- 1Guangdong Provincial Hospital of Traditional Chinese Medicine, The Second Clinical Medical College of Guangzhou University of Chinese Medicine, Guangzhou, China
- 2Guangzhou Key Laboratory of Chirality Research on Active Components of Traditional Chinese Medicine, Guangzhou, China
- 3Guangdong Provincial Key Laboratory of Clinical Research on Traditional Chinese Medicine Syndrome, Guangzhou, China
The root of Reynoutria multiflora Thunb. Moldenke (RM, syn.: Polygonum multiflorum Thunb.) has been widely used in TCM clinical practice for centuries. The raw R. multiflora (RRM) should be processed before use, in order to reduce toxicity and increase efficiency. However, the content of trans-2, 3, 5, 4′-tetrahydroxystilbene-2-O-β-D-glucopyranoside (trans-THSG), which is considered to be the main medicinal ingredient, decreases in this process. In order to understand the changes of stilbene glycosides raw R. multiflora (RRM) and processed R. multiflora (PRM), a simple and effective method was developed by ultra high performance liquid chromatography tandem quadrupole/electrostatic field orbitrap high-resolution mass spectrometry (UHPLC-Q-Exactive plus orbitrap MS/MS). The content and quantity of stilbene glycosideshave undergone tremendous changes during the process. Seven parent nucleus of stilbene glycosides and 55 substituents, including 5-HMF and a series of derivatives, were identified in PM. 146 stilbene glycosides were detected in RRM, The number of detected compounds increased from 198 to 219 as the processing time increased from 4 to 32 h. Among the detected compounds, 102 stilbene glycosides may be potential new compounds. And the changing trend of the compounds can be summarized in 3 forms: gradually increased, gradually decreased, first increased and then decreased or decreased first. The content of trans-THSG was indeed decreased during processing, as it was converted into a series of derivatives through the esterification reaction with small molecular compounds. The clarification of secondary metabolite group can provide a basis for the follow-up study on the mechanism of pharmacodynamics and toxicity of PM, and for screening of relevant quality markers.
1 Introduction
Traditional Chinese medicine processing is a unique pharmaceutical technology derived from the theory of traditional Chinese medicine. It has played a prominent role in the clinical practice of traditional Chinese medicine for thousands of years, ensuring the safety and effectiveness of treatment. After processing with different temperatures, durations, solvents or excipients, the components of traditional Chinese medicine have undergone different changes. Ingredients will be dissolved, decomposed or transformed into new components, resulting in increasing or decreasing of the compounds. All these changes are closely related to the property and efficacy of traditional Chinese medicine. Therefore, it is of great significance to study the changes of chemical components before and after processing of traditional Chinese medicine.
The root of Reynoutria multiflora Thunb. (Polygonum multiflorum Thunb.), well known as He-shou-wu in China, has been widely used in TCM clinical practice for centuries (Li et al., 2017). Lots of research have shown that RRM and its processed products have different pharmacological effects. RRM has the effect of detoxification, carbuncle elimination, relaxing bowel. And PRM shows the effect of tonifying liver and kidney, tonic medicines and hair-blacking (Cheung et al., 2014; Lin et al., 2015; Chinese Pharmacopoeias Commission, 2020). RRM is commonly processed by steaming with black bean or water, which has been officially documented in the Chinese pharmacopoeia. However, the processing time was not specified in the processing specification. Therefore, the processing time of PRM on the market varies greatly, ranging from 2 to 18 h (Lin et al., 2018). But in our previous studies, we have screened out that the best effect of PRM was processing for 24–32 h (Qiu et al., 2008). The quality of PRM is inhomogeneous in the market, the main reason for this phenomenon is that the processing mechanism of PRM is not clear. The increased reports of hepatotoxicity of RRM in recent years (Dong et al., 2014; Lei et al., 2015; Zhang et al., 2019) may also be related to incomplete processing.
Previous research indicated that the main chemical components of RM were secondary metabolites, including stilbene glycosides, anthraquinone and polyphenols were the most representative (Choi et al., 2007; Lin et al., 2015; Sun et al., 2015). The fragmentation pathways of typical constituents and chemical profiles of RM have been studied by an on-line UHPLC-ESI-linear ion trap-Orbitrap hybrid mass spectrometry method (Xu et al., 2012; Qiu et al., 2013). The secondary metabolites were quantitatively analyzed by HPLC/LC-MS/MS to study the chemical components before and after processing of R. multiflora, which showed that the content of some chemical substances was changed by processing. In our previous study, the contents of 5-HMF, THSG, emodin and physcion are changed during the processing (Chen et al., 2012). The content of THSG, a compound that possess anti-oxidative, anti-aging, anti-tumor, anti-inflammatory and liver protective activities (Lv et al., 2007; Shao et al., 2012; Lin et al., 2015; Yang et al., 2020), was decreased (Qiu et al., 2006; Fu, 2011). However, there is no research report on the secondary metabolite group produced by stilbene glycosides in the process, and the clarification of secondary metabolite group can provide a basis for the follow-up study on the mechanism of pharmacodynamics and toxicity of PM, and for screening of relevant quality markers.
In this study, a simple and rapid method for the determination of RRM and PRM by UHPLC-Q-Exactive plus orbitrap MS/MS was established, and the qualitative analysis of RRM and PRM were carried out in vitro to obtain a clear chemical map. The fragment ions at m/z 405.1087 and 243.0656 were selected as characteristic fragments, the secondary metabolites in RRM and processed PRM samples prepared with different durations were characterized and identified, then, the changes of stilbene glycosides during processing were further analyzed.
2 Materials and Methods
2.1 Materials
RRM and PRMs that had been processed for 4, 8, 12, 18, 24 and 32 h were provided by Shanghai Dehua Traditional Chinese Medicine CO., Ltd., and the corresponding batch numbers were HSW2018051101-S, HSW2018051101-4H, HSW2018051101-8H, HSW2018051101-12H, HSW2018051101-18H, HSW2018051101-24H, and HSW2018051101-32H. The samples were authenticated by Professor Zhihai Huang, and voucher specimens were deposited in the Materials Medica Preparation Lab of the Second Affiliated Hospital of the Guangzhou University of Chinese Medicine.
Trans-2, 3, 5, 4′-tetrahydroxystilbene-2-O-β-D-glucopyranoside (THSG), cis-THSG and polydatin were purchased from yuanye Bio-Technology Co., Ltd. Acetonitrile (No. H08J11E115101, P27A11P107214, T15A10F85743, purity ≥98%, Shanghai, China). acetonitrile and methanol (HPLC grade), were supplied by E. Merck (Darmstadt, Germany), formic acid (HPLC grade) was purchased from fisher (United States), ultra-pure water was prepared by a Mili-Q water purification system (Millipore, MA, United States).
2.2 Sample Processing Method
All the samples were prepared using following method: 1 g sample powder was ultrasonicated for 30 min with 25 ml of 70% ethanol, followed by filtration and then evaporated the filtrate. 5 ml of ultrapure water were added to dissolve the residue and then extracted twice with 15 ml of ethyl acetate. The resulting mixture was combined with an ethyl acetate solution and evaporated over a water bath; after that, 1 ml of methanol was added to dissolve the residue and centrifugation (15,000 rpm, 4°C) for 10 min by a 1.5 ml centrifuge tube. Finally, the supernatant of the treatment samples was injected into the UPLC-Q-Exactive plus orbitrap MS/MS system.
2.3 UHPLC-Q-Exactive Plus Orbitrap MS/MS Analysis
2.3.1 Liquid Chromatography
All the samples were analysed using an Ultimate 3000 UPLC system (Dionex, United States) that was controlled with Thermo Xcalibur software (Thermo Fisher Scientific, United States). The samples were separated using a Kinetex UPLC C18 column (100*2.1 mm, 1.7 µm) (Phenomenex, United States). The mobile phase consisted of solvent A (0.1% formic acid) and solvent B (acetonitrile). A gradient elution was applied using the following optimized gradient program: 8-8% B at 0–3 min, 8–28% B at 3–25 min, 28–40% B at 25–26 min, 40–50% B at 26–28 min, 50–70% B at 28–30 min, 70–90% B at 30–32 min, and 90–90% B at 32–35 min. The flow rate was kept at 0.4 ml/min, the sample injection volume was 1 μL, and the column temperature was maintained at 25°C.
2.4 Mass Spectrometry
Mass spectrometry was performed on a Q-Exactive Plus™ quadrupole-Orbitrap mass spectrometer (Thermo Fisher Scientific, United States) in negative ion mode. The scan mass range was set at m/z 100–1,200. The parameter settings were as follows: a full scan and fragment spectral resolution of 70,000 FWHM and 17, 50 FWHM, respectively; capillary temperature was 350°C; auxiliary gas heater temperature was 350°C; spray voltage was −3.2 KV; sheath gas flow rate was 40 Arb; auxiliary gas flow rate was 15 Arb; and S-lens RF level was set at 50. The acquisition mode of stepped NCE (normalized collision energy) was using with settings of 30, 50, and 70 eV. The accumulated resultant fragment ions were injected into the Orbitrap mass analyzer for single-scan detection.
Considering the possible elemental composition of the RM components, the types and quantities of expected atoms were set as follows: carbon ≤50, hydrogen ≤200, oxygen ≤20, nitrogen ≤3. The accuracy error threshold was fixed at 5 ppm.
3 Results and Discussion
3.1 Base Peak Chromatograms
The chemical profiles of RRM and processing PRMs were analyzed by UHPLC-Q-Exactive plus orbitrap MS/MS, the representative base peak chromatograms of RRM and processing PRM (24 h) are shown in Figure 1. Some differences were observed between the two base peak chromatograms. The stilbene glycosides and their derivatives were distributed from 7 to 22 min. The representative base peak chromatograms of processing of PRM (7–22 min) are shown in Figure 2.
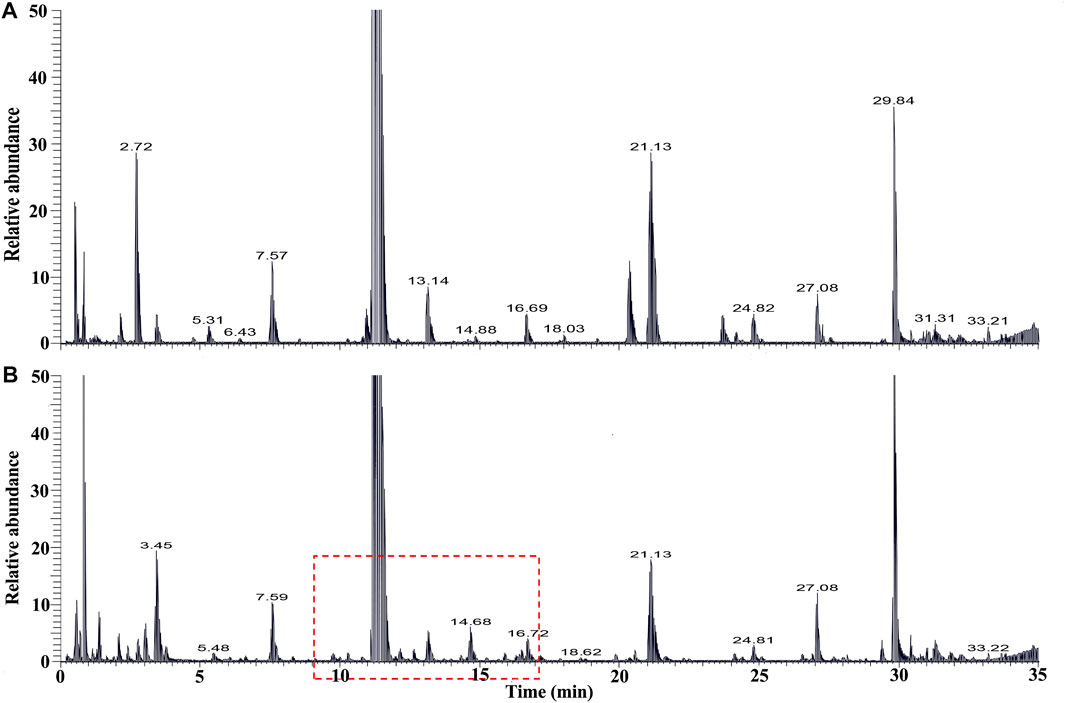
FIGURE 1. The Base peak intensity chromatograms of samples of raw Reynoutria multiflora Thunb. [RRM, (A)] and processed Reynoutria multiflora Thunb. [PRM for 24h, (B)] derived from UHPLC-Q-Exactive plus orbitrap MS/MS.
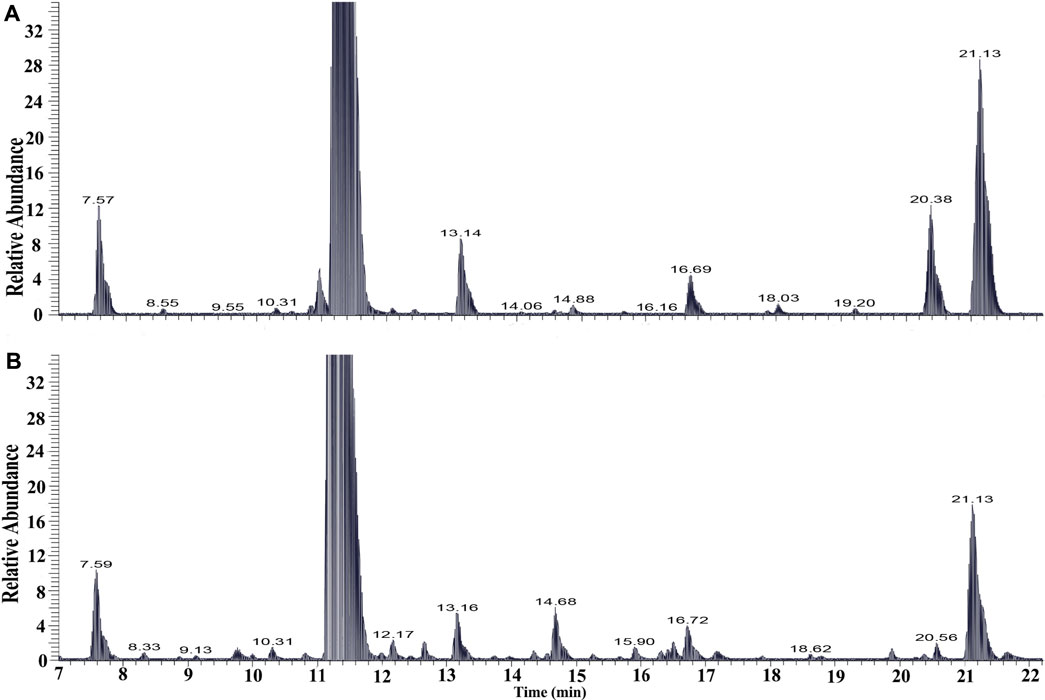
FIGURE 2. The base peak intensity chromatograms of samples raw Reynoutria multiflora Thunb. (RRM, A) and processed Reynoutria multiflora Thunb. (PRM for 24 h, B) derived from UHPLC-Q-Exactive plus orbitrap MS/MS (7–22 min).
3.2 Fragmentation Pathway of THSG and Derivatives
To identify the derivatives of THSG in the processing RM, the trans-THSG and cis-THSG standard were firstly analyzed by UPLC-Q-Exactive plus MS/MS under the above-mentioned conditions. Trans-THSG (A3-5, tR = 11.18 min) and cis-THSG (A3-2, tR = 7.58 min) had a [M-H]− ion at m/z 405.1187 with only a dominant ion at m/z 243.0654 (C14H11O4) in MS2 spectrum. These two ions could be used as a diagnostic ion for identify stilbene glycosides. Compound A3-1, A3-3 and A3-4 (tR = 6.67, 9.75 and 9.89 min) also had an [M-H]− ion at m/z 405.1187 (C20H21O9), and showed a fragment ion at m/z 243.0654 in their MS2 spectrum, indicating that they are isomers of THSG. A3-5 was identified as trans-THSG and A3-2 was cis-THSG, and A3-1 should be isomer of cis-THSG, A3-3 and A3-4 should be isomers of trans-THSG. (Figure 3).
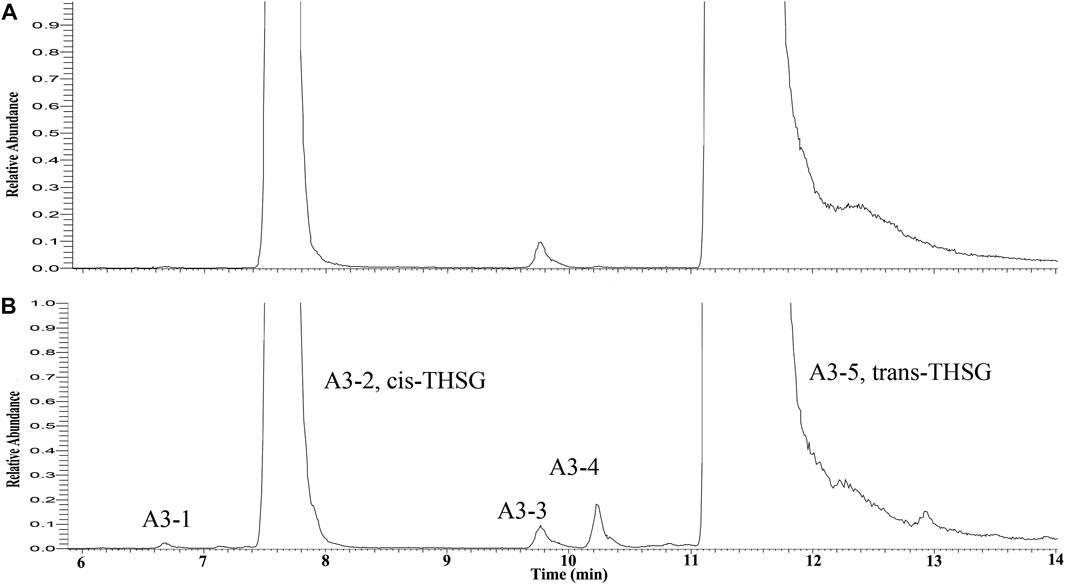
FIGURE 3. The extracted ion chromatograms of ion at m/z = 405.1187 (A: raw Reynoutria multiflora Thunb. RRM), B: processed Reynoutria multiflora Thunb. (PRM 24 h)).
3.3 Identification of Tetrahydroxystilbene-O-Hexoside Derivatives
During the processing, Maillard reaction occurred, producing a large number of compounds, including acetone alcohol, 2, 3-butanediol, succinic acid, 2, 3-dihydro-3, 5-dihydroxy-6-methyl-4H-pyranone (DDMP), 5-hydroxymethyl furfural (5-HMF) and its derivatives (Liu, 2018). Stilbene glycosides may react with products of Maillard reaction or small moleculars, such as gallic acid and catechuic acid, in high temperature and high humidity environment.
Most stilbene glycosides in RM showed common fragmentation pathways and two diagnostic fragment ions at m/z 405.1192(C20H21O9) and 243.0654 (C14H11O4). These were used for rapidly extracting and analyzing unknown stilbene glycosides. According to the structural characteristics of THSG, the linking points of stilbene derivatives with other compounds contain glycosyl hydroxyl moiety and phenyl hydroxyl moiety. According to the cleaved fragments, it can be inferred as follows: 1. if there is a fragment from loss of C6H10O5 by parent ion, the linking point should be phenyl hydroxyl moiety; 2. The cleavage fragment contains the ion at m/z 405.1187 of THSG and the ion at m/z (hexoside + substituent), and there is no fragment to loss of C6H10O5, so the linking point should be glycosyl hydroxyl moiety; 3. if the fragment is only the ion at m/z 243.0654, most of the glycosyl hydroxyl moiety may be linked, but there is also a probability that the hexoside of THSG and the substituent on the phenyl hydroxyl moiety will split at the same time, so the linking point cannot be determined in this case. We use tetrahydroxystilbene-O- (substituent)-hexoside to name them.
Compounds A1-1 and A1-2 displayed a [M-H]− ion at m/z 375.1081 (C19H19O8) and the product ion at m/z 243.0654 derived from the loss of a pentose (mostly arabinose). By comparing with literature, Compounds A1-1 and A1-2 were tentatively identified as tetrahydroxystilbene-O-pentose.
Compounds A2-1, A2-2, and A2-3 gave a [M-H]− ion at m/z 389.1242 (C20H21O8) and the product ion at m/z 243.0654 derived from the loss of a deoxyhexose (mostly rhamnose), indicated that it was a THSG derivative. Compounds A2-1, A2-2, and A2-3 were tentatively characterized as tetrahydroxystilbene-O-deoxyhexoside.
Compounds A4-1 and A4-2 displayed a high resolution [M-H]− ion at m/z 423.1295 and gave element composition of C20H23O10. The MS2 spectra gave identical ions at m/z 261.0764 (C14H13O5) and 243.0654 (C14H11O4), respectively. The loss of C6H10O5 (hexoside) and H2O to produce the deprotonated moiety ion at m/z 243.0655, indicated can be identified as stilbene derivatives, but the specific structure is not yet determined.
Compounds A5-1 ∼ A5-4 showed the same [M-H]− ion at m/z 433.1136 (C21H21O10) and the MS2 spectra gave ions at m/z 271.0608 (C15H11O5) and 243.0654 (C14H11O4). Without further information, compounds A5-1 ∼ A5-4 were tentatively characterized as tetrahydroxystilbene-O-hexoside-O-formic acid acyl (phenolic hydroxyl moiety).
Compounds A6-1 and A6-2 showed the same [M-H]− ion at m/z 437.1450 (C21H25O10) and the MS2 spectra gave ions at m/z 275.0922 (C15H15O5) and m/z 243.0655 (C14H11O4), the loss of C6H10O5 (hexoside) and CH4O to produce the deprotonated moiety ion at m/z 243.0655, allowed us to infer that they were tetrahydroxystilbene derivative, but the specific structure is not yet determined.
Compounds A7-1 ∼ A7-5 gave a [M-H]− ion at m/z 447.1300 (C22H23O10) and loss 204 Da to produce ion at m/z 243.0656 in the MS2 spectrum, which indicated that the presence of a hexose group and an acetyl. Thus, compounds A7-1∼ A7-5 were preliminarily characterized as tetrahydroxystilbene-O-(acetyl)-hexoside.
Compounds A8-1 and A8-2 showed the same [M-H]− ion at m/z 449.1086 (C21H21O11) and the MS2 spectra gave ions at m/z 287.0554 (C15H11O6) and 243.0654 (C14H11O4) form continuous loss of C6H10O5 and CO2. Thus, the carbonate acyl substituted THSG was detected and compounds A8-1 and A8-2 were identified as tetrahydroxystilbene-O-hexoside-O-carbonate acyl (phenolic hydroxyl moiety).
Compound A9 displayed a high resolution [M-H]− ion at m/z 457.1116 and gave element composition of C23H21O10, the product ion at m/z 243.0654 originated from the loss of C9H10O6 (hexoside + hydroxycyclopropenon). By investigating literature, compound A9 was preliminarily identified as tetrahydroxystilbene-O-(hydroxycyclopropenon)-hexoside. Similarly, compounds A10 and A11 were tentatively identified as tetrahydroxystilbene-O-(acrylic acid acyl)-hexoside and tetrahydroxystilbene-O-(propionyl)-hexoside, since the loss of C9H12O6 (hexoside + acrylic acid) and C9H14O6 (hexoside + propionic acid) were detected.
Compounds A12-1 and A12-2 showed the same [M-H]− ion at m/z 463.1244 (C22H23O11) and the MS2 spectra gave ion at m/z 243.0654 (C14H11O4) form loss of C8H12O7 (C6H10O5 and C2H2O2). Thus, the glycolic acid substituted THSG was detected and compounds A12-1 and A12-2 were identified as tetrahydroxystilbene-O- (glycolic acid acyl)-hexoside.
Compounds A13-1, A13-2, and A13-3 showed the same [M-H]− ion at m/z 477.1396 (C23H25O11) and the MS2 spectra gave ions at m/z 405.1184 (C20H2109), 315.0859 (C17H15O6), 297.0763 (C17H13O5) and 243.0655 (C14H11O4). The ion at m/z 477.1396 loss of C3H4O2 produce the ion at m/z 405.1184, By comparing literature, compounds A13-1, A13-2, and A13-3 were tentatively identified as tetrahydroxystilbene-O-hexoside-O-lactic acid acyl (phenolic hydroxyl moiety).
Compounds A14-1 ∼ A14-4 showed the same [M-H]− ion at m/z 489.1759 (C25H29O10) and the MS2 spectra gave identical ions at m/z 405.1176 (C20H2109), 327.1222 (C19H19O5) and 243.0656 (C14H11O4). The loss of C5H8O to produce the deprotonated THSG moiety ion at m/z 405.1176, Furthermore, the ion at m/z 327.1222 assigned as loss of C6H10O5 form the m/z 489.1759. By investigating literatures, compounds A14-1 ∼ A14-4 were identified as tetrahydroxystilbene-O-hexoside-O-valerate acyl (phenolic hydroxyl moiety).
Compound A15 displayed a high resolution [M-H]− ion at m/z 499.1241 and gave element composition of C25H23O11, the product ions at m/z 337.0704 (C19H13O6), 293.0812 (C18H13O4) and 243.0654 (C14H11O4) originated from the consecutive loss of C6H10O5 (hexoside), CO2 and C4H2 (5-hydroxyfuran-2-carbaldehyde). By investigating literature, compound A15 was preliminarily identified as tetrahydroxystilbene-O-hexoside-O-5-hydroxyfuran-2-carbaldehyde (phenolic hydroxyl moiety).
Compounds A16-1 and A16-2 showed the same [M-H]− ion at m/z 501.1393 (C25H25O11) and the MS2 spectra gave ions at m/z 339.0858, 321.0756 and 243.0654 form continuous loss of C6H10O5 (hexoside), CO2 and C4H4 (Figure 4). By investigating literature, compounds A16-1 and A16-2 were identified as tetrahydroxystilbene-O-hexoside-O-4-hydroxymethyl-5H-furan-2-one (phenolic hydroxyl moiety).
Compound A17 gave a [M-H]− ion at m/z 503.1553 (C25H27O11) and the product ions at m/z 341.1019 (C19H17O6), 297.1125 (C18H17O4) and 243.0656 (C14H11O4). By comparing literature, compound A17 was tentatively characterized as tetrahydroxystilbene-O-hexoside-O- 5-hydroxymethyl-4, 5-dihydrofuranone (phenolic hydroxyl moiety).
Compounds A18-1 ∼ A18-5 showed the same [M-H]− ion at m/z 505.1346 (C24H25O12) and in A18-1 and A18-2 MS2 spectra, gave ions at m/z 405.1178 and m/z 243.0655, in A18-3 ∼ A18-5 MS2 spectra, gave ions at m/z 343.0799 and m/z 243.0655. By comparing literature, compounds A18-1 and A18-2 were preliminarily characterized as tetrahydroxystilbene-O-(succinic acid acyl)-hexoside, and compounds A18-3 ∼ A18-5 were identified as tetrahydroxystilbene-O-hexoside-O-succinic acid acyl (phenolic hydroxyl moiety).
Compounds A19-1 and A19-2 were eluted at 11.85 and 12.00 min, and the molecular formula was C24H27O12 (m/z 507.1500). The MS2 spectra gave identical ions at m/z 345.0966 (C18H17O7), m/z 313.0709 (C17H13O6), m/z 285.0763 (C16H13O5), m/z 255.0656 (C15H11O4) and m/z 243.0654 (C14H11O4). By comparing literature, compounds A19-1 and A19-2 were identified as tetrahydroxystilbene-O-hexoside-O-dihydroxybutyrate (phenolic hydroxyl moiety).
Compound A20 gave a [M-H]− ion at m/z 511.1603 (C27H27O10) and the product ions at m/z 349.1068 (C21H17O5) and 243.0655 (C14H11O4) form continuous loss of C6H10O5 (hexoside) and C7H6O (salicyloyl). By comparing literature, compound A20 was tentatively characterized as tetrahydroxystilbene-O-hexoside-O-salicyloyl (phenolic hydroxyl moiety).
Compounds A21-1 and A21-2 showed the same [M-H]− ion at m/z 512.1555 (C26H26O10N) and the MS2 spectra gave ions at m/z 405.1175 (C20H21O9) and 243.0655 (C14H11O4). By comparing literature, compounds A21-1 and A21-2 were tentatively identified as tetrahydroxystilbene-O-(aminocatecholoyl)-hexosides. Similarly, compound A25 was tentatively identified as tetrahydroxystilbene-O-(pyroglutamyl)-hexoside.
Compounds A22-1 ∼ A22-4 showed the same [M-H]− ion at m/z 513.1497 (C26H25O11), compounds A22-1, A22-2 and A22-3 loss 162 Da to produce ion at m/z 351.0862, and then loss 108 Da (C6H4O2) to produce ion at m/z 243.0656 in the MS2 spectrum. And in compound A22-4 MS2 spectra, there was a fragment ion at m/z 243.0655, the proposal fragmentation pathway shown in Figure 5. By investigating literatures, compounds A22-1, A22-2, and A22-3 were identified as tetrahydroxystilbene-O-hexoside-O-5-HMF (phenolic hydroxyl moiety), A22-4 was identified as tetrahydroxystilbene-O-(5-HMF)-hexoside.
Compounds A23-1 and A23-2 were eluted at 15.29 and 15.69 min, and the molecular formula was C26H27O11 (m/z 515.1555). In addition, the product ions at m/z 353.1021 (C20H17O6) and m/z 243.0654 (C14H11O4) originated from the consecutive loss of hexoside (C6H10O5) and C6H6O2 (2, 5-bis-hydroxymethyl furan). By comparing literature, compounds A23-1 and A23-2 identified as tetrahydroxystilbene-O-hexoside-O-2, 5-bis-hydroxymethyl furan (phenolic hydroxyl moiety). And compound A24 displayed a high resolution [M-H]− ion at m/z 515.1179 and gave element composition of C25H23O12, the loss of C5H2O3 and C6H10O5 to produce the deprotonated moiety ion at m/z 243.0655. By investigating literatures, compound A24 was tentatively characterized as tetrahydroxystilbene-O-(5-hydroxyfuran-2-carboxylic acid)-hexoside.
Compounds A26-1 ∼ A26-4 showed the same [M-H]− ion at m/z 519.1495 (C25H27O12). In A26-1 MS2 spectra, the fragment ion at m/z 243.0655, in A26-2, A26-3 and A26-4 MS2 spectra, the fragment ions at m/z 405.1167 (C20H21O9), 357.0967 (C19H17O7), 339.0855 (C19H15O6), 297.0760 (C17H13O5) and 243.0655 (C14H11O4), by investigating literatures, compound A26-1 was identified as tetrahydroxystilbene-O-(glutaryl)-hexoside, A26-2, A26-3 and A26-4 were identified as tetrahydroxystilbene-O-hexoside-O-glutaryl (phenolic hydroxyl moiety).
Compounds A27-1 (tR = 12.84 min), A27-2 (tR = 13.07 min) and A27-3 (tR = 13.82 min) showed the same [M-H]− ion at m/z 521.1294 (C24H25O13) and the MS2 spectra gave identical ions at m/z 405.1177 (C20H21O9), 359.1115 (C19H19O7) and 243.0754 (C14H11O4). The loss of C4H4O4 to produce the deprotonated THSG moiety ion at m/z 405.1177, thus, compounds A27-1, A27-2, and A27-3 identified as tetrahydroxystilbene-O-hexoside-O-malic acid acyl (phenolic hydroxyl moiety).
Compounds A28-1 ∼ A28-5 showed the same [M-H]− ion at m/z 525.1398 (C27H25O11), the MS2 of A28-1 ∼ A28-3 spectra gave ions at m/z 525.1398, 405.1179, 363.0883, 243.0858 and 137.0228. The product ions at m/z 363.0883 originated from the loss of hexoside (C8H10O5). Thus, the salicylic acid acyl substituted THSG was detected and compounds A28-1 ∼ A28-3 identified as tetrahydroxystilbene-O-hexoside-O-salicylic acid acyl (phenolic hydroxyl moiety). The MS2 of A28-4 and A28-5 spectra gave ions 405.1179, 243.0858 and 137.0228, but there were no 363.0833 fragment ion. Thus, compounds A28-4 and A28-5 were identified as tetrahydroxystilbene-O- (salicylic acid acyl)-hexoside.
Compounds A29-1, A29-2 and A29-3 gave a [M-H]− ion at m/z 527.1190 (C26H23O12) and the MS2 spectra showed identical ions at m/z 365.0652 (C20H13O7) and 243.0659 (C14H11O4). The MS2 spectrum showed losses of C6H10O5 and C6H2O3, respectively, to produce characteristic aglycone ion at m/z 243.0659. By comparing literature, compounds A29-1, A29-2, and A29-3 were tentatively identified as tetrahydroxystilbene-O-hexoside-O-5-formylfuran-2-carboxylyl (phenolic hydroxyl moiety).
Compounds A30-1 ∼ A30 ∼ 7 showed the same [M-H]− ion at m/z 529.1345 (C26H25O12), the MS2 spectra of A30-1, A30-2 and A30-6 gave ions at m/z 367.0870 (C20H15O7), 323.0914 (C19H15O5) and 243.0651 (C14H11O4). The product ions originated from the consecutive loss of hexoside (C6H10O5), CO2 and C5H4O. In A30-3 and A30-4 spectra, gave ions at m/z 367.0807 (C20H15O7), 243.0656 (C14H11O4) and 123.0071 (C6H3O3), and in A30-5 and A30-7 spectra, gave ions at m/z 405.1176 (C20H21O9), 243.0656 (C14H11O4) and 123.0071 (C6H3O3). By investigating literatures, the substituent group of the compound was 5-hydroxymethyl-furfural. And according to the fragmentation fragments, it can be inferred that the binding sites are different (Figure 6). A30-1, A30-2, and A30-6 were tentatively identified as tetrahydroxystilbene (phenolic hydroxyl moiety)-O-5-hydroxymethylfuran-2-carboxylyl-hexosides (hydroxyl moiety), A30-3 and A30-4 were identified as tetrahydroxystilbene (phenolic hydroxyl moiety)-O-5-hydroxymethylfuran- 2-carboxylyl-hexoside (carboxyl moiety), A30-5 and A30-7 were identified as tetrahydroxystilbene-O-(5-hydroxymethylfuran-2-carboxylyl)-hexoside.
Compounds A31-1 ∼ A31-10 were eluted at 2.58, 2.75, 3.58, 4.66, 5.00 min 5.64, 12.14, 15.02, 15.36, 15.74 min, they both showed an accurate [M-H]− ion at m/z 531.1511 (C26H27O12), which were 128 Da higher than that of THSG. In their MS2 spectra, the [M-H]− ion showed fragment ions at m/z 405.1180 (C20H21O9), 369.0966 (C20H17O7), 351.0863 (C20H15O6), 319.0796 (C18H13O5), 295.0609 (C17H11O5), and 243.0655 (C14H11O4). All the MS2 of the compounds have molecular fragment m/z 369.0966, indicated that the compounds are formed by dehydration of substituents and phenolic hydroxyl groups of stilbene glycosides. By investigating literature (Liu, 2018), compounds A31-1 ∼ A31-10 were tentatively characterized as tetrahydroxystilbene-O-hexoside-DDMP (phenolic hydroxyl moiety).
Compounds A32-1 ∼ A32-5 showed the same [M-H]− ion at m/z 533.1658 (C26H29O12) and the MS2 spectra gave identical ions at m/z 371.1124 (C20H18O7), 327.0863 (C18H16O6) and 243.0657 (C14H11O4). The MS2 showed losses of C6H3O3 and C6H10O5 due to substituent and hexose moiety, respectively, to produce characteristic aglycone ion at m/z 243.0657 (C14H11O4). By comparing literature, compounds A32-1 ∼ A32-5 were tentatively characterized as tetrahydroxystilbene-O-hexoside-adipic acid acyl (phenolic hydroxyl moiety).
Compound A33-1 ad A33-2 gave a [M-H]− ion at m/z 537.1609 (C25H29O13) and the product ion at m/z 243.0655 (C14H11O4) form loss of C11H18O9 (C6H10O5 and C5H8O4). By comparing literature, compound A33-1 and A33-2 were tentatively characterized as tetrahydroxystilbene-O-(arabinoyl)-hexoside.
Compounds A34-1 ∼ A34-4 showed a [M-H]− ion at m/z 541.1353 (C27H25O12) and the MS2 spectra of A34-1 and A34-2, showed fragment ions at m/z 405.1175 (C20H21O9), 297.0610 (C13H13O8), 243.0657 (C14H11O4), 153.0179 (C7H5O4), respectively, indicated that they were THSG derivatives. The loss of C7H5O4 to produce the deprotonated THSG moiety ion at m/z 405.1175, allowed us to infer that they were protocatechuic acid substituted THSG products. And the ion at m/z 297.0610 assigned as protocatechuic acid acyl-hexoside moiety, produced protocatechuic acid ion at m/z 153.0179. A34-3 and A34-4 were eluted at 21.70 and 22.07 min, the [M-H]− ions showed fragment ions at m/z 379.0793 (C21H15O7) and 243.0657 (C14H11O4), respectively, originated from the consecutive loss of hexoside (C6H10O5) and protocatechuic acid (C7H5O4). There results indicate that protocatechuic acid substituted to the phenolic hydroxyl moiety in compounds A34-3 and A34-4. Therefore, compounds A34-1 and A34-2 were identified as tetrahydroxystilbene-O-hexoside-O-protocatechuic acid acyl (glycosyl hydroxyl moiety), and A34-3 and A34-4 were identified as tetrahydroxystilbene-O-hexoside-O-protocatechuic acid acyl (phenolic hydroxyl moiety).
Compound A35 gave a [M-H]− ion at m/z 543.1121 (C26H33O13) and the product ions at m/z 405.1196 (C20H21O9) and 243.0655 (C14H11O4) form consecutive loss of C6H12O4 and C6H10O5. By comparing literatures, compound A35 was tentatively identified as tetrahydroxystilbene-O- (furan-dicarboxylic acid acyl)-hexoside. And compound A36 gave a [M-H]− ion at m/z 543.1501 (C27H27O12) and the product ion at m/z 381.0963 (C21H17O7), 337.1069 (C20H17O5) and 243.0655 (C14H11O4) form consecutive loss of C6H10O5, CO2 and C6H6O. By investigating literatures, A36 was tentatively identified as tetrahydroxystilbene-O-hexoside-methoxymethyl-furancarboxylic acid acyl (phenolic hydroxyl moiety).
Compounds A37-1 and A37-2 showed the same [M-H]− ion at m/z 547.1453 (C26H27O13) and the MS2 spectra gave ions at m/z 385.0914 (C20H17O8) and 243.0655 (C14H11O4) from consecutive loss of C6H10O5 and C6H6O4. By comparing literature, compounds A37-1 and A37-2 were tentatively identified as tetrahydroxystilbene-O-hexoside-oxoadipic acid acyl (phenolic hydroxyl moiety). Similarly, compounds A38-1, A38-2 and A38-3 were tentatively identified as tetrahydroxystilbene-O-hexoside-hydroxyadipic acid acyl (phenolic hydroxyl moiety), since the ions at m/z 387.1066 (C20H19O8) and 243.0655 (C14H11O4) from consecutive loss of C6H10O5 and C6H8O4.
Compounds A39-1 ∼ A39-5 displayed a high resolution [M-H]− ion at m/z 551.1556 and gave element composition of C29H27O11. In A39-2 ∼ A39-5 MS2 spectra, the [M-H]− showed fragment ions at m/z 405.1180 (C20H21O9), 243.0656 (C14H11O4), 163.0397(C9H7O3) and 145.0280 (C9H5O2). The product ions at m/z 405.1180 and 234.0656 originated from the consecutive loss of p-hydroxycinnamoyl (C9H6O2) and hexoside (C6H10O5), Thus, p-hydroxycinnamoyl substituted THSG was detected and compounds A39-2 ∼ A39-5 were identified as tetrahydroxystilbene-O-(p-hydroxycinnamoyl)-hexoside. In A39-1 MS2 spectra, the [M-H]− showed fragment ions at 399.1018 (C23H17O6, M-C6H10O5), 243.0655, 163.0396 and 145.0279, indicated p-hydroxycinnamoyl was linked to phenolic hydroxyl moiety. Thus, compound A39-1 was identified as tetrahydroxystilbene-O-hexoside-p-hydroxycinnamoyl (phenolic hydroxyl moiety).
Compounds A40-1 ∼ A40-8 showed the same [M-H]− ion at m/z 557.1295 (C27H25O13) and the MS2 spectra gave identical ions at m/z 405.1179 (C20H21O9), 313.0555 (C13H13O9), 243.0654 (C14H11O4) and 169.0127 (C7H5O5). The loss of C6H10O5 and C7H4O4 to produce the deprotonated resveratrol moiety ion at m/z 243.0654, a galloyl group was present, the ion at 313 ([galloylglucose—H]) appeared as base peak in the MS2 spectra. It could further fragment ion m/z 169 ([gallic acid – H]−). Therefore, compounds A40-1 ∼ A40-8 were identified as tetrahydroxystilbene-O-hexoside-O-galloyl (glycosyl hydroxyl moiety) (Qiu et al., 2013).
Compounds 41-1 and 41-2 showed the same [M-H]− ion at m/z 561.1609 (C27H29O13) and the MS2 spectra gave identical ions at m/z 405.1174 (C20H21O9) and 243.0654 (C14H11O4). By comparing literatures, compounds 41-1 and 41-2 were identified as tetrahydroxystilbene-O- (gabosine C)-hexoside.
Compounds A 42-1 ∼ A 42-13 gave precursor ion [M-H]− at m/z 567.1712 (C26H31O14), in A42-2 ∼ A42-4 and A42-7 ∼ A42-13 MS2 spectra, the [M-H]− ion showed fragment ion at m/z 243.0654 (C14H11O4), indicated that the consecutive neutral loss of hexoside, they were disaccharide THSGs. By comparing literature, they were tentatively characterized as tetrahydroxystilbene-O-di-hexosides (Figure 7). But in A42-1, A42-5, and A42-6 MS2 spectra, the [M-H]− ion showed fragment ions at m/z 477.1401 (C23H25O11), 447.1278 (C22H23O10), 315.0857 (C17H15O6), 285.0761 (C16H13O5), and 243.0655 (C14H11O4), respectively. The fragment ions 477.1401 [M-H-90 Da, C3H6O3]−, 447.1278 [M-H-120 Da, C4H8O4]− are diagnostic the last two neutral loss fragments suggested that a C-glycoside was connected with the stilbene glycoside. Therefore, A42-1, A42-5 and A42-6 were determined as tetrahydroxystilbene-O-hexoside-C-glycoside.
A42-14 (tR = 18.29 min), A42-15 (tR = 19.50 min) and A42-16 (tR = 21.64 min) showed the molecular formula were C29H27H12 (m/z = 567.1504), which was 162 Da heavier than that of THSG, and different from A42-1 ∼ A42-13. In their MS2 spectra, the [M-H]− ion showed fragment ions at m/z 405.1203 (C20H21O9), 243.0654 (C14H11O4), 161.0229 (C9H5O3), respectively. Indicated that the consecutive loss of caffeoyl (C9H6O3) and hexoside (C6H10O5). Thus, the caffeoyl substituted THSG was detected and compound A42-14, A42-15 and A42-16 identified as tetrahydroxystilbene-O-(caffeoyl)-hexoside.
Compounds A43-1 ∼ A43-6 showed the same [M-H]− ion at m/z 573.1251 (C27H25O14) and the MS2 spectra gave identical ions at m/z 243.0657 (C14H11O4), 166.9971 (C7H3O5) and 123.0071 (C6H3O3). By comparing literature, Compounds A43-1 ∼ A43-6 were tentatively identified as tetrahydroxystilbene-O- (tetrahydroxybenzoic acid acyl)-hexoside.
Compound A44 gave a [M-H]− ion at m/z 575.1402 (C27H27O14) and the product ions at m/z 337.0707 (C19H13O6) and 244.0655 (C14H11O4). By comparing literatures, compound A44 was tentatively identified as tetrahydroxystilbene-O- (dioxoheptane-dicarboxylic acid acyl)-hexoside.
Compounds A45-1 ∼ A45-5 showed the same [M-H]− ion at m/z 581.1655 (C30H29O12), the MS2 spectra gave ions at m/z 405.1177 (C20H21O9), 337.0921(C16H17O8), 243.0655(C14H11O4), 193.0493 (C10H9O4) and 175.0387 (C10H7O3). Its MS2 spectrum gave characteristic ions at m/z 337.0921 ([feruoylglucose-H]−), m/z 193.0493 ([ferulic acid-H]−) and m/z 175.0387, therefore, compounds A45-1 ∼ A45-5 were identified as tetrahydroxystilbene-O-hexoside-O-feruloyl (glycosyl hydroxyl moiety).
Compound A46 gave a [M-H]− ion at m/z 591.2075 (C29H35O13) and the product ions at m/z 405.1203 (C20H21O9), 243.0655 (C14H11O4) and 185.0806 (C9H13O4). By comparing literature, compound A46 was tentatively identified as tetrahydroxystilbene-O- (hydroxynonanedioic acid acyl)-hexoside.
Compound A47 gave a [M-H]− ion at m/z 613.1769 (C27H33O16) and the product ions at m/z 405.1203 (C20H21O9) and 243.0655 (C14H11O4). By comparing literatures, compound A47 was tentatively identified as tetrahydroxystilbene-O-(Glucoheptanoyl)-hexoside.
Compounds A48-1, A48-2 and A48-3 showed the same [M-H]− ion at m/z 719.1825 (C33H35O18) and the MS2 spectra gave identical ions at m/z 557.1287 (C27H25O13), 405.1174 (C20H21O9), 313.0557 (C13H13O9), 243.0655 (C14H11O4) and 169.0126 (C7H5O5), the loss of C6H10O5 to produce compound A39 ion at m/z 557.1287, and the fragmentation ions were the same. Therefore, A48-1, A48-2 and A48-3 were identified as tetrahydroxystilbene-O-dihexoside -galloyl (glycosyl hydroxyl moiety).
Compounds A49-1 ∼ A49-6 displayed a high resolution [M-H]− ion at m/z 827.2399 and gave element composition of C40H43O19. The MS2 spectra gave ions at 405.1165 (C20H21O9), 259.0607 (C14H11O5) and 243.0656 (C14H11O4), forming the ions 405.1165 and 243.0656 indicated that they should be THSG derivatives. By comparing literature, compounds A49-1 ∼ A49-6 were tentatively identified as polygonumoside C/D.
Compounds A50-1 ∼ A50-8 displayed a high resolution [M-H]− ion at m/z 837.2601 and gave element composition of C42H45O18. The MS2 spectra gave ions at m/z 675.2068 (C36H35O13), 513.1556 (C30H25O8), 431.1342 (C22H23O9), 405.1165 (C20H21O9), 269.0813 (C16H13O4) and 243.0656 (C14H11O4). The consecutive neutral loss of hexoside, forming ions at m/z 675.2068 and 513.1556, and forming the ions 405.1165, 243.0656 and 431.1342, 269.0813 indicated that was cleavage into two glycosides. By comparing literature, compounds A50-1 ∼ A50-8 were identified as polygonumnolide D (Figure 8). Similarly, compound A52 was tentatively characterized as hydroxylation polygonumnolide D, since the [M-H]− ion at m/z 853.2560 (C42H45O19), which was 16 Da (O) higher than that of A50, and the MS2 spectra gave ions at m/z 447.1300 (C22H23O10), 405.1175 (C20H21O9), 285.0765 (C16H13O5) and 243.0656 (C14H11O4).
Compounds A51-1 ∼ A51-5 showed the same [M-H]− ion at m/z 841.2551 (C41H45O19), which was 14 Da (CH2) higher than that of A49. The MS2 spectra gave ions at m/z 405.1182 (C20H21O9), 273.0764(C15H13O5) and 243.0655(C14H11O4), and the ion m/z 273.0764 was 14 Da (CH2) higher than that of A49 ion m/z 259.0607. therefore, compounds A51-1 ∼ A51-5 were identified as methylation polygonumoside C/D. Similarly, compounds A53-1 ∼ A53-4 were identified as hydroxylation methylation polygonumoside C/D. since the [M-H]− ions at m/z 857.2502 (C41H45O20), which was 14 Da (CH2) higher than that of A51, and the MS2 spectra gave ions at m/z 405.1172 (C20H21O9), 289.0709 (C15H13O6) and 243.0656 (C14H11O4).
3.4 Identification of Trihydroxystilbene-O-Hexoside Derivatives
Compounds B1-1, B1-2 and B1-3 gave a [M-H]− ion at m/z 389.1240 (C20H21O8) and the product ion at m/z 227.0702 (C14H11O3). The loss of C6H10O5 was confirmed by MS2 spectra and indicated a hexose neutral loss. Compared with the control substance, B1-2 was identified as polydatin, compounds B1-1 and B1-3 were identified as isomer polydatin.
Compounds B2-1 ∼ B2-4 showed the same [M-H]− ion at m/z 541.1345 (C27H25O12) and the MS2 spectra gave ions at m/z 313.0559 (C13H13O9), 227.0702 (C14H11O3), 169.0128 (C7H5O5). Similar with compounds A40, compounds B2-1 ∼ B2-4 were identified as trihydroxystilbene-O-hexoside-O-galloyl (glycosyl hydroxyl moiety).
Compounds B3-1 and B3-2 showed the same [M-H]− ion at m/z 457.1136 (C23H21O10) and the MS2 spectra gave ions at m/z 295.0605 (C17H11O5) and 227.0702 (C14H11O3). By comparing literature, compounds B3-1 and B3-2 were identified as trihydroxystilbene-O-hexoside-O-acid deltique acyl (phenolic hydroxyl moiety).
Compound B4 gave a [M-H]− ion at m/z 535.1816 (C26H31O12) and the product ion at m/z 227.0702 (C14H11O3) derived from the loss of C6H10O5 (hexoside) and C6H10O4 (deoxyhexose, mostly rhamnose). By investigating literatures, compound B4 was identified as trihydroxystilbene-(deoxyhexose)-O-hexoside.
Compound B5 displayed a high resolution [M-H]− ion at m/z 359.1132 and gave element composition of C19H19O7, the product ions at m/z 359.1129 (C19H19O7) and 227.0701 (C14H11O3). The product ion at m/z 227.0701 originated from the loss of pentose (mostly arabinose). Therefore, compound B5 was identified as trihydroxystilbene-O-pentose.
3.5 Identification of Pentahydroxystilbene Glycoside Derivatives
Compounds C1-1 ∼ C1-7 displayed a high resolution [M-H]− ion at m/z 421.1138, and gave element composition of C20H21O10, which was 14 Da (CH2) higher than that of THSG, the MS2 spectra gave ion at 259.0609 (C14H11O5). By comparing literature, compounds C1-1 ∼ C1-7 were tentatively identified as pentahydroxystilbene glycosides.
Compound C2 gave a [M-H]− ion at m/z 545.1291 (C26H25O13) and the product ions at m/z 421.1128 (C20H21O10), 259 (C14H11O5) and 123.0070 (C6H3O3) derived from the loss of C6H5O5 (5-HMF) and C16H10O5 (hexoside). By investigating literatures, compound C2 was identified as pentahydroxystilbene-(5-HMF)-O-hexoside.
3.6 Identification of Tetrahydroxy-Phenanthrene-O-Hexoside Derivatives
Compounds D1-1 and D1-2 gave a [M-H]− ion at m/z 403.1030 (C20H19O9) and prominent fragment ion at m/z 241.0497 (C14H9O4) in MS2 spectrum, which were showed 2 Da less than that of THSG. It can be inferred that they were dehydrogenated product of THSG. By comparing literatures (Qiu et al., 2013), compounds D1-1 and D1-2 were identified as tetrahydroxy-phenanthrene-O-hexoside.
Compounds D2-1 ∼ D2-8 showed the same [M-H]− ion at m/z 549.1605 (C26H29O13) and the MS2 spectra gave ions at m/z 387.1072 (C20H19O8), 297.0760 (C17H13O5) and 241.0497 (C14H9O4). Similarly compounds A38, compounds D2-1 ∼ D2-8 were identified as tetrahydroxy-phenanthrene-O-hexoside-O-p-hydroxycinnamoyl (phenolic hydroxyl moiety).
3.7 Identification of Dihydrotetrahydroxystilbene-O-Hexoside Derivatives
Compound E1 gave a [M-H]− ion at m/z 407.1343 (C20H23O9) and prominent fragment ion at m/z 245.0811 (C14H13O4) in MS2 spectrum, which were showed 2 Da higher than that of THSG. It can be inferred that they were dihydrogenated product of THSG. By comparing literatures, compounds E1 was identified as dihydrotetrahydroxystilbene-O-hexoside.
Compounds E2-1 and E2-2 showed the same [M-H]− ion at m/z 527.1552 (C27H27O11) and the MS2 spectra gave ions at m/z 365.1017 (C21H17O6), 335.0918 (C20H15O5) and 245.0814 (C14H13O4). Similarly compounds A27, compounds E2-1 and E2-2 were identified as dihydrotetrahydroxystilbene-O-hexoside-salicylic acid acyl (phenolic hydroxyl moiety).
Compound E3 displayed a high resolution [M-H]− ion at m/z 539.1766, and gave element composition of C25H31O13. The MS2 spectra gave ion at 245.0811 (C14H13O4) derived from the loss of C6H10O5 (hexoside) and C5H8O4 (pentose, mostly arabinose). Compound E3 was identified as dihydrotetrahydroxystilbene-O-(pentose)-hexoside.
3.8 Identification of Pentahydroxy-Phenanthrene-O-Hexoside
Compounds F1-1 and F1-2 showed the same [M-H]− ion at m/z 419.0980 (C20H19O10) and the MS2 spectra gave ion at m/z 257.0542 (C14H9O5), which were showed 16 Da higher than that of compounds D1. Therefore, compounds F1-1 and F1-2 were identified as pentahydroxy-phenanthrene-O-hexosides.
3.9 Identification of Dihydroxystilbene-O-Hexoside Derivatives
Compound G1 gave a [M-H]− ion at m/z 373.1286 (C20H21O7) and prominent fragment ion at m/z 211.0751 (C14H13O2) in MS2 spectrum, which were showed 32 Da less than that of THSG. It can be inferred that they were dedihydroxylation product of THSG. Therefore, compounds G1 was identified as dihydroxystilbene-O-hexoside.
Compounds G2-1 and G2-2 showed the same [M-H]− ion at m/z 525.1396 (C27H25O11) and the fragment ions at m/z 525.1392, 313.0558 (C13H13O9), 211.0751 (C14H13O2), 169.0128 (C7H5O5) and 151.0020 (C7H3O4) in MS2 spectra. Similar with compounds A39 and compounds B2, compounds G2-1 and G2-2 were identified as dihydroxystilbene-O-hexoside-O-galloyl (glycosyl hydroxyl moiety).
3.10 Structural Changes of Stilbene Glycosides
3.10.1 Structural Changes of Parent Stilbene Glycosides
Stilbenes are regared to be derived from phenylalanine metabolism in plants (Isvett et al., 2009) (Figure 9). THSG is the highest and most reported compound in RM Studies have shown that resveratrol was the intermediate product of THSG, which was hydroxylated to form terahydroxystilbene, and then glycosylated to form THSG (compounds A3) (Zhao, 2017). Polydatin (compound B1-2) was glycosylated form resveratrol. Pentahydroxystilben-O-hexoside (compounds C1) was synthesized by rehydroxylation of tetrahydroxystilbene and then glycosylation. The phenanthrene moiety was formed by cyclization of 6-H and 2′-H positions of tetrahydroxystilbene, which can stabilize the cyclization of aglycone, and then glycosylated to form tetrahydroxy-phenanthrene-O-hexoside (compounds D1). The tetrahydroxy-phenanthrene was hydroxylated to form pentahydroxy-phenanthrene, then glycosylated to form pentahydroxy-phenanthrene-O-hexoside (compounds F1). Dihydrotetrahydroxystilbene-O-hexoside (compounds E1) was synthesized from tetrahydroxystilbene by double bond opening and then glycosylated. The resveratrol lost a hydroxyl group in the plant, and dihydroxystilbene-O-hexoside (G1) was obtained by the glycosylation of dihydroxystilbene (Figure 10).
3.11 Structural Changes in Stilbene Glycosides Substituents
Compounds containing malonyl, acetyl, caffeoyl and other aromatic acyls are very common in various plants (Barnes et al., 1994; Klejdus et al., 2001; Ye et al., 2007). And galloyl, malonyl, acetyl, caffeoyl, coumaroyl, feruloyl substituent have been reported in raw PM in our previous research (Qiu et al., 2013). In total, fifty-five substituents were found in this study. Except for the above-mentioned substituents, there was also some organic acids existed, such as formic acid, acetic acid, carbonic acid, propionic acid, glycolic acid, lactic acid, valeric acid, succinic acid, dihydroxy butyric acid, salicylic acid, glutaric acid, malic acid, catechuic acid and p-hydroxybenzoic acid. Maillard reaction products, mainly includes 2, 3-dihydro-3, 5-dihydroxy-6-methyl-4H-pyranone (DDMP, A31) and 5-hydromethylfurfura (5-HMF, A22) and its derivatives from glucose or glycine were also observed as stilbene glycosides substituents (Perez Locas and Yaylayan, 2008; Harishchandra et al., 2011; Liu, 2018). The aldehyde end of 5-HMF is oxidized to carboxylic acid, 5-hydroxymethylfuran-2-carboxyl acid (5-HMF-2-CC, A30) was produced, then methylation and oxidation produced methoxymethyl-furancarboxylic acid (MM-FBA, A36) and furan-dicarboxylic acid (FDA, A35). 2, 5-bis-(hydroxymethyl) furan (2, 5-BHMF, A23) was synthesized by the reduction of 5-HMF. 5-formylfuran-2-carboxyl acid (5-FF-2CC, A29) was synthesized by the oxidation of the hydroxyl end of 5-HMF. Then decarboxylation and reoxidation were carried out to form 5-hydroxyfuran-2-carbaldehyde (5HF-2CA, A15), then, 5-hydroxyfuran-2-carboxylic acid (5-HF-2CC, A24) was formed by oxidation of aldehyde end. 4-hydroxymethyl-5h-furan-2-one (4-HM-5H-2O, A16) was synthesized from 2, 4-dihydroxy-5- (hydroxymethyl) oxolane-2-carbaldehyde by dehydration and CH2O removal, then hydrogenation reaction was reacted to produce 5-hydroxymethyl-4,5-dihydrofuranone (5-HM-4, 5-DF, A17), and the change path of 5-HMF is shown in Figure 11) all substituents information is shown in Supplementary Table S1.
3.12 Content Change Trend of Stilbene Derivatives
The peak areas of all compounds were based on the extracted ion chromatographic peaks. The mean and SD values were calculated and the column diagram of each compound was drawn (Supplementary Table S1). A total of 219 compounds were identified in this study, 73 compounds were not found in RRM, 21 compounds were not found in 4 h PRM, 9 compounds were not found in 8 h PRM and 1 compound was not found in 12 h PRM. During the process of PM, THSG dehydrated with other small molecules to form new compounds.
The change trend of the highest content compound trans-THSG and its cis-THSG slightly increased before 8 h, then decreased gradually, and was lower than that of RRM at 24 h. This is a model of content change, but the peak time may be 4, 8, 12 or 18 h. The second model, such as compound A3-4 (isomer trans-THSG), gradually increase during the processing time. The third model, such as compound A45-1 (tetrahydroxystilbene-O-hexoside-feruloyl (glycosyl hydroxyl moiety), gradually decreased with processing time.
The results showed that the content and quantity of stilbene glycoside compounds have undergone tremendous changes during the processing process. Although the content of THSG in PRM is indeed lower than that in RRM, a large number of stilbene glycoside derivatives are produced in the processing process, so the total content of stilbene glycoside compounds in the PRM will not be reduced. Conventional understanding, RRM after processing can enhance efficiency and reduce toxicity, and the content of THSG also decreases with the processing time, is THSG toxic? After this experimental study, it can be proved that THSG should not be a toxic component, because its derivatives will metabolize into compounds similar to THSG in vivo, enhancing the efficacy of THSG.
4 Conclusion
In the present study, a simple and effective method was developed for characterization of stilbene compounds in the roots of RRM and PRMs by UHPLC-Q-Exactive plus orbitrap MS/MS. Stilbene glycosides were distinguished by diagnostic fragment ions at m/z 405.1087 and 243.0656, accurate mass measurements and fragmentation pathways. Based on the proposed strategy, the metabolic process of 7 stilbene glycosides in plants was identified, and 55 substituent and Maillard reaction process were identified. Finally, 219 stilbene glycosides derivatives were identified, of which 102 compounds may be potential new compounds. The 55 substituents include monosaccharide, disaccharide, organic acid and Maillard reaction products (DDMP, 5-HMF and its derivatives) and so on. The quality and quantity of stilbene glycosides changed during the processing of RM. 73 compounds were not found in RRM, 21 compounds were not found in 4 h PRM, 9 compounds were not found in 8 h PRM and 1 compound was not found in 12 h PRM, and the change trend of the compounds can be summarized into 3 models: gradually increased, gradually decreased, first increased and then decreased. 181 trans-THSG derivative products were obtained through the hydrolysis and dehydration reaction between trans-THSG and small molecules compounds, after this experimental study, it can be proved that THSG should not be a toxic component, because its derivatives will metabolize into compounds similar to THSG in vivo, enhancing the efficacy of THSG.
Data Availability Statement
The raw data supporting the conclusion of this article will be made available by the authors, without undue reservation.
Author Contributions
XQ and ZH conceived and designed the experiments; JB, WC, and HS preformed the experiments and analyzed the data; JH, WX, and JZ contributed reagents/materials/analysis tools; JB wrote the paper.
Funding
National Natural Science Foundations of China 81373967 XQ Guangdong Provincial Hospital of Chinese Medicine Special Fund YN2019QJ14 JH GuangDong Basic and Applied Basic Research Foundation 2020A1515110383 JH Science and Technology Planning Project of Guangdong Province 2017B030314166 XQ the special foundation of Guangzhou Key Laboratory XQ, ZH.
Conflict of Interest
The authors declare that the research was conducted in the absence of any commercial or financial relationships that could be construed as a potential conflict of interest.
Publisher’s Note
All claims expressed in this article are solely those of the authors and do not necessarily represent those of their affiliated organizations, or those of the publisher, the editors and the reviewers. Any product that may be evaluated in this article, or claim that may be made by its manufacturer, is not guaranteed or endorsed by the publisher.
Supplementary Material
The Supplementary Material for this article can be found online at: https://www.frontiersin.org/articles/10.3389/fphar.2022.757490/full#supplementary-material
Abbreviations
DDMP, 2, 3-dihydro-3, 5-dihydroxy-6-methyl-4H-pyranone; 5-HMF, 5-hydromethylfurfura; RM, Reynoutria multiflora Thunb; PRM, processed Reynoutria multiflora; RRM, raw Reynoutria multiflora; TCM, Traditional Chinese Medicine; Trans-THSG, tran-2, 3, 5, 4′-tetrahydroxystilbene-2-O-β-D-glucopyranoside; UHPLC-Q-Exactive plus orbitrap MS/MS, ultra high performance liquid chromatography tandem quadrupole/electrostatic field orbitrap high-resolution mass spectrometry.
References
Barnes, S., Kirk, M., and Coward, L. (1994). Isoflavones and Their Conjugates in Soy Foods: Extraction Conditions and Analysis by HPLC-Mass Spectrometry. J. Agric. Food Chem. 42, 2466–2474. doi:10.1021/jf00047a019
Chen, Q. T., Zhuo, L. H., Xu, W., Huang, Z. H., and Qiu, X. H. (2012). Content Changes of 5 Components in Polygonum Multiflorum during Processing. Chin. J. Exp. Tradit. Med. Form. 18, 66–71. doi:10.13422/j.cnki.syfjx.2012.05.028
Cheung, F. W., Leung, A. W., Liu, W. K., and Che, C. T. (2014). Tyrosinase Inhibitory Activity of a Glucosylated Hydroxystilbene in Mouse Melan-A Melanocytes. J. Nat. Prod. 77, 1270–1274. doi:10.1021/np4008798
Chinese Pharmacopoeia Commission (2020). Pharmacopoeia of the People’s Republic of China, 184. Beijing: China Medical Science and Technology Publishing House.
Choi, S. G., Kim, J., Sung, N. D., Son, K. H., Cheon, H. G., Kim, K. R., et al. (2007). Anthraquinones, Cdc25B Phosphatase Inhibitors, Isolated from the Roots of Polygonum Multiflorum Thunb. Nat. Prod. Res. 21, 487–493. doi:10.1080/14786410601012265
Dong, H., Slain, D., Cheng, J. C., Alma, W. H., and Liang, W. F. (2014). Eighteen Cases of Liver Injury Following Ingestion of Polygonum Multiflorum. Complement. Ther. Med. 22, 70–74. doi:10.1016/j.ctim.2013.12.008
Fu, W. H. (2011). HPLC Determination of the Main Chemical Ingredients Content in Polygonum Multiflorum during the Processing. Asia-pacific Tradit. Medic. 9, 50–51. doi:10.3969/j.issn.1673-2197.2013.07.021
Harishchandra, J., Christian, M. P., Theis, S., and Mikael, B. (2011). 3-Deoxy-Glucosone Is an Intermediate in the Formation of Furfurals from D-Glucose. Chem. Sus. Chem. 4, 1049–1051. doi:10.1002/cssc.201100249
Isvett, J., Flores, S., and Robert, V. (2009). Plant Polyketide Synthases: A Fascinating Group of Enzymes. Plant Physiol. Biochem 47, 167–174. doi:10.1016/j.plaphy.2008.11.005
Klejdus, B., Vitamvásová-Štěrbová, D., and Kubáň, V. (2001). Identification of Isoflavone Conjugates in Red clover (Trifolium Pratense) by Liquid Chromatography-Mass Spectrometry after Two-Dimensional Solid-phase Extraction. Analytica Chim. Acta 450, 81–97. doi:10.1016/S0003-2670(01)01370-8
Lei, X., Chen, J., Ren, J., Li, Y., Zhai, J., Mu, W., et al. (2015). Liver Damage Associated with Polygonum Multiflorum Thunb.: a Systematic Review of Case Reports and Case Series. Evid. Based Complement. Alternat. Med. 2015, 459749. doi:10.1155/2015/459749
Li, C., Niu, M., Bai, Z., Zhang, C., Zhao, Y., Li, R., et al. (2017). Screening for Main Components Associated with the Idiosyncratic Hepatotoxicity of a Tonic Herb, Polygonum Multiflorum. Front. Med. 11, 253–265. doi:10.1007/s11684-017-0508-9
Lin, L., Ni, B., Lin, H., Zhang, M., Li, X., Yin, X., et al. (2015). Traditional Usages, Botany, Phytochemistry, Pharmacology and Toxicology of Polygonum Multiflorum Thunb.: a Review. J. Ethnopharmacol. 159, 158–183. doi:10.1016/j.jep.2014.11.009
Lin, X. N., Chen, P. Y., Zhang, Q. F., Zhao, W. H., Cai, Y. K., and Mo, J. L. (2018). The Analyses on the Preparation Process and GMP of Polygonum Multiflorum. Guangdong Chem. Industry 15, 93–95. doi:10.3969/j.issn.1007-1865.2018.15.041
Liu, Z. L. (2018). Changes in Chemical Components of Root of Polygonum Multiflorum Thunb. And How Maillard Reaction Involves during the Heating Process. Beijing: China Academy of Chinese Medical Sciences.
Lv, L., Gu, X., Tang, J., and Ho, C. (2007). Antioxidant Activity of Stilbene Glycoside from Polygonum Multiflorum Thunb In Vivo. Food Chem. 104, 1678–1681. doi:10.1016/j.foodchem.2007.03.022
Perez Locas, C., and Yaylayan, V. A. (2008). Isotope Labeling Studies on the Formation of 5-(hydroxymethyl)-2-Furaldehyde (HMF) from Sucrose by Pyrolysis-GC/MS. J. Agric. Food Chem. 56, 6717–6723. doi:10.1021/jf8010245
Qiu, X. H., Li, J. H., and Huang, Z. H. (2006). Content Comparative Analysis of Water Soluble Saccharides in Radix Polygoni Multiflora before and after its Processing. J. China. Pharm. 17, 954–956. CNKI.SUN.ZGYA.0.2006-12-039.
Qiu, X. H., Song, Y. G., Sun, J. B., and Min, J. (2008). Effect of Radix Polygoni Multifori Praeparata by Different Processing on Model of Blood Deficiency Rats. Zhong Yao Cai 31, 14–17. doi:10.13863/j.issn1001-4454.2008.01.009
Qiu, X., Zhang, J., Huang, Z., Zhu, D., and Xu, W. (2013). Profiling of Phenolic Constituents in Polygonum Multiflorum Thunb. By Combination of Ultra-high-pressure Liquid Chromatography with Linear Ion Trap-Orbitrap Mass Spectrometry. J. Chromatogr. A. 1292, 121–131. doi:10.1016/j.chroma.2012.11.051
Shao, L., Zhao, S. J., Cui, T. B., Liu, Z. Y., and Zhao, W. (2012). 2,3,5,4'- Tetrahydroxystilbene-2-O-β-D-Glycoside Biosynthesis by Suspension Cells Cultures of Polygonum Multiflorum Thunb and Production Enhancement by Methyl Jasmonate and Salicylic Acid. Molecules 17, 2240–2247. doi:10.3390/molecules17022240
Sun, Y. N., Li, W., Kim, J. H., Yan, X. T., Kim, J. E., Yang, S. Y., et al. (2015). Chemical Constituents from the Root of Polygonum Multiflorum and Their Soluble Epoxide Hydrolase Inhibitory Activity. Arch. Pharm. Res. 38, 998–1004. doi:10.1007/s12272-014-0520-4
Xu, W., Zhang, J., Huang, Z., and Qiu, X. (2012). Identification of New Dianthrone Glycosides from Polygonum Multiflorum Thunb. Using High-Performance Liquid Chromatography Coupled with LTQ-Orbitrap Mass Spectrometry Detection: A Strategy for the Rapid Detection of New Low Abundant Metabolites from Traditional Chinese Medicines. Anal. Methods 4, 1806–1812. doi:10.1039/c2ay00009a
Yang, J. B., Ye, F., Tian, J. Y., Song, Y. F., Gao, H. Y., Liu, Y., et al. (2020). Multiflorumisides HK, Stilbene Glucosides Isolated from Polygonum Multiflorum and Their In Vitro PTP1B Inhibitory Activities. Fitoterapia 146, 104703. doi:10.1016/j.fitote.2020.104703
Ye, M., Han, J., Chen, H., Zheng, J., and Guo, D. (2007). Analysis of Phenolic Compounds in Rhubarbs Using Liquid Chromatography Coupled with Electrospray Ionization Mass Spectrometry. J. Am. Soc. Mass. Spectrom. 18, 82–91. doi:10.1016/j.jasms.2006.08.009
Zhang, G. P., Zhang, H. J., Chen, T. F., Hou, H. P., Su, P., Gao, Y. H., et al. (2019). Screening and Identifying Hepatotoxic Components in Polygoni Multiflori Radix and Polygoni Multiflori Radix Praeparata. World J. Tradit. Chin. Med. 3, 164–170. doi:10.4103/wjtcm.wjtcm_29_19
Keywords: Reynoutria multiflora, stilbene glycosides, processed, UHPLC-Q-Exactive plus orbitrap MS/MS, structural and content changes
Citation: Bai J, Chen W, Huang J, Su H, Zhang D, Xu W, Zhang J, Huang Z and Qiu X (2022) Transformation of Stilbene Glucosides From Reynoutria multiflora During Processing. Front. Pharmacol. 13:757490. doi: 10.3389/fphar.2022.757490
Received: 12 August 2021; Accepted: 11 March 2022;
Published: 25 April 2022.
Edited by:
Gokhan Zengin, Selcuk University, TurkeyReviewed by:
Xiaoxiao Huang, Shenyang Pharmaceutical University, ChinaSengul Uysal, Erciyes University, Turkey
Ying-Yong Zhao, Northwest University, China
Copyright © 2022 Bai, Chen, Huang, Su, Zhang, Xu, Zhang, Huang and Qiu. This is an open-access article distributed under the terms of the Creative Commons Attribution License (CC BY). The use, distribution or reproduction in other forums is permitted, provided the original author(s) and the copyright owner(s) are credited and that the original publication in this journal is cited, in accordance with accepted academic practice. No use, distribution or reproduction is permitted which does not comply with these terms.
*Correspondence: Zhihai Huang, zhhuang7308@163.com; Xiaohui Qiu, qiuxiaohui@gzucm.edu.cn