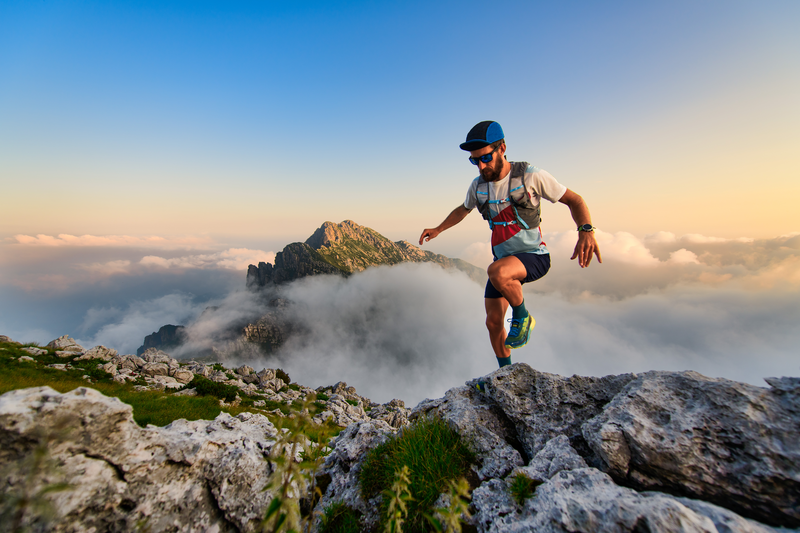
94% of researchers rate our articles as excellent or good
Learn more about the work of our research integrity team to safeguard the quality of each article we publish.
Find out more
ORIGINAL RESEARCH article
Front. Pharmacol. , 21 March 2022
Sec. Translational Pharmacology
Volume 13 - 2022 | https://doi.org/10.3389/fphar.2022.829830
This article is part of the Research Topic Women in Science - Translational Medicine 2021 View all 14 articles
At present, the drug treatment of osteoporosis is mostly focused on inhibiting osteoclastogenesis, which has relatively poor effects. Metformin is a drug that can potentially promote osteogenic differentiation and improve bone mass in postmenopausal women. We aimed to detect the molecular mechanism underlying the osteogenic effect of metformin. Our study indicated that metformin obviously increased the Alkaline phosphatase activity and expression of osteogenic marker genes at the mRNA and protein levels. The PI3K/AKT signaling pathway was revealed to play an essential role in the metformin-induced osteogenic process, as shown by RNA sequencing. We added LY294002 to inhibit the PI3K/AKT pathway, and the results indicated that the osteogenic effect of metformin was also blocked. Additionally, the sequencing data also indicated oxidation-reduction reaction was involved in the osteogenic process of osteoblasts. We used H2O2 to mimic the oxidative damage of osteoblasts, but metformin could attenuate it. Antioxidative Nrf2/HO-1 pathway, regarded as the downstream of PI3K/AKT pathway, was modulated by metformin in the protective process. We also revealed that metformin could improve bone mass and oxidative level of OVX mice. In conclusion, our study revealed that metformin promoted osteogenic differentiation and H2O2-induced oxidative damage of osteoblasts via the PI3K/AKT/Nrf2/HO-1 pathway.
Osteoporosis is characterized by a decrease in the amount of bone tissue per unit volume and mainly occurs in postmenopausal women and diabetes patients (Chapurlat et al., 2020; Zhou et al., 2020). Estrogen deficiency and glucose overload reduce the inhibition of osteoclast differentiation and enhances bone resorption, leading to the loss of bone mass (Ponte et al., 2020; Cho et al., 2021; Sha et al., 2021). The current strategy for osteoporosis treatment is mainly to inhibit osteoclasts with drugs such as bisphosphonates, estrogen receptor modulators and calcitonin (Ukon et al., 2019; Hsiao et al., 2020; Toriumi et al., 2020). However, these drugs are limited because they only prevent further loss of bone mass, and do not restore it. To improve this condition, researchers can increase the activity of osteoblasts in patients, which will be an effective means of treatment. Weakened differentiation and declined activity of osteoblasts aggravate the deterioration of osteoporosis (Xu et al., 2020; Yang et al., 2020). Therefore, enhancing differentiation and increasing activity is essential in improving the treatment of osteoporosis.
The acceleration of the aging population has resulted in a gradual increase in the incidence of osteoporotic fractures. Estrogen deficiency and impaired glucose tolerance are important factors that accelerate aging (He et al., 2020; Kashima et al., 2021). And the decline in body function related to aging is caused by oxidative damage with the accumulation of reactive oxygen species (ROS) (Buccellato et al., 2021). Recent studies have determined that metformin shows anti-aging effects and resistance to diseases caused by aging, which indicates the potential application of metformin in osteoporosis (Martel et al., 2021; Sharma et al., 2021). Metformin is a hypoglycemic drug that has been verified to improve bone mass loss in diabetes-induced osteoporosis by decreasing the blood glucose level (Tseng, 2021). And metformin also shows potential therapeutic effects in postmenopausal osteoporosis (Yang et al., 2021). However, the direct effect of metformin on bone metabolism is unclear. The molecular mechanism of metformin on osteogenic differentiation and prevention of oxidative damage remain to be determined.
A new generation of high-throughput transcriptome sequencing methods have been used in basic research, clinical diagnostics and drug development (Koborova et al., 2009; Wang et al., 2013). RNA sequencing technology has become an important method in transcriptomic research. The principle is to sequence genomic cDNA, calculate the expression of different mRNAs by counting the number of related small cDNA fragments and analyze the expression level of the transcript (Niemi et al., 2015). This technology can elucidate gene function and structure at the overall level and reveal specific biological processes and molecular mechanisms in the occurrence of diseases (Cui et al., 2020; Wang Y et al., 2021). In previous studies, RNA sequencing has been widely used to determine the targets and pathways of drugs in disease research (Chen et al., 2021; Zhou et al., 2021). Therefore, we aimed to detect the molecular mechanism of metformin in osteogenic differentiation by using RNA sequencing.
The reagents and chemicals used in this study are listed below. MC3T3-E1 cells were purchased from the Chinese Academy of Sciences Cell Bank. Metformin was purchased from Meilunbio (Dalian, China). Antibodies against Runx2 (1:1000; cat. no. ab236639), Collagen Ⅰ (1:2500; cat. no. ab260043) Osteocalcin (OCN) (1:100; cat. no. ab93876), Nrf2 (1:1000, cat. no. ab92946) and HO-1 (1:5000; cat. no. ab68477) were obtained from Abcam (Cambridge, MA). β-actin antibodies (1:2000; cat. no. 66009-1-Ig) and a peroxidase-conjugated anti-rat secondary antibody (1:2000; cat. nos. SA00001-15) were purchased from Protein Tech Group, Inc. (Chicago, IL, United States). antibodies against PI3K (1:1,000; cat. no. 4257), phosphorylated (p-)PI3K (1:1,000; cat. no. 4228), AKT (1:1,000; cat. no. 4691) and p-AKT (1:1,000; cat. no. 4060) were purchased from Cell Signaling Technology, Inc. The PI3K/AKT signaling inhibitor LY294002 and AKT inhibitor MK2206 was purchased from Beyotime (Shanghai, China).
MC3T3-E1 cells were cultured in α-MEM (HyClone, Logan, UT, United States). The media were supplemented with 10% fetal bovine serum, 100 U/ml streptomycin sulfate, and 100 mg/ml penicillin. Osteogenic induction medium was prepared according to the following criterion: 100 mM β-glycerophosphate, 50 mg/L ascorbic acid and 10 nM dexamethasone. The parameters of the humidified incubator for MC3T3-E1 cell culture were set to 5% CO2 and 37°C. Detection of ALP activity and mRNA and protein expression levels was performed after 7 days of osteogenic induction of MC3T3-E1 cells.
Cell Counting Kit-8 (CCK-8) (Dojindo Molecular Technologies, Inc. Japan) was used to detect cell viability after treatment with different concentrations of metformin. The reagent is an indicator of redox reactions. In the presence of the electron carrier 1-methoxy PMS, dehydrogenase in living cells can catalyze the tetrazolium salt WST-8 to generate formazan dyes, and the amount of formazan dye produced has a linear relationship with the number of living cells.
ALP is secreted by osteoblasts. ALP activity can directly reflect the differentiation level of osteoblasts. MC3T3-E1 cells were induced in osteogenic medium for 1 week. Then the ALP level was detected by ALP Analysis kits (Nanjing Jiancheng, China) according to the manufacturer’s instructions.
Calcium salt variation is an indicator of osteoblast proliferation and differentiation. Alizarin Red S can form a complex with calcium salt in a chelating manner to identify the calcium salt component of tissue cells and produce orange-red deposits. MC3T3-E1 cells were cultured in 6-well plates and treated with induced medium for 28 days before Alizarin red S staining. The cells were first washed twice with PBS, fixed with 95% ethanol for 10 min, and washed with distilled water 3 times. Then 0.1% Alizarin Red-Tris-Hcl (pH 8.3) was added at 37°C for 30 min.
A miRNeasy RNA mini kit (Qiagen, MD, United States) was used to extract total RNA. Then, GoScript™ reverse transcription mix and oligo (dT) (Promega, Wi, United States) were used to synthesize cDNA. qPCR was performed using GoTaq® qPCR master mix (Promega, Wi, United States). The data were collected using a Roche Light Cycler® 480 II system (Roche, Basel, Switzerland). The conditions of PCR cycles were as follows: 2 min at 90°C, 15 s at 95°C and 60 s at 60°C for 45 cycles. β-actin was used as a standardized control. And the sequences of primers were listed in Supplementary Table S1. Gene expression was calculated by the 2−ΔΔCt method.
Protein was extracted after 7 days of treatment, and then frozen in a refrigerator at −80°C for later use.
Then, proteins were resolved by SDS-PAGE and transferred to polyvinylidene difluoride (PVDF) membranes. The membranes with proteins of various molecular weights were immersed in blocking buffer for 1.5 h. After the membranes were washed with 1% TBST, they were incubated with a primary antibody at 4°C overnight and a secondary antibody at 4°C the next day. After the membranes were thoroughly washed, the protein bands were coated with luminescent solution and visualized using a chemiluminescence (ECL) system (UVP Inc., CA, United States). The protein level was normalized to that of β-actin (molecular weight of 43 kDa). Finally, ImageJ software was used to calculate the optical density and relative protein expression levels.
MC3T3-E1 cells were induced in osteogenic medium and metformin for 3 days. RNA sequencing analysis was performed by Novogene Institute (Tianjin, China). Differential expression analysis of two groups (three biological replicates per condition) was performed using the DESeq2 R package (1.20.0). Gene Ontology (GO) enrichment analysis of differentially expressed genes was implemented by the cluster Profiler R package, in which gene length bias was corrected. GO terms with corrected p values less than 0.05 were considered significantly enriched by differentially expressed genes. The KEGG pathway database was used in the cluster Profiler R package for the statistical enrichment of marker genes.
GO enrichment analysis of marker genes was performed with DAVID Bioinformatics Resources 6.8. The gene length bias was corrected, and the GO terms with corrected p values less than 0.05 were considered significantly enriched for the marker genes. The biological domains examined in the GO database included three categories: molecular function, cellular component and biological process.
The KEGG pathway database (KOBAS 3.0) was used for the statistical enrichment of marker genes. The database can help elucidate the high-level functions and utilities of cells, organisms and ecosystems and includes molecular-level information (especially large-scale molecular genome sequencing datasets) and information from other high-throughput experimental technologies.
Eight-week-old female mice were obtained from the Department of Laboratory Animal Science of China Medical University. The feeding environmental conditions were 20–26°C with constant temperature, 40–70% relative humidity, ≤14 mg/m3 ammonia concentration, ≤60 dB (A) noise and a 12 h/12 h alternating light and dark cycle. All animals were fed in this environment for 2 weeks before the experiments. Mice were randomly divided into three groups (n = 7 each group): a sham group, a bilateral ovariectomy (OVX) group and a OVX group with intragastric metformin (OVX + Met). We performed bilateral ovariectomy on mice under 1.4–1.5% isoflurane inhalation anesthesia with oxygen. The dosage of metformin for the OVX + Met mice was 100 mg/kg/day, which was dissolved in 0.9% normal saline. The mice in the other groups were fed only with equal amount of saline. After 8 weeks of treatment, all mice were sacrificed, and the bilateral femur and tibias were harvested for imaging and protein extraction. All animal experiments were approved by the Animal Ethics Committee of the First Affiliated Hospital of China Medical University and were performed according to the laboratory and animal welfare guidelines.
Collected femurs were fixed with 4% formaldehyde solution for imaging by microcomputed tomography (μCT, Skyscan1276, Bruker, Germany). When X-rays pass through the sample, each part of the sample has different absorption rates for X-rays. The X-rays penetrate the sample and are finally imaged on the detector. Micro-CT uses tapered X-ray beams to image samples at different angles above 360°. The cone beam method can obtain isotropic volumetric images, improve spatial resolution, and increase ray utilization. X-ray images at each angle were reconstructed into a 3-dimensional image analyzed by the CT-analyser software CTAn 1.19.11.1 (Bruker Corporation). The parameters of the scan were as follows: X-ray voltage 50 kV, X-ray current 200 uA, Filter 0.5 mm aluminum, Image pixel size 8.9um, Camera resolution setting High (4,000 pixel field width), Tomographic rotation (180°/360°), Rotation step (0.3–0.5°), Frame averaging 1, Scan duration 20–50 min.
Mice-specific total antioxidant activities (T-AOC) was purchased from Nanjing Jiancheng Bioengineering Institute, Nanjing, China (A015-2-1) and superoxide dismutase (SOD) kits was purchased from Beyotime (S0101) to measure the serum T-AOC and SOD activity in mice. All experiments were performed according to the manufacturer’s instructions.
The experimental data were means ± standard deviation (SD) by using GraphPad Prism 8 (San Diego, CA, United States) and SPSS 22.0 (Chicago, IL, United States). Student’s t-tests and one-way ANOVA were used for statistical analysis of three replicate experiments by SPSS. p < 0.05 was considered statistically significant.
We first performed CCK-8 cell viability assays to detect MC3T3-E1 cell viability after treatment with different concentrations of metformin, and the results indicated that metformin had no inhibitory effect on osteoblast proliferation (Figure 1A). To determine whether metformin promoted osteogenic differentiation and the optimal concentration, we detected ALP activity with ALP Analysis kits. As shown in Figure 1B, metformin at 0.2 mM maximized osteogenic differentiation, which was also verified by the mRNA expression of osteogenic genes including Runx2, OCN and COLL-1 (Figure 1C). Additionally, we performed Alizarin Red S staining and western blotting to evaluate the effect of metformin treatment on the differentiation of osteoblasts (Figures 1D,E). The protein levels of Collagen I, Runx2 and OCN increased most obviously with 0.2 mM metformin treatment (Figure 1F).
FIGURE 1. Metformin promotes osteogenic differentiation of MC3T3-E1 cells. (A) Cell viability after treatment with different concentrations of metformin (0.1, 0.2 and 0.3 mM). (B) ALP activity was detected to explicit the osteogenic effect of metformin. (C) The mRNA level of COLL-1, OCN and Runx2 after osteogenic induction and treatment with metformin. (D) The mineralization of osteoblast demonstrated by Alizarin red S staining was most obvious after treatment with 0.2 mM metformin. (E) The protein level of Collagen I, OCN and Runx2 under different concentrations of metformin. (F) Relative protein expression level of the proteins in (E) compared with control group. Experiments were implemented in triplicate. Data are means ± SD, *p < 0.05, **p < 0.01 compared with control cells and #p < 0.05, ##p < 0.01 compared with osteogenic induction alone analyzed by using ANOVA.
Based on the data mentioned above, we attempted to elucidate the mechanism by which metformin promoted osteoblast differentiation. We performed RNA sequencing to compare the differential expression of genes between osteogenic induction with and without 0.2 mM metformin treatment. Compared with osteogenic medium alone, a total of 1946 up-regulated and 1544 down-regulated genes were obtained when treating with metformin after quality control and differential analysis (Figure 2A). We performed KEGG pathway analysis of these up-regulated genes and the results showed that they were enriched in PI3K/AKT signaling pathway (Figure 2B). These genes included PI3K upstream regulators ITGA, ITGB and SYK; the AKT upstream regulators HSP90B1 and PPP2R5D; and the downstream regulators of the PI3K/AKT pathway GYS, PCK2 and CCND1 (Figure 2C). We firstly detected the effect of metformin on the PI3K/AKT pathway by western blotting. And the results indicated the expression of p-PI3K and p-AKT increased in osteogenic medium and metformin promoted it further (Figure 2D). To determine the role of PI3K/AKT signaling pathway in osteogenic induction by metformin, we added the PI3K/AKT signaling inhibitor LY294002 to MC3T3-E1 cells and assessed osteogenic indicators. LY294002 can inhibit the enzyme activity of PI3K through competitive inhibition of PI3K. In Figure 2E, ALP activity decreased after combined treatment with metformin and LY294002 compared to that with metformin treatment alone. Moreover, the protein levels of Collagen I, OCN and Runx2 decreased after combined treatment with metformin and LY294002 compared with metformin treatment alone (Figure 2F). Additionally, we performed Alizarin Red S staining to confirm the effect of the PI3K/AKT signaling pathway on osteoblast formation. The results indicated that LY294002 inhibited mineralization after osteogenic induction (Figure 2G).
FIGURE 2. Metformin induces osteogenic differentiation via the PI3K/AKT pathway. (A) Volcano map of differential genes as osteogenic induction (OI) vs. OI with metformin treatment (OI_Met). (B) Kyoto Encyclopedia of Genes and Genomes (KEGG) of up-regulated genes during metformin-induced osteogenic differentiation. (C) Heatmap of up-regulated genes involved in PI3K/AKT signaling pathway. (D) Protein expression of PI3K-AKT pathway, enhancing effect observed for metformin on PI3K/AKT expression. (E) ALP activity was detected to explicit metformin protection of osteogenic differentiation inhibited by H2O2 was blocked by LY294002. (F) Protein expression of COLL-1, OCN and Runx2 after adding LY294002. (G) The activity of osteoblast mineralization decreased with LY294002 treatment detected by using Alizarin red S staining. Experiments were implemented in triplicate. Data are means ± SD, *p < 0.05, **p < 0.01 compared with control cells and #p < 0.05, ##p < 0.01 compared with osteogenic induction alone and ^p < 0.05, ^^p < 0.01 compared with combined treatment of osteogenic induction and metformin analyzed by using ANOVA.
GO analysis revealed that the genes upregulated expression after metformin treatment were involved in the oxidation-reduction reaction (Figure 3A). These genes included many reductase genes such as CBR2, HMGCR, HSD17B7, RRM2, DHCR24, DHFR, and peroxidase gene PTGS2. All of these genes performed a reductive effect on oxidative substances. In order to detect the protective effect of metformin on osteogenic differentiation in peroxidative status, we added 0.2 mM H2O2 into osteogenic medium to stimulate oxidative damage and 0.2 mM metformin to improve. After osteogenic induction and 6 h incubation with H2O2 and metformin, we detected the protein expression of Collagen I, OCN and Runx2 (Figure 3B). The results indicated the protective effect of metformin (Figure 3C). Then, we attempted to explore the mechanism by which metformin protected the oxidative damage of osteoblasts. Our past studies have proved that metformin can attenuate H2O2-induced osteoblast apoptosis via the PI3K/AKT pathway (Yang et al., 2021). Nrf2 is an important transcription factor that regulates cellular oxidative stress response. And the Heme Oxygenase-1 (HO-1) is the downstream factor of Nrf2. HO-1 participated in the modulation of mitochondria function and further influenced cell differentiation and apoptosis. We used western blotting to detect the protein level of nuclear Nrf2 and HO-1. Metformin increased the expression of Nrf2 and HO-1 compared with H2O2 treatment alone, but which was blocked by adding AKT inhibitor MK2206(Figures 3D,E).
FIGURE 3. Metformin reserves H2O2-induced inhibition of osteogenic differentiation. (A) Heatmap of up-regulated genes involved in oxidation-reduction reaction. (B) Protein expression of Collagen I, Runx 2 and OCN indicated the protective effect of metformin on H2O2 inhibition (OM: osteogenic medium, OM + H: osteogenic medium with H2O2, OM + H + M: osteogenic medium mixed with H2O2 and MK2206). (C) Relative protein expression level of the proteins in (B). (D) Protein expression of p-AKT, nuclear Nrf2 and HO-1 after additing AKT inhibitor MK2206. (E) Relative protein expression level of the proteins in (D). Experiments were implemented in triplicate. Data are means ± SD, *p < 0.05, **p < 0.01 compared with control cells and #p < 0.05, ##p < 0.01 compared with H2O2 treatment alone and ^p < 0.05, ^^p < 0.01 compared with combined treatment of H2O2 and metformin analyzed by using ANOVA.
Finally, we attempted to determine whether metformin could improve bone mass and oxidative level in postmenopausal mice. We performed bilateral ovariectomy on mice to decrease estrogen secretion and fed them a normal diet. Additionally, we fed another set of OVX mice metformin. After 8 weeks, the bone mass of the OVX mice decreased significantly but increased after metformin feeding (Figure 4A). The micro-CT data also revealed the therapeutic effect of metformin on postmenopausal osteoporosis. Trabecular thickness (Tb.Th) and percent bone volume (bone volume/tissue volume, BV/TV) decreased in the OVX mice and increased with metformin treatment. Trabecular separation (Tb.Sp) and bone surface/volume ratio (bone surface/bone volume, BS/BV) increased in the OVX mice and metformin preserved these parameters (Figure 4B). To detect the osteogenic effect of metformin on postmenopausal mice, we also extracted protein from the mouse femur. As shown in Figure 4C, the osteogenic proteins Collagen I, OCN and Runx2 were decreased compared with those in the sham mice, and metformin improved their expression levels. And we also collected the blood of these mice and centrifuged it into serum. The level of superoxide dismutase (SOD) and total antioxidant capacity (T-AOC) were measured by the corresponding kit. And the results indicated metformin reserved the serum level of SOD and T-AOC (Figure 4D). We measured the protein expression of Nrf2 and HO-1 to validate the signaling pathway in bone tissue (Figure 4E). As mentioned above, metformin could improve bone mass loss by enhancing the osteogenic effects and reserving oxidative damage in the OVX mice.
FIGURE 4. Metformin reserved bone mass loss of OVX mice. (A) 2D and 3D reconstruction of the femur micro-CT images. (B) Related parameters obtained from image analysis (n = 7 specimens/group). (C) Protein level of osteogenic markers Collagen I, Runx2 and OCN in femur samples. (D) Serum anti-oxidative level SOD1 and T-AOC in mice (n = 5 specimens/group). (E) The protein expression of Nrf2 and HO-1 in bone tissue. Data are means ± SD, *p < 0.05, **p < 0.01, ***p < 0.001, ****p < 0.0001 compared with OVX analyzed by using ANOVA.
With increased global aging, osteoporosis is a major threat to human health and quality of life, and postmenopausal women have the greatest risk (Chen et al., 2019). The incidence of osteoporotic fractures, which increases the health care burden, is increasing (Jørgensen et al., 2017; Kanis et al., 2021). In recent years, metformin has been found to be a multipotent anti-aging drug with cardiovascular protective and tumor growth inhibitory effects (Bahrambeigi et al., 2019; Elgendy et al., 2019; Deshmukh et al., 2021; Kodali et al., 2021). However, previous studies focused on the hypoglycemic effect of metformin in diabetes-induced osteoporosis but ignored the direct effect on bone metabolism and the molecular mechanism underlying the osteogenic differentiation of metformin. Our study determined the osteogenic effect and optimal concentration of metformin on MC3T3-E1 cell differentiation and the therapeutic effect on postmenopausal osteoporosis. We also performed RNA sequencing to show that the PI3K/AKT signaling pathway and oxidation-reduction reaction were essentially involved in the osteogenic process induced by metformin.
In previous studies, there was no consistent conclusion regarding the pathogenesis of postmenopausal osteoporosis. Our transcriptional data indicated that metformin modulated the expression of genes involved in oxidation-reduction process of osteoblasts. We also believe that oxidation-reduction imbalance is a key factor leading to osteoporosis in postmenopausal women due to the reducibility of estrogen. It has been demonstrated that circulating levels of catalase, superoxide dismutase 2 (SOD 2) and peroxiredoxin 2 (PRX2) are lower in postmenopausal women with osteoporosis than health controls (Azizieh et al., 2019). Mitochondrial dysfunction leads to weakened internal oxidoreductase function, resulting in high levels of free radical, which attacks osteoblasts and induces apoptosis (Zhou et al., 2019). Osteoclasts showed strong differentiation when the inhibitory effect of estrogen was lost (Li et al., 2021). Metformin can improve the oxidative state and protect against oxidative stress damage, which also indicates the therapeutic effect of metformin on osteoporosis (Jia et al., 2021). Therefore, it is essential to determine the mechanism by which metformin acts on the oxidation-reduction process in osteoblasts.
PI3K/AKT signaling is an important pathway involved in the modulation of redox balance. Activation of PI3K/AKT enhances the antioxidant effect (Kim et al., 2021; Wang P et al., 2021). The PI3K/AKT signaling pathway has been demonstrated to have a positive effect on osteoblast differentiation and inhibition of PI3K/AKT signaling suppresses the osteoinduction process (Zhang et al., 2019; Dong et al., 2020). It was reported that multiple drugs promote osteogenic differentiation via the PI3K/AKT pathway (Xiong et al., 2020; Liu et al., 2021). In our study, we revealed that metformin promoted osteogenic differentiation of MC3T3-E1 cells by activating the PI3K/AKT pathway. Various osteogenic growth factors and extracellular matrix components can induce the activation of PI3K, including fibroblast growth factor, vascular endothelial growth factor, angiogenic protein I and insulin (Khodabandehloo et al., 2020). These factors activate receptor tyrosine kinases, cause autophosphorylation and induce PI3K/AKT-mediated differentiation. We also demonstrated that the osteogenic effect of metformin was blocked by adding a PI3K/AKT inhibitor. The inhibitor decreased the ratio of OPG/RANKL expression to inhibit osteogenesis (Xu et al., 2021). Additionally, we used H2O2 to mimic the oxidative damage of osteoblasts metformin to attenuate it, the anti-apoptosis effect of which has been proven in our previous study (Yang et al., 2021). And in this study, we further explored the downstream mechanism. Nrf2 is an factor involved in the modulation of cellular oxidation-reduction balance (Čipak Gašparović et al., 2021). Nrf2 participates in the expression and maturation of various anti-oxidative proteins to maintain the homeostasis of oxidation and reduction (Ying et al., 2018). HO-1 is an essential anti-oxidative factor modulated by Nrf2 (Ma et al., 2021). HO-1 decomposes heme to produce carbon monoxide (CO) that promotes the expression of glutamate-cysteine ligase for GSH transformation (Consoli et al., 2021). As mentioned above, PI3K/AKT/Nrf2/HO-1 pathway is closely related to osteogenic differentiation and anti-oxidative damage of osteoblasts. Our experimental results also indicated protective effect of metformin in postmenopausal osteoporosis via this pathway (Figure 5).
Due to the complex pathogenesis of osteoporosis, current treatment methods have limited efficacy. According to our experiments, metformin can directly increase the gene expression, protein formation of osteogenic markers and protect H2O2-induced oxidative damage. In vitro, we further verified that the bone mass of the OVX mice was significantly improved by metformin feeding. As estrogen is a reductive hormone, detecting the role of oxidoreduction in osteoporosis development and the therapeutic effect of metformin in improving the oxidative state in osteoblasts will be further directions for research on postmenopausal osteoporosis.
Our study demonstrated that metformin could promote osteogenic differentiation and improve H2O2-induced oxidative damage of osteoblasts via the PI3K/AKT/Nrf2/HO-1 pathway. Vitro experiments also demonstrated that metformin improved bone mass, enhanced osteogenic protein expression and increased anti-oxidative level in OVX mice. These results provide an important basis for the potential therapeutic effect of metformin in postmenopausal osteoporosis. Along with its effect in controlling blood glucose and reducing lipids, metformin may have applications in the treatment of osteoporosis.
The data presented in the study are deposited in the GEO (NCBI) repository, accession number (GSE198254). https://www.ncbi.nlm.nih.gov/geo/query/acc.cgi?acc=GSE198254/Supplementary Material.
The animal study was reviewed and approved by the Animal Ethics Committee of the First Affiliated Hospital of China Medical University (No. 2019014).
KY: Data curation, Formal analysis, Data curation, Methodology, Writing—original draft. FC: Data curation, Methodology, Software, SQ: Conceptualization, Writing—original draft, WJ: Investigation, Methodology, Software, LT: Conceptualization, Software, Validation, Writing—review and editing, YZ: Funding acquisition, Project administration, Resources, Writing—review and editing. All authors read and approved the manuscript.
Our study was supported by National Natural Science Foundation of China (NO. 81472044), Department of Laboratory Animal Science of China Medical University and Construction of Clinical Medical Research Center of Orthopedics in Liaoning Province.
The authors declare that the research was conducted in the absence of any commercial or financial relationships that could be construed as a potential conflict of interest.
All claims expressed in this article are solely those of the authors and do not necessarily represent those of their affiliated organizations, or those of the publisher, the editors and the reviewers. Any product that may be evaluated in this article, or claim that may be made by its manufacturer, is not guaranteed or endorsed by the publisher.
The Supplementary Material for this article can be found online at: https://www.frontiersin.org/articles/10.3389/fphar.2022.829830/full#supplementary-material
Azizieh, F. Y., Shehab, D., Jarallah, K. A., Gupta, R., and Raghupathy, R. (2019). Circulatory Levels of RANKL, OPG, and Oxidative Stress Markers in Postmenopausal Women with Normal or Low Bone Mineral Density. Biomark Insights 14, 1177271919843825. doi:10.1177/1177271919843825
Bahrambeigi, S., Yousefi, B., Rahimi, M., and Shafiei-Irannejad, V. (2019). Metformin; an Old Antidiabetic Drug with New Potentials in Bone Disorders. Biomed. Pharmacother. 109, 1593–1601. doi:10.1016/j.biopha.2018.11.032
Buccellato, F. R., D'Anca, M., Fenoglio, C., Scarpini, E., and Galimberti, D. (2021). Role of Oxidative Damage in Alzheimer's Disease and Neurodegeneration: From Pathogenic Mechanisms to Biomarker Discovery. Antioxidants (Basel) 10 (9), 1353. doi:10.3390/antiox10091353
Chapurlat, R., Bui, M., Sornay-Rendu, E., Zebaze, R., Delmas, P. D., Liew, D., et al. (2020). Deterioration of Cortical and Trabecular Microstructure Identifies Women with Osteopenia or Normal Bone Mineral Density at Imminent and Long-Term Risk for Fragility Fracture: A Prospective Study. J. Bone Miner Res. 35, 833–844. doi:10.1002/jbmr.3924
Chen, D. Y., Li, Y. J., Jiang, R. f., Li, Y. t., Feng, J., and Hu, W. (2021). Effects and Mechanism of lncRNA‐27785.1 that Regulates TGF‐β1 of Sika Deer on Antler Cell Proliferation. J. Cel Physiol 236, 5742–5756. doi:10.1002/jcp.30258
Chen, W. C., Lin, E. Y., and Kang, Y. N. (2019). Efficacy and Safety of Elcatonin in Postmenopausal Women with Osteoporosis: a Systematic Review with Network Meta-Analysis of Randomized Clinical Trials. Osteoporos. Int. 30, 1723–1732. doi:10.1007/s00198-019-04997-6
Cho, E., Chen, Z., Ding, M., Seong, J., Lee, S., Min, S. H., et al. (2021). PMSA Prevents Osteoclastogenesis and Estrogen-Dependent Bone Loss in Mice. Bone 142, 115707. doi:10.1016/j.bone.2020.115707
Čipak Gašparović, A., Milković, L., Rodrigues, C., Mlinarić, M., and Soveral, G. (2021). Peroxiporins are Induced upon Oxidative Stress Insult and are Associated with Oxidative Stress Resistance in Colon Cancer Cell Lines. Antioxidants 10, 1856. doi:10.3390/antiox10111856
Consoli, V., Sorrenti, V., Grosso, S., and Vanella, L. (2021). Heme Oxygenase-1 Signaling and Redox Homeostasis in Physiopathological Conditions. Biomolecules 11, 589. doi:10.3390/biom11040589
Cui, Q., Yang, H., Gu, Y., Zong, C., Chen, X., Lin, Y., et al. (2020). RNA Sequencing (RNA-Seq) Analysis of Gene Expression Provides New Insights into Hindlimb Unloading-Induced Skeletal Muscle Atrophy. Ann. Transl Med. 8, 1595. doi:10.21037/atm-20-7400
Deshmukh, A., Ghannam, M., Liang, J., Saeed, M., Cunnane, R., Ghanbari, H., et al. (2021). Effect of Metformin on Outcomes of Catheter Ablation for Atrial Fibrillation. J. Cardiovasc. Electrophysiol. 32, 1232–1239. doi:10.1111/jce.14954
Dong, J., Xu, X., Zhang, Q., Yuan, Z., and Tan, B. (2020). The PI3K/AKT Pathway Promotes Fracture Healing through its Crosstalk with Wnt/β-Catenin. Exp. Cel Res 394, 112137. doi:10.1016/j.yexcr.2020.112137
Elgendy, M., Cirò, M., Hosseini, A., Weiszmann, J., Mazzarella, L., Ferrari, E., et al. (2019). Combination of Hypoglycemia and Metformin Impairs Tumor Metabolic Plasticity and Growth by Modulating the PP2A-GSK3β-MCL-1 Axis. Cancer cell 35, 798–815. e795. doi:10.1016/j.ccell.2019.03.007
He, Q., Gu, L., Lin, Q., Ma, Y., Liu, C., Pei, X., et al. (2020). The Immp2l Mutation Causes Ovarian Aging through ROS-Wnt/β-Catenin-Estrogen Pathway: Preventive Effect of Melatonin. Endocrinology 161 (9), bqaa119. doi:10.1210/endocr/bqaa119
Hsiao, C. Y., Chen, T. H., Chu, T. H., Ting, Y. N., Tsai, P. J., and Shyu, J. F. (2020). Calcitonin Induces Bone Formation by Increasing Expression of Wnt10b in Osteoclasts in Ovariectomy-Induced Osteoporotic Rats. Front. Endocrinol. (Lausanne) 11, 613. doi:10.3389/fendo.2020.00613
Jia, W., Bai, T., Zeng, J., Niu, Z., Fan, D., Xu, X., et al. (2021). Combined Administration of Metformin and Atorvastatin Attenuates Diabetic Cardiomyopathy by Inhibiting Inflammation, Apoptosis, and Oxidative Stress in Type 2 Diabetic Mice. Front Cel Dev Biol 9, 634900. doi:10.3389/fcell.2021.634900
Jørgensen, N. R., Schwarz, P., Iversen, H. K., and Vestergaard, P. (2017). P2Y12 Receptor Antagonist, Clopidogrel, Does Not Contribute to Risk of Osteoporotic Fractures in Stroke Patients. Front. Pharmacol. 8, 821. doi:10.3389/fphar.2017.00821
Kanis, J. A., Johansson, H., Harvey, N. C., Gudnason, V., Sigurdsson, G., Siggeirsdottir, K., et al. (2021). The Effect on Subsequent Fracture Risk of Age, Sex, and Prior Fracture Site by Recency of Prior Fracture. Osteoporos. Int. 32, 1547–1555. doi:10.1007/s00198-020-05803-4
Kashima, S., Inoue, K., and Matsumoto, M. (2021). Low Creatinine Levels in Diabetes Mellitus Among Older Individuals: the Yuport Medical Checkup Center Study. Sci. Rep. 11, 15167. doi:10.1038/s41598-021-94441-9
Khodabandehloo, F., Taleahmad, S., Aflatoonian, R., Rajaei, F., Zandieh, Z., Nassiri-Asl, M., et al. (2020). Microarray Analysis Identification of Key Pathways and Interaction Network of Differential Gene Expressions during Osteogenic Differentiation. Hum. Genomics 14, 43. doi:10.1186/s40246-020-00293-1
Kim, M.-B., Kang, H., Li, Y., Park, Y.-K., and Lee, J.-Y. (2021). Fucoxanthin Inhibits Lipopolysaccharide-Induced Inflammation and Oxidative Stress by Activating Nuclear Factor E2-Related Factor 2 via the Phosphatidylinositol 3-kinase/AKT Pathway in Macrophages. Eur. J. Nutr. 60, 3315–3324. doi:10.1007/s00394-021-02509-z
Koborova, O. N., Filimonov, D. A., Zakharov, A. V., Lagunin, A. A., Ivanov, S. M., Kel, A., et al. (2009). In Silico method for Identification of Promising Anticancer Drug Targets. SAR QSAR Environ. Res. 20, 755–766. doi:10.1080/10629360903438628
Kodali, M., Attaluri, S., Madhu, L. N., Shuai, B., Upadhya, R., Gonzalez, J. J., et al. (2021). Metformin Treatment in Late Middle Age Improves Cognitive Function with Alleviation of Microglial Activation and Enhancement of Autophagy in the hippocampus. Aging Cell 20, e13277. doi:10.1111/acel.13277
Li, Q., Wang, H., Zhang, J., Kong, A. P., Li, G., Lam, T. P., et al. (2021). Deletion of SIRT3 Inhibits Osteoclastogenesis and Alleviates Aging or Estrogen Deficiency-Induced Bone Loss in Female Mice. Bone 144, 115827. doi:10.1016/j.bone.2020.115827
Liu, H., Li, X., Lin, J., and Lin, M. (2021). Morroniside Promotes the Osteogenesis by Activating PI3K/Akt/mTOR Signaling. Biosci. Biotechnol. Biochem. 85, 332–339. doi:10.1093/bbb/zbaa010
Ma, N., Wei, Z., Hu, J., Gu, W., and Ci, X. (2021). Farrerol Ameliorated Cisplatin-Induced Chronic Kidney Disease through Mitophagy Induction via Nrf2/PINK1 Pathway. Front. Pharmacol. 12, 768700. doi:10.3389/fphar.2021.768700
Martel, J., Chang, S. H., Wu, C. Y., Peng, H. H., Hwang, T. L., Ko, Y. F., et al. (2021). Recent Advances in the Field of Caloric Restriction Mimetics and Anti-Aging Molecules. Ageing Res. Rev. 66, 101240. doi:10.1016/j.arr.2020.101240
Niemi, J., Mittman, E., Landau, W., and Nettleton, D. (2015). Empirical Bayes Analysis of RNA-Seq Data for Detection of Gene Expression Heterosis. J. Agric. Biol. Environ. Stat. 20, 614–628. doi:10.1007/s13253-015-0230-5
Ponte, F., Kim, H. N., Iyer, S., Han, L., Almeida, M., and Manolagas, S. C. (2020). Cxcl12 Deletion in Mesenchymal Cells Increases Bone Turnover and Attenuates the Loss of Cortical Bone Caused by Estrogen Deficiency in Mice. J. Bone Miner Res. 35, 1441–1451. doi:10.1002/jbmr.4002
Sha, N. N., Zhang, J. L., Poon, C. C., Li, W. X., Li, Y., Wang, Y. F., et al. (2021). Differential Responses of Bone to Angiotensin II and Angiotensin(1-7): Beneficial Effects of ANG(1-7) on Bone with Exposure to High Glucose. Am. J. Physiol. Endocrinol. Metab. 320, E55–e70. doi:10.1152/ajpendo.00158.2020
Sharma, S., Nozohouri, S., Vaidya, B., and Abbruscato, T. (2021). Repurposing Metformin to Treat Age-Related Neurodegenerative Disorders and Ischemic Stroke. Life Sci. 274, 119343. doi:10.1016/j.lfs.2021.119343
Toriumi, S., Kobayashi, A., and Uesawa, Y. (2020). Comprehensive Study of the Risk Factors for Medication-Related Osteonecrosis of the Jaw Based on the Japanese Adverse Drug Event Report Database. Pharmaceuticals 13, 467. doi:10.3390/ph13120467
Tseng, C. H. (2021). Metformin Use Is Associated with a Lower Risk of Osteoporosis/Vertebral Fracture in Taiwanese Patients with Type 2 Diabetes Mellitus. Eur. J. Endocrinol. 184, 299–310. doi:10.1530/eje-20-0507
Ukon, Y., Makino, T., Kodama, J., Tsukazaki, H., Tateiwa, D., Yoshikawa, H., et al. (2019). Molecular-Based Treatment Strategies for Osteoporosis: A Literature Review. Int. J. Mol. Sci. 20 (10), 2557. doi:10.3390/ijms20102557
Wang, P., Tian, X., Tang, J., Duan, X., Wang, J., Cao, H., et al. (2021). Artemisinin Protects Endothelial Function and Vasodilation from Oxidative Damage via Activation of PI3K/Akt/eNOS Pathway. Exp. Gerontol. 147, 111270. doi:10.1016/j.exger.2021.111270
Wang, W., Qin, Z., Feng, Z., Wang, X., and Zhang, X. (2013). Identifying Differentially Spliced Genes from Two Groups of RNA-Seq Samples. Gene 518, 164–170. doi:10.1016/j.gene.2012.11.045
Wang, Y., Shi, H., Zhang, G., Wu, P., Chen, L., Shen, M., et al. (2021). Transcriptome Analysis of Long Noncoding RNAs and mRNAs in Granulosa Cells of Jinghai Yellow Chickens Illuminated with Red Light. Front. Genet. 12, 563623. doi:10.3389/fgene.2021.563623
Xiong, Y., Zhao, B., Zhang, W., Jia, L., Zhang, Y., and Xu, X. (2020). Curcumin Promotes Osteogenic Differentiation of Periodontal Ligament Stem Cells through the PI3K/AKT/Nrf2 Signaling Pathway. Iran J. Basic Med. Sci. 23, 954–960. doi:10.22038/ijbms.2020.44070.10351
Xu, A., Yang, Y., Shao, Y., Wu, M., and Sun, Y. (2020). Activation of Cannabinoid Receptor Type 2-induced Osteogenic Differentiation Involves Autophagy Induction and P62-Mediated Nrf2 Deactivation. Cell Commun Signal 18, 9. doi:10.1186/s12964-020-0512-6
Xu, L., He, X., Zhou, Y., Yu, K., Yuan, M., Zhang, Q., et al. (2021). Connectivity Map Analysis Identifies Fisetin as a Treatment Compound for Osteoporosis through Activating the PI3K-AKT Signaling Pathway in Mouse Pre-osteoblastic MC3T3-E1 Cells. Curr. Pharm. Biotechnol. 22, 2038–2047. doi:10.2174/1389201022666210301141238
Yang, J., Dong, D., Luo, X., Zhou, J., Shang, P., and Zhang, H. (2020). Iron Overload-Induced Osteocyte Apoptosis Stimulates Osteoclast Differentiation through Increasing Osteocytic RANKL Production In Vitro. Calcif Tissue Int. 107, 499–509. doi:10.1007/s00223-020-00735-x
Yang, K., Pei, L., Zhou, S., Tao, L., and Zhu, Y. (2021). Metformin Attenuates H2O2-Induced Osteoblast Apoptosis by Regulating SIRT3 via the PI3K/AKT Pathway. Exp. Ther. Med. 22, 1316. doi:10.3892/etm.2021.10751
Ying, Y., Jin, J., Ye, L., Sun, P., Wang, H., and Wang, X. (2018). Phloretin Prevents Diabetic Cardiomyopathy by Dissociating Keap1/Nrf2 Complex and Inhibiting Oxidative Stress. Front. Endocrinol. (Lausanne) 9, 774. doi:10.3389/fendo.2018.00774
Zhang, Z., Zhang, X., Zhao, D., Liu, B., Wang, B., Yu, W., et al. (2019). TGF-β1 Promotes the Osteoinduction of Human Osteoblasts via the PI3K/AKT/mTOR/S6K1 Signalling Pathway. Mol. Med. Rep. 19, 3505–3518. doi:10.3892/mmr.2019.10051
Zhou, L., Poon, C. C.-W., Wong, K.-Y., Cao, S., Dong, X., Zhang, Y., et al. (2021). Icariin Ameliorates Estrogen-Deficiency Induced Bone Loss by Enhancing IGF-I Signaling via its Crosstalk with Non-Genomic ERα Signaling. Phytomedicine 82, 153413. doi:10.1016/j.phymed.2020.153413
Zhou, R., Ma, Y., Tao, Z., Qiu, S., Gong, Z., Tao, L., et al. (2020). Melatonin Inhibits Glucose-Induced Apoptosis in Osteoblastic Cell Line through PERK-eIF2α-ATF4 Pathway. Front. Pharmacol. 11, 602307. doi:10.3389/fphar.2020.602307
Keywords: metformin, osteogenic differentiation, RNA sequencing, oxidative damage, PI3K/AKT/Nrf2/HO-1
Citation: Yang K, Cao F, Qiu S, Jiang W, Tao L and Zhu Y (2022) Metformin Promotes Differentiation and Attenuates H2O2-Induced Oxidative Damage of Osteoblasts via the PI3K/AKT/Nrf2/HO-1 Pathway. Front. Pharmacol. 13:829830. doi: 10.3389/fphar.2022.829830
Received: 20 January 2022; Accepted: 02 March 2022;
Published: 21 March 2022.
Edited by:
Andreas Nüssler, University of Tübingen, GermanyReviewed by:
Shyamsundar Pal China, University of California, San Diego, United StatesCopyright © 2022 Yang, Cao, Qiu, Jiang, Tao and Zhu. This is an open-access article distributed under the terms of the Creative Commons Attribution License (CC BY). The use, distribution or reproduction in other forums is permitted, provided the original author(s) and the copyright owner(s) are credited and that the original publication in this journal is cited, in accordance with accepted academic practice. No use, distribution or reproduction is permitted which does not comply with these terms.
*Correspondence: Lin Tao, dGFvbGluZHJAMTYzLmNvbQ==; Yue Zhu, emh1eXVlZHJAMTYzLmNvbQ==
Disclaimer: All claims expressed in this article are solely those of the authors and do not necessarily represent those of their affiliated organizations, or those of the publisher, the editors and the reviewers. Any product that may be evaluated in this article or claim that may be made by its manufacturer is not guaranteed or endorsed by the publisher.
Research integrity at Frontiers
Learn more about the work of our research integrity team to safeguard the quality of each article we publish.