- 1The Second Affiliated Hospital and Yuying Children’s Hospital of Wenzhou Medical University, Wenzhou, China
- 2School of Pharmaceutical Science, Liaoning University, Shenyang, China
Patrinia villosa (Thunb.) Juss (P.V) is widely used in the treatment of chronic diseases, such as appendicitis, enteritis and gynecological inflammation. Modern research indicated that the herb has pharmacological effect on liver injury caused by inflammation, but the metabolomics mechanism is not clear. For the purpose of discovering the therapeutic effect and metabolomic mechanism of P.V on liver injury, 40 Sprague-Dawley (SD) rats were divided into normal group, model group, and P.V groups (0.98, 1.97, and 2.96 g/kg). The model group and P.V groups were injected intraperitoneally with 40% CCl4 (v/v, olive oil) to establish liver injury model. After administration of P.V for seven consecutive days. Therapeutic effect of P.V on liver injury rats were analyzed. P.V could decrease serum alanine aminotransferase (ALT) and aspartate aminotransferase (AST) levels of liver injury rats as a dose-dependent manner. Compared with the model group, the pathological analysis of liver tissue of P.V groups exhibit significant decrease tendency of hepatic tissue structure destruction, cytoplasmic vacuolation, cellular swelling, and inflammatory cell infiltration as a dose-dependent manner. 82 endogenous metabolites in rat serum and liver were analyzed by Ultra-high performance liquid chromatography tandem mass spectrometry (UPLC-MS/MS). 14 metabolites in serum and 26 metabolites in liver were significantly different between the P.V group (2.96 g/kg) and the model group. Metabolic pathway analysis revealed that the main pathway including alanine, aspartate and glutamate metabolism, and TCA cycle were significantly altered. It is suggested that P.V can alleviate CCl4 induced liver injury, and its effect on metabolites may be an important mechanism of action.
1 Introduction
The liver is an important metabolic organ of the human body. It mainly has the physiological functions of storing glycogen, decomposing blood sugar, and participating in the metabolism of protein, fat, vitamins and hormones (Wu et al., 2017a). The liver not only regulates metabolism, but also has the function of detoxification, phagocytosis and defense against foreign toxic substances (Mega et al., 2021; Qian et al., 2021). The extensive application of toxic chemicals, drugs, alcohol, dietary supplements and food additives may cause liver injury (Yang et al., 2017). Liver cell injury will cause inflammatory reaction, oxidative stress, fat-like changes, and then a series of pathological changes such as liver cell necrosis, apoptosis and fibrosis (Navarro et al., 2017; Kong et al., 2021).
Liver injury is progresses to the stage of liver failure, which has clinical manifestations such as fatigue, anorexia, abdominal fullness and loose stools (Wang et al., 2021). It is often delayed due to insufficient attention, and severe cases can even cause liver failure and endanger life (Jaeschke et al., 2012). Poor prognosis after liver injury have always been a problem in the medical community (Khemichian et al., 2021). In modern medicine, symptomatic treatment is often used clinically to treat liver injury (Navarro et al., 2017). At the same time of treatment, it lacks comprehensive consideration of systemic pathological state, which will cause harm to the body (Sun et al., 2014).
Patrinia villosa (Thunb.) Juss (P.V) is a traditional Chinese medicine which is commonly used in the treatment of chronic inflammation diseases, such as intestinal carbuncle, pulmonary carbuncle, dysentery, postpartum petechial abdominal pain, and carbuncle (Huang et al., 2022). Modern medical research has found that the herb has various pharmacological activities such as anti-inflammatory, sedative and hypnotic, anti-viral, anti-tumor, liver-protecting, and immunity-enhancing actions (Zheng et al., 2012; Li et al., 2016; Sheng et al., 2019), but its mechanism of liver-protection action has not been reported.
Chemical investigation indicated that saponins, flavonoids, coumarins, cycloethenes terpenoids, lignans, and volatile oils are the main active ingredients of P.V (Peng et al., 2005; Xiang et al., 2016; Xin-Jia et al., 2016; Yang et al., 2016). P.V can inhibit the hepatitis virus, improve liver function, and promote hepatocyte regeneration and bile secretion (Liao et al., 2012; Dai et al., 2013). Previous study indicated apigenin in P.V could inhibit hepatocellular carcinoma cell growth by CyclinD1/CDK4 regulation via p38 MAPK-p21 signaling (Li et al., 2016). In this study, the therapeutic effect of P.V on liver injury was studied, and the metabolic mechanism was analyzed by UPLC-MS/MS.
2 Materials and methods
2.1 Materials and reagents
Patrinia villosa (Thunb.) Juss was obtained from Xinqi Traditional Chinese Medicine Pellets Co., Ltd (Hebei, China), and the herb was identified by Dr. Jian Wu from Harbin University of Commerce. A voucher specimen (No. PVJ20130821) was deposited at the Pharmacognosy Laboratory of Liaoning University. L-tryptophan (Try), L-kynurenine (Kyn), Oxidizided glutathione (GSSG), N-phenylacetylglycine (N-Phe), 5-hydroxytryptophan (5-HTP), L-leucine (Leu), 5-HT, cholic acid (CA), and Glutathione (GSH) were obtained from Dalian Melon Biology Technology Co., N-Ethylmaleimide (NEM) and formic acid were purchased from Sigma-Aldrich Chemical Co., PBS buffer was obtained from Hyclone Chemical Co., Internal standard (L-phenylalanine-d5, d5-Phe) was obtained from Cambridge isotope laboratories. All standard stock solutions were stored at -40 °C before use. Methanol and acetonitrile were purchased from ACS Chemical Co.
2.2 Preparation of Patrinia villosa (Thunb.) Juss extract
960 g of P.V was refluxed with 9.6 L of distilled water for 1.5 h, before being cooled, the supernatant was filtered and concentrated under reduced pressure gave extract residue of 90.29 g. Samples were stored at 4°C in a refrigerator before use.
2.3 Animals and treatments
A total of 40 male Sprague-Dawley rats, weighing about 200 ± 20 g, were divided into five groups (n = 8) including normal group, model group and P.V groups with three dosages (0.98, 1.97, and 2.96 g/kg). After 2 weeks of adaptive feeding, the P.V groups and model group were injected intraperitoneally with 40% CCl4 (v/v, olive oil) at the dose 2 ml/kg (Wang et al., 2021). P.V groups were intragastric administered once daily for 7 days, the normal group and model group were intragastric administered with the same volume of saline once daily for 7 days. All the rats were anesthetized with sodium pentobarbital intraperitoneally 2 h after administration on the seventh day and blood samples were collected from the abdominal aorta and exposed to laparotomy. Blood was collected in a non-anticoagulant tube, and serum was collected after centrifugation at 3,500 rpm and 4 °C for 10 min. Rat livers were washed with normal saline after routine sampling and then fixed in formalin solution for the preparation of pathological sections of liver tissue. The histopathological changes in the rat livers were observed by microscope after HE staining.
2.4 Metabolic analysis
Metabolic analysis was performed according to the previous report with minor modification (Wang et al., 2022). In this study, 82 endogenous metabolites involved in oxidative stress, inflammation, amino acid metabolism, purine metabolism, the tricarboxylic acid cycle, glycolysis, and lipid metabolism were selected as targets.
For the preparation of stripped serum, 1.8 g of activated carbon powder was added to 30 ml of rat serum. After mixing in a shaker for 2 h, the mixture was centrifuged at 13,500 rpm for 20 min at 4°C. The supernatant was collected and filtered with Millipore express PES membranes (Merck Millipore, Ltd.) in order of 5, 1.2, 0.45 and 0.22 μm, respectively, to prepare the stripped serum samples.
For the preparation of standard solutions, L-tryptophan (Try), L-kynurenine (Kyn), oxidizided glutathione (GSSG), N-phenylacetylglycine (N-Phe), 5-hydroxytryptophan (5-HTP), L-leucine (Leu), 5-HT and cholic acid (CA) were dissolved in methanol and prepared to a final concentration of 1 mg/ml. Glutathione (GSH) was dissolved in 10 mM of NEM PBS buffer to a final concentration of 0.5 mg/ml. All standard stock solutions were stored at -40°C and protected from light. L-phenylalanine-d5 (d5-Phe) was used as the internal standard, and a 10 ng/ml stock solution was prepared in methanol and stored at –40°C. The components were mixed, and distilled water was used to prepare standard curve solutions and diluted with 50% methanol-water to prepare a series of standard solutions. Because of the degradability of the GSH component, to ensure its quantitative assay accuracy and its component stability, GSH was derivatized with the reaction of NEM and GSH at an NEM concentration of 50 mM (Carretero et al., 2014; Younossi et al., 2016; Wang et al., 2022).
200 μL of PBS solution containing 10 mM of NEM and 1,200 μL of methanol (containing 10 ng/ml of d5-Phe) was added to 200 μL of serum in the tube and incubated at -20°C for 20 min. After vortex mixing, centrifugation was performed at 13,500 rpm and 4°C for 15 min 1,000 μL of supernatant were collected and blown dry by nitrogen to obtain samples. Samples were reconstituted with 50 μL of 5% acetonitrile (ACN) in water and centrifuged at 13,500 rpm and 4°C for 15 min. Then, 30 μL of the supernatant was pipetted into a sample tube for metabolic analysis.
0.2 g of liver tissue was weighted precisely, and 10 times the volume of normal saline were added in a centrifuge tube and homogenized, then the samples were centrifuged at 12,000 rpm for 15 min 100 μL of PBS solution containing 10 mM of NEM and 800 μL of methanol (containing 10 ng/ml of d5-Phe) was added to 100 μL of supernatant in the tube and incubated at -20°C for 20 min. After vortex mixing, centrifugation was performed at 13,500 rpm and 4°C for 15 min 800 μL of supernatant were collected and blown dry by nitrogen to obtain samples. Samples were reconstituted with 50 μL of 5% acetonitrile (ACN) in water and centrifuged at 13,500 rpm and 4°C for 15 min. Then, 30 μL of the supernatant was pipetted into a sample tube for metabolic analysis.
Metabolic analysis was performed on an AB4000Q TRAP LC/MS/MS mass spectrometer with deuterated internal standards (IS) (Phe-d5) as the internal standard. The column used was Waters X BridgeTM BEH C18 2.5 μm, 3.0 × 100 mm. Positive and negative ions were quantified and semi-quantified using an ESI ion source detector. UPLC was performed using 0.1% formic acid-water (A) and acetonitrile (B) as elution systems. The gradient separation conditions were 0–2 min, 5% (B); 2–5 min, 5–50% (B); 5–6 min, 50% (B); 6–17 min, 50–95% (B); 17–18 min, and 95%–5% (B). The injection volume was 10 μL and flow rate was 0.3 ml/min. Sample detecting were in an MRM mode with positive and negative model. Curtain gas (CUR): 10; collision gas (CAD): Medium; ion spray voltage: The Ion Spray voltage: ±4500V; Temperature: 450°C; ion source gas (GS1): 40; ion source gas (GS2): 40.
2.4.1 Methodology validation
Methodology validation was conducted in accordance with the “Guidance for Industry-Bio analysis Method Validation” (Food and Drug Administration, September 2013) issued by the United States Food and Drug Administration. A mixed standard solution of GSH, L-tryptophan, L-kynurenine, GSSG, N-phenylacetylglycine, 5-HTP, L-leucine, 5-HT, and cholic acid was prepared and diluted successively to prepare two solutions at each concentration. The standard curve was calculated by the ratio of the peak area of the sample to the area of the internal standard sample, and the least-squares method with 1/x weight were used to fit the linear regression equation of the standard curve.
2.4.2 Precision and accuracy assay
The assay precision of the UPLC-MS method was analyzed using quality control (QC) samples, and the QC samples were assayed using untreated rat serum to determine the intraday and interday precision.
High, medium and low concentration QC samples were prepared by adding standard solutions of L-tryptophan, L-kynurenine, GSSG, N-phenylacetylglycine, 5-HTP, L-leucine, 5-HT, cholic acid, and GSH-NEM to stripped plasma. The stability of the method was evaluated by calculating the sample concentration according to the sample test content calculation method.
2.4.3 Data analysis
The MS analysis was performed by the software of Analyst (1.6.4). The standard curve method was used to measure the ratio of the peak area (Area ratio) of the sample to the internal standard. The least-squares method with 1/x weight was used to fit the standard curve linear regression equation. For test metabolites where no standard exists, a semi-quantitative analysis was performed to evaluate the change trend of the same test component based on the ratio of the peak area of the test sample to the area of the internal standard.
2.4.4 Metabolic pathway analysis
Pattern discrimination analysis was performed on each group of relevant data using the principal component analysis (PCA) method with SIMCA-P software. Based on the differential metabolites, serum metabolite profiles were analyzed using the The Human Metabolome Database (HMDB) and Kyoto Encyclopedia of Genes and Genomes (KEGG)-related metabolomics databases to identify the metabolic pathways associated with the prevention of CCl4-induced liver injury in rats.
2.5 Statistics analysis
The statistical analysis was performed using SPSS 17.0. ALT, AST, and the metabolites contents were described by means and standard deviations (Mean ± SD). ALT and AST levels were compared between groups by t-tests. p < 0.05 was taken as a statistically significant.
3 Results
3.1 Protect effect of P.V on liver injury
3.1.1 Histopathological analysis
In terms of the gross anatomical appearance, the adhesion condition of the liver to peritoneum and intestine was severe in the model group, no adhesion condition was found in the normal group, and the adhesion degree was lower in the P.V groups.
Pathological analysis of rat liver tissue sections showed that the structure of rat liver tissues in the normal group was intact and clear, the cell morphology was regular, and no necrosis and inflammatory cell infiltration were observed. In the model group, the liver tissue structure was severely damaged, the cells were swollen and denatured, cytoplasmic vacuolation was observed, and inflammatory cell infiltration was observed to different degrees. Compared with the model group, hepatic tissue structure destruction, cytoplasmic vacuolation, and cellular swelling significantly decreased, and inflammatory cell infiltration decreased, in the P.V groups. The injury level tended to decrease as the dose dependant manner (Figure 1), which was consistent with the changes in ALT and AST levels.
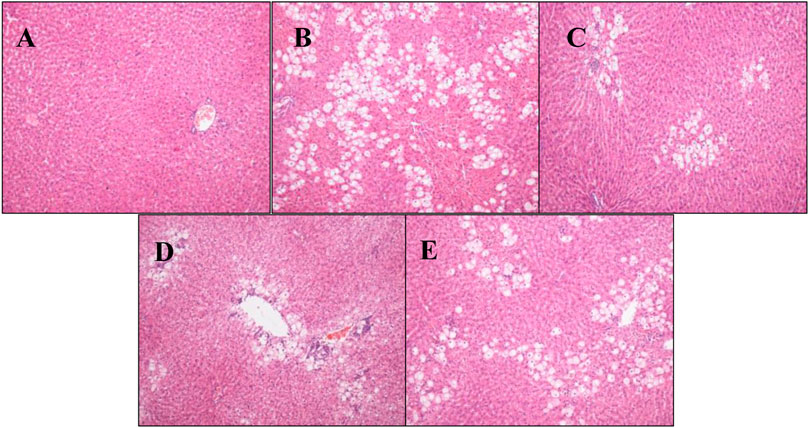
FIGURE 1. Pathological analysis results of rat liver tissue (H-E staining, × 100) (A) Blank group (B) Model group (C) P.V group (2.96 g/kg) (D) P.V group (1.97 g/kg) (E) P.V group (0.98 g/kg).
3.1.2 ALT and AST in serum
Compared with the normal group, the levels of ALT and AST in the model group were significantly higher (p < 0.01), indicating that the model of CCl4-induced liver injury was successful. The levels of ALT and AST in P.V groups decreased compared with those in the model group as a dose-dependent manner, indicating that P.V could reduce the levels of ALT and AST in the serum of rats with liver injury to a different extent and play a liver-protective role in liver injury (Figure 2).
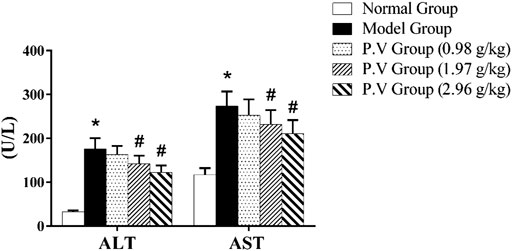
FIGURE 2. ALT and AST levels in serum. *p <0.05, model group vs normal group; #p <0.05, P.V group vs. Model group.
3.2 Metabolic analysis
3.2.1 Linearity
The linear regression equation of the standard curve was fitted using the least-squares method and 1/x weighting, using the concentration of the metabolites as the horizontal coordinate and the ratio of the peak area of the detected component to the internal standard component as the vertical coordinate (Table 1).
3.2.2 Accuracy and precision
The results indicate that the accuracy and precision of the method was appropriate for all study samples. Precision analysis of GSH, GSSG, L-Leu, L-Try, L-Kyn, 5-HTP, cholic acid, 5-HT, and N-phenylacetylglycine indicated that the intra-day precision of each metabolite ranged from 2.35% to 4.19%, and the inter-day precision ranged from 2.76% to 4.82%. All the values of these parameters were less than 5% which had satisfactory results within the acceptable criteria.
3.2.3 UPLC-MS/MS analysis
On the basis of establishing a stable and reliable method for the determination of metabolites by UPLC-MS/MS, 82 metabolites were simultaneously determined in normal group, model group, and P.V group (2.96 g/kg). We then compared the differences of 14 metabolites in serum (Figure 3) and 26 metabolites in liver (Figure 4) between the groups by combining the chromatograms with the relative standard deviation (RSD%) of the content of each component within the same group, which was less than 30%. The results indicated that there were significant changes in metabolites in the serum samples of the model group, and there were multiple related differential metabolites that could be differentiated between the normal group and the model group. The GSH/GSSG ratio decreased significantly in the model group compared with that in normal group, which was consistent with the decrease in the antioxidant capacity in the model group. The levels of phenylalanine and citric acid in the model group were significantly lower than those in the normal group, and the levels of phenylalanine and citric acid in the model group recovered to the level of the normal group after treatment. The levels of L-leucine, cholic acid, N-phenylacetylglycine, hippuric acid, carnitine, and hypoxanthine in the model group were significantly higher than those in the normal group.
3.2.4 Pathway analysis
As shown in the PCA analysis (Figure 5), the serum samples (Figure 5A) and liver samples (Figure 5B) of the normal group and model group were distributed in different regions and far away from each other, and the same group showed an obvious aggregation trend, indicating the difference in metabolite content between the normal group and model group. This further confirmed the success of the model of liver injury and also suggested that P.V could significantly adjust the biomarker content in the serum and liver of rats with liver injury, causing an obvious change in the metabolic network. P-test analysis also showed that the different components tested were replaced to ensure that there was no overfitting in the between-group separation models (Figure 6).
Metabolitic pathway analysis indicated that primary bile acid biosynthesis, glutathione metabolism, and TCA cycle were the most important metabolic pathway affected in the serum of the normal group vs. model group (Figure 7A). The alanine, aspartate and glutamate metabolism, TCA cycle, and tyrosine metabolism pathways were significantly affected by the administration of the P.V (Figure 7B). While in the liver tissue, arginine and proline metabolism, TCA cycle, alaine, aspartate and glutamate metabolism, Phenylalanine, tyrosine and tryptophan biosynthesis were the most important metabolic pathway affected in the normal group vs. model group (Figure 8A). Alanine, aspartate and glutamate metabolism, TCA cycle, glyoxylate and dicarboxylate metabolism pathways were significantly affected by the administration of P.V (Figure 8B).
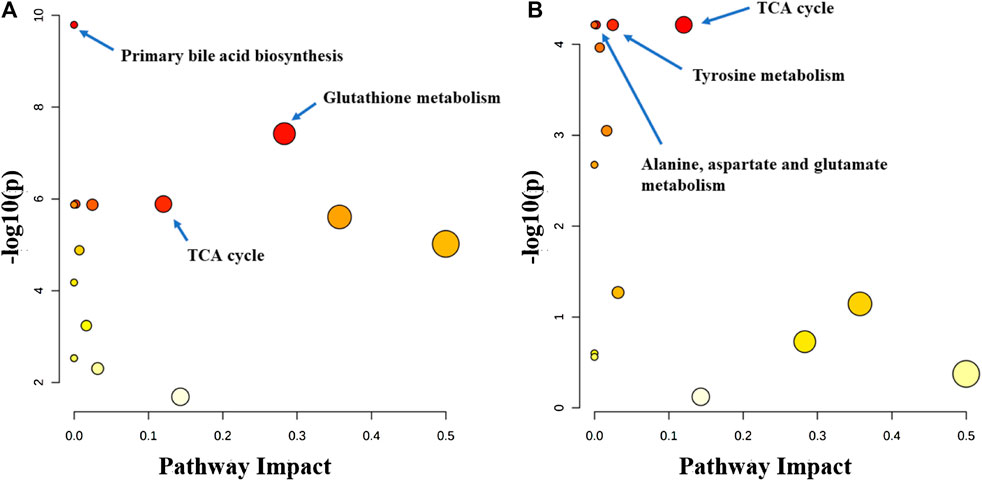
FIGURE 7. Metabolic pathway analysis of serum in rats (A) Normal group vs. Model group (B) P.V group vs. Model group.
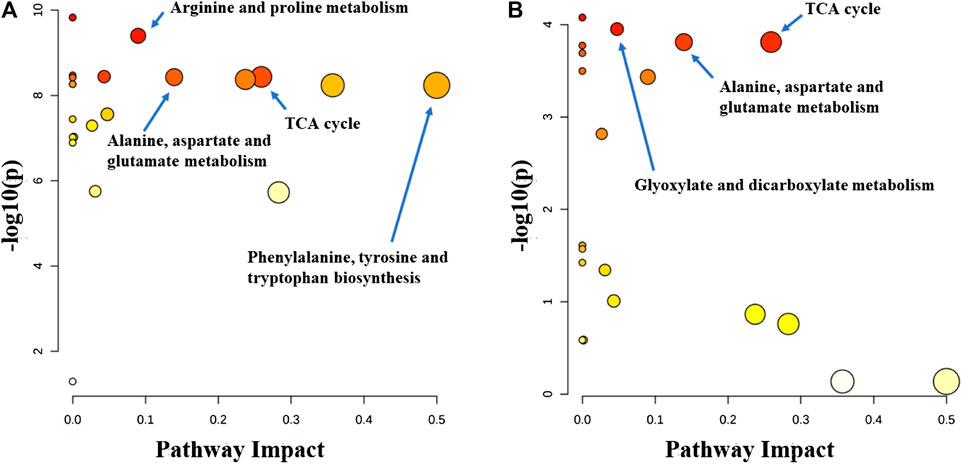
FIGURE 8. Metabolic pathway analysis of liver in rats (A) Normal group vs. Model group (B) P.V group vs. Model group.
4 Discussion
In this study, the liver injury induced by CCl4 was improved by P.V. Further, the elevation of serum ALT and AST in the rat model was reversed, the liver histological damage was alleviated to a certain extent, and the effect showed a dose-dependent manner. 82 molecules related to oxidative stress, inflammation, amino acid metabolism, purine metabolism, tricarboxylic acid cycle, glycolysis, and lipid metabolism were analyzed. It was found that 14 metabolites in serum and 26 metabolites in liver showed significant changes, of which appeared to be key different metabolizers.
It is well known that despite having different etiologies, liver injuries are frequently associated with excessive oxidative stress. Liver function is protected by enhancing the activity of the endogenous antioxidant defense system (Wang et al., 2017). Glutathione has two active forms, reduced and oxidized. GSH is an important scavenger to protect against oxidative stress in the liver. A decreased GSH/GSSG ratio is used as the index of oxidative stress. In this study, the serum GSH/GSSG values in the model group (23.7) were significantly decreased compared with those in the normal group (51.2), which is in accordance with the state of abrupt decrease of antioxidant capacity in the rat model of liver injury. The GSH/GSSG value in the P.V group (60.1) was similar to that in the normal group, suggesting that P.V could repair the liver injury by reducing the level of oxidative stress in the hepatocytes.
Nucleoside is the major component of nucleic acid, while hypoxanthine is a metabolite of nucleoside and is an important alkaloid purine. AMP first generates hypoxanthine, which is oxidized to xanthine under the action of xanthine oxidase and ultimately decomposes to form uric acid, which is excreted in the urine. This pathway is an important metabolic pathway in the body, and gout is a clinical condition caused by abnormal uric acid metabolism. In this study, the increase of hypoxanthine content in the serum of model rats is considered to be related to a decrease in xanthine oxidase activity and the hindrance of hypoxanthine oxidation.
The TCA cycle is a common pathway for the metabolism of the three major nutrients: sugar, fat and amino acids. Citric acid is the key intermediate product of the TCA cycle, and oxaloacetic acid and acetyl-CoA are the important regulatory points of the TCA cycle. In this study, citric acid levels in serum were significantly reduced in the model group, presumably due to impairment of the TCA cycle process in rat hepatocytes (Li et al., 2014; Wu et al., 2017b), partial recovery of the TCA cycle, and increased citric acid levels after treatment.
Amino acid metabolism can be used to synthesize specific proteins, peptides, and nitrogen-containing compounds, either through decarboxylation by deamination, transamination, and catabolism of ammonia, or by the release of energy through citric acid cycling. Therefore, these changes in amino acid metabolism may elicit important signaling events within hepatocytes. Our results suggest that P.V can cause metabolic abnormalities of amino acid. L-Leucine is one of the eight essential amino acids in the human body. It has been reported that CCl4 can cause decreases in leucine, glutamine/glutathione, and betaine contents, presumably due to inhibition of the expression of these amino acid synthesis genes (Li et al., 2014). Phenylacetylglycine could be involved in the metabolism of intestinal microorganisms. It has been suggested that the level of phenylacetylglycine in the urine of CCl4 liver injury model rats decreased (Wu et al., 2017b). In this study, the level of N-phenylacetylglycine in the serum of the model group increased and the level of acetylglycine in the P.V group decreased. We presume that this was related to the decrease in urinary excretion and the increase in the serum concentration in the model rats.
In this study, the therapeutic effect of P.V on liver injury was assayed. P.V could decrease the hepatic tissue structure destruction, cytoplasmic vacuolation, cellular swelling, and inflammatory cell infiltration obviously. And it could decrease ALT and AST levels in rats suffered liver injury as a dose dependant manner. Metabolic mechanism analysis revealed that P.V could treat liver injury by regulating alanine, aspartate and glutamate metabolism, and TCA cycle.
Data availability statement
The raw data supporting the conclusions of this article will be made available by the authors, without undue reservation.
Ethics statement
The animal study was reviewed and approved by Institutional Animal Ethics Committee of Liaoning University.
Author contributions
WL and DL were responsible for overall supervision. L-MQ was responsible for experiments and data collection. HZ analysed data. L-MQ and WL wrote the manuscript. All authors read and approved the final manuscript.
Funding
This study was supported by Wenzhou Science and Technology Bureau Project (Y20210220).
Conflict of interest
The authors declare that the research was conducted in the absence of any commercial or financial relationships that could be construed as a potential conflict of interest.
Publisher’s note
All claims expressed in this article are solely those of the authors and do not necessarily represent those of their affiliated organizations, or those of the publisher, the editors and the reviewers. Any product that may be evaluated in this article, or claim that may be made by its manufacturer, is not guaranteed or endorsed by the publisher.
References
Carretero, A., León, Z., García-Cañaveras, J. C., Zaragoza, A., Gómez-Lechón, M. J., Donato, M. T., et al. (2014). In vitro/in vivo screening of oxidative homeostasis and damage to DNA, protein, and lipids using UPLC/MS-MS. Anal. Bioanal. Chem. 406 (22), 5465–5476. doi:10.1007/s00216-014-7983-5
Dai, Y., Tu, F. J., Yao, Z. H., Ding, B., Xu, W., Qiu, X. H., et al. (2013). Rapid identification of chemical constituents in traditional Chinese medicine fufang preparation xianling gubao capsule by LC-linear ion trap/Orbitrap mass spectrometry. Am. J. Chin. Med. 41 (5), 1181–1198. doi:10.1142/S0192415X13500808
Huang, L. Y., Sun, Y. Z., Chen, Q. Q., Bai, X. L., Du, T. T., Xu, H. T., et al. (2022). New compounds from Patrinia villosa Juss. and their anti-inflammatory activities. Nat. Prod. Res. 2022, 1–9. doi:10.1080/14786419.2022.2035382
Jaeschke, H., McGill, M. R., and Ramachandran, A. (2012). Oxidant stress, mitochondria, and cell death mechanisms in druginduced liver injury: Lessons learned from acetaminophen hepatotoxicity. Drug Metab. Rev. 44 (1), 88–106. doi:10.3109/03602532.2011.602688
Khemichian, S., Francoz, C., Durand, F., Karvellas, C. J., and Nadim, M. K. (2021). Hepatorenal syndrome. Crit. Care Clin. 37 (2), 321–334. doi:10.1016/j.ccc.2020.11.011
Kong, M., Dong, W., Zhu, Y., Fan, Z., Miao, X., Guo, Y., et al. (2021). Redox-sensitive activation of CCL7 by BRG1 in hepatocytes during liver injury. Redox Biol. 46, 102079. doi:10.1016/j.redox.2021.102079
Li, X., Zhang, F., Wang, D., Li, Z., Qin, X., and Du, G. (2014). NMR-based metabonomic and quantitative real-time PCR in the profiling of metabolic changes in carbon tetrachloride-induced rat liver injury. J. Pharm. Biomed. Anal. 89, 42–49. doi:10.1016/j.jpba.2013.10.023
Li, Y., Cheng, X., Chen, C., Huijuan, W., Zhao, H., Liu, W., et al. (2016). Apigenin, a flavonoid constituent derived from P. villosa, inhibits hepatocellular carcinoma cell growth by CyclinD1/CDK4 regulation via p38 MAPK-p21 signaling. Pathol. Res. Pract. 216 (1), 152701. doi:10.1016/j.prp.2019.152701
Liao, J. C., Deng, J. S., Chiu, C. S., Huang, S. S., Hou, W. C., Lin, W. C., et al. (2012). Chemical compositions, anti-inflammatory, antiproliferative and radical-scavenging activities of Actinidia callosa var. ephippioides. Am. J. Chin. Med. 40 (5), 1047–1062. doi:10.1142/S0192415X12500772
Mega, A., Marzi, L., Kob, M., Piccin, A., and Floreani, A. (2021). Food and nutrition in the pathogenesis of liver damage. Nutrients 13 (4), 1326. doi:10.3390/nu13041326
Navarro, V., Khan, I., Björnsson, E., Seeff, L. B., Serrano, J., and Hoofnagle, J. H. (2017). Liver injury from herbal and dietary supplements. Hepatology 65 (1), 363–373. doi:10.1002/hep.28813
Peng, J., Fan, G., and Wu, Y. (2005). Studies on chemical constituents of Patrinia villosa Juss. Zhong Yao Cai 28 (10), 883.
Qian, H., Chao, X., Williams, J., Fulte, S., Li, T., Yang, L., et al. (2021). Autophagy in liver diseases: A review. Mol. Asp. Med. 82, 100973. doi:10.1016/j.mam.2021.100973
Sheng, L., Yang, Y., Zhang, Y., and Li, N. (2019). Chemical constituents of Patrinia heterophylla Bunge and selective cytotoxicity against six human tumor cells. J. Ethnopharmacol. 236, 129–135. doi:10.1016/j.jep.2019.03.005
Sun, T. F., Wang, P., and Zhang, C. L. (2014). Research progress on Chinese medicine treatment of liver injury. J. Pharm. Res. 33 (12), 715–717. doi:10.13506/j.cnki.jpr.2014.12.013
Wang, J., Wang, X., Ma, X., Xu, B., Chen, L., Chen, C., et al. (2022). Therapeutic effect of Patrinia villosa on TNBS-induced ulcerative colitis via metabolism, vitamin D receptor and NF-κB signaling pathways. J. Ethnopharmacol. 288, 114989. doi:10.1016/j.jep.2022.114989
Wang, M. F., Zhao, S. S., Thapa, D. M., Song, Y. L., and Xiang, Z. (2021). Metabolomics of Fuzi-Gancao in CCl4 induced acute liver injury and its regulatory effect on bile acid profile in rats. World J. Gastroenterol. 27 (40), 6888–6907. doi:10.3748/wjg.v27.i40.6888
Wang, M. Y., Srinivasan, M., Dasari, S., Narvekar, P., Samy, A., Dontaraju, V. S., et al. (2017). Antioxidant activity of yichun blue honeysuckle (YBHS) berry counteracts CCl₄-Induced toxicity in liver injury model of mice. Antioxidants (Basel) 6 (3), 50. doi:10.3390/antiox6030050
Wu, F., Zheng, H., Yang, Z. T., Cheng, B., Wu, J. X., Liu, X. W., et al. (2017a). Urinary metabonomics study of the hepatoprotective effects of total alkaloids from Corydalis saxicola Bunting on carbon tetrachloride-induced chronic hepatotoxicity in rats using 1H NMR analysis. J. Pharm. Biomed. Anal. 140, 199–209. doi:10.1016/j.jpba.2017.03.031
Wu, H. T., Zhang, G., Huang, L. S., Pang, H. Y., Zhang, N., Chen, Y. P., et al. (2017b2017). Hepatoprotective effect of polyphenol-enriched fraction from folium microcos on oxidative stress and apoptosis in acetaminophen-induced liver injury in mice. Oxid. Med. Cell. Longev. 2017, 3631565. doi:10.1155/2017/3631565
Xiang, Z., Chen, N., Xu, Y., Wu, J., Liu, Y. J., Tan, C., et al. (2016). New flavonoid from Patrinia villosa. Pharm. Biol. 54 (7), 1219–1222. doi:10.3109/13880209.2015.1064449
Xin-Jia, Y., Wei, L., Ying, Z., Ning, C., Ying, X., Jian, W., et al. (2016). A new biphenyl neolignan from leaves of Patrinia villosa (thunb.) Juss. Pharmacogn. Mag. 12 (45), 1–3. doi:10.4103/0973-1296.175988
Yang, Y. F., Ma, H. M., Chen, G., Wang, H. F., Xiang, Z., Feng, Q. M., et al. (2016). A new sesquiterpene lactone glycoside and a new quinic acid methyl ester from Patrinia villosa. J. Asian Nat. Prod. Res. 18 (10), 945–951. doi:10.1080/10286020.2016.1173678
Yang, Y., He, Q., Wang, H., Hu, X., Luo, Y., Liang, G., et al. (2017). The protection of meloxicam against chronic aluminium overload-induced liver injury in rats. Oncotarget 8 (14), 23448. doi:10.18632/oncotarget.15588
Younossi, Z. M., Koenig, A. B., Abdelatif, D., Fazel, Y., Henry, L., and Wymer, M. (2016). Global epidemiology of nonalcoholic fatty liver disease-Meta-analytic assessment of prevalence, incidence, and outcomes. Hepatology 64 (1), 73–84. doi:10.1002/hep.28431
Zheng, Y., Jin, Y., Zhu, H. B., Xu, S. T., Xia, Y. X., and Huang, Y. (2012). The anti-inflammatory and anti-nociceptive activities of Patrinia villosa and its mechanism on the proinflammatory cytokines of rats with pelvic inflammation. Afr. J. Tradit. Complement. Altern. Med. 9 (3), 295–302. doi:10.4314/ajtcam.v9i3.1
Keywords: Patrinia villosa (Thunb.) juss, liver injury, inflammation, metabolism, UPLC-MS
Citation: Qiao L-M, Zhang H, Liu W and Lou D (2022) Therapeutic effect and metabolomics mechanism of Patrinia Villosa (Thunb.) juss on liver injury in rats. Front. Pharmacol. 13:1058587. doi: 10.3389/fphar.2022.1058587
Received: 30 September 2022; Accepted: 11 October 2022;
Published: 21 October 2022.
Edited by:
Xiande Ma, Liaoning University of Traditional Chinese Medicine, ChinaReviewed by:
Yanwu Li, Guangzhou University of Chinese Medicine, ChinaSenling Feng, Third Affiliated Hospital of Guangzhou Medical University, China
Copyright © 2022 Qiao, Zhang, Liu and Lou. This is an open-access article distributed under the terms of the Creative Commons Attribution License (CC BY). The use, distribution or reproduction in other forums is permitted, provided the original author(s) and the copyright owner(s) are credited and that the original publication in this journal is cited, in accordance with accepted academic practice. No use, distribution or reproduction is permitted which does not comply with these terms.
*Correspondence: Wei Liu, liuweiolina@163.com; Dan Lou, loudan81@163.com