- 1Central Laboratory, Hainan General Hospital, Hainan Affiliated Hospital of Hainan Medical University, Haikou, China
- 2Department of Pharmacy, Hainan General Hospital, Hainan Affiliated Hospital of Hainan Medical University, Haikou, China
Introduction: Venlafaxine is one of the most commonly used anti-depressant and antineoplastic drug. Previous studies have predicted venlafaxine as an anti-cancer compound, but the therapeutic effects of venlafaxine in melanoma have not yet been demonstrated. Nur77 is an orphan nuclear receptor that highly expressed in melanoma cells and can interact with Bcl-2 to convert Bcl-2 from an antiapoptotic to a pro-apoptotic protein.
Method: We examined the effects of venlafaxine in MV3 cells in vitro and MV3 xenograft tumor in nude mice. Western-blot, PCR, TUNEL assay and immunofluorescence were used to reveal the growth of melanoma cells.
Results: Here, our data revealed that venlafaxine could reduce the growth, and induce apoptosis of melanoma cells through a Nur77-dependent way. Our results also showed that treatment with venlafaxine (20 mg/kg, i.p.) potently inhibited the growth of melanoma cells in nude mice. Mechanistically, venlafaxine activated JNK1/2 signaling, induced Nur77 expressions and mitochondrial localization, thereby promoting apoptosis of melanoma cells. Knockdown of Nur77 and JNK1/2, or inhibition of JNK1/2 signaling with its inhibitor SP600125 attenuated the anti-cancer effects of venlafaxine.
Conclusion: In summary, our results suggested venlafaxine as a potential therapy for melanoma.
1 Introduction
Melanoma is the leading cause of skin cancer death worldwide (Cullen et al., 2020). Currently, BRAF inhibitor vemurafenib and MEK inhibitor trametinib are the most common drugs used for the treatment of melanoma (Guo et al., 2021; Chiavarini et al., 2022; Porcelli et al., 2022). However, BRAF-mutated melanomas treated with these compounds almost invariably develop resistance (Siegel et al., 2020). Further research on the molecular mechanism of melanoma and the development of novel therapeutics with high efficiency and low toxicity is highly desired. Nur77 is an orphan nuclear receptor that widely expressed in different types of tumors, including melanoma (Hsu et al., 2004; To et al., 2012). Nur77 plays diverse roles in the regulation of cell proliferation, survival, and apoptosis (Hu et al., 2017; Wu and Chen, 2018; Safe and Karki, 2021). The mitogenic and survival effect of Nur77 may be associated with its transcriptional activity in the nucleus (Zhang, 2007). On the other hand, the pro-apoptotic effect of Nur77 involves its translocation from the nucleus to mitochondria, where it interacts with Bcl-2 and converts Bcl-2 from a survival to a killer of cancer cells (Lin et al., 2004; Kolluri et al., 2008; Zhou et al., 2014; Liu et al., 2017). These complex effects of Nur77 appear to be dependent on its posttranslational modifications (Zhou et al., 2014; Liu et al., 2017). For example, phosphorylation of Nur77 by protein kinase B (AKT) promotes its nuclear shuttling, resulting in the promotion of cancer cell proliferation and invasion, while its phosphorylation by c-Jun N-terminal kinase (JNK) involves the apoptosis in certain cancer cells (Han et al., 2006; Bourhis et al., 2008; Bliss et al., 2012). Thus, targeting Nur77 may offer new strategies to develop effective melanoma therapeutics.
To date, many potent Nur77 modulators have been developed (Crean and Murphy, 2021). However, it is extremely challenging to push these compounds towards clinical application. One way to expedite drug development is to discover new uses for approved or investigational drugs. Recently, a study conducted by Bennett et al. revealed that predicted venlafaxine as an anti-cancer compound (Bennett et al., 2022). Venlafaxine is a serotonin and norepinephrine reuptake inhibitor (Figure 1A) and has been used in therapy as an anti-depressant drug since 1993 (Roseboom and Kalin, 2000). Venlafaxine also can attenuate neuropathic pain and vasomotor symptoms in women after cancer (Pinkerton and Santen, 2019; van den Beuken-van Everdingen et al., 2017). Many serotonin reuptake inhibitors, e.g., fluoxetine and desipramine could inhibit melanoma solid tumor growth in vitro (Kubera et al., 2009; Grygier et al., 2013). Thus, it is possible that venlafaxine can reduce melanoma cell proliferation. In addition, venlafaxine contains a two-ring group, which is commonly seen in many potent Nur77 modulators, i.g., BI1071 and Triclosan (Ha et al., 2018; Chen et al., 2019). Venlafaxine is likely to act as a Nur77 modulator, exhibiting anti-cancer activities by regulation of Nur77 signaling. So far, venlafaxine has not been explored as a therapeutic approach for melanoma and whether venlafaxine can inhibit the growth of tumor cells in animals is still unknown.
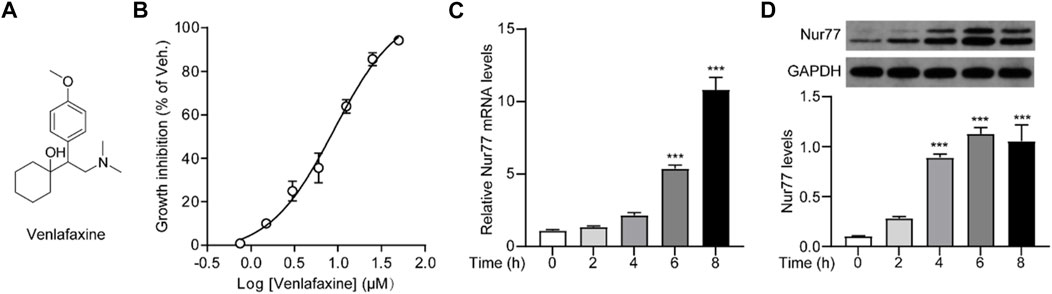
FIGURE 1. Cellular viability was correlated to Nur77 expression induced by venlafaxine. (A) Chemical structure of venlafaxine. (B) Dose-dependent inhibition of venlafaxine on the growth inhibition of MV3 cells at 72 h. (C) Relative mRNA expressions of Nur77 in MV3 cells were quantified after treatment with venlafaxine (10 μM) for 0–8 h. (D) The protein expression of Nur77 in MV3 cells was detected by Western blot after treatment with venlafaxine (10 μM) for 0–8 h. Intensity of the protein bands was quantified and normalized to loading control GAPDH. N = 3, ***, p < .001 vs. control (0 h).
As a proof of concept, we examined the capability of venlafaxine to inhibit the growth of MV3 melanoma cells, and its effects in theNur77 expression. We also studied the molecular mechanisms involved in venlafaxine-induced MV3 cell death. Our results showed that venlafaxine could reduce the growth and induce apoptosis of MV3 cells through the JNK1/2-Nur77 signaling pathway. Our results suggested venlafaxine as a potential therapy for melanoma.
2 Materials and methods
2.1 Chemicals
All reagents were purchased from Sinopharm (Shanghai, China) unless otherwise indicated. Venlafaxine hydrochloride (Cat. #V129637), SP600125 (Cat. #S125267) and PD98059 (Cat. #P126620) were purchased from Aladdin (Shanghai, China).
2.2 Cell culture and treatment
Human melanoma (MV3) cells were purchased from the China center for type culture collection (Cat. #GDC0615). MV3 cells were cultured in DMEM medium supplemented with 10% FBS, 100 units/ml penicillin, and 100 μg/ml streptomycin in humidified 5% CO2 atmosphere at 37°C until 80% confluence. MV3 cells were then treated with venlafaxine (0–100 μM), SP600125 (.5 μM) and PD98059 (15 μM) (a MEK/ERK inhibitor that can inhibit MEK activation and subsequent ERK phosphorylation) for 30 min, or human Nur77 siRNA (50 nM), JNK1/2 siRNA (Cell signaling, Cat. #6232S, 50 nM), ERK1/2 siRNA (Cell signaling, Cat. #6560S, 50 nM) and HiPerfect transfection reagent (Qiagen, 301704, United States) for 12 h, followed by incubation at 37°C for 0–72 h (Li et al., 2018). The siRNA sequences were described below:
Control siRNA: 5′-GCGCGCUUUGTAGGAUUCGdTdT-3′
Nur77 siRNA: 5′-CAGUCCAGCCAUGCUCCUCdTdT-3′
2.3 Cell viability
Cell viability was measured at 72 h, using the cell counting kit-8 (CCK8) according to the manufacturer’s instructions (Liu J et al., 2022; Qin et al., 2022). MV3 cells were collected and incubated with a CCK-8 reagent (Dojindo, Cat. #CK04, 5 mg/ml, 10 μl) at 37°C for 60 min. The OD values of the reaction mixture were measured at the wavelength of 450 nm. The cell viability was calculated using the following formula: Cell viability (%) = OD (control) − OD (test)/OD (control) − OD (blank).
2.4 Apoptosis assay
Apoptosis assay was conducted at 0, 4 and 8 h, using a dead cell apoptosis kit with Annexin V-FITC and propidium iodide (PI) (Thermo, Cat. #V13241) according to the manufacturer’s instructions (Chen et al., 2019; Li et al., 2022). MV3 cells were washed with PBS, resuspended in binding buffer, incubated with Annexin V-FITC and PI for 15 min according to the kit protocol, and analyzed immediately by cytoFLEX Flow Cytometry System (Beckman-Coulter) using FITC and PerCP.
2.5 Animals and treatments
The animal experiments were approved by the Animal Care and Use Committee of Hainan Medical University [Approval No. Med-Eth-Re (2022) 736]. Female BALB/c nude mice (20–25 g) were purchased from Shanghai SLAC Laboratory Animal Co., Ltd. Mice were maintained under specific pathogen-free conditions, group-housed in ventilated cages with controlled temperature (25°C ± 1°C) and relative humidity (55% ± 10%). Standard mouse chow and tap water were provided ad libitum. The nude mice were anesthetized by intravenous injection of pentobarbital sodium (25 mg/kg), followed by subcutaneous transplantation of MV3 or Nur77 knockout (Nur77 KO) MV3 melanoma cells in the right posterior axillary line (Liu Y. X et al., 2022). The dose of venlafaxine for the mice was based on the preliminary experiments and the references (Zhang et al., 2019; Madrigal-Bujaidar et al., 2021). Mice were treated with venlafaxine (20 mg/kg, i.p.) or its .1% DMSO-containing saline vehicle once daily after tumor size grew up to 50–100 mm3. Body weight and tumor volume were measured every 3 days. Mice were sacrificed by CO2 inhalation after 15-day drug treatment and the tumors were stripped for various assessments (Chen et al., 2019). Nur77 knockout (KO) MV3 cells were generated by CRISPR/Cas9 system using a previously reported method (Chen et al., 2019). The gRNA targeting sequence of Nur77 is 5′-ACCTTCATGGACGGCTACAC-3′. Protein lysates were prepared from tumors with previously reported method (Chen et al., 2015). Tumors were homogenized in cold 1× RIPA lysis buffer and centrifuged at 15,000 × g for 15 min at 4°C. The supernatants were collected and the protein concentration of sample was measured by Pierce BCA protein assay kit (Thermo, Cat. #23225). Ultimately, all samples were normalized to the same total protein concentration of 2 mg/ml. Frozen tumor tissues were thawed and then homogenated in Trizol reagent. Total RNA was extracted with chloroform, isopropanol, and 75% ethanol. The RNA concentration was measured with a spectrophotometer (Beckman Coulter, United States).
2.6 Western blot
Western blots were performed using the standard sodium dodecyl sulfate (SDS)-PAGE polyacrylamide gel electrophoresis method (Li et al., 2021a). The protein of cell or tumor lysates was prepared and measured by Pierce BCA protein assay kit (Thermo, Cat. #23225) (Xie et al., 2022a). The total protein (50 μg) was separated by 10% SDS-PAGE gels and transferred to a nitrocellulose membrane (Amersham Biosciences, Shanghai, China). Membranes were blocked in 5% (w/v) nonfat milk for 1 h at room temperature, washed with saline buffer (containing .05% tween-20) and then incubated at 4°C overnight with the primary antibody: Nur77 (Cell signaling, Cat. #3960S, dilution 1:500), cleaved caspase-3 (Cell signaling, Cat. #9661S, dilution 1:300), PARP (Santa Cruz, Cat. #sc-8001, dilution 1:500), p38 (Novus, Cat. #AF8691, dilution 1:500), p-p38 (Santa Cruz, Cat. #sc-166182, dilution 1:300), JNK1/2 (Santa Cruz, Cat. #sc-137019, dilution 1:800), p-JNK1/2 (R&D, Cat. #AF1205, dilution 1:400), c-Jun (Novus, Cat. #MAB8930, dilution 1:500), p-c-Jun (Cell signaling, Cat. #3270S, dilution 1:300), ERK1/2 (Cell signaling, Cat. #68303SF, dilution 1:600), p-ERK1/2 (Cell signaling, Cat. #9101S, dilution 1:300), GAPDH (Santa Cruz, Cat. #sc-47724, dilution 1:1000). The membranes were then incubated for 1 h at room temperature with horseradish peroxidase (HRP)-linked anti-rabbit IgG antibody (Santa Cruz, Cat. #sc-2004, dilution 1:5000) and detected with an electrochemiluminescence plus kit (Amersham Biosciences).
2.7 Real-time polymerase chain reaction (RT-PCR)
Total RNAs were extracted by Trizol (Invitrogen) and complemental DNA was synthesized using RevertAid First-Strand cDNA Synthesis Kits (Fermentas). RT-PCR was performed using SYBR Green dye and the Roche LightCycler® 480 II system following the manufacturer’s instructions on a 7300 real-time PCR system (Applied Biosystems) using respective primers (Hu et al., 2021):
Nur77: 5′-ACCCACTTCTCCACACCTTG-3′ (forward), 5′- ACTTGGCGTTTTTCT GCACT-3′ (reverse).
β-Actin: 5′-AGAGCTACGAGCTGCCTGAC-3’ (forward), 5′-AGCACTGTGTTG GCGTACAG-3′ (reverse).
Expression data were normalized to β-Actin mRNA expression.
2.8 Histological analysis
Tumor tissues were excised, sectioned and fixed in 10% (w/v) formalin for 24 h, followed by embedding in paraffin. The specimen was embedded in paraffin and cut into 5 μm sections for further assessments (Xie et al., 2022b).
2.9 Immunofluorescence
In vitro–cultured MV3 cells were fixed with 4% paraformaldehyde, permeabilized with .1% Triton X-100 in PBS for 20 min, and then blocked with goat serum in .3 M glycine in PBS at 25°C for 1 h. Sections were then incubated at 4°C overnight with the primary antibody: Nur77 (Cell signaling, Cat. #3960S, dilution 1:500) and Bcl2 (Abcam, Cat. #ab692, dilution 1:500). Sections were rinsed with .1 M PBS and exposed to donkey secondary antibodies conjugated with Alexa Fluor 488 or 647 (Abcam, dilution 1:1,000) at room temperature for 2 h. After an additional rinse, cells were then counterstained with 4′, 6-diamidino-2-phenylindole (DAPI) for nuclear labelling (Wang et al., 2022). Fluorescence images were captured with a confocal microscope.
The paraffin-embedded tumor sections were incubated with the following primary antibodies at 4°C overnight: Nur77 (Cell signaling, Cat. #3960S, dilution 1:500), cleaved caspase-3 (Cell signaling, Cat. #9661S, dilution 1:300), Ki-67 (Abcam, Cat. #ab15580, dilution 1:800). After incubation, sections were rinsed with .1 M PBS and exposed to goat secondary antibodies conjugated with Alexa Fluor 488 or 647 (Abcam, dilution 1:1,000) at room temperature for 1 h (Li et al., 2021b). After an additional rinse, sections were then counterstained with DAPI for nuclear labelling. Fluorescence images were captured with a confocal microscope.
Reactive oxygen species (ROS) were stained with cellular ROS assay kit (deep red) (Abcam, Cat. #ab186029) according to the manufacturer’s instructions. Terminal deoxynucleotidyl transferase-mediated dUTP nick end labeling (TUNEL) assays were carried out according to the manufacturer’s instructions (Promega, Cat. #G7131). The number of apoptotic cells was counted by Image J.
2.10 Statistical analysis
Data are presented as means ± SEM. Analyses were performed with GraphPad Prism 9.0.5. Three or more different groups were analyzed by one-way ANOVA with Dunnett’s post hoc multiple comparison tests. p < .05 was considered statistically significant.
3 Results
3.1 Cellular viability was correlated to Nur77 expression induced by venlafaxine
We first investigated whether venlafaxine could regulate the cellular viability of MV3 cells. As shown in Figure 1B, venlafaxine showed a great inhibition on the growth of MV3 cells with an LD50 = 9.01 ± .97 μM. To investigate whether the cytotoxicity effect of venlafaxine was associated with its induction of Nur77 expression, we then examined the effect of venlafaxine on Nur77 expression in MV3 cells. Based on the LD50 data of venlafaxine, MV3 cells were treated with venlafaxine at a concentration of 10 μM. qRT-PCR (Figure 1C) and western blot (Figure 1D) analyses showed that the mRNA and protein levels of Nur77 were low in MV3 cells, but were persistently elevated after venlafaxine treatment. Together, these results suggested that venlafaxine reduced the cell proliferation and introduced Nur77 expression in MV3 cells.
3.2 Venlafaxine-induced apoptosis in MV3 cells
Given that the death effect of Nur77 is associated with its induction of apoptosis, which has been observed in other studies (Zhang, 2007), we speculate that venlafaxine may exert an anti-proliferative effect through activating the Nur77-dependent apoptotic pathway. As a proof of concept, we examined the expression of BAX-2, cleaved caspase 3 and cleaved PARP, the indicators of apoptosis, in MV3 cells treated with venlafaxine (10 μM). Western-blot analysis showed that venlafaxine treatment persistently induced caspase 3 and PARP cleavage and BAX-2 expression in MV3 cells (Figure 2A). We further assessed the effect of venlafaxine on cell death using flow cytometry-based Annexin V/Propidium iodide (PI) apoptosis assay. As shown in Figure 2B, approximately 30% of MV3 cells were apoptotic when treated with venlafaxine for 8 h, whereas only about 2% of cells were apoptotic in vehicle control group. Moreover, TUNEL assay revealed extensive apoptosis in venlafaxine-treated MV3 cells (Figure 2C). Together, these results revealed that venlafaxine induces apoptosis in MV3 cells.
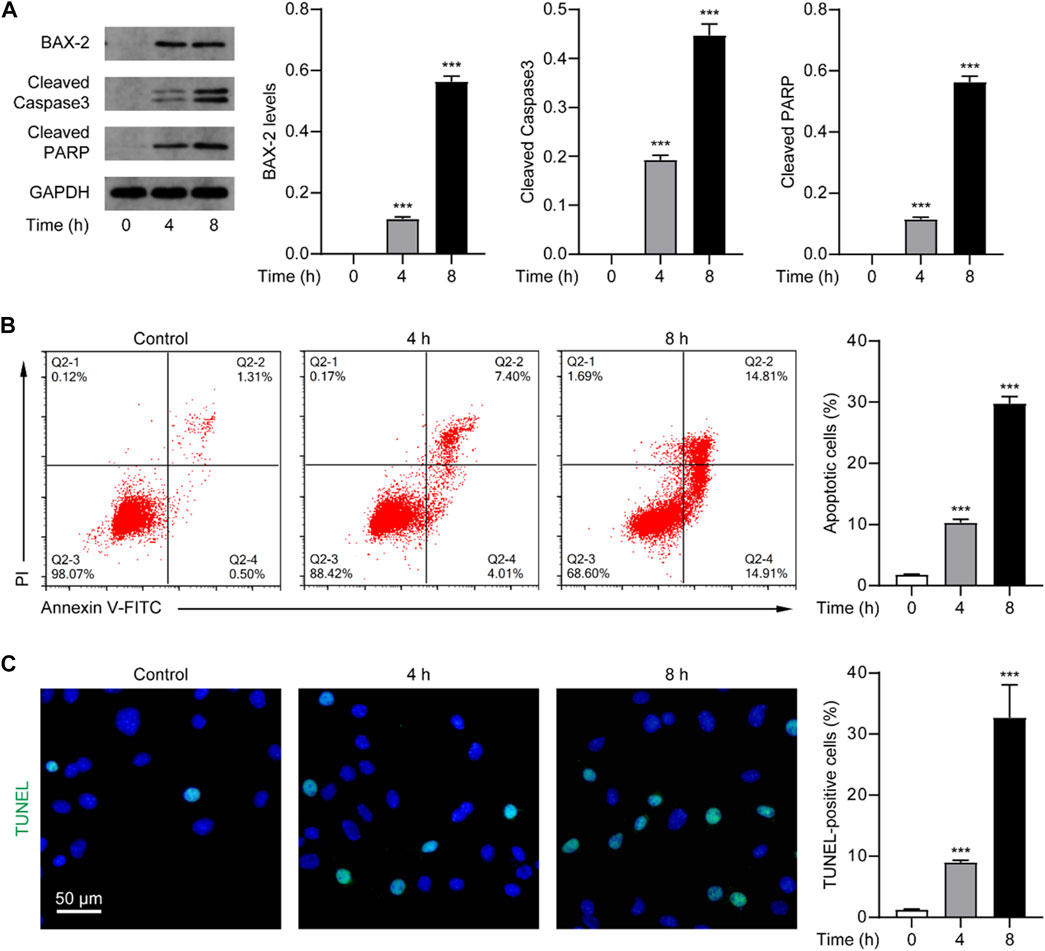
FIGURE 2. Venlafaxine induced apoptosis in MV3 cells. (A) The protein expression of BAX-2, cleaved caspase 3 and cleaved PARP in MV3 cells was detected by Western blot after treatment with venlafaxine (10 μM) for 0–8 h. Intensity of the protein bands was quantified and normalized to loading control GAPDH. (B) Apoptosis assays in MV3 cells treated with venlafaxine (10 μM) for 0–8 h were conducted by flow cytometry. (C) The apoptotic cells were detected by TUNEL assay in MV3 cells treated with venlafaxine (10 μM) for 0–8 h. N = 3, ***, p < .001 vs. control (0 h).
3.3 Venlafaxine induced Nur77 mitochondrial targeting and ROS production in MV3 cells
Nur77 can induce apoptosis by translocating to mitochondria where it binds to Bcl-2 to trigger cytochrome c release and ROS production (Lin et al., 2004; Chen et al., 2019). Thus, we further examined whether venlafaxine could induce Nur77 translocation from the nucleus to mitochondria. Immunofluorescence assay showed that Nur77 was mainly localized in the nucleus of MV3 cells, while treatment of venlafaxine promoted the mitochondrial translocation of this protein (Figure 3A). Furthermore, following Nur77 mitochondrial translocation, the production of ROS in MV3 cells was also enhanced by venlafaxine (Figure 3B). Together, these results revealed that venlafaxine induced Nur77 mitochondrial targeting and ROS production in MV3 cells.
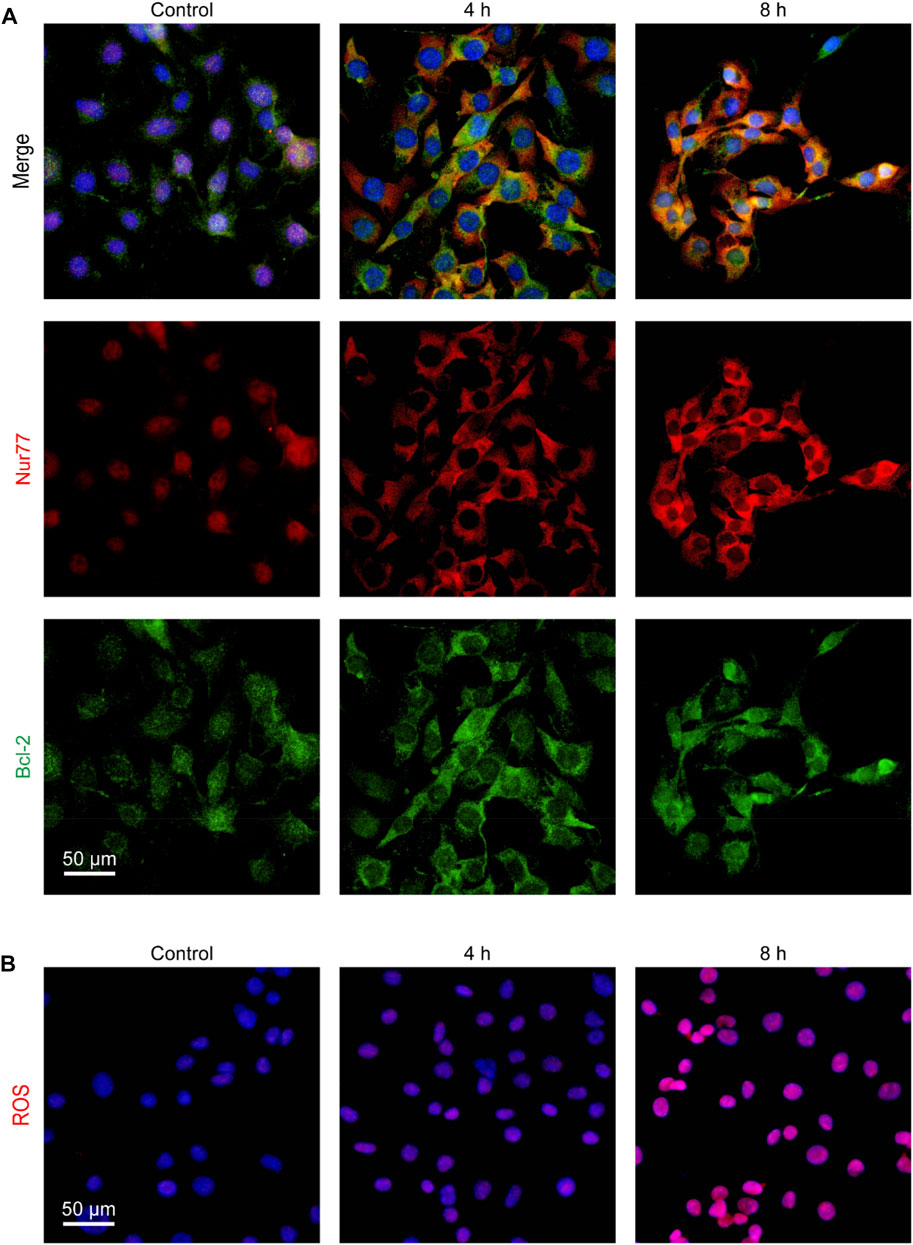
FIGURE 3. Venlafaxine induced Nur77 mitochondrial targeting and ROS production in MV3 cells. (A) MV3 cells treated with venlafaxine (10 μM) for 0–8 h were immunostained with Bcl-2 and Nur77 antibodies and visualized by confocal microscopy. (B) MV3 cells treated with the cellular ROS assay kit and visualized by confocal microscopy.
3.4 Nur77 expression was necessary for venlafaxine-induced apoptosis
We next determined whether venlafaxine-induced apoptosis was Nur77-dependent. MV3 cells were transfected with Nur77 siRNA and subjected to venlafaxine treatment for 8 h. We found that Nur77 knockdown decreased venlafaxine-induced expressions of BAX-2, cleaved caspase 3 and cleaved PARP were in MV3 cells (Figure 4A). Furthermore, venlafaxine-induced apoptosis was rescued by transfecting with Nur77 siRNA, but not control siRNA (Figures 4B, C). Additionally, venlafaxine induced lower levels of ROS in Nur77-knockdown cells than control MV3 cells (Figure 4D). Together, these data indicated that Nur77 expression was necessary for venlafaxine-induced apoptosis.
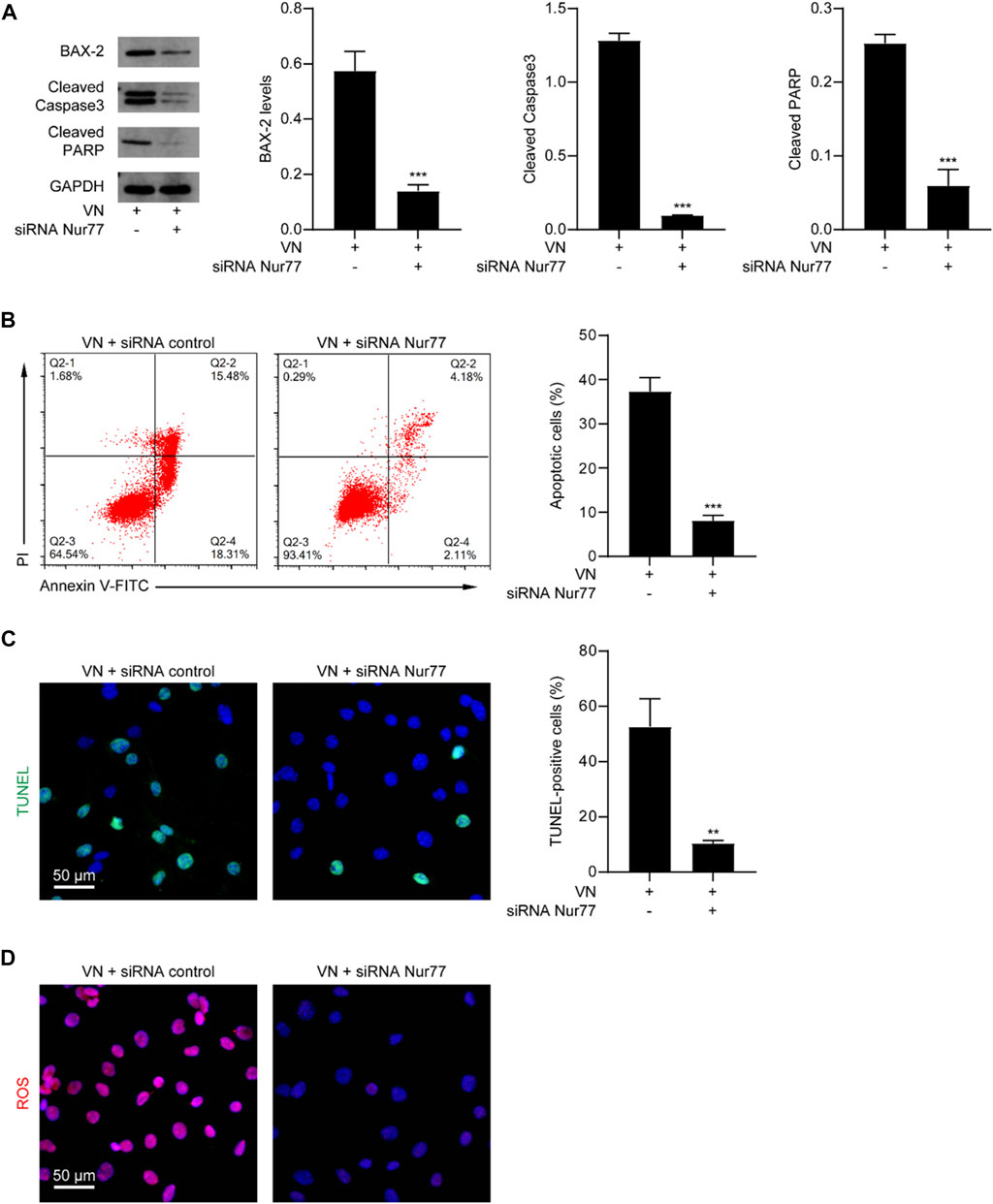
FIGURE 4. Nur77 expression was necessary for venlafaxine-induced apoptosis. MV3 cells were transfected with siRNA control or Nur77 siRNA, following by treatment with venlafaxine (10 μM) for 0–8 h. (A) The protein expression of BAX-2, caspase 3 and cleaved PARP in MV3 cells was detected by western blot. Inte cleaved nsity of the protein bands was quantified and normalized to loading control GAPDH. (B) Apoptosis assays in MV3 cells were conducted by flow cytometry. (C) The apoptotic cells were detected by TUNEL assay. (D) MV3 cells treated with the cellular ROS assay kit and visualized by confocal microscopy. N = 3, **, p < .01, ***, p < .001 vs. control.
3.5 Activation of JNK1/2 signaling was necessary for venlafaxine-induced cell death effects
Given that MAPKs play an important role in the regulation of melanoma cell survival and Nur77 post-translational modifications (Liu et al., 2017; Huo et al., 2020; Hu et al., 2021), we further studied whether venlafaxine affects Nur77 expression through MAPKs signaling. MV3 cells expressed relatively low levels of phosphorylated MEK (p-MEK), p-JNK1/2, p-c-Jun, and p-ERK. After treatment with venlafaxine, the levels of p-MEK, p-JNK1/2, p-c-Jun, and p-ERK were increased in MV3 cells (Figure 5A). To further study the roles of MAPK signaling in venlafaxine-induced apoptosis, MV3 cells were co-treated with venlafaxine and different inhibitors of MAPK kinases, including MEK/ERK signaling inhibitor PD98059 and JNK1/2 inhibitor SP600125. CCK8 assay showed that inhibition of JNK1/2 activity by SP600125 impaired venlafaxine-induced cell death effects, while PD98059 had no such effects (Figure 5B). Moreover, knockdown of JNK1/2 but not ERK increased cell viability in venlafaxine-treated MV3 cells (Figure 5C). These data demonstrated that JNK1/2 signaling is involved in venlafaxine-induced cell death effects. Next, we examined whether venlafaxine affects Nur77 expression in MV3 cells through JNK1/2 signaling. As shown in Figures 5D, E, both genetic inactivation and pharmacological administration of JNK1/2 inhibitor SP600125 significantly suppressed venlafaxine-induced Nur77 expression, indicating that venlafaxine increases Nur77 expression in MV3 cells through activation of JNK1/2 signaling. Furthermore, blockade of JNK1/2 signaling also suppressed mitochondrial translocation of Nur77 (Figure 5F). Taken together, these data suggested that venlafaxine induced MV3 cells death through activation of JNK1/2 signaling and induction of Nur77 expression.
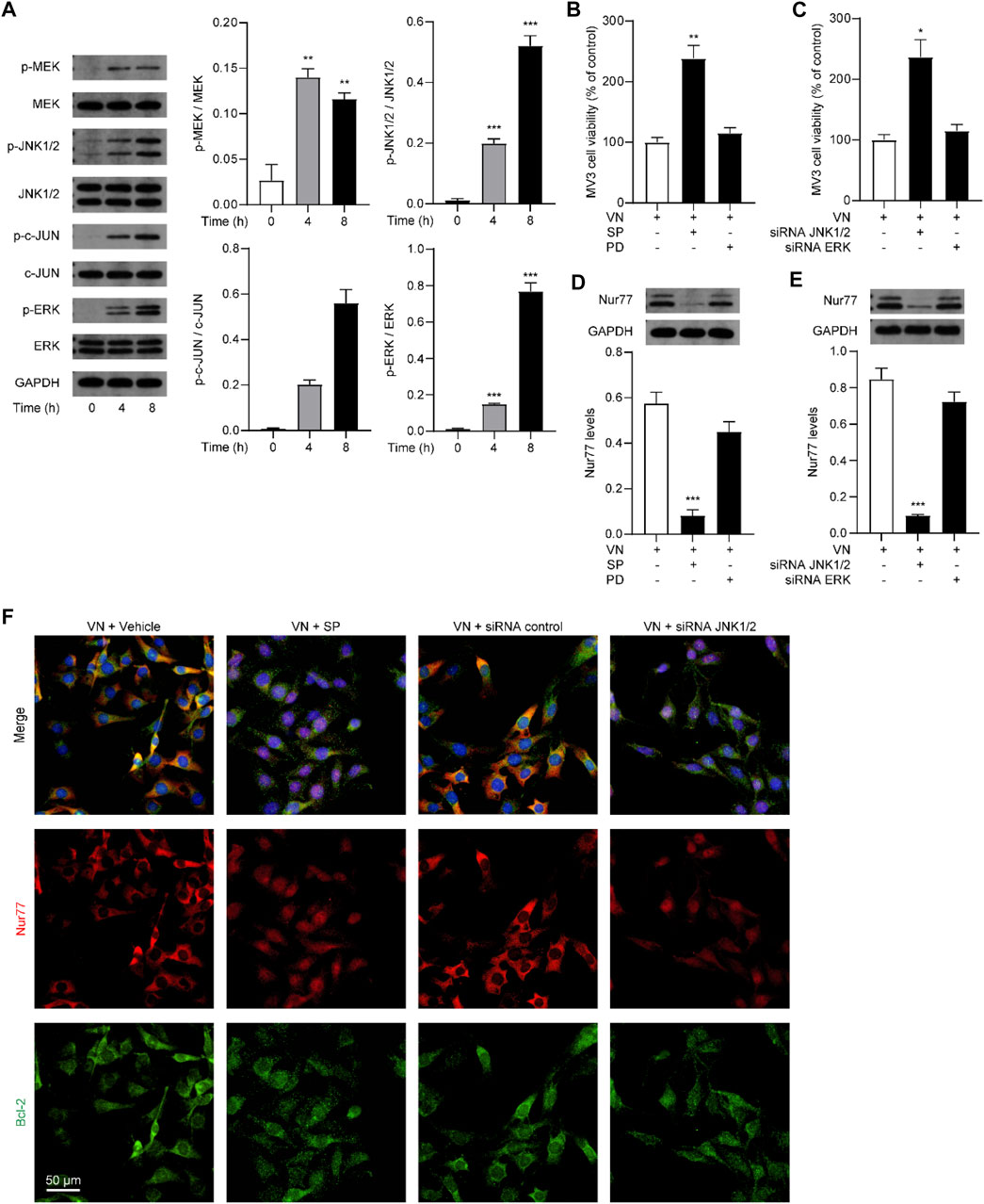
FIGURE 5. Activation of ERK1/2 signaling was necessary for venlafaxine-induced apoptosis. (A) Representative western-blot bands and quantification of the MAPK signal molecules abundances in MV3 cells treated with venlafaxine (10 μM) for 0–8 h (B) MV3 cells were treated with vehicle, JNK1/2 inhibitor SP600125 and MERK/ERK inhibitor PD98059 for 72 h. Cell viability were measured by CCK-8 kits at 72 h (C) MV3 cells were transfected with siRNA control, JNK1/2 siRNA, and ERK siRNA for 72 h. Cell viability were measured by CCK-8 kits at 72 h (D,E) Representative western-blot bands and quantification of Nur77 abundances in MV3 cells treated with venlafaxine (10 μM) for 8 h, or transfected with siRNA control, JNK1/2 siRNA, and ERK siRNA for 20 h (F) MV3 cells were immunostained with Bcl-2 and Nur77 antibodies and visualized by confocal microscopy. N = 3, *, p < .05, **, < .01, ***, p < .001 vs. control.
3.6 Anti-cancer efficacy of venlafaxine in mice
Encouraged by the in vitro activity of venlafaxine, we further investigated whether the induction of Nur77 by venlafaxine contribute to its growth inhibitory effect in vivo. BALB/c nude mice were inoculated subcutaneously with MV3 cells, and were treated with venlafaxine (20 mg/kg, i.p., once daily for 15 days) when the average tumor size grew up to 50–100 mm3. Consistent with the in vitro results, treatment with venlafaxine for 15 days promoted expressions of Nur77 and activation of JNK1/2 in tumor tissues (Figure 6A). Furthermore, at the 15 days after implantation, the average tumor volume of the vehicle control group was 1,732 mm3; and that of venlafaxine treatment group was 630 mm3, with the inhibition ratio of tumor growth was 64% (Figure 6B). These results demonstrated that venlafaxine induced Nur77 expression and inhibit the growth of MV3 xenograft tumor in vivo.
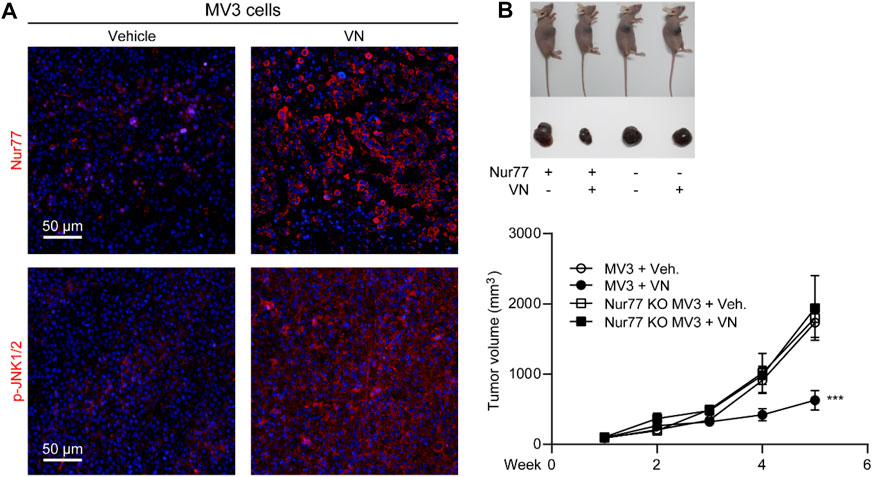
FIGURE 6. Anti-cancer efficacy of venlafaxine in mice. BALB/c nude mice bearing MV3 or Nur77 KO MV3 xenograft tumors were treated with venlafaxine (20 mg/kg, i.p.) or its vehicle once daily from week 1 to week 5 after implantation of MV3 or Nur77 KO MV3 cells. (A) Tumor tissues were immunostained with Nur77 and JNK1/2 antibodies and visualized by confocal microscopy. (B) Venlafaxine reduced cancer cell growth in nude mice through Nur77. One of five similar experiments is shown.
To study whether venlafaxine inhibit the growth of MV3 xenograft tumor associated with its induction of apoptosis. We examined the expression of BAX-2, cleaved caspase 3 and cleaved PARP in tumor tissues. Western-blot assay and immunostaining showed that venlafaxine treatment significantly increased the level of these protein (Figures 7A, B). Additionally, immunostaining assay demonstrated that venlafaxine enhanced apoptotic cell death, as assessed by TUNEL staining, and suppressed cell proliferation, as assessed by Ki-67 immunostaining, in tumor tissues (Figure 7C). When combined, these results suggested that venlafaxine potently inhibited the growth of melanoma cells in animals through its apoptotic cell death effects.
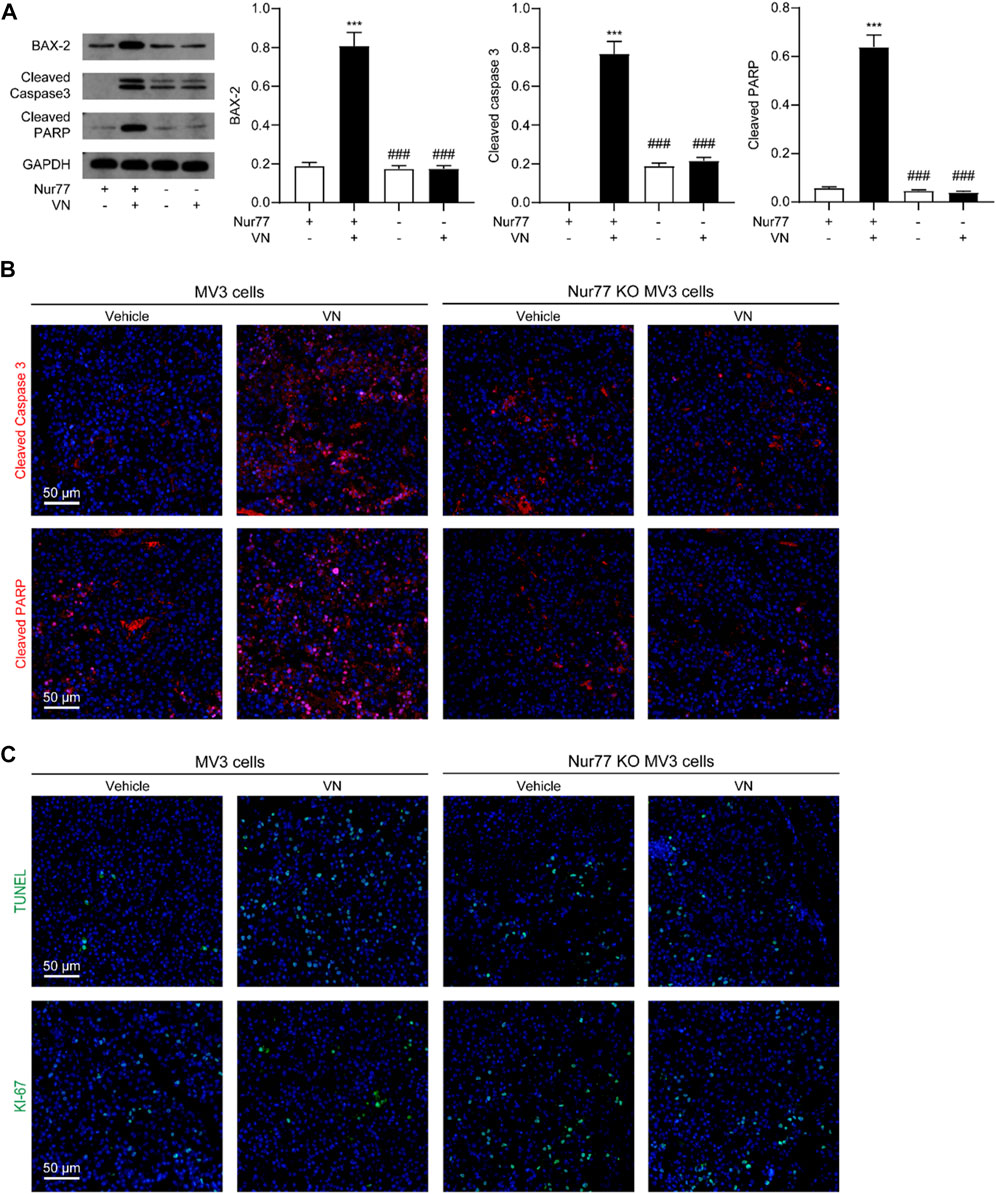
FIGURE 7. Venlafaxine-induced apoptosis in vivo. BALB/c nude mice bearing WT MV3 or Nur77 KO MV3 xenograft tumors were treated with venlafaxine (20 mg/kg, i.p.) or its vehicle once daily from week 1 to week 5 after implantation of MV3 or Nur77 KO MV3 cells. (A) Representative western-blot bands and quantification of BAX-2, cleaved caspase 3 and cleaved PARP abundances in tumor tissues. N = 5, *, p < .05, **, < .01, ***, p < .001 vs. MV3 vehicle control. ###, p < .001 vs. MV3 venlafaxine control. (B) Tumor tissues were immunostained with cleaved caspase 3 and cleaved PARP antibodies and visualized by confocal microscopy. (C) Tumor tissues were immunostained with TUNEL assay kits and antibodies and Ki-67 visualized by confocal microscopy. One of five similar experiments is shown.
Furthermore, we also studied the role of Nur77 in the anti-cancer effects of venlafaxine in vivo. We used the CRISPR/Cas9 technology to generate Nur77 knockout (KO) MV3 cells, and subcutaneously injected Nur77 KO MV3 melanoma cells into the BALB/c nude mice. At the 15 days after implantation, we found that the average tumor volume of the Nur77 KO MV3 group was similar to that of MV3 group, indicating Nur77 is not required for the growth of MV3 xenograft tumor. Furthermore, treatment with venlafaxine could not inhibit the growth of Nur77 KO MV3 xenograft tumor (Figures 6B, C), as well as the expression of BAX-2, cleaved caspase 3 and cleaved PARP in tumor tissues (Figures 7A, B). Moreover, treatment with venlafaxine had no effects on the numbers of TUNEL-positive and Ki-67-positive cells (Figure 7C). Taken together, these results suggested that venlafaxine suppresses the growth of melanoma cells through Nur77-dependent apoptotic pathway.
4 Discussion
Melanoma is one of the most aggressive and dangerous form of skin cancer (Ahmed et al., 2020). Although immunotherapies and targeted therapies are highly effective in ameliorating melanoma, their clinical use is hindered by the drug resistance (Ahmed et al., 2020). Therefore, novel approaches to melanoma treatment are still highly desired to reduce the mortality rate of patient with melanoma. One way to rapidly develop therapeutic agents for melanoma is drug repurposing, defined as the re-application of known drugs to target new indication. Some of the classic examples of successful repurposing are minoxidil and gabapentin. Minoxidil, originally an anti-hypertensive agent, is now commonly used to promote hair re-growth (Goren and Naccarato, 2018). Gabapentin, originally used as anti-epileptics, is now used to treat neuropathic pain (Moore and Gaines, 2019). Therefore, we switch our attention from newly synthesized anti-cancer compound to approved drugs to rapidly develop therapeutic agents for melanoma. As a proof of concept, we demonstrated that anti-depressant drug venlafaxine could inhibit the growth of MV3 melanoma cells through introduction of Nur77 expression. Mechanistically, venlafaxine activates JNK1/2 signaling pathway, thus trigger expression and mitochondrial localization of Nur77. Follow mitochondrial translocation, Nur77 binds to Bcl-2 and converts Bcl-2 from a survival to a killer of cancer cells, thereby inhibiting the growth and induce apoptosis of MV3 melanoma cells (Figure 8).
A significant finding presented here is that venlafaxine can induce apoptosis of MV3 human melanoma cells through a Nur77-dependent pathway. Nur77 is an orphan nuclear receptor. It often translocate to mitochondria and binds to Bcl-2 in response to different death signals, leading to a conformation change in Bcl-2 and conversion of Bcl-2 from performing an anti-apoptotic role to a pro-apoptotic role (Lin et al., 2004; Kolluri et al., 2008). Many anti-cancer drugs, including vinblastine, vincristine, taxol, and cisplatin also induce expression of Nur77 (Deacon et al., 2003; Achkar et al., 2018). Herein, we found that venlafaxine treatment could increase Nurr77 expression and interaction with Bcl-2 (Figures 1D, 3A), resulting in ROS production (Figure 3B), and apoptosis (Figure 2A). Interestingly, the mRNA expression of Nur77 after 4 h incubation is non-significant, although there is significant protein expression at the same time point (Figures 1C, D). The reason for the discrepancies between mRNA expressions and protein expression is not clear. There may be serval reasons attributed to these results. It has been reported that Nur77 can bind to Bcl-2 after phosphorylation by JNK1/2. It is possible that the certain posttranslational modifications of Nur77 may increase the binding affinity of Bur77 to its antibody, thus a significant band of Nur77 was also observed at 4 h. Furthermore, we cannot exclude the possibility that the degradation of Nur77 was reduced in MV3 cells after 4 h incubation with venlafaxine. Future studies need to be carried out to confirm the precise reason for this result. Furthermore, the Nur77 subgroup of nuclear hormone receptors subfamily has been implicated in the pathophysiology of the central nervous system, including manic depression, Parkinson’s disease, schizophrenia, and Alzheimer’s disease (Liu et al., 2021). Venlafaxine also exhibits pharmacological actions in these diseases (Mazeh et al., 2004; Mokhber et al., 2014; El-Saiy et al., 2022; Zhuo et al., 2022). It is possible that venlafaxine produces therapeutic effects in these diseases through introduction of Nur77 expression.
A number of studies suggested that MAPKs, including JNK1/2 and ERK and their related upstream or downstream signal molecules such as MEK can regulate Nur77 expression (Anjum et al., 2022). Our results showed that venlafaxine could activated the JNK/c-Jun and the MERK/ERK pathway. Both pathways are documented in the literature. For example, c-Jun is a transcription factor that positively modulated Nur77 mitochondrial localization and activation by directly binding to the Nur77 DNA promoter regions (Han et al., 2006; Ming et al., 2018). The transcriptional activity of c-Jun is associated with its abundance and posttranslational modification (Han et al., 2006; Ming et al., 2018). Ha et. al. (2018) have reported that inhibition of c-Jun phosphorylation by miR-6321/Map3k1 reduced Nur77 protein expression, while activation of the JNK/c-Jun pathway by anisomycin increased Nur77 levels (Han et al., 2006). In addition, the MERK/ERK pathway plays a critical role in inducing Nur77 expression. GnRH promoted Nur77 expression in alpha T3-1 cells through ERK signaling (Bliss et al., 2012; Oliveira et al., 2021; Liu Y. X et al., 2022). Scalarane sesterterpenoid 12-deacetyl-12-epi-scalaradial induces HeLa cells apoptosis through ERK-mediated expression and phosphorylation of Nur77 (Zhou et al., 2020). Furthermore, malayoside, a cardenolide glycoside extracted from Antiaris toxicaria Lesch, also induced Nur77 expression, phosphorylation, and human non-small lung cancer cells apoptosis through ERK signaling (Hu et al., 2021). Our results showed that venlafaxine-induced Nur77 expression and cell apoptosis can be blocked by treatment with JNK1/2 inhibitor SP600125, but not MERK/ERK inhibitor PD98059, suggesting that venlafaxine may induced MV3 cell apoptosis through the JNK/c-Jun signaling.
5 Conclusion
In conclusion, our results demonstrate that venlafaxine could reduce the growth and induce apoptosis of MV3 cells through the JNK1/2-Nur77 signaling pathway. Our results also identified venlafaxine as a promising therapy for melanoma.
Data availability statement
The raw data supporting the conclusion of this article will be made available by the authors, without undue reservation.
Ethics statement
The animal study was reviewed and approved by the Animal Care and Use Committee of Hainan Medical University.
Author contributions
TN conducted most of the experiments and helped with manuscript preparation. ZW, JF, and YW conduced cell culture, western blot and analyzed data. SC and RW conduced Flow analysis. RZ and TN designed the experiments, and wrote the manuscript.
Funding
This work was supported by National Natural Science Fund Cultivating 530 Project of Hainan General Hospital (No. 2021QNXM05 to TN).
Conflict of interest
The authors declare that the research was conducted in the absence of any commercial or financial relationships that could be construed as a potential conflict of interest.
Publisher’s note
All claims expressed in this article are solely those of the authors and do not necessarily represent those of their affiliated organizations, or those of the publisher, the editors and the reviewers. Any product that may be evaluated in this article, or claim that may be made by its manufacturer, is not guaranteed or endorsed by the publisher.
References
Achkar, I. W., Abdulrahman, N., Al-Sulaiti, H., Joseph, J. M., Uddin, S., and Mraiche, F. (2018). Cisplatin based therapy: The role of the mitogen activated protein kinase signaling pathway. J. Transl. Med. 16, 96. doi:10.1186/s12967-018-1471-1
Ahmed, B., Qadir, M. I., and Ghafoor, S. (2020). Malignant melanoma: Skin cancer-diagnosis, prevention, and treatment. Crit. Rev. Eukaryot. Gene Expr. 30, 291–297. doi:10.1615/CritRevEukaryotGeneExpr.2020028454
Anjum, J., Mitra, S., Das, R., Alam, R., Mojumder, A., Bin Emran, T., et al. (2022). A renewed concept on the MAPK signaling pathway in cancers: Polyphenols as a choice of therapeutics. Pharmacol. Res. 184, 106398. doi:10.1016/j.phrs.2022.106398
Bennett, A. N., Huang, R. X., He, Q., Lee, N. P., Sung, W. K., and Chan, K. H. K. (2022). Drug repositioning for esophageal squamous cell carcinoma. Front. Genet. 13, 991842. doi:10.3389/fgene.2022.991842
Bliss, S. P., Navratil, A. M., Xie, J., Miller, A., Baccarini, M., and Roberson, M. S. (2012). ERK signaling, but not c-Raf, is required for gonadotropin-releasing hormone (GnRH)-induced regulation of Nur77 in pituitary gonadotropes. Endocrinology 153, 700–711. doi:10.1210/en.2011-0247
Bourhis, E., Maheux, J., Rouillard, C., and Levesque, D. (2008). Extracellular signal-regulated kinases (ERK) and protein kinase C (PKC) activities are involved in the modulation of Nur77 and Nor-1 expression by dopaminergic drugs. J. Neurochem. 106, 875–888. doi:10.1111/j.1471-4159.2008.05455.x
Chen, L., Chen, H., Li, Y., Li, L., Qiu, Y., and Ren, J. (2015). Endocannabinoid and ceramide levels are altered in patients with colorectal cancer. Oncol. Rep. 34, 447–454. doi:10.3892/or.2015.3973
Chen, X., Cao, X., Tu, X., Alitongbieke, G., Xia, Z., Li, X., et al. (2019). BI1071, a novel Nur77 modulator, induces apoptosis of cancer cells by activating the nur77-bcl-2 apoptotic pathway. Mol. cancer Ther. 18, 886–899. doi:10.1158/1535-7163.MCT-18-0918
Chiavarini, M., Naldini, G., Giacchetta, I., and Fabiani, R. (2022). Postmenopausal exogenous hormone therapy and melanoma risk in women: A systematic review and time-response meta-analysis. Pharmacol. Res. 176, 106054. the official journal of the Italian Pharmacological Society. doi:10.1016/j.phrs.2021.106054
Crean, D., and Murphy, E. P. (2021). Targeting NR4A nuclear receptors to control stromal cell inflammation, metabolism, angiogenesis, and tumorigenesis. Front. Cell Dev. Biol. 9, 589770. doi:10.3389/fcell.2021.589770
Cullen, J. K., Simmons, J. L., Parsons, P. G., and Boyle, G. M. (2020). Topical treatments for skin cancer. Adv. Drug Deliv. Rev. 153, 54–64. doi:10.1016/j.addr.2019.11.002
Deacon, K., Mistry, P., Chernoff, J., Blank, J. L., and Patel, R. (2003). p38 Mitogen-activated protein kinase mediates cell death and p21-activated kinase mediates cell survival during chemotherapeutic drug-induced mitotic arrest. Mol. Biol. Cell 14, 2071–2087. doi:10.1091/mbc.e02-10-0653
El-Saiy, K. A., Sayed, R. H., El-Sahar, A. E., and Kandil, E. A. (2022). Modulation of histone deacetylase, the ubiquitin proteasome system, and autophagy underlies the neuroprotective effects of venlafaxine in a rotenone-induced Parkinson's disease model in rats. Chem. Biol. Interact. 354, 109841. doi:10.1016/j.cbi.2022.109841
Goren, A., and Naccarato, T. (2018). Minoxidil in the treatment of androgenetic alopecia. Dermatol Ther. 31, e12686. doi:10.1111/dth.12686
Grygier, B., Arteta, B., Kubera, M., Basta-Kaim, A., Budziszewska, B., Leskiewicz, M., et al. (2013). Inhibitory effect of antidepressants on B16F10 melanoma tumor growth. Pharmacol. Rep. 65, 672–681. doi:10.1016/s1734-1140(13)71045-4
Guo, W., Wang, H., and Li, C. (2021). Signal pathways of melanoma and targeted therapy. Signal Transduct. Target Ther. 6, 424. doi:10.1038/s41392-021-00827-6
Ha, M., Zhang, P., Li, L., and Liu, C. (2018). Triclosan suppresses testicular steroidogenesis via the miR-6321/JNK/Nur77 cascade. Cell Physiol. Biochem. 50, 2029–2045. doi:10.1159/000495049
Han, Y. H., Cao, X., Lin, B., Lin, F., Kolluri, S. K., Stebbins, J., et al. (2006). Regulation of Nur77 nuclear export by c-Jun N-terminal kinase and Akt. Oncogene 25, 2974–2986. doi:10.1038/sj.onc.1209358
Hsu, H. C., Zhou, T., and Mountz, J. D. (2004). Nur77 family of nuclear hormone receptors. Curr. Drug Targets Inflamm. Allergy 3, 413–423. doi:10.2174/1568010042634523
Hu, M., Luo, Q., Alitongbieke, G., Chong, S., Xu, C., Xie, L., et al. (2017). Celastrol-induced Nur77 interaction with TRAF2 alleviates inflammation by promoting mitochondrial ubiquitination and autophagy. Mol. Cell 66, 141–153. doi:10.1016/j.molcel.2017.03.008
Hu, Q. Y., Zhang, X. K., Wang, J. N., Chen, H. X., He, L. P., Tang, J. S., et al. (2021). Malayoside, a cardenolide glycoside extracted from Antiaris toxicaria Lesch, induces apoptosis in human non-small lung cancer cells via MAPK-Nur77 signaling pathway. Biochem. Pharmacol. 190, 114622. doi:10.1016/j.bcp.2021.114622
Huo, J., Xu, S., and Lam, K. P. (2020). ASK1 mediates Nur77 expression in T-cell receptor mediated thymocyte apoptosis. Cells 9, 585. doi:10.3390/cells9030585
Kolluri, S. K., Zhu, X., Zhou, X., Lin, B., Chen, Y., Sun, K., et al. (2008). A short Nur77-derived peptide converts Bcl-2 from a protector to a killer. Cancer Cell 14, 285–298. doi:10.1016/j.ccr.2008.09.002
Kubera, M., Grygier, B., Arteta, B., Urbanska, K., Basta-Kaim, A., Budziszewska, B., et al. (2009). Age-dependent stimulatory effect of desipramine and fluoxetine pretreatment on metastasis formation by B16F10 melanoma in male C57BL/6 mice. Pharmacol. Rep. 61, 1113–1126. doi:10.1016/s1734-1140(09)70174-4
Li, H., Li, X., Yang, B., Su, J., Cai, S., Huang, J., et al. (2021). The retinoid X receptor alpha modulator K-80003 suppresses inflammatory and catabolic responses in a rat model of osteoarthritis. Sci. Rep. 11, 16956. doi:10.1038/s41598-021-96517-y
Li, X. X., Wang, Z. J., Zheng, Y., Guan, Y. F., Yang, P. B., Chen, X., et al. (2018). Nuclear receptor Nur77 facilitates melanoma cell survival under metabolic stress by protecting fatty acid oxidation. Mol. Cell 69, 480–492. doi:10.1016/j.molcel.2018.01.001
Li, Y., Li, Y., Xu, S., Chen, Y., Zhou, P., Hu, T., et al. (2022). Corrigendum to "N-acylethanolamine acid amidase (NAAA) exacerbates psoriasis inflammation by enhancing dendritic cell (DCs) maturation" [Pharm. Res. 185 (2022) 1-16/YPHRS_106491]. Pharmacol. Res. 185, 106527. doi:10.1016/j.phrs.2022.106527
Li, Y., Zhou, P., Hu, T., Ren, J., Xu, Y., Qiu, Y., et al. (2021). NAAA inhibitor F96 attenuates BBB disruption and secondary injury after traumatic brain injury (TBI). Eur. J. Pharmacol. 912, 174561. doi:10.1016/j.ejphar.2021.174561
Lin, B., Kolluri, S. K., Lin, F., Liu, W., Han, Y. H., Cao, X., et al. (2004). Conversion of Bcl-2 from protector to killer by interaction with nuclear orphan receptor Nur77/TR3. Cell 116, 527–540. doi:10.1016/s0092-8674(04)00162-x
Liu, J., Wang, G. H., Duan, Y. H., Dai, Y., Bao, Y., Hu, M., et al. (2017). Modulation of the Nur77-Bcl-2 apoptotic pathway by p38α MAPK. Oncotarget 8, 69731–69745. doi:10.18632/oncotarget.19227
Liu, J., Zheng, X., Li, W., Ren, L., Li, S., Yang, Y., et al. (2022). Anti-tumor effects of Skp2 inhibitor AAA-237 on NSCLC by arresting cell cycle at G0/G1 phase and inducing senescence. Pharmacol. Res. 181, 106259. the official journal of the Italian Pharmacological Society. doi:10.1016/j.phrs.2022.106259
Liu, L., Ma, D., Zhuo, L., Pang, X., You, J., and Feng, J. (2021). Progress and promise of nur77-based therapeutics for central nervous system disorders. Curr. Neuropharmacol. 19, 486–497. doi:10.2174/1570159X18666200606231723
Liu, Y. X., Xu, B. W., Niu, X. D., Chen, Y. J., Fu, X. Q., Wang, X. Q., et al. (2022). Inhibition of Src/STAT3 signaling-mediated angiogenesis is involved in the anti-melanoma effects of dioscin. Pharmacol. Res. 175, 105983. doi:10.1016/j.phrs.2021.105983
Madrigal-Bujaidar, E., Gomez-Gonzalez, P., Camacho-Cantera, S., Morales-Gonzalez, J. A., Madrigal-Santillan, E., and Alvarez-Gonzalez, I. (2021). Genotoxic and cytotoxic evaluation of venlafaxine in an acute and a subchronic assay in mouse. Braz J. Biol. 84, e251289. doi:10.1590/1519-6984.251289
Mazeh, D., Shahal, B., Saraf, R., and Melamed, Y. (2004). Venlafaxine for the treatment of depressive episode during the course of schizophrenia. J. Clin. Psychopharmacol. 24, 653–655. doi:10.1097/01.jcp.0000144894.37611.0a
Ming, S., Shui-Yun, W., Wei, Q., Jian-Hui, L., Ru-Tai, H., Lei, S., et al. (2018). miR-139-5p inhibits isoproterenol-induced cardiac hypertrophy by targetting c-Jun. Biosci. Rep. 38, BSR20171430. doi:10.1042/BSR20171430
Mokhber, N., Abdollahian, E., Soltanifar, A., Samadi, R., Saghebi, A., Haghighi, M. B., et al. (2014). Comparison of sertraline, venlafaxine and desipramine effects on depression, cognition and the daily living activities in Alzheimer patients. Pharmacopsychiatry 47, 131–140. doi:10.1055/s-0034-1377041
Moore, J., and Gaines, C. (2019). Gabapentin for chronic neuropathic pain in adults. Br. J. Community Nurs. 24, 608–609. doi:10.12968/bjcn.2019.24.12.608
Oliveira, E. A., Chauhan, J., Silva, J. R. D., Carvalho, L., Dias, D., Carvalho, D. G., et al. (2021). TOP1 modulation during melanoma progression and in adaptative resistance to BRAF and MEK inhibitors. Pharmacol. Res. official J. Italian Pharmacol. Soc. 173, 105911. doi:10.1016/j.phrs.2021.105911
Pinkerton, J. V., and Santen, R. J. (2019). Managing vasomotor symptoms in women after cancer. Climacteric 22, 544–552. doi:10.1080/13697137.2019.1600501
Porcelli, L., Di Fonte, R., Pierri, C. L., Fucci, L., Saponaro, C., Armenio, A., et al. (2022). BRAF(V600E;K601Q) metastatic melanoma patient-derived organoids and docking analysis to predict the response to targeted therapy. Pharmacol. Res. 182, 106323. the official journal of the Italian Pharmacological Society. doi:10.1016/j.phrs.2022.106323
Qin, Y., Xie, J., Zheng, R., Li, Y., and Wang, H. (2022). Oleoylethanolamide as a new therapeutic strategy to alleviate doxorubicin-induced cardiotoxicity. Front. Pharmacol. 13, 863322. doi:10.3389/fphar.2022.863322
Roseboom, P. H., and Kalin, N. H. (2000). Neuropharmacology of venlafaxine. Depress Anxiety 12 (1), 20–29. doi:10.1002/1520-6394(2000)12:1+<20::AID-DA3>3.0.CO;2-M
Safe, S., and Karki, K. (2021). The paradoxical roles of orphan nuclear receptor 4A (NR4A) in cancer. Mol. Cancer Res. 19, 180–191. doi:10.1158/1541-7786.MCR-20-0707
Siegel, R. L., Miller, K. D., Goding Sauer, A., Fedewa, S. A., Butterly, L. F., Anderson, J. C., et al. (2020). Colorectal cancer statistics, 2020. CA Cancer J. Clin. 70, 145–164. doi:10.3322/caac.21601
To, S. K., Zeng, J. Z., and Wong, A. S. (2012). Nur77: A potential therapeutic target in cancer. Expert Opin. Ther. Targets 16, 573–585. doi:10.1517/14728222.2012.680958
van den Beuken-van Everdingen, M. H., de Graeff, A., Jongen, J. L., Dijkstra, D., Mostovaya, I., Vissers, K. C., et al. (2017). Pharmacological treatment of pain in cancer patients: The role of adjuvant analgesics, a systematic review. Pain Pract. official J. World Inst. Pain 17, 409–419. doi:10.1111/papr.12459
Wang, T., Zheng, R., and Sun, S. (2022). Drug Repurposing: Escitalopram attenuates acute lung injury by inhibiting the SIK2/HDAC4/NF-κB signaling cascade. Biochem. Biophys. Res. Commun. 599, 1–8. doi:10.1016/j.bbrc.2022.02.015
Wu, L., and Chen, L. (2018). Characteristics of Nur77 and its ligands as potential anticancer compounds (Review). Mol. Med. Rep. 18, 4793–4801. doi:10.3892/mmr.2018.9515
Xie, X., Li, Y., Xu, S., Zhou, P., Yang, L., Xu, Y., et al. (2022). Genetic blockade of NAAA cell-specifically regulates fatty acid ethanolamides (FAEs) metabolism and inflammatory responses. Front. Pharmacol. 12, 817603. doi:10.3389/fphar.2021.817603
Xie, X., Wu, X., Zhao, D., Liu, Y., Du, Q., Li, Y., et al. (2022). Fluvoxamine alleviates bleomycin-induced lung fibrosis via regulating the cGAS-STING pathway. Pharmacol. Res. 187, 106577. the official journal of the Italian Pharmacological Society. doi:10.1016/j.phrs.2022.106577
Zhang, X. K. (2007). Targeting Nur77 translocation. Expert Opin. Ther. Targets 11, 69–79. doi:10.1517/14728222.11.1.69
Zhang, Y., Bi, X., Adebiyi, O., Wang, J., Mooshekhian, A., Cohen, J., et al. (2019). Venlafaxine improves the cognitive impairment and depression-like behaviors in a cuprizone mouse model by alleviating demyelination and neuroinflammation in the brain. Front. Pharmacol. 10, 332. doi:10.3389/fphar.2019.00332
Zhou, M., Peng, B. R., Tian, W., Su, J. H., Wang, G., Lin, T., et al. (2020). 12-Deacetyl-12-epi-Scalaradial, a scalarane sesterterpenoid from a marine sponge hippospongia sp., induces HeLa cells apoptosis via MAPK/ERK pathway and modulates nuclear receptor Nur77. Mar. Drugs 18, 375. doi:10.3390/md18070375
Zhou, Y., Zhao, W., Xie, G., Huang, M., Hu, M., Jiang, X., et al. (2014). Induction of Nur77-dependent apoptotic pathway by a coumarin derivative through activation of JNK and p38 MAPK. Carcinogenesis 35, 2660–2669. doi:10.1093/carcin/bgu186
Zhuo, C., Zhou, C., Cai, Z., Chen, J., Yang, L., Li, Q., et al. (2022). Electrical stimulus combined with venlafaxine and mirtazapine improves brain Ca(2+) activity, pre-pulse inhibition, and immobility time in a model of major depressive disorder in schizophrenia. J. Affect Disord. 319, 610–617. doi:10.1016/j.jad.2022.09.037
Keywords: melanoma, Nur77, venlafaxine, drug repurposing, JNK1/2 kinase
Citation: Niu T, Wei Z, Fu J, Chen S, Wang R, Wang Y and Zheng R (2023) Venlafaxine, an anti-depressant drug, induces apoptosis in MV3 human melanoma cells through JNK1/2-Nur77 signaling pathway. Front. Pharmacol. 13:1080412. doi: 10.3389/fphar.2022.1080412
Received: 26 October 2022; Accepted: 21 December 2022;
Published: 04 January 2023.
Edited by:
Eswar Shankar, The Ohio State University, United StatesReviewed by:
Annapurna Gupta, Comprehensive Cancer Center, United StatesKate Ormiston, The Ohio State University, United States
Copyright © 2023 Niu, Wei, Fu, Chen, Wang, Wang and Zheng. This is an open-access article distributed under the terms of the Creative Commons Attribution License (CC BY). The use, distribution or reproduction in other forums is permitted, provided the original author(s) and the copyright owner(s) are credited and that the original publication in this journal is cited, in accordance with accepted academic practice. No use, distribution or reproduction is permitted which does not comply with these terms.
*Correspondence: Ruihe Zheng, cnVpaGV6aGVuZ0BoYWlubWMuZWR1LmNu, cnVpaGV6aGVuZ0AxMjYuY29t
†These authors have contributed equally to this work