- State Key Laboratory of Trauma, Department of Army Occupational Disease, The Molecular Biology Center, Burn and Combined Injury, Daping Hospital, Army Medical University (Third Military Medical University), Chongqing, China
As a nonspecific antagonist of the adenosine A2A receptor (A2AR), caffeine enhances learning and improves memory impairment. Simultaneously, the consumption of caffeine correlates with a feeling of anxiety. The hippocampus is functionally differentiated along its dorsal/ventral axis and plays a crucial role both in memory and anxiety. Whether caffeine exerts its regulation by inhibiting A2ARs in different subregions of the hippocampus is still unknown. In the present study, we found that after chronic intake of drinking water containing caffeine (1 g/L, 3 weeks), mice exhibited aggravated anxiety-like behavior and enhanced memory function. Tissue-specific, functional disruption of dorsal hippocampal A2ARs by the CRE-LoxP system prevented the memory-enhancing effect of caffeine, while selective disruption of ventral hippocampal A2ARs blocked the impact of caffeine on anxiety. These results, together with the enhanced memory of dorsal hippocampus A2AR knockout mice and greater anxiety-like behavior of ventral hippocampus A2AR knockout mice without caffeine, indicates a dissociation between the roles of ventral and dorsal hippocampal A2A receptors in caffeine’s effects on anxiety-like and memory-related behavioral measures, respectively. Furthermore, optogenetic activation of dorsal or ventral hippocampal A2ARs reversed the behavioral alterations caused by drinking caffeine, leading to impaired memory or decreased anxiety-like behaviors, respectively. Taken together, our findings suggest that the memory- and anxiety-enhancing effects of caffeine are related to the differential effects of inhibiting A2ARs in the dorsal and ventral hippocampus, respectively.
Introduction
As the most widely consumed psychotropic substance and a component of the most popular beverages, caffeine is used to counteract performance impairments associated with sleep loss (Irwin et al., 2020).The consumption of caffeine attenuates memory impairments associated with aging and Alzheimer’s disease (AD) (Solfrizzi et al., 2015; Jacobson et al., 2020; Londzin, et al., 2021)and enhances memory in healthy humans (Borota et al., 2014; Irwin et al., 2020). However, controlled studies have confirmed that caffeine produces negative effects such as increased anxiety (Garcia and Salloum, 2015; Khurana and Bansal, 2019). Similarly, mouse studies have also shown that caffeine ingestion improves memory and induces anxiety (Xu and Reichelt, 2018). The main molecular targets of caffeine in the brain are adenosine receptors, including the inhibitory A1 receptor (A1R) and facilitatory A2A receptor (A2AR) (Ikram et al., 2020). From results obtained in animal models, it was further concluded that the effect of caffeine on both anxiety and memory performance was mimicked by the selective blockade of A2A, but not of A1 receptor (Kaster et al., 2015; Machado et al., 2017).
The hippocampus belongs to the limbic system and plays an important role in memory, spatial navigation and emotion. The hippocampus can be divided into two segments along its longitudinal axis: the dorsal and ventral subregions. Early anatomical studies demonstrated differences between the afferent and efferent nerve projections of the dorsal hippocampus (dHPC) and ventral hippocampus (vHPC) (Swanson and Cowan, 1977). According to a current consensus, the role played by the most dorsally located hippocampal segment is on cognitive operations like spatial navigation, while internally monitoring functions related to emotionality are taken on by the ventral segment of the hippocampus (Trompoukis and Papatheodoropoulos, 2020). Moreover, some studies have found that caffeine reverts memory impairment in a depression-prone mouse strain with upregulation of adenosine A2A receptors in the hippocampus (Machado et al., 2017), while selective A2AR knockout in the forebrain region (striatum, hippocampus, and cortex) induces anxiety-like behavior (Wei et al., 2014). All the evidence suggests that the hippocampal adenosine A2A receptor is likely to emerge as an important receptor in the regulation of caffeine on memory and anxiety. However, whether the different effects of caffeine on overall function are derived from its action on adenosine A2A receptors in different subregions of the hippocampus is still unclear.
To explore the above question, we first verified that overall A2AR knockout reduced the effect of caffeine on mice. Furthermore, we found that localized knockout of dHPC A2ARs reduced the regulation of caffeine on memory but did not affect anxiety, whereas localized knockout of vHPC A2ARs reduced the regulation of caffeine on anxiety without affecting memory. To confirm the memory-enhancing effects of dHPC A2AR knockout, we assessed the level of SNAP-25, a synaptic marker reflecting synapse formation and remodeling (Batista et al., 2017). To confirm the anxiogenic effects of vHPC A2AR knockout, we assessed the level of SNAP-25, a glutamatergic-selective marker and labels excitatory glutamatergic neurons (Zheng et al., 2015; Martineau et al., 2017). Finally, specific stimulation of dorsal/ventral hippocampal A2ARs by optogenetic techniques demonstrated that dorsal hippocampal A2AR activation impaired memory while ventral hippocampal A2AR activation reduced anxiety. Therefore, our experiments showed that caffeine regulates memory and anxiety by inhibiting the dorsal and ventral hippocampal A2ARs, respectively.
Materials and Methods
Animals
Adult male C57BL/6 mice (weighing 25–30 g, 11–13 weeks old) were purchased and used in our study. Global A2AR knockout mice were established on a C57BL/6 background as our previously described (Zeng et al., 2020), and their littermates were used as wild-type (WT) mice in this experiment. Mice with a ‘floxed’ adenosine A2AR gene (A2Aflox/flox mice) created by insertion of loxP sequences into introns flanking an exon of the A2AR gene (Bastia et al., 2005; Yu et al., 2009) were provided by Dr. Chen. The experimental procedures were performed in accordance with the guidelines of the Animal Ethical and Welfare Committee of the Army Medical University.
Viral Production
For conditional knockout (Cre/loxp system) of neuronal A2AR, pAAV-hSyn-EGFP-2A-CRE virus and pAAV-hSyn-MCS-EGFP-3Flag virus (control virus) were packaged and supplied by OBiO Technology (Shanghai) Corp. Ltd.
For optogenetic manipulations of neuronal A2AR, a chimeric rhodopsin-A2AR protein (optoA2AR) was developed by replacing the intracellular domain of rhodopsin with that of A2AR as described in our previous research (Li et al., 2015). Extracellular adenosine or caffeine cannot react with optoA2AR. Similarly, pAAV-CaMKIIa-optoA2AR-mCherry virus and pAAV-CaMKIIa-MCS-mCherry-3FLAG virus (control virus) were constructed and supplied by OBiO Technology (Shanghai) Corp. Ltd. All viruses were used at titers of ∼4–8*1012 vg/ml.
Drug Treatments
In the first part of our experiment, mice (knockout or wild-type) were randomly allocated to two groups: a control group provided with drinking water without drug and a treatment group provided with drinking water containing caffeine (1 g/L, Sigma) starting 3 weeks before behavioral tests. This dose and schedule of administration of caffeine was chosen since it was sufficient to improve memory and increase anxiety according to a previous study (Kaster et al., 2015).
In the second part of the study, A2Aflox/flox mice were injected with CRE virus or EGFP virus. Half the mice in each group were then provided with caffeine-containing drinking water starting 3 weeks before behavioral tests, and the other half were provided with normal drinking water.
In the last part, wild-type mice were randomized into two groups: an experimental group injected with the optoA2AR virus and a control group were injected with the mCherry virus. Then, all mice underwent optical fiber implantation and were treated with caffeine starting 3 weeks before optogenetic manipulation.
In all experiments, the drinking water with or without caffeine were provided every day, until the mice were killed.
Animal Surgery: Virus Injection and Optical Fiber Implantation
For all surgical procedures, mice were anesthetized with 1.5% isoflurane at an oxygen flow rate of 1 L/min and then immobilized in a Robot Stereotaxic apparatus (Neurostar, Tübingen, Germany). The fur was shaved, and a midline scalp incision was made for surgical procedures. After surgery, the mice were given saline containing buprenorphine (0.13 mg/kg, subcutaneously) for 3 days for analgesia.
For brain region-specific knockout of A2AR, 1.5 μL pAAV-hSyn-EGFP-2A-CRE virus (or control virus) was injected into the each dHPC (AP: 1.1 mm; ML: ±1.25 mm; DV: +1.75 mm) or each vHPC (AP: 3.4 mm; ML: ±2.5 mm; DV: +4.0 mm) of A2Aflox/flox mice (Total 3.0 μL).
To express optoA2AR in hippocampal neurons, we injected 1.5 μL pAAV-CaMKIIa-OptoA2A (A400S)-mCherry virus (or control virus) into the left dorsal or ventral subregion (viral injection coordinates are described above). Four weeks after injection, a 200 μm optical fiber (Shanghai Fiblaser Technology Co., Ltd.) was implanted in the same coordinates. Via a patch cable, the optical fiber was connected to a 473 nm DPSS laser (100 mW; Shanghai Laser and Optics Century). The power density at the fiber tip was approximately 5 mW/mm2, and light was delivered with a 50 m pulse width (10Hz). For the optogenetic experiment, mice were habituated with an optical fiber connected to the optical patch cable without laser stimulation for 30 min before the behavior tests, and optical stimulation was delivered specifically according to the different behavioral tests (see below). For immunofluorescence analyses, mice were euthanized by cervical dislocation following 10 min of optical stimulation.
Behavioral Experiments
To eliminate the acute effects of caffeine while preserving its long-term chronic effects, all behavioral tests were performed within 24–48 h after 3-week caffeine treatment.
Open Field Test: Mice were placed in a square chamber (40 cm × 40 cm × 40 cm length-width-height) with a dimly lit (approximately 65 lux). The center zone was a 20 × 20 cm square. Mice were allowed to freely explore the environment for 5 min, while activities were recorded and analyzed by EthoVision XT behavioral tracking software (Noldus Information Technology Inc.). For optogenetic manipulation, light stimulation was maintained for the entire 5-min duration of the open-field test.
Elevated Plus Maze (EPM) Test: The elevated plus maze had open and closed arms (30 cm × 8.5 cm length-width, 20 cm tall closed arms) that extended from a central platform (8.5 × 8.5 cm). The maze was placed at a height of 58 cm and the room light was about 65 lux. The mouse was placed in the central platform of the maze facing an open arm and were allowed to freely explore the EPM for 5 min, and their activities were recorded and analyzed by EthoVision software (Noldus Information Technology Inc.). For optogenetic experiments, light stimulation was used for a total period of 5 min while mice explored the EPM.
Novel Object Recognition (NOR) Test: NOR test was carried out in a home-cage arena (45 cm × 45 cm × 50 cm length-width-height) which they were allowed to freely explore for 10 min on the previous day. In the training trial on the next day, mice were presented with two same objects (a red cube, 2.8 cm × 2.8 cm × 2.8 cm) placed in opposite corners for 10 min. The exploration of the objects, defined by mice showing investigative behaviors (head orientation or sniffing) or playing within 1 cm around the object, was measured. In the testing trial (24 h later), one of the identical objects was changed for a novel object (a yellow pyramid, height 3.0 cm, base 2.8 cm × 2.8 cm), and the animals were left in the cage for 10 min. The exploration time for the familiar and the novel object during the test phase was recorded with EthoVision software. For the optogenetic experiment, light stimuli were only delivered during the testing trial.
Y-Maze Test: Y-maze test was carried out in a gray maze formed by three arms (28 cm × 8.5 cm × 20 cm length-width-height, 58 cm above the ground) so as to form a Y shape. Each mouse was first allowed to explore the maze for 5 min while one arm was blocked (acquisition phase). After 2 h, mice had access to all three arms for a 5 min period (retrieval phase). During the second period, the time spent in each arm was measured by a video-tracking system (Noldus Information Technology Inc.). For the optogenetic experiment, light stimuli were only presented during the retrieval trial.
Immunofluorescence
Following behavioral experiments or light stimulation, mice were sequentially perfused with saline and 4% paraformaldehyde in PBS. Brains were postfixed, and coronal sections (30 μm) were cut and prepared for immunofluorescence. Free-floating sections were washed in PBS and then incubated for 30 min in 0.3% Triton X-100 and 3% BSA or goat serum. Sections were incubated with the following primary antibodies overnight at 4°C: anti-A2AR (1:200, Frontier Institute, AB_2571655), anti-c-Fos (1:50, Santa Cruz, sc-271243), anti-synaptosomal-associated protein 25 (SNAP-25; 1:100, Abcam, ab5666) and anti-vesicular glutamate transporter 1 (vGluT1; 1:100, Abcam, ab227805). Sections were then washed with PBS and incubated with fluorescence-tagged secondary antibodies including Cy3 (1:500, donkey anti-goat, Abcam, ab6949), Cy3 (1:500, goat anti-mouse, Abcam, ab97035), Cy3 (1:500, goat anti-rabbit, Abcam, ab6939), and Alexa Fluor 488 (1:500, goat anti-mouse, Abcam, ab150117) for 1 h at 37°C. Nuclei were subsequently stained with DAPI (Santa Cruz, sc-359850). High-magnification images were captured using a confocal laser-scanning microscope (Leica TCS-SP2, laser lines at 488, 543, 633) and analyzed with Image-Pro Plus 4.5 software. For quantification of immunofluorescence, at least 50 cells were evaluated in each field (3 fields per slice, three slices per mouse, three mice from each analyzed group).
Statistical Analysis
Results are expressed as the means ± SEM. All semi-quantitative assessments of histological staining were made by a single investigator blinded to the genotype and treatment of the experimental animals. Sample size was chosen according to previous reports and our pre-experiments. Two-way analyses of variance (ANOVAs) were used to assess the effects of caffeine, gene manipulation and the caffeine × gene manipulation interaction in the A2AR total (or region-specific) knockout mice. Two-way ANOVAs were used to assess the effects of optoA2AR virus, light stimulation and the optoA2AR virus × light stimulation interaction in the optoA2AR mice. A value of p < .05 was considered statistically significant.
Results
Global A2AR Knockout Blocked the Effect of Caffeine on Anxiety and Memory
The open-field test and EPM test were used to evaluate anxiety-like behaviors. Chronic caffeine consumption (3 weeks) significantly reduced the time (Figure 1A, for caffeine, F(1,28) = 5.741, p < .01; for knockout, F(1,28) = 16.341, p < .01; for caffeine × knockout interaction, F(3,28) = 4.124, p < .01) and distance (Figure 1B, for caffeine, F(1,28) = 56.114, p < .001; for knockout, F(1,28) = 23.554, p < .01; for caffeine × knockout interaction, F(3,28) = 4.451, p < .01) in the center of the open field, and had no significant effect on the total distance (Supplementary Figure S1A, for caffeine, F(1,28) = 0.372, p = .547; for knockout, F(1,28) = .001, p = .975; for caffeine × knockout interaction, F(3,28) = 0.985, p = 0.331). In the EPM, caffeine treatment decreased the percentage of time (Figure 1D, for caffeine, F(1,28) = 9.125, p < .01; for knockout, F(1,28) = 21.934, p < .01; for caffeine × knockout interaction, F(3,28) = 23.344, p < .01) and number of entries (Figure 1C, for caffeine, F(1,28) = 14.624, p < .01; for knockout, F(1,28) = 21.679, p < .01; for caffeine × knockout interaction F(3,28) = 8.824, p < .01) in the open arms which confirmed the open-field test results. We next examined object recognition to evaluate the role of caffeine in memory formation. The caffeine-treated mice showed greater exploration of the novel object over the familiar object (Figure 1E, for caffeine, F(1,28) = 12.347, p < .01; for knockout, F(1,28) = 15.472, p < .01; for caffeine × knockout interaction, F(3,28) = 14.147, p < .01). The spatial recognition memory of mice was probed using a two-trial Y-maze paradigm. Likewise, caffeine-treated mice showed increased time in the novel arm compared with control mice (Figure 1F, for caffeine, F(1,28) = 22.712, p < .01; for knockout, F(1,28) = 15.755, p < .01; for caffeine × knockout interaction, F(3,28) = 21.457, p < .01). This finding suggests that chronic caffeine consumption for 3 weeks enhanced memory performance in mice.
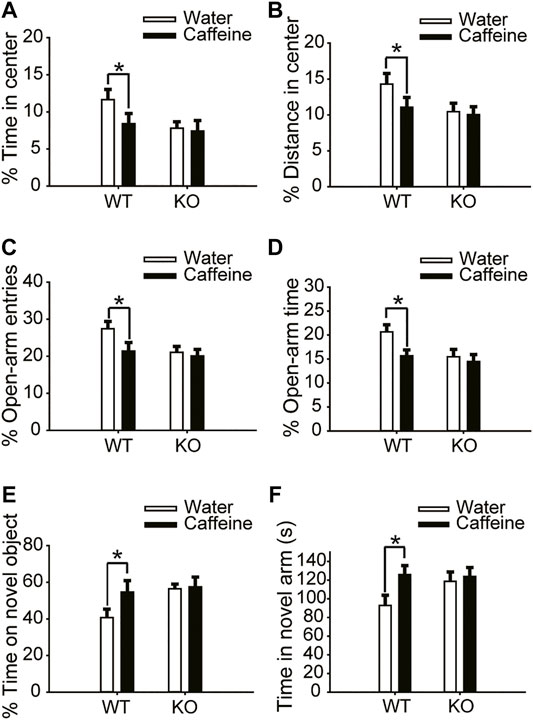
FIGURE 1. Effects of chronic caffeine consumption on wild-type mice and A2AR knockout mice in behavioral experiments. (A,B) Behavior of the four groups of mice in the open-field test. Caffeine induced anxiogenic behavior with decreased time in the center (A) and distance in the center (B) in wild-type mice. (C,D) Behavior of the four groups of mice in the Elevated Plus Maze (EPM) test. Caffeine decreased the percentage of open-arm entries (C) and the percentage of time spent in the open arms (D) in wild-type mice. (E) Behavior of the four groups of mice in the Novel Object Recognition (NOR) test. The time spent exploring a novel object is shown as the ratio of the total time spent exploring both objects in the testing trial. (F) Behavior of the four groups of mice in the Y-maze test. The time spent in the novel arm is shown during the retrieval phase. Data are presented as the mean ± SEM, n = 7 mice per group; *p < .05, two-way ANOVA, Bonferroni post hoc t-test.
However, chronic caffeine consumption did not alter anxiety-like behaviors in global A2AR knockout mice. There were no significant differences between caffeine-treated mice (caffeine + knockout) and control mice (water + knockout) in either the open-field test or the EPM test (Figure 1C and Figure 1D). At the same time, A2AR knockout blocked the effect of caffeine in the NOR test and Y-maze test (Figures 1E,F), suggesting that the effect of caffeine on memory was prohibited by A2AR inactivation. It is worth noting that in global A2AR knockout mice, there were baseline differences in behaviors that are independent of caffeine (Figures 1A–F). But the costimulation with caffeine and A2AR knockout did not produce additive effects which reflected the interaction between caffeine-A2AR function.
Dorsal Hippocampal A2AR Knockout Blocked the Caffeine-Induced Enhancement of Memory
Six weeks after the injection of pAAV-syn-EGFP-2A-CRE virus in the dHPC of A2Aflox/flox mice (Figure 2G), the A2AR level was significantly reduced (Figure 2H). Then, after drinking caffeine for 3 weeks, mice showed reduced center distance (Figure 2A, for caffeine, F(1,28) = 7.473, p < .01; for CRE, F(1,28) = 17.453, p = .413; for caffeine × CRE interaction, F(3,28) = 32.913, p = .302) and center time (Figure 2B, for caffeine, F(1,28) = 15.417, p < .01; for CRE, F(1,28) = 32.348, p = .235; for caffeine × CRE interaction, F(3,28) = 42.374, p = .328) in the open-field test, and had no significant effect on the total distance (Supplementary Figure S1B, for caffeine, F(1,28) = .042, p = 0.839; for CRE, F(1,28) = 1.817, p = .190; for caffeine × CRE interaction, F(3,28) = 0.767, p = .389). At the same time, caffeine reduced the number of entries (Figure 2C, for caffeine, F(1,28) = 5.113, p < .01; for CRE, F(1,28) = 15.457, p = .191; for caffeine × CRE interaction, F(3,28) = 21.479, p = .208) and duration of time (Figure 2D, for caffeine, F(1,28) = 3.323, p < .01; for CRE, F(1,28) = 16.278, p = .058; for caffeine × CRE interaction, F(3,28) = 22.143, p = .572) spent in the open arm of the EPM. These results indicate that dHPC A2AR knockout did not affect the caffeine-induced anxiety-like behavior in mice.
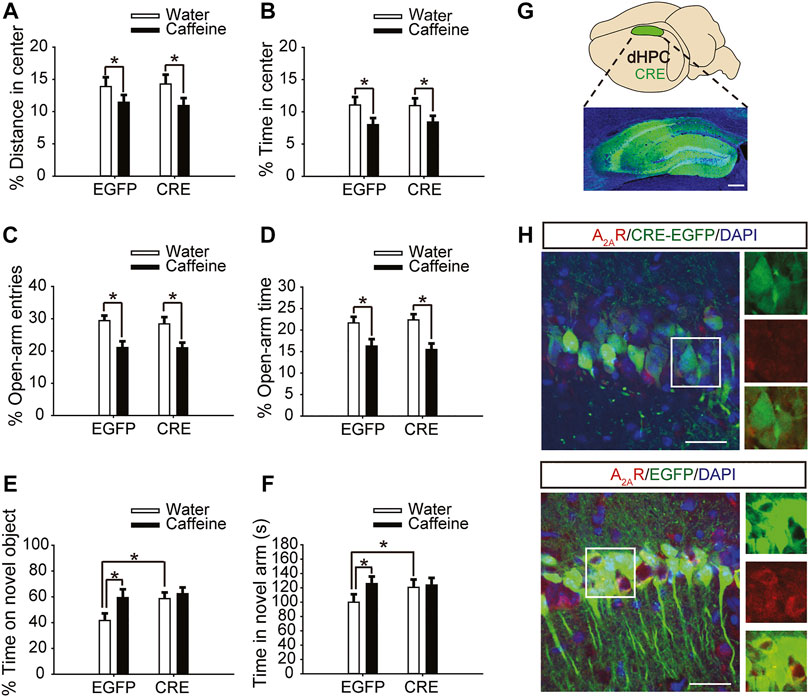
FIGURE 2. Selective expression of pAAV-CRE in the dHPC triggers memory enhancement. (A,B) Behavior of the four groups of mice in the open-field test. Caffeine decreased the time in the center (B) and distance in center (A) in CRE and EGFP mice. (C,D) Behavior of the four groups of mice in the Elevated Plus Maze (EPM) test. Caffeine decreased the percentage of open-arm entries (C) and the percentage of time spent in the open arms (D) in CRE and EGFP mice. (E) Behavior of the four groups of mice in the Novel Object Recognition (NOR) test. The time spent exploring a novel object was increased by caffeine only in EGFP mice during the testing trial. (F) Behavior of the four groups of mice in the Y-maze test. The time spent in the novel arm was increased by caffeine only in EGFP mice during the retrieval phase. (G) Selected expression of AAV-CRE in the dHPC (scale bar = 500 μm). (H) Images of hippocampal brain sections obtained from mice injected with AAV-CRE or AAV-EGFP (scale bar = 30 μm). The level of A2AR (red) was lower in CRE-positive cells (upper panel) than in cells transfected with pAAV-EGFP (lower panel). Data are presented as the mean ± SEM; n = 7 mice per group; *p < .05, two-way ANOVA, Bonferroni post hoc t-test.
However, after inactivation of dorsal hippocampal A2ARs, there was no significant difference in behavior in the NOR test (Figure 2E, for caffeine, F(1,28) = 1.413, p < .01; for CRE, F(1,28) = 19.413, p < .01; for caffeine × CRE interaction, F(3,28) = 15.439, p < .01) or Y-maze test (Figure 2F, for caffeine, F(1,28) = 17.435, p < .01; for CRE, F(1,28) = 10.235, p < 0.01; for caffeine × CRE interaction, F(3,28) = 5.636, p < .01) between the caffeine-treated group and the control group, indicating that dHPC A2AR knockout eliminated the effect of caffeine on memory. Immunofluorescence results showed that the level of SNAP-25 in the pAAV-EGFP group given caffeine (EGFP + caffeine) was significantly higher than that in the group given water (EGFP + water). However, caffeine did not alter the expression of SNAP-25 in the pAAV-CRE group (Figure 3). Interestingly, the NOR test and Y-maze test showed that dorsal hippocampal A2AR knockout alone improved memory similar to chronic caffeine consumption (Figures 2E,F), and these behavioral changes were in accordance with the trend observed in SNAP-25 expression (Figure 3). This evidence suggests that the effect of caffeine on memory in mice is derived from its action on dorsal hippocampal A2ARs.
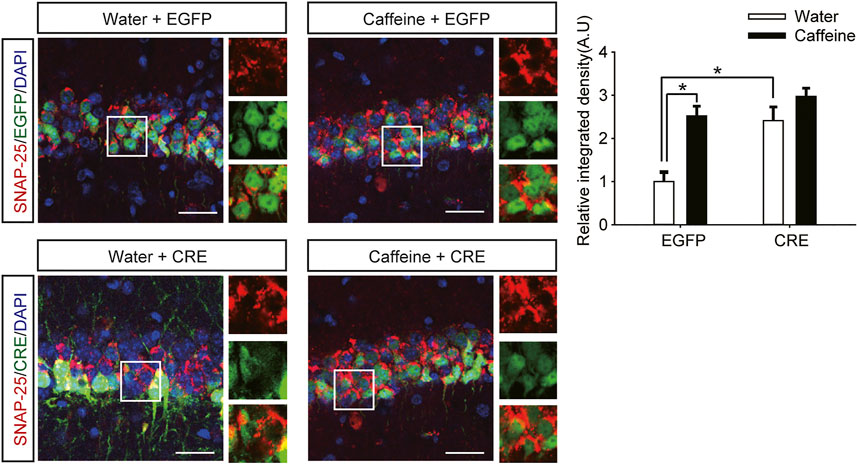
FIGURE 3. Selective deletion of dHPC A2ARs improves SNAP-25 expression. Caffeine promoted SNAP-25 expression more in mice with the dHPC transfected with pAAV-EGFP than in mice transfected with pAAV-CRE. pAAV-CRE transfection also produced an additive effect on SNAP-25 levels independently. Representative images (left) and data (right) are presented as the mean ± SEM; n = 27 fields per group (3 fields per section, three sections per mouse, three mice per group). Scale bar = 30 μm.
Ventral Hippocampal A2AR Knockout Prevented Caffeine-Induced Anxiety
After expression of pAAV-syn-EGFP-2A-CRE in the vHPC (Figure 4G), chronic caffeine consumption for 3 weeks had no significant effect on behavior in the open-field test or EPM test (Figures 4A–D), indicating that inactivation of vHPC A2ARs abolished the anxiogenic effect of caffeine. At the same time, mice in the pAAV-CRE group (CRE + water) showed greater anxiety-like behavior than pAAV-EGFP mice (EGFP + water) in the open-field test (Figure 4A, for caffeine, F(1,28) = 15.445, p < .01; for CRE, F(1,28) = 17.609, p < .01; for caffeine × CRE interaction, F(3,28) = 5.235, p < .01; Figure 4B, for caffeine, F(1,28) = 7.143, p < .01; for CRE, F(1,28) = 2.439, p < .01; for caffeine × CRE interaction, F(3,28) = 8.833, p < .01) and EPM test (Figure 4C, for caffeine, F(1,28) = 11.415, p < .01; for CRE, F(1,28) = 11.961, p < .01; for caffeine × CRE interaction, F(3,28) = 9.675, p < .01; Figure 4D, for caffeine, F(1,28) = 21.435, p < .01; for CRE, F(1,28) = 19.495, p < .01; for caffeine × CRE interaction, F(3,28) = 5.195, p < .01), and had no significant effect on the total distance in the open-field test (SupplementaryFigure S1C, for caffeine, F(1,28) = 0.108, p = .746; for CRE, F(1,28) = 1.838, p = .188; for caffeine × CRE interaction, F(3,28) = 1.367, p = .252). The levels of vGLuT1 in the four groups of mice (Figure 5) were consistent with the trend in behavior. Thus, these findings indicate that the anxiogenic effect of chronic caffeine consumption stems from its inhibition of ventral hippocampal A2ARs.
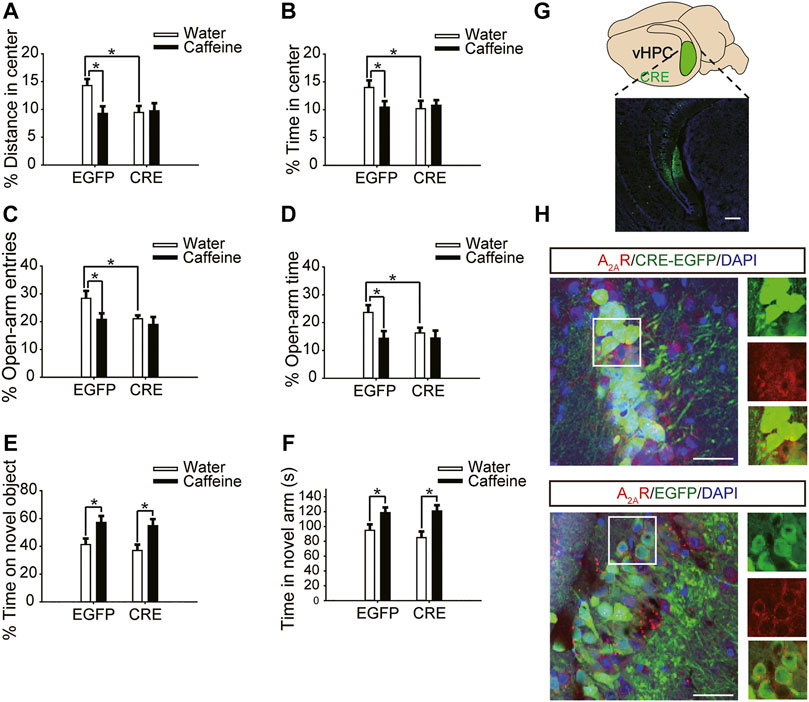
FIGURE 4. Selective deletion of vHPC A2ARs triggers anxiety-like behaviors. (A,B) Behavior of the four groups of mice in the open-field test. Caffeine induced anxiogenic behavior with decreased time in the center (B) and distance in the center (A) only in EGFP mice. (C,D) Behavior of the four groups of mice in the Elevated Plus Maze (EPM) test. Caffeine decreased the percentage of open-arm entries (C) and the percentage of time spent in the open arms (D) only in EGFP mice. (E) Behavior of the four groups of mice in the Novel Object Recognition (NOR) test. The time spent exploring a novel object was increased by caffeine in EGFP and CRE mice during the testing trial. (F) Behavior of the four groups of mice in the Y-maze test. The time spent in the novel arm was increased by caffeine in EGFP and CRE mice during the retrieval phase. (G) Expression of AAV-CRE or AAV-EGFP in the vHPC (scale bar = 500 μm). Data are presented as the mean ± SEM; n = 7 mice per group; *p < .05, two-way ANOVA, Bonferroni post hoc t-test.
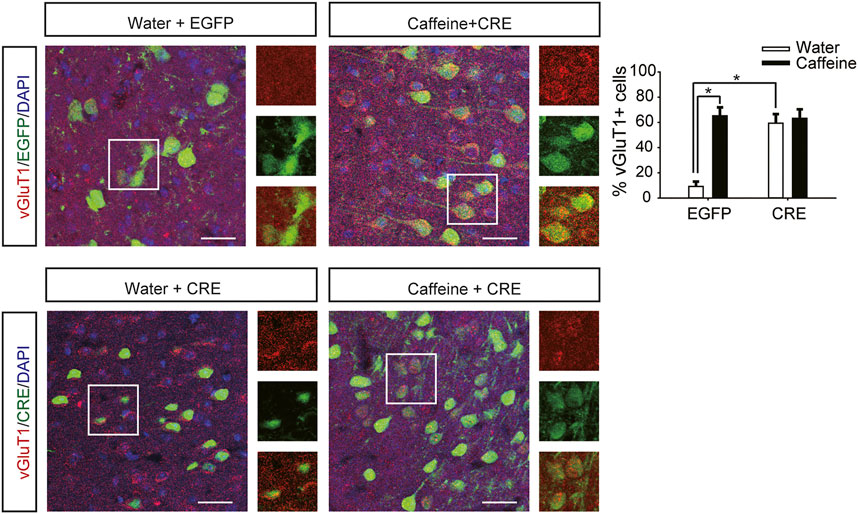
FIGURE 5. Selective deletion of vHPC A2ARs increases vGluT1 expression. Caffeine markedly induced vGluT1 expression in mice transfected with pAAV-EGFP but not in mice transfected with pAAV-CRE. pAAV-CRE transfection increased vGluT1 levels in mice exposed to water alone as well. Representative images (left) and Data (right) are presented as the mean ± SEM; n = 27 fields per group (3 fields per section, three sections per mouse, three mice per group). Scale bar = 30 μm.
Caffeine in drinking water still increased the time spent exploring a novel object (Figure 4E, for caffeine, F(1,28) = 3.239, p < .01; for CRE, F(1,28) = 13.319, p = .279; for caffeine × CRE interaction, F(3,28) = 21.173, p = .064) and the exploration time of the new arm (Figure 4F, for caffeine, F(1,28) = 7.631, p < .01; for CRE, F(1,28) = 23.659, p = .288; for caffeine × CRE interaction, F(3,28) = 31.849, p = 0.233) in both the pAAV-CRE and pAAV-EGFP mice, indicating that the ablation of ventral hippocampal A2ARs did not affect the memory-enhancing effect of caffeine.
Brain Region-specific Activation of dHPC/vHPC A2ARs Reversed the Regulation of Caffeine on Memory and Anxiety
Six weeks after dHPC/vHPC injection of pAAV-CaMKIIa-optoA2AR-mCherry (Figures 6A,7A), mice were exposed to caffeine in drinking water for 3 weeks. Light stimulation for 5 min significantly increased the levels of c-Fos in pAAV-optoA2AR mice but not in pAAV-mCherry mice (Figure 6D), indicating that illumination was sufficient to activate the optoA2AR signaling pathway.
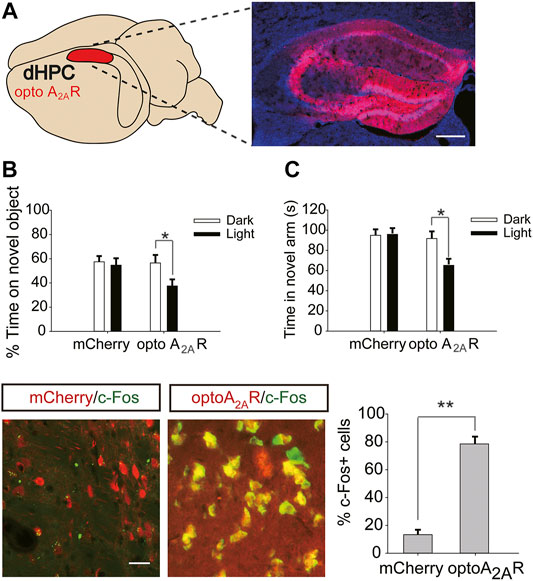
FIGURE 6. Targeted expression and light activation of optoA2AR in the dHPC prevents the memory improvement caused by caffeine consumption. (A) Selected expression of AAV-optoA2AR or AAV-mCherry in the dHPC (Scale bar = 500 μm). (B) Behavior of mice in the Novel Object Recognition (NOR) test (n = 7 mice per group). C Behavior of the four groups of mice in the Y-maze test (n = 7 mice per group). Light stimulation of opto-A2AR in the dHPC increased the time spent exploring a novel object during the testing trial (B; *p < .05 using a two-way ANOVA followed by Bonferroni post hoc t-test) and the time spent in the novel arm during the retrieval phase (C; *p < .05 using a two-way ANOVA followed by Bonferroni post hoc t-test). (D) Light stimulation for 5 min induced c-Fos expression in optoA2AR-positive cells but not in cells transfected with AAV-mCherry. Representative images (left) and data (right) are presented. n = 27 fields per group (3 fields per section, three sections per mouse, three mice per group); Scale bar = 50 μm; **p < .001, Student’s t-test comparing optoA2AR with mCherry. Data are presented as the mean ± SEM.
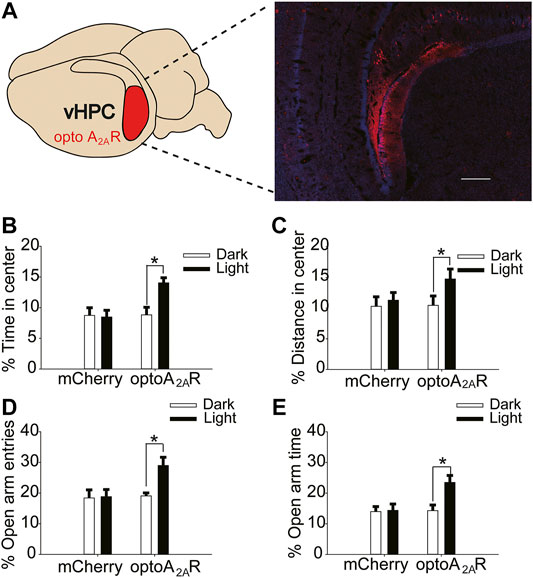
FIGURE 7. Targeted expression and light activation of optoA2AR in the vHPC alleviates caffeine-induced anxiety. (A) Selected expression of AAV-optoA2AR or AAV-mCherry in the vHPC (Scale bar = 500 μm). (B,C) Behavior of the four groups of mice in the open-field test (n = 7). Light stimulation of opto-A2AR in the dHPC increased the percentage of open-arm entries (B) and the percentage of time spent in the open arms (C). (D,E) Behavior of the four groups of mice in the Elevated Plus Maze (EPM) test (n = 7). Light stimulation of opto-A2AR in the dHPC increased the percentage of open-arm entries (D) and the percentage of time spent in the open arms (E). Data are presented as the mean ± SEM. *p < .01 using two-way ANOVA followed by Bonferroni post hoc t-test.
After optogenetic optoA2AR activation in the dHPC, both the time spent exploring novel objects (Figure 6B, for optoA2AR virus, F(1,28) = 3.891, p = .105; for light stimulation, F(1,28) = 11.391, p < .05; for virus × light interaction, F(3,28) = 25.317, p = .091) and time spent in the novel arm (Figure 6C for optoA2AR virus, F(1,28) = 16.132, p = .067; for light stimulation, F(1,28) = 13.639, p < .05; for virus × light interaction, F(3,28) = 12.558, p = .334) were significantly decreased, indicating that specific activation of dorsal hippocampal A2ARs triggered obvious memory impairments in mice exposed to caffeine and further suggesting that caffeine promotes memory function by inhibiting dorsal hippocampal A2ARs.
In addition, light activation of optoA2AR in the vHPC significantly enhanced the proportion of time spent in the center and distance in the center in the open-field test (Figure 7B, for optoA2AR virus, F(1,28) = 3.229, p = .255; for light stimulation, F(1,28) = 8.296, p < .01; for virus × light interaction, F(3,28) = 26.539, p = .539; Figure 7C, for optoA2AR virus, F(1,28) = 11.453, p = .548; for light stimulation, F(1,28) = 13.231, p < .05; for virus × light interaction, F(1,28) = 23.665, p = .792), and had no significant effect on the total distance (Figure S 1Dfor optoA2AR virus, F(1,28) = 0.299, p = .589; for light interaction, F(1,28) = 0.088, p = .769; for virus × light interaction, F(3,28) = 0.246, p = .624).Furthermore, the number of open-arm entries and duration of time spent in the open arms in the EPM increased markedly with light activation (Figure 7D, for optoA2AR virus, F(1,28) = 21.238, p = .459; for light stimulation, F(1,28) = 17.487, p < .001; for virus × light interaction, F(3,28) = 28.292, p = .428; Figure 7E, for optoA2AR virus, F(1,28) = 3.241, p = .536; for light stimulation, F(1,28) = 14.549, p < .01; for virus × light interaction, F(3,28) = 13.449, p = .413). These findings indicate that specific activation of ventral hippocampal A2ARs inhibited the anxiety-like behaviors of mice treated with caffeine and affirm that caffeine-induced anxiety originates from its suppression of ventral hippocampal A2ARs.
Discussion
In the present study, we demonstrated that chronic caffeine consumption (1 g/L, 3 weeks) increased anxiety-like behavior, as assessed by the open-field test and EPM, and enhanced memory, as reflected in the NOR and Y-maze. Consistent with our results, caffeine has been shown to reverse cognitive impairments in aging and Alzheimer’s disease (AD) (Solfrizzi et al., 2015; Londzin et al., 2021) and lead to depression and anxiety-like behaviors (Richards and Smith, 2015; Mikkelsen et al., 2017). Acute caffeine consumption is widely used to counteract mood and performance impairments associated with sleep loss (Irwin et al., 2020). To eliminate the acute effects of caffeine while preserving its long-term chronic effects, all behavioral tests were performed after 3-week caffeine treatment. Chronic but not acute treatment with caffeine is considered to induce those behavioral alterations. As caffeine is most widely consumed all over the world, our exploration of chronic caffeine consumption is more meaningful. Moreover, caffeine seemed to have no effects on anxiety and memory in our total A2AR knockout mice. Statistical analysis also showed the strong interactions between caffeine and A2AR function, which suggests that caffeine functions through the antagonism of A2ARs. The main targets for caffeine in the brain are the inhibitory A1R and the facilitatory A2AR (Fredholm et al., 2005). Which is different from anti-inflammatory effect of A1R against noxious brain conditions (Martins et al., 2015), A2AR may play a more important role in memory and anxiety.
Many evidence suggests that pathological brain conditions associated with memory impairment are accompanied by a local increase of the extracellular levels of adenosine (Chen et al., 2013) and an up-regulation and aberrant signaling of the brain A2AR (Cunha and Agostinho, 2010; Chen et al., 2013). Thus, A2AR blockade could attenuate the impairment of brain function (Cunha, 2016) and memory function in particular (Cunha and Agostinho, 2010). However, whether A2AR blockade increases learn and memory in healthy animals remains contentious. Several groups reported that A2AR blockade and caffeine did not increase memory performance in control rodents, whereas stressed mice displayed increased memory performance upon caffeine consumption or upon blocking A2AR (Prediger et al., 2005; Kaster et al., 2015; Laurent et al., 2016; Carvalho et al., 2019). But genetic KO studies have shown that inactivation of A2AR is sufficient to improve memory in healthy animals (Zhou et al., 2009; Wei et al., 2011). The mechanism by which genetic inactivation of A2ARs strengthens memory is not clear. loss of A2ARs may impact cortical function through neuronal networks such as basal ganglia loop (Zhou et al., 2009) or potentiate striatal dopaminergic signaling via D2Rs to produce the memory enhancement (Wei et al., 2011).
While studies have shown that intraperitoneal injection of the A2AR agonist CGS21680 produces strong anxiety-like behavior (El Yacoubi et al., 2000), in our study, the A2AR knockout mice showed greater anxiety and enhanced memory compared to wild-type littermates. Considering that A2AR is widely distributed in the brain, the overall effect of drinking caffeine is likely be a superposition of its antagonism towards A2AR in multiple brain regions. Therefore, A2AR in a particular brain subregion may be responsible for caffeine’s effects on anxiety-like and memory-related behaviors.
Another major advance provided by this study is the dissociation of the roles of the ventral and dorsal hippocampal A2A receptors in caffeine’s effects on anxiety-like and memory-related behavioral measures, respectively, which are also consistent with the reported roles of the dorsal and ventral hippocampus more generally (Fanselow and Dong, 2010; McHugh et al., 2011; Schumacher et al., 2018). We discovered that knocking out dorsal hippocampal A2ARs blocked the memory-enhancing effects of caffeine without affecting its anxiogenic effects, whereas knocking out ventral hippocampal A2ARs did not affect the memory-enhancing effects of caffeine but blocked its anxiogenic effects. In addition, there were baseline differences in the behaviors of site-specific A2AR knockout mice, and statistical analysis confirmed caffeine-A2AR interactions. These results indicate that caffeine modulates memory by inhibiting dorsal hippocampal A2AR and modulates anxiety by acting through ventral hippocampal A2AR.
To confirm the memory-enhancing effects dHPC A2AR knockout, we assessed the level of SNAP-25. Our study showed that inactivation of dHPC A2AR upregulated the density of synaptic proteins, consistent with a previous study that showed that activation of hippocampal A2AR is sufficient to attenuate synaptic plasticity and further impair memory (Li et al., 2015). In recent years, synaptic density and synaptic connections in the hippocampus have been associated with learning and memory (Benito and Barco, 2010; Latina et al., 2017), and the overexpression of adenosine receptors revealed a hippocampal LTD-to-LTP shift to impair synaptic plasticity (Temido-Ferreira et al., 2018). Thus, chronic caffeine consumption may affect synaptic function to enhance memory by inhibiting dHPC A2ARs. However, the specific mechanism remains to be further confirmed.
One of the most important hypotheses of anxiety disorder is inhibition/excitation imbalance (Colic et al., 2018). As vGluT1 is a glutamatergic-selective marker and labels excitatory glutamatergic neurons (Zheng et al., 2015; Martineau et al., 2017), upregulation of vGluT1 reflects excessive excitation and inhibition/excitation imbalance in vHPC to some extent. It is also consistent with the greater anxiety-like behavior induced by caffeine and dHPC adenosine A2A receptor knockout. It is unclear whether caffeine regulates anxiety through the alteration of vGluT1, but the activation of adenosine A2A receptor has been reported to reduce GluT and glutamate uptake in cultured astrocytes and gliosomes (Matos et al., 2012). Therefore, the upregulation of vGluT1 may underlie the effect of caffeine on anxiety via inhibition of vHPC adenosine A2A receptor.
As a supplement to the brain region-specific knockout of adenosine A2A receptor, an optoA2AR approach to mimic endogenous A2AR signaling was used. Due to the specific construct, overexpression of optoA2AR did not generate baseline effects and caffeine could not react with optoA2AR too. After 3-weeks antagonism by caffeine, endogenous A2AR signaling was inhibited, optogenetic activation of optoA2AR captured the physiological function of the native A2AR. Light activation of dHPC adenosine A2A receptors reversed the behavioral alterations caused by caffeine, leading to impaired memory, while light activation of vHPC adenosine A2A receptors decreased caffeine-induced anxiety, further confirming the dissociation between the roles of the ventral and dorsal hippocampal A2A receptors in caffeine’s effects. Considering that we examined the behavioral effects of withdrawal from a chronic regime of caffeine administration, caffeine may induce compensatory effects in response to long-term drug exposure. The instant but significant effect of optoA2AR suggests the specificity of A2AR signaling rather than the compensatory effects.
Since adeno-associated viral vectors driven by either the synapsin- (syn-) or CaMKIIa promoter were employed, our results suggested that the effects of caffeine may result from specific inhibition of neuronal adenosine A2A receptors, consistent with previous studies on the role of neuronal adenosine A2A receptor in cognition (Kaster et al., 2015; Viana da Silva et al., 2016; Temido-Ferreira et al., 2018). The prominent cortical connectivity of the dorsal hippocampus and the projection to the anterior cingulated cortices are involved in memory processing (Jones and Wilson, 2005; Lavenex et al., 2006). The CA1 and subiculum of the ventral hippocampus share massive bidirectional connectivity with amygdala nuclei, which plays a key role in control (Pitkanen et al., 2000; Liu and Carter, 2018). The neuronal adenosine A2A receptors in those projections may underlie the distinct regulation of adenosine A2A receptors in the dHPC and vHPC. No method was designed in this research to confirm the mechanism, and further research is needed to clarify the mechanisms of neuronal A2AR-mediated regulation and modulation of caffeine.
Conclusion
In the present study, we blocked and reversed the effects of caffeine by specifically inactivating and activating dHPC/vHPC adenosine A2A receptors. For the first time, caffeine was demonstrated to affect memory by inhibiting dorsal hippocampal adenosine A2A receptors and affect anxiety by inhibiting ventral hippocampal adenosine A2A receptors, explaining how caffeine triggers anxiety while enhancing memory. Our results may help to understand the mechanisms of anxiety and memory and provide an experimental basis for making better use of caffeine while avoiding its side effects.
Data Availability Statement
The original contributions presented in the study are included in the article/Supplementary Material, further inquiries can be directed to the corresponding authors.
Ethics Statement
The animal study was reviewed and approved by The experimental procedures were provided by the Welfare Committee of the Army Medical University.
Author Contributions
YX performed experiments, and wrote the manuscript. PL and YZ designed the study and wrote the manuscript. YN, YZ, YP, and FL performed experiments. YX, YN, YZ, and PL analyzed data. PL and YZ reviewed the manuscript. PL and YZ designed the study and worked on the final approval of the manuscript and financial support.
Funding
This work was supported by a grant from the National Natural Science Foundation of China (81772455).
Conflict of Interest
The authors declare that the research was conducted in the absence of any commercial or financial relationships that could be construed as a potential conflict of interest.
Publisher’s Note
All claims expressed in this article are solely those of the authors and do not necessarily represent those of their affiliated organizations, or those of the publisher, the editors and the reviewers. Any product that may be evaluated in this article, or claim that may be made by its manufacturer, is not guaranteed or endorsed by the publisher.
Acknowledgments
We would like to thank AJE (www.aje.com) for English language editing.
Supplementary Material
The Supplementary Material for this article can be found online at: https://www.frontiersin.org/articles/10.3389/fphar.2022.807330/full#supplementary-material
References
Bastia, E., Xu, Y. H., Scibelli, A. C., Day, Y. J., Linden, J., Chen, J. F., et al. (2005). A Crucial Role for Forebrain Adenosine A(2A) Receptors in Amphetamine Sensitization. Neuropsychopharmacology 30 (5), 891–900. doi:10.1038/sj.npp.1300630
Batista, A. F. R., Martínez, J. C., and Hengst, U. (2017). Intra-axonal Synthesis of SNAP25 Is Required for the Formation of Presynaptic Terminals. Cell Rep 20 (13), 3085–3098. doi:10.1016/j.celrep.2017.08.097
Benito, E., and Barco, A. (2010). CREB's Control of Intrinsic and Synaptic Plasticity: Implications for CREB-dependent Memory Models. Trends Neurosci. 33 (5), 230–240. doi:10.1016/j.tins.2010.02.001
Borota, D., Murray, E., Keceli, G., Chang, A., Watabe, J. M., Ly, M., et al. (2014). Post-study Caffeine Administration Enhances Memory Consolidation in Humans. Nat. Neurosci. 17 (2), 201–203. doi:10.1038/nn.3623
Carvalho, K., Faivre, E., Pietrowski, M. J., Marques, X., Gomez-Murcia, V., Deleau, A., et al. (2019). Exacerbation of C1q Dysregulation, Synaptic Loss and Memory Deficits in Tau Pathology Linked to Neuronal Adenosine A2A Receptor. Brain 142 (11), 3636–3654. doi:10.1093/brain/awz288
Chen, J. F., Eltzschig, H. K., and Fredholm, B. B. (2013). Adenosine Receptors as Drug Targets-Wwhat Are the Challenges? Nat. Rev. Drug Discov. 12 (4), 265–286. doi:10.1038/nrd3955
Colic, L., Li, M., Demenescu, L. R., Li, S., Müller, I., Richter, A., et al. (2018). GAD65 Promoter Polymorphism Rs2236418 Modulates Harm Avoidance in Women via Inhibition/Excitation Balance in the Rostral ACC. J. Neurosci. 38 (22), 5067–5077. doi:10.1523/JNEUROSCI.1985-17.2018
Cunha, R. A., and Agostinho, P. M. (2010). Chronic Caffeine Consumption Prevents Memory Disturbance in Different Animal Models of Memory Decline. J. Alzheimers Dis. 20 (Suppl. 1), S95–S116. doi:10.3233/JAD-2010-1408
Cunha, R. A. (2016). How Does Adenosine Control Neuronal Dysfunction and Neurodegeneration? J. Neurochem. 139 (6), 1019–1055. doi:10.1111/jnc.13724
El Yacoubi, M., Ledent, C., Parmentier, M., Costentin, J., and Vaugeois, J. M. (2000). The Anxiogenic-like Effect of Caffeine in Two Experimental Procedures Measuring Anxiety in the Mouse Is Not Shared by Selective A(2A) Adenosine Receptor Antagonists. Psychopharmacology (Berl) 148 (2), 153–163. doi:10.1007/s002130050037
Fanselow, M. S., and Dong, H. W. (2010). Are the Dorsal and Ventral hippocampus Functionally Distinct Structures? Neuron 65 (1), 7–19. doi:10.1016/j.neuron.2009.11.031
Fredholm, B. B., Chen, J. F., Cunha, R. A., Svenningsson, P., and Vaugeois, J. M. (2005). Adenosine and Brain Function. Int. Rev. Neurobiol. 63, 191–270. doi:10.1016/S0074-7742(05)63007-3
Garcia, A. N., and Salloum, I. M. (2015). Polysomnographic Sleep Disturbances in Nicotine, Caffeine, Alcohol, Cocaine, Opioid, and Cannabis Use: A Focused Review. Am. J. Addict. 24 (7), 590–598. doi:10.1111/ajad.12291
Ikram, M., Park, T. J., Ali, T., and Kim, M. O. (2020). Antioxidant and Neuroprotective Effects of Caffeine against Alzheimer's and Parkinson's Disease: Insight into the Role of Nrf-2 and A2AR Signaling. Antioxidants (Basel) 9 (9), 902. doi:10.3390/antiox9090902
Irwin, C., Khalesi, S., Desbrow, B., and McCartney, D. (2020). Effects of Acute Caffeine Consumption Following Sleep Loss on Cognitive, Physical, Occupational and Driving Performance: A Systematic Review and Meta-Analysis. Neurosci. Biobehav Rev. 108, 877–888. doi:10.1016/j.neubiorev.2019.12.008
Jacobson, K. A., Gao, Z. G., Matricon, P., Eddy, M. T., and Carlsson, J. (2020). Adenosine A 2A Receptor Antagonists: from Caffeine to Selective Non‐xanthines. Br. J. Pharmacol. [online ahead of print] doi:10.1111/bph.15103
Jones, M. W., and Wilson, M. A. (2005). Theta Rhythms Coordinate Hippocampal-Prefrontal Interactions in a Spatial Memory Task. Plos Biol. 3 (12), e402. doi:10.1371/journal.pbio.0030402
Kaster, M. P., Machado, N. J., Silva, H. B., Nunes, A., Ardais, A. P., Santana, M., et al. (2015). Caffeine Acts through Neuronal Adenosine A2A Receptors to Prevent Mood and Memory Dysfunction Triggered by Chronic Stress. Proc. Natl. Acad. Sci. U S A. 112 (25), 7833–7838. doi:10.1073/pnas.1423088112
Khurana, K., and Bansal, N. (2019). Lacidipine Attenuates Caffeine-Induced Anxiety-like Symptoms in Mice: Role of Calcium-Induced Oxido-Nitrosative Stress. Pharmacol. Rep. 71 (6), 1264–1272. doi:10.1016/j.pharep.2019.07.008
Latina, V., Caioli, S., Zona, C., Ciotti, M. T., Amadoro, G., and Calissano, P. (2017). Impaired NGF/TrkA Signaling Causes Early AD-Linked Presynaptic Dysfunction in Cholinergic Primary Neurons. Front Cell Neurosci 11, 68. doi:10.3389/fncel.2017.00068
Laurent, C., Burnouf, S., Ferry, B., Batalha, V. L., Coelho, J. E., Baqi, Y., et al. (2016). A2A Adenosine Receptor Deletion Is Protective in a Mouse Model of Tauopathy. Mol. Psychiatry 21 (1), 149. doi:10.1038/mp.2015.115
Lavenex, P. B., Amaral, D. G., and Lavenex, P. (2006). Hippocampal Lesion Prevents Spatial Relational Learning in Adult Macaque Monkeys. J. Neurosci. 26 (17), 4546–4558. doi:10.1523/JNEUROSCI.5412-05.2006
Li, P., Rial, D., Canas, P. M., Yoo, J. H., Li, W., Zhou, X., et al. (2015). Optogenetic Activation of Intracellular Adenosine A2A Receptor Signaling in the hippocampus Is Sufficient to Trigger CREB Phosphorylation and Impair Memory. Mol. Psychiatry 20 (11), 1481. doi:10.1038/mp.2015.43
Liu, X., and Carter, A. G. (2018). Ventral Hippocampal Inputs Preferentially Drive Corticocortical Neurons in the Infralimbic Prefrontal Cortex. J. Neurosci. 38 (33), 7351–7363. doi:10.1523/JNEUROSCI.0378-18.2018
Londzin, P., Zamora, M., Kąkol, B., Taborek, A., and Folwarczna, J. (2021). Potential of Caffeine in Alzheimer's Disease-A Review of Experimental Studies. Nutrients 13 (2), 537. doi:10.3390/nu13020537
Machado, N. J., Simões, A. P., Silva, H. B., Ardais, A. P., Kaster, M. P., Garção, P., et al. (2017). Caffeine Reverts Memory but Not Mood Impairment in a Depression-Prone Mouse Strain with Up-Regulated Adenosine A2A Receptor in Hippocampal Glutamate Synapses. Mol. Neurobiol. 54 (2), 1552–1563. doi:10.1007/s12035-016-9774-9
Martineau, M., Guzman, R. E., Fahlke, C., and Klingauf, J. (2017). VGLUT1 Functions as a Glutamate/proton Exchanger with Chloride Channel Activity in Hippocampal Glutamatergic Synapses. Nat. Commun. 8 (1), 2279. doi:10.1038/s41467-017-02367-610.1038/s41467-017-02367-6
Martins, D. F., Brito, R. N., Stramosk, J., Batisti, A. P., Madeira, F., Turnes, B. L., et al. (2015). Peripheral Neurobiologic Mechanisms of Antiallodynic Effect of Warm Water Immersion Therapy on Persistent Inflammatory Pain. J. Neurosci. Res. 93 (1), 157–166. doi:10.1002/jnr.23461
Matos, M., Augusto, E., Santos-Rodrigues, A. D., Schwarzschild, M. A., Chen, J. F., Cunha, R. A., et al. (2012). Adenosine A2A Receptors Modulate Glutamate Uptake in Cultured Astrocytes and Gliosomes. Glia 60 (5), 702–716. doi:10.1002/glia.22290
McHugh, S. B., Fillenz, M., Lowry, J. P., Rawlins, J. N., and Bannerman, D. M. (2011). Brain Tissue Oxygen Amperometry in Behaving Rats Demonstrates Functional Dissociation of Dorsal and Ventral hippocampus during Spatial Processing and Anxiety. Eur. J. Neurosci. 33 (2), 322–337. doi:10.1111/j.1460-9568.2010.07497.x
Mikkelsen, S. H., Obel, C., Olsen, J., Niclasen, J., and Bech, B. H. (2017). Maternal Caffeine Consumption during Pregnancy and Behavioral Disorders in 11-Year-Old Offspring: A Danish National Birth Cohort Study. J. Pediatr. 189, 120–e1. doi:10.1016/j.jpeds.2017.06.051
Pitkänen, A., Pikkarainen, M., Nurminen, N., and Ylinen, A. (2000). Reciprocal Connections between the Amygdala and the Hippocampal Formation, Perirhinal Cortex, and Postrhinal Cortex in Rat. A Review. Ann. N. Y Acad. Sci. 911, 369–391. doi:10.1111/j.1749-6632.2000.tb06738.x
Prediger, R. D., Batista, L. C., and Takahashi, R. N. (2005). Caffeine Reverses Age-Related Deficits in Olfactory Discrimination and Social Recognition Memory in Rats. Involvement of Adenosine A1 and A2A Receptors. Neurobiol. Aging 26 (6), 957–964. doi:10.1016/j.neurobiolaging.2004.08.012
Richards, G., and Smith, A. (2015). Caffeine Consumption and Self-Assessed Stress, Anxiety, and Depression in Secondary School Children. J. Psychopharmacol. 29 (12), 1236–1247. doi:10.1177/0269881115612404
Schumacher, A., Villaruel, F. R., Ussling, A., Riaz, S., Lee, A. C. H., and Ito, R. (2018). Ventral Hippocampal CA1 and CA3 Differentially Mediate Learned Approach-Avoidance Conflict Processing. Curr. Biol. 28 (8), 1318–e4. doi:10.1016/j.cub.2018.03.012
Solfrizzi, V., Panza, F., Imbimbo, B. P., D'Introno, A., Galluzzo, L., Gandin, C., et al. (2015). Coffee Consumption Habits and the Risk of Mild Cognitive Impairment: The Italian Longitudinal Study on Aging. J. Alzheimers Dis. 47 (4), 889–899. doi:10.3233/JAD-150333
Swanson, L. W., and Cowan, W. M. (1977). An Autoradiographic Study of the Organization of the Efferent Connections of the Hippocampal Formation in the Rat. J. Comp. Neurol. 172 (1), 49–84. doi:10.1002/cne.901720104
Temido-Ferreira, M., Ferreira, D. G., Batalha, V. L., Marques-Morgado, I., Coelho, J. E., Pereira, P., et al. (2018). Age-related Shift in LTD Is Dependent on Neuronal Adenosine A2A Receptors Interplay with mGluR5 and NMDA Receptors. Mol. Psychiatry 25, 1876–1900. doi:10.1038/s41380-018-0110-9
Trompoukis, G., and Papatheodoropoulos, C. (2020). Dorsal-Ventral Differences in Modulation of Synaptic Transmission in the Hippocampus. Front. Synaptic Neurosci. 12, 24. doi:10.3389/fnsyn.2020.00024
Viana da Silva, S., Haberl, M. G., Zhang, P., Bethge, P., Lemos, C., Gonçalves, N., et al. (2016). Early Synaptic Deficits in the APP/PS1 Mouse Model of Alzheimer's Disease Involve Neuronal Adenosine A2A Receptors. Nat. Commun. 7, 11915. doi:10.1038/ncomms11915
Wei, C. J., Augusto, E., Gomes, C. A., Singer, P., Wang, Y., Boison, D., et al. (2014). Regulation of Fear Responses by Striatal and Extrastriatal Adenosine A2A Receptors in Forebrain. Biol. Psychiatry 75 (11), 855–863. doi:10.1016/j.biopsych.2013.05.003
Wei, C. J., Singer, P., Coelho, J., Boison, D., Feldon, J., Yee, B. K., et al. (2011). Selective Inactivation of Adenosine A(2A) Receptors in Striatal Neurons Enhances Working Memory and Reversal Learning. Learn. Mem. 18 (7), 459–474. doi:10.1101/lm.2136011
Xu, T. J., and Reichelt, A. C. (2018). Sucrose or Sucrose and Caffeine Differentially Impact Memory and Anxiety-like Behaviours, and Alter Hippocampal Parvalbumin and Doublecortin. Neuropharmacology 137, 24–32. doi:10.1016/j.neuropharm.2018.04.012
Yu, C., Gupta, J., Chen, J. F., and Yin, H. H. (2009). Genetic Deletion of A2A Adenosine Receptors in the Striatum Selectively Impairs Habit Formation. J. Neurosci. 29 (48), 15100–15103. doi:10.1523/JNEUROSCI.4215-09.2009
Zeng, X. J., Li, P., Ning, Y. L., Zhao, Y., Peng, Y., Yang, N., et al. (2020). A2A R Inhibition in Alleviating Spatial Recognition Memory Impairment after TBI Is Associated with Improvement in Autophagic Flux in RSC. J. Cell Mol Med 24 (12), 7000–7014. doi:10.1111/jcmm.15361
Zheng, H., Stornetta, R. L., Agassandian, K., and Rinaman, L. (2015). Glutamatergic Phenotype of Glucagon-like Peptide 1 Neurons in the Caudal Nucleus of the Solitary Tract in Rats. Brain Struct. Funct. 220 (5), 3011–3022. doi:10.1007/s00429-014-0841-6
Keywords: caffeine, dorsal hippocampus, ventral hippocampus, adenosine A2A receptor, memory, anxiety
Citation: Xu Y, Ning Y, Zhao Y, Peng Y, Luo F, Zhou Y and Li P (2022) Caffeine Functions by Inhibiting Dorsal and Ventral Hippocampal Adenosine 2A Receptors to Modulate Memory and Anxiety, Respectively. Front. Pharmacol. 13:807330. doi: 10.3389/fphar.2022.807330
Received: 02 November 2021; Accepted: 11 January 2022;
Published: 02 February 2022.
Edited by:
Senthil S. Gounder, Johns Hopkins University, United StatesReviewed by:
Ana Elisa Speck, Federal University of Santa Catarina, BrazilYu Ohmura, Hokkaido University, Japan
Copyright © 2022 Xu, Ning, Zhao, Peng, Luo, Zhou and Li. This is an open-access article distributed under the terms of the Creative Commons Attribution License (CC BY). The use, distribution or reproduction in other forums is permitted, provided the original author(s) and the copyright owner(s) are credited and that the original publication in this journal is cited, in accordance with accepted academic practice. No use, distribution or reproduction is permitted which does not comply with these terms.
*Correspondence: Yuanguo Zhou, ygzhou@tmmu.edu.cn; Ping Li, ping__ping0074@sina.com