- 1Experimental Center, Shandong University of Traditional Chinese Medicine, Jinan, China
- 2Institute for Literature and Culture of Chinese Medicine, Shandong University of Traditional Chinese Medicine, Jinan, China
- 3Innovation Research Institute of Traditional Chinese Medicine, Shandong University of Traditional Chinese Medicine, Jinan, China
- 4Key Laboratory of Traditional Chinese Medicine for Classical Theory, Ministry of Education, Shandong University of Traditional Chinese Medicine, Jinan, China
- 5Shandong Provincial Key Laboratory of Traditional Chinese Medicine for Basic Research, Shandong University of Traditional Chinese Medicine, Jinan, China
The incidence of melanoma has increased rapidly over the past few decades, with mortality accounting for more than 75% of all skin cancers. The high metastatic potential of Melanoma is an essential factor in its high mortality. Vascular angiogenic system has been proved to be crucial for the metastasis of melanoma. An in-depth understanding of angiogenesis will be of great benefit to melanoma treatment and may promote the development of melanoma therapies. This review summarizes the recent advances and challenges of anti-angiogenic agents, including monoclonal antibodies, tyrosine kinase inhibitors, human recombinant Endostatin, and traditional Chinese herbal medicine. We hope to provide a better understanding of the mechanisms, clinical research progress, and future research directions of melanoma.
1 Introduction
Melanoma is one of the most aggressive and fatal skin cancer types, characterized by rapid growth, a long dormancy time, high rates of late-stage recurrence, and extensive metastasis (Eddy and Chen, 2020; Filippi et al., 2020). Its incidence has steadily increased over the past few decades, posing a significant threat to human health worldwide (Li et al., 2022). The considerable risk factor for Melanoma is UV radiation via direct DNA damage and harmful effects on the skin (Sample and He, 2018). Acquired and congenital nevus are also risk factors for melanoma (Li et al., 2019a). Approximately 25% of patients with melanoma develop from nevus, and 5%–15% of patients with a family history were susceptible to melanoma (Armstrong and Cust, 2017). Indeed, patients with melanoma who were diagnosed at an early stage could be cured by surgical removal. However, tumor metastasis always occurs after initial treatments and is a fundamental cause of the recurrence in patients with melanoma. Although, clinical therapeutic options for melanoma are plentiful, such as chemotherapy, immunotherapy, and other targeted therapies, the prognosis of advanced melanoma remains severe (Ramelyte et al., 2017; Xiao et al., 2019; Goldinger et al., 2022). Thus, a new and effective therapeutic method is still needed to treat melanoma.
Angiogenesis is a complex process of forming new blood vessels, generally regulated by pro-angiogenic and anti-angiogenic factors (Halder et al., 2018; Wang et al., 2018). However, it is not in dynamic balance in various solid tumors, such as melanoma (Luciano et al., 2021; Parmar and Apte, 2021). Developing a rich vascular network seems vital for melanoma cells during the vertical growth phase, because melanoma cells require lots of nutrients and oxygen to sustain their vertical growth (Pandita et al., 2021). Therefore, angiogenesis is essential for the occurrence and development of melanoma. In 1966, the concept of tumor angiogenesis in melanoma was first proposed by Warren and Shubik (Warren and Shubik, 1966). Since then, anti-angiogenic drugs have been identified as an essential therapeutic measure for treating melanoma (Liu et al., 2022a; Hu et al., 2022; Wohlfeil et al., 2022). These studies suggest that inhibiting angiogenesis will bring new insights into the treatment of melanoma.
In this review, we have elucidated the clinical trials and detailed mechanisms of anti-angiogenesis drugs in melanoma treatment, such as monoclonal antibodies (Bevacizumab, Ramucirumab, Aflibercept, Ontuxizumab), tyrosine kinase inhibitors (Sorafenib, Lenvatinib, Imatinib, Sunitinib, Pazopanib, Axitinib) and human recombinant Endostatin. At the same time, we will further discuss the anti-angiogenic activity of Traditional Chinese herbal medicine. In addition, we will also elucidate potential mechanisms of resistance to anti-angiogenic agents, giving an outlook on the specific targets which would be helpful to the successful therapy of malignant melanoma.
2 Mechanism of angiogenesis
Angiogenesis, forming new blood vessels depending on pre-existing vasculatures (Koo and Kume, 2013), is an essential indicator of tumor proliferation, survival, and distant metastasis in various solid tumors, including melanoma (Cho et al., 2019). Melanoma cells have acquired the ability to induce angiogenesis to meet the increasing nutritional and oxygen needs, especially when cells are in a vertical growth phase of continued proliferation (Straume et al., 1999; Sobierajska et al., 2020). Generally, pro-angiogenic and anti-angiogenic factors are in a dynamic balance (Kazerounian and Lawler, 2018). However, this balance of angiogenesis is often out of control in melanoma. As a result, large amounts of pro-angiogenic factors are release and the expression of the receptors of these factors upregulate in tumor cells. Pro-angiogenic factors will play a dominant role in angiogenesis, leading to the formation of new blood vessels (Rodrigues and Ferraz, 2020). Then, with an adequate supply of nutrients, tumor cells can increase rapidly without control and become more invasive, ultimately leading to metastasis. The growth factors and cytokines are the potential targets for angiogenesis and have been well-studied in melanoma therapy (Figure 1).
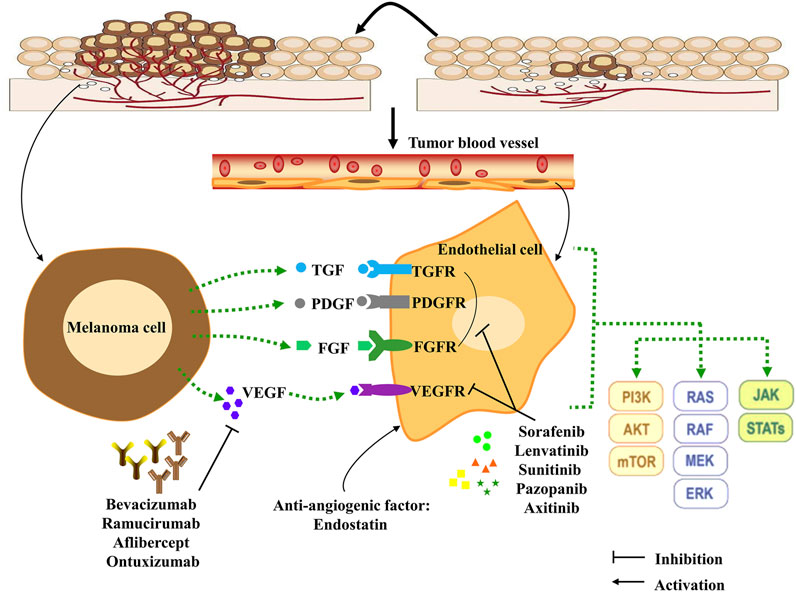
FIGURE 1. Angiogenesis, angiogenesis signaling pathways and anti-angiogenesis targets in melanoma cells. Pro-angiogenic factors are released by melanoma cells, and can bind receptors expressed on endothelial cells, which leads to initiation of the downstream signaling effects to stimulate melanoma proliferation, metastasis and differentiation. Combining this process with anti-angiogenesis compounds (monoclonal antibodies and TKIs) can effectively inhibit tumor angiogenesis. In addition, the anti-angiogenic factor Endostatin can interact with pro-angiogenic factors to influence angiogenesis in the tumor microenvironment. TKIs: tyrosine kinase inhibitors; TGF: transforming growth factor; TGFR: transforming growth factor receptor; VEGF: Vascular endothelial growth factor; VEGFR: Vascular endothelial growth factor receptor; PDGF: Platelet-derived growth factor; PDGFR: Platelet-derived growth factor receptor; FGF: Fibroblast growth factor; FGFR: Fibroblast growth factor receptor; PI3K: Phosphoinositide 3-kinases; STAT: Signal transducer and activator of transcription protein; JAK: Janus protein tyrosine kinase; MEK: Mitogen-activated protein kinase; mTOR: Mammalian target of rapamycin.
Some critical functional enzymes and adhesion factors have been discussed in melanoma, including vascular endothelial growth factor A (VEGF-A), placental growth factor (PlGF), interleukin-8 (IL-8), primary fibroblast growth factor (bFGF), platelet-derived growth factor (PDGF), angiopoietin (Ang), urokinase plasminogen activator (uPA), integrin, and MMPs (Singh et al., 2010; Laurenzana et al., 2017; Zhang et al., 2018a; Lacal and Graziani, 2018; Pekkonen et al., 2018; Zhang et al., 2019; Czarnecka et al., 2020; Ten Voorde et al., 2021). Vascular endothelial growth factor (VEGF), the first described cytokine, stimulates the formation of new blood vessels in tumors (Senger et al., 1983). There are seven types of this gene family, including VEGF-A, VEGF-B, VEGF-C, VEGF-D, VEGF-E, VEGF-F, and placental growth factors (Shibuya, 2011). Accordingly, the VEGF receptor (VEGFR) is a member of the tyrosine kinase receptor family, including five subtypes (VEGFR-1, VEGFR-2, VEGFR-3, NRP-1, and NRP-2) (Zhao et al., 2021). To our knowledge, VEGFRs are the most crucial factors in cancer angiogenesis. For instance, VEGF induces phosphorylation of VEGFR and activates its downstream signaling to enhance vascular expansion and permeability of tumor cells (Fernandez-Cruz et al., 2019). Meanwhile, the other factors (PlGF, IL-8, bFGF, PDGF, Ang, uPA, integrin, and MMPs) are also produced by melanoma cells and endothelial cells. These pro-angiogenic growth factors and cytokines commonly induce their downstream signaling effects through paracrine and autocrine mechanisms. Interestingly, their corresponding receptors are frequently overexpressed by melanoma cells (Peters et al., 2020). Many studies showed that inhibiting pro-angiogenic growth factors and cytokines could attenuate the formation of new blood vessels and inhibit angiogenesis (Muppala et al., 2017; Horvathova et al., 2019; Palanisamy et al., 2019). In this review, we summarized these clinical anti-angiogenic drugs and Traditional Chinese herbal medicine in melanoma (Table 1, Table 2, and Table 3), hoping to improve the clinical effectiveness of anti-angiogenic drugs in treating melanoma.
3 Anti-angiogenic agents in melanoma
3.1 Monoclonal antibody
3.1.1 Bevacizumab
Bevacizumab, a humanized VEGF monoclonal antibody, is the leading anti-angiogenic agent for clinical use in advanced melanoma (Corrie et al., 2018). It shows anti-tumor effects by preventing the binding of VEGF with its receptors and inhibiting the growth of endothelial cells and vessel formation (Presta et al., 1997). To evaluate the effects of Bevacizumab on patients with melanoma, 1344 patients (median age 56 years) who had resected cutaneous melanoma were recruited (Corrie et al., 2018). They were randomized into the adjuvant Bevacizumab (7.5 mg/kg intravenous every 3 weeks for 1 year) group and the standard observation group. Results showed that the overall survival (OS) at 5 years of the two groups was 64%. However, compared with the observation group, the disease-free interval (DFI) of the Bevacizumab group was 51%, implying that the DFI was improved in the Bevacizumab group. This clinical research also showed that patients with BRAF mutations tended to have poorer OS without Bevacizumab treatment (Corrie et al., 2018). Moreover, a phase II study assessed the activity of Bevacizumab in combination with paclitaxel and carboplatin in patients with advanced melanoma (Yan et al., 2021). 114 patients were enrolled in this research, and patients were randomly assigned to a CPB (carboplatin + paclitaxel + Bevacizumab) group and a CP (carboplatin + paclitaxel) group. The median progression-free survival (PFS) in the CPB group was 4.8 months, which was longer than that in the CP group (3.0 months). The overall response rate (ORR) of the two groups was 19.7% (CPB) and 13.2% (CP), respectively. Meanwhile, the median OS in the CPB group (13.6 months) was also significantly longer than in the CP group (9.0 months). A phase III trial was undertaken to evaluate the serum vitamin D in patients with resected stage IIB–IIIB melanoma after Bevacizumab treatment (Lipplaa et al., 2018). Patients with resected stage IIB-C and IIIA-C melanoma randomly receiving Bevacizumab (7.5 mg/kg every 3 weeks) or observation. One year later, vitamin D levels of patients did not predict prognostic markers DFI (HR = 0.98 per 10 nmol/L increase) or OS (HR = 0.96 per 10 nmol/L increase). Interestingly, longer DFI was observed in stage II melanoma patients after Bevacizumab treatment with higher vitamin D levels. Further exploration is warranted in the future.
3.1.2 Ramucirumab
Ramucirumab is a fully humanized anti-VEGF-2 monoclonal antibody that inhibits tumor growth and angiogenesis (Goode and Smyth, 2016; Jagiela et al., 2021). The safety, tolerability, and effectiveness of Ramucirumab when used alone or in combination with dacarbazine in patients with metastatic melanoma were assessed (Carvajal et al., 2014). In a phase II study, 106 patients with metastatic melanoma were enrolled from 14 centers in America. Patients received Ramucirumab at a dose of 10 mg/kg every 3 weeks (q3w) or Ramucirumab 10 mg/kg plus dacarbazine 1000 mg/m2 intravenously q3w. The Median PFS in the Ramucirumab group was 2.6 months compared with 1.7 months in the combination group. The Median OS of the Ramucirumab group was 8.7 months, and 11.1 months of combination therapy, respectively. (Carvajal et al., 2014). The combination group (Ramucirumab plus dacarbazine) showed safety at grade 3/4 toxicities. In conclusion, the preliminary effectiveness of Ramucirumab plus dacarbazine demonstrated the importance of VEGFR-2 inhibition in treating metastatic melanoma. A phase Ia/Ib study of LY3300054 (a new programmed cell death ligand 1 (PD-L1) inhibitor) as monotherapy or in combination with Ramucirumab, neratinib (a type II MET kinase inhibitor) or abemaciclib in patients with solid tumors was conducted. As a result, LY3300054 was well tolerated when administered alone or concurrently with Ramucirumab. No adverse events associated with the combination were observed. Durable clinical effects were observed in LY3300054 dose (phase Ia) as monotherapy or combined with Ramucirumab (Patnaik et al., 2021).
3.1.3 Aflibercept
Aflibercept (VEGF Trap) is a selective humanized IgG1 monoclonal antibody, which can block the interaction of VEGF and its receptors (VEGFR1 and VEGFR2). Angiogenesis could cause immune suppressions in multiple solid tumors (Li et al., 2021). To investigate the effects of the therapy (Ziv-Aflibercept + pembrolizumab) on melanoma, a phase IB trial was conducted (Rahma et al., 2022). Ziv-Aflibercept (2–4 mg/kg) or pembrolizumab (2 mg/kg) was administered intravenously every 2 weeks. No dose-limiting toxicities were observed at the initial dose level, and 2 of 33 patients had a complete response, and 1 had a partial response. The combination group showed an acceptable safety profile with anti-tumor activity in melanoma. The study is currently being carried out in patients with anti-PD-1-resistant melanoma (NCT02298959) (Rahma et al., 2022). The VEGF family takes a pivotal part in mediating tumor and lymph angiogenesis as well as the innate and adaptive immunities of the host (Fagiani et al., 2016; Boudria et al., 2019; Gloger et al., 2020; Menzel et al., 2020). As reported, Interleukin-2 (IL-2), a growth factor for T and NK cells, plays a significant role in melanoma (Pretto et al., 2014; Lee et al., 2016). To investigate the effect of Aflibercept with IL-2 on metastatic melanoma, a phase II study was implemented (Tarhini et al., 2018). 89 patients were enrolled and randomly divided into the combination group (Aflibercept + IL-2) or the IL-2 isolated group. Results showed that the PFS of the combination group was 6.9 months, and that of the IL-2 alone group was 2.3 months. Although there were no significant differences in OS between the two groups, the PFS of IL-2 and Ziv-Aflibercept group significantly improved compared with IL-2 alone group, suggesting that anti-VEGF combined with immunosuppressive agents might be an excellent therapeutic option for patients with melanoma.
3.1.4 Ontuxizumab
Ontuxizumab (MORAB-004), the first monoclonal antibody that interferes with the function of Endosialin, plays an essential role in tumor growth and angiogenesis. The clinical activity and tolerability of Ontuxizumab were evaluated in a phase II study (D’Angelo et al., 2018). In this trial, patients with metastatic melanoma who had received at least one prior systemic treatment received Ontuxizumab weekly at 2 or 4 mg/kg. The median PFS was 8.3 weeks in the Ontuxizumab group. Moreover, the overall grade 1 or 2 adverse events were nausea (36.8%), headache (55.3%), chills (42.1%), and fatigue (48.7%). In summary, the effectiveness of Ontuxizumab (2 or 4 mg/kg) in melanoma was poor. Clinical trials aimed at evaluating the effectiveness of Ontuxizumab alone, and in combination with other active drug may yield better results.
3.2 Tyrosine kinase inhibitors
Tyrosine kinase inhibitors (TKIs) are involved in tumorigenesis and progression, which aims to inhibits the catalytic function of kinases and then blocks the activation of downstream signaling cascade (Bellantoni and Wagner, 2021; Salmaso et al., 2021). In recent years, TKIs have identified as critical targets for drug discovery (Choi et al., 2021; Mohammadi and Gelderblom, 2021; Yang et al., 2022). Summary of clinical stage and ongoing evaluation of TKIs might contribute to a comprehensive understanding of TKIs therapies in cancer.
3.2.1 Sorafenib
Sorafenib is a raf kinase inhibitor, which can also inhibit the tyrosine kinase activity of various receptors, including VGFR-2, VEGF-3, PDGF-β, KIT, FLT-3, and other receptors. Sorafenib has dual anti-tumor effects (Ortega-Muelas et al., 2021), which can not only directly inhibit the proliferation of tumor cells by mediating RAF/MEK/ERK pathway but also cut off the nutrition of tumor cells through inhibiting the formation of new blood vessels (Spirli et al., 2012). To evaluate the safety, effectiveness, health-related quality of life (HRQoL), and the non-progression rate of Sorafenib (800 mg per day), a multicenter, single-arm phase II trial was conducted in patients with metastatic uveal melanoma. (Mouriaux et al., 2016). After 24 weeks of oral administration, the PFS and OS in the Sorafenib group were 31.2% and 62.5%, respectively. However, 41.4% of patients required dose adjustment due to toxicity and without improvement of HRQoL. Simultaneously, to evaluate the safety and effectiveness of Sorafenib plus chemotherapy (gemcitabine or cisplatin) in metastatic melanoma patients with collecting duct carcinoma, a randomized, single-arm, and multicenter study was carried out (Sheng et al., 2018). The data showed the median OS in the combination group (Sorafenib plus chemotherapy) was about 12.5 months, and the ORR was 30.8%. Delightfully, the PFS for Sorafenib plus chemotherapy was improved in metastatic melanoma patients with CDC.
3.2.2 Lenvatinib
Lenvatinib (E7080) is an oral multiple tyrosine kinase inhibitor (TKI) that shows effects on VEGFR1-3, FGFR1-4, PDGFR, and KIT to inhibit tumor angiogenesis (Okamoto et al., 2013). Moreover, Lenvatinib inhibits human umbilical vein endothelial cell proliferation and tube formations to reduce tumor growth (Capozzi et al., 2019; Iwasa et al., 2020). In a phase I trial, the safety and clinical effectiveness of Lenvatinib were assessed in patients (n = 77) with melanoma (Hong et al., 2015). 18 patients received Lenvatinib at a dose of .1–3.2 mg twice a day (BID) (7 days on, 7 days off), while 33 patients received it at an amount of 3.2–12 mg BID and the dose of 10 mg BID in 26 patients, respectively. Preliminary results from this phase I trial shows that Lenvatinib had partial clinical response of 15.6% with a stable disease (SD) ≥23 weeks. The authors also found the dose-limiting toxicities of Lenvatinib included fatigue, hypertension, and proteinuria (Hong et al., 2015). Besides, a decrease in the angiopoietin-1 ratio was considered a significant factor associated with prolonged PFS in melanoma patients. At the same time, angiogenesis and apoptosis-related biomarkers were related to PFS in melanoma patients treated with Lenvatinib. Subsequently, a multicenter, open-label phase Ib/II study (Arance et al., 2022) was taken in melanoma (ClinicalTrials.gov identifier: NCT03776136). Briefly, 103 patients with melanoma were enrolled. Preliminary results showed that the median study follow-up was 15.3 months, and ORR in the total population was 21.4%. The adverse events occurred in 47 (45.6%) patients in Grades 3–5 (Arance et al., 2022). Accordingly, the anti-angiogenic therapy combined with immunotherapy displays promising anti-tumor activities and expected safety profiles in patients with melanoma.
3.2.3 Imatinib
A successful TKI, Imatinib, demonstrates its anti-angiogenic activity against multiple targets, including v-Abl, c-Kit, and PDGFR (Knol et al., 2015). Recent reports showed that c-Kit mutations were more common in acral and mucosal melanomas (Knol et al., 2015; Sabbah et al., 2021). In a study, 130 KIT-altered melanoma patients were pooled from five medical centers (Jung et al., 2022). Mucosal melanoma was associated with high PFS, whereas exon 17 mutations were associated with low PFS. Imatinib has shown significant activity as a therapeutic agent in metastatic melanoma patients harboring aberrations in c-Kit. Thereby, promising prospects of Imatinib in melanoma applications could occur in the future.
3.2.4 Sunitinib
Sunitinib, an oral multi-kinase inhibitor, has been used in melanoma and targets VEGF receptor, KIT receptor, and other receptors (Yeramian et al., 2012). It is well known that the VEGF and KIT are potential targets for alternative therapeutics in malignant melanoma, and they are recognized to play a pivotal role in the pathogenesis and metastasis of melanoma (Graells et al., 2004; Curtin et al., 2006). Based on the part of VEGF and KIT in melanoma, they conducted a phase II trial of Sunitinib for patients with acral and mucosal melanomas (Buchbinder et al., 2015). Patients received 37.5 mg and 50 mg Sunitinib daily. The results showed that the toxicity was acceptable, and the disease control rate was 44%. Fortunately, 20% or more of the patients were alive, and progression-free at 2 months, encouraging the activity of Sunitinib in acral and mucosal melanomas. The results indicated Sunitinib had greater effectiveness in patients with primary KIT exon 9 mutations or wild-type status than in those with direct KIT exon 11 mutations. However, the responses of Sunitinib in patients with non-KIT-mutated indicated Sunitinib might have other targets associated with melanoma growth. The tolerance of Sunitinib was poor, and no lengthy response was observed in patients. With the multi-target features, a combination of Sunitinib and other inhibitors might provide a considerable promise in the future.
3.2.5 Pazopanib
Pazopanib is an oral TKI that binds to VEGFR1-3, c-KIT, PDGFR-α, and PDGFR-β, which are often abnormally activated during tumorigenesis (Goh et al., 2010). A clinical trial using Pazopanib combined with various cytotoxic chemotherapies was investigated in BRAF wild-type metastatic melanoma patients (Fruehauf et al., 2018). 60 patients were included in this study and received Pazopanib and paclitaxel. The final dates displayed that the combination of the Pazopanib plus paclitaxel was well-tolerated, and the significant activity was close to the current first-line therapy for metastatic melanoma. Moreover, the immunological events and metabolic responses induced by Pazopanib plus paclitaxel was evaluated in a study. 90 patients received Pazopanib/paclitaxel, Pazopanib was given 400 mg, BID. Paclitaxel was given 150 mg/m2 body surface. Interestingly, they observed that melanoma cells could be rescued by M2 macrophages after Pazopanib treatment (Thurneysen et al., 2016). Thus, therapies that inhibit tumor-associated macrophages might be feasible and have potential for melanoma patients.
3.2.6 Axitinib
Axitinib is a TKI that targets VEGFR-1, VEGFR-2, c-SRC, Kit, and RET (Roviello et al., 2016). Preclinical studies had demonstrated the vital role of the VEGF signaling pathway in melanoma (Peng et al., 2016). Importantly, Yuan et al. (2022) conducted a single-center, single-arm phase II trial in patients with advanced recurrent melanoma. Patients orally received Axitinib daily. Regarding the toxicity, results elaborated that Axitinib was well tolerated, and the observed toxicity levels were mild and manageable. In summary, Axitinib might play a vital role in metastatic melanoma, and further investigations of Axitinib alone or in combination with chemotherapy should be taken.
Mucosal Melanoma is a severe natural disease (Lian et al., 2017). Unfortunately, due to the rarity, there are no well-established therapeutic guidelines for treating mucosal melanoma (Yi et al., 2011; Lian et al., 2017). In the past decade, the application of targeted therapies and immunotherapies has brought light in metastatic cutaneous melanoma treatment (Kaufman et al., 2018). Xinan Sheng et al. (2019) had reported the safety and effectiveness of toripalimab and Axitinib in patients with advanced melanoma. They found high response rates (48.3% ORR) and prolonged median PFS for toripalimab and Axitinib. Moreover, tumor mutational burden (TMB) and PD-L1 expression were related to higher ORR, consistent with previous reports (Hellmann et al., 2018). Interestingly, three published signatures (angiogenesis signatures, inflammation signatures, and interferon-gamma signatures) for clinical outcomes were also discussed in their study (Hellmann et al., 2018), which might be relevant biomarkers for immuno-oncology plus VEGF therapy. In summary, Axitinib combined with toripalimab could be a promising option for mucosal melanoma treatment. Subsequently, a phase III study should be validated among non-Asian patients in the future.
3.3 Endostatin
Endostatin (20-kDa), a potent endogenous angiogenesis inhibitor, is the C-terminal fragment of type XVIII collagen (O'Reilly et al., 1997). Since 1997, Endostatin has shown anti-angiogenic effects on endothelial cells (Alahuhta et al., 2015; Jia et al., 2017; Lamattina et al., 2019; Wang et al., 2021a; Shin et al., 2021; Zhu et al., 2022). In 2005, Endostatin was approved by the Food and Drug Administration of China to treat non-small-cell lung cancer (NSCLC) (Wang et al., 2005; Han et al., 2011). However, the clinical effectiveness of Endostatin is controversial, which needs further investigation in the treatment of patients with metastatic melanoma. A real-world study was designed to evidence the effectiveness and safety of Endostatin plus chemotherapy for treating patients with metastatic melanoma (Zhang et al., 2022). In this trial, 43 patients with advanced or recurrent mucosal melanoma were recruited from Fudan University Shanghai Cancer Center (April 2017 and August 2020). They were randomly assigned to the two arms (dacarbazine plus cisplatin arm, temozolomide plus cisplatin). Simultaneously, patients in the two arms received a placebo or Endostatin (105 mg/m) intravenously for 168 h. At the end of this trial, the PFS and OS were 4.9 and 15.3 months, respectively. Endostatin plus chemotherapy represented well tolerability and a manageable toxicity profile (Zhang et al., 2022). Overall, Endostatin provided a novel option for anti-angiogenic treatment.
Endostatin has been recently identified as a prognostic biomarker for patients with metastatic melanoma (Nyakas et al., 2019). Many studies showed Endostatin levels were closely associated with aggressive phenotypes or poor outcomes in various malignancies (Alahuhta et al., 2015; Chen et al., 2018; Zamaratskaia et al., 2020; Zhang et al., 2021a; Zhu et al., 2022), such as metastatic melanoma (Fukuda et al., 2011; Liang et al., 2018; Zhang et al., 2022). A phase IV study demonstrated Endostatin could influence melanoma invasion by regulating T Cell activation (Nyakas et al., 2019). As a prognostic biomarker for metastatic melanoma patients, Endostatin might be helpful in selecting patients for anti-angiogenic therapy.
3.4 Traditional Chinese herbal medicine
In recent years, anti-cancer compounds extracted from Traditional Chinese Medicine (TCM) have become a research hotspot. Several studies have reported significant anti-angiogenic activities of these compounds in melanoma, the underlying mechanisms of which are still being studied. Firstly, Betulinic Acid (BA), an extract from the plane and birch trees, has shown anti-angiogenic effects in melanoma. An in vitro study indicates that BA significantly inhibits the proliferation of melanoma cell lines (Wroblewska-Luczka et al., 2022). Interestingly, the combination of BA with paclitaxel or docetaxel indicates ideal drug-drug synergy interactions (Wroblewska-Luczka et al., 2022). Another study reports that BA demonstrated inhibitory effects on A375 melanoma cells via mitochondrial apoptosis and glycolysis pathway (Coricovac et al., 2021). Next, genistein (GS), derived from the soybean, is a powerful anti-angiogenic agent in melanoma. It is reported that GS shows an effect on the Prostaglandin E2 (PGE2) pathway, which has been proven as essential for its anti-melanoma activity. Furthermore, the overexpression of IL-8 could be induced by GS through one of the PGE2 receptors (EP3) in melanoma cells (Venza et al., 2018). Simultaneously, apigenin, which is a naturally occurring flavonoid in vegetables, fruits, celery, and parsley, could inhibit the proliferation and angiogenesis of melanoma cells by suppressing the secretion of TNF-α and influencing PI3K/Akt/mTOR signaling pathway (Li et al., 2019b; Ghitu et al., 2019; Ghitu et al., 2021). Furthermore, jatrorrhizine hydrochloride (JH), a component of Coptis Chinensis, shows anti-metastatic and anti-proliferation effects on C8161 human melanoma cells. Mechanistic studies showed that JH induced G0/G1 cell cycle arrest in C8161 tumor cells. Moreover, JH reduced the neovascularization of C8161 cells and disturbed the expression of VE-cadherin, suggesting that JH is a new potential anti-melanoma drug candidate (Liu et al., 2013). To explore other effective strategies for treating melanoma, Vaid M et al. assessed the effects of Silymarin (an extract of Silybum marianum) on melanoma cells. The data showed the therapeutic effect of Silymarin was associated with angiogenic biomarkers (Vaid et al., 2015). Besides, honokiol, a compound isolated from the Magnolia tree, has a therapeutic impact on skin cancer (Leeman-Neill et al., 2010). More interestingly, the anti-angiogenic functions of some of these TMCs are strongly associated with hypoxia-inducible-factor (HIF) and other pro-angiogenic genes (Vavilala et al., 2012). For instance, Parthenolide (PT), an active component of the medicinal herb Feverfew, exhibits an anti-angiogenic effect by regulating the NF-кB/AP-1/VEGF signaling pathway, encouraging a promising agent for melanoma treatments (Talib and Al Kury, 2018; Tian et al., 2020). Particularly, Cryptotanshinone (CPT), isolates from Salvia miltiorrhiza, takes a crucial role in angiogenesis-related diseases. Zhang et al. (2018b) reported that CPT prevented the growth and metastasis of colon cancer cells via modulating PI3K/Akt/mTOR signaling, MMP/TIMP system, and HIF-1α nuclear translocation (Zhu et al., 2016). However, the clinical use of TCM still has severe limitations, which often reduce their therapeutic effectiveness. Consequently, it is urgent to improve their anti-tumor activities in patients.
4 Mechanisms of resistance
Angiogenesis plays a crucial role in regulating vital functions of tumor cells, including tumor growth, proliferation, and metastasis (Papaevangelou et al., 2018; Zhang et al., 2021b; Rampino et al., 2021). In recent years, multiple anti-angiogenic agents have been developed to treat melanoma (Huang et al., 2021a; Micheli et al., 2021). However, due to acquired resistance (Jimenez-Valerio and Casanovas, 2017; Pozas et al., 2019; Kuczynski and Reynolds, 2020; Watanabe, 2021), anti-angiogenic agents are limited, including vascular mimicry (VM), vascular co-option, metabolic symbiosis, upregulation of alternative pathways, and recruitment of tumor stromal cells. Moreover, autophagy, a highly mediated adaptive process of cancers, has been implicated in perturbing resistance to anti-angiogenic therapy. Firstly, a recent study determined the inhibitory effect of the BRAFV600E inhibitor vemurafenib on VM in invasive melanoma cells. As a result, vemurafenib failed to inhibit the VM ability of A375 melanoma cells in vitro (Andreucci et al., 2022). Another critical factor, vessel co-option, seems to play an essential role in mediating resistance to anti-angiogenic drugs (Kuczynski and Reynolds, 2020). For instance, several studies have shown vessel co-option is associated with primary melanoma and organ (brain, lung, and liver) metastases of melanoma (Lugassy et al., 2014; Szabo et al., 2015; Bentolila et al., 2016; Barnhill et al., 2018; Rodewald et al., 2019), which may be an essential factor for poor clinical effectiveness of anti-angiogenic drugs. Secondly, in terms of the resistance mechanism to anti-angiogenic therapy, metabolic symbiosis is also reported in both experimental and clinical studies (Jimenez-Valerio et al., 2016; Sebestyen et al., 2021). Both OXPHOS and glycolysis (metabolic symbiosis) have been identified to be critical for metabolic plasticity in melanoma, driving acquired resistance to anti-angiogenic chemotherapy (Kumar et al., 2021). Furthermore, the abnormal upregulation of both OXPHOS and glycolysis is significant for melanoma progression (Feichtinger et al., 2018; Ruocco et al., 2019). Thus, inhibition of glycolysis may be a promising strategy to overcome Bevacizumab resistance (Eriksson et al., 2018). Although anti-VEGF therapy is available, drug resistance often occurs, and malignant tumor patients are not always responsive. This acquired resistance to anti-VEGF treatment is involved in other angiogenic pathways, compensating for the inhibiting effects on cancer cells (Li et al., 2014; Choi et al., 2015; Mahdi et al., 2019; Yin et al., 2019). Consequently, combined with multitargeted inhibitors can refrain angiogenesis more efficiently than monotherapy therapy.
Drug resistance and tumor angiogenesis are affected by the tumor microenvironment (TME) (Maacha et al., 2019), which is composed of stromal cells, immune cells, cancer stem cells (CSCs), blood vessels, tumor cells, lymphatic vessels, and extracellular matrix (ECM) (Liu et al., 2022b). TME is a complex network of tumor cells and surrounding components, where various associated cells and components communicate to regulate tumor growth (De Palma et al., 2017; Wang et al., 2021b). Tumor cells generally prefer a hypoxic environment (Huang et al., 2021b). Unfortunately, long-term use of anti-angiogenic drugs often aggravates hypoxia. Hypoxia-induced upregulation of hypoxia-inducible factor (HIF)-1a can induce the differentiation of tumor cells into CSCs, which is also the main contribution to drug-resistance of anti-angiogenic therapy (Zheng et al., 2018; Jiang et al., 2020). As the host immune system is often disrupted in cancer patients, the increased number of immunosuppressive cells, such as tumor-mass associated macrophages (TAMs), T-regs, and myeloid-derived suppressor cells (MDSCs), will be is responsible for an unfavorable prognosis of cancer treatment (Hosseini et al., 2019; Qi et al., 2020). For this reason, stromal cells, a critical surrounding components of tumor cells, might act as potential therapeutic targets for tumor cells (Licarete et al., 2020). As discussed above, resistance mechanisms of anti-angiogenic therapy have been elucidated in cancer, most of which occurred at the later stage of tumor progression. Different from those, autophagy seems to be the first defense process that appears at the cellular level without extracellular matrix (ECM) or tissue remodeling and needs to understand better (Chandra et al., 2020; Jena et al., 2021; Wen et al., 2022). Additional studies have revealed autophagy as a resistance mechanism and could enhance anti-angiogenic therapeutic effects (Huang et al., 2018; Zhao et al., 2018; Malhotra et al., 2019). However, whether early or late autophagy inhibitors will overcome the resistance of anti-angiogenic therapy is imperative to determine.
5 Summary and future directions
Anti-angiogenic therapy for tumors has achieved specific clinical efficacies (Qin et al., 2019; Tong et al., 2019; Liu et al., 2021a; Yetkin-Arik et al., 2021; Choi et al., 2022), and mainly manifests the improvements of PFS, which is consistent with the fact that angiogenesis is a marker of cancer. The in-depth studies on tumor angiogenesis will become a hot topic in tumor research. In this review, we discussed the critical roles of angiogenesis in melanoma growth and progression. Angiogenesis is a highly complex and dynamic process mediated by pro-angiogenic and angiogenesis inhibitory factors, which is the basis of anti-angiogenesis resistance (Tiwari et al., 2018; Cho et al., 2019). We also discussed the limitations of anti-angiogenic therapies, challenges, safety, predictive biomarkers, and future directions. Simultaneously, we explained Traditional Chinese herbal medicine as a vital anti-angiogenesis option in melanoma therapy.
Angiogenesis provides an essential target for multiple therapeutic agents, including Bevacizumab. VEGF is overexpressed and associated with prognosis in melanoma patients (Liu et al., 2021b). However, anti-angiogenic therapy is not as effective as initially hoped, and drug resistance always occurs in patients with melanoma, especially in those treated with Bevacizumab monotherapy (Jour et al., 2016; Zhang et al., 2020). Combinational therapies might be advantageous as they have multi-mechanisms targeting individual ligands and receptors to avoid resistance. For instance, activating mutations at V600 of the BRAF gene is common in several cancers (Halle and Johnson, 2021), including approximately 50% of melanoma (Gutierrez-Castaneda et al., 2020). Therefore, BRAF/MEK inhibitors have been developed to treat patients with BRAF-mutant melanoma. Moreover, due to the development of drug resistance and tumor recurrence, patients with BRAF-mutant melanoma have a short response time to BRAF/MEK inhibitors (Kakadia et al., 2018). Fortunately, angiogenesis inhibitors might be suitable for BRAF-mutant melanoma patients with acquired resistance to BRAF/MEK inhibitors (Amann et al., 2017; Martin et al., 2018; Atzori et al., 2020). Besides, there is a rationale for anti-angiogenic drugs combined with PARP inhibitors. Combining PARP inhibitors with anti-angiogenic drugs could provide synergetic benefits to patients with solid tumors (Tentori et al., 2007; Ledermann, 2017; Russo and Giavazzi, 2018; Sachdev et al., 2019; Smith and Pothuri, 2022). Mechanistically, PARP1 is associated with the stabilizing of HIF-1α (Hulse et al., 2018), which plays a vital role in melanocyte transformation and represents an essential feature in malignant tumor growth, including melanoma (Malekan et al., 2021).
The therapy of malignant tumors has opened the era of immunotherapy (Wang et al., 2021c; Zhang and Xiao, 2021; Zimmermannova et al., 2021). Both immune checkpoint inhibitors (CPIs) and anti-angiogenic agents have been widely used in melanoma treatment. Numerous trials assessing the effectiveness and safety of anti-angiogenic agents plus CPIs have been taken. The combined strategy is frequent in clinical trials for patients with unresectable stage III or IV melanoma. Most importantly, the combined approaches of Bevacizumab and ipilimumab might synergistically increase the infiltration of CD163+ dendritic macrophages and CD8+ T Cells via tumor vasculatures (Hodi et al., 2014; Ott et al., 2015). Another combination study of Lenvatinib and pembrolizumab has shown manageable safety and promising anti-tumor activity in patients with melanoma (Taylor et al., 2020). Overall, immunotherapy combined with anti-angiogenic agents does bring survival benefits to patients. Unfortunately, there are currently no drugs that can successfully target both immune systems and blood vessels. Hence few of these drugs could improve tumor progression effectively without adverse reactions or drug resistance. We hope to develop specific targeted inhibitors that affect both immunity and blood vessels to achieve satisfactory anti-tumor effects and prolong the survival of patients in the future, such as evaluating the potential synergistic effects of combined immunotherapy with VEGF inhibitors.
Although modulating angiogenesis appears to be a potential strategy for melanoma treatment, vascular disrupting agents have poor selectivity to distinguish tumor blood vessels from normal blood vessels, thus limiting their ability to suppress tumor growth (Mukherjee et al., 2020; Smolarczyk et al., 2021). The development of drugs that selectively target tumor blood vessels and angiogenesis drivers may be a direction in the future. Furthermore, vascular normalization could not only improve the delivery ratios of drugs but also enhance the therapeutic effects of combination therapies (Zhou and Gallo, 2009). Interestingly, anti-angiogenic treatments could affect tumor vessel normalization, which has synergistic effects when combined with radiotherapy, chemotherapy, immunotherapy strategies, and other therapeutic methods (Viallard and Larrivee, 2017; Liu et al., 2021c; Wang et al., 2021d; Une et al., 2021). However, the main problem of anti-angiogenic therapies is how to confirm the optimized time point and suitable dose of anti-angiogenic agents, which is particularly relevant to expand the vascular normalization window and obtaining the most extended survival time of cancer patients. In addition, prognostic markers, including PFS and PSA responses, did not display their suitability in determining the activity of angiogenesis inhibitors, calling for more energy in this setting. We highlighted the understanding of the molecular pathways that contributed to the development and progression of melanoma, as well as the specific molecular markers and predictors of each melanoma subtype. However, it is debatable whether or not anti-angiogenic therapy should be used as preoperative or perioperative treatment, which needs to be further explored in cancers. Although the efficacies of anti-angiogenic drugs need to be further improved, anti-angiogenic therapies have become an essential milestone in the history of human cancer treatment. It is expected to enhance the effectiveness of anti-angiogenic drugs by understanding the mechanisms of drug resistance and identifying its reliable predictive markers. In conclusion, the drug resistances, side effects, limited survival advantage, and high cancer recurrence rates highlight the critical need for new targets and strategies for anti-angiogenic therapies.
Author contributions
All authors listed have made a substantial, direct, and intellectual contribution to the work and approved it for publication.
Funding
This work was funded by the National Key Research and Development Program of China (No. SQ2017YFC170600), “Taishan Scholar’’ program, the Key Research and Development Program of Shandong province (No. 2016CYJS08A01-1) and the Natural Science Foundation of Shandong Province of China (ZR2022QH185).
Conflict of interest
The authors declare that the research was conducted in the absence of any commercial or financial relationships that could be construed as a potential conflict of interest.
Publisher’s note
All claims expressed in this article are solely those of the authors and do not necessarily represent those of their affiliated organizations, or those of the publisher, the editors and the reviewers. Any product that may be evaluated in this article, or claim that may be made by its manufacturer, is not guaranteed or endorsed by the publisher.
References
Alahuhta, I., Aikio, M., Vayrynen, O., Nurmenniemi, S., Suojanen, J., Teppo, S., et al. (2015). Endostatin induces proliferation of oral carcinoma cells but its effect on invasion is modified by the tumor microenvironment. Exp. Cell Res. 336, 130–140. doi:10.1016/j.yexcr.2015.06.012
Amann, V. C., Ramelyte, E., Thurneysen, S., Pitocco, R., Bentele-Jaberg, N., Goldinger, S. M., et al. (2017). Developments in targeted therapy in melanoma. Eur. J. Surg. Oncol. 43, 581–593. doi:10.1016/j.ejso.2016.10.014
Andreucci, E., Laurenzana, A., Peppicelli, S., Biagioni, A., Margheri, F., Ruzzolini, J., et al. (2022). uPAR controls vasculogenic mimicry ability expressed by drug-resistant melanoma cells. Oncol. Res. 28, 873–884. doi:10.3727/096504021X16273798026651
Arance, A., de la Cruz-Merino, L., Petrella, T. M., Jamal, R., Ny, L., Carneiro, A., et al. (2022). Phase II LEAP-004 study of lenvatinib plus pembrolizumab for melanoma with confirmed progression on a programmed cell death protein-1 or programmed death ligand 1 inhibitor given as monotherapy or in combination. J. Clin. Oncol., JCO2200221. doi:10.1200/JCO.22.00221
Armstrong, B. K., and Cust, A. E. (2017). Sun exposure and skin cancer, and the puzzle of cutaneous melanoma: a perspective on fears et al. Mathematical models of age and ultraviolet effects on the incidence of skin cancer among whites in the United States. American journal of epidemiology 1977; 105: 420-427. Cancer Epidemiol.Cancer Epidemiol. 10548, 420147–427156. doi:10.1016/j.canep.2017.04.004
Atzori, M. G., Ceci, C., Ruffini, F., Trapani, M., Barbaccia, M. L., Tentori, L., et al. (2020). Role of VEGFR-1 in melanoma acquired resistance to the BRAF inhibitor vemurafenib. J. Cell. Mol. Med. 24, 465–475. doi:10.1111/jcmm.14755
Barnhill, R., Vermeulen, P., Daelemans, S., van Dam, P. J., Roman-Roman, S., Servois, V., et al. (2018). Replacement and desmoplastic histopathological growth patterns: a pilot study of prediction of outcome in patients with uveal melanoma liver metastases. J. Pathol. Clin. Res. 4, 227–240. doi:10.1002/cjp2.105
Bellantoni, A. J., and Wagner, L. M. (2021). Pursuing precision: receptor tyrosine kinase inhibitors for treatment of pediatric solid tumors. Cancers (Basel) 13, 3531. doi:10.3390/cancers13143531
Bentolila, L. A., Prakash, R., Mihic-Probst, D., Wadehra, M., Kleinman, H. K., Carmichael, T. S., et al. (2016). Imaging of angiotropism/vascular Co-option in a murine model of brain melanoma: implications for melanoma progression along extravascular pathways. Sci. Rep. 6, 23834. doi:10.1038/srep23834
Boudria, A., Abou Faycal, C., Jia, T., Gout, S., Keramidas, M., Didier, C., et al. (2019). VEGF165b, a splice variant of VEGF-A, promotes lung tumor progression and escape from anti-angiogenic therapies through a β1 integrin/VEGFR autocrine loop. Oncogene 38, 1050–1066. doi:10.1038/s41388-018-0486-7
Buchbinder, E. I., Sosman, J. A., Lawrence, D. P., McDermott, D. F., Ramaiya, N. H., Van den Abbeele, A. D., et al. (2015). Phase 2 study of Sunitinib in patients with metastatic mucosal or acral melanoma. Cancer 121, 4007–4015. doi:10.1002/cncr.29622
Capozzi, M., De Divitiis, C., Ottaiano, A., von Arx, C., Scala, S., Tatangelo, F., et al. (2019). Lenvatinib, a molecule with versatile application: from preclinical evidence to future development in anti-cancer treatment. Cancer Manag. Res. 11, 3847–3860. doi:10.2147/CMAR.S188316
Carvajal, R. D., Wong, M. K., Thompson, J. A., Gordon, M. S., Lewis, K. D., Pavlick, A. C., et al. (2014). A phase 2 randomised study of Ramucirumab (IMC-1121B) with or without dacarbazine in patients with metastatic melanoma. Eur. J. Cancer 50, 2099–2107. doi:10.1016/j.ejca.2014.03.289
Chandra, A., Rick, J., Yagnik, G., and Aghi, M. K. (2020). Autophagy as a mechanism for anti-angiogenic therapy resistance. Semin. Cancer Biol. 66, 75–88. doi:10.1016/j.semcancer.2019.08.031
Chen, J., Yao, Q., Huang, M., Wang, B., Zhang, J., Wang, T., et al. (2018). A randomized Phase III trial of neoadjuvant recombinant human endostatin, docetaxel and epirubicin as first-line therapy for patients with breast cancer (CBCRT01). Int. J. Cancer 142, 2130–2138. doi:10.1002/ijc.31217
Cho, W. C., Jour, G., and Aung, P. P. (2019). Role of angiogenesis in melanoma progression: Update on key angiogenic mechanisms and other associated components. Semin. Cancer Biol. 59, 175–186. doi:10.1016/j.semcancer.2019.06.015
Choi, H. J., Armaiz Pena, G. N., Pradeep, S., Cho, M. S., Coleman, R. L., and Sood, A. K. (2015). Anti-vascular therapies in ovarian cancer: moving beyond anti-VEGF approaches. Cancer Metastasis Rev. 34, 19–40. doi:10.1007/s10555-014-9538-9
Choi, G., Kim, D., and Oh, J. (2021). AI-based drug discovery of TKIs targeting L858R/T790M/C797S-mutant EGFR in non-small cell lung cancer. Front. Pharmacol. 12, 660313. doi:10.3389/fphar.2021.660313
Choi, S. H., Yoo, S. S., Lee, S. Y., and Park, J. Y. (2022). Anti-angiogenesis revisited: reshaping the treatment landscape of advanced non-small cell lung cancer. Arch. Pharm. Res. 45, 263–279. doi:10.1007/s12272-022-01382-6
Coricovac, D., Dehelean, C. A., Pinzaru, I., Mioc, A., Aburel, O. M., Macasoi, I., et al. (2021). Assessment of betulinic Acid cytotoxicity and mitochondrial metabolism impairment in a human melanoma cell line. Int. J. Mol. Sci. 22, 4870. doi:10.3390/ijms22094870
Corrie, P. G., Marshall, A., Nathan, P. D., Lorigan, P., Gore, M., Tahir, S., et al. (2018). Adjuvant Bevacizumab for melanoma patients at high risk of recurrence: survival analysis of the AVAST-M trial. Ann. Oncol. 29, 1843–1852. doi:10.1093/annonc/mdy229
Curtin, J. A., Busam, K., Pinkel, D., and Bastian, B. C. (2006). Somatic activation of KIT in distinct subtypes of melanoma. J. Clin. Oncol. 24, 4340–4346. doi:10.1200/JCO.2006.06.2984
Czarnecka, A. M., Bartnik, E., Fiedorowicz, M., and Rutkowski, P. (2020). Targeted therapy in melanoma and mechanisms of resistance. Int. J. Mol. Sci. 21, 4576. doi:10.3390/ijms21134576
D'Angelo, S. P., Hamid, O. A., Tarhini, A., Schadendorf, D., Chmielowski, B., Collichio, F. A., et al. (2018). A phase 2 study of Ontuxizumab, a monoclonal antibody targeting Endosialin, in metastatic melanoma. Invest. New Drugs 36, 103–113. doi:10.1007/s10637-017-0530-4
De Palma, M., Biziato, D., and Petrova, T. V. (2017). Microenvironmental regulation of tumour angiogenesis. Nat. Rev. Cancer 17, 457–474. doi:10.1038/nrc.2017.51
Eddy, K., and Chen, S. (2020). Overcoming immune evasion in melanoma. Int. J. Mol. Sci. 21, 8984. doi:10.3390/ijms21238984
Eriksson, J. A., Wanka, C., Burger, M. C., Urban, H., Hartel, I., von Renesse, J., et al. (2018). Suppression of oxidative phosphorylation confers resistance against Bevacizumab in experimental glioma. J. Neurochem. 144, 421–430. doi:10.1111/jnc.14264
Fagiani, E., Lorentz, P., Bill, R., Pavotbawan, K., Kopfstein, L., and Christofori, G. (2016). VEGF receptor-2-specific signaling mediated by VEGF-E induces hemangioma-like lesions in normal and in malignant tissue. Angiogenesis 19, 339–358. doi:10.1007/s10456-016-9508-7
Feichtinger, R. G., Lang, R., Geilberger, R., Rathje, F., Mayr, J. A., Sperl, W., et al. (2018). Melanoma tumors exhibit a variable but distinct metabolic signature. Exp. Dermatol. 27, 204–207. doi:10.1111/exd.13465
Fernandez-Cruz, E., Cerezo, A. B., Cantos-Villar, E., Richard, T., Troncoso, A. M., and Garcia-Parrilla, M. C. (2019). Inhibition of VEGFR-2 phosphorylation and effects on downstream signaling pathways in cultivated human endothelial cells by stilbenes from vitis spp. J. Agric. Food Chem. 67, 3909–3918. doi:10.1021/acs.jafc.9b00282
Filippi, L., Bruno, G., Domazetovic, V., Favre, C., and Calvani, M. (2020). Current therapies and new targets to fight melanoma: a promising role for the β3-adrenoreceptor. Cancers (Basel) 12, 1415. doi:10.3390/cancers12061415
Fruehauf, J. P., El-Masry, M., Osann, K., Parmakhtiar, B., Yamamoto, M., and Jakowatz, J. G. (2018). Phase II study of Pazopanib in combination with paclitaxel in patients with metastatic melanoma. Cancer Chemother. Pharmacol. 82, 353–360. doi:10.1007/s00280-018-3624-6
Fukuda, H., Mochizuki, S., Abe, H., Okano, H. J., Hara-Miyauchi, C., Okano, H., et al. (2011). Host-derived MMP-13 exhibits a protective role in lung metastasis of melanoma cells by local endostatin production. Br. J. Cancer 105, 1615–1624. doi:10.1038/bjc.2011.431
Ghitu, A., Schwiebs, A., Radeke, H. H., Avram, S., Zupko, I., Bor, A., et al. (2019). A comprehensive assessment of apigenin as an antiproliferative, proapoptotic, antiangiogenic and immunomodulatory phytocompound. Nutrients 11, 858. doi:10.3390/nu11040858
Ghitu, A., Pavel, I. Z., Avram, S., Kis, B., Minda, D., Dehelean, C. A., et al. (2021). An in vitro-in vivo evaluation of the antiproliferative and antiangiogenic effect of flavone apigenin against SK-MEL-24 human melanoma cell line. Anal. Cell. Pathol. 2021, 5552664. doi:10.1155/2021/5552664
Gloger, M., Menzel, L., Grau, M., Vion, A. C., Anagnostopoulos, I., Zapukhlyak, M., et al. (2020). Lymphoma angiogenesis is orchestrated by noncanonical signaling pathways. Cancer Res. 80, 1316–1329. doi:10.1158/0008-5472.CAN-19-1493
Goh, B. C., Reddy, N. J., Dandamudi, U. B., Laubscher, K. H., Peckham, T., Hodge, J. P., et al. (2010). An evaluation of the drug interaction potential of Pazopanib, an oral vascular endothelial growth factor receptor tyrosine kinase inhibitor, using a modified Cooperstown 5+1 cocktail in patients with advanced solid tumors. Clin. Pharmacol. Ther. 88, 652–659. doi:10.1038/clpt.2010.158
Goldinger, S. M., Buder-Bakhaya, K., Lo, S. N., Forschner, A., McKean, M., Zimmer, L., et al. (2022). Chemotherapy after immune checkpoint inhibitor failure in metastatic melanoma: a retrospective multicentre analysis. Eur. J. Cancer 162, 22–33. doi:10.1016/j.ejca.2021.11.022
Goode, E. F., and Smyth, E. C. (2016). Immunotherapy for gastroesophageal cancer. J. Clin. Med. 5, 84. doi:10.3390/jcm5100084
Graells, J., Vinyals, A., Figueras, A., Llorens, A., Moreno, A., Marcoval, J., et al. (2004). Overproduction of VEGF concomitantly expressed with its receptors promotes growth and survival of melanoma cells through MAPK and PI3K signaling. J. Invest. Dermatol. 123, 1151–1161. doi:10.1111/j.0022-202X.2004.23460.x
Gutierrez-Castaneda, L. D., Gamboa, M., Nova, J. A., Pulido, L., and Tovar-Parra, J. D. (2020). Mutations in the BRAF, NRAS, and C-KIT genes of patients diagnosed with melanoma in Colombia population. Biomed. Res. Int. 2020, 2046947. doi:10.1155/2020/2046947
Halder, S. K., Kant, R., and Milner, R. (2018). Chronic mild hypoxia promotes profound vascular remodeling in spinal cord blood vessels, preferentially in white matter, via an α5β1 integrin-mediated mechanism. Angiogenesis 21, 251–266. doi:10.1007/s10456-017-9593-2
Halle, B. R., and Johnson, D. B. (2021). Defining and targeting BRAF mutations in solid tumors. Curr. Treat. Options Oncol. 22, 30. doi:10.1007/s11864-021-00827-2
Han, B., Xiu, Q., Wang, H., Shen, J., Gu, A., Luo, Y., et al. (2011). A multicenter, randomized, double-blind, placebo-controlled study to evaluate the efficacy of paclitaxel-carboplatin alone or with endostar for advanced non-small cell lung cancer. J. Thorac. Oncol. 6, 1104–1109. doi:10.1097/JTO.0b013e3182166b6b
Hellmann, M. D., Callahan, M. K., Awad, M. M., Calvo, E., Ascierto, P. A., Atmaca, A., et al. (2018). Tumor mutational burden and efficacy of nivolumab monotherapy and in combination with ipilimumab in small-cell lung cancer. Cancer Cell 33, 853–861. doi:10.1016/j.ccell.2018.04.001
Hodi, F. S., Lawrence, D., Lezcano, C., Wu, X., Zhou, J., Sasada, T., et al. (2014). Bevacizumab plus ipilimumab in patients with metastatic melanoma. Cancer Immunol. Res. 2, 632–642. doi:10.1158/2326-6066.CIR-14-0053
Hong, D. S., Kurzrock, R., Wheler, J. J., Naing, A., Falchook, G. S., Fu, S., et al. (2015). Phase I dose-escalation study of the multikinase inhibitor lenvatinib in patients with advanced solid tumors and in an expanded cohort of patients with melanoma. Clin. Cancer Res. 21, 4801–4810. doi:10.1158/1078-0432.CCR-14-3063
Horvathova, J., Moravcik, R., Bohac, A., and Zeman, M. (2019). Synergic effects of inhibition of glycolysis and multikinase receptor signalling on proliferation and migration of endothelial cells. Gen. Physiol. Biophys. 38, 157–163. doi:10.4149/gpb_2018047
Hosseini, A., Masjedi, A., Baradaran, B., Hojjat-Farsangi, M., Ghalamfarsa, G., Anvari, E., et al. (2019). Dimethyl fumarate: Regulatory effects on the immune system in the treatment of multiple sclerosis. J. Cell. Physiol. 234, 9943–9955. doi:10.1002/jcp.27930
Hu, F., Fong, K. O., Cheung, M. P. L., Liu, J. A., Liang, R., Li, T. W., et al. (2022). DEPDC1B promotes melanoma angiogenesis and metastasis through sequestration of ubiquitin ligase CDC16 to stabilize secreted SCUBE3. Adv. Sci. 9, e2105226. doi:10.1002/advs.202105226
Huang, H., Song, J., Liu, Z., Pan, L., and Xu, G. (2018). Autophagy activation promotes Bevacizumab resistance in glioblastoma by suppressing Akt/mTOR signaling pathway. Oncol. Lett. 15, 1487–1494. doi:10.3892/ol.2017.7446
Huang, W., Xing, Y., Zhu, L., Zhuo, J., and Cai, M. (2021). Sorafenib derivatives-functionalized gold nanoparticles confer protection against tumor angiogenesis and proliferation via suppression of EGFR and VEGFR-2. Exp. Cell Res. 406, 112633. doi:10.1016/j.yexcr.2021.112633
Huang, C. H., Chong, K. Y., and Lei, K. F. (2021). Analysis of the internal hypoxic environment in solid tumor tissue using a folding paper system. ACS Appl. Mat. Interfaces 13, 33885–33893. doi:10.1021/acsami.1c08857
Hulse, M., Caruso, L. B., Madzo, J., Tan, Y., Johnson, S., and Tempera, I. (2018). Poly(ADP-ribose) polymerase 1 is necessary for coactivating hypoxia-inducible factor-1-dependent gene expression by Epstein-Barr virus latent membrane protein 1. PLoS Pathog. 14, e1007394. doi:10.1371/journal.ppat.1007394
Iwasa, S., Okita, N., Kuchiba, A., Ogawa, G., Kawasaki, M., Nakamura, K., et al. (2020). Phase II study of lenvatinib for metastatic colorectal cancer refractory to standard chemotherapy: the LEMON study (NCCH1503). ESMO Open 5, e000776. doi:10.1136/esmoopen-2020-000776
Jagiela, J., Bartnicki, P., and Rysz, J. (2021). Nephrotoxicity as a complication of chemotherapy and immunotherapy in the treatment of colorectal cancer, melanoma and non-small cell lung cancer. Int. J. Mol. Sci. 22, 4618. doi:10.3390/ijms22094618
Jena, B. C., Rout, L., Dey, A., and Mandal, M. (2021). Active autophagy in cancer-associated fibroblasts: Recent advances in understanding the novel mechanism of tumor progression and therapeutic response. J. Cell. Physiol. 236, 7887–7902. doi:10.1002/jcp.30419
Jia, L., Lu, X. A., Liu, G., Wang, S., Xu, M., Tian, Y., et al. (2017). Endostatin sensitizes p53-deficient non-small-cell lung cancer to genotoxic chemotherapy by targeting DNA-dependent protein kinase catalytic subunit. J. Pathol. 243, 255–266. doi:10.1002/path.4952
Jiang, N., Zou, C., Zhu, Y., Luo, Y., Chen, L., Lei, Y., et al. (2020). HIF-1ɑ-regulated miR-1275 maintains stem cell-like phenotypes and promotes the progression of LUAD by simultaneously activating Wnt/β-catenin and Notch signaling. Theranostics 10, 2553–2570. doi:10.7150/thno.41120
Jimenez-Valerio, G., and Casanovas, O. (2017). Angiogenesis and metabolism: entwined for therapy resistance. Trends Cancer 3, 10–18. doi:10.1016/j.trecan.2016.11.007
Jimenez-Valerio, G., Martinez-Lozano, M., Bassani, N., Vidal, A., Ochoa-de-Olza, M., Suarez, C., et al. (2016). Resistance to antiangiogenic therapies by metabolic symbiosis in renal cell carcinoma PDX models and patients. Cell Rep. 15, 1134–1143. doi:10.1016/j.celrep.2016.04.015
Jour, G., Ivan, D., and Aung, P. P. (2016). Angiogenesis in melanoma: an update with a focus on current targeted therapies. J. Clin. Pathol. 69, 472–483. doi:10.1136/jclinpath-2015-203482
Jung, S., Armstrong, E., Wei, A. Z., Ye, F., Lee, A., Carlino, M. S., et al. (2022). Clinical and genomic correlates of imatinib response in melanomas with KIT alterations. Br. J. Cancer 127, 1726–1732. doi:10.1038/s41416-022-01942-z
Kakadia, S., Yarlagadda, N., Awad, R., Kundranda, M., Niu, J., Naraev, B., et al. (2018). Mechanisms of resistance to BRAF and MEK inhibitors and clinical update of US Food and Drug Administration-approved targeted therapy in advanced melanoma. Onco. Targets. Ther. 11, 7095–7107. doi:10.2147/OTT.S182721
Kaufman, H. L., Margolin, K., and Sullivan, R. (2018). Management of metastatic melanoma in 2018. JAMA Oncol. 4, 857–858. doi:10.1001/jamaoncol.2018.0170
Kazerounian, S., and Lawler, J. (2018). Integration of pro- and anti-angiogenic signals by endothelial cells. J. Cell Commun. Signal. 12, 171–179. doi:10.1007/s12079-017-0433-3
Knol, A. C., Pandolfino, M. C., Vallee, A., Nguyen, F., Lella, V., Khammari, A., et al. (2015). Comparative analysis of BRAF, NRAS and c-KIT mutation status between tumor tissues and autologous tumor cell-lines of stage III/IV melanoma. Exp. Dermatol. 24, 70–73. doi:10.1111/exd.12584
Koo, H. Y., and Kume, T. (2013). FoxC1-dependent regulation of vascular endothelial growth factor signaling in corneal avascularity. Trends Cardiovasc. Med. 23, 1–4. doi:10.1016/j.tcm.2012.08.002
Kuczynski, E. A., and Reynolds, A. R. (2020). Vessel co-option and resistance to anti-angiogenic therapy. Angiogenesis 23, 55–74. doi:10.1007/s10456-019-09698-6
Kumar, P. R., Moore, J. A., Bowles, K. M., Rushworth, S. A., and Moncrieff, M. D. (2021). Mitochondrial oxidative phosphorylation in cutaneous melanoma. Br. J. Cancer 124, 115–123. doi:10.1038/s41416-020-01159-y
Lacal, P. M., and Graziani, G. (2018). Therapeutic implication of vascular endothelial growth factor receptor-1 (VEGFR-1) targeting in cancer cells and tumor microenvironment by competitive and non-competitive inhibitors. Pharmacol. Res. 136, 97–107. doi:10.1016/j.phrs.2018.08.023
Lamattina, A. M., Poli, S., Kidambi, P., Bagwe, S., Courtwright, A., Louis, P. H., et al. (2019). Serum endostatin levels are associated with diffusion capacity and with tuberous sclerosis- associated lymphangioleiomyomatosis. Orphanet J. Rare Dis. 14, 72. doi:10.1186/s13023-019-1050-4
Laurenzana, A., Chilla, A., Luciani, C., Peppicelli, S., Biagioni, A., Bianchini, F., et al. (2017). uPA/uPAR system activation drives a glycolytic phenotype in melanoma cells. Int. J. Cancer 141, 1190–1200. doi:10.1002/ijc.30817
Ledermann, J. A. (2017). Front-line therapy of advanced ovarian cancer: new approaches. Ann. Oncol. 28, viii46–viii50. doi:10.1093/annonc/mdx452
Lee, W. W., Teo, T. H., Lum, F. M., Andiappan, A. K., Amrun, S. N., Renia, L., et al. (2016). Virus infection drives IL-2 antibody complexes into pro-inflammatory agonists in mice. Sci. Rep. 6, 37603. doi:10.1038/srep37603
Leeman-Neill, R. J., Cai, Q., Joyce, S. C., Thomas, S. M., Bhola, N. E., Neill, D. B., et al. (2010). Honokiol inhibits epidermal growth factor receptor signaling and enhances the antitumor effects of epidermal growth factor receptor inhibitors. Clin. Cancer Res. 16, 2571–2579. doi:10.1158/1078-0432.CCR-10-0333
Li, D., Xie, K., Ding, G., Li, J., Chen, K., Li, H., et al. (2014). Tumor resistance to anti-VEGF therapy through up-regulation of VEGF-C expression. Cancer Lett. 346, 45–52. doi:10.1016/j.canlet.2013.12.004
Li, W. Q., Cho, E., Weinstock, M. A., Li, S., Stampfer, M. J., and Qureshi, A. A. (2019). Cutaneous nevi and risk of melanoma death in women and men: a prospective study. J. Am. Acad. Dermatol. 80, 1284–1291. doi:10.1016/j.jaad.2018.12.058
Li, C. Y., Wang, Q., Wang, X., Li, G., Shen, S., and Wei, X. (2019). Scutellarin inhibits the invasive potential of malignant melanoma cells through the suppression epithelial-mesenchymal transition and angiogenesis via the PI3K/Akt/mTOR signaling pathway. Eur. J. Pharmacol. 858, 172463. doi:10.1016/j.ejphar.2019.172463
Li, S. J., Chen, J. X., and Sun, Z. J. (2021). Improving antitumor immunity using antiangiogenic agents: Mechanistic insights, current progress, and clinical challenges. Cancer Commun. 41, 830–850. doi:10.1002/cac2.12183
Li, Z., Fang, Y., Chen, H., Zhang, T., Yin, X., Man, J., et al. (2022). Spatiotemporal trends of the global burden of melanoma in 204 countries and territories from 1990 to 2019: Results from the 2019 global burden of disease study. Neoplasia 24 (2022), 12–21. doi:10.1016/j.neo.2021.11.013
Lian, B., Cui, C. L., Zhou, L., Song, X., Zhang, X. S., Wu, D., et al. (2017). The natural history and patterns of metastases from mucosal melanoma: an analysis of 706 prospectively-followed patients. Ann. Oncol. 28, 868–873. doi:10.1093/annonc/mdw694
Liang, K., Liu, Q., Li, P., Han, Y., Bian, X., Tang, Y., et al. (2018). Endostatin gene therapy delivered by attenuated Salmonella typhimurium in murine tumor models. Cancer Gene Ther. 25, 167–183. doi:10.1038/s41417-018-0021-6
Licarete, E., Rauca, V. F., Luput, L., Drotar, D., Stejerean, I., Patras, L., et al. (2020). Overcoming intrinsic doxorubicin resistance in melanoma by anti-angiogenic and anti-metastatic effects of liposomal prednisolone phosphate on tumor microenvironment. Int. J. Mol. Sci. 21, 2968. doi:10.3390/ijms21082968
Lipplaa, A., Fernandes, R., Marshall, A., Lorigan, P., Dunn, J., Myers, K. A., et al. (2018). 25-hydroxyvitamin D serum levels in patients with high risk resected melanoma treated in an adjuvant Bevacizumab trial. Br. J. Cancer 119, 793–800. doi:10.1038/s41416-018-0179-6
Liu, R., Cao, Z., Pan, Y., Zhang, G., Yang, P., Guo, P., et al. (2013). Jatrorrhizine hydrochloride inhibits the proliferation and neovascularization of C8161 metastatic melanoma cells. Anticancer. Drugs 24, 667–676. doi:10.1097/CAD.0b013e328361ab28
Liu, Y., Huang, N., Liao, S., Rothzerg, E., Yao, F., Li, Y., et al. (2021). Current research progress in targeted anti-angiogenesis therapy for osteosarcoma. Cell Prolif. 54, e13102. doi:10.1111/cpr.13102
Liu, Q., Nie, R., Li, M., Li, L., Zhou, H., Lu, H., et al. (2021). Identification of subtypes correlated with tumor immunity and immunotherapy in cutaneous melanoma. Comput. Struct. Biotechnol. J. 19, 4472–4485. doi:10.1016/j.csbj.2021.08.005
Liu, Z., Zhao, Q., Zheng, Z., Liu, S., Meng, L., Dong, L., et al. (2021). Vascular normalization in immunotherapy: a promising mechanisms combined with radiotherapy. Biomed. Pharmacother. 139, 111607. doi:10.1016/j.biopha.2021.111607
Liu, Y. X., Xu, B. W., Niu, X. D., Chen, Y. J., Fu, X. Q., Wang, X. Q., et al. (2022). Inhibition of Src/STAT3 signaling-mediated angiogenesis is involved in the anti-melanoma effects of dioscin. Pharmacol. Res. 175, 105983. doi:10.1016/j.phrs.2021.105983
Liu, S., Zhao, H., Hu, Y., Yan, C., Mi, Y., Li, X., et al. (2022). Lactate promotes metastasis of normoxic colorectal cancer stem cells through PGC-1α-mediated oxidative phosphorylation. Cell Death Dis. 13, 651. doi:10.1038/s41419-022-05111-1
Luciano, A. M., Perez-Oliva, A. B., Mulero, V., and Del Bufalo, D. (2021). Bcl-xL: a focus on melanoma pathobiology. Int. J. Mol. Sci. 22, 2777. doi:10.3390/ijms22052777
Lugassy, C., Zadran, S., Bentolila, L. A., Wadehra, M., Prakash, R., Carmichael, S. T., et al. (2014). Angiotropism, pericytic mimicry and extravascular migratory metastasis in melanoma: an alternative to intravascular cancer dissemination. Cancer Microenviron. 7, 139–152. doi:10.1007/s12307-014-0156-4
Maacha, S., Bhat, A. A., Jimenez, L., Raza, A., Haris, M., Uddin, S., et al. (2019). Extracellular vesicles-mediated intercellular communication: roles in the tumor microenvironment and anti-cancer drug resistance. Mol. Cancer 18, 55. doi:10.1186/s12943-019-0965-7
Mahdi, A., Darvishi, B., Majidzadeh, A. K., Salehi, M., and Farahmand, L. (2019). Challenges facing antiangiogenesis therapy: the significant role of hypoxia-inducible factor and MET in development of resistance to anti-vascular endothelial growth factor-targeted therapies. J. Cell. Physiol. 234, 5655–5663. doi:10.1002/jcp.27414
Malekan, M., Ebrahimzadeh, M. A., and Sheida, F. (2021). The role of Hypoxia-Inducible Factor-1alpha and its signaling in melanoma. Biomed. Pharmacother. 141, 111873. doi:10.1016/j.biopha.2021.111873
Malhotra, J., Jabbour, S., Orlick, M., Riedlinger, G., Guo, Y., White, E., et al. (2019). Phase Ib/II study of hydroxychloroquine in combination with chemotherapy in patients with metastatic non-small cell lung cancer (NSCLC). Cancer Treat. Res. Commun. 21, 100158. doi:10.1016/j.ctarc.2019.100158
Martin, C. A., Cullinane, C., Kirby, L., Abuhammad, S., Lelliott, E. J., Waldeck, K., et al. (2018). Palbociclib synergizes with BRAF and MEK inhibitors in treatment naive melanoma but not after the development of BRAF inhibitor resistance. Int. J. Cancer 142, 2139–2152. doi:10.1002/ijc.31220
Menzel, L., Hopken, U. E., and Rehm, A. (2020). Angiogenesis in lymph nodes is a critical regulator of immune response and lymphoma growth. Front. Immunol. 11, 591741. doi:10.3389/fimmu.2020.591741
Micheli, L., Parisio, C., Lucarini, E., Vona, A., Toti, A., Pacini, A., et al. (2021). VEGF-A/VEGFR-1 signalling and chemotherapy-induced neuropathic pain: therapeutic potential of a novel anti-VEGFR-1 monoclonal antibody. J. Exp. Clin. Cancer Res. 40, 320. doi:10.1186/s13046-021-02127-x
Mohammadi, M., and Gelderblom, H. (2021). Systemic therapy of advanced/metastatic gastrointestinal stromal tumors: an update on progress beyond imatinib, Sunitinib, and regorafenib. Expert Opin. Investig. Drugs 30, 143–152. doi:10.1080/13543784.2021.1857363
Mouriaux, F., Servois, V., Parienti, J. J., Lesimple, T., Thyss, A., Dutriaux, C., et al. (2016). Sorafenib in metastatic uveal melanoma: efficacy, toxicity and health-related quality of life in a multicentre phase II study. Br. J. Cancer 115, 20–24. doi:10.1038/bjc.2016.119
Mukherjee, A., Madamsetty, V. S., Paul, M. K., and Mukherjee, S. (2020). Recent advancements of nanomedicine towards antiangiogenic therapy in cancer. Int. J. Mol. Sci. 21, 455. doi:10.3390/ijms21020455
Muppala, S., Xiao, R., Krukovets, I., Verbovetsky, D., Yendamuri, R., Habib, N., et al. (2017). Thrombospondin-4 mediates TGF-beta-induced angiogenesis. Oncogene 36, 5189–5198. doi:10.1038/onc.2017.140
Nyakas, M., Aamdal, E., Jacobsen, K. D., Guren, T. K., Aamdal, S., Hagene, K. T., et al. (2019). Prognostic biomarkers for immunotherapy with ipilimumab in metastatic melanoma. Clin. Exp. Immunol. 197, 74–82. doi:10.1111/cei.13283
Okamoto, K., Kodama, K., Takase, K., Sugi, N. H., Yamamoto, Y., Iwata, M., et al. (2013). Antitumor activities of the targeted multi-tyrosine kinase inhibitor lenvatinib (E7080) against RET gene fusion-driven tumor models. Cancer Lett. 340, 97–103. doi:10.1016/j.canlet.2013.07.007
O'Reilly, M. S., Boehm, T., Shing, Y., Fukai, N., Vasios, G., Lane, W. S., et al. (1997). Endostatin: an endogenous inhibitor of angiogenesis and tumor growth. Cell 88, 277–285. doi:10.1016/s0092-8674(00)81848-6
Ortega-Muelas, M., Roche, O., Fernandez-Aroca, D. M., Encinar, J. A., Albandea-Rodriguez, D., Arconada-Luque, E., et al. (2021). ERK5 signalling pathway is a novel target of Sorafenib: implication in EGF biology. J. Cell. Mol. Med. 25, 10591–10603. doi:10.1111/jcmm.16990
Ott, P. A., Hodi, F. S., and Buchbinder, E. I. (2015). Inhibition of immune checkpoints and vascular endothelial growth factor as combination therapy for metastatic melanoma: An overview of rationale, preclinical evidence, and initial clinical data. Front. Oncol. 5, 202. doi:10.3389/fonc.2015.00202
Palanisamy, K., Nareshkumar, R. N., Sivagurunathan, S., Raman, R., Sulochana, K. N., and Chidambaram, S. (2019). Anti-angiogenic effect of adiponectin in human primary microvascular and macrovascular endothelial cells. Microvasc. Res. 122, 136–145. doi:10.1016/j.mvr.2018.08.002
Pandita, A., Ekstrand, M., Bjursten, S., Zhao, Z., Fogelstrand, P., Le Gal, K., et al. (2021). Intussusceptive angiogenesis in human metastatic malignant melanoma. Am. J. Pathol. 191, 2023–2038. doi:10.1016/j.ajpath.2021.07.009
Papaevangelou, E., Boult, J. K. R., Whitley, G. S., Robinson, S. P., and Howe, F. A. (2018). Assessment of the direct effects of DDAH I on tumour angiogenesis in vivo. Angiogenesis 21, 737–749. doi:10.1007/s10456-018-9617-6
Parmar, D., and Apte, M. (2021). Angiopoietin inhibitors: a review on targeting tumor angiogenesis. Eur. J. Pharmacol. 899, 174021. doi:10.1016/j.ejphar.2021.174021
Patnaik, A., Yap, T. A., Chung, H. C., de Miguel, M. J., Bang, Y. J., Lin, C. C., et al. (2021). Safety and clinical activity of a new anti-PD-L1 antibody as monotherapy or combined with targeted therapy in advanced solid tumors: The PACT phase ia/ib trial. Clin. Cancer Res. 27, 1267–1277. doi:10.1158/1078-0432.CCR-20-2821
Pekkonen, P., Alve, S., Balistreri, G., Gramolelli, S., Tatti-Bugaeva, O., Paatero, I., et al. (2018). Lymphatic endothelium stimulates melanoma metastasis and invasion via MMP14-dependent Notch3 and β1-integrin activation. Elife 7, e32490. doi:10.7554/eLife.32490
Peng, S., Zhang, Y., Peng, H., Ke, Z., Xu, L., Su, T., et al. (2016). Intracellular autocrine VEGF signaling promotes EBDC cell proliferation, which can be inhibited by Apatinib. Cancer Lett. 373, 193–202. doi:10.1016/j.canlet.2016.01.015
Peters, M. A. M., Meijer, C., Fehrmann, R. S. N., Walenkamp, A. M. E., Kema, I. P., de Vries, E. G. E., et al. (2020). Serotonin and dopamine receptor expression in solid tumours including rare cancers. Pathol. Oncol. Res. 26, 1539–1547. doi:10.1007/s12253-019-00734-w
Pozas, J., San Roman, M., Alonso-Gordoa, T., Pozas, M., Caracuel, L., Carrato, A., et al. (2019). Targeting angiogenesis in pancreatic neuroendocrine tumors: resistance mechanisms. Int. J. Mol. Sci. 20, 4949. doi:10.3390/ijms20194949
Presta, L. G., Chen, H., O'Connor, S. J., Chisholm, V., Meng, Y. G., Krummen, L., et al. (1997). Humanization of an anti-vascular endothelial growth factor monoclonal antibody for the therapy of solid tumors and other disorders. Cancer Res. 57, 4593–4599.
Pretto, F., Elia, G., Castioni, N., and Neri, D. (2014). Preclinical evaluation of IL-2-based immunocytokines supports their use in combination with dacarbazine, paclitaxel and TNF-based immunotherapy. Cancer Immunol. Immunother. 63, 901–910. doi:10.1007/s00262-014-1562-7
Qi, F. L., Wang, M. F., Li, B. Z., Lu, Z. F., Nie, G. J., and Li, S. P. (2020). Reversal of the immunosuppressive tumor microenvironment by nanoparticle-based activation of immune-associated cells. Acta Pharmacol. Sin. 41, 895–901. doi:10.1038/s41401-020-0423-5
Qin, S., Li, A., Yi, M., Yu, S., Zhang, M., and Wu, K. (2019). Recent advances on anti-angiogenesis receptor tyrosine kinase inhibitors in cancer therapy. J. Hematol. Oncol. 12, 27. doi:10.1186/s13045-019-0718-5
Rahma, O. E., Tyan, K., Giobbie-Hurder, A., Brohl, A. S., Bedard, P. L., Renouf, D. J., et al. (2022). Phase IB study of ziv-Aflibercept plus pembrolizumab in patients with advanced solid tumors. J. Immunother. Cancer 10, e003569. doi:10.1136/jitc-2021-003569
Ramelyte, E., Schindler, S. A., and Dummer, R. (2017). The safety of anti PD-1 therapeutics for the treatment of melanoma. Expert Opin. Drug Saf. 16, 41–53. doi:10.1080/14740338.2016.1248402
Rampino, A., Annese, T., Margari, A., Tamma, R., and Ribatti, D. (2021). Nutraceuticals and their role in tumor angiogenesis. Exp. Cell Res. 408, 112859. doi:10.1016/j.yexcr.2021.112859
Rodewald, A. K., Rushing, E. J., Kirschenbaum, D., Mangana, J., Mittmann, C., Moch, H., et al. (2019). Eight autopsy cases of melanoma brain metastases showing angiotropism and pericytic mimicry. Implications for extravascular migratory metastasis. J. Cutan. Pathol. 46, 570–578. doi:10.1111/cup.13465
Rodrigues, T., and Ferraz, L. S. (2020). Therapeutic potential of targeting mitochondrial dynamics in cancer. Biochem. Pharmacol. 182, 114282. doi:10.1016/j.bcp.2020.114282
Roviello, G., Ravelli, A., Polom, K., Petrioli, R., Marano, L., Marrelli, D., et al. (2016). Apatinib: A novel receptor tyrosine kinase inhibitor for the treatment of gastric cancer. Cancer Lett. 372, 187–191. doi:10.1016/j.canlet.2016.01.014
Ruocco, M. R., Avagliano, A., Granato, G., Vigliar, E., Masone, S., Montagnani, S., et al. (2019). Metabolic flexibility in melanoma: a potential therapeutic target. Semin. Cancer Biol. 59, 187–207. doi:10.1016/j.semcancer.2019.07.016
Russo, M., and Giavazzi, R. (2018). Anti-angiogenesis for cancer: Current status and prospects. Thromb. Res. 164, S3–S6. doi:10.1016/j.thromres.2018.01.030
Sabbah, M., Krayem, M., Najem, A., Sales, F., Miller, W., Del Rincon, S., et al. (2021). Dasatinib stimulates its own mechanism of resistance by activating a CRTC3/MITF/Bcl-2 pathway in melanoma with mutant or amplified c-kit. Mol. Cancer Res. 19, 1221–1233. doi:10.1158/1541-7786.mcr-20-1040
Sachdev, E., Tabatabai, R., Roy, V., Rimel, B. J., and Mita, M. M. (2019). PARP inhibition in cancer: an update on clinical development. Target. Oncol. 14, 657–679. doi:10.1007/s11523-019-00680-2
Salmaso, S., Mastrotto, F., Roverso, M., Gandin, V., De Martin, S., Gabbia, D., et al. (2021). Tyrosine kinase inhibitor prodrug-loaded liposomes for controlled release at tumor microenvironment. J. Control. Release 340, 318–330. doi:10.1016/j.jconrel.2021.11.006
Sample, A., and He, Y. Y. (2018). Mechanisms and prevention of UV-induced melanoma. Photodermatol. Photoimmunol. Photomed. 34, 13–24. doi:10.1111/phpp.12329
Sebestyen, A., Danko, T., Sztankovics, D., Moldvai, D., Raffay, R., Cervi, C., et al. (2021). The role of metabolic ecosystem in cancer progression - metabolic plasticity and mTOR hyperactivity in tumor tissues. Cancer Metastasis Rev. 40, 989–1033. doi:10.1007/s10555-021-10006-2
Senger, D. R., Galli, S. J., Dvorak, A. M., Perruzzi, C. A., Harvey, V. S., and Dvorak, H. F. (1983). Tumor cells secrete a vascular permeability factor that promotes accumulation of ascites fluid. Science 219, 983–985. doi:10.1126/science.6823562
Sheng, X., Cao, D., Yuan, J., Zhou, F., Wei, Q., Xie, X., et al. (2018). Sorafenib in combination with gemcitabine plus cisplatin chemotherapy in metastatic renal collecting duct carcinoma: a prospective, multicentre, single-arm, phase 2 study. Eur. J. Cancer 100, 1–7. doi:10.1016/j.ejca.2018.04.007
Sheng, X., Yan, X., Chi, Z., Si, L., Cui, C., Tang, B., et al. (2019). Axitinib in combination with toripalimab, a humanized immunoglobulin G4 monoclonal antibody against programmed cell death-1, in patients with metastatic mucosal melanoma: An open-label phase IB trial. J. Clin. Oncol. 37, 2987–2999. doi:10.1200/JCO.19.00210
Shibuya, M. (2011). Vascular endothelial growth factor (VEGF) and its receptor (VEGFR) signaling in angiogenesis: A crucial target for anti- and pro-angiogenic therapies. Genes Cancer 2, 1097–1105. doi:10.1177/1947601911423031
Shin, S. U., Cho, H. M., Das, R., Gil-Henn, H., Ramakrishnan, S., Al Bayati, A., et al. (2021). Inhibition of vasculogenic mimicry and angiogenesis by an anti-EGFR IgG1-human endostatin-P125A fusion protein reduces triple negative breast cancer metastases. Cells 10, 2904. doi:10.3390/cells10112904
Singh, S., Singh, A. P., Sharma, B., Owen, L. B., and Singh, R. K. (2010). CXCL8 and its cognate receptors in melanoma progression and metastasis. Future Oncol. 6, 111–116. doi:10.2217/fon.09.128
Smith, M., and Pothuri, B. (2022). Appropriate selection of PARP inhibitors in ovarian cancer. Curr. Treat. Options Oncol. 23, 887–903. doi:10.1007/s11864-022-00938-4
Smolarczyk, R., Czapla, J., Jarosz-Biej, M., Czerwinski, K., and Cichon, T. (2021). Vascular disrupting agents in cancer therapy. Eur. J. Pharmacol. 891, 173692. doi:10.1016/j.ejphar.2020.173692
Sobierajska, K., Ciszewski, W. M., Sacewicz-Hofman, I., and Niewiarowska, J. (2020). Endothelial cells in the tumor microenvironment. Adv. Exp. Med. Biol. 1234, 71–86. doi:10.1007/978-3-030-37184-5_6
Spirli, C., Morell, C. M., Locatelli, L., Okolicsanyi, S., Ferrero, C., Kim, A. K., et al. (2012). Cyclic AMP/PKA-dependent paradoxical activation of Raf/MEK/ERK signaling in polycystin-2 defective mice treated with Sorafenib. Hepatology 56, 2363–2374. doi:10.1002/hep.25872
Straume, O., Salvesen, H. B., and Akslen, L. A. (1999). Angiogenesis is prognostically important in vertical growth phase melanomas. Int. J. Oncol. 15, 595–599. doi:10.3892/ijo.15.3.595
Szabo, V., Bugyik, E., Dezso, K., Ecker, N., Nagy, P., Timar, J., et al. (2015). Mechanism of tumour vascularization in experimental lung metastases. J. Pathol. 235, 384–396. doi:10.1002/path.4464
Talib, W. H., and Al Kury, L. T. (2018). Parthenolide inhibits tumor-promoting effects of nicotine in lung cancer by inducing P53 - dependent apoptosis and inhibiting VEGF expression. Biomed. Pharmacother. 107, 1488–1495. doi:10.1016/j.biopha.2018.08.139
Tarhini, A. A., Frankel, P., Ruel, C., Ernstoff, M. S., Kuzel, T. M., Logan, T. F., et al. (2018). NCI 8628: A randomized phase 2 study of ziv-aflibercept and high-dose interleukin 2 or high-dose interleukin 2 alone for inoperable stage III or IV melanoma. Cancer 124, 4332–4341. doi:10.1002/cncr.31734
Taylor, M. H., Lee, C. H., Makker, V., Rasco, D., Dutcus, C. E., Wu, J., et al. (2020). Phase IB/II trial of lenvatinib plus pembrolizumab in patients with advanced renal cell carcinoma, endometrial cancer, and other selected advanced solid tumors. J. Clin. Oncol. 38, 1154–1163. doi:10.1200/JCO.19.01598
Ten Voorde, A. M. W., Wierenga, A. P. A., Nell, R. J., van der Velden, P. A., Luyten, G. P. M., Verdijk, R. M., et al. (2021). In uveal melanoma, angiopoietin-2 but not angiopoietin-1 is increased in high-risk tumors, providing a potential druggable target. Cancers (Basel) 13, 3986. doi:10.3390/cancers13163986
Tentori, L., Lacal, P. M., Muzi, A., Dorio, A. S., Leonetti, C., Scarsella, M., et al. (2007). Poly(ADP-ribose) polymerase (PARP) inhibition or PARP-1 gene deletion reduces angiogenesis. Eur. J. Cancer 43, 2124–2133. doi:10.1016/j.ejca.2007.07.010
Thurneysen, S., Cheng, P. F., Nagel, H. W., Kunz, M., Jaberg-Bentele, N., Nageli, M., et al. (2016). An exploratory study investigating the metabolic activity and local cytokine profile in patients with melanoma treated with Pazopanib and paclitaxel. Br. J. Dermatol. 175, 966–978. doi:10.1111/bjd.14727
Tian, B., Xiao, Y., Ma, J., Ou, W., Wang, H., Wu, J., et al. (2020). Parthenolide inhibits angiogenesis in esophageal squamous cell carcinoma through suppression of VEGF. Onco. Targets. Ther. 13, 7447–7458. doi:10.2147/OTT.S256291
Tiwari, A., Mukherjee, B., and Dixit, M. (2018). MicroRNA key to angiogenesis regulation: MiRNA biology and therapy. Curr. Cancer Drug Targets 18, 266–277. doi:10.2174/1568009617666170630142725
Tong, M., Wang, J., Zhang, H., Xing, H., Wang, Y., Fang, Y., et al. (2019). Efficacy and safety of gemcitabine plus anti-angiogenesis therapy for advanced pancreatic cancer: a systematic review and meta-analysis of clinical randomized phase III trials. J. Cancer 10, 968–978. doi:10.7150/jca.26672
Une, N., Takano-Kasuya, M., Kitamura, N., Ohta, M., Inose, T., Kato, C., et al. (2021). The anti-angiogenic agent lenvatinib induces tumor vessel normalization and enhances radiosensitivity in hepatocellular tumors. Med. Oncol. 38, 60. doi:10.1007/s12032-021-01503-z
Vaid, M., Singh, T., Prasad, R., and Katiyar, S. K. (2015). Silymarin inhibits melanoma cell growth both in vitro and in vivo by targeting cell cycle regulators, angiogenic biomarkers and induction of apoptosis. Mol. Carcinog. 54, 1328–1339. doi:10.1002/mc.22208
Vavilala, D. T., Ponnaluri, V. K., Vadlapatla, R. K., Pal, D., Mitra, A. K., and Mukherji, M. (2012). Honokiol inhibits HIF pathway and hypoxia-induced expression of histone lysine demethylases. Biochem. Biophys. Res. Commun. 422, 369–374. doi:10.1016/j.bbrc.2012.04.143
Venza, I., Visalli, M., Oteri, R., Beninati, C., Teti, D., and Venza, M. (2018). Genistein reduces proliferation of EP3-expressing melanoma cells through inhibition of PGE2-induced IL-8 expression. Int. Immunopharmacol. 62, 86–95. doi:10.1016/j.intimp.2018.06.009
Viallard, C., and Larrivee, B. (2017). Tumor angiogenesis and vascular normalization: alternative therapeutic targets. Angiogenesis 20, 409–426. doi:10.1007/s10456-017-9562-9
Wang, J., Sun, Y., Liu, Y., Yu, Q., Zhang, Y., Li, K., et al. (2005). Results of randomized, multicenter, double-blind phase III trial of rh-endostatin (YH-16) in treatment of advanced non-small cell lung cancer patients. Zhongguo Fei Ai Za Zhi 8, 283–290. doi:10.3779/j.issn.1009-3419.2005.04.07
Wang, J. C., Li, X. X., Sun, X., Li, G. Y., Sun, J. L., Ye, Y. P., et al. (2018). Activation of AMPK by simvastatin inhibited breast tumor angiogenesis via impeding HIF-1α-induced pro-angiogenic factor. Cancer Sci. 109, 1627–1637. doi:10.1111/cas.13570
Wang, Z. Q., Wang, D. S., Wang, F. H., Ren, C., Tan, Q., and Li, Y. H. (2021). Recombinant human endostatin plus paclitaxel/nedaplatin for recurrent or metastatic advanced esophageal squamous cell carcinoma: a prospective, single-arm, open-label, phase II study. Invest. New Drugs 39, 516–523. doi:10.1007/s10637-020-01021-1
Wang, G., Zhang, M., Cheng, M., Wang, X., Li, K., Chen, J., et al. (2021). Tumor microenvironment in head and neck squamous cell carcinoma: Functions and regulatory mechanisms. Cancer Lett. 507, 55–69. doi:10.1016/j.canlet.2021.03.009
Wang, Y., Wang, M., Wu, H. X., and Xu, R. H. (2021). Advancing to the era of cancer immunotherapy. Cancer Commun. (Lond). 41, 803–829. doi:10.1002/cac2.12178
Wang, K., Chen, Q., Liu, N., Zhang, J., and Pan, X. (2021). Recent advances in, and challenges of, anti-angiogenesis agents for tumor chemotherapy based on vascular normalization. Drug Discov. Today 26, 2743–2753. doi:10.1016/j.drudis.2021.07.024
Warren, B. A., and Shubik, P. (1966). The growth of the blood supply to melanoma transplants in the hamster cheek pouch. Lab. Invest. 15, 464–478.
Watanabe, T. (2021). Realization of osteolysis, angiogenesis, immunosuppression, and drug resistance by extracellular vesicles: Roles of RNAs and proteins in their cargoes and of ectonucleotidases of the immunosuppressive adenosinergic noncanonical pathway in the bone marrow niche of multiple myeloma. Cancers (Basel) 13, 2969. doi:10.3390/cancers13122969
Wen, Y., Chelariu-Raicu, A., Umamaheswaran, S., Nick, A. M., Stur, E., Hanjra, P., et al. (2022). Endothelial p130cas confers resistance to anti-angiogenesis therapy. Cell Rep. 38, 110999. doi:10.1016/j.celrep.2022.110999
Wohlfeil, S. A., Hafele, V., Dietsch, B., Weller, C., Sticht, C., Jauch, A. S., et al. (2022). Angiogenic and molecular diversity determine hepatic melanoma metastasis and response to anti-angiogenic treatment. J. Transl. Med. 20, 62. doi:10.1186/s12967-022-03255-4
Wroblewska-Luczka, P., Cabaj, J., Bak, W., Bargiel, J., Grabarska, A., Goralczyk, A., et al. (2022). Additive interactions between betulinic Acid and two taxanes in in vitro tests against four human malignant melanoma cell lines. Int. J. Mol. Sci. 23, 9641. doi:10.3390/ijms23179641
Xiao, R., Mansour, A. G., Huang, W., Chrislip, L. A., Wilkins, R. K., Queen, N. J., et al. (2019). Adipocytes: A novel target for IL-15/IL-15rα cancer gene therapy. Mol. Ther. 27, 922–932. doi:10.1016/j.ymthe.2019.02.011
Yan, X., Sheng, X., Chi, Z., Si, L., Cui, C., Kong, Y., et al. (2021). Randomized phase II study of Bevacizumab in combination with carboplatin plus paclitaxel in patients with previously untreated advanced mucosal melanoma. J. Clin. Oncol. 39, 881–889. doi:10.1200/JCO.20.00902
Yang, T., Zhang, W., Cao, S., Sun, S., Cai, X., Xu, L., et al. (2022). Discovery of highly potent and selective EGFR(T790M/L858R) TKIs against NSCLC based on molecular dynamic simulation. Eur. J. Med. Chem. 228, 113984. doi:10.1016/j.ejmech.2021.113984
Yeramian, A., Sorolla, A., Velasco, A., Santacana, M., Dolcet, X., Valls, J., et al. (2012). Inhibition of activated receptor tyrosine kinases by Sunitinib induces growth arrest and sensitizes melanoma cells to Bortezomib by blocking Akt pathway. Int. J. Cancer 130, 967–978. doi:10.1002/ijc.26096
Yetkin-Arik, B., Kastelein, A. W., Klaassen, I., Jansen, C., Latul, Y. P., Vittori, M., et al. (2021). Angiogenesis in gynecological cancers and the options for anti-angiogenesis therapy. Biochim. Biophys. Acta. Rev. Cancer 1875, 188446. doi:10.1016/j.bbcan.2020.188446
Yi, J. H., Yi, S. Y., Lee, H. R., Lee, S. I., Lim, D. H., Kim, J. H., et al. (2011). Dacarbazine-based chemotherapy as first-line treatment in noncutaneous metastatic melanoma: multicenter, retrospective analysis in asia. Melanoma Res. 21, 223–227. doi:10.1097/CMR.0b013e3283457743
Yin, X., Lin, X., Ren, X., Yu, B., Liu, L., Ye, Z., et al. (2019). Novel multi-targeted inhibitors suppress ocular neovascularization by regulating unique gene sets. Pharmacol. Res. 146, 104277. doi:10.1016/j.phrs.2019.104277
Yuan, S., Fu, Q., Zhao, L., Fu, X., Li, T., Han, L., et al. (2022). Efficacy and safety of apatinib in patients with recurrent or refractory melanoma. Oncologist 27, e463–e470. doi:10.1093/oncolo/oyab068
Zamaratskaia, G., Mhd Omar, N. A., Brunius, C., Hallmans, G., Johansson, J. E., Andersson, S. O., et al. (2020). Consumption of whole grain/bran rye instead of refined wheat decrease concentrations of TNF-R2, e-selectin, and endostatin in an exploratory study in men with prostate cancer. Clin. Nutr. 39, 159–165. doi:10.1016/j.clnu.2019.01.007
Zhang, N., and Xiao, X. H. (2021). Integrative medicine in the era of cancer immunotherapy: Challenges and opportunities. J. Integr. Med. 19, 291–294. doi:10.1016/j.joim.2021.03.005
Zhang, X., Li, N. L., Guo, C., Li, Y. D., Luo, L. L., Liu, Y. Q., et al. (2018). A vaccine targeting basic fibroblast growth factor elicits a protective immune response against murine melanoma. Cancer Biol. Ther. 19, 518–524. doi:10.1080/15384047.2018.1435223
Zhang, L., Chen, C., Duanmu, J., Wu, Y., Tao, J., Yang, A., et al. (2018). Cryptotanshinone inhibits the growth and invasion of colon cancer by suppressing inflammation and tumor angiogenesis through modulating MMP/TIMP system, PI3K/Akt/mTOR signaling and HIF-1α nuclear translocation. Int. Immunopharmacol. 65, 429–437. doi:10.1016/j.intimp.2018.10.035
Zhang, Q., Lu, S., Li, T., Yu, L., Zhang, Y., Zeng, H., et al. (2019). ACE2 inhibits breast cancer angiogenesis via suppressing the VEGFa/VEGFR2/ERK pathway. J. Exp. Clin. Cancer Res. 38, 173. doi:10.1186/s13046-019-1156-5
Zhang, X., Zhang, Y., Jia, Y., Qin, T., Zhang, C., Li, Y., et al. (2020). Bevacizumab promotes active biological behaviors of human umbilical vein endothelial cells by activating TGFβ1 pathways via off-VEGF signaling. Cancer Biol. Med. 17, 418–432. doi:10.20892/j.issn.2095-3941.2019.0215
Zhang, J., Chen, H., Chen, C., Liu, H., He, Y., Zhao, J., et al. (2021). Systemic administration of mesenchymal stem cells loaded with a novel oncolytic adenovirus carrying IL-24/endostatin enhances glioma therapy. Cancer Lett. 509, 26–38. doi:10.1016/j.canlet.2021.03.027
Zhang, S., Yang, J., and Shen, L. (2021). Extracellular vesicle-mediated regulation of tumor angiogenesis- implications for anti-angiogenesis therapy. J. Cell. Mol. Med. 25, 2776–2785. doi:10.1111/jcmm.16359
Zhang, X., Jin, F., Jiang, S., Cao, J., Meng, Y., Xu, Y., et al. (2022). Rh-endostatin combined with chemotherapy in patients with advanced or recurrent mucosal melanoma: retrospective analysis of real-world data. Invest. New Drugs 40, 453–460. doi:10.1007/s10637-021-01172-9
Zhao, Z., Xia, G., Li, N., Su, R., Chen, X., and Zhong, L. (2018). Autophagy inhibition promotes bevacizumab-induced apoptosis and proliferation inhibition in colorectal cancer cells. J. Cancer 9, 3407–3416. doi:10.7150/jca.24201
Zhao, Z., Zhang, D., Wu, F., Tu, J., Song, J., Xu, M., et al. (2021). Sophoridine suppresses lenvatinib-resistant hepatocellular carcinoma growth by inhibiting RAS/MEK/ERK axis via decreasing VEGFR2 expression. J. Cell. Mol. Med. 25, 549–560. doi:10.1111/jcmm.16108
Zheng, J., Zhang, M., and Weng, H. (2018). Induction of the mitochondrial NDUFA4L2 protein by HIF-1a regulates heart regeneration by promoting the survival of cardiac stem cell. Biochem. Biophys. Res. Commun. 503, 2226–2233. doi:10.1016/j.bbrc.2018.06.142
Zhou, Q., and Gallo, J. M. (2009). Differential effect of Sunitinib on the distribution of temozolomide in an orthotopic glioma model. Neuro. Oncol. 11, 301–310. doi:10.1215/15228517-2008-088
Zhu, Z., Zhao, Y., Li, J., Tao, L., Shi, P., Wei, Z., et al. (2016). Cryptotanshinone, a novel tumor angiogenesis inhibitor, destabilizes tumor necrosis factor-alpha mRNA via decreasing nuclear-cytoplasmic translocation of RNA-binding protein HuR. Mol. Carcinog. 55, 1399–1410. doi:10.1002/mc.22383
Zhu, J., Chen, G., Niu, K., Feng, Y., Xie, L., Qin, S., et al. (2022). Efficacy and safety of recombinant human endostatin during peri-radiotherapy period in advanced non-small-cell lung cancer. Future Oncol. 18, 1077–1087. doi:10.2217/fon-2021-1239
Keywords: angiogenesis, melanoma, clinical treatment, pharmacology, mechanism
Citation: Wu Z, Bian Y, Chu T, Wang Y, Man S, Song Y and Wang Z (2022) The role of angiogenesis in melanoma: Clinical treatments and future expectations. Front. Pharmacol. 13:1028647. doi: 10.3389/fphar.2022.1028647
Received: 26 August 2022; Accepted: 30 November 2022;
Published: 15 December 2022.
Edited by:
Nathaniel Edward Bennett Saidu, INSERM U1016 Institut Cochin, FranceReviewed by:
Sandra Donnini, University of Siena, ItalyFrancesca Bianchini, University of Florence, Italy
Copyright © 2022 Wu, Bian, Chu, Wang, Man, Song and Wang. This is an open-access article distributed under the terms of the Creative Commons Attribution License (CC BY). The use, distribution or reproduction in other forums is permitted, provided the original author(s) and the copyright owner(s) are credited and that the original publication in this journal is cited, in accordance with accepted academic practice. No use, distribution or reproduction is permitted which does not comply with these terms.
*Correspondence: Shuai Man, MTM2MTU0MjI1ODlAMTYzLmNvbQ==; Yongmei Song, c29uZ3ltMDIwMEAxNjMuY29t; Zhenguo Wang, emhlbmd1b3dAMTI2LmNvbQ==