- 1NICM Health Research Institute, Western Sydney University, Penrith, NSW, Australia
- 2Reserach Centre for Chinese Medicine Innovation, The Hong Kong Polytechnic University, Kowloon, Hong Kong SAR, China
- 3Department of Applied Biology and Chemical Technology, The Hong Kong Polytechnic University, Kowloon, Hong Kong SAR, China
Crocins (CRs) and the related active constituents derived from Crocus sativus L. (Saffron) have demonstrated protective effects against cerebral ischemia and ischemic stroke, with various bioactivities including neuroprotection, anti-neuroinflammation, antioxidant, and cardiovascular protection. Among CRs, crocin (CR) has been shown to act on multiple mechanisms and signaling pathways involved in ischemic stroke, including mitochondrial apoptosis, nuclear factor kappa light chain enhancer of B cells pathway, S100 calcium-binding protein B, interleukin-6 and vascular endothelial growth factor-A. CR is generally safe and well-tolerated. Pharmacokinetic studies indicate that CR has poor bioavailability and needs to convert to crocetin (CC) in order to cross the blood-brain barrier. Clinical studies have shown the efficacy of saffron and CR in treating various conditions, including metabolic syndrome, depression, Alzheimer’s disease, and coronary artery disease. There is evidence supporting CR as a treatment for ischemic stroke, although further studies are needed to confirm their efficacy and safety in clinical settings.
Introduction
Stroke (cerebral apoplexy) is a serious cerebrovascular disease and the second leading cause of death globally (WHO, 2020). There are three different categories of stroke, namely ischemic stroke (IS), hemorrhagic stroke (Waziry et al., 2020), and transient ischemic attack (Martinez et al., 2020). The prevalence of stroke varies in different countries, with a high incidence in Oceania, Asia, North Africa, and parts of America (Venketasubramanian, 2021; Zhou et al., 2021). Globally, 101.5 million people suffered from a stroke in 2019, causing 6.6 million deaths. Of these, 77.2 million were IS, resulting in 3.3 million deaths (AMA, 2021; Yang et al., 2021).
IS is caused by a blood clot formed in the brain vasculature (thrombotic stroke) or in the peripheral system travelling to the brain through the bloodstream (embolic stroke) (Shiber et al., 2010; Khan et al., 2017; Ye et al., 2021). Currently, there are still limited therapies for IS, either by surgical intervention to remove the thrombus via thrombectomy (Yoo and Andersson, 2017) or pharmacological interventions using recombinant tissue plasminogen activator (rtPA) intravenous (IV) and lipid-lowering drugs such as statins (Fuentes et al., 2009; Kazley et al., 2013; Alakbarzade and Pereira, 2020; Barthels and Das, 2020; Wechsler, 2020; Orset et al., 2021). These therapies have limited efficacy (Singh and Singh, 2019), low prognosis, and are associated with adverse reactions and the risk of complications (Zheng et al., 2019; Cui et al., 2020; Pergolizzi Jr et al., 2020). In addition, IS patients often have limited access to rtPA (Barthels and Das, 2020) due to the narrow therapeutic window (which must be administered within 4.5 h of IS onset) (Fukuta et al., 2017; Barthels and Das, 2020). The adverse effects of rtPA, such as hemorrhagic transformation with increased matrix metalloprotein (MMP) (Orset et al., 2021), anaphylaxis and systemic bleeding (Chapman et al., 2014; Khandelwal et al., 2021) have restricted its clinical prescription (Cao et al., 2021).
Due to the limitations of the aforementioned therapies, there have been continuing efforts to discover, develop, research, and implement new therapies for IS (Marquez-Romero et al., 2020; Williams et al., 2020). A number of natural ingredients and traditional herbal medicines have been investigated as potential therapies for IS, including ginsenoside Rg1 and Rb1 (Gao, Bai et al., 2020), Naoxinqing (NXQ) (Bei et al., 2004), Buyang Huanwu Decoction (Hao et al., 2012), saffron (SF) (Sharma et al., 2020) and related formulas including Naodesheng (Sugawara and Chan, 2003; Hao et al., 2015), Weinaokang (Zhang et al., 2010), also known as Sailoutong (SLT) (Fan et al., 2021). SLT is a standardized combination of SF that has been shown to be effective for vascular dementia in Phase I and Phase II clinical trials and is currently under the phase III trial for vascular dementia (Chang et al., 2016; Jia et al., 2018; Steiner et al., 2018). NXQ and SLT have shown vigorous antioxidant activity that may play a role in their neuroprotective effects (Bei et al., 2004; Bei et al., 2009; Fan et al., 2021). Ginsenoside Rg1 was demonstrated with a protective effect in animal models against ischemia/reperfusion (I/R) induced injuries (Xie et al., 2015b; Chen et al., 2019; Gao et al., 2020) possibly via alleviating blood-brain barrier (BBB) disruption (Zhou et al., 2014), downregulating inflammatory mediators (Zheng et al., 2019) and ameliorating protease-activated receptor-1 expression (Xie et al., 2015a). Additionally, Gj-4, a CR enrichment extract from Gardenia Jasminoides J. Ellis improved neurovascular protection, mitigating endothelial cell damage (Yang, 2020) and protecting memory deficit in rodents focal cerebral ischemia (Li et al., 2014; Pang et al., 2020; Liu et al., 2021a).
C. sativus L. (Iridaceae) in the superorder of monocots and subdivision of spermatophytes (Crocus-sativus, 2010) yields saffron (SF—the dried stigma) which demonstrated various pharmacological effects, including aphrodisiac (Kashani et al., 2013), anticonvulsant (El Khoudri et al., 2021), antitussive, and antianxiety (Khazdair et al., 2019). SF has long been used as a folk medicine to treat a variety of diseases and conditions, including neurodegenerative diseases, memory disorders, atherosclerosis, hyperlipidaemia, diabetes, high blood pressure, ulcers, and fatty liver disease (Abe and Saito, 2000; Sheikhani et al., 2017; Awasthi and Kulkarni, 2020). C. sativus L. is known to be native to Greece and Iran, and has been extensively cultivated in other countries such as southern Europe, Tibet, and India (Jan et al., 2014). The global production of SF is expected to increase by 12.09% in 2020–2027 (Kothari et al., 2021b), with 90% from Iran (Dhar and Mir, 1997; Kothari et al., 2021a). Iran has been reported as a country with a highly sustainable cultivation source of C. sativus L. based on climatic and edaphic conditions, and production and processing practices (Ghorbani and Koocheki, 2017). CR compounds can also be obtained from other sustainable species. For example, an extraction containing 17% of CRs can be obtained from Gardenia Jasminoides (Sommano et al., 2020). In addition petals of C. sativus L. which are considered a waste product in saffron production, may also be a sustainable source of CR (Zeka et al., 2020) with an estimated 0.6% (w/w) of CR can be recovered from dried petals (Zeka et al., 2015).
More than 100 compounds have been identified from SF, mainly terpenes, flavonoids, and anthraquinones (Chang et al., 2013), including CRs and crocetin (CC), which are responsible for the color of SF (Akbari et al., 2018; Kermanshahi et al., 2020; Csupor et al., 2021; Pandita, 2021). Given that CR is the main active ingredient of SF and SF-containing products such as SLT, it is of prime interest to review the current evidence for CRs in IS and related conditions. The effect of SF on IS has recently been briefly reviewed, although CRs and related compounds were not covered in detail (Azami et al., 2021). Thus, the focus of this review is to evaluate the current evidence on CR and associated analogues for IS, including pre-clinical and clinical studies, molecular mechanisms, toxicity, and safety, as well as current gaps and future directions.
Literature Search Strategy
Electronic databases including PubMed, Cochrane Library, Medline, Embase, Scopus, China National Knowledge Infrastructure (CNKI), and Web of Science were searched for relevant studies from their inception to 16 February 2022. The search terms include “crocin and analogues,” AND “Saffron” OR “Crocus sativus L.” AND “stroke” OR “ischemic stroke” AND “clinical trials” AND “pharmacokinetics,” “crocin” AND “neurons, astrocytes, microglial cells” OR “neuroprotection” OR “cytokines” OR “neuroinflammation” OR “neurotoxicity” OR “antioxidant” OR “apoptosis” OR “signaling pathways” OR “molecular targets” OR “mitochondria” OR “pharmacokinetics” OR “acute/chronic toxicity” OR “clinical trial,” OR “nanoparticle formulation,” “safranal,” “picrocrocin,” “crocetin” and their combinations. 287 research items including peer-reviewed papers, websites, and Chinese data-related research papers were considered suitable according to the review search criterion. Our inclusion criterion was all primary studies involving ischemic stroke, CR, and related compounds OR stroke OR ischemia OR hypoxia. All items identified were screened for relevant references excluding duplicates and non-peer-reviewed articles. Chemical structures of CR compounds were drawn using ChemDraw software (Table 1). Structural information of CRs such as isomeric SMILE was retrieved from PubChem and SciFinder Databases. Human metabolomics data were retrieved from the Human Metabolome Database v4 (HMDB) Canadian Database System (Wishart et al., 2018). All the diagrams (Figure 1) have been created with Adobe Illustrator (Adobe Inc., 2019), Preview MacOS v10.0 (944.5) and BioRender.com.
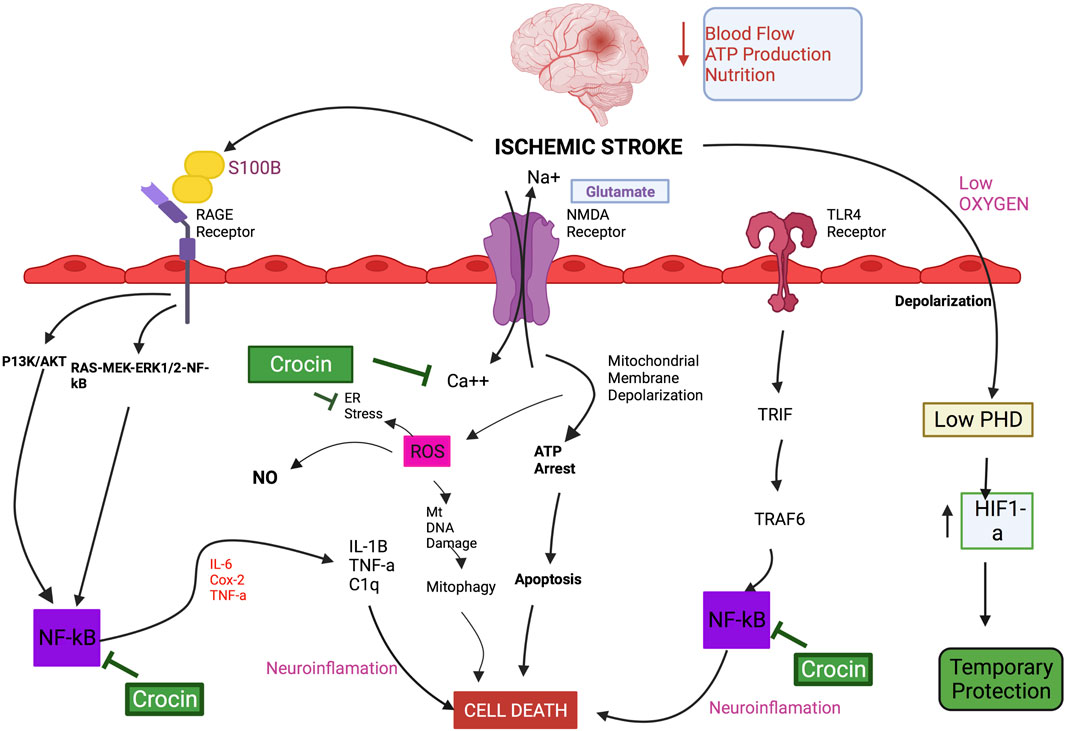
FIGURE 1. Schematic diagram of the mechanistic actions of crocin on the molecular pathways involved in IS.
Crocin and Related Compounds
Crocins (CRs) are a group of carotenoid compounds isolated from SF that also contain other active compounds, including picrocrocin and safranal (Suchareau et al., 2021). Seven different natural CR analogues have been identified from SF, namely CR (crocin 1, CR, CR1), CR 2 (crocin 2, CR II), CR 3 (crocin 3), CR 4 (crocin 4), CR 5 (crocin 5), CR 6 (crocin 6), and CR 7 (crocin 7) (Zhang et al., 2004; Mehrnia et al., 2017; Song et al., 2021b). All are mono or di glycosyl polyene esters of CC (Mohajeri et al., 2010a; Nam et al., 2010; Pang et al., 2020). CR and CR2 are the disaccharide analogues, whilst CR3 and CR4 are the monosaccharide analogues, which are more potent than CR and CR2 because of their structural orientations (Ulbricht et al., 2011). The biosynthesis of CRs is related to several enzymes such as cytochrome p450 (Gao et al., 2021), carotenoid cleavage dioxygenase (CCD), UDP-glycosyl transferase (UGT), and aldehyde dehydrogenase (Nagatoshi et al., 2012; Liu et al., 2020; Pu et al., 2020).
CR is one of the main bioactive ingredients in SF (Kothari et al., 2021b). The level of CRs in SF depends on their origin and quality. A high-quality SF contains about 30% of CRs (Azami et al., 2021). It was shown that Spanish SF contains more CRs, especially CR (9%), than that from other sources (Li et al., 1999). According to the Chinese Pharmacopeia, the total content of CR and CR2 in dried SF used in traditional Chinese medicine shall not be less than 10.0%, and the content of picrocrocin shall not be less than 5.0% (PPRC, 2020). On the other hand, dicrocin and CR3 are the isomers. Tricrocin, CR II or CR 2 are synonyms with the same molecular structure amidst isomeric structural orientation differentiations. To date, studies on CR3, CR4, CR5, CR6, and CR7 are still lacking. Table 1 shows the chemical structures of CR and related compounds.
CR molecule embodies two D-gentiobiose moieties (Mohajeri et al., 2010b; Ebrahim-Habibi et al., 2010). Studies on its structural activity relationship (SAR) revealed that the sugar moieties of CR related to its antioxidant activity and water solubility (Akhtari et al., 2013; Rahaiee et al., 2015; Akbari et al., 2018). Gentiobiose terminus is known to be involved in the conversion of CR to CC in enterocytes (Singla and Giliyaru, 2011). On the other hand, some synthetic CR analogues have been developed. For example, a-glucosyl-(1-6)-trans CRs have been shown with improved water solubility, and antioxidant and neuroprotective activities in mouse neuronal cell line (HT22) neuronal cells (Mok et al., 2020).
CC is a carotenoid (lacking provitamin functionality) recognized by diterpenic and symmetrical structure along with seven double bonds, four methyl functional groups, and two carboxylic groups (Giaccio, 2004) (Table 1). Its sodium salt, trans-sodium crocetinate (TSC), has been developed as a potential drug candidate (Gainer, 2000; Xi and Qian, 2006; Gainer, 2008; Wang et al., 2014; Shah et al., 2021). CC and TSC have similar pharmacological activities as CR (see below). Some bioactive synthetic analogues of CC have also been synthesized such as the diamide derivative of CC (Gao et al., 2017).
Pharmacological Actions of Crocin and Related Compounds Against Ischemic Stroke
Effect on Experimental Ischemic Stroke
The injuries caused by cerebral ischemic and IS are mainly caused by oxidative and nitrosative stress, and are also related to inflammation, apoptosis, BBB dysfunction, and edema formation, which increases the intracranial pressure and decreases the cerebral blood perfusion of ischemic areas (Akbari et al., 2018). The commonly used animal models of cerebral ischemia and IS injuries include middle cerebral artery occlusion (MCAO) in rodents to mimic the I/R injuries in humans after stroke. A number of studies have demonstrated the protective effects of CRs in vitro and in vivo (Table 2). Similar findings were reported by a group showing the protective effects of CR (20 mg/kg) and Weinaokang (10 and 20 mg/kg, which contains CR), against cerebral microvessel injury induced by global ischemia (Zheng et al., 2010). Vakili et al. (2014) reported CR (30, 60, and 120 mg/kg, i.p., given at the start of ischemia) dose-dependently decreased the infarct volume of cerebral I/R injuries. They also found that CR (60 mg/kg, given 1 h before, at the start, or 1 h after ischemia) reduced brain edema by 48%, 52%, and 51%, respectively. Sarshoori et al. (2014) showed in MCAO rats that CR (50 and 80 mg/kg, p.o.) reduced the cortical infarct volume by 48%–60%, and decreased striatal infarct volume by 45%–75%, respectively, with improved neurological deficit scores and decreased number of eosinophilic (prenecrotic) neurons,fiber demyelination and axonal damage in ischemic regions. Similar findings were obtained by Oruc et al. (2016) who used a global cerebral I/R model in rats (bilateral occlusion for 30 min followed by 30 min of reperfusion) and demonstrated that CR (40 mg/kg/day, orally) reduced the histopathological changes and apoptosis, and improved tissues oxidative index (Oruc et al., 2016). CR (10, 20, and 40 mg/kg/day i.p., given 7 days before the operation) attenuated the brain injury compared to that of the model group, with improved symptom score, attenuated brain edema, and improved pathological morphological and structural changes (Finley et al., 2017). Furthermore, CR was also shown to protect the BBB function during cerebral hypoxia/ischemia (Zhang et al., 2017). Similarly, Huang et al. (2019) demonstrated that CR at 50 and 100 mg/kg (p.o. for 7 days) decreased infarct volume and neurological scores in MCAO rats. Bin Wen et al. (2020) found significant results, stating that CR protected brain tissue against cerebral I/R damage, and that this effect was linked to its anti-oxidant and anti-inflammatory properties.
In addition, the curative effect of CR has also been demonstrated. Zheng et al. (2007) used a transient global cerebral ischemia mice model and demonstrated that CR (5, 10, and 20 mg/kg, given intragastrically from day 0 to day 21 after ischemia) significantly improved the capillary integrity and reduced mitochondria damage caused by I/R. Ochiai et al. (2004) reported that CR (10 mg/kg, IV, administrated immediately and 3 h after MCAO) significantly reduced the infarct volume in mice. They also showed that CR was more effective than other CR analogues and SF compounds (tricrocin, dicrocin, and picrocrocin) in promoting the expression of γ-glutamylcysteinyl synthase (γ-GCS) mRNA. γ-GCS is involved in the de novo synthesis of glutathione (GSH) as a rate-limiting enzyme reaction and plays a crucial role in IS (Su et al., 2020; Wu et al., 2020). CR (10 mg/kg) has also been shown to reduce the brain edema and infarct areas induced by hypoxia-ischemia when given immediately or after hypoxia-ischemia in post-natal C57BL/6J mice (Huang et al., 2019). Furthermore, it has been reported recently that CR (30 mg/kg and 60 mg/kg, p.o. for 7 days, administrated after cerebral ischemia) improved the memory loss in a rat model of cerebral ischemia, which was linked to increased hippocampal acetylcholine (ACh) level and reduced apoptosis (Yuan et al., 2020), a finding similar to an earlier observation using CR (25 mg/kg, i.p.) and hydroalcoholic extract of SF (250 mg/kg, i.p.) in a rat model of vascular dementia after permanent bilateral ligation of the common carotid arteries (Hosseinzadeh et al., 2012).
A recent study reported that the cerebral-protective effects of CR against cerebral I/R injury may involve gut microbiota (Zhang et al., 2019). Using a rat transient MCAO model, the investigators showed that the oral administration of CR was more effective than IV injection in reducing infarct volume and improving neurological behaviour changes. Since CC was detected in plasma after oral administration of CR but not after IV injection of CR, and the orally administered CC showed similar protection to that of CR, it indicated the importance of gut microbiota in facilitating the transformation of CR into CC. This was confirmed by the finding that CR could be deglycosylated to CC in the gut content of normal rats, but not in pseudo-germ-free rats. Metabolomics studies also indicated that gut microbiota facilitated the transformation of CR into CC (Zhang et al., 2019).
CC has demonstrated similar pharmacological actions to CR, including neuroprotection, anti-oxidation, and anti-inflammation (Tseng et al., 1995; Li et al., 2018; Hashemi and Hosseinzadeh, 2019; Cerdá-Bernad et al., 2020) as well as protective against cardiac ischemic mitochondrial injury (Gao et al., 2017). Recently Liu et al. (2021) showed that CC (5–50 mg/L) protected hypoxia-induced cell injuries and inhibited apoptosis in cultured human U87 glioma cells, and CC (5,10, and 50 mg/kg) reduced the infarct size and apoptotic cell numbers in brain tissue and improved pathological status in rats. These effects were associated with modulation of miR145-5p, toll-like receptor 4 (TLR4) and nuclear factor kappa light chain enhancer of B cells (NFκB) (p65) (Liu et al., 2021b). CC and related oxygen diffusion-enhancing compounds have been recently reviewed (Shah et al., 2021). These compounds have been shown with properties that improve the diffusion of oxygen in plasma, thus increasing oxygenation in ischemic brain tissue (Manabe et al., 2010; Wang et al., 2014; Bahr-Hosseini et al., 2021; Shah et al., 2021). TSC has shown potential as a therapeutic drug for early stroke intervention reducing the infarct and hemorrhagic volume in rodent models of ischemic and hemorrhagic stroke (Lapchak, 2010; Wang et al., 2014; Shah et al., 2021). In obese MCAO mice, TSC (0.14 mg/kg) showed a significant improvement in neurological deficit and neuroprotective effects evidenced by lowered brain edema, MMP-2, MMP-9, and inflammatory cytokine markers in brain tissues (Deng et al., 2015).
Neuroprotection
One strategy for developing new therapies for IS to target neuroprotective signaling pathways (Rodriguez et al., 2021; Liu et al., 2012). CR has been extensively studied for its neuroprotective effects in vitro and in vivo (Table 2) (Yuan et al., 2020). Studies have shown that CR and related compounds protected CNS neurons in various conditions. For example, CR was demonstrated to protect against PC-12 cells injury in rats by increasing the synthesis of GSH (Ochiai et al., 2004b; Soeda et al., 2007), nitric oxide (NO), and decreasing MMP (Zheng et al., 2007). It induced the proliferation and migration of neural stem cells and inhibited the apoptosis of neural stem cells in cerebral I/R conditions, the effect involved Notch1 signaling and inhibition of inflammatory factors (An et al., 2020). CC also modulated the amyloidogenic pathway and tau misprocessing in neuronal cells (Shah et al., 2021). In the retinal ganglionic cells (RGC), CR prevented apoptosis induced by ischemic injury (Qi et al., 2013). In diabetic rats, CR was shown to act as a neuroprotective agent by lowering the malondialdehyde (MDA) and xanthine oxidase levels in the brain and cerebellum tissue (Altinzo et al., 2014). In addition, CR dose-dependently inhibited the ischemic cerebral neuronal apoptosis of proinflammatory cytokines in the ischemic tissue (Oruc et al., 2016). Huo et al. (2012) investigated the neuroprotective effect of CR in mice with traumatic brain injury. It was found that the intraperitoneal injection of CR (50–200 mg/kg) markedly reduced brain edema and motor functional deficits after the traumatic brain injury induced by physical damage from cortical impact injury. The traumatic brain injury restricted the supply of blood and oxygen in the area which led to the accumulation of reactive oxygen species and subsequent neuronal death.
Recently, CR was found to protect against hippocampal neuron damage in IS (Wu et al., 2020) and dopaminergic neuron damage in 1-methyl-4-phenyl-1,2,3,6-tetrahydropyridine (MPTP)-induced Parkinson’s disease mouse models (Haeri et al., 2019). CR (25 mg/kg) has also been shown to protect neurons against neurotoxicity induced by rotenone, methamphetamine, and acrylamide in mice and Wistar rats (Rao et al., 2019b; Salama et al., 2020; Mehri et al., 2015; Shafahi et al., 2018), as well as improving neuronal survival in hypoxic ischemia related brain damage (Huang and Jia, 2019b). Another study showed that CR (30 mg/kg) alleviated apoptosis, neurodegeneration, and enhanced protection in rotenone-induced Parkinson’s disease rats via mammalian target of rapamycin (mTOR) pathway activation (Salama et al., 2020). CR, via its metabolite crocetin monoglucuronide (CM), was also shown to inhibit ACh activity as predicted by docking studies (Zhu et al., 2019b). In addition, CR improved gut microbiota in stressed mice and decreased serum levels of interleukin (IL-6) and necrosis factor—α (TNF-α) (Wu et al., 2020).
Both CR and CR2 have been shown to enhance neuronal survival by downregulating caspase-3 (Casp3) and Nfkb1 mRNA expression after hypoxic ischemic CNS amelioration (Lv et al., 2019). In addition, CR has been shown to reduce the neurological deficit in a heme oxygenase-1 (HO-1) knockout mouse model of intracerebral hemorrhage (Duan et al., 2019) and reduce cytotoxicity induced by lethal agents. In glutamate-damaged HT22 cells, CR improved cell viability, suppressed reactive oxygen species (ROS) accumulation, calcium ion (Ca+2) load, and apoptosis (Wang et al., 2019). In diazinon induced subacute toxicities, CR at 50, 100, or 200 mg/kg doses (i.p.) significantly ameliorated the adverse effect of diazinon on enzyme levels, and biochemical indices and downregulated the levels of S100 calcium-binding protein B (S100B) (Hariri et al., 2010). Interestingly, S100B is the prime secretary cytokine from astrocyte during metabolic stress and is related to astrocyte activation (Gerlach et al., 2006). Thus, CR may affect the function of astrocytes in its anti-IS action, although its exact effect is still not clear, it has been suggested that neuroprotection in the central nervous system (CNS) may involve astrocyte support (Teo and Bourne, 2018). Targeting astrocytic survival has been shown to lead to lowered neurodegeneration (Freitas-Andrade and Naus, 2016), especially in IS conditiosn (Becerra-Calixto and Cardona-Gómez, 2017).
Anti-Neuroinflammation
There is strong evidence for the involvement of CRs anti-neuroinflammatory effects in their neuroprotective actions (Deslauriers et al., 2011; Ahmed et al., 2020). Studies have shown that CR inhibited the production of certain inflammatory mediators such as TNF-α and interleukin-1B (IL-1B) in microglial cells (Nam et al., 2010; Bin Wen et al., 2020). CR at a dose of 40 mg/kg significantly lowered levels of IL-6 and TNF-α in chronic restraint stress mice (Xiao et al., 2020), as well as inhibited IL-1B in depression-induced mice (Xiao et al., 2019). The anti-neuroinflammatory effect of CR was also shown in the methamphetamine-induced neurotoxicity model in rats (Shafahi et al., 2018), and inhibited inflammation-related microglial activation in mice (Fernández-Albarral et al., 2019), and also mitigated neuroinflammation in rat striatum (Eteghadi et al., 2021). CR (50 mg/kg and 100 mg/kg) inhibited lipopolysaccharide (LPS) induced neuroinflammation in rats, whilst the effect was not dose-dependent (Azmand and Rajaei, 2021). Furthermore, CR downregulated IL-1β, NO, IL-6, and TNF-α generation in rats with hemorrhagic shock (HS) and increased the level of IL-10 (Yang and Dong, 2017). IL-10 is an anti-neuroinflammatory cytokine expressed by immune cells (Saraiva and O’garra, 2010). The upregulation of IL-10 by CR is also supported by other studies (Bakshi et al., 2018; Badavi et al., 2020; Yousefi et al., 2021), although there is a contradictory finding that CR decreased IL-10 level (Dianat and Radan, 2014). The reason for this discrepancy is not clear. It may be related to experimental or disease conditions. CR (10 mg/kg) attenuated TNF-a, inducible nitric oxide synthase (iNOS), NFκB expressions during doxorubicin-induced nephrotoxicity in rats (Hussain et al., 2021). In addition, CR has also been shown to regulate cyclooxygenase-1 (COX-1) and COX-2 enzymes in LPS induced RAW264.7 cells (Xu et al., 2009). In 5XFAD (5X Familial AD) mice, CR (10 mg/kg/day) improved BBB integrity and lowered amyloid ß (Aß) associated neuroinflammation while this effect was accompanied by suppressing mitogen-activated protein kinase (MAPK) and NFκB but activating nuclear factor-erythroid factor 2 related factor 2 (Nrf2) pathways (Batarseh et al., 2017; Hashemzaei et al., 2020). The role of anti-inflammatory effect of CRs on neuronal pain has been recently reviewed, showing suppression of NFκB in turn downregulates the levels of IL-6, IL-10, IL-1β, and TNF-α (Hashemzaei et al., 2020). NFκB relates closely to IS involving inflammatory biosensors (Harari and Liao, 2010). Similarly, anti-neuroinflammatory activities of CC and TSC have also been demonstrated, including inhibiting the formation of proinflammatory mediators, such as NO and cytokines, and regulating NFκB pathway (Deng et al., 2015; Hashemi and Hosseinzadeh, 2019; Liu et al., 2021b; Shah et al., 2021).
Antioxidant Activity
Numerous studies have demonstrated that endogenous antioxidant levels were lowered after acute IS due to oxidative stress (Ullegaddi et al., 2006), and increased cellular ROS levels could ultimately lead to mitochondrial injury and cell death (Shahbaz, 2017a; Shahbaz, 2017b). Hence, antioxidants have long been investigated as a potential therapy for reducing IS injury (Shirley et al., 2014; Cichoń et al., 2017). The antioxidant activity of CR and related compounds have been well established, with increasing superoxide dismutase (SOD) and GSH synthesis and activities in brain tissue (Bandegi et al., 2014; Chen et al., 2015; Mehri et al., 2015; Zhang et al., 2017a; Krishnaswamy et al., 2020; Yousefi et al., 2021), decreasing oxidized lipids and oxidative stress in PC-12 cells (Ochiai et al., 2004b; Soeda et al., 2007), and reducing hypoxia-induced cell damage (Javandoost et al., 2017; Bukhari et al., 2018; Ghaffari and Roshanravan, 2019). In human myoblast cells, 0.3 μM CR inhibited hydrogen peroxide (H2O2) induced toxicity accompanied by decreased ROS and increased antioxidant enzyme activity (Nassar et al., 2020). In addition, CR was shown to upregulate MMP-2 and MMP-9 protein expression which contributes to neuroprotection and maintenance of BBB integrity (Yang and Rosenberg, 2015; Zhang et al., 2017a). In particular, Vakili et al. (2014) showed that the anti-IS effect of CR was associated with increased SOD and glutathione peroxidase (GPx) activity and reduced MDA content in the ischemic cortex. Oruc et al. (2016) reported that CR-induced protection of cerebral I/R is associated with improved tissues oxidative index. CR was also shown to curb ROS in depression-induced mice (Xiao et al., 2019). In addition, a study indicates that the antioxidant effect of CR may contribute to its protection against organ damage in HS (Yang and Dong, 2017). Similarly, the antioxidant activity of CC and TSC have been demonstrated, including inhibition of ROS formation, improving antioxidant enzyme activities, and suppression of related mitochondrial apoptosis, and Aβ mechanisms (Hashemi and Hosseinzadeh, 2019; Shah et al., 2021). It has been reported that the free radical quenching activity of CR is weaker than SF, indicating that SF may contain other antioxidant constituents or has a synergistic effect among its ingredients (Asdaq and Inamdar, 2010). It was found that trans-crocin-4 exhibited more potent antioxidant activity than CC in the human brain cells, indicating the sugar molecules in CR may be important for its antioxidant activity and Aß fibril formation inhibition (Papandreou et al., 2006). In addition, SLT, a CR-containing formula was shown to reduce H2O2 related injury in EA hy926 cells (Seto et al., 2017). However, it is not clear if this effect of SLT relates to CR.
Cardiovascular Protection
Cardiovascular function is important for blood supply to the brain, thus the effect of CRs on cardiovascular functions may affect or contribute to their effects against IS. CR has demonstrated anti-ischemia effects in cardiac and vascular tissue. For example, CR (20 mg/kg) was shown to exhibit cardioprotective activity in I/R-related myocardial injury and decreased infarct size in ischemia the rats’ hearts by altering antioxidant status (Jahanbakhsh et al., 2012; Dianat et al., 2014). CR (100 mg/kg/day) attenuated cardiac inflammation and improved antioxidant capacity in female rats (Kocaman et al., 2021). Additionally, a study using cardiac ischemic rats showed CR at 20 mg/kg/day i.p. protected cardiac injury and increased SOD, MDA, and GSH antioxidant markers (Jahanbakhsh et al., 2012). Another study found that pre-treatment with CR followed by I/R (2 h hypoxia, 4 h reoxygenation) protected myocardial ischemic injury and regulated autophagy AMP-activated protein kinase (AMPK) mechanistic pathway (Zeng et al., 2016). Fan et al. (2021) showed CR protected myocardial mitochondria injury and acute myocardial infarction in rats (Leijiao Fan, 2021b). CR (15 and 30 mg/kg) significantly attenuated mitochondrial damage by increasing the membrane potential and reducing the mitochondrial permeability transition pore openness. The mechanism was associated with increased viability of mitochondrial respiratory enzymes, adenosine triphosphate (ATP), Na+-K+-ATP enzyme and Ca2+-ATP enzyme, and reduced mitochondrial Ca2+ concentration (Leijiao Fan, 2021b). Similar activities have been reported for CC and TCS, including cardioprotective effects against I/R injury, inhibiting myocardial infraction and cardiac hypertrophy, reducing blood pressure, and inhibiting platelet aggregation (Hashemi and Hosseinzadeh, 2019; Shah et al., 2021). For example, in a myocardial I/R rats model TSC (50 and 100 µg/kg) significantly alleviated I/R-induced cell injury via the SIRT3/FOXO3a/SOD2 signaling pathway (Chang et al., 2019). CR and related compounds have also been shown to possess vascular protective activity which may also contribute to their anti-IS actions. For example, CR improved vasodilation by acting on endogenous NO and endothelial NO synthase (Sai Li and ding, 2017) and reduced I/R injury in mice cerebral microvessels (Zheng et al., 2007). CC (25 and 50 mg/kg/day) was also shown to protect vascular function in stroke-prone spontaneously hypertensive rats (SHRSPs), with downregulated thrombogenesis, improved vasodilation, and endothelial function (elicits NO production), and antioxidant capacity (Higashino et al., 2014).
Mechanism of Actions of Crocin and Related Compounds
Potential Cellular Targets
CR has been shown to act on multiple brain cells including microglia, neurons, and astrocytes (Hosseinzadeh et al., 2014b; Zhu et al., 2019a). Table 2 shows the effects of CR and related compounds on different cells including microglia, endothelial cells and neurons (Table 2). For example, in syncytin-1-expressed primary human foetal astrocytes, CR (100, 200, and 400 µM) inhibited endoplasmic reticulum (ER) stress and nitric oxide synthase 2 and interferon gamma (IFN-α) expressions (Deslauriers et al., 2011). Although CR mitigated TNF-a release in astrocytes, microglia, and neurons after LPS stimulation (Gullo et al., 2017), the effect of CRs on astrocytes involving IS microvessels is still not clear. Astrocytes may play a critical role in neuroprotection during IS through their specialized functional and structural properties in the CNS (Liu and Chopp, 2016; Sun et al., 2019) including regulation of metabolic and homeostasis (Liddelow et al., 2017; Escartin et al., 2021). It is also necessary to investigate the effect of CRs on different phenotypes of astrocytes such as A1 (neuroinflammatory), A2 (neuroprotective), and A0 (nascent astrocytes).
Potential Molecular Targets
As mentioned above, CRs have therapeutic action on multiple pathways, related to cell signalingsignaling, transportations, energy production, and redox homeostasis (Hosseinzadeh et al., 2014a), in particular neuroinflammation, antioxidation, and apoptosis mechanisms (Table 2). For example, CR inhibited hypoxia-inducible factor-1α which is an important molecular target in IS (Oruc et al., 2016) (Table 2). The action of CR on mitochondrial apoptosis is also important for their neuroprotective effects against IS (Li et al., 2021; Yousefsani et al., 2021), including casp 3, casp 8, casp 9, B-cell lymphoma-2 (Bcl-2), and Bcl-2 Associated X-protein (Bax) (Table 2). The key ROS-related enzymes targeted by CR and associated compounds or analogues include SOD, reduced nicotinamide adenine dinucleotide phosphate (NADPH) oxidase, and GPx (Bandegi et al., 2014; Chen et al., 2015; Zhang et al., 2017b). In addition, it has been suggested that matrix MMPs activation plays a role in IS (Yang and Rosenberg, 2015) and amelioration of MMP-2 and MMP-9 expressions by CR may provide neuroprotection and protection of BBB functions under cerebral ischemia (Zhang et al., 2017b). The key inflammatory signaling molecules influenced by CR and related compounds include TNF-α, IL-1β, IL-1α, IL-6, IL-1, and MMP-9, monocyte chemoattractant protein-1 (MCP-1), macrophage inflammatory proten-1α (MIP-1α), vascular adhesion molecule-1 (VCAM-1), E-selectin and fractalkine (CX3CL1) and related signaling pathways namely NFκB, MAPK, TLR4, c-Jun N-terminal kinase (JNK), and P38 (Che et al., 2001; Hussein et al., 2019; Barthels and Das, 2020). In addition, autophagy is another important cellular mechanism involved in various cellular functions (Shen et al., 2021), and is a significant adaptive mechanism in IS (Ajoolabady et al., 2021). It has been suggested that activation of autophagy is involved in the protective effect of CR against IS (Zeng et al., 2016) CR enhanced autophagy by downregulating the LC3-II/I and upregulating the p62 and mTOR expression in IS model of HT22 cells (Huang et al., 2019). CC and TSC have shown with similar activities affecting cellular redox signaling and inflammatory pathways, as well as regulating autophagy, which contribute to their anti-IS and neuroprotective pharmacological actions (Tseng et al., 1995; Hashemi and Hosseinzadeh, 2019; Cerdá-Bernad et al., 2020; Shah et al., 2021; Wani et al., 2021).
Table 3 shows the IS-related human proteins potentially regulated by CR, based on the human metabolomic data retrieved from the standard HMDB Canadian Database System. We identified 11 proteins as the possible targets of CRs, including S100β, vascular endothelial growth factor (VEGF), glial fibrillary acidic protein (GFAP) and compliment component 1q (C1q). Since S100B is elevated in patients after IS (Lasek-Bal et al., 2019) and higher S100B levels were observed in the hospitalized IS patients (Shotar et al., 2019), S100B may be a potential diagnostic and therapeutic biomarker of IS. Intracellularly S100B can act as a regulator of Ca++ homeostasis (Donato et al., 2009). Extracellularly, it can activate inflammatory and other signaling pathways, such as the mitogenic Ras-MEK-ERK1/2-NF-κB pathway. SF has also been shown to regulate glial GFAP, VEGF, and C1q in MCAO animals (Yang et al., 2019; Abdel-Rahman et al., 2020; Zhong et al., 2020), although the exact role of CR and related compounds in these actions is not clear. Further studies are mandatory to elucidate the mechanisms involved in the actions of CRs against IS and related conditions in human (Karkoula et al., 2020).
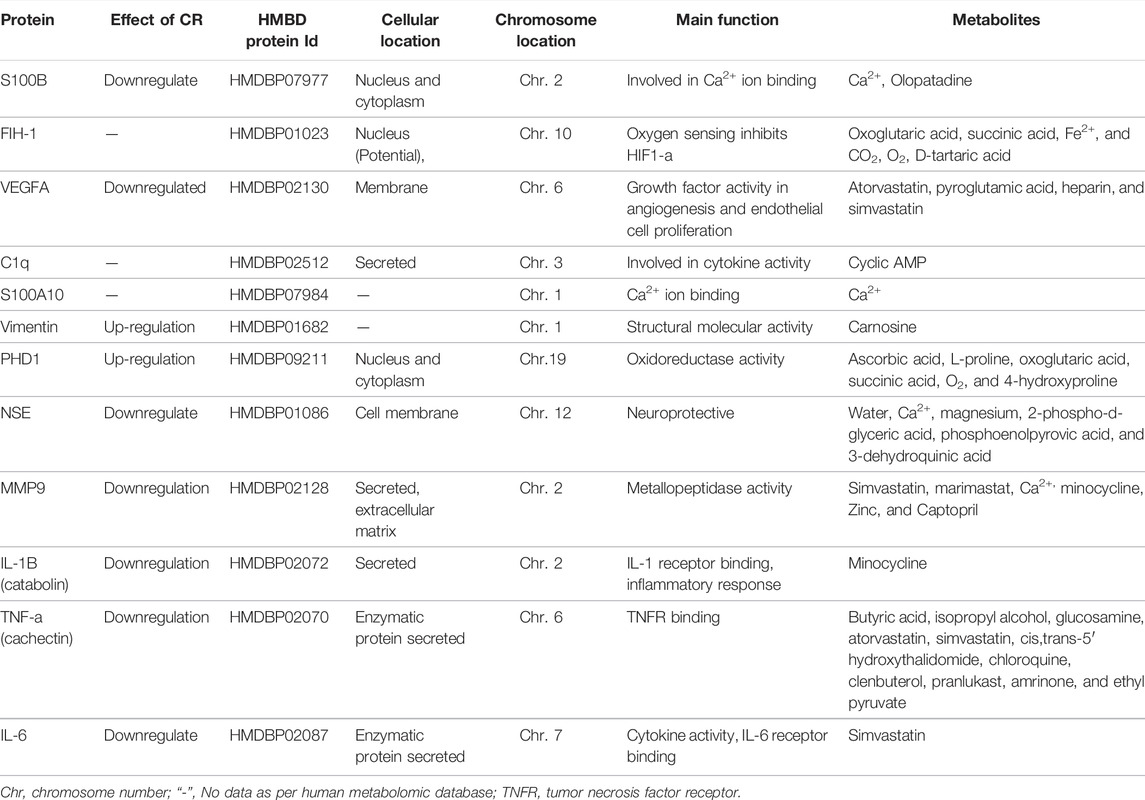
TABLE 3. IS-related human proteins targeted by CR, based on the metabolomic data by CRs (retrieved from the HMDB Canadian Database System).
Pharmacokinetics of Crocins
The pharmacokinetics of CR and related compounds have been reviewed (Xi and Qian, 2006; Khorasany and Hosseinzadeh, 2016; Hosseini et al., 2018; Hashemi and Hosseinzadeh, 2019; Veisi et al., 2020; Song et al., 2021a; Shah et al., 2021).
Asai et al. (2005) studied the absorption of CR and CC in mice and showed that neither CR nor CR2 were detectable in the plasma after the oral administration of a mixed micelle solution containing CC or CRs, whereas CC was rapidly absorbed into the blood and detected in plasma as free form and glucuronide conjugates, indicating the metabolism of CRs mainly involves glucuronidation in the intestine and liver. Another study confirmed that CM (CR metabolite) was detected in blood and brain after oral administration of CR (Zhang et al., 2012). Xi et al. (2007) studied the absorption of CR and CC in rats after single or repeated oral doses (40 mg/kg by oral gavage), and found that CR was not detectable, while CC was present in the plasma at low concentrations. They also demonstrated that CR was excreted through the intestinal tract following oral administration, indicating the intestinal tract may serve as a site for CR via hydrolysis. Another study in stroke-prone spontaneously hypertensive rats found a high level of CC in plasma and brain after oral administration of 100 mg/kg CC (Yoshino et al., 2011). The elimination half-life (t1/2k) after an oral dose of CR (1 mg/kg) was reported as 3.0 ± 0.6 h (Zhang et al., 2012). Studies in humans confirm that CC is rapidly absorbed after oral administration (Hosseini et al., 2018). In healthy adult volunteers, it was reported that the peak plasm (Cmax) level of CC after receiving 7.5, 15, and 22.5 mg doses was 100.9–279.7 ng/ml, and the mean time to reach maximum concentration (Tmax) was 4–4.8 h, AUC0–24 h ranged from 556.5 to 1720.8 ng h/ml and the mean elimination half-life (T1/2) was 6.1–7.5 h (Umigai et al., 2011). The pharmacokinetics of CC after oral and IV administrations is consistent with a two-compartment model (Xi and Qian, 2006).
There is evidence that CR could not penetrate Caco-2 monolayers, while trans-crocetin permeated the intestinal barrier (Lautenschläger et al., 2015). It has been suggested that CRs are hydrolyzed in the intestine by intestinal cells to the deglycosylated trans-crocetin, which is subsequently absorbed by passive transcellular diffusion (Lautenschläger et al., 2015; Karkoula et al., 2018; Shah et al., 2021), most likely via gut microbiota mediated biotransformation as mentioned above (Zhang et al., 2019). This mechanism may be important for CRs to exert their anti-IS and neuroprotective actions in the brain, as CC has been shown to be able to permeate BBB and accumulate in the brain (Lautenschläger et al., 2015; Karkoula et al., 2018). On the other hand, some CRs such as trans-crocin 4 were shown to be able to across BBB in mice after ip administration despite its highly hydrophilic character (Lautenschläger et al., 2015; Karkoula et al., 2018). Studies showed that CC can be rapidly distributed into different tissues, including the liver and kidneys, partly due to its weak binding to plasma albumin (Xi and Qian, 2006; Hosseini et al., 2018; Christodoulou et al., 2019; Hashemi and Hosseinzadeh, 2019; Shah et al., 2021). A study on rats by Zhang et al., showed that T1/2 was estimated as 2.5–2.9 h after oral administration of different doses of CC (Zhang et al., 2017a). CR is excreted primarily through the intestinal tract in feces after oral administration (40 mg/kg), with 59.507% ± 13.56% excreted (Asai et al., 2005), and via urine after IV administration with a cumulative excretion fraction of 67.17% ± 4.79% within 48 h (Zhang et al., 2019).
Nanoparticle Formulation and Green Synthesis
Nanoscale drug delivery is an important tool to improve the pharmacokinetics and bioavailability of drugs and natural drugs (Puglia et al., 2010). Nanoparticle (NP)-based drug delivery systems have shown advantages in improvised bioavailability, bioadhesion, and controlled drug release in the gastrointestinal (GI) tract (Kim et al., 2006; Dudhani and Kosaraju, 2010). For example, some chitosan-alginate biofilm forming agents have been used for pH-sensitive nanomolecular formulations to control drug movement across the GI tract (George and Abraham, 2006). Thus, NP-based formulations can be used to enhance the bioavailability and activity of CRs and CC (Shah et al., 2021). A nano-encapsulated formulation of chitosan alginate biofilm-forming agents has been shown with improved CR stability and bioavailability, and controlled release (Rahaiee et al., 2015) (Mirhadi et al., 2020). CR chitosan-alginate NPs also showed improved antioxidant and anticancer activities in vivo, suggesting potential therapeutic applications for these preparations (Rahaiee et al., 2017). Another recent study explored an NP-CR formulation with dextran/chitosan sulphate (DS/CH) coated NPs loaded with CR, and demonstrated its activity in downregulating VEGF and AB142 levels accompanied by a stronger antioxidant capacity in SHSY5Y cells (Song et al., 2022). In addition, a water-soluble crocetin-γ-cyclodextrin formulation significantly increased the bioavailability of CC and facilitated it crossing the BBB to enter the brain (Wong et al., 2020). Furthermore, CR-NPs have been formulated with polymeric carriers to improve the stability of CR (Mirhadi et al., 2020).
Green synthesis is an advanced method that uses natural reducing agents, plugs, and stabilizers surpassing the employment of toxic and expensive chemicals and high energy costs (Hussain et al., 2016). There is an increasing need for optimal eco-friendly and non-toxic methods of developing NPs such as gold NPs (AuNPs) preparation with CR (Hoshyar et al., 2016) and SF (Abootorabi et al., 2016). Solid lipid nanoparticles belong to the lipid nanotransporter family that can solubilize hydrophilic and lipophilic molecules in physiological environments. This is controlling their release and protects them from degradation (Tapeinos et al., 2017). CC and CR solid lipid nanoparticles can be prepared using Softisan 100 (hydrogenated coco glyceride) and Pluronic F68 (Poloxamer 188) with these lipid substrates commonly regarded as the best with low melting point (35°C), which is an important property related to the stability of NPs (Puglia et al., 2019). These nanoparticles have been tested in cancer cell lines and showed a prolonged antioxidant activity, and better antitumor cytotoxicity than free CR (Puglia et al., 2019).
Clinical Evidence
A number of clinical studies on SF and CR have been conducted, involving healthy subjects or patients with various conditions such as metabolic syndrome (Kermani et al., 2017), depression, and coronary artery disease (CAD) (Abedimanesh et al., 2017). Table 4 summarizes the clinical studies on SF and CR. For example, a study with SF extract capsules (200 mg/day) showed that it was effective against IS with a long term (up to 3 months) neuroprotective effect, based on the National Institute of Health Stroke Scale (NIHSS), with improved Barthel index and brain-derived neurotrophic factor (BDNF) levels, and decreased stroke severity with lowered levels of serum neuron-specific enolase (NSE) and S100 (Asadollahi et al., 2019). A similar finding was obtained in an RCT involving 40 patients with acute IS, and revealed that SF at 400 mg/day decreased the severity of stroke as assessed by the NIHSS score, with an improved MDA level (Gudarzi et al., 2020). Other studies demonstrated the effectiveness of SF, in combination with Ritalin, for patients with attention deficit hyperactivity disorder (ADHD) (Pazoki et al., 2022), or CAD with a significant inhibition of circulating MCP-1 (Abedimanesh et al., 2020). In addition, a study showed that 30 mg of SF supplement for 16 weeks improved cognition function (change in both AD Scale-cognitive subscale (ADAS-cog) and clinical dementia ratings-scale sums of boxes (CDR-SB) in patients with mild to moderate Alzheimer’s disease (Akhondzadeh et al., 2010). This is supported by a recent systematic review of five RCTs involving 325 subjects on AD and mild cognitive impairment, suggesting that SF may be as efficacious as common drugs against AD, although it should be taken with caution as there may be an unknown or high risk of bias due to the low quality of some of the studies included (Avgerinos et al., 2020). On the other hand, a recent trial involving 50 patients with type 2 diabetes (T2D) confirmed a significant improvement in glycaemic control and insulin resistance after administration of 15 mg CR twice daily for up to 12 weeks (Behrouz et al., 2020). Furthermore, a double-blind RCT involving 62 participants with mild erectile dysfunction (ED) showed that administering 15 mg SF twice a day improved erectile function without obvious side effects, indicating it may be effective against ED, especially in patients reluctant to accept the prescription of phosphodiesterase type 5 inhibitors (Najafabadi et al., 2022). A small trial (40 patients) on depression compared the effect of SF (30 mg/day) and fluoxetine (40 mg/day) and found no significant difference between the two groups in reduction of Hamilton depression rating scale, and the frequency of adverse events, indicating SF has similar antidepressant activity as fluoxetine, although further research with larger sample size is needed (Shahmansouri et al., 2014). Currently, there is no published RCT on CR or related compounds for treating IS. Zhu et al. (2010) described earlier a study using a CR injection (40 and 80 mg/day for 2 weeks) treating 60 patients with thrombotic cerebral infarction, and showed a significant improvement in symptoms, with an overall effective rate of 91% and 80%, respectively, and without obvious side effects. Given that CR has been demonstrated with efficacy in various conditions (Table 4), further clinical trials on the effect of CR on IS are mandatory. On the other hand, TSC has been studied as a synthetic carotenoid drug to enhance oxygenation of hypoxic tissue in addition to the standard of care, including Covid-19 and a Phase 2 trial on efficacy and safety for suspected stroke. However, the stroke trial was terminated due to the COVID-19 pandemic, according to the information posted on Clinicaltrials.gov (Zhu, 2010; Shah et al., 2021; Streinu-Cercel et al., 2021).
Toxicity and Safety of Saffron, Crocin, and Related Compounds
The toxicity of SF has been well studied (Alavizadeh and Hosseinzadeh, 2014b; Abu-Izneid et al., 2020) with a lethal dose (LD50) ranging from 1 to 5 g/kg, indicating it is mildly toxic compared to nontoxic compounds (LD50 > 5 g/kg) (Abu-Izneid et al., 2020). A recent study on the acute toxicity of orally administrated SF showed its LD50 as 4.12 ± 0.55 g/kg in mice (Gupta and Pandey, 2020). SF at the therapeutic doses (30–60 mg/day) has been shown with certain side effects including hypomania, sedation, nausea, mild headache and anxiety, dry mouth, dizziness, vomiting, and fatigue after 6 or 22 weeks (Akhondzadeh et al., 2005; Akhondzadeh et al., 2010). In general, SF at 1.5 g/day has been considered to be safe with no significant adverse drug effects (Ghaffari and Roshanravan, 2019). At a high dosage of 10 g/day in humans, SF manifested abortion or life-threatening complications (Mollazadeh et al., 2015), such as temporary paralysis after hallucination and abortion accompanied by maternal morbidity (Moghaddasi, 2010; Ghorbani and Koocheki, 2017). In comparison, the LD50 for CR could not be obtained as it did not cause mortality in mice after i.p. administration at 0.5–3 g/kg (Hosseinzadeh et al., 2010). An early study found that a high dose of CR (100 mg/kg for 2 weeks) caused liver injury and black pigmentation in rats (Wang et al., 1984). However, a recent comprehensive study on the acute and subacute toxicity of CR (up to 3 g/kg po and i.p.) in rodent found it did not cause damage to major organs (Hosseinzadeh et al., 2010). Another study found that CR at doses of 50,100, and 200 mg/kg (once a week for 4 weeks, i.p.) in rats did not cause significant changes in liver enzymatic profile and non-pathological tissue changes (Hariri et al., 2010). Rats fed with 1% CR for 4 months showed a reversible pigmentation (Wang et al., 1984; Imran et al., 2019). These findings indicate that CR is generally safe and well-tolerated. However, CR at 200 and 600 mg/kg i.p. was found to affect skeleton formation in pregnant mice indicating it may have developmental toxicity at very high doses (Moallem et al., 2016). On the other hand, CC was reported with a teratogenic effect at high concentration (200 µM) in frog (Xenopus) embryos (Martin et al., 2002), although no genotoxicity was observed for CC in V79 Chinese hamster cells (Ozaki et al., 2002), and no retinal toxicity was observed for CC in rabbit eyes (Wang et al., 2019). CR and CC have been well demonstrated for their cytotoxic activity against several cancer cells (Hoshyar and Mollaei, 2017; Hashemi and Hosseinzadeh, 2019; Veisi et al., 2020).
The clinical safety of CR has been demonstrated in several human trials (Ahmed et al., 2020). In a randomized double-blind placebo-controlled trial in healthy volunteers (n = 42), CR at 20 mg/day administrated for 1 month showed a safe profile of CR, with only minor adverse reactions in conjunction with decreased partial thromboplastin time, amylase, and mixed monocytes, basophils, and eosinophils (Mohamadpour et al., 2013). Another double-blind placebo-controlled study in schizophrenic patients (n = 22) found that CR tablet (15 mg/twice a day) caused no side effects or significant changes in liver, kidney, and thyroid markers and hepatological components (Mousavi et al., 2015). A randomized trial on diabetic maculopathy diagnosed 60 patients, and showed that CR tablets (5 or 15 mg/day for 3 months) caused some minor adverse reactions including polyphagia (4 patients), foot swelling (2 patients ), burning of eyes (3 patient), red-eye (2 patients), subconjunctival hemorrhage (5 patients), eye swelling (3 patients ) and stomach ache (1 patient) (Sepahi et al., 2018). Another clinical trial on CR as an adjunct therapy to methadone against opioid withdrawal in 50 patients found that CR at 15 mg twice a day for 8 weeks caused some minor side effects including headache, insomnia, nausea, and dyspnoea (Khalatbari-mohseni et al., 2019). Similarly, CC at 7.5 mg/day for 14 days in a randomized, double-blind, placebo-controlled, crossover trial on sleep quality was found to improve subjective sleep quality and no obvious adverse events linked to CC intake were observed, indicating that CC at this dose and treatment protocol is safe (Umigai et al., 2011). A 12-week RCT involving 32 healthy adult volunteers showed that CC (7.5 mg/day) had no significant adverse effects (Yamashita et al., 2018) while increased delta power and enhanced the refreshing feeling while waking up (Umigai et al., 2018). Overall, CR and CC at common therapeutic doses are generally safe in humans. Animal toxicology studies and phase I clinical trials had been conducted for TSC and showed it was well tolerated and safe in humans, although no published data are available (Gainer, 2008). Nevertheless, Mohler III et al. (2011) showed that TSC at a dose range (0.25 to 2.0 mg/kg, IV, once daily for 5 days) was safe and well-tolerated in patients with peripheral artery disease (PAD).
Further remarks and Conclusions
Significant progress has been made recently in understanding the actions of CR and related compounds on IS-related conditions and the mechanisms involved. The current pre-clinical and clinical evidence indicates a great potential of CR, CC, and TSC to treat IS and related conditions. However, these studies have some limitations and there are still gaps that curb the clinical translation of research findings. For example, although there is strong pre-clinical evidence for the beneficial actions of CR against IS, the current clinical trials on CR and related compounds are limited to other conditions, and specific clinical trials on IS are still lacking. In addition, many published studies are with small sample sizes and some are subject to potential bias, thus, high-quality clinical studies are needed to further confirm their efficacy and safety. It is also important to establish the proper treatment protocol and compare the effects of these compounds under short-term and long-term treatments. More research is needed to improve the bioavailability of CR, including developing formulations or delivery systems to maximize their efficacy and safety, with proper dosage regimen and route of administration. Further research is also needed to elucidate the mechanism(s) involved in the actions of CR and related compounds, including transformation and site of actions, molecular targets, and sensitive markers. Among these, the synergy of these compounds with other drugs or ingredients needs to be explored. For example, it has been shown that TSC, given in combination with rtPA either before or after embolization, improved the treatment outcomes in experimental acute IS (Lapchak, 2010). CR has also been shown with a synergistic effect with zinc sulfate to reduce hepatic I/R injury in Rats (Mard et al., 2017). Further research in this area will warrant the potential therapeutic value of these compounds not only as drug candidates by themselves but also as a complementary therapy with existing therapies and other drugs available. Finally, current studies have focused on CR and CC, more research on other forms of CRs is needed.
In conclusion CRs, most importantly CR, are key active compounds of C. sativus L. (SF). These compounds have been demonstrated with beneficial pharmacological actions in preventing or reducing IS-induced injury via multiple mechanisms including neuroprotective, antioxidant, anti-inflammation, and other cerebral protective activities. CR can act on various cellular and molecular mechanisms related to IS in particular neuroinflammatory mitochondrial signaling pathways, HIF1a, VEGF, and cytokines. CR has low bioavailability and its conversion to CC by gut microbiota may be important in mediating its therapeutic effect. Toxicological and clinical studies indicate that CR is generally safe in humans. The current evidence indicates that CR and related products may have potential as stand-alone or adjuvant therapy for treating IS, although further confirming clinical studies are needed. The elucidation of CRs molecular targets and synergistic mechanisms with other drugs or ingredients may help translate preclinical findings into novel therapies for the intervention, management, and prognosis of IS and related conditions.
Author Contributions
CL and KS conceptualised the review. KS and CL drafted and revised the manuscript. DC, XZ, ML, and SS contributed to the revision of the manuscript. KS, CL, and XZ contributed to reference collection. KS drew all chemical structures and figures. All authors approved the submitted version.
Funding
This work was supported by Western Sydney University (WSU) Research Training Scheme. KS was supported by a WSU Postgraduate Research Scholarship.
Conflict of Interest
The authors declare that the research was conducted in the absence of any commercial or financial relationships that could be construed as a potential conflict of interest.
Publisher’s Note
All claims expressed in this article are solely those of the authors and do not necessarily represent those of their affiliated organizations, or those of the publisher, the editors, and the reviewers. Any product that may be evaluated in this article, or claim that may be made by its manufacturer, is not guaranteed or endorsed by the publisher.
References
Abdel-rahman, R. F., El awdan, S. A., Hegazy, R. R., Mansour, D. F., Ogaly, H. A., and Abdelbaset, M. (2020). Neuroprotective Effect of Crocus Sativus against Cerebral Ischemia in Rats. Metab. Brain Dis. 35, 427–439. doi:10.1007/s11011-019-00505-1
Abe, K., and Saito, H. (2000). Effects of Saffron Extract and its Constituent Crocin on Learning Behaviour and Long-Term Potentiation. Phytother. Res. 14, 149–152. doi:10.1002/(sici)1099-1573(200005)14:3<149::aid-ptr665>3.0.co;2-5
Abedimanesh, N., Motlagh, B., Abedimanesh, S., Bathaie, S. Z., Separham, A., and Ostadrahimi, A. (2020). Effects of Crocin and Saffron Aqueous Extract on Gene Expression of SIRT1, AMPK, LOX1, NF-Κb, and MCP-1 in Patients with Coronary Artery Disease: A Randomized Placebo-Controlled Clinical Trial. Phytother. Res. 34, 1114–1122. doi:10.1002/ptr.6580
Abedimanesh, N., Ostadrahimi, A., Bathaie, S. Z., Abedimanesh, S., Motlagh, B., Jafarabadi, M. A., et al. (2017). Effects of Saffron Aqueous Extract and its Main Constituent, Crocin, on Health-Related Quality of Life, Depression, and Sexual Desire in Coronary Artery Disease Patients: A Double-Blind, Placebo-Controlled, Randomized Clinical Trial. Iran. Red Crescent Med. J. 19. doi:10.5812/ircmj.13676
Abootorabi, Z., Poorgholami, M., Hanafi-Bojd, M. Y., and Hoshyar, R. (2016). Green Synthesis of Gold Nanoparticles Using Barberry and Saffron Extracts. Mod. Care J. 13, e13000. doi:10.5812/modernc.13000
Abu-Izneid, T., Rauf, A., Khalil, A. A., Olatunde, A., Khalid, A., Alhumaydhi, F. A., et al. (2020). Nutritional and Health Beneficial Properties of Saffron (Crocus Sativus L): A Comprehensive Review. Crit. Rev. food Sci. Nutr., 1–24.
Adobe inc (2019). Adobe Illustrator. [Online]. Available at: https://adobe.com/products/illustrator. [accessed].
Ahmed, S., Hasan, M. M., Heydari, M., Rauf, A., Bawazeer, S., Abu-Izneid, T., et al. (2020). Therapeutic Potentials of Crocin in Medication of Neurological Disorders. Food Chem. Toxicol. 145, 111739. doi:10.1016/j.fct.2020.111739
Ajoolabady, A., Wang, S., Kroemer, G., Penninger, J. M., Uversky, V. N., Pratico, D., et al. (2021). Targeting Autophagy in Ischemic Stroke: from Molecular Mechanisms to Clinical Therapeutics. Pharmacol. Ther. 225, 107848. doi:10.1016/j.pharmthera.2021.107848
Akbari, G., Ali Mard, S., and Veisi, A. (2018). A Comprehensive Review on Regulatory Effects of Crocin on Ischemia/reperfusion Injury in Multiple Organs. Biomed. Pharmacother. 99, 664–670. doi:10.1016/j.biopha.2018.01.113
Akhondzadeh, S., Sabet, M. S., Harirchian, M. H., Togha, M., Cheraghmakani, H., Razeghi, S., et al. (2010). Saffron in the Treatment of Patients with Mild to Moderate Alzheimer’s Disease: A 16‐week, Randomized and Placebo‐Controlled Trial. J. Clin. Pharm. Ther. 35, 581–588. doi:10.1111/j.1365-2710.2009.01133.x
Akhondzadeh, S., Tahmacebi‐Pour, N., Noorbala, A. A., Amini, H., Fallah‐Pour, H., Jamshidi, A. H., et al. (2005). Crocus Sativus L. In the Treatment of Mild to Moderate Depression: A Double‐blind, Randomized and Placebo‐controlled Trial. Phytotherapy Res. Int. J. Devoted Pharmacol. Toxicol. Eval. Nat. Prod. Deriv. 19, 148–151. doi:10.1002/ptr.1647
Akhtari, K., Hassanzadeh, K., Fakhraei, B., Fakhraei, N., Hassanzadeh, H., and Zarei, S. A. (2013). A Density Functional Theory Study of the Reactivity Descriptors and Antioxidant Behavior of Crocin. Comput. Theor. Chem. 1013, 123–129. doi:10.1016/j.comptc.2013.03.015
Alakbarzade, V., and Pereira, A. C. (2020). What Proportion of Patients Admitted with Stroke or Transient Ischemic Attack May Be Suitable for Newer Cholesterol-Lowering Treatment? J. Stroke Cerebrovasc. Dis. 29, 104457. doi:10.1016/j.jstrokecerebrovasdis.2019.104457
Alavizadeh, S. H., and Hosseinzadeh, H. (2014a). Bioactivity Assessment and Toxicity of Crocin: A Comprehensive Review. Food Chem. Toxicol. 64, 65–80. doi:10.1016/j.fct.2013.11.016
Alavizadeh, S. H., and Hosseinzadeh, H. (2014b). Bioactivity Assessment and Toxicity of Crocin: A Comprehensive Review. Food Chem. Toxicol. 64, 65–80. doi:10.1016/j.fct.2013.11.016
Altinzo, E., Oner, Z., Elbe, H., and Vardi, N. (2014). Neuro-Protective Effects of Crocin on Brain and Cerebellum Tissues in Diabetic Rats. Afr. J. Traditional, Complementary Altern. Med. 11, 33–39.
Ama (2021). Global Burden of Disease-2021statistical Fact Sheet. American Heart Association. [Online] (Accessed 08 11, 21).
An, B., Ma, Y., Xu, Y., Liu, X., Zhang, X., Zhang, J., et al. (2020). Crocin Regulates the Proliferation and Migration of Neural Stem Cells After Cerebral Ischemia by Activating the Notch1 Pathway. Folia Neuropathol. 58 (3), 201–212.
Asadollahi, M., Nikdokht, P., Hatef, B., Sadr, S. S., Sahraei, H., Assarzadegan, F., et al. (2019). Protective Properties of the Aqueous Extract of Saffron (Crocus Sativus l.) in Ischemic Stroke, Randomized Clinical Trial. J. Ethnopharmacol. 238, 111833. doi:10.1016/j.jep.2019.111833
Asai, A., Nakano, T., Takahashi, M., and Nagao, A. (2005). Orally Administered Crocetin and Crocins Are Absorbed into Blood Plasma as Crocetin and its Glucuronide Conjugates in Mice. J. Agric. Food Chem. 53, 7302–7306. doi:10.1021/jf0509355
Asdaq, S. M. B., and Inamdar, M. N. (2010). Potential of Crocus Sativus (Saffron) and its Constituent, Crocin, as Hypolipidemic and Antioxidant in Rats. Appl. Biochem. Biotechnol. 162, 358–372. doi:10.1007/s12010-009-8740-7
Avgerinos, K. I., Vrysis, C., Chaitidis, N., Kolotsiou, K., Myserlis, P. G., and Kapogiannis, D. (2020). Effects of Saffron (Crocus Sativus l.) on Cognitive Function. A Systematic Review of Rcts. Neurol. Sci. 41, 2747–2754. doi:10.1007/s10072-020-04427-0
Awasthi, R., and Kulkarni, G. (2020). Therapeutic Benefits of Saffron in Brain Diseases: New Lights on Possible Pharmacological Mechanisms. Saffron Age-Old Panacea a New Light, 117.
Ayatollahi, H., Javan, A. O., Khajedaluee, M., Shahroodian, M., and Hosseinzadeh, H. (2014). Effect of Crocus Sativus l. (Saffron) on Coagulation and Anticoagulation Systems in Healthy Volunteers. Phytotherapy Res. 28, 539–543. doi:10.1002/ptr.5021
Azami, S., Shahriari, Z., Asgharzade, S., Farkhondeh, T., Sadeghi, M., Ahmadi, F., et al. (2021). Therapeutic Potential of Saffron (Crocus Sativus l.) in Ischemia Stroke. In Evidence-based Complementary and Alternative Medicine. doi:10.1155/2021/6643950
Azmand, M. J., and Rajaei, Z. (2021). Effects of Crocin on Spatial or Aversive Learning and Memory Impairments Induced by Lipopolysaccharide in Rats. Avicenna J. Phytomedicine 11, 79.
Badavi, M., Mard, S. A., Dianat, M., and Dashtbozorgi, N. (2020). Crocin Attenuates Oxidative Stress and Inflammation in Myocardial Infarction Induced by Isoprenaline via Pparγ Activation in Diabetic Rats. J. Diabetes & Metabolic Disord. 19, 1517–1525. doi:10.1007/s40200-020-00686-y
Bahr-Hosseini, M., Bikson, M., Iacoboni, M., Liebeskind, D. S., Hinman, J. D., Carmichael, S. T., et al. (2021). Primed2 Preclinical Evidence Scoring Tool to Assess Readiness for Translation of Neuroprotection Therapies. Transl. Stroke Res., 1–6. doi:10.1007/s12975-021-00922-4
Bakshi, H. A., Hakkim, F. L., Sam, S., Javid, F., and Rashan, L. (2018). Dietary Crocin Reverses Melanoma Metastasis. J. Biomed. Res. 32, 39.
Bandegi, A. R., Rashidy-Pour, A., Vafaei, A. A., and Ghadrdoost, B. (2014). Protective Effects of Crocus Sativus L. Extract and Crocin against Chronic-Stress Induced Oxidative Damage of Brain, Liver and Kidneys in Rats. Adv. Pharm. Bull. 4, 493. doi:10.5681/apb.2014.073
Barthels, D., and Das, H. (2020). Current Advances in Ischemic Stroke Research and Therapies. Biochimica Biophysica Acta (bba)-Molecular Basis Dis. 1866, 165260. doi:10.1016/j.bbadis.2018.09.012
Batarseh, Y. S., Bharate, S. S., Kumar, V., Kumar, A., Vishwakarma, R. A., Bharate, S. B., et al. (2017). Crocus Sativus Extract Tightens the Blood-Brain Barrier, Reduces Amyloid β Load and Related Toxicity in 5xfad Mice. Acs Chem. Neurosci. 8, 1756–1766. doi:10.1021/acschemneuro.7b00101
Becerra-Calixto, A., and Cardona-Gómez, G. P. (2017). The Role of Astrocytes in Neuroprotection after Brain Stroke: Potential in Cell Therapy. Front. Mol. Neurosci. 10, 88. doi:10.3389/fnmol.2017.00088
Behrouz, V., Dastkhosh, A., Hedayati, M., Sedaghat, M., Sharafkhah, M., and Sohrab, G. (2020). The Effect of Crocin Supplementation on Glycemic Control, Insulin Resistance and Active Ampk Levels in Patients with Type 2 Diabetes: A Pilot Study. Diabetology Metabolic Syndrome 12, 1–9. doi:10.1186/s13098-020-00568-6
Bei, W., Peng, W., Ma, Y., and Xu, A. (2004). Naoxinqing, an Anti-stroke Herbal Medicine, Reduces Hydrogen Peroxide-Induced Injury in Ng108-15 Cells. Neurosci. Lett. 363, 262–265. doi:10.1016/j.neulet.2004.04.031
Bei, W., Zang, L., Guo, J., Peng, W., Xu, A., Good, D. A., et al. (2009). Neuroprotective Effects of a Standardized Flavonoid Extract from Diospyros Kaki Leaves. J. Ethnopharmacol. 126, 134–142. doi:10.1016/j.jep.2009.07.034
Bin Wen, Q. Z., Jin, L., Kang, L., and Yang, Y. (2020). Protective Effect of Crocin Pretreatment on Global Cerebral Ischemia Reperfusion Injury in Rats. Drug Eval. Res. 43, 429–435.
Bukhari, S. I., Manzoor, M., and Dhar, M. (2018). A Comprehensive Review of the Pharmacological Potential of Crocus Sativus and its Bioactive Apocarotenoids. Biomed. Pharmacother. 98, 733–745. doi:10.1016/j.biopha.2017.12.090
Cao, H., Seto, S. W., Bhuyan, D. J., Chan, H. H., and Song, W. (2021). Effects of Thrombin on the Neurovascular Unit in Cerebral Ischemia. Cell. Mol. Neurobiol. doi:10.1007/s10571-020-01019-6
Carmona, M., Zalacain, A., Sánchez, A. M., Novella, J. L., and Alonso, G. L. (2006). Crocetin Esters, Picrocrocin and its Related Compounds Present in Crocus Sativus Stigmas and Gardenia Jasminoides Fruits. Tentative Identification of Seven New Compounds by Lc-Esi-Ms. J. Agric. Food Chem. 54, 973–979. doi:10.1021/jf052297w
Cerdá-Bernad, D., Valero-Cases, E., Pastor, J.-J., and Frutos, M. J. (2020). Saffron Bioactives Crocin, Crocetin and Safranal: Effect on Oxidative Stress and Mechanisms of Action. Crit. Rev. Food Sci. Nutr., 1–18.
Chang, D., Colagiuri, B., and Luo, R. (2013). 10 Chinese Medicine Used. In Advances in Natural Medicines. Boca Raton, Fla: Nutraceuticals and Neurocognition, 205.
Chang, D., Liu, J., Bilinski, K., Xu, L., Steiner, G. Z., Seto, S. W., et al. (2016). Herbal Medicine for the Treatment of Vascular Dementia: An Overview of Scientific Evidence-Based Complementary and Alternative Medicine, 2016.
Chang, G., Chen, Y., Zhang, H., and Zhou, W. (2019). Trans Sodium Crocetinate Alleviates Ischemia/reperfusion-Induced Myocardial Oxidative Stress and Apoptosis via the Sirt3/foxo3a/sod2 Signaling Pathway. Int. Immunopharmacol. 71, 361–371. doi:10.1016/j.intimp.2019.03.056
Chapman, S. N., Mehndiratta, P., Johansen, M. C., Mcmurry, T. L., Johnston, K. C., and Southerland, A. M. (2014). Current Perspectives on the Use of Intravenous Recombinant Tissue Plasminogen Activator (Tpa) for Treatment of Acute Ischemic Stroke. Vasc. Health Risk Manag. 10, 75. doi:10.2147/VHRM.S39213
Che, X., Ye, W., Panga, L., Wu, D.-C., and Yang, G.-Y. (2001). Monocyte Chemoattractant Protein-1 Expressed in Neurons and Astrocytes during Focal Ischemia in Mice. Brain Res. 902, 171–177. doi:10.1016/s0006-8993(01)02328-9
Chen, J., Zhang, X., Liu, X., Zhang, C., Shang, W., Xue, J., et al. (2019). Ginsenoside Rg1 Promotes Cerebral Angiogenesis via the Pi3k/akt/mtor Signaling Pathway in Ischemic Mice. Eur. J. Pharmacol. 856, 172418. doi:10.1016/j.ejphar.2019.172418
Chen, L., Qi, Y., and Yang, X. (2015). Neuroprotective Effects of Crocin against Oxidative Stress Induced by Ischemia/reperfusion Injury in Rat Retina. Ophthalmic Res. 54, 157–168. doi:10.1159/000439026
Chen, Y., Zhang, H., Tian, X., Zhao, C., Cai, L., Liu, Y., et al. (2008). Antioxidant Potential of Crocins and Ethanol Extracts of Gardenia Jasminoides Ellis and Crocus Sativus l.: A Relationship Investigation between Antioxidant Activity and Crocin Contents. Food Chem. 109, 484–492. doi:10.1016/j.foodchem.2007.09.080
Christodoulou, E., Grafakou, M.-E., Skaltsa, E., Kadoglou, N., Kostomitsopoulos, N., and Valsami, G. (2019). Preparation, Chemical Characterization and Determination of Crocetin's Pharmacokinetics after Oral and Intravenous Administration of Saffron (Crocus Sativus l.) Aqueous Extract to C57/bl6j Mice. J. Pharm. Pharmacol. 71, 753–764. doi:10.1111/jphp.13055
Cichoń, N., Bijak, M., Miller, E., and Saluk, J. (2017). Extremely Low Frequency Electromagnetic Field (Elf‐emf) Reduces Oxidative Stress and Improves Functional and Psychological Status in Ischemic Stroke Patients. Bioelectromagnetics 38, 386–396. doi:10.1002/bem.22055
Crocus-sativus (2010). Iridaceae of North America Update. Database (Version 2010), Updated for Itis by the Fora of North America Expertise Network, in Connection with an Update for Usda Plants (2007-2010) itis.gov.
Csupor, D., Tóth, B., Mottaghipisheh, J., Zangara, A., and Al-Dujaili, E. A. S. (2021). “Chapter 15 - The Psychopharmacology of Saffron, a Plant with Putative Antidepressant and Neuroprotective Properties,” in Nutraceuticals in Brain Health and Beyond. Editor d. ghosh (Academic Press).
Cui, C., Dong, S., Chen, N., Bao, J., and He, L. (2020). Low-Dose Statin Pretreatment Improves Function and Prognosis of Recurrent Ischemic Stroke Patients. Ther. Adv. Neurological Disord. 13, 1756286420920078. doi:10.1177/1756286420920078
Deng, J., Xiong, L., and Zuo, Z. (2015). Trans‐Sodium Crocetinate Provides Neuroprotection Against Cerebral Ischemia and Reperfusion in Obese Mice. J. Neurosci. Res. 93, 615–622. doi:10.1002/jnr.23522
Deslauriers, A. M., Afkhami-Goli, A., Paul, A. M., Bhat, R. K., Acharjee, S., Ellestad, K. K., et al. (2011). Neuroinflammation and Endoplasmic Reticulum Stress Are Coregulated by Crocin to Prevent Demyelination and Neurodegeneration. J. Immunol. 187, 4788–4799. doi:10.4049/jimmunol.1004111
Dhar, A., and Mir, G. (1997). Saffron in Kashmir-Vi: A Review of Distribution and Production. J. Herbs, Spices Med. Plants 4, 83–90. doi:10.1300/j044v04n04_09
Dianat, M., Esmaeilizadeh, M., Badavi, M., Samarbafzadeh, A., and Naghizadeh, B. (2014). Protective Effects of Crocin on Hemodynamic Parameters and Infarct Size in Comparison with Vitamin e After Ischemia Reperfusion in Isolated Rat Hearts. Planta Medica. 80, 393–398. doi:10.1055/s-0033-1360383
Dianat, M., and Radan, M. (2014). A Review on the Effective Properties of Crocin in the Management of Cardiopulmonary Dysfunction.
Donato, R., Sorci, G., Riuzzi, F., Arcuri, C., Bianchi, R., Brozzi, F., et al. (2009). S100b's Double Life: Intracellular Regulator and Extracellular Signal. Biochimica Biophysica Acta (bba) - Mol. Cell. Res. 1793, 1008–1022. doi:10.1016/j.bbamcr.2008.11.009
Du, J., Li, y., Song, d., Liu, j., Huang, q., Li, J., et al. (2021). Protective Effects of Crocin Against Endogenous Aβ-Induced Neurotoxicity in N2a/app695swe Cells. Berl: Psychopharmacology.
Duan, Z., Li, H., Qi, X., Wei, Y., Guo, X., Li, Y., et al. (2019). Crocin Attenuation of Neurological Deficits in a Mouse Model of Intracerebral Hemorrhage. Brain Res. Bull. 150, 186–195. doi:10.1016/j.brainresbull.2019.05.023
Dudhani, A. R., and Kosaraju, S. L. (2010). Bioadhesive Chitosan Nanoparticles: Preparation and Characterization. Carbohydr. Polym. 81, 243–251. doi:10.1016/j.carbpol.2010.02.026
Ebrahim‐Habibi, M. B., Amininasab, M., Ebrahim‐Habibi, A., Sabbaghian, M., and Nemat‐Gorgani, M. (2010). Fibrillation of α‐lactalbumin: Effect of Crocin and Safranal, Two Natural Small Molecules from Crocus Sativus. Biopolymers 93, 854–865.
El Khoudri, M., Ouahhoud, S., Lahmass, M., Khoulati, A., Benyoussef, S., Mamri, S., et al. (2021). Biological Effects and Pharmacological Activities of Saffron of Crocus Sativus. Arabian J. Med. Aromatic Plants 7, 254–268.
Escartin, C., Galea, E., Lakatos, A., O’callaghan, J. P., Petzold, G. C., Serrano-Pozo, A., et al. (2021). Reactive Astrocyte Nomenclature, Definitions, and Future Directions. Nat. Neurosci. 24, 312–325. doi:10.1038/s41593-020-00783-4
Eteghadi, M.-R., Nasehi, M., Vaseghi, S., and Hesami-Tackallou, S. (2021). The Effect of Crocin on Tfam and Pgc-1α Expression and Catalase and Superoxide Dismutase Activities Following Cholestasis-Induced Neuroinflammation in the Striatum of Male Wistar Rats. Metab. Brain Dis., 1–11. doi:10.1007/s11011-021-00748-x
Fan, X.-D., Yao, M.-J., Yang, B., Han, X., Wang, G.-R., Li, P., et al. (2021). Chinese Herbal Preparation Sailuotong Alleviates Brain Ischemia via Nrf2 Antioxidation Pathway Dependent Cerebral Microvascular Protection.
Fernández-Albarral, J. A., Ramírez, A. I., De Hoz, R., López-Villarín, N., Salobrar-García, E., López-Cuenca, I., et al. (2019). Neuroprotective and Antiinflammatory Effects of a Hydrophilic Saffron Extract in a Model of Glaucoma. Int. J. Mol. Sci. 20, 4110.
Finley, J. W., Gao, S. J. J. O. A., and Chemistry, F. 2017. A Perspective on Crocus Sativus l.(Saffron) Constituent Crocin: A Potent Water-Soluble Antioxidant and Potential Therapy for Alzheimer’s Disease. 65, 1005–1020. doi:10.1021/acs.jafc.6b04398
Frederico, D., Donate, P. M., Constantino, M. G., Bronze, E. S., and Sairre, M. I. (2003). A Short and Efficient Synthesis of Crocetin-Dimethylester and Crocetindial. J. Org. Chem. 68, 9126–9128. doi:10.1021/jo034545y
Freitas-Andrade, M., and Naus, C. (2016). Astrocytes in Neuroprotection and Neurodegeneration: The Role of Connexin43 and Pannexin1. Neuroscience 323, 207–221. doi:10.1016/j.neuroscience.2015.04.035
Fuentes, B., Martínez-Sánchez, P., and Díez-Tejedor, E. (2009). Lipid-lowering Drugs in Ischemic Stroke Prevention and Their Influence on Acute Stroke Outcome. Cerebrovasc. Dis. 27, 126–133. doi:10.1159/000200450
Fukuta, T., Asai, T., Yanagida, Y., Namba, M., Koide, H., Shimizu, K., et al. (2017). Combination Therapy with Liposomal Neuroprotectants and Tissue Plasminogen Activator for Treatment of Ischemic Stroke. faseb J. 31, 1879–1890. doi:10.1096/fj.201601209R
Gainer, J. L. (2008). Trans-sodium Crocetinate for Treating Hypoxia/ischemia. Expert Opin. Investigational Drugs 17, 917–924. doi:10.1517/13543784.17.6.917
Gainer, J. L. (2000). Trans-Sodium Crocetinate, Methods of Making and Methods of Use Thereof. Alexandria, United States: Google Patents.
Gao, G., Wu, J., Li, B., Jiang, Q., Wang, P., and Li, J. (2021). Transcriptomic Analysis of Saffron at Different Flowering Stages Using Rna Sequencing Uncovers Cytochrome P450 Genes Involved in Crocin Biosynthesis. Mol. Biol. Rep. 48, 3451–3461. doi:10.1007/s11033-021-06374-1
Gao, J., Bai, P., Li, Y., Li, J., Jia, C., Wang, T., et al. (2020). Metabolomic Profiling of the Synergistic Effects of Ginsenoside Rg1 in Combination with Neural Stem Cell Transplantation in Ischemic Stroke Rats. J. Proteome Res. 19, 2676–2688. doi:10.1021/acs.jproteome.9b00639
Gao, J., Chen, M., Ren, X.-C., Zhou, X.-B., Shang, Q., Lu, W.-Q., et al. (2017). Synthesis and Cardiomyocyte Protection Activity of Crocetin Diamide Derivatives. Fitoterapia 121, 106–111. doi:10.1016/j.fitote.2017.06.014
George, M., and Abraham, T. E. (2006). Polyionic Hydrocolloids for the Intestinal Delivery of Protein Drugs: Alginate and Chitosan—A Review. J. Control. Release 114, 1–14. doi:10.1016/j.jconrel.2006.04.017
Gerlach, R., Demel, G., König, H.-G., Gross, U., Prehn, J., Raabe, A., et al. (2006). Active Secretion of S100b from Astrocytes During Metabolic Stress. Neuroscience 141, 1697–1701. doi:10.1016/j.neuroscience.2006.05.008
Ghaderi, A., Rasouli‐Azad, M., Vahed, N., Banafshe, H. R., Soleimani, A., Omidi, A., et al. (2019). Clinical and Metabolic Responses to Crocin in Patients under Methadone Maintenance Treatment: A Randomized Clinical Trial. Phytotherapy Res. 33, 2714–2725. doi:10.1002/ptr.6445
Ghaffari, S., and Roshanravan, N. (2019). Saffron; An Updated Review on Biological Properties with Special Focus on Cardiovascular Effects. Biomed. Pharmacother. 109, 21–27. doi:10.1016/j.biopha.2018.10.031
Ghorbani, R., and Koocheki, A. (2017). Sustainable Cultivation of Saffron in Iran. Sustainable Agriculture Reviews. Springer. doi:10.1007/978-3-319-58679-3_6
Giaccio, M. (2004). Crocetin from Saffron: An Active Component of an Ancient Spice. Crit. Rev. Food Sci. Nutr. 44, 155–172. doi:10.1080/10408690490441433
Girme, A., Pawar, S., Ghule, C., Shengule, S., Saste, G., Balasubramaniam, A. K., et al. (2021). Bioanalytical Method Development and Validation Study of Neuroprotective Extract of Kashmiri Saffron Using Ultra-fast Liquid Chromatography-Tandem Mass Spectrometry (Uflc-ms/ms): In Vivo Pharmacokinetics of Apocarotenoids and Carotenoids. Molecules 26, 1815. doi:10.3390/molecules26061815
Gudarzi, S., Jafari, M., Pirzad Jahromi, G., Eshrati, R., Asadollahi, M., and Nikdokht, P. (2020). Evaluation of Modulatory Effects of Saffron (Crocus Sativus l.) Aqueous Extract on Oxidative Stress in Ischemic Stroke Patients: A Randomized Clinical Trial. Nutr. Neurosci., 1–10. doi:10.1080/1028415X.2020.1840118
Gullo, F., Ceriani, M., D'aloia, A., Wanke, E., Constanti, A., Costa, B., et al. (2017). Plant Polyphenols and Exendin-4 Prevent Hyperactivity and Tnf-α Release in Lps-Treated In Vitro Neuron/astrocyte/microglial Networks. Front. Neurosci. 11, 500. doi:10.3389/fnins.2017.00500
Gupta, A., and Pandey, A. K. (2020). Saffron and its Active Ingredients: A Natural Product with Potent Anticancer Property. Drug Development for Cancer and Diabetes. Apple Academic Press. doi:10.1201/9780429330490-17
Hadizadeh, F., Mohajeri, S., and Seifi, M. (2010). Extraction and Purification of Crocin from Saffron Stigmas Employing a Simple and Efficient Crystallization Method. Pak. J. Biol. Sci. 13, 691–698. doi:10.3923/pjbs.2010.691.698
Haeri, P., Mohammadipour, A., Heidari, Z., and Ebrahimzadeh-Bideskan, A. (2019). Neuroprotective Effect of Crocin on Substantia Nigra in Mptp-Induced Parkinson’s Disease Model of Mice. Anatomical Sci. Int. 94, 119–127. doi:10.1007/s12565-018-0457-7
Hao, C.-H., Xi, W.-G., Zheng, H.-J., Wang, W.-T., and Zhao, Z.-Y. (2015). Effect of Chinese Patent Medicine Naodesheng against Repeated Transient Global Cerebral Ischemia in Mice. Chin. Herb. Med. 7, 339–343. doi:10.1016/s1674-6384(15)60062-4
Hao, C.-Z., Wu, f., Shen, j., Lu, l., Fu, D.-L., Liao, W.-J., et al. (2012). Clinical Efficacy and Safety of Buyang Huanwu Decoction for Acute Ischemic Stroke: A Systematic Review and Meta-Analysis of 19 Randomized Controlled Trials Evidence-Based Complementary and Alternative Medicine.
Harari, O. A., and Liao, J. K. (2010). Nf-κb and Innate Immunity in Ischemic Stroke. Ann. N. Y. Acad. Sci. 1207, 32–40. doi:10.1111/j.1749-6632.2010.05735.x
Hariri, A. T., Moallem, S. A., Mahmoudi, M., Memar, B., and Hosseinzadeh, H. (2010). Sub-acute Effects of Diazinon on Biochemical Indices and Specific Biomarkers in Rats: Protective Effects of Crocin and Safranal. Food Chem. Toxicol. 48, 2803–2808. doi:10.1016/j.fct.2010.07.010
Hashemi, M., and Hosseinzadeh, H. (2019). A Comprehensive Review on Biological Activities and Toxicology of Crocetin. Food Chem. Toxicol. 130, 44–60. doi:10.1016/j.fct.2019.05.017
Hashemzaei, M., Mamoulakis, C., Tsarouhas, K., Georgiadis, G., Lazopoulos, G., Tsatsakis, A., et al. (2020). Crocin: A Fighter against Inflammation and Pain. Food Chem. Toxicol. 143, 111521. doi:10.1016/j.fct.2020.111521
He, S.-Y., Qian, Z.-Y., Tang, F.-T., Wen, N., Xu, G.-L., and Sheng, L. (2005). Effect of Crocin on Experimental Atherosclerosis in Quails and its Mechanisms. Life Sci. 77, 907–921. doi:10.1016/j.lfs.2005.02.006
Higashino, S., Sasaki, Y., Giddings, J. C., Hyodo, K., Fujimoto Sakata, S., Matsuda, K., et al. (2014). Crocetin, a Carotenoid from Gardenia Jasminoides Ellis, Protects against Hypertension and Cerebral Thrombogenesis in Stroke‐prone Spontaneously Hypertensive Rats. Phytotherapy Res. 28, 1315–1319. doi:10.1002/ptr.5130
Hoshyar, R., Khayati, G. R., Poorgholami, M., and Kaykhaii, M. (2016). A Novel Green One-step Synthesis of Gold Nanoparticles Using Crocin and Their Anti-cancer Activities. J. Photochem. Photobiol. B Biol. 159, 237–242. doi:10.1016/j.jphotobiol.2016.03.056
Hoshyar, R., and Mollaei, H. (2017). A Comprehensive Review on Anticancer Mechanisms of the Main Carotenoid of Saffron, Crocin. J. Pharm. Pharmacol. 69, 1419–1427. doi:10.1111/jphp.12776
Hosseini, A., Razavi, B. M., and Hosseinzadeh, H. (2018). Pharmacokinetic Properties of Saffron and its Active Components. Eur. J. Drug Metabolism Pharmacokinet. 43, 383–390. doi:10.1007/s13318-017-0449-3
Hosseinzadeh, H., Mehri, S., Heshmati, A., Ramezani, M., Sahebkar, A., and Abnous, K. (2014a). Proteomic Screening of Molecular Targets of Crocin. Daru J. Pharm. Sci. 22, 5. doi:10.1186/2008-2231-22-5
Hosseinzadeh, H., Mehri, S., Heshmati, A., Ramezani, M., Sahebkar, A., and Abnous, K. (2014b). Screening of Molecular Targets of Crocin. Daru J. Fac. Pharm. Berlin/Heidelberg, Germany: Springer: Google Patents. 22, 5. doi:10.1186/2008-2231-22-5
Hosseinzadeh, H., Sadeghnia, H. R., Ghaeni, F. A., Motamedshariaty, V. S., and Mohajeri, S. A. (2012). Effects of Saffron (Crocus Sativus l.) and its Active Constituent, Crocin, on Recognition and Spatial Memory after Chronic Cerebral Hypoperfusion in Rats. Phytotherapy Res. 26, 381–386. doi:10.1002/ptr.3566
Hosseinzadeh, H., Shariaty, V. M., Sameni, A. K., and Vahabzadeh, M. (2010). Acute and Sub-acute Toxicity of Crocin, a Constituent of Crocus Sativus l. (Saffron), in Mice and Rats. Pharmacologyonline 2, 943–951.
Huang, A., and Jia, L. (2019a). Crocin Enhances Hypothermia Therapy in Hypoxic Ischemia-Induced Brain Injury in Mice. Acta Neurol. Belg. doi:10.1007/s13760-019-01198-0
Huang, A., and Jia, L. (2019b). Crocin Enhances Hypothermia Therapy in Hypoxic Ischemia-Induced Brain Injury in Mice. Acta Neurol. Belg., 1–8. doi:10.1007/s13760-019-01198-0
Huang, Z., Xu, J., Huang, X., Sun, G., Jiang, R., Wu, H., et al. (2019). Crocin Induces Anti-ischemia in Middle Cerebral Artery Occlusion Rats and Inhibits Autophagy by Regulating the Mammalian Target of Rapamycin. Eur. J. Pharmacol. 857, 172424. doi:10.1016/j.ejphar.2019.172424
Hussain, I., Singh, N. B., Singh, A., Singh, H., and Singh, S. C. (2016). Green Synthesis of Nanoparticles and its Potential Application. Biotechnol. Lett. 38, 545–560. doi:10.1007/s10529-015-2026-7
Hussain, M. A., Abogresha, N. M., Abdelkader, G., Hassan, R., Abdelaziz, E. Z., and Greish, S. M. (2021). Antioxidant and Antiinflammatory Effects of Crocin Ameliorate Doxorubicin-Induced Nephrotoxicity in Rats. Oxidative Med. Cell. Longev. 2021, 8841726. doi:10.1155/2021/8841726
Hussein, S. A., Ahmed, T. E., Amin, A., and Ali, A. H. (2019). The Antiinflammatory and Anti-apoptotic Role of Crocin against Expression of Mmp-9, Timp-1, Mcp-1, Caspase-3, Pparα in Heart Tissue, and Metamorphoses of Microrna 188 in Hyperhomocysteinemic Rats. Recent Adv. Biol. Med. 5, 10601. doi:10.18639/rabm.2019.883878
Imran, M., Imran, A., Arshad, M. U., and Suleria, H. A. R. (2019). Crocin: A Mechanistic Treatise. Bioactive Compounds from Plant Origin. Apple Academic Press. doi:10.1201/9780429029288-5
Jackson, P. A., Forster, J., Khan, J., Pouchieu, C., Dubreuil, S., Gaudout, D., et al. (2021). Effects of Saffron Extract Supplementation on Mood, Well-Being, and Response to a Psychosocial Stressor in Healthy Adults: A Randomized, Double-Blind, Parallel Group, Clinical Trial. Front. Nutr., 365. doi:10.3389/fnut.2020.606124
Jahanbakhsh, Z., Rasoulian, B., Jafari, M., Shekarforoush, S., Esmailidehaj, M., Taghi Mohammadi, M., et al. (2012). Protective Effect of Crocin against Reperfusion-Induced Cardiac Arrhythmias in Anaesthetized Rats. Excli J. 11, 20.
Jan, S., Wani, A. A., Kamili, A. N., and Kashtwari, M. (2014). Distribution, Chemical Composition and Medicinal Importance of Saffron (Crocus Sativus l.). Afr. J. plant Sci. 8, 537–545.
Javandoost, A., Afshari, A., Nikbakht-Jam, I., Khademi, M., Eslami, S., Nosrati, M., et al. (2017). Effect of Crocin, a Carotenoid from Saffron, on Plasma Cholesteryl Ester Transfer Protein and Lipid Profile in Subjects with Metabolic Syndrome: A Double Blind Randomized Clinical Trial. Arya Atheroscler. 13, 245–252.
Jia, J., Wei, C., Chen, S., Li, F., Tang, Y., Qin, W., et al. (2018). Efficacy and Safety of the Compound Chinese Medicine Sailuotong in Vascular Dementia: A Randomized Clinical Trial. Alzheimer's Dementia Transl. Res. Clin. Interventions 4, 108–117. doi:10.1016/j.trci.2018.02.004
Karkoula, E., Dagla, I.-V., Baira, E., Kokras, N., Dalla, C., Skaltsounis, A.-L., et al. (2020). A Novel Uhplc-Hrms-Based Metabolomics Strategy Enables the Discovery of Potential Neuroactive Metabolites in Mice Plasma, Following I.P. Administration of the Main Crocus Sativus L. Bioactive Component. J. Pharm. Biomed. Analysis 177, 112878. doi:10.1016/j.jpba.2019.112878
Karkoula, E., Lemonakis, N., Kokras, N., Dalla, C., Gikas, E., Skaltsounis, A.-L., et al. (2018). Trans-crocin 4 Is Not Hydrolyzed to Crocetin Following Ip Administration in Mice, While it Shows Penetration through the Blood Brain Barrier. Fitoterapia 129, 62–72. doi:10.1016/j.fitote.2018.06.012
Kashani, L., Raisi, F., Saroukhani, S., Sohrabi, H., Modabbernia, A., Nasehi, A. A., et al. (2013). Saffron for Treatment of Fluoxetine‐induced Sexual Dysfunction in Women: Randomized Double‐blind Placebo‐controlled Study. Hum. Psychopharmacol. Clin. Exp. 28, 54–60. doi:10.1002/hup.2282
Kazley, A. S., Simpson, K. N., Simpson, A., Jauch, E., and Adams, R. J. (2013). Optimizing the Economic Impact of Rtpa Use in a Stroke Belt State: the Case of South Carolina. Am. Health & Drug Benefits 6, 155.
Kell, G., Rao, A., Beccaria, G., Clayton, P., Inarejos-García, A. M., and Prodanov, M. (2017). Affron® a Novel Saffron Extract (Crocus Sativus l.) Improves Mood in Healthy Adults over 4 Weeks in a Double-Blind, Parallel, Randomized, Placebo-Controlled Clinical Trial. Complementary Ther. Med. 33, 58–64. doi:10.1016/j.ctim.2017.06.001
Kermani, T., Kazemi, T., Molki, S., Ilkhani, K., Sharifzadeh, G., and Rajabi, O. (2017). The Efficacy of Crocin of Saffron (Crocus Sativus l.) on the Components of Metabolic Syndrome: A Randomized Controlled Clinical Trial. J. Res. Pharm. Pract. 6, 228. doi:10.4103/jrpp.JRPP_17_26
Kermanshahi, S., Ghanavati, G., Abbasi-Mesrabadi, M., Gholami, M., Ulloa, L., Motaghinejad, M., et al. (2020). Novel Neuroprotective Potential of Crocin in Neurodegenerative Disorders: an Illustrated Mechanistic Review. Neurochem. Res. 45, 2573–2585. doi:10.1007/s11064-020-03134-8
Khalatbari-Mohseni, A., Banafshe, H. R., Mirhosseini, N., Asemi, Z., Ghaderi, A., and Omidi, A. (2019). The Effects of Crocin on Psychological Parameters in Patients under Methadone Maintenance Treatment: A Randomized Clinical Trial. Subst. Abuse Treat. Prev. Policy 14, 9. doi:10.1186/s13011-019-0198-1
Khan, M. T., Ikram, A., Saeed, O., Afridi, T., Sila, C. A., Smith, M. S., et al. (2017). Deep Vein Thrombosis in Acute Stroke-A Systemic Review of the Literature. Cureus 9. doi:10.7759/cureus.1982
Khandelwal, P., Martínez-Pías, E., Bach, I., Prakash, T., Hillen, M. E., Martínez-Galdámez, M., et al. (2021). Severe Epistaxis after Tissue Plasminogen Activator Administration for Acute Ischemic Stroke in Sars-Cov-2 Infection. Brain Circ. 7, 135. doi:10.4103/bc.bc_17_21
Khazdair, M. R., Anaeigoudari, A., Hashemzehi, M., and Mohebbati, R. (2019). Neuroprotective Potency of Some Spice Herbs, a Literature Review. J. Traditional Complementary Med. 9, 98–105. doi:10.1016/j.jtcme.2018.01.002
Khorasany, A. R., and Hosseinzadeh, H. (2016). Therapeutic Effects of Saffron (Crocus Sativus l.) in Digestive Disorders: A Review. Iran. J. Basic Med. Sci. 19, 455.
Kianbakht, S., and Hajiaghaee, R. (2011). Anti-Hyperglycemic Effects of Saffron and its Active Constituents, Crocin and Safranal, in Alloxan-Induced Diabetic Rats. Jmpir 10, 82–89.
Kim, D.-G., Jeong, Y.-I., Choi, C., Roh, S.-H., Kang, S.-K., Jang, M.-K., et al. (2006). Retinol-encapsulated Low Molecular Water-Soluble Chitosan Nanoparticles. Int. J. Pharm. 319, 130–138. doi:10.1016/j.ijpharm.2006.03.040
Kocaman, G., Altinoz, E., Erdemli, M. E., Gul, M., Erdemli, Z., Zayman, E., et al. (2021). Crocin Attenuates Oxidative and Inflammatory Stress-Related Periodontitis in Cardiac Tissues in Rats. Adv. Clin. Exp. Med. 30, 517–524. doi:10.17219/acem/133753
Kothari, D., Thakur, R., and Kumar, R. (2021a). Saffron (Crocus Sativus l.): Gold of the Spices—A Comprehensive Review. Hortic. Environ. Biotechnol. 62, 661–677. doi:10.1007/s13580-021-00349-8
Kothari, D., Thakur, R., and Kumar, R. (2021b). Saffron (Crocus Sativus l.): Gold of the Spices—A Comprehensive Review. Hortic. Environ. Biotechnol., 1–17.
Krishnaswamy, V., Alugoju, P., and Periyasamy, L. (2020). Effect of Short-Term Oral Supplementation of Crocin on Age-Related Oxidative Stress, Cholinergic, and Mitochondrial Dysfunction in Rat Cerebral Cortex. Life Sci. 263, 118545. doi:10.1016/j.lfs.2020.118545
Lapchak, P. A. (2010). Efficacy and Safety Profile of the Carotenoid Trans Sodium Crocetinate Administered to Rabbits Following Multiple Infarct Ischemic Strokes: A Combination Therapy Study with Tissue Plasminogen Activator. Brain Res. 1309, 136–145. doi:10.1016/j.brainres.2009.10.067
Lasek-Bal, A., Jedrzejowska-Szypulka, H., Student, S., Warsz-Wianecka, A., Zareba, K., Puz, P., et al. (2019). The Importance of Selected Markers of Inflammation and Blood-Brain Barrier Damage for Short-Term Ischemic Stroke Prognosis. J. Physiology Pharmacol. 70, 209–217. doi:10.26402/jpp.2019.2.04
Lautenschläger, M., Sendker, J., Hüwel, S., Galla, H., Brandt, S., Düfer, M., et al. (2015). Intestinal Formation of Trans-crocetin from Saffron Extract (Crocus Sativus l.) and In Vitro Permeation through Intestinal and Blood Brain Barrier. Phytomedicine 22, 36–44.
Lech, K., Witowska‐Jarosz, J., and Jarosz, M. (2009). Saffron Yellow: Characterization of Carotenoids by High Performance Liquid Chromatography with Electrospray Mass Spectrometric Detection. J. Mass Spectrom. 44, 1661–1667. doi:10.1002/jms.1631
Leijiao Fan, G. H. (2021a). Protective Effect of Crocin on Myocardial Mitochondria in Rats with Acute Myocardial Infaction. Chin. J. Arterioscler. 29, 383–388.
Leijiao Fan, G. H. (2021b). Protective Effect of Crocin on Myocardial Mitochondria in Rats with Acute Myocardial Infaction. Chin. J. Arterioscler. 29, 383–388.
Li, N., Lin, G., Kwan, Y.-W., and Min, Z.-D. (1999). Simultaneous Quantification of Five Major Biologically Active Ingredients of Saffron by High-Performance Liquid Chromatography. J. Chromatogr. A 849, 349–355. doi:10.1016/s0021-9673(99)00600-7
LI, W., Li, X., Du, Q., Li, F., Zhu, Y., Liu, Y., et al. (2014). Effect of Tongluojiunao Injection Made from Sanqi (Radix Notoginseng) and Zhizi (Fructus Gardeniae) on Brain Microvascular Endothelial Cells and Astrocytes in an In Vitro Ischemic Model. J. Traditional Chin. Med. 34, 725–732. doi:10.1016/s0254-6272(15)30088-1
Li, X., Liu, Y., Cao, A., Li, C., Wang, L., Wu, Q., et al. (2021). Crocin Improves Endothelial Mitochondrial Dysfunction via gpx1/ros/kca3.1 Signal Axis in Diabetes. Front. Cell. Dev. Biol. 9, 651434. doi:10.3389/fcell.2021.651434
Li, Y., Kakkar, R., and Wang, J. (2018). In Vivo and In Vitro Approach to Anti-arthritic and Anti-inflammatory Effect of Crocetin by Alteration of Nuclear Factor-E2-Related Factor 2/hem Oxygenase (Ho)-1 and Nf-Κb Expression. Front. Pharmacol. 9, 1341. doi:10.3389/fphar.2018.01341
Liddelow, S. A., Guttenplan, K. A., Clarke, L. E., Bennett, F. C., Bohlen, C. J., Schirmer, L., et al. (2017). Neurotoxic Reactive Astrocytes Are Induced by Activated Microglia. Nature 541, 481–487. doi:10.1038/nature21029
Liu, H., Zhang, Z., Zang, C., Wang, L., Yang, H., Sheng, C., et al. (2021a). Gj-4 Ameliorates Memory Impairment in Focal Cerebral Ischemia/reperfusion of Rats via Inhibiting Jak2/stat1-Mediated Neuroinflammation. J. Ethnopharmacol. 267, 113491. doi:10.1016/j.jep.2020.113491
Liu, J., Ye, C., Lv, G., Li, G., Gao, Y., and Ji, X. (2021b). Crocetin Improves Ischaemic Stroke in Vitro and Vivo. Berlin/Heidelberg, Germany: Springer.
Liu, R., Yuan, H., Yuan, F., and Yang, S.-H. (2012). Neuroprotection Targeting Ischemic Penumbra and Beyond for the Treatment of Ischemic Stroke. Neurological Res. 34, 331–337. doi:10.1179/1743132812Y.0000000020
Liu, T., Yu, S., Xu, Z., Tan, J., Wang, B., Liu, Y.-G., et al. (2020). Prospects and Progress on Crocin Biosynthetic Pathway and Metabolic Engineering. Comput. Struct. Biotechnol. J. 18, 3278–3286. doi:10.1016/j.csbj.2020.10.019
Liu, Z., and Chopp, M. (2016). Astrocytes, Therapeutic Targets for Neuroprotection and Neurorestoration in Ischemic Stroke. Prog. Neurobiol. 144, 103–120. doi:10.1016/j.pneurobio.2015.09.008
Lv, B., Yin, J., Feng, C., and Li, Y. (2019). Neuroprotective Effects of Crocin I and Ii in an Ischemia-Reperfusion Injury Model. New York, United States: Biorxiv, 757971.
Manabe, H., Okonkwo, D. O., Gainer, J. L., Clarke, R. H., and Lee, K. S. (2010). Protection against Focal Ischemic Injury to the Brain by Trans-sodium Crocetinate. J. Neurosurg. 113, 802–809. doi:10.3171/2009.10.JNS09562
Mard, S. A., Akbari, G., Dianat, M., and Mansouri, E. (2017). Protective Effects of Crocin and Zinc Sulfate on Hepatic Ischemia-Reperfusion Injury in Rats: A Comparative Experimental Model Study. Biomed. Pharmacother. 96, 48–55.
Marquez-Romero, J. M., Góngora-Rivera, F., Hernández-Curiel, B. C., Aburto-Murrieta, Y., García-Cazares, R., Delgado-Garzón, P., et al. (2020). Endovascular Treatment of Ischemic Stroke in a Developing Country. Vasc. Endovascular Surg. 54, 305–312. doi:10.1177/1538574420906941
Martin, G., Goh, E., and Neff, A. (2002). Evaluation of the Developmental Toxicity of Crocetin on Xenopus. Food Chem. Toxicol. 40, 959–964. doi:10.1016/s0278-6915(02)00040-6
Martinez, C., Wallenhorst, C., Rietbrock, S., and Freedman, B. (2020). Ischemic Stroke and Transient Ischemic Attack Risk Following Vitamin K Antagonist Cessation in Newly Diagnosed Atrial Fibrillation: a Cohort Study. J. Am. Heart Assoc. 9, e014376. doi:10.1161/JAHA.119.014376
Mehri, S., Abnous, K., Khooei, A., Mousavi, S. H., Shariaty, V. M., and Hosseinzadeh, H. (2015). Crocin Reduced Acrylamide-Induced Neurotoxicity in Wistar Rat through Inhibition of Oxidative Stress. Iran. J. basic Med. Sci. 18, 902.
Mehri, S., Abnous, K., Mousavi, S. H., Shariaty, V., and Hosseinzadeh, H. (2011). Neuroprotective Effect of Crocin on Acrylamide-Induced Cytotoxicity in Pc12 Cells. Cell. Mol. Neurobiol. 32, 227–235. doi:10.1007/s10571-011-9752-8
Mehrnia, M.-A., Jafari, S.-M., Makhmal-Zadeh, B. S., and Maghsoudlou, Y. J. F. H. (2017). Rheological and Release Properties of Double Nano-Emulsions Containing Crocin Prepared with Angum Gum. Arabic Gum Whey Protein 66, 259–267. doi:10.1016/j.foodhyd.2016.11.033
Mirhadi, E., Nassirli, H., and Malaekeh-Nikouei, B. (2020). An Updated Review on Therapeutic Effects of Nanoparticle-Based Formulations of Saffron Components (Safranal, Crocin, and Crocetin). J. Pharm. Investigation 50, 47–58. doi:10.1007/s40005-019-00435-1
Moallem, S. A., Afshar, M., Etemad, L., Razavi, B. M., and Hosseinzadeh, H. (2016). Evaluation of Teratogenic Effects of Crocin and Safranal, Active Ingredients of Saffron, in Mice. Toxicol. Industrial Health 32, 285–291. doi:10.1177/0748233713500818
Modaghegh, M.-H., Shahabian, M., Esmaeili, H.-A., Rajbai, O., and Hosseinzadeh, H. (2008). Safety Evaluation of Saffron (Crocus Sativus) Tablets in Healthy Volunteers. Phytomedicine 15, 1032–1037. doi:10.1016/j.phymed.2008.06.003
Mohajeri, S. A., Hosseinzadeh, H., Keyhanfar, F., and Aghamohammadian, J. (2010a). Extraction of Crocin from Saffron (Crocus Sativus) Using Molecularly Imprinted Polymer Solid-Phase Extraction. J. Sep. Sci. 33, 2302–2309. doi:10.1002/jssc.201000183
Mohajeri, S. A., Hosseinzadeh, H., Keyhanfar, F., and Aghamohammadian, J. (2010b). Extraction of Crocin from Saffron (Crocus Sativus) Using Molecularly Imprinted Polymer Solid‐Phase Extraction. J. Sep. Sci. 33, 2302–2309. doi:10.1002/jssc.201000183
Mohamadpour, A. H., Ayati, Z., Parizadeh, M. R., Rajbai, O., and Hosseinzadeh, H. (2013). Safety Evaluation of Crocin (A Constituent of Saffron) Tablets in Healthy Volunteers. Iran. J. Basic Med. Sci. 16, 39.
Mohler, E. R., Gainer, J. L., Whitten, K., Eraso, L. H., Thanaporn, P. K., and Bauer, T. (2011). Evaluation of Trans Sodium Crocetinate on Safety and Exercise Performance in Patients with Peripheral Artery Disease and Intermittent Claudication. Vasc. Med. 16, 346–353. doi:10.1177/1358863X11422742
Mok, I.-K., Nguyen, T. T. H., Kim, D. H., Lee, J. W., Lim, S., Jung, H.-Y., et al. (2020). Enhancement of Neuroprotection, Antioxidant Capacity, and Water-Solubility of Crocins by Transglucosylation Using Dextransucrase under High Hydrostatic Pressure. Enzyme Microb. Technol. 140, 109630. doi:10.1016/j.enzmictec.2020.109630
Mollazadeh, H., Emami, S. A., and Hosseinzadeh, H. (2015). Razi’s Al-Hawi and Saffron (Crocus Sativus): A Review. Iran. J. basic Med. Sci. 18, 1153.
Moratalla-López, N., Parizad, S., Habibi, M. K., Winter, S., Kalantari, S., Bera, S., et al. (2021). Impact of Two Different Dehydration Methods on Saffron Quality, Concerning the Prevalence of Saffron Latent Virus (Salv) in Iran. Food Chem. 337, 127786. doi:10.1016/j.foodchem.2020.127786
Mousavi, B., Bathaie, S. Z., Fadai, F., Ashtari, Z., Ali Beigi, N., Farhang, S., et al. (2015). Safety Evaluation of Saffron Stigma (Crocus Sativus l.) Aqueous Extract and Crocin in Patients with Schizophrenia. Avicenna J. Phytomedicine 5, 413–419.
Nagatoshi, M., Terasaka, K., Owaki, M., Sota, M., Inukai, T., Nagatsu, A., et al. (2012). Ugt75l6 and ugt94e5 Mediate Sequential Glucosylation of Crocetin to Crocin in Gardenia Jasminoides. Febs Lett. 586, 1055–1061. doi:10.1016/j.febslet.2012.03.003
Najafabadi, B. T., Farsinejad, M., Shokraee, K., Momtazmanesh, S., Violette, P. D., Esalatmanesh, S., et al. (2022). Possible Effects of Saffron (Crocus Sativus) in the Treatment of Erectile Dysfunction: A Randomized, Double-Blind, Placebo-Controlled Trial. J. Herb. Med., 100551. doi:10.1016/j.hermed.2022.100551
Nam, K. N., Park, Y.-M., Jung, H.-J., Lee, J. Y., Min, B. D., Park, S.-U., et al. (2010). Antiinflammatory Effects of Crocin and Crocetin in Rat Brain Microglial Cells. Eur. J. Pharmacol. 648, 110–116. doi:10.1016/j.ejphar.2010.09.003
Nassar, R., Eid, S., Chahine, R., Chabi, B., Bonnieu, A., Sabban, M. E., et al. (2020). Antioxidant Effects of Lebanese Crocus Sativus L. And its Main Components, Crocin and Safranal, on Human Skeletal Muscle Cells. Eur. J. Integr. Med. 40, 101250. doi:10.1016/j.eujim.2020.101250
Ncbi, P. (2021). Crocin 7. [Online]. Pubchem.ncbi.nih. Available: https://pubchem.ncbi.nlm.nih.gov/compound/101124079 (Accessed 11 08, 2021).
Nikbakht-Jam, I., Khademi, M., Nosrati, M., Eslami, S., Foroutan-Tanha, M., Sahebkar, A., et al. (2016). Effect of Crocin Extracted from Saffron on Pro-oxidant–anti-oxidant Balance in Subjects with Metabolic Syndrome: A Randomized, Placebo-Controlled Clinical Trial. Eur. J. Integr. Med. 8, 307–312. doi:10.1016/j.eujim.2015.12.008
Ochiai, T., Ohno, S., Soeda, S., Tanaka, H., Shoyama, Y., and Shimeno, H. (2004a). Crocin Prevents the Death of Rat Pheochromyctoma (Pc-12) Cells by its Antioxidant Effects Stronger Than Those of α-tocopherol. Neurosci. Lett. 362, 61–64. doi:10.1016/j.neulet.2004.02.067
Ochiai, T., Soeda, S., Ohno, S., Tanaka, H., Shoyama, Y., and Shimeno, H. (2004b). Crocin Prevents the Death of Pc-12 Cells through Sphingomyelinase-Ceramide Signaling by Increasing Glutathione Synthesis. Neurochem. Int. 44, 321–330. doi:10.1016/s0197-0186(03)00174-8
Orset, C., Arkelius, K., Anfray, A., Warfvinge, K., Vivien, D., and Ansar, S. (2021). Combination Treatment with U0126 and Rt-Pa Prevents Adverse Effects of the Delayed Rt-Pa Treatment after Acute Ischemic Stroke. Sci. Rep. 11, 1–10. doi:10.1038/s41598-021-91469-9
Oruc, S., Gönül, Y., Tunay, K., Oruc, O. A., Bozkurt, M. F., Karavelioğlu, E., et al. (2016). The Antioxidant and Antiapoptotic Effects of Crocin Pretreatment on Global Cerebral Ischemia Reperfusion Injury Induced by Four Vessels Occlusion in Rats. Life Sci. 154, 79–86. doi:10.1016/j.lfs.2016.04.028
Ozaki, A., Kitano, M., Furusawa, N., Yamaguchi, H., Kuroda, K., and Endo, G. (2002). Genotoxicity of Gardenia Yellow and its Components. Food Chem. Toxicol. 40, 1603–1610. doi:10.1016/s0278-6915(02)00118-7
Pandita, D. (2021). Saffron (Crocus Sativus l.): Phytochemistry, Therapeutic Significance and Omics-Based Biology. Medicinal and Aromatic Plants. Elsevier.
Pang, Q., Zhang, W., Li, C., Li, H., Zhang, Y., Li, L., et al. (2020). Antidementia Effects, Metabolic Profiles and Pharmacokinetics of Gj-4, a Crocin-Rich Botanical Candidate from Gardeniae Fructus. Food & Funct. 11, 8825–8836. doi:10.1039/d0fo01678k
Papandreou, M. A., Kanakis, C. D., Polissiou, M. G., Efthimiopoulos, S., Cordopatis, P., Margarity, M., et al. (2006). Inhibitory Activity on Amyloid-β Aggregation and Antioxidant Properties of Crocus Sativus Stigmas Extract and its Crocin Constituents. J. Agric. Food Chem. 54, 8762–8768. doi:10.1021/jf061932a
Pazoki, B., Zandi, N., Assaf, Z., Moghaddam, H. S., Zeinoddini, A., Mohammadi, M. R., et al. (2022). Efficacy and Safety of Saffron as Adjunctive Therapy in Adults with Attention-Deficit/hyperactivity Disorder: A Randomized, Double-Blind, Placebo-Controlled Clinical Trial. In Advances in Integrative Medicine. doi:10.1016/j.aimed.2022.01.002
Pergolizzi, J. V., Coluzzi, F., Colucci, R. D., Olsson, H., Lequang, J. A., Al-Saadi, J., et al. (2020). Expert Review of Clinical Pharmacology. doi:10.1080/17512433.2020.1734451 Statins and Muscle Pain.
Pfister, S., Meyer, P., Steck, A., and Pfander, H. (1996). Isolation and Structure Elucidation of Carotenoid−Glycosyl Esters in Gardenia Fruits (Gardenia Jasminoides Ellis) and Saffron (Crocus Sativus Linne). J. Agric. Food Chem. 44, 2612–2615. doi:10.1021/jf950713e
Pprc, C. P. C. (2020). Pharmacopoeia of the People's Republic of China. Beijing, China: China Medical Science Press. (Chinese Edition).
Pu, X., He, C., Yang, Y., Wang, W., Hu, K., Xu, Z., et al. (2020). In Vivo Production of Five Crocins in the Engineered Escherichia Coli. Acs Synth. Biol. 9, 1160–1168. doi:10.1021/acssynbio.0c00039
Puglia, C., Rizza, L., Drechsler, M., and Bonina, F. (2010). Nanoemulsions as Vehicles for Topical Administration of Glycyrrhetic Acid: Characterization and In Vitro and In Vivo Evaluation. Drug Deliv. 17, 123–129. doi:10.3109/10717540903581679
Puglia, C., Santonocito, D., Musumeci, T., Cardile, V., Graziano, A. C. E., Salerno, L., et al. (2019). Nanotechnological Approach to Increase the Antioxidant and Cytotoxic Efficacy of Crocin and Crocetin. Planta Medica. 85, 258–265. doi:10.1055/a-0732-5757
QI, Y., Chen, L., Zhang, L., Liu, W.-B., Chen, X.-Y., and Yang, X.-G. (2013). Crocin Prevents Retinal Ischaemia/reperfusion Injury-Induced Apoptosis in Retinal Ganglion Cells through the Pi3k/akt Signaling Pathway. Exp. Eye Res. 107, 44–51. doi:10.1016/j.exer.2012.11.011
Qin, L., Liu, H., Wang, J., Wang, W., and Zhang, L. (2021). Crocetin Exerts a Cardio-Protective Effect on Mice with Coxsackievirus B3-Induced Acute Viral Myocarditis. J. Oleo Sci. 70, 1115–1124. doi:10.5650/jos.ess21100
Rahaiee, S., Hashemi, M., Shojaosadati, S. A., Moini, S., and Razavi, S. H. (2017). Nanoparticles Based on Crocin Loaded Chitosan-Alginate Biopolymers: Antioxidant Activities, Bioavailability and Anticancer Properties. Int. J. Biol. Macromol. 99, 401–408. doi:10.1016/j.ijbiomac.2017.02.095
Rahaiee, S., Shojaosadati, S. A., Hashemi, M., Moini, S., and Razavi, S. H. (2015). Improvement of Crocin Stability by Biodegradeble Nanoparticles of Chitosan-Alginate. Int. J. Biol. Macromol. 79, 423–432. doi:10.1016/j.ijbiomac.2015.04.041
Rao, S. V., Hemalatha, P., Yetish, S., Muralidhara, M., and Rajini, P. S. (2019a). Prophylactic Neuroprotective Propensity of Crocin, a Carotenoid against Rotenone Induced Neurotoxicity in Mice: Behavioural and Biochemical Evidence. Metab. Brain Dis., 1–13. doi:10.1007/s11011-019-00451-y
Rao, S. V., Hemalatha, P., Yetish, S., Muralidhara, M., and Rajini, P. S. (2019b). Prophylactic Neuroprotective Propensity of Crocin, a Carotenoid against Rotenone Induced Neurotoxicity in Mice: Behavioural and Biochemical Evidence. Metab. Brain Dis. 34, 1341–1353. doi:10.1007/s11011-019-00451-y
Rodriguez, C., Agulla, J., and Delgado-Esteban, M. (2021). Refocusing the Brain: New Approaches in Neuroprotection against Ischemic Injury, 46. Berlin/Heidelberg, Germany: Springer. doi:10.1007/s11064-020-03016-z
Sai Li, H. Y., and Xuansheng, D. (2017). The Effect of Crocin on Thoracic Aorta Artery Systolic and Diastolic Function on Diabetic Rats. Pharm. Clin. Res. 25, 183–186.
Salama, R. M., Abdel-Latif, G. A., Abbas, S. S., Hekmat, M., and Schaalan, M. F. (2020). Neuroprotective Effect of Crocin against Rotenone-Induced Parkinson's Disease in Rats: Interplay between Pi3k/akt/mtor Signaling Pathway and Enhanced Expression of Mirna-7 and Mirna-221. Neuropharmacology 164, 107900. doi:10.1016/j.neuropharm.2019.107900
Saraiva, M., and O'garra, A. (2010). The Regulation of Il-10 Production by Immune Cells. Nat. Rev. Immunol. 10, 170–181. doi:10.1038/nri2711
Sarshoori, J. R., Asadi, M. H., and Mohammadi, M. T. (2014). Neuroprotective Effects of Crocin on the Histopathological Alterations Following Brain Ischemia-Reperfusion Injury in Rat. Iran. J. Basic Med. Sci. 17, 895.
Sepahi, S., Mohajeri, S. A., Hosseini, S. M., Khodaverdi, E., Shoeibi, N., Namdari, M., et al. (2018). Effects of Crocin on Diabetic Maculopathy: A Placebo-Controlled Randomized Clinical Trial. Am. J. Ophthalmol. 190, 89–98. doi:10.1016/j.ajo.2018.03.007
Seto, S., Chang, D., Ko, W., Zhou, X., Kiat, H., Bensoussan, A., et al. (2017). Sailuotong Prevents Hydrogen Peroxide (H2o2)-induced Injury in Ea. Hy926 Cells. Int. J. Mol. Sci. 18, 95. doi:10.3390/ijms18010095
Shafahi, M., Vaezi, G., Shajiee, H., Sharafi, S., and Khaksari, M. (2018). Crocin Inhibits Apoptosis and Astrogliosis of hippocampus Neurons against Methamphetamine Neurotoxicity via Antioxidant and Antiinflammatory Mechanisms. Neurochem. Res. 43, 2252–2259. doi:10.1007/s11064-018-2644-2
Shah, H. M., Jain, A. S., Joshi, S. V., and Kharkar, P. S. (2021). Crocetin and Related Oxygen Diffusion‐enhancing Compounds: Review of Chemical Synthesis, Pharmacology, Clinical Development, and Novel Therapeutic Applications. Hoboken, New Jersey, United States: Wiley.
Shahbaz, K. (2017a). Oxidative Stress Causes Aging: Genetics and Epigenetics. Merit Res. J. Med. Med. Sci. 5, 604–610.
Shahmansouri, N., Farokhnia, M., Abbasi, S.-H., Kassaian, S. E., Tafti, A.-A. N., Gougol, A., et al. (2014). A Randomized, Double-Blind, Clinical Trial Comparing the Efficacy and Safety of Crocus Sativus L. With Fluoxetine for Improving Mild to Moderate Depression in Post Percutaneous Coronary Intervention Patients. J. Affect. Disord. 155, 216–222. doi:10.1016/j.jad.2013.11.003
Sharma, B., Kumar, H., Kaushik, P., Mirza, R., Awasthi, R., and Kulkarni, G. (2020). Therapeutic Benefits of Saffron in Brain Diseases: New Lights on Possible Pharmacological Mechanisms. Saffron. Elsevier.
Sheikhani, A., Kholdi, N., and Mokhtari, M. (2017). An Overview of the Most Effective Tranquilizer Herbs. J. Basic Clin. Pathophysiol. 5, 27–34. doi:10.22342/jims.23.1.257.27-42
Shen, J. Z., Wu, G., and Guo, S. (2021). Amino Acids in Autophagy: Regulation and Function Amino Acids in Nutrition and Health. Springer.
Shiber, J. R., Fontane, E., and Adewale, A. (2010). Stroke Registry: Hemorrhagic vs Ischemic Strokes. Am. J. Emerg. Med. 28, 331–333. doi:10.1016/j.ajem.2008.10.026
Shirley, R., Ord, E. N., and Work, L. M. (2014). Oxidative Stress and the Use of Antioxidants in Stroke. Antioxidants 3, 472–501. doi:10.3390/antiox3030472
Shotar, E., Amouyal, C., Jacquens, A., Mathon, B., Boulouis, G., Monneret, D., et al. (2019). S100b Serum Elevation Predicts In-Hospital Mortality after Brain Arteriovenous Malformation Rupture. Stroke 50, 1250–1253. doi:10.1161/STROKEAHA.119.025033
Singh, P., and Singh, R. K. (2019). The Advantage of Higher Statin Concentrations to Improve Outlook after Ischemic Stroke.
Soeda, S., Ochiai, T., Paopong, L., Tanaka, H., Shoyama, Y., and Shimeno, H. (2001). Crocin Suppresses Tumor Necrosis Factor-α-Induced Cell Death of Neuronally Differentiated Pc-12 Cells. Life Sci. 69, 2887–2898. doi:10.1016/s0024-3205(01)01357-1
Soeda, S., Ochiai, T., Shimeno, H., Saito, H., Abe, K., Tanaka, H., et al. (2007). Pharmacological Activities of Crocin in Saffron. J. Nat. Med. 61, 102–111. doi:10.1007/s11418-006-0120-9
Sommano, S. R., Suppakittpaisarn, P., Sringarm, K., Junmahasathien, T., and Ruksiriwanich, W. (2020). Recovery of Crocins from Floral Tissue of Gardenia Jasminoides Ellis. Front. Nutr. 7, 106. doi:10.3389/fnut.2020.00106
Song, G., Liu, J., Wang, Q., Wang, D., Chu, B., Li, L., et al. (2022). Layer-by-layer Self-Assembly of Hollow Dextran Sulfate/chitosan-Coated Zein Nanoparticles Loaded with Crocin: Fabrication, Structural Characterization and Potential Biological Fate. Food Hydrocoll. 125, 107420. doi:10.1016/j.foodhyd.2021.107420
Song, Y.-N., Wang, Y., Zheng, Y.-H., Liu, T.-L., and Zhang, C. (2021a). Crocins: A Comprehensive Review of Structural Characteristics, Pharmacokinetics and Therapeutic Effects. Amsterdam, Netherlands: Elsevier. 104969. doi:10.1016/j.fitote.2021.104969
Song, Y.-N., Wang, Y., Zheng, Y.-H., Liu, T.-L., and Zhang, C. (2021b). Crocins: A Comprehensive Review of Structural Characteristics, Pharmacokinetics and Therapeutic Effects. Fitoterapia 153, 104969. doi:10.1016/j.fitote.2021.104969
Steiner, G. Z., Bensoussan, A., Liu, J., Hohenberg, M. I., and Chang, D. H. (2018). Study Protocol for a Randomised, Double-Blind, Placebo-Controlled 12-week Pilot Phase Ii Trial of Sailuotong (Slt) for Cognitive Function in Older Adults with Mild Cognitive Impairment. Trials 19, 1–10. doi:10.1186/s13063-018-2912-0
Streinu-Cercel, A., Sandulescu, O., Miron, V. D., Oana, A.-A., Motoi, M. M., and Galloway, C. D. (2021). Trans Sodium Crocetinate (Tsc) to Improve Oxygenation in Covid-19. London: Cold Spring Harbor Laboratory.
SU, X.-T., Wang, L., Ma, S.-M., Cao, Y., Yang, N.-N., Lin, L.-L., et al. (2020). Mechanisms of Acupuncture in the Regulation of Oxidative Stress in Treating Ischemic Stroke. Oxidative Med. Cell. Longev. doi:10.1155/2020/7875396
Suchareau, M., Bordes, A., and Lemée, L. (2021). Improved Quantification Method of Crocins in Saffron Extract Using Hplc-Dad after Qualification by Hplc-Dad-Ms. Food Chem., 130199. doi:10.1016/j.foodchem.2021.130199
Sugawara, T., and Chan, P. H. (2003). Reactive Oxygen Radicals and Pathogenesis of Neuronal Death after Cerebral Ischemia. Antioxidants Redox Signal. 5, 597–607. doi:10.1089/152308603770310266
Sun, L., Zhang, Y., Liu, E., Ma, Q., Anatol, M., Han, H., et al. (2019). The Roles of Astrocyte in the Brain Pathologies Following Ischemic Stroke. Brain Inj. 33, 712–716. doi:10.1080/02699052.2018.1531311
Sun, P.-D., Wang, J.-X., Fang, Z.-K., and Tang, D.-D. (2012). Synthesis of Crocetin Dimethyl Ester with Wittig and Wittig-Horner Reaction. Beijing, China: Chemical World, 06.
TAPEINOS, C., Battaglini, M., and Ciofani, G. (2017). Advances in the Design of Solid Lipid Nanoparticles and Nanostructured Lipid Carriers for Targeting Brain Diseases. J. Control. Release 264, 306–332. doi:10.1016/j.jconrel.2017.08.033
Teo, L., and Bourne, J. A. (2018). Current Opinion on a Role of the Astrocytes in Neuroprotection. Neural Regen. Res. 13, 797. doi:10.4103/1673-5374.232466
Tseng, T.-H., Chu, C.-Y., Huang, J.-M., Shiow, S.-J., and Wang, C.-J. (1995). Crocetin Protects against Oxidative Damage in Rat Primary Hepatocytes. Cancer Lett. 97, 61–67. doi:10.1016/0304-3835(95)03964-x
Ulbricht, C., Conquer, J., Costa, D., Hollands, W., Iannuzzi, C., Isaac, R., et al. 2011. An Evidence-Based Systematic Review of Saffron (Crocus Sativus) by the Natural Standard Research Collaboration. 8, 58–114. doi:10.3109/19390211.2011.547666
Ullegaddi, R., Powers, H. J., and Gariballa, S. E. (2006). Antioxidant Supplementation with or without B‐group Vitamins after Acute Ischemic Stroke: A Randomized Controlled Trial. J. Parenter. Enter. Nutr. 30, 108–114. doi:10.1177/0148607106030002108
Umigai, N., Murakami, K., Ulit, M., Antonio, L., Shirotori, M., Morikawa, H., et al. (2011). The Pharmacokinetic Profile of Crocetin in Healthy Adult Human Volunteers after a Single Oral Administration. Phytomedicine 18, 575–578. doi:10.1016/j.phymed.2010.10.019
Umigai, N., Takeda, R., and Mori, A. (2018). Effect of Crocetin on Quality of Sleep: a Randomized, Double-Blind, Placebo-Controlled, Crossover Study. Complementary Ther. Med. 41, 47–51. doi:10.1016/j.ctim.2018.09.003
Vahdati Hassani, F., Naseri, V., Razavi, B. M., Mehri, S., Abnous, K., and Hosseinzadeh, H. (2014). Antidepressant Effects of Crocin and its Effects on Transcript and Protein Levels of Creb, Bdnf, and Vgf in Rat hippocampus. Daru J. Fac. Pharm. Tehran Univ. Med. Sci. 22, 16. doi:10.1186/2008-2231-22-16
Vakili, A., Einali, M. R., and Bandegi, A. R. (2014). Protective Effect of Crocin against Cerebral Ischemia in a Dose-dependent Manner in a Rat Model of Ischemic Stroke. J. Stroke Cerebrovasc. Dis. 23, 106–113. doi:10.1016/j.jstrokecerebrovasdis.2012.10.008
Veisi, A., Akbari, G., Mard, S. A., Badfar, G., Zarezade, V., and Mirshekar, M. A. (2020). Role of Crocin in Several Cancer Cell Lines: An Updated Review. Iran. J. Basic Med. Sci. 23, 3. doi:10.22038/IJBMS.2019.37821.8995
Venketasubramanian, N. (2021). Stroke Epidemiology in Oceania: A Review. Neuroepidemiology, 1–10. doi:10.1159/000512972
Verma, R. S., and Middha, D. (2010). Analysis of Saffron (Crocus Sativus L. Stigma) Components by Lc–Ms–Ms. Chromatographia 71, 117–123. doi:10.1365/s10337-009-1398-z
Wang, C., Cai, X., Hu, W., Li, Z., Kong, F., Chen, X., et al. (2019). Investigation of the Neuroprotective Effects of Crocin via Antioxidant Activities in Ht22 Cells and in Mice with Alzheimer's Disease. Int. J. Mol. Med. 43, 956–966. doi:10.3892/ijmm.2018.4032
Wang, C., Hwang, L. S., and Lin, J. (1984). Reversible Hepatic Black Pigmentation and Enzyme Alteration Induced by Prolonged Feeding of High Dose of Crocin Dyes in Rats. Proc. Natl. Sci. Counc. Repub. China. Part B, Life Sci. 8, 246–253.
Wang, Y., Han, T., Zhu, Y., Zheng, C.-J., Ming, Q.-L., Rahman, K., et al. (2009). Antidepressant Properties of Bioactive Fractions from the Extract of Crocus Sativus L. J. Nat. Med. 64, 24. doi:10.1007/s11418-009-0360-6
Wang, Y., Yoshimura, R., Manabe, H., Schretter, C., Clarke, R., Cai, Y., et al. (2014). Trans-sodium Crocetinate Improves Outcomes in Rodent Models of Occlusive and Hemorrhagic Stroke. Brain Res. 1583, 245–254. doi:10.1016/j.brainres.2014.08.013
Wani, A., Al Rihani, S. B., Sharma, A., Weadick, B., Govindarajan, R., Khan, S. U., et al. (2021). Crocetin Promotes Clearance of Amyloid-β by Inducing Autophagy via the Stk11/lkb1-Mediated Ampk Pathway. Autophagy, 1–20. doi:10.1080/15548627.2021.1872187
Waziry, R., Chibnik, L. B., Bos, D., Ikram, M. K., and Hofman, A. (2020). Risk of Hemorrhagic and Ischemic Stroke in Patients with Alzheimer Disease: A Synthesis of the Literature. Neurology 94, 265–272. doi:10.1212/WNL.0000000000008924
Wechsler, L. R. (2020). Statins and Stroke—It’s Complicated. England, United Kingdom: Mass Medical Soc.
Williams, E. I., Betterton, R. D., Davis, T. P., and Ronaldson, P. T. (2020). Transporter-mediated Delivery of Small Molecule Drugs to the Brain: A Critical Mechanism that Can Advance Therapeutic Development for Ischemic Stroke. Pharmaceutics 12, 154. doi:10.3390/pharmaceutics12020154
Wishart, D. S., Feunang, Y. D., Marcu, A., Guo, A. C., Liang, K., Vázquez-Fresno, R., et al. (2018). Hmdb 4.0: The Human Metabolome Database for 2018. Nucleic Acids Res. 46, d608–d617. doi:10.1093/nar/gkx1089
Wong, K. H., Xie, Y., Huang, X., Kadota, K., Yao, X.-S., Yu, Y., et al. (2020). Delivering Crocetin across the Blood-Brain Barrier by Using γ-cyclodextrin to Treat Alzheimer’s Disease. Sci. Rep. 10, 1–12. doi:10.1038/s41598-020-60293-y
Wu, L., Xiong, X., Wu, X., Ye, Y., Jian, Z., Zhi, Z., et al. (2020). Targeting Oxidative Stress and Inflammation to Prevent Ischemia-Reperfusion Injury. Front. Mol. Neurosci. 13, 28. doi:10.3389/fnmol.2020.00028
Xi, L., Qian, Z., Du, P., and Fu, J. (2007). Pharmacokinetic Properties of Crocin (Crocetin Digentiobiose Ester) Following Oral Administration in Rats. Phytomedicine 14, 633–636. doi:10.1016/j.phymed.2006.11.028
Xi, L., and Qian, Z. (2006). Pharmacological Properties of Crocetin and Crocin (Digentiobiosyl Ester of Crocetin) from Saffron. Nat. Product. Commun. 1, 1934578x0600100112. doi:10.1177/1934578x0600100112
Xiao, Q., Shu, R., Wu, C., Tong, Y., Xiong, Z., Zhou, J., et al. (2020). Crocin-i Alleviates the Depression-like Behaviors Probably via Modulating “Microbiota-gut-brain” Axis in Mice Exposed to Chronic Restraint Stress. J. Affect. Disord. 276, 476–486. doi:10.1016/j.jad.2020.07.041
Xiao, Q., Xiong, Z., Yu, C., Zhou, J., Shen, Q., Wang, L., et al. (2019). Antidepressant Activity of Crocin-I Is Associated with Amelioration of Neuroinflammation and Attenuates Oxidative Damage Induced by Corticosterone in Mice. Physiology Behav. 212, 112699. doi:10.1016/j.physbeh.2019.112699
Xie, C.-L., Li, J.-H., Wang, W.-W., Zheng, G.-Q., and Wang, L.-X. (2015a). Neuroprotective Effect of Ginsenoside-Rg1 on Cerebral Ischemia/reperfusion Injury in Rats by Downregulating Protease-Activated Receptor-1 Expression. Life Sci. 121, 145–151. doi:10.1016/j.lfs.2014.12.002
Xie, C.-L., Wang, W.-W., Xue, X.-D., Zhang, S.-F., Gan, J., and Liu, Z.-G. (2015b). A Systematic Review and Meta-Analysis of Ginsenoside-Rg1 (G-rg1) in Experimental Ischemic Stroke. Sci. Rep. 5, 7790. doi:10.1038/srep07790
Xu, G.-L., Li, G., Ma, H.-P., Zhong, H., Liu, F., and Ao, G.-Z. (2009). Preventive Effect of Crocin in Inflamed Animals and in Lps-Challenged Raw 264.7 Cells. J. Agric. Food Chem. 57, 8325–8330. doi:10.1021/jf901752f
Yamashita, S. I., Umigai, N., Kakinuma, T., and Takara, T. (2018). Safety Evaluation of Excessive Intake of Crocetin in Healthy Adult Volunteers-A Randomized, Double-Blind, Placebo-Controlled, Parallel-Group Comparison Trial. Pharmacol. Treat. 46, 393–401.
Yang, C., Fan, F., Sawmiller, D., Tan, J., Wang, Q., and Xiang, Y. (2019). C1q/tnf‐related Protein 9: A Novel Therapeutic Target in Ischemic Stroke? J. Neurosci. Res. 97, 128–136. doi:10.1002/jnr.24353
Yang, L., and Dong, X. (2017). Inhibition of Inflammatory Response by Crocin Attenuates Hemorrhagic Shock-Induced Organ Damages in Rats. J. Interferon & Cytokine Res. 37, 295–302. doi:10.1089/jir.2016.0137
Yang, W. Z. A. L. (2020). Study on the Protective Effect of Saffron on Organ Damage Caused by Hemorrhagic Shock in Rats. Modern Journal of Integrated. Tradit. Chin. West. Med. 29, 1742–1746.
Yang, Y., Han, K., Park, S. H., Kim, M. K., Yoon, K.-H., and Lee, S.-H. (2021). High-density Lipoprotein Cholesterol and the Risk of Myocardial Infarction, Stroke, and Cause-Specific Mortality: A Nationwide Cohort Study in Korea. J. Lipid Atheroscler. 10, 74. doi:10.12997/jla.2021.10.1.74
Yang, Y., and Rosenberg, G. A. (2015). Matrix Metalloproteinases as Therapeutic Targets for Stroke. Brain Res. 1623, 30–38. doi:10.1016/j.brainres.2015.04.024
YE, F., Garton, H. J., Hua, Y., Keep, R. F., and Xi, G. (2021). The Role of Thrombin in Brain Injury after Hemorrhagic and Ischemic Stroke. Transl. Stroke Res. 12, 496–511. doi:10.1007/s12975-020-00855-4
Yoo, A. J., and Andersson, T. (2017). Thrombectomy in Acute Ischemic Stroke: Challenges to Procedural Success. J. Stroke 19, 121. doi:10.5853/jos.2017.00752
Yoshino, F., Yoshida, A., Umigai, N., Kubo, K., and Masaichi, C.-I. L. (2011). Crocetin Reduces the Oxidative Stress Induced Reactive Oxygen Species in the Stroke-Prone Spontaneously Hypertensive Rats (Shrsps) Brain. J. Clin. Biochem. Nutr. 49, 182–187. doi:10.3164/jcbn.11-01
Yousefi, F., Arab, F. L., Rastin, M., Tabasi, N. S., Nikkhah, K., and Mahmoudi, M. (2021). Comparative Assessment of Immunomodulatory, Proliferative, and Antioxidant Activities of Crocin and Crocetin on Mesenchymal Stem Cells. J. Cell. Biochem. 122, 29–42. doi:10.1002/jcb.29826
Yousefsani, B. S., Mehri, S., Pourahmad, J., and Hosseinzadeh, H. (2021). Protective Effect of Crocin against Mitochondrial Damage and Memory Deficit Induced by Beta-Amyloid in the Hippocampus of Rats. Iran. J. Pharm. Res. 20, 79–94. doi:10.22037/ijpr.2020.112206.13604
Yuan, Y., Shan, X., Men, W., Zhai, H., Qiao, X., Geng, L., et al. (2020). The Effect of Crocin on Memory, Hippocampal Acetylcholine Level, and Apoptosis in a Rat Model of Cerebral Ischemia. Biomed. Pharmacother. 130, 110543. doi:10.1016/j.biopha.2020.110543
Zeka, K., Marrazzo, P., Micucci, M., Ruparelia, K. C., Arroo, R. R., Macchiarelli, G., et al. (2020). Activity of Antioxidants from Crocus Sativus L. Petals: Potential Preventive Effects Towards Cardiovascular System. Antioxidants 9, 1102. doi:10.3390/antiox9111102
Zeka, K., Ruparelia, K. C., Continenza, M. A., Stagos, D., Vegliò, F., and Arroo, R. R. (2015). Petals of Crocus Sativus L. As a Potential Source of the Antioxidants Crocin and Kaempferol. Fitoterapia 107, 128–134. doi:10.1016/j.fitote.2015.05.014
Zeng, C., Li, H., Fan, Z., Zhong, L., Guo, Z., Guo, Y., et al. (2016). Crocin-elicited Autophagy Rescues Myocardial Ischemia/reperfusion Injury via Paradoxical Mechanisms. Am. J. Chin. Med. 44, 515–530. doi:10.1142/S0192415X16500282
Zhang, H., Zeng, Y., Yan, F., Chen, F., Zhang, X., Liu, M., et al. (2004). Semi-preparative Isolation of Crocins from Saffron (Crocus Sativus l.). Chromatographia 59, 691–696. doi:10.1365/s10337-004-0311-z
Zhang, H., Zhang, X., Yan, F., Zeng, Y., and Chen, F. (2001). Separation and Preparation of Crocins in Saffron by Low-Pressure Liquid Chromatography. Chin. J. Anal. Chem.
Zhang, X., Fan, Z., and Jin, T. (2017a). Crocin Protects against Cerebral-Ischemia-Induced Damage in Aged Rats through Maintaining the Integrity of Blood-Brain Barrier. Restor. Neurology Neurosci. 35, 65–75. doi:10.3233/RNN-160696
Zhang, X., Fan, Z., and Ting, J. (2017b). Crocin Protects against Cerebral- Ischemia-Induced Damage in Aged Rats through Maintaining the Integrity of Blood-Brain Barrier. Restor. Neurology Neurosci. 35, 65–75. doi:10.3233/RNN-160696
Zhang, Y., Geng, J., Hong, Y., Jiao, L., Li, S., Sun, R., et al. (2019). Orally Administered Crocin Protects against Cerebral Ischemia/reperfusion Injury through the Metabolic Transformation of Crocetin by Gut Microbiota. Front. Pharmacol. 10, 440. doi:10.3389/fphar.2019.00440
Zhang, Y., Liu, J., Lin, L., and Li, L. (2012). Pharmacokinetics of Crocin-1 after Oral Administration in Rats. Chin. Pharm. J. 47, 136–140.
Zheng, T., Jiang, H., Jin, R., Zhao, Y., Bai, Y., Xu, H., et al. (2019). Ginsenoside Rg1 Attenuates Protein Aggregation and Inflammatory Response Following Cerebral Ischemia and Reperfusion Injury. Eur. J. Pharmacol. 853, 65–73. doi:10.1016/j.ejphar.2019.02.018
Zheng, Y.-Q., Liu, J.-X., Li, X.-Z., and Xu, L. (2010). Effects and Mechanism of Weinaokang (维脑康) on Reperfusioninduced Vascular Injury to Cerebral Microvessels after Global Cerebral Ischemia. Chin. J. Integr. Med. 16, 145–150. doi:10.1007/s11655-010-0145-5
Zheng, Y.-Q., Liu, J.-X., Wang, J.-N., and Xu, L. (2007). Effects of Crocin on Reperfusion-Induced Oxidative/nitrative Injury to Cerebral Microvessels after Global Cerebral Ischemia. Brain Res. 1138, 86–94. doi:10.1016/j.brainres.2006.12.064
Zhong, K., Wang, R.-X., Qian, X.-D., Yu, P., Zhu, X.-Y., Zhang, Q., et al. (2020). Neuroprotective Effects of Saffron on the Late Cerebral Ischemia Injury through Inhibiting Astrogliosis and Glial Scar Formation in Rats. Biomed. Pharmacother. 126, 110041. doi:10.1016/j.biopha.2020.110041
Zhou, B., Perel, P., Mensah, G. A., and Ezzati, M. (2021). Global Epidemiology, Health Burden and Effective Interventions for Elevated Blood Pressure and Hypertension. Nat. Rev. Cardiol., 1–18. doi:10.1038/s41569-021-00559-8
Zhou, Y., Li, H.-Q., Lu, L., Fu, D.-L., Liu, A.-J., Li, J.-H., et al. (2014). Ginsenoside Rg1 Provides Neuroprotection against Blood Brain Barrier Disruption and Neurological Injury in a Rat Model of Cerebral Ischemia/reperfusion through Downregulation of Aquaporin 4 Expression. Phytomedicine 21, 998–1003. doi:10.1016/j.phymed.2013.12.005
Zhu, A., Lao, C., Wang, Z., Chen, Y., and Bai, C. (2019a). Characterization of Crocetin-Monoglucuronide as a Neuron-Protective Metabolite of Crocin-1. Mol. Nutr. Food Res. 63, 1900024. doi:10.1002/mnfr.201900024
Zhu, A., Lao, C., Wang, Z., Chen, Y., and Bai, C. (2019b). Characterization of Crocetin‐monoglucuronide as a Neuron‐protective Metabolite of Crocin‐1. Mol. Nutr. Food Res. 63, 1900024. doi:10.1002/mnfr.201900024
Zhu, Y. (2010). Clinical Study on Crocin Injection in the Treatment of Arteriosclerotic Thrombotic Cerebral Infarction (Stroke Recovery Period-Blood Stasis Blocking Collaterals Syndrome). Jilin, China: Changchun University of Traditional Chinese Medicine. Master Thesis (in chinese).
Keywords: ischemic stroke, Crocins, saffron, neuroinflammation, antioxidant, molecular targets, toxicity and safety, clinical trial
Citation: Shahbaz K, Chang D, Zhou X, Low M, Seto SW and Li CG (2022) Crocins for Ischemic Stroke: A Review of Current Evidence. Front. Pharmacol. 13:825842. doi: 10.3389/fphar.2022.825842
Received: 30 November 2021; Accepted: 28 April 2022;
Published: 05 August 2022.
Edited by:
Aiping Lu, Hong Kong Baptist University, Hong Kong SAR, ChinaReviewed by:
Seyed Zachariah Moradi, Kermanshah University of Medical Sciences, IranNeda Roshanravan, Tabriz University of Medical Sciences, Iran
Copyright © 2022 Shahbaz, Chang, Zhou, Low, Seto and Li. This is an open-access article distributed under the terms of the Creative Commons Attribution License (CC BY). The use, distribution or reproduction in other forums is permitted, provided the original author(s) and the copyright owner(s) are credited and that the original publication in this journal is cited, in accordance with accepted academic practice. No use, distribution or reproduction is permitted which does not comply with these terms.
*Correspondence: Kiran Shahbaz, k.shahbaz@westernsydney.edu.au; Chung Guang Li, c.li@westernsydney.edu.au