- 1Unit of Neuropharmacology and Translational Neurosciences, Oasi Research Institute-IRCCS, Troina, Italy
- 2Department of Drug and Health Sciences, University of Catania, Catania, Italy
- 3Department of Biomedical and Biotechnological Sciences, University of Catania, Catania, Italy
- 4Bio-nanotech Research and Innovation Tower (BRIT), University of Catania, Catania, Italy
- 5Department of Pharmacological and Biomolecular Sciences, University of Milano, Milan, Italy
- 6Department of Physiology and Pharmacology “Vittorio Erspamer”, Sapienza University of Rome, Rome, Italy
- 7Neurobiology of Behavior Laboratory, Santa Lucia Foundation, Rome, Italy
- 8Department of Clinical and Experimental Medicine, University of Foggia, Foggia, Italy
- 9Department of Life Sciences, University of Modena and Reggio Emilia, Modena, Italy
- 10Biological Psychiatry Unit, IRCCS Istituto Centro San Giovanni di Dio Fatebenefratelli, Brescia, Italy
Stressful experiences early in life, especially in the prenatal period, can increase the risk to develop depression during adolescence. However, there may be important qualitative and quantitative differences in outcome of prenatal stress (PNS), where some individuals exposed to PNS are vulnerable and develop a depressive-like phenotype, while others appear to be resilient. PNS exposure, a well-established rat model of early life stress, is known to increase vulnerability to depression and a recent study demonstrated a strong interaction between transforming growth factor-β1 (TGF-β1) gene and PNS in the pathogenesis of depression. Moreover, it is well-known that the exposure to early life stress experiences induces brain oxidative damage by increasing nitric oxide levels and decreasing antioxidant factors. In the present work, we examined the role of TGF-β1 pathway in an animal model of adolescent depression induced by PNS obtained by exposing pregnant females to a stressful condition during the last week of gestation. We performed behavioral tests to identify vulnerable or resilient subjects in the obtained litters (postnatal day, PND > 35) and we carried out molecular analyses on hippocampus, a brain area with a key role in the pathogenesis of depression. We found that female, but not male, PNS adolescent rats exhibited a depressive-like behavior in forced swim test (FST), whereas both male and female PNS rats showed a deficit of recognition memory as assessed by novel object recognition test (NOR). Interestingly, we found an increased expression of type 2 TGF-β1 receptor (TGFβ-R2) in the hippocampus of both male and female resilient PNS rats, with higher plasma TGF-β1 levels in male, but not in female, PNS rats. Furthermore, PNS induced the activation of oxidative stress pathways by increasing inducible nitric oxide synthase (iNOS), NADPH oxidase 1 (NOX1) and NOX2 levels in the hippocampus of both male and female PNS adolescent rats. Our data suggest that high levels of TGF-β1 and its receptor TGFβ-R2 can significantly increase the resiliency of adolescent rats to PNS, suggesting that TGF-β1 pathway might represent a novel pharmacological target to prevent adolescent depression in rats.
1 Introduction
Major depressive disorder (MDD) is one of the most common mood disorder characterized by affective and cognitive symptoms affecting the life’s quality of patients (Bhatt et al. (2020) with a prevalence of 5.7% in children and 11.3% in adolescents (Bowman and Daws 2019). Recent studies also show that the rates of depression increase after puberty in girls more than in boys (Silberg et al., 1999). Although depression in adolescents could be considered as a familial disorder resulting in approximately 37% of heritability, where genetic factors have a strong role in this transmission, environmental factors play a key role in the development of adolescent depression (Zalsman et al., 2006; Thapar et al., 2012). Several epidemiological studies demonstrate that the exposure to adverse experiences during pregnancy and stressful early life conditions during childhood can increase the vulnerability to depression (Laplante et al., 2008; Vedhara et al., 2012). Nevertheless, not all individuals exposed to prenatal or postnatal stress develop depressive-like phenotypes, but they can exhibit a notable degree of resilience (Russo et al., 2012). Different studies have been conducted in the last ten years to identify the genetic and molecular mechanisms underlying resilience, but discrepancies exist between human and animal studies in this field, and animal models remain an essential tool to investigate which factors may be involved in the stress resilience mechanisms (Russo et al., 2012). According to this scenario, animal models of prenatal stress (PNS) are used to reproduce the effects of early adverse life events in humans during early life and represent a useful tool to examine the long-term effects of stressful events during pregnancy and to identify the molecular mechanisms underlying vulnerability/resilience to depression in adolescence (Maccari et al., 2014).
PNS exposure is known to increase vulnerability to depression in adult rats (Cattaneo et al., 2020) and different studies have demonstrated that adult animals exposed to PNS procedure show depressive-like or anxious-like phenotypes paralleled by hypothalamic-pituitary-adrenal (HPA) axis dysfunction and an impairment of neuronal plasticity mechanisms (Fumagalli et al., 2004; Maccari and Morley-Fletcher 2007; Luoni et al., 2015; Caruso et al., 2019c). Oxidative stress plays a key role in the pathogenesis of depression by the reduction of antioxidant enzymes and by increasing reactive oxygen species (ROS) production (Bajpai et al., 2014; Caruso et al., 2019a; Caruso et al., 2019c; Bhatt et al., 2020). Starting from the evidence obtained in pediatric and adolescent patients with depressive disorders of increased blood levels of oxidative stress markers combined with a reduction in antioxidant factors, such as superoxide dismutase and glutathione peroxidase (Katrenčíková et al., 2021), it can be hypothesized an activation of oxidative and nitrosative stress pathways in animal models of adolescent depression. It has been suggested a strong neurobiological link between early life adverse experiences (e.g., maternal separation and social isolation) and redox state dysfunctions in PNS-induced depression (Schiavone et al., 2013), and also that stress-related conditions are followed by changes in the pro-oxidant/antioxidant ratio in various tissues (Liu et al., 2015; Moniczewski et al., 2015).
Depressed children and adolescents have shown HPA axis dysregulation and higher basal cortisol levels compared to healthy controls (Silberg et al., 1999). This enhanced cortisol secretion is associated with: i) increased oxidative tissue damage in adolescent children with depression (Oravcova et al., 2022); ii) a deficit of serotoninergic system (Hughes et al., 1996) combined with a lower expression/function of the serotonin transporter than in adults and a reduced clinical response to selective serotonin reuptake inhibitors (SSRIs) (Bowman And Daws 2019).
It is also well-known that chronic stress can act as a risk factor for the development of MDD through the impairment of neurotrophins signaling such as brain-derived neurotrophic factor (BDNF) and transforming growth factor-β1 (TGF-β1) (Pittenger and Duman 2008; Guerrera et al., 2020). Adult rats subjected to PNS in utero show a reduction of BDNF levels in the prefrontal cortex (Luoni et al., 2014).
An impairment of TGF-β1 was observed in animal models of depression with cognitive dysfunction (Yu et al., 2011), whereas a reduction of plasma TGF-β1 levels was detected in depressed patients and correlated with depression symptoms severity and treatment resistance (Myint et al., 2005; Rush et al., 2016; Caraci et al., 2018a). We have recently demonstrated a deficit of hippocampal TGF-β1 levels paralleled by a depressive-like phenotype and cognitive impairment in an animal model of amyloid-related depression, whereas no studies have been conducted yet in animal models of adolescent depression (Applebaum and Wilson 1988). Interestingly, recent studies in humans have provided a strong evidence for a key role of TGF-β1 signaling in depression (Cattaneo et al., 2018; Qiu et al., 2021). A first study has examined the transcriptome and miRNome profiles from the hippocampus of adult rats exposed to PNS with transcriptome data obtained from blood mRNA of adult humans exposed to early life trauma, demonstrating a strong interaction between TGF-β1 gene and PNS in the regulation of mechanisms relevant to stress and depression (Cattaneo et al., 2018). A second study analyzed the TGF-β1 SMAD-dependent pathway in the neurodevelopment of children exposed to maternal depression in utero showing that children with a lower gene expression score of TGF-β type I transmembrane receptor (TGF-βRI) exhibited larger amygdala volumes in relation to greater prenatal maternal depressive symptoms (Qiu et al., 2021). An open question remains to understand whether a deficit of TGF-β1 signaling might increase the vulnerability to depression in children and adolescence and, most importantly, whether TGF-β1 signaling might be studied as a new pharmacological target for the prevention or treatment of maternal depression during pregnancy, with a positive effect in the offspring in the context of brain development (Qiu et al., 2021). TGF-β1 plays also a key role in cognitive dysfunction in depression (Caraci et al., 2018b), but it is presently unknown the role of TGF-β1 pathway in animal models of adolescent depression induced by PNS.
Starting from the above evidence, we used an animal model of PNS in pregnancy, obtained by exposing pregnant females to a stressful condition during the last week of gestation, in order to: i) assess whether it can induce the development of a depressive-like phenotype in offspring during adolescence; ii) to identify the molecular mechanisms mediating the vulnerability or resilience to PNS. In particular, we hypothesized that an impairment of the TGF-β1 pathway and oxidative stress increase the vulnerability to adolescent depression induced by PNS. In the present work, we show, for the first time, that an increased response of the TGF-β1 pathway due to PNS can increase the resiliency to adolescent depression.
2 Materials and methods
2.1 Animals
A total of 26 adult male (weight 400 g) and female (weight 250 g) Sprague-Dawley rats were obtained from Envigo RMS s. r.l. laboratories (San Pietro al Natisone, Italy) and group-housed for 8 days after arrival to eliminate stress from shipping. Then, nulliparous female rats were separately housed in the presence of sexually experienced male rats and a vaginal smear was performed daily, by introducing a small amount of saline into the vagina using a pipette and placing drops of the cell suspension onto a slide and by labeling with methylene blue, to check by light microscopy the presence of sperm and to establish the first day of pregnancy. Pregnant female rats, randomly assigned to control and PNS groups, were individually housed with access to food and water ad libitum and maintained in an air-conditioned (23°C ± 1°C) room with constant humidity (60% ± 10%) and a 12 h light/12 h dark cycle.
2.2 PNS model
The experimental design carried out in this study is reported in Figure 1A. We used a PNS model obtained by exposing pregnant dams to a stressful condition during the last week of gestation as previously described (Luoni et al., 2014; Marchisella et al., 2021). Briefly, PNS pregnant female rats were placed into plexiglass transparent cylinders (9 cm diameter, 9 cm height, and 20 cm length) and exposed to bright light (1,500 lux) for 45 min, three times a day (09:00 a.m.–12:00 a.m.–17:00 p.m. ± 2 h), at different periods of the day in order to reduce possible habituation to repeated stress. Control pregnant female rats were left undisturbed in home cages. Male and female offspring (CTRL male n = 29; CTRL female n = 43; PNS male n = 37; PNS female n = 25) were weaned 21 days after birth, randomly housed in the same sex groups of 2 or 3 per cage and maintained under control conditions. Then animals were handled daily before behavioral test sessions.
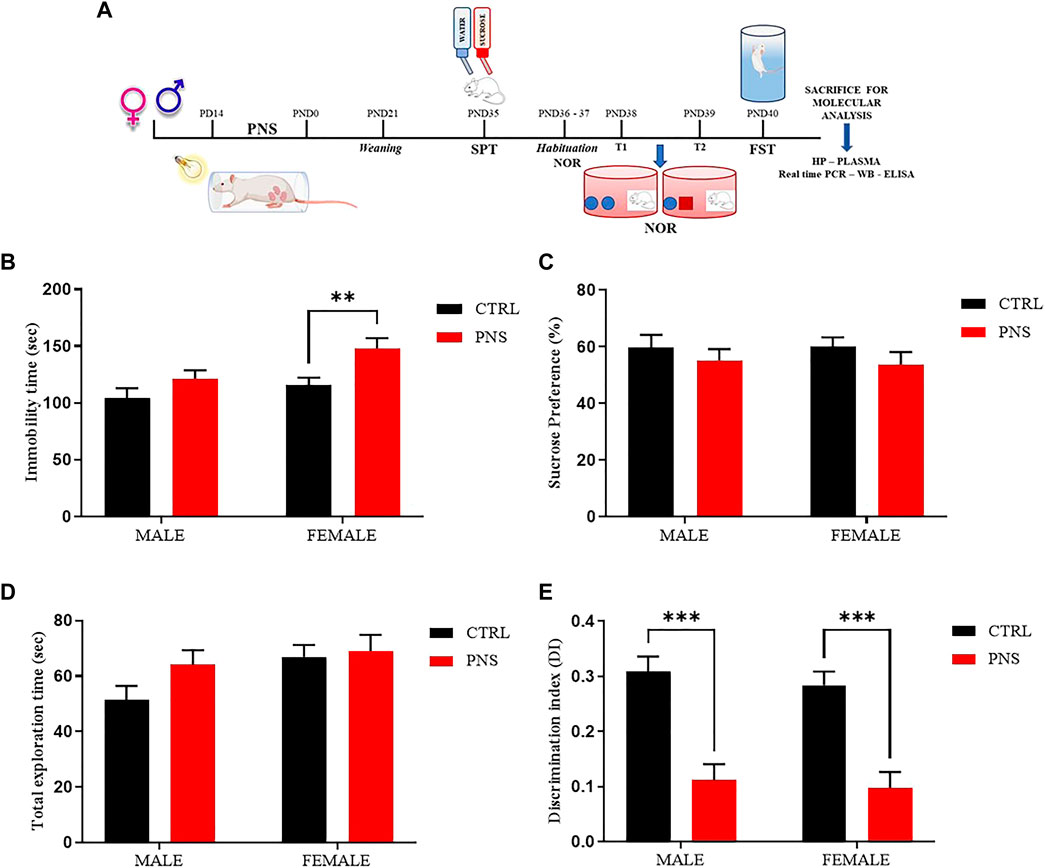
FIGURE 1. PNS induces depressive-like behavior and recognition memory deficits in adolescent rats. (A) Schematic representation of the experimental design. CTRL = Control, PNS = Prenatal Stress, PD = Pregnancy Day, PND = Post Natal Day, SPT = Sucrose Preference Test, NOR = Novel Object Recognition, FST = Forced Swim Test, Real time-PCR = Real time-Polymerase Chain Reaction, WB = Western Blot, ELISA = Enzyme-linked immunosorbent assay, and HP = Hippocampus. (B) Depressive-like behavior assessed in the FST by measuring immobility time (sec) in PNS and CTRL rats. **p < 0.01 vs. CTRL female. (C) Sucrose preference (%) of CTRL and PNS males and female rats. (D) Total Exploration Time and (E) Discrimination Index (D.I) were used to evaluate recognition memory in rats. ***p < 0.001 vs. CTRL groups. All data are shown as mean ± SEM of CTRL male n = 29; CTRL female n = 43; PNS male n = 37; PNS female n = 25.
2.3 Behavioral assessment
We performed specific behavioral tests to assess anhedonia-like behavior, cognitive functions, and depressive-like behavior in male and female offspring during adolescence (PND 35-40) from stressed and control dams in order to identify vulnerable (V) and resilient (R) litters. At the end of behavioral characterization, animals were sacrificed at PND 47 for molecular investigations in hippocampus and plasma in order to identify possible mechanisms underlying the vulnerability and/or the resilience to PNS (Figure 1A).
2.3.1 Sucrose preference test
In order to assess anhedonia-like behavior we performed the Sucrose Preference Test (SPT) as previously described by Marchisella et al. (2021). Briefly, the night before the test (PND 34), adolescent PNS and control rats, habituated to having two water bottles during normal maintenance conditions, were singly housed and water deprived for 12 h. The next day (PND 35), all animals were given 3 h access to one bottle of 1% (wt/vol) sucrose solution and one bottle of regular water. Each bottle was weighed before and after the test. At the end of the test, all animals were group-housed again with food and water ad libitum. Anhedonia-like behavior, as a reduction in sucrose preference ratio relative to control group, was evaluated using the following formula: sucrose preference = (sucrose intake/total intake) *100, with the total intake as the sum of sucrose and water intake (in grams).
2.3.2 Novel object recognition test
The Novel Object Recognition (NOR) test, a paradigm for the investigation of recognition memory in preclinical field, was performed as previously described by Mhillaj et al. (2018). All animals (PND 36-39) were transferred to the test room 30 min prior to the experiment’s start to allow the acclimation. During the NOR test, rats received two 5 min-habituation sessions to explore the circular arena (75 cm diameter, 33 cm height). In the first trial (T1) day test, rats were submitted to a 10-min exposure to two identical (familiar) objects placed in the central part of the arena equally distant from the perimeter, and then animals returned to their home cage. The next day (T2), rats were exposed to one familiar object (F) and to one novel object (N) for 10 min. After each trial, arena and objects were cleaned with a 50% ethanol solution and dried. We waited 10 min to allow the evaporation of ethanol solution, avoiding the presence of possible olfactory cues. The number of exploration seconds of each object was recorded. Exploration was defined as sniffing and touching the object with the nose and quantified as total exploration time = TN (sec) + TF (sec) and discrimination index (D.I.) = (TN – TF)/TN + TF. A reduction in discrimination index ratio relative to control group indicates a recognition memory impairment.
2.3.3 Forced swim test (FST)
In order to evaluate the possible depressive-like behavior caused by PNS procedure in offspring, we performed the Forced Swim Test (FST) in adolescent animals (PND 40) according to the original protocol employed by Porsolt et al. (1978) with slight modifications following the published protocol by Monteggia et al. (2007). All animals were transferred to the test room 30 min prior to the experiment’s start to allow the acclimation. PNS and control rats were placed in a plexiglass transparent cylinder tank containing 2/3 of temperature controlled water (24°C ± 1°C) for a total time of 6 min. Water was changed after each test and tested animals were dried with a clean paper towel and placed underneath a heat source (37°C) in order to restore their body temperature. After an initial habituation period of 2 min, the mobility, defined as any movements other than those necessary to balance the body and keep the head above the water (Cryan et al., 2002), and immobility time was recorded during the last 4 min of the test. An increase in the immobility time relative to control group, evaluated with the following formula: Immobility (sec) = 240 sec– mobility time (sec), indicates a depressive-like behaviour.
2.4 Behavioral z-scoring
To identify vulnerable and resilient rats obtained by PNS procedure we applied the z-normalization (Total z-score), a simple mathematical and integrative tool widely used in clinical and preclinical studies (Guilloux et al., 2011) to measure cognitive and emotional dimensions, generated from mean of every z-score value obtained from different tests. This allows all parameters to be of the same magnitude, so that a possible outlier in the dataset has been transformed to prevent it from being a massive outlier, and thus directly comparable. The benefit of performing this type of normalization is to reduce the behavioral noise related to the use of multiple tests (Torrisi et al., 2021).
Z-score indicates how many standard deviations (σ) an individual observation (X) is above or below the mean of a control group (μ): Z-score = (X–μ)/σ.
After calculating the z-score for each behavioral test, we measured the z-normalization Total z-score for each animal by using the following formula: Total z-score = (z-score SPT + z-score NOR + z-score FST)/(n) behavioral tests, with the aim to classify vulnerable and resilient animals for both emotional and cognitive dimensions. PNS rats were separated into resilient and vulnerable on the basis of the results obtained on three different behavioral tests, which assess depressive- (FST) and anhedonia- (SPT) like behavior and cognitive memory deficit (NOR). A rat was considered to have a PNS- induced depressive-like phenotype when the mean performance resulting from all three different tests differed by ½ standard deviation (SD) from the mean level of the performance obtained by the control group.
2.5 Gene expression analysis by quantitative real-time PCR
The extraction of total RNA from each hippocampal sample was performed as previously described (Marchisella et al., 2021), with slight modifications. Briefly, tissue samples were homogenized in a homogenization buffer, containing sucrose, phenylmethylsulfonyl fluoride, HEPES, MgCl2, NaHCO, cOmplete (protease inhibitor cocktail tablets, EDTA-free, Sigma-Aldrich, 11836170001), phosphatase and RNase inhibitors (Sigma-Aldrich, St. Louis, MO, United States), and sterile H2O, by using ULTRA-TURRAX homogenizer (IKA®-Werke GmbH & Co. KG, Darmstadt, Germany). Once completely homogenized, a half of each sample was used for RNA extraction, while the other half was used for protein extraction.
In order to extract the RNA from each hippocampal sample, homogenized solutions were sonicated, added with PureZOL (Bio-Rad Laboratories, Inc., Milan, Italy), and chloroform, and centrifuged (12,000 rpm at 4°C for 15 min). The aqueous phase was transferred into a new vial and incubated with isopropanol overnight at −20°C. The day after, each solution was centrifuged (12,000 rpm at 4°C for 30 min), the supernatant was discarded, an appropriate quantity of 75% ethanol solution was added followed by centrifugation (12,000 rpm at 4°C for 15 min), the supernatant was discarded, and the pellet was left to dry at room temperature for 5 min. The pellet was then resuspended in an adequate volume of RNase-Free Water (Qiagen, Hilden, Germany).
Total RNA concentration was assessed by using NanoDrop® ND-1000 (Thermo Fisher Scientific, Waltham, MA, United States), while RNA quality was determined using Qubit® 3.0 Fluorometer (Thermo Fisher Scientific) (Fresta et al., 2020a). The reverse transcription of 1 μg of RNA (for each sample) was accomplished by using QuantiTect Reverse Transcription Kit (Qiagen) according to manufacturer instructions, while the quantification of each cDNA sample loaded in a 384-well plate was obtained by employing a LightCycler® 480 System (Roche Molecular Systems, Inc., Pleasanton, CA, United States). The primer sequences (QuantiTect Primer Assays, Qiagen) used for gene expression analysis are shown in Table 1, with the exception of those for NOX1 (forward: 5′-CTT CCT CAC TGG CTG GGA TA-3′; reverse: 5′-CGA CAG CAT TTG CGC AGG CT-3′) (Uchizono et al., 2006) that were purchased by Life Technologies Italia (Monza, Italy). The protocol used to achieve sample amplification, fluorescence data collection, and sample quantification is the same as previously described (Caruso et al., 2019b; Fresta et al., 2020b). Glyceraldehyde-3-phosphate dehydrogenase (GAPDH) and β-actin were selected as the combination of reference genes.
2.6 Western blot analysis
After initial homogenization using a homogenization buffer containing protease (cOmplete, Sigma-Aldrich, 11836170001) and phosphatase inhibitors cocktail (Sigma-Aldrich, P0044), part of each hippocampus sample isolated from PNS and control rats was used for Western Blot analysis as previously described (Fidilio et al., 2021). Briefly, samples were sonicated (10 sec, 3 cycles), centrifuged at 14,000 g at 4°C for 15 min and then the supernatants were collected. Protein concentrations were quantified by using a Pierce™ BCA protein assay kit with bovine serum albumin as a standard (Thermo Fisher Scientific, 23,227), according to the manufacturer’s specifications; subsequently, 20 μg of total proteins were denatured at 95 °C for 10 min, separated by NuPage™ 4%–12% bis–tris gel electrophoresis (Thermo Fisher Scientific, NP0322BOX) and transferred to nitrocellulose membranes. After a blocking step, the membranes were incubated overnight at 4°C with the following primary antibodies: rabbit anti-TGF-β1 (1:500, Abcam, Cambridge, United Kingdom; ab92486), rabbit anti-TGFβ-R2 (1:500, Cell signaling Technology Inc., Danvers, MA, United States; 79,424), rabbit anti-NOX1 (1:1,000, Abcam; ab131088), rabbit anti-NOX2 (1:4,000, Abcam; ab80508), rabbit anti-iNOS (1:500, Abcam; ab136918), and mouse anti-actin (1:1,000, Sigma-Aldrich, A4700) used as housekeeping. Secondary goat anti-rabbit labeled with IRDye 800CW (Li-COR Biosciences; 1:15,000) and goat anti-mouse labeled with IRDye 680LT (Li-COR Biosciences; 1:15,000) were used at room temperature for 60 min in the dark. Hybridization signals were detected using an Odyssey® Infrared Imaging System (LI-COR Biosciences, Lincoln, NE, United States), and the densitometric analysis was performed by Image J software.
2.7 TGF-β1 measurement in plasma samples
In order to measure TGF-β1 levels in plasma samples obtained from vulnerable and resilient rats (both males and females) as well as in control rats, we carried out the enzyme-linked immunosorbent assay (ELISA) in accordance to manufacturer’s instructions (Bio-techne, R&D system, DB100C). After the activation procedure from latent TGF-β1 to the immunoreactive form, plasma samples were assayed at a 1:10 dilution. TGF-β1 standard curve and activated samples were assayed in duplicate. The sensitivity of the ELISA kit, as reported in the data sheet, is 15.4 pg/ml. The optical density of each well was determined using a microplate reader Synergy HT (Agilent BioTek, Santa Clara, CA, United States) set to 450 nm, 540 nm, and 570 nm as suggested by the producer. Data were analyzed subtracting readings at 540 nm from the readings at 450 nm in order to correct the optical imperfections in the plate.
2.8 Statistical analysis
All statistical analyses were carried out by using the software GraphPad Prism®, version 9.0 (GraphPad, La Jolla, CA, United States). Results obtained by behavioral assessment were analyzed using two-way Analysis Of Variance (ANOVA), followed by Bonferroni’s post hoc multiple comparisons, while data from molecular investigations were analyzed by using one-way ANOVA followed by Bonferroni’s post-hoc test. Data were expressed as mean ± Standard Error of Mean (SEM) and only p-values < 0.05 were considered statistically significant. GraphPad Prism®, version 9.0 was also used to identify possible outliers in all experimental groups. The Spearman rank test was used for bivariate correlation analysis between peripheral and central TGF-β1 levels in both male and female PNS rats.
2.9 Study approval
The study was authorized by the Institutional Animal Care and Use Committee (IACUC) of the University of Catania and by the Italian Ministry of Health (DDL 26/2014 and previous legislation; OPBA Project #337/2020; Authorization n. 300/2020-PR). Animal care followed Italian (D.M. 116192) and EEC (O.J. of E.C.L 358/1 12/18/1986) regulations on protection of animals used for experimental and scientific purposes.
3 Results
3.1 PNS exposure induces a depressive-like behavior during adolescence only in females, but causes a deficit in recognition memory in both sexes
To assess if PNS procedure contributes to emotional impairment, FST and SPT were adopted to evaluate a possible depressive- and anhedonia-like behavior, respectively. Figure 1B shows an increase of immobility time in female rats exposed to PNS if compared to the control group (p < 0.01); whereas no significant changes were observed in immobility time in male PNS and CTRL rats. We also explored the anhedonic-like behaviour by employing SPT and we found only a small decrease in sucrose preference, but not statistically relevant, in both male and female PNS rats compared to the control group (Figure 1C).
In order to extend our investigation to the effect of PNS on cognitive dimension, we tested adolescent rats using NOR test, a task based on the natural tendency of rodents to explore unfamiliar objects, which depends upon integrity of the perirhinal cortex, the hippocampus, and the medial temporal lobe (Broadbent et al., 2010). Figure 1E reveals a strong significant reduction of discrimination index values in both male and female PNS rats compared to the control group (p < 0.001), suggesting that PNS animals were not able to keep the information acquired during the T1 training trial and they did not discriminate between the familiar and the novel object compared to control rats. Results were not affected by differences in total exploration time between all experimental animal groups (Figure 1D).
These data show for the first time that PNS exposure induces an impairment of object recognition memory both in male and female adolescent rats and a depressive-like behavior only in adolescent female rats, suggesting that PNS is able to cause a strong deficit in a cognitive dimension, also relevant in humans, and a gender difference in emotional response.
3.2 Characterization of vulnerable and resilient rats exposed to PNS using total z-score
To identify vulnerable and resilient rats exposed to PNS procedure, we analyzed both emotional and cognitive behavioral data using the z-normalization. Z-score values of single behavioral tests indicate that PNS rats showed an increase of immobility time (Figure 2A, male PNS V = 33%; female PNS V = 44%, p < 0.01), a significant reduction in discrimination index (Figure 2C, male PNS V = 65%, p < 0.001; female PNS V = 68%, p < 0.001), without significant changes in sucrose preference intake (Figure 2B), compared to the control group. Conversely, resilient rats exhibited a similar behavioral response to the control animals. After calculating the z-score of FST, SPT, and NOR (Figures 2A,B,C), we applied z-normalization (Total z-score) (Figure 2D) for all behavioral data using the formula: Total z-score = (Z-score FST + Z-score SPT + Z-score NOR)/3 (see above paragraph 2.4). Z-normalization data reveal a slightly higher percentage of vulnerability in female rats than males exposed to PNS procedure (male PNS V = 62%, p < 0.001 vs. CTRL and PNS R; female PNS V = 68%, p < 0.001 vs. CTRL and PNS R). By applying the z-normalization, we obtained n = 23 and n = 14 PNS males V and R, respectively, while n = 17 and n = 8 were obtained in the case of PNS females V and R, respectively (Figure 2D).
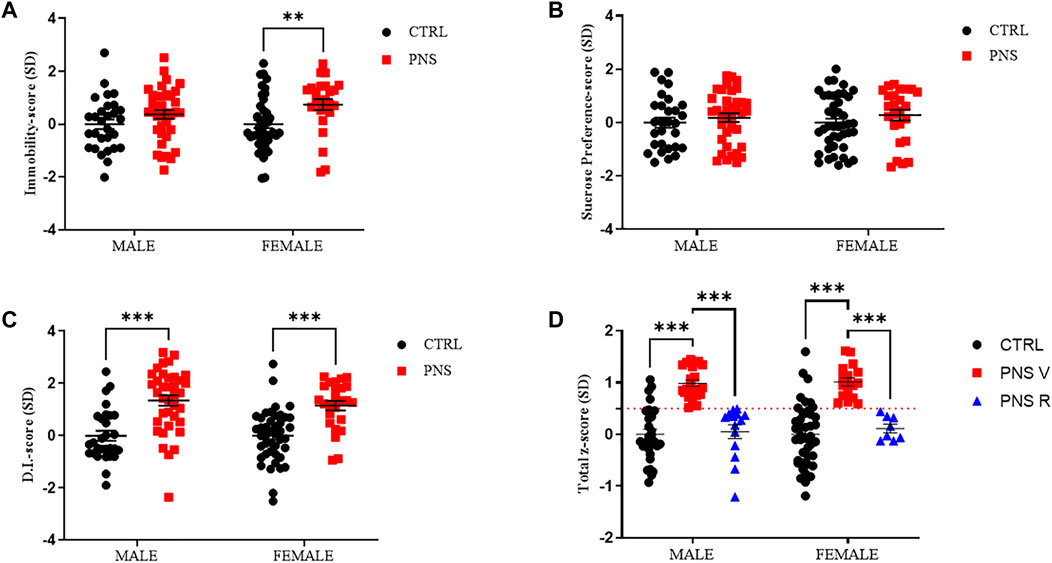
FIGURE 2. Identification of vulnerable and resilient rats by applying z-score method. (A) z-score values in CTRL and PNS male and female adolescent rats in FST. **p < 0.01 vs. CTRL female. (B) z-score values in CTRL and PNS male and female adolescent rats in SPT. (C) z-score values in CTRL and PNS male and female adolescent rats in NOR test. ***p < 0.001 vs. CTRL groups. (D) Total z-score = (Z-score FST + Z-score SPT + Z-score NOR)/3). Male PNS V = 62%, ***p < 0.001 vs. CTRL and PNS R; female PNS V = 68%, ***p < 0.001 vs. CTRL and PNS R.
3.3 Molecular mechanisms underlying vulnerability to depression and memory deficits in PNS rats: A key role of TGF-β1
Starting from the evidence that chronic stress, by impairing TGF-β1 pathway and promoting oxidative stress, can represent a risk factor for the development of depression (Caraci et al., 2018b; Cattaneo et al., 2018; Caruso et al., 2021b), we first examined the role of TGF-β1 signaling in mediating the PNS response by evaluating the mRNA levels of this neurotrophic factor and its receptor TGFβ-R2 in the hippocampus of animals exposed to PNS procedure (Figures 3A–D) in parallel to the analysis of the pro-oxidant enzymes inducible nitric oxide synthase (iNOS), responsible for nitric oxide production (Metto et al., 2013), and NADPH oxidase 1 (NOX1) and 2 (NOX2), responsible for superoxide production (de Campos et al., 2015; Ogboo et al., 2022) (Figures 3E,I).
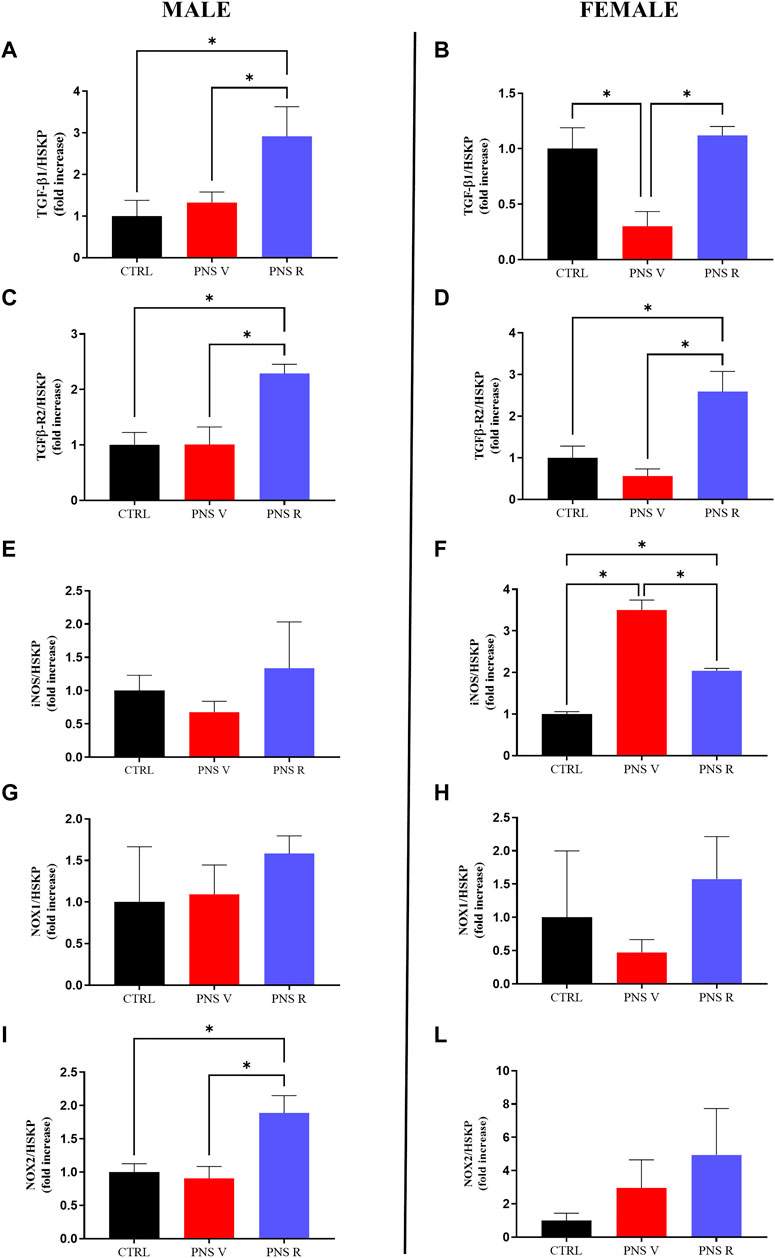
FIGURE 3. Effects of PNS on gene expression in the hippocampus: impact on TGF-β1 pathway and oxidative stress machinery. Effects induced by PNS exposure on (A,B) TGF-β1, (C,D) TGFβ-R2, (E,F) iNOS, (G,H) NOX1, and (I,J) NOX2 mRNAs expression measured by qRT-PCR. The abundance of each mRNA of interest was expressed relative to the abundance of GAPDH/β-actin (HSKP) mRNA, as endogenous controls. As a negative control, a reaction in absence of cDNA (no template control, NTC) was performed. qRT-PCR amplifications were performed at least in triplicate. Data are shown as mean ± S.E.M. *p < 0.05.
Interestingly, we found that PNS exposure reduced the expression of TGF-β1 gene only in the hippocampus of vulnerable female (PNS V) (p < 0.05 vs. CTRL and PNS R), but not in PNS V male, whereas TGF-β1 mRNA levels were comparable to that of controls in resilient female (PNS R) and significantly increased (p < 0.05) compared to controls in resilient male (PNS R) (Figures 3A,B). Recent studies in depressed patients suggest that TGF-β1 receptor genes play a central role in the pathogenesis of child and adolescent depression (Qiu et al., 2021). Interestingly, along this line, we observed, in our model of adolescent depression, significantly increased TGFβ-R2 mRNA levels only in resilient male and female when compared both to controls and vulnerable rats (Figures 3C,D; p < 0.05), suggesting a key role of TGF-β1 pathway in the pathophysiology of adolescent depression.
In our in vivo model we found that PNS induced a significant increase of iNOS mRNA levels in PNS V females compared to controls and PNS R (Figure 3F; p < 0.05). With regard to NOX2 mRNA levels, it was observed that the expression of this gene was significantly increased in PNS R males compared to both controls and PNS V (Figure 3I; p < 0.05). A different effect was observed when considering the mRNA expression levels of NOX2 in female rats, where a trend of increase was observed in PNS V and PNS R compared to controls (Figure 3J). In the case of NOX1, a trend of increase was observed for PNS R males only compared to both PNS V and controls (Figure 3G), while no significant differences in the expression of this gene were detected among the different experimental groups in the case of female rats (Figure 3H).
To better understand the alterations of the TGF-β1 pathway in our model of adolescent depression, we carried out western blot analysis in hippocampus of control and PNS rats, also starting from the well-known evidence that TGF-β1 final activity is regulated not only at a transcriptional level, but also at a post-transcriptional level and primarily through the conversion of latent TGF-β1 to active TGF-β1 by a variety of proteases (Annes et al., 2003). Figure 4 showed a significant increase of TGF-β1 expression in both male and female PNS resilient rats (PNS R) compared to PNS V rats (Figures 4A,B; p < 0.05). Furthermore, we confirmed at a protein level a statistically significant deficit of TGF-β1 in female PNS V compared to both control and PNS R rats (Figure 4B; p < 0.05). When examining the protein levels of TGF-β1 receptor (TGFβ-R2), it was observed a significant increase in the expression of TGFβ-R2 in both male and female PNS R rats (Figures 4C,D) compared to PNS V (p < 0.001 for males; p < 0.05 for females) and control (p < 0.01 for males; p < 0.05 for females) groups. Finally, we examined the levels of active TGF-β1 in the plasma from control and PNS rats and we found an increase of TGF-β1 plasmatic levels only in male PNS V and PNS R rats compared to control group (Figure 4E; p < 0.01). On the contrary, in PNS female rats we observed a reduction, although not statistically significant, of this neurotrophic factor, but not an increase in females PNS R compared to control group (Figure 4F). No correlation was found between peripheral and central TGF-β1 levels in both male and female PNS rats.
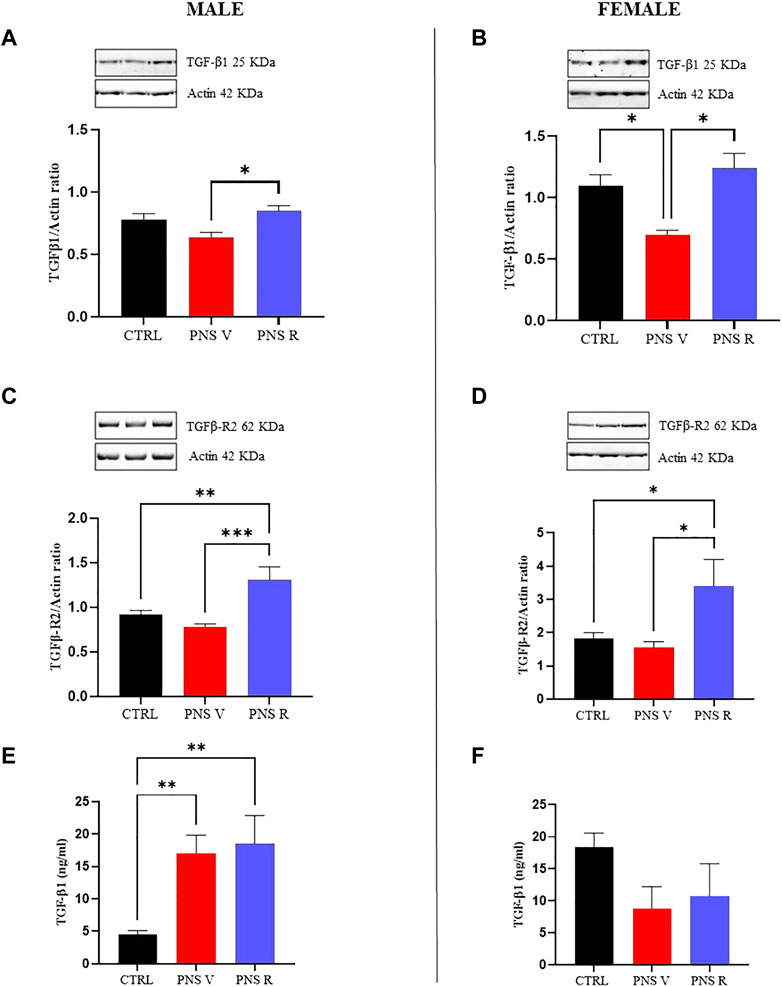
FIGURE 4. Role of TGF-β1 pathway in the mechanisms of vulnerability and resilience to PNS exposure. Effects induced by PNS exposure on TGF-β1 levels and of its receptor TGFβ-R2 in total protein extracts from hippocampus of CTRL and PNS rats evaluated by Western Blot analysis. (A) Representative immunoblot and histogram of TGF-β1 (44 kDa) in CTRL and PNS male adolescent rats (*p < 0.05 vs. PNS V). (B) Representative immunoblot and histogram of TGF-β1 (44 kDa) in CTRL and PNS female adolescent rats (*p < 0.05 vs. CTRL; *p < 0.05 vs. PNS V). (C) Representative immunoblot and histogram of TGFβ-R2 (65 kDa) in CTRL and PNS male adolescent rats (**p < 0.01 vs. CTRL; ***p < 0.001 vs. PNS V). (D) Representative immunoblot and histogram of TGFβ-R2 (65 kDa) in CTRL and PNS female adolescent rats (*p < 0.05 vs. CTRL; *p < 0.05 vs. PNS V). TGF-β1 and TGFβ-R2 densitometric values were normalized against actin used as internal control. Plasmatic levels of TGF-β1 (ng/ml) in male (E) (**p < 0.01 vs. CTRL) and female (F). All data are shown as mean ± SEM of CTRL male n = 10; PNS V male n = 9; PNS R male n = 5; CTRL female n = 10; PNS V female n = 5; PNS R female n = 4.
When we analyzed the oxidative response after PNS procedure and potential changes of pro-oxidant factors in hippocampus of PNS rats, we found an increased expression of NOX1 (Figure 5C, p < 0.05 vs. PNS V and CTRL), and NOX2 (Figure 5E, p < 0.001 vs. PNS V and CTRL) in male PNS R rats while a trend toward increased levels of iNOS protein was observed in the same group compared to both PNS V and controls (Figure 5A). The oxidative stress response to PNS exposure was significantly different in female rats, where we detected high and significantly increased levels of iNOS in both PNS V and PNS R (Figure 5B, p < 0.01 vs. CTRL), whereas both NOX1 (Figure 5D) and NOX2 (Figure 5F) showed a non-significant increase.
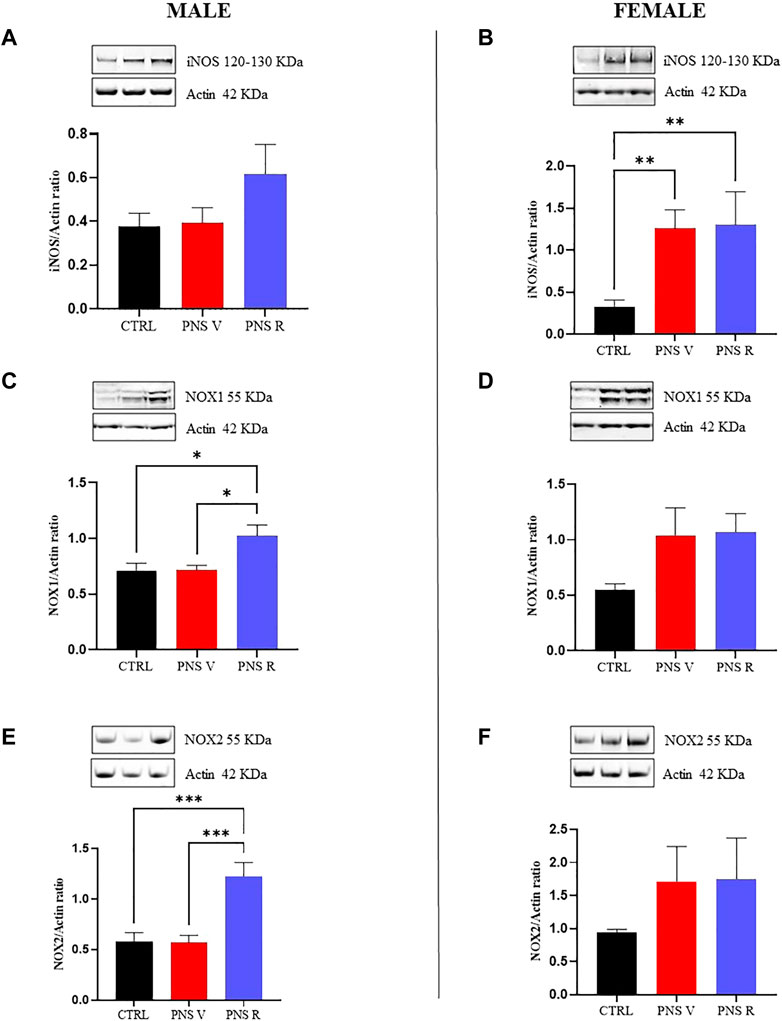
FIGURE 5. Oxidative stress response in vulnerable and resilient PNS rats. Effects induced by PNS exposure on iNOS, NOX1, and NOX2 expression in total protein extracts from hippocampus of CTRL and PNS rats evaluated by Western Blot analysis. Representative immunoblots and histograms of iNOS (120 kDa–130 kDa) in CTRL and PNS male (A) and female (B) adolescent rats (**p < 0.01 vs. CTRL). Representative immunoblots and histograms of NOX1 (55 kDa) in CTRL and PNS male (C) (*p < 0.05 vs. CTRL and PNS V) and female (D) adolescent rats. Representative immunoblots and histograms of NOX2 (55 kDa) in CTRL and PNS male (E) (***p < 0.001 vs. CTRL and PNS V) and female (F) adolescent rats. Densitometric values were normalized against actin used as internal control. All data are shown as mean ± SEM of CTRL male n = 10; PNS V male n = 9; PNS R male n = 5; CTRL female n = 10; PNS V female n = 5; PNS R female n = 4.
4 Discussion
Epidemiological and preclinical studies demonstrated that the exposure to stress or adverse life events during pregnancy increases the risk to neuropsychiatric disorders such as depression in the offspring. Indeed, PNS, especially during pregnancy, can lead to long-term effects in the offspring impacting on emotional, behavioral, and cognitive outcomes and increasing the risk of depression development in early adulthood (Sohr-Preston and Scaramella 2006; Plant et al., 2015; Brooker et al., 2020).
In this study, by employing a validated PNS animal model, we have found for the first time that the exposure to early adverse life events is able to induce a depressive-like phenotype paralleled by memory deficits more evident in adolescent female compared to male rats (Figure 1) and we also examined the percentage of vulnerable and resilient rats detected with the z-normalization, observing that male vulnerable rats are 62% compared to 68% of female vulnerable adolescent rats (Figure 2). Our data are in accordance with other studies reported in literature, where a positive association has been found among female offspring between prenatal maternal stress and cognitive dysfunction during early childhood (Simcock et al., 2017; Sutherland and Brunwasser 2018). Preclinical and clinical data also show that females are more susceptible to several types of stressful events leading to a higher prevalence of depression compared to male subjects (Schmidt et al., 2018; Kalinichenko et al., 2019).
Moreover, by employing the PNS animal model, previous studies have found memory deficits measured by NOR test in male and female rats at adulthood (PND73 and PND80, respectively) (Cattaneo et al., 2019), and the stressful events during the last week of gestation are able to induce in the offspring cognitive deficit, which in females is dependent on the estrous cycle phase (Moura et al., 2020). In our cohort of PNS adolescent male and female rats (PND 35) no differences were observed with regard to sucrose preference among all experimental groups. As recently highlighted by Markov (Markov 2022), this test could give inconsistent results which may be influenced by several factors such as differences in sucrose preference concentration threshold, water and food deprivation, and differences in animals’ susceptibility to stress. We cannot exclude that PNS exposure during pregnancy can impair the responsiveness in our cohort only in adult rats. Future studies are planned to evaluate the onset of anhedonic-like behavior in adult rats.
To understand the molecular mechanisms underlying the vulnerability or resilience to PNS, we focused on neuroinflammatory phenomena in the hippocampus of male and female adolescent rats exposed to PNS because previous studies have shown that early life stress exposure may trigger pro-inflammatory system activation in the brain, an event known to contribute to the development of depression (Ślusarczyk et al., 2016; Caruso et al., 2020). Several studies carried out in animal models of depression have shown increased levels of pro-inflammatory cytokines paralleled by decreased TGF-β1 levels in hippocampus (Torrisi et al., 2019). Furthermore, TGF-β1 signaling in the CNS plays a key role in mediating cellular and behavioral plasticity related to depression and the rescue of TGF-β1 canonical pathway in the hippocampus mediates behavioral effects of antidepressant treatment (Gergues et al., 2021; Mitra et al., 2022). In particular, a selective atrophy of the hippocampus, a brain area essential in the storage/consolidation of short-term memory and learning, has been found in depressed patients compared with healthy controls (Koolschijn et al., 2009), and, similarly, adolescent subjects at a higher risk to develop depression after early life adversity possess smaller hippocampal volumes (Rao et al., 2010). We found for the first time a gender-dependent deficit of hippocampal TGF-β1 levels which seems to contribute significantly to increase the vulnerability to depression, whereas the increased expression of TGF-β1 protein was able to promote resiliency in adolescent male rats (Figure 4). Our data are in agreement with a study carried out by Trojan et al., where a deficit of TGF-β1 gene expression was found in hippocampus and frontal cortex of adult rats previously exposed to PNS during pregnancy, paralleled by anxiety and a depressive-like phenotype, which were normalized by a chronic antidepressant treatment (Trojan et al., 2017).
When considering the differences in gender specific PNS effects on TGF-β1 pathway, but not on oxidative stress, we believe that it may depend on the bidirectional regulation existing between sex hormones and TGF-β1 and its receptor. In fact, it has been already demonstrated that TGF-β1 is involved in gonads and secondary sex organs development, spermatogenesis, and ovarian function (Ingman and Robertson 2002). Interactions exist between follicle-stimulating hormone (FSH), estradiol-17 beta, and TGF-β1 expression and function (Dorrington et al., 1993). Additionally, TGF-β1 secreted by astrocytes can regulate GnRH release via the SMAD-dependent pathway (Galbiati et al., 2005). In this context, it has been recently reported, by using an in vivo model of diabetic nephropathy, that male and female sex hormones seem to influence the TGF-β1/TGF-βR axis by different mechanisms; in particular estradiol via TGFβ-R1 and dihydrotestosterone via TGFβ-R2 (Ziller et al., 2020). Further studies are needed in the PNS model to understand the impact of estradiol-17 beta on TGF-β1 signaling in the brain.
The novelty of the present study stems from the evidence that we identified for the first time the deficit of TGF-β1 pathway as an early event in PNS-induced depression that can be detected in adolescent depression and might then represent a novel pharmacological target to reduce vulnerability to adult depression. Along this line, it has been recently demonstrated that children at 6 years of age with a lower gene expression score of TGFβ-R2 showed larger amygdala volumes in relation to greater prenatal maternal depressive symptoms (Qiu et al., 2021), suggesting a great impact of prenatal stress and prenatal depression on TGF-β1 pathway as a vulnerability factor for child and adolescent depression. In the present work we have focused our attention only on TGF-β1, starting from the evidence that a selective deficit of this cytokine/neurotrophic factor has been found in major depressed patients (Myint et al., 2005), whereas no studies both in humans and animal models of depression show a deficit of TGF-β2. We cannot exclude a role for TGF-β2 in depression, because this neurotrophic factor is expressed in the dentate gyrus and it is also known to modulate serotonin synthesis and metabolism (Chleilat et al., 2019).
In the present study, we planned to increase the translational value of our model by combining the analysis of TGF-β1 pathway in the hippocampus with ELISA assays on plasma samples in the same PNS rats. We found an increase in gene and protein expression levels of TGFβ-R2 in the hippocampus of both male and female resilient rats (Figures 3, 4), whereas at peripheral level we detected an increase of TGF-β1 only in vulnerable and resilient adolescent male rats (Figures 4E,F). Unfortunately, we did not find an increase of plasma TGF-β1 levels in female PNS R rats, suggesting that further studies are needed to understand whether plasma TGF-β1 levels can contribute or not to increase resiliency in female PNS rats.
It is known that the interactions between chronic inflammation and oxidative stress have been implicated in the pathophysiology of depression (Chauhan and Chauhan 2006; Berk et al., 2011; Capuron and Miller 2011). Neuroinflammation can trigger oxidative stress through several mechanisms, such as through the overproduction of free oxygen radicals by activated microglia and astrocytes, and oxidative stress can stimulate several transcription factors to induce a higher release of circulating cytokines (Closa and Folch-Puy 2004; Hayley et al., 2005). Moreover, starting from the evidence of an interaction between early life adverse experience and redox state dysfunctions in PNS-induced mental disorders such as depression (Schiavone et al., 2013), we focused our attention on the potential modulation of pro-oxidant factors in the hippocampus of PNS adolescent rats. In the present study, the modulation of iNOS, NOX1, and NOX2 pro-enzymes, under our experimental conditions, was measured at both gene and protein expression levels since they represent well-demonstrated markers of oxidative stress. In particular, as previously mentioned, iNOS is responsible for nitric oxide production, while NOX1 and NOX2 are responsible for superoxide production. It is also well-known that superoxide anions easily react with NO producing peroxynitrite, an extremely reactive and toxic molecule able to damage the four major classes of biological macromolecules (DNA, carbohydrates, fatty acids and proteins) and mitochondria (Caruso et al., 2021a). We have also recently demonstrated that oxidative stress, taking place as a consequence of pro-oxidant enzymes (i.e., iNOS and NOX2) activation (Caruso et al., 2021b), along with the previously showed deficit of TGF-β1 (Torrisi et al., 2019), is responsible for depressive-like phenotype in an animal model of amyloid-induced depression. We also recently showed as the rescue the TGF-β1 pathway can contribute to prevent amyloid-induced depression and cognitive decline by counteracting oxidative stress. Interestingly, we found that the oxidative stress response to PNS exposure was significantly different in female compared to male rats (Figures 3, 5). Several studies reported that the exposure to early life stress conditions (e.g., maternal deprivation) can influence the brain functions in the offspring and can lead to an increase in oxidative stress at the CNS level (Mumtaz et al., 2018; Safarpour et al., 2021). Furthermore, it has been demonstrated that rats exposed to social isolation at weaning (PND 21) show an increase in NOX2 expression associated with high levels of oxidative stress markers (Schiavone et al., 2009). In this context, we found a strong increase in the expression of iNOS in both PNS V and PNS R female rats compared to control animals (Figures 3F, 5B), (Figures 3E, 5A). In addition, NOX2 showed a significant increase in the hippocampus of male PNS R rats compared to PNS V rats at gene and protein level (Figures 3I, 5E); conversely, NOX1 was significant increased only at protein level in male PNS R rats (Figures 5D,F). These data suggest a prominent activation in the oxidant response following stress exposure in early life and that initial differences in pro-oxidant markers levels may determine the individual characteristic response to chronic stress. In this context, it has been demonstrated that NOX2 gene is under the control of TGF-β1 pathway and activated SMAD signaling (Zhang et al., 2014) and then we can hypothesize that an increased activation of TGF-β1 pathway in male resilient rats can promote the increased expression of NOX2 in PNS R male rats. Further studies in neuronal and glial cell cultures as well as in animal models of depression are needed to validate this hypothesis.
Our study shows some limitations, because it is a correlative study rather than a mechanistic one, and future studies are needed to understand whether TGFβ-R2 silencing or lowering TGF-β1 level can increase susceptibility of rats to PNS-induced depressive like behavior. Further studies are also needed, in the PNS model, to understand the impact of gender and estradiol-17 beta on TGF-β1 signaling in the brain. Our study has analyzed the impact of PNS procedure on adolescent rats, but we cannot exclude possible long-term consequences of exposure to PNS into adulthood. Finally, we did not find a correlation between the central and the peripheral levels of TGF-β1 in both male and female PNS rats. It is also possible that no correlation exists between a central deficit of TGF-β1 after PNS procedure and the reduced levels of this cytokine in the periphery. A recent study conducted in non-human primates, exposed to two consecutive acute confinement stress periods, seems to suggest this hypothesis, with the stress condition able to decrease TGF-β1 concentrations only in cerebrospinal fluid, but not in serum (Coplan et al., 2017).
Overall, our data, obtained in animal model of PNS, indicate that PNS procedure during the last week of gestation can induce in female adolescent rats a depressive-like phenotype combined with recognition memory deficit mimicking both affective and cognitive symptoms observable in adolescent depression. We demonstrated for the first time that an impairment of the TGF-β1 pathway as well as oxidative stress can contribute to increase the vulnerability to adolescent depression induced by PNS and suggest that the rescue of TGF-β1 signaling might represent a new pharmacological strategy to increase resiliency to chronic stress.
Data availability statement
The original contributions presented in the study are included in the article/Supplementary Material, further inquiries can be directed to the corresponding author.
Ethics statement
The animal study was reviewed and approved by Institutional Animal Care and Use Committee (IACUC) of the University of Catania and the Italian Ministry of Health (DDL 26/2014 and previous legislation; OPBA Project #337/2020; Authorization n. 300/2020-PR).
Author contributions
FC gave substantial contributions to the conception and design of the work. AF, MG, GC, AP, and NM performed the experiments. AF, MG, GC, SC, and VB analyzed the data. AF, GML, SAT, SS, and FD participated in the design of the study. FC, MR, AF, MG, and GC drafted the work. All authors approved the version to be published.
Funding
This work was funded by the Italian Ministry of University and Research PRIN 2017 (Program of Relevant National interest–2017AY8BP4004 to FC) and partially supported by RC-2022 (Oasi Research Institute-IRCCS, Troina, Italy).
Acknowledgments
The authors would like to thank the International PhD Program in Neuroscience at University of Catania (AP) and the BRIT laboratory at the University of Catania (Italy) for the valuable technical assistance and use of their laboratories.
Conflict of interest
The authors declare that the research was conducted in the absence of any commercial or financial relationships that could be construed as a potential conflict of interest.
Publisher’s note
All claims expressed in this article are solely those of the authors and do not necessarily represent those of their affiliated organizations, or those of the publisher, the editors and the reviewers. Any product that may be evaluated in this article, or claim that may be made by its manufacturer, is not guaranteed or endorsed by the publisher.
References
Annes, J. P., Munger, J. S., and Rifkin, D. B. (2003). Making sense of latent TGFbeta activation. J. Cell Sci. 116, 217–224. doi:10.1242/jcs.00229
Applebaum, R. A., and Wilson, N. L. (1988). Training needs for providing case management for the long-term care client: Lessons from the national channeling demonstration. Gerontologist 28 (2), 172–176. doi:10.1093/geront/28.2.172
Bajpai, A., Verma, A. K., Srivastava, M., and Srivastava, R. (2014). Oxidative stress and major depression. J. Clin. Diagn. Res. 8 (12), Cc04–07. doi:10.7860/JCDR/2014/10258.5292
Berk, M., Kapczinski, F., Andreazza, A. C., Dean, O. M., Giorlando, F., Maes, M., et al. (2011). Pathways underlying neuroprogression in bipolar disorder: Focus on inflammation, oxidative stress and neurotrophic factors. Neurosci. Biobehav. Rev. 35 (3), 804–817. doi:10.1016/j.neubiorev.2010.10.001
Bhatt, S., Nagappa, A. N., and Patil, C. R. (2020). Role of oxidative stress in depression. Drug Discov. Today 25 (7), 1270–1276. doi:10.1016/j.drudis.2020.05.001
Bowman, M. A., and Daws, L. C. (2019). Targeting serotonin transporters in the treatment of juvenile and adolescent depression. Front. Neurosci. 13, 156. doi:10.3389/fnins.2019.00156
Broadbent, N. J., Gaskin, S., Squire, L. R., and Clark, R. E. (2010). Object recognition memory and the rodent hippocampus. Learn. Mem. 17 (1), 5–11. doi:10.1101/lm.1650110
Brooker, R. J., Kiel, E. J., MacNamara, A., Nyman, T., John-Henderson, N. A., Schmidt, L. A., et al. (2020). Maternal neural reactivity during pregnancy predicts infant temperament. Infancy 25 (1), 46–66. doi:10.1111/infa.12316
Capuron, L., and Miller, A. H. (2011). Immune system to brain signaling: Neuropsychopharmacological implications. Pharmacol. Ther. 130 (2), 226–238. doi:10.1016/j.pharmthera.2011.01.014
Caraci, F., Calabrese, F., Molteni, R., Bartova, L., Dold, M., Leggio, G. M., et al. (2018a). International union of basic and clinical Pharmacology CIV: The neurobiology of treatment-resistant depression: From antidepressant classifications to novel pharmacological targets. Pharmacol. Rev. 70 (3), 475–504. doi:10.1124/pr.117.014977
Caraci, F., Spampinato, S. F., Morgese, M. G., Tascedda, F., Salluzzo, M. G., Giambirtone, M. C., et al. (2018b). Neurobiological links between depression and AD: The role of TGF-β1 signaling as a new pharmacological target. Pharmacol. Res. 130, 374–384. doi:10.1016/j.phrs.2018.02.007
Caruso, G., Benatti, C., Blom, J. M. C., Caraci, F., and Tascedda, F. (2019a). The many faces of mitochondrial dysfunction in depression: From pathology to treatment. Front. Pharmacol. 10, 995. doi:10.3389/fphar.2019.00995
Caruso, G., Benatti, C., Musso, N., Fresta, C. G., Fidilio, A., Spampinato, G., et al. (2021a). Carnosine protects macrophages against the toxicity of aβ1-42 oligomers by decreasing oxidative stress. Biomedicines 9 (5), 477. doi:10.3390/biomedicines9050477
Caruso, G., Fresta, C. G., Grasso, M., Santangelo, R., Lazzarino, G., Lunte, S. M., et al. (2020). Inflammation as the common biological link between depression and cardiovascular diseases: Can carnosine exert a protective role? Curr. Med. Chem. 27 (11), 1782–1800. doi:10.2174/0929867326666190712091515
Caruso, G., Fresta, C. G., Musso, N., Giambirtone, M., Grasso, M., Spampinato, S. F., et al. (2019b). Carnosine prevents aβ-induced oxidative stress and inflammation in microglial cells: A key role of TGF-β1. Cells 8 (1), E64. doi:10.3390/cells8010064
Caruso, G., Grasso, M., Fidilio, A., Torrisi, S. A., Musso, N., Geraci, F., et al. (2021b). Fluoxetine and vortioxetine reverse depressive-like phenotype and memory deficits induced by aβ1-42 oligomers in mice: A key role of transforming growth factor-β1. Front. Pharmacol. 12, 693. doi:10.3389/fphar.2019.00693
Caruso, G., Spampinato, S. F., Cardaci, V., Caraci, F., Sortino, M. A., and Merlo, S. (2019c). β-Amyloid and oxidative stress: Perspectives in Drug development. Curr. Pharm. Des. 25 (45), 4771–4781. doi:10.2174/1381612825666191209115431
Cattaneo, A., Begni, V., Malpighi, C., Cattane, N., Luoni, A., Pariante, C., et al. (2019). Transcriptional signatures of cognitive impairment in rat exposed to prenatal stress. Mol. Neurobiol. 56 (9), 6251–6260. doi:10.1007/s12035-019-1523-4
Cattaneo, A., Cattane, N., Malpighi, C., Czamara, D., Suarez, A., Mariani, N., et al. (2018). FoxO1, A2M, and TGF-β1: Three novel genes predicting depression in gene X environment interactions are identified using cross-species and cross-tissues transcriptomic and miRNomic analyses. Mol. Psychiatry 23 (11), 2192–2208. doi:10.1038/s41380-017-0002-4
Cattaneo, A., Suderman, M., Cattane, N., Mazzelli, M., Begni, V., Maj, C., et al. (2020). Long-term effects of stress early in life on microRNA-30a and its network: Preventive effects of lurasidone and potential implications for depression vulnerability. Neurobiol. Stress 13, 100271. doi:10.1016/j.ynstr.2020.100271
Chauhan, V., and Chauhan, A. (2006). Oxidative stress in Alzheimer's disease. Pathophysiology 13 (3), 195–208. doi:10.1016/j.pathophys.2006.05.004
Chleilat, E., Mallmann, R., Spanagel, R., Klugbauer, N., Krieglstein, K., and Roussa, E. (2019). Spatiotemporal role of transforming growth factor beta 2 in developing and mature mouse hindbrain serotonergic neurons. Front. Cell. Neurosci. 13, 427. doi:10.3389/fncel.2019.00427
Closa, D., and Folch-Puy, E. (2004). Oxygen free radicals and the systemic inflammatory response. IUBMB Life 56 (4), 185–191. doi:10.1080/15216540410001701642
Coplan, J. D., Gopinath, S., Abdallah, C. G., Margolis, J., Chen, W., Scharf, B. A., et al. (2017). Effects of acute confinement stress-induced hypothalamic-pituitary adrenal Axis Activation and concomitant peripheral and central transforming growth factor-β1 measures in nonhuman primates. Chronic Stress (Thousand Oaks) 1, 247054701668869. doi:10.1177/2470547016688693
Cryan, J. F., Markou, A., and Lucki, I. (2002). Assessing antidepressant activity in rodents: Recent developments and future needs. Trends Pharmacol. Sci. 23 (5), 238–245. doi:10.1016/s0165-6147(02)02017-5
de Campos, R. P., Siegel, J. M., Fresta, C. G., Caruso, G., da Silva, J. A., and Lunte, S. M. (2015). Indirect detection of superoxide in RAW 264.7 macrophage cells using microchip electrophoresis coupled to laser-induced fluorescence. Anal. Bioanal. Chem. 407 (23), 7003–7012. doi:10.1007/s00216-015-8865-1
Dorrington, J. H., Bendell, J. J., and Khan, S. A. (1993). Interactions between FSH, estradiol-17 beta and transforming growth factor-beta regulate growth and differentiation in the rat gonad. J. Steroid Biochem. Mol. Biol. 44 (4-6), 441–447. doi:10.1016/0960-0760(93)90248-u
Fidilio, A., Grasso, M., Turnaturi, R., Caruso, G., Spitale, F. M., Vicario, N., et al. (2021). The multimodal MOPr/DOPr agonist LP2 reduces allodynia in chronic constriction injured rats by rescue of TGF-β1 signalling. Front. Pharmacol. 12, 749365. doi:10.3389/fphar.2021.749365
Fresta, C. G., Caruso, G., Fidilio, A., Platania, C. B. M., Musso, N., Caraci, F., et al. (2020a). Dihydrotanshinone, a natural diterpenoid, preserves blood-retinal barrier integrity via P2X7 receptor. Int. J. Mol. Sci. 21 (23), E9305. doi:10.3390/ijms21239305
Fresta, C. G., Fidilio, A., Lazzarino, G., Musso, N., Grasso, M., Merlo, S., et al. (2020b). Modulation of pro-oxidant and pro-inflammatory activities of M1 macrophages by the natural dipeptide carnosine. Int. J. Mol. Sci. 21 (3), E776. doi:10.3390/ijms21030776
Fumagalli, F., Bedogni, F., Perez, J., Racagni, G., and Riva, M. A. (2004). Corticostriatal brain-derived neurotrophic factor dysregulation in adult rats following prenatal stress. Eur. J. Neurosci. 20 (5), 1348–1354. doi:10.1111/j.1460-9568.2004.03592.x
Galbiati, M., Saredi, S., Romanò, N., Martini, L., Motta, M., and Melcangi, R. C. (2005). Smad proteins are targets of transforming growth factor beta1 in immortalised gonadotrophin-releasing hormone releasing neurones. J. Neuroendocrinol. 17 (11), 753–760. doi:10.1111/j.1365-2826.2005.01366.x
Gergues, M. M., Yohn, C. N., Bharadia, A., Levinstein, M. R., and Samuels, B. A. (2021). Dentate gyrus activin signaling mediates the antidepressant response. Transl. Psychiatry 11 (1), 7. doi:10.1038/s41398-020-01156-y
Guerrera, C. S., Furneri, G., Grasso, M., Caruso, G., Castellano, S., Drago, F., et al. (2020). Antidepressant drugs and physical activity: A possible synergism in the treatment of major depression? Front. Psychol. 11, 857. doi:10.3389/fpsyg.2020.00857
Guilloux, J. P., Seney, M., Edgar, N., and Sibille, E. (2011). Integrated behavioral z-scoring increases the sensitivity and reliability of behavioral phenotyping in mice: Relevance to emotionality and sex. J. Neurosci. Methods 197 (1), 21–31. doi:10.1016/j.jneumeth.2011.01.019
Hayley, S., Poulter, M. O., Merali, Z., and Anisman, H. (2005). The pathogenesis of clinical depression: Stressor- and cytokine-induced alterations of neuroplasticity. Neuroscience 135 (3), 659–678. doi:10.1016/j.neuroscience.2005.03.051
Hughes, C. W., Petty, F., Sheikha, S., and Kramer, G. L. (1996). Whole-blood serotonin in children and adolescents with mood and behavior disorders. Psychiatry Res. 65 (2), 79–95. doi:10.1016/s0165-1781(96)02932-0
Ingman, W. V., and Robertson, S. A. (2002). Defining the actions of transforming growth factor beta in reproduction. Bioessays 24 (10), 904–914. doi:10.1002/bies.10155
Kalinichenko, L. S., Kornhuber, J., and Müller, C. P. (2019). Individual differences in inflammatory and oxidative mechanisms of stress-related mood disorders. Front. Neuroendocrinol. 55, 100783. doi:10.1016/j.yfrne.2019.100783
Katrenčíková, B., Vaváková, M., Paduchová, Z., Nagyová, Z., Garaiova, I., Muchová, J., et al. (2021). Oxidative stress markers and antioxidant enzymes in children and adolescents with depressive disorder and impact of omega-3 fatty acids in randomised clinical trial. Antioxidants (Basel) 10 (8), 1256. doi:10.3390/antiox10081256
Koolschijn, P. C., van Haren, N. E., Lensvelt-Mulders, G. J., Hulshoff Pol, H. E., and Kahn, R. S. (2009). Brain volume abnormalities in major depressive disorder: A meta-analysis of magnetic resonance imaging studies. Hum. Brain Mapp. 30 (11), 3719–3735. doi:10.1002/hbm.20801
Laplante, D. P., Brunet, A., Schmitz, N., Ciampi, A., and King, S. (2008). Project ice storm: Prenatal maternal stress affects cognitive and linguistic functioning in 5 1/2-year-old children. J. Am. Acad. Child. Adolesc. Psychiatry 47 (9), 1063–1072. doi:10.1097/CHI.0b013e31817eec80
Liu, T., Zhong, S., Liao, X., Chen, J., He, T., Lai, S., et al. (2015). A meta-analysis of oxidative stress markers in depression. PLoS One 10 (10), e0138904. doi:10.1371/journal.pone.0138904
Luoni, A., Berry, A., Calabrese, F., Capoccia, S., Bellisario, V., Gass, P., et al. (2014). Delayed BDNF alterations in the prefrontal cortex of rats exposed to prenatal stress: Preventive effect of lurasidone treatment during adolescence. Eur. Neuropsychopharmacol. 24 (6), 986–995. doi:10.1016/j.euroneuro.2013.12.010
Luoni, A., Richetto, J., Racagni, G., and Molteni, R. (2015). The long-term impact of early adversities on psychiatric disorders: Focus on neuronal plasticity. Curr. Pharm. Des. 21 (11), 1388–1395. doi:10.2174/1381612821666150105142824
Maccari, S., Krugers, H. J., Morley-Fletcher, S., Szyf, M., and Brunton, P. J. (2014). The consequences of early-life adversity: Neurobiological, behavioural and epigenetic adaptations. J. Neuroendocrinol. 26 (10), 707–723. doi:10.1111/jne.12175
Maccari, S., and Morley-Fletcher, S. (2007). Effects of prenatal restraint stress on the hypothalamus-pituitary-adrenal axis and related behavioural and neurobiological alterations. Psychoneuroendocrinology 32, S10–S15. doi:10.1016/j.psyneuen.2007.06.005
Marchisella, F., Creutzberg, K. C., Begni, V., Sanson, A., Wearick-Silva, L. E., Tractenberg, S. G., et al. (2021). Exposure to prenatal stress is associated with an excitatory/inhibitory imbalance in rat prefrontal cortex and amygdala and an increased risk for emotional dysregulation. Front. Cell Dev. Biol. 9, 653384. doi:10.3389/fcell.2021.653384
Markov, D. D. (2022). Sucrose preference test as a measure of anhedonic behavior in a chronic unpredictable mild stress model of depression: Outstanding issues. Brain Sci. 12 (10), 1287. doi:10.3390/brainsci12101287
Metto, E. C., Evans, K., Barney, P., Culbertson, A. H., Gunasekara, D. B., Caruso, G., et al. (2013). An integrated microfluidic device for monitoring changes in nitric oxide production in single T-lymphocyte (Jurkat) cells. Anal. Chem. 85 (21), 10188–10195. doi:10.1021/ac401665u
Mhillaj, E., Morgese, M. G., Tucci, P., Furiano, A., Luongo, L., Bove, M., et al. (2018). Celecoxib prevents cognitive impairment and neuroinflammation in soluble amyloid β-treated rats. Neuroscience 372, 58–73. doi:10.1016/j.neuroscience.2017.12.046
Mitra, S., Werner, C., and Dietz, D. M. (2022). Neuroadaptations and TGF-β signaling: Emerging role in models of neuropsychiatric disorders. Mol. Psychiatry 27 (1), 296–306. doi:10.1038/s41380-021-01186-y
Moniczewski, A., Gawlik, M., Smaga, I., Niedzielska, E., Krzek, J., Przegaliński, E., et al. (2015). Oxidative stress as an etiological factor and a potential treatment target of psychiatric disorders. Part 1. Chemical aspects and biological sources of oxidative stress in the brain. Pharmacol. Rep. 67 (3), 560–568. doi:10.1016/j.pharep.2014.12.014
Monteggia, L. M., Luikart, B., Barrot, M., Theobold, D., Malkovska, I., Nef, S., et al. (2007). Brain-derived neurotrophic factor conditional knockouts show gender differences in depression-related behaviors. Biol. Psychiatry 61 (2), 187–197. doi:10.1016/j.biopsych.2006.03.021
Moura, C. A., Oliveira, M. C., Costa, L. F., Tiago, P. R. F., Holanda, V. A. D., Lima, R. H., et al. (2020). Prenatal restraint stress impairs recognition memory in adult male and female offspring. Acta Neuropsychiatr. 32, 122–127. doi:10.1017/neu.2020.3
Mumtaz, F., Khan, M. I., Zubair, M., and Dehpour, A. R. (2018). Neurobiology and consequences of social isolation stress in animal model-A comprehensive review. Biomed. Pharmacother. 105, 1205–1222. doi:10.1016/j.biopha.2018.05.086
Myint, A. M., Leonard, B. E., Steinbusch, H. W., and Kim, Y. K. (2005). Th1, Th2, and Th3 cytokine alterations in major depression. J. Affect. Disord. 88 (2), 167–173. doi:10.1016/j.jad.2005.07.008
Ogboo, B. C., Grabovyy, U. V., Maini, A., Scouten, S., van der Vliet, A., Mattevi, A., et al. (2022). Architecture of the NADPH oxidase family of enzymes. Redox Biol. 52, 102298. doi:10.1016/j.redox.2022.102298
Oravcova, H., Katrencikova, B., Garaiova, I., Durackova, Z., Trebaticka, J., and Jezova, D. (2022). Stress hormones cortisol and aldosterone, and selected markers of oxidative stress in response to long-term supplementation with omega-3 fatty acids in adolescent children with depression. Antioxidants (Basel) 11 (8), 1546. doi:10.3390/antiox11081546
Pittenger, C., and Duman, R. S. (2008). Stress, depression, and neuroplasticity: A convergence of mechanisms. Neuropsychopharmacology 33 (1), 88–109. doi:10.1038/sj.npp.1301574
Plant, D. T., Pariante, C. M., Sharp, D., and Pawlby, S. (2015). Maternal depression during pregnancy and offspring depression in adulthood: Role of child maltreatment. Br. J. Psychiatry 207 (3), 213–220. doi:10.1192/bjp.bp.114.156620
Porsolt, R. D., Bertin, A., and Jalfre, M. (1978). Behavioural despair" in rats and mice: Strain differences and the effects of imipramine. Eur. J. Pharmacol. 51 (3), 291–294. doi:10.1016/0014-2999(78)90414-4
Qiu, A., Zhang, H., Wang, C., Chong, Y. S., Shek, L. P., Gluckman, P. D., et al. (2021). Canonical TGF-β signaling regulates the relationship between prenatal maternal depression and amygdala development in early life. Transl. Psychiatry 11 (1), 170. doi:10.1038/s41398-021-01292-z
Rao, U., Chen, L. A., Bidesi, A. S., Shad, M. U., Thomas, M. A., and Hammen, C. L. (2010). Hippocampal changes associated with early-life adversity and vulnerability to depression. Biol. Psychiatry 67 (4), 357–364. doi:10.1016/j.biopsych.2009.10.017
Rush, G., O'Donovan, A., Nagle, L., Conway, C., McCrohan, A., O'Farrelly, C., et al. (2016). Alteration of immune markers in a group of melancholic depressed patients and their response to electroconvulsive therapy. J. Affect. Disord. 205, 60–68. doi:10.1016/j.jad.2016.06.035
Russo, S. J., Murrough, J. W., Han, M. H., Charney, D. S., and Nestler, E. J. (2012). Neurobiology of resilience. Nat. Neurosci. 15 (11), 1475–1484. doi:10.1038/nn.3234
Safarpour, S., Zabihi, E., Ghasemi-Kasman, M., Nosratiyan, N., and Feizi, F. (2021). Prenatal and breastfeeding exposure to low dose of diethylhexyl phthalate induces behavioral deficits and exacerbates oxidative stress in rat hippocampus. Food Chem. Toxicol. 154, 112322. doi:10.1016/j.fct.2021.112322
Schiavone, S., Jaquet, V., Trabace, L., and Krause, K. H. (2013). Severe life stress and oxidative stress in the brain: From animal models to human pathology. Antioxid. Redox Signal. 18 (12), 1475–1490. doi:10.1089/ars.2012.4720
Schiavone, S., Sorce, S., Dubois-Dauphin, M., Jaquet, V., Colaianna, M., Zotti, M., et al. (2009). Involvement of NOX2 in the development of behavioral and pathologic alterations in isolated rats. Biol. Psychiatry 66 (4), 384–392. doi:10.1016/j.biopsych.2009.04.033
Schmidt, M., Braun, K., Brandwein, C., Rossetti, A. C., Guara Ciurana, S., Riva, M. A., et al. (2018). Maternal stress during pregnancy induces depressive-like behavior only in female offspring and correlates to their hippocampal Avp and Oxt receptor expression. Behav. Brain Res. 353, 1–10. doi:10.1016/j.bbr.2018.06.027
Silberg, J., Pickles, A., Rutter, M., Hewitt, J., Simonoff, E., Maes, H., et al. (1999). The influence of genetic factors and life stress on depression among adolescent girls. Arch. Gen. Psychiatry 56 (3), 225–232. doi:10.1001/archpsyc.56.3.225
Simcock, G., Kildea, S., Elgbeili, G., Laplante, D. P., Cobham, V., and King, S. (2017). Prenatal maternal stress shapes children's theory of mind: The QF2011 queensland flood study. J. Dev. Orig. Health Dis. 8 (4), 483–492. doi:10.1017/S2040174417000186
Ślusarczyk, J., Trojan, E., Wydra, K., Głombik, K., Chamera, K., Kucharczyk, M., et al. (2016). Beneficial impact of intracerebroventricular fractalkine administration on behavioral and biochemical changes induced by prenatal stress in adult rats: Possible role of NLRP3 inflammasome pathway. Biochem. Pharmacol. 113, 45–56. doi:10.1016/j.bcp.2016.05.008
Sohr-Preston, S. L., and Scaramella, L. V. (2006). Implications of timing of maternal depressive symptoms for early cognitive and language development. Clin. Child. Fam. Psychol. Rev. 9 (1), 65–83. doi:10.1007/s10567-006-0004-2
Sutherland, S., and Brunwasser, S. M. (2018). Sex differences in vulnerability to prenatal stress: A review of the recent literature. Curr. Psychiatry Rep. 20 (11), 102. doi:10.1007/s11920-018-0961-4
Thapar, A., Collishaw, S., Pine, D. S., and Thapar, A. K. (2012). Depression in adolescence. Lancet 379 (9820), 1056–1067. doi:10.1016/S0140-6736(11)60871-4
Torrisi, S. A., Geraci, F., Tropea, M. R., Grasso, M., Caruso, G., Fidilio, A., et al. (2019). Fluoxetine and vortioxetine reverse depressive-like phenotype and memory deficits induced by aβ(1-42) oligomers in mice: A key role of transforming growth factor-β1. Front. Pharmacol. 10, 693. doi:10.3389/fphar.2019.00693
Torrisi, S. A., Lavanco, G., Maurel, O. M., Gulisano, W., Laudani, S., Geraci, F., et al. (2021). A novel arousal-based individual screening reveals susceptibility and resilience to PTSD-like phenotypes in mice. Neurobiol. Stress 14, 100286. doi:10.1016/j.ynstr.2020.100286
Trojan, E., Ślusarczyk, J., Chamera, K., Kotarska, K., Głombik, K., Kubera, M., et al. (2017). The modulatory properties of chronic antidepressant drugs treatment on the brain chemokine - chemokine receptor network: A molecular study in an animal model of depression. Front. Pharmacol. 8, 779. doi:10.3389/fphar.2017.00779
Uchizono, Y., Takeya, R., Iwase, M., Sasaki, N., Oku, M., Imoto, H., et al. (2006). Expression of isoforms of NADPH oxidase components in rat pancreatic islets. Life Sci. 80 (2), 133–139. doi:10.1016/j.lfs.2006.08.031
Vedhara, K., Metcalfe, C., Brant, H., Crown, A., Northstone, K., Dawe, K., et al. (2012). Maternal mood and neuroendocrine programming: Effects of time of exposure and sex. J. Neuroendocrinol. 24 (7), 999–1011. doi:10.1111/j.1365-2826.2012.02309.x
Yu, T., Guo, M., Garza, J., Rendon, S., Sun, X. L., Zhang, W., et al. (2011). Cognitive and neural correlates of depression-like behaviour in socially defeated mice: An animal model of depression with cognitive dysfunction. Int. J. Neuropsychopharmacol. 14 (3), 303–317. doi:10.1017/S1461145710000945
Zalsman, G., Oquendo, M. A., Greenhill, L., Goldberg, P. H., Kamali, M., Martin, A., et al. (2006). Neurobiology of depression in children and adolescents. Child. Adolesc. Psychiatr. Clin. N. Am. 15 (4), 843–868. doi:10.1016/j.chc.2006.05.010
Zhang, J., Pan, X., Li, N., Li, X., Wang, Y., Liu, X., et al. (2014). Grape seed extract attenuates arsenic-induced nephrotoxicity in rats. Exp. Ther. Med. 7 (1), 260–266. doi:10.3892/etm.2013.1381
Keywords: depression, memory, TGF-β1, oxidative stress, prenatal stress
Citation: Fidilio A, Grasso M, Caruso G, Musso N, Begni V, Privitera A, Torrisi SA, Campolongo P, Schiavone S, Tascedda F, Leggio GM, Drago F, Riva MA and Caraci F (2022) Prenatal stress induces a depressive-like phenotype in adolescent rats: The key role of TGF-β1 pathway. Front. Pharmacol. 13:1075746. doi: 10.3389/fphar.2022.1075746
Received: 20 October 2022; Accepted: 21 November 2022;
Published: 02 December 2022.
Edited by:
Heike Wulff, University of California, Davis, United StatesReviewed by:
Wladyslaw–Lason, Polish Academy of Sciences, PolandKarina S. MacDowell, Universidad Complutense de Madrid, Spain
Copyright © 2022 Fidilio, Grasso, Caruso, Musso, Begni, Privitera, Torrisi, Campolongo, Schiavone, Tascedda, Leggio, Drago, Riva and Caraci. This is an open-access article distributed under the terms of the Creative Commons Attribution License (CC BY). The use, distribution or reproduction in other forums is permitted, provided the original author(s) and the copyright owner(s) are credited and that the original publication in this journal is cited, in accordance with accepted academic practice. No use, distribution or reproduction is permitted which does not comply with these terms.
*Correspondence: Filippo Caraci, carafil@hotmail.com
†These authors have contributed equally to this work and share first authorship