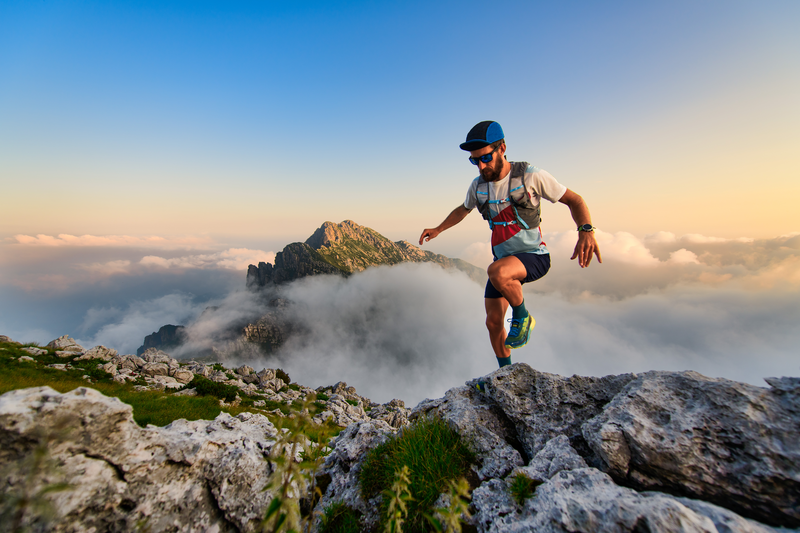
94% of researchers rate our articles as excellent or good
Learn more about the work of our research integrity team to safeguard the quality of each article we publish.
Find out more
REVIEW article
Front. Pharmacol. , 03 November 2022
Sec. Ethnopharmacology
Volume 13 - 2022 | https://doi.org/10.3389/fphar.2022.1040350
Fibrosis is the abnormal deposition of extracellular matrix, characterized by accumulation of collagen and other extracellular matrix components, which causes organ dysfunction and even death. Despite advances in understanding fibrosis pathology and clinical management, there is no treatment for fibrosis that can prevent or reverse it, existing treatment options may lead to diarrhea, nausea, bleeding, anorexia, and liver toxicity. Thus, effective drugs are needed for fibrotic diseases. Traditional Chinese medicine has played a vital role in fibrotic diseases, accumulating evidence has demonstrated that Astragalus (Astragalus mongholicus Bunge) can attenuate multiple fibrotic diseases, which include liver fibrosis, pulmonary fibrosis, peritoneal fibrosis, renal fibrosis, cardiac fibrosis, and so on, mechanisms may be related to inhibition of epithelial-mesenchymal transition (EMT), reactive oxygen species (ROS), transforming growth factor beta 1 (TGF-β1)/Smads, apoptosis, inflammation pathways. The purpose of this review was to summarize the pharmacology and mechanisms of Astragalus in treating fibrotic diseases, the data reviewed demonstrates that Astragalus is a promising anti-fibrotic drug, its main anti-fibrotic components are Calycosin, Astragaloside IV, Astragalus polysaccharides and formononetin. We also review formulas that contain Astragalus with anti-fibrotic effects, in which Astragalus and Salvia miltiorrhiza Bunge, Astragalus and Angelica sinensis (Oliv.) Diels are the most commonly used combinations. We propose that combining active components into new formulations may be a promising way to develop new drugs for fibrosis. Besides, we expect Astragalus to be accepted as a clinically effective method of treating fibrosis.
Fibrosis may be caused by normal healing or by pathological deposition of fibrous connective tissue (Zhang and Zhang, 2020), which occurs in various organs such as lung, heart, kidney, peritoneum, and is responsible for approximately 45% of all deaths in western developed countries (Wynn, 2004). Owing to inappropriate assignment, adequate epidemiological evidence is lacking (Asrani et al., 2019; Sgalla et al., 2019), available data highlights that up to 3 million people worldwide are affected by idiopathic pulmonary fibrosis (Martinez et al., 2017). More than 1 million people died for liver cirrhosis in 2010 (Mokdad et al., 2014), nearly all forms of heart diseases lead to cardiac fibrosis (Czubryt and Hale, 2021), 10% of the world’s population suffer from chronic kidney disease and renal fibrosis (Humphreys, 2018), indicating a high prevalence of fibrotic diseases.
The process of fibrosis is dynamic and occurs as a reaction to repeated or chronic tissue injuries. Trauma, toxic, drug-induced, infectious, or autoimmune injuries can all contribute to fibrosis (Thannickal et al., 2004). Inflammation is the most common precipitating factor (Aydın and Akçalı, 2018), however, in some cases removing the trigger does not stop the fibrosis process (Roehlen et al., 2020). Even though fibrosis plays a pivotal role in restoring normal tissue architecture, relentlessly progressive and irreversible fibrosis caused by repetitive or severe injury may lead to organ dysfunction and ultimately organ failure (Henderson et al., 2020).
Although understanding of the pathogenesis and management of fibrotic diseases have been greatly improved, there’s currently no cure for them (Martinez et al., 2017; Nastase et al., 2018; Roehlen et al., 2020; Czubryt and Hale, 2021). What’s more, current treatments may lead to diarrhea, nausea, bleeding, anorexia, and liver toxicity (Martinez et al., 2017; Richeldi et al., 2017). Thus, the main aim of treatment is to relieve symptoms as much as possible and slow down fibrosis progression (Martinez et al., 2017). Therefore, alternative approaches are urgently needed, natural products have always held a privileged position as valuable sources and inspirations for new drug development (Newman and Cragg, 2020). Some Chinese medicines show promising anti-fibrotic effects (El-Tantawy and Temraz, 2022; Zhang et al., 2022a).
Astragalus (Astragalus mongholicus Bunge), known as Huangqi in China, has a long history of medicinal use for more than 2000 years (Bi et al., 2020). In Chinese Pharmacopoeia, the most commonly used are Astragalus membranaceus (Fisch.) Bge. and Astragalus membranaceus (Fisch.) Bge. Var. mongholicus (Bge.) Hsiao. As an adaptogenic herb, Astragalus holds an important place in traditional Chinese medicine and is a popular herbal medicine worldwide. The medicinal value of Astragalus was first recorded in Wu shi er bing fang (Formularies for 52 Kinds of Disorders) (Gu et al., 2018). While in Shennong’s Materia Medica Classic (200–300 AD, Han Dynasty) (Zhao et al., 2022), Astragalus was classified as top grade and used for “tonifying deficiency”, which means Astragalus has fewer side-effects and excellent clinical efficacies (Bi et al., 2020). Astragalus has been widely used in foods and clinics, it is one of the approved medicine food homology species in China (Li et al., 2020). Traditionally, Astragalus is used to improve the body’s vital functions in chronic disease patients and healthy persons (Liu et al., 2017). More importantly, “deficiency” is the major initiator of fibrosis. Thus, Astragalus is commonly used for fibrosis treatment (Yao and Jiang, 2003; Xu and Liu, 2020; Sun et al., 2022). Astragalus contains more than 200 compounds, including triterpene saponins, flavonoids, and polysaccharides (Shan et al., 2019). Pharmacological studies have shown that Astragalus has anticancer, anti-aging, anti-oxidation, anti-photoaging, anti-inflammation, and improvement of cardiomyocyte functions (Li et al., 2014a; Liu et al., 2017).
In recent years, numerous studies have demonstrated the anti-fibrotic properties of Astragalus and its active components, including pulmonary, cardiac, liver, renal and peritoneal fibrosis. Mechanisms may be related to the inhibition of epithelial-mesenchymal transition (EMT), reactive oxygen species (ROS), transforming growth factor beta 1 (TGF-β1)/Smads, apoptosis, and inflammation pathways (Yu et al., 2016; Qian et al., 2018; Zheng et al., 2021; Li et al., 2022). In this review, we summarized Astragalus’s antifibrotic effects and mechanisms to provide a reference for the follow-up studies.
An online literature search was carried out at PubMed, Web of Science, Google Scholar, and China National Knowledge Infrastructure, covering 2012 until April 2022. The following keywords were used: “Astragalus” and “fibrosis”, “pulmonary fibrosis”, “liver fibrosis”, or “renal fibrosis”, “cardiac fibrosis”, and “peritoneal fibrosis”. The references of all retrieved articles were also reviewed to include relevant literature.
Myofibroblasts are cells that produce collagens and are involved in the fibrosis of different tissues, which are gradually activated by inflammatory and mechanical conditions (Pakshir et al., 2020). The origins of myofibroblasts are extensive and incompletely elucidated, including fibroblasts, endothelial cells, pericytes, and bone marrow-derived cells (Yuan et al., 2019). Since TGF-β1 can be activated in inflammatory and mechanical conditions and active TGF-β1 leads to myofibroblast activation, this signal is involved in fibrosis of almost all tissues and is a common signaling pathway in many organs (Pakshir et al., 2020). In some organs, such as the lung, kidney, and peritoneum, EMT is also involved in fibrosis process (Humphreys, 2018; Balzer, 2020; Muthuramalingam et al., 2020).
Activated hepatic stellate cells are the major effectors during hepatic fibrosis (Tsuchida and Friedman, 2017). Multiple signaling pathways regulate them, including platelet-derived growth factor (PDGF) signaling and TGF-β1. After being stimulated, activated hepatic stellate cells transform into myofibroblasts (Aydın and Akçalı, 2018), which secrete collagens I, II, and fibronectin (FN) and lead to liver fibrosis (Brown et al., 2006; Dewidar et al., 2019).
Pulmonary fibrosis can be induced by EMT, myofibroblast activation, and mechanical tension (Thannickal et al., 2004; Yang et al., 2020). Mechanisms include TGF-β1, sonic hedgehog, Notch, Wnt, fibroblast growth factor, and PDGF (Chanda et al., 2019). Common extracellular matrix components (ECM) are collagens I, III, and VI (Deng et al., 2020).
Long-term peritoneal dialysis causes fibrosis and inflammation in the peritoneal membrane. These two processes are frequently bidirectional (Zhou et al., 2016a). In addition, EMT, TGF-β1, and mechanical tension also induce peritoneal fibrosis (Balzer, 2020; Terri et al., 2021).
In kidney, myofibroblast activation can be induced by TGF-β1 and Notch pathway upregulation (Humphreys, 2018). Inflammatory cells such as macrophages and obstructive uropathy can active TGF-β1 to induce renal fibrosis (Gu et al., 2020; Yoon et al., 2020). Common ECM are collagen I, III, V, VI, VII, XV, and FN (Genovese et al., 2014).
Cardiac fibrosis is often caused by inflammation and overexpansion, both of these factors activate myofibroblasts, which secrete ECM such as collagen I, III, and IV (Yuan et al., 2019; Lafuse et al., 2020; Liu et al., 2021a).
Astragalus is shown to exert multiple antifibrosis effects (Table 1), and the active antifibrotic components are Calycosin (C16H12O5), Astragaloside IV (C41H68O14), Astragalus polysaccharides, and formononetin (C16H12O4) (Figure 1).
Studies on the pharmacokinetics of Astragalus are rare, a study showed that the primary metabolites and secondary metabolites of Astragali Radix from different origins were very different in mice after oral administration. Therefore, studies on the pharmacokinetics of Astragalus need to limit the origin, season, and planting years (Li et al., 2015). Water-soluble extracts of Astragali Radix include flavonoids, saponins, carbohydrates, amino acids, organic acids, and nucleotide derivatives (Li et al., 2015). The water extracts of Astragali Radix contain Calycosin 0.1934 mg/g, formononetin 0.16 mg/g, and Astragaloside IV 0.29 mg/g, the mean half-life (t1/2) was between 1 and 5 h (Shi et al., 2015). In the human body, flavonoids can be absorbed orally, Calycosin and formononetin are important flavonoids, and the major metabolites are glucuronides, such as calycosin-7-O-β-D-glucoside, formononetin-7-O-β-D-glucoside (Xu et al., 2006). In rats, the oral bioavailability of formononetin is 21.8% (Luo et al., 2018). In rats, up to 170 compounds (23 original constituents and 147 metabolites) were found in vivo after oral administration of Astragali Radix total flavonoids, Calycosin-3′-O-glucuronide was identified as the main metabolite of Calycosin. Calycosin and formononetin were widely distributed within rat tissues, including kidneys, lungs, heart, spleen, liver, thymus, and colon, except the brain (Liu et al., 2020).
However, lower plasma concentrations of saponins were observed in rat and human plasma, which may be associated with low bioavailability and intestinal bacterial metabolism (Xu et al., 2006; Shi et al., 2015). The absolute oral bioavailability of Astragaloside IV is only 7.4% and 2.2% in beagle dogs and rats, respectively (Gu et al., 2004; Zhang et al., 2007). After oral administration of Astragalus aqueous extract (4 g/kg raw herb), the peak concentration (Cmax) and elimination half-life (t1/2) of Astragaloside IV is 7.99 ± 5.97 ng/ml and 5.09 ± 2.26 h in beagle dogs (Yu et al., 2022). Intravenous administration has greater bioavailability than oral administration, about 50% of Astragaloside IV can be metabolized in vivo by intravenous administration in rats (Du et al., 2005). Moreover, Astragaloside IV is rapidly absorbed, metabolized by the liver, and widely distributed in the body. After intravenous administration of Astragaloside IV at a dose of 4 mg/kg for 10 min, Astragaloside IV can be found in the liver, kidney, lung, heart, and spleen in rats, with the highest content in the liver and kidney (Chang et al., 2012). In addition, Astragalus polysaccharides also have low bioavailability due to molecular weight and low solubility (Du Y. et al., 2022).
In terms of safety, Astragalus may be safe for most adults. In rats, the acute oral median lethal dose was more than 250.00 g/kg BW, and no harmful effects were found in the 90-day oral toxicity test at a dose of 15.00 g/kg BW (Li H. et al., 2021). No significant adverse effects were found in rat and beagle dog models when Astragalus extract was administered intraperitoneally or intravenously for three consecutive months. For rats, the safe dosage range is 5.7–39.9 g/kg and for beagle dogs, it is 2.85–19.95 g/kg, which is equal to 70 or 35 times that of human (0.57 g/kg, average BW 70 kg), respectively (Yu et al., 2007). In the human body, after intravenous administration of Astragalosides injection of 200–500 ml for 7 days, only transient adverse events were found, such as raised total bilirubin and rash (Xu et al., 2013).
However, intravenous administration of Astragaloside IV (0.5 and 1.0 mg/kg) affects fetal survival in rats or rabbits (Jiangbo et al., 2009), another study found that intravenous administration of 0.25 mg/kg to 1.0 mg/kg of astragaloside IV inhibited fertility in rats (Xuying et al., 2010). Therefore, Astragalus should be used cautiously in pregnant women.
Here, we summarize the antifibrosis mechanisms of Astragalus (Figure 2).
FIGURE 2. Astragalus exerts antifibrosis effects by inhibiting EMT, ROS, TGF-β1/Smads, apoptosis, and inflammation pathways. ASIV is the main antifibrotic component of Astragalus. ASIV downregulates mir-21/Wnt/β-catenin/EMT pathway (Wang et al., 2018), TGF-β1/PI3K/AKT/Foxo3a/EMT pathway (Qian et al., 2018), and sirt1 AS/EMT pathway (Qian et al., 2020). ASIV also regulates mir-135a/TGF-β/Smads pathway (Wei et al., 2020). ASIV reduces oxidative stress by upregulating p62/Keap1/Nrf2 pathway (Gao et al., 2020), ASIV enhances Nrf2 signaling to inhibit ROS-induced ferroptosis (Luo et al., 2021). In addition, ASIV can modulate MAPK and NF-κB signaling pathways to inhibit inflammation and apoptosis (Xu et al., 2014; Che et al., 2015; Dai et al., 2017; Zhou et al., 2017). ASIV inhibits NLRP3/caspase1 pathway (Wan et al., 2018; Zhang et al., 2022b). ASIV downregulates FAS/FASL pathway to inhibit apoptosis (Liu et al., 2019b). ASIV also reduces TNF-α and IL-6 expression to inhibit inflammation (Qian et al., 2018). Cal can suppress TGFBR1 signaling pathway (Chen et al., 2022). Cal also modulates PI3K/AKT and IL33/ST2 pathways to suppress inflammation (Elsherbiny et al., 2020; Wang et al., 2022b). Cal activates FXR to reduce triglyceride (Duan et al., 2017). Cal reduces ROS and balances MMP-1/TIMP-1 system to inhibit collagen synthesis (Zhang et al., 2021a). AI inhibits Jagged1/Notch1//TGF-β1/Smads/EMT and TGF-β1/NADPH oxidase/ROS pathways (Liu et al., 2014; Zhou et al., 2016b), AI can reduce MCP-1 expression to inhibit inflammation (Li et al., 2014b). FORM can regulate sirt1/Nrf2/ARE/ROS signaling pathway (Zhuang et al., 2020). AM modulates Smad7/β-catenin/EMT pathway (Yu et al., 2018). AS regulates numb/Notch/TGF-β1 pathway (Yongping et al., 2015). APS inhibits TGF-β1/ILK pathway to reduce inflammation (Zheng et al., 2021). ATS modulates PGC-1α and Bax/Bcl2/caspase3 pathway to inhibit apoptosis (Li et al., 2022).
In previous studies, the PDGF family was shown to induce hepatic stellate cell activation to aggravate hepatic fibrosis. However, Astragaloside IV can suppress PDGF-BB-induced hepatic stellate cells activation to reduce collagen I, α-smooth muscle actin (α-SMA), and FN deposition by activating the nuclear factor kappa-B (NF-κB) pathway in vitro (Chen et al., 2019). In addition, bile stasis causes cholestatic liver fibrosis (Hasegawa et al., 2021), intraperitoneal administration of Astragaloside in a bile duct ligation model of hepatic fibrosis in rats attenuated the development of fibrosis as assessed by collagen deposition, myofibroblast activation, and hepatobiliary function (Yongping et al., 2015). Furthermore, fibrosis leads to changes in cellular structure and mechanical properties (Natarajan et al., 2017), Astragalus polysaccharide can increase the stiffness of liver sinusoidal endothelial cells, which may provide a new mechanism for Chinese medicine to fight hepatic fibrosis (Lu et al., 2018). Calycosin can reduce α-SMA in both carbon tetrachloride-induced hepatic fibrosis mice and non-alcoholic steatohepatitis mice, collagen I decrease is also observed in the carbon tetrachloride model, this is related to multiple mechanisms, including inhibiting oxidative stress, matrix metalloproteinase-1/tissue inhibitor of metalloproteinase-1 (MMP-1/TIMP-1) system and activating janus kinase 2- signal transducer and activator of transcription 3 (JAK2-STAT3) pathway, while Calycosin activates farnesoid X receptor (FXR) to attenuate hepatic fibrosis in non-alcoholic steatohepatitis mice (Duan et al., 2017; Zhang et al., 2021a).
In pulmonary fibrosis rats, Astragaloside IV significantly inhibits the TGF-β1/Smad signaling pathway and attenuates extracellular matrix deposition, such as collagen I, collagen III, laminin, hyaluronic acid, hydroxyproline, high-mobility group box1 (Li et al., 2017; Li et al., 2019; Li N. et al., 2021). Besides, EMT plays a vital role in pulmonary fibrosis (Muthuramalingam et al., 2020). The inhibitory effect of Astragalus active ingredients on EMT has been confirmed in bleomycin induced-pulmonary fibrosis rats. Astragaloside IV inhibits TGF-β1/phosphoinositide-3-kinase (PI3K)/protein kinase B (AKT)-induced forkhead box O3a (Foxo3a) hyperphosphorylation and down-regulation to reverse EMT (Qian et al., 2018). Furthermore, long noncoding RNAs sirt1 antisense (sirt1 AS) is involved in organ fibrosis, sirt1 AS enhances the stability of sirt1 and increases sirt1 expression, thereby inhibiting EMT in idiopathic pulmonary fibrosis (IPF). Interestingly, Astragaloside IV treatment increases sirt1 AS expression and suppresses EMT (Qian et al., 2020). Additionally, Calycosin suppresses the AKT/glycogen synthase kinase 3β (GSK3β)/β-catenin signaling pathway to inhibit EMT (Liu et al., 2021b), Astragalus polysaccharides inhibit TGF-β1/EMT and NF-κB pathway activation (Zhang et al., 2020a). Astragalus injection also reduces collagen accumulation and α-SMA protein overexpression in bleomycin-induced-pulmonary fibrosis rats, this protective effects may be related to Jagged1/Notch1 downregulation in the lung (Zhou et al., 2016b).
Monocytes/macrophages are the principal cells in inflammation and monocyte chemoattractant protein (MCP)-1 is an effective chemokine that activates macrophages and promotes monocytes migration into tissue during inflammation (Reinecker et al., 1995; Shi and Pamer, 2011; Oishi and Manabe, 2018). Happily, Astragalus injection effectively reduces MCP-1 expression, inhibits monocytes/macrophages recruitment and activation, and suppresses TGF-β1 production in rats submitted to peritoneal dialysis, indicating its mechanisms of anti-peritoneal fibrosis effects may involve both MCP-1 and the TGF-β/Smad pathways (Li et al., 2014b). In addition, EMT is crucial for causing fibrosis and the accompanying decline in peritoneal membrane function (Strippoli et al., 2016; Balzer, 2020). Astragalus and Astragalus injection can inhibit rat and human peritoneal mesothelial cell EMT in vitro. Mechanisms are related to downregulating β-catenin and nicotinamide-adenine dinucleotide phosphate (NADPH) oxidase-dependent formation of ROS (Liu et al., 2014; Yu et al., 2018). Furthermore, Astragalus total saponins can promote the peroxisome proliferator-activated receptor gamma co-activator (PGC-1α) in peritoneal fibrosis rats, which increases mitochondrial synthesis to inhibit apoptosis and fibrosis, evidenced by decreased Smad2, α-SMA, caspase3, and Bax (Li et al., 2022).
Nearly all kidney disorders have a common ultimate pathway, and growing renal fibrosis is linked to functional impairment (Nastase et al., 2018). In hypertensive mice, TGF-β1/integrin-linked kinase (ILK) signaling pathway is activated, while it is downregulated after Astragalus polysaccharide treatment, as evidenced by reduced IL (interleukin)-1β, IL-6, α-SMA, collagen I, and collagen III (Zheng et al., 2021). Renal tubulointerstitial fibrosis is made worse by EMT. Astragalus treatment reduces TGF-β1/Smad2/3 signaling pathway to antagonize tubular EMT and deposition of FN and collagen I, significantly ameliorating renal interstitial fibrosis in a mouse model of unilateral ureteral obstruction (UUO) (Shan et al., 2016). In vitro, Astragaloside IV inhibits mitogen-activated protein kinase (MAPK) and NF-κB signaling pathways in mice renal fibroblasts, which reduces α-SMA, FN, and collagen I (Che et al., 2015), which is verified in UUO mice. A UUO mouse model for intraperitoneal injection of Astragaloside IV reduced α-SMA, FN, and collagen IV in vivo via suppressing the MAPK pathway (Xu et al., 2014). Recently, Astragaloside IV has been proven to ameliorate renal fibrosis by inhibiting inflammation via the toll-like receptor 4 (TLR4)/NF-кB signaling pathway in UUO mice (Zhou et al., 2017). Besides, Astragaloside IV significantly alleviates tubulointerstitial fibrosis by upregulating p62- Kelch-like ECH-associated protein 1 (Keap1)- Nuclear factor erythroid 2-related factor 2 (Nrf2) pathway in tacrolimus treated mice (Gao et al., 2020). A db/db (diabetic) mouse model of diabetic renal fibrosis is improved by formononetin through activating the Nrf2/antioxidant response element (ARE) signaling cascade via sirt1 (Zhuang et al., 2020). Astragaloside IV also reduces collagen IV, FN, and CD36 expression in the kidney tissues of diabetic nephropathy rats, thus delaying the process of renal fibrosis (Su et al., 2019; Zhang et al., 2020b). Recent studies also demonstrated that Astragaloside IV functioned an inhibitory role in renal fibrosis by inhibiting mir-192 and mir-21 in animal models, which contributes to suppressing TGF-β1 (Wang et al., 2018; Cao et al., 2019; Mao et al., 2019). In addition, Calycosin regulates both oxidative stress and inflammation process to suppress renal fibrosis in diabetic rats, which is related to Nrf2 upregulation and IL33/ST2 inhibition (Elsherbiny et al., 2020).
Astragalus polysaccharide could suppress the TLR4/NF-κB p65 signal pathway and protects mice from coxsackievirus B3 (CVB3)-induced virus myocarditis in vivo (Liu et al., 2019c). Similarly, Astragaloside IV suppresses the FAS/FASL signaling pathway and protects mice against CVB3-induced myocardial damage and fibrosis in vivo (Liu et al., 2019b). Fibrosis is the principal pathological change of radiation-induced heart disease and Astragalus saponin decreased collagen I, and TGF-β1 expression of rats’ cardiac fibroblasts in vitro, which may be closely related to its antioxidant action (Gu et al., 2014). Calycosin can ameliorate myocardial infarction-induced inflammation and fibrosis via activation of PI3K-AKT-IκB kinase α/β (IKKα/β) in heart failure rats and transforming growth factor-beta receptor 1 (TGFBR1) pathways in heart failure mice (Wang et al., 2022b; Chen et al., 2022). Astragaloside IV significantly downregulates the TRPM7 channel to reduce hypoxia-induced cardiac fibrosis, further study verifies that Astragaloside IV inhibits cardiac fibrosis by targeting the mir-135a-TRPM7-TGF-β/Smads pathway in rats and in vitro, α-SMA and collagen I are also decreased (Lu et al., 2017; Wei et al., 2020). What’s more, oxidative stress is directly or indirectly involved in cardiac fibrosis (Kong et al., 2014). Fortunately, Astragaloside IV has proven to be effective at regulating ROS through multiple pathways. ROS level is involved in TGF-β-mediated fibrosis and ferroptosis is involved in lipid ROS generation. Study shows that Astragaloside IV has an anti-ferroptotic action by enhancing the Nrf2 signal pathway, which may play a protective role against adriamycin-induced myocardial fibrosis in rats as assessed by collagen I, III, and TGF-β (Luo et al., 2021). Astragaloside IV treatment also relieves NADPH oxidase 2, 4 expression and oxidative stress in cardiomyocytes in vitro, leading to decreased myocardial fibrosis (Lin et al., 2019). Astragaloside IV may suppress ROS-mediated MAPK activation and cardiotrophin-1 (CT-1) upregulation to inhibit cardiac fibrosis in vitro, as Astragaloside IV reduces isoprenaline-induced rat cardiac fibroblast proliferation and collagen Ι deposition (Dai et al., 2017; Jia et al., 2017). Astragaloside IV also alleviates myocardial fibrosis by suppressing ROS/caspase1/gasdermin D (GSDMD) signaling pathway in mice (Zhang et al., 2022b). Besides, Astragaloside IV exhibits antifibrosis effects through inhibition of the NOD-like receptors family pyrin domain-containing 3 (NLRP3)/caspase1/IL-18 pathway in isoproterenol-induced cardiac fibrosis mice, evidenced by decreased collagen I, collagen III, and TGF-β1 (Wan et al., 2018). Gut microbiota is important to cardiac health, Astragaloside IV may decrease α-SMA expression and ameliorate cardiac fibrosis by increasing the Akkermansia, Defluviitaleaceae_UCG-011, and Rikenella abundance and modulating amino acid metabolism in isoprenaline-induced cardiac fibrosis mice (Du X. Q. et al., 2022).
Astragalus possesses broad anti-fibrotic effects, Astragalus polysaccharide may inhibit TGF-β1 production to manage systemic scleroderma fibrotic disorders in bleomycin-induced scleroderma murine model (Hao et al., 2015). Similarly, Calycosin may reduce α-SMA, collagen I and modulate the TGF-β signaling pathway to inhibit intestinal fibrosis on CCD-18Co cells in vitro (Liu et al., 2019a). Astragaloside IV decreases TGF-β2 and FN, collagen I to reduce mouse glaucomatous trabecular meshwork fibrosis in vivo and in vitro (Kasetti et al., 2021). Astragaloside IV and Astragalus polysaccharides promote wound healing and inhibit scar formation in vivo (Chen et al., 2013; Zhao et al., 2017). The former process may be related to inducing cell proliferation, cell migration, and angiogenesis (Chen et al., 2012), and the latter process may be related to suppressing excessive inflammation and reducing collagen I, collagen III, and FN deposition (Chen et al., 2012; Luo et al., 2016; Wang et al., 2022a). Although both processes involve collagen deposition, more inflammatory responses can be detected in scar formation, so this two-way regulation may be related to suppressing the inflammatory response.
Compatibility of herbs is one of the advantages of traditional Chinese medicine and is believed to elicit therapeutic effects (Wang et al., 2021). As shown in Table 2, Astragalus is often combined with other herbs, such as Angelica sinensis (Oliv.) Diels and Salvia miltiorrhiza Bunge or in various complex prescription formulas.
Huang Qi Decoction prevents cholestatic liver fibrosis by inhibiting the Numb/Notch signal pathway in rats (Zhang et al., 2017; Xu et al., 2020). Yangfei Huoxue Decoction downregulates vascular endothelial growth factor (VEGF), IL-1β, and Notch signal pathways to prevent bleomycin-induced pulmonary fibrosis in rats (Liu et al., 2019d; Chen et al., 2020). Danggui Buxue Decoction was found to have anti-multiorgan fibrosis effects in rats model. For example, Danggui Buxue Decoction inhibits MMP-1, 9, TIMP-1 and downregulates the level of oxidative stress in lung tissue (Gao et al., 2012; Zhao et al., 2015). Danggui Buxue Decoction prevents renal fibrosis through decreasing TIMP-1, TGF-β1 gene, NLRP3 inflammasome expressions and increasing MMP-9 gene expression, leading to decreased collagen IV, collagen I, FN deposition and α-SMA expression in rats (Wei et al., 2012; Wang et al., 2016). What’s more, Danggui Buxue Decoction could prevent renal fibrosis by suppressing the tumour necrosis factor (TNF), MAPK, and PI3K-AKT signaling pathways in UUO mice (Yuan et al., 2022).
The combination of Astragalus and Salvia miltiorrhiza Bunge is also commonly used in fibrotic diseases. Studies have proven this combination has favorable therapeutic efficacy in hypertrophic scar (Wu et al., 2014), liver fibrosis (Cao et al., 2020), cardiac fibrosis (Mao et al., 2015; Zhang et al., 2021b), renal fibrosis (Han et al., 2021).
Fibrosis causes considerable morbidity and mortality with no safe and effective treatment, and its mechanisms are poorly understood. Astragalus, with significant antifibrosis activities, has been used in traditional Chinese medicine for thousands of years, and its predominant components are saponins, flavonoids, and polysaccharides. This review summarizes the different anti-fibrotic effects of Astragalus and its anti-fibrotic components, including liver, pulmonary, peritoneal, renal, and cardiac fibrosis. Its active anti-fibrotic components are Calycosin, Astragaloside IV, Astragalus polysaccharides, and formononetin. Major mechanisms are inhibition of EMT, ROS, TGF-β1/Smads, apoptosis, and inflammation pathways. As shown in Table 1 and Figure 2, the inhibition of TGF-β1 may be the primary anti-fibrotic mechanisms of Astragalus. Astragaloside IV plays the main anti-fibrotic effects among the four active components. Astragaloside IV, Calycosin, and Astragalus polysaccharides can inhibit TGF-β1 to exert anti-fibrotic effects. In addition, Calycosin could inhibit ROS, EMT, and inflammation to exert anti-fibrotic effects, Astragalus polysaccharides could inhibit EMT and inflammation to exert anti-fibrotic effects, while formononetin plays an anti-fibrotic role by reducing ROS. We also review formulas containing Astragalus with anti-fibrotic effects, in which Astragalus and Salvia miltiorrhiza Bunge, Astragalus and Angelica sinensis (Oliv.) Diels are the most commonly used combinations.
Traditional Chinese medicine has the advantages of “multiple ingredients, multiple targets and less side effects” (Wang et al., 2019). Reasonable compatibility of medicinal herbs can increase effectiveness. However, there are still some questions, Astragalus contains more than 200 components, the pharmacology of them have not been fully elucidated, simultaneous analysis of two kinds of components is rare (Li et al., 2014a), and even the anti-fibrotic effects of the four active components are still incompletely understood despite extensive studies. Meanwhile, there are over 2000 Astragalus species (Li et al., 2014a), and amounts of main components vary in different species, even in the same species among locations and years (Ma et al., 2002; Ma et al., 2022). Astragalus is often used in combination with multiple drugs in treating fibrotic diseases. Considering the interference of other components, combining active components into new formulations may be a promising way to develop new drugs (Wu et al., 2014; Mao et al., 2015). For example, Astragaloside I, Calycosin, and levistilide A may be the three main bioactive components in Danggui Buxue Decoction, their combination exerts anti-liver fibrosis effects in mice (Guo et al., 2018). The combination of Astragaloside IV and ferulic acid can improve pulmonary and hepatic fibrosis in animal models (Zhao et al., 2020; Tong et al., 2021). Astragaloside IV combined with Ginsenoside Rg1 ameliorates renal fibrosis in rats with diabetic nephropathy (Du et al., 2018). However, fundamental and therapeutic studies are needed for optimal dose ratio and interactions between active components. More importantly, the oral bioavailability of Astragalus is limited, and its pharmacokinetics have not been fully clarified. It is feasible to use its active components as alternations, but their bioavailability is still worthy of attention. Taking Astragaloside IV as an example, its derivative LS-102 has higher bioavailability than Astragaloside IV, with definite efficacy and high safety (Qing et al., 2019). Derivatizations, modifications, co-administration, and nanotechnology can significantly improve the oral bioavailability of drugs. It is suggested that future studies should use these methods to improve the active components’ bioavailability (Zhu et al., 2022). In additon, Astragaloside IV is proven to ameliorate cardiac fibrosis in mice via modulating gut microbiota and fecal metabolites (Du X. Q. et al., 2022), given that oral administration is the main way for Astragalus, gut microbiota may be involved in fibrotic diseases, how Astragalus interacts with gut microbiota in fibrotic diseases is worthy of further study (Bajaj, 2019; Rayego-Mateos and Valdivielso, 2020; Gong et al., 2021). In recent years, multi-omics technology has been developing rapidly, providing new means for traditional Chinese medicine. It is expected to have a deep understanding of the pharmacokinetics and anti-fibrosis mechanisms of Astragalus by using multi-omics technology (Guo et al., 2020). Although Astragalus is generally considered non-toxic, the interaction between its active components and western medicine still deserves attention, and more research is needed.
Previous small clinical trial show that Salvia miltiorrhiza Bunge and Astragalus could improve liver fibrosis (Tan et al., 2001). In recent years, there are many clinical trials about Astragalus and its active components concentrated on immunomodulatory function (Brush et al., 2006; Latour et al., 2021), anticancer effects (Guo et al., 2012; Hsieh et al., 2020) and cardioprotective effects (Li et al., 2018). Therefore, large sample, multi-center, long-period studies to confirm the anti-fibrotic effects of Astragalus are highly warranted.
In conclusion, Astragalus is a promising anti-fibrotic drug, and its main anti-fibrotic components are Calycosin, Astragaloside IV, Astragalus polysaccharides, and formononetin. Future research should concentrate on these active components to confirm anti-fibrotic effects.
YZ, YL, and WM reviewed the literature, YZ and GX wrote this manuscript. YC, WX, and XX draught diagrams, PZ guided diagrams modification. CZ and LL contributed to the manuscript revision. All authors read and approved the submitted version.
Financial support was provided by the Sichuan Science and technology program, Grant/Award Number: 2020JDRC0114, 2020YFH0164, 2022YFS0392; Special subject of scientific research on traditional Chinese Medicine of Sichuan Administration of Traditional Chinese Medicine, Grant/Award Number: 2021MS093; “100 Talent Plan” Project of Hospital of Chengdu University of Traditional Chinese Medicine, Grant/Award Number: Hospital office [2021] 42.
The authors declare that the research was conducted in the absence of any commercial or financial relationships that could be construed as a potential conflict of interest.
All claims expressed in this article are solely those of the authors and do not necessarily represent those of their affiliated organizations, or those of the publisher, the editors and the reviewers. Any product that may be evaluated in this article, or claim that may be made by its manufacturer, is not guaranteed or endorsed by the publisher.
AM, Astragalus mongholicus Bunge; ASIV, astragaloside IV; APS, Astragalus polysaccharides; AI, Astragalus injection; Cal, calycosin; ATS, Astragalus total saponins; AS, Astragalus saponin; FORM, formononetin (Figure 2 was created with BioRender.com).
Asrani, S. K., Devarbhavi, H., Eaton, J., and Kamath, P. S. (2019). Burden of liver diseases in the world. J. Hepatol. 70, 151–171. doi:10.1016/j.jhep.2018.09.014
Aydın, M. M., and Akçalı, K. C. (2018). Liver fibrosis. Turk. J. Gastroenterol. 29, 14–21. doi:10.5152/tjg.2018.17330
Bajaj, J. S. (2019). Alcohol, liver disease and the gut microbiota. Nat. Rev. Gastroenterol. Hepatol. 16, 235–246. doi:10.1038/s41575-018-0099-1
Balzer, M. S. (2020). Molecular pathways in peritoneal fibrosis. Cell. Signal. 75, 109778. doi:10.1016/j.cellsig.2020.109778
Bi, Y., Bao, H., Zhang, C., Yao, R., and Li, M. (2020). Quality control of Radix astragali (the root of Astragalus membranaceus var. Mongholicus) along its value chains. Front. Pharmacol. 11, 562376. doi:10.3389/fphar.2020.562376
Brown, B., Lindberg, K., Reing, J., Stolz, D. B., and Badylak, S. F. (2006). The basement membrane component of biologic scaffolds derived from extracellular matrix. Tissue Eng. 12, 519–526. doi:10.1089/ten.2006.12.519
Brush, J., Mendenhall, E., Guggenheim, A., Chan, T., Connelly, E., Soumyanath, A., et al. (2006). The effect of echinacea purpurea, Astragalus membranaceus and Glycyrrhiza glabra on Cd69 expression and immune cell activation in humans. Phytother. Res. 20, 687–695. doi:10.1002/ptr.1938
Cao, T., Lu, Y., Zhu, M., Cheng, J., Ye, B., Fang, N., et al. (2020). Effects of Salvia miltiorrhiza and Radix astragali on the TGF-β/Smad/Wnt pathway and the pathological process of liver fibrosis in rats. Cell. Mol. Biol. 66, 46–51. doi:10.14715/cmb/2020.66.6.9
Cao, Y., Zhang, L., Wang, Y., Fan, Q., and Cong, Y. (2019). Astragaloside IV attenuates renal fibrosis through repressing epithelial-to-mesenchymal transition by inhibiting microrna-192 expression: In vivo and in vitro studies. Am. J. Transl. Res. 11, 5029–5038.
Chanda, D., Otoupalova, E., Smith, S. R., Volckaert, T., De Langhe, S. P., and Thannickal, V. J. (2019). Developmental pathways in the pathogenesis of lung fibrosis. Mol. Asp. Med. 65, 56–69. doi:10.1016/j.mam.2018.08.004
Chang, Y. X., Sun, Y. G., Li, J., Zhang, Q. H., Guo, X. R., Zhang, B. L., et al. (2012). The experimental study of Astragalus membranaceus on meridian tropsim: The distribution study of astragaloside iv in rat tissues. J. Chromatogr. B Anal. Technol. Biomed. Life Sci. 911, 71–75. doi:10.1016/j.jchromb.2012.10.024
Che, X., Wang, Q., Xie, Y., Xu, W., Shao, X., Mou, S., et al. (2015). Astragaloside IV suppresses transforming growth factor-β1 induced fibrosis of cultured mouse renal fibroblasts via inhibition of the MAPK and NF-κB signaling pathways. Biochem. Biophys. Res. Commun. 464, 1260–1266. doi:10.1016/j.bbrc.2015.07.116
Chen, G., Xu, H., Xu, T., Ding, W., Zhang, G., Hua, Y., et al. (2022). Calycosin reduces myocardial fibrosis and improves cardiac function in post-myocardial infarction mice by suppressing TGFBR1 signaling pathways. Phytomedicine. 104, 154277. doi:10.1016/j.phymed.2022.154277
Chen, H., Liu, X., Su, Y., Chang, K., Wu, H., Su, G., et al. (2020). Notch signaling pathway mediates the immunomodulatory mechanism of yangfei huoxue decoction alleviating bleomycin-induced pulmonary fibrosis in rats. J. Tradit. Chin. Med. 40, 204–211.
Chen, X., Peng, L. H., Li, N., Li, Q. M., Li, P., Fung, K. P., et al. (2012). The healing and anti-scar effects of astragaloside IV on the wound repair in vitro and in vivo. J. Ethnopharmacol. 139, 721–727. doi:10.1016/j.jep.2011.11.035
Chen, X., Peng, L. H., Shan, Y. H., Li, N., Wei, W., Yu, L., et al. (2013). Astragaloside IV-loaded nanoparticle-enriched hydrogel induces wound healing and anti-scar activity through topical delivery. Int. J. Pharm. 447, 171–181. doi:10.1016/j.ijpharm.2013.02.054
Chen, Z., Yao, L., Liu, Y., Pan, Z., Peng, S., Wan, G., et al. (2019). Astragaloside IV regulates NF-κB-Mediated cellular senescence and apoptosis of hepatic stellate cells to suppress pdgf-bb-induced activation. Exp. Ther. Med. 18, 3741–3750. doi:10.3892/etm.2019.8047
Czubryt, M. P., and Hale, T. M. (2021). Cardiac fibrosis: Pathobiology and therapeutic targets. Cell. Signal. 85, 110066. doi:10.1016/j.cellsig.2021.110066
Dai, H., Jia, G., Lu, M., Liang, C., Wang, Y., and Wang, H. (2017). Astragaloside IV inhibits isoprenaline-induced cardiac fibrosis by targeting the reactive oxygen species/mitogen-activated protein kinase signaling Axis. Mol. Med. Rep. 15, 1765–1770. doi:10.3892/mmr.2017.6220
Deng, Z., Fear, M. W., Suk Choi, Y., Wood, F. M., Allahham, A., Mutsaers, S. E., et al. (2020). The extracellular matrix and mechanotransduction in pulmonary fibrosis. Int. J. Biochem. Cell Biol. 126, 105802. doi:10.1016/j.biocel.2020.105802
Dewidar, B., Meyer, C., Dooley, S., and Meindl-Beinker, A. N. (2019). TGF-β in hepatic stellate cell activation and liver fibrogenesis-updated 2019. Cells 8, E1419. doi:10.3390/cells8111419
Du, N., Xu, Z., Gao, M., Liu, P., Sun, B., and Cao, X. (2018). Combination of Ginsenoside Rg1 and astragaloside IV reduces oxidative stress and inhibits TGF-β1/smads signaling cascade on renal fibrosis in rats with diabetic nephropathy. Drug Des. devel. Ther. 12, 3517–3524. doi:10.2147/dddt.S171286
Du, X. Q., Shi, L. P., Chen, Z. W., Hu, J. Y., Zuo, B., Xiong, Y., et al. (2022a). Astragaloside IV ameliorates isoprenaline-induced cardiac fibrosis in mice via modulating gut microbiota and fecal metabolites. Front. Cell. Infect. Microbiol. 12, 836150. doi:10.3389/fcimb.2022.836150
Du, Y., Wan, H., Huang, P., Yang, J., and He, Y. (2022b). A critical review of Astragalus polysaccharides: From therapeutic mechanisms to pharmaceutics. Biomed. Pharmacother. 147, 112654. doi:10.1016/j.biopha.2022.112654
Du, Y., Zhang, Q., Chen, G. G., Wei, P., and Tu, C. Y. (2005). Pharmacokinetics of astragaloside iv in rats by liquid chromatography coupled with tandem mass spectrometry. Eur. J. Drug Metab. Pharmacokinet. 30, 269–273. doi:10.1007/bf03190631
Duan, X., Meng, Q., Wang, C., Liu, Z., Liu, Q., Sun, H., et al. (2017). Calycosin attenuates triglyceride accumulation and hepatic fibrosis in murine model of non-alcoholic steatohepatitis via activating farnesoid X receptor. Phytomedicine 25, 83–92. doi:10.1016/j.phymed.2016.12.006
El-Tantawy, W. H., and Temraz, A. (2022). Anti-fibrotic activity of natural products, herbal extracts and nutritional components for prevention of liver fibrosis: Review. Arch. Physiol. Biochem. 128, 382–393. doi:10.1080/13813455.2019.1684952
Elsherbiny, N. M., Said, E., Atef, H., and Zaitone, S. A. (2020). Renoprotective effect of calycosin in high fat diet-fed/STZ injected rats: Effect on IL-33/ST2 signaling, oxidative stress and fibrosis suppression. Chem. Biol. Interact. 315, 108897. doi:10.1016/j.cbi.2019.108897
Gao, J., Feng, L. J., Huang, Y., Li, P., Xu, D. J., Li, J., et al. (2012). Total glucosides of Danggui Buxue tang attenuates bleomycin-induced pulmonary fibrosis via inhibition of extracellular matrix remodelling. J. Pharm. Pharmacol. 64, 811–820. doi:10.1111/j.2042-7158.2012.01490.x
Gao, P., Du, X., Liu, L., Xu, H., Liu, M., Guan, X., et al. (2020). Astragaloside IV alleviates tacrolimus-induced chronic nephrotoxicity via P62-keap1-nrf2 pathway. Front. Pharmacol. 11, 610102. doi:10.3389/fphar.2020.610102
Genovese, F., Manresa, A. A., Leeming, D. J., Karsdal, M. A., and Boor, P. (2014). The extracellular matrix in the kidney: A source of novel non-invasive biomarkers of kidney fibrosis? Fibrogenes. Tissue Repair 7, 4. doi:10.1186/1755-1536-7-4
Gong, G. C., Song, S. R., and Su, J. (2021). Pulmonary fibrosis alters gut microbiota and associated metabolites in mice: An integrated 16s and metabolomics analysis. Life Sci. 264, 118616. doi:10.1016/j.lfs.2020.118616
Gu, J., Liu, K., Li, H., Wang, X., and Yang, K. (2014). Astragalus saponin attenuates the expression of fibrosis-related molecules in irradiated cardiac fibroblasts. Acta Biochim. Biophys. Sin. 46, 492–501. doi:10.1093/abbs/gmu021
Gu, Y., Wang, G., Pan, G., Fawcett, J. P., Jiye, A., and Sun, J. (2004). Transport and bioavailability studies of astragaloside IV, an active ingredient in Radix astragali. Basic Clin. Pharmacol. Toxicol. 95, 295–298. doi:10.1111/j.1742-7843.2004.t01-1-pto950508.x
Gu, Y. Y., Liu, X. S., Huang, X. R., Yu, X. Q., and Lan, H. Y. (2020). TGF-β in renal fibrosis: Triumphs and challenges. Future Med. Chem. 12, 853–866. doi:10.4155/fmc-2020-0005
Gu, Z. R., Ge, B., Xu, A. X., Zheng, X. L., Song, P. S., and Li, F. (2018). Study on the efficacy and contraindicated medication use of Astragalus based on the traditional Chinese medicine. Chin. Tradit. Pat. Med. 11, 2524–2530.
Guo, L., Bai, S. P., Zhao, L., and Wang, X. H. (2012). Astragalus polysaccharide injection integrated with vinorelbine and cisplatin for patients with advanced non-small cell lung cancer: Effects on quality of life and survival. Med. Oncol. 29, 1656–1662. doi:10.1007/s12032-011-0068-9
Guo, R., Luo, X., Liu, J., Liu, L., Wang, X., and Lu, H. (2020). Omics strategies decipher therapeutic discoveries of traditional Chinese medicine against different diseases at multiple layers molecular-level. Pharmacol. Res. 152, 104627. doi:10.1016/j.phrs.2020.104627
Guo, T., Liu, Z. L., Zhao, Q., Zhao, Z. M., and Liu, C. H. (2018). A combination of astragaloside I, levistilide A and calycosin exerts anti-liver fibrosis effects in vitro and in vivo. Acta Pharmacol. Sin. 39, 1483–1492. doi:10.1038/aps.2017.175
Han, C., Jiang, Y. H., Li, W., and Liu, Y. (2021). Astragalus membranaceus and Salvia miltiorrhiza ameliorates cyclosporin A-induced chronic nephrotoxicity through the "Gut-Kidney Axis. J. Ethnopharmacol. 269, 113768. doi:10.1016/j.jep.2020.113768
Hao, Z. F., Su, Y. M., Liu, J. Y., Wang, C. M., and Yang, R. Y. (2015). Astragalus polysaccharide suppresses excessive collagen accumulation in a murine model of bleomycin-induced scleroderma. Int. J. Clin. Exp. Med. 8, 3848–3854.
Hasegawa, S., Yoneda, M., Kurita, Y., Nogami, A., Honda, Y., Hosono, K., et al. (2021). Cholestatic liver disease: Current treatment strategies and new therapeutic agents. Drugs 81, 1181–1192. doi:10.1007/s40265-021-01545-7
Henderson, N. C., Rieder, F., and Wynn, T. A. (2020). Fibrosis: From mechanisms to medicines. Nature 587, 555–566. doi:10.1038/s41586-020-2938-9
Hsieh, C. H., Lin, C. Y., Hsu, C. L., Fan, K. H., Huang, S. F., Liao, C. T., et al. (2020). Incorporation of Astragalus polysaccharides injection during concurrent chemoradiotherapy in advanced pharyngeal or laryngeal squamous cell carcinoma: Preliminary experience of a phase II double-blind, randomized trial. J. Cancer Res. Clin. Oncol. 146, 33–41. doi:10.1007/s00432-019-03033-8
Humphreys, B. D. (2018). Mechanisms of renal fibrosis. Annu. Rev. Physiol. 80, 309–326. doi:10.1146/annurev-physiol-022516-034227
Jia, G., Leng, B., Wang, H., and Dai, H. (2017). Inhibition of cardiotrophin-1 overexpression is involved in the anti-fibrotic effect of astrogaloside IV. Mol. Med. Rep. 16, 8365–8370. doi:10.3892/mmr.2017.7676
Jiangbo, Z., Xuying, W., Yuping, Z., Xili, M., Yiwen, Z., and Tianbao, Z. (2009). Effect of astragaloside iv on the embryo-fetal development of sprague-dawley rats and New Zealand white rabbits. J. Appl. Toxicol. 29, 381–385. doi:10.1002/jat.1422
Kasetti, R. B., Maddineni, P., Kodati, B., Nagarajan, B., and Yacoub, S. (2021). Astragaloside IV attenuates ocular hypertension in a mouse model of TGFβ2 induced primary open angle glaucoma. Int. J. Mol. Sci. 22, 12508. doi:10.3390/ijms222212508
Kong, P., Christia, P., and Frangogiannis, N. G. (2014). The pathogenesis of cardiac fibrosis. Cell. Mol. Life Sci. 71, 549–574. doi:10.1007/s00018-013-1349-6
Lafuse, W. P., Wozniak, D. J., and Rajaram, M. V. S. (2020). Role of cardiac macrophages on cardiac inflammation, fibrosis and tissue repair. Cells 10, E51. doi:10.3390/cells10010051
Latour, E., Arlet, J., Latour, E. E., Juszkiewicz, A., Luczkowska, K., Marcinkiewicz, A., et al. (2021). Standardized Astragalus extract for attenuation of the immunosuppression induced by strenuous physical exercise: Randomized controlled trial. J. Int. Soc. Sports Nutr. 18, 57. doi:10.1186/s12970-021-00425-5
Li, A. P., Li, Z. Y., Sun, H. F., Li, K., Qin, X. M., and Du, G. H. (2015). Comparison of two different astragali Radix by a 1h nmr-based metabolomic approach. J. Proteome Res. 14, 2005–2016. doi:10.1021/pr501167u
Li, H., Li, J. H., Cui, Y., Ma, D. Z., Zhang, K. Y., and Liu, J. C. (2021a). Evaluation of acute oral toxicity, genetic toxicity, and subchronic toxicity of Astragalus stem and leaf. Mod. Food Sci. Technol. 37 (10), 332–339. doi:10.13982/J.Mfst.1673-9078.2021.10.0036
Li, K., Cui, L. J., Cao, Y. X., Li, S. Y., Shi, L. X., Qin, X. M., et al. (2020). Uhplc Q-exactive ms-based serum metabolomics to explore the effect mechanisms of immunological activity of Astragalus polysaccharides with different molecular weights. Front. Pharmacol. 11, 595692. doi:10.3389/fphar.2020.595692
Li, L. C., Xu, L., Hu, Y., Cui, W. J., Cui, W. H., Zhou, W. C., et al. (2017). Astragaloside IV improves bleomycin-induced pulmonary fibrosis in rats by attenuating extracellular matrix deposition. Front. Pharmacol. 8, 513. doi:10.3389/fphar.2017.00513
Li, N., Feng, F., Wu, K., Zhang, H., Zhang, W., and Wang, W. (2019). Inhibitory effects of astragaloside IV on silica-induced pulmonary fibrosis via inactivating TGF-β1/smad3 signaling. Biomed. Pharmacother. 119, 109387. doi:10.1016/j.biopha.2019.109387
Li, N., Wu, K., Feng, F., Wang, L., Zhou, X., and Wang, W. (2021b). Astragaloside IV alleviates silica-induced pulmonary fibrosis via inactivation of the TGF-β1/smad2/3 signaling pathway. Int. J. Mol. Med. 47, 16. doi:10.3892/ijmm.2021.4849
Li, N. Y., Yu, H., Li, X. L., Wang, Q. Y., Zhang, X. W., Ma, R. X., et al. (2018). Astragalus membranaceus improving asymptomatic left ventricular diastolic dysfunction in postmenopausal hypertensive women with metabolic syndrome: A prospective, open-labeled, randomized controlled trial. Chin. Med. J. 131, 516–526. doi:10.4103/0366-6999.226077
Li, X., Qu, L., Dong, Y., Han, L., Liu, E., Fang, S., et al. (2014a). A review of recent research progress on the Astragalus genus. Molecules 19, 18850–18880. doi:10.3390/molecules191118850
Li, Z. H., Xu, R., Shi, J., Yu, M. S., Zhong, Y., He, W. M., et al. (2022). Astragalus total saponins ameliorate peritoneal fibrosis by promoting mitochondrial synthesis and inhibiting apoptosis. Am. J. Chin. Med. 50, 261–274. doi:10.1142/s0192415x22500094
Li, Z., Zhang, L., He, W., Zhu, C., Yang, J., and Sheng, M. (2014b). Astragalus membranaceus inhibits peritoneal fibrosis via monocyte chemoattractant protein (Mcp)-1 and the transforming growth factor-β1 (TGF-β1) pathway in rats submitted to peritoneal dialysis. Int. J. Mol. Sci. 15, 12959–12971. doi:10.3390/ijms150712959
Lin, J., Fang, L., Li, H., Li, Z., Lyu, L., Wang, H., et al. (2019). Astragaloside IV alleviates doxorubicin induced cardiomyopathy by inhibiting nadph oxidase derived oxidative stress. Eur. J. Pharmacol. 859, 172490. doi:10.1016/j.ejphar.2019.172490
Liu, J., Deng, T., Wang, Y., Zhang, M., Zhu, G., Fang, H., et al. (2019a). Calycosin inhibits intestinal fibrosis on ccd-18co cells via modulating transforming growth factor-β/smad signaling pathway. Pharmacology 104, 81–89. doi:10.1159/000500186
Liu, L. J., Li, H. F., Xu, F., Wang, H. Y., Zhang, Y. F., Liu, G. X., et al. (2020). Exploring the in vivo existence forms (23 original constituents and 147 metabolites) of astragali Radix total flavonoids and their distributions in rats using hplc-dad-esi-it-tof-ms(N). Molecules 25, E5560. doi:10.3390/molecules25235560
Liu, M., Lopez De Juan Abad, B., and Cheng, K. (2021a). Cardiac fibrosis: Myofibroblast-mediated pathological regulation and drug delivery strategies. Adv. Drug Deliv. Rev. 173, 504–519. doi:10.1016/j.addr.2021.03.021
Liu, P., Zhao, H., and Luo, Y. (2017). Anti-aging implications of Astragalus membranaceus (Huangqi): A well-known Chinese tonic. Aging Dis. 8, 868–886. doi:10.14336/ad.2017.0816
Liu, T., Yang, F., Liu, J., Zhang, M., Sun, J., Xiao, Y., et al. (2019b). Astragaloside IV reduces cardiomyocyte apoptosis in a murine model of coxsackievirus B3-induced viral myocarditis. Exp. Anim. 68, 549–558. doi:10.1538/expanim.19-0037
Liu, T., Zhang, M., Niu, H., Liu, J., Ruilian, M., Wang, Y., et al. (2019c). Astragalus polysaccharide from Astragalus melittin ameliorates inflammation via suppressing the activation of TLR-4/NF-κB P65 signal pathway and protects mice from CVB3-induced virus myocarditis. Int. J. Biol. Macromol. 126, 179–186. doi:10.1016/j.ijbiomac.2018.12.207
Liu, X., Chen, H., Su, G., Song, P., Jiang, M., and Gong, J. (2019d). An animal research and a chemical composition analysis of a Chinese prescription for pulmonary fibrosis: Yangfei huoxue decoction. J. Ethnopharmacol. 245, 112126. doi:10.1016/j.jep.2019.112126
Liu, X., Shao, Y., Zhang, X., Ji, X., Xie, M., and Liu, H. (2021b). Calycosin attenuates pulmonary fibrosis by the epithelial-mesenchymal transition repression upon inhibiting the AKT/GSK3β/β-catenin signaling pathway. Acta Histochem. 123, 151746. doi:10.1016/j.acthis.2021.151746
Liu, X. X., Zhou, H. J., Cai, L., Zhang, W., Ma, J. L., Tao, X. J., et al. (2014). NADPH oxidase-dependent formation of reactive oxygen species contributes to transforming growth factor β1-induced epithelial-mesenchymal transition in rat peritoneal mesothelial cells, and the role of Astragalus intervention. Chin. J. Integr. Med. 20, 667–674. doi:10.1007/s11655-012-1176-x
Lu, J., Wang, Q. Y., Zhou, Y., Lu, X. C., Liu, Y. H., Wu, Y., et al. (2017). Astragaloside IV against cardiac fibrosis by inhibiting TRPM7 channel. Phytomedicine 30, 10–17. doi:10.1016/j.phymed.2017.04.002
Lu, W. L., Li, J. M., Yang, J., Xu, C. G., Zhang, S. S., Yan, J., et al. (2018). Effects of Astragalus polysaccharide on mechanical characterization of liver sinusoidal endothelial cells by atomic force microscopy at nanoscale. Chin. J. Integr. Med. 24, 455–459. doi:10.1007/s11655-017-2964-0
Luo, L. F., Guan, P., Qin, L. Y., Wang, J. X., Wang, N., and Ji, E. S. (2021). Astragaloside IV inhibits adriamycin-induced cardiac ferroptosis by enhancing Nrf2 signaling. Mol. Cell. Biochem. 476, 2603–2611. doi:10.1007/s11010-021-04112-6
Luo, L. Y., Fan, M. X., Zhao, H. Y., Li, M. X., Wu, X., and Gao, W. Y. (2018). Pharmacokinetics and bioavailability of the isoflavones formononetin and ononin and their in vitro absorption in ussing chamber and caco-2 cell models. J. Agric. Food Chem. 66, 2917–2924. doi:10.1021/acs.jafc.8b00035
Luo, X., Huang, P., Yuan, B., Liu, T., Lan, F., Lu, X., et al. (2016). Astragaloside IV enhances diabetic wound healing involving upregulation of alternatively activated macrophages. Int. Immunopharmacol. 35, 22–28. doi:10.1016/j.intimp.2016.03.020
Ma, X. Q., Shi, Q., Duan, J. A., Dong, T. T., and Tsim, K. W. (2002). Chemical analysis of Radix astragali (Huangqi) in China: A comparison with its adulterants and seasonal variations. J. Agric. Food Chem. 50, 4861–4866. doi:10.1021/jf0202279
Ma, Y. C., Hu, J. H., Wu, W. X., Duan, Y., Fan, C. C., Feng, T. T., et al. (2022). Research progress on chemical constituents and pharmacological effects of Radix astragali. Acta Chin. Med. Pharmacol. 50, 92–95. doi:10.19664/j.cnki.1002-2392.220092
Mao, B., Nuan, L., Yang, L., and Zeng, X. (2015). Compatibility of Astragalus and Salvia extract inhibits myocardial fibrosis and ventricular remodeling by regulation of protein kinase D1 protein. Int. J. Clin. Exp. Med. 8, 3716–3724.
Mao, Q., Chen, C., Liang, H., Zhong, S., Cheng, X., and Li, L. (2019). Astragaloside IV inhibits excessive mesangial cell proliferation and renal fibrosis caused by diabetic nephropathy via modulation of the TGF-β1/smad/mir-192 signaling pathway. Exp. Ther. Med. 18, 3053–3061. doi:10.3892/etm.2019.7887
Martinez, F. J., Collard, H. R., Pardo, A., Raghu, G., Richeldi, L., Selman, M., et al. (2017). Idiopathic pulmonary fibrosis. Nat. Rev. Dis. Prim. 3, 17074. doi:10.1038/nrdp.2017.74
Mokdad, A. A., Lopez, A. D., Shahraz, S., Lozano, R., Mokdad, A. H., Stanaway, J., et al. (2014). Liver cirrhosis mortality in 187 countries between 1980 and 2010: A systematic analysis. BMC Med. 12, 145. doi:10.1186/s12916-014-0145-y
Muthuramalingam, K., Cho, M., and Kim, Y. (2020). Cellular senescence and emt crosstalk in bleomycin-induced pathogenesis of pulmonary fibrosis-an in vitro analysis. Cell Biol. Int. 44, 477–487. doi:10.1002/cbin.11248
Nastase, M. V., Zeng-Brouwers, J., Wygrecka, M., and Schaefer, L. (2018). Targeting renal fibrosis: Mechanisms and drug delivery systems. Adv. Drug Deliv. Rev. 129, 295–307. doi:10.1016/j.addr.2017.12.019
Natarajan, V., Harris, E. N., and Kidambi, S. (2017). Secs (sinusoidal endothelial cells), liver microenvironment, and fibrosis. Biomed. Res. Int. 2017, 4097205. doi:10.1155/2017/4097205
Newman, D. J., and Cragg, G. M. (2020). Natural products as sources of new drugs over the nearly four decades from 01/1981 to 09/2019. J. Nat. Prod. 83, 770–803. doi:10.1021/acs.jnatprod.9b01285
Oishi, Y., and Manabe, I. (2018). Macrophages in inflammation, repair and regeneration. Int. Immunol. 30, 511–528. doi:10.1093/intimm/dxy054
Pakshir, P., Noskovicova, N., Lodyga, M., Son, D. O., Schuster, R., Goodwin, A., et al. (2020). The myofibroblast at a glance. J. Cell Sci. 133, jcs227900. doi:10.1242/jcs.227900
Qian, W., Cai, X., and Qian, Q. (2020). Sirt1 antisense long non-coding rna attenuates pulmonary fibrosis through sirt1-mediated epithelial-mesenchymal transition. Aging (Albany NY) 12, 4322–4336. doi:10.18632/aging.102882
Qian, W., Cai, X., Qian, Q., Zhang, W., and Wang, D. (2018). Astragaloside IV modulates TGF-β1-dependent epithelial-mesenchymal transition in bleomycin-induced pulmonary fibrosis. J. Cell. Mol. Med. 22, 4354–4365. doi:10.1111/jcmm.13725
Qing, L. S., Chen, T. B., Sun, W. X., Chen, L., Luo, P., Zhang, Z. F., et al. (2019). Pharmacokinetics comparison, intestinal absorption and acute toxicity assessment of a novel water-soluble astragaloside IV derivative (astragalosidic acid, ls-102). Eur. J. Drug Metab. Pharmacokinet. 44, 251–259. doi:10.1007/s13318-018-0515-5
Rayego-Mateos, S., and Valdivielso, J. M. (2020). New therapeutic targets in chronic kidney disease progression and renal fibrosis. Expert Opin. Ther. Targets 24, 655–670. doi:10.1080/14728222.2020.1762173
Reinecker, H. C., Loh, E. Y., Ringler, D. J., Mehta, A., Rombeau, J. L., and Macdermott, R. P. (1995). Monocyte-chemoattractant protein 1 gene expression in intestinal epithelial cells and inflammatory bowel disease mucosa. Gastroenterology 108, 40–50. doi:10.1016/0016-5085(95)90006-3
Richeldi, L., Collard, H. R., and Jones, M. G. (2017). Idiopathic pulmonary fibrosis. Lancet 389, 1941–1952. doi:10.1016/s0140-6736(17)30866-8
Roehlen, N., Crouchet, E., and Baumert, T. F. (2020). Liver fibrosis: Mechanistic concepts and therapeutic perspectives. Cells 9, E875. doi:10.3390/cells9040875
Sgalla, G., Kulkarni, T., Antin-Ozerkis, D., Thannickal, V. J., and Richeldi, L. (2019). Update in pulmonary fibrosis 2018. Am. J. Respir. Crit. Care Med. 200, 292–300. doi:10.1164/rccm.201903-0542UP
Shan, G., Zhou, X. J., Xia, Y., and Qian, H. J. (2016). Astragalus membranaceus ameliorates renal interstitial fibrosis by inhibiting tubular epithelial-mesenchymal transition in vivo and in vitro. Exp. Ther. Med. 11, 1611–1616. doi:10.3892/etm.2016.3152
Shan, H., Zheng, X., and Li, M. (2019). The effects of Astragalus membranaceus active extracts on autophagy-related diseases. Int. J. Mol. Sci. 20, E1904. doi:10.3390/ijms20081904
Shi, C., and Pamer, E. G. (2011). Monocyte recruitment during infection and inflammation. Nat. Rev. Immunol. 11, 762–774. doi:10.1038/nri3070
Shi, J., Zheng, L., Lin, Z., Hou, C., Liu, W., Yan, T., et al. (2015). Study of pharmacokinetic profiles and characteristics of active components and their metabolites in rat plasma following oral administration of the water extract of astragali Radix using uplc-ms/ms. J. Ethnopharmacol. 169, 183–194. doi:10.1016/j.jep.2015.04.019
Strippoli, R., Moreno-Vicente, R., Battistelli, C., Cicchini, C., Noce, V., Amicone, L., et al. (2016). Molecular mechanisms underlying peritoneal emt and fibrosis. Stem Cells Int. 2016, 3543678. doi:10.1155/2016/3543678
Su, Y., Chen, Q., Ma, K., Ju, Y., Ji, T., Wang, Z., et al. (2019). Astragaloside IV inhibits palmitate-mediated oxidative stress and fibrosis in human glomerular mesangial cells via downregulation of CD36 expression. Pharmacol. Rep. 71, 319–329. doi:10.1016/j.pharep.2018.12.008
Sun, Q., Li, S. S., Yang, S. N., and Yu, X. Y. (2022). Research progress of traditional Chinese medicine targeting TGF-β1/smad signaling pathway in prevention and treatment of renal fibrosis. Pharmacol. Clin. Chin. Materia Medica, 1–14. doi:10.13412/j.cnki.zyyl.20220323.00
Tan, Y. W., Yin, Y. M., and Yu, X. J. (2001). [Influence of Salvia miltiorrhizae and Astragalus membranaceus on hemodynamics and liver fibrosis indexes in liver cirrhotic patients with portal hypertension]. Zhongguo Zhong Xi Yi Jie He Za Zhi 21, 351–353.
Terri, M., Trionfetti, F., Montaldo, C., Cordani, M., Tripodi, M., Lopez-Cabrera, M., et al. (2021). Mechanisms of peritoneal fibrosis: Focus on immune cells-peritoneal stroma interactions. Front. Immunol. 12, 607204. doi:10.3389/fimmu.2021.607204
Thannickal, V. J., Toews, G. B., White, E. S., Lynch, J. P., and Martinez, F. J. (2004). Mechanisms of pulmonary fibrosis. Annu. Rev. Med. 55, 395–417. doi:10.1146/annurev.med.55.091902.103810
Tong, J., Wu, Z., Wang, Y., Hao, Q., Liu, H., Cao, F., et al. (2021). Astragaloside IV synergizing with ferulic acid ameliorates pulmonary fibrosis by TGF-β1/smad3 signaling. Evid. Based. Complement. Altern. Med. 2021, 8845798. doi:10.1155/2021/8845798
Tsuchida, T., and Friedman, S. L. (2017). Mechanisms of hepatic stellate cell activation. Nat. Rev. Gastroenterol. Hepatol. 14, 397–411. doi:10.1038/nrgastro.2017.38
Wan, Y., Xu, L., Wang, Y., Tuerdi, N., Ye, M., and Qi, R. (2018). Preventive effects of astragaloside IV and its active sapogenin cycloastragenol on cardiac fibrosis of mice by inhibiting the NLRP3 inflammasome. Eur. J. Pharmacol. 833, 545–554. doi:10.1016/j.ejphar.2018.06.016
Wang, J., Zhang, D., Zhu, Y., Mo, X., Mchugh, P. C., and Tong, Q. (2022a). Astragalus and human mesenchymal stem cells promote wound healing by mediating immunomodulatory effects through paracrine signaling. Regen. Med. 17, 219–232. doi:10.2217/rme-2021-0076
Wang, L., Ding, T., Gong, W. L., Yang, C. H., and Liu, F. (2019). [Effective components of traditional Chinese medicine for regulating tgf-beta1/smads signaling pathway in hepatic fibrosis]. Zhongguo Zhong Yao Za Zhi 44, 666–674. doi:10.19540/j.cnki.cjcmm.20181221.006
Wang, L., Ma, J., Guo, C., Chen, C., Yin, Z., Zhang, X., et al. (2016). Danggui Buxue tang attenuates tubulointerstitial fibrosis via suppressing NLRP3 inflammasome in a rat model of unilateral ureteral obstruction. Biomed. Res. Int. 2016, 9368483. doi:10.1155/2016/9368483
Wang, X., Gao, Y., Tian, N., Zou, D., Shi, Y., and Zhang, N. (2018). Astragaloside IV improves renal function and fibrosis via inhibition of mir-21-induced podocyte dedifferentiation and mesangial cell activation in diabetic mice. Drug Des. devel. Ther. 12, 2431–2442. doi:10.2147/dddt.S170840
Wang, X., Li, W., Zhang, Y., Sun, Q., Cao, J., Tan, N., et al. (2022b). Calycosin as a novel PI3k activator reduces inflammation and fibrosis in heart failure through akt-ikk/stat3 Axis. Front. Pharmacol. 13, 828061. doi:10.3389/fphar.2022.828061
Wang, Y., Lin, C., Ren, Q., Liu, Y., and Yang, X. (2015). Astragaloside effect on TGF-β1, smad2/3, and Α-sma expression in the kidney tissues of diabetic kkay mice. Int. J. Clin. Exp. Pathol. 8, 6828–6834.
Wang, Y., Yang, H., Chen, L., Jafari, M., and Tang, J. (2021). Network-based modeling of herb combinations in traditional Chinese medicine. Brief. Bioinform. 22, bbab106. doi:10.1093/bib/bbab106
Wei, M. G., Sun, W., Xiong, P. H., and Shao, J. D. (2012). Antifibrotic effect of the Chinese herbs modified Danggui Buxue decoction on adriamycin-induced nephropathy in rats. Chin. J. Integr. Med. 18, 591–598. doi:10.1007/s11655-011-0816-x
Wei, Y., Wu, Y., Feng, K., Zhao, Y., Tao, R., Xu, H., et al. (2020). Astragaloside IV inhibits cardiac fibrosis via mir-135a-TRPM7-TGF-β/smads pathway. J. Ethnopharmacol. 249, 112404. doi:10.1016/j.jep.2019.112404
Wu, C., Jiang, J., Boye, A., Jiang, Y., and Yang, Y. (2014). Compound Astragalus and Salvia miltiorrhiza extract suppresses rabbits' hypertrophic scar by modulating the tgf-β/smad signal. Dermatology 229, 363–368. doi:10.1159/000365784
Wynn, T. A. (2004). Fibrotic disease and the T(H)1/T(H)2 paradigm. Nat. Rev. Immunol. 4, 583–594. doi:10.1038/nri1412
Xu, F., Zhang, Y., Xiao, S., Lu, X., Yang, D., Yang, X., et al. (2006). Absorption and metabolism of astragali Radix decoction: In silico, in vitro, and a case study in vivo. Drug Metab. Dispos. 34, 913–924. doi:10.1124/dmd.105.008300
Xu, L. M., and Liu, P. (2020). Guidelines for diagnosis and treatment of hepatic fibrosis with integrated traditional Chinese and western medicine (2019 edition). J. Integr. Med. 18, 203–213. doi:10.1016/j.joim.2020.03.001
Xu, M., Yin, J., Xie, L., Zhang, J., Zou, C., Zou, J., et al. (2013). Pharmacokinetics and tolerance of toal astragalosides after intravenous infusion of astragalosides injection in healthy Chinese volunteers. Phytomedicine 20, 1105–1111. doi:10.1016/j.phymed.2013.05.004
Xu, W., Shao, X., Tian, L., Gu, L., Zhang, M., Wang, Q., et al. (2014). Astragaloside IV ameliorates renal fibrosis via the inhibition of mitogen-activated protein kinases and antiapoptosis in vivo and in vitro. J. Pharmacol. Exp. Ther. 350, 552–562. doi:10.1124/jpet.114.214205
Xu, W., Xu, Y. N., Zhang, X., Xu, Y., Jian, X., Chen, J. M., et al. (2020). Hepatic stem cell numb gene is a potential target of huang Qi decoction against cholestatic liver fibrosis. Sci. Rep. 10, 17486. doi:10.1038/s41598-020-74324-1
Xuying, W., Jiangbo, Z., Yuping, Z., Xili, M., Yiwen, Z., Tianbao, Z., et al. (2010). Effect of astragaloside IV on the general and peripartum reproductive toxicity in sprague-dawley rats. Int. J. Toxicol. 29, 505–516. doi:10.1177/1091581810376840
Yang, J., Pan, X., Wang, L., and Yu, G. (2020). Alveolar cells under mechanical stressed niche: Critical contributors to pulmonary fibrosis. Mol. Med. 26, 95. doi:10.1186/s10020-020-00223-w
Yao, C. F., and Jiang, S. L. (2003). Prevention and treatment of pulmonary-fibrosis by traditional Chinese medicine. Zhong Xi Yi Jie He Xue Bao 1, 234–238. doi:10.3736/jcim20030327
Yongping, M., Zhang, X., Xuewei, L., Fan, W., Chen, J., Zhang, H., et al. (2015). Astragaloside prevents bdl-induced liver fibrosis through inhibition of Notch signaling activation. J. Ethnopharmacol. 169, 200–209. doi:10.1016/j.jep.2015.04.015
Yoon, H., Lee, Y. S., Lim, B. J., Han, K., Shin, H. J., Kim, M. J., et al. (2020). Renal elasticity and perfusion changes associated with fibrosis on ultrasonography in a rabbit model of obstructive uropathy. Eur. Radiol. 30, 1986–1996. doi:10.1007/s00330-019-06547-4
Yu, M., Shi, J., Sheng, M., Gao, K., Zhang, L., Liu, L., et al. (2018). Astragalus inhibits epithelial-to-mesenchymal transition of peritoneal mesothelial cells by down-regulating β-catenin. Cell. Physiol. biochem. 51, 2794–2813. doi:10.1159/000495972
Yu, S. Y., Ouyang, H. T., Yang, J. Y., Huang, X. L., Yang, T., Duan, J. P., et al. (2007). Subchronic toxicity studies of Radix astragali extract in rats and dogs. J. Ethnopharmacol. 110, 352–355. doi:10.1016/j.jep.2006.09.024
Yu, W. N., Sun, L. F., and Yang, H. (2016). Inhibitory effects of astragaloside IV on bleomycin-induced pulmonary fibrosis in rats via attenuation of oxidative stress and inflammation. Inflammation 39, 1835–1841. doi:10.1007/s10753-016-0420-5
Yu, X., Xia, K., Wu, S., Wang, Q., Cheng, W., Ji, C., et al. (2022). Simultaneous determination and pharmacokinetic study of six components in beagle dog plasma by uplc-ms/ms after oral administration of Astragalus membranaceus aqueous extract. Biomed. Chromatogr., e5488. doi:10.1002/bmc.5488
Yuan, H., Wu, X., Wang, X., and Yuan, C. (2022). Chinese herbal decoction Astragalus and Angelica exerts its therapeutic effect on renal interstitial fibrosis through the inhibition of MAPK, PI3k-AKT and TNF signaling pathways. Genes Dis. 9, 510–521. doi:10.1016/j.gendis.2020.06.001
Yuan, Q., Tan, R. J., and Liu, Y. (2019). Myofibroblast in kidney fibrosis: Origin, activation, and regulation. Adv. Exp. Med. Biol. 1165, 253–283. doi:10.1007/978-981-13-8871-2_12
Zhang, M., Wang, Y., Zhu, G., Sun, C., and Wang, J. (2021a). Hepatoprotective effect and possible mechanism of phytoestrogen calycosin on carbon tetrachloride-induced liver fibrosis in mice. Naunyn. Schmiedeb. Arch. Pharmacol. 394, 189–204. doi:10.1007/s00210-020-01891-5
Zhang, M., and Zhang, S. (2020). T cells in fibrosis and fibrotic diseases. Front. Immunol. 11, 1142. doi:10.3389/fimmu.2020.01142
Zhang, Q., Qian, D., Tang, D. D., Liu, J., Wang, L. Y., Chen, W., et al. (2022a). Glabridin from Glycyrrhiza glabra possesses a therapeutic role against keloid via attenuating pi3k/akt and transforming growth factor-β1/smad signaling pathways. J. Agric. Food Chem. 70, 10782–10793. doi:10.1021/acs.jafc.2c02045
Zhang, Q., Zhu, L. L., Chen, G. G., and Du, Y. (2007). Pharmacokinetics of astragaloside IV in beagle dogs. Eur. J. Drug Metab. Pharmacokinet. 32, 75–79. doi:10.1007/bf03190995
Zhang, R., Xu, L., An, X., Sui, X., and Lin, S. (2020a). Astragalus polysaccharides attenuate pulmonary fibrosis by inhibiting the epithelial-mesenchymal transition and NF-κB pathway activation. Int. J. Mol. Med. 46, 331–339. doi:10.3892/ijmm.2020.4574
Zhang, X., Qu, H., Yang, T., Liu, Q., and Zhou, H. (2022b). Astragaloside IV attenuate mi-induced myocardial fibrosis and cardiac remodeling by inhibiting ros/caspase-1/gsdmd signaling pathway. Cell Cycle 21, 2309–2322. doi:10.1080/15384101.2022.2093598
Zhang, X., Xu, Y., Chen, J. M., Liu, C., Du, G. L., Zhang, H., et al. (2017). Huang Qi decoction prevents bdl-induced liver fibrosis through inhibition of Notch signaling activation. Am. J. Chin. Med. 45, 85–104. doi:10.1142/s0192415x17500070
Zhang, Y., Tao, C., Xuan, C., Jiang, J., and Cao, W. (2020b). Transcriptomic analysis reveals the protection of astragaloside IV against diabetic nephropathy by modulating inflammation. Oxid. Med. Cell. Longev. 2020, 9542165. doi:10.1155/2020/9542165
Zhang, Y., Wang, J., Liu, Y. M., Chen, Y. Y., Yang, X. C., and Duan, L. (2021b). The synergistic effects of Astragalus mongholicus and Salvia miltiorrhiza on coronary heart disease identified by network pharmacology and experiment. Drug Des. devel. Ther. 15, 4053–4069. doi:10.2147/dddt.S326024
Zhao, B., Zhang, X., Han, W., Cheng, J., and Qin, Y. (2017). Wound healing effect of an Astragalus membranaceus polysaccharide and its mechanism. Mol. Med. Rep. 15, 4077–4083. doi:10.3892/mmr.2017.6488
Zhao, J. C., Wang, Y. H., Jin, Y., Liu, H. H., Chen, S. B., Zhang, W., et al. (2022). Herbal textual research on astragali Radix in famous classical formulas. Chin. J. Exp. Traditional Med. Formulae 10, 337–346. doi:10.13422/j.cnki.syfjx.20211659
Zhao, P., Zhou, W. C., Li, D. L., Mo, X. T., Xu, L., Li, L. C., et al. (2015). Total glucosides of Danggui Buxue tang attenuate BLM-induced pulmonary fibrosis via regulating oxidative stress by inhibiting NOX4. Oxid. Med. Cell. Longev. 2015, 645814. doi:10.1155/2015/645814
Zhao, X. M., Zhang, J., Liang, Y. N., and Niu, Y. C. (2020). Astragaloside IV synergizes with ferulic acid to alleviate hepatic fibrosis in bile duct-ligated cirrhotic rats. Dig. Dis. Sci. 65, 2925–2936. doi:10.1007/s10620-019-06017-3
Zheng, W., Huang, T., Tang, Q. Z., Li, S., Qin, J., and Chen, F. (2021). Astragalus polysaccharide reduces blood pressure, renal damage, and dysfunction through the TGF-β1-ILK pathway. Front. Pharmacol. 12, 706617. doi:10.3389/fphar.2021.706617
Zhou, Q., Bajo, M. A., Del Peso, G., Yu, X., and Selgas, R. (2016a). Preventing peritoneal membrane fibrosis in peritoneal dialysis patients. Kidney Int. 90, 515–524. doi:10.1016/j.kint.2016.03.040
Zhou, X., Sun, X., Gong, X., Yang, Y., Chen, C., Shan, G., et al. (2017). Astragaloside IV from Astragalus membranaceus ameliorates renal interstitial fibrosis by inhibiting inflammation via TLR4/NF-кB in vivo and in vitro. Int. Immunopharmacol. 42, 18–24. doi:10.1016/j.intimp.2016.11.006
Zhou, Y., Liao, S., Zhang, Z., Wang, B., and Wan, L. (2016b). Astragalus injection attenuates bleomycin-induced pulmonary fibrosis via down-regulating jagged1/notch1 in lungs. J. Pharm. Pharmacol. 68, 389–396. doi:10.1111/jphp.12518
Zhu, Y., Xie, N., Chai, Y., Nie, Y., Liu, K., Liu, Y., et al. (2022). Apoptosis induction, a sharp edge of berberine to exert anti-cancer effects, focus on breast, lung, and liver cancer. Front. Pharmacol. 13, 803717. doi:10.3389/fphar.2022.803717
Keywords: Astragalus mongholicus Bunge, active components, fibrosis, anti-fibrosis effects, traditional Chinese medicine
Citation: Zhu Y, Chai Y, Xiao G, Liu Y, Xie X, Xiao W, Zhou P, Ma W, Zhang C and Li L (2022) Astragalus and its formulas as a therapeutic option for fibrotic diseases: Pharmacology and mechanisms. Front. Pharmacol. 13:1040350. doi: 10.3389/fphar.2022.1040350
Received: 09 September 2022; Accepted: 18 October 2022;
Published: 03 November 2022.
Edited by:
Somasundaram Arumugam, National Institute of Pharmaceutical Education and Research, Kolkata, IndiaReviewed by:
David Dolivo, Northwestern University, United StatesCopyright © 2022 Zhu, Chai, Xiao, Liu, Xie, Xiao, Zhou, Ma, Zhang and Li. This is an open-access article distributed under the terms of the Creative Commons Attribution License (CC BY). The use, distribution or reproduction in other forums is permitted, provided the original author(s) and the copyright owner(s) are credited and that the original publication in this journal is cited, in accordance with accepted academic practice. No use, distribution or reproduction is permitted which does not comply with these terms.
*Correspondence: Chuantao Zhang, emhhbmdjaHVhbnRhb0BjZHV0Y20uZWR1LmNu; Liuying Li, YXJlbmFsbHlAc2luYS5jb20=
† These authors have contributed equally to this work and share the first authorship
Disclaimer: All claims expressed in this article are solely those of the authors and do not necessarily represent those of their affiliated organizations, or those of the publisher, the editors and the reviewers. Any product that may be evaluated in this article or claim that may be made by its manufacturer is not guaranteed or endorsed by the publisher.
Research integrity at Frontiers
Learn more about the work of our research integrity team to safeguard the quality of each article we publish.