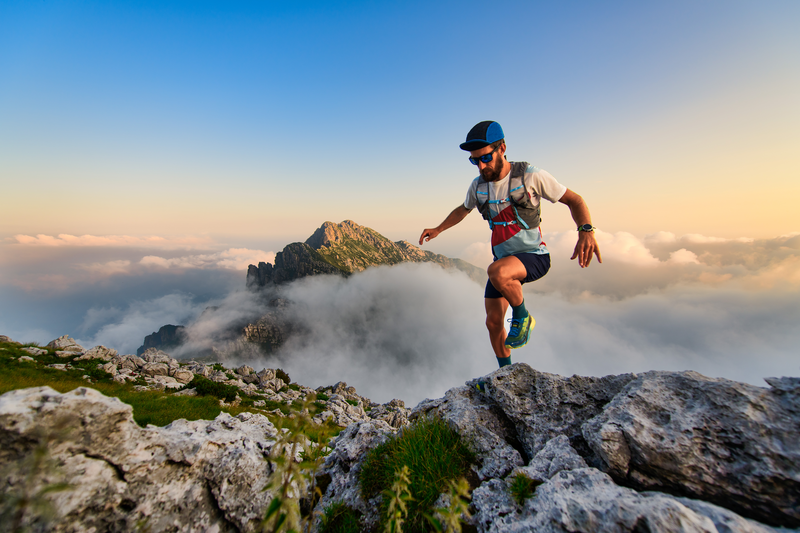
94% of researchers rate our articles as excellent or good
Learn more about the work of our research integrity team to safeguard the quality of each article we publish.
Find out more
REVIEW article
Front. Pharmacol. , 16 November 2022
Sec. Pharmacology of Anti-Cancer Drugs
Volume 13 - 2022 | https://doi.org/10.3389/fphar.2022.1036140
This article is part of the Research Topic The Roles of Ion-Induced Cell Death in Cancer Treatment View all 19 articles
Ferroptosis is referred as a novel type of cell death discovered in recent years with the feature of the accumulation of iron-dependent lipid reactive oxygen species. Breast cancer is one of the most common malignant cancers in women. There is increasing evidence that ferroptosis can inhibit breast cancer cell growth, improve the sensitivity of chemotherapy and radiotherapy and inhibit distant metastases. Therefore, ferroptosis can be regarded a new target for tumor suppression and may expand the landscape of clinical treatment of breast cancer. This review highlights the ferroptosis mechanism and its potential role in breast cancer treatment to explore new therapeutic strategies of breast cancer.
Ferroptosis is a kind of regulated cell death (RCD) characterized by iron dependent-mechanisms and excessive lipid peroxidation (Stockwell et al., 2017). It participates in tumor progression and disorders of the nervous system and cardiovascular system (Tang et al., 2021). However, despite breakthroughs in cancer treatment, the therapeutic response of cancer is limited by the acquired and intrinsic resistance of the tumor to apoptosis. Therefore, targeting non-apoptotic cell death offers a new option for cancer treatment (Hanahan and Weinberg, 2011). Unique metabolism, high levels of reactive oxygen species (ROS), and related gene mutations confer cancer cells inherently prone to the ferroptosis, while other cancer cells appear to rely particularly on the defense system of ferroptosis to survive under metabolic and oxidative stress conditions (Chen X. et al., 2021). Therefore, the destruction of these defense systems will cause fatal damage to these cancer cells, while normal cells will not be affected. Ferroptosis is also considered an important cell death mechanism caused by a number of therapies, including chemotherapy, radiotherapy (RT), targeted therapy and immunotherapy (Hassannia et al., 2019). It is also found that ferroptosis plays a controversial role in immunogenic cell death (ICD). The tumor cells with ferroptosis could diminish anti-tumor immune response by inhibiting the antigen presenting cells (Sacco et al., 2021; Wiernicki et al., 2022). However, the ferroptosis inducers (FINS) could enhance the efficacy of conventional treatment has been regarded as the potential drugs in cancer treatment (Mou et al., 2019). Breast cancer is currently the tumor with the highest incidence rate in the world (Duggan et al., 2021). The breast is in a unique microenvironment with plentiful adipocytes infiltrating. Previous studies have shown that adipocytes can regulate fatty acid metabolism, enhance the invasion and metastasis of breast cancer. Ferroptosis has been shown to participate in the development and treatment of breast cancer. Studies have found that highly invasive triple negative breast cancer (TNBC) cells are susceptive to ferroptosis and are particularly vulnerable to FINs. The susceptibility is attributed to several metabolic characteristics including high polyunsaturated fatty acids (PUFA) levels, expanded liable iron pool, and weakened Glutathione Peroxidase 4 (GPX4)-glutathione (GSH) defense system. The ferroptosis induced by the drugs in breast cancer is a potential and valuable research direction, which indicates that the it is an attractive target for the treatment of “refractory” tumors (Sui et al., 2022). We summarize the mechanisms of ferroptosis and explore its prospective on breast cancer in the review, which hopefully will provide novel strategies to improve the therapeutic response of breast cancer.
Ferroptosis is characterized by serious damage to mitochondrial morphology including volume shrinkage, condensed bilayer membrane density and ballooning phenotype (a clear, rounded cell consisting mainly of empty cytosol) (Battaglia et al., 2022). Ferroptosis is the result of antagonism of its prerequisites (drivers) and defense systems (Dixon et al., 2012). Prerequisites for ferroptosis include iron metabolism, mitochondrial metabolism, synthesis of polyunsaturated fatty acid phospholipid (PUFA-PL) and lipid peroxidation (Tang and Kroemer, 2020). The ferroptosis defense system mainly includes the GPX4/GSH system, FSP1-CoQH2 system, DHODH-CoQH2 system and GCH1-BH4 system. Ferroptosis occurs when the drivers of ferroptosis significantly exceed the detoxification capabilities of the defense system. When the dysregulation of iron hemostasis induce free iron increasing in cells, ROS is catalyzed by free iron through Fenton reaction, thus promotes lipid peroxidation, accumulates lipid peroxides and ultimately induces ferroptosis. System Xc–, the cystine/glutamate reverse transporter, participates in the GSH synthesis. GPX4 utilizes GSH as substrate to reduce lipid peroxides, decrease the accumulation of lipid peroxides, and inhibit the occurrence of ferroptosis (Lei et al., 2022); (Figure 1).
FIGURE 1. Molecular mechanisms of ferroptosis in breast cancer. lipid peroxidation leads to ferroptosis. PUFA is catalyzed by LPCAT3, ACSL4 and LOX to generate PUFA-PLOOH, which induces ferroptosis. In addition, PUFAs also promote ferroptosis through POR-mediated conversion to lipid peroxides. The MVA pathway suppresses ROS by regulating Sec-tRNA and CoQ10. The iron mediates ferroptosis. FPN regulates the intracellular iron level by converting Fe2+ to extracellular iron. TF binds to the TFR to convert Fe3+ to Fe2+ and enter cells. Free Fe2+ then induces the lipid ROS production by Fenton reaction.
Increased intracellular iron induces ROS production through the Fenton reaction, implying that iron overload may be an activator of ferroptosis. Ferroptosis sensitivity can be improved by increased iron uptake, reduced iron consumption and impaired cellular iron export (Liu et al., 2020). The iron is mainly stored in ferritin in the form of bound iron. Ferritinophagy releases the iron from ferritin into the liable iron pool which induce the ferroptosis. Tranferrin (TF) binds to the Tranferrin receptor to convert Fe3+ to Fe2+. Ferroportin (FPN) is required for transferring excess Fe2+ out of cells. FPN knocking out can induced ferroptosis by iron overload. FPN is lower in breast cancer patients compared with healthy people, which has great impact on the progression of breast cancer (Pinnix et al., 2010).
FINs can promote the production of mitochondrial ROS (MitoROS) to make mitochondria disappearance and ATP depletion by the unfolding of mitochondrial permeability transition pore (MPTP). MitoROS plays a positive feedback role in the process of mitochondrial homeostasis and ferroptosis. The impairment of CDGSH Iron Sulfur Domain 2 (CISD2) function in breast cancer can induce the destruction of mitochondrial labile iron pool, which improve the mitochondrial ROS level, thus leading to the activation of ferroptosis (Karmi et al., 2021).
PUFAs can be oxidized by ROS to produce unstable lipid hydroperoxide (LOH/LOOH) and lipid peroxide. Studies have shown that with the increased intake of PUFAs in diet, the level of ferroptosis-related genes such as GPX4, SLC7A11, DHFR and FSP1 in tumor cells has changed, which can promote the lipid peroxidation of cancer cells in the acidic tumor microenvironment, thus induce the ferroptosis in cancer cells (Dierge et al., 2021). ACSL4 and LPCAT3 are two key enzymes in the formation of lipid peroxides. Some studies have found that the level of lipid oxidation in breast cancer plasma and tumor tissue is negatively related to the survival. The expression of ACSL4 in TNBC is related to the sensitivity to ferroptosis. Studies have shown that ACSL4 is underlying enriched in basal-like subtypes and regulate the enrichment of long polyunsaturated–ω-6 fatty acids on cell membrane to inpede the ferroptosis (Doll et al., 2017). Some lipoxygenases (LOXs) are non heme dioxygenases which can oxidize the PUFAs to trigger the ferroptosis. The serum ALOX5 level in breast cancer patients was two times higher than that in the healthy people. It was also found that ALOX5 is positively correlated with tumor stage of breast cancer which could accelerate the growth of breast cancer cells (Kumar et al., 2016). Recent studies suggest that cytochrome P450 oxidoreductase (POR) also facilitates the lipid peroxidation (Zou et al., 2020).
Lipid oxidation is strictly regulated by the antioxidant defense system. GPX4 is considered to be a vital antioxidant enzyme, which directly eliminates hydroperoxides in lipid bilayers, thus preventing lethal lipid ROS production (Yang et al., 2014). GSH/GPX4 pathway, FSP1/COQ10/NADPH pathway, DHODH pathway and GCH1/BH4 pathway are identified as defense system in ferroptosis.
GSH/GPX4 pathway includes cystine (Cys) import through System Xc-, the production of cysteine via the transsulfuration pathway, and production of selenocysteine through the mevalonate pathway (Ingold et al., 2018). ROS neutralization is catalyzed by GSH during the occurrence of ferroptosis. Selective anti-GSH biosynthesis can be considered to target the metabolic vulnerability ER + breast cancer (Xiong et al., 2019). GSH is found in higher level in tumor lesion than in noncancerous tissue and has been shown to increase the risk of breast cancer (Mehdi et al., 2018). When system Xc- is prohibited, it prevents cystine from entering the cells and blocking GSH synthesis, leading to the inactivation of GPX4, resulting in the accumulation of Lip-ROS (Lee et al., 2021). The subtypes of TNBC have different characteristics of ferroptosis. Luminal androgen receptor (LAR) subtype is the most vulnerable to the ferroptosis treatment. The GPX4 which is driven by androgen receptor is a key factor of ferroptosis in LAR subtype breast cancer. The GPX4 inhibitor not only suppress the proliferation but also reshape the tumor microenvironment of LAR subtype (Yang F. et al., 2022).
Ferroptosis suppressor protein 1 (FSP1), a suppressor of ferroptosis, acts as a NADH-dependent coenzyme Q oxidoreductase (Doll et al., 2019). Coenzyme Q 10 (CoQ10) is generated in the mitochondrial electron transport chain by the mevalonate (MVA) pathway, which restrain the mitochondria. 3-hydroxy-methylglutaryl-CoA reductase (HMGCR) is the enzyme that catalyzes HMG-CoA to mevalonate, which increases GPX4 biosynthesis and CoQ10 (Shimada et al., 2016). The inhibition of ferroptosis by FSP1 is mediated by CoQ10. The reduced form of CoQ10 captures lipid peroxidative radicals, while FSP1 catalyzes CoQ10 by using NAD(P)H. The FSP1-CoQ10-NAD(P)H pathway is independent and cooperates with the GSH/GPX4 pathway to defense ferroptosis (Bersuker et al., 2019). Statins can inhibit HMGCR and reduce FSP1-mediated ferroptosis resistance which could be considered as a potential drug for the treatment of TNBC (Yao et al., 2021).
Dihydrolactate dehydrogenase (DHODH) is located in the inner membrane of mitochondria which is a flavin-dependent enzyme. The DHODH is mainly to catalyze pyrimidine nucleotide synthesis pathway. The electrons are transferred in the mitochondria membrane to make ubiquinone convert to dihydroubiquinone, which induces resistance to ferroptosis in mitochondria. It is suggested that DHODH may regulate ferroptosis independently of the pyrimidine nucleotide synthesis function (Mao et al., 2021). Moreover, the DHODH inhibition could reduce the production of ATP and endogenous ROS and promote the cell cycle arrest in S phase of breast cancer cells (Mohamad Fairus et al., 2017).
Guanosine triphosphate cyclohydrolase 1 (GCH1) is a rate-limiting enzyme for tetrahydrobiopterin BH4 synthesis to inhibit ferroptosis independently of GPX4. BH4 is an effective free radical trapping antioxidant that can prevent lipid peroxidation and can be regenerated by dihydrofolate reductase (DHFR). The inhibition of DHFR cooperates with GPX4 deletion could induce ferroptosis (Kraft et al., 2020). GCH1 promotes the immunosuppression of TNBC by regulating tryptophan metabolism (Wei et al., 2021). Further studies are needed to clarify the molecular mechanism of GCH1/BH4 in ferroptosis defense of breast cancer.
Sufficient intracellular iron is a necessary condition for ferroptosis (Pinnix et al., 2010). A recent study showed that compared with non TNBC tumor, iron-regulatory genes are significantly overexpressed in TNBC (Table 1). In particular, a large number of low-level iron export transporters are observed in TNBC, accompanied by high-level expression of iron import transferrin receptors. A European randomized controlled study showed there was a positive association between serum transferrin level and the incidence of ER- breast cancer (Hou et al., 2021). A Canadian cohort study showed that dietary iron supplementation was associated with a low risk of breast cancer, and heme iron was positively related with the risk of the ER and or PR positive breast cancer subtypes in postmenopausal women (Chang et al., 2020). High ferroportin and hepcidin expression play a protective role in patients with breast cancer (Pinnix et al., 2010). Transferrin receptor 1 (TFR1), an independent prognostic factor, is positively related to the amounts of immune cells (CD8+ T cells, CD4+ T cells, neutrophils, B cells, macrophages, and dendritic cells) in breast cancer patients (Chen F. et al., 2021).
Ferroptosis-related genes (FRGs) are found to be promising targets for the treatment of breast cancer. Two subgroups, a high-risk group and a low-risk group, can be stratified by nine FRGs, namely SQLE, G6PD, ALDH3A2, SLC1A4, CHAC1, SIAH2, FLT3, EGLN2, and SFXN5, which are associated with histological type, grade, stage, Her-2 status, and subtype of breast cancer. A high-risk subgroup of patients with positive or negative CTLA4 and PD-1 status demonstrated a significant benefit of combined anti-CTLA4 and anti-PD-1 therapy (Li P. et al., 2021). This new genome panel consisting of nine FRGs which are identified to predict the prognosis and immune status of breast cancer patients. The high expression of ferroptosis-related gene aldo-keto reductase family 1member C1 (AKR1C1) was associated with better survival and various immune infiltrating cells amount in breast cancer (Zhang et al., 2021d). Changes in the genes expression involved in the regulation of iron metabolism may help increase the liable iron pool of cells, promote iron-dependent lipid peroxidation, and make TNBC an iron-enriched tumor prone to ferroptosis. Iron overload leads to the reactivation of oxygen components, lipid peroxidation, and DNA damage. Recently, it has been shown that high iron level of the inflammatory microenvironment may promote the progression and metastasis of breast cancer. Heme oxygenase (HO) level was found to have great impact for the onset of ferroptosis in TNBC (Consoli et al., 2022). MYC increases ADHFE1 and induces iron overload in breast cancer, resulting in poor survival (Mishra et al., 2018). Ferroptosis has been shown to be involved in breast cancer brain metastases, HOMX1, LPCAT3, PEBP1, and KEAP1, and are associated with relapse and overall survival. The ferroptosis score is associated with iron metabolism, interstitial and immune cell numbers, and iron mortality indicators, which can be used as prognostic markers (Zhu et al., 2021). Taken together, these findings indicates that multiple iron-regulatory genes are related with the survival of breast cancer.
Several cell signaling pathways and molecules can directly or indirectly act on key factors that initiate or regulate ferroptosis, thus positively or negatively regulating ferroptosis (Figure 2).
FIGURE 2. The diagram of cell signaling pathways and molecules of ferroptosis in breast cancer. SUFU deletion increase the ferroptosis by activated by RSL3 via ACSL4. PKCβII activate ACSL4 to induce lipid peroxidation and improves the efficacy of immunotherapy. DKK1 inhibit their stem cell properties. Because CSCs are highly sensitive to ferroptosis, DKK1 induces SLC7A11 increasing and promote the development of metastase og CSC. DMOCPTL inhibit GPX4 to induce ferroptosis by EGR-1. 27HC exposure induce the overexpression of GPX4 to inhibit ferroptosis and increase the proliferation and metastasis. The upregulation of DDR2 elicits ferroptosis to recurrent breast cancer through the Hippo pathway. EMT induce DDR2 upregulation to active ferroptodis by regulating YAP/TAZ. GSK-3 β promoting erastin-induced ferroptosis by blocking Nrf2. p53 reduces the expression of SLC7A11 by inhibiting H2Bub1. FGFR4 suppress GSH synthesis and efflux of Fe2+ through β-catenin/TCF4/SLC7A1 signaling pathway.
Nuclear factor erythroid2-related factor 2 (Nrf2) can regulate ferroptosis through p62-Keap1-NRF2 pathway. The activation of p62-Keap1-NRF2 signal pathway can also promote the expression of System Xc-, accelerate the transportation of cystine/glutamic acid, and thus eliminate the accumulated lipid peroxidation. The activation of Nrf2 promotes the iron storage, reduces the iron consumption, increasing the expression of target genes NADH Dehydrogenase Quinone 1 (NQO1) and HO-1 which are related to iron and ROS (Xu et al., 2019). Glycogen synthase kinase-3 β (GSK-3 β) promoting erastin-induced ferroptosis by blocking Nrf2 in breast cancer (Wu et al., 2020). Suppressor of fused homolog (SUFU) is a key element of the Hedgehog pathway (Fang et al., 2022). The deletion of SUFU can increase the ferroptosis of breast cancer cells activated by RSL3 by decreasing ACSL4. Vincristine can promote the RSL3 sensitivity of breast cancer cells by downregulating SUFU. Protein kinase C-βII (PKCβII) is activated by lipid peroxidation during ferroptosis, which can directly phosphorylate the Thr328 site of ACSL4 to activate ACSL4, strengthen the biosynthesis of PUFA-containing lipids, and induce lipid peroxidation. PKCβII induces ferroptosis and improves the response of immunotherapy (Zhang et al., 2022).
Recent studies have shown that cancer stem cells (CSC) are relatively enriched in lung metastases due to their high invasive properties, and enriched CSCs can secrete negative Dickkopf1 (DKK1) feedback to inhibit their stem cell properties. Because CSCs are highly sensitive to ferroptosis, DKK1 induces an increase in SLC7A11 expression and regulates inhibition of CSC properties and thus can protect lung metastatic cells from ferroptosis and promote the development of metastases. In multiple models of breast cancer metastasis, small-molecule inhibitors of DKK1 can almost completely block the occurrence of lung metastases (Wu M. et al., 2022). p53 induces ferroptosis by SLC7A11 (Magri et al., 2021). In fact, mutant p53 inhibits Nrf2-dependent SLC7A11 transcription to promote SLC7A11 expression (Clemons et al., 2017). In addition, p53 promotes the nuclear translocation of ubiquitin-specific protease (USP7) and reduces the expression of SLC7A11 by inhibiting H2B monoubiquitination (H2Bub1) (Wang Y. et al., 2019).
DMOCPTL can induce EGR1-mediated apoptosis and ferroptosis and in TNBC by GPX4 ubiquitination. Long-term exposure to the cholesterol metabolite 27-hydroxycholesterol (27HC) causes breast cancer cells to be resistant to 27HC and overexpress GPX4, which inhibits ferroptosis and increases tumorigenicity and tumor metastasis (Liu W. et al., 2021). Up-regulation of the discoidin domain receptor 2 (DDR2) elicits the susceptibility of ferroptosis by GSH consumption of recurrent breast cancer through the Hippo pathway. Epithelial mesenchymal transition-driven upregulation of DDR2 suppresses tumor proliferation in recurrent breast cancer by regulating YAP/TAZ-mediated ferroptosis (Lin et al., 2021). The inhibiting of Fibroblast Growth Factor Receptor 4 (FGFR4) mediated by m6A hypomethylation significantly suppress GSH synthesis and efflux of Fe2+ through β-catenin/TCF4-SLC7A11/FPN1 signaling pathway, which trigger the excessive production of ROS and abnormal iron pool accumulation in Her-2 positive breast cancer (Zou et al., 2022).
The tumor microenvironment (TME), especially immune cells, has an important impact on ferroptosis in cancer cells. Interferon-γ (IFN-γ) secreted by CD8+ cytotoxic T cells inhibits cystine uptake by cancer cells by downregulating SLC7A11, thus triggering lipid peroxidation and ferroptosis in various tumors (Wang W. et al., 2019). Tumor-associated macrophages (TAM) secrete transforming growth factor beta 2 (TGF-β2) to regulate liver leukemia factor (HLF). HLF inhibits ferroptosis by activating GGT1 and induces TNBC chemoresistance (Li H. et al., 2022). Adipocytes induce ferroptosis resistance in breast cancer by secreting specific fatty acids. Mono-unsaturated Fatty Acid (MUFA) regulates breast cancer cells by binding with acyl-CoA synthetase long chain family member 3 (ACSL3) to phospholipids, and it is confirmed that the regulation of ferroptosis in breast cancer by adipocytes and exogenous MUFA is dependent on ACSL3 (Xie et al., 2022).
Non-coding RNAs (ncRNAs) are regarded as novel regulators of various cellular processes in cancer (Zuo et al., 2022); (Figure 3). Some recent studies have shown that they participate in the molecular regulation of ferroptosis. The ectopic overexpression of SLC7A11 inhibits miR-5096-mediated ferroptosis and diminishes antitumor effects in breast cancer (Yadav et al., 2021). PGM5P3-AS1 regulates MAP1LC3C to confer ferroptosis and thus inhibits the progression of TNBC (Qi et al., 2022). Circular RNA RHOT1 prohibits the occurrence of ferroptosis by targeting the miR-106a-5p/STAT3 signaling pathway and increases breast cancer proliferation (Zhang H. et al., 2021). CircGFRA1 facilitates progression of the malignant phenotype of Her-2 positive breast cancer by sponging with miR-1228 and improving FSP1 expression (Bazhabayi et al., 2021). Circ-BGN binds to OTUB1 and SLC7A11 and inhibits ferroptosis to confer resistance to trastuzumab in HER-2+ breast cancer (Wang S. et al., 2022). A integrity understanding of these regulatory network might facilicate the discovery of vulnerable therapeutic target of ferroptosis in breast cancer.
FIGURE 3. The diagram of cell signaling pathways of ncRNAs and ferroptosis in breast cancer. The overexpression of SLC7A11 inhibits miR-5096 to induce ferroptosis. PGM5P3-AS1 regulates MAP1LC3C to induce the occurrence of ferroptosis. CircRHOT1 inhibits ferroptosis by targeting the miR-106a-5p via STAT3. CircGFRA1 sponge with miR-1228 and improves FSP1 expression. CircBGN binds to OTUB1 and SLC7A1 and inhibits ferroptosis.
The development of cancer treatment strategies based on ferroptosis targets is actively in progress. Loading FINS through nanocarriers can effectively reduce the toxicity of drug delivery systems, overcome the drug resistance of cancer cells, and significantly improve the ferroptosis efficacy.
The demand of tumor cells for iron has increased significantly indicating that iron chelators may be an effective anti-cancer strategy iron chelators exert their anticancer potential by consuming intracellular iron, inhibiting DNA synthesis, inducing apoptosis, and oxidative stress. Furthermore, the reduction of intracellular iron through iron chelation can sensitize breast cancer cells to chemotherapy (Tury et al., 2018). For example, deferoxamine (DFO) and deferoxazole (DFX) show potential antitumor activity in breast cancer by limiting iron bioavailability. DFO promote iron uptake to activate TRF1 and divalent metal transporter 1 (DMT1) via the IL6/PI3K/AKT axis (Chen et al., 2019). The physiologically achievable concentration of DFO monotherapy significantly active the apoptosis of breast cancer cells and the xenotransplantation of mouse breast tumors by activating the tumor necrosis factor-α (TNF-α)/nuclear factor kappa B (NF-κB) axis and transform growth factor β (TGF-β). DFO promoted migration in the ROS-dependent NF-κB and TGF-β signaling pathway by enhancing mitochondrial iron accumulation in TNBC (Liu et al., 2016). Although preclinical studies have confirmed that iron chelating agents inhibit tumor growth and metastasis by certain signaling pathways. However, in clinical studies, iron chelating agents only show moderate therapeutic benefits, and may promote anti-tumor drug resistance due to ferroptosis. It is urgent to improve the bioavailability of iron chelating agents and combination therapy.
Some Chinese medicine and natural compounds have been shown to induce ferroptosis in breast cancer by targeting iron overload. Shuganning induces ferroptosis via Heme oxygenase-1(HO-1) to increase intracellular iron accumulation and suppresses tumor growth in TNBC (Du et al., 2021). Artemisinin is activated by Fe2+ to generate free radicals. The iron supplements can improve the anticancer efficacy of artemisinin. Artemisinin can induce ferroptosis by promoting ferritin phagocytosis and thus increasing intracellular iron levels (Chen et al., 2020). A completed phase I clinical trial (NCT00764036) showed that for patients with metastatic breast cancer, oral artemisinin of up to 200 mg per day was deemed safe and well tolerated (von Hagens et al., 2017).
Ferritinophagy serves as a link between ferroptosis and autophagy (Latunde-Dada, 2017). Ferritinophagy transports ferritin to the lysosome for degradation. The release of free iron leads to increased iron, and the Fenton reaction occurs to generate oxidative stress, which is essential for ferroptosis. Autophagic degradation reduces the expression of the ferritin storage protein. Decreased ferritin expression promotes the onset of ferroptosis. The BRD4 inhibitor (+)-JQ1 (JQ1) confer ferroptosis by autophagy in MDA-MB-231 cells. JQ1 was shown to induce ferritin phagocytosis or decreased the expression of GPX4, SLC7A11, and SLC3A2 (Sui et al., 2019). Dihydroartemisinin (DAT) combined with RSL3 was used to treat breast cancer by targeting ferritin. DAT induces lysosomal degradation of ferritin and impact the iron regulatory protein (IRP)/iron response element (IRE) controlled iron homeostasis to promote the level of cellular free iron (Chen et al., 2020).
System Xc–, a glutamate-cystine antiporter, is consists of the light chain subunit SLC7A11 and the heavy chain subunit SLC3A2. SLC3A2 anchors SLC7A11 to the plasma membrane and maintains the stability of SLC7A11 (Liu M. R. et al., 2021). Several compounds-such as erastin, sulfasalazine, and sorafenib—have been utilized as inhibitors of SLC7A11. The erastin is a classic ferroptosis agonist that inhibits the function of System Xc- by irreversibly binding to SLC7A11 and inactivating SLC7A11, resulting in the depletion of GPX4 and GSH. The erastin-induced ferroptosis by increasing cellular lipid ROS in TNBC (Wang H. et al., 2022). Fascin directly interacts with System Xc- and decreases its stability via ubiquitination. Fascin enhances anti-tumor effect to erastin-induced ferroptosis (Chen C. et al., 2022). Sulfasalazine (SAS) can active ROS production to induce the ferroptosis in breast cancer.
Metformin increases Fe2+ and lipid ROS, reduces the stability of SLC7A11 by inhibiting the ultrafiltration process of SLC7A11, and metformin combined with SAS can synergistically activate ferroptosis and inhibit the growth of breast cancer cells (Yang et al., 2021). The pH regulator carbonic anhydrase IX (CAIX) induced by hypoxia prevents ferroptosis in cancer cells, and co-targeting of CAIX/XII and xCT induce ferroptosis in TNBC. The novel synthetic lethal interaction will help researchers develop new strategies for solid tumor therapy (Chafe et al., 2021). p53 and BRCA1 Associated Protein 1 (BAP1) inhibit carcinogenesis in part by antagonizing SLC7A11 and induce ferroptosis (Zhang Y. et al., 2019). Eupaformosanin was shown to induce ferroptosis by ubiquitination of the mutant p53 in TNBC (Wei et al., 2022).
GPX4 is an essential enzyme for the elimination of lipid hydrogen peroxide. GPX4 reduces the production of ROS radicals in membrane lipids and protects normal breast cells from ROS DNA damage to inhibit ferroptosis. GPX4 inhibitors include RSL3, the RSL3 analogs DPI7, DPI12, DPI13, DPI17, DPI18 and DPI19, ML210, JKE1647, altretamine, FIN56, FINO2, dihydroartemisinin, and Withaferin A. RSL3 inactivates GPX4, leading to ferroptosis by ROS accumulation (Stockwell, 2022). Erastin also suppresses the expression of GPX4 in breast cancer, especially in TNBC. Vitamin C induces TNBC cells death by suppressing GPX4. Furthermore, sequential treatment with VC and quercetin (Q) significantly increased ROS. A study suggested that vitamin C and Q can be developed as an adjuvant agents for cancer patients by Nrf2 (Mostafavi-Pour et al., 2017). Statins, the mevalonate pathway inhibitors, are identified as ferroptosis agents that target HMGCR. Statins suppress CoQ10 production to induce ferroptosis and decrease GPX4 (Gobel et al., 2019). Lycium barbarum polysaccharide (LBP), a traditional Chinese medicine, promotes ferroptosis in breast cancer by modulating the xCT/GPX4 pathway (Du et al., 2022). Ketamine inhibits GPX4 levels and inhibits histone H3 lysine 27 acetylation (H3K27ac) and enrichment of RNA polymerase II (RNA pol II) by attenuating KAT5 on the GPX4 promoter in breast cancer cells (Li H. et al., 2021). Glycyrrhetinic acid (GA) induces lipid peroxidation to trigger ferroptosis in TNBC cells by reducing the activity of GSH and GPX4 (Wen et al., 2021). DMOCPTL can induce EGR1 mediated apoptosis and ferroptosis and in TNBC by GPX4 ubiquitination (Ding et al., 2021). Researchers screened drug combinations using a synthetic lethal mechanism (simultaneous inhibition of two or more non-lethal genes leading to cell death) and found that low doses of targeted drugs targeting BET and cytoplasmic proteins in vitro could reduce the levels of GPX4 and trigger ferroptosis in TNBC (Verma et al., 2020).
Nanomedicine is a favorable solution for the development of ferroptosis because of its better efficacy and improved targeting ability, low systemic toxicity, and high safety (Zafar et al., 2021). The mechanism of emerging nanomedical therapy is mainly including: 1) triggering Fenton reaction; 2) inhibiting the expression of GPX4; 3) exogenous regulation of lipid peroxidation. The diversified multifunctional nanomaterials can produce H2O2, eliminate GSH, and provide Fenton reaction catalyst. In addition, some iron-containing nanomaterials can also induce ferroptosis. Azo-CA4 was loaded into nanocarriers and is converted by near-infrared light to ultraviolet light. After irradiation, Azo-CA4-loaded nanocarriers reduced the viability of TNBC through ferroptosis (Zhu et al., 2020). Sonodynamically amplified ferroptotic red blood cells (SAFEs), a state-of-the-art red blood cell was engineered for simultaneous activation of ferroptosis and oxygen-enriched sonodynamic therapy (SDT). SAFE contains internalized RGD peptide and erythrocyte membrane mixed with camouflaged hemoglobin nanocomplex, perfluorocarbon, sonosensitizer verteporfin, and FINS (dihomo-gamma-linolenic acid, DGLA). SAFE can not only provide abundant oxygen to the hypoxia-related therapeutic resistance of the defect by ultrasound stimulation, but also activate ferroptosis by the combination of ROS production and lipid peroxidation, which selectively accumulates and induces desirable anticancer effects in the tumor (Zhou A. et al., 2022). Heparinase-driven continuous release nanoparticles (HSPE) can enhance the killing power of tumor cells via DOX-induced ferroptosis, regulating the tumor microenvironment, and effectively inhibiting breast cancer metastasis (Zhang J. et al., 2021). The lapatinib and pseudolaric acid B (PAB) were delivered by ferritin-containing nanoparticles, which showed an enhanced tumor suppression ability by increasing ROS (Wu X. et al., 2022).
A tumor-targeted MOF nanosheet system with multienzyme-like catalytic activity can inhibit the antiferroptotic mechanism mediated by GPX4 and FSP1 in tumor cells, thus enhancing ferroptotic damage. After iRGD promoted tumor cells to uptake Au/Cu-TCPP(Fe)@RSL3-PEG-iRGD nanosheets, besides the ability of RSL3 to activate GPX4, the glucose oxidase (Gox) activity of gold nanoparticles could easily comsume intracellular glucose and inhibit the production of NADPH, resulting in reduced expression of GSH and GPX4 (Li K. et al., 2022). Rosuvastatin (RSV) was loaded into silk fibroin (SF) nanoparticles (NPs) (Cu-SF(RSV), to disrupt redox stabilization regulated by the CoQ10/FSP1 axis by interfering with the mevalonate pathway to improve ferroptosis sensitivity in TNBC (Yang J. et al., 2022). In one study, the drug simvastatin (SIM) was loaded into zwitterionic polymer-coated magnetic nanoparticles (Fe3O4@PCBMA). PCBMA promoted the accumulation of Fe3O4@PCBMA in tumor lesions, while SIM inhibited the expression of HMGCR, thereby downregulating mevalonate pathway and GPX4 expression, and inducing ferroptosis (Yao et al., 2021). Cinnamaldehyde dimers (CDCs) induce lipid materials to form dimers, which enable them to deplete GSH and deliver therapeutics to enhance ferroptosis and immunotherapeutic effects against breast cancer (Zhou Z. et al., 2022). The nanoparticles consisting of ferritin and a pH-sensitive molecular switch (FPBC@SN) disintegrate in the acidic cytoplasm and release sorafenib and an indoleamine2,3-dioxygenase (IDO) inhibitor NLG919. NLG919 reduces tryptophan metabolism by inhibiting IDO, thus improving tumor immunity and antitumor efficacy. Sorafenib upregulates nuclear receptor coactivator 4 (NCOA4), which produces lipid peroxide (LPO) through ferritin autophagy. Down-regulation of GPX4 and LPO clearance elicit the ferroptosis in tumor cells (Zuo et al., 2021).
The combination of immunotherapy and ferroptosis death nanoparticles can also play an ideal therapeutic effect on breast cancer. A bionic Fe3O4 magnetosome (PaM/Ti NC), TGF-β Inhibitor (Ti) is carried in the membrane and PD-1 antibody (Pa) is anchored on the outer surface of the nanoparticles. The level of intracellular H2O2 can be increased under immunogenic microenvironment, thus the ferroptosis can be triggered by Fenton reaction. The ideal combined anti-tumor effect is achieved through the mutual promotion of ferroptosis and immunomodulation (Zhang F. et al., 2019).
Combining ferroptosis inducers with immunotherapy and radiation therapy may be a suitable strategy to enhance ferroptosis in breast cancer and make tumors sensitive to immunotherapy and radiation therapy, especially for those with high expression of antiferroptosis genes or low ferroptosis-promoting genes (Sun et al., 2021). Furthermore, ferroptosis has been confirmed to induce synergetic crosstalk between immunotherapy and RT (Table 2).
Lapatinib is a potent double tyrosine kinase inhibitor of the epidermal growth factor receptor (EGFR) and HER-2 which inhibits the proliferation of breast cancer cells with overexpression of ErbB2 and EGFR (Guarneri et al., 2021). Siramesine is a lysosomotropic agent that releases cathepsin B from lysosomes, leading to increased ROS production and cell death (Petersen et al., 2013). The combination of lapatinib and siramesine could induce ferroptosis in TNBC by boosting intracellular Fe2+ (Ma et al., 2016).
RT is a common cancer treatment that uses the ionizing radiation (IR) to eradicate cancer cells (Delaney et al., 2005). Binding of FINS targeting GPX4 or SLC7A11 to RT can sensitize breast cancer cell lines or xenografts to radiation by enhancing ferroptosis sensitivity. Zhang et al. found that iron-saturated lactoferrin (holo-lactoferrin, Holo-Lf) significantly decreased GPX4 expression in MDA-MB-231 cells and cell viability, and combination therapy with erastin could further inhibit cell viability. The combination of Holo-Lf with RT can significantly increase the production of lipid ROS in MDA-MB-231 cells, indicating that RT can promote cell ferroptosis and improve radiosensitivity (Zhang et al., 2021c). Pre-red laser irradiation combined with gallic acid could enhance anticancer effects by decreasing GPX4 activity (Khorsandi et al., 2020). γ-radiation combined with erastin improves GSH synthesis and controls ROS generation, the therapeutic effectors of radiation therapy (Cobler et al., 2018). Knocking down ESR1 expression may enhance the efficacy of radiation-induced ferroptosis via the NEDD4L/CD71 pathway in breast cancer (Liu et al., 2022). Although the combination of RT and FINS appears to be safe in preclinical studies, some researches have suggested that ferroptosis may also be associated with RT-induced damage of normal tissue (Li et al., 2019). Therefore, it is urgent to clarify whether this combination therapy has negligible toxicity to normal tissues.
Dichloroacetate (DCA) combined with albiziabioside A (AlbA) can inhibit GPX4 and induce ferroptosis to inhibit tumor progression (Wei et al., 2019). DCA can also improve glycolytic lactate immunosuppression and promote the effect of immunotherapy (Ohashi et al., 2013). AlbA has the characteristic of selective antitumor effect without affecting normal cells (Wei et al., 2014). The synergistic effect of the AlbA-DCA conjugate which can affect the polarization of M2-TAM to reprogram the tumor immune microenvironment and ferroptosis will enhance therapeutic efficacy.
Photodynamic therapy (PDT) gather lymphocyte infiltration in tumor lesions and stimulates IFN-γ secretion (Xu et al., 2020). Hemoglobin (Hb) can be used as an oxygen provider in PDT due to its excellent oxygen-carrying properties and can also be used as an iron supplement in the treatment of ferroptosis due to the iron component (Wang et al., 2015). Synergistically induced ferroptosis in cancer cells could be a potential strategy for treating breast cancer (Xu et al., 2020).
The development of various FINS and inhibitors has accelerated research on the ferroptosis and chemosensitivity. ACSL4 in tumor lesions can be used as a predictive factor of complete pathological remission and progression-free survival of neoadjuvant chemotherapy in breast cancer. Importantly, the imbalance between ACSL4/GPX4 can indicate the level of ferroptosis after undergoing neoadjuvant chemotherapy, effectively distinguishing patients with different complete pathological response rates and disease-free survival. With the increase in ACSL4 and the decrease in GPX4, the pathological complete remission rate of patients has gradually increased (Sha et al., 2021).
The iron chelator deferasirox can synergistically enhance the efficacy of doxorubicin and cisplatin in TNBC without increasing side effects (Tury et al., 2018). Saponin formosanin C (FC) is a potent FIN that showed the strongest cytostatic effect among all anticancer drugs, including cisplatin, using the MDA-MB-231 cell line as a model (Chen H. C. et al., 2022). The excessive intake of dietary iron leads to the production of oxidative stress and DNA damage. A collaborative group clinical trial revealed that the use of antioxidant supplements during chemotherapy, such as iron and vitamin B12, increased intracellular iron to serve as a propellant and could increase recurrence and mortality in breast cancer. This finding should be considered when discussing the use of dietary supplements during chemotherapy with breast cancer patients (Ambrosone et al., 2020).
As a hotspot in tumor research, ferroptosis plays a crucial role in a variety of tumor cells. New therapeutic targets have the potential to stimulate the development of strategies for the treatment of tumors. Research on ferroptosis in breast cancer focuses on the cellular level in vitro, which provides a relevant theoretical basis for clinical application.
Despite the rapid progress of ferroptosis research in breast cancer, some challenges remain to be elucidated, including the identification of the best candidate drugs and targeting of ferroptosis in clinical trials. FINS are used in combination with other therapeutic approaches, such as immunotherapy or RT, which may induce mixed RCD to inhibit tumor growth. How to select the subtype of breast cancer and subgroups of patients who are sensitive to ferroptosis for targeted treatment should also be elucidated.
Genomics provides a platform for identifying the gene mutations that drive tumor progression and constitute therapeutic targets. Therefore, the integration of genetic information may help distinguish tumors that respond (or do not respond) to ferroptosis. If the expression of pro-apoptotic genes is low but that of ferroptosis-promoting genes is high, patients may derive more benefit from ferroptosis drugs. In addition, depending on the vulnerability of specific breast cancer tumors, different FINS can be used to target endogenous or exogenous pathways.
Ferroptosis is considered as a double-edged sword, and the potential toxic side effects of inducers or inhibitors should be fully elucidate to ensure the activation of the Fenton response and avoid off-target toxicity to normal organ and tissue. In the future, the rational design of safe and effective ferroptosis-based antitumor drugs should be mutifaceted. There are still significant challenges in basic research and clinical translation based on ferroptosis. However, we believe that with the advancement of basic research on ferroptosis, it will be possible to translate FINS or enhancers into clinical practice of breast cancer therapy.
Conception and design: XG and YL. Drafting of the manuscript: YH and YL. Drawing of figures: YJ and JB. Conceiving and Critical revision of the manuscript for important intellectual content: XG and YL. All authors read and approved the final manuscript.
This work was supported by the National Natural Science foundation of China (Grant No.82103468 to YL), Natural Science foundation of Liaoning Province of China (Grant No.2020-MS-178 to XG) and 345 Talent Project of Shengjing Hospital of China Medical University (to YL).
We thank Charlesworth Author Service (https://www.cwauthors.com.cn/) for editing this manuscript.
The authors declare that the research was conducted in the absence of any commercial or financial relationships that could be construed as a potential conflict of interest.
All claims expressed in this article are solely those of the authors and do not necessarily represent those of their affiliated organizations, or those of the publisher, the editors and the reviewers. Any product that may be evaluated in this article, or claim that may be made by its manufacturer, is not guaranteed or endorsed by the publisher.
Ambrosone, C. B., Zirpoli, G. R., Hutson, A. D., Mccann, W. E., Mccann, S. E., Barlow, W. E., et al. (2020). Dietary supplement use during chemotherapy and survival outcomes of patients with breast cancer enrolled in a cooperative group clinical trial (SWOG S0221). J. Clin. Oncol. 38, 804–814. doi:10.1200/JCO.19.01203
Battaglia, A. M., Sacco, A., Perrotta, I. D., Faniello, M. C., Scalise, M., Torella, D., et al. (2022). Iron administration overcomes resistance to erastin-mediated ferroptosis in ovarian cancer cells. Front. Oncol. 12, 868351. doi:10.3389/fonc.2022.868351
Bazhabayi, M., Qiu, X., Li, X., Yang, A., Wen, W., Zhang, X., et al. (2021). CircGFRA1 facilitates the malignant progression of HER-2-positive breast cancer via acting as a sponge of miR-1228 and enhancing AIFM2 expression. J. Cell. Mol. Med. 25, 10248–10256. doi:10.1111/jcmm.16963
Bersuker, K., Hendricks, J. M., Li, Z., Magtanong, L., Ford, B., Tang, P. H., et al. (2019). The CoQ oxidoreductase FSP1 acts parallel to GPX4 to inhibit ferroptosis. Nature 575, 688–692. doi:10.1038/s41586-019-1705-2
Chafe, S. C., Vizeacoumar, F. S., Venkateswaran, G., Nemirovsky, O., Awrey, S., Brown, W. S., et al. (2021). Genome-wide synthetic lethal screen unveils novel CAIX-NFS1/xCT axis as a targetable vulnerability in hypoxic solid tumors. Sci. Adv. 7, eabj0364. doi:10.1126/sciadv.abj0364
Chang, V. C., Cotterchio, M., Bondy, S. J., and Kotsopoulos, J. (2020). Iron intake, oxidative stress-related genes and breast cancer risk. Int. J. Cancer 147, 1354–1373. doi:10.1002/ijc.32906
Chen, C., Liu, P., Duan, X., Cheng, M., and Xu, L. X. (2019). Deferoxamine-induced high expression of TfR1 and DMT1 enhanced iron uptake in triple-negative breast cancer cells by activating IL-6/PI3K/AKT pathway. Onco. Targets. Ther. 12, 4359–4377. doi:10.2147/OTT.S193507
Chen, C., Xie, B., Li, Z., Chen, L., Chen, Y., Zhou, J., et al. (2022a). Fascin enhances the vulnerability of breast cancer to erastin-induced ferroptosis. Cell Death Dis. 13, 150. doi:10.1038/s41419-022-04579-1
Chen, F., Fan, Y., Hou, J., Liu, B., Zhang, B., Shang, Y., et al. (2021a). Integrated analysis identifies TfR1 as a prognostic biomarker which correlates with immune infiltration in breast cancer. Aging (Albany NY) 13, 21671–21699. doi:10.18632/aging.203512
Chen, G. Q., Benthani, F. A., Wu, J., Liang, D., Bian, Z. X., and Jiang, X. (2020). Artemisinin compounds sensitize cancer cells to ferroptosis by regulating iron homeostasis. Cell Death Differ. 27, 242–254. doi:10.1038/s41418-019-0352-3
Chen, H. C., Tang, H. H., Hsu, W. H., Wu, S. Y., Cheng, W. H., Wang, B. Y., et al. (2022b). Vulnerability of triple-negative breast cancer to saponin formosanin C-induced ferroptosis. Antioxidants (Basel) 11, 298. doi:10.3390/antiox11020298
Chen, X., Kang, R., Kroemer, G., and Tang, D. (2021b). Broadening horizons: The role of ferroptosis in cancer. Nat. Rev. Clin. Oncol. 18, 280–296. doi:10.1038/s41571-020-00462-0
Clemons, N. J., Liu, D. S., Duong, C. P., and Phillips, W. A. (2017). Inhibiting system xC(-) and glutathione biosynthesis - a potential Achilles' heel in mutant-p53 cancers. Mol. Cell. Oncol. 4, e1344757. doi:10.1080/23723556.2017.1344757
Cobler, L., Zhang, H., Suri, P., Park, C., and Timmerman, L. A. (2018). xCT inhibition sensitizes tumors to gamma-radiation via glutathione reduction. Oncotarget 9, 32280–32297. doi:10.18632/oncotarget.25794
Consoli, V., Sorrenti, V., Pittala, V., Greish, K., D'amico, A. G., Romeo, G., et al. (2022). Heme oxygenase modulation drives ferroptosis in TNBC cells. Int. J. Mol. Sci. 23, 5709. doi:10.3390/ijms23105709
Delaney, G., Jacob, S., Featherstone, C., and Barton, M. (2005). The role of radiotherapy in cancer treatment: Estimating optimal utilization from a review of evidence-based clinical guidelines. Cancer 104, 1129–1137. doi:10.1002/cncr.21324
Dierge, E., Debock, E., Guilbaud, C., Corbet, C., Mignolet, E., Mignard, L., et al. (2021). Peroxidation of n-3 and n-6 polyunsaturated fatty acids in the acidic tumor environment leads to ferroptosis-mediated anticancer effects. Cell Metab. 33, 1701–1715.e5. e1705. doi:10.1016/j.cmet.2021.05.016
Ding, Y., Chen, X., Liu, C., Ge, W., Wang, Q., Hao, X., et al. (2021). Identification of a small molecule as inducer of ferroptosis and apoptosis through ubiquitination of GPX4 in triple negative breast cancer cells. J. Hematol. Oncol. 14, 19. doi:10.1186/s13045-020-01016-8
Dixon, S. J., Lemberg, K. M., Lamprecht, M. R., Skouta, R., Zaitsev, E. M., Gleason, C. E., et al. (2012). Ferroptosis: An iron-dependent form of nonapoptotic cell death. Cell 149, 1060–1072. doi:10.1016/j.cell.2012.03.042
Doll, S., Freitas, F. P., Shah, R., Aldrovandi, M., Da Silva, M. C., Ingold, I., et al. (2019). FSP1 is a glutathione-independent ferroptosis suppressor. Nature 575, 693–698. doi:10.1038/s41586-019-1707-0
Doll, S., Proneth, B., Tyurina, Y. Y., Panzilius, E., Kobayashi, S., Ingold, I., et al. (2017). ACSL4 dictates ferroptosis sensitivity by shaping cellular lipid composition. Nat. Chem. Biol. 13, 91–98. doi:10.1038/nchembio.2239
Du, J., Wang, L., Huang, X., Zhang, N., Long, Z., Yang, Y., et al. (2021). Kanglaite injection plus platinum-based chemotherapy for stage III/IV non-small cell lung cancer: A meta-analysis of 27 RCTs. Phytomedicine 85, 153154. doi:10.1016/j.phymed.2019.153154
Du, X., Zhang, J., Liu, L., Xu, B., Han, H., Dai, W., et al. (2022). A novel anticancer property of Lycium barbarum polysaccharide in triggering ferroptosis of breast cancer cells. J. Zhejiang Univ. Sci. B 23, 286–299. doi:10.1631/jzus.B2100748
Duggan, C., Trapani, D., Ilbawi, A. M., Fidarova, E., Laversanne, M., Curigliano, G., et al. (2021). National health system characteristics, breast cancer stage at diagnosis, and breast cancer mortality: A population-based analysis. Lancet. Oncol. 22, 1632–1642. doi:10.1016/S1470-2045(21)00462-9
Fang, K., Du, S., Shen, D., Xiong, Z., Jiang, K., Liang, D., et al. (2022). SUFU suppresses ferroptosis sensitivity in breast cancer cells via Hippo/YAP pathway. iScience 25, 104618. doi:10.1016/j.isci.2022.104618
Gobel, A., Breining, D., Rauner, M., Hofbauer, L. C., and Rachner, T. D. (2019). Induction of 3-hydroxy-3-methylglutaryl-CoA reductase mediates statin resistance in breast cancer cells. Cell Death Dis. 10, 91. doi:10.1038/s41419-019-1322-x
Guarneri, V., Dieci, M. V., Griguolo, G., Miglietta, F., Girardi, F., Bisagni, G., et al. (2021). Trastuzumab-lapatinib as neoadjuvant therapy for HER2-positive early breast cancer: Survival analyses of the CHER-Lob trial. Eur. J. Cancer 153, 133–141. doi:10.1016/j.ejca.2021.05.018
Hanahan, D., and Weinberg, R. A. (2011). Hallmarks of cancer: The next generation. Cell 144, 646–674. doi:10.1016/j.cell.2011.02.013
Hassannia, B., Vandenabeele, P., and Vanden Berghe, T. (2019). Targeting ferroptosis to iron out cancer. Cancer Cell 35, 830–849. doi:10.1016/j.ccell.2019.04.002
Hou, C., Hou, Q., Xie, X., Wang, H., Chen, Y., Lu, T., et al. (2021). Serum iron status and the risk of breast cancer in the European population: A two-sample mendelian randomisation study. Genes Nutr. 16, 9. doi:10.1186/s12263-021-00691-7
Ingold, I., Berndt, C., Schmitt, S., Doll, S., Poschmann, G., Buday, K., et al. (2018). Selenium utilization by GPX4 is required to prevent hydroperoxide-induced ferroptosis. Cell 172, 409–422. doi:10.1016/j.cell.2017.11.048
Karmi, O., Sohn, Y. S., Zandalinas, S. I., Rowland, L., King, S. D., Nechushtai, R., et al. (2021). Disrupting CISD2 function in cancer cells primarily impacts mitochondrial labile iron levels and triggers TXNIP expression. Free Radic. Biol. Med. 176, 92–104. doi:10.1016/j.freeradbiomed.2021.09.013
Khorsandi, K., Kianmehr, Z., Hosseinmardi, Z., and Hosseinzadeh, R. (2020). Anti-cancer effect of gallic acid in presence of low level laser irradiation: ROS production and induction of apoptosis and ferroptosis. Cancer Cell Int. 20, 18. doi:10.1186/s12935-020-1100-y
Kraft, V. a. N., Bezjian, C. T., Pfeiffer, S., Ringelstetter, L., Muller, C., Zandkarimi, F., et al. (2020). GTP cyclohydrolase 1/tetrahydrobiopterin counteract ferroptosis through lipid remodeling. ACS Cent. Sci. 6, 41–53. doi:10.1021/acscentsci.9b01063
Kumar, R., Singh, A. K., Kumar, M., Shekhar, S., Rai, N., Kaur, P., et al. (2016). Serum 5-LOX: A progressive protein marker for breast cancer and new approach for therapeutic target. Carcinogenesis 37, 912–917. doi:10.1093/carcin/bgw075
Latunde-Dada, G. O. (2017). Ferroptosis: Role of lipid peroxidation, iron and ferritinophagy. Biochim. Biophys. Acta. Gen. Subj. 1861, 1893–1900. doi:10.1016/j.bbagen.2017.05.019
Lee, N., Carlisle, A. E., Peppers, A., Park, S. J., Doshi, M. B., Spears, M. E., et al. (2021). xCT-driven expression of GPX4 determines sensitivity of breast cancer cells to ferroptosis inducers. Antioxidants (Basel) 10, 317. doi:10.3390/antiox10020317
Lei, G., Zhuang, L., and Gan, B. (2022). Targeting ferroptosis as a vulnerability in cancer. Nat. Rev. Cancer 22, 381–396. doi:10.1038/s41568-022-00459-0
Li, H., Liu, W., Zhang, X., Wu, F., Sun, D., and Wang, Z. (2021a). Ketamine suppresses proliferation and induces ferroptosis and apoptosis of breast cancer cells by targeting KAT5/GPX4 axis. Biochem. Biophys. Res. Commun. 585, 111–116. doi:10.1016/j.bbrc.2021.11.029
Li, H., Yang, P., Wang, J., Zhang, J., Ma, Q., Jiang, Y., et al. (2022a). HLF regulates ferroptosis, development and chemoresistance of triple-negative breast cancer by activating tumor cell-macrophage crosstalk. J. Hematol. Oncol. 15, 2. doi:10.1186/s13045-021-01223-x
Li, K., Lin, C., Li, M., Xu, K., He, Y., Mao, Y., et al. (2022b). Multienzyme-like reactivity cooperatively impairs glutathione peroxidase 4 and ferroptosis suppressor protein 1 pathways in triple-negative breast cancer for sensitized ferroptosis therapy. ACS Nano 16, 2381–2398. doi:10.1021/acsnano.1c08664
Li, P., Yang, B., Xiu, B., Chi, Y., Xue, J., and Wu, J. (2021b). Development and validation of a robust ferroptosis-related gene panel for breast cancer disease-specific survival. Front. Cell Dev. Biol. 9, 709180. doi:10.3389/fcell.2021.709180
Li, X., Duan, L., Yuan, S., Zhuang, X., Qiao, T., and He, J. (2019). Ferroptosis inhibitor alleviates Radiation-induced lung fibrosis (RILF) via down-regulation of TGF-β1. J. Inflamm. 16, 11. doi:10.1186/s12950-019-0216-0
Lin, C. C., Yang, W. H., Lin, Y. T., Tang, X., Chen, P. H., Ding, C. C., et al. (2021). DDR2 upregulation confers ferroptosis susceptibility of recurrent breast tumors through the Hippo pathway. Oncogene 40, 2018–2034. doi:10.1038/s41388-021-01676-x
Liu, J., Kuang, F., Kroemer, G., Klionsky, D. J., Kang, R., and Tang, D. (2020). Autophagy-dependent ferroptosis: Machinery and regulation. Cell Chem. Biol. 27, 420–435. doi:10.1016/j.chembiol.2020.02.005
Liu, L., Zhang, C., Qu, S., Liu, R., Chen, H., Liang, Z., et al. (2022). ESR1 inhibits ionizing radiation-induced ferroptosis in breast cancer cells via the NEDD4L/CD71 pathway. Arch. Biochem. Biophys. 725, 109299. doi:10.1016/j.abb.2022.109299
Liu, M. R., Zhu, W. T., and Pei, D. S. (2021a). System Xc(-): A key regulatory target of ferroptosis in cancer. Invest. New Drugs 39, 1123–1131. doi:10.1007/s10637-021-01070-0
Liu, P., He, K., Song, H., Ma, Z., Yin, W., and Xu, L. X. (2016). Deferoxamine-induced increase in the intracellular iron levels in highly aggressive breast cancer cells leads to increased cell migration by enhancing TNF-α-dependent NF-κB signaling and TGF-β signaling. J. Inorg. Biochem. 160, 40–48. doi:10.1016/j.jinorgbio.2016.04.014
Liu, W., Chakraborty, B., Safi, R., Kazmin, D., Chang, C. Y., and Mcdonnell, D. P. (2021b). Dysregulated cholesterol homeostasis results in resistance to ferroptosis increasing tumorigenicity and metastasis in cancer. Nat. Commun. 12, 5103. doi:10.1038/s41467-021-25354-4
Ma, S., Henson, E. S., Chen, Y., and Gibson, S. B. (2016). Ferroptosis is induced following siramesine and lapatinib treatment of breast cancer cells. Cell Death Dis. 7, e2307. doi:10.1038/cddis.2016.208
Magri, J., Gasparetto, A., Conti, L., Calautti, E., Cossu, C., Ruiu, R., et al. (2021). Tumor-associated antigen xCT and mutant-p53 as molecular targets for new combinatorial antitumor strategies. Cells 10, 108. doi:10.3390/cells10010108
Mao, C., Liu, X., Zhang, Y., Lei, G., Yan, Y., Lee, H., et al. (2021). DHODH-mediated ferroptosis defence is a targetable vulnerability in cancer. Nature 593, 586–590. doi:10.1038/s41586-021-03539-7
Mehdi, M., Menon, M. K. C., Seyoum, N., Bekele, M., Tigeneh, W., and Seifu, D. (2018). Blood and tissue enzymatic activities of GDH and LDH, index of glutathione, and oxidative stress among breast cancer patients attending referral hospitals of addis ababa, Ethiopia: Hospital-based comparative cross-sectional study. Oxid. Med. Cell. Longev. 2018, 6039453. doi:10.1155/2018/6039453
Mishra, P., Tang, W., and Ambs, S. (2018). ADHFE1 is a MYC-linked oncogene that induces metabolic reprogramming and cellular de-differentiation in breast cancer. Mol. Cell. Oncol. 5, e1432260. doi:10.1080/23723556.2018.1432260
Mohamad Fairus, A. K., Choudhary, B., Hosahalli, S., Kavitha, N., and Shatrah, O. (2017). Dihydroorotate dehydrogenase (DHODH) inhibitors affect ATP depletion, endogenous ROS and mediate S-phase arrest in breast cancer cells. Biochimie 135, 154–163. doi:10.1016/j.biochi.2017.02.003
Mostafavi-Pour, Z., Ramezani, F., Keshavarzi, F., and Samadi, N. (2017). The role of quercetin and vitamin C in Nrf2-dependent oxidative stress production in breast cancer cells. Oncol. Lett. 13, 1965–1973. doi:10.3892/ol.2017.5619
Mou, Y., Wang, J., Wu, J., He, D., Zhang, C., Duan, C., et al. (2019). Ferroptosis, a new form of cell death: Opportunities and challenges in cancer. J. Hematol. Oncol. 12, 34. doi:10.1186/s13045-019-0720-y
Ohashi, T., Akazawa, T., Aoki, M., Kuze, B., Mizuta, K., Ito, Y., et al. (2013). Dichloroacetate improves immune dysfunction caused by tumor-secreted lactic acid and increases antitumor immunoreactivity. Int. J. Cancer 133, 1107–1118. doi:10.1002/ijc.28114
Petersen, N. H., Olsen, O. D., Groth-Pedersen, L., Ellegaard, A. M., Bilgin, M., Redmer, S., et al. (2013). Transformation-associated changes in sphingolipid metabolism sensitize cells to lysosomal cell death induced by inhibitors of acid sphingomyelinase. Cancer Cell 24, 379–393. doi:10.1016/j.ccr.2013.08.003
Pinnix, Z. K., Miller, L. D., Wang, W., D'agostino, R., Kute, T., Willingham, M. C., et al. (2010). Ferroportin and iron regulation in breast cancer progression and prognosis. Sci. Transl. Med. 2, 43ra56. doi:10.1126/scisignal.3001127
Qi, L., Sun, B., Yang, B., and Lu, S. (2022). PGM5P3-AS1 regulates MAP1LC3C to promote cell ferroptosis and thus inhibiting the malignant progression of triple-negative breast cancer. Breast Cancer Res. Treat. 193, 305–318. doi:10.1007/s10549-021-06501-3
Sacco, A., Battaglia, A. M., Botta, C., Aversa, I., Mancuso, S., Costanzo, F., et al. (2021). Iron metabolism in the tumor microenvironment-implications for anti-cancer immune response. Cells 10, 303. doi:10.3390/cells10020303
Sha, R., Xu, Y., Yuan, C., Sheng, X., Wu, Z., Peng, J., et al. (2021). Predictive and prognostic impact of ferroptosis-related genes ACSL4 and GPX4 on breast cancer treated with neoadjuvant chemotherapy. EBioMedicine 71, 103560. doi:10.1016/j.ebiom.2021.103560
Shimada, K., Skouta, R., Kaplan, A., Yang, W. S., Hayano, M., Dixon, S. J., et al. (2016). Global survey of cell death mechanisms reveals metabolic regulation of ferroptosis. Nat. Chem. Biol. 12, 497–503. doi:10.1038/nchembio.2079
Stockwell, B. R. (2022). Ferroptosis turns 10: Emerging mechanisms, physiological functions, and therapeutic applications. Cell 185, 2401–2421. doi:10.1016/j.cell.2022.06.003
Stockwell, B. R., Friedmann Angeli, J. P., Bayir, H., Bush, A. I., Conrad, M., Dixon, S. J., et al. (2017). Ferroptosis: A regulated cell death nexus linking metabolism, redox biology, and disease. Cell 171, 273–285. doi:10.1016/j.cell.2017.09.021
Sui, S., Xu, S., and Pang, D. (2022). Emerging role of ferroptosis in breast cancer: New dawn for overcoming tumor progression. Pharmacol. Ther. 232, 107992. doi:10.1016/j.pharmthera.2021.107992
Sui, S., Zhang, J., Xu, S., Wang, Q., Wang, P., and Pang, D. (2019). Ferritinophagy is required for the induction of ferroptosis by the bromodomain protein BRD4 inhibitor (+)-JQ1 in cancer cells. Cell Death Dis. 10, 331. doi:10.1038/s41419-019-1564-7
Sun, L. L., Linghu, D. L., and Hung, M. C. (2021). Ferroptosis: A promising target for cancer immunotherapy. Am. J. Cancer Res. 11, 5856–5863.
Tang, D., Chen, X., Kang, R., and Kroemer, G. (2021). Ferroptosis: Molecular mechanisms and health implications. Cell Res. 31, 107–125. doi:10.1038/s41422-020-00441-1
Tang, D., and Kroemer, G. (2020). Ferroptosis. Curr. Biol. 30, R1292–R1297. doi:10.1016/j.cub.2020.09.068
Tury, S., Assayag, F., Bonin, F., Chateau-Joubert, S., Servely, J. L., Vacher, S., et al. (2018). The iron chelator deferasirox synergises with chemotherapy to treat triple-negative breast cancers. J. Pathol. 246, 103–114. doi:10.1002/path.5104
Verma, N., Vinik, Y., Saroha, A., Nair, N. U., Ruppin, E., Mills, G., et al. (2020). Synthetic lethal combination targeting BET uncovered intrinsic susceptibility of TNBC to ferroptosis. Sci. Adv. 6, eaba8968. doi:10.1126/sciadv.aba8968
Von Hagens, C., Walter-Sack, I., Goeckenjan, M., Osburg, J., Storch-Hagenlocher, B., Sertel, S., et al. (2017). Prospective open uncontrolled phase I study to define a well-tolerated dose of oral artesunate as add-on therapy in patients with metastatic breast cancer (ARTIC M33/2). Breast Cancer Res. Treat. 164, 359–369. doi:10.1007/s10549-017-4261-1
Wang, H., Wang, P., and Zhu, B. T. (2022a). Mechanism of erastin-induced ferroptosis in MDA-MB-231 human breast cancer cells: Evidence for a critical role of protein disulfide isomerase. Mol. Cell. Biol. 42, e0052221. doi:10.1128/mcb.00522-21
Wang, S., Wang, Y., Li, Q., Li, X., and Feng, X. (2022b). A novel circular RNA confers trastuzumab resistance in human epidermal growth factor receptor 2-positive breast cancer through regulating ferroptosis. Environ. Toxicol. 37, 1597–1607. doi:10.1002/tox.23509
Wang, S., Yuan, F., Chen, K., Chen, G., Tu, K., Wang, H., et al. (2015). Synthesis of hemoglobin conjugated polymeric micelle: A ZnPc carrier with oxygen self-compensating ability for photodynamic therapy. Biomacromolecules 16, 2693–2700. doi:10.1021/acs.biomac.5b00571
Wang, W., Green, M., Choi, J. E., Gijon, M., Kennedy, P. D., Johnson, J. K., et al. (2019a). CD8(+) T cells regulate tumour ferroptosis during cancer immunotherapy. Nature 569, 270–274. doi:10.1038/s41586-019-1170-y
Wang, Y., Yang, L., Zhang, X., Cui, W., Liu, Y., Sun, Q. R., et al. (2019b). Epigenetic regulation of ferroptosis by H2B monoubiquitination and p53. EMBO Rep. 20, e47563. doi:10.15252/embr.201847563
Wei, G., Sun, J., Luan, W., Hou, Z., Wang, S., Cui, S., et al. (2019). Natural product albiziabioside A conjugated with pyruvate dehydrogenase kinase inhibitor dichloroacetate to induce apoptosis-ferroptosis-M2-TAMs polarization for combined cancer therapy. J. Med. Chem. 62, 8760–8772. doi:10.1021/acs.jmedchem.9b00644
Wei, G., Wang, S., Cui, S., Guo, J., Liu, Y., Liu, Y., et al. (2014). Synthesis and evaluation of the anticancer activity of albiziabioside A and its analogues as apoptosis inducers against human melanoma cells. Org. Biomol. Chem. 12, 5928–5935. doi:10.1039/c4ob00874j
Wei, J. L., Wu, S. Y., Yang, Y. S., Xiao, Y., Jin, X., Xu, X. E., et al. (2021). GCH1 induces immunosuppression through metabolic reprogramming and Ido1 upregulation in triple-negative breast cancer. J. Immunother. Cancer 9, e002383. doi:10.1136/jitc-2021-002383
Wei, Y., Zhu, Z., Hu, H., Guan, J., Yang, B., and Zhao, H. (2022). Eupaformosanin induces apoptosis and ferroptosis through ubiquitination of mutant p53 in triple-negative breast cancer. Eur. J. Pharmacol. 924, 174970. doi:10.1016/j.ejphar.2022.174970
Wen, Y., Chen, H., Zhang, L., Wu, M., Zhang, F., Yang, D., et al. (2021). Glycyrrhetinic acid induces oxidative/nitrative stress and drives ferroptosis through activating NADPH oxidases and iNOS, and depriving glutathione in triple-negative breast cancer cells. Free Radic. Biol. Med. 173, 41–51. doi:10.1016/j.freeradbiomed.2021.07.019
Wiernicki, B., Maschalidi, S., Pinney, J., Adjemian, S., Vanden Berghe, T., Ravichandran, K. S., et al. (2022). Cancer cells dying from ferroptosis impede dendritic cell-mediated anti-tumor immunity. Nat. Commun. 13, 3676. doi:10.1038/s41467-022-31218-2
Wu, M., Zhang, X., Zhang, W., Chiou, Y. S., Qian, W., Liu, X., et al. (2022a). Cancer stem cell regulated phenotypic plasticity protects metastasized cancer cells from ferroptosis. Nat. Commun. 13, 1371. doi:10.1038/s41467-022-29018-9
Wu, X., Liu, C., Li, Z., Gai, C., Ding, D., Chen, W., et al. (2020). Regulation of GSK3β/Nrf2 signaling pathway modulated erastin-induced ferroptosis in breast cancer. Mol. Cell. Biochem. 473, 217–228. doi:10.1007/s11010-020-03821-8
Wu, X., Sheng, H., Zhao, L., Jiang, M., Lou, H., Miao, Y., et al. (2022b). Co-loaded lapatinib/PAB by ferritin nanoparticles eliminated ECM-detached cluster cells via modulating EGFR in triple-negative breast cancer. Cell Death Dis. 13, 557. doi:10.1038/s41419-022-05007-0
Xie, Y., Wang, B., Zhao, Y., Tao, Z., Wang, Y., Chen, G., et al. (2022). Mammary adipocytes protect triple-negative breast cancer cells from ferroptosis. J. Hematol. Oncol. 15, 72. doi:10.1186/s13045-022-01297-1
Xiong, H., Wang, C., Wang, Z., Jiang, Z., Zhou, J., and Yao, J. (2019). Intracellular cascade activated nanosystem for improving ER+ breast cancer therapy through attacking GSH-mediated metabolic vulnerability. J. Control. Release 309, 145–157. doi:10.1016/j.jconrel.2019.07.029
Xu, T., Ding, W., Ji, X., Ao, X., Liu, Y., Yu, W., et al. (2019). Molecular mechanisms of ferroptosis and its role in cancer therapy. J. Cell. Mol. Med. 23, 4900–4912. doi:10.1111/jcmm.14511
Xu, T., Ma, Y., Yuan, Q., Hu, H., Hu, X., Qian, Z., et al. (2020). Enhanced ferroptosis by oxygen-boosted phototherapy based on a 2-in-1 nanoplatform of ferrous hemoglobin for tumor synergistic therapy. ACS Nano 14, 3414–3425. doi:10.1021/acsnano.9b09426
Yadav, P., Sharma, P., Sundaram, S., Venkatraman, G., Bera, A. K., and Karunagaran, D. (2021). SLC7A11/xCT is a target of miR-5096 and its restoration partially rescues miR-5096-mediated ferroptosis and anti-tumor effects in human breast cancer cells. Cancer Lett. 522, 211–224. doi:10.1016/j.canlet.2021.09.033
Yang, F., Xiao, Y., Ding, J. H., Jin, X., Ma, D., Li, D. Q., et al. (2022a). Ferroptosis heterogeneity in triple-negative breast cancer reveals an innovative immunotherapy combination strategy. Cell Metab. 2022, S1550. doi:10.1016/j.cmet.2022.09.021
Yang, J., Jia, Z., Zhang, J., Pan, X., Wei, Y., Ma, S., et al. (2022b). Metabolic intervention nanoparticles for triple-negative breast cancer therapy via overcoming FSP1-mediated ferroptosis resistance. Adv. Healthc. Mat. 11, e2102799. doi:10.1002/adhm.202102799
Yang, J., Zhou, Y., Xie, S., Wang, J., Li, Z., Chen, L., et al. (2021). Metformin induces Ferroptosis by inhibiting UFMylation of SLC7A11 in breast cancer. J. Exp. Clin. Cancer Res. 40, 206. doi:10.1186/s13046-021-02012-7
Yang, W. S., Sriramaratnam, R., Welsch, M. E., Shimada, K., Skouta, R., Viswanathan, V. S., et al. (2014). Regulation of ferroptotic cancer cell death by GPX4. Cell 156, 317–331. doi:10.1016/j.cell.2013.12.010
Yao, X., Xie, R., Cao, Y., Tang, J., Men, Y., Peng, H., et al. (2021). Simvastatin induced ferroptosis for triple-negative breast cancer therapy. J. Nanobiotechnology 19, 311. doi:10.1186/s12951-021-01058-1
Zafar, H., Raza, F., Ma, S., Wei, Y., Zhang, J., and Shen, Q. (2021). Recent progress on nanomedicine-induced ferroptosis for cancer therapy. Biomater. Sci. 9, 5092–5115. doi:10.1039/d1bm00721a
Zhang, F., Li, F., Lu, G. H., Nie, W., Zhang, L., Lv, Y., et al. (2019a). Engineering magnetosomes for ferroptosis/immunomodulation synergism in cancer. ACS Nano 13, 5662–5673. doi:10.1021/acsnano.9b00892
Zhang, H., Ge, Z., Wang, Z., Gao, Y., Wang, Y., and Qu, X. (2021a). Circular RNA RHOT1 promotes progression and inhibits ferroptosis via mir-106a-5p/STAT3 axis in breast cancer. Aging (Albany NY) 13, 8115–8126. doi:10.18632/aging.202608
Zhang, H. L., Hu, B. X., Li, Z. L., Du, T., Shan, J. L., Ye, Z. P., et al. (2022). PKCβII phosphorylates ACSL4 to amplify lipid peroxidation to induce ferroptosis. Nat. Cell Biol. 24, 88–98. doi:10.1038/s41556-021-00818-3
Zhang, J., Yang, J., Zuo, T., Ma, S., Xokrat, N., Hu, Z., et al. (2021b). Heparanase-driven sequential released nanoparticles for ferroptosis and tumor microenvironment modulations synergism in breast cancer therapy. Biomaterials 266, 120429. doi:10.1016/j.biomaterials.2020.120429
Zhang, Y., Zhuang, L., and Gan, B. (2019b). BAP1 suppresses tumor development by inducing ferroptosis upon SLC7A11 repression. Mol. Cell. Oncol. 6, 1536845. doi:10.1080/23723556.2018.1536845
Zhang, Z., Lu, M., Chen, C., Tong, X., Li, Y., Yang, K., et al. (2021c). Holo-lactoferrin: The link between ferroptosis and radiotherapy in triple-negative breast cancer. Theranostics 11, 3167–3182. doi:10.7150/thno.52028
Zhang, Z., Qiu, X., Yan, Y., Liang, Q., Cai, Y., Peng, B., et al. (2021d). Evaluation of ferroptosis-related gene AKR1C1 as a novel biomarker associated with the immune microenvironment and prognosis in breast cancer. Int. J. Gen. Med. 14, 6189–6200. doi:10.2147/IJGM.S329031
Zhou, A., Fang, T., Chen, K., Xu, Y., Chen, Z., and Ning, X. (2022a). Biomimetic activator of sonodynamic ferroptosis amplifies inherent peroxidation for improving the treatment of breast cancer. Small 18, e2106568. doi:10.1002/smll.202106568
Zhou, Z., Liang, H., Yang, R., Yang, Y., Dong, J., Di, Y., et al. (2022b). Glutathione depletion-induced activation of dimersomes for potentiating the ferroptosis and immunotherapy of "cold" tumor. Angew. Chem. Int. Ed. Engl. 61, e202202843. doi:10.1002/anie.202202843
Zhu, J., Dai, P., Liu, F., Li, Y., Qin, Y., Yang, Q., et al. (2020). Upconverting nanocarriers enable triggered microtubule inhibition and concurrent ferroptosis induction for selective treatment of triple-negative breast cancer. Nano Lett. 20, 6235–6245. doi:10.1021/acs.nanolett.0c00502
Zhu, L., Chen, M., Huang, B., Zhang, T., Chen, K., Lian, H., et al. (2021). Genomic analysis uncovers immune microenvironment characteristics and drug sensitivity of ferroptosis in breast cancer brain metastasis. Front. Genet. 12, 819632. doi:10.3389/fgene.2021.819632
Zou, Y., Li, H., Graham, E. T., Deik, A. A., Eaton, J. K., Wang, W., et al. (2020). Cytochrome P450 oxidoreductase contributes to phospholipid peroxidation in ferroptosis. Nat. Chem. Biol. 16, 302–309. doi:10.1038/s41589-020-0472-6
Zou, Y., Zheng, S., Xie, X., Ye, F., Hu, X., Tian, Z., et al. (2022). N6-methyladenosine regulated FGFR4 attenuates ferroptotic cell death in recalcitrant HER2-positive breast cancer. Nat. Commun. 13, 2672. doi:10.1038/s41467-022-30217-7
Zuo, T., Fang, T., Zhang, J., Yang, J., Xu, R., Wang, Z., et al. (2021). pH-sensitive molecular-switch-containing polymer nanoparticle for breast cancer therapy with ferritinophagy-cascade ferroptosis and tumor immune activation. Adv. Healthc. Mat. 10, e2100683. doi:10.1002/adhm.202100683
Zuo, Y. B., Zhang, Y. F., Zhang, R., Tian, J. W., Lv, X. B., Li, R., et al. (2022). Ferroptosis in cancer progression: Role of noncoding RNAs. Int. J. Biol. Sci. 18, 1829–1843. doi:10.7150/ijbs.66917
AA Arachidonic acid
ACSL acyl CoA synthase long chain family member
ADA Adrenaline
ADHFE1 Alcohol Dehydrogenase Iron Containing 1
AlbA Albiziabioside A
ALOX5 Arachidonate 5-Lipoxygenase
AKR1C1 Aldo-keto reductase family 1member C1
BAP1 BRCA1 associated protein 1
BC breast cancer
BRCA1 BRCA1 associated protein 1
BRD4 Bromodomain containing 4
CAIX carbonic anhydrase IX
CDCs Cinnamaldehyde dimers
COQ10 ubiquinone
CSC cancer stem cell
CISD2 CDGSH Iron Sulfur Domain 2
DAT Dihydroartemisinin
DCA Dichloroacetate
DDR2 discoidin domain receptor tyrosine kinase 2
DFO Deferoxamine
DFX desferoxazole
DGLA dihomo-gamma-linolenic acid
DHFR dihydrofolate reductase
DHODH Dihydroorotate dehydrogenase (Quinone)
DKK1 Dickkopf WNT Signaling Pathway Inhibitor 1
DMT1 divalent metal transporter 1
DMT1 divalent metal transporter 1,
EGFR epidermal growth factor receptor
EGR1 early growth response 1
EMT Epithelial-to-mesenchymal transition
ESR1 estrogen receptor 1
FC formosanin C
Fins ferroptosis inducers
FPN ferroportin,
FRG Ferroptosis-related gene
FSP1 Ferroptosis suppressor protein
FTH1 ferritin heavy chain 1
FTL ferritin light chain
FGFR4 Fibroblast Growth Factor Receptor 4
GA Glycyrrhetinic acid
GCH1 Guanosine triphosphate cyclohydrolase 1
GCL γ-glutamylcysteine ligase
GFRA1 GDNF Family Receptor Alpha 1
GGT1 gamma-glutamyltransferase 1
Gox glucose oxidase
GPX4 glutathione peroxidase 4
GS Glutathione synthetase
GSH Glutathione
GSK-3 β Glycogen synthase kinase-3 β
H3K27ac H3 lysine 27 acetylation
Hb Hemoglobin
HLF hepatic leukemia factor
HMGB1 high mobility group box 1
HMGCR 3-Hydroxy-3-methylglutaryl coenzyme A reductase
HO-1 Heme oxygenase-1
Holo-Lf holo-lactoferrin
H2Bub1 H2B monoubiquitination
IDO indoleamine2,3-dioxygenase
IFN-γ interferon-γ
IR ionizing radiation
IRE iron response element
IRP iron regulatory proteins
LBP Lycium barbarum polysaccharide, Lipopolysaccharide Binding Protein
LOX lipoxygenase
LPCAT3 lysophosphatidylcholine acyltransferase 3
LPO lipid peroxide
MUFA Mono-unsaturated Fatty Acid
NCOA4 nuclear receptor coactivator 4
NF-κB nuclear factor-κB
Nrf2 nuclear factor erythroid
NQO1 NADH Dehydrogenase Quinone 1
OTUB1 OTU Deubiquitinase, Ubiquitin Aldehyde Binding 1
PDT Photodynamic therapy
PE phosphatidylethanolamine
PGM5P3-AS1 PGM5P3 antisense RNA 1
PLOOH phospholipid hydroperoxide
PLS phospholipids
POR P450 oxidoreductase
PUFA-PL polyunsaturated fatty acid phospholipids
PKCβII Protein kinase C-βII
RCD regulated cell death
RHOT1 Ras homolog family member T1
RNA pol II RNA polymerase II
ROS reactive oxygen species
RSV Rosuvastatin
RT radiotherapy
SAFE Sonodynamically amplified ferroptotic red blood cells
SAS Sulfasalazine
SDT sonodynamic therapy
SF silk fibroin
SIM simvastatin
SLC3A2 Solute carrier family 3 member 2
SLC7A11 Solute carrier family 7 member 11
SOD superoxide dismutase
SRF sorafenib
STAT3 signal transducer and activator of transcription 3
TAMs Tumor-associated macrophages
TF transferrin,
TFR1 Transferrin Receptor 1
TFR1 transferrin receptor 1,
TGF-β2 transforming growth factor-beta 2
TME tumor microenvironment
TNBC triple-negative breast cancer
TNF-α tumor necrosis factor-α
VC Vitamin C
27HC 27-hydroxycholesterol
USP7 ubiquitin-specific protease 7
Keywords: iron, breast cancer, ferroptosis, therapy, lipid
Citation: Liu Y, Hu Y, Jiang Y, Bu J and Gu X (2022) Targeting ferroptosis, the achilles’ heel of breast cancer: A review. Front. Pharmacol. 13:1036140. doi: 10.3389/fphar.2022.1036140
Received: 04 September 2022; Accepted: 31 October 2022;
Published: 16 November 2022.
Edited by:
Zhijie Xu, Xiangya Hospital, Central South University, ChinaReviewed by:
Xufeng Fu, Ningxia Medical University, ChinaCopyright © 2022 Liu, Hu, Jiang, Bu and Gu. This is an open-access article distributed under the terms of the Creative Commons Attribution License (CC BY). The use, distribution or reproduction in other forums is permitted, provided the original author(s) and the copyright owner(s) are credited and that the original publication in this journal is cited, in accordance with accepted academic practice. No use, distribution or reproduction is permitted which does not comply with these terms.
*Correspondence: Xi Gu, amFkZWd4QDE2My5jb20=
Disclaimer: All claims expressed in this article are solely those of the authors and do not necessarily represent those of their affiliated organizations, or those of the publisher, the editors and the reviewers. Any product that may be evaluated in this article or claim that may be made by its manufacturer is not guaranteed or endorsed by the publisher.
Research integrity at Frontiers
Learn more about the work of our research integrity team to safeguard the quality of each article we publish.