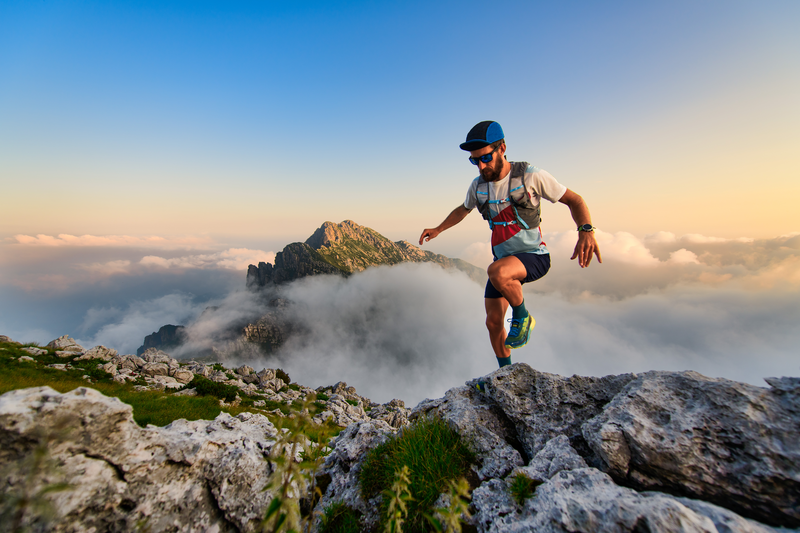
95% of researchers rate our articles as excellent or good
Learn more about the work of our research integrity team to safeguard the quality of each article we publish.
Find out more
REVIEW article
Front. Pharmacol. , 09 January 2023
Sec. Obstetric and Pediatric Pharmacology
Volume 13 - 2022 | https://doi.org/10.3389/fphar.2022.1065243
Polycystic ovary syndrome (PCOS) is one of the most common endocrine diseases in women of reproductive age and features complex pathological symptoms and mechanisms. Existing medical treatments have, to some extent, alleviated the deterioration of PCOS. However, these strategies only temporarily control symptoms, with a few side effects and no preventive effect. Phytochemicals extracted from medicinal herbs and plants are vital for discovering novel drugs. In recent years, many kinds of research have proven that phytochemicals isolated from traditional Chinese medicine (TCM) and medicinal plants show significant potential in preventing, alleviating, and treating PCOS. Nevertheless, compared to the abundance of experimental literature and minimal specific-topic reviews related to PCOS, there is a lack of systematic reviews to summarize these advancements in this promising field. Under this background, we systematically document the progress of bioactive phytochemicals from TCM and medicinal plants in treating PCOS, including flavonoids, polyphenols, and alkaloids. According to the literature, these valuable phytochemicals demonstrated therapeutic effects on PCOS supported by in vivo and in vitro experiments, mainly depending on anti-inflammatory, antioxidation, improvement of hormone disorder and insulin resistance (IR), and alleviation of hyperinsulinemia. Based on the current progress, future research directions should emphasize 1) exploring bioactive phytochemicals that potentially mediate bone metabolism for the treatment of PCOS; 2) improving unsatisfactory bioavailability by using advanced drug delivery systems such as nanoparticles and antibody-conjugated drugs, as well as a chemical modification; 3) conducting in-depth research on the pathogenesis of PCOS to potentially impact the gut microbiota and its metabolites in the evolution of PCOS; 4) revealing the pharmacological effects of these bioactive phytochemicals on PCOS at the genetic level; and 5) exploring the hypothetical and unprecedented functions in regulating PCOS by serving as proteolysis-targeting chimeras and molecular glues compared with traditional small molecule drugs. In brief, this review aims to provide detailed mechanisms of these bioactive phytochemicals and hopefully practical and reliable insight into clinical applications concerning PCOS.
Polycystic ovary syndrome (PCOS) is one of the most common endocrine diseases with a heterogeneous genetic condition in women of reproductive age (15–49 years) (Palomba et al., 2015; Balen et al., 2016; Escobar-Morreale, 2018). Data from the Global Burden of Disease (GBD) in 2019 showed that the incidence of PCOS increased by 30.4% between 1990 and 2019, reaching 66 million cases globally in 2019 (Murray et al., 2020). According to the authoritative Rotterdam criteria formulated by the American Society for Reproductive Medicine (ASRM) and European Society of Human Reproduction and Embryology (ESHRE) in 2003 (Rotterdam ESHRE/ASRM-Sponsored PCOS consensus workshop group), PCOS can be diagnosed with two symptoms of the following three: 1) chronic oligo-anovulation or anovulation; 2) clinical or biochemical hyperandrogenism; and 3) polycystic ovarian morphology. With the development of clinical research on POCS, according to recommendations from the International Evidence-based Guideline for the Assessment and Management of Polycystic Ovary Syndrome from 2018 (Teede et al., 2018), PCOS has been further distinguished into four phenotypes: phenotype A manifested as the excess of androgens, ovulatory dysfunction, and polycystic ovary on ultrasound; phenotype B manifested as the excess of androgens and ovulatory dysfunction; phenotype C manifested as the excess of androgens and polycystic ovary on ultrasound; and phenotype D manifested as ovulatory dysfunction and polycystic ovary on ultrasound. Although the guidelines mentioned above permit the diagnosis of PCOS, the clinical manifestations of PCOS are complex and reference multiple symptoms, such as ovarian enlargement (Azziz et al., 2016; Escobar-Morreale, 2018), hyperandrogenism (Rosenfield and Ehrmann, 2016; Ruth et al., 2020), insulin resistance (Diamanti-Kandarakis and Dunaif, 2012; Azziz et al., 2016), hyperinsulinemia (Housman and Reynolds, 2014; Muscogiuri et al., 2015; Cai et al., 2022), menstrual irregularity (Jayasena and Franks, 2014; Pena et al., 2020), anovulation (Dewailly et al., 2016; Carson and Kallen, 2021) or oligo-anovulation (Hickey et al., 2012; Tay et al., 2020), infertility (Carson and Kallen, 2021), and others. At the same time, PCOS significantly increases the risk of cardiovascular disease (Okoth et al., 2020; O'Kelly et al., 2022), type 2 diabetes (Diamanti-Kandarakis and Dunaif, 2012; Azziz et al., 2016; Zhu et al., 2021), obesity (Lim et al., 2012; Lim et al., 2013; Sermondade et al., 2019), and metabolic disorders (Rosenfield and Ehrmann, 2016; Escobar-Morreale, 2018).
Currently, oral contraceptives, antiandrogens, insulin sensitizers, and ovulation-stimulating drugs are mainly used to treat different symptoms of PCOS. However, these treatments only temporarily control symptoms, with a few side effects and no preventive effect. For example, oral contraceptives aggravate insulin resistance while increasing the risk of inflammation and coagulation disorders in women with PCOS (Manzoor et al., 2019; Okoth et al., 2020). Spironolactone, as a commonly used antiandrogen, plays an antagonistic role by binding to the androgen receptor despite potentially leading to menstrual irregularity and even feminizing male fetuses (Happe et al., 2021; Rashid et al., 2022). Although metformin is universally utilized as an insulin sensitizer in clinical practice, side effects (such as nausea, vomiting, and diarrhea) limit its extensive clinical application (Morley et al., 2017). For infertility caused by PCOS, assisted reproductive technology can be applied as an alternative method. However, ovulation-stimulating drugs may induce poor ovarian response and hyperstimulation syndrome, which limits the clinical application of this technology (La Marca and Sunkara, 2014). Therefore, it is urgent to discover drugs with novel mechanisms and few side effects to solve clinical problems.
Phytochemicals extracted from medicinal herbs and plants are a vital resource for discovering novel drugs (Choudhari et al., 2020; Rahman et al., 2021; Khatoon et al., 2022). In recent years, it has been found that phytochemicals isolated from traditional Chinese medicine (TCM) and medicinal plants (Figure 1) can improve symptoms of PCOS, such as oxidative stress, insulin resistance, hyperinsulinemia, hyperandrogenism, abnormalities in ovarian morphology and function, obesity, anovulation or oligomenorrhea, miscarriage, infertility, and others. Further mechanistic studies showed that these phytochemicals mainly prevent, alleviate, and treat PCOS symptoms through multiple mechanisms (Figure 2). Although the above research progress has laid a critical research foundation for the discovery of new drugs targeting PCOS, there is a lack of systematic reviews (despite the abundance of experimental literature and tiny specific-topic reviews (Mihanfar et al., 2021a) to summarize these advancements in this rapidly developing and promising field. In this context, this review systematically documents the progress of bioactive phytochemicals from TCM and plants in treating PCOS. For the convenience of readers, this review is divided into three parts (flavonoids, polyphenols, and alkaloids) according to the structural characteristics of these valuable phytochemicals.
Although the specific pathological mechanism of PCOS remains unclear, numerous studies have shown that oxidative stress (Murri et al., 2013), insulin resistance (Diamanti-Kandarakis and Dunaif, 2012), and androgen excess (Azziz et al., 2016; Rosenfield and Ehrmann, 2016) play an essential role in the occurrence and development of polycystic ovary syndrome. PCOS can be considered a state of oxidative stress (Merhi et al., 2019). Specifically, the antioxidant function in the human body cannot deal with excessive reactive oxygen species (ROS) (Sies and Jones, 2020; Cheung and Vousden, 2022), which further exacerbates the clinicopathologic features of PCOS, such as chronic oligo-anovulation or anovulation, clinical or biochemical signs of hyperandrogenism, and polycystic ovarian morphology in women. Meanwhile, high levels aggravate the oxidative stress reaction and are often accompanied by insulin resistance (Diamanti-Kandarakis and Dunaif, 2012), worsening PCOS symptoms. In addition, many factors, such as the maternal environment and genetics (Stener-Victorin and Deng, 2021), have influenced the evolution of PCOS. Namely, high prenatal maternal androgen levels cause PCOS in female infants (Filippou and Homburg, 2017). Moreover, recent studies disclose that multiple genes are highly related to PCOS, and data analysis shows that 241 gene mutations are involved in the etiology of PCOS (Joseph et al., 2016). These pathological factors indicate that PCOS is a comprehensive disease referring to multiple signaling pathways and targets.
Recent studies have found that the process of chronic inflammation plays an essential role in the pathogenesis of PCOS (Rudnicka et al., 2021). Numerous studies have shown that PCOS patients have higher inflammatory markers, such as TNF-α, IL-6, IL-1β, IL-18, and C-reactive protein (CRP) (Ganie et al., 2019; Liu et al., 2021). The nuclear factor κB (NF-κB) pathway and phosphatidylinositol 3-kinase (PI3K)/AKT serine (Akt) pathway are associated with high levels of inflammatory markers. It has been validated that the upregulation of these pathways is related to the mechanism of PCOS (Zhao et al., 2015). The higher activity of proinflammatory processes in adipocytes is related to insulin resistance (Zatterale et al., 2020). Therefore, obesity, inflammatory factors, and insulin resistance will jointly affect the occurrence and development of PCOS. Obesity is a symptom most PCOS patients face (de Zegher et al., 2018), and overweight and obese women have lower ovulation rates, conception rates, pregnancy rates, and live birth rates (Gao et al., 2021). Moreover, obesity can promote the molecular mechanism of androgen expression, which may be the cause of obesity leading to PCOS (Rangel et al., 2021). In addition, the latest studies have revealed that the gut microbiota is also connected with the pathogenesis of PCOS (Qi et al., 2019). Studies have shown that the abundance of Firmicutes and Bacteroides in the gut microbiota of PCOS patients is changed. As beneficial bacteria, Lactobacilli and Bifidobacteria can regulate the levels of sex hormones, manage the synthesis and secretion of insulin, and reduce the production of proinflammatory cytokines (He and Li, 2020; He et al., 2020; Yurtdas and Akdevelioglu, 2020). All these studies prove that restoring the abundance of some gut microbiota can be used as a new strategy to relieve PCOS symptoms.
Flavonoids are a class of essential and valuable compounds frequently found in nature and demonstrate a variety of physiological activities, such as anti-inflammatory (Choy et al., 2019; Maleki et al., 2019), antioxidation (Shen et al., 2022), hypoglycemic (Shamsudin et al., 2022), antiviral (Ninfali et al., 2020; Badshah et al., 2021), and antitumor (Sun et al., 2022) activities. This part mainly summarizes the pharmacological effects of quercetin, soy isoflavones, naringenin, baicalin, apigenin, luteolin, rutin, and anthocyanins on PCOS (Table 1).
Quercetin (3,5,7,3′,4′-pentahydroxyflavone) is a common dietary flavonoid in vegetables and fruits. In recent years, quercetin has been widely studied because of its antioxidation, anti-inflammatory, antitumor, hypoglycemic, cardiovascular protection, and other effects in regulating ovarian function and protecting ovarian morphology. Through network pharmacology and bioinformatics, Li et al. found that quercetin, as the core ingredient of the Kuntai capsule (a proprietary Chinese medicine), overlaps with potential therapeutic targets in treating PCOS (Li et al., 2022). Hong et al. speculated that the phenolic ring B of quercetin reduced the activity of steroidogenic enzyme 3β-hydroxysteroid dehydrogenase (3β-HSD) and/or 17β-hydroxysteroid dehydrogenase (17β-HSD), thus effectively relieving the symptoms of the metabolic disorder (Hong et al., 2018). In addition, quercetin decreases the expression of the 17α-hydroxylase/C17-20-lyase (CYP17; Cyp17a1) gene to reduce the activity of its enzyme (the critical enzyme that converts progesterone to androgens) (Shah and Patel, 2016) and regulates the state of androgen receptor (AR) binding to the specific sequence of C-type natriuretic peptide (CNP)/natriuretic peptide receptor 2 (NPR2) gene promoters (Zheng et al., 2022), thereby restoring the normal meiosis of oocytes and improving the state of high androgen and anovulation in patients with PCOS. In addition to high androgen levels, Khorchani et al. found that the overexpression of aromatase is associated with an increase in estrogen levels. In contrast, estrogen inhibits the secretion of gonadotropin-releasing hormone (GnRH) and follicle-stimulating hormone (FSH) through negative feedback, leading to decreased oogenesis (Khorchani et al., 2020). Quercetin, functionally similar to estradiol, can reduce the expression of aromatase and the estrogen level, effectively increasing oogenesis (Khorchani et al., 2020). Estrogen receptor α (ERα) plays a vital role in fertility. Neisy et al. found that the ERα gene can be increased by quercetin. Compared with the control group, the expression of the ERα gene reached five times higher in the rats treated with quercetin, which provided a method for treating infertility caused by PCOS (Neisy et al., 2019).
Obesity is one of the crucial factors attributed to PCOS. Khorchani et al. reported that low expression of the Nesfatin-1 and AdipoR1 genes was associated with obesity, and treatment with quercetin increased the expression of the Nesfatin-1 and AdipoR1 genes, showing a good effect on weight loss and other symptoms caused by obesity (Khorchani et al., 2020). Adiponectin is an essential human adipose factor controlled by the receptors AdipoR1 and AdipoR2, which can metabolize glucose and fatty acids by stimulating AMP-activated protein kinase (AMPK) (Rezvan et al., 2018). The level of adiponectin is low in PCOS with or without adiposity. Clinical data showed that quercetin (1 g daily for 12 weeks) improved adiponectin-mediated IR and metabolic disorder by increasing total adiponectin and high molecular weight adiponectin and reducing resistin, which promoted IR and hyperinsulinemia (Khorshidi et al., 2018). Furthermore, Rezvan et al. revealed that by activating the adiponectin pathway and AMPK in peripheral blood mononuclear cells (PBMCs), quercetin improved the metabolic pathway and the expression of adipokines (Rezvan et al., 2018). Moreover, the imbalance of AMPK-SIRT-1 (as a metabolic sensor) is highly related to IR, weight gain, and fat formation. Mihanfar et al. reported that quercetin could activate the expression of AMPK-SIRT-1 protein, thus increasing insulin sensitivity and improving other symptoms of PCOS (Mihanfar et al., 2021b).
Oxidative stress is another prominent pathological feature of PCOS. Research has found that quercetin enhances the levels of superoxide dismutase (SOD), catalase (CAT), glutathione peroxidase (GPX), glutathione s-transferase (GST), glutathione (GSH), and glutathione reductase (GR) in menopausal female Sprague‒Dawley (SD) rats to reverse the changes in ovarian morphology induced by oxidative stress (Hong et al., 2018; Wang et al., 2018). In addition, quercetin also has promising potential to alleviate IR. Neisy et al. discovered that quercetin increased insulin sensitivity and restored glucose homeostasis by restoring the activities of hexokinase (HK) and glucokinase (GK) in the liver and the expression of the intrauterine glucose transporter type 4 (GLUT4) gene (Neisy et al., 2019). Later, Wang et al. found that quercetin reduced the levels of the inflammatory cytokines IL-1β, IL-6, and TNF-α by regulating the oxidized low-density lipoprotein (OX-LDL)/Toll-like receptor 4 (TLR-4)/nuclear factor κB (NF-κB) pathway, improving the inflammatory microenvironment of ovarian tissue and reversing IR in dehydroepiandrosterone (DHEA)-induced PCOS rats (Wang et al., 2017).
Soy isoflavones are a kind of secondary metabolite formed in the growth of soy and display diverse bioactivities. In recent years, soybean isoflavones have attracted much attention due to their antioxidation, anti-inflammatory, and cholesterol-lowering properties. Farkhad et al. found that treatment with soy isoflavones could reduce the total oxidative state (TOS) and inflammatory cytokines IL-6, IL-12, IL-1β, and TNF-α in estradiol valerate (EV)-induced PCOS rats and increase the total antioxidant capacity (TAC) (Farkhad and Khazali, 2019). Their report revealed that soy isoflavones reduced the number of cystic follicles and the outer layer of theca cells (Lan et al., 2017). In addition, Ma et al. found that soy isoflavones regulated the morphology and function of ovaries by inhibiting the NF-κB pathway to achieve antioxidation and anti-inflammatory effects, improving hormone disorders in PCOS rats (Ma et al., 2021). Aromatase is a critical enzyme in converting androgen to estrogen, and insufficient aromatase activity may contribute to excessive androgen accumulation. Soy isoflavone decreased testosterone concentration by increasing aromatase activity and improving hyperandrogenism in letrozole-induced PCOS rats (Rajan et al., 2017). Clinical data studies showed that soy isoflavones (50 mg daily for 12 weeks), a reduction in BMI, blood glucose, insulin resistance markers (such as insulin), total serum testosterone, sex hormone-binding globulin (SHBG), free androgen index, triglyceride, VLDL-cholesterol, and malondialdehyde were observed, as well as increases in GSH, SOD, and GPX (Jamilian and Asemi, 2016; Karamali et al., 2018). These experiments indicated that soy isoflavones have beneficial effects on IR, hormone disorders, and oxidative stress related to PCOS. For gut microbiota, Liyanage et al. have found that letrozole-induced PCOS rats with soy isoflavones and resistant starch can restore the menstrual cycle and improve the polycystic ovary through the genus-level of gut microbiota returned to normal (Liyanage et al., 2021). However, the poor effect of soy isoflavone used exclusively was documented, which implied that soy isoflavone could be applied as an auxiliary drug to regulate gut microbiota (Liyanage et al., 2021). In contrast to the above studies, Patisaul et al. have shown that lifetime exposure to a soy diet containing endocrine-active phytoestrogens could induce the symptoms of PCOS caused by endocrine-disrupting compounds (EDCs) (Patisaul et al., 2014). Therefore, attention should be given to this phenomenon in treating PCOS.
Naringin, one of the valuable flavonoids widely found in traditional Chinese medicine, possesses a variety of pharmacological activities, such as anti-inflammation and antioxidation. Due to the existing B-ring moiety, naringin can reduce the activities of steroidogenic enzymes 3β-HSD and 17β-HSD in the letrozole-induced PCOS rats and improve the symptoms of hormone disorder (Hong et al., 2019). Additionally, naringin can enhance the bioactivities of SOD, CAT, and GPX while scavenging ROS and relieving the oxidative stress of PCOS (Hong et al., 2019). Wu et al. found that naringin could affect the level of sex hormones, reduce IR, alleviate chronic inflammation and maintain normal ovarian morphology and function by activating the AMPK/SIRT-1/PGC-1α signaling pathway and regulating the composition of intestinal flora (Wu et al., 2022). Furthermore, Yang et al. disclosed that naringin combined with morin could inhibit the proliferation of human endometrial adenocarcinoma cells. In their study, naringin inhibited PCOS-induced endometrial hyperplasia and regulated autophagy by blocking the mammalian target of rapamycin complex 1 (mTORC1)/mammalian target of rapamycin complex 2 (mTORC2) signaling, and morin increased the levels of Caspase-3 and LC3-phosphatidylethanolamine conjugate (LC3-II) (Yang et al., 2022). In addition, naringin could change the elevated serum insulin level and maintain the typical morphology of the ovary in rats with PCOS (Wu et al., 2022). Undoubtedly, naringin shows specific therapeutic potential in insulin-resistant PCOS.
Scutellaria baicalensis Georgi has been used for thousands of years in Asia. As an effective flavonoid isolated from Scutellaria baicalensis Georgi, baicalin has a wide range of pharmacological activities, such as anti-inflammatory, antitumor, and antioxidation activities. Overexpression of 3β-hydroxysteroid dehydrogenase type II (HSD3B2) in ovarian tissue or adrenal cortex leads to excessive androgen synthesis and results in PCOS (Yu et al., 2019). In NCI-H295R cells and the DHEA-induced PCOS rat model, Yu et al. discovered that baicalin could improve the hyperandrogenism of PCOS by destroying the binding of the HSD3B2 gene to the promoter GATA1 and reducing the expression of ovarian HSD3B2, resulting in reduced testosterone secretion (Yu et al., 2019). Additionally, previous studies have shown that AMPK, a sensor of cellular energy changes and a key enzyme in regulating glucose and lipid metabolism, participates in the synthesis and oxidative decomposition of glucose and fatty acids (You et al., 2018). Wang et al. reported that baicalin increased AMPK to inhibit the PI3K/Akt signaling pathway and reduce the protein level of 5α-R1 in ovarian tissue, contributing to increasing insulin sensitivity and regulating androgen levels (reducing the hormone levels of free testosterone, total testosterone, luteinizing hormone (LH), FSH, progesterone, and estradiol). Likewise, baicalin decreased TNF-α, IL-1β, and IL-18 and increased IL-10, resulting in the amelioration of inflammation by proinflammatory and anti-inflammatory cytokines in ovarian tissues (Wang et al., 2019).
Apigenin is a natural flavonoid from many sources, such as fruits and vegetables, with anti-inflammatory, antioxidation, and antitumor activities. Darabi et al. revealed that apigenin could reduce testosterone, estrogen, and LH/FSH levels in EV-induced PCOS rats, normalizing the hormone levels (Darabi et al., 2020). Moreover, they speculated that the observed decrease in hormone levels might be achieved by reducing the activity of aromatase and its 17β-hydroxysteroid dehydrogenases. PCOS is a chronic inflammatory disease highly related to oxidative stress. The experiment showed that apigenin reduced TNF-α and IL-6, which may be accomplished by reducing the expression of the NF-κB transcription factor gene (Darabi et al., 2020). Peng et al. found that apigenin could decrease the TOS and increase the TAC and the activities of SOD, CAT, peroxidase (POD), nicotinamide adenine dinucleotide phosphate (NADPH), and glutathione reductase (GR), thus inhibiting oxidative stress (Peng et al., 2022). As a result, the antioxidant effect of apigenin restored ovarian tissue, increased the number of healthy follicles, and contributed to regaining the pleura of follicles (Darabi et al., 2020; Peng et al., 2022).
Luteolin is a natural flavonoid in fruits, vegetables, and TCM used to treat hypertension, diabetes, cancer, and allergies. It is generally accepted that IR, which is closely related to hyperinsulinemia, is one of the pathogenic mechanisms of PCOS. The abundance of insulin receptor substrate (IRS) is related to decreased PI3K activity in women with PCOS (Chahal et al., 2021). The phosphorylation of IRS in PCOS results in the dysfunction of PI3K/Akt transduction, eventually leading to exaggerated IR and disturbance of glucose homeostasis. Luteolin showed significant glucose homeostasis recovery and IR improvement in letrozole-induced PCOS rats by regulating PI3K/Akt transduction (Huang and Zhang, 2021). Additionally, PCOS patients always showed a state of oxidative stress. The decrease in antioxidants, such as SOD, CAT, GSH, GPX, and glutathione peroxidase (GSH-Px), and the increase in ROS affected the ovary’s physiological functions, such as ovulation, folliculogenesis, and oocyte maturation. Moreover, Nrf2 is a transcription factor mediating the antioxidant pathway. Huang et al. reported that luteolin exerted antioxidative effects by activating Nrf2 to promote the expression of heme oxygenase-1 (Hmox1) and quinone oxidoreductase 1 (NQO1), as well as increasing the levels of CAT, GPX, SOD, GSH, and GSH-Px. As a result, reduced oxidative stress and restored ovarian function were observed in ovarian granulosa cells of rats with letrozole-induced PCOS (Huang and Zhang, 2021).
Rutin is a flavonol compound from many sources with antioxidative, anti-inflammatory, and hypoglycemic effects. Dyslipidemia, IR, hormonal imbalances, and oxidative stress often exist in PCOS. Rutin can restore lipid profiles by reducing the expression levels of adipogenic genes (Han et al., 2016). For IR, rutin could enhance the activity of insulin-dependent receptor kinase and increase the expression of GLUT4, thus increasing glucose uptake, controlling blood sugar, and reducing the risk of diabetes in patients with PCOS (Jahan et al., 2016). The function and morphology of aberrant adipose tissue in PCOS patients are closely related to IR. BAT (brown adipose tissue) can participate in whole-body metabolic homeostasis through uncoupling protein 1 (UCP1)-mediated thermogenesis and secretory cytokines such as adiponectin. Previous research experimentally showed that BAT transplantation in DHEA-induced PCOS rats improved lipid metabolism and reversed anovulation, hyperandrogenism, and polycystic ovaries (Yuan et al., 2016). However, BAT transplantation is unfeasible in clinical applications. Moreover, rutin could activate BAT, increase the expression of UCP1 to increase energy expenditure, and upregulate adiponectin expression in BAT, thereby improving adiposity and IR in DHEA-induced PCOS rats (Hu et al., 2017). Treatment with rutin activated ovarian steroidogenic enzymes such as P450C17, aromatase, 3β-HSD, 17β-HSD, and STAR at normal levels and improved the hormonal imbalances of PCOS rats (Hu et al., 2017). Furthermore, rutin reduced ROS accumulation and improved oxidative stress in PCOS rats. As a rutin derivative, troxerutin was capable of reducing the expression of GnRH (as the master hormone), reducing the secretion of LH and testosterone, and improving the reproductive endocrine dysfunction of 5α-DHT-induced PCOS rats (Gao et al., 2020).
Crocus sativus (saffron) possesses anti-inflammation, antioxidation, and antitumor properties in the clinical application of TCM. Anthocyanins belong to the flavonoid family and are used to alleviate diabetes and control obesity, which contribute to PCOS. Moshfegh et al. utilized saffron petal anthocyanins (SPA) to treat testosterone-induced PCOS mice and disclosed that SPA could improve the hormone disorder, which was evidenced by the recovery of serum levels of LH, FSH, and steroid hormones (estrogen, progesterone, and testosterone) in mice (Moshfegh et al., 2022). In addition, SPA reduced the levels of the inflammatory cytokines TNF-α, IL-6, IL-1β, IL-18, and C-reactive protein (CRP), indicating that SPA demonstrated an anti-inflammatory effect by reducing the mRNA levels of inflammatory genes and restoring NF-κB and inhibitor of NF-κB (IκB) (key mediators of inflammatory genes), resulting in improvement of the inflammatory response of PCOS mice (Moshfegh et al., 2022). In addition, SPA showed antioxidation, which was reflected in the increased levels of GPX, SOD, CAT, GST, and GSH enzymes in the plasma of PCOS mice after SPA treatment. Subsequently, these results ensured that the number of follicles and corpus luteum increased, and the number of cystic follicles decreased in PCOS mice (Moshfegh et al., 2022).
Polyphenols play a restricted role in modern medicine because of their poor bioavailability (Dei Cas and Ghidoni, 2019; Zhang et al., 2020a). However, polyphenols possess significantly beneficial effects in treating PCOS, which has been confirmed in recent studies (Mirabelli et al., 2020; Mihanfar et al., 2021a). This section mainly focuses on the pharmacological effects of polyphenols such as resveratrol, curcumin, catechins, cinnamon, gallic acid, and mangiferin on PCOS (Table 2).
PCOS often presents with hyperandrogenism, oxidative stress, and infertility, accompanied by cardiovascular diseases and others. Resveratrol (RVT) is a polyphenolic compound derived from peanuts, grapes, and other plants featuring broad biological functions, such as antioxidation, anti-inflammatory, and cardiovascular protective effects.
Hyperandrogenism in PCOS induces the expression of VEGF (highly related to ovarian hyperstimulation syndrome) and leads to abnormal angiogenic irregularities in ovaries (Herr et al., 2015). Ortega and Bahramrezaie et al. illustrated that RVT improved irregular angiogenesis by reducing the expression of VEGF mRNA, VEGF protein, and the intermediate factor HIF1 to improve disorders of ovulation, subfertility, and endometriosis caused by ovarian hyperstimulation syndrome (Ortega et al., 2012b; Bahramrezaie et al., 2019). The high testosterone secreted by the ovary and high DHEA secreted by the adrenal gland could induce hyperandrogenism. Banaszewska et al. found that RVT reduced the levels of testosterone and DHEA in serum and affected the production of androgen in the ovary and adrenal glands, thus improving hyperandrogenism in PCOS (Banaszewska et al., 2016). Further study showed that CYP17/Cyp17a1 was the key rate-limiting enzyme in androgen biosynthesis. RVT reduced androgen production by inhibiting the Akt/PKB signaling pathway and reducing the protein expression of CYP17/Cyp17a1 in theca-interstitial cells (Ortega et al., 2012a; Marti et al., 2017). Moreover, Israel et al. confirmed that RVT, combined with simvastatin (a drug that inhibits steroidogenesis in theca-interstitial cells), could effectively reduce steroidogenesis by inhibiting the mevalonate pathway and Akt/PKB phosphorylation (Ortega et al., 2014).
Hyperandrogenism also induces ovarian oxidative stress (OS) and fibrosis in PCOS rats, leading to infertility. Wang et al. reported that RVT, as a SIRT-1 agonist, mediated deacetylation of the 66-kDa Src homology 2 domain-containing protein (p66Shc) while reducing the production of ROS and fibrotic factors, resulting in improved ovarian oxidative stress and fibrosis (Wang et al., 2020). Endoplasmic reticulum stress (ERS) in granulosa cells (GCs) is related to chronic inflammation and oxidative stress in PCOS. Studies have shown that RVT can regulate ERS in GCs by altering the expression of genes involved in the unfolded protein response (UPR) process, reducing the levels of proinflammatory factors, such as IL-6, IL-1β, TNF-α, IL-18, and CPR, and contributing to the amelioration of inflammation and oxidative stress (Ghowsi et al., 2018; Brenjian et al., 2020). Rencber et al. further disclosed that RVT could not only reduce the level of these factors by activating the AMPK-SIRT-1 pathway but also downregulate the levels of anti-Müllerian hormone (AMH), T, LH, and LH/FSH, giving rise to ameliorate the hormone disorders of PCOS and the structure of follicular cells (Rencber et al., 2018). Recent studies have pointed out that damage to transzonal projections (TZPs) could mediate oocyte-granulosa cell (GC) communication in follicles, which may play a vital role in the etiology of PCOS. Chen et al. disclosed that RVT resumed the inward flow of calcium ions and activated calcium-/calmodulin-dependent protein kinase II beta (CaMKIIβ), thus enabling the synthesis of TZPs. Chen’s work provided a new strategy for treating PCOS (Chen et al., 2022).
Curcumin is a yellow polyphenol pigment extracted from turmeric (Curcuma longa L.), which is low-insoluble in water. Curcumin has received attention from the scientific community due to its anti-inflammatory, antioxidation, and antiapoptotic properties. In PCOS, hyperandrogenism induces ERs and activation of the UPR (to maintain ER homeostasis). Zhang et al. illustrated that long-term ERs led to GC autophagy or apoptosis and caused follicle development disorders, including the accumulation of small follicles around the ovary, polycystic morphology, anovulation, and damage to follicular maturation (Zhang et al., 2021). Curcumin (200 mg/kg daily for 8 weeks) combined with aerobic exercise ameliorated follicle development disorders by reducing hyperandrogen-induced ERs, inhibiting the IRE1α-XBP1 pathway in ovarian GCs, and downregulating follicular development-related genes (Ar, Cyp11α1, and Cyp19α1) (Zhang et al., 2021). Heshmati et al. found that in a randomized, placebo-controlled double-blind trial, curcumin (1500 mg daily for 12 weeks) significantly reduced fasting plasma glucose (FPG) and DHEA in PCOS patients. This result suggested that curcumin could reduce blood glucose, regulate hormone levels, and prevent type 2 diabetes and other complications with PCOS (Heshmati et al., 2021). Due to curcumin’s low oral bioavailability, medium-high curcumin was utilized in the above experiments.
To improve curcumin’s solubility and bioavailability, many researchers have applied curcumin nanoparticles (curcumin NPs) to improve its therapeutic effects. Abuelezz et al. reported that impaired PI3k/Akt/mammalian target of rapamycin (mTOR) signaling in the pancreas and increased levels of TNF-α in PCOS led to pancreatic β-cell secretory dysfunction and IR to disturb glucose metabolism. Curcumin NPs, as an anti-inflammatory agent, could regulate the level of PI3K/Akt/mTOR in the pancreas and reduce TNF-α, thus alleviating abnormal glucose metabolism (Abuelezz et al., 2020). In addition, Abhari et al. revealed that the imbalance between increased ROS levels and decreased GSH initiates oxidative stress and induces apoptosis (Abhari et al., 2020). Oxidative stress could increase the levels of AMH and estrogens via lipid peroxidation of GCs and then bring about follicular atresia, polycystic morphology, anovulation, and other symptoms in PCOS. Interestingly, curcumin’s antioxidation could reduce the level of ROS and inhibit GC apoptosis and oxidative stress by reducing the expression of apoptotic factors and increasing the expression of BAX, B-cell lymphoma 2 (Bcl2) genes, and Caspase3, followed by restoring ovarian function (Abhari et al., 2020). Inspired by the above studies, Raja et al. modified curcumin NPs, which encapsulated arginine (Arg)- and N-acetyl histidine (NAcHis)-modified chitosan (Arg-CS-NAcHis/Cur) nanoparticles (NPs), to construct a better natural drug delivery system (sustained release pattern) against PCOS (Raja et al., 2021).
Bioactive ingredients extracted from tea potentially improve IR, hyperandrogenism, abnormalities in ovarian morphology, and overweight in PCOS through antioxidation and anti-inflammatory pathways. Catechins from oolong tea inhibited the expression of STAT3 signaling, MMP2, and MMP9 in the uterus, increased insulin receptor substrate-1 (IRS-1) and PI3K signals, and downregulated NF-κB, contributing to ameliorating hyperandrogenism and IR, reversing abnormalities in ovarian morphology and reducing uterine inflammation in insulin- and hCG-induced PCOS mice (Hong et al., 2020). The phenolic content in marjoram (Origanum majorana L.) tea aqueous extract mainly includes caffeic acid derivatives and rosmarinic acid. Haj-Husein et al. illustrated that marjoram tea increased PCOS-induced insulin sensitivity by activating peroxisome proliferator-activated receptor-α (PPAR-α) and peroxisome proliferator-activated receptor-γ (PPAR-γ) (Haj-Husein et al., 2016). A host of PCOS patients are overweight or obese, which will not only affect metabolism but also lead to reproductive disorders. Mombaini et al. demonstrated that green tea reduced body mass index, weight, waist circumference, and body fat percentage in women with PCOS and revealed that catechins and caffeine in green tea could prolong the pharmacological effects of catecholamines and increase levels of norepinephrine (increasing energy expenditure and fat oxidation) (Mombaini et al., 2017). These experiments suggested that long-term tea consumption was beneficial in preventing PCOS.
Cinnamon polyphenol compounds isolated from cinnamon have attracted much attention because of their potential in patients with type 2 diabetes. Kort et al. showed that cinnamon could significantly reduce FPG and improve IR and menstrual cyclicity in patients with PCOS, but the specific mechanism has not been clarified (Kort and Lobo, 2014). Further mechanistic studies showed that cinnamon extract had insulin-like properties, enhancing insulin sensitivity and reducing insulin levels and LDL. As a result, the improvement of hormone levels, recovety of the normal menstrual cycle, and reduction of the risk of PCOS complications such as type 2 diabetes and cardiovascular disease were observed (Keefe, 2015; Hajimonfarednejad et al., 2018; Khan and Begum, 2019). In addition, the imbalance between insulin-like growth factor-1 (IGF-1) and insulin-like growth factor-binding protein-1 (IGFBP-1) affects follicular maturation. Patients with PCOS always feature higher IGF-1 and lower IGFBP-1 than healthy women. Dou et al. found that cinnamon extract could reduce the plasma IGF-1 level, increase the plasma IGFBP-1 level, and downregulate the serum levels of testosterone and insulin, followed by restoring the estrous cyclicity and ovary morphology of DHEA-induced PCOS mice (Dou et al., 2018).
Gallic acid (GA), with the chemical formula C7H6O5 and a molecular weight of 170.12 g/mol, is a polyphenol found in many plants with anti-inflammatory and antioxidation properties. Mazloom et al. showed that proinflammatory cytokines were significantly increased, and estrous cyclicity was disrupted in the EV-induced PCOS rat model (Mazloom et al., 2019). The decreased concentrations of inflammatory cytokines in the ovaries of rats treated with GA indicated that GA played an anti-inflammatory role in PCOS (Mazloom et al., 2019). As an antioxidant, GA could effectively protect the ovary and improve the blocked development of follicular formation and follicular atresia in PCOS by inhibiting ROS production, protecting DNA, and preventing lipid peroxidation (Mazloom et al., 2019).
Mangiferin is a bioactive compound isolated from the leaves and bark of mango tree (Mangifera indica) and kinds of TCM, which is widely reported due to its anti-inflammatory and regulation of blood glucos. Qian et al. demonstrated that mangiferin could ameliorate ovarian function and IR by reducing the ratio of ovarian weight/body weight, levels of blood glucose, and insulin, which was highly connected with POCS (Qian et al., 2020). In Qian’s experiment, mangiferin improved inflammation in the DHEA-induced PCOS rat model by reducing the pp65/p65 ratio and blocking NF-κB signaling and the expression of the inflammatory cytokines IL-6, IL-1β, and TNF-α. They also found that inhibition of inflammation improved IR symptoms in DHEA-induced PCOS rats (Qian et al., 2020). Their research showed that the utilization of mangiferin could be considered a potential therapeutic strategy for PCOS.
Alkaloids are a class of compounds with various therapeutic effects that play a massive role in treating diseases (Peng et al., 2019; Bai et al., 2021; Rampogu et al., 2022). However, according to the literature, alkaloids rarely show potential in treating PCOS. This phenomenon indicates that research on alkaloids may provide an opportunity to treat PCOS (Table 3).
PCOS patients usually present with IR, an excess of androgens, an inflammatory response, infertility, or miscarriage. Metformin and insulin sensitizers are usually used to relieve the above symptoms in the clinic. Berberine (BBR) is an isoquinoline alkaloid that is mainly isolated from TCMs, such as Berberis vulgaris L. and Coptis chinensis Franch. BBR is regarded as an insulin sensitizer, and An et al. discovered that BBR was similar to metformin in reducing fasting glucose, fasting insulin, HOMA-IR, and androgen and increasing SHBG. Moreover, women who received berberine were associated with an increase in the live birth rate and fewer side effects after in vitro fertilization (an assisted reproductive technology) treatment than metformin (An et al., 2014). In addition, BBR increases insulin sensitivity by regulating IRS-1 and the mTOR signaling pathway (Kuang et al., 2020). Likewise, BBR upregulated GLUT4 to alleviate IR through dual regulation of PI3K/Akt and mitogen-activated protein kinases (MAPKs) (Zhang et al., 2020b). Additionally, Shen et al. discovered that BBR might alleviate PCOS by inhibiting apoptosis and regulating the expression levels of toll-like receptor 4 (TLR4), tyrosine kinase (LYN), PI3K, Akt, NF-κB, TNF-α, IL-1, IL-6, and caspase-3, which contributed to the improvement of IR (Shen et al., 2021a). For infertility and miscarriage caused by PCOS, Yu et al. confirmed that BBR promoted proliferation and inhibited apoptosis of ovarian granulosa cells through the PI3K/Akt pathway, which is beneficial to improving the pregnancy rate (Yu et al., 2021). High levels of lysophosphatidic acid receptor 3 (LPAR3) and integrin αvβ3 led to reduced endometrial receptivity and subsequent miscarriage. Wang et al. revealed that intervention with BBR could downregulate the levels of LPAR3 and αvβ3 in PCOS rats (Wang et al., 2021). Furthermore, BBR alleviates ovarian glucose metabolism disorders in PCOS. Li et al. indicated that BBR activated the AMPK pathway by promoting the ubiquitination of SIRT-3 and resulted in ovarian cell glucose uptake to maintain ovarian glucose homeostasis (Li et al., 2020). Regarding the effects of the gut microbiota on PCOS, Shen et al. found that berberine improved the symptoms of PCOS by regulating the structure of the gut microbiota and its metabolites (including glutamine, unsaturated acids, and glucose), bringing about decreased Firmicutes and increased Bacteroidetes at the phylum level, as well as increasing glutamine and decreasing glucose and unsaturated acids (Shen et al., 2021b). Their study offered a new direction for berberine in the improvement of PCOS.
Although modern medical treatments, including drugs and surgery, play an important role in treating PCOS, their accompanying side effects further limit their clinical application. Phytochemicals are a vital source of drug discovery and show the potential to improve the symptoms of PCOS and even possess some preventive effects. In this review, we summarized the advancement of these bioactive phytochemicals isolated from TCM and medicinal plants in treating PCOS, including flavonoids, polyphenols, and alkaloids. These phytochemicals show therapeutic effects on PCOS supported by in vivo and in vitro studies, mainly depending on anti-inflammatory, antioxidation, improvement of hormone disorder and IR, and alleviation of hyperinsulinemia (Figure 2). For instance, the anti-inflammatory effect is achieved by inhibiting the combination of ox-LDL and TLR4 from avoiding NF-κB pathway activation, which then elevates the expression of proinflammatory cytokines such as IL-1β, IL-6, IL-12, and TNF-α; activating AMPK to inhibit the NF-κB pathway; and restraining UPR activation by decreasing the production of proinflammatory cytokines. The antioxidation effect is primarily triggered by the initiation of UCP1 to increase the interaction between adiponectin and AdipoR1, leading to the activation of AMPK-SIRT-1 and decreased ROS production. As a result, antioxidant enzymes (such as GST, CAT, and GSH) are enhanced, leading to the activation of Nrf2 to produce Hmox1 and NQO1, followed by the downregulation of ROS. For IR or hyperinsulinemia, these valuable phytochemicals exhibit a potential capacity to sensitize insulin receptors attributed to their antioxidant and anti-inflammatory features. These phytochemicals can activate AMPK to increase GLUT4 translocation to regulate GLUT4 and the PI3K/Akt signaling pathway to increase insulin sensitivity. Moreover, LH binding with LH-R is implicated in androgen production. Some phytochemicals, such as baicalein, apigenin, rutin, SPA, and RVT, can directly decrease LH levels, which decreases androgen release. Several individual reports found that inhibition of the expression of 3β-HSD and 17β-HSD reduced the levels of androstenedione and testosterone. Because of the B-ring structure of flavonoids, these flavonoids are identical to the substrates for the enzymes 3β-HSD and 17β-HSD. Therefore, the binding of 3β-HSD and 17β-HSD with endogenous steroids can be reduced, thus indirectly reducing the activities of 3β-HSD and 17β-HSD. It can also reduce the levels of androgens such as androstenedione and testosterone. The structure-activity relationship of flavonoids has been preliminarily studied according to the literature, and detailed information on the binding sites and forms of flavonoids in ER and AR is summarized in Table 4. Furthermore, inhibition of GnRH can decrease the level of testosterone; regulation of PI3K/Akt in decreasing the expression of CYP17A1 avoids the conversion of pregnenolone to DHEA; and downregulation of FSH inhibits the combination between FSH and the FSH receptor to reduce cAMP through the PKA pathway for downregulating aromatase, showing potential therapeutic effects on PCOS. In addition to the above advancements, some bioactive phytochemicals indicate that regulating the activities of GK and HK to increase ERα gene expression and inhibiting the IRE1α-XBP1 pathway to downregulate Ar, Cyp11α1 and Cyp19α1 gene expression are beneficial to the improvement of PCOS.
In brief, flavonoids reduce androgen levels and improve hyperandrogenism mainly by inhibiting the activities of 3β-HSD, 17β-HSD, and aromatase, thereby inhibiting androgen conversion. For example, quercetin decreases the activities of 3β-HSD and 17β-HSD to reduce testosterone and estradiol levels. Regretfully, only a limited number of clinical studies have been designed to evaluate the efficacy of flavonoids in treating PCOS. Due to their phenolic groups, polyphenols have redox properties, which are responsible for antioxidant effects in treating PCOS. In addition, polyphenols also reduce the release of inflammatory factors by downregulating NF-κB, thereby achieving anti-inflammatory effects. For example, resveratrol, which affects the SIRT-1 pathway by mediating the deacetylation of p66Shc, reduces ROS to achieve antioxidant effects. The anti-inflammatory effect of resveratrol is to reduce the release of inflammatory factors by affecting the UPR process. However, the poor solubility and bioavailability of polyphenolic compounds, such as curcumin, hamper their clinical application. For alkaloids, berberine increases insulin sensitivity and alleviates IR by regulating IRS-1 and activating the PI3K/Akt pathway. Nevertheless, other valuable alkaloids have received less attention in treating PCOS. Of note, according to the literature, we detected those flavonoids and polyphenols accounted for most PCOS therapies, compared to a minor proportion of alkaloids. Continuous investment and attention to flavonoids and polyphenols will benefit the discovery of new drugs for PCOS.
There is a close relationship between hormonal alterations and bone metabolism in PCOS. For example, an increase in androgen leads to an increase in inflammatory factors and impairs bone formation. Estrogen is mainly used to maintain the development of female bones, while the decrease in estrogen in PCOS will cause some damage to bones. Other hormonal alterations, such as changes in LH, FSH, and insulin levels, also affect bone formation and development (Krishnan and Muthusami, 2017). Interestingly, some of the phytochemicals can directly or indirectly affect bone metabolism. For example, quercetin and curcumin can specifically mediate bone metabolism and osteoclast-related pathologies (Bian et al., 2021; Inchingolo et al., 2022); soy isoflavones can directly participate in bone metabolism and maintain the development of bones (Uehara, 2013; Pawlowski et al., 2015); resveratrol can stimulate the proliferation and differentiation of OB cells or inhibit the osteoclastic resorption (Vidoni et al., 2019; Inchingolo et al., 2022); and berberine can not only directly participate in bone metabolism, but also reduce the production of adipocytes (Jia et al., 2019). Notably, there is an inverse relationship between adipocytes and osteoblasts in bone marrow, which can indirectly affect bone metabolism by reducing the production of bone marrow adipocytes (Rayalam et al., 2011). As a result, these phytochemicals can potentially treat PCOS by affecting hormone and bone metabolism (Table 5). This conjecture needs to be validated by abundant experiments.
In addition to these encouraging outcomes, some problems need to be solved. Based on our review, these phytochemicals displayed therapeutic effects in alleviating the symptoms of PCOS. However, most of them are still in the preclinical research stage, except for quercetin, soy isoflavones, berberine, resveratrol, and cinnamon. The following reasons may explain this phenomenon. First, unsatisfactory bioavailability hinders their development in clinical application. A few compounds, such as curcumin, need to overcome poor bioavailability before entering clinical studies (Patel et al., 2020; Raja et al., 2021). In addition to chemical modification, advanced drug delivery systems such as nanoparticles (Luther et al., 2020; Nile et al., 2020) and antibody-conjugated drugs (Drago et al., 2021) can effectively solve the challenges of low bioavailability and precise drug delivery to tissues and cells, avoiding severe side effects. However, until now, only limited attention has been given to these valuable compounds. Conduction of the research above could accelerate the transformation of these powerful and valuable phytochemicals into clinical applications. Second, we still lack in-depth research on the pathogenesis of PCOS regardless of the known symptoms and possible mechanisms. Interestingly, a few studies have shown that gut microbiota and its metabolites significantly impact the evolution of PCOS, such as soy isoflavones, naringenin, and berberine, which can alleviate the symptoms by restoring the gut microbiota of PCOS models to a normal state. Further exploring the relationship between gut microbiota (Aron-Wisnewsky and Clement, 2016; Schuijt et al., 2016; Zuo and Ng, 2018) and PCOS may become a new research direction. Third, we found that many genes related to ovarian function were responsible for the evolution of PCOS, such as ERα, Ar, Cyp11α1, and Cyp19α1 genes. Nevertheless, current research on these valuable phytochemicals mainly focuses on the molecular level, except for quercetin, baicalin, apigenin, rutin, anthocyanins, and resveratrol. Hence, exploring the effects of other phytochemicals on PCOS at the genetic level might offer a different solution (Ruth et al., 2020; Mimouni et al., 2021) to treating PCOS in the future. Fourth, these molecules may play a different role in regulating PCOS than traditional small-molecule drugs. In recent years, the rise of targeted protein degradation (TPD) (Schapira et al., 2019; Dale et al., 2021) technology has revolutionized small molecule drugs, especially proteolysis-targeting chimeras (PROTACs) (Burslem and Crews, 2020; Bekes et al., 2022) and molecular glues (Mayor-Ruiz et al., 2020; Dale et al., 2021). These breakthrough technologies inspired us to determine whether these bioactive molecules play similar roles in alleviating or treating PCOS. Of course, sufficient experiments need to be conducted to test this hypothesis. In brief, this review aims to provide detailed mechanisms of these bioactive phytochemicals and hopefully practical and reliable insight into clinical applications concerning PCOS.
Conceptualization, E-DL and H-MJ; formal analysis, E-DL and H-MJ; investigation, E-DL, WC and YW; data curation, E-DL and H-MJ; writing and original draft preparation, E-DL, H-MJ and WC; writing, reviewing, and editing, XY, H-YD, and MT; visualization (partly created by Figdraw), H-MJ, W-MG, and N-YC; supervision, S-SX and YB; funding acquisition, S-SX and YB. All authors have read and agreed to the published version of the manuscript.
We are grateful for financial support from the Fund of Healthy City Development Research Center—Chengdu Key Research Base of Philosophy and Social Sciences (2019ZZ003); Chengdu Key Medical Disciplines Construction Project 2022; Zhou Cong-rong Sichuan Famous Traditional Chinese Medicine Physician Studio Construction Project 2022; Science and Technology Development Fund, Macau SAR (SKL-QRCM(UM)-2020-2022); University of Macau Research Funding (QRCM-IRG2022-001).
The authors declare that the research was conducted in the absence of any commercial or financial relationships that could be construed as a potential conflict of interest.
All claims expressed in this article are solely those of the authors and do not necessarily represent those of their affiliated organizations, or those of the publisher, the editors and the reviewers. Any product that may be evaluated in this article, or claim that may be made by its manufacturer, is not guaranteed or endorsed by the publisher.
Abhari, S. M. F., Khanbabaei, R., Roodbari, N. H., Parivar, K., and Yaghmaei, P. (2020). Curcumin-loaded super-paramagnetic iron oxide nanoparticle affects on apoptotic factors expression and histological changes in a prepubertal mouse model of polycystic ovary syndrome-induced by dehydroepiandrosterone - a molecular and stereological study. Life Sci. 249, 117515. doi:10.1016/j.lfs.2020.117515
Abuelezz, N. Z., Shabana, M. E., Abdel-Mageed, H. M., Rashed, L., and Morcos, G. N. B. (2020). Nanocurcumin alleviates insulin resistance and pancreatic deficits in polycystic ovary syndrome rats: Insights on PI3K/AkT/mTOR and TNF-alpha modulations. Life Sci. 256, 118003. doi:10.1016/j.lfs.2020.118003
An, Y., Sun, Z., Zhang, Y., Liu, B., Guan, Y., and Lu, M. (2014). The use of berberine for women with polycystic ovary syndrome undergoing IVF treatment. Clin. Endocrinol. 80 (3), 425–431. doi:10.1111/cen.12294
Aron-Wisnewsky, J., and Clement, K. (2016). The gut microbiome, diet, and links to cardiometabolic and chronic disorders. Nat. Rev. Nephrol. 12 (3), 169–181. doi:10.1038/nrneph.2015.191
Azziz, R., Carmina, E., Chen, Z., Dunaif, A., Laven, J. S. E., Legro, R. S., et al. (2016). Polycystic ovary syndrome. Nat. Rev. Dis. Prim. 2, 16057. doi:10.1038/nrdp.2016.57
Badshah, S. L., Faisal, S., Muhammad, A., Poulson, B. G., Emwas, A. H., and Jaremko, M. (2021). Antiviral activities of flavonoids. Biomed. Pharmacother. 140, 111596. doi:10.1016/j.biopha.2021.111596
Bai, R. R., Yao, C. S., Zhong, Z. C., Ge, J. M., Bai, Z. Q., Ye, X. Y., et al. (2021). Discovery of natural anti-inflammatory alkaloids: Potential leads for the drug discovery for the treatment of inflammation. Eur. J. Med. Chem. 213, 113165. doi:10.1016/j.ejmech.2021.113165
Bahramrezaie, M., Amidi, F., Aleyasin, A., Saremi, A., Aghahoseini, M., Brenjian, S., et al. (2019). Effects of resveratrol on VEGF & HIF1 genes expression in granulosa cells in the angiogenesis pathway and laboratory parameters of polycystic ovary syndrome: A triple-blind randomized clinical trial. J. Assisted Reproduction Genet. 36 (8), 1701–1712. doi:10.1007/s10815-019-01461-6
Balen, A. H., Morley, L. C., Misso, M., Franks, S., Legro, R. S., Wijeyaratne, C. N., et al. (2016). The management of anovulatory infertility in women with polycystic ovary syndrome: An analysis of the evidence to support the development of global WHO guidance. Hum. Reprod. Update 22 (6), 687–708. doi:10.1093/humupd/dmw025
Banaszewska, B., Wrotynska-Barczynska, J., Spaczynski, R. Z., Pawelczyk, L., and Duleba, A. J. (2016). Effects of resveratrol on polycystic ovary syndrome: A double-blind, randomized, placebo-controlled trial. J. Clin. Endocrinol. Metabolism 101 (11), 4322–4328. doi:10.1210/jc.2016-1858
Bekes, M., Langley, D. R., and Crews, C. M. (2022). PROTAC targeted protein degraders: The past is prologue. Nat. Rev. Drug Discov. 21 (3), 181–200. doi:10.1038/s41573-021-00371-6
Bian, W., Xiao, S. Q., Yang, L., Chen, J., and Deng, S. F. (2021). Quercetin promotes bone marrow mesenchymal stem cell proliferation and osteogenic differentiation through the H19/miR-625-5p axis to activate the Wnt/β-catenin pathway. BMC Complement. Med. Ther. 21 (1), 243. doi:10.1186/s12906-021-03418-8
Brenjian, S., Moini, A., Yamini, N., Kashani, L., Faridmojtahedi, M., Bahramrezaie, M., et al. (2020). Resveratrol treatment in patients with polycystic ovary syndrome decreased pro-inflammatory and endoplasmic reticulum stress markers. Am. J. Reproductive Immunol. 83 (1), e13186. doi:10.1111/aji.13186
Burslem, G. M., and Crews, C. M. (2020). Proteolysis-targeting chimeras as therapeutics and tools for biological discovery. Cell. 181 (1), 102–114. doi:10.1016/j.cell.2019.11.031
Cai, W.-Y., Luo, X., Song, J., Ji, D., Zhu, J., Duan, C., et al. (2022). Effect of hyperinsulinemia and insulin resistance on endocrine, metabolic, and reproductive outcomes in non-PCOS women undergoing assisted reproduction: A retrospective cohort study. Front. Med. 8, 736320. doi:10.3389/fmed.2021.736320
Carson, S. A., and Kallen, A. N. (2021). Diagnosis and management of infertility A review. Jama-Journal Am. Med. Assoc. 326 (1), 65–76. doi:10.1001/jama.2021.4788
Chahal, N., Geethadevi, A., Kaur, S., Lakra, R., Nagendra, A., Shrivastav, T. G., et al. (2021). Direct impact of gonadotropins on glucose uptake and storage in preovulatory granulosa cells: Implications in the pathogenesis of polycystic ovary syndrome. Metabolism-Clinical Exp. 115, 154458. doi:10.1016/j.metabol.2020.154458
Chen, M., He, C., Zhu, K., Chen, Z., Meng, Z., Jiang, X., et al. (2022). Resveratrol ameliorates polycystic ovary syndrome via transzonal projections within oocyte-granulosa cell communication. Theranostics 12 (2), 782–795. doi:10.7150/thno.67167
Cheung, E. C., and Vousden, K. H. (2022). The role of ROS in tumour development and progression. Nat. Rev. Cancer 22 (5), 280–297. doi:10.1038/s41568-021-00435-0
Choudhari, A. S., Mandave, P. C., Deshpande, M., Ranjekar, P., and Prakash, O. (2020). Phytochemicals in cancer treatment: From preclinical studies to clinical practice. Front. Pharmacol. 10, 1614. doi:10.3389/fphar.2019.01614
Choy, K. W., Murugan, D., Leong, X. F., Abas, R., Alias, A., and Mustafa, M. R. (2019). Flavonoids as natural anti-inflammatory agents targeting nuclear factor-kappa B (NF kappa B) signaling in cardiovascular diseases: A mini review. Front. Pharmacol. 10. doi:10.3389/fphar.2019.01295
D'Arrigo, G., Gianquinto, E., Rossetti, G., Cruciani, G., Lorenzetti, S., and Spyrakis, F. (2021). Binding of androgen- and estrogen-like flavonoids to their cognate (Non)Nuclear receptors: A comparison by computational prediction. Molecules 26 (6). doi:10.3390/molecules26061613
Dale, B., Cheng, M., Park, K.-S., Kaniskan, H. U., Xiong, Y., and Jin, J. (2021). Advancing targeted protein degradation for cancer therapy. Nat. Rev. Cancer 21 (10), 638–654. doi:10.1038/s41568-021-00365-x
Darabi, P., Khazali, H., and Mehrabani Natanzi, M. (2020). Therapeutic potentials of the natural plant flavonoid apigenin in polycystic ovary syndrome in rat model: Via modulation of pro-inflammatory cytokines and antioxidant activity. Gynecol. Endocrinol. 36 (7), 582–587. doi:10.1080/09513590.2019.1706084
de Zegher, F., Lopez-Bermejo, A., and Ibanez, L. (2018). Central obesity, faster maturation, and 'PCOS' in girls. Trends Endocrinol. Metabolism 29 (12), 815–818. doi:10.1016/j.tem.2018.09.005
Dei Cas, M., and Ghidoni, R. (2019). Dietary curcumin: Correlation between bioavailability and health potential. Nutrients 11 (9), 2147. doi:10.3390/nu11092147
Dewailly, D., Robin, G., Peigne, M., Decanter, C., Pigny, P., and Catteau-Jonard, S. (2016). Interactions between androgens, FSH, anti-Mullerian hormone and estradiol during folliculogenesis in the human normal and polycystic ovary. Hum. Reprod. Update 22 (6), 709–724. doi:10.1093/humupd/dmw027
Diamanti-Kandarakis, E., and Dunaif, A. (2012). Insulin resistance and the polycystic ovary syndrome revisited: An update on mechanisms and implications. Endocr. Rev. 33 (6), 981–1030. doi:10.1210/er.2011-1034
Dou, L., Zheng, Y., Li, L., Gui, X., Chen, Y., Yu, M., et al. (2018). The effect of cinnamon on polycystic ovary syndrome in a mouse model. Reproductive Biol. Endocrinol. 16, 99. doi:10.1186/s12958-018-0418-y
Drago, J. Z., Modi, S., and Chandarlapaty, S. (2021). Unlocking the potential of antibody-drug conjugates for cancer therapy. Nat. Rev. Clin. Oncol. 18 (6), 327–344. doi:10.1038/s41571-021-00470-8
Escobar-Morreale, H. F. (2018). Polycystic ovary syndrome: Definition, aetiology, diagnosis and treatment. Nat. Rev. Endocrinol. 14 (5), 270–284. doi:10.1038/nrendo.2018.24
Farkhad, S. A., and Khazali, H. (2019). Therapeutic effects of isoflavone-aglycone fraction from soybean (Glycine max L. Merrill) in rats with estradiol valerate-induced polycystic ovary syndrome as an inflammatory state. Gynecol. Endocrinol. 35 (12), 1078–1083. doi:10.1080/09513590.2019.1624715
Filippou, P., and Homburg, R. (2017). Is foetal hyperexposure to androgens a cause of PCOS? Hum. Reprod. Update 23 (4), 421–432. doi:10.1093/humupd/dmx013
Ganie, M. A., Sahar, T., Rashid, A., Wani, I. A., Nisar, S., Sathyapalan, T., et al. (2019). Comparative evaluation of biomarkers of inflammation among Indian women with polycystic ovary syndrome (PCOS) consuming vegetarian vs. Non-vegetarian diet. Front. Endocrinol. 10, 699. doi:10.3389/fendo.2019.00699
Gao, J. S., Ma, H. L., Wang, Y., Yang, X. M., Cao, Y. J., Zhang, B., et al. (2021). Hospital-based phenotypic features and treatment outcomes of Chinese women with polycystic ovary syndrome: The effect of body mass index and geographic distribution. Engineering 7 (2), 170–177. doi:10.1016/j.eng.2020.12.006
Gao, Z., Ma, X., Liu, J., Ge, Y., Wang, L., Fu, P., et al. (2020). Troxerutin protects against DHT-induced polycystic ovary syndrome in rats. J. Ovarian Res. 13 (1), 106. doi:10.1186/s13048-020-00701-z
Ghowsi, M., Khazali, H., and Sisakhtnezhad, S. (2018). Evaluation of TNF-α and IL-6 mRNAs expressions in visceral and subcutaneous adipose tissues of polycystic ovarian rats and effects of resveratrol. J. Basic Med. Sci. 21 (2), 165–174. doi:10.22038/ijbms.2017.24801.6167
Haj-Husein, I., Tukan, S., and Alkazaleh, F. (2016). The effect of marjoram (origanum majorana) tea on the hormonal profile of women with polycystic ovary syndrome: A randomised controlled pilot study. J. Hum. Nutr. Dietetics 29 (1), 105–111. doi:10.1111/jhn.12290
Hajimonfarednejad, M., Nimrouzi, M., Heydari, M., Zarshenas, M. M., Raee, M. J., and Jahromi, B. N. (2018). Insulin resistance improvement by cinnamon powder in polycystic ovary syndrome: A randomized double-blind placebo controlled clinical trial. Phytotherapy Res. 32 (2), 276–283. doi:10.1002/ptr.5970
Han, Y. H., Kee, J. Y., Park, J., Kim, D. S., Shin, S., Youn, D. H., et al. (2016). Lipin1-Mediated repression of adipogenesis by rutin. Am. J. Chin. Med. 44 (3), 565–578. doi:10.1142/s0192415x16500312
Happe, L., Puente, J., Beveridge, M., Wixson, S., and Loy, B. (2021). High-dose spironolactone for acne in patients with polycystic ovarian syndrome: A single-institution retrospective study. J. Am. Acad. Dermatology 85 (3), 741–743. doi:10.1016/j.jaad.2019.08.079
Hatefi, M., Ahmadi, M. R. H., Rahmani, A., Dastjerdi, M. M., and Asadollahi, K. (2018). Effects of curcumin on bone loss and biochemical markers of bone turnover in patients with spinal cord injury. World Neurosurg. 114, E785–E791. doi:10.1016/j.wneu.2018.03.081
He, F. F., and Li, Y. M. (2020). Role of gut microbiota in the development of insulin resistance and the mechanism underlying polycystic ovary syndrome: A review. J. Ovarian Res. 13 (1), 73. doi:10.1186/s13048-020-00670-3
He, Y. F., Wang, Q. Q., Li, X., Wang, G., Zhao, J. X., Zhang, H., et al. (2020). Lactic acid bacteria alleviate polycystic ovarian syndrome by regulating sex hormone related gut microbiota. Food & Funct. 11 (6), 5192–5204. doi:10.1039/c9fo02554e
Herr, D., Bekes, I., and Wulff, C. (2015). Regulation of endothelial permeability in the primate corpora lutea: Implications for ovarian hyperstimulation syndrome. Reproduction 149 (2), R71–R79. doi:10.1530/rep-13-0296
Heshmati, J., Moini, A., Sepidarkish, M., Morvaridzadeh, M., Salehi, M., Palmowski, A., et al. (2021). Effects of curcumin supplementation on blood glucose, insulin resistance and androgens in patients with polycystic ovary syndrome: A randomized double-blind placebo-controlled clinical trial. Phytomedicine 80, 153395. doi:10.1016/j.phymed.2020.153395
Hickey, M., Higham, J. M., and Fraser, I. (2012). Progestogens with or without oestrogen for irregular uterine bleeding associated with anovulation. Cochrane Database Syst. Rev. 2012 (9), CD001895. doi:10.1002/14651858.CD001895.pub3
Hong, G., Wu, H., Ma, S.-T., and Su, Z. (2020). Catechins from oolong tea improve uterine defects by inhibiting STAT3 signaling in polycystic ovary syndrome mice. Chin. Med. 15 (1), 125. doi:10.1186/s13020-020-00405-y
Hong, Y., Yin, Y., Tan, Y., Hong, K., and Zhou, H. (2019). The flavanone, naringenin, modifies antioxidant and steroidogenic enzyme activity in a rat model of letrozole-induced polycystic ovary syndrome. Med. Sci. Monit. 25, 395–401. doi:10.12659/msm.912341
Hong, Y., Yin, Y., Tan, Y., Hong, K., Jiang, F., and Wang, Y. (2018). Effect of quercetin on biochemical parameters in letrozole-induced polycystic ovary syndrome in rats. Trop. J. Pharm. Res. 17 (9), 1783–1788. doi:10.4314/tjpr.v17i9.15
Housman, E., and Reynolds, R. V. (2014). Polycystic ovary syndrome: A review for dermatologists Part I. Diagnosis and manifestations. J. Am. Acad. Dermatology 71 (5), e1–e847. doi:10.1016/j.jaad.2014.05.007
Hu, T., Yuan, X., Ye, R., Zhou, H., Lin, J., Zhang, C., et al. (2017). Brown adipose tissue activation by rutin ameliorates polycystic ovary syndrome in rat. J. Nutr. Biochem. 47, 21–28. doi:10.1016/j.jnutbio.2017.04.012
Huang, Y., and Zhang, X. (2021). Luteolin alleviates polycystic ovary syndrome in rats by resolving insulin resistance and oxidative stress. Am. J. Physiology-Endocrinology Metabolism 320 (6), E1085–E1092. doi:10.1152/ajpendo.00034.2021
Inchingolo, A. D., Inchingolo, A. M., Malcangi, G., Avantario, P., Azzollini, D., Buongiorno, S., et al. (2022). Effects of resveratrol, curcumin and quercetin supplementation on bone metabolism-A systematic review. Nutrients 14 (17), 3519. doi:10.3390/nu14173519
Jahan, S., Munir, F., Razak, S., Mehboob, A., Ul Ain, Q., Ullah, H., et al. (2016). Ameliorative effects of rutin against metabolic, biochemical and hormonal disturbances in polycystic ovary syndrome in rats. J. Ovarian Res. 9, 86. doi:10.1186/s13048-016-0295-y
Jamilian, M., and Asemi, Z. (2016). The effects of soy isoflavones on metabolic status of patients with polycystic ovary syndrome. J. Clin. Endocrinol. Metabolism 101 (9), 3386–3394. doi:10.1210/jc.2016-1762
Jayasena, C. N., and Franks, S. (2014). The management of patients with polycystic ovary syndrome. Nat. Rev. Endocrinol. 10 (10), 624–636. doi:10.1038/nrendo.2014.102
Jia, X., Jia, L., Mo, L., Yuan, S., Zheng, X., He, J., et al. (2019). Berberine ameliorates periodontal bone loss by regulating gut microbiota. J. Dent. Res. 98 (1), 107–116. doi:10.1177/0022034518797275
Joseph, S., Barai, R. S., Bhujbalrao, R., and Idicula-Thomas, S. (2016). PCOSKB: A KnowledgeBase on genes, diseases, ontology terms and biochemical pathways associated with PolyCystic ovary syndrome. Nucleic Acids Res. 44 (D1), D1032–D1035. doi:10.1093/nar/gkv1146
Karamali, M., Kashanian, M., Alaeinasab, S., and Asemi, Z. (2018). The effect of dietary soy intake on weight loss, glycaemic control, lipid profiles and biomarkers of inflammation and oxidative stress in women with polycystic ovary syndrome: A randomised clinical trial. J. Hum. Nutr. Dietetics 31 (4), 533–543. doi:10.1111/jhn.12545
Keefe, D., and Lobo, R. A. (2015). Preliminary evidence that cinnamon improves menstrual cyclicity in women with polycystic ovary syndrome: A randomized controlled trial editorial comment. Obstetrical Gynecol. Surv. 70 (2), 94–95. doi:10.1097/01.ogx.0000461902.16853.84
Khan, A. A., and Begum, W. (2019). Efficacy of darchini in the management of polycystic ovarian syndrome: A randomized clinical study. J. Herb. Med. 15, 100249. doi:10.1016/j.hermed.2018.11.005
Khatoon, E., Banik, K., Harsha, C., Sailo, B. L., Thakur, K. K., Khwairakpam, A. D., et al. (2022). Phytochemicals in cancer cell chemosensitization: Current knowledge and future perspectives. Seminars Cancer Biol. 80, 306–339. doi:10.1016/j.semcancer.2020.06.014
Khorchani, M. J., Zal, F., and Neisy, A. (2020). The phytoestrogen, quercetin, in serum, uterus and ovary as a potential treatment for dehydroepiandrosterone-induced polycystic ovary syndrome in the rat. Reproduction Fertil. Dev. 32 (3), 313–321. doi:10.1071/rd19072
Khorshidi, M., Moini, A., Alipoor, E., Rezvan, N., Gorgani-Firuzjaee, S., Yaseri, M., et al. (2018). The effects of quercetin supplementation on metabolic and hormonal parameters as well as plasma concentration and gene expression of resistin in overweight or obese women with polycystic ovary syndrome. Phytotherapy Res. 32 (11), 2282–2289. doi:10.1002/ptr.6166
Kort, D. H., and Lobo, R. A. (2014). Preliminary evidence that cinnamon improves menstrual cyclicity in women with polycystic ovary syndrome: A randomized controlled trial. Am. J. Obstetrics Gynecol. 211 (5), e1–e6. doi:10.1016/j.ajog.2014.05.009
Krishnan, A., and Muthusami, S. (2017). Hormonal alterations in PCOS and its influence on bone metabolism. J. Endocrinol. 232 (2), R99–R113. doi:10.1530/joe-16-0405
Kuang, H., Duan, Y., Li, D., Xu, Y., Ai, W., Li, W., et al. (2020). The role of serum inflammatory cytokines and berberine in the insulin signaling pathway among women with polycystic ovary syndrome. Plos One 15 (8), e0235404. doi:10.1371/journal.pone.0235404
La Marca, A., and Sunkara, S. K. (2014). Individualization of controlled ovarian stimulation in IVF using ovarian reserve markers: From theory to practice. Hum. Reprod. Update 20 (1), 124–140. doi:10.1093/humupd/dmt037
Lan, Z. J., Krause, M. S., Redding, S. D., Li, X., Wu, G. Z., Zhou, H. X., et al. (2017). Selective deletion of Pten in theca-interstitial cells leads to androgen excess and ovarian dysfunction in mice. Mol. Cell. Endocrinol. 444 (C), 26–37. doi:10.1016/j.mce.2017.01.043
Li, W., Li, D., Kuang, H., Feng, X., Ai, W., Wang, Y., et al. (2020). Berberine increases glucose uptake and intracellular ROS levels by promoting Sirtuin 3 ubiquitination. Biomed. Pharmacother. 121, 109563. doi:10.1016/j.biopha.2019.109563
Li, X., Ma, J., Guo, L., Dong, C., Zhu, G., Hong, W., et al. (2022). Identification of bioactive compounds and potential mechanisms of Kuntai capsule in the treatment of polycystic ovary syndrome by integrating network pharmacology and bioinformatics. Oxidative Med. Cell. Longev. 2022, 3145938. doi:10.1155/2022/3145938
Lim, S. S., Davies, M. J., Norman, R. J., and Moran, L. J. (2012). Overweight, obesity and central obesity in women with polycystic ovary syndrome: A systematic review and meta-analysis. Hum. Reprod. Update 18 (6), 618–637. doi:10.1093/humupd/dms030
Lim, S. S., Norman, R. J., Davies, M. J., and Moran, L. J. (2013). The effect of obesity on polycystic ovary syndrome: A systematic review and meta-analysis. Obes. Rev. 14 (2), 95–109. doi:10.1111/j.1467-789X.2012.01053.x
Liu, Y. S., Liu, H., Li, Z. T., Fan, H. L., Yan, X. M., Liu, X., et al. (2021). The release of peripheral immune inflammatory cytokines promote an inflammatory cascade in PCOS patients via altering the follicular microenvironment. Front. Immunol. 12, 685724. doi:10.3389/fimmu.2021.685724
Liyanage, G. S. G., Inoue, R., Fujitani, M., Ishijima, T., Shibutani, T., Abe, K., et al. (2021). Effects of soy isoflavones, resistant starch and antibiotics on polycystic ovary syndrome (PCOS)-Like features in letrozole-treated rats. Nutrients 13 (11), 3759. doi:10.3390/nu13113759
Luther, D. C., Huang, R., Jeon, T., Zhang, X., Lee, Y.-W., Nagaraj, H., et al. (2020). Delivery of drugs, proteins, and nucleic acids using inorganic nanoparticles. Adv. Drug Deliv. Rev. 156, 188–213. doi:10.1016/j.addr.2020.06.020
Ma, X., Li, X., Ma, L., Chen, Y., and He, S. (2021). Soy isoflavones alleviate polycystic ovary syndrome in rats by regulating NF- kappa B signaling pathway. Bioengineered 12 (1), 7215–7223. doi:10.1080/21655979.2021.1979864
Manzoor, S., Ganie, M. A., Amin, S., Shah, Z. A., Bhat, I. A., Yousuf, S. D., et al. (2019). Oral contraceptive use increases risk of inflammatory and coagulatory disorders in women with Polycystic Ovarian Syndrome: An observational study. Sci. Rep. 9, 10182. doi:10.1038/s41598-019-46644-4
Maleki, S. J., Crespo, J. F., and Cabanillas, B. (2019). Anti-inflammatory effects of flavonoids. Food Chem. 299, 125124. doi:10.1016/j.foodchem.2019.125124
Marti, N., Bouchoucha, N., Sauter, K.-S., and Fluck, C. E. (2017). Resveratrol inhibits androgen production of human adrenocortical H295R cells by lowering CYP17 and CYP21 expression and activities. Plos One 12 (3), e0174224. doi:10.1371/journal.pone.0174224
Mayor-Ruiz, C., Bauer, S., Brand, M., Kozicka, Z., Siklos, M., Imrichova, H., et al. (2020). Rational discovery of molecular glue degraders via scalable chemical profiling. Nat. Chem. Biol. 16(11), 1199-+. doi: 1207. doi:10.1038/s41589-020-0594-x
Mazloom, B. F., Edalatmanesh, M. A., and Hosseini, S. E. (2019). Gallic acid reduces inflammatory cytokines and markers of oxidative damage in a rat model of estradiol-induced polycystic ovary. Comp. Clin. Pathol. 28 (5), 1281–1286. doi:10.1007/s00580-019-02920-3
Merhi, Z., Kandaraki, E. A., and Diamanti-Kandarakis, E. (2019). Implications and future perspectives of AGEs in PCOS pathophysiology. Trends Endocrinol. Metabolism 30 (3), 150–162. doi:10.1016/j.tem.2019.01.005
Mihanfar, A., Nouri, M., Roshangar, L., and Khadem-Ansari, M. H. (2021a). Polyphenols: Natural compounds with promising potential in treating polycystic ovary syndrome. Reprod. Biol. 21 (2), 100500. doi:10.1016/j.repbio.2021.100500
Mihanfar, A., Nouri, M., Roshangar, L., and Khadem-Ansari, M. H. (2021b900). Therapeutic potential of quercetin in an animal model of PCOS: Possible involvement of AMPK/SIRT-1 axis. Eur. J. Pharmacol. 900, 174062. doi:10.1016/j.ejphar.2021.174062
Mimouni, N. E. H., Paiva, I., Barbotin, A.-L., Timzoura, F. E., Plassard, D., Le Gras, S., et al. (2021). Polycystic ovary syndrome is transmitted via a transgenerational epigenetic process. Cell. Metab. 33(3), 513-+. doi: 530.e8. doi:10.1016/j.cmet.2021.01.004
Mirabelli, M., Chiefari, E., Arcidiacono, B., Corigliano, D. M., Brunetti, F. S., Maggisano, V., et al. (2020). Mediterranean diet nutrients to turn the tide against insulin resistance and related diseases. Nutrients 12 (4), 1066. doi:10.3390/nu12041066
Mombaini, E., Jafarirad, S., Husain, D., Haghighizadeh, M. H., and Padfar, P. (2017). The impact of green tea supplementation on anthropometric indices and inflammatory cytokines in women with polycystic ovary syndrome. Phytotherapy Res. 31 (5), 747–754. doi:10.1002/ptr.5795
Morley, L. C., Tang, T., Yasmin, E., Norman, R. J., and Balen, A. H. (2017). Insulin-sensitising drugs (metformin, rosiglitazone, pioglitazone, D-chiro-inositol) for women with polycystic ovary syndrome, oligo amenorrhoea and subfertility. Cochrane Database Syst. Rev. 11, CD003053. doi:10.1002/14651858.CD003053.pub6
Moshfegh, F., Balanejad, S. Z., Shahrokhabady, K., and Attaranzadeh, A. (2022). Crocus sativus (saffron) petals extract and its active ingredient, anthocyanin improves ovarian dysfunction, regulation of inflammatory genes and antioxidant factors in testosterone-induced PCOS mice. J. Ethnopharmacol. 282, 114594. doi:10.1016/j.jep.2021.114594
Murray, C. J. L., Aravkin, A. Y., Zheng, P., Abbafati, C., Abbas, K. M., Abbasi-Kangevari, M., et al. (2020). Global burden of 87 risk factors in 204 countries and territories, 1990-2019: A systematic analysis for the global burden of disease study 2019. Lancet 396 (10258), 1223–1249. doi:10.1016/s0140-6736(20)30752-2
Murri, M., Luque-Ramirez, M., Insenser, M., Ojeda-Ojeda, M., and Escobar-Morreale, H. F. (2013). Circulating markers of oxidative stress and polycystic ovary syndrome (PCOS): A systematic review and meta-analysis. Hum. Reprod. Update 19 (3), 268–288. doi:10.1093/humupd/dms059
Muscogiuri, G., Colao, A., and Orio, F. (2015). Insulin-mediated diseases: Adrenal mass and polycystic ovary syndrome. Trends Endocrinol. Metabolism 26 (10), 512–514. doi:10.1016/j.tem.2015.07.010
Neisy, A., Zal, F., Seghatoleslam, A., and Alaee, S. (2019). Amelioration by quercetin of insulin resistance and uterine GLUT4 and ERα gene expression in rats with polycystic ovary syndrome (PCOS). Reproduction, Fertil. Dev. 31 (2), 315–323. doi:10.1071/RD18222
Nile, S. H., Baskar, V., Selvaraj, D., Nile, A., Xiao, J., and Kai, G. (2020). Nanotechnologies in food science: Applications, recent trends, and future perspectives. Nano-Micro Lett. 12 (1), 45. doi:10.1007/s40820-020-0383-9
Ninfali, P., Antonelli, A., Magnani, M., and Scarpa, E. S. (2020). Antiviral properties of flavonoids and delivery strategies. Nutrients 12 (9), 2534. doi:10.3390/nu12092534
O'Kelly, A. C., Michos, E. D., Shufelt, C. L., Vermunt, J. V., Minissian, M. B., Quesada, O., et al. (2022). Pregnancy and reproductive risk factors for cardiovascular disease in women. Circulation Res. 130 (4), 652–672. doi:10.1161/circresaha.121.319895
Okoth, K., Chandan, J. S., Marshall, T., Thangaratinam, S., Thomas, G. N., Nirantharakumar, K., et al. (2020). Association between the reproductive health of young women and cardiovascular disease in later life: Umbrella review. Bmj-British Med. J. 371, m3502. doi:10.1136/bmj.m3502
Ortega, I., Villanueva, J. A., Wong, D. H., Cress, A. B., Sokalska, A., Stanley, S. D., et al. (2014). Resveratrol potentiates effects of simvastatin on inhibition of rat ovarian theca-interstitial cells steroidogenesis. J. Ovarian Res. 7, 21. doi:10.1186/1757-2215-7-21
Ortega, I., Villanueva, J. A., Wong, D. H., Cress, A. B., Sokalska, A., Stanley, S. D., et al. (2012a). Resveratrol reduces steroidogenesis in rat ovarian theca-interstitial cells: The role of inhibition of Akt/PKB signaling pathway. Endocrinology 153 (8), 4019–4029. doi:10.1210/en.2012-1385
Ortega, I., Wong, D. H., Villanueva, J. A., Cress, A. B., Sokalska, A., Stanley, S. D., et al. (2012b). Effects of resveratrol on growth and function of rat ovarian granulosa cells. Fertil. Steril. 98 (6), 1563–1573. doi:10.1016/j.fertnstert.2012.08.004
Palomba, S., de Wilde, M. A., Falbo, A., Koster, M. P. H., La Sala, G. B., and Fauser, B. C. J. M. (2015). Pregnancy complications in women with polycystic ovary syndrome. Hum. Reprod. Update 21 (5), 575–592. doi:10.1093/humupd/dmv029
Patel, S. S., Acharya, A., Ray, R. S., Agrawal, R., Raghuwanshi, R., and Jain, P. (2020). Cellular and molecular mechanisms of curcumin in prevention and treatment of disease. Crit. Rev. Food Sci. Nutr. 60 (6), 887–939. doi:10.1080/10408398.2018.1552244
Patisaul, H. B., Mabrey, N., Adewale, H. B., and Sullivan, A. W. (2014). Soy but not bisphenol A (BPA) induces hallmarks of polycystic ovary syndrome (PCOS) and related metabolic co-morbidities in rats. Reprod. Toxicol. 49, 209–218. doi:10.1016/j.reprotox.2014.09.003
Pawlowski, J. W., Martin, B. R., McCabe, G. P., McCabe, L., Jackson, G. S., Peacock, M., et al. (2015). Impact of equol-producing capacity and soy-isoflavone profiles of supplements on bone calcium retention in postmenopausal women: A randomized crossover trial. Am. J. Clin. Nutr. 102 (3), 695–703. doi:10.3945/ajcn.114.093906
Pena, A. S., Witchel, S. F., Hoeger, K. M., Oberfield, S. E., Vogiatzi, M. G., Misso, M., et al. (2020). Adolescent polycystic ovary syndrome according to the international evidence-based guideline. Bmc Med. 18 (1), 72. doi:10.1186/s12916-020-01516-x
Peng, J., Zheng, T. T., Li, X., Liang, Y., Wang, L. J., Huang, Y. C., et al. (2019). Plant-Derived alkaloids: The promising disease-modifying agents for inflammatory bowel disease. Front. Pharmacol. 10, 351. doi:10.3389/fphar.2019.00351
Peng, F., Hu, Y., Peng, S., Zeng, N., and Shi, L. (2022). Apigenin exerts protective effect and restores ovarian function in dehydroepiandrosterone induced polycystic ovary syndrome rats: A biochemical and histological analysis. Ann. Med. 54 (1), 578–587. doi:10.1080/07853890.2022.2034933
Qi, X. Y., Yun, C. Y., Sun, L. L., Xia, J. L., Wu, Q., Wang, Y., et al. (2019). Gut microbiota-bile acid-interleukin-22 axis orchestrates polycystic ovary syndrome. Nat. Med. 25(8), 1225-+. doi: 1233. doi:10.1038/s41591-019-0509-0
Qian, Q., Tang, M., Li, X., Cao, Q., and Zhu, Z. (2020). Mangiferin ameliorates insulin resistance in a rat model of polycystic ovary syndrome via inhibition of inflammation. Trop. J. Pharm. Res. 19 (1), 89–94. doi:10.4314/tjpr.v19i1.14
Rahman, M. A., Hannan, M. A., Dash, R., Rahman, M. D. H., Islam, R., Uddin, M. J., et al. (2021). Phytochemicals as a complement to cancer chemotherapy: Pharmacological modulation of the autophagy-apoptosis pathway. Front. Pharmacol. 12, 639628. doi:10.3389/fphar.2021.639628
Raja, M. A., Maldonado, M., Chen, J., Zhong, Y., and Gu, J. (2021). Development and evaluation of curcumin encapsulated self-assembled nanoparticles as potential remedial treatment for PCOS in a female rat model. Int. J. Nanomedicine 16, 6231–6247. doi:10.2147/ijn.S302161
Rajan, R. K., Kumar, S. S. M., and Balaji, B. (2017). Soy isoflavones exert beneficial effects on letrozole-induced rat polycystic ovary syndrome (PCOS) model through anti-androgenic mechanism. Pharm. Biol. 55 (1), 242–251. doi:10.1080/13880209.2016.1258425
Rampogu, S., Balasubramaniyam, T., and Lee, J. H. (2022). Phytotherapeutic applications of alkaloids in treating breast cancer. Biomed. Pharmacother. 155, 113760. doi:10.1016/j.biopha.2022.113760
Rangel, N., Villegas, V. E., and Rondon-Lagos, M. (2021). Obesity and androgen receptor signaling: Associations and potential crosstalk in breast cancer cells. Cancers 13 (9), 2218. doi:10.3390/cancers13092218
Rashid, R., Mir, S. A., Kareem, O., Ali, T., Ara, R., Malik, A., et al. (2022). Polycystic ovarian syndrome-current pharmacotherapy and clinical implications. Taiwan. J. Obstetrics Gynecol. 61 (1), 40–50. doi:10.1016/j.tjog.2021.11.009
Rayalam, S., Della-Fera, M. A., and Baile, C. A. (2011). Synergism between resveratrol and other phytochemicals: Implications for obesity and osteoporosis. Mol. Nutr. Food Res. 55 (8), 1177–1185. doi:10.1002/mnfr.201000616
Rencber, S. F., Ozbek, S. K., Eraldemir, C., Sezer, Z., Kum, T., Ceylan, S., et al. (2018). Effect of resveratrol and metformin on ovarian reserve and ultrastructure in PCOS: An experimental study. J. Ovarian Res. 11, 55. doi:10.1186/s13048-018-0427-7
Rezvan, N., Moini, A., Gorgani-Firuzjaee, S., and Hosseinzadeh-Attar, M. J. (2018). Oral quercetin supplementation enhances adiponectin receptor transcript expression in polycystic ovary syndrome patients: A randomized placebo-controlled double-blind clinical trial. Cell. J. 19 (4), 627–633. doi:10.22074/cellj.2018.4577
Rosenfield, R. L., and Ehrmann, D. A. (2016). The pathogenesis of polycystic ovary syndrome (PCOS): The hypothesis of PCOS as functional ovarian hyperandrogenism revisited. Endocr. Rev. 37 (5), 467–520. doi:10.1210/er.2015-1104
Rudnicka, E., Suchta, K., Grymowicz, M., Calik-Ksepka, A., Smolarczyk, K., Duszewska, A. M., et al. (2021). Chronic low grade inflammation in pathogenesis of PCOS. Int. J. Mol. Sci. 22 (7), 3789. doi:10.3390/ijms22073789
Ruth, K. S., Day, F. R., Tyrrell, J., Thompson, D. J., Wood, A. R., Mahajan, A., et al. (2020). Using human genetics to understand the disease impacts of testosterone in men and women. Nat. Med. 26(2), 252-+. doi: 258. doi:10.1038/s41591-020-0751-5
Schapira, M., Calabrese, M. F., Bullock, A. N., and Crews, C. M. (2019). Targeted protein degradation: Expanding the toolbox. Nat. Rev. Drug Discov. 18 (12), 949–963. doi:10.1038/s41573-019-0047-y
Schuijt, T. J., Lankelma, J. M., Scicluna, B. P., e Melo, F. d. S., Roelofs, J. J. T. H., de Boer, J. D., et al. (2016). The gut microbiota plays a protective role in the host defence against pneumococcal pneumonia. Gut 65 (4), 575–583. doi:10.1136/gutjnl-2015-309728
Sermondade, N., Huberlant, S., Bourhis-Lefebvre, V., Arbo, E., Gallot, V., Colombani, M., et al. (2019). Female obesity is negatively associated with live birth rate following IVF: A systematic review and meta-analysis. Hum. Reprod. Update 25 (4), 439–451. doi:10.1093/humupd/dmz011
Shah, K. N., and Patel, S. S. (2016). Phosphatidylinositide 3-kinase inhibition: A new potential target for the treatment of polycystic ovarian syndrome. Pharm. Biol. 54 (6), 975–983. doi:10.3109/13880209.2015.1091482
Rotterdam ESHRE/ASRM-Sponsored PCOS consensus workshop group (2004). Revised 2003 consensus on diagnostic criteria and long?term health risks related to polycystic ovary syndrome (PCOS). Hum. Reprod. 19 (1), 41–47. doi:10.1093/humrep/deh098
Shamsudin, N. F., Ahmed, Q. U., Mahmood, S., Shah, S. A. A., Sarian, M. N., Khattak, M., et al. (2022). Flavonoids as antidiabetic and anti-inflammatory agents: A review on structural activity relationship-based studies and meta-analysis. Int. J. Mol. Sci. 23 (20), 12605. doi:10.3390/ijms232012605
Shen, H.-R., Xu, X., and Li, X.-L. (2021a). Berberine exerts a protective effect on rats with polycystic ovary syndrome by inhibiting the inflammatory response and cell apoptosis. Reproductive Biol. Endocrinol. 19 (1), 3. doi:10.1186/s12958-020-00684-y
Shen, H.-R., Xu, X., Ye, D., and Li, X.-L. (2021b). Berberine improves the symptoms of DHEA-induced PCOS rats by regulating gut microbiotas and metabolites. Gynecol. Obstetric Investigation 86 (4), 388–397. doi:10.1159/000518040
Shen, N., Wang, T. F., Gan, Q., Liu, S., Wang, L., and Jin, B. (2022). Plant flavonoids: Classification, distribution, biosynthesis, and antioxidant activity. Food Chem. 383, 132531. doi:10.1016/j.foodchem.2022.132531
Sies, H., and Jones, D. P. (2020). Reactive oxygen species (ROS) as pleiotropic physiological signalling agents. Nat. Rev. Mol. Cell. Biol. 21 (7), 363–383. doi:10.1038/s41580-020-0230-3
Stener-Victorin, E., and Deng, Q. (2021). Epigenetic inheritance of polycystic ovary syndrome - challenges and opportunities for treatment. Nat. Rev. Endocrinol. 17 (9), 521–533. doi:10.1038/s41574-021-00517-x
Sun, Q., Liu, Q., Zhou, X. T., Wang, X. M., Li, H. Y., Zhang, W. F., et al. (2022). Flavonoids regulate tumor-associated macrophages - from structure-activity relationship to clinical potential (Review). Pharmacol. Res. 184, 106419. doi:10.1016/j.phrs.2022.106419
Tay, C. T., Hart, R. J., Hickey, M., Moran, L. J., Earnest, A., Doherty, D. A., et al. (2020). Updated adolescent diagnostic criteria for polycystic ovary syndrome: Impact on prevalence and longitudinal body mass index trajectories from birth to adulthood. Bmc Med. 18 (1), 389. doi:10.1186/s12916-020-01861-x
Teede, H. J., Misso, M. L., Costello, M. F., Dokras, A., Laven, J., Moran, L., et al. (2018). Recommendations from the international evidence-based guideline for the assessment and management of polycystic ovary syndrome. Hum. Reprod. 33 (9), 1602–1618. doi:10.1093/humrep/dey256
Uehara, M. (2013). Isoflavone metabolism and bone-sparing effects of daidzein-metabolites. J. Clin. Biochem. Nutr. 52 (3), 193–201. doi:10.3164/jcbn.13-2
Vidoni, C., Ferraresi, A., Secomandi, E., Vallino, L., Gardin, C., Zavan, B., et al. (2019). Autophagy drives osteogenic differentiation of human gingival mesenchymal stem cells. Cell Commun. Signal. 17 (1), 98. doi:10.1186/s12964-019-0414-7
Wang, W., Zheng, J., Cui, N., Jiang, L., Zhou, H., Zhang, D., et al. (2019). Baicalin ameliorates polycystic ovary syndrome through AMP-activated protein kinase. J. Ovarian Res. 12 (1), 109. doi:10.1186/s13048-019-0585-2
Wang, D., Wang, T., Wang, R., Zhang, X., Wang, L., Xiang, Z., et al. (2020). Suppression of p66Shc prevents hyperandrogenism-induced ovarian oxidative stress and fibrosis. J. Transl. Med. 18 (1), 84. doi:10.1186/s12967-020-02249-4
Wang, J., Qian, X., Gao, Q., Lv, C., Xu, J., Jin, H., et al. (2018). Quercetin increases the antioxidant capacity of the ovary in menopausal rats and in ovarian granulosa cell culture in vitro. J. Ovarian Res. 11, 51. doi:10.1186/s13048-018-0421-0
Wang, Z., Nie, K., Su, H., Tang, Y., Wang, H., Xu, X., et al. (2021). Berberine improves ovulation and endometrial receptivity in polycystic ovary syndrome. Phytomedicine 91, 153654. doi:10.1016/j.phymed.2021.153654
Wang, Z., Zhai, D., Zhang, D., Bai, L., Yao, R., Yu, J., et al. (2017). Quercetin decreases insulin resistance in a polycystic ovary syndrome rat model by improving inflammatory microenvironment. Reprod. Sci. 24 (5), 682–690. doi:10.1177/1933719116667218
Wu, Y.-X., Yang, X.-Y., Han, B.-S., Hu, Y.-Y., An, T., Lv, B.-H., et al. (2022). Naringenin regulates gut microbiota and SIRT1/PGC-1? Signaling pathway in rats with letrozole-induced polycystic ovary syndrome. Biomed. Pharmacother. 153, 113286. doi:10.1016/j.biopha.2022.113286
Yang, Q. C., Leong, S. A., Chan, K. P., Yuan, X. L., and Ng, T. K. (2021). Complex effect of continuous curcumin exposure on human bone marrow-derived mesenchymal stem cell regenerative properties through matrix metalloproteinase regulation. Basic Clin. Pharmacol. Toxicol. 128 (1), 141–153. doi:10.1111/bcpt.13477
Yang, Y., Liu, J., and Xu, W. (2022). Naringenin and morin reduces insulin resistance and endometrial hyperplasia in the rat model of polycystic ovarian syndrome through enhancement of inflammation and autophagic apoptosis. Acta Biochim. Pol. 69 (1), 91–100. doi:10.18388/abp.2020_5722
You, J. W., Cheng, J., Yu, B., Duan, C. H., and Peng, J. H. (2018). Baicalin, a Chinese herbal medicine, inhibits the proliferation and migration of human non-small cell lung carcinoma (NSCLC) cells, A549 and H1299, by activating the SIRT1/AMPK signaling pathway. Med. Sci. Monit. 24, 2126–2133. doi:10.12659/msm.909627
Yu, J., Ding, C., Hua, Z., Jiang, X., and Wang, C. (2021). Protective effects of berberine in a rat model of polycystic ovary syndrome mediated via the PI3K/AKT pathway. J. Obstet. Gynaecol. Res. 47 (5), 1789–1803. doi:10.1111/jog.14730
Yu, J., Liu, Y., Zhang, D., Zhai, D., Song, L., Cai, Z., et al. (2019). Baicalin inhibits recruitment of GATA1 to the HSD3B2 promoter and reverses hyperandrogenism of PCOS. J. Endocrinol. 240 (3), 497–507. doi:10.1530/joe-18-0678
Yuan, X., Hu, T., Zhao, H., Huang, Y., Ye, R., Lin, J., et al. (2016). Brown adipose tissue transplantation ameliorates polycystic ovary syndrome. Proc. Natl. Acad. Sci. U. S. A. 113 (10), 2708–2713. doi:10.1073/pnas.1523236113
Yurtdas, G., and Akdevelioglu, Y. (2020). A new approach to polycystic ovary syndrome: The gut microbiota. J. Am. Coll. Nutr. 39 (4), 371–382. doi:10.1080/07315724.2019.1657515
Zatterale, F., Longo, M., Naderi, J., Raciti, G. A., Desiderio, A., Miele, C., et al. (2020). Chronic adipose tissue inflammation linking obesity to insulin resistance and type 2 diabetes. Front. Physiology 10, 1607. doi:10.3389/fphys.2019.01607
Zhang, L., McClements, D. J., Wei, Z. L., Wang, G. Q., Liu, X. B., and Liu, F. G. (2020a). Delivery of synergistic polyphenol combinations using biopolymer-based systems: Advances in physicochemical properties, stability and bioavailability. Crit. Rev. Food Sci. Nutr. 60 (12), 2083–2097. doi:10.1080/10408398.2019.1630358
Zhang, N., Liu, X., Zhuang, L., Liu, X., Zhao, H., Shan, Y., et al. (2020b). Berberine decreases insulin resistance in a PCOS rats by improving GLUT4: Dual regulation of the PI3K/AKT and MAPK pathways. Regul. Toxicol. Pharmacol. 110, 104544. doi:10.1016/j.yrtph.2019.104544
Zhang, Y., Weng, Y., Wang, D., Wang, R., Wang, L., Zhou, J., et al. (2021). Curcumin in combination with aerobic exercise improves follicular dysfunction via inhibition of the hyperandrogen-induced ire1α/XBP1 endoplasmic reticulum stress pathway in PCOS-like rats. Oxid. Med. Cell. Longev. 2021, 7382900. doi:10.1155/2021/7382900
Zhao, Y., Zhang, C. M., Huang, Y., Yu, Y., Li, R., Li, M., et al. (2015). Up-regulated expression of WNT5a increases inflammation and oxidative stress via PI3K/AKT/NF-kappa B signaling in the granulosa cells of PCOS patients. J. Clin. Endocrinol. Metab. 100 (1), 201–211. doi:10.1210/jc.2014-2419
Zheng, S., Chen, Y., Ma, M., and Li, M. (2022). Mechanism of quercetin on the improvement of ovulation disorder and regulation of ovarian CNP/NPR2 in PCOS model rats. J. Formos. Med. Assoc. 121 (6), 1081–1092. doi:10.1016/j.jfma.2021.08.015
Zhu, T., Cui, J., and Goodarzi, M. O. (2021). Polycystic ovary syndrome and risk of type 2 diabetes, coronary heart disease, and stroke. Diabetes 70 (2), 627–637. doi:10.2337/db20-0800
Keywords: polycystic ovary syndrome, phytochemicals, flavonoid, polyphenol, alkaloid
Citation: Luo E-D, Jiang H-M, Chen W, Wang Y, Tang M, Guo W-M, Diao H-Y, Cai N-Y, Yang X, Bian Y and Xing S-S (2023) Advancements in lead therapeutic phytochemicals polycystic ovary syndrome: A review. Front. Pharmacol. 13:1065243. doi: 10.3389/fphar.2022.1065243
Received: 09 October 2022; Accepted: 12 December 2022;
Published: 09 January 2023.
Edited by:
Qiwei Yang, The University of Chicago, United StatesReviewed by:
Sridhar Muthusami, Karpagam Academy of Higher Education, IndiaCopyright © 2023 Luo, Jiang, Chen, Wang, Tang, Guo, Diao, Cai, Yang, Bian and Xing. This is an open-access article distributed under the terms of the Creative Commons Attribution License (CC BY). The use, distribution or reproduction in other forums is permitted, provided the original author(s) and the copyright owner(s) are credited and that the original publication in this journal is cited, in accordance with accepted academic practice. No use, distribution or reproduction is permitted which does not comply with these terms.
*Correspondence: Ying Bian, eGluZ3NoYXNoYTEyMzBAMTI2LmNvbQ==; Sha-Sha Xing, QmlhbnlpbmdAdW0uZWR1Lm1v
†These authors have contributed equally to this work and share first authorship
Disclaimer: All claims expressed in this article are solely those of the authors and do not necessarily represent those of their affiliated organizations, or those of the publisher, the editors and the reviewers. Any product that may be evaluated in this article or claim that may be made by its manufacturer is not guaranteed or endorsed by the publisher.
Research integrity at Frontiers
Learn more about the work of our research integrity team to safeguard the quality of each article we publish.