to anti-neoplastic therapies has been reported in T lymphoma patients, especially for bexarotene, vorinostat, and fenofibrate, or retinoids. Methotrexate has also been associated with cutaneous TLS (Hsu et al., 2006; Steinhoff et al., 2008; Pfistershammer et al., 2010; Sánchez et al., 2019).
CADRs in Pediatric Patients
Differences in the drug metabolism and lack of substantial evidence on drug efficacy and toxic effects could be the reason for the occurrence of CADRs in the pediatric population. Most of the time, drug-induced cutaneous adverse reactions are mistakenly diagnosed as viral exanthema. A retrospective study conducted in India reported 33 CADRs among children. Most of the reactions were MPE, but one death was reported in AED-induced TEN cases. 14% of these reactions were due to antimicrobial, 5% were due to AEDs. SJS was reported mainly with AEDs and antimicrobial (Satyanarayana et al., 2020). Another study from Turkey, whose research population was with 1.99 years of mean age group, found that the most common form of CADRs was urticarial angioedema, which was produced mostly by antibiotics, analgesics, anti-inflammatory, and antipyretic medicines (AAA) (Dilek1 et al., 2014).
Genetic Markers Associated with Cutaneous Adverse Drug Reactions to the Clinical PGx Implementation in Thailand
Following the accomplishment of the human genome project in 2001, the fields of pharmacogenomics and personalized medicine have exploded globally. In 2004, the Thailand Centre of Excellence for Life Sciences began working on several initiatives. In Thailand, pharmacogenetic biomarkers linked to the top 10 ADRs-involved medicines, such as AEDs, antiretroviral therapy (ART), and allopurinol, have been widely reported. In the Thai population, HLA-B* 15:02 and HLA-B* 58:01 were linked to CBZ-induced SJS/TEN and allopurinol-induced SCARs, respectively (Tassaneeyakul et al., 2009b; Tassaneeyakul et al., 2010b). Patients found to be negative for HLA-B* 15:02 but still developed SCARs were associated with HLA-B* 15:13 and HLA-B* 15:21 in the same serotype 75 (Jaruthamsophon et al., 2017; Sukasem et al., 2021). Other than these drugs, other AEDs such as lamotrigine (HLA-A* 02:07, HLA-A*33: 03, HLA-B*15:02, HLA-B*44:03) (Napatrupron et al., 2017), phenytoin (HLA-B*15:02, HLA-B*15:13, HLA-B*56:02/04) (Kano et al., 2010), oxcarbamazepine (HLA-B* 15:02) (Chen et al., 2017), and phenobarbital (HLA-B* 13:01 and HLA-B* 51:01) (Manuyakorn et al., 2016b) were studied well for their association with HLA alleles and cutaneous adverse reactions. Other than HLA alleles, CYP2C9, CYP2C19, and omeprazole were other predictors of PHT-induced CADRs. Likewise, CYP2C19 was associated with phenobarbital-induced CADRs (Manuyakorn et al., 2016b).
Apart from AEDs, cotrimoxazole- (CTX-) induced DRESS has been connected to HLA-B* 13:01 in the Thai population, while CTX-induced SJS/TEN has been linked to HLA-B* 15:02 in the same group (Sukasem et al., 2020b). HLA-B*13:01 has been linked to CADRs caused by dapsone, indicating phenotypic non-specific reactivity (Tangamornsuksan and Lohitnavy, 2018). In the Thai population, HLA-DQB1*03:01 has been associated with DPP4 inhibitor-induced bullous pemphigoid (Chanprapaph et al., 2021).
The risks can be minimized by genotyping the susceptible HLA alleles in the vulnerable population. HLA-A* 31:01, HLA-B* 15:02, HLA-B* 57:01, and HLA-B* 58:01 alleles were evaluated in Thailand even before administering such medications to avoid SCARs. In Thailand, the cost-effectiveness of pharmacogenetic testing was evaluated for two distinct alleles: HLA-B* 15:02 and HLA-B* 58:01. Thailand, China, and Malaysia are among the middle-income countries that reimburse the expense of genetic testing. Only Singapore and Thailand have all of the necessary elements for PGx implementation ready and available.
Severe Cutaneous Adverse Drug Reactions in Thai Population: Lessons Learned from ThaiSCAR
ThaiSCAR is a multicenter registry of patients with severe cutaneous adverse reactions (SCARs) from drugs among tertiary medical institutes/hospitals in Thailand. ThaiSCAR is aims to study etiologies, clinical characteristics, therapeutic outcomes, quality of life, and the values of laboratory techniques for culprit drugs identification in Thai patients suffering from SCARs. The network was established in October 2013 with eight participating medical schools and hospitals from all country regions. The registry includes patients aged more than 18 years who suffered from SCARs, SJS/TEN, DRESS, AGEP, and GBFDE. The diagnosis is made based on the RegiSCAR diagnostic criteria (Bastuji-Garin et al., 1993; Sidoroff et al., 2001; Kardaun et al., 2007; Lipowicz et al., 2013). Only cases considered probable or definite are included. Importantly, all cases are assessed by dermatologists/allergists at their point of care.
A multidisciplinary team including immunologists, pharmacogeneticists, allergists, and ophthalmologists, in addition to dermatologists, were incorporated into the data gathering of the registry. The correct culprit drug identification is of paramount importance in the management of SCARs. The methods used to identify the causative drugs in the registry are lymphocyte transformation test (LTT) and enzyme-linked immunospot assay (ELISpot) to detect drug-specific IFN-γ-releasing cells from the patients’ peripheral blood mononuclear cells (PBMC). We demonstrated that IFN-γ ELISpot assay yielded positive results in 73.9% of SCARs patients while that of LTT was 52.2%. Interestingly, in this study, the ELISpot assay was undertaken within 2 weeks after the incidence of SCARs, while LTT was performed between 3 and 6 months after the skin eruptions had improved. Therefore, during the acute phase of the disease, ELISpot assay may be a beneficial tool (Suthumchai et al., 2018b). Our further study has confirmed the utility of IFN-γ ELISpot in SCARs. The culprit drugs can be identified in 45.7% (53 of 116) of the patients by the technique. The positive rate was highest in DRESS (50.0%), followed by SJS/TEN (46.0%) and AGEP (31.3%), respectively (Klaewsongkram et al., 2019).
Ocular involvement in SJS/TEN is one of the most devastating manifestations of the disease. Clinical features and serum biomarkers to predict acute severe ocular complications (SOCs) would be crucial to aid in the management of clinicians. From our ThaiSCAR recent study, body surface area detachment of ≥10% was a significant predictive factor for acute SOCs. In addition, serum levels of inflammatory mediators, especially S100A8/A9 and granulysin, showed a significantly higher trend in the severe group compared to those of non-severe individuals (Panpruk et al., 2021).
A study conducted using data obtained from the ThaiSCAR registry shows a slight female preponderance (female: male, n = 174:147, 54.2:45.8%). Of 155 patients with SJS/TEN, anticonvulsants, allopurinol, beta-lactams, sulfonamides/sulfones, and anti-tuberculosis drugs were the leading culprits. One hundred and fifteen (115) patients were seen with DRESS, and anticonvulsants, allopurinol, sulfonamides/sulfones, anti-tuberculosis drugs, and antiretroviral drugs were the most common causative agents. AGEP was observed in 46 patients, with beta-lactam and non-beta-lactam antibiotics being the most common. Lastly, GBFDE was seen in five patients from beta-lactams, sulfonamides/sulfones, non-beta-lactam antibiotics, and analgesic drugs (Sukasem et al., 2020c). The study also found that skin lightening cream was the reason for one case of overlap SJS-TEN. The oral supplements available for lighter skin in Thailand usually contain glutathione, tomato extract, and vitamin C (Chottawornsak et al., 2021).
Future Perspective
Consensual diagnostic criteria are also necessary for severe cutaneous adverse effects other than SJS/TEN and DRESS. Likewise, regular updates on diagnosis and the relevance of clinical risk factors are required for the accepted diagnostic criteria for SJS/TEN and DRESS. For example, the established diagnostic criteria contained unambiguous clinical manifestations for skin and mucous membrane involvement, showing the involvement of internal organs, particularly liver involvement, is still difficult. The pattern of liver injury must be thoroughly investigated and updated in the criteria that can distinguish SCARs with (drug-induced liver injury) DILI from DILI without SCARs (Sanabria-Cabrera et al., 2019). Similarly, aside from the causality assessment tools, there are no specific or collective tools available to evaluate SCARs in relation to liver injury. Because of this, less effective treatment for SCARs with systemic involvement may be chosen (Villanueva-Paz et al., 2021).
Although many pharmacogenetic biomarkers are being studied in several populations, how these data can be used at the maximum level to predict and prevent SCARs in the real clinical world is the issue for future research to explore. Likewise, more research is needed to investigate various barriers to PGx implementation such as bringing awareness about PGx and reeducating the PGx to sectors of the health care system. Attempts to incorporate PGx into government healthcare plans and insurance schemes could be very useful to the literature. Similarly, the pharmacoeconomic aspects of PGx, such as the cost and clinical advantages of single genetic testing and the range of panel testing, must be thoroughly investigated. This evidence will aid patients in obtaining funding for genetic testing through insurance and government healthcare programs.
Author Contributions
CS and TT contributed to the conception and designed and reviewed the manuscript. TT, CS, SJ, PR, and PS contributed to the writing of the manuscript. PR and PS drew the figures. All authors contributed to the article and approved the submitted version.
Conflict of Interest
The authors declare that the research was conducted in the absence of any commercial or financial relationships that could be construed as a potential conflict of interest.
Publisher’s Note
All claims expressed in this article are solely those of the authors and do not necessarily represent those of their affiliated organizations or those of the publisher, the editors, and the reviewers. Any product that may be evaluated in this article, or claim that may be made by its manufacturer, is not guaranteed or endorsed by the publisher.
References
Al-Quteimat, O. M. (2016). Phenytoin-induced Toxic Epidermal Necrolysis: Review and Recommendations. J. Pharmacol. Pharmacother. 7 (3), 127–132. doi:10.4103/0976-500X.189662
Alvestad, S., Lydersen, S., and Brodtkorb, E. (2007). Rash from Antiepileptic Drugs: Influence by Gender, Age, and Learning Disability. Epilepsia 48 (July 7), 1360–1365. doi:10.1111/j.1528-1167.2007.01109.x
Anderson, M., Egunsola, O., Cherrill, J., Millward, C., Fakis, A., and Choonara, I. (2015). A Prospective Study of Adverse Drug Reactions to Antiepileptic Drugs in Children. BMJ Open 5 (6), e008298. doi:10.1136/bmjopen-2015-008298
Asakura, E., Mori, K., Komori, Y., Takano, M., Hasegawa, T., Kobayashi, W., et al. (2001). The Trend of the Drug Eruptions in the Last Fifteen Years. Yakugaku Zasshi 121, 145–151. doi:10.1248/yakushi.121.145
Barbaud, A. (2014). Skin Testing and Patch Testing in Non-IgE-mediated Drug Allergy. Curr. Allergy Asthma Rep. 14, 442. doi:10.1007/s11882-014-0442-8
Bastuji-Garin, S., Rzany, B., Stern, R. S., Shear, N. H., Naldi, L., and Roujeau, J. C. (1993). Clinical Classification of Cases of Toxic Epidermal Necrolysis, Stevens-Johnson Syndrome, and Erythema Multiforme. Arch. Dermatol. 129 (1), 92–96. doi:10.1001/archderm.129.1.92
Becker, L. C. (1973). Allergy in Systemic Lupus Erythematosus. Johns Hopkins Med. J. 133 (1), 38–44.
Bigby, M. (2001). Rates of Cutaneous Reactions to Drugs. Arch. Dermatol. 137, 765–770. doi:10.1001/archderm.137.12.1639
Bocquet, H., Bagot, M., and Roujeau, J. C. (1996). Drug-induced Pseudolymphoma and Drug Hypersensitivity Syndrome (Drug Rash with Eosinophilia and Systemic Symptoms: DRESS). Semin. Cutan. Med. Surg. 15, 250–257. doi:10.1016/s1085-5629(96)80038-1
Bodmer, H., Viville, S., Benoist, C., and Mathis, D. (1994). Diversity of Endogenous Epitopes Bound to MHC Class II Molecules Limited by Invariant Chain. Science 263 (5151), 1284–1286. doi:10.1126/science.7510069
Borras-Blasco, J., Navarro-Ruiz, A., Borras, C., and Castera, E. (2008). Adverse Cutaneous Reactions Associated with the Newest Antiretroviral Drugs in Patients with Human Immunodeficiency Virus Infection. J. Antimicrob. Chemother. 62, 879–888. doi:10.1093/jac/dkn292
Britschgi, M., and Pichler, W. J. (2002). Acute Generalized Exanthematous Pustulosis, a Clue to Neutrophil-Mediated Inflammatory Processes Orchestrated by T Cells. Curr. Opin. Allergy Clin. Immunol. 2 (4), 325–331. doi:10.1097/00130832-200208000-00006
Brodie, M. J., Overstall, P. W., and Giorgi, L. (1999). Multicentre, Double-Blind, Randomised Comparison between Lamotrigine and Carbamazepine in Elderly Patients with Newly Diagnosed Epilepsy. The UK Lamotrigine Elderly Study Group. Epilepsy Res. 37 (1), 81–87. doi:10.1016/s0920-1211(99)00039-x
Burkhart, C., Britschgi, M., Strasser, I., Depta, J. P., von Greyerz, S., Barnaba, V., et al. (2002). Non-covalent Presentation of Sulfamethoxazole to Human CD4+ T Cells Is Independent of Distinct Human Leucocyte Antigen-Bound Peptides. Clin. Exp. Allergy 32, 1635–1643. doi:10.1046/j.1365-2222.2002.01513.x
Cacoub, P., Musette, P., Descamps, V., Meyer, O., Speirs, C., Finzi, L., et al. (2011). The DRESS Syndrome: a Literature Review. Am. J. Med. 124, 588–597. doi:10.1016/j.amjmed.2011.01.017
Campos-Fernández, M., Ponce-De-León-Rosales, S., Archer-Dubon, C., and Orozco-Topete, R. (2005). Incidence and Risk Factors for Cutaneous Adverse Drug Reactions in an Intensive Care Unit. Rev. Invest. Clin. 57 (6), 770–774.
Carr, D. F., Chaponda, M., Jorgensen, A. L., Castro, E. C., van Oosterhout, J. J., Khoo, S. H., et al. (2013). Association of Human Leukocyte Antigen Alleles and Nevirapine Hypersensitivity in a Malawian HIV-Infected Population. Clin. Infect. Dis. 56, 1330–1339. doi:10.1093/cid/cit021
Carr, D. F., Chung, W-H., Jenkiins, R. E., Chaponda, M., Nwikue, G., Cornejo Castro, E. M., et al. (2016). 7th Drug Hypersensitivity Meeting: Part One. ClinTransl Allergy 6 (S3), 31.
Castillo, E. F., and Schluns, K. S. (2012). Regulating the Immune System via IL-15 Transpresentation. Cytokine 59 (3), 479–490. doi:10.1016/j.cyto.2012.06.017
Chang, C. C., Too, C. L., Murad, S., and Hussein, S. H. (2011). Association of HLA-B*1502 Allele with Carbamazepine-Induced Toxic Epidermal Necrolysis and Stevens-Johnson Syndrome in the Multi-Ethnic Malaysian Population. Int. J. Dermatol. 50 (2), 221–224. doi:10.1111/j.1365-4632.2010.04745.x
Chanprapaph, K., Pratumchart, N., Limtong, P., Rutnin, S., Sukasem, C., Kungvalpivat, P., et al. (2021). Dipeptidyl Peptidase-4 Inhibitor-Related Bullous Pemphigoid: A Comparative Study of 100 Patients with Bullous Pemphigoid and Diabetes Mellitus. J. Dermatol. 48 (4), 486–496. doi:10.1111/1346-8138.15778
Chen, C. B., Hsiao, Y. H., Wu, T., Hsih, M. S., Tassaneeyakul, W., Jorns, T. P., et al. (2017). Risk and Association of HLA with Oxcarbazepine-Induced Cutaneous Adverse Reactions in Asians. Neurology 88 (1), 78–86. doi:10.1212/WNL.0000000000003453
Chiou, C. C., Yang, L. C., Hung, S. I., Chang, Y. C., Kuo, T. T., Ho, H. C., et al. (2008). Clinicopathological Features and Prognosis of Drug Rash with Eosinophilia and Systemic Symptoms: a Study of 30 Cases in Taiwan. J. Eur. Acad. Dermatol. Venereol. 22, 1044–1049. doi:10.1111/j.1468-3083.2008.02585.x
Cho, Y.-T., Yang, C.-W., and Chu, C.-Y. (2017). Drug Reaction with Eosinophilia and Systemic Symptoms (DRESS): An Interplay Among Drugs, Viruses, and Immune System. Ijms 18 (6), 1243. doi:10.3390/ijms18061243
Choon, S. E., and Lai, N. M. (2012). An Epidemiological and Clinical Analysis of Cutaneous Adverse Drug Reactions Seen in a Tertiary Hospital in Johor, Malaysia. Indian J. Dermatol. Venereol. Leprol. 78 (6), 734–739. doi:10.4103/0378-6323.102367
Chottawornsak, N., Tansrisawad, N., Tubtimrattana, A., Thantiworasit, P., Asawanonda, P., Klaewsongkram, J., et al. (2021). Glutathione Whitening Pills Induced Toxic Epidermal Necrolysis: An Unusual Case Confirmed by Enzyme-Linked Immunospot Assay and Liquid Chromatography-Mass Spectrometry. Dermatitis 32 (6), e115–e117. doi:10.1097/DER.0000000000000782
Chung, W. H., Hung, S. I., Hong, H. S., Hsih, M. S., Yang, L. C., Ho, H. C., et al. (2004). Medical Genetics: a Marker for Stevens-Johnson Syndrome. Nature 428 (6982), 486. doi:10.1038/428486a
Chung, W. H., Hung, S. I., Yang, J. Y., Su, S. C., Huang, S. P., Wei, C. Y., et al. (2008). Granulysin Is a Key Mediator for Disseminated Keratinocyte Death in Stevens-Johnson Syndrome and Toxic Epidermal Necrolysis. Nat. Med. 14, 1343–1350. doi:10.1038/nm.1884
Clayberger, C., and Krensky, A. M. (2003). Granulysin. Curr. Opin. Immunol. 15 (5), 560–565. doi:10.1016/s0952-7915(03)00097-9
Cloyd, J. (1991). Pharmacokinetic Pitfalls of Present Antiepileptic Medications. Epilepsia 32 (Suppl. 5), S53–S65.
Cohen, P. R., Prieto, V. G., and Kurzrock, R. (2021). Tumor Lysis Syndrome: Introduction of a Cutaneous Variant and a New Classification System. Cureus 13 (3), e13816. doi:10.7759/cureus.13816
Correa-de-Castro, B., Paniago, A. M., Takita, L. C., Murback, N. D., and Hans-Filho, G. (2016). Drug Reaction with Eosinophilia and Systemic Symptoms: a Clinicopathological Study of Six Cases at a Teaching Hospital in Midwestern Brazil. Int. J. Dermatol. 55, 328–334. doi:10.1111/ijd.12899
Correa-de-Castro, B., Paniago, A. M., Takita, L. C., Murback, N. D., and Hans-Filho, G. (2016). Drug Reaction with Eosinophilia and Systemic Symptoms: a Clinicopathological Study of Six Cases at a Teaching Hospital in Midwestern Brazil. Int. J. Dermatol. 55, 328–334. doi:10.1111/ijd.12899
Cottu, P. H., Fourchotte, V., Vincent-Salomon, A., Kriegel, I., and Fromantin, I. (2011). Necrosis in Breast Cancer Patients with Skin Metastases Receiving Bevacizumab-Based Therapy. J. Wound Care 20, 403–passim. doi:10.12968/jowc.2011.20.9.403
Craig, K. S., Edward, W. C., and Anthony, A. G. (2001). Cutaneous Drug Reactions. Pharmacol. Rev. 53, 357–379.
Cristallo, A. F., Schroeder, J., Citterio, A., Santori, G., Ferrioli, G. M., Rossi, U., et al. (2011). A Study of HLA Class I and Class II 4-digit Allele Level in Stevens-Johnson Syndrome and Toxic Epidermal Necrolysis. Int. J. Immunogenet. 38, 303–309. doi:10.1111/j.1744-313X.2011.01011.x
Da Silva, J. A. (1999). Sex Hormones and Glucocorticoids: Interactions with the Immune System. Ann. N. Y Acad. Sci. 876, 102–108. doi:10.1111/j.1749-6632.1999.tb07628.x
Descamps, V., Valance, A., Edlinger, C., Fillet, A. M., Grossin, M., Lebrun-Vignes, B., et al. (2001). Association of Human Herpesvirus 6 Infection with Drug Reaction with Eosinophilia and Systemic Symptoms. Arch. Dermatol. 137, 301–304.
Dilek1, N., Özkol2, H. U., Akbaş3, A., Kılınç3, F., Dilek4, A. R., Saral1, Y., et al. (2014). Ömer Çalka2 Cutaneous Drug Reactions in Children: A Multicentric Study Postępy Dermatologii I Alergologii 6.
Dreifuss, F. E., Santilli, N., Langer, D. H., Sweeney, K. P., Moline, K. A., and Menander, K. B. (1987). Valproic Acid Hepatic Fatalities: a Retrospective Review. Neurology 37 (3), 379–385. doi:10.1212/wnl.37.3.379
Dreifuss, F. E., Santilli, N., Langer, D. H., Sweeney, K. P., Moline, K. A., and Menander, K. B. (1987). Valproic Acid Hepatic Fatalities: a Retrospective Review. Neurology 37 (3), 379–385. doi:10.1212/wnl.37.3.379
Elzagallaai, A. A., Knowles, S. R., Rieder, M. J., Bend, J. R., Shear, N. H., and Koren, G. (2009). Patch Testing for the Diagnosis of Anticonvulsant Hypersensitivity Syndrome: a Systematic Review. Drug Saf. 32 (5), 391–408. doi:10.2165/00002018-200932050-00003
Fernando, S. L.. Drug-reaction Eosinophilia and Systemic Symptoms and Drug-Induced Hypersensitivity Syndrome. Australas. J. Dermatol. 55, 15–23.
Ferrandiz-Pulido, C., and Garcia-Patos, V. (2013). A Review of Causes of Stevens-Johnson Syndrome and Toxic Epidermal Necrolysis in Children. Arch. Dis. Child. 98, 998–1003. doi:10.1136/archdischild-2013-303718
Fiszenson-Albala, F., Auzerie, V., Mahe, E., Farinotti, R., Durand-Stocco, C., Crickx, B., et al. (2003). A 6-month Prospective Survey of Cutaneous Drug Reactions in a Hospital Setting. Br. J. Dermatol. 149 (5), 1018–1022. doi:10.1111/j.1365-2133.2003.05584.x
Fowler, T., Bansal, A. S., and Lozsádi, D. (2019). Risks and Management of Antiepileptic Drug Induced Skin Reactions in the Adult Out-Patient Setting. Seizure 72, 61–70. doi:10.1016/j.seizure.2019.07.003
Funck-Brentano, E., Duong, T. A., Bouvresse, S., Bagot, M., Wolkenstein, P., Roujeau, J. C., et al. (2015). Therapeutic Management of DRESS: a Retrospective Study of 38 Cases. J. Am. Acad. Dermatol. 72, 246–252. doi:10.1016/j.jaad.2014.10.032
Galindo, P. A., Borja, J., Gómez, E., Mur, P., Gudín, M., García, R., et al. (2002). Anticonvulsant Drug Hypersensitivity. J. Investig. Allergol. Clin. Immunol. 12 (4), 299–304.
Gamadia, L. E., Remmerswaal, E. B., Surachno, S., Lardy, N. M., Wertheim-van Dillen, P. M., van Lier, R. A., et al. (2004). Cross-reactivity of Cytomegalovirus-specific CD8+ T Cells to Allo-Major Histocompatibility Complex Class I Molecules. Transplantation 77, 1879–1885. doi:10.1097/01.tp.0000131158.81346.64
Genin, E., Chen, D. P., Hung, S. I., Sekula, P., Schumacher, M., Chang, P. Y., et al. (2014). HLA-A*31:01 and Different Types of Carbamazepine-Induced Severe Cutaneous Adverse Reactions: an International Study and Meta-Analysis. Pharmacogenomics J. 14, 281–288. doi:10.1038/tpj.2013.40
Gonçalo, M., Coutinho, I., Teixeira, V., Gameiro, A. R., Brites, M. M., Nunes, R., et al. (2013). HLA-B*58:01 Is a Risk Factor for Allopurinol-Induced DRESS and Stevens-Johnson Syndrome/toxic Epidermal Necrolysis in a Portuguese Population. Br. J. Dermatol. 169, 660–665. doi:10.1111/bjd.12389
Hadavand, M. A., Kaffenberger, B., Cartron, A. M., and Trinidad, J. C. (2020). Clinical Presentation and Management of Atypical and Recalcitrant Acute Generalized Exanthematouspustulosis(AGEP). J. Am. Acaddermatol2020 S0190-9622 (20), 32609–32618.
Halevy, S., Ghislain, P. D., Mockenhaupt, M., Fagot, J. P., Bouwes Bavinck, J. N., Sidoroff, A.., et al. (2008). Allopurinol Is the Most Common Cause of Stevens-Johnson Syndrome and Toxic Epidermal Necrolysis in Europe and Israel. J. Am. Acad. Dermatol. 58, 25–32. doi:10.1016/j.jaad.2007.08.036
Halevy, S. (2009). Acute Generalized Exanthematous Pustulosis. CurrOpin Allergy ClinImmunol. 9, 322–328. doi:10.1097/aci.0b013e32832cf64e
Hashizume, H., Fujiyama, T., and Tokura, Y. (2016). Reciprocal Contribution of Th17 and Regulatory T Cells in Severe Drug Allergy. J. Dermatol. Sci. 81 (2), 131–134. doi:10.1016/j.jdermsci.2015.11.002
Hassoun-Kheir, N., Bergman, R., and Weltfriend, S. (2016). The Use of Patch Tests in the Diagnosis of Delayed Hypersensitivity Drug Eruptions. Int. J. Dermatol. 55, 1219–1224. doi:10.1111/ijd.13306
He, X. J., Jian, L. Y., He, X. L., Wu, Y., Xu, Y. Y., Sun, X. J., et al. (2013). Association between the HLA-B*15:02 Allele and Carbamazepine-Induced Stevens-Johnson Syndrome/toxic Epidermal Necrolysis in Han Individuals of Northeastern China. Pharmacol. Rep. 65, 1256–1262. doi:10.1016/s1734-1140(13)71483-x
Hernández-Salazar, A., Rosales, S. P., Rangel-Frausto, S., Criollo, E., Archer-Dubon, C., and Orozco-Topete, R. (2006). Epidemiology of Adverse Cutaneous Drug Reactions. A Prospective Study in Hospitalized Patients. Arch. Med. Res. 37 (7), 899–902. doi:10.1016/j.arcmed.2006.03.010
Hoosen, K., Mosam, A., Dlova, N. C., and Grayson, W. (2019). An Update on Adverse Cutaneous Drug Reactions in HIV/AIDS. Dermatopathology 6, 111–125. doi:10.1159/000496389
Howard, S. C., Jones, D. P., and Pui, C. H. (2011). The Tumor Lysis Syndrome. N. Engl. J. Med. 364 (19), 1844–1854. doi:10.1056/NEJMra0904569
Hsiao, Y. H., Hui, R. C., Wu, T., Chang, W. C., Hsih, M. S., Yang, C. H., et al. (2014). Genotype-phenotype Association between HLA and Carbamazepine-Induced Hypersensitivity Reactions: Strength and Clinical Correlations. J. Dermatol. Sci. 73, 101–109. doi:10.1016/j.jdermsci.2013.10.003
Hsu, C. K., Hsu, M. M., and Lee, J. Y. (2006). Fusariosis Occurring in an Ulcerated Cutaneous CD8+ T Cell Lymphoma Tumor. Eur. J. Dermatol. 16, 297–301.
Huang, Y. C., Li, Y. C., and Chen, T. J. (2012). The Efficacy of Intravenous Immunoglobulin for the Treatment of Toxic Epidermal Necrolysis: a Systematic Review and Meta-Analysis. Br. J. Dermatol. 167, 424–432. doi:10.1111/j.1365-2133.2012.10965.x
Hughes, A. R., Brothers, C. H., Mosteller, M., Spreen, W. R., and Burns, D. K. (2009). Genetic Association Studies to Detect Adverse Drug Reactions: Abacavir Hypersensitivity as an Example. Pharmacogenomics 10 (2), 225–233. doi:10.2217/14622416.10.2.225
Hung, S. I., Chung, W. H., Jee, S. H., Chen, W. C., Chang, Y. T., Lee, W. R., et al. (2006). Genetic Susceptibility to Carbamazepine-Induced Cutaneous Adverse Drug Reactions. Pharmacogenet. Genomics 16, 297–306. doi:10.1097/01.fpc.0000199500.46842.4a
Hung, S. I., Chung, W. H., Liou, L. B., Chu, C. C., Lin, M., Huang, H. P., et al. (2005). HLA-B*5801 Allele as a Genetic Marker for Severe Cutaneous Adverse Reactions Caused by Allopurinol. Proc. Natl. Acad. Sci. U S A. 102, 4134–4139. doi:10.1073/pnas.0409500102
Hung, S. I., Chung, W. H., Liu, Z. S., Chen, C. H., Hsih, M. S., Hui, R. C., et al. (2010). Common Risk Allele in Aromatic Antiepileptic-Drug Induced Stevens-Johnson Syndrome and Toxic Epidermal Necrolysis in Han Chinese. Pharmacogenomics 11, 349–356. doi:10.2217/pgs.09.162
Husain, Z., Reddy, B. Y., and Schwartz, R. A. (2013). DRESS Syndrome: Part I. Clinical Perspectives. J. Am. Acad. Dermatol. 68, 693–698. doi:10.1016/j.jaad.2013.01.033
Husain, Z., Reddy, B. Y., and Schwartz, R. A. (2013). DRESS Syndrome: Part II. Management and Therapeutics. J. Am. Acad. Dermatol. 68, 709–720. doi:10.1016/j.jaad.2013.01.032
Ikediobi, O., and Schneider, J. A. (2021). Pharmacogenomics of Allopurinol and Sulfamethoxazole/Trimethoprim: Case Series and Review of the Literature. J. Pers Med. 11 (2), 71. doi:10.3390/jpm11020071
Illing, P. T., Vivian, J. P., Dudek, N. L., Kostenko, L., Chen, Z., Bharadwaj, M., et al. (2012). Immune Self-Reactivity Triggered by Drug-Modified HLA-Peptide Repertoire. Nature 486, 554–558. doi:10.1038/nature11147
Jaruthamsophon, K., Tipmanee, V., Sangiemchoey, A., Sukasem, C., and Limprasert, P. (2017). HLA-B*15:21 and Carbamazepine-Induced Stevens-Johnson Syndrome: Pooled-Data and In Silico Analysis. Sci. Rep. 7, 45553. doi:10.1038/srep45553
John, S., Anand, T. C. V., Sukasem, C., Canyuk, B., and Pattharachayakul, S. (2021). Patient, Disease, and Drug-Related Risk Factors Associated with Phenytoin-Induced Cutaneous Adverse Drug Reactions in South Indian Epileptic Patients. Curr. Drug Saf. 18. doi:10.2174/157488631602211118122907
John, S., Balakrishnan, K., Sukasem, C., Anand, T. C. V., Canyuk, B., and Pattharachayakul, S. (2021). Association of HLA-B*51:01, HLA-B*55:01, CYP2C9*3, and Phenytoin-Induced Cutaneous Adverse Drug Reactions in the South Indian Tamil Population. J. Pers Med. 11 (8), 737. doi:10.3390/jpm11080737Dsg
Joshi, R., Tripathi, M., Gupta, P., Gulati, S., and Gupta, Y. K. (2017). Adverse Effects & Drug Load of Antiepileptic Drugs in Patients with Epilepsy: Monotherapy versus Polytherapy. Indian J. Med. Res. 145 (3), 317–326. doi:10.4103/ijmr.IJMR_710_15
Kabashima, R., Sugita, K., Sawada, Y., Hino, R., Nakamura, M., and Tokura, Y. (2011). Increased Circulating Th17 Frequencies and Serum IL-22 Levels in Patients with Acute Generalized Exanthematous Pustulosis. J. Eur. Acad. Dermatol. Venereol. 25 (4), 485–488. doi:10.1111/j.1468-3083.2010.03771.x
Kamada, N., Kinoshita, K., Togawa, Y., Kobayashi, T., Matsubara, H., Kohno, M., et al. (2006). Chronic Pulmonary Complications Associated with Toxic Epidermal Necrolysis: Report of a Severe Case with Anti-ro/SS-A and a Review of the Published Work. J. Dermatol. 33, 616–622. doi:10.1111/j.1346-8138.2006.00142.x
Kang, H. R., Jee, Y. K., Kim, Y. S., Lee, C. H., Jung, J. W., Kim, S. H., et al. (2011). Positive and Negative Associations of HLA Class I Alleles with Allopurinol-Induced SCARs in Koreans. Pharmacogenet Genomics 21, 303–307. doi:10.1097/FPC.0b013e32834282b8
Kang, M. G., Sohn, K. H., Kang, D. Y., Park, H. K., Yang, M. S., Lee, J. Y., et al. (2019). Analysis of Individual Case Safety Reports of Severe Cutaneous Adverse Reactions in Korea. Yonsei Med. J. 60 (2), 208–215. doi:10.3349/ymj.2019.60.2.208
Kaniwa, N., Saito, Y., Aihara, M., Matsunaga, K., Tohkin, M., Kurose, K., et al. (2010). HLA-B*1511 Is a Risk Factor for Carbamazepine-Induced Stevens-Johnson Syndrome and Toxic Epidermal Necrolysis in Japanese Patients. Epilepsia 51, 2461–2465. doi:10.1111/j.1528-1167.2010.02766.x
Kaniwa, N., Sugiyama, E., Saito, Y., Kurose, K., Maekawa, K., Hasegawa, R., et al. (2013). Specific HLA Types Are Associated with Antiepileptic Drug-Induced Stevens-Johnson Syndrome and Toxic Epidermal Necrolysis in Japanese Subjects. Pharmacogenomics 14, 1821–1831. doi:10.2217/pgs.13.180
Kano, Y., Hirahara, K., Mitsuyama, Y., Takahashi, R., and Shiohara, T. (2007). Utility of the Lymphocyte Transformation Test in the Diagnosis of Drug Sensitivity: Dependence on its Timing and the Type of Drug Eruption. Allergy 62, 1439–1444. doi:10.1111/j.1398-9995.2007.01553.x
Kano, Y., Ishida, T., Hirahara, K., and Shiohara, T. (2010). Visceral Involvements and Long-Term Sequelae in Drug-Induced Hypersensitivity Syndrome. Med. Clin. North. Am. 94, 743–xi. doi:10.1016/j.mcna.2010.03.004
Kano*, Y., and Shiohara, T. (2013). Long-term Outcome of Patients with Severe Cutaneous Adverse Reactions. Dermatologica Sinica 31, 211–216. doi:10.1016/j.dsi.2013.09.004
Kardaun, S. H., Sekula, P., Valeyrie-Allanore, L., Liss, Y., Chu, C. Y., Creamer, D.., et al. (2013). Drug Reaction with Eosinophilia and Systemic Symptoms (DRESS): an Original Multisystem Adverse Drug Reaction. Results from the Prospective RegiSCAR Study. Br. J. Dermatol. 169, 1071–1080. doi:10.1111/bjd.12501
Kardaun, S. H., Sidoroff, A., Valeyrie-Allanore, L., Halevy, S., Davidovici, B. B., Mockenhaupt, M., et al. (2007). Variability in the Clinical Pattern of Cutaneous Side-Effects of Drugs with Systemic Symptoms: Does a DRESS Syndrome Really Exist? Br. J. Dermatol. 156 (3), 609–611. doi:10.1111/j.1365-2133.2006.07704.x
Kashiwagi, M., Aihara, M., Takahashi, Y., Yamazaki, E., Yamane, Y., Song, Y., et al. (2008). Human Leukocyte Antigen Genotypes in Carbamazepine-Induced Severe Cutaneous Adverse Drug Response in Japanese Patients. J. Dermatol. 35 (10), 683–685. doi:10.1111/j.1346-8138.2008.00548.x
Kazeem, G. R., Cox, C., Aponte, J., Messenheimer, J., Brazell, C., Nelsen, A. C., et al. (2009). High-resolution HLA Genotyping and Severe Cutaneous Adverse Reactions in Lamotrigine-Treated Patients. Pharmacogenet Genomics 19, 661–665. doi:10.1097/FPC.0b013e32832c347d
Khor, A. H., Lim, K. S., Tan, C. T., Wong, S. M., and Ng, C. C. (2014). HLA-B*15:02 Association with Carbamazepine-Induced Stevens-Johnson Syndrome and Toxic Epidermal Necrolysis in an Indian Population: a Pooled-Data Analysis and Meta-Analysis. Epilepsia 55, e120–4. doi:10.1111/epi.12802
Kiertiburanakul, S., Bushyakanist, A., Puangpetch, A., and Sukasem, C. (2015). The Use of Pharmacogenetics in Clinical Practice for the Treatment of Individuals with HIV Infection in Thailand. Pgpm 2015, 163–170. doi:10.2147/PGPM.S86444
Kijima, Y., Yoshinaka, H., Hirata, M., Nakajo, A., Arima, H., Shinden, Y., et al. (2015). Impaired Wound Healing and Expansion of a Large Ulcer after Bevacizumab with Paclitaxel for Skin Metastases from Breast Cancer: Report of a Case. Surg. Today 45, 498–502. doi:10.1007/s00595-014-0912-6
Kim, G. Y., Anderson, K. R., Davis, D. M. R., Hand, J. L., and Tollefson, M. M. (2020). Drug Reaction with Eosinophilia and Systemic Symptoms (DRESS) in the Pediatric Population: A Systematic Review of the Literature. J. Am. Acad. Dermatol. 83, 1323–1330. doi:10.1016/j.jaad.2020.03.081
Kim, S. H., Kim, M., Lee, K. W., Kim, S. H., Kang, H. R., Park, H. W., et al. (2010). HLA-B*5901 Is Strongly Associated with Methazolamide-Induced Stevens-Johnson Syndrome/toxic Epidermal Necrolysis. Pharmacogenomics 11, 879–884. doi:10.2217/pgs.10.54
Kim, S. H., Lee, K. W., Song, W. J., Kim, S. H., Jee, Y. K., Lee, S. M., et al. (2011). Carbamazepine-induced Severe Cutaneous Adverse Reactions and HLA Genotypes in Koreans. Epilepsy Res. 97, 190–197. doi:10.1016/j.eplepsyres.2011.08.010
Kim, S. H., Lee, S. K., Kim, S. H., Park, H. W., Chang, Y. S., Lee, K. W., et al. (2013). Antituberculosis Drug-Induced Hypersensitivity Syndrome and its Association with Human Leukocyte Antigen. Tuberculosis (Edinb) 93, 270–274. doi:10.1016/j.tube.2012.10.010
Klaewsongkram, J., Sukasem, C., Thantiworasit, P., Suthumchai, N., Rerknimitr, P., Tuchinda, P., et al. (2019). Analysis of HLA-B Allelic Variation and IFN-γ ELISpot Responses in Patients with Severe Cutaneous Adverse Reactions Associated with Drugs. J. Allergy Clin. Immunol. Pract. 7 (1), 219–e4. doi:10.1016/j.jaip.2018.05.004
Klaewsongkram, J., Thantiworasit, P., Suthumchai, N., Rerknimitr, P., Sukasem, C., Tuchinda, P., et al. (2016). In Vitro test to Confirm Diagnosis of Allopurinol-Induced Severe Cutaneous Adverse Reactions. Br. J. Dermatol. 175, 994–1002. doi:10.1111/bjd.14701
Kongpan, T., Mahasirimongkol, S., Konyoung, P., Kanjanawart, S., Chumworathayi, P., Wichukchinda, N., et al. (2015). Candidate HLA Genes for Prediction of Co-Trimoxazole-Induced Severe Cutaneous Reactions. Pharmacogenet. Genomics 25 (8), 402–411. doi:10.1097/FPC.0000000000000153
Kunimi, Y., Hirata, Y., Aihara, M., Yamane, Y., and Ikezawa, Z. (2011). Statistical Analysis of Stevens-Johnson Syndrome Caused by Mycoplasma Pneumonia Infection in Japan. Allergol. Int. 60, 525–532. doi:10.2332/allergolint.11-OA-0309
Landsteiner, K., and Jacobs, J.. Studies on the Sensitization of Animals with Simple Chemical Compounds. J. Exp. Med. 1936, 64–625.
Li, L. F., and Ma, C. (2006). Epidemiological Study of Severe Cutaneous Adverse Drug Reactions in a City District of China. Clin. Exp. Dermatol. 31 (5), 642–647. doi:10.1111/j.1365-2230.2006.02185
Lipowicz, S., Sekula, P., Ingen-Housz-Oro, S., Liss, Y., Sassolas, B., Dunant, A., et al. (2013). Prognosis of Generalized Bullous Fixed Drug Eruption: Comparison with Stevens-Johnson Syndrome and Toxic Epidermal Necrolysis. Br. J. Dermatol. 168 (4), 726–732. doi:10.1111/bjd.12133
Locharernkul, C., Loplumlert, J., Limotai, C., Korkij, W., Desudchit, T., Tongkobpetch, S., et al. (2008). Carbamazepine and Phenytoin Induced Stevens-Johnson Syndrome Is Associated with HLA-B*1502 Allele in Thai Population. Epilepsia 49, 2087–2091. doi:10.1111/j.1528-1167.2008.01719.x
Loo, C. H., Tan, W. C., Khor, Y. H., and Chan, L. C. (2018). A 10-years Retrospective Study on Severe Cutaneous Adverse Reactions (SCARs) in a Tertiary Hospital in Penang, Malaysia. Med. J. Malaysia 73 (2), 73–77.
Maggs, J. L., Naisbitt, D. J., Tettey, J. N., Pirmohamed, M., and Park, B. K. (2000). Metabolism of Lamotrigine to a Reactive Arene Oxide Intermediate. Chem. Res. Toxicol. 13 (11), 1075–1081. doi:10.1021/tx0000825
Mallal, S., Nolan, D., Witt, C., Masel, G., Martin, A. M., Moore, C., et al. (2002). Association between Presence of HLA-B*5701, HLA-DR7, and HLA-DQ3 and Hypersensitivity to HIV-1 Reverse-Transcriptase Inhibitor Abacavir. Lancet 359 (9308), 727–732. doi:10.1016/s0140-6736(02)07873-x
Manuyakorn, W., Mahasirimongkol, S., Likkasittipan, P., Kamchaisatian, W., Wattanapokayakit, S., Inunchot, W., et al. (2016). Association of HLA Genotypes with Phenobarbital Hypersensitivity in Children. Epilepsia 57 (10), 1610–1616. doi:10.1111/epi.13509
Manuyakorn, W., Mahasirimongkol, S., Likkasittipan, P., Kamchaisatian, W., Wattanapokayakit, S., Inunchot, W., et al. (2016). Association of HLA Genotypes with Phenobarbital Hypersensitivity in Children. Epilepsia 57 (10), 1610–1616. doi:10.1111/epi.13509
Mariuzza, R. A., Agnihotri, P., and Orban, J. (2020). The Structural Basis of T-Cell Receptor (TCR) Activation: An Enduring enigma. J. Biol. Chem. 295 (Issue 4), 914–925. doi:10.1016/S0021-9258(17)49904-210.1074/jbc.REV119.009411
McCormack, M., Alfirevic, A., Bourgeois, S., Farrell, J. J., Kasperavičiūtė, D., Carrington, M., et al. (2011). HLA-A*3101 and Carbamazepine-Induced Hypersensitivity Reactions in Europeans. N. Engl. J. Med. 364 (12), 1134–1143. doi:10.1056/NEJMoa1013297
Mehta, T. Y., Prajapati, L. M., Mittal, B., Joshi, C. G., Sheth, J. J., Patel, D. B., et al. (2009). Association of HLA-B*1502 Allele and Carbamazepine-Induced Stevens-Johnson Syndrome Among Indians. Indian J. Dermatol. Venereol. Leprol. 75 (6), 579–582. doi:10.4103/0378-6323.57718
Meier-Schiesser, B., Feldmeyer, L., Jankovic, D., Mellett, M., Satoh, T. K., Yerly, D., et al. (2019). Culprit Drugs Induce Specific IL-36 Overexpression in Acute Generalized Exanthematous Pustulosis. J. Invest. Dermatol. 139, 848–858. doi:10.1016/j.jid.2018.10.023
Meier-Schiesser, B., Feldmeyer, L., Jankovic, D., Mellett, M., Satoh, T. K., Yerly, D., et al. (2019). Culprit Drugs Induce Specific IL-36 Overexpression in Acute Generalized Exanthematous Pustulosis. J. Invest. Dermatol. 139, 848–858. doi:10.1016/j.jid.2018.10.023
Messenheimer, J., MullensYoung, E. L. F., Giorgi, L., and Young, F. (1998). Safety Review of Adult Clinical Trial Experience with Lamotrigine. Drug Saf. 18, 281–296. doi:10.2165/00002018-199818040-00004
Mitre, V., Applebaum, D. S., Albahrani, Y., and Hsu, S. (2017). Generalized Bullous Fixed Drug Eruption Imitating Toxic Epidermal Necrolysis: A Case Report and Literature Review. Dermatol. Online J. 23 (7), 13030/qt25v009gs.
Mockenhaupt, M. (2012). Epidemiology of Cutaneous Adverse Drug Reactions. ChemImmunol Allergy 97, 1–17. doi:10.1159/000335612
Napatrupron, K., Jirawat, P., Thawinee, J., Voralaksana, T., Wichittra, T., Jettanong, K., et al. (2017). Association of HLA-A and HLA-B Alleles with Lamotrigine-Induced Cutaneous Adverse Drug Reactions in the Thai Population Frontiers in Pharmacology 8, 879. Available at: https://www.frontiersin.org/article/10.3389/fphar.2017.00879.
Neefjes, J. J., and Ploegh, H. L. (1992). Intracellular Transport of MHC Class II Molecules. Immunol. Today 13 (5), 179–184. doi:10.1016/0167-5699(92)90123-O
Nguyen, D. V., Chu, H. C., Nguyen, D. V., Phan, M. H., Craig, T., Baumgart, K., et al. (2015). HLA-B*1502 and Carbamazepine-Induced Severe Cutaneous Adverse Drug Reactions in Vietnamese. Asia Pac. Allergy 5 (2), 68–77. doi:10.5415/apallergy.2015.5.2.68
Niihara, H., Kaneko, S., Ito, T., Sugamori, T., Takahashi, N., Kohno, K., et al. (2013). HLA-B*58:01 Strongly Associates with Allopurinol-Induced Adverse Drug Reactions in a Japanese Sample Population. J. Dermatol. Sci. 71, 150–152. doi:10.1016/j.jdermsci.2013.04.013
Norcross, M. A., Luo, S., Lu, L., Boyne, M. T., Gomarteli, M., Rennels, A. D., et al. 2012, Abacavir Induces Loading of Novel Self-Peptides into HLA-B*57; 26(11):F21–F29.doi:10.1097/qad.0b013e328355fe8f
Oberlin, K. E., Rahnama-Moghadam, S., Alomari, A. K., and Haggstrom, A. N. (2019). Drug Reaction with Eosinophilia and Systemic Symptoms: Pediatric Case Series and Literature Review. Pediatr. Dermatol. 36, 887–892. doi:10.1111/pde.13949
Ogawa, K., Morito, H., Hasegawa, A., Daikoku, N., Miyagawa, F., Okazaki, A., et al. (2013). Identification of Thymus and Activation-Regulated Chemokine (TARC/CCL17) as a Potential Marker for Early Indication of Disease and Prediction of Disease Activity in Drug-Induced Hypersensitivity Syndrome (DIHS)/drug Rash with Eosinophilia and Systemic Symptoms (DRESS). J. Dermatol. Sci. 69 (1), 38–43. doi:10.1016/j.jdermsci.2012.10.002
Ogawa, K., Morito, H., Hasegawa, A., Miyagawa, F., Kobayashi, N., Watanabe, H., et al. (2014). Elevated Serum Thymus and Activation-Regulated Chemokine (TARC/CCL17) Relates to Reactivation of Human Herpesvirus 6 in Drug Reaction with Eosinophilia and Systemic Symptoms (DRESS)/drug-induced Hypersensitivity Syndrome (DIHS). Br. J. Dermatol. 171 (2), 425–427. doi:10.1111/bjd.12948
Ohtoshi, S., Kitami, Y., Sueki, H., and Nakada, T. (2014). Utility of Patch Testing for Patients with Drug Eruption. Clin. Exp. Dermatol. 39 (3), 279–283. doi:10.1111/ced.12239
Olteanu, C., Shear, N. H., Chew, H. F., Hashimoto, R., Alhusayen, R., Whyte-Croasdaile, S., et al. (2018). Severe Physical Complications Among Survivors of Stevens-Johnson Syndrome and Toxic Epidermal Necrolysis. Drug Saf. 41, 277–284. doi:10.1007/s40264-017-0608-0
Ono, M., Ito, T., Kanai, T., Murayama, K., Koyama, H., Maeno, K., et al. (2013). Rapid Tumor Necrosis and Massive Hemorrhage Induced by Bevacizumab and Paclitaxel Combination Therapy in a Case of Advanced Breast Cancer. Onco Targets Ther. 6, 1393–1398. doi:10.2147/OTT.S51164
Osman, M. (2003). Therapeutic Implications of Sex Differences in Asthma and Atopy. Arch. Dis. Child. 88 (7), 587–590. doi:10.1136/adc.88.7.587
Palmieri, T. L., Greenhalgh, D. G., Saffle, J. R., Spence, R. J., Peck, M. D., Jeng, J. C., et al. (2002). A Multicenter Review of Toxic Epidermal Necrolysis Treated in U.S. Burn Centers at the End of the Twentieth century. J. Burn Care Rehabil. 23, 87–96. doi:10.1097/00004630-200203000-00004
Panpruk, R., Puangsricharern, V., Klaewsongkram, J., Rerknimitr, P., Kittipibul, T., Chongpison, Y., et al. (2021). Clinical Parameters and Biological Markers Associated with Acute Severe Ocular Complications in Stevens-Johnson Syndrome and Toxic Epidermal Necrolysis. Sci. Rep. 11 (1), 20275. doi:10.1038/s41598-021-99370-1
Park, H. J., Park, J. W., Kim, S. H., Choi, S. Y., Kim, H. K., Jung, C. G., et al. (2020). The HLA-B*13:01 and the Dapsone Hypersensitivity Syndrome in Korean and Asian Populations: Genotype- and Meta-Analyses. Expert Opin. Drug Saf. 19 (10), 1349–1356. doi:10.1080/14740338.2020.1796965
Patel, T. K., Barvaliya, M. J., Sharma, D., and Tripathi, C. (2013). A Systematic Review of the Drug-Induced Stevens-Johnson Syndrome and Toxic Epidermal Necrolysis in Indian Population. Indian J. Dermatol. Venereol. Leprol. 79 (3), 389–398. doi:10.4103/0378-6323.110749
Patel, T. K., Thakkar, S. H., and Sharma, D. (2014). Cutaneous Adverse Drug Reactions in Indian Population: A Systematic Review. Indian Dermatol. Online J. 5 (6), S76–S86. doi:10.4103/2229-5178.146165
Pfistershammer, K., Petzelbauer, P., Stingl, G., Mastan, P., Chott, A., Jäger, U., et al. (2010). Methotrexate-induced Primary Cutaneous Diffuse Large B-Cell Lymphoma with an 'angiocentric' Histological Morphology. Clin. Exp. Dermatol. 35, 59–62. doi:10.1111/j.1365-2230.2009.03293.x
Pichler, W. J. (2008). The P-I Concept: Pharmacological Interaction of Drugs with Immune Receptors. World Allergy Organ. J. 1 (6), 96–102. doi:10.1097/WOX.0b013e3181778282
Pinho, A., Coutinho, I., Gameiro, A., Gouveia, M., and Gonçalo, M. (2017). Patch Testing - a Valuable Tool for Investigating Non-immediate Cutaneous Adverse Drug Reactions to Antibiotics. J. Eur. Acad. Dermatol. Venereol. 31, 280–287. doi:10.1111/jdv.13796
Porebski, G., Pecaric-Petkovic, T., Groux-Keller, M., Bosak, M., Kawabata, T. T., and Pichler, W. J. (2013). In Vitro drug Causality Assessment in Stevens-Johnson Syndrome - Alternatives for Lymphocyte Transformation Test. Clin. Exp. Allergy 43, 1027–1037. doi:10.1111/cea.12145
Rana, S., Gupta, K., Agarwal, N., and Ahamed, A. N. M. (2021). A Study of Cutaneous Adverse Drug Reactions and Their Association with Autoimmune Diseases at a Tertiary Centre in South-West Rajasthan, India. Indian J. Dermatol. 66 (4), 445. doi:10.4103/ijd.IJD_261_17
Raucci, U., Rossi, R., Da Cas, R., Rafaniello, C., Mores, N., Bersani, G., et al. (2013). Stevens-johnson Syndrome Associated with Drugs and Vaccines in Children: a Case-Control Study. PLoS One 8 (7), e68231. doi:10.1371/journal.pone.0068231
Romagnani, S., Maggi, E., Liotta, F., Cosmi, L., and Annunziato, F. (2009). Properties and Origin of Human Th17 Cells. Mol. Immunol. 47 (1), 3–7. doi:10.1016/j.molimm.2008.12.019
Rozieres, A., Hennino, A., Rodet, K., Gutowski, M. C., Gunera-Saad, N., Berard, F., et al. (2009). Detection and Quantification of Drug-specific T Cells in Penicillin Allergy. Allergy 64, 534–542. doi:10.1111/j.1398-9995.2008.01674.x
Ryder, E. N. C., and Perkins, W. (2018). Acute Localised Exanthematous Pustulosis: Case Report, Review of the Literature and Proposed Diagnostic Criteria. Australas. J. Dermatol. 59, 226–227. doi:10.1111/ajd.12734
Saha, A., Das, N. K., Hazra, A., Gharami, R. C., Chowdhury, S. N., and Datta, P. K. (2012). Cutaneous Adverse Drug Reaction Profile in a Tertiary Care Out Patient Setting in Eastern India. Indian J. Pharmacol. 44 (6), 792–797. doi:10.4103/0253-7613.103304
Sanabria-Cabrera, J., Medina-Cáliz, I., Stankevičiūtė, S., Rodríguez-Nicolás, A., Almarza-Torres, M., Lucena, M. I., et al. (2019). Drug-Induced Liver Injury Associated with Severe Cutaneous Hypersensitivity Reactions: A Complex Entity in Need of a Multidisciplinary Approach. Curr. Pharm. Des. 25 (36), 3855–3871. doi:10.2174/1381612825666191107161912
Sánchez, A. R., Sambucety, P. S., García, C. P., and Prieto, M. A. R. (2019). Atypical Presentation of Primary Cutaneous CD8 Positive Aggressive Epidermotropic Cytotoxic T-Cell Lymphoma. Indian Dermatol. Online J. 10, 298–299. doi:10.4103/idoj.IDOJ_257_18
Sassolas, B., Haddad, C., Mockenhaupt, M., Dunant, A., Liss, Y., Bork, K., et al. (2010). ALDEN, an Algorithm for Assessment of Drug Causality in Stevens-Johnson Syndrome and Toxic Epidermal Necrolysis: Comparison with Case-Control Analysis. Clin. Pharmacol. Ther. 88, 60–68. doi:10.1038/clpt.2009.252
Sassolas, B., Haddad, C., Mockenhaupt, M., Dunant, A., Liss, Y., Bork, K., et al. (2010). ALDEN, an Algorithm for Assessment of Drug Causality in Stevens-Johnson Syndrome and Toxic Epidermal Necrolysis: Comparison with Case-Control Analysis. Clin. Pharmacol. Ther. 88, 60–68. doi:10.1038/clpt.2009.252
Satyanarayana, V. V., Purushothaman, S., and Chandipriya, B. (2020). Clinical Spectrum of Cutaneous Adverse Drug Reactions in Pediatric Population in East Coast of Andhra Pradesh: An Observational Study. Indian J. Paediatr. Dermatol. 22, 37–42.
Schaerli, P., Britschgi, M., Keller, M., Steiner, U. C., Steinmann, L. S., Moser, B., et al. (2004). Characterization of Human T Cells that Regulate Neutrophilic Skin Inflammation. J. Immunol. 173 (3), 2151–2158. doi:10.4049/jimmunol.173.3.2151
Schmitz, B., and Bergmann, L. (2007). The Use of Lamotrigine in Female Patients. Nervenarzt 78 (August8), 912–922. doi:10.1007/s00115-006-2060-8
Schwartz, R. A., McDonough, P. H., and Lee, B. W. (2013). Toxic Epidermal Necrolysis: Part II. Prognosis, Sequelae, Diagnosis, Differential Diagnosis, Prevention, and Treatment. J. Am. Acad. Dermatol. 69, 187.e1–204. doi:10.1016/j.jaad.2013.05.002
Shanbhag, S. S., Chodosh, J., Fathy, C., Goverman, J., Mitchell, C., and Saeed, H. N. (2020). Multidisciplinary Care in Stevens-Johnson Syndrome. Ther. Adv. Chronic Dis. 11, 2040622319894469. doi:10.1177/2040622319894469
Sharma, K., and Marcus, J. R. (2013). Bevacizumab and Wound-Healing Complications: Mechanisms of Action, Clinical Evidence, and Management Recommendations for the Plastic Surgeon. Ann. Plast. Surg. 71, 434–440. doi:10.1097/SAP.0b013e31824e5e57
Shear, N. H., and Spielberg, S. P. (1988). Anticonvulsant Hypersensitivity Syndrome. In Vitro Assessment of Risk. J. Clin. Invest. 82, 1826–1832. doi:10.1172/JCI113798
Shi, Y. W., Min, F. L., Qin, B., Zou, X., Liu, X. R., Gao, M. M., et al. (2012). Association between HLA and Stevens-Johnson Syndrome Induced by Carbamazepine in Southern Han Chinese: Genetic Markers besides B*1502? Basic Clin. Pharmacol. Toxicol. 111, 58–64. doi:10.1111/j.1742-7843.2012.00868.x
Sidoroff, A., Halevy, S., Bavinck, J. N., Vaillant, L., and Roujeau, J. C. (2001). Acute Generalized Exanthematous Pustulosis (AGEP)--a Clinical Reaction Pattern. J. Cutan. Pathol. 28 (3), 113–119. doi:10.1034/j.1600-0560.2001.028003113.x
Sousa-Pinto, B., Pinto-Ramos, J., Correia, C., Gonçalves-Costa, G., Gomes, L., Gil-Mata, S., et al. (2015). Pharmacogenetics of Abacavir Hypersensitivity: A Systematic Review and Meta-Analysis of the Association with HLA-B*57:01. J. Allergy Clin. Immunol. 136, 1092–e3. doi:10.1016/j.jaci.2015.03.019
Spriet, S., and Banks, T. A. (2015). Drug Reaction with Eosinophilia and Systemic Symptoms Syndrome. Allergy Asthma Proc. 36, 501–505. doi:10.2500/aap.2015.36.3903
Spriet, S., and Banks, T. A. (2015). Drug Reaction with Eosinophilia and Systemic Symptoms Syndrome. Allergy Asthma Proc. 36, 501–505. doi:10.2500/aap.2015.36.3903
Spriet, S., and Banks, T. A. (2015). Drug Reaction with Eosinophilia and Systemic Symptoms Syndrome. Allergy Asthma Proc. 36, 501–505. doi:10.2500/aap.2015.36.3903
Steinhoff, M., Beyer, M., Roewert-Huber, J., Lukowsky, A., Assaf, C., and Sterry, W. (2008). Complete Clinical Remission of Tumor-Stage Mycosis Fungoides after Acute Extensive Skin Necroses, Granulomatous Reaction, and Fever under Treatment with Bexarotene, Vorinostat, and High-Dose Fenofibrate. J. Am. Acad. Dermatol. 58, S88–S91. doi:10.1016/j.jaad.2007.07.012
Stern, R. S., and Divito, S. J. (2017). Stevens-Johnson Syndrome and Toxic Epidermal Necrolysis: Associations, Outcomes, and Pathobiology-Thirty Years of Progress but Still Much to Be Done. J. Invest. Dermatol. 137 (5), 1004–1008. doi:10.1016/j.jid.2017.01.003
Su, P., and Aw, C. W. (2014). Severe Cutaneous Adverse Reactions in a Local Hospital Setting: a 5-year Retrospective Study. Int. J. Dermatol. 53 (11), 1339–1345. doi:10.1111/ijd.12118
Su, S. C., Mockenhaupt, M., Wolkenstein, P., Dunant, A., Le Gouvello, S., Chen, C. B., et al. (2017). Interleukin-15 Is Associated with Severity and Mortality in Stevens-Johnson Syndrome/Toxic Epidermal Necrolysis. J. Invest. Dermatol. 137 (5), 1065–1073. doi:10.1016/j.jid.2016.11.034
Sukasem, C., Katsila, T., Tempark, T., Patrinos, G. P., and Chantratita, W. (2018). Drug-Induced Stevens-Johnson Syndrome and Toxic Epidermal Necrolysis Call for Optimum Patient Stratification and Theranostics via Pharmacogenomics. Annu. Rev. Genomics Hum. Genet. 19, 329–353. doi:10.1146/annurev-genom-083115-022324
Sukasem, C., Pratoomwun, J., Satapornpong, P., Klaewsongkram, J., Rerkpattanapipat, T., Rerknimitr, P., et al. (2020). Genetic Association of Co-trimoxazole-induced Severe Cutaneous Adverse Reactions Is Phenotype-specific: HLA Class I Genotypes and Haplotypes. Clin. Pharmacol. Ther. 108 (5), 1078–1089. doi:10.1002/cpt.1915
Sukasem, C., Sririttha, S., Tempark, T., Klaewsongkram, J., Rerkpattanapipat, T., Puangpetch, A., et al. (2020). Genetic and Clinical Risk Factors Associated with Phenytoin-Induced Cutaneous Adverse Drug Reactions in Thai Population. Pharmacoepidemiol. Drug Saf. 29 (5), 565–574. doi:10.1002/pds.4979
Sukasem, C., Sririttha, S., Chaichan, C., Nakkrut, T., Satapornpong, P., Jaruthamsophon, K., et al. (2021). Spectrum of Cutaneous Adverse Reactions to Aromatic Antiepileptic Drugs and Human Leukocyte Antigen Genotypes in Thai Patients and Meta-Analysis. Pharmacogenomics J. 21, 682–690. doi:10.1038/s41397-021-00247-3
Sullivan, J. R., and Shear, N. H. (2002). Drug Eruptions and Other Adverse Drug Effects in Aged Skin. Clin. Geriatr. Med. 18 (1), 21–42. doi:10.1016/s0749-0690(03)00032-6
Suthumchai, N., Srinoulprasert, Y., Thantiworasit, P., Rerknimitr, P., Tuchinda, P., Chularojanamontri, L., et al. (2018). The Measurement of Drug-Induced Interferon γ-releasing Cells and Lymphocyte Proliferation in Severe Cutaneous Adverse Reactions. J. Eur. Acad. Dermatol. Venereol. 32, 992–998. doi:10.1111/jdv.14890
Szatkowski, J., and Schwartz, R. A. (2015). Acute Generalized Exanthematous Pustulosis (AGEP): A Review and Update. J. Am. Acad. Dermatol. 73, 843–848. doi:10.1016/j.jaad.2015.07.017
Talal, N. (1987). Autoimmune Mechanisms in Patients and Animal Models. Toxicol. Pathol. 15 (3), 272–275. doi:10.1177/019262338701500303
Tan, S. K., and Tay, Y. K. (2012). Profile and Pattern of Stevens-Johnson Syndrome and Toxic Epidermal Necrolysis in a General Hospital in Singapore: Treatment Outcomes. Acta Derm Venereol. 92 (1), 62–66. doi:10.2340/00015555-1169
Tangamornsuksan, W., Chaiyakunapruk, N., Somkrua, R., Lohitnavy, M., and Tassaneeyakul, W. (2013). Relationship between the HLA-B*1502 Allele and Carbamazepine-Induced Stevens-Johnson Syndrome and Toxic Epidermal Necrolysis: a Systematic Review and Meta-Analysis. JAMA Dermatol. 149, 1025–1032. doi:10.1001/jamadermatol.2013.4114
Tangamornsuksan, W., and Lohitnavy, M. (2018). Association between HLA-B*1301 and Dapsone-Induced Cutaneous Adverse Drug Reactions: A Systematic Review and Meta-Analysis. JAMA Dermatol. 154 (4), 441–446. doi:10.1001/jamadermatol.2017.6484
Tapia, B., Padial, A., Sánchez-Sabaté, E., Alvarez-Ferreira, J., Morel, E., Blanca, M., et al. (2004). Involvement of CCL27-CCR10 Interactions in Drug-Induced Cutaneous Reactions. J. Allergy Clin. Immunol. 114 (2), 335–340. doi:10.1016/j.jaci.2004.04.034
Tassaneeyakul, W., Jantararoungtong, T., Chen, P., Lin, P. Y., Tiamkao, S., Khunarkornsiri, U., et al. (2009). Strong Association between HLA-B*5801 and Allopurinol-Induced Stevens-Johnson Syndrome and Toxic Epidermal Necrolysis in a Thai Population. Pharmacogenet Genomics 19 (9), 704–709. doi:10.1097/FPC.0b013e328330a3b8
Tassaneeyakul, W., Tiamkao, S., Jantararoungtong, T., Chen, P., Lin, S. Y., Chen, W. H., et al. (2010). Association between HLA-B*1502 and Carbamazepine-Induced Severe Cutaneous Adverse Drug Reactions in a Thai Population. Epilepsia 51 (5), 926–930. doi:10.1111/j.1528-1167.2010.0253310.1111/j.1528-1167.2010.02533.x
Tempark, T., Deekajorndech, T., Chatproedprai, S., Supornsilchai, V., and Wananukul, S. (2022). Late Sequelae of Drug Reaction with Eosinophilia and Systemic Symptoms (DRESS) Cause Thyroid Dysfunction and Thyroiditis: Review of Literature. J. Pediatr. Endocrinol. Metab. doi:10.1515/jpem-2021-0685
Tempark, T., Satapornpong, P., Rerknimitr, P., Nakkam, N., Saksit, N., Wattanakrai, P., et al. (2022). Dapsone-induced severe cutaneous adverse drug reactions are strongly linked with HLA-B*13: 01 allele in the Thai population. Pharmacogenet Genomics 2017; 27 (12): 429–437.
Teyton, L., O'Sullivan, D., Dickson, P. W., Lotteau, V., Sette, A., Fink, P., et al. (1990). Invariant Chain Distinguishes between the Exogenous and Endogenous Antigen Presentation Pathways. Nature 348 (6296), 39–44. doi:10.1038/348039a0
Thong, B. Y., Leong, K. P., Tang, C. Y., and Chng, H. H. (2003). Drug Allergy in a General Hospital: Results of a Novel Prospective Inpatient Reporting System. Ann. Allergy Asthma Immunol. 90 (3), 342–347. doi:10.1016/S1081-1206(10)61804-2
Tran, C., Knowles, S. R., Liu, B. A., and Shear, N. H. (1998). Gender Differences in Adverse Drug Reactions. J. Clin. Pharmacol. 38 (11), 1003–1009. doi:10.1177/009127009803801103
Tsai, Y. G., Liou, J. H., Hung, S. I., Chen, C. B., Chiu, T. M., Wang, C. W., et al. . Increased Type 2 Innate Lymphoid Cells in Patients with Drug Reaction with Eosinophilia and Systemic Symptom. J. Investigdermatol2019 78, 98.
Ueta, M., Kaniwa, N., Sotozono, C., Tokunaga, K., Saito, Y., Sawai, H., et al. (2014). Independent strong Association of HLA-A*02:06 and HLA-B*44:03 with Cold Medicine-Related Stevens-Johnson Syndrome with Severe Mucosal Involvement. Sci. Rep. 4, 4862. doi:10.1038/srep04862
Ueta, M., Kannabiran, C., Wakamatsu, T. H., Kim, M. K., Yoon, K. C., Seo, K. Y., et al. (2014). Trans-ethnic Study Confirmed Independent Associations of HLA-A*02:06 and HLA-B*44:03 with Cold Medicine-Related Stevens-Johnson Syndrome with Severe Ocular Surface Complications. Sci. Rep. 4, 5981. doi:10.1038/srep05981
van der Ven, A. J., Koopmans, P. P., Vree, T. B., and van der Meer, J. W. (1991). Adverse Reactions to Co-trimoxazole in HIV Infection. Lancet 338 (8764), 431–433. doi:10.1016/0140-6736(91)91046-w
Verma, R., Vasudevan, B., and Pragasam, V. (2013). Severe Cutaneous Adverse Drug Reactions. Med. J. Armed Forces India 69 (4), 375–383. doi:10.1016/j.mjafi.2013.01.007
Villani, A., Baldo, A., De Fata Salvatores, G., Desiato, V., Ayala, F., and Donadio, C. (2017). Acute Localized Exanthematous Pustulosis (ALEP): Review of Literature with Report of Case Caused by Amoxicillin-Clavulanic Acid. Dermatol. Ther. (Heidelb) 7, 563–570. doi:10.1007/s13555-017-0206-1
Villanueva-Paz, M., Niu, H., Segovia-Zafra, A., Medina-Caliz, I., Sanabria-Cabrera, J., Lucena, M. I., et al. (2021). Critical Review of Gaps in the Diagnosis and Management of Drug-Induced Liver Injury Associated with Severe Cutaneous Adverse Reactions. J. Clin. Med. 10 (22), 5317. doi:10.3390/jcm10225317
Wang, Q., Zhou, J. Q., Zhou, L. M., Chen, Z. Y., Fang, Z. Y., Chen, S. D., et al. (2011). Association between HLA-B*1502 Allele and Carbamazepine-Induced Severe Cutaneous Adverse Reactions in Han People of Southern China mainland. Seizure 20 (6), 446–448. doi:10.1016/j.seizure.2011.02.003
Watanabe, H. (2018). Recent Advances in Drug-Induced Hypersensitivity syndrome/Drug Reaction with Eosinophilia and Systemic Symptoms. J. Immunol. Res. 2018, 5163129. doi:10.1155/2018/5163129
Watkins, S., and Pichler, W. (2013). Activating Interactions of Sulfanilamides with T Cell Receptors. Open J. Immunol. 3, 139–157. doi:10.4236/oji.2013.33019
Wucherpfennig, K. W., Gagnon, E., Call, M. J., Huseby, E. S., and Call, M. E. (2010). Structural Biology of the T-Cell Receptor: Insights into Receptor Assembly, Ligand Recognition, and Initiation of Signaling. Cold Spring Harb Perspect. Biol. 2 (4), a005140. doi:10.1101/cshperspect.a005140
Yampayon, K., Sukasem, C., Limwongse, C., Chinvarun, Y., Tempark, T., Rerkpattanapipat, T., et al. (2017). Influence of Genetic and Non-genetic Factors on Phenytoin-Induced Severe Cutaneous Adverse Drug Reactions. Eur. J. Clin. Pharmacol. 73 (7), 855–865. doi:10.1007/s00228-017-2250-2
Yang, D., Han, Z., and Oppenheim, J. J. (2017). Alarmins and Immunity. Immunol. Rev. 280 (1), 41–56. doi:10.1111/imr.12577
Yang, F., Gu, B., Zhang, L., Xuan, J., Luo, H., Zhou, P., et al. (2014). HLA-B*13:01 Is Associated with Salazosulfapyridine-Induced Drug Rash with Eosinophilia and Systemic Symptoms in Chinese Han Population. Pharmacogenomics 15, 1461–1469. doi:10.2217/pgs.14.69
Yang, F., Xuan, J., Chen, J., Zhong, H., Luo, H., Zhou, P., et al. (2016). HLA-B*59:01: a Marker for Stevens-Johnson Syndrome/toxic Epidermal Necrolysis Caused by Methazolamide in Han Chinese. Pharmacogenomics J. 16, 83–87. doi:10.1038/tpj.2015.25
Yang, S. C., Hu, S., Zhang, S. Z., Huang, J. W., Zhang, J., Ji, C., et al. (2018). The Epidemiology of Stevens-Johnson Syndrome and Toxic Epidermal Necrolysis in China. J. Immunol. Res. 11, 4320195. doi:10.1155/2018/4320195
Yuliwulandari, R., Kristin, E., Prayuni, K., Sachrowardi, Q., Suyatna, F. D., Menaldi, S. L., et al. (2017). Association of the HLA-B Alleles with Carbamazepine-Induced Stevens-Johnson Syndrome/toxic Epidermal Necrolysis in the Javanese and Sundanese Population of Indonesia: the Important Role of the HLA-B75 Serotype. Pharmacogenomics 18, 1643–1648. doi:10.2217/pgs-2017-0103
Yun, J., Cai, F., Lee, F. J., and Pichler, W. J. (2016). T-cell-mediated Drug Hypersensitivity: Immune Mechanisms and Their Clinical Relevance. Asia Pac. Allergy 6 (2), 77–89. doi:10.5415/apallergy.2016.6.2.77
Zanni, M. P., von Greyerz, S., Schnyder, B., Wendland, T., and Pichler, W. J. (1998). Allele-Unrestricted Presentation of Lidocaine by HLA-DR Molecules to Specific Alphabeta+ T Cell Clones. Int. Immunol. 10 (4), 507–515. doi:10.1093/intimm/10.4.507
Zhang, F. R., Liu, H., Irwanto, A., Fu, X. A., Li, Y., Yu, G. Q., et al. (2013). HLA-B*13:01 and the Dapsone Hypersensitivity Syndrome. N. Engl. J. Med. 369, 1620–1628. doi:10.1056/NEJMoa1213096
Zhang, S., Tang, S., Li, S., Pan, Y., and Ding, Y. (2020). Biologic TNF-Alpha Inhibitors in the Treatment of Stevens-Johnson Syndrome and Toxic Epidermal Necrolysis: a Systemic Review. J. Dermatolog Treat. 31, 66–73. doi:10.1080/09546634.2019.1577548
Zhou, P., Zhang, S., Wang, Y., Yang, C., and Huang, J. (20161936). Structural Modeling of HLA-Landsteiner K, Jacobs J. Studies on the Sensitization of Animals with Simple Chemical Compounds. J. Exp. Med. 64, 625‐639.
Zhu, J., Peng, T., Johnston, C., Phasouk, K., Kask, A. S., Klock, A., et al. (2013). Immune Surveillance by CD8αα+ Skin-Resident T Cells in Human Herpes Virus Infection. Nature 497 (7450), 494–497. doi:10.1038/nature12110
Keywords: SCARs, pharmacogenomics, PGx implementation, Thailand, immunopathogenesis of SCARs, risk factors
Citation: Tempark T, John S, Rerknimitr P, Satapornpong P and Sukasem C (2022) Drug-Induced Severe Cutaneous Adverse Reactions: Insights Into Clinical Presentation, Immunopathogenesis, Diagnostic Methods, Treatment, and Pharmacogenomics. Front. Pharmacol. 13:832048. doi: 10.3389/fphar.2022.832048
Received: 14 December 2021; Accepted: 01 March 2022;
Published: 20 April 2022.
Edited by:
José Antonio Cornejo-García, Universidad de Málaga, SpainReviewed by:
M. Isabel Lucena, University of Malaga, SpainMarc Pallardy, Université Paris-Saclay, France
Copyright © 2022 Tempark, John, Rerknimitr, Satapornpong and Sukasem. This is an open-access article distributed under the terms of the Creative Commons Attribution License (CC BY). The use, distribution or reproduction in other forums is permitted, provided the original author(s) and the copyright owner(s) are credited and that the original publication in this journal is cited, in accordance with accepted academic practice. No use, distribution or reproduction is permitted which does not comply with these terms.
*Correspondence: Chonlaphat Sukasem, Y2hvbmxhcGhhdC5zdWtAbWFoaWRvbC5hYy50aA==
†These authors share first authorship