- 1Comprehensive Department of Traditional Chinese Medicine, First Department of Integration, Department of Neurology, Putuo Hospital, Shanghai University of Traditional Chinese Medicine, Shanghai, China
- 2Department of Neurology, Renji Hospital Baoshan Branch, School of Medicine, Shanghai Jiao Tong University, Shanghai, China
- 3Department of Neurology, Shanghai Jinshan Hospital of Integrated Traditional Chinese and Western Medicine, Shanghai, China
- 4Department of Neurology, Yueyang Hospital of Integrated Traditional Chinese and Western Medicine, Shanghai University of Traditional Chinese Medicine, Shanghai, China
Ischemic stroke, mostly caused by thromboembolic or thrombotic arterial occlusions, is a primary leading cause of death worldwide with high morbidity and disability. Unfortunately, no specific medicine is available for the treatment of cerebral I/R injury due to its limitation of therapeutic window. Hydroxysafflor yellow A, a natural product extracted from Carthamus tinctorius, has been extensively investigated on its pharmacological properties in cerebrovascular diseases. However, review focusing on the beneficial role of HSYA against cerebral I/R injury is still lacking. In this paper, we reviewed the neuroprotective effect of HSYA in preclinical studies and the underlying mechanisms involved, as well as clinical data that support the pharmacological activities. Additionally, the sources, physicochemical properties, biosynthesis, safety and limitations of HSYA were also reviewed. As a result, HSYA possesses a wide range of beneficial effects against cerebral I/R injury, and its action mechanisms include anti-excitotoxicity, anti-oxidant stress, anti-apoptosis, anti-inflammation, attenuating BBB leakage and regulating autophagy. Collectively, HSYA might be applied as one of the promising alternatives in ischemic stroke treatment.
1 Introduction
Ischemic stroke, which accounts for more than 80% of all strokes, mostly induced by thromboembolic or thrombotic arterial occlusions, is a primary leading cause of death with high morbidity and disability worldwide in current years (Prabhakaran et al., 2015; Katan and Luft, 2018). More than half of the stroke victims remain with neurological deficits including numbness, hemiplegia, balance problems, loss of sensory, decreased reflexes, visual field defects, apraxia, and aphasia which require prolonged rehabilitation (Tsuchiya et al., 1992; Assayag et al., 2012). These neurological deficits are mainly attributed to cerebral ischemia and reperfusion (I/R) injury, which is characterized by a series of pathological events, including inflammatory response (Linnerbauer et al., 2020), oxidative stress (Liu et al., 2020), glutamate toxicity (Dohmen et al., 2005), energy metabolism disorders (Gong et al., 2018), apoptosis (Wen et al., 2019), and many other factors. Since the prevalence rate continues to increase, and the affected population has represented a younger trend, the prevention and treatment of stroke is the significant problem in China (Fu et al., 2020). Recently, treatment options for this stubborn disease are still limited due to the narrow therapeutic window. The recombinant tissue-type plasminogen activators (rtPAs) are nowadays the standard therapeutics in acute ischemic stroke, however the time to initiate intravenous thrombolysis is generally limited to within 4.5 h after the onset of symptoms (Ma et al., 2019).
Based on the theories of traditional Chinese medicine, blood stasis syndrome is thought to be a common clinical syndrome type of ischemic stroke which requires activating blood and resolving stasis method (Wang et al., 2020). The treatment of cardio-cerebra-vascular ischemic diseases by natural medicine is featured by high efficacy and low adverse effects and has a long history with a unique theoretical system (Gu et al., 2014; Chen et al., 2017). Carthamus tinctorius L. is a branching, thistle-like herbaceous annual plant (Figure 1A). The dried florets of Carthamus tinctorius (Figure 1B) has been widely applied in the treatment of cerebrovascular disease for thousands of years (Yu et al., 2018), which is described in the Compendium of Materia Medica as being able to “invigorate the circulation of blood”, suggesting its positive role in the circulation system (Xu et al., 2012).
Hydroxysafflor yellow A (HSYA) is a major bioactive component first isolated from Carthami flos, the flower of Carthamus tinctorius L. in 1993 (Yue et al., 2013), and has been used in the clinical treatment for ischemci cerebrovascular disease. Emerging evidences have revealed that HSYA possesses a wide range of biological activities against I/R injury, such as anti-oxidative, anti-inflammatory, anti-apoptotic effects, relieving BBB damage (Xu et al., 2021), reducing cerebral infarction, attenuating cerebral edema, promoting neurological recovery and improving cognitive function (Yu et al., 2018; Yu et al., 2020a). Thus, HSYA is expected to be developed as a promising candidate for combating ischemic stroke. Up to now, several reviews on traditional Chinese herb have been conducted, in which the therapeutic effects of HSYA on cardio-cerebrovascular diseases were mentioned. However, the pharmacological properties of HSYA against cerebral I/R injury has not been comprehensively reviewed. In this article, we focused on the neuropharmacological properties of HSYA, the therapeutic effects of HSYA in preclinical models of ischemic stroke and the underlying mechanisms involved, as well as clinical data that support its neuroprotective action. Moreover, the sources, physicochemical properties, biosynthesis, and safety of HSYA were also reviewed here.
2 Sources of HSYA
Safflower (Carthamus tinctorius L.), as the natural source of HSYA, is widely planted worldwide. In China, it is also cultivated with planting area of about 30,000 hm2–58,000 hm2 and Xinjiang province is the major safflower production area providing more than 80% dried flowers and seeds (Zhao et al., 2020). Carthami flos, the dried flower of safflower, is a classic medicine for promoting blood circulation and removing blood stasis. Geographical origins, color and harvest time are the main factors influencing the content of HSYA containing in safflower. For example, HSYA cultivars in China are higher than that in Turkey, India and Kenya. The content of HSYA is higher in safflower with darker colors (Xu et al., 2018). And the most appropriate time to pick safflower is the morning of the third or fourth day after the onset of flowering (Tian et al., 2007).
3 Physicochemical properties and biosynthesis
It is well known that safflower yellow is the main active components in Carthami flos extract, including safflower yellow A, safflower yellow B, hydroxysafflow yellow A (HSYA), etc., (Zhang et al., 2016). Among these components, HSYA is the major bioactive component of Carthami flos, accounting for 85% of safflower yellow (Su et al., 2018). HSYA has a stable structure at pH 3-7 and below 60°C. However, it is easily degraded by light, high temperature, strong acidic and alkaline conditions (Fan et al., 2011b). In 1981, a quinochalcone C-glycoside, named safflomin A, was firstly isolated from C. tinctorius by Onodera et al. (1981). Since its 1H and 13C NMR data and other related information were aligned with that of HSYA, the tentative structure Figure 2A was proposed. In 1993, Meselhy et al. (1993) isolated the compound from C. tinctorius and described it as a new quinochalcone C-glycoside. Meanwhile, its structure was identified as Figure 2B and was formally named hydroxysafflor yellow A. In 2013, Feng et al. put forward that HSYA was a mixture of two keto-enol tautomeric forms (Figures 2C,D), with the 1-enol-3,7-diketo form, is the preferred tautomer (Feng et al., 2013) which amended the conclusion that HSYA belongs to “quinochalcone-C-glycoside structures”. Nowadays, HSYA is mainly obtained from plants at about 1%–3%. Planting environment, harvesting time and anthropogenic induction are the significant factors to increase the yield of HSYA. Additionally, HSYA is produced mainly through the phenylalanine metabolic pathway, of which chalcone synthase genes (CHSs) are the rate-limiting enzymes (Xue et al., 2021). CHSs expression and HSYA accumulation are peaked after 3–4 days of flowering (Kang, 2014). It’s worth noting that enzymatic catalysis is efficient to promote biosynthesis of natural products. Methyl jasmonate (MeJA), a well-known exogenous inducing factor, has been reported to promote the biosynthesis of HSYA through regulating the expression of upstream and downstream genes in the flavonoid biosynthesis pathway, such as CHSs, CHIs, F3Ms, ANRs etc (Chen et al., 2020).
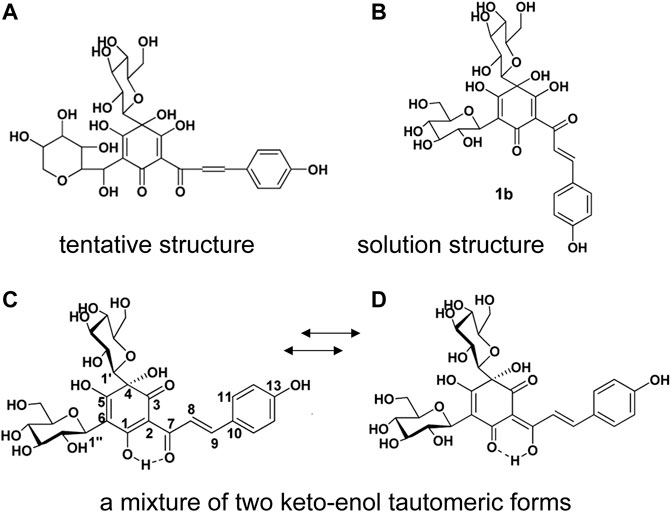
FIGURE 2. The structure of HSYA. (A) The tentative structure identified by Onodera et al., 1981, (B) the structure identified by Meselhy et al., (C, D) the structure identified by Feng et al., 2013.
4 Protective effects and mechanisms
Ischemic stroke occurs when the blockage of brain artery causes a reduction of regional cerebral blood flow, resulting in deleterious effects on neurons followed by a series of pathological processes such as excitotoxicity, oxidative stress, inflammatory response, neuronal apoptosis, BBB disruption and autophagy (Figure 3). Since extensive work aims to explore neuroprotective therapeutics for stroke, multiple animal models have been developed to reproduce both focal and global ischemic stroke. Middle cerebral artery occlusion (MCAO) model is the most widely used experimental model for inducing focal cerebral ischemia in rodents, while four vessel occlusion and two vessel occlusion methods are commonly used in global cerebral ischemia (Bacigaluppi et al., 2010). Various studies have indicated that HSYA dose-dependently improves neurological deficit scores, reduces cerebral infarct volume, attenuates brain edema and recovers cognitive impairment (Zhu et al., 2005; Yu et al., 2018; Yu et al., 2020a). The initial mechanisms are closely associated with inhibitory effect of HSYA on thrombosis formation and platelet aggregation following focal cerebral ischemia. And HSYA could improve blood rheological parameters as well (Zhu et al., 2005). In contrast, it is reported that HSYA administration has no impact on cerebral blood flow, blood pressure and heart rate in Beagle dogs (Sun et al., 2018). The discrepancies would be explained by differences in animal model. In vitro studies revealed that HSYA protected brain microvascular endothelial cells (BMECs) injury induced by oxygen and glucose deprivation/reoxygenation (OGD/R) via inhibiting autophagy, which was associated with its regulation of the Class I PI3K/AKT/mTOR pathway (Yang et al., 2018). Moreover, HSYA exhibits protective action on neuronal damage following glutamate and sodium cyanide (NaCN) exposure in fetal cortical cells (Zhu et al., 2003). These results indicate that HSYA is a promising therapeutic agent for cerebral I/R injury treatment. To further support the its positive role in ischemic stroke, we review the underlying mechanisms which are correlated with the activities of HSYA to inhibit excitotoxicity, oxidative stress, inflammation, apoptosis, BBB damage, as well as regulate autophagy on below.
4.1 Inhibiting excitotoxicity
Excitotoxicity is a primary stage of neuronal injury following cerebral ischemia. It is triggered by neuronal stimulation with high concentration of glutamate and overactivation of glutamate receptors (Wang et al., 2018). N-methyl-d-aspartate (NMDA) subtype of glutamate receptors plays an important role in mediating glutamate accumulation at synapses, which is caused by high permeability of calcium (Lai et al., 2014). Overactivation of NMDA receptors (NMDARs) containing the NR2A and NR2B subunits is the pivotal reason in glutamate-provoked excitotoxic neuronal damage (Yang et al., 2010). Yang et al. (2010) conducted an in vitro study on NMDA-induced injury in rat primary neurons to investigate the effect of HSYA on NMDAR-mediated neurotoxicity. HSYA was claimed to attenuate the excitotoxic neuronal death, meanwhile over-expression of NR2B subtype by NMDA stimuli was reversed by HSYA, which indicated the neuroprotection of HSYA against NMDA-induced neuronal glutamate excitotoxicity. Since an excessive glutamate release triggers excitotoxic damage through the overactivation of NMDARs following brain ischemia (Soriaet al., 2014), Wang et al. further investigated the related mechanism of HSYA’s protective effect against glutamatergic excitotoxicity in NMDA-mediated and OGD-induced neuronal injury (Wang et al., 2016). HSYA was observed to inhibit postsynaptic NMDAR activity and NMDAR-mediated neuronal membrane depolarization under oxygen and glucose deprivation circumstance. Meanwhile, it was further confirmed to suppress pre-synaptic glutamate transmitter release (Wang et al., 2016). Moreover, through NMDAR-dependent manner, intracellular rapid influx of calcium initiated by glutamate release has been verified to be responsible for neuronal excitotoxicity (Tehse and Taghibiglou, 2019). Apart from that, HSYA was demonstrated to inhibit the increase of NMDAR-mediated Ca2+ concentration and NMDAR-dependent ischemic long-term potentiation (LTP) induced by OGD for protecting hippocampal neurons from excitotoxic damage (Wang et al., 2016). Taken together, these studies indicate that HSYA could ameliorate neuronal excitotoxicity after cerebral I/R injury via suppressing the overactivation of NMDARs, and consequently inhibiting excessive neurotransmitter release, neuronal membrane depolarization, overload of calcium and ischemic LTP, which are mediated by or depend on NMDAR.
4.2 Ameliorating oxidative stress
It is well known that anti-free radical system of nervous tissues is relatively weaker than other organs in the human body, which means that neurons are more prone to oxidative damage than other tissues (Xie et al., 2018). Under cerebral ischemic conditions, several pathological mechanisms, including neuronal excitotoxicity, excessive Ca2+ influx, mitochondrial dysfunction, may cause free radical damage (Yu et al., 2020b). In rat brain mitochondria, Tian et al. (2008) revealed that HSYA could inhibit Ca2+- and H2O2-induced swelling of mitochondria and generation of ROS, enhance ATP levels and improve mitochondrial energy metabolism. In PC12 cells and primary hippocampal neurons, Fan and Fangma et al. provided the in vitro evidence that HSYA could attenuate neuronal damage via reversing the decrease of superoxide dismutase (SOD) and glutathioneperoxidase (GSH-Px) activity, suppressing the increase of reactive oxygen species (ROS) and malondialdehyde (MDA) levels after OGD/R-induced injury (Wei et al., 2005; Fan et al., 2011a; Fangma et al., 2021). Since the release of cytochrome c from mitochondria has been evidenced to be mediated by ROS (Chung et al., 2021), HSYA was further demonstrated to significantly decrease the cytochrome c in the cytosol (Fan et al., 2011a). The exact mechanisms underlying the antioxidant effects of HSYA remain unclear. Silent information regulator 1 (SIRT1), a deacetylase, is involved in the regulation of cell survival, energy metabolism, anti-apoptosis (Ding et al., 2017). It has been proved to exert a positive role in cerebral ischemic injury (Ding et al., 2017). In MCAO rats and OGD/R-injured primary neurons, Fangma et al. (2021) provided the evidence that HSYA regulated the SIRT1 pathway. However, the effect of HSYA on SIRT1 was restrained with SIRT1-specific inhibitor EX527, suggesting the pivotal role of SIRT1 in neuroprotection of HSYA. Ferroptosis and parthanatos are two types of programmed cell death associated with cerebral ischemia. Excessive ROS may stimulate cell death pathway and trigger a series inflammation reaction (Tang et al., 2019). In OGD/R-insulted PC12 cells, Chen et al. (2022) found that HSYA limited ferroptosis and parthanatos to alleviate oxidative stress through suppressing PARP-1 overactivation and attenuating the production of excessive PAR polymer and translocation of AIF nuclear. Moreover, the excessive generation of ROS activates opening of mitochondrial permeability transition pore (mPTP) during I/R injury to further increase ROS production resulting in mitochondrial dysfunction, which is considered as a critical contributor to neuronal damage (Granger and Kvietys, 2015). In MCAO rats, Ramagiri et al. verified that HSYA could inhibit mPTP opening induced by oxidative stress (Ramagiri and Taliyan, 2016). HSYA was also proved to suppress the overexpression of 12/15-LOX, the enzyme involved in oxidative stress after MCAO (Sun et al., 2012). Collectively, these studies demonstrate that HSYA could mitigate oxidative stress evoked by I/R injury through increasing SOD and GSH-Px activity, inhibiting ROS and MDA levels, decreasing the cytochrome c in the cytosol, upregulating the SIRT1 pathway, suppressing mPTP opening, as well as limiting ferroptosis and parthanatos.
4.3 Anti-inflammation
Neuroinflammation has been recognized as a crucial pathological process following cerebral ischemia-reperfusion injury (Sun et al., 2020), which is characterized by the production of inflammatory cyto- and chemokines, as well as the infiltration of leukocyte into ischemic tissues (Franke et al., 2021). HSYA has been exhibited an anti-inflammatory role in both MCAO rats and OGD/R-injured neurons (Ye and Gao, 2008). In MCAO mice and LPS-treated microglia and neurons, HSYA was found to suppress the excessive secretion of inflammatory cytokines through inhibiting TLR4-mediated signaling pathway (Lv et al., 2015; Lv et al., 2016). In another study, HSYA was demonstrated to improve OGD/R-injured BV2 microglia viability by limiting pro-inflammatory cytokines (Li et al., 2013). Glycogen synthase kinase-3 (GSK-3) is a serine-threonine kinase composed of both alpha and beta isoforms (Eldar-Finkelman and Martinez, 2011), which has been evidenced to participate in the production of pro-inflammatory factors. Thus, inhibition of this kinase has been recognized as a molecular brake to limit inflammatory response (Cai et al., 2021). In MCAO rats, Yang et al. (2020) found that HSYA elevated GSK-3β phosphorylation levels and suppressed nuclear factor kappa B (NF-κB) activation in the ischemic penumbra, which manifested its anti-inflammatory properties by regulating GSK-3β. Glial fibrillary acidic protein (GFAP) is a crucial cytoskeletal component of astrocytes, as the contributor to trigger inflammatory response once excessively activated. Deng et al. revealed that HSYA attenuated inflammatory response through upregulating GFAP and reversing the increasing level of intercellular adhesion molecular 1(ICAM-1) in MCAO rats (Deng et al., 2018). Meanwhile, elevated inflammatory mediators, such as IL-1β, TNF-α and NF-κB were suppressed by HSYA (Deng et al., 2018). These results suggest that suppressing the TLR4-mediated pathway and TLR4-induced downstream effectors, increasing GSK-3β phosphorylation and GFAP expression contributed to the anti-inflammatory effects of HSYA following cerebral I/R injury.
4.4 Anti-apoptosis
Apoptosis is one of the two types of cell death produced by cerebral ischemia injury, which is triggered by either extrinsic or intrinsic stimuli (Radak et al., 2017). The intrinsic stimuli for apoptosis are via a series of mitochondrial signaling pathways (Yu et al., 2020b). Huang et al. found that HSYA increased viability of brain microvascular endothelial cells (BMECs) after OGD/R (Huang et al., 2021). Additionally, HSYA decreased the export of cytochrome c from mitochondrial by inhibiting mPTP opening via the regulation of MEK/ERK/CypD pathway in both OGD/R and MCAO models (Huang et al., 2021). Moreover, HSYA was identified to enhance mitochondrial function and biogenesis via inhibiting phenylalanine synthesis in OGD/R-injured primary neurons and PC12 cells (Chen et al., 2019). PH domain leucine-rich repeat protein phosphatase-1 (PHLPP1) has been found to participate in the regulation of cell survival and cell apoptosis (Aviv and Krishenbaum, 2010). PHLPP1 gene deletion could ameliorate cerebral ischemic injury implying its critical role in neuroprotection (Chen B. et al., 2013). In OGD/R-treated BMECs, HSYA was found to reverse the increased PHLPP1 evoked by OGD/R and its protective action was abolished once PHLPP1 knockout, which indicated that HSYA attenuated cellular apoptosis following ischemic-reperfusion injury in PHLPP1-dependent manner (Cao et al., 2020). A growing number of literatures demonstrated that the activation of PI3K/Akt pathway ameliorated I/R-induced apoptosis (Chang et al., 2018; Zhou et al., 2021). Moreover, glycogen synthase kinase 3β (GSK3β), an important downstream target of the Akt signaling, has been evidenced to regulate key steps involved in intrinsic apoptotic and extrinsic apoptotic pathways (Chen et al., 2014). In MCAO rats, Chen et al. (2013b) provided the consistent results that HSYA inhibited cellular apoptosis following I/R injury by increasing the phosphorylation levels of Akt and GSK3β. Furthermore, the application of wortmannin, an inhibitor of PI3K, revealed that PI3K/Akt pathway played a positive role in HSYA-mediated neuroprotective effect (Chen L. et al., 2013). Altogether, these studies demonstrate that HSYA could attenuate apoptosis after cerebral I/R injury by inhibiting mPTP open via MEK/ERK/CypD pathway, enhancing mitochondrial function and biogenesis, increasing PHLPP1 level, as well as regulating PI3K/Akt/GSK3β pathway.
4.5 Attenuating BBB damage
The blood-brain barrier (BBB), a unique anatomical and physiological interface between peripheral circulation and central nervous system (Daneman and Prat, 2015), regulates the trafficking of solutes, fluid and cells at blood-brain interface (Jiang et al., 2018). BBB integrity will be damaged under cerebral ischemia condition, leading to the development of brain injury and subsequent neurological impairment (Abdullahi et al., 2018). Tan et al. constructed an in vitro BBB model to verify that FDA-approved adenosine receptor agonist Lexiscan (Lex) promoted HSYA accumulation in the brain by transitory enhancement of BBB permeability. Accordingly, the combination of HSYA and Lex exhibited a better protective performance against I/R injury in MCAO rats than the single HSYA (Tan et al., 2020). Moreover, in MCAO rats, Sun et al. (2012) demonstrated that HSYA reduced the increase of serum IgG following brain ischemia by more than 50%, indicating the amelioration effect of HSYA against BBB disruption subjected to cerebral ischemia. Lv et al. used the integrated method of serial affinity chromatography and shotgun proteomics analysis to explore the underlying mechanism of HSYA’s protective effect on BBB damage in anti-inflammatory patterns in MCAO mice (Lv and Fu, 2018). The data showed that HSYA regulated the tight junction via TLR4/PI3K/AKT/JNK1/2/14-3-3ε/NF-κBp65 pathway and modulated BBB permeability via suppressing inflammation (Lv and Fu, 2018). Li et al. (2022) performed photothrombotic stroke model in C57BL/6J mice to imitate cerebral ischemia, in which HSYA was verified to protect ZO-1 stability, a tight junction protein, for reducing cerebral vascular leakage via blocking HIF-1α/NOX2 signaling cascades (Li et al., 2022). The findings demonstrated the significant role of HIF-1α in NOXs activation and the regulatory effect of HSYA on HIF-1α/NOX2 signaling cascades for protecting cerebral vessel integrity. Apart from that, the caveolin pathway has been found to play an important role in preserving and protecting BBB integrity (Huang et al., 2018). Caveolin-1 (Cav-1) could reduce BBB permeability destroyed by ischemic stroke through downregulating MMP9 (Huang et al., 2018). In OGD/R-injured BMECs, Cao et al. provided consistent results that HSYA exerted neuroprotective property by stimulating Cav-1 pathway, which validated the functioning of HSYA in rescuing BBB (Cao et al., 2020). These results indicate that HSYA may attenuate BBB leakage after I/R injury via regulating the tight junction, stimulating the caveolin-1 pathway and blocking HIF-1α/NOX2 signaling cascades.
4.6 Regulating autophagy
Autophagy is a cellular catabolic process that acts as a double-edged sword under pathological conditions, contributing to either cell survival or cell damage (Martinet et al., 2009). It is commonly agreed that autophagy is closely associated with heart disease, cancer and neurodegenerative disease (Martinet et al., 2009). In recent years, growing evidence has revealed that autophagy confers cytoprotection against various pathological stresses, including ischemia/reperfusion injury (Lai et al., 2020). In MCAO rats, Qi et al. (2014) found that HSYA promoted autophagy in the penumbra through activating AKT-dependent autophagy pathway, which was subsequently verified as a potential mechanism in the HSYA-mediated neuroprotection. In contrast, Yang et al. (2018); Zhang et al. (2022) observed that HSYA inhibited autophagy following ischemia for exerting neuroprotection in OGD/R-insulted BMECs and MCAO rats, which is inconsistent with the observation of Qi et al. A body of studies has demonstrated that autophagy increases in cerebral I/R injury, and both harmful and protective potentials of autophagy have been reported (Wang et al., 2013; Luo et al., 2014). Yang et al. (2018) found that HSYA suppressed excessive autophagy evoked by OGD/R injury in BMECs and such inhibition was partially associated with the activation of Class I PI3K/AKT/mTOR pathway. Similarly, Zhang et al. (2022) revealed that HSYA suppressed autophagy induced by brain ischemia probably through limiting the expressions of HIF-1, BNIP3 and Notch 1. All together, these results demonstrate that HSYA may regulate autophagy induced by cerebral I/R injury via activating AKT-related pathway and the Class I PI3K/AKT/mTOR pathway, as well as decreasing the expressions of HIF-1, BNIP3 and Notch 1.
It is known that ischemic stroke involves several major pathogeneses including excitotoxicity, oxidative stress, inflammation, apoptosis and BBB damage etc. As described above, HSYA exerts potent neuroprotection against cerebral I/R injury through complex signaling pathways and exhibits a definite therapeutic effect for brain ischemia treatment (Figure 4).
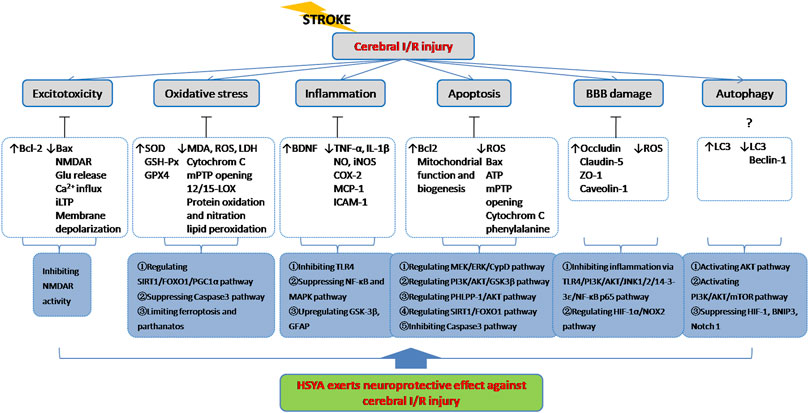
FIGURE 4. HSYA acts on the functional targets and signaling pathways of cerebral I/R injury. Indicator: ↑, upgrade; ↓, downgrade; ?, undetermined.
5 Clinical application
Safflow yellow injection (SYI) contains 90% HSYA (45 mg HSYA per 50 mg SYI), and it has been reported to use clinically for ischemic stroke (Li L. J. et al., 2015). Li et al. (2015b) provided clinical evidence that SYI exerts beneficial effect for acute cerebral infarction. The study was a prospective, single-blinded, and randomized controlled trial and conducted in 108 patients after informed consent and screening. All patients were randomized to either SYI group or control group. SYI (80 mg) was given to the SYI group and placebo (0 mg) injection was given to the control group by intravenous drop once daily for 14 days. The results showed that SYI improved neurological deficits and hemorheological index, including red blood cell deformation and red blood cell aggregation. Prothrombin time was increased and fibrinogen, TNF-α, IL-1β and IL-6 were decreased in patients treated with SYI on day 14 after treatment (Li L. J. et al., 2015). Hu et al. conducted a multicenter, randomized, double-blind, multiple-dose and active-controlled clinical trial for assessing effect and safety of HSYA injection in 266 patients with acute ischemic stroke of blood stasis syndrome. 25 mg/d, 50 mg/d, and 70 mg/d HSYA injection were administrated by intravenous infusion for 14 consecutive days. Scores of NIHSS and BI at days 90 after treatment as well as improvement degree of blood stasis syndrome at days 30 and 60 after treatment in the medium- and high-dose HSYA groups were higher than the control group. Thus, HSYA injection was proved to be safe and well-tolerated at all doses for acute ischemic stroke patients with blood stasis syndrome (Hu et al., 2020). Although a growing number of preclinical studies have displayed the significant protective potential of HSYA against cerebral I/R injury, there is still a lack of convincing evidence with high methodological quality for the efficacy and safety of HSYA in acute cerebral infarction treatment.
6 Safety
Subchronic toxicity studies in SD rats signified a safety concern of HSYA (Liu et al., 2004). The exposure of HSYA at the doses of 180, 60 mg/kg by daily intraperitoneal injection for 90 days period caused a prolonged blood coagulation time. However, the normal blood coagulation process wasn’t influenced. No animal was observed to die from hemorrhaging. Kidney injury, including round tubular figures and a breaking-off of the tubular epithelium in histological slices, was observed in the rats with 180 mg/kg HSYA. Although the liver index was increased with 180 mg/kg HSYA, no pathological change of liver histiocytes has been found. The data indicated that the great amount of HSYA is excreted by kidney and induces a slight nephrotoxicity (Liu et al., 2004). On the other hand, some factors in drug metabolism will cause unsafe events. In a clinical experiment of 36 Chinese healthy adults, single doses (25 mg, 50 mg, and 75 mg) of injectable powder of pure HSYA (IPPH) displayed moderate linear pharmacokinetic properties. And seven successive days’ administration of IPPH didn’t cause the in vivo drug accumulation, but leaded to the decrease of its system exposure and prolonging of the drug elimination time (Li C. Y. et al., 2015). It is important to note that HSYA can either inhibit or induce activities of CYP1A2, CYP2C11 and CYP3A1, which may be correlated with the significant changes of maximum plasma concentration (Cmax) and area under the plasma concentration versus time curve (AUC) after multiple drug administration. Thus, co-administration of some CYP substrates with HSYA may need dose adjustment to avoid some herb-drug interaction (Xu et al., 2014). Besides, the Cmax and AUC of female was generally larger than that of male, which may be influenced by sex differences in body weight, proportions of muscular and adipose tissues, gastrointestinal and renal blood flows, drug enzyme activity and hormonal factors (Li et al., 2011). Therefore, in the clinical application of HSYA, the drug dosage, the combination of drugs and the physical condition of patients should be fully considered to avoid adverse reaction.
7 Limitations and further perspectives
Although HSYA might be applied as one of the promising alternatives in ischemic stroke treatment, there still remain some limitations. Firstly, HSYA is easily degraded in the process of storage, extraction and separation procedure due to the chemical instability. Secondly, although oral administration is of great significance among many administration routes because of its convenience and safety, HSYA generally possesses low oral bioavailability probably caused by its low membrane permeability, which decreases the effective concentration in vivo (Sajid et al., 2021). Since many challenges still exist to limit HSYA clinical application, a large number of studies have been conducted to explore the improvement of chemical instability and bioavailability. Self-double-emulsifying drug delivery system (SDEDDS) has been used to enhance the oral absorption of HSYA and verified no significant toxicity in vitro and in vivo (Lv et al., 2012). HSYA solid lipid nanoparticles prepared by a warm microemulsion process using approved drug excipients for oral delivery has been demonstrated to increase the oral absorption of HSYA with little cytotoxicity (Zhao et al., 2018). Generally speaking, studies in synthetic biology and metabolic engineering should be further conducted to help improve efficacy, stability, bioavailability and pharmacokinetic properties for accelerating HSYA clinical application.
Since Carthami flos is a common part of preparation used in traditional Chinese medicine (TCM), it is necessary to further carry out the compatibility research of HSYA. For example, HSYA and Danshensu achieve synergistic protective effects on cerebral I/R injury through anti-inflammatory and oxidative pathways (Xu et al., 2017). HSYA and astragaloside IV could decrease blood viscosity, plasma viscosity, and attenuate oxidative stress in MCAO rats (Cao et al., 2014). On the other hand, combinations with existing western medication may provide new therapy option. It is reported that HSYA together with acetylglutamine attenuated inflammation and apoptosis process following brain ischemia, and the combination of two drugs exhibited a synergetic effect (Deng et al., 2018). However, it should be pointed out that HSYA can either inhibit or induce activities of CYP1A2, CYP2C11 and CYP3A1 (Xu et al., 2014). Comedication of HSYA with drugs metabolized by CYP1A2 and CYP2C11 will probably result in herb-drug interactions. Hence, more advanced clinical studies should be conducted for fully assessing the safety of HSYA and exploring new compound formulas with HSYA, which may bring benefits not only to brain ischemia patients but also TCM modernization as well.
8 Conclusion
In the present review, we summarized the materials about HSYA, including sources, physicochemical properties, safety, protective effects against cerebral I/R and molecular mechanisms in vivo (Table 1) and in vitro (Table 2). As a natural compound extracted from Chinese herbal, HSYA exerts extensive pharmacological effects in ischemic stroke treatment, including inhibiting excitotoxicity, ameliorating oxidative stress, suppressing inflammation and apoptosis, modulating BBB permeability and regulating autophagy. HSYA suppresses the overactivation of NMDARs following cerebral I/R injury and inhibits excessive release of neurotransmitters, neuronal membrane depolarization, overload of calcium and ischemic LTP mediated by NMDAR to ameliorate excitotoxicity. On the other hand, HSYA increases SOD activity and decrease ROS generation for suppressing oxidative stress, accompanied by attenuating mPTP opening. Apart from that, HSYA has been found to limit ferroptosis and parthanatos for protecting cells from oxidative stress. In addition, HSYA exerts anti-inflammatory effects under cerebral I/R circumstance mainly through activating TLR4-mediated pathway. Moreover, attenuated apoptosis by enhancement of mitochondrial function and improved BBB leakage via tight junction regulation also contribute to HSYA-mediated neuroprotective effect against brain ischemic insult. Finally, HSYA ameliorates cerebral I/R injury by regulating autophagy signaling pathway. Therefore, as a potential therapeutic agent for HSYA with a significant application prospect, further study is necessary to be carried out by adopting advanced technologies and methods.
The last but not the least, although a growing number of preclinical studies show the neuroprotective potential of HSYA against cerebral I/R injury, only few clinical studies included patients with acute ischemic stroke have been reported (Li L. J. et al., 2015; Hu et al., 2020). The randomized controlled clinical trial (RCT) is regarded as the “gold standard” for evaluating the effectiveness of drugs. To evaluate the efficacy and safety of HSYA in treating ischemic stroke, more high-quality, multi-center, large-sample, randomized double-blind controlled trials are urgently needed.
Author contributions
LY and QZ designed and drafted the review. ZJ, ML, and HL searched the literature and modified the manuscript. JT and CX examined the literature. LW made the figures and edited the manuscript. All authors read and approved the final manuscript.
Funding
This work was supported in part by grants from the National Natural Science Foundation of China (81503370 to LY), Natural Science Foundation of Shanghai (19ZR1447900 to LY), Clinical Research Project of Shanghai Municipal Health Commission (202040150 to LY), Youth Talent Promotion Project of China Association of Chinese Medicine (CACM-2019-QNRC2-C10 to JT), Construction Project of Putuo District TCM Clinical Key Specialty (ptzyzk2108), Project for Capacity Promotion of Putuo District Clinical Special Disease (2019tszb02).
Conflict of interest
The authors declare that the research was conducted in the absence of any commercial or financial relationships that could be construed as a potential conflict of interest.
Publisher’s note
All claims expressed in this article are solely those of the authors and do not necessarily represent those of their affiliated organizations, or those of the publisher, the editors and the reviewers. Any product that may be evaluated in this article, or claim that may be made by its manufacturer, is not guaranteed or endorsed by the publisher.
References
Abdullahi, W., Tripathi, D., and Ronaldson, P. T. (2018). Blood-brain barrier dysfunction in ischemic stroke: Targeting tight junctions and transporters for vascular protection. Am. J. Physiol. Cell Physiol. 315, C343–c356. doi:10.1152/ajpcell.00095.2018
Assayag, E. B., Korczyn, A. D., Giladi, N., Goldbourt, U., Berliner, A. S., Shenhar-Tsarfaty, S., et al. (2012). Predictors for poststroke outcomes: The tel aviv brain acute stroke cohort (TABASCO) study protocol. Int. J. Stroke 7, 341–347. doi:10.1111/j.1747-4949.2011.00652.x
Aviv, Y., and Kirshenbaum, L. A. (2010). Novel phosphatase PHLPP-1 regulates mitochondrial Akt activity and cardiac cell survival. Circ. Res. 107, 448–450. doi:10.1161/CIRCRESAHA.110.225896
Bacigaluppi, M., Comi, G., and Hermann, D. M. (2010). Animal models of ischemic stroke. Part two: Modeling cerebral ischemia. Open Neurol. J. 4, 34–38. doi:10.2174/1874205X01004020034
Cai, M., Sun, S., Wang, J., Dong, B., Yang, Q., Tian, L., et al. (2021). Sevoflurane preconditioning protects experimental ischemic stroke by enhancing anti-inflammatory microglia/macrophages phenotype polarization through GSK-3β/Nrf2 pathway. CNS Neurosci. Ther. 27, 1348–1365. doi:10.1111/cns.13715
Cao, J., Chen, Z., Zhu, Y., Li, Y., Guo, C., Cao, K., et al. (2014). Huangqi-Honghua combination and its main components ameliorate cerebral infarction with Qi deficiency and blood stasis syndrome by antioxidant action in rats. J. Ethnopharmacol. 155, 1053–1060. doi:10.1016/j.jep.2014.05.061
Cao, J., Wang, K., Lei, L., Bai, L., Liang, R., Qiao, Y., et al. (2020). Astragaloside and/or hydroxysafflor yellow A attenuates oxygen-glucose deprivation-induced cultured brain microvessel endothelial cell death through downregulation of PHLPP-1. Evid. Based. Complement. Altern. Med. 2020, 3597527. doi:10.1155/2020/3597527
Chang, Y., Huang, W., Sun, Q., Li, S., Yan, Z., Wang, Q., et al. (2018). MicroRNA-634 alters nerve apoptosis via the PI3K/Akt pathway in cerebral infarction. Int. J. Mol. Med. 42, 2145–2154. doi:10.3892/ijmm.2018.3777
Chen, B., Winkle, J. A. Van., Lyden, P. D., Brown, J. H., and Purcell, N. H. (2013a). PHLPP1 gene deletion protects the brain from ischemic injury. J. Cereb. Blood Flow. Metab. 33, 196–204. doi:10.1038/jcbfm.2012.150
Chen, G., Li, C., Zhang, L., Yang, J., Meng, H., Wan, H., et al. (2022). Hydroxysafflor yellow A and anhydrosafflor yellow B alleviate ferroptosis and parthanatos in PC12 cells injured by OGD/R. Free Radic. Biol. Med. 179, 1–10. doi:10.1016/j.freeradbiomed.2021.12.262
Chen, H. S., Qi, S. H., and Shen, J. G. (2017). One-Compound-Multi-Target: Combination prospect of natural compounds with thrombolytic therapy in acute ischemic stroke. Curr. Neuropharmacol. 15, 134–156. doi:10.2174/1570159x14666160620102055
Chen, J., Wang, J., Wang, R., Xian, B., Ren, C., Liu, Q., et al. (2020). Integrated metabolomics andtranscriptome analysis on flavonoidbiosynthesis in safflower (Carthamustinctorius L.) under MeJA treatment. BMC Plant Biol. 20, 353. doi:10.1186/s12870-020-02554-6
Chen, L., Wei, X., Hou, Y., Liu, X., Li, S., Sun, B., et al. (2014). Tetramethylpyrazine analogue CXC195 protects against cerebral ischemia/reperfusion-induced apoptosis through PI3K/Akt/GSK3β pathway in rats. Neurochem. Int. 66, 27–32. doi:10.1016/j.neuint.2014.01.006
Chen, L., Xiang, Y., Kong, L., Zhang, X., Sun, B., Wei, X., et al. (2013b). Hydroxysafflor yellow A protects against cerebral ischemia-reperfusion injury by anti-apoptotic effect through PI3K/Akt/GSK3β pathway in rat. Neurochem. Res. 38, 2268–2275. doi:10.1007/s11064-013-1135-8
Chen, S., Sun, M., Zhao, X., Yang, Z., Liu, W., Cao, J., et al. (2019). Neuroprotection of hydroxysafflor yellow A in experimental cerebral ischemia/reperfusion injury via metabolic inhibition of phenylalanine and mitochondrial biogenesis. Mol. Med. Rep. 19, 3009–3020. doi:10.3892/mmr.2019.9959
Chung, Y., Kim, Y., Yun, N., and Oh, J. Y. (2021). Dysregulated autophagy is linked to BAX oligomerization and subsequent cytochrome c release in 6-hydroxydopmaine-treated neuronal cells. Biochem. Biophys. Res. Commun. 548, 20–26. doi:10.1016/j.bbrc.2021.02.045
Daneman, R., and Prat, A. (2015). The blood-brain barrier. Cold Spring Harb. Perspect. Biol. 7, a020412. doi:10.1101/cshperspect.a020412
Deng, L., Wan, H., Zhou, H., Yu, L., and He, Y. (2018). Protective effect of hydroxysafflor yellow A alone or in combination with acetylglutamine on cerebral ischemia reperfusion injury in rat: A pet study using (18)F-fuorodeoxyglucose. Eur. J. Pharmacol. 825, 119–132. doi:10.1016/j.ejphar.2018.02.011
Ding, P., Ren, D., He, S., He, M., Zhang, G., Chen, Y., et al. (2017). Sirt1 mediates improvement in cognitive defects induced by focal cerebral ischemia following hyperbaric oxygen preconditioning in rats. Physiol. Res. 66, 1029–1039. doi:10.33549/physiolres.933544
Dohmen, C., Kumura, E., Rosner, G., Heiss, W., D., and Graf, R. (2005). Extracellular correlates of glutamate toxicity in short-term cerebral ischemia and reperfusion: A direct in vivo comparison between white and gray matter. Brain Res. 1037, 43–51. doi:10.1016/j.brainres.2004.12.046
Eldar-Finkelman, H., and Martinez, A. (2011). GSK-3 inhibitors: Preclinical and clinical focus on CNS. Front. Mol. Neurosci. 4, 32. doi:10.3389/fnmol.2011.00032
Fan, L., Dang, X., Shi, Z., Zhang, C., and Wang, K. (2011a). Hydroxysafflor yellow A protects PC12 cells against the apoptosis induced by oxygen and glucose deprivation. Cell. Mol. Neurobiol. 31, 1187–1194. doi:10.1007/s10571-011-9720-3
Fan, L., Pu, R., Zhao, H. Y., Liu, X., Ma, C., Wang, B. R., et al. (2011b). Stability anddegradation of hydroxysafflor yellow A and anhydrosafflor yellow B in the Safflowerinjection studied by HPLC-DAD-ESI-MS. J. Chin. Pharm. Sci. 20, 47–56.
Fangma, Y., Zhou, H., Shao, C., Yu, L., Yang, J., Wan, H., et al. (2021). Hydroxysafflor yellow A and anhydrosafflor yellow B protect against cerebral ischemia/reperfusion injury by attenuating oxidative stress and apoptosis via the silent information regulator 1 signaling pathway. Front. Pharmacol. 12, 739864. doi:10.3389/fphar.2021.739864
Feng, Z. M., He, J., Jiang, J. S., Chen, Z., Yang, Y. N., and Zhang, P. C. (2013). NMR solution structure study of the representative ComponentHydroxysafflor yellow A and other quinochalcone C-glycosides fromCarthamus tinctorius. J. Nat. Prod. 76, 270–274. doi:10.1021/np300814k
Franke, M., Bieber, M., Kraft, P., Weber, A. N. R., Stoll, G., and Schuhmann, M. K. (2021). The NLRP3 inflammasome drives inflammation in ischemia/reperfusion injury after transient middle cerebral artery occlusion in mice. Brain Behav. Immun. 92, 223–233. doi:10.1016/j.bbi.2020.12.009
Fu, H., Zhang, D., Zhu, R., Cui, L., Qiu, L., Lin, S., et al. (2020). Association between lipoprotein (a) concentration and the risk of stroke in the Chinese han population: A retrospective case-control study. Ann. Transl. Med. 8, 212. doi:10.21037/atm.2020.01.38
Gong, Z., Pan, J., Shen, Q., Li, M., and Peng, Y. (2018). Mitochondrial dysfunction induces NLRP3 inflammasome activation during cerebral ischemia/reperfusion injury. J. Neuroinflammation 15, 242. doi:10.1186/s12974-018-1282-6
Granger, D. N., and Kvietys, P. R. (2015). Reperfusion injury and reactive oxygen species: The evolution of a concept. Redox Biol. 6, 524–551. doi:10.1016/j.redox.2015.08.020
Gu, Y., Chen, J., and Shen, J. (2014). Herbal medicines for ischemic stroke: Combating inflammation as therapeutic targets. J. Neuroimmune Pharmacol. 9, 313–339. doi:10.1007/s11481-014-9525-5
Hu, M., Zhou, Z., Zhou, Z., Lu, H., Gao, M., Liu, L., et al. (2020). Effect and safety of hydroxysafflor yellow A for injection in patients with acute ischemic stroke of blood stasis syndrome: A phase II, multicenter, randomized, double-blind, multiple-dose, active-controlled clinical trial. Chin. J. Integr. Med. 26, 420–427. doi:10.1007/s11655-020-3094-7
Huang, P., Wu, S. P., Wang, N., Seto, S., and Chang, D. (2021). Hydroxysafflor yellow A alleviates cerebral ischemia reperfusion injury by suppressing apoptosis via mitochondrial permeability transition pore. Phytomedicine. 85, 153532. doi:10.1016/j.phymed.2021.153532
Huang, Q., Zhong, W., Hu, Z., and Tang, X. (2018). A review of the role of cav-1 in neuropathology and neural recovery after ischemic stroke. J. Neuroinflammation 15, 348. doi:10.1186/s12974-018-1387-y
Jiang, X., Andjelkovic, A. V., Zhu, L., Yang, T., Bennett, M. V. L., Chen, J., et al. (2018). Blood-brain barrier dysfunction and recovery after ischemic stroke. Prog. Neurobiol. 163-164, 144–171. doi:10.1016/j.pneurobio.2017.10.001
Kang, Y. L. (2014). The expression of chalcone synthase gene and the analysis of the accumulation of safflor yellow in Carthamus tinctorius L. Chengdu: Chengdu University of Traditional Chinese Medicine.
Katan, M., and Luft, A. (2018). Global burden of stroke. Semin. Neurol. 38, 208–211. doi:10.1055/s-0038-1649503
Lai, T. W., Zhang, S., and Wang, Y. T. (2014). Excitotoxicity and stroke: Identifying novel targets for neuroprotection. Prog. Neurobiol. 115, 157–188. doi:10.1016/j.pneurobio.2013.11.006
Lai, Z., Gu, L., Yu, L., Chen, H., Yu, Z., Zhang, C., et al. (2020). Delta opioid peptide [d-Ala2, d-Leu5] enkephalin confers neuroprotection by activating delta opioid receptor-AMPK-autophagy axis against global ischemia. Cell Biosci. 10, 79. doi:10.1186/s13578-020-00441-z
Li, C. Y., Yin, J. G., Zhang, J., Wang, X. X., Xu, M. J., Liu, F., et al. (2015a). Pharmacokinetic profiles of hydroxysafflor yellow A followingintravenous administration of its pure preparations in healthyChinese volunteers. J. Ethnopharmacol. 162, 225–230. doi:10.1016/j.jep.2014.12.068
Li, J., Zhang, S., Lu, M., Chen, Z., Chen, C., Han, L., et al. (2013). Hydroxysafflor yellow A suppresses inflammatory responses of BV2 microglia after oxygen-glucose deprivation. Neurosci. Lett. 535, 51–56. doi:10.1016/j.neulet.2012.12.056
Li, L. J., Li, Y. M., Qiao, B. Y., Jiang, S., Li, X., Du, H. M., et al. (2015b). Corrigendum to the value of safflower yellow injection for the treatment of acute cerebral infarction: A randomized controlled trial". Evid. Based. Complement. Altern. Med. 2015, 4270317. doi:10.1155/2016/4270317
Li, Y., Liu, X. T., Zhang, P. L., Li, Y. C., Sun, M. R., Wang, Y. T., et al. (2022). Hydroxysafflor yellow A blocks HIF-1α induction of NOX2 and protects ZO-1 protein in cerebral microvascular endothelium. Antioxidants (Basel) 11, 728. doi:10.3390/antiox11040728
Li, Z., TenHoor, C., Marbury, T., Swan, S., Ticho, B., Rogge, M., et al. (2011). Clinical pharmacokinetics of tonapofylline: Evaluation of dose proportionality, oral bioavailability, and gender and food effects in healthy human subjects. J. Clin. Pharmacol. 51, 1004–1014. doi:10.1177/0091270010377633
Linnerbauer, M., Wheeler, M. A., and Quintana, F. J. (2020). Astrocyte crosstalk in CNS inflammation. Neuron 108, 608–622. doi:10.1016/j.neuron.2020.08.012
Liu, D., Wang, H., Zhang, Y., and Zhang, Z. (2020). Protective effects of chlorogenic acid on cerebral ischemia/reperfusion injury rats by regulating oxidative stress-related Nrf2 pathway. Drug Des. devel. Ther. 14, 51–60. doi:10.2147/DDDT.S228751
Liu, Z., Li, C., Li, M., Li, D., and Liu, K. (2004). The subchronic toxicity of hydroxysafflor yellow A of 90 days repeatedly intraperitoneal injections in rats. Toxicology 203, 139–143. doi:10.1016/j.tox.2004.06.007
Luo, T., Liu, G., Ma, H., Lu, B., Xu, H., Wang, Y., et al. (2014). Inhibition of autophagy via activation of PI3K/Akt pathway contributes to the protection of ginsenoside Rb1 against neuronal death caused by ischemic insults. Int. J. Mol. Sci. 15, 15426–15442. doi:10.3390/ijms150915426
Lv, L. Z., Tong, C. Q., Lv, Q., Tang, X. J., Li, L. M., Fang, Q. X., et al. (2012). Enhanced absorption of hydroxysafflor yellow A using a self-double-emulsifying drug delivery system: In vitro and in vivo studies. Int. J. Nanomedicine 7, 4099–4107. doi:10.2147/IJN.S33398
Lv, Y., and Fu, L. (2018). The potential mechanism for Hydroxysafflor yellow A attenuating blood-brain barrier dysfunction via tight junction signaling pathways excavated by an integrated serial affinity chromatography and shotgun proteomics analysis approach. Neurochem. Int. 112, 38–48. doi:10.1016/j.neuint.2017.10.012
Lv, Y., Qian, Y., Fu, L., Chen, X., Zhong, H., and Wei, X. (2015). Hydroxysafflor yellow A exerts neuroprotective effects in cerebral ischemia reperfusion-injured mice by suppressing the innate immune TLR4-inducing pathway. Eur. J. Pharmacol. 769, 324–332. doi:10.1016/j.ejphar.2015.11.036
Lv, Y., Qian, Y., Ou-Yang, A., and Fu, L. (2016). Hydroxysafflor yellow A attenuates neuron damage by suppressing the lipopolysaccharide-induced TLR4 pathway in activated microglial cells. Cell. Mol. Neurobiol. 36, 1241–1256. doi:10.1007/s10571-015-0322-3
Ma, H., Campbell, B. C. V., Parsons, M. W., Churilov, L., Levi, C. R., Hsu, C., et al. (2019). Thrombolysis guided by perfusion imaging up to 9 hours after onset of stroke. N. Engl. J. Med. 380, 1795–1803. doi:10.1056/NEJMoa1813046
Martinet, W., Agostinis, P., Vanhoecke, B., Dewaele, M., and De Meyer, G. R. (2009). Autophagy in disease: A double-edged sword with therapeutic potential. Clin. Sci. (Lond). 116, 697–712. doi:10.1042/CS20080508
Meselhy, M. R., Kadota, S., Momose, Y., Hatakeyama, N., Kusai, A., Hattori, M., et al. (1993). Two new quinochalcone yellow pigments from Carthamus tinctorius and Ca2+ antagonistic activity of tinctormine. Chem. Pharm. Bull. (Tokyo). 41, 1796–1802. doi:10.1248/cpb.41.1796
Onodera, J., Obara, H., Osone, M., Maruyama, Y., and Sato, S. (1981). The structure of safflomin-A, a component of safflower yellow. Chem. Lett. 10, 433–436. doi:10.1246/cl.1981.433
Prabhakaran, S., Ruff, I., and Bernstein, R. A. (2015). Acute stroke intervention: A systematic review. Jama 313, 1451–1462. doi:10.1001/jama.2015.3058
Qi, Z., Yan, F., Shi, W., Zhang, C., Dong, W., Zhao, Y., et al. (2014). AKT-related autophagy contributes to the neuroprotective efficacy of hydroxysafflor yellow A against ischemic stroke in rats. Transl. Stroke Res. 5, 501–509. doi:10.1007/s12975-014-0346-x
Radak, D., Katsiki, N., Resanovic, I., Jovanovic, A., Sudar-Milovanovic, E., Zafirovic, S., et al. (2017). Apoptosis and acute brain ischemia in ischemic stroke. Curr. Vasc. Pharmacol. 15, 115–122. doi:10.2174/1570161115666161104095522
Ramagiri, S., and Taliyan, R. (2016). Neuroprotective effect of hydroxy safflor yellow A against cerebral ischemia-reperfusion injury in rats: Putative role of mPTP. J. Basic Clin. Physiol. Pharmacol. 27, 1–8. doi:10.1515/jbcpp-2015-0021
Sajid, M., Channakesavula, C. N., Stone, S. R., and Kaur, P. (2021). Synthetic biology towards improved flavonoid pharmacokinetics. Biomolecules 11, 754. doi:10.3390/biom11050754
Soria, F. N., Pérez-Samartín, A., Martin, A., Gona, K. B., Llop, J., Szczupak, B., et al. (2014). Extrasynaptic glutamate release through cystine/glutamate antiporter contributes to ischemic damage. J. Clin. Invest. 124, 3645–3655. doi:10.1172/JCI71886
Su, J., Tang, H., Li, L., Jiang, D., and Kuai, S. (2018). A study on the separation ofHydroxysafflor yellow A from safflower yellow pigment. J.Kunming. Univ.Sci.Technol (NatSci). 43, 95–99.
Sun, L., Yang, L., Xu, Y. W., Liang, H., Han, J., Zhao, R. J., et al. (2012). Neuroprotection of hydroxysafflor yellow A in the transient focal ischemia: Inhibition of protein oxidation/nitration, 12/15-lipoxygenase and blood-brain barrier disruption. Brain Res. 1473, 227–235. doi:10.1016/j.brainres.2012.07.047
Sun, R., Peng, M., Xu, P., Huang, F., Xie, Y., Li, J., et al. (2020). Low-density lipoprotein receptor (LDLR) regulates NLRP3-mediated neuronal pyroptosis following cerebral ischemia/reperfusion injury. J. Neuroinflammation 17, 330. doi:10.1186/s12974-020-01988-x
Sun, Y., Xu, D. P., Qin, Z., Wang, P. Y., Hu, B. H., Yu, J. G., et al. (2018). Protective cerebrovascular effects of hydroxysafflor yellow A (HSYA) on ischemic stroke. Eur. J. Pharmacol. 818, 604–609. doi:10.1016/j.ejphar.2017.11.033
Tan, L., Wang, Y., Jiang, Y., Wang, R., Zu, J., and Tan, R. (2020). Hydroxysafflor yellow A together with blood-brain barrier regulator lexiscan for cerebral ischemia reperfusion injury treatment. ACS Omega 5, 19151–19164. doi:10.1021/acsomega.0c02502
Tang, D., Kang, R., Berghe, T. V., Vandenabeele, P., and Kroemer, G. (2019). The molecular machinery of regulated cell death. Cell Res. 29, 347–364. doi:10.1038/s41422-019-0164-5
Tehse, J., and Taghibiglou, C. (2019). The overlooked aspect of excitotoxicity: Glutamate-independent excitotoxicity in traumatic brain injuries. Eur. J. Neurosci. 49, 1157–1170. doi:10.1111/ejn.14307
Tian, J., Li, G., Liu, Z., and Fu, F. (2008). Hydroxysafflor yellow A inhibits rat brain mitochondrial permeability transition pores by a free radical scavenging action. Pharmacology 82, 121–126. doi:10.1159/000141653
Tian, L., Wu, G., and Wang, Y. (2007). Quality assessment of carthamustinctorius L. In emin country, tacheng prefecture, Xinjiang. China. Pharm. 16, 5–7.
Tsuchiya, M., Sako, K., Yura, S., and Yonemasu, Y. (1992). Cerebral blood flow and histopathological changes following permanent bilateral carotid artery ligation in Wistar rats. Exp. Brain Res. 89, 87–92. doi:10.1007/BF00229004
Wang, J., Gan, Y., Han, P., Yin, J., Liu, Q., Ghanian, S., et al. (2018). Ischemia-induced neuronal cell death is mediated by chemokine receptor CX3CR1. Sci. Rep. 8, 556. doi:10.1038/s41598-017-18774-0
Wang, P. R., Wang, J. S., Zhang, C., Song, X. F., Tian, N., and Kong, L. Y. (2013). Huang-Lian-Jie-Du-Decotion induced protective autophagy against the injury of cerebral ischemia/reperfusion via MAPK-mTOR signaling pathway. J. Ethnopharmacol. 149, 270–280. doi:10.1016/j.jep.2013.06.035
Wang, X., Ma, Z., Fu, Z., Gao, S., Yang, L., Jin, Y., et al. (2016). Hydroxysafflor yellow A protects neurons from excitotoxic death through inhibition of NMDARs. ASN Neuro 8, 1759091416642345. doi:10.1177/1759091416642345
Wang, Y., Zhang, L., Pan, Y., Fu, W., Huang, S., Xu, B., et al. (2020). Investigation of invigorating Qi and activating blood circulation prescriptions in treating Qi deficiency and blood stasis syndrome of ischemic stroke patients: Study protocol for a randomized controlled trial. Front. Pharmacol. 11, 892. doi:10.3389/fphar.2020.00892
Wei, X., Liu, H., Sun, X., Fu, F., Zhang, X., Wang, J., et al. (2005). Hydroxysafflor yellow A protects rat brains against ischemia-reperfusion injury by antioxidant action. Neurosci. Lett. 386, 58–62. doi:10.1016/j.neulet.2005.05.069
Wen, L., Liu, L., Li, J., Tong, L., Zhang, K., Zhang, Q., et al. (2019). NDRG4 protects against cerebral ischemia injury by inhibiting p53-mediated apoptosis. Brain Res. Bull. 146, 104–111. doi:10.1016/j.brainresbull.2018.12.010
Xie, W., Zhou, P., Sun, Y., Meng, X., Dai, Z., Sun, G., et al. (2018). Protective effects and target network analysis of ginsenoside Rg1 in cerebral ischemia and reperfusion injury: A comprehensive overview of experimental studies. Cells 7, 270.
Xu, H., Liu, W., Liu, T., Su, N., Guo, C., Feng, X., et al. (2017). Synergistic neuroprotective effects of Danshensu and hydroxysafflor yellow A on cerebral ischemia-reperfusion injury in rats. Oncotarget 8, 115434–115443. doi:10.18632/oncotarget.23272
Xu, J., Wang, Y., Li, N., Xu, L., Yang, H., and Yang, Z. (2012). L-3-n-butylphthalide improves cognitive deficits in rats with chronic cerebral ischemia. Neuropharmacology 62, 2424–2429. doi:10.1016/j.neuropharm.2012.02.014
Xu, J., Zhan, T., Zheng, W., Huang, Y. K., Chen, K., Zhang, X. H., et al. (2021). Hydroxysafflor yellow A acutely attenuates blood-brain barrier permeability, oxidative stress, inflammation and apoptosis in traumatic brain injury in rats1. Acta Cir. Bras. 35, e351202. doi:10.1590/ACB351202
Xu, L., Liang, H., Yu, Y., Tan, Z., Yang, H., Dong, W., et al. (2018). Carthamus tinctorius L.: Evaluation on correlations of hydroxysafflor with flower color and the difference among cultivars. Chin. Agricu. Sci. Bull. 34, 41–45.
Xu, R. A., Xu, Z. S., and Ge, R. S. (2014). Effects of hydroxysafflor yellow A on the activity and mRNA expression of four CYP isozymes in rats. J. Ethnopharmacol. 151, 1141–1146. doi:10.1016/j.jep.2013.12.025
Xue, X., Deng, Y., Wang, J., Zhou, M., Liao, L., Wang, C., et al. (2021). Hydroxysafflor yellow A, a natural compound from Carthamus tinctorius L with good effect of alleviating atherosclerosis. Phytomedicine. 91, 153694. doi:10.1016/j.phymed.2021.153694
Yang, G., Wang, N., Seto, S. W., Chang, D., and Liang, H. (2018). Hydroxysafflor yellow a protects brain microvascular endothelial cells against oxygen glucose deprivation/reoxygenation injury: Involvement of inhibiting autophagy via class I PI3K/Akt/mTOR signaling pathway. Brain Res. Bull. 140, 243–257. doi:10.1016/j.brainresbull.2018.05.011
Yang, Q., Yang, Z. F., Liu, S. B., Zhang, X. N., Hou, Y., Li, X. Q., et al. (2010). Neuroprotective effects of hydroxysafflor yellow A against excitotoxic neuronal death partially through down-regulation of NR2B-containing NMDA receptors. Neurochem. Res. 35, 1353–1360. doi:10.1007/s11064-010-0191-6
Yang, X., Chen, L., Li, Y., Gao, F., Yan, Z., Zhang, P., et al. (2020). Protective effect of Hydroxysaf flor Yellow A on cerebral ischemia reperfusion-injury by regulating GSK3β-mediated pathways.flor Yellow A on cerebral ischemia reperfusion-injury by regulating GSK3β-mediated pathways. Neurosci. Lett. 736, 135258. doi:10.1016/j.neulet.2020.135258
Ye, S. Y., and Gao, W. Y. (2008). Hydroxysafflor yellow A protects neuron against hypoxia injury and suppresses inflammatory responses following focal ischemia reperfusion in rats. Arch. Pharm. Res. 8, 1010–1015. doi:10.1007/s12272-001-1261-y
Yu, L., Duan, Y., Zhao, Z., He, W., Xia, M., Zhang, Q., et al. (2018). Hydroxysafflor yellow A (HSYA) improves learning and memory in cerebral ischemia reperfusion-injured rats via recovering synaptic plasticity in the Hippocampus. Front. Cell. Neurosci. 12, 371. doi:10.3389/fncel.2018.00371
Yu, L., Liu, Z., He, W., Chen, H., Lai, Z., Duan, Y., et al. (2020a). Hydroxysafflor yellow A confers neuroprotection from focal cerebral ischemia by modulating the crosstalk between JAK2/STAT3 and SOCS3 signaling pathways. Cell. Mol. Neurobiol. 40, 1271–1281. doi:10.1007/s10571-020-00812-7
Yu, L., Tao, J., Zhao, Q., Xu, C., and Zhang, Q. (2020b). Confirmation of potential neuroprotective effects of natural bioactive compounds from traditional medicinal herbs in cerebral ischemia treatment. J. Integr. Neurosci. 19, 373–384. doi:10.31083/j.jin.2020.02.63
Yue, S., Tang, Y., Li, S., and Duan, J. A. (2013). Chemical and biological properties of quinochalcone C-glycosides from the florets of Carthamus tinctorius. Molecules 18, 15220–15254. doi:10.3390/molecules181215220
Zhang, L. L., Tian, K., Tang, Z. H., Chen, X. J., Bian, Z. X., Wang, Y. T., et al. (2016). Phytochemistry and Pharmacology of Carthamus tinctorius L. Am. J. Chin. Med. 44, 197–226. doi:10.1142/S0192415X16500130
Zhang, Y., Liu, Y., Cui, Q., Fu, Z., Yu, H., Liu, A., et al. (2022). Hydroxysafflor yellow A alleviates ischemic stroke in rats via HIF-1[Formula: See text], BNIP3, and notch1-mediated inhibition of autophagy. Am. J. Chin. Med. 50, 799–815. doi:10.1142/S0192415X22500331
Zhao, B., Gu, S., Du, Y., Shen, M., Liu, X., and Shen, Y. (2018). Solid lipid nanoparticles as carriers for oral delivery of hydroxysafflor yellow A. Int. J. Pharm. 535, 164–171. doi:10.1016/j.ijpharm.2017.10.040
Zhao, F., Wang, P., Jiao, Y., Zhang, X., Chen, D., and Xu, H. (2020). Hydroxysafflor yellow A: ASystematical review on BotanicalResources, PhysicochemicalProperties, drug delivery system, pharmacokinetics, andPharmacological effects. Front. Pharmacol. 11, 579332. doi:10.3389/fphar.2020.579332
Zhou, Z., Xu, N., Matei, N., McBride, D. W., Ding, Y., Liang, H., et al. (2021). Sodium butyrate attenuated neuronal apoptosis via GPR41/Gβγ/PI3K/Akt pathway after MCAO in rats. J. Cereb. Blood Flow. Metab. 41, 267–281. doi:10.1177/0271678X20910533
Zhu, H. B., Zhang, L., Wang, Z. H., Tian, J. W., Fu, F. H., Liu, K., et al. (2005). Therapeutic effects of hydroxysafflor yellow A on focal cerebral ischemic injury in rats and its primary mechanisms. J. Asian Nat. Prod. Res. 7, 607–613. doi:10.1080/10286020310001625120
Zhu, H., Wang, Z., Ma, C., Tian, J., Fu, F., Li, C., et al. (2003). Neuroprotective effects of hydroxysafflor yellow A: In vivo and in vitro studies. Planta Med. 69, 429–433. doi:10.1055/s-2003-39714
Glossary
I/R ischemia/reperfusion
BI brain ischemia
tPA tissue-type plasminogen activator
FDA Food and Drug Adminstration
HSYA hydroxysafflor yellow A
MCAO middle cerebral artery occlusion
CHS chalcone synthase
CHI chalcone isomerase
F3Ms flavonoid 3′-monooxygenase
ANRs anthocyanidin reductase
MeJA methyl jasmonate
BMECs brain microvascular endothelial cells
OGD/R oxygen and glucose deprivation/reoxygenation
PI3K phosphoinositide 3-kinase
Akt protein kinase B
mTOR mammalian target of rapamycin
NaCN sodium cyanide
NMDA N-methyl-d-aspartate
NMDAR N-methyl-d-aspartate-receptor
LTP long-term potentiation
SOD superoxide dismutase
GSH-Px glutathione peroxidase
ROS reactive oxygen species
MDA malondialdehyde
SIRT1 silent information regulator 1
GPX4 glutathione peroxidase 4
PAR poly (ADP-ribose)
PARP-1 poly (ADP-ribose) polymerase-1
AIF apoptosis inducing factor
mPTP mitochondrial permeability transition pore
LPS lipopolysaccharide
GSK-3 glycogen synthase knase-3
NF-κB nuclear factor kappa B
GFAP glial fibrillary acidic protein
ICAM-1 intercellular adhesion molecular 1
IL-1β interleukin 1β
TNF-α tumor necrosis factor-α
TLR-4 Toll-linke receptor-4
MEK mitogen-activated protein kinase kinase
ERK extracellular signal-regulated kinase
CypD cyclophilin D
PHLPP1 PH domain leucine-rich repeat protein phosphatase-1
BBB blood-brain barrier
Lex Lexiscan
JNK c-Jun NH2-terminal kinase
ZO-1 zonula occludens-1
HIF-1α hypoxia-inducible transcription factor-1α
NOXs NADPH oxidases
Cav-1 caveolin-1
MMP9 matrix metalloproteinase 9
SYI safflow yellow injection
IPPH injectable powder of pure HSYA
CYP cytochrome P450
Cmax changes of maximum plasma concentration
AUC area under the plasma concentration versus time curve
SDEDDS self-double-emulsifying drug delivery system
NIHSS National Institute of Health Stroke Scale
BI Barthel Index
TCM traditional Chinese medicine
RCT controlled clinical trial.
Keywords: hydroxysafflor yellow A, cerebral ischemia-reperfusion injury, natural bioactive compounds, neuroprotection, pathophysiological mechanism, review
Citation: Yu L, Jin Z, Li M, Liu H, Tao J, Xu C, Wang L and Zhang Q (2022) Protective potential of hydroxysafflor yellow A in cerebral ischemia and reperfusion injury: An overview of evidence from experimental studies. Front. Pharmacol. 13:1063035. doi: 10.3389/fphar.2022.1063035
Received: 06 October 2022; Accepted: 06 December 2022;
Published: 15 December 2022.
Edited by:
Kah Hui Wong, University of Malaya, MalaysiaReviewed by:
Yunxia Luo, Shenzhen Hospital of Guangzhou University of Chinese Medicine, ChinaJaydeep Roy, The University of Hong Kong, Hong Kong SAR, China
Copyright © 2022 Yu, Jin, Li, Liu, Tao, Xu, Wang and Zhang. This is an open-access article distributed under the terms of the Creative Commons Attribution License (CC BY). The use, distribution or reproduction in other forums is permitted, provided the original author(s) and the copyright owner(s) are credited and that the original publication in this journal is cited, in accordance with accepted academic practice. No use, distribution or reproduction is permitted which does not comply with these terms.
*Correspondence: Lu Yu, lyy299@hotmail.com; Qiujuan Zhang, qiujuanzhang0000@hotmail.com; Liwei Wang, wlw_pt@hotmail.com
†These authors have contributed equally to this work