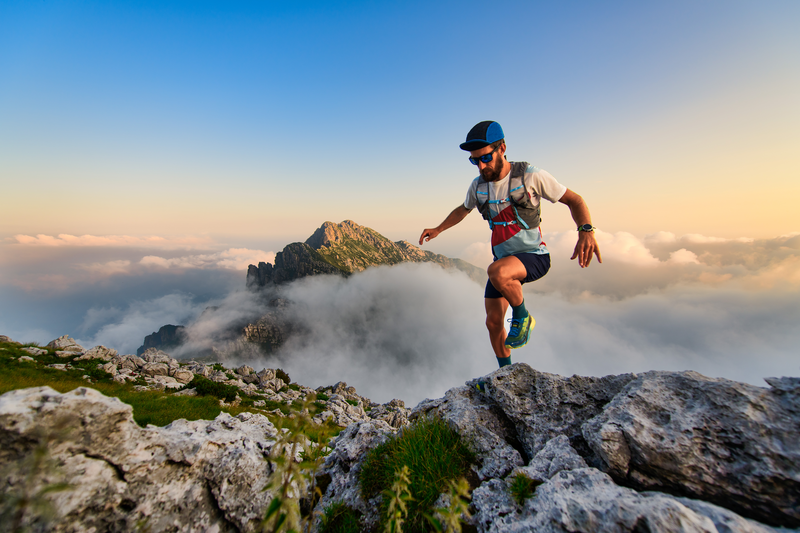
95% of researchers rate our articles as excellent or good
Learn more about the work of our research integrity team to safeguard the quality of each article we publish.
Find out more
REVIEW article
Front. Pharmacol. , 24 June 2022
Sec. Pharmacology of Anti-Cancer Drugs
Volume 13 - 2022 | https://doi.org/10.3389/fphar.2022.814971
This article is part of the Research Topic Current Aspects in Chemopreventive Strategies, Volume II View all 13 articles
Objective (s): In this mini-review, we aimed to discuss the Wnt/β-catenin signaling pathway modulation in triple-negative breast cancer, particularly the contribution of lncRNAs and miRNAs in its regulation and their possible entwining role in breast cancer pathogenesis, proliferation, migration, or malignancy.
Background: Malignant tumor formation is very high for breast cancer in women and is a leading cause of death all over the globe. Among breast cancer subtypes, triple-negative breast cancer is rife in premenopausal women, most invasive, and prone to metastasis. Complex pathways are involved in this cancer’s pathogenesis, advancement, and malignancy, including the Wnt/β-catenin signaling pathway. This pathway is conserved among vertebrates and is necessary for sustaining cell homeostasis. It is regulated by several elements such as transcription factors, enhancers, non-coding RNAs (lncRNAs and miRNAs), etc.
Methods: We evaluated lncRNAs and miRNAs differentially expressed in triple-negative breast cancer (TNBC) from the cDNA microarray data set literature survey. Using in silico analyses combined with a review of the current literature, we anticipated identifying lncRNAs and miRNAs that might modulate the Wnt/β-catenin signaling pathway.
Result: The miRNAs and lncRNAs specific to triple-negative breast cancer have been identified based on literature and database searches. Tumorigenesis, metastasis, and EMT were all given special attention. Apart from cross-talk being essential for TNBC tumorigenesis and treatment outcomes, our results indicated eight upregulated and seven downregulated miRNAs and 19 upregulated and three downregulated lncRNAs that can be used as predictive or diagnostic markers. This consolidated information could be useful in the clinic and provide a combined literature resource for TNBC researchers working on the Wnt/β-catenin miRNA/lncRNA axis.
Conclusion: In conclusion, because the Wnt pathway and miRNAs/lncRNAs can modulate TNBC, their intertwinement results in a cascade of complex reactions that affect TNBC and related processes. Their function in TNBC pathogenesis has been highlighted in molecular processes underlying the disease progression.
Breast cancer represents the most common type of cancer worldwide, with high morbidity and mortality rates, (Agustsson et al., 2020). Breast cancer shows high heterogeneity, which impacts the clinical course of the disease. Differential expression profiles among patients lead to tumor tissue heterogeneity, resulting in variations in malignant behavior, prognosis, and responsiveness to standard therapies (Padmanaban et al., 2019). This cancer is prevalent in women and is the first leading cause of cancerous death in women. Breast cancer has been progressively increasing in most nations (Ferlay et al., 2015). According to WHO data (https://www.who.int/news-room/fact-sheets/detail/breast-cancer), 2.3 million women were diagnosed with breast cancer in 2020, with about 685,000 fatalities. This year, in the United States alone, around 281,550 cases of invasive and 49,290 cases of non-invasive breast cancer cases were diagnosed in women, according to the American Cancer Society. Most deaths occur due to metastasis instead of the primary tumor in breast cancer (Geng et al., 2014). Metastasis is linked with stem cells, which have characteristics such as self-renewal, differentiation ability, drug resistance, etc. (Phi et al., 2018). These properties favor aggressive behavior in breast cancer stem cells, leading to recurrent and aggressive tumors on and away from the primary site. Upregulation of Wnt/β-catenin signaling has been observed in triple-negative breast cancer (TNBC)/basal-like cancer when collated with other breast cancer subtypes (luminal A, B, and HER2 positive) or normal tissues (Gangrade et al., 2018).
TNBC can be classified into at least six distinct subtypes with differences in clinical behavior and treatment response. TNBC is highly invasive and, due to the non-expression of estrogen receptor, progesterone receptor, or HER-2, it has poor prognosis, high metastatic potential, and is disposed to relapse (Yin et al., 2020). TNBCs are more common in younger and obese women, with premenopausal African American women having the highest prevalence. BRCA1 and BRCA2 gene mutations are identified in approximately 20% of TNBC patients. P53 and Rb1 mutations are also quite common in TNBC tumors (van Barele et al., 2021). Differentially expressed ncRNAs have been found in a variety of human cancers, including breast cancer. Several lncRNA molecules have been linked to tumorigenesis, and their differential expression could constitute a potential new category of biomarkers. The lncRNA HOTAIR (HOX transcript antisense intergenic RNA) was associated with the polycomb repressive complex 2 (PRC2) and the histone demethylation enzyme LSD1 (lysine-specific demethylase 1) in cancer cells, resulting in epigenetic changes that promote tumor development and metastasis (Rodrigues de Bastos and Nagai, 2020). Other circulating lncRNAs, including MALAT1, GAS5, H19, and MEG3, have also been linked to survival and treatment response. LncRNAs have opened up a new field of study for researchers all over the world, and these molecules have been assigned major roles that may have a direct impact on patient survival and therapeutic responsiveness (Gupta et al., 2010). The SPARC gene (secreted protein acidic and rich in cysteine, also known as osteonectin or basement-membrane protein 40) encodes a 32-kDa matricellular glycoprotein involved in a variety of biological activities, including differentiation, proliferation, migration, and adhesion (Zhang et al., 2019).
The canonical Wnt/β-catenin signaling pathway contributes to instigation (Figure 1), differentiation, and proliferation of TNBC cells (Zhang et al., 2018), leading to primary tumor formation (Xu et al., 2015), cellular transition of the epithelial-to-mesenchymal (EMT) state, and metastasis (Pohl et al., 2017). Chemoresistance has also been linked with Wnt/β-catenin signaling, with impaired pathways leading to drug-resistant TNBC (Merikhian et al., 2021). This is due to the synergistic contact between the Wnt target gene c-MYC and HIF-1α. This dual gene interaction diminishes cancer cell response to the given drugs. However, knockdown of β-catenin has been reported to cause TNBC cells to respond to doxorubicin or cisplatin (Xu et al., 2015).
FIGURE 1. Wnt/β-catenin pathway in TNBC instigation. Figure adapted from the KEGG pathway (https://www.genome.jp/pathway/map05224).
This is why the study of this pathway is essential in TNBC, and a comprehensive updated literature review was undertaken to integrate information regarding the Wnt/β-catenin signaling pathway and TNBC.
The Wnt pathway is tangled with low-density lipoprotein receptor-related protein5/6 (LRP5/6) and frizzled (FZD) receptors for its activation (King et al., 2012). If Wnts are insufficient or non-functional, β-catenin pools with a tetrad of proteins (axin, casein kinase 1 (CK1), adenomatous polyposis coli (APC) tumor suppressor, and glycogen synthase kinase-3b (GSK3b). Phosphorylation (by CK1 and GSK3b) is followed by ubiquitination and ultimate degradation of β-catenin (26S proteasome). Conversely, in the presence of the Wnt signal, β-catenin attaches to FZD and LRP5/6 receptors, leading to inhibition of GSK3b and stabilization of cytosolic β-catenin. This β-catenin is then shifted to the nucleus. It associates with T-cell factor/lymphoid-enhancing factor (TCF/LEF) to incite the downstream expression of cell development and cell cycle control genes (MacDonald et al., 2009). Secreted proteins regulate this signaling at the cell surface, where the central modulators are Wnt and R-spondin (Rspo). Inhibitors include Wnt Inhibitory Factor 1 (WIF1), Dickkopf (Dkk), soluble Frizzled-related protein (sFRP), and sclerostin (SOST) (Yao et al., 2011; Danieau et al., 2019). Abnormal Wnt signaling has been implicated in TNBC tumorigenesis (Xu et al., 2015; Mohammadi Yeganeh et al., 2017), stemness, metastasis, and prognosis (Ryu et al., 2020). Dey et al. (2013) identified that patients with dysregulated Wnt/β-catenin signaling had a higher chance of lung and brain metastases. Dysregulation of the canonical pathway is responsible for metastasis in more than half of breast cancer patients as the nucleolar β-catenin level is elevated. However, mutations in the relevant genes are uncommon (Yu et al., 2019). It implies that the role of the β-catenin is indispensable to the Wnt signaling in TNBC advancement, with the dissemination of non-phosphorylated cytoplasmic β-catenin to the nucleus having an imperative role in TNBC metastasis (Breuer et al., 2019; Satriyo et al., 2019).
Green et al. (2013) discovered that Wnt ligands caused enhanced transcription in the majority of TNBC cell lines, while Xu et al. (2015) reported that nuclear accumulation of β-catenin is linked with TNBC characteristics. The role of the key modulators of this pathway in TNBC includes the action of FZD receptors, LRP5/6, Receptor Tyrosine Kinase–Like Orphan Receptors (RORs), and Dead Box Proteins (DDX3 and DDX5) (Pohl et al., 2017). LRP5/6 is crucial in mammary development and is allied with tumorigenesis (Goel et al., 2012). Overexpression of LRP5/6 has been seen in TNBC (Maubant et al., 2018). LRP6 has also been linked with migration and invasion of cells (Ma et al., 2017). Elevated FZD7 expression in TNBC also promotes tumorigenesis (Yang et al., 2011) via transformation-related protein 63 (p63) (Chakrabarti et al., 2014), while FZD8-driven Wnt signaling mediated by c-Myc overexpression drives chemoresistance (Yin et al., 2013). Dead box proteins DDX3 and DDX5 have shown increased EMT in TNBC. DDX3 is associated with increased motility and invasiveness. In comparison, DDX5 is linked with tumorigenesis and cancer cell progression (Moore et al., 2010; Wang et al., 2012; Guturi et al., 2014; Pohl et al., 2017).
Cancer stem cells (CSCs) or cancer stem-like cells within the tumor, being less responsive to environmental stop signals, are responsible for cancer progression, metastasis, chemoresistance, and hence, cancer relapse (Clarke, 2019). They differ from other cancer cells as they use mitochondrial reactive oxygen species (ROS) for respiration, which means higher oxygen consumption, increased mitochondrial mass, and high resistance to DNA damage (Peiris-Pagès et al., 2018; Scatena et al., 2018). Abnormal Wnt/β-catenin signaling is linked with CSC formation and, hence, tumorigenesis, stemness, migration, and chemoresistance in TNBC (Alraouji et al., 2020). Upregulation of β-catenin triggers the CSC phenotype of TNBC, and repression by cadherin leads to suppression of this phenotype (Satriyo et al., 2019). Jang et al. (2015) observed that Wnt/β-catenin pathway genes (WNT1, FZD1, TCF4, and LEF1) are upregulated in CSC-enriched mammospheres in breast cancer, while signaling proteins (LEF1, TCF4, and β-catenin) were increased in high CSC activity, depicting cell fraction, compared to that with low CSC activity. However, recently, Brilliant et al. (2019) reported 11% of Wnt signaling expression in high vs. 33% in the low content of cancer stem cells. In contrast, some researchers have reported inhibiting TNBC CSCs by drugs targeting the Wnt/β-catenin pathway (like hydroxytyrosol) (Cruz-Lozano et al., 2019). Others have reported that β-catenin is also responsible for drug resistance (e.g., doxorubicin resistance) in TNBC CSCs (Xu et al., 2015).
According to recent research, more than 90% of the transcripts in the human genome may not be able to code for proteins (Wilusz et al., 2009) but regulate the expression of nearby genes (He and Hannon, 2004; Trzybulska et al., 2018). They are categorized according to their size and function (Trzybulska et al., 2018), with microRNAs (miRNAs) being 19–24 nucleotides long and long non-coding RNAs (lncRNAs) being >200 nucleotides in length. There are other non-coding RNAs such as piwiRNAs, free circulating RNAs, and snoRNAs, but our focus will be on miRNAs and lncRNAs in this review. Long non-coding RNAs are abundant in human cells and play critical roles in a range of biological processes, including cell cycle regulation (Lu et al., 2016), genomic expression (Ballantyne et al., 2016), and cell differentiation (Chen et al., 2016). Increasing evidence has recently indicated that abnormal lncRNA expression is linked with various tumor forms (Mendell, 2016). Researchers have proved that miRNA dysregulation also leads to human cancer via different mechanisms (Karimzadeh et al., 2021), including altered epigenetics (Arif et al., 2020) and abnormal transcriptional control (Müller et al., 2019; Ali Syeda et al., 2020). Apart from performing as oncogenes, where they support proliferative signaling (Miao et al., 2017), invasion (Chen et al., 2017), repelling cell death, eluding progression suppressors, and metastasis, they act as tumor suppressors too (Hong et al., 2019). This has led to their demarcation as potential biomarkers of cancer (Gai et al., 2018; Dai et al., 2019).
miRNA expression in BCSCs and cancer cells signals that they are crucial for promoting characteristics such as stemness and tumorigenesis. Piasecka et al. (2018) reviewed 121 articles demonstrating the role of miRNAs in TNBC. After scanning a plethora of literature, it was revealed that the miRNAs not only serve as predictive markers of TNBC but also have prognostic clinical utility. They assist in attaining CSC properties in TNBC and EMT. Since these properties are conditions for metastasis, miRNAs play an essential role in cell transition to the metastasis stage. Several miRNAs, which are differently expressed in TNBC cells compared to normal cells and entangled with the Wnt/β-catenin pathway, have also been identified (Avery-Kiejda et al., 2014; Goh et al., 2016; Pohl et al., 2017).
More than 70 miRNAs (previously implicated in BC) have shown differential expression in TNBC, targeting 16 genes from the Wnt pathway, causing their increased expression in TNBC metastasis. The impacting miRNAs comprised the miR-17-92 oncogenic cluster members and the miR-200 family, revealing that most miRNAs are not mainly associated with a cancer subtype (Pohl et al., 2017). miR-340 alters CTNNB1, MYC, and ROCK1 gene expression of the Wnt pathway and causes apoptosis in TNBC (Mohammadi Yeganeh et al., 2017). miR-203 expresses higher methylation and is downregulated in TNBC, along with downregulation of the Wnt pathway (Taube et al., 2013). Telonis and Rigoutsos (2018) have identified miR-200c, miR-21, miR-17/92 cluster, and the miR-183/96/182 cluster to be upregulated in TNBC. Wang et al. (2019) demonstrated that miR-125, MiR, MiR-145, MiR-381, MiR-136, and MiR-4324 are associated with the poorest prognosis in TNBC patients. Thus, miRNAs play a critical role in TNBC and are intertwined with the Wnt pathway gene regulation, making them essential players in Wnt-mediated TNBC progression, prognosis, and other outcomes. Previously reported miRNAs with a role in TNBC regulation have been collected in Table 1 below:
LncRNAs may support transcription; aid RNA interference; act as a decoy, peptide, and scaffold; or function as a guide/enhancer RNA (Li et al., 2014). They may deactivate miRNAs in cancer via the “sponge” effect, that is, act as a competing molecule or decoy to attach to miRNAs and perturb them from their target (Fan et al., 2018; Huang et al., 2019) (Figure 2). They have been demarcated as diagnostic and therapeutic targets. Here, we review lncRNA intertwined with the Wnt pathway and TNBC progression, pathogenesis, prognosis, or invasion. LncRNA Lung Cancer–Associated Transcript 1 (LUCAT1) is interlinked with miR-5582 and regulates breast cancer stemness via the Wnt/β-catenin pathway (Zheng et al., 2019). UCA1 promotes EMT (Xiao et al., 2016), while actin filament–associated protein 1 antisense RNA1 (AFAP1-AS1) promotes EMT and tumorigenesis (Zhang et al., 2018). and differentiation antagonizing non-protein coding RNA (DANCR) negatively regulates the Wnt pathway (Li and Zhou, 2018) to uplift tumorigenesis (Tao et al., 2019), EMT, and stemness in TNBC (Zhang and Wang, 2020). HOX Antisense Intergenic RNA (HOTAIR) modulates the Wnt pathway (Li et al., 2016) and leads to metastasis (Collina et al., 2019) and poor prognosis in TNBC via upregulation by miR-146a (Liang et al., 2019).
Jiang et al. (2020) reported that DiGeorge Syndrome Critical Region Gene 5 (DGCR5) induces tumorigenesis in TNBC. LncRNA associated with poor prognosis of hepatocellular carcinoma (AWPPH) promotes TNBC growth by upregulating the frizzled homolog 7 (FZD7) ligand of the Wnt pathway (Wang et al., 2018b) and decreased manifestation of lncRNA has been reported to increase the malignant spread of TNBCs (Liu et al., 2017). Long intergenic non-protein coding RNA 1234 (LINC01234) modulates TNBC cell growth, invasion, and EMT positively (Xiao et al., 2021). We also mined lncRNAs with a role in TNBC and impacted by Wnt pathway genes (Table 2) from the lnc2Cancer 3.0 database (Gao et al., 2021).
In addition, lncRNA–miRNA interactions entwining the Wnt pathway have also been noted in TNBC (Volovat et al., 2020). Among these, intranuclear Metastasis-Related Lung Adenocarcinoma Transcript 1 (MALAT1) acts as a sponge of miR-129-5p (Dong et al., 2015), and silencing of this non-coding gene causes a decline in cell propagation and movement, illustrating its role in TNBC pathology (Zuo et al., 2017). AWPPH stimulates cell proliferation and contributes to drug therapy resistance when combined with miRNA-21 and is being exploited as a diagnostic biomarker (Cascione et al., 2013; Liu et al., 2019). At the same time, TUG1 impacts miR-197, prompts NLK expression, and incapacitates the Wnt signaling pathway, making the TNBC cells susceptible to cisplatin therapy (Tang et al., 2018b). AFAP1-AS1 controls miRNA-2110, leading to tumorigenesis and cell invasion (Zuo et al., 2017). The diminished NEF and boosted miRNA-155 levels segregate TNBC patients from controls, suggesting an interlinked modulation prompting enhanced invasion and cell migration in the case of increased miRNA-155 (Song et al., 2019).
This narrative review was centered on TNBC association with the Wnt/β-catenin pathway. Moreover, miRNAs and lncRNAs shown to be specific to triple-negative breast carcinoma were listed, both from literature and database searches. Since the Wnt pathway and miRNAs/lncRNAs can modulate TNBC and their intertwinement forms a cascade of complex reactions that impact TNBC and associated processes, their role in TNBC pathology was highlighted concerning molecular processes underlying disease progression. Particular emphasis was put on tumorigenesis, metastasis, and EMT. Apart from cross-talk critical for TNBC tumorigenesis and treatment outcomes, miRNA and lncRNA can serve as predictive or diagnostic markers, so this consolidated information might be of clinical use and offer a consolidated literature resource to scientists working on the Wnt/β-catenin and miRNA/lncRNA axis of TNBC. They also have a role in chemotherapy resistance. However, information on detailed analyses of Wnt/miRNA/lncRNA axis mechanisms in TNBC is still scant, and more work needs to be carried out to infer the pivotal role of these moieties in TNBC. Owing to the heterogeneity of TNBC, recognition of subgroups or their pathologies based on the varied signatures of this axis could be interesting to explore. Furthermore, knock-out or knock-in functional studies in model organisms could be beneficial to understanding the comprehensive role of this axis in TNBC.
Conception/Design: WW, QZ. Collection and assembly of data: XH, WX. Manuscript writing: XH, WW. Final approval of manuscript: WW, QZ, XH, WX.
This study was supported by the China Postdoctoral Science Foundation Grant (No. 2019M651216).
The authors declare that the research was conducted in the absence of any commercial or financial relationships that could be construed as a potential conflict of interest.
All claims expressed in this article are solely those of the authors and do not necessarily represent those of their affiliated organizations, or those of the publisher, the editors, and the reviewers. Any product that may be evaluated in this article, or claim that may be made by its manufacturer, is not guaranteed or endorsed by the publisher.
We acknowledge the China Postdoctoral Science Foundation for supporting this study.
Agustsson, A. S., Birgisson, H., Agnarsson, B. A., Jonsson, T., Stefansdottir, H., Wärnberg, F., et al. (2020). In Situ breast Cancer Incidence Patterns in Iceland and Differences in Ductal Carcinoma In Situ Treatment Compared to Sweden. Sci. Rep. 10 (1), 17623. doi:10.1038/s41598-020-74134-5
Ali Syeda, Z., Langden, S. S. S., Munkhzul, C., Lee, M., and Song, S. J. (2020). Regulatory Mechanism of MicroRNA Expression in Cancer. Int. J. Mol. Sci. 21 (5). doi:10.3390/ijms21051723
Alraouji, N. N., Al-Mohanna, F. H., Ghebeh, H., Arafah, M., Almeer, R., Al-Tweigeri, T., et al. (2020). Tocilizumab Potentiates Cisplatin Cytotoxicity and Targets Cancer Stem Cells in Triple-Negative Breast Cancer. Mol. Carcinog. 59 (9), 1041–1051. doi:10.1002/mc.23234
Arif, K. M. T., Elliott, E. K., Haupt, L. M., and Griffiths, L. R. (2020). Regulatory Mechanisms of Epigenetic miRNA Relationships in Human Cancer and Potential as Therapeutic Targets. Cancers (Basel) 12 (10). doi:10.3390/cancers12102922
Avery-Kiejda, K. A., Braye, S. G., Mathe, A., Forbes, J. F., and Scott, R. J. (2014). Decreased Expression of Key Tumour Suppressor microRNAs Is Associated with Lymph Node Metastases in Triple Negative Breast Cancer. BMC Cancer 14, 51. doi:10.1186/1471-2407-14-51
Ballantyne, R. L., Zhang, X., Nuñez, S., Xue, C., Zhao, W., Reed, E., et al. (2016). Genome-wide Interrogation Reveals Hundreds of Long Intergenic Noncoding RNAs that Associate with Cardiometabolic Traits. Hum. Mol. Genet. 25 (14), 3125–3141. doi:10.1093/hmg/ddw154
Bonetti, P., Climent, M., Panebianco, F., Tordonato, C., Santoro, A., Marzi, M. J., et al. (2019). Dual Role for miR-34a in the Control of Early Progenitor Proliferation and Commitment in the Mammary Gland and in Breast Cancer. Oncogene 38 (3), 360–374. doi:10.1038/s41388-018-0445-3
Breuer, E. K., Fukushiro-Lopes, D., Dalheim, A., Burnette, M., Zartman, J., Kaja, S., et al. (2019). Potassium Channel Activity Controls Breast Cancer Metastasis by Affecting β-catenin Signaling. Cell Death Dis. 10 (3), 180. doi:10.1038/s41419-019-1429-0
Brilliant, A., Brilliant, Y., Sazonov, S., and Wnt, (2019). WNT, Hedgehog and NOTCH Signaling Pathways in Triple Negative Breast Cancer with High and Low Content of Cancer Stem Cells. Ann. Oncol. 30, iii13–iii14. doi:10.1093/annonc/mdz095.039
Cai, J., Guan, H., Fang, L., Yang, Y., Zhu, X., Yuan, J., et al. (2013). MicroRNA-374a Activates Wnt/β-Catenin Signaling to Promote Breast Cancer Metastasis. J. Clin. Invest. 123 (2), 566–579. doi:10.1172/JCI65871
Cascione, L., Gasparini, P., Lovat, F., Carasi, S., Pulvirenti, A., Ferro, A., et al. (2013). Integrated microRNA and mRNA Signatures Associated with Survival in Triple Negative Breast Cancer. PLoS One 8 (2), e55910. doi:10.1371/journal.pone.0055910
Chakrabarti, R., Wei, Y., Hwang, J., Hang, X., Andres Blanco, M., Choudhury, A., et al. (2014). ΔNp63 Promotes Stem Cell Activity in Mammary Gland Development and Basal-like Breast Cancer by Enhancing Fzd7 Expression and Wnt Signalling. Nat. Cell Biol. 16 (10), 10041–11513. doi:10.1038/ncb3040
Chen, C., Li, Z., Yang, Y., Xiang, T., Song, W., and Liu, S. (2015). Microarray Expression Profiling of Dysregulated Long Non-coding RNAs in Triple-Negative Breast Cancer. Cancer Biol. Ther. 16 (6), 856–865. doi:10.1080/15384047.2015.1040957
Chen, Y., Min, L., Ren, C., Xu, X., Yang, J., Sun, X., et al. (2017). miRNA-148a Serves as a Prognostic Factor and Suppresses Migration and Invasion through Wnt1 in Non-small Cell Lung Cancer. PLoS One 12 (2), e0171751. doi:10.1371/journal.pone.0171751
Chen, Z., Yu, C., Zhan, L., Pan, Y., Chen, L., and Sun, C. (2016). Lncrna crnde promotes hepatic carcinoma cell proliferation, migration and invasion by suppressing mir-384. Am. J. Cancer Res. 6 (10), 2299–2309.
Cheng, S., Huang, Y., Lou, C., He, Y., Zhang, Y., and Zhang, Q. (2019). FSTL1 Enhances Chemoresistance and Maintains Stemness in Breast Cancer Cells via Integrin β3/Wnt Signaling under miR-137 Regulation. Cancer Biol. Ther. 20 (3), 328–337. doi:10.1080/15384047.2018.1529101
Clarke, M. F. (2019). Clinical and Therapeutic Implications of Cancer Stem Cells. N. Engl. J. Med. 380 (23), 2237–2245. doi:10.1056/NEJMra1804280
Collina, F., Aquino, G., Brogna, M., Cipolletta, S., Buonfanti, G., De Laurentiis, M., et al. (2019). Lncrna hotair up-regulation is strongly related with lymph nodes metastasis and lar subtype of triple negative breast cancer. J. Cancer 10 (9), 2018–2024. doi:10.7150/jca.29670
Cruz-Lozano, M., González-González, A., Marchal, J. A., Muñoz-Muela, E., Molina, M. P., Cara, F. E., et al. (2019). Hydroxytyrosol Inhibits Cancer Stem Cells and the Metastatic Capacity of Triple-Negative Breast Cancer Cell Lines by the Simultaneous Targeting of Epithelial-To-Mesenchymal Transition, Wnt/β-Catenin and TGFβ Signaling Pathways. Eur. J. Nutr. 58 (8), 3207–3219. doi:10.1007/s00394-018-1864-1
Dai, W., He, J., Zheng, L., Bi, M., Hu, F., Chen, M., et al. (2019). miR-148b-3p, miR-190b, and miR-429 Regulate Cell Progression and Act as Potential Biomarkers for Breast Cancer. J. Breast Cancer 22 (2), 219–236. doi:10.4048/jbc.2019.22.e19
Danieau, G., Morice, S., Rédini, F., Verrecchia, F., and Royer, B. B. (2019). New Insights about the Wnt/β-Catenin Signaling Pathway in Primary Bone Tumors and Their Microenvironment: A Promising Target to Develop Therapeutic Strategies? Int. J. Mol. Sci. 20 (15). doi:10.3390/ijms20153751
Dey, N., Barwick, B. G., Moreno, C. S., Ordanic-Kodani, M., Chen, Z., Oprea-Ilies, G., et al. (2013). Wnt Signaling in Triple Negative Breast Cancer Is Associated with Metastasis. BMC Cancer 13, 537. doi:10.1186/1471-2407-13-537
Dong, Y., Liang, G., Yuan, B., Yang, C., Gao, R., and Zhou, X. (2015). MALAT1 Promotes the Proliferation and Metastasis of Osteosarcoma Cells by Activating the PI3K/Akt Pathway. Tumour Biol. 36 (3), 1477–1486. doi:10.1007/s13277-014-2631-4
Drago-Ferrante, R., Pentimalli, F., Carlisi, D., De Blasio, A., Saliba, C., Baldacchino, S., et al. (2017). Suppressive Role Exerted by microRNA-29b-1-5p in Triple Negative Breast Cancer through SPIN1 Regulation. Oncotarget 8 (17), 28939–28958. doi:10.18632/oncotarget.15960
Fan, C. N., Ma, L., and Liu, N. (2018). Systematic Analysis of lncRNA-miRNA-mRNA Competing Endogenous RNA Network Identifies Four-lncRNA Signature as a Prognostic Biomarker for Breast Cancer. J. Transl. Med. 16 (1), 264. doi:10.1186/s12967-018-1640-2
Fan, H., Yuan, J., Li, X., Ma, Y., Wang, X., Xu, B., et al. (2020). LncRNA LINC00173 Enhances Triple-Negative Breast Cancer Progression by Suppressing miR-490-3p Expression. Biomed. Pharmacother. 125, 109987. doi:10.1016/j.biopha.2020.109987
Ferlay, J., Soerjomataram, I., Dikshit, R., Eser, S., Mathers, C., Rebelo, M., et al. (2015). Cancer Incidence and Mortality Worldwide: Sources, Methods and Major Patterns in GLOBOCAN 2012. Int. J. Cancer 136 (5), E359–E386. doi:10.1002/ijc.29210
Gai, C., Camussi, F., Broccoletti, R., Gambino, A., Cabras, M., Molinaro, L., et al. (2018). Salivary Extracellular Vesicle-Associated miRNAs as Potential Biomarkers in Oral Squamous Cell Carcinoma. BMC Cancer 18 (1), 439. doi:10.1186/s12885-018-4364-z
Gangrade, A., Pathak, V., Augelli-Szafran, C. E., Wei, H. X., Oliver, P., Suto, M., et al. (2018). Preferential Inhibition of Wnt/β-Catenin Signaling by Novel Benzimidazole Compounds in Triple-Negative Breast Cancer. Int. J. Mol. Sci. 19 (5). doi:10.3390/ijms19051524
Gao, Y., Shang, S., Guo, S., Li, X., Zhou, H., Liu, H., et al. (2021). Lnc2Cancer 3.0: an Updated Resource for Experimentally Supported lncRNA/circRNA Cancer Associations and Web Tools Based on RNA-Seq and scRNA-Seq Data. Nucleic Acids Res. 49 (D1), D1251–D1258. doi:10.1093/nar/gkaa1006
Geng, S. Q., Alexandrou, A. T., and Li, J. J. (2014). Breast Cancer Stem Cells: Multiple Capacities in Tumor Metastasis. Cancer Lett. 349 (1), 1–7. doi:10.1016/j.canlet.2014.03.036
Goel, S., Chin, E. N., Fakhraldeen, S. A., Berry, S. M., Beebe, D. J., and Alexander, C. M. (2012). Both LRP5 and LRP6 Receptors Are Required to Respond to Physiological Wnt Ligands in Mammary Epithelial Cells and Fibroblasts. J. Biol. Chem. 287 (20), 16454–16466. doi:10.1074/jbc.M112.362137
Goh, J. N., Loo, S. Y., Datta, A., Siveen, K. S., Yap, W. N., Cai, W., et al. (2016). microRNAs in Breast Cancer: Regulatory Roles Governing the Hallmarks of Cancer. Biol. Rev. Camb Philos. Soc. 91 (2), 409–428. doi:10.1111/brv.12176
Green, J. L., La, J., Yum, K. W., Desai, P., Rodewald, L. W., Zhang, X., et al. (2013). Paracrine Wnt Signaling Both Promotes and Inhibits Human Breast Tumor Growth. Proc. Natl. Acad. Sci. U. S. A. 110 (17), 6991–6996. doi:10.1073/pnas.1303671110
Gupta, R. A., Shah, N., Wang, K. C., Kim, J., Horlings, H. M., Wong, D. J., et al. (2010). Long non-coding rna hotair reprograms chromatin state to promote cancer metastasis. Nature 464 (7291), 1071–1076. doi:10.1038/nature08975
Guturi, K. K., Sarkar, M., Bhowmik, A., Das, N., and Ghosh, M. K. (2014). DEAD-box Protein P68 Is Regulated by β-catenin/transcription Factor 4 to Maintain a Positive Feedback Loop in Control of Breast Cancer Progression. Breast Cancer Res. 16 (6), 496. doi:10.1186/s13058-014-0496-5
Han, C., Fu, Y., Zeng, N., Yin, J., and Li, Q. (2020). LncRNA FAM83H-AS1 Promotes Triple-Negative Breast Cancer Progression by Regulating the miR-136-5p/metadherin axis. Aging (Albany NY) 12 (4), 3594–3616. doi:10.18632/aging.102832
Han, C., Li, X., Fan, Q., Liu, G., and Yin, J. (2019). CCAT1 Promotes Triple-Negative Breast Cancer Progression by Suppressing miR-218/ZFX Signaling. Aging (Albany NY) 11 (14), 4858–4875. doi:10.18632/aging.102080
Han, J., Han, B., Wu, X., Hao, J., Dong, X., Shen, Q., et al. (2018). Knockdown of lncRNA H19 Restores Chemo-Sensitivity in Paclitaxel-Resistant Triple-Negative Breast Cancer through Triggering Apoptosis and Regulating Akt Signaling Pathway. Toxicol. Appl. Pharmacol. 359, 55–61. doi:10.1016/j.taap.2018.09.018
He, L., and Hannon, G. J. (2004). MicroRNAs: Small RNAs with a Big Role in Gene Regulation. Nat. Rev. Genet. 5 (7), 522–531. doi:10.1038/nrg1379
Hong, B. S., Ryu, H. S., Kim, N., Kim, J., Lee, E., Moon, H., et al. (2019). Tumor Suppressor miRNA-204-5p Regulates Growth, Metastasis, and Immune Microenvironment Remodeling in Breast Cancer. Cancer Res. 79 (7), 1520–1534. doi:10.1158/0008-5472.CAN-18-0891
Huang, Q. Y., Liu, G. F., Qian, X. L., Tang, L. B., Huang, Q. Y., and Xiong, L. X. (2019). Long Non-coding RNA: Dual Effects on Breast Cancer Metastasis and Clinical Applications. Cancers (Basel) 11 (11). doi:10.3390/cancers11111802
Isobe, T., Hisamori, S., Hogan, D. J., Zabala, M., Hendrickson, D. G., Dalerba, P., et al. (2014). miR-142 Regulates the Tumorigenicity of Human Breast Cancer Stem Cells through the Canonical WNT Signaling Pathway. Elife 3. doi:10.7554/eLife.01977
Jang, G. B., Kim, J. Y., Cho, S. D., Park, K. S., Jung, J. Y., Lee, H. Y., et al. (2015). Blockade of Wnt/β-Catenin Signaling Suppresses Breast Cancer Metastasis by Inhibiting CSC-like Phenotype. Sci. Rep. 5, 12465. doi:10.1038/srep12465
Jiang, D., Wang, C., and He, J. (2020). Long Non-coding RNA DGCR5 Incudes Tumorigenesis of Triple-Negative Breast Cancer by Affecting Wnt/β-Catenin Signaling Pathway. J. BUON 25 (2), 702–708.
Karimzadeh, M. R., Pourdavoud, P., Ehtesham, N., Qadbeigi, M., Asl, M. M., Alani, B., et al. (2021). Regulation of DNA Methylation Machinery by Epi-miRNAs in Human Cancer: Emerging New Targets in Cancer Therapy. Cancer Gene Ther. 28 (3-4), 157–174. doi:10.1038/s41417-020-00210-7
King, T. D., Suto, M. J., and Li, Y. (2012). The Wnt/β-Catenin Signaling Pathway: a Potential Therapeutic Target in the Treatment of Triple Negative Breast Cancer. J. Cell Biochem. 113 (1), 13–18. doi:10.1002/jcb.23350
Li, H. Y., Liang, J. L., Kuo, Y. L., Lee, H. H., Calkins, M. J., Chang, H. T., et al. (2017). miR-105/93-3p Promotes Chemoresistance and Circulating miR-105/93-3p Acts as a Diagnostic Biomarker for Triple Negative Breast Cancer. Breast Cancer Res. 19 (1), 133. doi:10.1186/s13058-017-0918-2
Li, J., Yang, S., Su, N., Wang, Y., Yu, J., Qiu, H., et al. (2016). Overexpression of long non-coding rna hotair leads to chemoresistance by activating the wnt/β-catenin pathway in human ovarian cancer. Tumour Biol. 37 (2), 2057–2065. doi:10.1007/s13277-015-3998-6
Li, J., and Zhou, L. (2018). Overexpression of lncrna dancr positively affects progression of glioma via activating wnt/β-catenin signaling. Biomed. Pharmacother. 102, 602–607. doi:10.1016/j.biopha.2018.03.116
Li, S., Zhou, J., Wang, Z., Wang, P., Gao, X., and Wang, Y. (2018). Long Noncoding RNA GAS5 Suppresses Triple Negative Breast Cancer Progression through Inhibition of Proliferation and Invasion by Competitively Binding miR-196a-5p. Biomed. Pharmacother. 104, 451–457. doi:10.1016/j.biopha.2018.05.056
Li, X., Hou, L., Yin, L., and Zhao, S. (2020). Lncrna xist interacts with mir-454 to inhibit cells proliferation, epithelial mesenchymal transition and induces apoptosis in triple-negative breast cancer. J. Biosci. 45. doi:10.1007/s12038-020-9999-7
Li, X., Wu, Z., Fu, X., and Han, W. (2014). lncRNAs: Insights into Their Function and Mechanics in Underlying Disorders. Mutat. Res. Rev. Mutat. Res. 762, 1–21. doi:10.1016/j.mrrev.2014.04.002
Liang, H., Huang, W., Wang, Y., Ding, L., and Zeng, L. (2019). Overexpression of MiR-146a-5p Upregulates lncRNA HOTAIR in Triple-Negative Breast Cancer Cells and Predicts Poor Prognosis. Technol. Cancer Res. Treat. 18, 1533033819882949. doi:10.1177/1533033819882949
Liu, A. N., Qu, H. J., Gong, W. J., Xiang, J. Y., Yang, M. M., and Zhang, W. (2019). LncRNA AWPPH and miRNA-21 Regulates Cancer Cell Proliferation and Chemosensitivity in Triple-Negative Breast Cancer by Interacting with Each Other. J. Cell Biochem. 120 (9), 14860–14866. doi:10.1002/jcb.28747
Liu, L., Yu, D., Shi, H., Li, J., and Meng, L. (2017). Reduced lncRNA Aim Enhances the Malignant Invasion of Triple-Negative Breast Cancer Cells Mainly by Activating Wnt/β-catenin/mTOR/PI3K Signaling. Pharmazie 72 (10), 599–603. doi:10.1691/ph.2017.7547
Liu, S., Wang, Z., Liu, Z., Shi, S., Zhang, Z., Zhang, J., et al. (2018). miR-221/222 Activate the Wnt/β-Catenin Signaling to Promote Triple-Negative Breast Cancer. J. Mol. Cell Biol. 10 (4), 302–315. doi:10.1093/jmcb/mjy041
Liu, X., Song, J., Kang, Y., Wang, Y., and Chen, A. (2020). Long Noncoding RNA SOX21-AS1 Regulates the Progression of Triple-Negative Breast Cancer through Regulation of miR-520a-5p/ORMDL3 axis. J. Cell Biochem. 121 (11), 4601–4611. doi:10.1002/jcb.29674
Lu, L., Xu, H., Luo, F., Liu, X., Lu, X., Yang, Q., et al. (2016). Epigenetic Silencing of miR-218 by the lncRNA CCAT1, Acting via BMI1, Promotes an Altered Cell Cycle Transition in the Malignant Transformation of HBE Cells Induced by Cigarette Smoke Extract. Toxicol. Appl. Pharmacol. 304, 30–41. doi:10.1016/j.taap.2016.05.012
Luo, N., Zhang, K., Li, X., and Hu, Y. (2020). ZEB1 Induced-Upregulation of Long Noncoding RNA ZEB1-AS1 Facilitates the Progression of Triple Negative Breast Cancer by Binding with ELAVL1 to Maintain the Stability of ZEB1 mRNA. J. Cell Biochem. 121 (10), 4176–4187. doi:10.1002/jcb.29572
Lv, M., Xu, P., Wu, Y., Huang, L., Li, W., Lv, S., et al. (2016). LncRNAs as New Biomarkers to Differentiate Triple Negative Breast Cancer from Non-triple Negative Breast Cancer. Oncotarget 7 (11), 13047–13059. doi:10.18632/oncotarget.7509
Ma, J., Lu, W., Chen, D., Xu, B., and Li, Y. (2017). Role of Wnt Co-receptor LRP6 in Triple Negative Breast Cancer Cell Migration and Invasion. J. Cell Biochem. 118 (9), 2968–2976. doi:10.1002/jcb.25956
MacDonald, B. T., Tamai, K., and He, X. (2009). Wnt/beta-catenin Signaling: Components, Mechanisms, and Diseases. Dev. Cell 17 (1), 9–26. doi:10.1016/j.devcel.2009.06.016
Maubant, S., Tahtouh, T., Brisson, A., Maire, V., Némati, F., Tesson, B., et al. (2018). LRP5 Regulates the Expression of STK40, a New Potential Target in Triple-Negative Breast Cancers. Oncotarget 9 (32), 22586–22604. doi:10.18632/oncotarget.25187
Mendell, J. T. (2016). Targeting a Long Noncoding RNA in Breast Cancer. N. Engl. J. Med. 374 (23), 2287–2289. doi:10.1056/NEJMcibr1603785
Merikhian, P., Eisavand, M. R., and Farahmand, L. (2021). Triple-negative Breast Cancer: Understanding Wnt Signaling in Drug Resistance. Cancer Cell Int. 21 (1), 419. doi:10.1186/s12935-021-02107-3
Miao, Y., Zheng, W., Li, N., Su, Z., Zhao, L., Zhou, H., et al. (2017). MicroRNA-130b Targets PTEN to Mediate Drug Resistance and Proliferation of Breast Cancer Cells via the PI3K/Akt Signaling Pathway. Sci. Rep. 7, 41942. doi:10.1038/srep41942
Mohammadi Yeganeh, S., Vasei, M., Tavakoli, R., Kia, V., and Paryan, M. (2017). The Effect of miR-340 Over-expression on Cell-Cycle-Related Genes in Triple-Negative Breast Cancer Cells. Eur. J. Cancer Care (Engl) 26 (6). doi:10.1111/ecc.12496
Moore, H. C., Jordan, L. B., Bray, S. E., Baker, L., Quinlan, P. R., Purdie, C. A., et al. (2010). The RNA Helicase P68 Modulates Expression and Function of the Δ133 Isoform(s) of P53, and Is Inversely Associated with Δ133p53 Expression in Breast Cancer. Oncogene 29 (49), 6475–6484. doi:10.1038/onc.2010.381
Mou, E., and Wang, H. (2019). LncRNA LUCAT1 Facilitates Tumorigenesis and Metastasis of Triple-Negative Breast Cancer through Modulating miR-5702. Biosci. Rep. 39 (9). doi:10.1042/BSR20190489
Müller, S., Glaß, M., Singh, A. K., Haase, J., Bley, N., Fuchs, T., et al. (2019). IGF2BP1 Promotes SRF-dependent Transcription in Cancer in a m6A- and miRNA-dependent Manner. Nucleic Acids Res. 47 (1), 375–390. doi:10.1093/nar/gky1012
Nie, J., Jiang, H. C., Zhou, Y. C., Jiang, B., He, W. J., Wang, Y. F., et al. (2019). MiR-125b Regulates the Proliferation and Metastasis of Triple Negative Breast Cancer Cells via the Wnt/β-Catenin Pathway and EMT. Biosci. Biotechnol. Biochem. 83 (6), 1062–1071. doi:10.1080/09168451.2019.1584521
Padmanaban, V., Krol, I., Suhail, Y., Szczerba, B. M., Aceto, N., Bader, J. S., et al. (2019). E-cadherin Is Required for Metastasis in Multiple Models of Breast Cancer. Nature 573 (7774), 439–444. doi:10.1038/s41586-019-1526-3
Peiris-Pagès, M., Bonuccelli, G., Sotgia, F., and Lisanti, M. P. (2018). Mitochondrial Fission as a Driver of Stemness in Tumor Cells: mDIVI1 Inhibits Mitochondrial Function, Cell Migration and Cancer Stem Cell (CSC) Signalling. Oncotarget 9 (17), 13254–13275. doi:10.18632/oncotarget.24285
Phi, L. T. H., Sari, I. N., Yang, Y. G., Lee, S. H., Jun, N., Kim, K. S., et al. (2018). Cancer Stem Cells (CSCs) in Drug Resistance and Their Therapeutic Implications in Cancer Treatment. Stem Cells Int. 2018, 5416923. doi:10.1155/2018/5416923
Piasecka, D., Braun, M., Kordek, R., Sadej, R., and Romanska, H. (2018). MicroRNAs in Regulation of Triple-Negative Breast Cancer Progression. J. Cancer Res. Clin. Oncol. 144 (8), 1401–1411. doi:10.1007/s00432-018-2689-2
Pohl, S. G., Brook, N., Agostino, M., Arfuso, F., Kumar, A. P., and Dharmarajan, A. (2017). Wnt Signaling in Triple-Negative Breast Cancer. Oncogenesis 6 (4), e310. doi:10.1038/oncsis.2017.14
Poodineh, J., Sirati-Sabet, M., Rajabibazl, M., and Mohammadi-Yeganeh, S. (2020). MiR-130a-3p Blocks Wnt Signaling Cascade in the Triple-Negative Breast Cancer by Targeting the Key Players at Multiple Points. Heliyon 6 (11), e05434. doi:10.1016/j.heliyon.2020.e05434
Rodrigues de Bastos, D., and Nagai, M. A. (2020). In Silico analyses Identify lncRNAs: WDFY3-AS2, BDNF-AS and AFAP1-AS1 as Potential Prognostic Factors for Patients with Triple-Negative Breast Tumors. PLoS One 15 (5), e0232284. doi:10.1371/journal.pone.0232284
Ryu, W. J., Lee, J. D., Park, J. C., Cha, P. H., Cho, Y. H., Kim, J. Y., et al. (2020). Destabilization of β-catenin and RAS by Targeting the Wnt/β-Catenin Pathway as a Potential Treatment for Triple-Negative Breast Cancer. Exp. Mol. Med. 52 (5), 832–842. doi:10.1038/s12276-020-0440-y
Satriyo, P. B., Bamodu, O. A., Chen, J. H., Aryandono, T., Haryana, S. M., Yeh, C. T., et al. (2019). Cadherin 11 Inhibition Downregulates β-catenin, Deactivates the Canonical WNT Signalling Pathway and Suppresses the Cancer Stem Cell-like Phenotype of Triple Negative Breast Cancer. J. Clin. Med. 8 (2). doi:10.3390/jcm8020148
Scatena, C., Roncella, M., Di Paolo, A., Aretini, P., Menicagli, M., Fanelli, G., et al. (2018). Doxycycline, an Inhibitor of Mitochondrial Biogenesis, Effectively Reduces Cancer Stem Cells (CSCs) in Early Breast Cancer Patients: A Clinical Pilot Study. Front. Oncol. 8, 452. doi:10.3389/fonc.2018.00452
Shi, R., Wu, P., Liu, M., Chen, B., and Cong, L. (2020). Knockdown of lncRNA PCAT6 Enhances Radiosensitivity in Triple-Negative Breast Cancer Cells by Regulating miR-185-5p/TPD52 Axis. Onco Targets Ther. 13, 3025–3037. doi:10.2147/OTT.S237559
Shin, V. Y., Chen, J., Cheuk, I. W., Siu, M. T., Ho, C. W., Wang, X., et al. (2019). Long Non-coding RNA NEAT1 Confers Oncogenic Role in Triple-Negative Breast Cancer through Modulating Chemoresistance and Cancer Stemness. Cell Death Dis. 10 (4), 270. doi:10.1038/s41419-019-1513-5
Song, X., Liu, Z., and Yu, Z. (2019). Lncrna nef is downregulated in triple negative breast cancer and correlated with poor prognosis. Acta Biochim. Biophys. Sin. (Shanghai) 51 (4), 386–392. doi:10.1093/abbs/gmz021
Taipaleenmäki, H., Farina, N. H., van Wijnen, A. J., Stein, J. L., Hesse, E., Stein, G. S., et al. (2016). Antagonizing miR-218-5p Attenuates Wnt Signaling and Reduces Metastatic Bone Disease of Triple Negative Breast Cancer Cells. Oncotarget 7 (48), 79032–79046. doi:10.18632/oncotarget.12593
Tang, J., Zhong, G., Zhang, H., Yu, B., Wei, F., Luo, L., et al. (2018). Lncrna dancr upregulates pi3k/akt signaling through activating serine phosphorylation of rxra. Cell Death Dis. 9 (12), 1167. doi:10.1038/s41419-018-1220-7
Tang, T., Cheng, Y., She, Q., Jiang, Y., Chen, Y., Yang, W., et al. (2018). Long Non-coding RNA TUG1 Sponges miR-197 to Enhance Cisplatin Sensitivity in Triple Negative Breast Cancer. Biomed. Pharmacother. 107, 338–346. doi:10.1016/j.biopha.2018.07.076
Tao, W., Wang, C., Zhu, B., Zhang, G., and Pang, D. (2019). Lncrna dancr contributes to tumor progression via targetting mir-216a-5p in breast cancer: lncrna dancr contributes to tumor progression. Biosci. Rep. 39 (4). doi:10.1042/BSR20181618
Taube, J. H., Malouf, G. G., Lu, E., Sphyris, N., Vijay, V., Ramachandran, P. P., et al. (2013). Epigenetic Silencing of microRNA-203 Is Required for EMT and Cancer Stem Cell Properties. Sci. Rep. 3, 2687. doi:10.1038/srep02687
Telonis, A. G., and Rigoutsos, I. (2018). Race Disparities in the Contribution of miRNA Isoforms and tRNA-Derived Fragments to Triple-Negative Breast Cancer. Cancer Res. 78 (5), 1140–1154. doi:10.1158/0008-5472.CAN-17-1947
Trzybulska, D., Vergadi, E., and Tsatsanis, C. (2018). miRNA and Other Non-coding RNAs as Promising Diagnostic Markers. EJIFCC 29 (3), 221–226.
Tu, Z., Schmöllerl, J., Cuiffo, B. G., and Karnoub, A. E. (2019). Microenvironmental Regulation of Long Noncoding RNA LINC01133 Promotes Cancer Stem Cell-like Phenotypic Traits in Triple-Negative Breast Cancers. Stem Cells 37 (10), 1281–1292. doi:10.1002/stem.3055
van Barele, M., Heemskerk-Gerritsen, B. A. M., Louwers, Y. V., Vastbinder, M. B., Martens, J. W. M., Hooning, M. J., et al. (2021). Estrogens and Progestogens in Triple Negative Breast Cancer: Do They Harm? Cancers (Basel) 13 (11). doi:10.3390/cancers13112506
Volovat, S. R., Volovat, C., Hordila, I., Hordila, D. A., Mirestean, C. C., Miron, O. T., et al. (2020). MiRNA and LncRNA as Potential Biomarkers in Triple-Negative Breast Cancer: A Review. Front. Oncol. 10, 526850. doi:10.3389/fonc.2020.526850
Wang, D., Huang, J., and Hu, Z. (2012). RNA Helicase DDX5 Regulates microRNA Expression and Contributes to Cytoskeletal Reorganization in Basal Breast Cancer Cells. Mol. Cell Proteomics 11 (2), M111–M011932. doi:10.1074/mcp.M111.011932
Wang, D. Y., Gendoo, D. M. A., Ben-David, Y., Woodgett, J. R., and Zacksenhaus, E. (2019). A Subgroup of microRNAs Defines PTEN-Deficient, Triple-Negative Breast Cancer Patients with Poorest Prognosis and Alterations in RB1, MYC, and Wnt Signaling. Breast Cancer Res. 21 (1), 18. doi:10.1186/s13058-019-1098-z
Wang, K., Li, X., Song, C., and Li, M. (2018). Lncrna awpph promotes the growth of triple-negative breast cancer by up-regulating frizzled homolog 7 (fzd7). Biosci. Rep. 38 (6). doi:10.1042/BSR20181223
Wang, L., Wang, R., Ye, Z., Wang, Y., Li, X., Chen, W., et al. (2018). PVT1 Affects EMT and Cell Proliferation and Migration via Regulating P21 in Triple-Negative Breast Cancer Cells Cultured with Mature Adipogenic Medium. Acta Biochim. Biophys. Sin. (Shanghai) 50 (12), 1211–1218. doi:10.1093/abbs/gmy129
Wang, Y., Zhang, Z., and Wang, J. (2018). MicroRNA-384 Inhibits the Progression of Breast Cancer by Targeting ACVR1. Oncol. Rep. 39 (6), 2563–2574. doi:10.3892/or.2018.6385
Wilusz, J. E., Sunwoo, H., and Spector, D. L. (2009). Long Noncoding RNAs: Functional Surprises from the RNA World. Genes Dev. 23 (13), 1494–1504. doi:10.1101/gad.1800909
Wu, J., Shuang, Z., Zhao, J., Tang, H., Liu, P., Zhang, L., et al. (2018). Linc00152 Promotes Tumorigenesis by Regulating DNMTs in Triple-Negative Breast Cancer. Biomed. Pharmacother. 97, 1275–1281. doi:10.1016/j.biopha.2017.11.055
Wu, R., Zhao, B., Ren, X., Wu, S., Liu, M., Wang, Z., et al. (2020). MiR-27a-3p Targeting GSK3β Promotes Triple-Negative Breast Cancer Proliferation and Migration through Wnt/β-Catenin Pathway. Cancer Manag. Res. 12, 6241–6249. doi:10.2147/CMAR.S255419
Xiao, C., Wu, C. H., and Hu, H. Z. (2016). LncRNA UCA1 Promotes Epithelial-Mesenchymal Transition (EMT) of Breast Cancer Cells via Enhancing Wnt/beta-Catenin Signaling Pathway. Eur. Rev. Med. Pharmacol. Sci. 20 (13), 2819–2824.
Xiao, F., Jiao, H., Wu, D., Zhang, Z., Li, S., and Guo, J. (2021). LINC01234 Aggravates Cell Growth and Migration of Triple-Negative Breast Cancer by Activating the Wnt Pathway. Environ. Toxicol.36, 1999–2012. doi:10.1002/tox.23318
Xu, J., Prosperi, J. R., Choudhury, N., Olopade, O. I., and Goss, K. H. (2015). β-Catenin Is Required for the Tumorigenic Behavior of Triple-Negative Breast Cancer Cells. PLoS One 10 (2), e0117097. doi:10.1371/journal.pone.0117097
Xu, S. T., Xu, J. H., Zheng, Z. R., Zhao, Q. Q., Zeng, X. S., Cheng, S. X., et al. (2017). Long non-coding rna anril promotes carcinogenesis via sponging mir-199a in triple-negative breast cancer. Biomed. Pharmacother. 96, 14–21. doi:10.1016/j.biopha.2017.09.107
Yang, L., Wu, X., Wang, Y., Zhang, K., Wu, J., Yuan, Y. C., et al. (2011). FZD7 Has a Critical Role in Cell Proliferation in Triple Negative Breast Cancer. Oncogene 30 (43), 4437–4446. doi:10.1038/onc.2011.145
Yang, W., Cui, G., Ding, M., Yang, M., and Dai, D. (2020). MicroRNA-124-3p.1 Promotes Cell Proliferation through Axin1-dependent Wnt Signaling Pathway and Predicts a Poor Prognosis of Triple-Negative Breast Cancer. J. Clin. Lab. Anal. 34 (7), e23266. doi:10.1002/jcla.23266
Yao, H., Ashihara, E., and Maekawa, T. (2011). Targeting the Wnt/β-Catenin Signaling Pathway in Human Cancers. Expert Opin. Ther. Targets 15 (7), 873–887. doi:10.1517/14728222.2011.577418
Yin, L., Duan, J. J., Bian, X. W., and Yu, S. C. (2020). Triple-negative Breast Cancer Molecular Subtyping and Treatment Progress. Breast Cancer Res. 22 (1), 61. doi:10.1186/s13058-020-01296-5
Yin, S., Xu, L., Bonfil, R. D., Banerjee, S., Sarkar, F. H., Sethi, S., et al. (2013). Tumor-initiating Cells and FZD8 Play a Major Role in Drug Resistance in Triple-Negative Breast Cancer. Mol. Cancer Ther. 12 (4), 491–498. doi:10.1158/1535-7163.MCT-12-1090
Yu, L., Wang, C., Pan, F., Liu, Y., Ren, X., Zeng, H., et al. (2019). HePTP Promotes Migration and Invasion in Triple-Negative Breast Cancer Cells via Activation of Wnt/β-Catenin Signaling. Biomed. Pharmacother. 118, 109361. doi:10.1016/j.biopha.2019.109361
Yuan, C., Luo, X., Duan, S., and Guo, L. (2020). Long Noncoding RNA LINC00115 Promotes Breast Cancer Metastasis by Inhibiting miR-7. FEBS Open Bio 10 (7), 1230–1237. doi:10.1002/2211-5463.12842
Zhang, K., Liu, P., Tang, H., Xie, X., Kong, Y., Song, C., et al. (2018). AFAP1-AS1 Promotes Epithelial-Mesenchymal Transition and Tumorigenesis through Wnt/β-Catenin Signaling Pathway in Triple-Negative Breast Cancer. Front. Pharmacol. 9, 1248. doi:10.3389/fphar.2018.01248
Zhang, T., Hu, H., Yan, G., Wu, T., Liu, S., Chen, W., et al. (2019). Long Non-coding RNA and Breast Cancer. Technol. Cancer Res. Treat. 18, 1533033819843889. doi:10.1177/1533033819843889
Zhang, Y., and Wang, X. (2020). Targeting the Wnt/β-Catenin Signaling Pathway in Cancer. J. Hematol. Oncol. 13 (1), 165. doi:10.1186/s13045-020-00990-3
Zheng, A., Song, X., Zhang, L., Zhao, L., Mao, X., Wei, M., et al. (2019). Long Non-coding RNA LUCAT1/miR-5582-3p/TCF7L2 axis Regulates Breast Cancer Stemness via Wnt/β-Catenin Pathway. J. Exp. Clin. Cancer Res. 38 (1), 305. doi:10.1186/s13046-019-1315-8
Keywords: breast cancer, lncRNA, microRNA, wnt/β-catenin, pathogenesis
Citation: Hu X, Zhang Q, Xing W and Wang W (2022) Role of microRNA/lncRNA Intertwined With the Wnt/β-Catenin Axis in Regulating the Pathogenesis of Triple-Negative Breast Cancer. Front. Pharmacol. 13:814971. doi: 10.3389/fphar.2022.814971
Received: 14 November 2021; Accepted: 17 May 2022;
Published: 24 June 2022.
Edited by:
Mukerrem Betul Yerer Aycan, Erciyes University, TurkeyReviewed by:
Aklank Jain, Central University of Punjab, IndiaCopyright © 2022 Hu, Zhang, Xing and Wang. This is an open-access article distributed under the terms of the Creative Commons Attribution License (CC BY). The use, distribution or reproduction in other forums is permitted, provided the original author(s) and the copyright owner(s) are credited and that the original publication in this journal is cited, in accordance with accepted academic practice. No use, distribution or reproduction is permitted which does not comply with these terms.
*Correspondence: Wan Wang, d3dhbkBqbHUuZWR1LmNu
Disclaimer: All claims expressed in this article are solely those of the authors and do not necessarily represent those of their affiliated organizations, or those of the publisher, the editors and the reviewers. Any product that may be evaluated in this article or claim that may be made by its manufacturer is not guaranteed or endorsed by the publisher.
Research integrity at Frontiers
Learn more about the work of our research integrity team to safeguard the quality of each article we publish.