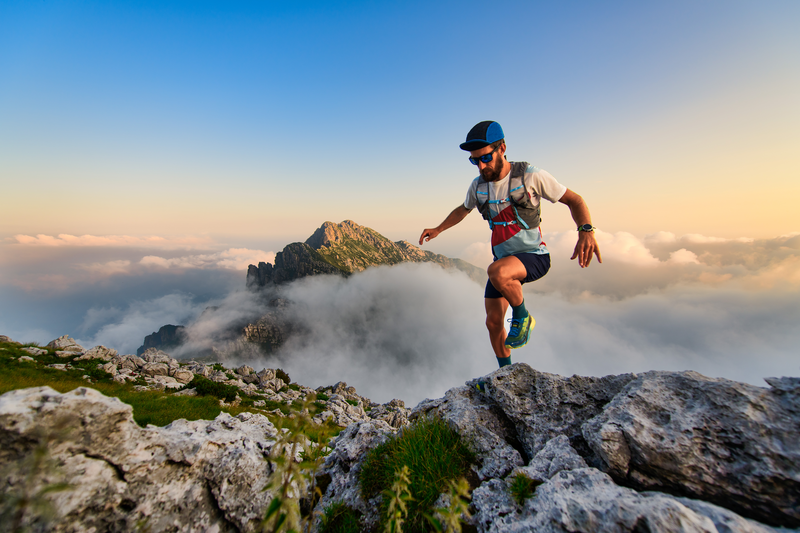
95% of researchers rate our articles as excellent or good
Learn more about the work of our research integrity team to safeguard the quality of each article we publish.
Find out more
MINI REVIEW article
Front. Pharmacol. , 02 November 2022
Sec. Ethnopharmacology
Volume 13 - 2022 | https://doi.org/10.3389/fphar.2022.1051952
This article is part of the Research Topic New Plant-Based Therapeutics against Cancer and Associated Complications View all 4 articles
Pancreatic cancer is one of the most malignant tumors of the digestive tract, with the poor prognosis and low 5-year survival rate less than 10%. Although surgical resection and chemotherapy as gemcitabine (first-line treatment) has been applied to the pancreatic cancer patients, the overall survival rates of pancreatic cancer are quite low due to drug resistance. Therefore, it is of urgent need to develop alternative strategies for its treatment. In this review, we summarized the major herbal drugs and metabolites, including curcumin, triptolide, Panax Notoginseng Saponins and their metabolites etc. These compounds with antioxidant, anti-angiogenic and anti-metastatic activities can inhibit the progression and metastasis of pancreatic cancer. Expecting to provide comprehensive information of potential natural products, our review provides valuable information and strategies for pancreatic cancer treatment.
Pancreatic cancer (PC) is one of the leading causes of cancer-related deaths worldwide. It has been known for its difficult in diagnosis and poor prognosis even with surgery and chemotherapy. The symptom of PC is hard to be perceptible, therefore the majority of patients were found to be the late stage of disease. Moreover, these patients are inoperable due to the severe metastasis and invasiveness (Eiznhamer and Xu, 2004). Lots of factors contribute to the oncogenesis and progression of pancreatic cancer, including the environment and lifestyle, alcohol, high fat diet (Rawla et al., 2019), genetic mutation of onco-hub genes as KRAS and other oncogenes as CDKN2A, TP53 and SMAD4 (Dixon et al., 2012; Zhang et al., 2020), epigenetic regulation (Xiao et al., 2015; Yu et al., 2018; Yu et al., 2020; Yu et al., 2021), hyperglycemia (Chien K and Liao, 2016; Hu et al., 2019), and chronic pancreatitis (Kichler and Jang, 2020; Piersma et al., 2020).
Currently, surgery and adjuvant chemotherapy are considered to be the only viable options for pancreatic cancer treatment. However, 80% of patients are not suitable for surgery due to the invasiveness and metastasis of tumor cells. Some chemotherapeutic drugs and immunotherapy have been applied for these patients, but the pharmacological effects are limited (Hanahan and Weinberg, 2011; Holohan et al., 2013; Wang et al., 2022a). Hence, there is an urgent need for finding novel potential therapeutic drugs.
Traditional Chinese medicine has been applied in east asia for thousands of years and some of these natural botanical drugs, including paclitaxel, camptothecin and podophyllotoxin have successfully applied in clinics for years (Xu et al., 2011; Fedoros et al., 2018; Pan et al., 2020; Zhou et al., 2022a). Therefore, in light of summarizing the potential natural products for pancreatic cancer treatment, we comprehensively addressed the natural products and some major anti-cancer metabolites.
Current treatment options for pancreatic cancer include surgery, immunotherapy and targeted therapy. Surgical resection is the major treatment for pancreatic cancer (Bockhorn et al., 2014). Immunotherapy and targeted therapies are currently new approaches to the treatment of pancreatic cancer. Immunotherapy includes tumor vaccine, adoptive cell immunotherapy, oncolytic virus therapy, immune checkpoint inhibitors, etc (Conroy et al., 2018). Targeted therapy mainly includes targeted therapy for tumor angiogenesis, targeted therapy of KRAS or other proteins of oncogenes and related signaling pathways. (Cao et al., 2021; Liu et al., 2021).
Natural products refer to the constituents or their metabolites in animals, plant extracts or insects, marine organisms and microorganisms, which are collectively referred to as natural products. For decades, natural products played an important role in the development of anti-tumor drugs, and many natural products such as paclitaxel, vincristine or their analogs have been widely used in clinical practice (Flores-Bustamante et al., 2010). These natural products exert their antitumor activities through different or novel mechanisms of action. Hence, we updated more information for botanical drugs other than paclitaxel and vincristine. Table 1 summarizes the therapeutic effects of some natural products on pancreatic cancer.
Curcumin is a plant polyphenolic compound extracted from the Curcuma longa L. of the ginger plant turmeric. It is also known as diferuloylmethane, and was first isolated in 1815. Curcumin has a broad variety of pharmacological effects, including anti-inflammatory, antioxidant, anti-tumor, enhancing radiotherapy and chemotherapy sensitivity, and protecting liver and kidney functions (Hewlings and Kalman, 2017). It has been broadly investigated in cancers, including acute myeloid leukemia (Zhou et al., 2022b), prostate cancer (Al-Rabia et al., 2022), breast cancer, lung cancer etc (Giordano and Tommonaro, 2019).
For the pre-clinical study of curcumin, great progress has been made on both its mechanisms. In BxPC-3 cells, curcumin could restore mutant p53(Y220C) function and promote apoptosis in a dose- and time-dependent manner (ranging from 2 µM to 10 μM, 12–72 h, respectively) (Malhotra et al., 2021; Malhotra et al., 2022). Curcumin could synergically suppress the pancreatic cancer cell (SW1990 cell) proliferation with 10058-F4, an inhibitor of c-Myc both in vitro and in vivo (Jie et al., 2022). Curcumin analogue C66 has been reported to inhibit the proliferation and migration of pancreatic cancer in a dose- and time-dependent manner (25–100 μM, 24–72 h, respectively). Further this analogue could inhibit the inflammatory cytokines (IL-1β, IL-6, IL-8, and IL-15) secretion via the inhibition of JNK pathway (Chen et al., 2022a). The anti-pancreatic cancer effects and mechanisms of curcumin and its derivatives are described in detail (Huang et al., 2022).
Curcumin is the only metabolite or botanical drug under clinical study for pancreatic cancer. From 2004 to 2010, a total of 44 patients diagnosed of pancreatic neoplasms adenocarcinoma, were enrolled in a phase II clinical trial of curcumin. In collaboration with Sabinsa Corporation, this trial is performed at M.D. Anderson Cancer Center. Curcumin is delivered orally at the dosage of 8 g/day for 8 weeks. The median age included in this study is 65 (range: 40-87) with 56% female. Meanwhile, it is neither an open-label study nor randomized trial. The outcome of this trial is not disclosed by the researchers. We only know that nine of the 44 patients has serious adverse effects, including cardiac disorders (chest pain, multiple pulmonary emboli, etc.), gastrointestinal disorders (chronic cancer progression, metastasis, abdomen pain, etc.) and other disorders (Kim et al., 2021).
Triptolide is an epoxy diterpene lactone compound extracted from the roots, leaves, flowers and fruits of Tripterygium wilfordii Hook. f. Triptolide has a broad range of pharmacological effects on autoimmune diseases, including rheumatoid arthritis (Li et al., 2022a), systemic lupus erythematosus (Zhang et al., 2022a), ankylosing spondylitis (Ji et al., 2022), and so on. Due to poor water solubility (0.017 mg/ml) and severe toxicity including excessive immune responses, its clinical application is greatly limited (Ding et al., 2017), and several strategies has been applied to reduce its toxicity and to improve its solubility (Kang et al., 2022a; Zhang et al., 2022b; Rao et al., 2022).
Triptolide has also been applied in a broad range of cancers. It could synergistically increase apoptosis of gastric cancer cells with Tumor necrosis factor-α (TNF-α) via the inhibition of H19/miR-204-5p/NF-κB/FLIP axis (Yuan et al., 2022). Followed by the decreased DNA (cytosine-5-)-methyltransferase 1 (DNMT1) and DNA (cytosine-5-)-methyltransferase 3a (DNMT3a) expression and the inhibition of Wnt inhibition factor 1 (WIF1), Sex-determining region Y-box (SOX17), cadherin 1 (CDH1) and Secreted Frizzled Related Protein 5 (SFRP5) demethylation, triptolide could inhibit the Wnt pathway, and thereby it inhibits T-cell acute lymphoblastic leukaemia (Ma et al., 2022). For the malignant melanoma treatment, targeted delivery of triptolide was applied with cyclopeptide and αvβ3 integrin-specific exosomes (Gu et al., 2022). Accumulating evidences revealed that triptolide has a strong antitumor effect in a broad range of cancers, including non-small cell lung cancer (Zhou et al., 2022c), colorectal cancer (Liskova et al., 2022; Song et al., 2022), hepatocellular carcinoma (Li et al., 2022b), acute myeloid leukemia (Chen et al., 2022b; Kang et al., 2022b) and so on.
For the treatment of pancreatic cancer, triptolide at the dose of 10–40 nM could inhibit pancreatic cancer cell proliferation through the suppression of hedgehog signaling pathway (Feng et al., 2019), or via the inhibition of plasminogen activator urokinase (PLAU) (Zhao et al., 2020). In another study, 50 nM triptolide could increase TRAIL sensitivity, downregulate the PUM1, and stimulate autophagy of pancreatic cancer cell (Dai et al., 2019). Study on BxPC-3 cells, PANC-1 cells and transplanted tumor models revealed that triptolide, at the dose of 0.1–1 µM could improve the gemcitabine sensitivity via the suppression of TLR4/NF-κB signaling pathway (Qiao et al., 2016; Ma et al., 2019). At the dose of 10–50 ng/ml, triptolide could also inhibits the proliferation by suppressing hypoxia-inducible factor-1α (HIF-1α) and c-Myc expression (Ding et al., 2015). It induces cell death by O-GlcNAc modification of transcription factor Sp1((Banerjee et al., 2013)). It can also be modified as prodrug. Chitosan oligosaccharide (CSO) conjugated triptolide could improve the water solubility to 15 mg/ml) (Wang et al., 2022b). Triptolide could also be conjugated with 2-(pyridin-2-yldisul-fanyl)ethyl acrylate (PDA)- poly (ethylene glycol) methyl (PEG) and lactobionic acid (LBA) (Sui et al., 2021). Three amino acids (tryptophan, valine, and lysine) based triptolide prodrug could also inhibit the growth of pancreatic cancer (Lou et al., 2021). In combined with a cytoprotective agent, diammonium glycyrrhizinate (DG), a complex lipid emulsion (TP/DG-CLE) could increase the therapeutic effects of triptolide (Mu et al., 2022).
Panax Notoginseng Saponins (PNS) is the main chemical component of the Panax notoginseng (Burkill) F. H. Chen ex C. H. PNS has multiple pharmacological functions, including the inhibition of the platelet aggregation, improving microcirculation, inhibiting the inflammation responses and reducing the oxidative stress (Zhang et al., 2018a).
Recent research on PNS revealed its anti-cancer activity and low toxicity in various cancers, including prostate cancer, colorectal cancer, retinoblastoma, and so on (Han et al., 2018; Li et al., 2022c; Hawthorne et al., 2022; Zhong et al., 2022). Study on pancreatic cancer Miapaca2 and PANC-1 cells revealed that the IC50 of PNS are 377.1 and 492.5 µM, respectively, whiles gemcitabine is the positive control. PNS could limit the proliferation, migration, invasion of pancreatic cell proliferation, and induce the autophagy of these cells. Importantly, it could stimulate the apoptosis and chemosentivity to gemcitabine via caspase-dependent pathway (Yao et al., 2021). Gold nanoparticles from the leaf of PNS has been proved to possess anti-pancreatic cancer activity in PANC-1 cells (Wang et al., 2019).
Further studies on the metabolites of PNS revealed that there are ginsengosides have anti-proliferative function on pancreatic cells. Haixia Pan et al. found that 50–200 µM ginsenoside Rg3 could increase the chemosensitivity of gemcitabine on pancreatic adenocarcinoma through the inhibition of Zinc finger protein 91 homolog (ZFP91) mediated testis specific Y-encoded-like protein 2 (TSPYL2) destabilization (Pan et al., 2022). Another study on ginsenoside Rg3 demonstrated that same dose of ginsenoside Rg3 could suppresses the growth of gemcitabine-resistant pancreatic cancer cells (Panc‐1/GEM and SW1990/GEM cells). Molecular mechanism investigation revealed that ginsenoside Rg3 could upregulate lncRNA-CASC2 and activates PTEN signaling pathway (Zou et al., 2020). In BxPC-3 and AsPC-1 cells, it stimulates the apoptosis and increase the anti-proliferative effects of erlotinib via the decreased phosphorylation of EGFR, PI3K, and Akt (Jiang et al., 2017). It inhibits the angiogenesis of pancreatic cancer via the downregulation of VE-cadherin/EphA2/MMP9/MMP2 signaling pathway (Guo et al., 2014). Furthermore, 20–60 µM ginsengoside Rh2 could also induce Bxpc-3 cell cycle arrest, and reduced migration and invasion in a caspase dependent manner (Tang et al., 2013).
Toosendanin is a tetracyclic triterpenoid extracted from the fruit or bark of the plant Melia toosendan Sieb. et Zucc. or Melia azedarach L. Toosendanin is a white crystalline powder, easily soluble in methanol, ethanol and pyridine (Shi and Li, 2007). With an IC50 of 26 nM, toosendanin could inhibit the growth of a broad range of tumors. By inhibiting the Hh-involved Hedgehog pathway, toosendanin coud inhibit colorectal cancer cell growth (Zhang et al., 2022c). As an autophagy inhibitor, it could block autophagy by inhibiting the activity of vacuolar-type H+-translocating ATPase, induce necroptosis and promote apoptosis in triple-negative breast cancer cells and tumor xenograft models (Dong et al., 2022; Zhang et al., 2022d; Zhang et al., 2022e). Through the inhibition of the PI3K/Akt/mTOR signaling pathways, toosendanin could inhibit the growth of glioma cells and tumor growth in vivo animal models (Zhang et al., 2021a). In the hepatocellular carcinoma, toosendanin functions as WW-domain containing oxidoreductase (WWOX) agonist and thereby inhibits tumor metastasis by the inhibition of JAK2/Stat3 and Wnt/β-catenin signaling pathways (Yang et al., 2021a; Yang et al., 2021b). It could induce the apoptosis of ovarian cancer and gastric cancer in a caspase-dependent pathway and through the κ-opioid receptor/β-catenin signaling axis (Shao et al., 2020; Wang et al., 2020; Wang et al., 2021a).
Pancreatic cancer cells were treated with different concentrations of toosendanin (50, 100 and 200 nM, respectively), and the results showed that pancreatic cancer cell lines had apoptosis in a dose dependent manner (Pei et al., 2017). Besides, toosendanin can also inhibit the migration and invasion of pancreatic cancer cells at the dosage of 200 μM.
Further mechanism investigation revealed that toosendanin could reverse the TGF-β induced epithelial-mesenchymal transition through increasing the expression of Ecadherin and reducing the expression of Vimentin, ZEB1 and SNAIL. It also suppressed TGF-β mediated EMT via the deactivation of Akt/mTOR signaling pathway. In the in vivo xenograft mice experiments, toosendanin at a dose of 0.2 mg/kg. Taken together, toosendanin shows an activity that can specifically inhibit pancreatic cancer cells (Pei et al., 2017).
Xanthohumol, a flavonoid isolated from Humulus lupulus L., has anti-inflammatory, anti-tumor and anti-angiogenesis properties (Krajka-Kuźniak et al., 2013; Niederau et al., 2022; Vesaghhamedani et al., 2022). It could reduce cell viability and cause G2/M arrest at the dose of 40 μM through MAPK JNK pathway for human nasopharyngeal carcinoma cells (Hsieh et al., 2022). At 10 nM dose, it could inhibit the colon cancer cellproliferation and progression via downregulating inflammatory signals (TNF-α and IL-6) and glucose metabolism (Torrens-Mas et al., 2022). By increasing p53-upregulated modulator of apoptosis (PUMA)-mediated apoptosis, it also inhibits non-small cell lung cancer (Li et al., 2022d). It hampers glutamine uptake in triple negative breast cancer (Carmo et al., 2022). It also inhibits a variety of cancers as prostate, B-chronic lymphocytic leukemia, hepatocellular, and medullary thyroid cancers (Lust et al., 2005; Szliszka et al., 2009; Cook et al., 2010; Dorn et al., 2010).
Xanthohumol could inhibit the growth of MiaPaCa-2, PANC-1, AsPC-1, and L3.6 pl cells at a dose-dependent manner, ranging from 5 mM to 30 mM. It can also inhibit PC patient-derived cells in a dose-dependent manner as well. Further mechanisms studies revealed that xanthohumol mediated cell apoptosis via the inhibition of Notch 1, HES-1 and survivin at both transcription and translation levels (Kunnimalaiyaan et al., 2015). It could also induce cell cycle arrest of PANC-1 and BxPC-3 cells by inactivating signal transducer and activator of transcription 3 (STAT3) and downstream genes as cyclinD1, survivin, and Bcl-xL (98). Xanthohumol has been reported to suppress angiogenesis by inactivation of NF-κB, and inhibiting the vascular endothelial growth factor (VEGF) and interleukin-8 (IL-8) expression both in vitro (BxPC-3 cells, AsPC-1 cells and MIA PaCa-2 cells, 10–25 µM) and in vivo (10 mg/kg) (Saito et al., 2018). Xanthohumol, in combination with phenethyl isothiocyanate (extracted from Oenanthe javanica (Bl.) DC.) could inhibit the PSN-1 cell growth with an IC50 46 µM and inhibit the MS1 cell growth with an IC50 49 µM. This combination could greatly reduce the expression of NF-κB p65 subunits, and activate Nrf2 and downstream genes as GSTP, NQO1, and SOD in PANC-1 cells (Krajka-Kuźniak et al., 2020) and PSN-1 cells (Cykowiak et al., 2021). Further investigation revealed that the combination of xanthohumol (40 mg/kg) and phenethyl isothiocyanate (15 mg/kg) could inhibit the plasma COX-2 and nuclear STAT3 expression (Cykowiak et al., 2021).
Pterostilbene (3,5-dimethoxy-40 -hydroxystilbene) is a phytoalexin, the secondary metabolite isolated from the heartwood of red sandalwood pTocarpus santalinus. Pterostilbene has been reported to be an efficient anti-cancer agents in hepatocellular carcinoma with IC50 of about 20–40 μM in various HCC cell lines. Meanwhile, it has similar inhibitory function in drug (sorafenib and lamivudine)-resistant HCC cell through the inhibition of ribonucleotide reductase activity, and thereby inhibit virus replication (Qian et al., 2018; Wang et al., 2021b).
Studies on pancreatic ductal adenocarcinoma (PDAC) demonstrated that 10–75 µM pterostilbene could enhance chemosensitivity by the inhibition of MIA PaCa-2 cell proliferation and cell cycle arrest. It could also induce both apoptosis and autophagy. Further mechanic study revealed that pterostilbene inhibited multidrug resistance protein 1 (MDR1) expression through the Receptor for advanced glycosylation end products (RAGE)/PI3K/Akt signaling pathway (Hsu et al., 2020). It can also increase cell death by inducing lysosomal membrane permeabilization (Obrador et al., 2021). Chloroquine could substantiate the anti-tumor effects of pterostilbene on PC through the inhibition of autophagy via downregulating RAGE/STAT3 and protein kinase B (AKT)/mammalian target of rapamycin (mTOR) signaling pathway (Chen et al., 2021a). In vivo study using AsPC-1 and BxPC-3 cells xenograft mice revealed that 20–40 mg/kg pterostilbene downregulates the glucocorticoid secretion via reducing glucocorticoid receptor and inhibiting Nrf2-dependent signaling pathway (Benlloch et al., 2016).
Cordyceps militaris is the mycelium of Cordyceps militaris (L.) Link. It has the functions of anti-tumor and immune regulation. Cordycepin is one of its main active components, which has the activity of regulating immunity, antibacterial and anti-inflammatory (Pan et al., 2015; Kong et al., 2022). It has been broadly investigated in various cancers, including triple-negative breast cancer (Wei et al., 2022; Wu et al., 2022), lymphoma (Shi et al., 2022), colon cancer (Deng et al., 2022), glioblastoma (Chen et al., 2022c) and so on both in vitro and in vivo. It could also increase the radiosensitivity by cell cycle arrest, ER stress and caspase dependent apoptosis (Lee et al., 2022). It could induce autophagy in tumor cells (Chen et al., 2021b).
Cordycepin has been reported to induce the caspase-dependent apoptosis in a dose dependent manner. 50–100 µM cordycepin suppress the growth pancreatic cancer cell growth with an IC50 of 38.85, 72.99, 150.1, 213.1, and 349.3 μM for BxPC-3, CFPAC-1, AsPC-1, PANC-1 and SW1990 cells, respectively. It induces S-phase arrest via activating checkpoint kinase 2 (Chk2) and downregulating cyclin A2 and CDK2 phosphorylation, inhibits Ras/ERK pathway (Li et al., 2020). In human pancreatic cancer cells (MIAPaCa-2 and Capan-1 cells), cordycepin could induce apoptosis in a dose- and time-dependent manner in vitro (ranging 50–600 μM, 24–72 h, respectively) and in vivo (15and 50 mg/kg/d for 28 days) via mitochondrial mediated intrinsic apoptosis pathways (Zhang et al., 2018b). Furthermore, for acute pancreatitis therapy, cordycepin could also be potential treatment by inhibiting the pro-inflammatory cytokines as IL-6, IL-1β, and TNF-α via the inhibition of nuclear factor-κB (NF-κB) and NLR family pyrin domain-containing protein 3 (NLRP3) (Yang et al., 2020).
Libertellenone H (LH) is a marine-derived diterpene-type diterpenoid isolated from the high latitude lived arctic fungus Eutypella sp. D-1 (120). In the anti-tumor assay, the positive controls are adriamycin, 5-fluorouracil and paclitaxel, and LH has been proved to inhibit the growth of U251 cells, SW-1990 cells, SG7901 cells, MCF-7 cells, Huh-7 cells, Hela cells, and H460 cells (Lu et al., 2014).
For pancreatic cancer treatment, it could inhibit the growth of human pancreatic cancer cell lines PANC-1 (IC50, 3.21 μM), SW 1990 (IC50, 0.67 μM), AsPC-1 (IC50, 2.78 μM), BxPC-3 (IC50, 5.53 μM) and human pancreatic duct epithelial cells HPDE6-C7 (IC50, 10.86 μM) in a dose-dependent manner (Zhang et al., 2021b). Further investigation revealed that it could stimulate ROS accumulation via the suppression on the Trx and the corresponding receptor system. Covalently binds toTrx1 and TrxR, it activates ASK1/JNK signaling pathway (Zhang et al., 2021b).
Mangiferin, 1,3,6,7-tetrahydroxyxanthone-C2-β-D-glucoside, is extracted from the leaves and barks of mango tree (Mangifera indica L.). It possesses a variety of pharmacological activities, such as antioxidative, antitumor, antibacterial, antiviral, anti-diabetes, and so on (Wang et al., 2014; Du et al., 2018; Wang et al., 2018). It could inhibit the proliferation of lung cancer (Shi et al., 2016), ovarian cancer (Zou et al., 2017; He et al., 2019), breast cancer, glioblastoma (Mu et al., 2018) and other cancers (Du et al., 2018). Recent studies on mangiferin revealed that it could inhibit the Mia-PaCa2 cell proliferation in a dose-dependent manner ranging from 3.06 to 100 µM. Further mechanic study indicated that 5, 10, and 20 µM mangiferin could induce autophagy, and caspase-dependent apoptosis. Additionally, it could induce the G2/M arrest and endogenous ROS production (Yu et al., 2019).
Propolis is a natural product obtained by mixing resin collected by bees and their own secretions. The chemical composition of propolis is very complex, which is affected by various factors such as its plant source, the type of bees collected and the collection time. In recent years, many studies have proved that propolis from Mexico, Brazil, Vietnam and other places has an inhibitory effect on the proliferation of pancreatic cancer cells (Awale et al., 2008; Li et al., 2010; Nguyen et al., 2017). For example, the Algerian bee limb can enhance the anti-pancreatic cancer effect mediated by doxorubicin by regulating apoptosis, cell cycle and inhibiting the activity of P-glycoprotein (Rouibah et al., 2018). The active ingredient CAPE in propolis can induce apoptosis of pancreatic cancer Panc-1 cells through mitochondrial dysfunction and activation of caspase-3/-7 (Chen et al., 2008). In addition, Chinese propolis inhibits the proliferation of human pancreatic cancer Panc-1 cells through the Hippo-YAP signaling pathway (Liu et al., 2018; He et al., 2019). The IC50 value of Propolis on human pancreatic cancer Panc-1 cell is about 50 μg/ml. Treatment with 50μg/ml Propolis for 48 h resulted in 34.25 ± 3.81% apoptosis of human pancreatic cancer Panc-1 cells. After treatment with propolis, the expression of YAP in human pancreatic cancer Panc-1 cells was significantly reduced, and its nuclear entry was inhibited. At the same time, the expression levels of the main upstream proteins MST1 and LATS1 and the downstream phosphorylated effector protein p-YAP were significantly increased. These studies will justify the research and development of pharmaceuticals and related health products containing propolis (Tao et al., 2021).
Accumulating evidences revealed that metabolites or botanical drugs could efficiently inhibits pancreatic cell proliferation and induces apoptosis or autophagy in vitro and alleviates the progression of cancer in xenograft mouse models. Because botanical drugs have been used in the world for thousands of years, especially in east asia, the advantages and adverse effects have been tested known. However, most studies of these botanical drugs are in the primitive stage. Data from in vitro cell study and in vivo xeograft mouse research are not enough for pre-clinical study. More animal studies as rabbit, beagle and non-human primate studies are needed for the new drug development of these botanical drugs. Meanwhile, these studies will cover the gap from bench to bed. Among these studies, curcumin is under phase II clinical trial of pancreatic neoplasms adenocarcinoma sponsored by researchers from M.D. Anderson Cancer Center. However, the detailed outcome of this trial is not released by the researcher and we could only find pieces of information about the adverse effects of curcumin, including cancer progression and metastasis. As most of these metabolites/botanical drugs are still based on in vitro cell study and in vivo rodent data, the major bottleneck for the clinical trial is no large animal study is available. How to shorten the gap between pre-clinical research and clinical trial is still challenges for researchers and clinicians. And more non-human primate studies should be performed for the efficacy and safety of these metabolites/botanical drugs before clinical trials. With more and more in-depth investigations on large animals, metabolites or botanical drugs will ultimately be applied in clinics in the near future.
YW and XHu contributed to the conception and design of the work. XHe and NW drafted the manuscript. YZ prepared the table. YW and XHu substantively revised the manuscript. All authors read and approved the final manuscript.
This research was supported by the National Natural Science Foundation of China (81802504, 81872207), Sichuan Science and Technology Bureau (2019YFS0439, 2020JDJQ0067, 2020JDRC0118, 2021YJ0564, 2022YFH0005, 2022QN07, 2022YFS0272), the Science and Technology Innovation Project of Chengdu, China (No. 2021-YF05-00225-SN), Sichuan Medical Association grant (No. Q19037).
The authors declare that the research was conducted in the absence of any commercial or financial relationships that could be construed as a potential conflict of interest.
All claims expressed in this article are solely those of the authors and do not necessarily represent those of their affiliated organizations, or those of the publisher, the editors and the reviewers. Any product that may be evaluated in this article, or claim that may be made by its manufacturer, is not guaranteed or endorsed by the publisher.
Al-Rabia, M. W., Alhakamy, N. A., Rizg, W. Y., Alghaith, A. F., Ahmed, O. A. A., and Fahmy, U. A. (2022). Boosting curcumin activity against human prostatic cancer PC3 cells by utilizing scorpion venom conjugated phytosomes as promising functionalized nanovesicles. Drug Deliv. 29 (1), 807–820. doi:10.1080/10717544.2022.2048133
Awale, S., Li, F., Onozuka, H., Esumi, H., Tezuka, Y., and Kadota, S. (2008). Constituents of Brazilian red propolis and their preferential cytotoxic activity against human pancreatic PANC-1 cancer cell line in nutrient-deprived condition. Bioorg. Med. Chem. 16 (1), 181–189. doi:10.1016/j.bmc.2007.10.004
Banerjee, S., Sangwan, V., McGinn, O., Chugh, R., Dudeja, V., Vickers, S. M., et al. (2013). Triptolide-induced cell death in pancreatic cancer is mediated by O-GlcNAc modification of transcription factor Sp1. J. Biol. Chem. 288 (47), 33927–33938. doi:10.1074/jbc.M113.500983
Benlloch, M., Obrador, E., Valles, S. L., Rodriguez, M. L., Sirerol, J. A., Alcacer, J., et al. (2016). Pterostilbene decreases the antioxidant defenses of aggressive cancer cells in vivo: A physiological glucocorticoids- and nrf2-dependent mechanism. Antioxid. Redox Signal. 24 (17), 974–990. doi:10.1089/ars.2015.6437
Bockhorn, M., Uzunoglu, F. G., Adham, M., Imrie, C., Milicevic, M., Sandberg, A. A., et al. (2014). Borderline resectable pancreatic cancer: A consensus statement by the international study group of pancreatic surgery (ISGPS). Surgery 155 (6), 977–988. doi:10.1016/j.surg.2014.02.001
Cao, D., Song, Q., Li, J., Jiang, Y., Wang, Z., and Lu, S. (2021). Opportunities and challenges in targeted therapy and immunotherapy for pancreatic cancer. Expert Rev. Mol. Med. 23, e21. doi:10.1017/erm.2021.26
Carmo, F., Silva, C., and Martel, F. (2022). Inhibition of glutamine cellular uptake contributes to the cytotoxic effect of xanthohumol in triple-negative breast cancer cells. Nutr. Cancer 74 (9), 3413–3430. doi:10.1080/01635581.2022.2076889
Chen, H., Jiang, Y., Liu, R., Deng, J., Chen, Q., Chen, L., et al. (2022). Curcumin derivative C66 suppresses pancreatic cancer progression through the inhibition of JNK-mediated inflammation. Molecules 27 (10), 3076. doi:10.3390/molecules27103076
Chen, J., Zhuang, Y. D., Zhang, Q., Liu, S., Zhuang, B. B., Wang, C. H., et al. (2022). Exploring the mechanism of cordycepin combined with doxorubicin in treating glioblastoma based on network pharmacology and biological verification. PeerJ 10, e12942. doi:10.7717/peerj.12942
Chen, M. J., Chang, W. H., Lin, C. C., Liu, C. Y., Wang, T. E., Chu, C. H., et al. (2008). Caffeic acid phenethyl ester induces apoptosis of human pancreatic cancer cells involving caspase and mitochondrial dysfunction. Pancreatology 8 (6), 566–576. doi:10.1159/000159843
Chen, Q., Deng, S., Deng, M., Shi, Y., Zhong, M., Ding, L., et al. (2022). Therapeutic synergy of Triptolide and MDM2 inhibitor against acute myeloid leukemia through modulation of p53-dependent and -independent pathways. Exp. Hematol. Oncol. 11 (1), 23. doi:10.1186/s40164-022-00276-z
Chen, R. J., Lyu, Y. J., Chen, Y. Y., Lee, Y. C., Pan, M. H., Ho, Y. S., et al. (2021). Chloroquine potentiates the anticancer effect of pterostilbene on pancreatic cancer by inhibiting autophagy and downregulating the RAGE/STAT3 pathway. Molecules 26 (21), 6741. doi:10.3390/molecules26216741
Chen, Y. Y., Chen, C. H., Lin, W. C., Tung, C. W., Chen, Y. C., Yang, S. H., et al. (2021). The role of autophagy in anti-cancer and health promoting effects of cordycepin. Molecules 26 (16), 4954. doi:10.3390/molecules26164954
Chien K, W. H. P., and Liao, W. C. (2016). Blood glucose concentration and risk of pancreatic cancer: Systematic review and dose-response meta-analysis. Bmj 354, i4089. doi:10.1136/bmj.i4089
Conroy, T., Hammel, P., Hebbar, M., Ben Abdelghani, M., Wei, A. C., Raoul, J. L., et al. (2018). FOLFIRINOX or gemcitabine as adjuvant therapy for pancreatic cancer. N. Engl. J. Med. 379 (25), 2395–2406. doi:10.1056/NEJMoa1809775
Cook, M. R., Luo, J., Ndiaye, M., Chen, H., and Kunnimalaiyaan, M. (2010). Xanthohumol inhibits the neuroendocrine transcription factor achaete-scute complex-like 1, suppresses proliferation, and induces phosphorylated ERK1/2 in medullary thyroid cancer. Am. J. Surg. 199 (3), 315–318. discussion 318 (2010). doi:10.1016/j.amjsurg.2009.08.034
Cykowiak, M., Kleszcz, R., Kucińska, M., Paluszczak, J., Szaefer, H., Plewiński, A., et al. (2021). Attenuation of pancreatic cancer in vitro and in vivo via modulation of Nrf2 and NF-κB signaling pathways by natural compounds. Cells 10 (12), 3556. doi:10.3390/cells10123556
Dai, H., Jiang, Y., Luo, Y., Bie, P., and Chen, Z. (2019). Triptolide enhances TRAIL sensitivity of pancreatic cancer cells by activating autophagy via downregulation of PUM1. Phytomedicine 62, 152953. doi:10.1016/j.phymed.2019.152953
Deng, Q., Li, X., Fang, C., Li, X., Zhang, J., Xi, Q., et al. (2022). Cordycepin enhances anti-tumor immunity in colon cancer by inhibiting phagocytosis immune checkpoint CD47 expression. Int. Immunopharmacol. 107, 108695. doi:10.1016/j.intimp.2022.108695
Ding, B., Wahid, M. A., Wang, Z., Xie, C., Thakkar, A., Prabhu, S., et al. (2017). Triptolide and celastrol loaded silk fibroin nanoparticles show synergistic effect against human pancreatic cancer cells. Nanoscale 9 (32), 11739–11753. doi:10.1039/c7nr03016a
Ding, X., Zhou, X., Jiang, B., Zhao, Q., and Zhou, G. (2015). Triptolide suppresses proliferation, hypoxia-inducible factor-1α and c-Myc expression in pancreatic cancer cells. Mol. Med. Rep. 12 (3), 4508–4513. doi:10.3892/mmr.2015.3960
Dixon, S. J., Lemberg, K. M., Lamprecht, M. R., Skouta, R., Zaitsev, E. M., Gleason, C. E., et al. (2012). Ferroptosis: An iron-dependent form of nonapoptotic cell death. Cell 149 (5), 1060–1072. doi:10.1016/j.cell.2012.03.042
Dong, Y., Zhu, G., Wang, S. F., Keon, K. A., Rubinstein, J. L., Zeng, S. X., et al. (2022). Toosendanin, a novel potent vacuolar-type H(+)-translocating ATPase inhibitor, sensitizes cancer cells to chemotherapy by blocking protective autophagy. Int. J. Biol. Sci. 18 (7), 2684–2702. doi:10.7150/ijbs.71041
Dorn, C., Weiss, T. S., Heilmann, J., and Hellerbrand, C. (2010). Xanthohumol, a prenylated chalcone derived from hops, inhibits proliferation, migration and interleukin-8 expression of hepatocellular carcinoma cells. Int. J. Oncol. 36 (2), 435–441. doi:10.3892/ijo_00000517
Du, S., Liu, H., Lei, T., Xie, X., Wang, H., He, X., et al. (2018). Mangiferin: An effective therapeutic agent against several disorders (Review). Mol. Med. Rep. 18 (6), 4775–4786. doi:10.3892/mmr.2018.9529
Eiznhamer, D. A., and Xu, Z. Q. (2004). Betulinic acid: A promising anticancer candidate. IDrugs. 7 (4), 359–373.
Fedoros, E. I., Orlov, A. A., Zherebker, A., Gubareva, E. A., Maydin, M. A., Konstantinov, A. I., et al. (2018). Novel water-soluble lignin derivative BP-Cx-1: Identification of components and screening of potential targets in silico and in vitro. Oncotarget 9 (26), 18578–18593. doi:10.18632/oncotarget.24990
Feng, J., Rao, M., Wang, M., Liang, L., Chen, Z., Pang, X., et al. (2019). Triptolide suppresses pancreatic cancer cell proliferation by inhibiting hedgehog signaling pathway activity. Sci. China. Life Sci. 62 (10), 1409–1412. doi:10.1007/s11427-018-9477-3
Flores-Bustamante, Z. R., Rivera-Orduna, F. N., Martinez-Cardenas, A., and Flores-Cotera, L. B. (2010). Microbial paclitaxel: Advances and perspectives. J. Antibiot. 63 (8), 460–467. doi:10.1038/ja.2010.83
Giordano, A., and Tommonaro, G. (2019). Curcumin and cancer. Nutrients 11 (10), E2376. doi:10.3390/nu11102376
Gu, Y., Du, Y., Jiang, L., Tang, X., Li, A., Zhao, Y., et al. (2022). αvβ3 integrin-specific exosomes engineered with cyclopeptide for targeted delivery of triptolide against malignant melanoma. J. Nanobiotechnology 20 (1), 384. doi:10.1186/s12951-022-01597-1
Guo, J. Q., Zheng, Q. H., Chen, H., Chen, L., Xu, J. B., Chen, M. Y., et al. (2014). Ginsenoside Rg3 inhibition of vasculogenic mimicry in pancreatic cancer through downregulation of VEcadherin/EphA2/MMP9/MMP2 expression. Int. J. Oncol. 45 (3), 1065–1072. doi:10.3892/ijo.2014.2500
Han, H., Du, L., Cao, Z., Zhang, B., and Zhou, Q. (2018). Triptonide potently suppresses pancreatic cancer cell-mediated vasculogenic mimicry by inhibiting expression of VE-cadherin and chemokine ligand 2 genes. Eur. J. Pharmacol. 818, 593–603. doi:10.1016/j.ejphar.2017.11.019
Hanahan, D., and Weinberg, R. A. (2011). Hallmarks of cancer: The next generation. Cell 144 (5), 646–674. doi:10.1016/j.cell.2011.02.013
Hawthorne, B., Lund, K., Freggiaro, S., Kaga, R., and Meng, J. (2022). The mechanism of the cytotoxic effect of Panax notoginseng extracts on prostate cancer cells. Biomed. Pharmacother. 149, 112887. doi:10.1016/j.biopha.2022.112887
He, W., You, Y., Du, S., Lei, T., Wang, H., Li, X., et al. (2019). Anti-neoplastic effect of mangiferin on human ovarian adenocarcinoma OVCAR8 cells via the regulation of YAP. Oncol. Lett. 17 (1), 1008–1018. doi:10.3892/ol.2018.9708
Hewlings, S. J., and Kalman, D. S. (2017). Curcumin: A review of its effects on human health. Foods 6 (10), E92. doi:10.3390/foods6100092
Holohan, C., Van Schaeybroeck, S., Longley, D. B., and Johnston, P. G. (2013). Cancer drug resistance: An evolving paradigm. Nat. Rev. Cancer 13 (10), 714–726. doi:10.1038/nrc3599
Hsieh, M. Y., Hsieh, M. J., Lo, Y. S., Lin, C. C., Chuang, Y. C., Chen, M. K., et al. (2022). Xanthohumol targets the JNK1/2 signaling pathway in apoptosis of human nasopharyngeal carcinoma cells. Environ. Toxicol. 37 (6), 1509–1520. doi:10.1002/tox.23502
Hsu, Y. H., Chen, S. Y., Wang, S. Y., Lin, J. A., and Yen, G. C. (2020). Pterostilbene enhances cytotoxicity and chemosensitivity in human pancreatic cancer cells. Biomolecules 10 (5), E709. doi:10.3390/biom10050709
Hu, C. M., Tien, S. C., Hsieh, P. K., Jeng, Y. M., Chang, M. C., Chang, Y. T., et al. (2019). High glucose triggers nucleotide imbalance through O-GlcNAcylation of key enzymes and induces KRAS mutation in pancreatic cells. Cell Metab. 29 (6), 1334e10–1349. doi:10.1016/j.cmet.2019.02.005
Huang, Q., Zhang, Y., Zheng, Y., Yang, H., Yang, Y., Mo, Y., et al. (2022). Molecular mechanism of curcumin and its analogs as multifunctional compounds against pancreatic cancer. Nutr. Cancer 74 (9), 3096–3108. doi:10.1080/01635581.2022.2071451
Ji, W., Lu, Y., Ma, Z., Gan, K., Liu, Y., Cheng, Y., et al. (2022). Triptolide attenuates inhibition of ankylosing spondylitis-derived mesenchymal stem cells on the osteoclastogenesis through modulating exosomal transfer of circ-0110634. J. Orthop. Transl. 36, 132–144. doi:10.1016/j.jot.2022.05.007
Jiang, J., Yuan, Z., Sun, Y., Bu, Y., Li, W., and Fei, Z. (2017). Ginsenoside Rg3 enhances the anti-proliferative activity of erlotinib in pancreatic cancer cell lines by downregulation of EGFR/PI3K/Akt signaling pathway. Biomed. Pharmacother. 96, 619–625. doi:10.1016/j.biopha.2017.10.043
Jiang, W., Zhao, S., Xu, L., Lu, Y., Lu, Z., Chen, C., et al. (2015). The inhibitory effects of xanthohumol, a prenylated chalcone derived from hops, on cell growth and tumorigenesis in human pancreatic cancer. Biomed. Pharmacother. 73, 40–47. doi:10.1016/j.biopha.2015.05.020
Jie, Z., Jinna, Z., Jingjun, Z., Pengcheng, L., Fang, Y., Qinyang, C., et al. (2022). Antitumor effects of 10058-F4 and curcumin in combination therapy for pancreatic cancer in vitro and in vivo. J. Healthc. Eng. 2022, 1620802. doi:10.1155/2022/1620802
Kang, D., Liu, Y., Song, Y., Fang, B., Zhang, Q., and Hu, L. (2022). Triptolide shows high sensitivity and low toxicity against acute myeloid leukemia cell lines through inhibiting WSTF-RNAPII complex. Front. Oncol. 12, 811850. doi:10.3389/fonc.2022.811850
Kang, D., Pan, X., Song, Y., Liu, Y., Wang, D., Zhu, X., et al. (2022). Discovery of a novel water-soluble, rapid-release triptolide prodrug with improved drug-like properties and high efficacy in human acute myeloid leukemia. Eur. J. Med. Chem. 243, 114694. doi:10.1016/j.ejmech.2022.114694
Kichler, A., and Jang, S. (2020). Chronic pancreatitis: Epidemiology, diagnosis, and management updates. Drugs 80 (12), 1155–1168. doi:10.1007/s40265-020-01360-6
Kim, A., Ha, J., Kim, J., Cho, Y., Ahn, J., Cheon, C., et al. (2021). Natural products for pancreatic cancer treatment: From traditional medicine to modern drug discovery. Nutrients 13 (11), 3801. doi:10.3390/nu13113801
Kong, W., Liu, W., Wang, M., Hui, W., Feng, Y., Lu, J., et al. (2022). Cordycepin exhibits anti-bacterial and anti-inflammatory effects against gastritis in Helicobacter pylori-infected mice. Pathog. Dis. 80 (1), ftac005. doi:10.1093/femspd/ftac005
Krajka-Kuźniak, V., Cykowiak, M., Szaefer, H., Kleszcz, R., and Baer-Dubowska, W. (2020). Combination of xanthohumol and phenethyl isothiocyanate inhibits NF-κB and activates Nrf2 in pancreatic cancer cells. Toxicol. Vitro 65, 104799. doi:10.1016/j.tiv.2020.104799
Krajka-Kuźniak, V., Paluszczak, J., and Baer-Dubowska, W. (2013). Xanthohumol induces phase II enzymes via Nrf2 in human hepatocytes in vitro. Toxicol. Vitro 27 (1), 149–156. doi:10.1016/j.tiv.2012.10.008
Kunnimalaiyaan, S., Trevino, J., Tsai, S., Gamblin, T. C., and Kunnimalaiyaan, M. (2015). Xanthohumol-mediated suppression of Notch1 signaling is associated with antitumor activity in human pancreatic cancer cells. Mol. Cancer Ther. 14 (6), 1395–1403. doi:10.1158/1535-7163.MCT-14-0915
Lee, Y. P., Huang, W. R., Wu, W. S., Wu, Y. H., Ho, S. Y., Wang, Y. J., et al. (2022). Cordycepin enhances radiosensitivity to induce apoptosis through cell cycle arrest, caspase pathway and ER stress in MA-10 mouse Leydig tumor cells. Am. J. Cancer Res. 12 (8), 3601–3624.
Li, F., Awale, S., Tezuka, Y., Esumi, H., and Kadota, S. (2010). Study on the constituents of Mexican propolis and their cytotoxic activity against PANC-1 human pancreatic cancer cells. J. Nat. Prod. 73 (4), 623–627. doi:10.1021/np900772m
Li, M., Wang, G., Yan, Y., Jiang, M., Wang, Z., Zhang, Z., et al. (2022). Triptolide and l-ascorbate palmitate co-loaded micelles for combination therapy of rheumatoid arthritis and side effect attenuation. Drug Deliv. 29 (1), 2751–2758. doi:10.1080/10717544.2022.2115162
Li, X., Jin, L., Ma, Y., Jiang, Z., Tang, H., and Tong, X. (2022). Xanthohumol inhibits non-small cell lung cancer by activating PUMA-mediated apoptosis. Toxicology 470, 153141. doi:10.1016/j.tox.2022.153141
Li, X. M., Yuan, D. Y., Liu, Y. H., Zhu, L., Qin, H. K., Yang, Y. B., et al. (2022). Panax notoginseng saponins prevent colitis-associated colorectal cancer via inhibition Ido1 mediated immune regulation. Chin. J. Nat. Med. 20 (4), 258–269. doi:10.1016/S1875-5364(22)60179-1
Li, X. Y., Tao, H., Jin, C., Du, Z. Y., Liao, W. F., Tang, Q. J., et al. (2020). Cordycepin inhibits pancreatic cancer cell growth in vitro and in vivo via targeting FGFR2 and blocking ERK signaling. Chin. J. Nat. Med. 18 (5), 345–355. doi:10.1016/S1875-5364(20)30041-8
Li, Z., Yang, G., Wang, R., Wang, Y., Wang, J., Yang, M., et al. (2022). γ-Cyclodextrin metal-organic framework as a carrier to deliver triptolide for the treatment of hepatocellular carcinoma. Drug Deliv. Transl. Res. 12 (5), 1096–1104. doi:10.1007/s13346-021-00978-7
Liskova, V., Kajsik, M., Chovancova, B., Roller, L., and Krizanova, O. (2022). Camptothecin, triptolide, and apoptosis inducer kit have differential effects on mitochondria in colorectal carcinoma cells. FEBS Open Bio 12 (5), 913–924. doi:10.1002/2211-5463.13401
Liu, H., Du, S., Lei, T., Wang, H., He, X., Tong, R., et al. (2018). Multifaceted regulation and functions of YAP/TAZ in tumors (Review). Oncol. Rep. 40 (1), 16–28. doi:10.3892/or.2018.6423
Liu, X., Li, Z., and Wang, Y. (2021). Advances in targeted therapy and immunotherapy for pancreatic cancer. Adv. Biol. 5 (3), e1900236. doi:10.1002/adbi.201900236
Lou, D., Lou, Z., Lin, Y., Shangguan, H., Lin, Y., Luo, Q., et al. (2021). ATB(0, +)-targeted delivery of triptolide prodrugs for safer and more effective pancreatic cancer therapy. Bioorg. Med. Chem. Lett. 33, 127728. doi:10.1016/j.bmcl.2020.127728
Lu, X. L., Liu, J. T., Liu, X. Y., Gao, Y., Zhang, J., Jiao, B. H., et al. (2014). Pimarane diterpenes from the arctic fungus Eutypella sp. D-1. J. Antibiot. 67 (2), 171–174. doi:10.1038/ja.2013.104
Lust, S., Vanhoecke, B., Janssens, A., Philippe, J., Bracke, M., and Offner, F. (2005). Xanthohumol kills B-chronic lymphocytic leukemia cells by an apoptotic mechanism. Mol. Nutr. Food Res. 49 (9), 844–850. doi:10.1002/mnfr.200500045
Ma, J. X., Sun, Y. L., Yu, Y., Zhang, J., Wu, H. Y., and Yu, X. F. (2019). Triptolide enhances the sensitivity of pancreatic cancer PANC-1 cells to gemcitabine by inhibiting TLR4/NF-κB signaling. Am. J. Transl. Res. 11 (6), 3750–3760.
Ma, Y., Li, Y., Huang, M., and Meng, Y. (2022). Triptolide inhibits T-cell acute lymphoblastic leukaemia by affecting aberrant epigenetic events in the Wnt signalling pathway. J. Chemother., 1–10. doi:10.1080/1120009X.2022.2082347
Malhotra, L., Goyal, H. K. V., Jhuria, S., Dev, K., Kumar, S., Kumar, M., et al. (2021). Curcumin rescue p53Y220C in BxPC-3 pancreatic adenocarcinomas cell line: Evidence-based on computational, biophysical, and in vivo studies. Biochim. Biophys. Acta. Gen. Subj. 1865 (2), 129807. doi:10.1016/j.bbagen.2020.129807
Malhotra, L., Sharma, S., Hariprasad, G., Dhingra, R., Mishra, V., Sharma, R. S., et al. (2022). Mechanism of apoptosis activation by Curcumin rescued mutant p53Y220C in human pancreatic cancer. Biochim. Biophys. Acta. Mol. Cell Res. 1869 (12), 119343. doi:10.1016/j.bbamcr.2022.119343
Mu, F., Liu, T., Zheng, H., Xie, X., Lei, T., He, X., et al. (2018). Mangiferin induces radiosensitization in glioblastoma cells by inhibiting nonhomologous end joining. Oncol. Rep. 40 (6), 3663–3673. doi:10.3892/or.2018.6756
Mu, L., Wu, P., Zhang, Y., Li, S., Yang, R., and Wang, S. (2022). Development of a novel oral complex lipid emulsion containing triptolide for targeting pancreatic cancer. Pharm. Dev. Technol., 1–11. doi:10.1080/10837450.2022.2127767
Nguyen, H. X., Nguyen, M. T. T., Nguyen, N. T., and Awale, S. (2017). Chemical constituents of propolis from Vietnamese trigona minor and their antiausterity activity against the PANC-1 human pancreatic cancer cell line. J. Nat. Prod. 80 (8), 2345–2352. doi:10.1021/acs.jnatprod.7b00375
Niederau, C., Bhargava, S., Schneider-Kramman, R., Jankowski, J., Craveiro, R. B., and Wolf, M. (2022). Xanthohumol exerts anti-inflammatory effects in an in vitro model of mechanically stimulated cementoblasts. Sci. Rep. 12 (1), 14970. doi:10.1038/s41598-022-19220-6
Obrador, E., Salvador-Palmer, R., Jihad-Jebbar, A., Lopez-Blanch, R., Dellinger, T. H., Dellinger, R. W., et al. (2021). Pterostilbene in cancer therapy. Antioxidants (Basel) 10 (3), 492. doi:10.3390/antiox10030492
Pan, B. S., Wang, Y. K., Lai, M. S., Mu, Y. F., and Huang, B. M. (2015). Cordycepin induced MA-10 mouse Leydig tumor cell apoptosis by regulating p38 MAPKs and PI3K/AKT signaling pathways. Sci. Rep. 5, 13372. doi:10.1038/srep13372
Pan, H., Yang, L., Bai, H., Luo, J., and Deng, Y. (2022). Ginsenoside Rg3 increases gemcitabine sensitivity of pancreatic adenocarcinoma via reducing ZFP91 mediated TSPYL2 destabilization. J. Ginseng Res. 46 (5), 636–645. doi:10.1016/j.jgr.2021.08.004
Pan, Q., Tian, J., Zhu, H., Hong, L., Mao, Z., Oliveira, J. M., et al. (2020). Tumor-Targeting polycaprolactone nanoparticles with codelivery of paclitaxel and IR780 for combinational therapy of drug-resistant ovarian cancer. ACS Biomater. Sci. Eng. 6 (4), 2175–2185. doi:10.1021/acsbiomaterials.0c00163
Pei, Z., Fu, W., and Wang, G. (2017). A natural product toosendanin inhibits epithelial-mesenchymal transition and tumor growth in pancreatic cancer via deactivating Akt/mTOR signaling. Biochem. Biophys. Res. Commun. 493 (1), 455–460. doi:10.1016/j.bbrc.2017.08.170
Piersma, B., Hayward, M. K., and Weaver, V. M. (2020). Fibrosis and cancer: A strained relationship. Biochim. Biophys. Acta. Rev. Cancer 1873 (2), 188356. doi:10.1016/j.bbcan.2020.188356
Qian, Y. Y., Liu, Z. S., Yan, H. J., Yuan, Y. F., Levenson, A. S., and Li, K. (2018). Pterostilbene inhibits MTA1/HDAC1 complex leading to PTEN acetylation in hepatocellular carcinoma. Biomed. Pharmacother. 101, 852–859. doi:10.1016/j.biopha.2018.03.022
Qiao, Z., He, M., He, M. U., Li, W., Wang, X., Wang, Y., et al. (2016). Synergistic antitumor activity of gemcitabine combined with triptolide in pancreatic cancer cells. Oncol. Lett. 11 (5), 3527–3533. doi:10.3892/ol.2016.4379
Rao, Q., Ma, G. C., Wu, H., Li, M., Xu, W., Wang, G. J., et al. (2022). Dendritic cell combination therapy reduces the toxicity of triptolide and ameliorates colitis in murine models. Drug Deliv. 29 (1), 679–691. doi:10.1080/10717544.2022.2044935
Rawla, P., Sunkara, T., and Gaduputi, V. (2019). Epidemiology of pancreatic cancer: Global trends, etiology and risk factors. World J. Oncol. 10 (1), 10–27. doi:10.14740/wjon1166
Rouibah, H., Kebsa, W., Lahouel, M., Zihlif, M., Ahram, M., Aburmeleih, B., et al. (2018). Algerian propolis potentiates doxorubicin mediated anticancer effect against human pancreatic PANC-1 cancer cell line through cell cycle arrest, apoptosis induction and P-glycoprotein inhibition. Anticancer. Agents Med. Chem. 18 (3), 375–387. doi:10.2174/1871520618666180110143239
Saito, K., Matsuo, Y., Imafuji, H., Okubo, T., Maeda, Y., Sato, T., et al. (2018). Xanthohumol inhibits angiogenesis by suppressing nuclear factor-κB activation in pancreatic cancer. Cancer Sci. 109 (1), 132–140. doi:10.1111/cas.13441
Shao, S., Li, S., Liu, C., Zhang, W., Zhang, Z., Zhu, S., et al. (2020). Toosendanin induces apoptosis of MKN45 human gastric cancer cells partly through miR23a3pmediated downregulation of BCL2. Mol. Med. Rep. 22 (3), 1793–1802. doi:10.3892/mmr.2020.11263
Shi, L., Cao, H., Fu, S., Jia, Z., Lu, X., Cui, Z., et al. (2022). Cordycepin enhances hyperthermia-induced apoptosis and cell cycle arrest by modulating the MAPK pathway in human lymphoma U937 cells. Mol. Biol. Rep. 49 (9), 8673–8683. doi:10.1007/s11033-022-07705-6
Shi, W., Deng, J., Tong, R., Yang, Y., He, X., Lv, J., et al. (2016). Molecular mechanisms underlying mangiferin-induced apoptosis and cell cycle arrest in A549 human lung carcinoma cells. Mol. Med. Rep. 13 (4), 3423–3432. doi:10.3892/mmr.2016.4947
Shi, Y. L., and Li, M. F. (2007). Biological effects of toosendanin, a triterpenoid extracted from Chinese traditional medicine. Prog. Neurobiol. 82 (1), 1–10. doi:10.1016/j.pneurobio.2007.02.002
Song, X., He, H., Zhang, Y., Fan, J., and Wang, L. (2022). Mechanisms of action of triptolide against colorectal cancer: Insights from proteomic and phosphoproteomic analyses. Aging (Albany NY) 14 (7), 3084–3104. doi:10.18632/aging.203992
Sui, B., Cheng, C., Shi, S., Wang, M., and Xu, P. (2021). Esterase-activatable and GSH-responsive triptolide nano-prodrug for the eradication of pancreatic cancer. Adv. Nanobiomed Res. 1 (11), 2100040. doi:10.1002/anbr.202100040
Szliszka, E., Czuba, Z. P., Mazur, B., Sedek, L., Paradysz, A., and Krol, W. (2009). Chalcones enhance TRAIL-induced apoptosis in prostate cancer cells. Int. J. Mol. Sci. 11 (1), 1–13. doi:10.3390/ijms11010001
Tang, X. P., Tang, G. D., Fang, C. Y., Liang, Z. H., and Zhang, L. Y. (2013). Effects of ginsenoside Rh2 on growth and migration of pancreatic cancer cells. World J. Gastroenterol. 19 (10), 1582–1592. doi:10.3748/wjg.v19.i10.1582
Tao, L., Chen, X., Zheng, Y., Wu, Y., Jiang, X., You, M., et al. (2021). Chinese propolis suppressed pancreatic cancer panc-1 cells proliferation and migration via hippo-YAP pathway. Molecules 26 (9), 2803. doi:10.3390/molecules26092803
Torrens-Mas, M., Alorda-Clara, M., Martinez-Vigara, M., Roca, P., Sastre-Serra, J., Oliver, J., et al. (2022). Xanthohumol reduces inflammation and cell metabolism in HT29 primary colon cancer cells. Int. J. Food Sci. Nutr. 73 (4), 471–479. doi:10.1080/09637486.2021.2012561
Vesaghhamedani, S., Ebrahimzadeh, F., Najafi, E., Shabgah, O. G., Askari, E., Shabgah, A. G., et al. (2022). Xanthohumol: An underestimated, while potent and promising chemotherapeutic agent in cancer treatment. Prog. Biophys. Mol. Biol. 172, 3–14. doi:10.1016/j.pbiomolbio.2022.04.002
Wang, G., Fan, X. Q., Li, L., Li, Y., Shi, B., Xing, K. X., et al. (2021). Toosendanin shows potent efficacy against human ovarian cancer through caspase-dependent mitochondrial apoptotic pathway. Am. J. Chin. Med. 49 (7), 1757–1772. doi:10.1142/S0192415X2150083X
Wang, H., He, X., Lei, T., Liu, Y., Huai, G., Sun, M., et al. (2018). Mangiferin induces islet regeneration in aged mice through regulating p16INK4a. Int. J. Mol. Med. 41 (6), 3231–3242. doi:10.3892/ijmm.2018.3524
Wang, H. L., Li, C. Y., Zhang, B., Liu, Y. D., Lu, B. M., Shi, Z., et al. (2014). Mangiferin facilitates islet regeneration and beta-cell proliferation through upregulation of cell cycle and beta-cell regeneration regulators. Int. J. Mol. Sci. 15 (5), 9016–9035. doi:10.3390/ijms15059016
Wang, H., Wen, C., Chen, S., Wang, F., He, L., Li, W., et al. (2020). Toosendanin-induced apoptosis in colorectal cancer cells is associated with the κ-opioid receptor/β-catenin signaling axis. Biochem. Pharmacol. 177, 114014. doi:10.1016/j.bcp.2020.114014
Wang, L., Xu, J., Yan, Y., Liu, H., and Li, F. (2019). Synthesis of gold nanoparticles from leaf Panax notoginseng and its anticancer activity in pancreatic cancer PANC-1 cell lines. Artif. Cells Nanomed. Biotechnol. 47 (1), 1216–1223. doi:10.1080/21691401.2019.1593852
Wang, R., Xu, Z., Tian, J., Liu, Q., Dong, J., Guo, L., et al. (2021). Pterostilbene inhibits hepatocellular carcinoma proliferation and HBV replication by targeting ribonucleotide reductase M2 protein. Am. J. Cancer Res. 11 (6), 2975–2989.
Wang, X., Zeng, H., Zhu, X., Xu, D., Tian, Q., Wang, C., et al. (2022). TP-CSO: A triptolide prodrug for pancreatic cancer treatment. Molecules 27 (12), 3686. doi:10.3390/molecules27123686
Wang, Y., Wang, Y., Ren, Y., Zhang, Q., Yi, P., and Cheng, C. (2022). Metabolic modulation of immune checkpoints and novel therapeutic strategies in cancer. Semin. Cancer Biol. 86, 542–565. doi:10.1016/j.semcancer.2022.02.010
Wei, C., Khan, M. A., Du, J., Cheng, J., Tania, M., Leung, E. L., et al. (2022). Cordycepin inhibits triple-negative breast cancer cell migration and invasion by regulating EMT-TFs SLUG, TWIST1, SNAIL1, and ZEB1. Front. Oncol. 12, 898583. doi:10.3389/fonc.2022.898583
Wu, W., Li, X., Qi, M., Hu, X., Cao, F., Wu, X., et al. (2022). Cordycepin inhibits growth and metastasis formation of MDA-MB-231 xenografts in nude mice by modulating the hedgehog pathway. Int. J. Mol. Sci. 23 (18), 10362. doi:10.3390/ijms231810362
Xiao, Y., Yu, F., Pang, L., Zhao, H., Liu, L., Zhang, G., et al. (2015). MeSiC: A model-based method for estimating 5 mC levels at single-CpG resolution from MeDIP-seq. Sci. Rep. 5, 14699. doi:10.1038/srep14699
Xu, H., Wang, Q., and Guo, Y. (2011). Stereoselective synthesis of 4α-alkyloxy-2-α/β-bromopodophyllotoxin derivatives as insecticidal agents. Chemistry 17 (30), 8299–8303. doi:10.1002/chem.201100855
Yang, J., Zhou, Y., and Shi, J. (2020). Cordycepin protects against acute pancreatitis by modulating NF-κB and NLRP3 inflammasome activation via AMPK. Life Sci. 251, 117645. doi:10.1016/j.lfs.2020.117645
Yang, T., Huo, J., Xu, R., and Zhang, Y. (2021). Synergistic effect of toosendanin and regorafenib against cell proliferation and migration by regulating WWOX signaling pathway in hepatocellular carcinoma. Phytother. Res. 35 (8), 4567–4578. doi:10.1002/ptr.7174
Yang, T., Xu, R., Huo, J., Wang, B., Du, X., Dai, B., et al. (2021). WWOX activation by toosendanin suppresses hepatocellular carcinoma metastasis through JAK2/Stat3 and Wnt/β-catenin signaling. Cancer Lett. 513, 50–62. doi:10.1016/j.canlet.2021.05.010
Yao, L. C., Wu, L., Wang, W., Zhai, L. L., Ye, L., Xiang, F., et al. (2021). Panax notoginseng saponins promote cell death and chemosensitivity in pancreatic cancer through the apoptosis and autophagy pathways. Anticancer. Agents Med. Chem. 21 (13), 1680–1688. doi:10.2174/1871520620999201110191459
Yu, F., Li, K., Li, S., Liu, J., Zhang, Y., Zhou, M., et al. (2020). Cfea: A cell-free epigenome atlas in human diseases. Nucleic Acids Res. 48 (1), D40-D44–D44. doi:10.1093/nar/gkz715
Yu, F., Sankaran, V. G., and Yuan, G. C. (2021). CUT&RUNTools 2.0: A pipeline for single-cell and bulk-level CUT&RUN and CUT&Tag data analysis. Bioinformatics 38, 252–254. doi:10.1093/bioinformatics/btab507
Yu, F., Zhang, G., Shi, A., Hu, J., Li, F., Zhang, X., et al. (2018). LnChrom: A resource of experimentally validated lncRNA-chromatin interactions in human and mouse. Oxford: Database, 2018. doi:10.1093/database/bay039
Yu, L., Chen, M., Zhang, R., and Jin, Z. (2019). Inhibition of cancer cell growth in gemcitabine-resistant pancreatic carcinoma by mangiferin phytochemical involves induction of autophagy, endogenous ROS production, cell cycle disruption, mitochondrial mediated apoptosis and suppression of cancer cell migration and invasion. J. BUON 24 (4), 1581–1586.
Yuan, W., Huang, J., Hou, S., Li, H., Bie, L., Chen, B., et al. (2022). The antigastric cancer effect of triptolide is associated with H19/NF-κB/FLIP Axis. Front. Pharmacol. 13, 918588. doi:10.3389/fphar.2022.918588
Zhang, C., Gao, H., Liu, Z., Lai, J., Zhan, Z., Chen, Y., et al. (2021). Mechanisms involved in the anti-tumor effects of Toosendanin in glioma cells. Cancer Cell Int. 21 (1), 492. doi:10.1186/s12935-021-02186-2
Zhang, H., Yuan, Z., Zhu, Y., Yuan, Z., Wang, J., Nong, C., et al. (2022). Th17/Treg imbalance mediates hepatic intolerance to exogenous lipopolysaccharide and exacerbates liver injury in triptolide induced excessive immune response. J. Ethnopharmacol. 295, 115422. doi:10.1016/j.jep.2022.115422
Zhang, J., Yang, F., Mei, X., Yang, R., Lu, B., Wang, Z., et al. (2022). Toosendanin and isotoosendanin suppress triple-negative breast cancer growth via inducing necrosis, apoptosis and autophagy. Chem. Biol. Interact. 351, 109739. doi:10.1016/j.cbi.2021.109739
Zhang, M., Tao, Z., Gao, L., Chen, F., Ye, Y., Xu, S., et al. (2022). Toosendanin inhibits colorectal cancer cell growth through the Hedgehog pathway by targeting Shh. Drug Dev. Res. 83 (5), 1201–1211. doi:10.1002/ddr.21951
Zhang, S., Chen, C., Lu, W., and Wei, L. (2018). Phytochemistry, pharmacology, and clinical use of Panax notoginseng flowers buds. Phytother. Res. 32 (11), 2155–2163. doi:10.1002/ptr.6167
Zhang, S., Dong, Y., Chen, X., Tan, C. S. H., Li, M., Miao, K., et al. (2022). Toosendanin, a late-stage autophagy inhibitor, sensitizes triple-negative breast cancer to irinotecan chemotherapy. Chin. Med. 17 (1), 55. doi:10.1186/s13020-022-00605-8
Zhang, W., Zhu, Y., Yu, H., Liu, X., Jiao, B., and Lu, X. (2021). Libertellenone H, a natural pimarane diterpenoid, inhibits thioredoxin system and induces ROS-mediated apoptosis in human pancreatic cancer cells. Molecules 26 (2), E315. doi:10.3390/molecules26020315
Zhang, Y., Zhang, F., Gao, Y., Wang, M., Gao, Y., Li, H., et al. (2022). Triptolide in the treatment of systemic lupus erythematosus - regulatory effects on miR-146a in B cell TLR7 signaling pathway in mice. Front. Pharmacol. 13, 952775. doi:10.3389/fphar.2022.952775
Zhang, Y., Zhang, X. X., Yuan, R. Y., Ren, T., Shao, Z. Y., Wang, H. F., et al. (2018). Cordycepin induces apoptosis in human pancreatic cancer cells via the mitochondrial-mediated intrinsic pathway and suppresses tumor growth in vivo. Onco. Targets. Ther. 11, 4479–4490. doi:10.2147/OTT.S164670
Zhang, Y., Zhang, Y., Hu, J., Zhang, J., Guo, F., Zhou, M., et al. (2020). scTPA: a web tool for single-cell transcriptome analysis of pathway activation signatures. Bioinformatics 36 (14), 4217–4219. doi:10.1093/bioinformatics/btaa532
Zhao, X., Liu, Z., Ren, Z., Wang, H., Wang, Z., Zhai, J., et al. (2020). Triptolide inhibits pancreatic cancer cell proliferation and migration via down-regulating PLAU based on network pharmacology of Tripterygium wilfordii Hook F. Eur. J. Pharmacol. 880, 173225. doi:10.1016/j.ejphar.2020.173225
Zhong, C.-C., Zhao, T., Hogstrand, C., Chen, F., Song, C.-C., and Luo, Z. (2022). Copper (Cu) induced changes of lipid metabolism through oxidative stress-me diated autophagy and Nrf2/PPAR gamma pathways. J. Nutr. Biochem. 100, 108883. doi:10.1016/j.jnutbio.2021.108883
Zhou, J., Luo, J., Li, P., Zhou, Y., Li, P., Wang, F., et al. (2022). Triptolide promotes degradation of the unfolded gain-of-function Tp53(R175H/Y220C) mutant protein by initiating heat shock protein 70 transcription in non-small cell lung cancer. Transl. Lung Cancer Res. 11 (5), 802–816. doi:10.21037/tlcr-22-312
Zhou, Q., Zhang, Q., Wang, K., Huang, T., Deng, S., Wang, Y., et al. (2022). Anti-rheumatic drug-induced Hepatitis B virus reactivation and preventive strategies for hepatocellular carcinoma. Pharmacol. Res. 178, 106181. doi:10.1016/j.phrs.2022.106181
Zhou, Y., Kong, Y., Jiang, M., Kuang, L., Wan, J., Liu, S., et al. (2022). Curcumin activates NLRC4, AIM2, and IFI16 inflammasomes and induces pyroptosis by up-regulated ISG3 transcript factor in acute myeloid leukemia cell lines. Cancer Biol. Ther. 23 (1), 328–335. doi:10.1080/15384047.2022.2058862
Zou, B., Wang, H., Liu, Y., Qi, P., Lei, T., Sun, M., et al. (2017). Mangiferin induces apoptosis in human ovarian adenocarcinoma OVCAR3 cells via the regulation of Notch3. Oncol. Rep. 38 (3), 1431–1441. doi:10.3892/or.2017.5814
Keywords: pancreatic cancer, natural products, curcumin, toosendanin, triptolide, panax notoginseng saponins
Citation: He X, Wang N, Zhang Y, Huang X and Wang Y (2022) The therapeutic potential of natural products for treating pancreatic cancer. Front. Pharmacol. 13:1051952. doi: 10.3389/fphar.2022.1051952
Received: 23 September 2022; Accepted: 20 October 2022;
Published: 02 November 2022.
Edited by:
Chunpeng Wan, Jiangxi Agricultural University, ChinaReviewed by:
Jing Hu, University of Texas Southwestern Medical Center, United StatesCopyright © 2022 He, Wang, Zhang, Huang and Wang. This is an open-access article distributed under the terms of the Creative Commons Attribution License (CC BY). The use, distribution or reproduction in other forums is permitted, provided the original author(s) and the copyright owner(s) are credited and that the original publication in this journal is cited, in accordance with accepted academic practice. No use, distribution or reproduction is permitted which does not comply with these terms.
*Correspondence: Xiaobo Huang, ZHJodWFuZ3hiQDE2My5jb20=; Yi Wang, d195aTIwMjJAMTYzLmNvbQ==
†These authors have contributed equally to this work
Disclaimer: All claims expressed in this article are solely those of the authors and do not necessarily represent those of their affiliated organizations, or those of the publisher, the editors and the reviewers. Any product that may be evaluated in this article or claim that may be made by its manufacturer is not guaranteed or endorsed by the publisher.
Research integrity at Frontiers
Learn more about the work of our research integrity team to safeguard the quality of each article we publish.