- State Institution “Institute of Nephrology National Academy of Medical Science of Ukraine”, Kyiv, Ukraine
A comprehensive understanding of urinary tract infections (UTIs), one of the most common human infections, is required as they are complex and poorly understood diseases. Periurethral and vaginal colonization by rectal flora, with the constant presence of pathogens in the urethra, is the initial step of the recurrent UTIs pathway. Current scientific data describe the genetic, etiological, biological, and behavioral risk factors for recurring UTIs, but they do not include the effect of intestinal barrier function on the disease. Although gut microbiota has been proposed as the main source for UTIs, the cross-talk between intestinal barrier dysfunction and the recurrence of UTIs has not yet been supported by scientific data. In this opinion review, based on published data and the results of our clinical studies, I aimed to outline the possible contribution of intestinal barrier dysfunction to the pathogenesis of recurrent UTIs. I believe that the unanswered questions raised by this review can guide further experimental and controlled studies to clarify the mechanisms underlying the role of intestinal barrier dysfunction in the pathogenesis of recurrent UTIs.
Introduction
Urinary tract infections (UTIs) are a worldwide weighty medical, economic, and social burden due to their high prevalence among sexually active women (Kolesnyk et al., 2014; Kolesnyk et al., 2016; Gaĭ;seniuk et al., 2013; Kolman, 2019). About 70% of sexually active premenopausal women have at least one episode of UTIs in their lives, while 25–44% have recurrent forms of UTIs (i.e., two episodes per 6 months or three or more recurrences during a year) (Foxman, 2014; Ke et al., 2021). Recurrent UTIs (RUTIs) are associated with significant financial expenses for both patients and government because of the cost of diagnostic tests and antibacterial therapy (Glover et al., 2014; Magruder et al., 2019). The emergence of multidrug-resistant bacterial strains and, more importantly, unsatisfactory treatment outcomes for both patients and physicians necessitate the search for alternative and advanced medical solutions.
The normal functioning of the gastrointestinal tract is balanced between the microbiota composition and the maintenance of the permeability of the mucosal barrier (Turner, 2009; Meijers et al., 2018; Ghosh et al., 2021). Under physiological conditions, the intestinal barrier is both permeable to nutrients and macromolecules and serves as a protective shield against pathogens and harmful substances (Turner, 2009; Ghosh et al., 2021). An imbalance in either the microbiota composition or the structural components of the intestinal barrier can result in increased intestinal permeability, leading to bacterial translocation and inflammation (Turner, 2009; Vancamelbeke and Vermeire, 2017; Muehler et al., 2020; Ghosh et al., 2021). However, the view of the intestinal barrier as simply a physical barrier that separates our body from the external environment has lost its relevance. In addition to the gut microbiome, many elements, such as short-chain fatty acids, tight junction proteins, mucins, secretory immunoglobulin A (sIg A), antimicrobial peptides, enzymes, and various other cellular regulators, play crucial roles in appropriate intestinal barrier functional integrity (Nagpal and Yadav, 2017; Vancamelbeke and Vermeire, 2017). Moreover, cytokines and chemokines, secreted by epithelial cells and stimulated by the gut microbiota, modulate host immune responses, which maintain the host’s immune system (Okumura and Takeda, 2017; Vancamelbeke and Vermeire, 2017). Accordingly, disruption of the composition and functioning of the microbiota and/or the intestinal barrier may be associated not only with intestinal inflammation but also inflammatory bowel diseases. Current knowledge of the relevance of the intestinal barrier’s function is providing increasing evidence of its interaction with neurological and autoimmune disease, non-alcoholic fatty liver disease, obesity and diabetes, rheumatoid arthritis, and chronic kidney diseases (Turner, 2009; Okumura and Takeda, 2017; Vancamelbeke and Vermeire, 2017; Meijers et al., 2018; Barbosa and Barbosa, 2020; Muehler et al., 2020). However, although antibiotics remain the basis of RUTIs treatment and prevention, there is a general lack of evidence for altered intestinal barrier function in patients with RUTIs. In this opinion review, I summarized the latest scientific data to outline the possible role of intestinal barrier dysfunction in the pathogenesis of RUTIs.
Routes of Urinary Tract Infection: An Old Issue With New Questions
Currently, two main routes of uropathogenic invasion are considered: an ascending route, which is realized through bacterial entry to the urothelium from colonies in the periurethral area, vagina, and/or rectum, and a hematogenous route (Stapleton, 2016; Meštrović et al., 2020). In physiological conditions, the glycosaminoglycan layers of urothelial plaques protect against bacterial colonization through urothelial cells and prevent microbial penetration (Abraham and Miao, 2015; Dalghi et al., 2020). The presence of any risk or complicating factor (e.g., female sex, UTI history, anatomical or functional abnormalities of the urinary tract, obstruction, vesicoureteral reflux, urine catheterization, and pregnancy or post-menopausal age) compromises the urothelial barrier function, that in response to uropathogenic Escherichia coli (UPEC) entry, leads to host inflammatory responses (Hooton, 2012; Abraham and Miao, 2015; Meštrović et al., 2020). However, most cases of uncomplicated UTIs cannot be explained by the presence of anatomical abnormalities or functional disorders. Therefore, obvious questions arise: Why doesn’t everyone develop UTIs under the same conditions? and Why do recurrences develop in the absence of complicating factors? However, unambiguous answers to these and many other questions are yet to be found. The value of genetic, hormonal, and/or metabolic factors; emotional and behavioral influences; the effect of urine or vaginal pH; inadequate local antibody production; and numerous other factors in the pathogenesis of uncomplicated UTIs have been actively discussed (Hooton, 2012; Foxman, 2014; Flores-Mireles et al., 2015; Behzadi, 2019; Storme et al., 2019; Wagenlehner et al., 2020). Moreover, the ability of UPEC to form virulence factors (e.g., adhesins, capsules, siderophores, and toxins) is a crucial facilitator of bacterial colonization and urothelium damage resulting in inflammation (Abraham and Miao, 2015; Terlizzi et al., 2017; Bunduki et al., 2021).
Nevertheless, despite the absence of exact answers to these questions, the ascending route of UTIs seems to be the most convincing, while the possibility of the hematogenous route of UTIs, in particular pyelonephritis, is a “standard” statement, which is yet to be confirmed in the absence of complicating factors. On the one hand, the hematogenous route is logical because the kidneys receive 20–25% of cardiac output (Kaufman et al., 2021), and any microorganism can be delivered to them. On the other hand, the same conditions of blood supply must provide sufficient resistance to the renal parenchyma and, therefore, the necessity of other conditions for pyelonephritis development to become obvious. Several early experimental studies have proven the hematogenous spread of UPEC to the kidneys (Larsson et al., 1980; Tanaka et al., 1981). However, no one has shown the possibility of pyelonephritis as a consequence of the bloodstream dissemination of Gram-negative bacteria without temporary occlusion of the ureter (Tancheva et al., 2011; Sullivan and Ulett, 2020). It should be emphasized that, unlike Gram-negative bacteria, group B streptococcus bacteria or Candida spp. can be disseminated to the kidneys via the hematogenous route even with a single injection of an appropriate volume and number of virulent bacteria (Sullivan and Ulett, 2020). These data can explain pyelonephritis in patients with septicemia caused by Gram-positive bacteria and negative urine cultures but not the development of RUTIs. In this context, the only explanation for uncomplicated RUTIs is an ascending route via intestinal, vaginal, or urinary dysbiosis. Nonetheless, should we always assume the obvious as true?
Altered Gut Microbiota: A Source or Consequence of Recurrent Urinary Tract Infections
The gut microbiota has been proposed as the main source of UTIs (Flores-Mireles et al., 2015; Stapleton, 2016; Magruder et al., 2019; Meštrović et al., 2020). Indeed, the intestinal origin of UPEC in patients with UTIs is supported by numerous studies (Paalanne et al., 2018; Magruder et al., 2019, 2020; Klein and Hultgren, 2020; Meštrović et al., 2020). For example, recent studies by Magruder et al. have demonstrated that an increased gut abundance of Enterobacteriaceae is associated with bacteriuria and the future development of UTIs in kidney transplant recipients (Magruder et al., 2019, 2020). Forde et al., after observing the dynamics of Escherichia coli (E. coli) strain 131 over 5 years in a woman with RUTIs, indicated the intestine as a reservoir for the recurrences (Forde et al., 2019). Moreover, current progress in metagenomic approaches has overturned the existing medical dogma of urinary tract sterility and demonstrated a large diversity of microbial species in the urine of patients without any clinical symptoms (Lewis et al., 2013; Neugent et al., 2020; Price et al., 2020). In addition, recent data provided by Thomas-White et al. (Thomas-White et al., 2018) and Price et al. (Price et al., 2020) have evidenced that not only UPEC but other different commensals, such as Lactobacillus spp., can be identified in the urinary bladder microbiota, suggesting a gut-derived source of pathogens. These new findings have thrown up many questions in need of further investigation: Does the gut microbiome cause the diversity of the urine microbiome? Does the urine microbiome change over time? Can we change the diversity of urinary commensals by influencing the gut microbiome?
UPEC are a pathotype of extraintestinal pathogenic E. coli (ExPEC) that can adapt to the competitive and volatile intestinal environment and colonize the urinary tract due to their numerous virulence factors and adhesive ability (Mann et al., 2017; Sarowska et al., 2019). The virulence factors of UPEC can be roughly divided into two groups: the bacterial cell surface factors (a number of different types of fimbriae, flagellum, capsular lipopolysaccharide, outer membrane proteins) and the secreted virulence factors (α-hemolysin, cytotoxic necrotizing factor 1, siderophores) (Behzadi, 2019; Sarowska et al., 2019; Shah et al., 2019; Khonsari et al., 2021). Both type 1 and P fimbriae encoded by fim and pap operon genes, respectively, are among the best-characterized bacterial fimbriae that promote adhesion to host cell surfaces, tissue invasion, biofilm formation, and cytokine expression (Terlizzi et al., 2017; Behzadi, 2019; Shah et al., 2019; Khonsari et al., 2021). Apart from adhesins, hemolysin, cytotoxic-necrotizing-factor, and siderophores encoded by hlyA, cnf1, and fyuA genes, respectively, are involved in intracellular survival, iron acquisition, host immune response, and tissue damage (Behzadi, 2019; Shah et al., 2019; Hozzari et al., 2020). To put the mentioned simply, UPEC possesses a huge number of virulent genes and factors, which allows them to develop different types of UTIs. It has been demonstrated that the phylogenetic group status which is based on the genomic Pathogenicity Islands and their associated virulence genes may contribute to the development of RUTIs and correlate with antibiotic resistance patterns (Luo et al., 2012; Shah et al., 2019; Hozzari et al., 2020). The phylogenetic groups B2 and D are traditionally considered to be the most virulent strains of UPEC (Behzadi, 2019; Khonsari et al., 2021) but recently, high percentages of phylogroup A strains have also been identified in UTI cases (Khairy et al., 2019). Nevertheless, despite the fundamental role of virulence factors in UPEC colonization and persistence, host epithelial dysfunction and its immune response are an integral part of UTI causing (Hooton, 2012; Sarowska et al., 2019; Meštrović et al., 2020).
In healthy individuals, ExPEC ingested with contaminated food or water are destroyed by the gut’s microbial community, while the intestinal epithelial layer creates a barrier against bacterial invasion (Mann et al., 2017; Govindarajan et al., 2020). Host epithelium dysfunction leads to bacterial imbalance and the overabundance of ExPEC in the intestine, resulting in the overproduction of toxins, local inflammation, and dysbiosis (Mann et al., 2017; Govindarajan et al., 2020). Additionally, ExPEC has been shown to specifically interact with the intestinal epithelial barrier (Bischoff et al., 2014; Francino, 2016; Poole et al., 2017) and trigger intestinal barrier dysfunction before the onset of disease (Turner, 2009; Nagpal and Yadav, 2017). The best example of this interaction is the regulation of epithelial barrier function by the activation of Toll-like receptors (TLRs). The main function of TLRs is the rapid recognition of pathogens (e.g., bacteria, viruses, and fungi) and the signaling of their unauthorized penetration of anatomical barriers (Vijay, 2018; El-Zayat et al., 2019; Behzadi et al., 2021). TLR signals regulate the activation of innate immunity and provide a relationship with acquired immunity through antigen-presenting cells (APCs) (Behzadi and Behzadi, 2016; Vijay, 2018; El-Zayat et al., 2019; Behzadi et al., 2021; Ghosh et al., 2021). Once the pathogenic bacteria adhere to the epithelium, TLRs on the surface of the APCs bind to components of the infected cells, such as the lipopolysaccharides (LPSs) of Gram-negative bacteria, and induce APCs to produce cytokines (Behzadi and Behzadi, 2016; Ghosh et al., 2021). In turn, LPSs disrupt junctional protein complexes and activate inflammatory cytokines released by TLR signaling, leading to increased gut permeability and bacterial translocation (Behzadi and Behzadi, 2016; Vijay, 2018; El-Zayat et al., 2019; Ghosh et al., 2021).
However, besides the overabundance of ExPEC per se, antibiotics can violate the quantitative and qualitative composition of gut microbiota and promote dysbiosis and the intestinal colonization of antibiotic-resistant bacteria (Lau et al., 2015; Yang et al., 2018; Stepanova, 2021). Patients with RUTIs must constantly take antibiotics, including long-term antibiotic prophylaxis (Fine et al., 2019; Issakhanian and Behzadi, 2019; Toor et al., 2019; Meštrović et al., 2020). Although antibiotics prevent UTI recurrences, antibiotic-induced dysbiosis leads to increased production and accumulation of LPSs and other toxic products of bacterial activity (e.g., P-cresol, trimethylamine-N-oxidoreductase, and indoxyl sulfate) and intestinal epithelial barrier dysfunction that accelerates excessive production of cytokines and chemokines and facilitates their translocation, which causes extraintestinal and/or systemic inflammation (Driyanska et al., 2014; Francino, 2016; Stepanova et al., 2018a; Gagliardi et al., 2018). It should be noted that the effect of antibiotics on intestinal barrier function is still poorly understood. In a recent in vivo study, Feng et al. examined the mechanisms of the effect of antibiotics on intestinal barrier function and suggested they induce tight junction dysfunction via decreasing expression of their proteins and the alteration zonula occludens protein 1 morphology (Feng et al., 2019). Moreover, the authors demonstrated antibiotic-derived activation of NLRP3 inflammasome and autophagy, which has been reported to be associated with intestinal barrier dysfunction (Feng et al., 2019). Holota et al. in a rat model showed an increase in cecum weight and a decrease in the level of mucus glycoproteins with a change in their carbohydrate composition in 56 days after antibiotic withdrawal (Holota et al., 2019). They also found changes in matrix metalloproteinase-2 and -9 activity, morphological remodeling of colon tissue, and increased colonic epithelial permeability to Evans blue dye, which was further confirmed by increased bacterial translocation from the lumen to the blood as late as 56 days after the antibiotic withdrawal (Holota et al., 2019). These experimental data provide evidence of long-term antibiotic-induced impairment of intestinal permeability that facilitates the translocation of pathogenic bacteria, antigens, and other microbial products to the systemic circulation leading to chronic inflammation (Johnson et al., 2015; Poole et al., 2017; Sharapatov et al., 2021). Gut microbiota-derived inflammation, so-called metabolic endotoxemia, has been recently demonstrated to play a key role in the pathogenesis of many diseases such as obesity, type 2 diabetes, pancreatitis, cardiovascular, systemic, kidney, liver, and bone diseases, as well as brain and mental disorders (Durack and Lynch, 2019; Barbosa and Barbosa, 2020; Ilchmann-Diounou and Menard, 2020; Ren et al., 2020; Skinner et al., 2020; Zhou et al., 2020). Interestingly, endotoxemia and low-level inflammation caused by increased intestinal permeability have also been demonstrated in psychological stress and depression, which often coexist with RUTIs (de Punder and Pruimboom, 2015; Trzeciak and Herbet, 2021). Given that after entering the bloodstream, pathogens and toxins can be delivered within one to 2 minutes to the vascular bed in any part of the body (Nash et al., 2015), why do we consider such a possibility for the above diseases and exclude the same for RUTIs?
Unfortunately, there are no evidenced clinical data on bacterial translocation in uncomplicated UTIs partially because of the methodological limitations. However, in a recent in vitro study, Owrangi et al. have demonstrated that UPEC strains isolated from patients with community-acquired UTIs not only had a similar rate of translocation through culturing human gut epithelium Caco-2 cells, but also translocated significantly more compared to UPEC strains from patients with urosepsis. Nonetheless, the virulence potential of urosepsis UPEC strains was significantly greater compared to community-acquired UTI strains, which allowed them to cause blood infection (Owrangi et al., 2018). Again, bacterial translocation is a constant physiological process that at a low level can be observed in healthy people and does not always manifest clinically. Most of the patients with bacterial translocation to mesenteric lymph nodes (the most reliable method of bacterial translocation evaluating in humans) showed no clinical infectious complications (Vaishnavi, 2013; Mohammad and Thiemermann, 2021). Besides, similar to the urinary microbiome, recent advances in culture-independent methods rejected the dogma of blood “sterility” and the existence of a “healthy” human blood microbiome has been demonstrated (D’Aquila et al., 2021; Castillo et al., 2019; Shah N. B et al., 2019). Unlike the clinical relevance of gut dysbiosis to human health, the problem of blood microbiome dysbiosis has only been addressed in a limited number of studies. However, the published data have indicated the changes of blood microbial taxonomic diversity in different diseases (Potgieter et al., 2015; Castillo et al., 2019; Shah N. B et al., 2019). Therefore, it is quite possible that the clinical relevance of bacterial translocation, and the “dormant” blood microbiome in patients with RUTIs, could depend on immune response and bacterial virulence properties influencing the severity and frequency of the infection.
Together, in my opinion, both the composition of the vaginal and urinary microbiota, as a consequence of gut dysbiosis, and the possible impact of the intestinal barrier dysfunction on bacterial translocation and chronic low-grade inflammation can trigger recurrences of uncomplicated UTI.
Is Intestinal Barrier Dysfunction Clinically Significant in Recurrent Urinary Tract Infections
Since 1885, when Theodor Escherich first identified E. coli (Shulman et al., 2007), this pathogen has remained the postulated dominant causative agent of UTIs. However, currently, Gram-positive bacteria (i.e., Enterococcus spp., Streptococcus spp., Staphylococcus saprophyticus, Staphylococcus epidermidis, and S. aureus) have confidently filled the niche of UTIs pathogens (Kline and Lewis, 2016; Kolesnyk et al., 2016; Gajdács et al., 2020; Petca et al., 2020). As stated in various research, the identification of Gram-positive bacteria in outpatients with UTIs rose steadily from 2% in 1971, up to 5.6% in 1990 (Felmingham et al., 1992), and up to 26% between 2008 and 2017 (Gajdács et al., 2020). According to our data, the frequency of Gram-positive bacteria identification depends on the recurrence rate, and it accounts for 40% of cases of uncomplicated RUTIs (Kolesnyk et al., 2016). However, compared to E. coli, Gram-positive bacteria are more often shed in complicated UTIs and associated with catheters, functional or anatomical abnormalities, comorbidities, immunosuppression, and nosocomial infections (Kline and Lewis, 2016; Petca et al., 2020). Therefore, the following question arises: How can we explain the significant spread of Gram-positive bacteria in uncomplicated RUTIs?
Again, the use of broad-spectrum antibiotics resulting in intestinal barrier dysfunction is the obvious explanation. However, unfortunately, in the current era of microbiome research, this issue has not been supported by scientific data. Based on comprehensive clinical examinations, we have previously demonstrated that all women with uncomplicated RUTIs were diagnosed with gut dysbiosis and decreased contents of Lactobacillus spp. and Bifidobacteria, and an increased number of conditionally pathogenic enterobacteria, E. coli, Clostridium, and Staphylococcus spp. in feces samples (Stepanova et al., 2017; Stepanova et al., 2018b). It should be noted, that all the examined patients had increased levels of sIg A and myeloperoxidase activity in coprofiltrates, which suggested enteric inflammation, while only 20% had clinical symptoms of gut dysbiosis. These data have been recently confirmed in a year-long study by Worby et al. in which the authors showed strong similarities between gut dysbiosis in RUTIs and inflammatory bowel disease characterized by depleted levels of butyrate-producing bacteria and diminished microbial richness (Worby et al., 2021). Moreover, the authors found a higher plasma marker of intestinal inflammation, eotaxin-1, in women with RUTIs compared to healthy controls, suggesting low-level enteric inflammation (Worby et al., 2021). The presence of such subclinical enteric inflammation in patients with RUTIs indicates the formation of a vicious circle. The overgrowth of ExPEC or antibiotic-induced intestinal barrier dysfunction causes enteric and systemic inflammation. In turn, the impaired intestinal barrier is the main source of the constant persistence of ExPEC in the urinary tract and a consequent predisposition for RUTIs (Figure 1). This leads to the next question: Should intestinal barrier dysfunction be considered a complicating factor for RUTIs? If yes, understanding the approaches that can improve intestinal barrier function may unlock new therapeutic strategies for the treatment and prevention of RUTIs. In addition, an honest answer to the question, How often do we prescribe an additional examination to exclude intestinal dysbiosis or barrier dysfunction in women without clinical manifestations? could significantly reduce the prevalence of uncomplicated RUTIs. In our experience, in the absence of clinical symptoms, the diagnosis of gut dysbiosis and/or intestinal barrier dysfunction is usually not carried out and remains unrecognized. I in no way urge readers to prescribe unreasonable, numerous, and expensive diagnostic methods, and do not underestimate the significance of other complicating factors for RUTIs. I simply call on readers to think about the diagnostic necessity and significance of intestinal barrier dysfunction in the pathogenesis of RUTIs.
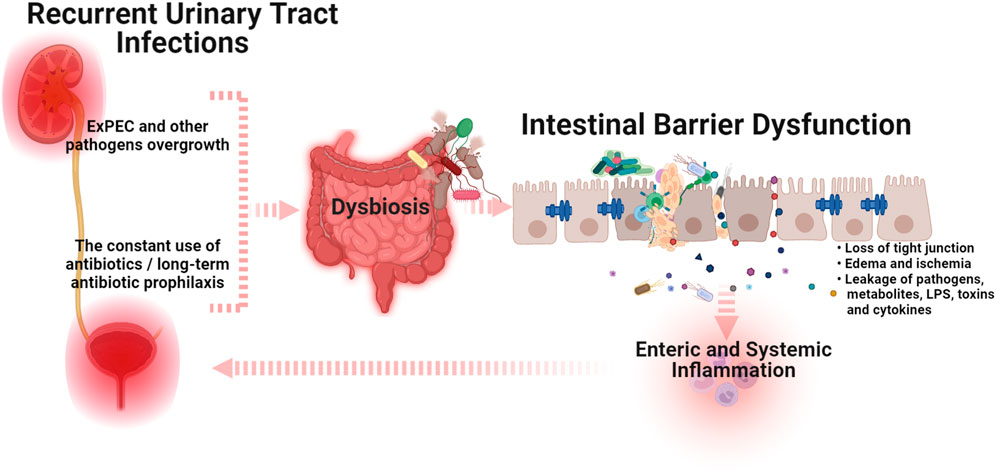
FIGURE 1. A schematic illustration of the cross-talk between intestinal barrier dysfunction and recurrent urinary tract infections (Graphic created by BioRender.com). The overgrowth of extraintestinal pathogenic E. coli (ExPEC) and/or the use of antibiotics that induce dysbiosis can trigger intestinal barrier dysfunction, which causes enteric and systemic inflammation. In turn, the impaired intestinal barrier is the main source of the constant persistence of ExPEC in the urinary tract and a consequent predisposition for RUTIs. Abbreviations: ExPEC, extraintestinal pathogenic E. coli; LPS, lipopolysaccharides.
Conclusion and Perspectives
Multiple environmental, genetic, host-related, and virulence risk factors play a determining role in the development of uncomplicated RUTIs. However, whatever the trigger, impaired intestinal barrier function appears to play a central role in urinary tract, enteric, and/or systemic inflammation. Although cross-talk between intestinal barrier dysfunction and RUTIs is not yet thoroughly understood, in this opinion review, I have presented a scientific rationale and underlined the possible role of intestinal barrier dysfunction in the pathogenesis of RUTIs. I believe that addressing the questions posed and many other questions will fill the knowledge gap on how intestinal barrier dysfunction is caused and how it can be restored in patients with RUTIs. As more and more research focuses on intestinal barrier function and its modulation, further potential therapeutic targets will result in the expansion of existing strategies for the treatment and prevention of RUTIs and provide new options for minimizing the prescription of antibiotics.
Author Contributions
The author confirms being the sole contributor to this work and has approved it for publication.
Conflict of Interest
The author declares that the research was conducted in the absence of any commercial or financial relationships that could be construed as a potential conflict of interest.
Publisher’s Note
All claims expressed in this article are solely those of the authors and do not necessarily represent those of their affiliated organizations, or those of the publisher, the editors and the reviewers. Any product that may be evaluated in this article, or claim that may be made by its manufacturer, is not guaranteed or endorsed by the publisher.
References
Abraham, S. N., and Miao, Y. (2015). The Nature of Immune Responses to Urinary Tract Infections. Nat. Rev. Immunol. 15, 655–663. doi:10.1038/NRI3887
Barbosa, P. M., and Barbosa, E. R. (2020). The Gut Brain-Axis in Neurological Diseases. Int. J. Cardiovas Sc 33, 528–536. doi:10.36660/IJCS.20200039
Behzadi, E., and Behzadi, P. (2016). The Role of Toll-like Receptors (TLRs) in Urinary Tract Infections (UTIs). Cent. Eur. J Urol 69, 404–410. doi:10.5173/ceju.2016.871
Behzadi, P. (20192019). Classical Chaperone-Usher (CU) Adhesive Fimbriome: Uropathogenic Escherichia coli (UPEC) and Urinary Tract Infections (UTIs). Folia Microbiol. (Praha) 65 (1 65), 45–65. doi:10.1007/S12223-019-00719-X
Behzadi, P., García-Perdomo, H. A., and Karpiński, T. M. (2021). Toll-Like Receptors: General Molecular and Structural Biology. J. Immunol. Res. 2021, 9914854. doi:10.1155/2021/9914854
Bischoff, S. C., Barbara, G., Buurman, W., Ockhuizen, T., Schulzke, J. D., Serino, M., et al. (2014). Intestinal Permeability-Aa New Target for Disease Prevention and Therapy. BMC Gastroenterol. 18, 14–189. doi:10.1186/s12876-014-0189-7
Bunduki, G. K., Heinz, E., Phiri, V. S., Noah, P., Feasey, N., and Musaya, J. (2021). Virulence Factors and Antimicrobial Resistance of Uropathogenic Escherichia coli (UPEC) Isolated from Urinary Tract Infections: a Systematic Review and Meta-Analysis. BMC Infect. Dis. 21 (1), 753. doi:10.1186/s12879-021-06435-7
Castillo, D. J., Rifkin, R. F., Cowan, D. A., and Potgieter, M. (2019). The Healthy Human Blood Microbiome: Fact or Fiction? Front Cell Infect Microbiol 9, 148. doi:10.3389/fcimb.2019.00148
D'Aquila, P., Giacconi, R., Malavolta, M., Piacenza, F., Bürkle, A., Villanueva, M. M., et al. (2021). Microbiome in Blood Samples from the General Population Recruited in the MARK-AGE Project: A Pilot Study. Front. Microbiol. 12, 707515. doi:10.3389/FMICB.2021.707515
Dalghi, M. G., Montalbetti, N., Carattino, M. D., and Apodaca, G. (2020). The Urothelium: Life in a Liquid Environment. Physiol. Rev. 100, 1621–1705. doi:10.1152/physrev.00041.2019
de Punder, K., and Pruimboom, L. (2015). Stress Induces Endotoxemia and Low-Grade Inflammation by Increasing Barrier Permeability. Front. Immunol. 6, 223. doi:10.3389/fimmu.2015.00223
Driyanska, V. E., Drannik, G. N., Stepanova, N. M., Lebed, L. O., Kruglikov, V. T., Driyanska, V. V., et al. (2014). Positive Effects of Modern Immunocorrection According to the Analysis of Cytokines and Slpi in Patients with Pyelonephritis. Lik Sprava 12, 45–56. PMID: 26638467.
Durack, J., and Lynch, S. V. (2019). The Gut Microbiome: Relationships with Disease and Opportunities for Therapy. J. Exp. Med. 216 (1), 20–40. doi:10.1084/JEM.20180448
El-Zayat, S. R., Sibaii, H., and Mannaa, F. A. (2019). Toll-like Receptors Activation, Signaling, and Targeting: an Overview. Bull. Natl. Res. Cent. 43, 1–12. doi:10.1186/s42269-019-0227-2
Felmingham, D., Wilson, A. P., Quintana, A. I., and Grüneberg, R. N. (1992). Enterococcus Species in Urinary Tract Infection. Clin. Infect. Dis. 15, 295–301. doi:10.1093/CLINIDS/15.2.295
Feng, Y., Huang, Y., Wang, Y., Wang, P., Song, H., and Wang, F. (2019). Antibiotics Induced Intestinal Tight junction Barrier Dysfunction Is Associated with Microbiota Dysbiosis, Activated NLRP3 Inflammasome and Autophagy. PLoS ONE 14, e0218384. doi:10.1371/journal.pone.0218384
Fine, R. L., Manfredo Vieira, S., Gilmore, M. S., and Kriegel, M. A. (2019). Mechanisms and Consequences of Gut Commensal Translocation in Chronic Diseases. Gut Microbes 11 (2), 217–230. doi:10.1080/19490976.2019.1629236
Flores-Mireles, A. L., Walker, J. N., Caparon, M., and Hultgren, S. J. (2015). Urinary Tract Infections: Epidemiology, Mechanisms of Infection and Treatment Options. Nat. Rev. Microbiol. 13, 269–284. doi:10.1038/NRMICRO3432
Forde, B. M., Roberts, L. W., Phan, M. D., Peters, K. M., Fleming, B. A., Russell, C. W., et al. (2019). Population Dynamics of an Escherichia coli ST131 Lineage during Recurrent Urinary Tract Infection. Nat. Commun. 10, 3643. doi:10.1038/S41467-019-11571-5
Foxman, B. (2014). Urinary Tract Infection Syndromes: Occurrence, Recurrence, Bacteriology, Risk Factors, and Disease Burden. Infect. Dis. Clin. North. Am. 28, 1–13. doi:10.1016/J.IDC.2013.09.003
Francino, M. P. (2016). Antibiotics and the Human Gut Microbiome: Dysbioses and Accumulation of Resistances. Front. Microbiol. 6 (6), 1543. doi:10.3389/fmicb.2015.01543
Gagliardi, A., Totino, V., Cacciotti, F., Iebba, V., Neroni, B., Bonfiglio, G., et al. (2018). Rebuilding the Gut Microbiota Ecosystem. Int. J. Environ. Res. Public Health 15, 1679. doi:10.3390/ijerph15081679
Gaĭseniuk, F. Z., Driianskaia, V. E., Drannik, G. N., Rudenko, M. I., Lavrenchuk, O. V., Stepanova, N. M., et al. (2013). Proinflammatory Cytokines in Patients with Pyelonephritis. Lik Sprava 6, 32–37. PMID: 25510086.
Gajdács, M., Ábrók, M., Lázár, A., and Burián, K. (2020). Increasing Relevance of Gram-Positive Cocci in Urinary Tract Infections: a 10-year Analysis of Their Prevalence and Resistance Trends. Sci. Rep. 10, 1–11. doi:10.1038/s41598-020-74834-y
Ghosh, S., Whitley, C. S., Haribabu, B., and Jala, V. R. (2021). Regulation of Intestinal Barrier Function by Microbial Metabolites. Cell Mol Gastroenterol Hepatol 11 (5), 1463–1482. doi:10.1016/j.jcmgh.2021.02.007
Glover, M., Moreira, C. G., Sperandio, V., and Zimmern, P. (2014). Recurrent Urinary Tract Infections in Healthy and Nonpregnant Women. Urol. Sci. 25, 1–8. doi:10.1016/j.urols.2013.11.007
Govindarajan, D. K., Viswalingam, N., Meganathan, Y., and Kandaswamy, K. (2020). Adherence Patterns of Escherichia coli in the Intestine and its Role in Pathogenesis. Med. Microecology 5, 100025. doi:10.1016/J.MEDMIC.2020.100025
Holota, Y., Dovbynchuk, T., Kaji, I., Vareniuk, I., Dzyubenko, N., Chervinska, T., et al. (2019). The Long-Term Consequences of Antibiotic Therapy: Role of Colonic Short-Chain Fatty Acids (SCFA) System and Intestinal Barrier Integrity. PLoS ONE 2214 (8), e0220642. doi:10.1371/JOURNAL.PONE.0220642
Hooton, T. M. (2012). Clinical Practice. Uncomplicated Urinary Tract Infection. N. Engl. J. Med. 366, 1028–1037. doi:10.1056/NEJMcp1104429
Hozzari, A., Behzadi, P., Kerishchi Khiabani, P., Sholeh, M., and Sabokroo, N. (2020). Clinical Cases, Drug Resistance, and Virulence Genes Profiling in Uropathogenic Escherichia coli. J. Appl. Genet. 61 (22), 265–273. doi:10.1007/S13353-020-00542-Y
Ilchmann-Diounou, H., and Menard, S. (2020). Psychological Stress, Intestinal Barrier Dysfunctions, and Autoimmune Disorders: An Overview. Front. Immunol. 11, 1823. doi:10.3389/fimmu.2020.01823
Issakhanian, L., and Behzadi, P. (2019). Antimicrobial Agents and Urinary Tract Infections. Curr. Pharm. Des. 25, 1409–1423. doi:10.2174/1381612825999190619130216
Johnson, J. R., Porter, S., Johnston, B., Kuskowski, M. A., Spurbeck, R. R., Mobley, H. L., et al. (2015). Host Characteristics and Bacterial Traits Predict Experimental Virulence for Escherichia coli Bloodstream Isolates from Patients with Urosepsis. Open Forum Infect. Dis. 2 (3), ofv083. doi:10.1093/ofid/ofv083
Kaufman, D., Basit, H., and Knohl, S. (2021). “Physiology, Glomerular Filtration Rate,” in StatPearls. Treasure Island ((FL: StatPearls Publishing). PMID: 29763208.
Ke, Q. S., Lee, C. L., and Kuo, H. C. (2021). Recurrent Urinary Tract Infection in Women and Overactive Bladder - Is There a Relationship? Tzu Chi Med. J. 33, 13–21. doi:10.4103/TCMJ.TCMJ_38_20
Khairy, R. M., Mohamed, E. S., Abdel Ghany, H. M., and Abdelrahim, S. S. (2019). Phylogenic Classification and Virulence Genes Profiles of Uropathogenic E. coli and Diarrhegenic E. coli Strains Isolated from Community Acquired Infections. PLoS One 14 (9), e0222441. doi:10.1371/JOURNAL.PONE.0222441
Khonsari, M. S., Behzadi, P., and Foroohi, F. (2021). The Prevalence of Type 3 Fimbriae in Uropathogenic Escherichia coli Isolated from Clinical Urine Samples. Meta Gene 28, 100881. doi:10.1016/J.MGENE.2021.100881
Klein, R. D., and Hultgren, S. J. (2020). Urinary Tract Infections: Microbial Pathogenesis, Host-Pathogen Interactions and New Treatment Strategies. Nat. Rev. Microbiol. 18, 211–226. doi:10.1038/s41579-020-0324-0
Kline, K. A., and Lewis, A. L. (2016). Gram-Positive Uropathogens, Polymicrobial Urinary Tract Infection, and the Emerging Microbiota of the Urinary Tract. Microbiol. Spectr. 4 (2). doi:10.1128/microbiolspec.UTI-0012-201210.1128/microbiolspec.UTI-0012-2012
Kolesnyk, M., Stepanova, N., Korol, L., Romanenko, O., and Mygal, L. (2014). Prediction of Recurrent Pyelonephritis by an Index of Oxidative Stress. Lik Sprava 9-10, 81–88. PMID: 26492781.
Kolesnyk, M., Stepanova, N., Kruglikov, V., and Rudenko, A. (2016). The Etiological Spectrum and Antibiotic Resistance Pattern of Bacteria Causing Uncomplicated Urinary Tract Infections: a Ten-Year Surveillance Study (2005-2015). Ukr. Journ. Nephrol. Dial. 49, 32–41. doi:10.31450/UKRJND.1(49).2016.02
Kolman, K. B. (2019). Cystitis and Pyelonephritis: Diagnosis, Treatment, and Prevention. Prim. Care 46, 191–202. doi:10.1016/j.pop.2019.01.001
Larsson, P., Kaijser, B., Baltzer, I. M., and Olling, S. (1980). An Experimental Model for Ascending Acute Pyelonephritis Caused by Escherichia coli or proteus in Rats. J. Clin. Pathol. 33, 408–412. doi:10.1136/jcp.33.4.408
Lau, W. L., Kalantar-Zadeh, K., and Vaziri, N. D. (2015). The Gut as a Source of Inflammation in Chronic Kidney Disease. Nephron 130, 92–98. doi:10.1159/000381990
Lewis, D. A., Brown, R., Williams, J., White, P., Jacobson, S. K., Marchesi, J. R., et al. (2013). The Human Urinary Microbiome; Bacterial DNA in Voided Urine of Asymptomatic Adults. Front. Cell. Infect. Microbiol. 3, 41. doi:10.3389/fcimb.2013.00041
Luo, Y., Ma, Y., Zhao, Q., Wang, L., Guo, L., Ye, L., et al. (2012). Similarity and Divergence of Phylogenies, Antimicrobial Susceptibilities, and Virulence Factor Profiles of Escherichia coli Isolates Causing Recurrent Urinary Tract Infections that Persist or Result from Reinfection. J. Clin. Microbiol. 50 (12), 4002–4007. doi:10.1128/JCM.02086-12
Magruder, M., Edusei, E., Zhang, L., Albakry, S., Satlin, M. J., Westblade, L. F., et al. (2020). Gut Commensal Microbiota and Decreased Risk for Enterobacteriaceae Bacteriuria and Urinary Tract Infection. Gut Microbes 912 (1), 1805281. doi:10.1080/19490976.2020.1805281
Magruder, M., Sholi, A. N., Gong, C., Zhang, L., Edusei, E., Huang, J., et al. (2019). Gut Uropathogen Abundance Is a Risk Factor for Development of Bacteriuria and Urinary Tract Infection. Nat. Commun. 10, 5521–5529. doi:10.1038/s41467-019-13467-w
Mann, R., Mediati, D. G., Duggin, I. G., Harry, E. J., and Bottomley, A. L. (2017). Metabolic Adaptations of Uropathogenic E. coli in the Urinary Tract. Front. Cell. Infect. Microbiol. 7, 241. doi:10.3389/fcimb.2017.00241
Meijers, B., Farré, R., Dejongh, S., Vicario, M., and Evenepoel, P. (2018). Intestinal Barrier Function in Chronic Kidney Disease. Toxins (Basel) 10 (7), 298. doi:10.3390/toxins10070298
Meštrović, T., Matijašić, M., Perić, M., Čipčić Paljetak, H., Barešić, A., and Verbanac, D. (2020). The Role of Gut, Vaginal, and Urinary Microbiome in Urinary Tract Infections: From Bench to Bedside. Diagnostics 11, 7. doi:10.3390/diagnostics11010007
Mohammad, S., and Thiemermann, C. (2020). Role of Metabolic Endotoxemia in Systemic Inflammation and Potential Interventions. Front. Immunol. 11, 594150. doi:10.3389/fimmu.2020.594150
Muehler, A., Slizgi, J. R., Kohlhof, H., Groeppel, M., Peelen, E., and Vitt, D. (2020). Clinical Relevance of Intestinal Barrier Dysfunction in Common Gastrointestinal Diseases. World J. Gastrointest. Pathophysiol 11, 114–130. doi:10.4291/WJGP.V11.I6.114
Nagpal, R., and Yadav, H. (2017). Bacterial Translocation from the Gut to the Distant Organs: An Overview. Ann. Nutr. Metab. 71 Suppl 1, 11–16. doi:10.1159/000479918
Neugent, M. L., Hulyalkar, N. V., Nguyen, V. H., Zimmern, P. E., and de Nisco, N. J. (2020). Advances in Understanding the Human Urinary Microbiome and its Potential Role in Urinary Tract Infection. mBio 11. doi:10.1128/MBIO.00218-20
Okumura, R., and Takeda, K. (2017). Roles of Intestinal Epithelial Cells in the Maintenance of Gut Homeostasis. Exp. Mol. Med. 49, e338. doi:10.1038/EMM.2017.20
Owrangi, B., Masters, N., Kuballa, A., O'Dea, C., Vollmerhausen, T. L., and Katouli, M. (2018). Invasion and Translocation of Uropathogenic Escherichia coli Isolated from Urosepsis and Patients with Community-Acquired Urinary Tract Infection. Eur. J. Clin. Microbiol. Infect. Dis. 37 (5), 833–839. doi:10.1007/S10096-017-3176-4
Paalanne, N., Husso, A., Salo, J., Pieviläinen, O., Tejesvi, M. V., Koivusaari, P., et al. (2018). Intestinal Microbiome as a Risk Factor for Urinary Tract Infections in Children. Eur. J. Clin. Microbiol. Infect. Dis. 37 (10 37), 1881–1891. doi:10.1007/S10096-018-3322-7
Petca, R. C., Mareș, C., Petca, A., Negoiță, S., Popescu, R. I., Boț, M., et al. (2020). Spectrum and Antibiotic Resistance of Uropathogens in Romanian Females. Antibiotics (Basel) 9, 472–479. doi:10.3390/ANTIBIOTICS9080472
Poole, N. M., Green, S. I., Rajan, A., Vela, L. E., Zeng, X. L., Estes, M. K., et al. (2017). Role for FimH in Extraintestinal Pathogenic Escherichia coli Invasion and Translocation through the Intestinal Epithelium. Infect. Immun. 85 (11), e00581–17. doi:10.1128/IAI.00581-17
Potgieter, M., Bester, J., Kell, D. B., and Pretorius, E. (2015). The Dormant Blood Microbiome in Chronic, Inflammatory Diseases. FEMS Microbiol. Rev. 39 (4), 567–591. doi:10.1093/femsre/fuv013
Price, T. K., Hilt, E. E., Thomas-White, K., Mueller, E. R., Wolfe, A. J., and Brubaker, L. (2020). The Urobiome of Continent Adult Women: a Cross-Sectional Study. BJOG 127, 193–201. doi:10.1111/1471-0528.15920
Ren, Z., Fan, Y., Li, A., Shen, Q., Wu, J., Ren, L., et al. (2020). Alterations of the Human Gut Microbiome in Chronic Kidney Disease. Adv. Sci. (Weinh) 7, 2001936. doi:10.1002/advs.202001936
Sarowska, J., Futoma-Koloch, B., Jama-Kmiecik, A., Frej-Madrzak, M., Ksiazczyk, M., Bugla-Ploskonska, G., et al. (2019). Virulence Factors, Prevalence and Potential Transmission of Extraintestinal Pathogenic Escherichia coli Isolated from Different Sources: Recent Reports. Gut Pathog. 11, 10. doi:10.1186/S13099-019-0290-0
Shah, C., Baral, R., Bartaula, B., and Shrestha, L. B. (2019). Virulence Factors of Uropathogenic Escherichia coli (UPEC) and Correlation with Antimicrobial Resistance. BMC Microbiol. 19 (1), 204–206. doi:10.1186/S12866-019-1587-3/TABLES/4
Shah, N. B., Allegretti, A. S., Nigwekar, S. U., Kalim, S., Zhao, S., Lelouvier, B., et al. (2019). Blood Microbiome Profile in CKD : A Pilot Study. Clin. J. Am. Soc. Nephrol. 14 (5), 692–701. doi:10.2215/CJN.12161018/-/DCSUPPLEMENTAL
Sharapatov, Y., Turgunov, Y., and Lavrinenko, A. (2021). Pathogenic Mechanisms of Acute Obstructive Pyelonephritis. Open Access Maced J. Med. Sci. 9, 124–128. doi:10.3889/oamjms.2021.5876
Shulman, S. T., Friedmann, H. C., and Sims, R. H. (2007). Theodor Escherich: The First Pediatric Infectious Diseases Physician? Clin. Infect. Dis. 45, 1025–1029. doi:10.1086/521946
Skinner, C., Thompson, A. J., Thursz, M. R., Marchesi, J. R., and Vergis, N. (2020). Intestinal Permeability and Bacterial Translocation in Patients with Liver Disease, Focusing on Alcoholic Aetiology: Methods of Assessment and Therapeutic Intervention. Therap Adv. Gastroenterol. 13, 1756284820942616. doi:10.1177/1756284820942616
Stapleton, A. E. (2016). The Vaginal Microbiota and Urinary Tract Infection. Microbiol. Spectr. 4 (6). doi:10.1128/microbiolspec.UTI-0025-201610.1128/MICROBIOLSPEC.UTI-0025-2016
Stepanova, N., Driyanska, V., Korol, L., Mihal, L., and Savchenko, V. (2018a). The Effects of Gut Indigenous Microbiota on Intensity of Oxidative Stress and the Cytokine Immunity in Women with Recurrent Pyelonephritis. Med. Perspekt 23 (part1), 129–135. doi:10.26641/2307-0404.2018.1(part1).127251
Stepanova, N. (2021). Hyperoxaluria; a Risk Factor or a Consequence of Recurrent Pyelonephritis? J. Nephropathol 10 (4), e36. doi:10.34172/jnp.2021.36
Stepanova, N., Stashevska, N., Driyanska, V., and Kolesnyk, M. (2017). Intestinal Colonization Resistance Is Associated with Hyperoxaluria in the Patients with Recurrent Pyelonephritis. Eur. Urol. Supplements 16, e402–e403. doi:10.1016/S1569-9056(17)30298-1
Stepanova, N., Tolstanova, G., Sergiychuk, T., and Akulenko, I. (2018b). Intestinal Barrier Dysfunction in Hyperoxaluria Women with Recurrent Pyelonephritis. Eur. Urol. Supplements 17, e473. doi:10.1016/S1569-9056(18)31175-8
Storme, O., Tirán Saucedo, J., Garcia-Mora, A., Dehesa-Dávila, M., and Naber, K. G. (2019). Risk Factors and Predisposing Conditions for Urinary Tract Infection. Ther. Adv. Urol. 11, 1756287218814382. doi:10.1177/1756287218814382
Sullivan, M. J., and Ulett, G. C. (2020). Evaluation of Hematogenous Spread and Ascending Infection in the Pathogenesis of Acute Pyelonephritis Due to Group B streptococcus in Mice. Microb. Pathog. 138, 103796. doi:10.1016/j.micpath.2019.103796
Tanaka, N., Une, T., and Ogawa, H. (1981). Experimental Pyelonephritis in Mice Following Ascending Infection with E. coli. Chronic Phase. Acta Pathol. Jpn. 31, 189–198. doi:10.1111/J.1440-1827.1981.TB01364.X
Tancheva, S., Valcheva-Kuzmanova, S. V., Radev, R. Z., Marinov, M. D., Boychev, B., Stoeva, T., et al. (2011). A Model of Experimental Acute Hematogenous Pyelonephritis in the Rat. Folia Med. (Plovdiv) 53, 63–68. doi:10.2478/v10153-010-0039-5
Terlizzi, M. E., Gribaudo, G., and Maffei, M. E. (2017). UroPathogenic Escherichia coli (UPEC) Infections: Virulence Factors, Bladder Responses, Antibiotic, and Non-antibiotic Antimicrobial Strategies. Front. Microbiol. 8, 1566. doi:10.3389/fmicb.2017.01566
Thomas-White, K., Forster, S. C., Kumar, N., Van Kuiken, M., Putonti, C., Stares, M. D., et al. (2018). Culturing of Female Bladder Bacteria Reveals an Interconnected Urogenital Microbiota. Nat. Commun. 9, 1557. doi:10.1038/S41467-018-03968-5
Toor, D., Wsson, M. K., Kumar, P., Karthikeyan, G., Kaushik, N. K., Goel, C., et al. (2019). Dysbiosis Disrupts Gut Immune Homeostasis and Promotes Gastric Diseases. Int. J. Mol. Sci. 20 (10), 2432. doi:10.3390/ijms20102432
Trzeciak, P., and Herbet, M. (2021). Role of the Intestinal Microbiome, Intestinal Barrier and Psychobiotics in Depression. Nutrients 13 (3), 927. doi:10.3390/nu13030927
Turner, J. R. (2009). Intestinal Mucosal Barrier Function in Health and Disease. Nat. Rev. Immunol. 9 (11 9), 799–809. doi:10.1038/nri2653
Vaishnavi, C. (2013). Translocation of Gut flora and its Role in Sepsis. Indian J. Med. Microbiol. 31, 334–342. doi:10.4103/0255-0857.118870
Vancamelbeke, M., and Vermeire, S. (2017). The Intestinal Barrier: a Fundamental Role in Health and Disease. Expert Rev. Gastroenterol. Hepatol. 11, 821–834. doi:10.1080/17474124.2017.1343143
Vijay, K. (2018). Toll-like Receptors in Immunity and Inflammatory Diseases: Past, Present, and Future. Int. Immunopharmacology 59, 391–412. doi:10.1016/j.intimp.2018.03.002
Wagenlehner, F. M. E., Bjerklund Johansen, T. E., Cai, T., Koves, B., Kranz, J., Pilatz, A., et al. (2020). Epidemiology, Definition and Treatment of Complicated Urinary Tract Infections. Nat. Rev. Urol. 17, 586–600. doi:10.1038/s41585-020-0362-4
Worby, C. J., Schreiber, H. L., Straub, T. J., van Dijk, L. R., Bronson, R. A., Olson, B., et al. (2021). Gut-bladder axis Syndrome Associated with Recurrent UTIs in Humans. medRxiv. doi:10.1101/2021.11.15.21266268
Keywords: recurrent urinary tract infections, intestinal barrier dysfunction, dysbiosis, enteric inflammation, uropathogen
Citation: Stepanova N (2022) How Advanced Is Our Understanding of the Role of Intestinal Barrier Dysfunction in the Pathogenesis of Recurrent Urinary Tract Infections. Front. Pharmacol. 13:780122. doi: 10.3389/fphar.2022.780122
Received: 20 September 2021; Accepted: 24 February 2022;
Published: 10 March 2022.
Edited by:
Stefania Marzocco, University of Salerno, ItalyReviewed by:
Agata Mulak, Wroclaw Medical University, PolandPayam Behzadi, Islamic Azad University, ShahreQods, Iran
Leszek Rudzki, Medical University of Bialystok, Poland
Copyright © 2022 Stepanova. This is an open-access article distributed under the terms of the Creative Commons Attribution License (CC BY). The use, distribution or reproduction in other forums is permitted, provided the original author(s) and the copyright owner(s) are credited and that the original publication in this journal is cited, in accordance with accepted academic practice. No use, distribution or reproduction is permitted which does not comply with these terms.
*Correspondence: Natalia Stepanova, nmstep88@gmail.com