- 1Department of Pharmaceutical Sciences, University of Milan, Milan, Italy
- 2Centro Cardiologico Monzino IRCCS, Milan, Italy
Leukotrienes are important pro-inflammatory lipid mediators derived from the arachidonic acid metabolism. In particular, cysteinyl leukotrienes, namely LTC4, LTD4, and LTE4 are involved in many of the principal features of asthma, while more recently they have also been implicated in cardiovascular diseases. COVID-19 is characterized by an overwhelming state of inflammation, sometimes resulting in an acute respiratory distress syndrome. Furthermore, severe COVID-19 patients present an endothelial cell damage characterized by a hyperinflammatory/procoagulant state and a widespread thrombotic disease. Leukotriene receptor antagonists, such as montelukast, have long been proven to have an efficacy in asthma, while more recently they have been suggested to have a protective role also in cardiovascular diseases. As elevated levels of LTE4 have been detected in bronchoalveolar lavage of COVID-19 patients, and montelukast, in addition to its anti-inflammatory properties, has been suggested to have a protective role in cardiovascular diseases, we decided to investigate whether this drug could also affect the platelet activation characteristic of COVID-19 syndrome. In this contribution, we demonstrate that montelukast inhibits platelet activation induced by plasma from COVID-19 patients by preventing the surface expression of tissue factor (TF) and P-selectin, reducing the formation of circulating monocyte– and granulocyte–platelet aggregates, and, finally, in completely inhibiting the release of TFpos-circulating microvesicles. These data suggest the repurposing of montelukast as a possible auxiliary treatment for COVID-19 syndrome.
Introduction
Leukotrienes (LTs) are important pro-inflammatory lipid mediators derived from the metabolism of the arachidonic acid. In particular, cysteinyl leukotrienes (cysteinyl-LTs), namely LTC4, LTD4, and LTE4 are synthesized in vivo by immunocompetent cells such as mast cells, eosinophils, basophils, and monocytes/macrophages (Haeggstrom and Funk, 2011). They are involved in many principal features of asthma, such as bronchoconstriction, hyperresponsiveness, and inflammatory cell recruitment (Sokolowska et al., 2021); more recently, they have also been implicated in other inflammatory conditions, including immune and neurodegenerative disorders, cancer, and particularly in cardiovascular diseases (Capra et al., 2013; Hoxha et al., 2017; Bäck et al., 2019). The biological actions of cysteinyl-LTs are mediated by two officially recognized G-protein-coupled receptors: CysLT1 and CysLT2. They differ in localization and binding affinities for the different cysteinyl-LTs as well as in their biological activities (Back et al., 2014).
Leukotriene receptor antagonists (LTRAs), such as pranlukast (Onon™), zafirlukast (Accolate™), and montelukast (Singulair™), have long been proven to have an efficacy in asthma therapy. LTRAs have been in use for the last 20 years to treat the airway inflammatory symptoms of mild-to-moderate asthma and allergic rhinitis (Capra et al., 2006; Trinh et al., 2019). In particular, montelukast, the most prescribed drugs among LTRAs in asthma, has been shown to have an excellent safety profile both in adults and children (Virchow and Bachert, 2006).
The SARS-CoV-2 (severe acute respiratory syndrome coronavirus 2) is the cause of the coronavirus disease 2019 (COVID-19) and, while the vast majority of the infected patients range from asymptomatic to mild symptoms, a minority of the patients will eventually develop severe symptoms leading rapidly to hypoxia and acute respiratory distress syndrome (ARDS) requiring hospitalization and oxygen supplementation (Wu and McGoogan, 2020). This severe form is prevalent in the elderly with underlying comorbidities such as hypertension, diabetes, or cardiovascular diseases (Nishiga et al., 2020). COVID-19 is characterized by an overwhelming state of inflammation with an elevated level of circulating chemokines (e.g., MCP-1 and RANTES) and cytokines, such as IL-6, IL-8, and TNF-α eventually leading to a multi-organ dysfunction that has been called COVID-19 cytokine storm syndrome (CSS) (England et al., 2021). Furthermore, an increasing amount of clinical data has documented how SARS-CoV-2 may also predispose patients to endothelial cell damage particularly in the pulmonary vessels (Ackermann et al., 2020), leading to a unique vascular hyperinflammatory/procoagulant state and a widespread thrombotic disease, both in the venous and arterial vascular beds (Teuwen et al., 2020).
In addition to mitigate airway inflammatory symptoms, over the years, a number of in vitro and animal studies have suggested LTRAs to have a protective role in cardiovascular diseases (Funk, 2005; Hoxha et al., 2017). Furthermore, in two separate observational studies, a nationwide cohort study in the all Swedish population (Ingelsson et al., 2012) and a retrospective study in 800 asthmatic adults in Albania (Hoxha et al., 2021), exposure to montelukast seems to protect patients from major cardiovascular events. This leads us to speculate on a potential effect of LTRAs on platelet activation, a well-established player in ischemic events. Of note, platelets from COVID-19 patients are characterized by a sustained activation status (Canzano et al., 2021), with cytokines, chemokines, and growth factors released in significantly large amounts upon their stimulation (Taus et al., 2020). Furthermore, we have recently provided evidences that the addition of COVID-19 plasma to plasma-depleted blood from healthy subjects (HS) reproduced the platelet activation, especially in terms of the prothrombotic phenotype, observed in vivo in COVID-19 patients (Canzano et al., 2021).
Considering that the elevated levels of LTs have been previously detected in aspirates of patients with ARDS (Sala et al., 1991), while high levels of LTE4, a biomarker of total body cysteinyl-LT production, have been detected in bronchoalveolar lavage (BAL) of hospitalized patients with severe COVID-19 syndrome (Archambault et al., 2021), suggesting a role for eicosanoids in the pathological response to SARS-CoV-2 infection, we decided to investigate whether montelukast, in addition to its anti-inflammatory properties, could also affect the expression of the major markers of platelet activation such as tissue factor (TF) and P-selectin as well as the formation of platelet–leukocyte aggregates and microvesicle (MV) release observed in COVID-19 syndrome.
Methods
Antibodies and Reagents
Antibodies were obtained from the following sources: mouse anti-human P-selectin APC (CD62P), mouse anti-human CD14 PerCP, mouse anti-human CD41 PerCP Cy5.5, mouse IgG FITC, and mouse IgG APC were from Becton Dickinson; mouse anti-human TF BV421 (clone HTF-1) and mouse anti-human CD41 PE were from Beckman Coulter; and mouse anti-human tissue factor FITC (clone VIC-7) was from Biomedica. Calcein AM was from Invitrogen.
Patient Selection
The study took advantage from an existing biobank of plasma samples prepared from a cohort of 46 consecutive COVID-19 patients, whose characteristics are reported in Supplementary Table S1 (Canzano et al., 2021).
Patients with a positive SARS-CoV-2 polymerase chain reaction test and requiring oxygen supplementation were included. The criteria for hospital admission were defined as those requiring inpatient care as a result of the severity of illness based on laboratory and radiological parameters as well as clinical findings. Following admission, all patients received supportive care in line with best international practice. Biochemical variables, including inflammatory and thrombotic parameters (IL-6, C-reactive protein (CRP), lactate dehydrogenase (LDH), fibrinogen, D-dimer, and procalcitonin) and arterial blood gas analysis (pO2/FiO2 ratio and oxygen saturation) were recorded at hospital admission and immediately before or soon after oxygen supplementation, concomitantly with the blood sampling.
Blood Collection and Plasma Preparation
Whole blood (WB), sampled at initiation of mechanical ventilation or low-flow oxygen therapy, was drawn using a 19-gauge needle without venous stasis into citrate (1/10 volume of 0.129 M sodium citrate)- and K2-EDTA-containing tubes (Vacutainer, Becton Dickinson) and processed within 15 min. For citrate plasma preparation, WB was centrifuged at 1,700 g for 10 min at 4°C. Absence of blood cells in plasma samples was evaluated using a cell counter (Sysmex XS-1000i).
In Vitro Studies
To test the effect of COVID-19 patient plasma on platelet activation, three pools made with plasma from 12 patients were prepared (4 patients/pool). The characteristics of the 12 patients are reported in Supplementary Table S1. Blood from healthy subjects (HS, n = 4–6, comparable for blood cell counts) was plasma-depleted by centrifuging WB at 1000×g for 10 min, at room temperature (RT). The plasma-free sample obtained after centrifugation was analyzed using a cell counter (Sysmex XS-1000i) to verify that all cellular components were left in the pelleted fraction. Healthy plasma was then replaced with the COVID-19 plasma pools or with autologous plasma as control. To assess the effect of montelukast on cell activation, blood from healthy subjects was preincubated with the drug (at the indicated concentrations) for 30 min, at room temperature. Blood was then centrifuged at 1000×g for 10 min, at room temperature, and plasma was replaced with the COVID-19 plasma pool or with autologous plasma. The effect of LTE4 (0.1–30 nM, 15 min, RT) on platelet-associated TF- and P-selectin-expression was also investigated.
Platelet Activation and Microvesicle Characterization
Circulating cell-associated TF expression, platelet activation markers, and MV release were analyzed by flow cytometry as previously described (Canzano et al., 2021). In brief, WB (5 µL for platelet and 100 µL for leukocyte analysis) was labeled for 15 min at room temperature in the dark with saturating concentration of αTF and αP-selectin MoAbs together with αCD41 and αCD14 or αCD66 to identify platelets, monocytes, and granulocytes, respectively. Leukocyte–platelet aggregates were identified as double positive events for platelet and leukocyte population markers (CD14pos/CD41pos or CD66pos/CD41pos for monocyte–platelet or granulocyte–platelet aggregates, respectively). The gating strategies are reported in the Supplementary Figure S1. A total of 10,000 CD41 pos events and 3,000 CD14pos events per sample were acquired on a Gallios flow cytometer (Beckman Coulter). Fluorochrome-conjugated isotype controls were used in order to quantify the background labeling and to set the dot plot quadrant marker for data analysis (Kaluza Analysis software v1.5; Beckman Coulter). The results are reported as percentage ± SD of positive cells.
For MV characterization, 50 μL of WB was diluted in 150 μL of 0.22 µm-filtered buffer [Hepes (10 mM), NaCl (140 mM), and CaCl2 (2.5 mM); pH 7.4] containing phe-pro-arg chloromethyl ketone (PPACK, 15 µM)) to prevent clot formation. To identify intact MVs, excluding cell debris, samples were incubated with calcein AM (10 µM) at 37°C in the dark for 25 min followed by the addition of saturating concentrations of αTF and αCD41 MoAbs. Fluorescence minus one (FMO) control was used to correct gating. The samples were immediately analyzed on a Gallios flow cytometer equipped with four solid-state lasers and enhanced wide forward angle light scatter optimized for MV detection. Flow-check Pro Fluorospheres were daily used to monitor cytometer performance. Megamix-FSC Plus beads (0.5, 0.9, and 3 µm) were used to define the analysis gate and BD Trucount tubesTM to have the absolute count of MVs.
Statistics
The results are expressed as mean ± standard deviation (SD). Continuous variables among groups were compared by repeated measure one-way analysis of variance (ANOVA) followed by Tukey’s multiple comparisons test. A p-value of 0.05 was considered statistically significant. Analyses were performed using SPSS statistical package (v9.4).
Results
In order to reproduce the platelet activation observed during COVID-19, blood from HS was plasma-depleted and reconstituted with plasma pools from COVID-19 patients or from the same HS blood donors. As expected, plasma from COVID-19 patients significantly increased the number of TFpos- and P-selectinpos-platelets (Figure 1) and the formation of total and TFpos platelet–monocyte and platelet–granulocyte aggregates (Figure 2) as well as the release of total and platelet-derived TFpos circulating MVs (Figure 3), highlighting the marked prothrombotic phenotype associated with both TF-bearing cells and MVs.
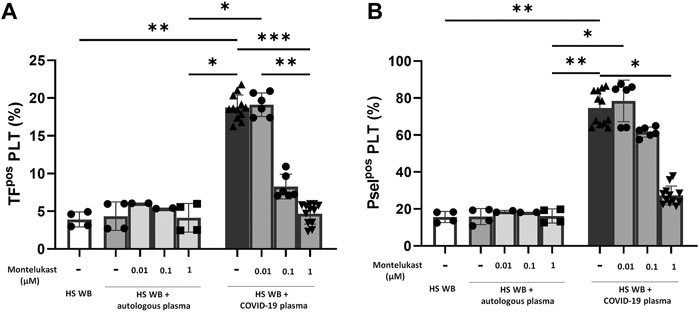
FIGURE 1. In vitro effect of montelukast on platelet-associated tissue factor (A) and P-selectin (B) expression induced by COVID-19 plasma. Whole blood (WB) from healthy subjects (HS; n = 4), pre-incubated with montelukast (0.01–1 µM), was plasma-depleted and reconstituted with COVID-19 plasma pools (n = 3 for each HS, dark gray bars) or autologous plasma (light gray bars). The percentage of TFpos- and P-selectinpos-platelets was assessed by flow cytometry. Data are reported as mean ± SD. *p < 0.05; **p < 0.01; ***p < 0.001 (TF, tissue factor; Psel, P-selectin; PLT, platelets).
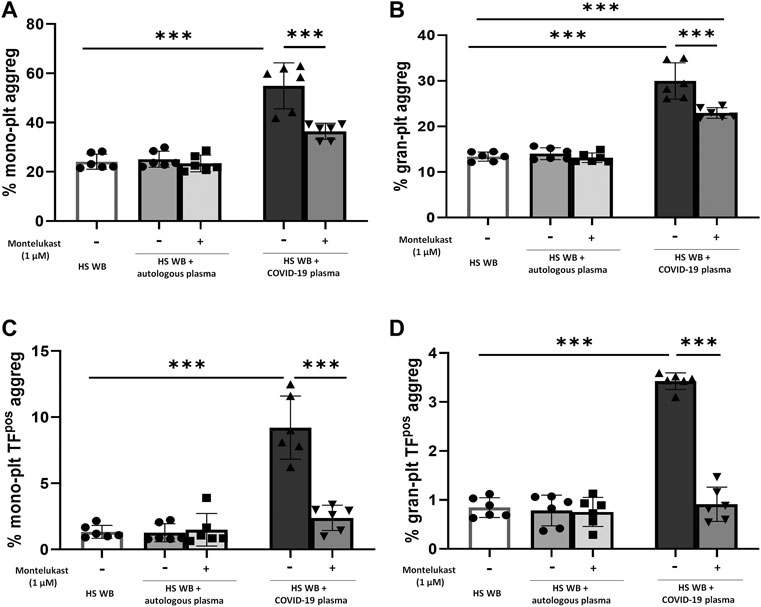
FIGURE 2. In vitro effect of montelukast on platelet–leukocyte aggregate formation induced by COVID-19 plasma. Whole blood (WB) from healthy subjects (HS; n = 6), pre-incubated with montelukast (1 µM) as indicated, was plasma-depleted and reconstituted with COVID-19 plasma pools (dark gray bars) or autologous plasma (light gray bars). Total (A,B) and TFpos(C,D)-platelet–leukocyte aggregates was measured by flow cytometry. Data are reported as mean ± SD. ***p < 0.001 (TF, tissue factor; plt, platelets; mono, monocytes; gran, granulocytes).
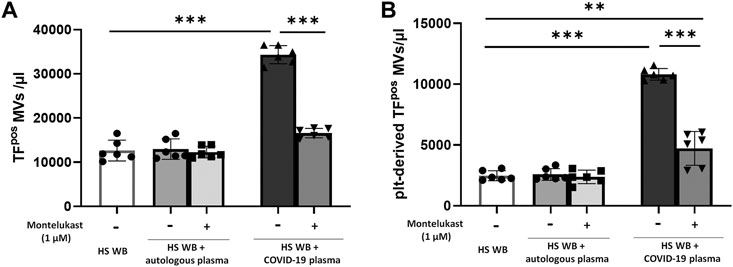
FIGURE 3. In vitro effect of montelukast on microvesicle (MV) release induced by COVID-19 plasma. Whole blood (WB) from healthy subjects (HS; n = 3), pre-incubated with montelukast (1 µM) as indicated, was plasma-depleted and reconstituted with the COVID-19 plasma pool (dark gray bars) or autologous plasma (light gray bars). The number of TFpos (A) and platelet-derived TFpos-MVs (B) was measured by flow cytometry. Data are reported as mean ± SD. **p < 0.01; ***p < 0.001 (TF, tissue factor; plt, platelets).
Interestingly, preincubation of HS platelets with montelukast concentration dependently prevented the induction of TFpos- and P-selectinpos-platelets by COVID-19 plasma, being both completely inhibited at 1 µM (Figure 1). It also significantly reduced the effect of COVID-19 plasma on the formation of circulating monocyte–platelet and granulocyte–platelet aggregates (Figure 2, panel A and B), decreasing the number of those TFpos by 4-times (Figure 2, panel C and D). Finally, montelukast was effective in completely preventing the release of TFpos-circulating MVs induced by COVID-19 plasma, reducing by more than 2-times those derived from platelets (Figure 3).
Overall, these data point to an involvement of LTs in the platelet activation induced by COVID-19 plasma. Indeed, in vitro stimulation of platelets from HS with LTE4, the most stable of cysteinyl-LTs (Sokolowska et al., 2021), concentration dependently induced TF and P-selectin expression (Figure 4).
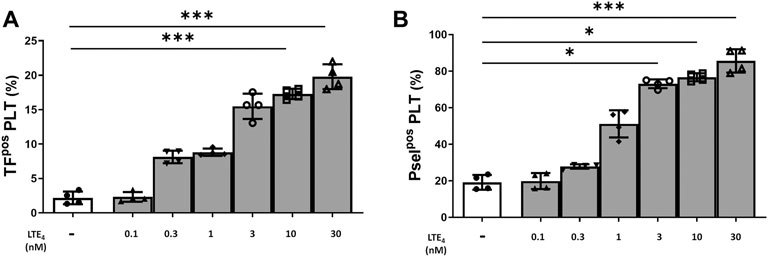
FIGURE 4. In vitro effect of LTE4 on platelet-associated tissue factor (A) and P-selectin (B) expression. Whole blood was stimulated with LTE4 (0.1–30 nM), for 15 min at room temperature. The percentage of TFpos- and P-selectinpos-platelets is shown. Data are reported as mean ± SD. *p < 0.05; ***p < 0.001 (TF, tissue factor; Psel, P-selectin; PLT, platelets).
Discussion
The present study reports, for the first time, that montelukast significantly inhibits platelet activation induced by plasma from COVID-19 patients, suggesting its use as a possible auxiliary treatment in this syndrome.
There is now a wide consensus in the literature that COVID-19 is associated not only with a CSS characterized by an hyperimmune or hyperinflammatory response (England et al., 2021) but also with endothelial cell damage ultimately leading to micro- and macro-thrombosis in the pulmonary vessels as well as in other organs (Ackermann et al., 2020; Teuwen et al., 2020). Despite several different drugs/strategies are available to treat COVID-19 patients today, from antiviral drugs to SARS-CoV-2-neutralizing antibodies, from IL-6 receptor monoclonal antibodies to corticosteroids or aspirin, and while several new approaches are tested in ongoing clinical trials, an unanimous consensus to treat this disease has not yet been achieved (COVID-19 Treatment Guidelines Panel, NIH. Available at https://www.covid19treatmentguidelines.nih.gov/).
Cysteinyl-LTs, in addition to being the most potent broncho-constrictors known in man, have a well-recognized role in immune cell and macrophages activation as well as cytokine (IL-6, IL-8, TNF-α, and MIP-1β) or chemokine (MCP-1 and RANTES) release (Back et al., 2014). Accordingly, LTRAs significantly inhibited pro-inflammatory cytokine production mostly through inhibition of NF-kB (Maeba et al., 2005; Tahan et al., 2008), a transcription factor that is known to control several genes involved in inflammation including IL-6, IL-8, and TNF-α, all of which would enhance the hyperimmune/inflammatory response. Of note, the severity of pulmonary complications in COVID-19 seems to be closely related to IL-6 and TNF-α peak levels (Chen et al., 2020). For these reasons, largely speculative until now, eicosanoids have been hypothesized to be involved in various aspects of COVID-19 pathology (Arnardottir et al., 2020; Hammock et al., 2020). In particular, the use of montelukast has been proposed as a possible therapy, particularly due to its anti-inflammatory activities (Aigner et al., 2020; Barré et al., 2020; Funk and Ardakani, 2020; Sanghai and Tranmer, 2020). Accordingly, the first phase III clinical trial testing the cysteinyl-LT receptor antagonist montelukast in COVID-19 patients has already been planned (https://clinicaltrials.gov/ct2/show/NCT04389411).
Despite LT levels cannot be reliably measured in plasma (Heavey et al., 1987; Sokolowska et al., 2021), as mentioned before, the elevated levels of LTE4, the only sufficiently stable LT to be prominent in biologic fluids (Sokolowska et al., 2021), have been detected in BAL of patients with severe COVID-19 (Archambault et al., 2021), while elevated levels of LTs have been previously detected in aspirates of patients with ARDS (Sala et al., 1991). Here we demonstrate that, indeed, exogenous LTE4 is able to induce expression of known markers of platelet activation at concentrations comparable to those obtained in BAL of COVID-19 patients (Archambault et al., 2021). Thus, the data presented here suggest that montelukast, if administered in the early phase of the disease, not only has the potential to limit the acute and chronic lung tissue damage in COVID-19 patients by treating the hyperimmune/hyperinflammatory response and taming the CSS, but it may also limit the massive platelet activation and prothrombotic phenotype, thus serving as a potential “single” approach for the two most prominent aspects of COVID-19 syndrome. To this point, two very recent retrospective analyses demonstrated that either asthmatic patients receiving montelukast had fewer episodes of confirmed COVID-19 or experienced significantly fewer events of clinical deterioration (Bozek and Winterstein, 2020; Khan et al., 2021).
Another interesting aspect may involve the thrombotic complications of SARS-CoV2 vaccination. In fact, while the exact mechanism by which adenovirus-vectored COVID-19 vaccines trigger the vaccine-induced immune thrombotic thrombocytopenia (VITT) is still unclear; this syndrome is thought to involve a FcγRIIA receptors-dependent platelet activation causing platelet P-selectin’s expression, secretion of alpha granules, and release of procoagulant MVs, leading to TF accumulation into developing thrombi (Marchandot et al., 2021). Therefore, montelukast treatment might also help to prevent or mitigate this rare, but serious adverse event upon vaccination.
At this stage, we can only speculate on the mechanism of antiplatelet activity of montelukast. While it is known that human platelets express both CysLT1 and CysLT2 receptors, and that pranlukast inhibits cysteinyl-LT-induced RANTES release (Hasegawa et al., 2010), contrasting data are present in the literature on platelet activation by LTs (Austen et al., 2009; Foster et al., 2013). While the results of our in vitro experiments showing that LTE4 concentration dependently induced TF and P-selectin expression suggest that LTE4 itself plays a central role in the described platelet activation, we cannot rule out the involvement of other players. Of note, in our model, IL-6, the expression of which can be modulated by montelukast, at concentrations comparable to those found in COVID-19 patients, significantly potentiated the effect of low-concentration adenosine diphosphate (ADP) or thromboxane A2, and accordingly, aspirin and the P2Y12 inhibitor AR-C69931MX prevented platelet activation induced by COVID-19 plasma (Canzano et al., 2021). Therefore, considering that montelukast has been shown to have some off-target effects, namely inhibition of some P2Y receptors (Mamedova et al., 2005; Woszczek et al., 2010), the data presented here might be either due to inhibition of its primary target or to a secondary target directly involved in platelet activation.
In conclusion, we believe that our data, highlighting the possible contribution of montelukast not only to mitigate the CSS but also in alleviating the peculiar platelet prothrombotic phenotype of COVID-19, should foster the scientific community to further consider the repurposing of montelukast, or other approved LTRAs, as an innovative strategy for COVID-19 syndrome.
Data Availability Statement
The raw data supporting the conclusion of this article will be made available by the authors, without undue reservation.
Ethics Statement
The studies involving human participants were reviewed and approved by the Ethical Committee of the institution (number 2020_06_16_18). The patients/participants provided their written informed consent to participate in this study.
Author Contributions
MC: helped in designing the study and was a major contributor in the discussion of results and the writing of the manuscript. PC and MB: performed the experiments and participated in writing the manuscript. GR: conceived the study and was a major contributor in the discussion of the data and in the writing of the manuscript.
Conflict of Interest
The authors declare that the research was conducted in the absence of any commercial or financial relationships that could be construed as a potential conflict of interest.
Publisher’s Note
All claims expressed in this article are solely those of the authors and do not necessarily represent those of their affiliated organizations, or those of the publisher, the editors, and the reviewers. Any product that may be evaluated in this article, or claim that may be made by its manufacturer, is not guaranteed or endorsed by the publisher.
Supplementary Material
The Supplementary Material for this article can be found online at: https://www.frontiersin.org/articles/10.3389/fphar.2022.784214/full#supplementary-material
Abbreviations
LTs, leukotrienes; cysteinyl-LTs, cysteinyl leukotrienes; LTRAs, leukotriene receptor antagonists; SARS-CoV-2, severe acute respiratory syndrome coronavirus 2; COVID-19, coronavirus disease 2019; ARDS, acute respiratory distress syndrome; CSS, cytokine storm syndrome; BAL, bronchoalveolar lavage; TF, tissue factor; MV, microvesicle.
References
Ackermann, M., Verleden, S. E., Kuehnel, M., Haverich, A., Welte, T., Laenger, F., et al. (2020). Pulmonary Vascular Endothelialitis, Thrombosis, and Angiogenesis in Covid-19. N. Engl. J. Med. 383, 120–128. doi:10.1056/NEJMoa2015432
Aigner, L., Pietrantonio, F., Bessa de Sousa, D. M., Michael, J., Schuster, D., Reitsamer, H. A., et al. (2020). The Leukotriene Receptor Antagonist Montelukast as a Potential COVID-19 Therapeutic. Front. Mol. Biosci. 7, 610132. doi:10.3389/fmolb.2020.610132
Archambault, A. S., Zaid, Y., Rakotoarivelo, V., Turcotte, C., Doré, É., Dubuc, I., et al. (2021). High Levels of Eicosanoids and Docosanoids in the Lungs of Intubated COVID-19 Patients. FASEB J. 35, e21666. doi:10.1096/fj.202100540R
Arnardottir, H., Pawelzik, S. C., Öhlund Wistbacka, U., Artiach, G., Hofmann, R., Reinholdsson, I., et al. (2020). Stimulating the Resolution of Inflammation through Omega-3 Polyunsaturated Fatty Acids in COVID-19: Rationale for the COVID-Omega-F Trial. Front. Physiol. 11, 624657. doi:10.3389/fphys.2020.624657
Austen, K. F., Maekawa, A., Kanaoka, Y., and Boyce, J. A. (2009). The Leukotriene E(4) Puzzle: Finding the Missing Pieces and Revealing the Pathobiologic Implications. J. Allergy Clin. Immunol. 124 (3), 406–414. doi:10.1016/j.jaci.2009.05.046
Bäck, M., Powell, W. S., Dahlén, S. E., Drazen, J. M., Evans, J. F., Serhan, C. N., et al. (2014). Update on Leukotriene, Lipoxin and Oxoeicosanoid Receptors: IUPHAR Review 7. Br. J. Pharmacol. 171, 3551–3574. doi:10.1111/bph.12665
Bäck, M., Yurdagul, A., Tabas, I., Öörni, K., and Kovanen, P. T. (2019). Inflammation and its Resolution in Atherosclerosis: Mediators and Therapeutic Opportunities. Nat. Rev. Cardiol. 16, 389–406. doi:10.1038/s41569-019-0169-2
Barré, J., Sabatier, J.-M., and Annweiler, C. (2020). Montelukast Drug May Improve COVID-19 Prognosis: A Review of Evidence. Front. Pharmacol. 11, 1344. doi:10.3389/fphar.2020.01344
Bozek, A., and Winterstein, J. (2020). Montelukast's Ability to Fight COVID-19 Infection. J. Asthma 58 (10), 1348–1349. doi:10.1080/02770903.2020.1786112
Canzano, P., Brambilla, M., Porro, B., Cosentino, N., Tortorici, E., Vicini, S., et al. (2021). Platelet and Endothelial Activation as Potential Mechanisms behind the Thrombotic Complications of COVID-19 Patients. JACC: Basic Translational Sci. 6, 202–218. doi:10.1016/j.jacbts.2020.12.009
Capra, V., Ambrosio, M., Riccioni, G., and Rovati, G. E. (2006). Cysteinyl-leukotriene Receptor Antagonists: Present Situation and Future Opportunities. Curr. Med. Chem. 13, 3213–3226. doi:10.2174/092986706778742963
Capra, V., Bäck, M., Barbieri, S. S., Camera, M., Tremoli, E., and Rovati, G. E. (2013). Eicosanoids and Their Drugs in Cardiovascular Diseases: Focus on Atherosclerosis and Stroke. Med. Res. Rev. 33, 364–438. doi:10.1002/med.21251
Chen, L. Y. C., Hoiland, R. L., Stukas, S., Wellington, C. L., and Sekhon, M. S. (2020). Confronting the Controversy: Interleukin-6 and the COVID-19 Cytokine Storm Syndrome. Eur. Respir. J. 56. doi:10.1183/13993003.03006-2020
England, J. T., Abdulla, A., Biggs, C. M., Lee, A. Y. Y., Hay, K. A., Hoiland, R. L., et al. (2021). Weathering the COVID-19 Storm: Lessons from Hematologic Cytokine Syndromes. Blood Rev. 45, 100707. doi:10.1016/j.blre.2020.100707
Foster, H. R., Fuerst, E., Lee, T. H., Cousins, D. J., and Woszczek, G. (2013). Characterisation of P2Y(12) Receptor Responsiveness to Cysteinyl Leukotrienes. PLoS One 8, e58305. doi:10.1371/journal.pone.0058305PONE-D-12-35349
Funk, C. D., and Ardakani, A. (2020). A Novel Strategy to Mitigate the Hyperinflammatory Response to COVID-19 by Targeting Leukotrienes. Front. Pharmacol. 11, 1214. doi:10.3389/fphar.2020.01214
Funk, C. D. (2005). Leukotriene Modifiers as Potential Therapeutics for Cardiovascular Disease. Nat. Rev. Drug Discov. 4, 664–672. doi:10.1038/nrd1796
Haeggström, J. Z., and Funk, C. D. (2011). Lipoxygenase and Leukotriene Pathways: Biochemistry, Biology, and Roles in Disease. Chem. Rev. 111, 5866–5898. doi:10.1021/cr200246d
Hammock, B. D., Wang, W., Gilligan, M. M., and Panigrahy, D. (2020). Eicosanoids: The Overlooked Storm in Coronavirus Disease 2019 (COVID-19)? Am. J. Pathol. 190, 1782–1788. doi:10.1016/j.ajpath.2020.06.010
Hasegawa, S., Ichiyama, T., Hashimoto, K., Suzuki, Y., Hirano, R., Fukano, R., et al. (2010). Functional Expression of Cysteinyl Leukotriene Receptors on Human Platelets. Platelets 21, 253–259. doi:10.3109/09537101003615394
Heavey, D. J., Soberman, R. J., Lewis, R. A., Spur, B., and Austen, K. F. (1987). Critical Considerations in the Development of an Assay for Sulfidopeptide Leukotrienes in Plasma. Prostaglandins 33, 693–708. doi:10.1016/0090-6980(87)90035-9
Hoxha, M., Rovati, G. E., and Cavanillas, A. B. (2017). The Leukotriene Receptor Antagonist Montelukast and its Possible Role in the Cardiovascular Field. Eur. J. Clin. Pharmacol. 73, 799–809. doi:10.1007/s00228-017-2242-2
Hoxha, M., Tedesco, C. C., Quaglin, S., Malaj, V., Pustina, L., Capra, V., et al. (2021). Montelukast Use Decreases Cardiovascular Events in Asthmatics. Front. Pharmacol. 11. doi:10.3389/fphar.2020.611561
Ingelsson, E., Yin, L., and Bäck, M. (2012). Nationwide Cohort Study of the Leukotriene Receptor Antagonist Montelukast and Incident or Recurrent Cardiovascular Disease. J. Allergy Clin. Immunol. 129, 702–707.e2. doi:10.1016/j.jaci.2011.11.052
Khan, A. R., Misdary, C., Yegya-Raman, N., Kim, S., Narayanan, N., Siddiqui, S., et al. (2021). Montelukast in Hospitalized Patients Diagnosed with COVID-19. J. Asthma, 1–7. [Online ahead of print] doi:10.1080/02770903.2021.1881967
Maeba, S., Ichiyama, T., Ueno, Y., Makata, H., Matsubara, T., and Furukawa, S. (2005). Effect of Montelukast on Nuclear Factor kappaB Activation and Proinflammatory Molecules. Ann. Allergy Asthma Immunol. 94, 670–674. doi:10.1016/S1081-1206(10)61326-9
Mamedova, L., Capra, V., Accomazzo, M. R., Gao, Z. G., Ferrario, S., Fumagalli, M., et al. (2005). CysLT1 Leukotriene Receptor Antagonists Inhibit the Effects of Nucleotides Acting at P2Y Receptors. Biochem. Pharmacol. 71, 115–125. doi:10.1016/j.bcp.2005.10.003
Marchandot, B., Curtiaud, A., Trimaille, A., Sattler, L., Grunebaum, L., and Morel, O. (2021). Vaccine-induced Immune Thrombotic Thrombocytopenia: Current Evidence, Potential Mechanisms, Clinical Implications, and Future Directions. Eur. Hear. J. Open 1. doi:10.1093/ehjopen/oeab014
Nishiga, M., Wang, D. W., Han, Y., Lewis, D. B., and Wu, J. C. (2020). COVID-19 and Cardiovascular Disease: from Basic Mechanisms to Clinical Perspectives. Nat. Rev. Cardiol. 17, 543–558. doi:10.1038/s41569-020-0413-9
Sala, A., Murphy, R. C., and Voelkel, N. F. (1991). Direct Airway Injury Results in Elevated Levels of Sulfidopeptide Leukotrienes, Detectable in Airway Secretions. Prostaglandins 42, 1–7. doi:10.1016/0090-6980(91)90088-W
Sanghai, N., and Tranmer, G. K. (2020). Taming the Cytokine Storm: Repurposing Montelukast for the Attenuation and Prophylaxis of Severe COVID-19 Symptoms. Drug Discov. Today 25, 2076–2079. doi:10.1016/j.drudis.2020.09.013
Sokolowska, M., Rovati, G. E., Diamant, Z., Untersmayr, E., Schwarze, J., Lukasik, Z., et al. (2021). Current Perspective on Eicosanoids in Asthma and Allergic Diseases: EAACI Task Force Consensus Report, Part I. Allergy 76, 114–130. doi:10.1111/all.14295
Tahan, F., Jazrawi, E., Moodley, T., Rovati, G. E., and Adcock, I. M. (2008). Montelukast Inhibits Tumour Necrosis Factor-Alpha-Mediated Interleukin-8 Expression through Inhibition of Nuclear Factor-kappaB P65-Associated Histone Acetyltransferase Activity. Clin. Exp. Allergy 38, 805–811. doi:10.1111/j.1365-2222.2008.02963.x
Taus, F., Salvagno, G., Canè, S., Fava, C., Mazzaferri, F., Carrara, E., et al. (2020). Platelets Promote Thromboinflammation in SARS-CoV-2 Pneumonia. Arterioscler. Thromb. Vasc. Biol. 40, 2975–2989. doi:10.1161/ATVBAHA.120.315175
Teuwen, L. A., Geldhof, V., Pasut, A., and Carmeliet, P. (2020). COVID-19: the Vasculature Unleashed. Nat. Rev. Immunol. 20, 389–391. doi:10.1038/s41577-020-0343-0
Trinh, H. K. T., Lee, S. H., Cao, T. B. T., and Park, H. S. (2019). Asthma Pharmacotherapy: an Update on Leukotriene Treatments. Expert Rev. Respir. Med. 13, 1169–1178. doi:10.1080/17476348.2019.1670640
Virchow, J. C., and Bachert, C. (2006). Efficacy and Safety of Montelukast in Adults with Asthma and Allergic Rhinitis. Respir. Med. 100, 1952–1959. doi:10.1016/j.rmed.2006.02.026
Woszczek, G., Chen, L. Y., Alsaaty, S., Nagineni, S., and Shelhamer, J. H. (2010). Concentration-dependent Noncysteinyl Leukotriene Type 1 Receptor-Mediated Inhibitory Activity of Leukotriene Receptor Antagonists. J. Immunol. 184, 2219–2225. doi:10.4049/jimmunol.0900071
Keywords: COVID-19, leukotrienes, leukotriene antagonists, platelet activation, tissue factor, P-selectin
Citation: Camera M, Canzano P, Brambilla M and Rovati GE (2022) Montelukast Inhibits Platelet Activation Induced by Plasma From COVID-19 Patients. Front. Pharmacol. 13:784214. doi: 10.3389/fphar.2022.784214
Received: 27 September 2021; Accepted: 14 January 2022;
Published: 08 February 2022.
Edited by:
Daniel Merk, Goethe University Frankfurt, GermanyReviewed by:
Suresh Kumar Kalangi, Amity University Gurgaon, IndiaAnthony Ashton, The University of Sydney, Australia
Copyright © 2022 Camera, Canzano, Brambilla and Rovati. This is an open-access article distributed under the terms of the Creative Commons Attribution License (CC BY). The use, distribution or reproduction in other forums is permitted, provided the original author(s) and the copyright owner(s) are credited and that the original publication in this journal is cited, in accordance with accepted academic practice. No use, distribution or reproduction is permitted which does not comply with these terms.
*Correspondence: G. Enrico Rovati, Z2Vucmljby5yb3ZhdGlAdW5pbWkuaXQ=