- 1State Key Laboratory of Quality Research in Chinese Medicine, Macau University of Science and Technology, Macao, Macao SAR, China
- 2School of Biotechnology and Health Sciences, Wuyi University, Jiangmen, China
- 3Antibody Engineering Laboratory, School of Life Science & Technology, China Pharmaceutical University, Nanjing, China
There are technical obstacles in the safety evaluation of traditional Chinese medicine (TCM) injections due to their complex chemical nature and the lack of rapid and accurate in vitro methods. Here, we established a dual in vitro mitochondrial toxicity approach combing the conventional “glucose/galactose” assay in HepG2 cells with the cytotoxic assay in mitochondrial respiration deficient cells. Using this dual in vitro approach, for the first time, we systematically assessed the mitochondrial toxicity of TCM injections. Four of the 35 TCM injections, including Xiyanping, Dengzhanhuasu, Shuanghuanglian, and Yinzhihuang, significantly reduced cellular ATP production in galactose medium in the first assay, and presented less cytotoxic in the respiration deficient cells in the second assay, indicating that they have mitochondrial toxicity. Furthermore, we identified scutellarin, rutin, phillyrin, and baicalin could be the potential mitochondrial toxic ingredients in the 4 TCM injections by combining molecular docking analysis with experimental validation. Collectively, the dual in vitro approach is worth applying to the safety evaluation of more TCM products, and mitochondrial toxic TCM injections and ingredients found in this study deserve more attention.
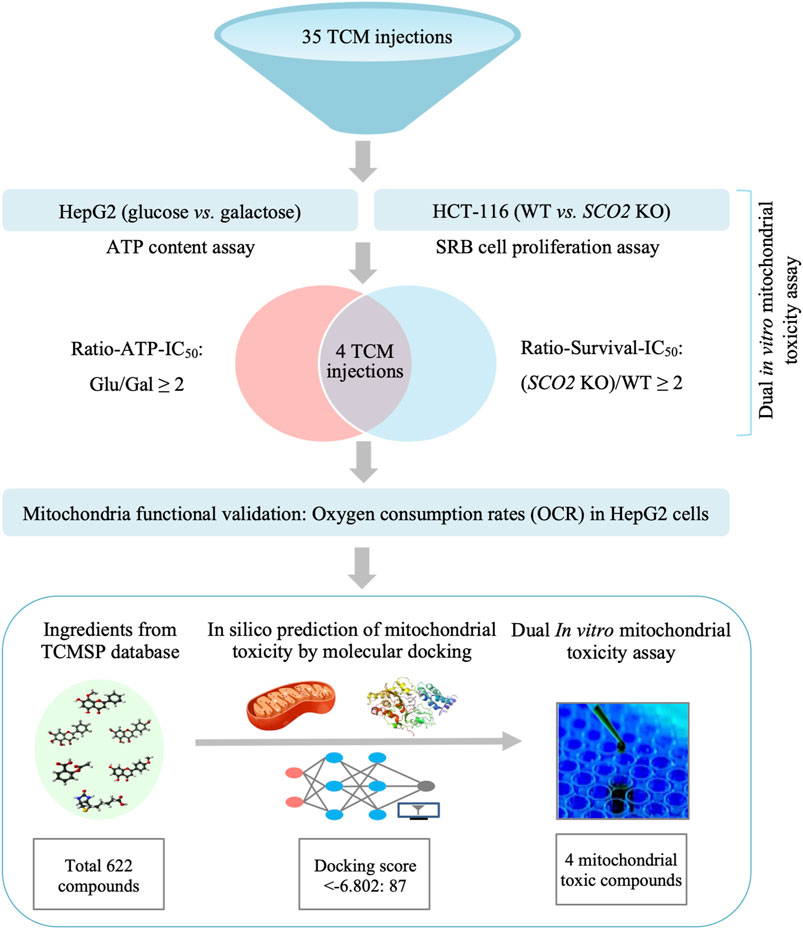
Graphical Abstract. A dual in vitro mitochondrial toxicity assay approach combing the conventional “glucose/galactose” assay in HepG2 cells with the cytotoxic assay in mitochondrial respiration deficient cells was established in this study. Using this platform, we systematically assessed the mitochondrial toxicity of TCM injections for the first time. Four TCM injections were identified with potential mitochondrial toxicity. Their toxic ingredients were predicted by molecular docking and validated by the dual in vitro approach.
Introduction
Traditional Chinese medicine (TCM) injections are a unique class of dosage forms consisting of active extracts from TCM products. TCM injections have been widely used in China since Chaihu (Bupleurum) injection was first developed in the 1940s, and have benefitted many patients, especially considering the cost-benefit tradeoffs (Xue 1984). However, increasing concerns have been raised regarding the safety of TCM injections in recent years. According to the Annual Report for National Adverse Drug Reaction Monitoring, adverse drug reactions (ADRs) caused by TCM injections accounted for 50% of all ADRs of TCMs in 2015-2015 (National Center for ADR Monitoring, 2015-2019). In contrast to conventional injections, TCM injections have a similar incidence of serious ADRs (6.02% vs. 6.72%) but a much higher incidence of unknown ADRs (46.74% vs. 24.13%) (Li et al., 2019). Therefore, significant efforts have been devoted to developing platforms for toxicity evaluation of TCMs using genomic, metabolomic, microtoxicity techniques, or animal models, which have been extensively reviewed elsewhere (Gao et al., 2019; Xie et al., 2019; Yu et al., 2020; He et al., 2021; Tu et al., 2021; Jiang et al., 2022; Wang et al., 2022). However, unlike chemical or biological drugs, the complexity of TCM ingredients makes safety assessment extremely difficult. Therefore, there is an unmet need for more explicit methods for toxicity testing of TCM injections.
Mitochondria are intracellular powerhouses that generate most of the ATP required for a cell’s biochemical reactions. They are also signaling hubs associated with thermogenesis, metabolite synthesis and transport, redox singling, calcium, copper and iron homeostasis, and cell death (Osellame et al., 2012; Mottis et al., 2019). Mitochondria are characterized by high lipid content in their membranes and proximity to respiratory byproducts of reactive oxygen species, leading them more vulnerable to chemical exposure (Meyer et al., 2018). Consequently, drugs that impede mitochondrial function can cause severe adverse effects. Approximately 35% of pharmaceutically relevant compounds are mitotoxic (Meyer and Chan 2017). Mitochondrial toxicity was the main cause of troglitazone-induced liver injury and cerivastatin-induced rhabdomyolysis, which ultimately led to the withdrawal of these two blockbusters from the market in 1997 and 2001, respectively (Hu et al., 2015; Huang et al., 2019). Drug-induced mitochondrial dysfunction has been observed in many different drug types, such as antivirals, antibiotics, chemotherapy agents, antipsychotics, antiepileptics, and antidepressants, which can lead to ADRs affecting the liver, muscle, kidney, and central nervous system (Stoker et al., 2019). However, mitochondrial toxicity is rarely detected in traditional preclinical animal models (Will and Dykens 2014). Therefore, a series of in vitro methods to detect mitochondrial toxicity have been developed over the past decade. One of the most widely employed high-throughput methods is called the “glucose/galactose” assay, in which glucose in the cell culture media is replaced with galactose, forcing the cells to produce ATP through oxidative phosphorylation (OXPHOS) rather than glycolysis and is therefore susceptible to mitochondrial toxicants (Swiss and Will 2011; Kamalian et al., 2015). In our studies, the human colorectal cancer cell line HCT116 SCO2 KO is deficient in the mitochondrial complex IV assembly SCO2 gene. Compared with the parental wild-type cells (HCT116 WT), HCT116 SCO2 KO cells have insufficient respiratory function and are resistant to the inhibition of cell proliferation by the mitochondrial toxic drug metformin (Sung et al., 2010; Wang et al., 2017). Therefore, the correlation and the scientific basis for combining the two assays is that mitochondrial toxicants can decrease intracellular ATP levels in galactose medium in the first assay, while are less cytotoxic in HCT116 SCO2 KO cells in the second assay.
Although herbal or TCM products are generally considered harmless, it has been aware in recent years that they also contain mitochondrial toxicants. Ligularia dentat Hara and Ligularia hodgsonii Hook herbs have been used for the treatment of cough, hepatitis, and inflammation in TCM. Their hepatotoxicity is caused by the high abundance of clivorine, an otonecine-type pyrrolizidine alkaloid that induces mitochondrial-dependent cell death (Ji et al., 2008; Ji et al., 2010). Neo-clerodane diterpenes present in the extensively used TCM herb skullcap are transformed into reactive metabolites by cytochrome P-450 in hepatocytes, which subsequently cause mitochondrial permeability transition, caspase activation, and apoptosis (Haouzi et al., 2000). The TCM herb Ephedra sinica, also known as Chinese ephedra or Ma Huang, induces mitophagy and inhibits mitochondrial biogenesis (Lee et al., 2017). The US Food and Drug Administration (FDA) banned the sale of ephedra-containing products, such as weight loss formulas and energy boosters, in 2004 due to cardiotoxicity (Zell-Kanter et al., 2015). However, compared with routine screening in the modern pharmaceutical industry, a systematic evaluation of the mitochondrial toxicity of TCM products is lacking.
In this study, we established a platform integrating two in vitro assays to enhance the prediction of mitochondrial toxicants. After the initial screen with the “glucose/galactose” assay, the resulting mitochondrial toxicants were counter-proved in a cell line deficient in mitochondrial respiration. Using this dual in vitro approach, for the first time, we systematically assessed mitochondrial toxicity of 35 TCM injections. Xiyanping (XYP), Dengzhanhuasu (DZHS), Shuanghuanglian (SHL), and Yinzhihuang (YZH) injections were identified as potential mitotoxicants which may correlate with their reported ADRs. Subsequently, the mitotoxic ingredients in the 4 TCM injections were identified by molecular docking and validated by functional assays.
Materials and methods
Drugs and reagents
TCM injections, TCM ingredients, rotenone, oligomycin A, antimycin A, metformin, as well as cytotoxic digoxin and tamoxifen were respectively purchased from commercial suppliers. Detailed information was shown Supplementary Tables S1, S2. Sulforhodamine B (SRB), Tris-base, puromycin, trichloroacetic acid (TCA), and crystal violet were obtained from Sigma Aldrich (St. Louis, MO, United States). Fetal bovine serum (FBS) and Dulbecco’s modified Eagle’s medium (DMEM) without glucose were obtained from Gibco (Brooklyn, NY, United States). Glucose and galactose were obtained from Psaitong (Beijing, China). HEPES was obtained from Aladdin (Shanghai, China). Sodium pyruvate was obtained from Innochem (Atlanta, GA, United States). CellTiter-Glo assay kit was purchased from Promega (Madison, WI, United States).
Cell lines and cell culture
The HCT116 and HepG2 cell lines were purchased from the American Type Culture Collection (ATCC, Manassas, VA, United States), and the HCT116 SCO2 KO cell line was a generous gift from Dr. Paul M. Hwang of the National Institutes of Health (NHLBI/NIH, United States). All three cell lines were cultured in DMEM supplemented with 1% antibiotics (0.1 mg/ml streptomycin and 100 U/ml penicillin) and 10% fetal bovine serum (FBS) at 37°C and 5% CO2 in a humidified incubator. The high-glucose media consisted of DMEM, containing 1 mM sodium pyruvate, 25 mM glucose, supplemented with 10% FBS, 1% antibiotics, and 5 mM N-2-hydro-xyethylpiperazine-N′-2-ethanesulfonic acid (HEPES). The galactose media is made up of DMEM supplemented with 10 mM galactose (without glucose), 5 mM HEPES, 1 mM sodium pyruvate, 10% FBS, and 1% streptomycin/penicillin as above.
Glucose/galactose assay
The glucose/galactose assay was performed in HepG2 cells after treatment with TCM injections or ingredients as described previously (Swiss and Will 2011; Kamalian et al., 2015; Orlicka-Płocka et al., 2020). Briefly, HepG2 cells were seeded at a density of 1× 104 cells/well in 96-well plates and cultured in either galactose or glucose medium. After 48 h of treatment with various concentrations of compounds or DMSO vehicle, total cellular adenosine triphosphate (ATP) content was examined with the CellTiter-Glo™ Luminescent Cell Viability Assay Kit from Promega (Madison, WI, United States) according to the manufacturer’s protocol. And the luminescence signal was measured by the SpectraMax paradigm microplate reader from Molecular Devices (Sunnyvale, CA, United States). The IC50 values, defined as the drug concentrations producing a 50% reduction in cellular ATP content, were calculated by fitting the data to the “log (inhibitor) vs. response --Variable slope (four parameters)” equation.
Cell proliferation assay
The antiproliferative activity of TCM injections and ingredients on HCT116 WT and HCT116 SCO2 KO cell lines were determined by the sulforhodamine B (SRB) colorimetric assay as described previously (Huang et al., 2021). In brief, cells were inoculated in 96-well plates in a volume of 90 μl/well at densities of 5,000 (HCT116 WT) or 15,000 (HCT116 SCO2 KO) cells/well. Next, cells were incubated at 37°C and 5% CO2 in a humidified incubator overnight before adding a 10 μl medium containing compounds (10X indicated concentrations). After 72 h of treatment, cells were fixed by 50 μl cold 50% (w/v) TCA at 4°C for 1 h and then stained with 100 μl 0.4% (w/v) SRB. The protein-bound dye was solubilized with 200 μl 10 mM Tris-base solution (pH 10.5), and the absorbance at 515 nm was determined using the SpectraMax paradigm microplate reader. The IC50 value was defined as a concentration that inhibited cell growth by 50% and calculated using the equation of the “log (inhibitor) vs. response --Variable slope (four parameters)”
Cellular mitochondrial respiratory function detection
The mitochondrial respiratory activity of HepG2 cells was determined using a fluorescence lifetime micro-oxygen monitoring system from Instech instruments (Plymouth, PA, United States) at 25°C according to the product manual. Briefly, HepG2 cells were inoculated in 6-well plates at a volume of 2500 μl/well at densities of 1 × 106−5 × 106 cells/well and incubated overnight. Then, cells were treated with TCM injections or ingredients at indicated concentrations for 24 h. Changes in %O2 per minute in HepG2 cells were measured according to the manufacturer’s protocol by Fluorescence Lifetime Micro Oxygen Monitoring System. The oxygen consumption rate (OCR) was calculated by the slope of the O2 data using linear regression and the results were reported as %O2 per minute, normalized to the number of cells.
Molecular docking simulation
The 3D structure of the target protein NDUFV1 (PDB ID: 5LC5), a complex composed of multiple subunits, was collected from the Protein Data Bank (PDB) (https://www.rcsb.org/). The structure files of compounds were derived from the traditional Chinese medicine systems pharmacology (TCMSP) database (https://tcmsp-e.com/) and uploaded in the 2D SDF format. Then, the target protein and compounds were transformed into PDBQT format by the molecular docking Schrödinger (Maestro Version 11.1.011) software. A crystal structure docking grid box for the target protein was constructed using Schrödinger (Maestro Version 11.1.011) software. Next, docking analysis was performed, and the docking score for each pair of compounds interacting with the target protein NDUFV1 was determined. The docking score was expressed as a negative value, the lower the value, the stronger the predicted binding strength of the compound to the target protein.
Statistical analysis
All data from the Glucose/galactose assay, SRB assay, and OCR were analyzed by GraphPad Prism 8.0 software and presented as the mean ± SD. Two-tailed Student’s t-test was used to analyze the significance of these results, and p values < 0.05 were considered statistically significant. The number of biological repeats was listed in each diagram.
Results
The establishment and validation of the dual In Vitro mitochondrial toxicity assay system
In this study, we established a cell-based in vitro mitochondrial toxicity assay system coupling a first-step screening and a second-step reverse validation. The “glucose/galactose” assay was adopted in the first-step screening, in which cells switch their energy source from glycolysis to OXPHOS. So, cells are more sensitive to mitochondrial toxicants when glucose is replaced with galactose (Swiss and Will 2011; Kamalian et al., 2015; Orlicka-Płocka et al., 2020). In this step, the human hepatocarcinoma HepG2 cell line was selected because of its super sensitivity for mitochondrial toxicity assay than other cell lines (Bugelski et al., 2000; Ma et al., 2001; Schoonen et al., 2005). In the second step, we took advantage of the human colorectal adenocarcinoma cell line HCT116 SCO2 KO, in which the mitochondrial complex IV assembly SCO2 gene is depleted. Compared to parental wild-type cells (HCT116 WT), HCT116 SCO2 KO cells are deficient in respiration and resistant to cell proliferative inhibition by the mitochondrial toxicant metformin (Sung et al., 2010; Wang et al., 2017).
Mitochondrial toxicants will decrease intracellular ATP levels in galactose medium in the first assay, while are less cytotoxic in HCT116 SCO2 KO cells in the second assay. To validate this system, we chose a well-defined set of mitochondrial toxicants, including rotenone (complex I), metformin (complex I), antimycin A (complex III), and oligomycin A (complex V), as well as cytotoxic digoxin and tamoxifen, as positive and negative control compounds, respectively. In the first assay, the IC50-ATP value, the concentration that results in a 50% reduction in ATP content, was calculated from dose-response curves for HepG2 cells cultured in glucose or galactose medium. As shown in Figure 1A; Supplementary Tables S3, the IC50-ATP values of rotenone, metformin, oligomycin A, and antimycin A were significantly reduced in galactose medium compared with that in glucose medium, while digoxin and tamoxifen did not differ significantly. According to previous publications, an IC50-ATP-Glu/IC50-ATP-Gal ratio ≥2 was defined as mitochondrial toxicity (Swiss and Will 2011; Kamalian et al., 2015; Orlicka-Płocka et al., 2020).
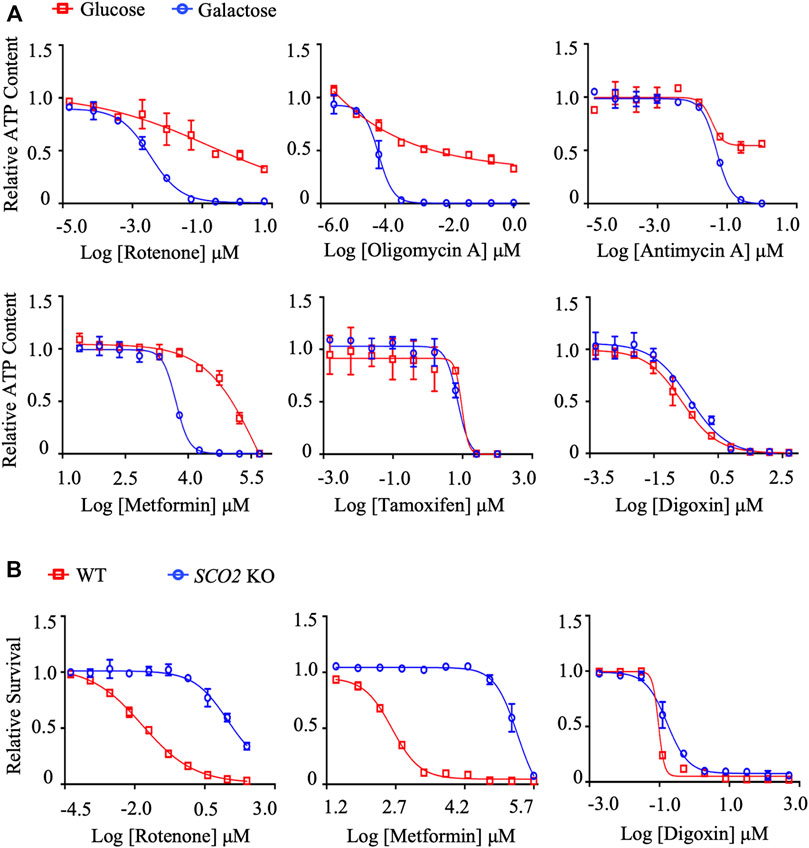
FIGURE 1. The establishment and validation of the dual in vitro mitochondrial toxicity assay system. (A) Concentration responses for high-glucose-grown (25 mM) and galactose-grown (10 mM) (blue) HepG2 cells treated with rotenone, oligomycin A, antimycin A, metformin, tamoxifen, and digoxin. After 48 h of treatment, the ATP content was assessed. (B) HCT-116 (WT; SCO2 KO) cells were treated with indicated concentrations of rotenone, metformin, and digoxin for 72 h. Cell viability was assessed by the SRB assay. Average values are from three independent experiments performed in duplicate (n = 3). Data are shown as mean ± SD.
In the second assay, cytotoxicity was assessed by the SRB assay. Half-maximum proliferative concentrations, IC50 values, were calculated from dose-response curves. As expected, the positive compounds rotenone and metformin significantly inhibited cell proliferation in HCT116 WT cells compared to HCT116 SCO2 KO cells (Figure 1B; Supplementary Tables S4). The dose-response curves and the IC50 values of the cytotoxic compound digoxin were not significantly different between the two cell lines, excluding its effect on mitochondrial function (Figure 1; Supplementary Tables S3, S4). Similarly, we defined the IC50-SCO2 KO/IC50-WT ratio ≥2 as mitochondrial toxicity.
Systematic evaluation of mitochondrial toxicity of TCM injections
Using the dual in vitro assay approach, we systematically evaluated the mitochondrial toxicity of TCM injections we could collect. Among the 35 TCM injections tested, Xiyanping (XYP), Dengzhanhuasu (DZHS), Shuanghuanglian (SHL) and Yinzhihuang (YZH) injections exhibited the IC50-ATP-Glu/IC50-ATP-Gal ratio ≥2, ranging from 2.1061 to 5.5678, implying the potential mitochondrial toxicity (Figure 2A; Table 1). This was validated by the secondary cytotoxic assay, in which the 4 TCM injections showed greater cell proliferation inhibition in HCT116 WT cells than in HCT116 SCO2 KO cells, and all the IC50-SCO2 KO/IC50-WT ratios were ≥2 (Figure 2B; Supplementary Tables S2). In addition, three TCM injections, including Salvianolate, Sodium aescinate, and Shugannin, were moderately toxic to the mitochondrion in the first assay, with IC50-ATP-Glu/IC50-ATP-Gal ratios between 1 and 2 (Table 1). Two out of the three TCM injections, except Sodium aescinate, were also defined as weak mitochondrial toxicants in the second assay (Table 2). Therefore, the dual in vitro assays exhibited high consistency.
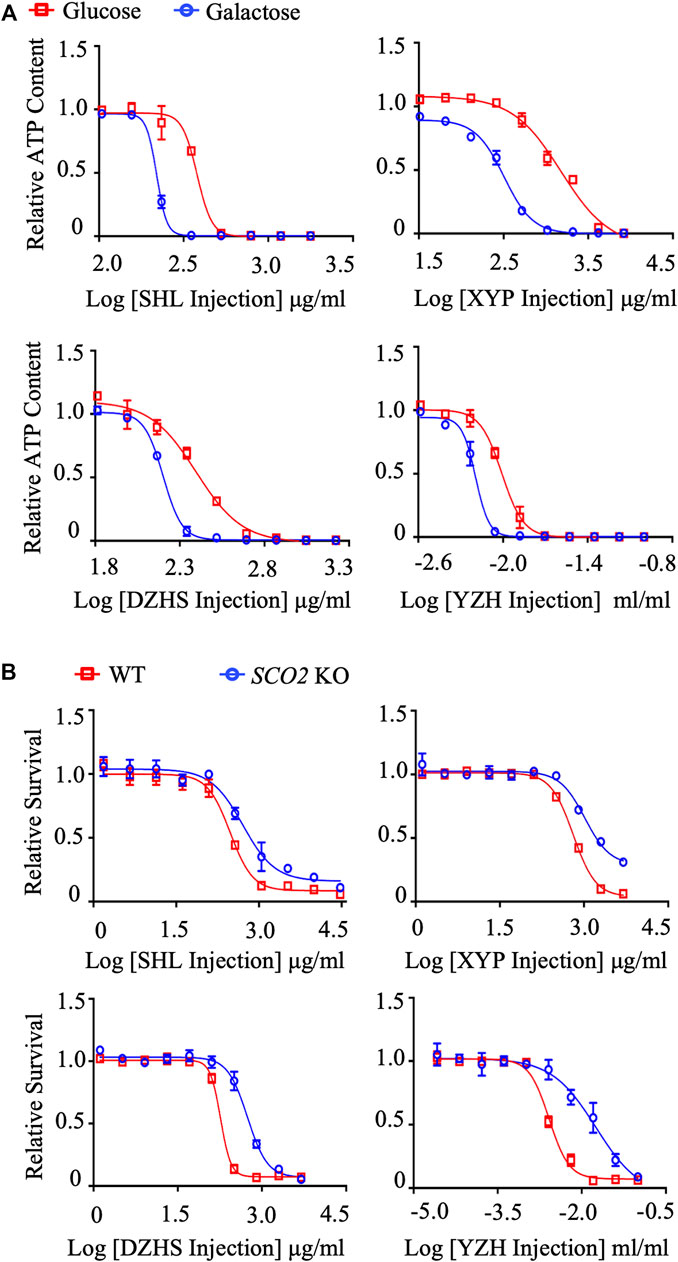
FIGURE 2. Systematic evaluation of mitochondrial toxicity of TCM injections. (A) Concentration responses for high-glucose-grown (25 mM) and galactose-grown (10 mM) (blue) HepG2 cells treated with XYP, DZHS, SHL and YZH injections. After 48 h of treatment, the ATP content was assessed. (B) HCT-116 (WT; SCO2 KO) cells were treated with indicated concentrations of XYP, DZHS, SHL, and YZH injections for 72 h. Cell viability was assessed by the SRB assay. Average values are from three independent experiments performed in duplicate (n = 3). Data are shown as mean ± SD.
The safety issues of the above 4 TCM injections have been widely reported. As summarized in Table 3, body as a whole-general disorders as well as skin and appendages disorders, both have allergic pathology, are the most common ADRs. The Adverse Drug Reaction Information Bulletin, issued by the National Medical Products Administration (NMPA), is the major source of information on drug safety issues in China. Due to severe allergic reactions, SHL injection was notified twice in 2005 (National Center for ADR Monitoring, 2005) and 2009 (National Center for ADR Monitoring, 2009), and XYP injection was notified once in 2012 (National Center for ADR Monitoring, 2012). In addition, DZHS injection caused liver and kidney dysfunction (Chengfeng et al., 2020; Zhang et al., 2021b). YZH and XYP injections affected the cardiovascular and nervous systems (Chen et al., 2012; Chen et al., 2016; National Medical Products Administration, 2016). As drug-induced mitochondrial toxicity can affect multiple organs, such as liver, heart, kidney, skeletal muscle, and brain (Will and Dykens 2014), we speculate that these ADRs may be related to their effects on mitochondria.
Identification of mitochondrial toxic ingredients by molecular docking
It is of great significance to identify mitochondrial toxic ingredients from the 4 TCM injections. On the one hand, it can be inferred that other TCM products containing the same ingredients may have similar concerns. On the other hand, by minimizing the content of the harmful components, the safety of related products can be improved. To this end, we first retrieved all known ingredients of the 4 TCM injections from the TCMSP database (https://tcmsp-e.com/). A total of 622 ingredients from the seven common herbs were found after eliminating the overlaps, including 143 in Scutellariae Radix, 53 in Artemisiae Scopariae Herba, 49 in Erigeron Breviscapus, 236 in Lonicerae Japonicae Flos, 150 in Forsythiae Fructus, 98 in Gardeniae Fructus, and 1 in Andrographis Herba (Figure 3A). Next, we employed molecular docking to identify potential mitochondrial toxic compounds. Complex I (NADH: ubiquinone oxidoreductase), the largest enzyme of the mitochondrial respiratory chain, is the most common binding target of mitochondrial toxic drugs (Imaizumi et al., 2015; Murai and Miyoshi 2016). NADH dehydrogenase [ubiquinone] flavoprotein 1, mitochondrial (NDUFV1) is the core subunit of complex I, and its binding with compounds results in defects in the electron transfer chain (Zhu et al., 2016). In addition, by empirical judgment and Sitemap (the module of Schrödinger (Maestro Version 11.1.011) software) analysis, NDUFV1 is the best protein-ligand binding pocket in complex I. Therefore, we took NDUFV1 as an example to perform molecular docking with 622 compounds from 4 TCM injections.
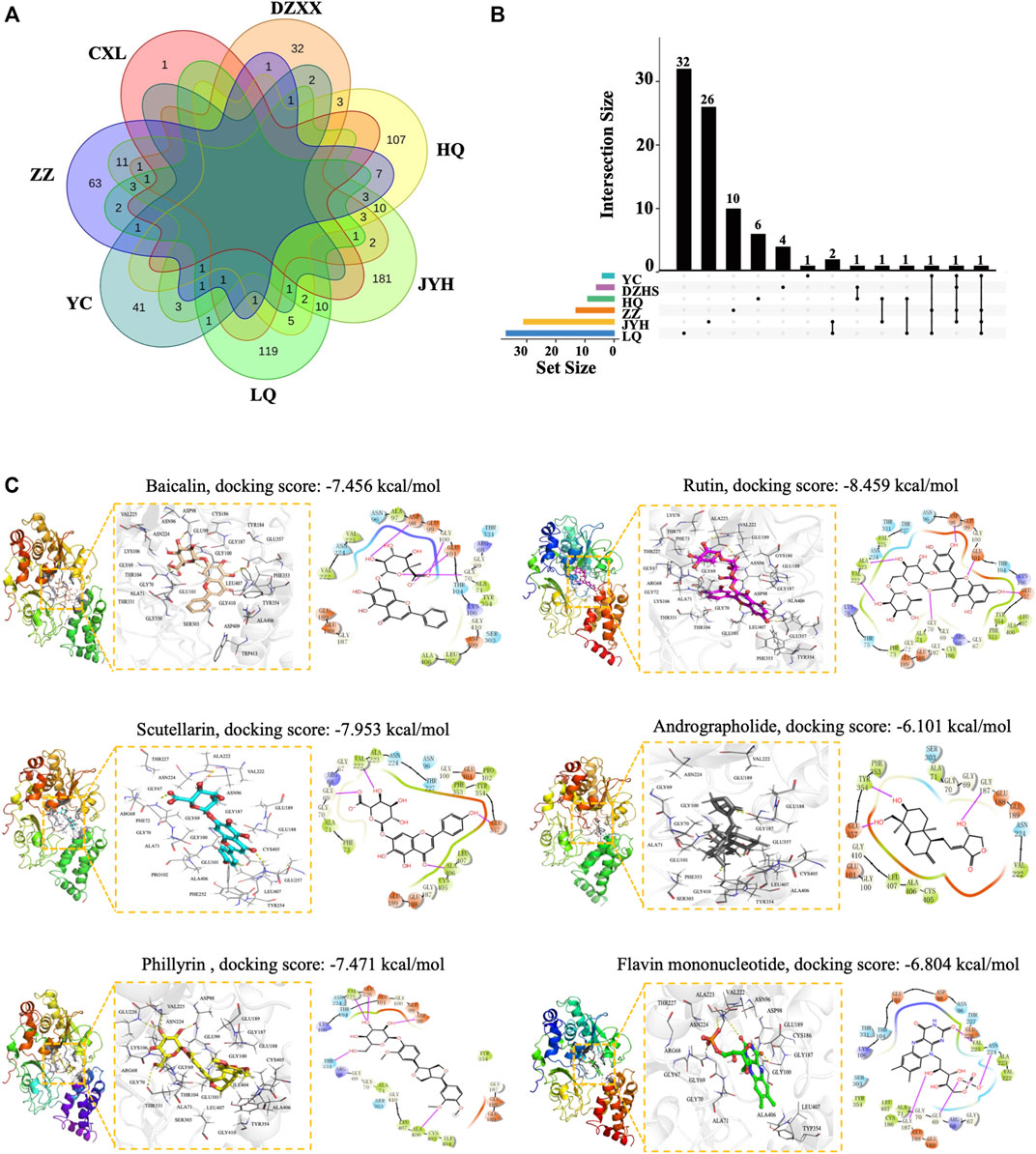
FIGURE 3. Identification of mitochondrial toxic ingredients by molecular docking. (A) Venn diagram of ingredients from Huangqin (HQ), Jinyinhua (JYH), Lianqiao (LQ), Yinchen (YC), Zhizi Dengzhanxixin (DZXX), and Chuanxinlian (CXL). (B) UpSetR plot of ingredients with a docking score below −6.804. (C) Molecular modes of the binding of compounds with NDUFV1 complex shown as 3D and 2D diagrams. The hydrogen bond is indicated by a yellow dotted line (left) and the purple arrows represent hydrogen bond interactions between molecules. Baicalin, scutellarin, rutin, phillyrin, andrographolide, and the natural ligand flavin mononucleotide are shown in orange, cyan, yellow, magenta, gray, and green sticks respectively, and key residues are shown as a line in white (left).
After eliminating the overlaps, a total of 87 compounds showed a strong binding ability to NDUFV1 with a docking score lower than −6.804, the value for the natural ligand flavin mononucleotide. Among the 87 compounds, there are 9 in Scutellariae Radix, 4 in Artemisiae Scopariae Herba, 6 in Erigeron Breviscapus, 31 in Lonicerae Japonicae Flos, 37 in Forsythiae Fructus, and 13 in Gardeniae Fructus (Figure 3B; Supplementary Tables S5). Baicalin and phillyrin in SHL and YZH and scutellarin in DZHS are quality control ingredients in the “Chinese Pharmacopeia 2020 Edition” (Commission 2020). In addition, baicalin, rutin, and phillyrin have been reported to be associated with various ADRs (Table 4). XYP injection is a mixture of andrographolide sulfate, which is a water-soluble medicament prepared from andrographolide extracted from Andrographis Herba through sulfonating reaction (Chong et al., 2013; Zheng et al., 2016). Therefore, we further analyzed the binding modes of interaction between these compounds and the target protein. From the 3D crystal structure of the small molecule-NDUFV1 complex, it can be seen that the distance between the amino acid residue and the small molecule was less than five Å (Figure 3C). The docking scores of scutellarin, rutin, phillyrin, and baicalin were −7.456 kcal/mol, −7.953 kcal/mol, −7.471 kcal/mol, and −8.459 kcal/mol, respectively (Figure 3C). So, these compounds can bind strongly to NDUFV1, suggesting their potential mitochondrial toxicity. In line with our findings, it has been recently reported that anthracene-9,10-dione and phthalaldehyde, a series of flavonoid derivative substructures, including baicalin, scutellarin, and rutin, were mitochondrial toxic identified by machine learning (Zhao P. et al., 2021).
In Vitro validation of mitochondrial toxicity of identified TCM ingredients
To validate the findings of molecular docking, these compounds were subjected to the dual in vitro mitochondrial toxicity assay. As expected, all compounds showed an IC50-ATP-Glu/IC50-ATP-Gal ratio ≥2 (Figure 4A; Table 5) and an IC50-SCO2 KO/IC50-WT ratio ≥2 (Figure 4B; Table 6), which demonstrated to be mitochondrial toxic. As summarized in Table 4, baicalin and rutin are the main components in SHL injection causing the anaphylactoid reaction (Zhang et al., 2017; Wang et al., 2020). Baicalin, also present in YZH injection, has been reported to induce kidney damage and renal fibrosis (Cai et al., 2017). Rutin, a plant pigment contained in SHL and YZH injections, is generally safe, but overdose can cause cardiovascular and neurological disorders (Wilson 2017). Although phillyrin in SHL injection and scutellarin in DZHS injection were identified as potential mitochondrial toxicants by molecular docking analysis (Figure 3C) and our dual in vitro approach (Figures 4A,B), there are few reports of their ADRs (Table 4).
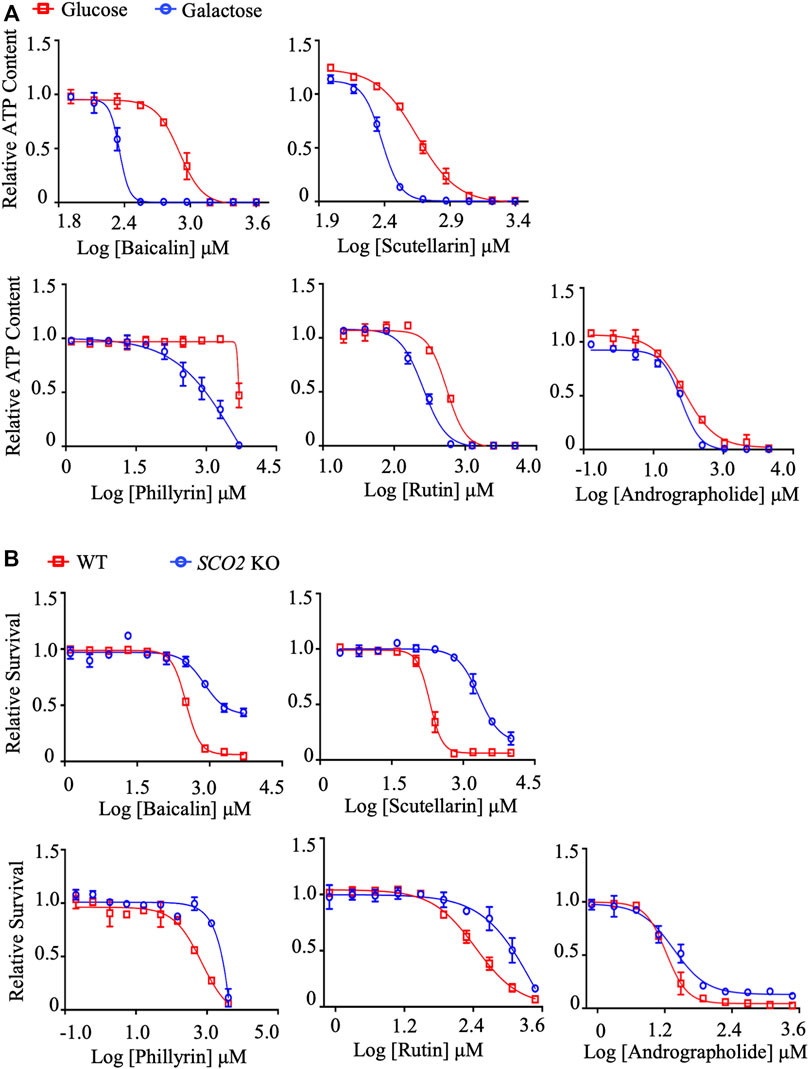
FIGURE 4. In vitro validation of mitochondrial toxicity of identified TCM ingredients. (A) Concentration responses for high-glucose-grown (25 mM) and galactose-grown (10 mM) (blue) HepG2 cells treated with baicalin, scutellarin, rutin, phillyrin, and andrographolide. After 48 h of treatment, the ATP content was assessed. (B) HCT-116 (WT; SCO2 KO) cells were treated with indicated concentrations of baicalin, scutellarin, rutin, phillyrin, and andrographolide for 72 h. Cell viability was assessed by the SRB assay. Average values are from three independent experiments performed in duplicate (n = 3). Data are shown as mean ± SD.
XYP injection is a mixture of andrographolide sulfate made from andrographolide extracted from Andrographis Herba through sulfonation reaction to increase water solubility, bioavailability, and stability (Loureiro Damasceno et al., 2022; Zou et al., 2022). Andrographolide is the main component of XYP injection. However, its docking score with NDUFV1 was not significant (−6.101 kcal/mol) (Figure 3C), which led us to speculate that its mitochondrial toxicity may not be due to its binding to complex I. To our surprise, neither the IC50-ATP-Glu/IC50-ATP-Gal ratio (Figure 4A; Table 5) nor the IC50-SCO2 KO/IC50-WT ratio (Figure 4B; Table 6) of andrographolide was ≥2, indicating lower mitochondrial toxicity compared with XYP injection (Figure 2; Table 1, 2). This discrepancy indicates that the mitochondrial toxicity of XYP injection may come from uncharacterized components during the extraction process or during the sulfonation reaction, which needs further investigation.
Effects of mitochondrial toxic TCM injections and ingredients on the respiration of HepG2 cells
Measurement of oxygen consumption rate (OCR) has long been used as the gold standard for evaluating drug-induced mitochondrial toxicity (Meyer et al., 2018). To validate the identified mitochondrial toxic TCM injections and ingredients, we performed OCR assays in HepG2 cells using a fluorescence lifetime micro-oxygen monitoring system. As expected, positive mitochondrial toxicants, such as rotenone, oligomycin A and metformin, significantly inhibited OCR levels (Figure 5A). Similar effects were observed for the identified TCM injections and their ingredients (Figures 5B,C). Taken together, these results suggest that XYP, DZHS, SHL, and YZH injections have potential mitochondrial toxicity, which may be related to their ADRs. Their adverse effects on mitochondria are due at least in part to the toxic components baicalin, rutin, scutellarin, and phillyrin.
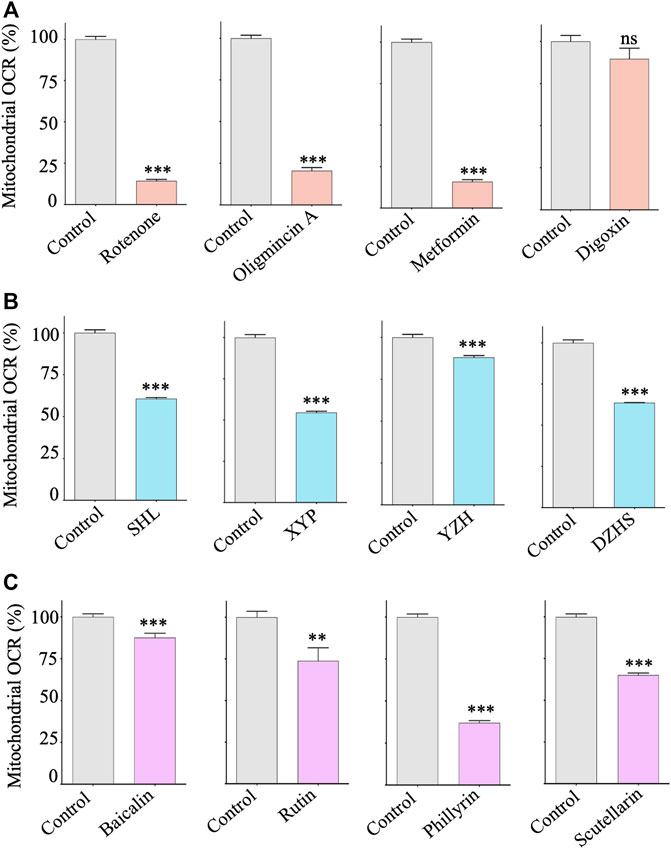
FIGURE 5. Effects of mitochondrial toxic TCM injections and ingredients on the respiration of HepG2 cells. HepG2 cells were treated with control compounds, TCM injections and ingredients with indicated concentrations for 24 h. The mitochondrial respiration rate (OCR) was assessed by the Instech Fiber Optic Oxygen Monitor System. (A) Control compounds: rotenone (2 μM), oligomycin A (2 μM), metformin (10 mM), and digoxin (2 μM). (B) TCM injections: SHL (200 μg/ml), XYP (500 μg/ml), YZH (0.01 ml/ml), and DZHS (200 μg/ml). (C) TCM ingredients: baicalin (200 μM), rutin (200 μM), phillyrin (500 μM), and scutellarin (200 μM). Average values are from three independent experiments performed in duplicate (n = 3). Data are analyzed by two-tailed Student’s t-test and presented as mean ± SD. (*p < 0.05, **p < 0.01, *** p <0.001; ns, nonsignificant).
Discussion
Although TCM injections have benefited China’s health system for decades, concerns about their safety have grown in recent years. The China National Medical Products Administration (NMPA) thus put forward mandatory requirements for post-marketing re-evaluation of TCM injections in 2018 (Drug Evaluation Center 2018). Drug-induced mitochondrial dysfunctions are one of the major underlying mechanisms of ADRs, and related detection methods have been widely used in the modern pharmaceutical industry but rarely in TCM products. Wang et al. (2015) carried out a pilot mitochondrial toxicity study using high content microscopy of four TCM injections, including Danhong, Xiangdan, Mailuoning, and Fufangkushen. They found that mitochondrial dysfunction may be related to the hepatotoxicity of Fufangkushen injection. However, the requirements for expensive equipment limit the application of this assay. In this study, we adopted the more affordable “glucose/galactose” assay for mitotoxicants screening, combined with the SRB cytotoxicity assay on a cell line deficient in mitochondrial respiration for validation. The dual in vitro mitochondrial toxicity assay system provides additional accuracy for mitochondrial toxicity prediction compared to the one-step “glucose/galactose” assay. Furthermore, the system is suitable for high-throughput detection.
It should be noted that there are other well-established methods and assays to study drug-induced mitochondrial toxicity that can be assessed in isolated mitochondria, cells, and tissue. As summarized by Mihajlovic et al. these methods include mitochondrial membrane potential (MMP), mitochondrial permeability transition pore (MPTP) opening, mitochondrial NADPH and NADH, mitochondrial ROS, mtDNA copy number, and mitochondrial morphology, size, and number (Mihajlovic and Vinken 2022). In addition, in silico prediction approaches have been recently developed (Rana et al., 2019; Hemmerich et al., 2020; Troger et al., 2020). However, above methods also have some disadvantages, including high toxicity, low sensitivity, poor specificity, and high cost of some probes when used alone. Compared with these methods, the advantages of the dual in vitro approach were inexpensive, consistent, reproducible, high-throughput and additional accuracy for mitochondrial toxicity prediction. And the disadvantages of the dual in vitro approach were the lack of the overall response, such as live kidney function and myocardial enzymes, etc. Therefore, the selection and combination of these methods can provide more convenient and robust strategies for mitochondrial toxicity assessment and can contribute to the TCM safety research society.
Mechanistically, one of the most prominent causes of mitochondrial toxicity of compounds is the interaction with respiratory chain complexes. For the first time, we carried out molecular docking studies to screen mitochondrial toxic compounds from TCM products. However, our attempts were limited to NDUFV1, the core subunit of complex I. To comprehensively predict the mitochondrial toxic ingredients of TCM injections, the targets of other complexes should also be considered. The 3D structures of SDHA subunit in complex II (Sun et al., 2005), UQCRFS1 subunit in complex III (Xia et al., 2013), NDUFA4 subunit in complex IV (Balsa et al., 2012), and α (ATP5A1) subunit in complex V (Abbas et al., 2020) have been solved and can be used for molecular docking analysis of TCM injection compounds. Meanwhile, our molecular docking analysis revealed a large number of TCM compounds with a high binding ability to mitochondria, providing a rich database for machine learning. Although the TCMSP database is very professional and reliable, in future studies, we should consider combining with other techniques, such as chromatography tandem mass spectrometry, to predict the mitochondrial toxicity of TCM ingredients.
Currently, after Lianbizhi withdraws from the market in 2022, there are 133 TCM injections approved by the NMPA (http://www.nmpa.gov.cn/WS04/CL2042/). Although we could not collect all of them due to the temporary suspension of sales for some TCM injections, 35 is an acceptable representative number. Therefore, we systematically evaluated the mitochondrial toxicity of TCM injections for the first time. The percentage of TCM injections with mitochondrial toxicity, 4 out of 35, is relatively high, implying all the other TCM injections need to be screened immediately. It is also suggested the necessity of mitochondrial toxicity evaluation for other dosage forms of TCM.
On the one hand, mitochondrial toxicants are intrinsic constituents of TCM products, such as those mentioned in the introduction section, as well as scutellarin, baicalin, rutin, and phillyrin found in this study. On the other hand, they may also come from environmental containments introduced during the cultivation and processing of TCM products, such as air pollutants, pesticides, and heavy metals known to be toxic to mitochondria (Roubicek and Souza-Pinto, 2017). This suggests that mitochondrial toxicity assessment should be considered throughout the entire process of TCM production, and re-emphasizes the importance of Good Agricultural Practice (GAP) and Good Manufacturing Practice (GMP) to the TCM industry.
The identified mitochondrial toxic compounds exist in a variety of herbal medicines. For example, according to the HERB database (http://herb.ac.cn/), Chinese herbal medicines such as Radix Scutellariae, Radix Bupleuri, Flos Carthami, and Radix Salviae liguliobae contain baicalin and rutin. Therefore, attention should be paid to other TCM products containing these mitochondrial toxic compounds. Although Chaihu, Danhong, and Danshen injections contain baicalin and rutin, and Tanreqing injection contains baicalin, rutin, and phillyrin, they did not exhibit strong mitochondrial toxicity in our dual in vitro system. This may be due to the relatively low concentrations of these compounds in these TCM injections.
Anaphylactic shock and anaphylactoid reaction are the most common ADRs of TCM injections and have been reported in the 4 mitochondrial toxic TCM injections identified in this study (Li et al., 2019). Like other mitochondrial toxicants, mitochondria damage induced by TCM injections results in the release of damage-associated molecular patterns (mtDAMPs), including ATP, ROS, cardiolipin, mitochondrial DNA (mtDNA), and formyl peptides. Once leak into the cytosol, mtDAMPs can potentiate inflammatory and type I interferon responses through the engagement of innate sensors, such as TLR9, cGAS, NLRs, and FPRs (West, 2017).
Certain populations are more susceptible to mitochondrial toxicants. Primary mitochondrial disease (PMD) refers to a group of metabolic disorders due to mitochondrial dysfunction caused by germline mutations in mtDNA or nuclear DNA (Niyazov et al., 2016). PMDs, such as Leigh syndrome, Kearns-Sayre syndrome, Alpers-Huttenlocher syndrome, mitochondrial encephalomyopathy with lactic acidosis and stroke-like episodes (MELAS) syndrome, and ataxia neuropathy syndrome, share similar mechanisms and symptoms with mitochondrial toxicants induced ADRs. So, the latter play important roles in the penetrance and pathogenesis of PMD patients (Chan, 2017). The “mitochondrial theory of aging” proposes that mitochondrial function declines with age and has a casualty relationship with age-related diseases such as cardiovascular disease, Parkinson’s, Alzheimer’s and Huntington’s disease, hearing loss, sarcopenia, inflammation, and cancer (Bratic and Larsson 2013). Mitochondrial toxicants can increase the frequency and severity of these diseases (Will et al., 2019). Therefore, particular attention should be paid to the use of TCM products among the elderly, who are the main consumer population, usually on a long-term basis (De Souza Silva et al., 2014).
Conclusion
In summary, we have established a dual in vitro mitochondrial toxicity screen system by combing the “glucose/galactose” assay in HepG2 cells with the cytotoxicity assay in mitochondrial respiration deficient cells. Using this system, we systematically evaluated mitochondrial toxicity in TCM injections for the first time. The method is worth applying to the safety evaluation of more TCM products.
Data availability statement
The raw data supporting the conclusions of this article will be made available by the authors, without undue reservation.
Author contributions
WM, NF, and YS conceived and designed the experiments, YS wrote the manuscript, and WM corrected the draft, WM supervised the experimentators, YS, KG, ZH, JD, JC, QL, CW, ZW, FZ, JZ, and WL carried out the experiments and analyzed the data. All data were generated in-house, and no paper mill was used. All authors agree to be accountable for all aspects of work ensuring integrity and accuracy.
Funding
This work was funded by the Science and Technology Development Fund, Macau SAR, China (File no. 0036/2020/A1 and 0013/2019/A1), and the Hong Kong-Macao Joint Research and Development Fund of Wuyi University (File no. 2019WGALH12).
Conflict of interest
The authors declare that the research was conducted in the absence of any commercial or financial relationships that could be construed as a potential conflict of interest.
Publisher’s note
All claims expressed in this article are solely those of the authors and do not necessarily represent those of their affiliated organizations, or those of the publisher, the editors and the reviewers. Any product that may be evaluated in this article, or claim that may be made by its manufacturer, is not guaranteed or endorsed by the publisher.
Supplementary material
The Supplementary Material for this article can be found online at: https://www.frontiersin.org/articles/10.3389/fphar.2022.1039235/full#supplementary-material
References
Abbas, Y. M., Wu, D., Bueler, S. A., Robinson, C. V., and Rubinstein, J. L. (2020). Structure of V-ATPase from the mammalian brain. Science 367 (6483), 1240–1246. doi:10.1126/science.aaz2924
Balsa, E., Marco, R., Perales, C. E., Szklarczyk, R., Calvo, E., Landázuri, M. O., et al. (2012). NDUFA4 is a subunit of complex IV of the mammalian electron transport chain. Cell Metab. 16 (3), 378–386. doi:10.1016/j.cmet.2012.07.015
Bratic, A., and Larsson, N. G. (2013). The role of mitochondria in aging. J. Clin. Invest. 123 (3), 951–957. doi:10.1172/jci64125
Bugelski, P. J., Atif, U., Molton, S., Toeg, I., Lord, P. G., and Morgan, D. G. (2000). A strategy for primary high throughput cytotoxicity screening in pharmaceutical toxicology. Pharm. Res. 17 (10), 1265–1272. doi:10.1023/a:1026495503939
Cai, Y., Ma, W., Xiao, Y., Wu, B., Li, X., Liu, F., et al. (2017). High doses of baicalin induces kidney injury and fibrosis through regulating TGF-β/Smad signaling pathway. Toxicol. Appl. Pharmacol. 333, 1–9. doi:10.1016/j.taap.2017.08.003
Chan, S. S. L. (2017). Inherited mitochondrial genomic instability and chemical exposures. Toxicology 391, 75–83. doi:10.1016/j.tox.2017.07.014
Chen, L., Titch, T., Luo, Z., Xu, Y., Li, X., Huang, F., et al. (2012). Confirmation of a proarrhythmic risk underlying the clinical use of common Chinese herbal intravenous injections. J. Ethnopharmacol. 142 (3), 829–835. doi:10.1016/j.jep.2012.06.008
Chen, Y. Y., Xie, Y. M., Liao, X., and Chen, H. Y. (2016). Systematic review of medication safety of Xiyanping injection in conformity with indications of package inserts. Zhongguo Zhong Yao Za Zhi 41 (18), 3463–3472. doi:10.4268/cjcmm20161824
Chen, S., Kwong, J. S. W., Zheng, R., Wang, Y., and Shang, H. (2018). Normative application of xiyanping injection: A systematic review of adverse case reports. Evid. Based. Complement. Altern. Med. 2018, 4013912. doi:10.1155/2018/4013912
Chengfeng, J. M., Xie, Y. M., Wang, L. X., Li, Y. Y., and Yang, X. C. (2020). Real world study on the influencing factors of breviscapine injection suspected allergic reaction. J. Tradit. Chin. Med. 61 (24), 2174–2178. doi:10.13288/j.11-2166/r.2020.24.012
Chong, L., Chen, W., Luo, Y., and Jiang, Z. (2013). Simultaneous determination of 9-dehydro-17-hydro-andrographolide and sodium 9-dehydro-17-hydro-andrographolide-19-yl sulfate in rat plasma by UHPLC-ESI-MS/MS after administration of xiyanping injection: application to a pharmacokinetic study. Biomed. Chromatogr. 27 (7), 825–830. doi:10.1002/bmc.2866
Commission, C. P. (2020). Pharmacopoeia of people’s Republic of China. Beijing, China: China Medical Science Press.
De Souza Silva, J. E., Santos Souza, C. A., Da Silva, T. B., Gomes, I. A., Brito Gde, C., De Souza Araújo, A. A., et al. (2014). Use of herbal medicines by elderly patients: A systematic review. Arch. Gerontol. Geriatr. 59 (2), 227–233. doi:10.1016/j.archger.2014.06.002
Deng, Y. Y., Hu, Z. M., Zhou, Y. L., Chen, S. Z., Liu, J. P., and Zhang, E. H. (2017). Determination of specific antibodies in serum of rabbits sensitized by baicalin in shuanghuanglian injection. Hebei J. Tradit. Chin. Med. 39 (6), 880–883. doi:10.3969/j.issn.1002-2619.2017.06.019
Drug Evaluation Center (2018). Annual drug approval report. Available at: https://www.nmpa.gov.cn/xxgk/fgwj/gzwj/gzwjyp/20190701175801236.html (Accessed July 01, 2019).
Feng, X., Fang, S. N., Gao, Y. X., Liu, J. P., and Chen, W. (2018). Current research situation of nephrotoxicity of Chinese herbal medicine. Chin. J. Chin. Mater Med. 43 (3), 417–424. doi:10.19540/j.cnki.cjcmm.2018.0009
Feng, C. Y., Zhao, L., and Liu, D. (2022). 385 cases of adverse drug reactions/adverse drug events caused by Yinzhihuang preparations. Chin. J. Pharmacovigil. 19 (3), 325–327. doi:10.19803/j.1672-8629.2022.03.21
Gao, N., Gao, Y., Tian, F. Q., and Qiao, H. L. (2014). Sensitization of baicalin in guinea pigs and its possible mechanism. Chin. J. Pharmacol. Toxicol. 28 (6), 857–862. doi:10.3867/j.issn.1000-3002.2014.06.007
Gao, Y., Hou, R., Han, Y., Fei, Q., Cai, R., and Qi, Y. (2018). Shuang-Huang-Lian injection induces an immediate hypersensitivity reaction via C5a but not IgE. Sci. Rep. 8 (1), 3572. doi:10.1038/s41598-018-21843-7
Gao, Y., Liang, A., Fan, X., Hu, L., Hao, F., and Li, Y. (2019). Safety research in traditional Chinese medicine: Methods, applications, and outlook. Engineering 5 (1), 76–82. doi:10.1016/j.eng.2018.11.019
Gu, Y. Y., Shi, L., Zhang, D. D., Huang, X., and Chen, D. Z. (2018). Metabonomics delineates allergic reactions induced by Shuang-huang-lian injection in rats using ultra performance liquid chromatography-mass spectrometry. Chin. J. Nat. Med. 16 (8), 628–640. doi:10.1016/s1875-5364(18)30101-8
Guo, T., and Li, Y. Y. (2012). Progresses on pharmacological and toxicological effects of Dengzhanxixin injection. Chin. J. Chin. Mater Med. 37 (18), 2820–2823.
Han, Z., Lei, X. L., Zhang, H., Liu, L., Chen, Z. S., Yang, W., et al. (2017). Evaluating the safety of forsythin from forsythia suspensa leaves by acute and sub-chronic oral administration in rodent models. Asian pac. J. Trop. Med. 10 (1), 47–51. doi:10.1016/j.apjtm.2016.10.011
Han, J., Zhao, Y., Zhang, Y., Li, C., Yi, Y., Pan, C., et al. (2018). RhoA/ROCK signaling pathway mediates shuanghuanglian injection-induced pseudo-allergic reactions. Front. Pharmacol. 9, 87. doi:10.3389/fphar.2018.00087
Han, Z., Guo, J., Meng, F., Liao, H., Deng, Y., Huang, Y., et al. (2021). Genetic toxicology and safety pharmacological evaluation of forsythin. Evid. Based. Complement. Altern. Med. 2021, 6610793. doi:10.1155/2021/6610793
Haouzi, D., Lekéhal, M., Moreau, A., Moulis, C., Feldmann, G., Robin, M. A., et al. (2000). Cytochrome P450-generated reactive metabolites cause mitochondrial permeability transition, caspase activation, and apoptosis in rat hepatocytes. Hepatology 32 (2), 303–311. doi:10.1053/jhep.2000.9034
He, J., and Wei, Z. Q. (2017). Literature analysis of adverse reactions of Xiyanping injection of Chinese patent medicine. World Latest Med. Inf. 17 (28), 165–168. doi:10.3969/j.issn.1671-3141.2017.28.116
He, Z. H., Wang, Q., Liu, M., Wu, T., and Ouyang, D. W. (2021). Research progress on quality control of traditional Chinese medicine injection. Chin. J. Pharmacovigil. 52 (05), 599–610. doi:10.16522/j.cnki.cjph.2021.05.002
Hemmerich, J., Troger, F., Füzi, B., and Ecker, G. F. (2020). Using machine learning methods and structural alerts for prediction of mitochondrial toxicity. Mol. Inf. 39 (5), e2000005. doi:10.1002/minf.202000005
Hu, D., Wu, C. Q., Li, Z. J., Liu, Y., Fan, X., Wang, Q. J., et al. (2015). Characterizing the mechanism of thiazolidinedione-induced hepatotoxicity: An in vitro model in mitochondria. Toxicol. Appl. Pharmacol. 284 (2), 134–141. doi:10.1016/j.taap.2015.02.018
Huang, J., Du, J., Lin, W., Long, Z., Zhang, N., Huang, X., et al. (2019). Regulation of lactate production through p53/β-enolase axis contributes to statin-associated muscle symptoms. EBioMedicine 45, 251–260. doi:10.1016/j.ebiom.2019.06.003
Huang, X. M., Huang, J. J., Du, J. J., Zhang, N., Long, Z., Yang, Y., et al. (2021). Autophagy inhibitors increase the susceptibility of KRAS-mutant human colorectal cancer cells to a combined treatment of 2-deoxy-D-glucose and lovastatin. Acta Pharmacol. Sin. 42 (11), 1875–1887. doi:10.1038/s41401-021-00612-9
Imaizumi, N., Kwang Lee, K., Zhang, C., and Boelsterli, U. A. (2015). Mechanisms of cell death pathway activation following drug-induced inhibition of mitochondrial complex I. Redox Biol. 4, 279–288. doi:10.1016/j.redox.2015.01.005
Ji, L., Chen, Y., Liu, T., and Wang, Z. (2008). Involvement of Bcl-xL degradation and mitochondrial-mediated apoptotic pathway in pyrrolizidine alkaloids-induced apoptosis in hepatocytes. Toxicol. Appl. Pharmacol. 231 (3), 393–400. doi:10.1016/j.taap.2008.05.015
Ji, L., Liu, T., and Wang, Z. (2010). Protection of epidermal growth factor against clivorine-induced mitochondrial-mediated apoptosis in hepatocytes. Environ. Toxicol. 25 (3), 304–309. doi:10.1002/tox.20500
Jiang, H., Zhang, Y., Liu, Z., Wang, X., He, J., and Jin, H. (2022). Advanced applications of mass spectrometry imaging technology in quality control and safety assessments of traditional Chinese medicines. J. Ethnopharmacol. 284, 114760. doi:10.1016/j.jep.2021.114760
Kamalian, L., Chadwick, A. E., Bayliss, M., French, N. S., Monshouwer, M., Snoeys, J., et al. (2015). The utility of HepG2 cells to identify direct mitochondrial dysfunction in the absence of cell death. Toxicol. Vitro 29 (4), 732–740. doi:10.1016/j.tiv.2015.02.011
Lee, A. Y., Jang, Y., Hong, S. H., Chang, S. H., Park, S., Kim, S., et al. (2017). Ephedrine-induced mitophagy via oxidative stress in human hepatic stellate cells. J. Toxicol. Sci. 42 (4), 461–473. doi:10.2131/jts.42.461
Li, H., Wang, S., Yue, Z., Ren, X., and Xia, J. (2018). Traditional Chinese herbal injection: Current status and future perspectives. Fitoterapia 129, 249–256. doi:10.1016/j.fitote.2018.07.009
Li, H., Deng, J., Deng, L., Ren, X., and Xia, J. (2019). Safety profile of traditional Chinese herbal injection: An analysis of a spontaneous reporting system in China. Pharmacoepidemiol. Drug Saf. 28 (7), 1002–1013. doi:10.1002/pds.4805
Loureiro Damasceno, J. P., Silva da Rosa, H., Silva de Araújo, L., and Jacometti Cardoso Furtado, N. A. (2022). Andrographis paniculata formulations: Impact on diterpene lactone oral bioavailability. Eur. J. Drug Metab. Pharmacokinet. 47 (1), 19–30. doi:10.1007/s13318-021-00736-7
Ma, S. C., But, P. P., Ooi, V. E., He, Y. H., Lee, S. H., Lee, S. F., et al. (2001). Antiviral amentoflavone from selaginella sinensis. Biol. Pharm. Bull. 24 (3), 311–312. doi:10.1248/bpb.24.311
Melamed-Gal, S., Loupe, P., Timan, B., Weinstein, V., Kolitz, S., Zhang, J., et al. (2018). Physicochemical, biological, functional and toxicological characterization of the European follow-on glatiramer acetate product as compared with Copaxone. eNeurologicalSci 13, 53–55. doi:10.1016/j.ensci.2018.11.007
Meyer, J. N., and Chan, S. S. L. (2017). Sources, mechanisms, and consequences of chemical-induced mitochondrial toxicity. Toxicology 391, 2–4. doi:10.1016/j.tox.2017.06.002
Meyer, J. N., Hartman, J. H., and Mello, D. F. (2018). Mitochondrial toxicity. Toxicol. Sci. 162 (1), 15–23. doi:10.1093/toxsci/kfy008
Mihajlovic, M., and Vinken, M. (2022). Mitochondria as the target of hepatotoxicity and drug-induced liver injury: molecular mechanisms and detection methods. Int. J. Mol. Sci. 23 (6), 3315. doi:10.3390/ijms23063315
Mottis, A., Herzig, S., and Auwerx, J. (2019). Mitocellular communication: Shaping health and disease. Science 366 (6467), 827–832. doi:10.1126/science.aax3768
Murai, M., and Miyoshi, H. (2016). Current topics on inhibitors of respiratory complex I. Biochim. Biophys. Acta 1857 (7), 884–891. doi:10.1016/j.bbabio.2015.11.009
National Center for ADR Monitoring (2005). Notification on adverse drug reactions (Phase 1). Available at: https://www.cdr-adr.org.cn/drug_1/aqjs_1/drug_aqjs_xxtb/200806/t20080626_36883.html (Accessed May 31, 2005).
National Center for ADR Monitoring (2009). Notification on adverse drug reactions (Phase 22)Available at: . https://www.cdr-adr.org.cn/drug_1/aqjs_1/drug_aqjs_xxtb/200905/t20090521_36870.html (Accessed May 21, 2009).
National Center for ADR Monitoring (2012). Notification on adverse drug reactions (Phase 48). Available at: https://www.cdr-adr.org.cn/drug_1/aqjs_1/drug_aqjs_xxtb/201206/t20120628_36843.html (Accessed June 28, 2012).
National Center for ADR Monitoring (2015-2019). Annual report for national adverse drug reaction monitoring. Available at: https://www.nmpa.gov.cn/xxgk/yjjsh/ypblfytb/20200413094901811.html (Accessed April 13, 2020).
National Medical Products Administration (2016). Notice of the general administration on the revision of the instructions for Yinzhihuang injection. Available at: https://www.nmpa.gov.cn/xxgk/ggtg/ypshmshxdgg/20160831171101146.html (Accessed Auguest 31, 2016).
Niyazov, D. M., Kahler, S. G., and Frye, R. E. (2016). Primary mitochondrial disease and secondary mitochondrial dysfunction: Importance of distinction for diagnosis and treatment. Mol. Syndromol. 7 (3), 122–137. doi:10.1159/000446586
Orlicka-Płocka, M., Gurda-Wozna, D., Fedoruk-Wyszomirska, A., and Wyszko, E. (2020). Circumventing the crabtree effect: forcing oxidative phosphorylation (OXPHOS) via galactose medium increases sensitivity of HepG2 cells to the purine derivative kinetin riboside. Apoptosis 25 (11-12), 835–852. doi:10.1007/s10495-020-01637-x
Osellame, L. D., Blacker, T. S., and Duchen, M. R. (2012). Cellular and molecular mechanisms of mitochondrial function. Best. Pract. Res. Clin. Endocrinol. Metab. 26 (6), 711–723. doi:10.1016/j.beem.2012.05.003
Rana, P., Aleo, M. D., Gosink, M., and Will, Y. (2019). Evaluation of in vitro mitochondrial toxicity assays and physicochemical properties for prediction of organ toxicity using 228 pharmaceutical drugs. Chem. Res. Toxicol. 32 (1), 156–167. doi:10.1021/acs.chemrestox.8b00246
Roubicek, D. A., and Souza-Pinto, N. C. d. (2017). Mitochondria and mitochondrial DNA as relevant targets for environmental contaminants. Toxicology 391, 100–108. doi:10.1016/j.tox.2017.06.012
Sanz-Serrano, J., Vettorazzi, A., Muruzabal, D., López de Cerain, A., and Azqueta, A. (2021). In vitro genotoxicity assessment of functional ingredients: DHA, rutin and α-tocopherol. Food Chem. Toxicol. 153, 112237. doi:10.1016/j.fct.2021.112237
Schoonen, W. G., de Roos, J. A., Westerink, W. M., and Débiton, E. (2005). Cytotoxic effects of 110 reference compounds on HepG2 cells and for 60 compounds on HeLa, ECC-1 and CHO cells. II mechanistic assays on NAD(P)H, ATP and DNA contents. Toxicol. Vitro 19 (4), 491–503. doi:10.1016/j.tiv.2005.01.002
Shii, T., Kuroda, M., Shamoto, N., and Mimaki, Y. (2020). An analysis of the ingredients in decoctions and extracts of Kampo medicines: Amounts of baicalin and baicalein in Kampo medicines containing Scutellariae Radix. Nihon Ronen Igakkai Zasshi. 57 (1), 72–80. doi:10.3143/geriatrics.57.72
Stoker, M. L., Newport, E., Hulit, J. C., West, A. P., and Morten, K. J. (2019). Impact of pharmacological agents on mitochondrial function: a growing opportunity? Biochem. Soc. Trans. 47 (6), 1757–1772. doi:10.1042/bst20190280
Sun, F., Huo, X., Zhai, Y., Wang, A., Xu, J., Su, D., et al. (2005). Crystal structure of mitochondrial respiratory membrane protein complex II. Cell 121 (7), 1043–1057. doi:10.1016/j.cell.2005.05.025
Sung, H. J., Ma, W., Wang, P. Y., Hynes, J., O'Riordan, T. C., Combs, C. A., et al. (2010). Mitochondrial respiration protects against oxygen-associated DNA damage. Nat. Commun. 1, 5. doi:10.1038/ncomms1003
Swiss, R., and Will, Y. (2011). Assessment of mitochondrial toxicity in HepG2 cells cultured in high-glucose- or galactose-containing media. Curr. Protoc. Toxicol. 2, Unit2.20–20. doi:10.1002/0471140856.tx0220s49
Tan, L., Li, M., and Lin, Y. (2019). Safety concerns of traditional Chinese medicine injections used in Chinese children. Evid. Based. Complement. Altern. Med. 2019, 8310368. doi:10.1155/2019/8310368
Troger, F., Delp, J., Funke, M., van der Stel, W., Colas, C., Leist, M., et al. (2020). Identification of mitochondrial toxicants by combined in silico and in vitro studies – a structure-based view on the adverse outcome pathway. Comput. Toxicol. 14, 100123. doi:10.1016/j.comtox.2020.100123
Tu, Y., Li, L., Wang, Z., and Yang, L. (2021). Advances in analytical techniques and quality control of traditional Chinese medicine injections. J. Pharm. Biomed. Anal. 206, 114353. doi:10.1016/j.jpba.2021.114353
Wang, L., Cheng, L., Yuan, Q., Cui, X., Shang, H., Zhang, B., et al. (2010a). Adverse drug reactions of shuanghuanglian injection: a systematic review of public literatures. J. Evid. Based. Med. 3 (1), 18–26. doi:10.1111/j.1756-5391.2010.01067.x
Wang, L., Yuan, Q., Marshall, G., Cui, X., Cheng, L., Li, Y., et al. (2010b). Adverse drug reactions and adverse events of 33 varieties of traditional Chinese medicine injections on national essential medicines list (2004 edition) of China: an overview on published literatures. J. Evid. Based. Med. 3 (2), 95–104. doi:10.1111/j.1756-5391.2010.01073.x
Wang, M., Liu, C. X., Dong, R. R., He, S., Liu, T. T., Zhao, T. C., et al. (2015). Safety evaluation of Chinese medicine injections with a cell imaging-based multiparametric assay revealed a critical involvement of mitochondrial function in hepatotoxicity. Evid. Based. Complement. Altern. Med. 2015, 379586. doi:10.1155/2015/379586
Wang, P. Y., Li, J., Walcott, F. L., Kang, J. G., Starost, M. F., Talagala, S. L., et al. (2017). Inhibiting mitochondrial respiration prevents cancer in a mouse model of Li-Fraumeni syndrome. J. Clin. Invest. 127 (1), 132–136. doi:10.1172/jci88668
Wang, J., Zhang, Y. J., Che, D. L., Zeng, Y. N., Wu, Y. Y., Qin, Q. H., et al. (2020). Baicalin induces Mrgprb2-dependent pseudo-allergy in mice. Immunol. Lett. 226, 55–61. doi:10.1016/j.imlet.2020.07.006
Wang, C., Pang, X., Zhu, T., Ma, S., Liang, Y., Zhang, Y., et al. (2022). Rapid discovery of potential ADR compounds from injection of total saponins from Panax notoginseng using data-independent acquisition untargeted metabolomics. Anal. Bioanal. Chem. 414 (2), 1081–1093. doi:10.1007/s00216-021-03734-5
West, A. P. (2017). Mitochondrial dysfunction as a trigger of innate immune responses and inflammation. Toxicology 391, 54–63. doi:10.1016/j.tox.2017.07.016
Will, Y., and Dykens, J. (2014). Mitochondrial toxicity assessment in industry--a decade of technology development and insight. Expert Opin. Drug Metab. Toxicol. 10 (8), 1061–1067. doi:10.1517/17425255.2014.939628
Will, Y., Shields, J. E., and Wallace, K. B. (2019). Drug-induced mitochondrial toxicity in the geriatric population: Challenges and future directions. Biol. (Basel) 8 (2), E32. doi:10.3390/biology8020032
Wilson, D. R. (2017). The potential health benefits of rutin. Available at: https://www.healthline.com/health/potential-benefits-of-rutin (Accessed September 1, 2017).
Wu, R., Liang, Y., Xu, M., Fu, K., Zhang, Y., Wu, L., et al. (2021). Advances in chemical constituents, clinical applications, pharmacology, pharmacokinetics and toxicology of erigeron breviscapus. Front. Pharmacol. 12, 656335. doi:10.3389/fphar.2021.656335
Xia, D., Esser, L., Tang, W. K., Zhou, F., Zhou, Y., Yu, L., et al. (2013). Structural analysis of cytochrome bc1 complexes: implications to the mechanism of function. Biochim. Biophys. Acta 1827 (11-12), 1278–1294. doi:10.1016/j.bbabio.2012.11.008
Xiao, P., Hou, L., Liu, Z., Mu, Y., Liu, Z., Wang, J., et al. (2020). Animal models for analysis of hypersensitivity reactions to Shuanghuanglian injection. Mol. Immunol. 122, 62–68. doi:10.1016/j.molimm.2020.03.014
Xie, L. P., and Ye, J. F. (2016). Adverse reactions and safety analysis of Yinzhihuang injection. J. Hunan Univ. Chin. Med. 403, 380. Available at: https://kns.cnki.net/kcms/detail/detail.aspx?FileName=HNZY201611001522&DbName=CPFD2017.
Xie, J. J., Zhang, L. B., Yi, J. J., Kang, L. J., and Zhao, L. (2019). Establishment of new evaluation standards for systemic anaphylactoid reactions using mouse model. Zhongguo Zhong Yao Za Zhi 44 (17), 3763–3772. doi:10.19540/j.cnki.cjcmm.20190422.401
Yang, Q. W., Li, Q., Zhang, J., Xu, Q., Yang, X., Li, Z. Y., et al. (2019). Crystal structure and anti-inflammatory and anaphylactic effects of andrographlide sulphonate E in Xiyanping, a traditional Chinese medicine injection. J. Pharm. Pharmacol. 71 (2), 251–259. doi:10.1111/jphp.13028
Yi, Y., Liang, A., Li, C., Zhao, Y., Wang, Y., and Li, G. (2012). Relationship between specific immunocyte and pseudoanaphylactoid reactions induced by Shuanghuanglian injection. Chin. J. Chin. Mater Med. 37 (13), 1898–1900. doi:10.4268/cjcmm20121308
Yu, S., Qin, X., and Li, Z. (2020). Quality assessment of Shuxuening injection based on widely targeted metabolomics approach. J. Pharm. Biomed. Anal. 189, 113398. doi:10.1016/j.jpba.2020.113398
Zell-Kanter, M., Quigley, M. A., and Leikin, J. B. (2015). Reduction in ephedra poisonings after FDA ban. N. Engl. J. Med. 372 (22), 2172–2174. doi:10.1056/NEJMc1502505
Zhang, W., Song, D. R., Wang, Y. L., and Zhu, Z. (2012). Evaluation of embryotoxicity of baicalin based on embryonic stem cell test system. Chin. J. Pharmacol. Toxicol. 26 (6), 864–869. doi:10.3867/j.issn.1000-3002.2012.06.015
Zhang, Q., Cai, C., Wang, P., Ding, N., Gao, J., Zhang, Y., et al. (2017). Baicalin and rutin are major constituents in Shuanghuanglian injection involving anaphylactoid reaction. J. Tradit. Chin. Med. 37 (3), 412–420. doi:10.1016/s0254-6272(17)30079-1
Zhang, Y., Zhang, X., Fan, S., Song, L., Yang, Z., Zhuang, P., et al. (2018). Desmoglein1 deficiency Is a potential cause of cutaneous eruptions induced by Shuanghuanglian injection. Molecules 23 (6), E1477. doi:10.3390/molecules23061477
Zhang, G. H., Lyu, J., Wu, X. W., Xie, Y. M., Sun, L. X., and Wang, H. (2021a). Effect of breviscapine for injection on liver function in the real world. Liaoning J. Tradit. Chin. Med. 48 (5), 145–150. doi:10.13192/j.issn.1000-1719.2021.05.039
Zhang, G. H., Sun, L. X., Wu, X. W., Xie, Y. M., Geng, H. J., and Yang, X. C. (2021b). Influencing factors of abnormal renal function of breviscapine for injection analyzed via nested case-control study combined with SMOTE algorithm. Chin. J. Pharmacovigil. 18 (12), 1150–1154. doi:10.19803/j.1672-8629.2021.12.11
Zhang, Y. Y., Bai, J., Xu, M. Y., Zhang, F., and Bo, C. X. (2021c). Study on baicalin on liver and kidney toxicity in male rats. Zhonghua Lao Dong Wei Sheng Zhi Ye Bing Za Zhi 39 (3), 169–172. doi:10.3760/cma.j.cn121094-20200326-00158
Zhang, Y. Y., Wang, C., Huang, H., Li, J., Bo, C. X., and F, Z. (2021d1626). Study on liver and kidney toxicity of baicalin in silicosis model rats. Occup. Health 37 (12), 1619–1621. doi:10.13329/j.cnki.zyyjk.2021.0404
Zhao, J., Zhang, Q., Zou, G., Gao, G., and Yue, Q. (2021a). Arenobufagin, isolated from toad venom, inhibited epithelial-to-mesenchymal transition and suppressed migration and invasion of lung cancer cells via targeting IKKβ/NFκB signal cascade. J. Ethnopharmacol. 265, 113313. doi:10.1016/j.jep.2020.113313
Zhao, P., Peng, Y., Xu, X., Wang, Z., Wu, Z., Li, W., et al. (2021b). In silico prediction of mitochondrial toxicity of chemicals using machine learning methods. J. Appl. Toxicol. 41 (10), 1518–1526. doi:10.1002/jat.4141
Zheng, D., Shao, J., Chen, W., and Luo, Y. (2016). In vitro metabolism of sodium 9-dehydro-17-hydro-andrographolide-19-yl sulfate in rat liver S9 by liquid chromatography-mass spectrometry method. Pharmacogn. Mag. 12, S102–S108. doi:10.4103/0973-1296.182194
Zheng, Q. Y. (2019). Analysis of adverse reactions of breviscapine for injection. Med J Thepresent Clin 32 (2), 172–175. doi:10.3969/j.issn.2095-9559.2019.02.048
Zhu, J., Vinothkumar, K. R., and Hirst, J. (2016). Structure of mammalian respiratory complex I. Nature 536 (7616), 354–358. doi:10.1038/nature19095
Keywords: TCM injections, mitochondrial toxicity, adverse drug reactions, glucose/galactose assay, HepG2 cells
Citation: Shen Y, Guo K, Ma A, Huang Z, Du J, Chen J, Lin Q, Wei C, Wang Z, Zhang F, Zhang J, Lin W, Feng N and Ma W (2022) Mitochondrial toxicity evaluation of traditional Chinese medicine injections with a dual in vitro approach. Front. Pharmacol. 13:1039235. doi: 10.3389/fphar.2022.1039235
Received: 07 September 2022; Accepted: 18 October 2022;
Published: 02 November 2022.
Edited by:
Albert P. Li, In Vitro ADMET Laboratories, United StatesReviewed by:
Guoshun Shan, Liaoning University of Traditional Chinese Medicine, ChinaYongshen Ren, South-Central University for Nationalities, China
Min Cao, Shanghai University of Traditional Chinese Medicine, China
Copyright © 2022 Shen, Guo, Ma, Huang, Du, Chen, Lin, Wei, Wang, Zhang, Zhang, Lin, Feng and Ma. This is an open-access article distributed under the terms of the Creative Commons Attribution License (CC BY). The use, distribution or reproduction in other forums is permitted, provided the original author(s) and the copyright owner(s) are credited and that the original publication in this journal is cited, in accordance with accepted academic practice. No use, distribution or reproduction is permitted which does not comply with these terms.
*Correspondence: Na Feng, wyuchemfn@126.com; Wenzhe Ma, wzma@must.edu.mo
†These authors have contributed equally to this work