- 1University Institute of Pharma Sciences, Chandigarh University, Mohali, Punjab, India
- 2University School of Pharmaceutical Sciences, Rayat Bhara University, Mohali, Punjab, India
- 3Natural Products for Neuroprotection and Anti-Ageing Research Unit, Chulalongkorn University, Bangkok, Thailand
- 4Department for Pharmaceutical Research and Development, Institute for Medicinal Plants Research “Dr. Josif Pančić”, Belgrade, Serbia
- 5Department of Pharmaceutical Sciences, College of Pharmacy, Shaqra University, Shaqra, Saudi Arabia
- 6Department of Pharmacy, Faculty of Biological Sciences, University of Malakand, Chakdara, Pakistan
- 7Shanghai Key Laboratory for Molecular Engineering of Chiral Drugs, School of Pharmacy, Shanghai Jiao Tong University, Shanghai, China
- 8Department of Natural Product Chemistry, School of Pharmacy, Shanghai Jiao Tong University, Shanghai, China
- 9Department of Pharmacy, Faculty of Medicine and Pharmacy, University of Oradea, Oradea, Romania
Depression is a condition characterized by low mood and an aversion to activity, that causes behavioral problems, poor quality of life and limits daily life activities. It is considered as the fourth leading cause of disability worldwide. Selective Serotonin Reuptake Inhibitors (SSRIs) Monoamine Oxidase (MAO) inhibitors, Tricyclic Antidepressants (TCAs), and atypical antidepressants are some of the conventional medications used to treat depression. However, only about half of patients with major depressive disorder (MDD) respond effectively to first-line antidepressant therapy. Additionally, there are a number of drawbacks to standard antidepressants, such as anti-cholinergic side effects, drug-drug interactions, and food-drug interactions, which prompts researchers to look at alternative approaches to the treatment of depression. Medicinal plants and their metabolites are extensively tested for their efficacy against depression. Electronic databases such as Google scholar, Science Direct, SciFinder and PubMed were used to search relevant literature on the role of polyphenols in depression. Plants-derived Polyphenols represent a major class of compounds extensively distributed in plants. Number of polyphenols have demonstrated antidepressant activity, among which berberine, piperine, curcumin, naringenin, ascorbic acid and ginsenosides are extensively evaluated. The medicinal plants and their derived compounds mediated synthesized green nanoparticles have also exhibited considerable efficacy in the management of depression. The therapeutic effects of these phytochemicals is mediated via differentiation and inhibition of neuronal cell apoptosis, promotion of neuronal cell survival and modulation of key neurotransmitters. The aim of this study is to review compressively the chemical, pharmacological and neurological evidence showing the potential of polyphenols in depression.
Introduction
Depression is a condition characterized by low mood and an aversion to activity. Low mood is a regular and expected part of the human condition, particularly when grieving loved ones. However, major depression disorder (MDD) is defined as a constant state of low mood that lasts for at least 2 weeks (Otte et al., 2016). Persistent depressive disorder (dysthymia), which may be less severe than MDD, lasts much longer (typically two or more years) (Baldwin et al., 1995). Other forms of depression include perinatal depression (occurring during or shortly after pregnancy in women) and seasonal affective disorder (occurring typically in the autumn or winter, with symptoms fading in the spring and summer). Depression affects a patient’s thoughts, mood, behavior, sense of well-being, and motivation. Patients may also have feelings of hopelessness, dejection, and possibly thoughts of suicide (de Zwart et al., 2019). Depression is thought to affect 5% of the world’s population (Institute of Health Metrics and Evaluation, 2021), and suicide rates in the United States of America rose alarmingly between 1999 and 2016 (Stone et al., 2018). According to the 2017 Global Burden of Disease (GBD) study the global incidence of depression cases between 1990 and 2017 has increased by 49.86% (Liu et al., 2020). The point prevalence rate of depressive symptoms was 34% from 2001 to 2020 globally, and for MDD was 8%. Among adolescents, the point prevalence of elevated depressive symptoms increased from 24% (between 2001 and 2010) to 37% (between 2011 and 2020) (Shorey et al., 2022). Furthermore, the World Health Organization lists suicide as the fourth leading cause of death in 15–29-year-olds worldwide (World Health Organization, 2021). Despite several clinically effective drugs for treating depression, people in more than 75% of middle and low-income counties do not receive treatment (Evans-Lacko et al., 2018; Ahsan et al., 2022).
Number of antidepressant agents such as SSRIs, MAO inhibitors and TCAs etc are commercially available which are in practice for the treatment of depression. The acute or chronic use of these agents may result in serious effects such as adverse drug reactions, drug-drug interactions or drug-food interactions (Pathak et al., 2013). These factors prompt the researchers to develop new antidepressant agents having minimal side effects and better efficacy. Medicinal plants and their metabolites are extensively tested for their efficacy against depression. Number of polyphenols have demonstrated antidepressant activity, among which berberine, piperine, curcumin, naringenin, ascorbic acid and ginsenosides are extensively evaluated. The therapeutic effects of these phytochemicals is mediated via differentiation and inhibition of neuronal cell apoptosis, promotion of neuronal cell survival and modulation of key neurotransmitters (Dhir, 2017). The present review aims to discuss compressively the antidepressant potential of polyphenols, including their mechanisms of action.
The monoamine hypothesis
The monoamine (catecholamine) hypothesis of depression (Figure 1) was proposed in early 1965's (Schildkraut, 1995) based on pharmacological evidence from drugs. For instance, reserpine and tetrabenazine deplete and inactivate monoamines intracellularly thus causing depression (Ruhé et al., 2007). On the contrary, amphetamine causes an increase in the synaptic norepinephrine levels and thus exhibit stimulant properties. Likewise, the monoaminoxidase (MAO) inhibitors prevent the breakdown of the monoamines within the synaptic cleft, giving rise to apparent antidepressant effects. Finally, the tricyclic class of antidepressants blocks serotonin and norepinephrine reuptake by serotonin and norepinephrine reuptake transporters (SERT and NET) in the synaptic cleft (Gillman, 2007). The hypothesis existed in the background until the late 1970s with the development of the first generation of selective serotonin reuptake inhibitors (SSRIs) (Spinks and Spinks, 2002) using rational design to improve the specificity of a drug to its intended target (Carlsson, 1999). Several antihistamines were known to have some antidepressant effects, which led to the development of zimelidine from the antihistamine drug bromopheniramine (Fagius et al., 1985), and later fluoxetine from the antihistaminic drug diphenhydramine (Wong et al., 1995). Fluoxetine (marketed as Prozac or Sarafem) was approved by FDA in the USA for the management of depression in 1987 and has subsequently become a prototype for the next generation of SSRI antidepressants. The development of SSRIs has continued, and there are now several on the market including citalopram (Celexa), escitalopram (Lexapro), fluvoxamine (Luvox), paroxetine (Paxil), and sertraline (Zoloft).
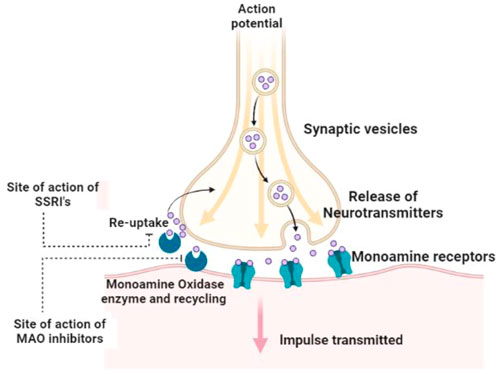
FIGURE 1. The monoamine (MAO) theory of depression; antidepressant drugs increase monoamine concentrations in the synaptic cleft leading to further signaling and the postsynaptic neuron.
It is proposed that SSRIs cause short-term increase serotonin levels in the synaptic cleft, thus stimulating more serotonin receptors on the postsynaptic nerve terminal. Subsequently, it augment serotonin signaling in neurons where serotonin is the primary neurotransmitter. The use of selective drugs that target the reuptake of serotonin in the synaptic cleft and thus increase serotonin’s availability appears to confirm the monoamine hypothesis. However, there are still several problems with the hypothesis. While meta analysis studies of SSRIs for patients with MDD have shown statistically significant improvements. Only in cases of severe symptoms, compared to placebo controls, many of these trials showed a high risk of bias, and the benefits were often outweighed by the risk of harmful side effects (Fournier et al., 2010; Jakobsen et al., 2017).
Furthermore, despite seeing an immediate increase in serotonin levels after treatment with SSRIs and the immediate antidepressant-like effects of SSRIs in animal studies, patients treated for depression with SSRIs do not see an immediate improvement in their condition and often requiring 2 weeks before improvements are observed (Edinoff et al., 2021). Additionally, during that time, there may be an apparent worsening of symptoms. With chronic dosing, the highly occupied serotonin receptors on the postsynaptic membrane trigger feedback signaling, resulting in less serotonin at the presynaptic neuron (Siesser et al., 2013). Furthermore, chronic dosing with SSRIs reduces the density of serotonin transporters (SERT) (Benmansour et al., 1999). Therefore, the regulation on serotonin levels in the synapse and the monoamine theory of depression does not adequately explain the pathophysiology of depression.
The sigma-1 receptor
The sigma-1 receptor (σ1R) is a chaperone protein found at the endoplasmic reticulum (ER) and mitochondrial membrane (MEM) and at the cell membrane. The σ1R is expressed throughout the body, but it is particularly densely expressed in the CNS. Expression of the σ1R appears to act as a cell survival signal, making it a potential target in many diseases, including cancer (Spruce et al., 2004; Brimson et al., 2020a), Alzheimer’s disease (AD) (Prasanth et al., 2021), Parkinson’s disease (PD) (Brimson et al., 2018), Huntington’s disease (HD), multiple sclerosis, amyotrophic lateral sclerosis (ALS) (Brimson et al., 2020b), heart disease, viral infections (Brimson et al., 2021) and depression (Brimson et al., 2020a).
Sigma-1 receptor is a promising therapeutic target in the treatment of neurodegenerative diseases including depression and schizophrenia as it stabilizes the function of several intracellular systems through its role as a chaperone when activated by a variety of ligands with neuroprotective properties. σ Receptor ligands may cause an inhibition of ischemic-induced presynaptic release of excitotoxic amino acids, indicating that σ ligands could also serve as neuroprotective agents (Maurice and Lockhart, 1997). The saffron extracts and crocetin has been reported to have antidepressant effects due to involvement of σ1Rs (Lechtenberg et al., 2008).
The σ1Rs involvement in the pathophysiology of depression is often hotly debated; however, there is plenty of evidence regarding its possible role. Initial interest in the σ1R for depression treatment stems from the observation that most antidepressant drugs have moderate to high affinity for the σ1R (Narita et al., 1996; Brimson et al., 2020b). Furthermore, many antidepressant drugs act as σ1R agonists (with the notable exceptions of sertraline, a σ1R antagonist, and paroxetine which does not affect the σ1R). The order in affinity of the SSRIs for the σ1R is as follows, fluvoxamine > sertraline > fluoxetine > escitalopram > citalopram >> paroxetine. Among these, fluvoxamine, fluoxetine, and escitalopram can potentiate neurite outgrowth in PC-12 cells in a σ1R antagonist sensitive manner.
Furthermore, fluvoxamine improved phencyclidine-induced cognitive deficits in mice, and this could be reversed by the σ1R antagonist NE-100, whereas sertraline (σ1R antagonist) and paroxetine (low affinity for σ1R) had no such effects on phencyclidine treated mice (Albayrak and Hashimoto, 2017). Clinical evidence also suggests a prominent role for the σ1R in the pathophysiology of the σ1R, as when switching patients with psychotic depression from fluvoxamine (SSRI with strong σ1R agonist activity) to sertraline (SSRI and σ1R antagonist), the patient’s symptoms worsened (Kishimoto et al., 2010). This has led to the implication that the σ1R is involved in the beneficial mechanism of fluvoxamine (Hayashi, 2005; Ishikawa and Hashimoto, 2010; Albayrak and Hashimoto, 2017).
Studies using animal models have further provided evidence of the σ1R’s involvement in depression. The σ1R ligands such as PRE-084 (Skuza and Rogóż, 2009), (+)-pentazocine, DTG, SKF-10,047 (Urani et al., 2004) and igmesine (Urani et al., 2001) all dose-dependently reduce immobility time in the forced swim test in an antagonist sensitive fashion. Furthermore, σ1R knockout mice show an apparent depressive-like phenotype in the forced swim test (Sabino et al., 2009). Moreover, mice treated with aortic banding and a high salt diet in a heart failure model showed depressive-like symptoms in the tail suspension test and had reduced expression of the σ1R in the brain (Ito et al., 2012).
Clinical studies have been conducted with σ1R specific ligands igmesine (Roman et al., 1990) and opimarol (structurally similar to imipramine) (Müller et al., 2004; Volz and Stoll, 2004). Igmesine, showed a response rate as high as 83%, although later, larger trials were less conclusive (Pande et al., 1999). However, this trial did appear to have a high response rate in the placebo group, which could have clouded the effects.
Brain regions and depression
Studies have shown that several regions of the brain are affected in patients suffering from depression. Additionally, the heterogeneity among depressed patients makes it difficult to identify specific brain regions among depressed populations and interpret findings (Graham et al., 2013). However, studies have shown the hippocampus as one such region of the brain with atrophy in MDD (Sheline et al., 1996; Lloyd et al., 2004; Cole et al., 2011). Stress can lead to depression-like symptoms and an excess of cortisol production. The hippocampus is particularly rich in glucocorticoid receptors making it more vulnerable to long-term stress than other regions of the brain, and this is the potential cause of hippocampal atrophy (Campbell and Macqueen, 2004). For instance, σ1R knockout mice show decreased neurogenesis in the hippocampus (Sha et al., 2015) and a depressive-like phenotype. Moreover, SSRIs with σ1R affinity such as fluoxetine can prevent stress-induced atrophy (Luo and Tan, 2001) and even induce further neurogenesis (Santarelli et al., 2003).
Other regions of the brain have been identified as either hyper or hypoactive in cases of MDD. The amygdala, part of the brain involved in emotional processing, is hyperactive in those with MDD. The size of the amygdala appears larger in medicated patients than un-medicated, although there is no noticeable difference between depressed patients and healthy people (Hamilton et al., 2008). The prefrontal cortex (PFC) has been identified as underactive in MDD. The PFR regulates emotional processing; its low activity may be involved in the etiology of depression. Treatment with norepinephrine reuptake inhibitors (SNRIs) has been shown to increase activity in the PFR, whereas SSRIs are amygdaloid-hippocampal regions (Outhred et al., 2013). The different brain regions affected by different antidepressant classes may explain the different efficacies of different drugs between patients.
The immune system, inflammation, and depression
While it is unlikely that inflammation is the primary cause of the MDD, there is evidence that it plays a role. Approximately 33% of MDD cases show an increase in inflammatory markers compared to healthy controls (Krishnadas and Cavanagh, 2012). Also, patients with inflammatory diseases are at greater risk of MDD (Choi et al., 2019), and treatment with anti-inflammatory drugs can reduce depression symptoms (Köhler et al., 2014). Moreover, treatment with cytokines such as interferon-alpha (IFN- ɑ) (used in treatments for cancer and chronic hepatitis C) can result in an increased risk of depression, with studies suggesting a prevalence of 20%–30% (Sockalingam et al., 2011).
Multiple studies in animals have identified the effects of inflammatory cytokines serotonin turnover in the brain (Dunn and Wang, 1995; Dunn et al., 1999; Anisman et al., 2005). Clinical studies have shown that the SSRI paroxetine can reduce symptoms of depression in patients treated with IFN-ɑ (Musselman et al., 2001; Lotrich et al., 2009). Furthermore, pro-inflammatory cytokines have been shown to affect dopamine production and reuptake in the brain (Morón et al., 2003; Wu et al., 2007). Glutamate is another excitatory neurotransmitter associated with inflammation and neurological disease through excitotoxicity and oxidative stress (Brimson et al., 2018). IL-1β prevents the reuptake of glutamate by glial cells, which leads to increased glutamate in the synapse and causes further NMDA-mediated excitotoxicity. Furthermore, IL-1β induces nitric oxide synthase production and thus an increase in nitric oxide (NO) production, leading to further glutamate release causing further excitotoxicity and oxidative stress (Hu et al., 2000).
Genetics of depression
The heritability of depression has been identified in twin studies, with estimates suggesting heritability ranged from 22% to 37% (McGue and Christensen, 2003) and as high as 70% in another (Kendler et al., 1993). This strongly suggests a genetic link when it comes to the development of the MDD.
While there may well be a genetic link to many forms of depression, once again, the heterogeneous nature of MDD has made it difficult to identify genes that contribute to increased risk. Two possible candidate genes include the serotonin transporter genes and brain-derived neurotrophic factor (BDNF). The serotonin transporter genes and genes related to its expression and the serotonin receptor 2A (5Ht-2A receptor) have long been candidates due to their proposed role in the monoamine hypothesis of depression (Lohoff and Ferraro, 2010). A 44 base pair repeat polymorphism in the promoter of the serotonin transporter (5-HTTLPR) has been shown, in vitro, to influence the expression of the transporter (Lesch et al., 1996). The Val66Met polymorphism in BDNF has been extensively studied in bipolar disorder and MDD. The results for the association between the Val66Met single nucleotide polymorphism (SNP) and other polymorphisms in BDNF and MDD have been mixed. Haplotype analysis of BDNF indicated an association with MDD (Schumacher et al., 2005), whereas studies of the Val66Met SNP have yielded negative results (Surtees et al., 2007).
Plant polyphenols: Sources and chemistry
Medicinal plants is a rich source of bioactive compounds with diverse pharmacological potentials (Mir et al., 2019; Muhammad et al., 2021a; Muhammad et al., 2021b; Muhammad et al., 2021c). Polyphenols represent greatly varying group of compounds extensively distributed in plants (Ovais et al., 2018b; Zohra et al., 2019). Their chemical structure as well as their composition are significantly influenced by environmental factors and plant species (Ofosu et al., 2020). According to their structure and complexity, they can be classified in two main sub-groups: flavonoids (flavones, flavonols, flavanones, flavanonols, isoflavonoids, flavanols, anthocyanidins and chalcones) and non-flavonoid compounds (phenolic acids, stilbenes, lignans and tannins) (Ayaz et al., 2019) Figure 2.
Flavonoid compounds
Flavonoids constitute a wide group of at least 6000 polyphenolic compounds which differ in their structure but generally, they contain two aromatic rings (A and B rings) linked by a 3-carbon chain making an oxygenated heterocyclic ring (C ring). Based on variations in structure of C ring, as well as functional groups on the rings and the position at which the B ring is connected with the C ring, there are six subclasses of flavonoids, namely flavones, flavonols, flavanones, flavanols, isoflavones and anthocyanidins (Ayaz et al., 2019). Six-member ring linked with the benzene ring is either a α-pyrone (flavonols and flavanones) or its dihydroderivative (flavonols and flavanones). According to the position of the benzenoid substituent flavonoids are separated into flavonoids (2-position) and isoflavonoids (3-position). Flavonols, the most common flavonoids in food, vary from flavanones based on hydroxyl group at the 3-position and a C2–C3 double bond. Flavonoids are usually hydroxylated in positions 3,5,7,2,3′,4′ and 5′ (Ayaz et al., 2019). The most investigated flavonol compounds are rutin and quercetin, and they are mainly present in buckwheat, asparagus, and citrus fruits, as well as in peaches, apples, and green tea. In relation to flavones (e.g., apigenin, luteolin) as the greatest sources of this subclass stand out celery, red peppers, chamomile, mint, parsley, rosemary, oregano, and ginkgo biloba (Truzzi et al., 2021). Grapes and berries are well known sources of flavanols where they are present in the form of (+)-catechin and (−)-epicatechin (Gadkari and Balaraman, 2015). Isoflavones, particularly daidzein, dominantly exist in leguminous plants, and they can be found in large quantities in soybeans and soymilk (Truzzi et al., 2021). Widely-known flavanones including naringin, neoeriodictyol and neohesperidin are usually found in citrus fruits and their products. Other flavanones are present in some medicinal plants such as mentha (Nazzaro et al., 2020). Dietary sources of anthocyanins (e.g., cyandin, malvidin) involve different red and purple berries, grapes, as well as apples, plums, cabbage, and other types of foods comprising high level of natural colorants (Mattioli et al., 2020).
Flavonoids exist in various forms such as aglycones, glycosides and their methylated derivatives. In case of glycosides, the glycosidic bond is ordinarily placed at positions 3 or 7 and the carbohydrate can be L-rhamnose, D-glucose, glucorhamnose, galactose, or arabinose. Methyl ethers and acetyl esters of the alcohol group are also identified in nature (Kumar and Pandey, 2013).
Non-flavonoid compounds
Tannins
Tannins is a group of naturally occurring high molecular weight polyphenols with a relatively complex structure. In nature they can be found in complexes with alkaloids, polysaccharides, and proteins and further divided into hydrolysable tannins and condensed tannins (also known as proanthocyanidins). Polymers of catechin, epicatechin, and/or leucoanthocyanidin, commonly named condensed tannins, are the most abundant polyphenols in woody plants. The degree of polymerization differs significantly and can range from several to more than 50 flavanol molecules. While small molecules of condensed tannins are soluble in aqueous or organic solvents, the large polymers are insoluble, and this disturbs their analysis, comprising determination of their content in food (Khanbabaee and Van Ree, 2001). Esters of gallic and ellagic acids are termed hydrolysable tannins. The center of the hydrolysable tannin molecule represents a glucose ring and its hydroxyl group can be esterified with gallic or ellagic acid, obtaining gallotannins or ellagitannins, respectively (Singla et al., 2019).
Phenolic acids
Polyphenols with a carboxylic acid are called phenolic acids. They are abundant in plant-based foods with highest content in seeds, fruits peel and vegetables leaves. Generally, they can be found in bounded form such, in particular as amides, esters, or glycosides and sparsely as free (Kumar and Goel, 2019). There are two representative parent structures of phenolic acids. In the case when the carboxyl group is directly attached to the phenol ring, the phenolic compound is known as hydroxybenzoic acid. In the case when C=C bond separated the carboxyl group and phenolic ring, these molecules are named hydroxycinnamic acids (Al Mamari, 2021). Substituted derivatives of hydroxybenzoic and hydroxycinnamic acids are the principal phenolic acids in plants, and hydroxycinnamic acids are with greater incidence. Phenolic acids can varying in the pattern of the hydroxylation and methoxylation in their aromatic rings. The most usual hydroxycinnamic acids are caffeic, p-coumaric and ferulic acids. They are often present in foods as simple esters with quinic acid or glucose. Perheps the most well-known bound hydroxycinnamic acid is chlorogenic acid, which provide combination of caffeic and quinic acids. Diverse from hydroxycinnamates, hydroxybenzoic acid derivatives are generally found in foods in the form of glucosides and molecules as p-hydroxybenzoic, vanillic and protocatechuic acids are the most common forms (Shahidi and Ambigaipalan, 2015).
Lignans
Lignans are a non-flavonoid compounds characterized with two propylbenzene units (C6-C3) linked together between the β-position in C8 of the propane side chains. Positions C9 and C9ˋ are substituted in various arrengements, having as a result in a high diversity of their structural forms. Accordingly, lignans are organized in eight subgroups: furans, furofurans, dibenzylbutanes, dibenzylbutyrolactones, dibenzocyclooctadienes, dibenzylbutyrolactols, aryltetralins and arylnaphthalenes. Lignans are phytoestrogens and can be found in legumes, seeds, and vegetable oils. In high amounts they can be present in flaxseed and flaxseed oil, with the prominent compounds being secoisolariciresinol and matairesinol (Amawi et al., 2017). Lignans are generally present in their free forms, whereas glycosylated molecules are not abundant (Ayres and Loike, 1990).
Stilbens
Another comparably minor class of non-flavonoids is stilbenes (1,2-diarylethenes) which are characterized by two phenyl moieties connected together by a two-carbon methylene groups. In stilbenes, the m-positions in ring A are usually substituted by two hydroxyl groups, while different positions in ring B may be substituted by hydroxyl and methoxyl groups. Stilbenes occur as cis and trans isomers, and they can exist in free (minor) and glycosylated (major) forms. Stilbenes are not usual in plants and they are generated just upon pathogen invasion (Amawi et al., 2017). One of the most analysed stilbenes is resveratrol (3,5,4′- trihydroxystilbene), that is produced by several plant species, in particular grapes, peanuts, and berries (Shen et al., 2009). Generally, the red wine is accepted as source for stilbenes, and those are resveratrol, piceid, piceatannol, astringin and pterostilbene and their dimers (Zhang et al., 2021). Also, some plant species from Polygonum genus, like Polygonum cuspidatum, which have not been applied as dietary ingredients, contain high content of stilbenes (Nonaka et al., 1982).
Nanoformulations of plant polyphenols used in depression
Nanotechnology and bio-inspired nanoparticles have got great attention these days and are extensively reported in pre-clinical studies against various diseases (Ovais et al., 2018a; Qasim Nasar et al., 2019; Chittaranjan Patra et al., 2021; Sani et al., 2021). The application of non-targeted antidepressants can provoke various side effects besides their low efficacy (Haque et al., 2014). One of the strategies in treatment of depression is to develop nanotechnology-based drug delivery systems. Nano-formulations have numerous advantages since they have specific control, as well as continued and targeted release characteristics (Ovais et al., 2018c; Khalil et al., 2018; Ovais et al., 2019; Ayaz et al., 2020; Nasar et al., 2022).
Curcumin
Antidepressant activity of curcumin has been tested in various animal models, and it was shown that it acts through decreasing inflammation, ameliorating oxidative stress induced apoptosis, and regulating the release of serotonin and dopamine. Animal and in vitro investigations have demonstrated that administration of curcumin could regulate the level of serotonin and dopamine in the central nervous system (Asadi et al., 2020). Main drawbacks of curcumin use as a therapeutic agent is its considerably low water solubility, insufficient permeability cross the blood-brain barrier (BBB), and poor bioavailability. These major challenges make curcumin problematic for use as an optimal antidepressant agent. Researchers utilized solid lipid nanoparticles (SLNs) to encapsulate curcumin (Cur) together with dexanabinol (HU-211). HU-211 represents an artificially synthesized cannabinoid derivative without cannabimimetic effects. Due to its highly lipophilic nature, HU-211 may present a new treatment procedure for MDD. On the contrary to curcumin, HU-211 use is interrupted by its low stability in biological systems and poor cellular uptake. To overreach these limitations, nanotechnology-based drug delivery systems are prospective strategy. The antidepressant effects of the dual-drug nanoparticles (Cur/SLNs-HU-211) for MDD treatment were analyzed in corticosterone-induced cellular and animal models of MDD. According to the results, Cur/SLNs-HU-211 effectively protected PC12 cells from corticosterone-induced apoptosis and induced higher dopamine level release, which may be connected with the higher uptake of Cur/SLNs-HU-211 exhibited by cellular uptake behavior analysis. Moreover, Cur/SLNs-HU-211 nanoparticles significantly decreased the immobility time in forced swimming test, improved fall latency in rotarod test, and increased the dopamine level in mice blood. Cur/SLNs-HU-211 can deliver more Cur to the brain providing that way a significant enhancement in neurotransmitters level in brain tissue, primarily in the hippocampus and striatum. The data obtained using Western blot and immunofluorescence demonstrated that Cur/SLNs-HU-211 nanoparticles can significantly improve the expression of CB1, p-MEK1, and p-ERK1/2.
Also, Asadi et al. (2020) performed a double-blind, randomized clinical trial designed to examine impact of nano-curcumin supplement on depression, anxiety and stress level in diabetic patients with peripheral neuropathy (Asadi et al., 2020). Eighty patients entered the study and the participants were divided randomly in two groups: intervention and control groups. They received 80 mg of nano−curcumin or placebo capsules per day during 8 weeks. At baseline of the investigation, as well after it, anthropometric measurements, dietary intake, physical activity, glycemic indices, and severity of neuropathy were evaluated. Depression, anxiety, stress scale questionnaire was used for measuring of the depression, anxiety, and stress level before and at the end of the intervention. According to the obtained data, there was a significant reduction in the mean score of depression in the nano-curcumin group after the study completion (from 16.7 [3.1] to 15.3 [2.6]) in comparison to placebo group (17.5 [3.2] to 17.3 [3.1]). These outcomes implied that application of nano-formulation with curcumin during 8 weeks was beneficial in alleviating depression.
Baicalein
Chen et al. (2018) analysed anti-depressant effect of baicalein-loaded solid lipid nanoparticles in animal model. These particles were modified using N-acet Pro-Gly-Pro (PGP) peptide aiming to promote binding of nanoparticles to neutrophiles in vivo and achieve brain-targeted delivery (Chen et al., 2018). In previous study, Lee at al. (2013) showed both acute and chronic anti-depressant effects of baicalein (Lee et al., 2013). After i.p application 1–4 mg kg−1 of baicalein the immobility time was significantly lowered in forced swimming test and tail suspending test. As stated by the same group of authors, chronic administration of equal baicalein doses during 21 days decreased the immobility time and enhanced locomotor activity in chronic unpredictable mild stress model in rats. Higher doses (10–40 mg kg−1) applied i.p. 30 min prior to daily exposure to continual restrained stress reduced depression-like behavior 14 days afterwards the application. According to Chen et al. (2018) brain distribution assay showed that baicalein-loaded solid lipid nanoparticles modified using PGP enhanced drug concentration in BLA region greatly (Chen et al., 2018). This region is the major one connected with emotional and psychiatric disturbances. Results of the study also revealed that the tested nanoparticles managed to lower immobility time, improve swimming and climbing time and weaken locomotion in olfactory-bulbectomized rats.
Silymarin
Ashraf et al. (2019) investigated the effect of silymarin alone or its nanostructured lipid carrier formulation on depressive-like behavior triggered by chronic unpredictable mild stress (CUMS) (Ashraf et al., 2019). During this research mice were exposed to CUMS pattern for 14 days. Within 2 weeks animals received silymarin (200 mg kg−1, p.o.) by itself or its nanoparticle formulation, or fluoxetine (10 mg kg−1, p.o.). On the 15th day behavioral and biochemical parameters were analyzed. According to the results of behavioral despair tests, oral application of silymarin (200 mg kg−1), especially in the form of nanoparticles, demonstrated an antidepressant-like effect that was comparable with the one showed by fluoxetine. Silymarin nanoparticles achieved reduction of weight gain, increased immobility time in both the teil suspension test and forced swimming test, in addition to the decreased time spent grooming in the splash test. In the same study silymarin reversed prefrontal cortical and hippocampal oxidative stress and neuroinflammation induced by CUMS. Additionally, it increased neurotransmitter levels (NE and 5-HT levels), strengthened neurogenesis and suppressed the activation of nod-like receptor protein 3 inflammasome. For certain parameters, nanoparticles of silymarin exhibited higher effect compared to silymarin most likely as a result of significantly higher brain concentration of silybinin that represent the principal active component of silymarin. Namely, its concentration was almost 12-fold higher in the group that received silymarin nanoparticles in comparison to the group treated with silymarin alone. The authors concluded that antidepressant-like activity of silymarin might be assigned to its antioxidant and anti-inflammatory effects together with enhanced neurogenesis in the prefrontal cortex and hippocampus.
Gallic acid
Chhillar and Dhingra (2013) demonstrated recently anti-depressant effect of gallic acid in mice subjected to unpredictable chronic mild stress (Chhillar and Dhingra, 2013). Similarly, Nabavi et al. (2016) showed antidepressant-like activity of gallic acid applied intraperitoneally in balb/c mice with post-stroke depression (Nabavi et al., 2016). Aiming to enhance its therapeutic effect, targeted delivery of gallic acid to brain is the imperative for showing an effective; better tolerated anti-depressant activity. Nagpal et al. (2012) investigated the anti-depressant activity of gallic acid loaded chitosan nanoparticles (GANP) and accordant tween 80 coated batch (cGANP) in mice (Nagpal et al., 2012). Both types of nanoparticles were applied in a quantity equivalent to 10 mg kg−1 gallic acid once per day during 1 week. Anti-depressant activity was evaluated using Despair Swim Test and Tail Suspension Test. Based on the significant enhancement of in vivo pharmacodynamic activity; better MAO-A inhibition; and stronger in vivo antioxidant activity obtained using cGANP authors presented the favorable outcome of ligand coated nanoparticulate system for the delivery of gallic acid across brain.
Quercetin
Bhutada et al. (2010) showed the promising role of quercetin in depressive-like disorders (Bhutada et al., 2010). It improved behavioral disorders in mice and rats with anxiety and depressive-like behaviors provoked with the corticotropin-releasing factor. Nevertheless, low absorption, fast metabolism and narrow capacity for crossing the blood-brain-barrier are restraints for its application in the treatment of neuropsychological diseases. Due to excessive first-pass metabolism, the effective dose of quercetin required to achieve the neurological activities might be considerably high. Nasal utilization provides various advantages such as avoiding the hepatic presystemic metabolism, simple dose regulation, continuous absorption and great convenience of application in patients (Xu et al., 2020). Also, nasal drug delivery bypasses the blood-brain barrier; it targets drugs directly to brain across neural connections between the olfactory epithelium, olfactory bulb, trigeminal nerve, and finally, the brain (Trevino et al., 2020). Tong-Un et al. (2010) determined the anti-depression like activity of quercetin liposomes applied nasally. Male Wistar rats were received quercetin liposomes, containing 20 μg in one dose, via intranasal route once daily continually for 4 weeks (Tong-Un et al., 2010). Anti-depressant effect was assessed employing forced swimming test and the results exhibited that free liposomes and vehicle (PEG) treatment alone did not provide significant changes in immobility time at all treatment intervals applied in this investigation. At the same time, quercetin liposomes induced a considerable decrease in the immobility time at all treatment duration. The anti-depressant generally reduces immobility time without stimulating motor activity. According to the same group of authors, the forced swimming test data showed that the acute and the repeated administration of quercetin liposomes acted like an anti-depressant drug in rat, by decrease of the immobility time. Altogether, the obtained data proposed that the mechanism underlying the anti-depression activity of nasally applied quercetin liposomes may include the inhibition MAO-A and increase the serotonin level. Furthermore, the electropharmacogram of adult rats which received quercetin have the same pattern as the well-known antidepressant moclobemide, MAO-A inhibitor (Dimpfel, 2009).
Mechanisms underlying the anti-depressant effects of phytochemicals
Efficacy of poly-phenols in depression
The subgroups of phenolic compounds includes flavonoids, coumarins, chromones, antraquinones, phenolic acids (carboxylic acid derivatives), lignanes, and stilbenes (Garcia-Salas et al., 2010; Ayaz et al., 2017b) (Figure 2). Flavonoids are phenolic compounds whose antiviral, antibacterial, hepatoprotective, antioxidant and anti-inflammatory activities have also been reported (Kumar and Pandey, 2013). They have been widely investigated for their antidepressant effects (Figures 3, 4; Table 1). Genistein is an isoflavone that can cross blood brain barrier (BBB) in mice (Si and Liu, 2008) and rats (Tsai, 2005) and its antidepressant effects on chronic use for 21 days, have been reported in male mice on forced swimming test (FST) and tail suspension test (TST) (Hu et al., 2017). In one study, the antidepressant effects of quercetin 4′-O-glucoside or quercetin has been reported in mice on FST and it was demonstrated that quercetin 4′-O-glucoside at a dose of 20 mg kg−1 produced the same effects as produced by fluoxetine at 20 mg kg−1 (Singh et al., 2021). Another study showed that quercetin administration at 10 mg kg−1 for 14 days decreased immobility time on TST but did not reduce immobility time on FST, however it was further found immobility time was reduced on both tests (TST and FST) at 25 and 50 mg kg−1 (Holzmann et al., 2015). The antidepressant mechanism of quercetin may be due to NMDA receptors inhibition that result in reduced intracellular calcium level which further leads to inhibition of protein calmodulin and then neuronal nitric oxide synthase resulting in decreased nitric oxide levels (NO) (Holzmann et al., 2015). In the brain, glutamate is the main excitatory neurotransmitter and its increased level in the synapse causes excessive stimulation of N-methyl-D-aspartate (NMDA) receptors that result in NMDA-mediated excitotoxicity and various forms of damage, such as a massive influx of calcium and the release of nitric oxide (NO) (Bishop and Anderson, 2005). Increase production of nitric oxide (NO) leading to further glutamate release causing further oxidative stress and excitotoxicity. Additionally, several studies have reported that nitric oxide synthase inhibition can cause antidepressant-like effect (Zeni et al., 2011).
Another study explored the antidepressant potential of chrysin at 20 mg kg−1 for 14 days in male OB C57B/6J mice using splash test (SP), where antidepressant effects were measured as increased in gromming time at 5 and 20 mg kg−1 doses decreased immobility time in obese (OB) mice on FST, but the hippocampal concentration of 5-HT and brain-derived neurotrophic factor (BDNF) was found increased (Borges Filho et al., 2016).
In another study, fisetin administration at 5 mg kg−1 increased the activation of the tropomyosin kinase B receptor (TrkB) through its phosphorylation in the hippocampus, which may result in pro-neurogenesis (Wang et al., 2017) reflecting its antidepressant effect on TST and FST. Apart from this fisten reversed depression in mice induced by spatial restraint stress which was demonstrated on FST and TST (Wang et al., 2017). Other studies have shown that dihydromyricetin triggered the ERK1/2-CREB pathway and enhanced glycogen synthase kinase-3 beta (GSK-3 beta) phosphorylation at ser-9, resulting in elevated BDNF expression in the hippocampus while suppressing neuroinflammation. These results might be explained by the antidepressant effect seen on TST and FST after administration of 10 and 20 mg kg−1 for 3 days (Ren et al., 2018). Hesperidin is another flavonoid, which may raise hippocampal BDNF levels at 0.3 and 1 mg kg−1 daily dose for 21 days. Hesperidin produced antidepressant effect at 0.3 and 1 mg kg−1 on TST was found similar to the effects produced by imipramine at 15 mg/kg and fluoxetine at 32 mg kg−1 (García-Ríos et al., 2020).
Baicalin is another flavonoid that has been proven to have CNS effects. It may enhance neuronal differentiation by increasing BDNF and extracellular signal-regulated kinase (ERK) phosphorylation (Xiong et al., 2011). The glucuronide glycoside of baicalin showed antidepressant activity by reducing monoamine oxidase (MAO)-A and B enzymes (Zhu et al., 2006). It has been suggested that baicalin at a 60 mg kg−1 produced the same effect on TST and Standard Penetration Test (SPT) as that produced by fluoxetine at 15 mg kg−1 (Zhang R. et al., 2019). Another flavonoid with antidepressant and antioxidant properties is naringin, which decreased immobility on the FST when administered at a dose of 2.5, 5, and 10 mg kg−1 for 7 days. The antidepressant effect of these doses was associated with increased cholinergic transmission as a result of decreased activity of the enzyme acetylcholinesterase and antioxidant defence systems caused by increased GSH levels, as well as increased activity of superoxide dismutase (SOD) and catalase (CAT) in mice brains.
Apart from this, naringin decrease ROS level and nitrogen species resulting in inhibition of nitrosative processes and lipid peroxidation (Ben-Azu et al., 2019). Naringenin is a major flavonoid of grapefruit, whose antidepressant activity has been reported, it increase NE, 5-HT, and BDNF levels and glucocorticoid receptors (Yi et al., 2014). Luteolin is a major flavonoid of the Cirsium japonicum extract, decreased the immobility time on FST at 5 and 10 mg kg−1 dose in the same manner as decreased by the antidepressant imipramine at a dose of 5 mg kg−1 (De La Peña et al., 2014).
Antidepressant effects of other phenolic compounds
Apart from flavonoids, other phenolic compounds are also reported for their antidepressant effects. Bis-eugenol showed antidepressant effect due to synthesis of 5-HT and participation of dopamine (DA) receptors (do Amaral et al., 2013). In depression models, magnolol from Magnolia officinalis bark altered brain BDNF level as well as serotonergic, noradrenergic and dopaminergic neurotransmission (Li et al., 2013). Among coumarins related compounds, scopoletin by affecting DA D1, D2, and 5-HT2a receptors and α1 and α2 adrenoceptors and psoralen by altering HPA axis demonstrated antidepressant effect in animal depression models (Capra et al., 2010).
Among the phenolic acids related compounds, the antidepressant like effects of paeoniflorin, galic acids, eugenol, freulic acid and ascorbic acid have been reported previously. Paeoniflorin from Paeonia lactiflora root (ancient Chinese antidepressant) demonstrated antidepressant effect by affecting HPA axis and by up-regulation of serotonergic system (Qiu et al., 2013). Gallic acid decreased plasma nitrite, corticosterone and malondialdehyde levels as well as prevented MAO-A activity induced by stress (Chhillar and Dhingra, 2013). Eugenol showed antidepressant like effects by increasing hippocampal BDNF level and inhibiting MAO-A and MAO-B effects (Irie, 2006). Ferulic acid showed antidepressant effects by interaction with 5-HT1a and 5-HT2a receptors (Zeni et al., 2012). Another phenolic acid is ascorbic acid (vitamin C), demonstrated antidepressant effects by serotonergic, dopaminergic and noradrenergic systems, NMDA receptors, K+ channels, alteration of mammalian target of rapamycin pathway and l-arginine-nitric oxide-cyclic guanosine monophosphate (l-arginine-NO-cGMP) pathway (Moretti et al., 2014). Another phenolic compound is curcumin, showed antidepressant effects by elevation of BDNF and brain monoamine levels, reduction of pro-inflammatory cytokines and prevention of MAO-A and MAO-B activities (Jiang et al., 2013).
Alkaloids as potential anti-depressant agents
Alkaloids are nitrogenous pharmacologically active secondary plant metabolites having diverse chemical structures and obtained from crude acid-base extracts (Perviz et al., 2016) (Figure 5). The anti-inflammatory tranquilizer and antiarthritic potential of isoquinoline alkaloids, morphine and colchicine respectively have been demonstrated previously (Lopes et al., 2018). Using FST in rats, berberine at a dose of 50 mg kg−1, increases climbing behavior and decreases immobility which reflects antidepressants effects (Lee et al., 2012). The antidepressants effects of Annona cherimola extract containing number of alkaloids including liriodenine, anonaine, nornuciferine and 1,2-dimethoxy-5,6,6a,7-tetrahydro-4H-dibenzoquinoline-3,8,9,10-tetraol, have been reported in mice FST. These alkaloids have been suggested to increase 5-HT and DA (Martínez-Vázquez et al., 2012).
The dose dependent antidepressant effects of β-carbolines derivatives such as harmane, norharmane and harmine have been reported previously (Farzin and Mansouri, 2006). The antidepressants effects of harmine has been compared with imipramine at different doses for 14 days in rats using FST, BDNF protein levels were increased by harmine in the hippocampus of rats while imipramine did not increase BDNF protein levels, reflecting that antidepressant effect is due to rise in hippocampal BDNF level (Fortunato et al., 2010a; b). One study reported that harman infusion increases 5-HT concentration and decreases degradation of 5-HT metabolite levels due to MAO-A inhibition (Baum et al., 1996). Another study suggested that harmane injection increase plasma concentration of corticosterone, adrenocorticotrophic hormone (ACTH), noradrenaline (NA) and 5-hydroxytryptamine (5-HT) in the structures of limbic system, suggesting that harman can modulate brain neurochemistry, behavioral alterations and neuroendocrine functions by inhibiting MAO-A (Smith et al., 2013).
The alkaloid fractions of Rhazya stricta extract containing rhaziminine, akuammidine, and tetrahydrosecamine inhibited MAO-B enzyme after oral administration to male rats for 21 days, which is responsible for antidepressant-like effect (Ali et al., 1998). Napelline, hypaconitine, songorine, and mesaconitine are diterpene alkaloids of Aconitum baicalense which have shown antidepressant effects in animals depression model by improving the activity serotonergic system (Perviz et al., 2016).
Mitragynine is Mitragyna speciosa alkaloid, whose antidepressant effects have been shown on TST and FST in mice, where it reduced immobility time (Idayu et al., 2011). Administration of punarnavine for 14 days at a dose of 20 and 40 mg kg−1 resulted in decrease corticosterone levels, MAO-A activity and immobility on FST in both unstressed and stressed mice (Dhingra and Valecha, 2014). Apart from this, piperine on FST and protopine on TST showed antidepressant effects which may be due serotonergic mechanism (Wattanathorn et al., 2008) and 5-HT inhibition respectively (Xu et al., 2016). The antidepressant potentials of alkaloids have been clearly demonstrated in various preclinical studies but more studies are required to further evaluate their efficacy, potency and safety.
Antidepressant effects of saponins and sapogenins
Saponins are secondary metabolites of plants and mostly found in glycosilated forms (Ayaz et al., 2016). Saponins contain one to three sugar chains which may be straight or branched and usually composed of d-glucose, d-galactose, d-glucuronic acid, l-rhamnose, l-arabinose, d -fucose or d-xylose (Vincken et al., 2007). Sapogenins are generally classified into triterpenoidal (with 4–5 rings skeleton) and steroidal (with 5–6 rings backbone) groups. The antidepressant effect of bacopaside I from Bacopa monniera in various animal models has reported (Liu et al., 2013). Ginsenosides from ginseng and intestinal metabolite of ginseng, 20(S)-protopanaxadiol demonstrated antidepressant effects by enhancing brain monoamine levels, HPA axis, BDNF signaling pathway and hippocampal neurogenesis (Xu et al., 2010). Glycyrrhizin is a triterpene saponin, exhibit antidepressant effect due to participation of α1 adrenoceptor and DA D2 receptor (Dhingra and Sharma, 2005). Hederagenin of Akebia quinata, showed antidepressant effect by HPA axis modulation (Jin et al., 2012). Sarsasapogenin from Anemarrhena asphodeloides possess antidepressant activity by preventing MAO-A and MAO-B activities and increasing NE and 5-HT levels of the hippocampus and hypothalamus (Ren et al., 2006).
Phyto-sterols in depression
The chemical structure of plant sterols, also called phytosterols is similar to the structure of cholesterol and mostly found in nuts, cereals, fruits and vegetables (Ayaz et al., 2017a; Shah et al., 2019) (Figure 6). β-sitosterol, stigmasterol fucosterol, and campesterol are among the different identified types of sterols. Sterols can cross blood brain barrier (BBB) to exert their effects on CNS due to their glycosylated forms and lipidic nature (Tabata et al., 2008). Trevisan et al. suggested that α-spinasterol exhibits antagonistic effects on transient potential receptors V1 (TRPV1). The activation of these receptors in various parts of brain may increase the release of glutamate, GABA, or other catecholamines (Martins et al., 2014), underlying the mechanism of anxiety and depression due to involvement of TRPV1. The antidepressant effect of α-spinasterol in male mice subjected to FST, was verified by Socała and Wlaź (2016). Socała and Wlaź further suggested that α-spinasterol may activate CB1 receptors which in turn activate TRPV1 receptors simultaneously to inhibit their anxiolytic effects (Socała and Wlaź, 2016).
One study reported that administration of fucosterol (20 and 30 mg kg−1) produced antidepressant effects in male mice FST and TST similar to that of fluoxetine at a dose of 20 mg kg−1 (Zhen et al., 2015). In mice brains, fucosterol prevented the reduction in the levels of NA, 5-HT and 5-HTIIA caused by stress of FST, suggesting that the antidepressant effects are due to increased monoamines and decreased metabolism rate of 5-HT. Administration of β-sitosterol (30 mg kg−1) produced antidepressant effects in male mice on FST and TST similar to that of fluoxetine at a dose of 20 mg kg−1 (García-Ríos et al., 2020). Another study reported the antidepressants potential of α- and β-amyrin (αβAMY) isolated from the stem resin of Protium heptaphyllum on FST. Administration of αβAMY via p.o route at a dose of 2.5 and 5 mg kg−1 decreased immobility time, as decreased by imipramine at 30 and 10 mg kg−1 (García-Ríos et al., 2020).
Clinical trials on polyphenols as potential anti-depressant agents
Number of clinical studies have reported that polyphenols rich foods or dietary polyphenols intake play a significant role in the prevention and treatment of depression (Godos et al., 2018). A recent clinical trial in elderly Japanese demonstrated that higher consumption of green tea is associated with lower prevalence of depression (Niu et al., 2009). The anti-depressive potential of saffron has been reported to be similar to that of synthetic antidepressant drugs like imipramine and fluoxetine, without side effects (Basti et al., 2007). It has been concluded in the meta-analysis review of clinical trials by Hausenblas that saffron supplementation can ameliorate symptoms of depression in adults having MDD (Hausenblas et al., 2013).
Conclusion and future perspectives
Medicinal plants and their metabolites are being used for the management of various mental disorders. The phytochemicals are widely available, more tolerable and have fewer side effects as compared to synthetic drugs. The phytochemicals with antidepressant activity include rosmanol, ursolic acid, oleanolic acid, linalool, carnosol, quercetin, fisetin, naringenin, baicalin, genistein, harmine, mitragynine, piperine, protopine, beta sitosterol, fucosterol, alpha spinnasterol, alpha amirine and beta amirine belonging to different chemical classes such as terpenes and terpenoids, saponins and sapogenins, sterols, alkaloids, polyphenols, amines and carbohydrates. Among them, the most studied phytochemicals in animal depression models are berberine, piperine, curcumin, naringenin, ascorbic acid, and ginsenosides. The antidepressant activity of these phytochemicals seems to be associated with various mechanisms that include activation of tyrosine hydroxylase enzymes, inhibition of alteration of MAO-A and MAO-B, alteration in brain monoamine levels and receptors, prevention of reactive oxygen species (ROS) and NO synthesis, involvement of D1, D2, 5-HT1A, 5-HT2A, GABAA receptors and α1, α2, β-adrenoceptors, CREB (cyclic adenosine monophosphate response element-binding protein), l-arginine-NO-cGMP pathway, and BDNF signaling pathway. All these mechanisms involves differentiation and inhibition of neuronal cell apoptosis and promotion of neuronal cell survival.
Numerous phytochemicals have been reported for their antidepressant activity but only few of them have subjected into clinical trials. Unfortunately, very few clinical trials have demonstrated antidepressant effects of phytochemicals including clinical study of curcumin in human and hence further randomized and placebo-controlled clinical trials are needed to confirm their antidepressant potential of these phytochemicals. Further research studies are also needed to investigate their antidepressant mechanisms and to develop cost effective formulations for the treatment of depression.
Author contributions
AK, RG, and MA conceptualized the project. AK, RG, JB, JŽ, MA, AN, SH, and SB collected data, analyzed, wrote the manuscript and prepared the figures. SA revised the manuscript including the clinical aspects, prepared Figures 1, 2, revised the table and the mechanism part. All authors read and approved the manuscript for publishing.
Acknowledgments
The authors wish to thank to University of Oradea, Oradea, Romania for financial support in publishing this paper. The authors would like to thank the Deanship of Scientific Research at Shaqra University for supporting this work.
Conflict of interest
The authors declare that the research was conducted in the absence of any commercial or financial relationships that could be construed as a potential conflict of interest.
Publisher’s note
All claims expressed in this article are solely those of the authors and do not necessarily represent those of their affiliated organizations, or those of the publisher, the editors and the reviewers. Any product that may be evaluated in this article, or claim that may be made by its manufacturer, is not guaranteed or endorsed by the publisher.
Abbreviations
5-HT, 5-hydroxytryptamine; BDNF, brain-derived neurotrophic factor; CUMS, chronic unpredictable mild stress; Cur, curcumin; DASS, Depression, Anxiety, Stress Scale; ER, endoplasmic reticulum; FDA, U.S. Food and Drug Administration; SERT, serotonin transporters; FST, forced swimming test; GANP, gallic acid loaded chitosan nanoparticles; MAO, monoamine oxidase; MDD, major depressive disorder; MEM, mitochondrial membrane; NET, norepinephrine reuptake transporters; NMDA, N-methyl D-aspartate; NO, nitric oxide; SERT, serotonin reuptake transporters; SLNs, solid lipid nanoparticles; SNP, single nucleotide polymorphism; SNRIs, Serotonin and norepinephrine reuptake inhibitors; SPT, Standard Penetration Test; SSRIs, Selective serotonin reuptake inhibitors; TCAs, tricyclic antidepressants; TrkB, tropomyosin kinase B receptor; TST, tail suspension test.
References
Abdelhalim, A., Karim, N., Chebib, M., Aburjai, T., Khan, I., Johnston, G. A., et al. (2015). Antidepressant, anxiolytic and antinociceptive activities of constituents from Rosmarinus officinalis. J. Pharm. Pharm. Sci. 18 (4), 448–459. doi:10.18433/j3pw38
Ahsan, H., Ayub, M., Irfan, H. M., Saleem, M., Anjum, I., Haider, I., et al. (2022). Tumor necrosis factor-alpha, prostaglandin-E2 and interleukin-1β targeted anti-arthritic potential of fluvoxamine: Drug repurposing. Environ. Sci. Pollut. Res., 1–12. doi:10.1007/s11356-022-23142-1
Al Mamari, H. H. (2021). “Phenolic compounds: Classification, chemistry, and updated techniques of analysis and synthesis,” in Phenolic compounds-chemistry, synthesis, diversity, non-conventional industrial, pharmaceutical and therapeutic applications. London, United Kingdom: IntechOpen.
Albayrak, Y., and Hashimoto, K. (2017). “Sigma-1 receptor agonists and their clinical implications in neuropsychiatric disorders,” in Sigma receptors: Their role in disease and as therapeutic targets. Editors S. B. Smith, and T.-P. Su (Cham: Springer International Publishing), 153–161.
Ali, B., Bashir, A., and Tanira, M. (1998). The effect of Rhazya stricta Decne, a traditional medicinal plant, on the forced swimming test in rats. Pharmacol. Biochem. Behav. 59 (2), 547–550. doi:10.1016/s0091-3057(97)00470-x
Amawi, H., Ashby, C. R., and Tiwari, A. K. (2017). Cancer chemoprevention through dietary flavonoids: what’s limiting? Chin. J. Cancer 36 (1), 50–13. doi:10.1186/s40880-017-0217-4
Anisman, H., Merali, Z., Poulter, M. O., and Hayley, S. (2005). Cytokines as a precipitant of depressive illness: Animal and human studies. Curr. Pharm. Des. 11 (8), 963–972. doi:10.2174/1381612053381701
Aragão, G., Carneiro, L., Junior, A., Vieira, L., Bandeira, P., Lemos, T., et al. (2006). A possible mechanism for anxiolytic and antidepressant effects of alpha-and beta-amyrin from Protium heptaphyllum (Aubl.) March. Pharmacol. Biochem. Behav. 85 (4), 827–834. doi:10.1016/j.pbb.2006.11.019
Asadi, S., Gholami, M. S., Siassi, F., Qorbani, M., and Sotoudeh, G. (2020). Beneficial effects of nano‐curcumin supplement on depression and anxiety in diabetic patients with peripheral neuropathy: A randomized, double‐blind, placebo‐controlled clinical trial. Phytother. Res. 34 (4), 896–903. doi:10.1002/ptr.6571
Ashraf, A., Mahmoud, P. A., Reda, H., Mansour, S., Helal, M. H., Michel, H. E., et al. (2019). Silymarin and silymarin nanoparticles guard against chronic unpredictable mild stress induced depressive-like behavior in mice: Involvement of neurogenesis and NLRP3 inflammasome. J. Psychopharmacol. 33 (5), 615–631. doi:10.1177/0269881119836221
Ayaz, M., Junaid, M., Ullah, F., Sadiq, A., Subhan, F., Khan, M. A., et al. (2016). Molecularly characterized solvent extracts and saponins from Polygonum hydropiper L. show high anti-angiogenic, anti-tumor, brine shrimp, and fibroblast NIH/3T3 cell line cytotoxicity. Front. Pharmacol. 7, 74. doi:10.3389/fphar.2016.00074
Ayaz, M., Junaid, M., Ullah, F., Subhan, F., Sadiq, A., Ali, G., et al. (2017a). Anti-Alzheimer’s studies on β-sitosterol isolated from Polygonum hydropiper L. Front. Pharmacol. 8, 697. doi:10.3389/fphar.2017.00697
Ayaz, M., Ovais, M., Ahmad, I., Sadiq, A., Khalil, A. T., and Ullah, F. (2020). “Biosynthesized metal nanoparticles as potential Alzheimer’s disease therapeutics,” in Metal nanoparticles for drug delivery and diagnostic applications. Netherlands: Elsevier, 31–42.
Ayaz, M., Sadiq, A., Junaid, M., Ullah, F., Ovais, M., Ullah, I., et al. (2019). Flavonoids as prospective neuroprotectants and their therapeutic propensity in aging associated neurological disorders. Front. Aging Neurosci. 11, 155. doi: doi:10.3389/fnagi.2019.00155
Ayaz, M., Subhan, F., Sadiq, A., Ullah, F., Ahmed, J., and Sewell, R. D. E. (2017b). Cellular efflux transporters and the potential role of natural products in combating efflux mediated drug resistance. Front. Biosci. 22 (4), 732–756. doi:10.2741/4513
Ayres, D. C., and Loike, J. D. (1990). Lignans: Chemical, biological and clinical properties. New York, NY: Cambridge University Press.
Baldwin, D., Rudge, S., and Thomas, S. (1995). Dysthymia. CNS Drugs 4 (6), 422–431. doi:10.2165/00023210-199504060-00005
Basti, A. A., Moshiri, E., Noorbala, A.-A., Jamshidi, A.-H., Abbasi, S. H., and Akhondzadeh, S. (2007). Comparison of petal of crocus sativus L. And fluoxetine in the treatment of depressed outpatients: A pilot double-blind randomized trial. Prog. Neuropsychopharmacol. Biol. Psychiatry 31 (2), 439–442. doi:10.1016/j.pnpbp.2006.11.010
Baum, S. S., Hill, R., and Rommelspacher, H. (1996). Harman-induced changes of extracellular concentrations of neurotransmitters in the nucleus accumbens of rats. Eur. J. Pharmacol. 314 (1-2), 75–82. doi:10.1016/s0014-2999(96)00543-2
Ben-Azu, B., Nwoke, E. E., Aderibigbe, A. O., Omogbiya, I. A., Ajayi, A. M., Olonode, E. T., et al. (2019). Possible neuroprotective mechanisms of action involved in the neurobehavioral property of naringin in mice. Biomed. Pharmacother. 109, 536–546. doi:10.1016/j.biopha.2018.10.055
Benmansour, S., Cecchi, M., Morilak, D. A., Gerhardt, G. A., Javors, M. A., Gould, G. G., et al. (1999). Effects of chronic antidepressant treatments on serotonin transporter function, density, and mRNA level. J. Neurosci. 19 (23), 10494–10501. doi:10.1523/jneurosci.19-23-10494.1999
Bhutada, P., Mundhada, Y., Bansod, K., Ubgade, A., Quazi, M., Umathe, S., et al. (2010). Reversal by quercetin of corticotrophin releasing factor induced anxiety-and depression-like effect in mice. Prog. Neuropsychopharmacol. Biol. Psychiatry 34 (6), 955–960. doi:10.1016/j.pnpbp.2010.04.025
Bishop, A., and Anderson, J. E. (2005). NO signaling in the CNS: From the physiological to the pathological. Toxicology 208 (2), 193–205. doi:10.1016/j.tox.2004.11.034
Borges Filho, C., Jesse, C. R., Donato, F., Del Fabbro, L., de Gomes, M. G., Goes, A. T. R., et al. (2016). Neurochemical factors associated with the antidepressant-like effect of flavonoid chrysin in chronically stressed mice. Eur. J. Pharmacol. 791, 284–296. doi:10.1016/j.ejphar.2016.09.005
Brimson, J. M., Akula, K. K., Abbas, H., Ferry, D. R., Kulkarni, S. K., Russell, S. T., et al. (2020a). Simple ammonium salts acting on sigma-1 receptors yield potential treatments for cancer and depression. Sci. Rep. 10 (1), 9251. doi:10.1038/s41598-020-65849-6
Brimson, J. M., Brimson, S., Chomchoei, C., and Tencomnao, T. (2020b). Using Sigma-ligands as part of a multi-receptor approach to target diseases of the brain. Expert Opin. Ther. Targets 24 (10), 1009–1028. doi:10.1080/14728222.2020.1805435
Brimson, J. M., Prasanth, M. I., Malar, D. S., Brimson, S., Thitilertdecha, P., and Tencomnao, T. (2021). Drugs that offer the potential to reduce hospitalization and mortality from SARS-CoV-2 infection: The possible role of the Sigma-1 receptor and autophagy. Expert Opin. Ther. Targets 25 (5), 435–449. doi:10.1080/14728222.2021.1952987
Brimson, J. M., Safrany, S. T., Qassam, H., and Tencomnao, T. (2018). Dipentylammonium binds to the sigma-1 receptor and protects against glutamate toxicity, attenuates dopamine toxicity and potentiates neurite outgrowth in various cultured cell lines. Neurotox. Res. 34 (2), 263–272. doi:10.1007/s12640-018-9883-5
Campbell, S., and Macqueen, G. (2004). The role of the hippocampus in the pathophysiology of major depression. J. Psychiatry Neurosci. 29 (6), 417–426.
Capra, J. C., Cunha, M. P., Machado, D. G., Zomkowski, A. D., Mendes, B. G., Santos, A. R. S., et al. (2010). Antidepressant-like effect of scopoletin, a coumarin isolated from polygala sabulosa (polygalaceae) in mice: Evidence for the involvement of monoaminergic systems. Eur. J. Pharmacol. 643 (2-3), 232–238. doi:10.1016/j.ejphar.2010.06.043
Carlsson, A. (1999). “The discovery of the SSRIs: A milestone in neuropsychopharmacology and rational drug design,” in Selective serotonin reuptake inhibitors: Past, present and future. Texas, United States: R.G. Landes Company Austin, 1–7.
Cassani, J., Dorantes-Barrón, A. M., Mayagoitia Novales, L., Alva Real, G., and Estrada-Reyes, R. (2014). Anti-depressant-like effect of kaempferitrin isolated from Justicia spicigera schltdl (Acanthaceae) in two behavior models in mice: Evidence for the involvement of the serotonergic system. Molecules 19 (12), 21442–21461. doi:10.3390/molecules191221442
Chen, B., Luo, M., Liang, J., Zhang, C., Gao, C., Wang, J., et al. (2018). Surface modification of PGP for a neutrophil–nanoparticle co-vehicle to enhance the anti-depressant effect of baicalein. Acta Pharm. Sin. B 8 (1), 64–73. doi:10.1016/j.apsb.2017.11.012
Chhillar, R., and Dhingra, D. (2013). Antidepressant‐like activity of gallic acid in mice subjected to unpredictable chronic mild stress. Fundam. Clin. Pharmacol. 27 (4), 409–418. doi:10.1111/j.1472-8206.2012.01040.x
Chittaranjan Patra, I. A., Ayaz, M., Khalil, A. T., Mukherjee, S., and Ovais, M. (2021). Biogenic nanoparticles for cancer theranostics. Netherlands: Elsevier.
Choi, K., Chun, J., Han, K., Park, S., Soh, H., Kim, J., et al. (2019). Risk of anxiety and depression in patients with inflammatory bowel disease: A nationwide, population-based study. J. Clin. Med. 8 (5), 654. doi:10.3390/jcm8050654
Coelho, V., Mazzardo-Martins, L., Martins, D. F., Santos, A. R. S., da Silva Brum, L. F., Picada, J. N., et al. (2013). Neurobehavioral and genotoxic evaluation of (−)-linalool in mice. J. Nat. Med. 67 (4), 876–880. doi:10.1007/s11418-013-0751-6
Cole, J., Costafreda, S. G., McGuffin, P., and Fu, C. H. (2011). Hippocampal atrophy in first episode depression: A meta-analysis of magnetic resonance imaging studies. J. Affect. Disord. 134 (1-3), 483–487. doi:10.1016/j.jad.2011.05.057
Colla, A. R., Pazini, F. L., Lieberknecht, V., Camargo, A., and Rodrigues, A. L. S. (2021). Ursolic acid abrogates depressive-like behavior and hippocampal pro-apoptotic imbalance induced by chronic unpredictable stress. Metab. Brain Dis. 36 (3), 437–446. doi:10.1007/s11011-020-00658-4
Colla, A. R., Rosa, J. M., Cunha, M. P., and Rodrigues, A. L. S. (2015). Anxiolytic-like effects of ursolic acid in mice. Eur. J. Pharmacol. 758, 171–176. doi:10.1016/j.ejphar.2015.03.077
De La Peña, J. B. I., Kim, C. A., Lee, H. L., Yoon, S. Y., Kim, H. J., Hong, E. Y., et al. (2014). Luteolin mediates the antidepressant-like effects of Cirsium japonicum in mice, possibly through modulation of the GABAA receptor. Arch. Pharm. Res. 37 (2), 263–269. doi:10.1007/s12272-013-0229-9
de Zwart, P. L., Jeronimus, B. F., and de Jonge, P. (2019). Empirical evidence for definitions of episode, remission, recovery, relapse and recurrence in depression: A systematic review. Epidemiol. Psychiatr. Sci. 28 (5), 544–562. doi:10.1017/s2045796018000227
Dhingra, D., and Sharma, A. (2005). Evaluation of antidepressant-like activity of glycyrrhizin in mice. Indian J. Pharmacol. 37 (6), 390. doi:10.4103/0253-7613.19077
Dhingra, D., and Valecha, R. (2014). Punarnavine, an alkaloid isolated from ethanolic extract of Boerhaavia diffusa Linn. reverses depression-like behaviour in mice subjected to chronic unpredictable mild stress. Indian J. Exp. Biol. 52 (8), 799–807.
Dhir, A. (2017). “Potential of polyphenols in the treatment of major depression,” in Neuroprotective effects of phytochemicals in neurological disorders, 265–282. doi:10.1002/9781119155195.ch12
Dimpfel, W. (2009). Rat electropharmacograms of the flavonoids rutin and quercetin in comparison to those of moclobemide and clinically used reference drugs suggest antidepressive and/or neuroprotective action. Phytomedicine 16 (4), 287–294. doi:10.1016/j.phymed.2009.02.005
do Amaral, J. F., Silva, M. I. G., de Aquino Neto, M. R., Moura, B. A., de Carvalho, A. M. R., Vasconcelos, P. F., et al. (2013). Antidepressant‐like effect of bis‐eugenol in the mice forced swimming test: Evidence for the involvement of the monoaminergic system. Fundam. Clin. Pharmacol. 27 (5), 471–482. doi:10.1111/j.1472-8206.2012.01058.x
Donato, F., de Gomes, M. G., Goes, A. T. R., Borges Filho, C., Del Fabbro, L., Antunes, M. S., et al. (2014). Hesperidin exerts antidepressant-like effects in acute and chronic treatments in mice: Possible role of l-arginine-NO-cGMP pathway and BDNF levels. Brain Res. Bull. 104, 19–26. doi:10.1016/j.brainresbull.2014.03.004
Dunn, A. J., Wang, J., and Ando, T. (1999). Effects of cytokines on cerebral neurotransmission. Comparison with the effects of stress. Adv. Exp. Med. Biol. 461, 117–127. doi:10.1007/978-0-585-37970-8_8
Dunn, A. J., and Wang, J. (1995). Cytokine effects on CNS biogenic amines. Neuroimmunomodulation 2 (6), 319–328. doi:10.1159/000097211
Edinoff, A. N., Akuly, H. A., Hanna, T. A., Ochoa, C. O., Patti, S. J., Ghaffar, Y. A., et al. (2021). Selective serotonin reuptake inhibitors and adverse effects: a narrative review. Neurology International 13 (3), 387–401.
Evans-Lacko, S., Aguilar-Gaxiola, S., Al-Hamzawi, A., Alonso, J., Benjet, C., Bruffaerts, R., et al. (2018). Socio-economic variations in the mental health treatment gap for people with anxiety, mood, and substance use disorders: Results from the WHO world mental health (WMH) surveys. Psychol. Med. 48 (9), 1560–1571. doi:10.1017/S0033291717003336
Fagius, J., Osterman, P. O., Sidén, A., Wiholm, B. E., and Siden, A. (1985). Guillain-Barré syndrome following zimeldine treatment. J. Neurol. Neurosurg. Psychiatry 48 (1), 65–69. doi:10.1136/jnnp.48.1.65
Farzin, D., and Mansouri, N. (2006). Antidepressant-like effect of harmane and other β-carbolines in the mouse forced swim test. Eur. Neuropsychopharmacol. 16 (5), 324–328. doi:10.1016/j.euroneuro.2005.08.005
Fortunato, J. J., Réus, G. Z., Kirsch, T. R., Stringari, R. B., Fries, G. R., Kapczinski, F., et al. (2010a). Chronic administration of harmine elicits antidepressant-like effects and increases BDNF levels in rat hippocampus. J. Neural Transm. 117 (10), 1131–1137. doi:10.1007/s00702-010-0451-2
Fortunato, J. J., Réus, G. Z., Kirsch, T. R., Stringari, R. B., Fries, G. R., Kapczinski, F., et al. (2010b). Effects of β-carboline harmine on behavioral and physiological parameters observed in the chronic mild stress model: Further evidence of antidepressant properties. Brain Res. Bull. 81 (4-5), 491–496. doi:10.1016/j.brainresbull.2009.09.008
Fournier, J. C., DeRubeis, R. J., Hollon, S. D., Dimidjian, S., Amsterdam, J. D., Shelton, R. C., et al. (2010). Antidepressant drug effects and depression severity: A patient-level meta-analysis. Jama 303 (1), 47–53. doi:10.1001/jama.2009.1943
Gadkari, P. V., and Balaraman, M. (2015). Catechins: Sources, extraction and encapsulation: A review. Food Bioprod. Process. 93, 122–138. doi:10.1016/j.fbp.2013.12.004
García-Ríos, R. I., Mora-Pérez, A., Ramos-Molina, A. R., and Soria-Fregozo, C. (2020). “Neuropharmacology of secondary metabolites from plants with anxiolytic and antidepressant properties,” in Behavioral pharmacology-from basic to clinical research. London United Kingdom: IntechOpen.
Garcia-Salas, P., Morales-Soto, A., Segura-Carretero, A., and Fernández-Gutiérrez, A. (2010). Phenolic-compound-extraction systems for fruit and vegetable samples. Molecules 15 (12), 8813–8826. doi:10.3390/molecules15128813
Gillman, P. K. (2007). Tricyclic antidepressant pharmacology and therapeutic drug interactions updated. Br. J. Pharmacol. 151 (6), 737–748. doi:10.1038/sj.bjp.0707253
God`os, J., Castellano, S., Ray, S., Grosso, G., and Galvano, F. (2018). Dietary polyphenol intake and depression: Results from the mediterranean healthy eating, lifestyle and aging (meal) study. Molecules 23 (5), 999. doi:10.3390/molecules23050999
Graham, J., Salimi-Khorshidi, G., Hagan, C., Walsh, N., Goodyer, I., Lennox, B., et al. (2013). Meta-analytic evidence for neuroimaging models of depression: State or trait? J. Affect. Disord. 151 (2), 423–431. doi:10.1016/j.jad.2013.07.002
Guzmán-Gutiérrez, S. L., Bonilla-Jaime, H., Gómez-Cansino, R., and Reyes-Chilpa, R. (2015). Linalool and β-pinene exert their antidepressant-like activity through the monoaminergic pathway. Life Sci. 128, 24–29. doi:10.1016/j.lfs.2015.02.021
Hamilton, J. P., Siemer, M., and Gotlib, I. H. (2008). Amygdala volume in major depressive disorder: A meta-analysis of magnetic resonance imaging studies. Mol. Psychiatry 13 (11), 993–1000. doi:10.1038/mp.2008.57
Haque, S., Md, S., Sahni, J. K., Ali, J., and Baboota, S. (2014). Development and evaluation of brain targeted intranasal alginate nanoparticles for treatment of depression. J. Psychiatr. Res. 48 (1), 1–12. doi:10.1016/j.jpsychires.2013.10.011
Hausenblas, H. A., Saha, D., Dubyak, P. J., and Anton, S. D. (2013). Saffron (crocus sativus L.) and major depressive disorder: A meta-analysis of randomized clinical trials. J. Integr. Med. 11 (6), 377–383. doi:10.3736/jintegrmed2013056
Hayashi, T. (2005). Understanding the role of sigma-1 receptors in psychotic depression. Psychiatr. Times 22 (11), 54.
Holzmann, I., da Silva, L. M., da Silva, J. A. C., Steimbach, V. M. B., and de Souza, M. M. (2015). Antidepressant-like effect of quercetin in bulbectomized mice and involvement of the antioxidant defenses, and the glutamatergic and oxidonitrergic pathways. Pharmacol. Biochem. Behav. 136, 55–63. doi:10.1016/j.pbb.2015.07.003
Hu, P., Ma, L., Wang, Y.-g., Ye, F., Wang, C., Zhou, W.-H., et al. (2017). Genistein, a dietary soy isoflavone, exerts antidepressant-like effects in mice: Involvement of serotonergic system. Neurochem. Int. 108, 426–435. doi:10.1016/j.neuint.2017.06.002
Hu, S., Sheng, W. S., Ehrlich, L. C., Peterson, P. K., and Chao, C. C. (2000). Cytokine effects on glutamate uptake by human astrocytes. Neuroimmunomodulation 7 (3), 153–159. doi:10.1159/000026433
Idayu, N. F., Hidayat, M. T., Moklas, M., Sharida, F., Raudzah, A. N., Shamima, A., et al. (2011). Antidepressant-like effect of mitragynine isolated from Mitragyna speciosa Korth in mice model of depression. Phytomedicine 18 (5), 402–407. doi:10.1016/j.phymed.2010.08.011
Institute of Health Metrics and Evaluation (2021). Global health data exchange [Online]. Available: http://ghdx.healthdata.org/gbd-results-tool?params=gbd-api-2019-permalink/d780dffbe8a381b25e1416884959e88b (Accessed 08 28, 2021).
Irie, Y. (2006). Effects of eugenol on the central nervous system: Its possible application to treatment of Alzheimer's disease, depression, and Parkinson's disease. Curr. Bioact. Compd. 2 (1), 57–66. doi:10.2174/1573407210602010057
Ishikawa, M., and Hashimoto, K. (2010). The role of sigma-1 receptors in the pathophysiology of neuropsychiatric diseases. J. Recept. Ligand Channel Res. 3, 25–36. doi:10.2147/JRLCR.S8453
Ito, K., Hirooka, Y., Matsukawa, R., Nakano, M., and Sunagawa, K. (2012). Decreased brain sigma-1 receptor contributes to the relationship between heart failure and depression. Cardiovasc. Res. 93 (1), 33–40. doi:10.1093/cvr/cvr255
Jakobsen, J. C., Katakam, K. K., Schou, A., Hellmuth, S. G., Stallknecht, S. E., Leth-Møller, K., et al. (2017). Selective serotonin reuptake inhibitors versus placebo in patients with major depressive disorder. A systematic review with meta-analysis and Trial Sequential Analysis. BMC Psychiatry 17 (1), 58. doi:10.1186/s12888-016-1173-2
Jiang, H., Wang, Z., Wang, Y., Xie, K., Zhang, Q., Luan, Q., et al. (2013). Antidepressant-like effects of curcumin in chronic mild stress of rats: Involvement of its anti-inflammatory action. Prog. Neuropsychopharmacol. Biol. Psychiatry 47, 33–39. doi:10.1016/j.pnpbp.2013.07.009
Jiang, M.-L., Zhang, Z.-X., Li, Y.-Z., Wang, X.-H., Yan, W., and Gong, G.-Q. (2015). Antidepressant-like effect of evodiamine on chronic unpredictable mild stress rats. Neurosci. Lett. 588, 154–158. doi:10.1016/j.neulet.2014.12.038
Jin, Z.-L., Gao, N., Zhou, D., Chi, M.-G., Yang, X.-M., and Xu, J.-P. (2012). The extracts of fructus akebiae, a preparation containing 90% of the active ingredient hederagenin: Serotonin, norepinephrine and dopamine reuptake inhibitor. Pharmacol. Biochem. Behav. 100 (3), 431–439. doi:10.1016/j.pbb.2011.10.001
Kendler, K. S., Neale, M. C., Kessler, R. C., Heath, A. C., and Eaves, L. J. (1993). The lifetime history of major depression in women: Reliability of diagnosis and heritability. Arch. Gen. Psychiatry 50 (11), 863–870. doi:10.1001/archpsyc.1993.01820230054003
Khalil, A. T., Ayaz, M., Ovais, M., Wadood, A., Ali, M., Shinwari, Z. K., et al. (2018). In vitro cholinesterase enzymes inhibitory potential and in silico molecular docking studies of biogenic metal oxides nanoparticles. Inorg. Nano-Metal Chem. 48 (9), 441–448. doi:10.1080/24701556.2019.1569686
Khanbabaee, K., and Van Ree, T. (2001). Tannins: Classification and definition. Nat. Prod. Rep. 18 (6), 641–649. doi:10.1039/b101061l
Kishimoto, A., Todani, A., Miura, J., Kitagaki, T., and Hashimoto, K. (2010). The opposite effects of fluvoxamine and sertraline in the treatment of psychotic major depression: A case report. Ann. Gen. Psychiatry 9 (1), 23–3. doi:10.1186/1744-859X-9-23
Köhler, O., Benros, M. E., Nordentoft, M., Farkouh, M. E., Iyengar, R. L., Mors, O., et al. (2014). Effect of anti-inflammatory treatment on depression, depressive symptoms, and adverse effects: A systematic review and meta-analysis of randomized clinical trials. JAMA psychiatry 71 (12), 1381–1391. doi:10.1001/jamapsychiatry.2014.1611
Krishnadas, R., and Cavanagh, J. (2012). Depression: An inflammatory illness? J. Neurol. Neurosurg. Psychiatry 83 (5), 495–502. doi:10.1136/jnnp-2011-301779
Kumar, N., and Goel, N. (2019). Phenolic acids: Natural versatile molecules with promising therapeutic applications. Biotechnol. Rep. 24, e00370. doi:10.1016/j.btre.2019.e00370
Kumar, S., and Pandey, A. K. (2013). Chemistry and biological activities of flavonoids: An overview. ScientificWorldJournal. 2013, 162750. doi:10.1155/2013/162750
Lechtenberg, M., Schepmann, D., Niehues, M., Hellenbrand, N., Wünsch, B., and Hensel, A. (2008). Quality and functionality of saffron: Quality control, species assortment and affinity of extract and isolated saffron compounds to NMDA and sigma1 (sigma-1) receptors. Planta Med. 74 (07), 764–772. doi:10.1055/s-2008-1074535
Lee, B., Sur, B., Park, J., Kim, S.-H., Kwon, S., Yeom, M., et al. (2013). Chronic administration of baicalein decreases depression-like behavior induced by repeated restraint stress in rats. Korean J. Physiol. Pharmacol. 17 (5), 393–403. doi:10.4196/kjpp.2013.17.5.393
Lee, B., Sur, B., Yeom, M., Shim, I., Lee, H., and Hahm, D.-H. (2012). Effect of berberine on depression-and anxiety-like behaviors and activation of the noradrenergic system induced by development of morphine dependence in rats. Korean J. Physiol. Pharmacol. 16 (6), 379–386. doi:10.4196/kjpp.2012.16.6.379
Lesch, K. P., Bengel, D., Heils, A., Sabol, S. Z., Greenberg, B. D., Petri, S., et al. (1996). Association of anxiety-related traits with a polymorphism in the serotonin transporter gene regulatory region. Science 274 (5292), 1527–1531. doi:10.1126/science.274.5292.1527
Li, L.-F., Yang, J., Ma, S.-P., and Qu, R. (2013). Magnolol treatment reversed the glial pathology in an unpredictable chronic mild stress-induced rat model of depression. Eur. J. Pharmacol. 711 (1-3), 42–49. doi:10.1016/j.ejphar.2013.04.008
Liu, Q., He, H., Yang, J., Feng, X., Zhao, F., and Lyu, J. (2020). Changes in the global burden of depression from 1990 to 2017: Findings from the global burden of disease study. J. Psychiatr. Res. 126, 134–140. doi:10.1016/j.jpsychires.2019.08.002
Liu, X., Liu, F., Yue, R., Li, Y., Zhang, J., Wang, S., et al. (2013). The antidepressant-like effect of bacopaside I: Possible involvement of the oxidative stress system and the noradrenergic system. Pharmacol. Biochem. Behav. 110, 224–230. doi:10.1016/j.pbb.2013.07.007
Lloyd, A. J., Ferrier, I. N., Barber, R., Gholkar, A., Young, A. H., and O'Brien, J. T. (2004). Hippocampal volume change in depression: Late-and early-onset illness compared. Br. J. Psychiatry 184 (6), 488–495. doi:10.1192/bjp.184.6.488
Lohoff, F. W., and Ferraro, T. N. (2010). Pharmacogenetic considerations in the treatment of psychiatric disorders. Expert Opin. Pharmacother. 11 (3), 423–439. doi:10.1517/14656560903508762
Lopes, I. S., Oliveira, I. C. M., Capibaribe, V. C. C., Valentim, J. T., da Silva, D. M. A., de Souza, A. G., et al. (2018). Riparin II ameliorates corticosterone-induced depressive-like behavior in mice: Role of antioxidant and neurotrophic mechanisms. Neurochem. Int. 120, 33–42. doi:10.1016/j.neuint.2018.07.007
Lotrich, F. E., Ferrell, R. E., Rabinovitz, M., and Pollock, B. G. (2009). Risk for depression during interferon-alpha treatment is affected by the serotonin transporter polymorphism. Biol. Psychiatry 65 (4), 344–348. doi:10.1016/j.biopsych.2008.08.009
Luo, L., and Tan, R.-X. (2001). Fluoxetine inhibits dendrite atrophy of hippocampal neurons by decreasing nitric oxide synthase expression in rat depression model. Acta Pharmacol. Sin. 22 (10), 865–870.
Machado, D. G., Cunha, M. P., Neis, V. B., Balen, G. O., Colla, A., Bettio, L. E., et al. (2013). Antidepressant-like effects of fractions, essential oil, carnosol and betulinic acid isolated from Rosmarinus officinalis L. Food Chem. 136 (2), 999–1005. doi:10.1016/j.foodchem.2012.09.028
Machado, D., Neis, V., Balen, G., Colla, A., Cunha, M., Dalmarco, J., et al. (2012). Antidepressant-like effect of ursolic acid isolated from rosmarinus officinalis L. In mice: Evidence for the involvement of the dopaminergic system. Pharmacol. Biochem. Behav. 103 (2), 204–211. doi:10.1016/j.pbb.2012.08.016
Martínez-Vázquez, M., Estrada-Reyes, R., Escalona, A. A., Velázquez, I. L., Martínez-Mota, L., Moreno, J., et al. (2012). Antidepressant-like effects of an alkaloid extract of the aerial parts of Annona cherimolia in mice. J. Ethnopharmacol. 139 (1), 164–170. doi:10.1016/j.jep.2011.10.033
Martins, J. L. R., Rodrigues, O. R. L., da Silva, D. M., Galdino, P. M., de Paula, J. R., Romão, W., et al. (2014). Mechanisms involved in the gastroprotective activity of Celtis iguanaea (Jacq.) Sargent on gastric lesions in mice. J. Ethnopharmacol. 155 (3), 1616–1624. doi:10.1016/j.jep.2014.08.006
Mattioli, R., Francioso, A., Mosca, L., and Silva, P. (2020). Anthocyanins: A comprehensive review of their chemical properties and health effects on cardiovascular and neurodegenerative diseases. Molecules 25 (17), 3809. doi:10.3390/molecules25173809
Maurice, T., and Lockhart, B. P. (1997). Neuroprotective and anti-amnesic potentials of sigma (sigma) receptor ligands. Prog. Neuropsychopharmacol. Biol. Psychiatry 21 (1), 69–102. doi:10.1016/s0278-5846(96)00160-1
McGue, M., and Christensen, K. (2003). The heritability of depression symptoms in elderly Danish twins: Occasion-specific versus general effects. Behav. Genet. 33 (2), 83–93. doi:10.1023/a:1022545600034
Mir, N. T., Saleem, U., Anwar, F., Ahmad, B., Ullah, I., Hira, S., et al. (2019). Lawsonia Inermis markedly improves cognitive functions in animal models and modulate oxidative stress markers in the brain. Medicina 55 (5), 192. doi:10.3390/medicina55050192
Moretti, M., Budni, J., Freitas, A. E., Rosa, P. B., and Rodrigues, A. L. S. (2014). Antidepressant-like effect of ascorbic acid is associated with the modulation of mammalian target of rapamycin pathway. J. Psychiatr. Res. 48 (1), 16–24. doi:10.1016/j.jpsychires.2013.10.014
Morón, J. A., Zakharova, I., Ferrer, J. V., Merrill, G. A., Hope, B., Lafer, E. M., et al. (2003). Mitogen-activated protein kinase regulates dopamine transporter surface expression and dopamine transport capacity. J. Neurosci. 23 (24), 8480–8488. doi:10.1523/jneurosci.23-24-08480.2003
Muhammad, I., Luo, W., Shoaib, R. M., Li, G.-l., ul Hassan, S. S., Yang, Z.-h., et al. (2021a). Guaiane-type sesquiterpenoids from Cinnamomum migao HW Li: And their anti-inflammatory activities. Phytochemistry 190, 112850. doi:10.1016/j.phytochem.2021.112850
Muhammad, I., ul Hassan, S. S., Cheung, S., Li, X., Wang, R., Zhang, W.-D., et al. (2021b). Phytochemical study of Ligularia subspicata and valuation of its anti-inflammatory activity. Fitoterapia 148, 104800. doi:10.1016/j.fitote.2020.104800
Muhammad, I., Wang, R.-B., Xiao, Y., Xie, Y.-G., Wu, G., Qian, X.-P., et al. (2021c). Chemical constituents of parasenecio quinquelobus. Chem. Nat. Compd. 57 (1), 190–193. doi:10.1007/s10600-021-03316-y
Müller, W. E., Siebert, B., Holoubek, G., and Gentsch, C. (2004). Neuropharmacology of the anxiolytic drug opipramol, a sigma site ligand. Pharmacopsychiatry 37 (3), 189–197. doi:10.1055/s-2004-832677
Musselman, D. L., Lawson, D. H., Gumnick, J. F., Manatunga, A. K., Penna, S., Goodkin, R. S., et al. (2001). Paroxetine for the prevention of depression induced by high-dose interferon alfa. N. Engl. J. Med. 344 (13), 961–966. doi:10.1056/NEJM200103293441303
Nabavi, S. F., Habtemariam, S., Di Lorenzo, A., Sureda, A., Khanjani, S., Nabavi, S. M., et al. (2016). Post-stroke depression modulation and in vivo antioxidant activity of gallic acid and its synthetic derivatives in a murine model system. Nutrients 8 (5), 248. doi:10.3390/nu8050248
Nagpal, K., Singh, S. K., and Mishra, D. N. (2012). Nanoparticle mediated brain targeted delivery of gallic acid: In vivo behavioral and biochemical studies for improved antioxidant and antidepressant-like activity. Drug Deliv. 19 (8), 378–391. doi:10.3109/10717544.2012.738437
Narita, N., Hashimoto, K., Tomitaka, S.-i., and Minabe, Y. (1996). Interactions of selective serotonin reuptake inhibitors with subtypes of σ receptors in rat brain. Eur. J. Pharmacol. 307 (1), 117–119. doi:10.1016/0014-2999(96)00254-3
Nasar, M. Q., Shah, M., Khalil, A. T., Kakar, M. Q., Ayaz, M., Dablool, A. S., et al. (2022). Ephedra intermedia mediated synthesis of biogenic silver nanoparticles and their antimicrobial, cytotoxic and hemocompatability evaluations. Inorg. Chem. Commun. 137, 109252. doi:10.1016/j.inoche.2022.109252
Nazzaro, F., Fratianni, F., De Feo, V., Battistelli, A., Da Cruz, A. G., and Coppola, R. (2020). “Polyphenols, the new frontiers of prebiotics,” in Advances in food and nutrition research. United States: Elsevier, 35–89.
Niu, K., Hozawa, A., Kuriyama, S., Ebihara, S., Guo, H., Nakaya, N., et al. (2009). Green tea consumption is associated with depressive symptoms in the elderly. Am. J. Clin. Nutr. 90 (6), 1615–1622. doi:10.3945/ajcn.2009.28216
Nonaka, G.-I., Miwa, N., and Nishioka, I. (1982). Stilbene glycoside gallates and proanthocyanidins from Polygonum multiflorum. Phytochemistry 21 (2), 429–432. doi:10.1016/s0031-9422(00)95282-8
Ofosu, F. K., Daliri, E. B.-M., Elahi, F., Chelliah, R., Lee, B.-H., and Oh, D.-H. (2020). New insights on the use of polyphenols as natural preservatives and their emerging safety concerns. Front. Sustain. Food Syst. 4, 525810. doi:10.3389/fsufs.2020.525810
Otte, C., Gold, S. M., Penninx, B. W., Pariante, C. M., Etkin, A., Fava, M., et al. (2016). Major depressive disorder. Nat. Rev. Dis. Prim. 2 (1), 16065. doi:10.1038/nrdp.2016.65
Outhred, T., Hawkshead, B. E., Wager, T. D., Das, P., Malhi, G. S., and Kemp, A. H. (2013). Acute neural effects of selective serotonin reuptake inhibitors versus noradrenaline reuptake inhibitors on emotion processing: Implications for differential treatment efficacy. Neurosci. Biobehav. Rev. 37 (8), 1786–1800. doi:10.1016/j.neubiorev.2013.07.010
Ovais, M., Ahmad, I., Khalil, A. T., Mukherjee, S., Javed, R., Ayaz, M., et al. (2018a). Wound healing applications of biogenic colloidal silver and gold nanoparticles: Recent trends and future prospects. Appl. Microbiol. Biotechnol. 102, 4305–4318. doi:10.1007/s00253-018-8939-z
Ovais, M., Ayaz, M., Khalil, A. T., Shah, S. A., Jan, M. S., Raza, A., et al. (2018b). HPLC-DAD finger printing, antioxidant, cholinesterase, and α-glucosidase inhibitory potentials of a novel plant Olax nana. BMC Complement. Altern. Med. 18 (1), 1–13. doi:10.1186/s12906-017-2057-9
Ovais, M., Zia, N., Ahmad, I., Khalil, A. T., Raza, A., Ayaz, M., et al. (2018c). Phyto-therapeutic and nanomedicinal approaches to cure Alzheimer's disease: Present status and future opportunities. Front. Aging Neurosci. 10, 284. doi:10.3389/fnagi.2018.00284
Ovais, M., Zia, N., Khalil, A. T., Ayaz, M., Khalil, A., and Ahmad, I. (2019). Nanoantibiotics: Recent developments and future prospects. Front. Clin. Drug Res. Anti. Infect. 5, 158.
Pande, A. C., Genève, J., Scherrer, B., Smith, F., Leadbetter, R. A., and De Meynard, C. (1999). A placebo-controlled trial of igmesine in the treatment of major depression. Eur. Neuropsychopharmacol. 9, 138. doi:10.1016/s0924-977x(99)80011-x
Pathak, L., Agrawal, Y., and Dhir, A. (2013). Natural polyphenols in the management of major depression. Expert Opin. Investig. Drugs 22 (7), 863–880. doi:10.1517/13543784.2013.794783
Perviz, S., Khan, H., and Pervaiz, A. (2016). Plant alkaloids as an emerging therapeutic alternative for the treatment of depression. Front. Pharmacol. 7, 28. doi:10.3389/fphar.2016.00028
Prasanth, M. I., Malar, D., Tencomnao, T., and Brimson, J. (2021). The emerging role of the sigma-1 receptor in autophagy: Hand-in-hand targets for the treatment of Alzheimer's. Expert Opin. Ther. Targets 25 (6), 401–414. doi:10.1080/14728222.2021.1939681
Qasim Nasar, M., Zohra, T., Khalil, A. T., Saqib, S., Ayaz, M., Ahmad, A., et al. (2019). Seripheidium quettense mediated green synthesis of biogenic silver nanoparticles and their theranostic applications. Green Chem. Lett. Rev. 12 (3), 310–322. doi:10.1080/17518253.2019.1643929
Qiu, F.-M., Zhong, X.-M., Mao, Q.-Q., and Huang, Z. (2013). Antidepressant-like effects of paeoniflorin on the behavioural, biochemical, and neurochemical patterns of rats exposed to chronic unpredictable stress. Neurosci. Lett. 541, 209–213. doi:10.1016/j.neulet.2013.02.029
Ren, L.-X., Luo, Y.-F., Li, X., Zuo, D.-Y., and Wu, Y.-L. (2006). Antidepressant-like effects of sarsasapogenin from Anemarrhena asphodeloides B UNGE (liliaceae). Biol. Pharm. Bull. 29 (11), 2304–2306. doi:10.1248/bpb.29.2304
Ren, Z., Yan, P., Zhu, L., Yang, H., Zhao, Y., Kirby, B. P., et al. (2018). Dihydromyricetin exerts a rapid antidepressant-like effect in association with enhancement of BDNF expression and inhibition of neuroinflammation. Psychopharmacology 235 (1), 233–244. doi:10.1007/s00213-017-4761-z
Roman, F. J., Pascaud, X., Martin, B., Vauche, D., and Junien, J. L. (1990). JO 1784, a potent and selective ligand for rat and mouse brain σ‐sites. J. Pharm. Pharmacol. 42 (6), 439–440. doi:10.1111/j.2042-7158.1990.tb06588.x
Ruhé, H. G., Mason, N. S., and Schene, A. H. (2007). Mood is indirectly related to serotonin, norepinephrine and dopamine levels in humans: A meta-analysis of monoamine depletion studies. Mol. Psychiatry 12 (4), 331–359. doi:10.1038/sj.mp.4001949
Sabino, V., Cottone, P., Parylak, S. L., Steardo, L., and Zorrilla, E. P. (2009). Sigma-1 receptor knockout mice display a depressive-like phenotype. Behav. Brain Res. 198 (2), 472–476. doi:10.1016/j.bbr.2008.11.036
Sani, A., Hassan, D., Khalil, A. T., Mughal, A., El-Mallul, A., Ayaz, M., et al. (2021). Floral extracts-mediated green synthesis of NiO nanoparticles and their diverse pharmacological evaluations. J. Biomol. Struct. Dyn. 39 (11), 4133–4147. doi:10.1080/07391102.2020.1775120
Santarelli, L., Saxe, M., Gross, C., Surget, A., Battaglia, F., Dulawa, S., et al. (2003). Requirement of hippocampal neurogenesis for the behavioral effects of antidepressants. science 301 (5634), 805–809. doi:10.1126/science.1083328
Schildkraut, J. J. (1995). The catecholamine hypothesis of affective disorders: A review of supporting evidence. J. Neuropsychiatry Clin. Neurosci. 7 (4), 524–533. doi:10.1176/jnp.7.4.524
Schumacher, J., Jamra, R. A., Becker, T., Ohlraun, S., Klopp, N., Binder, E. B., et al. (2005). Evidence for a relationship between genetic variants at the brain-derived neurotrophic factor (BDNF) locus and major depression. Biol. Psychiatry 58 (4), 307–314. doi:10.1016/j.biopsych.2005.04.006
Sha, S., Hong, J., Qu, W.-J., Lu, Z.-H., Li, L., Yu, W.-F., et al. (2015). Sex-related neurogenesis decrease in hippocampal dentate gyrus with depressive-like behaviors in sigma-1 receptor knockout mice. Eur. Neuropsychopharmacol. 25 (8), 1275–1286. doi:10.1016/j.euroneuro.2015.04.021
Shah, S. M., Ullah, F., Ayaz, M., Sadiq, A., Hussain, S., Shah, S. A. A., et al. (2019). β-Sitosterol from ifloga spicata (forssk.) sch. Bip. As potential anti-leishmanial agent against leishmania tropica: Docking and molecular insights. Steroids 148, 56–62. doi:10.1016/j.steroids.2019.05.001
Shahidi, F., and Ambigaipalan, P. (2015). Phenolics and polyphenolics in foods, beverages and spices: Antioxidant activity and health effects–A review. J. Funct. Foods 18, 820–897. doi:10.1016/j.jff.2015.06.018
Sheline, Y. I., Wang, P. W., Gado, M. H., Csernansky, J. G., and Vannier, M. W. (1996). Hippocampal atrophy in recurrent major depression. Proc. Natl. Acad. Sci. U. S. A. 93 (9), 3908–3913. doi:10.1073/pnas.93.9.3908
Shen, T., Wang, X.-N., and Lou, H.-X. (2009). Natural stilbenes: An overview. Nat. Prod. Rep. 26 (7), 916–935. doi:10.1039/b905960a
Shorey, S., Ng, E. D., and Wong, C. H. (2022). Global prevalence of depression and elevated depressive symptoms among adolescents: A systematic review and meta‐analysis. Br. J. Clin. Psychol. 61 (2), 287–305. doi:10.1111/bjc.12333
Si, H., and Liu, D. (2008). Genistein, a soy phytoestrogen, upregulates the expression of human endothelial nitric oxide synthase and lowers blood pressure in spontaneously hypertensive rats. J. Nutr. 138 (2), 297–304. doi:10.1093/jn/138.2.297
Siesser, W. B., Sachs, B. D., Ramsey, A. J., Sotnikova, T. D., Beaulieu, J. M., Zhang, X., et al. (2013). Chronic SSRI treatment exacerbates serotonin deficiency in humanized Tph2 mutant mice. ACS Chem. Neurosci. 4 (1), 84–88. doi:10.1021/cn300127h
Singh, V., Chauhan, G., and Shri, R. (2021). Anti-depressant like effects of quercetin 4'-O-glucoside from Allium cepa via regulation of brain oxidative stress and monoamine levels in mice subjected to unpredictable chronic mild stress. Nutr. Neurosci. 24 (1), 35–44. doi:10.1080/1028415X.2019.1587247
Singla, R. K., Dubey, A. K., Garg, A., Sharma, R. K., Fiorino, M., Ameen, S. M., et al. (2019). Natural polyphenols: Chemical classification, definition of classes, subcategories, and structures. Journal of AOAC International 102 (5), 1397–1400. Oxford University Press.
Skuza, G., and Rogóż, Z. (2009). Antidepressant-like effect of PRE-084, a selective sigma1 receptor agonist, in Albino Swiss and C57BL/6J mice. Pharmacol. Rep. 61 (6), 1179–1183. doi:10.1016/s1734-1140(09)70181-1
Smith, T. T., Levin, M. E., Schassburger, R. L., Buffalari, D. M., Sved, A. F., and Donny, E. C. (2013). Gradual and immediate nicotine reduction result in similar low-dose nicotine self-administration. Nicotine Tob. Res. 15 (11), 1918–1925. doi:10.1093/ntr/ntt082
Socała, K., and Wlaź, P. (2016). Evaluation of the antidepressant-and anxiolytic-like activity of α-spinasterol, a plant derivative with TRPV1 antagonistic effects, in mice. Behav. Brain Res. 303, 19–25. doi:10.1016/j.bbr.2016.01.048
Sockalingam, S., Links, P., and Abbey, S. (2011). Suicide risk in hepatitis C and during interferon-alpha therapy: A review and clinical update. J. Viral Hepat. 18 (3), 153–160. doi:10.1111/j.1365-2893.2010.01393.x
Spinks, D., and Spinks, G. (2002). Serotonin reuptake inhibition: An update on current research strategies. Curr. Med. Chem. 9 (8), 799–810. doi:10.2174/0929867024606795
Spruce, B. a., Campbell, L. a., McTavish, N., Cooper, M. a., Appleyard, M. V. L., O'Neill, M., et al. (2004). Small molecule antagonists of the sigma-1 receptor cause selective release of the death program in tumor and self-reliant cells and inhibit tumor growth in vitro and in vivo. Cancer Res. 64 (14), 4875–4886. doi:10.1158/0008-5472.CAN-03-3180
Stone, D. M., Simon, T. R., Fowler, K. A., Kegler, S. R., Yuan, K., Holland, K. M., et al. (2018). Vital signs: Trends in state suicide rates - United States, 1999-2016 and circumstances contributing to suicide - 27 states, 2015. MMWR. Morb. Mortal. Wkly. Rep. 67 (22), 617–624. doi:10.15585/mmwr.mm6722a1
Surtees, P. G., Wainwright, N. W., Willis-Owen, S. A., Sandhu, M. S., Luben, R., Day, N. E., et al. (2007). No association between the BDNF Val66Met polymorphism and mood status in a non-clinical community sample of 7389 older adults. J. Psychiatr. Res. 41 (5), 404–409. doi:10.1016/j.jpsychires.2006.01.004
Tabata, R., Wilson, J., Ly, P., Zwiegers, P., Kwok, D., Van Kampen, J., et al. (2008). Chronic exposure to dietary sterol glucosides is neurotoxic to motor neurons and induces an ALS–PDC phenotype. Neuromolecular Med. 10 (1), 24–39. doi:10.1007/s12017-007-8020-z
Tong-Un, T., Wannanon, P., Wattanathorn, J., and Phachonpai, W. (2010). Quercetin liposomes via nasal administration reduce anxiety and depression-like behaviors and enhance cognitive performances in rats. Am. J. Pharmacol. Toxicol. 5 (2), 80–88. doi:10.3844/ajptsp.2010.80.88
Trevino, A. E., Sinnott-Armstrong, N., Andersen, J., Yoon, S.-J., Huber, N., Pritchard, J. K., et al. (2020). Chromatin accessibility dynamics in a model of human forebrain development. science 367 (6476), eaay1645. doi:10.1126/science.aay1645
Truzzi, F., Tibaldi, C., Zhang, Y., Dinelli, G., and D Amen, E. (2021). An overview on dietary polyphenols and their biopharmaceutical classification system (BCS). Int. J. Mol. Sci. 22 (11), 5514. doi:10.3390/ijms22115514
Tsai, T.-H. (2005). Concurrent measurement of unbound genistein in the blood, brain and bile of anesthetized rats using microdialysis and its pharmacokinetic application. J. Chromatogr. A 1073 (1-2), 317–322. doi:10.1016/j.chroma.2004.10.048
Urani, A., Roman, F. J., Phan, V.-L., Su, T.-P., and Maurice, T. (2001). The antidepressant-like effect induced by sigma(1)-receptor agonists and neuroactive steroids in mice submitted to the forced swimming test. J. Pharmacol. Exp. Ther. 298 (3), 1269–1279.
Urani, A., Romieu, P., Roman, F. J., Yamada, K., Noda, Y., Kamei, H., et al. (2004). Enhanced antidepressant efficacy of sigma1 receptor agonists in rats after chronic intracerebroventricular infusion of beta-amyloid-(1-40) protein. Eur. J. Pharmacol. 486 (2), 151–161. doi:10.1016/j.ejphar.2003.12.018
Vincken, J.-P., Heng, L., de Groot, A., and Gruppen, H. (2007). Saponins, classification and occurrence in the plant kingdom. Phytochemistry 68 (3), 275–297. doi:10.1016/j.phytochem.2006.10.008
Volz, H. P., and Stoll, K. D. (2004). Clinical trials with sigma ligands. Pharmacopsychiatry 37 (3), 214–220. doi:10.1055/s-2004-832680
Wang, Y., Wang, B., Lu, J., Shi, H., Gong, S., Wang, Y., et al. (2017). Fisetin provides antidepressant effects by activating the tropomyosin receptor kinase B signal pathway in mice. J. Neurochem. 143 (5), 561–568. doi:10.1111/jnc.14226
Wattanathorn, J., Chonpathompikunlert, P., Muchimapura, S., Priprem, A., and Tankamnerdthai, O. (2008). Piperine, the potential functional food for mood and cognitive disorders. Food Chem. Toxicol. 46 (9), 3106–3110. doi:10.1016/j.fct.2008.06.014
Wong, D. T., Bymaster, F. P., and Engleman, E. A. (1995). Prozac (fluoxetine, lilly 110140), the first selective serotonin uptake inhibitor and an antidepressant drug: Twenty years since its first publication. Life Sci. 57 (5), 411–441. doi:10.1016/0024-3205(95)00209-o
World Health Organization (2021). Depression [online]. WHO. Available: https://www.who.int/news-room/fact-sheets/detail/depression (Accessed 09 27, 2021).
Wu, H.-Q., Rassoulpour, A., and Schwarcz, R. (2007). Kynurenic acid leads, dopamine follows: A new case of volume transmission in the brain? J. Neural Transm. 114 (1), 33–41. doi:10.1007/s00702-006-0562-y
Xiong, Z., Jiang, B., Wu, P.-F., Tian, J., Shi, L.-L., Gu, J., et al. (2011). Antidepressant effects of a plant-derived flavonoid baicalein involving extracellular signal-regulated kinases cascade. Biol. Pharm. Bull. 34 (2), 253–259. doi:10.1248/bpb.34.253
Xu, C., Teng, J., Chen, W., Ge, Q., Yang, Z., Yu, C., et al. (2010). 20 (S)-protopanaxadiol, an active ginseng metabolite, exhibits strong antidepressant-like effects in animal tests. Prog. Neuropsychopharmacol. Biol. Psychiatry 34 (8), 1402–1411. doi:10.1016/j.pnpbp.2010.07.010
Xu, J., Tao, J., and Wang, J. (2020). Design and application in delivery system of intranasal antidepressants. Front. Bioeng. Biotechnol. 8, 626882. doi:10.3389/fbioe.2020.626882
Xu, L.-F., Chu, W.-J., Qing, X.-Y., Li, S., Wang, X.-S., Qing, G.-W., et al. (2006). Protopine inhibits serotonin transporter and noradrenaline transporter and has the antidepressant-like effect in mice models. neuropharmacology 50 (8), 934–940. doi:10.1016/j.neuropharm.2006.01.003
Xu, Y., Zhang, C., Wu, F., Xu, X., Wang, G., Lin, M., et al. (2016). Piperine potentiates the effects of trans-resveratrol on stress-induced depressive-like behavior: Involvement of monoaminergic system and cAMP-dependent pathway. Metab. Brain Dis. 31 (4), 837–848. doi:10.1007/s11011-016-9809-y
Yi, L.-T., Liu, B.-B., Li, J., Luo, L., Liu, Q., Geng, D., et al. (2014). BDNF signaling is necessary for the antidepressant-like effect of naringenin. Prog. Neuropsychopharmacol. Biol. Psychiatry 48, 135–141. doi:10.1016/j.pnpbp.2013.10.002
Zeni, A. L. B., Zomkowski, A. D. E., Dal-Cim, T., Maraschin, M., Rodrigues, A. L. S., and Tasca, C. I. (2011). Antidepressant-like and neuroprotective effects of aloysia gratissima: Investigation of involvement of L-arginine-nitric oxide-cyclic guanosine monophosphate pathway. J. Ethnopharmacol. 137 (1), 864–874. doi:10.1016/j.jep.2011.07.009
Zeni, A. L. B., Zomkowski, A. D. E., Maraschin, M., Rodrigues, A. L. S., and Tasca, C. I. (2012). Ferulic acid exerts antidepressant-like effect in the tail suspension test in mice: Evidence for the involvement of the serotonergic system. Eur. J. Pharmacol. 679 (1-3), 68–74. doi:10.1016/j.ejphar.2011.12.041
Zhang, B., Ivanova-Petropulos, V., Duan, C., and Yan, G. (2021). Distinctive chemical and aromatic composition of red wines produced by Saccharomyces cerevisiae co-fermentation with indigenous and commercial non-Saccharomyces strains. Food Biosci. 41, 100925. doi:10.1016/j.fbio.2021.100925
Zhang, K., He, M., Wang, F., Zhang, H., Li, Y., Yang, J., et al. (2019). Revealing antidepressant mechanisms of baicalin in hypothalamus through systems approaches in corticosterone-induced depressed mice. Front. Neurosci. 13, 834. doi:10.3389/fnins.2019.00834
Zhang, R., Ma, Z., Liu, K., Li, Y., Liu, D., Xu, L., et al. (2019). Baicalin exerts antidepressant effects through Akt/FOXG1 pathway promoting neuronal differentiation and survival. Life Sci. 221, 241–248. doi:10.1016/j.lfs.2019.02.033
Zhao, D., Zheng, L., Qi, L., Wang, S., Guan, L., Xia, Y., et al. (2016). Structural features and potent antidepressant effects of total sterols and β-sitosterol extracted from Sargassum horneri. Mar. Drugs 14 (7), 123. doi:10.3390/md14070123
Zhen, X.-H., Quan, Y.-C., Jiang, H.-Y., Wen, Z.-S., Qu, Y.-L., and Guan, L.-P. (2015). Fucosterol, a sterol extracted from Sargassum fusiforme, shows antidepressant and anticonvulsant effects. Eur. J. Pharmacol. 768, 131–138. doi:10.1016/j.ejphar.2015.10.041
Zhu, W., Ma, S., Qu, R., Kang, D., and Liu, Y. (2006). Antidepressant effect of baicalin extracted from the root of Scutellaria baicalensis. in mice and rats. Pharm. Biol. 44 (7), 503–510. doi:10.1080/13880200600878684
Keywords: antidepressants, polyphenols, natural products, depression, herbal medicine
Citation: Kabra A, Garg R, Brimson J, Živković J, Almawash S, Ayaz M, Nawaz A, Hassan SSU and Bungau S (2022) Mechanistic insights into the role of plant polyphenols and their nano-formulations in the management of depression. Front. Pharmacol. 13:1046599. doi: 10.3389/fphar.2022.1046599
Received: 16 September 2022; Accepted: 24 October 2022;
Published: 07 November 2022.
Edited by:
Ajmal Khan, University of Nizwa, OmanReviewed by:
Muhammad Shafique, Shaqra University, Saudi ArabiaBogdan Tița, Vasile Goldiș Western University of Arad, Romania
Jolanta Orzelska-Gorka, Medical University of Lublin, Poland
Xiaobo Chen, Kunming Medical University, China
Copyright © 2022 Kabra, Garg, Brimson, Živković, Almawash, Ayaz, Nawaz, Hassan and Bungau. This is an open-access article distributed under the terms of the Creative Commons Attribution License (CC BY). The use, distribution or reproduction in other forums is permitted, provided the original author(s) and the copyright owner(s) are credited and that the original publication in this journal is cited, in accordance with accepted academic practice. No use, distribution or reproduction is permitted which does not comply with these terms.
*Correspondence: Saud Almawash, c2FsbWF3YXNoQHN1LmVkdS5zYQ==; Muhammad Ayaz, QXlhenVvcEBnbWFpbC5jb20=; Syed Shams Ul Hassan, U2hhbXMxMzI3QHlhaG9vLmNvbQ==; Simona Bungau, c2ltb25hYnVuZ2F1QGdtYWlsLmNvbQ==