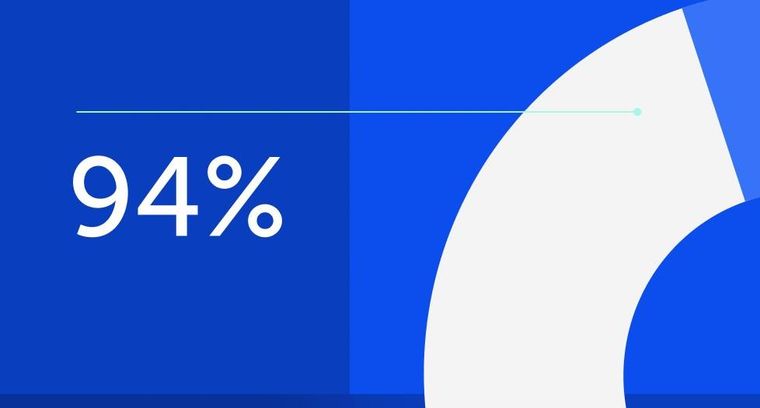
94% of researchers rate our articles as excellent or good
Learn more about the work of our research integrity team to safeguard the quality of each article we publish.
Find out more
ORIGINAL RESEARCH article
Front. Pharmacol., 20 October 2022
Sec. Ethnopharmacology
Volume 13 - 2022 | https://doi.org/10.3389/fphar.2022.1025540
This article is part of the Research TopicEfficacy and Mechanism of Herbal Medicines and Their Functional Compounds in Preventing and Treating Cardiovascular Diseases and Cardiovascular Disease Risk FactorsView all 15 articles
Objective: Network pharmacology provides new methods and references for the research of traditional Chinese medicine, but some problems remain, such as single evaluation components and index methods, imperfect relevant databases, unscientific prediction results, and lack of verification of results. Herein, we used a modified network pharmacology research method to explore the potential network analysis mechanism of Huoxue Qingre decoction in the treatment of coronary heart disease and utilized clinical trials for assessment.
Methods: Based on literature research, the targets corresponding to the drug were obtained with the assistance of the TCMSP database and Swiss Target Prediction, and the target proteins were corrected using the UniProt database. The targets related to coronary heart disease was obtained through the GeneCards database. A protein-protein interaction network diagram was constructed, and a “component-intersection target” network diagram was drawn based on Cytoscape 3.6.2 software. The mapped targets were imported into the DAVID bioinformatics platform, which underwent Gene Ontology (GO) function and Kyoto Encyclopedia of Genes and Genomes (KEGG) pathway enrichment analysis, and the network pharmacology prediction results were evaluated through clinical trials.
Results: We obtained 151 compounds related to Huoxue Qingre decoction, 286 genes after evaluation and deduplication, and 426 genes related to coronary heart disease. Finally, 81 common target genes were obtained with 32 pathways according to the KEGG pathway enrichment analysis. The validation results of the clinical trials showed that a total of 98 differential metabolites were found in the treatment of coronary heart disease with Huoxue Qingre decoction, involving a total of 16 metabolic pathways. Compared with the network pharmacology prediction results, it was found that only the pathways in cancer (hsa05200) were the common pathways in the top 32 signaling pathways predicted by network pharmacology. The expanded network pharmacology prediction results revealed that the sphingolipid signaling pathway (hsa04071) and prostate cancer pathway (hsa05215) matched the predicted metabolic pathways, with differential metabolites of N-oleoyl-D-sphingomyelin and 1-methyl-6-phenyl-1h-imidazole[4,5-b]pyridine-2-amine.
Conclusion: Through the network analysis and metabolomic evaluation, there may be three signaling pathways that involve the Huoxue Qingre decoction in the treatment of coronary heart disease: pathways in cancer (hsa05200), sphingolipid signaling pathway (hsa04071), and prostate cancer pathway (hsa05215).
Network pharmacology is an emerging, cross and cutting-edge discipline in the systematic study of drugs in the era of artificial intelligence and big data. It emphasizes that the molecular associations between drugs and therapeutic objects can be obtained from the perspective of the system level and the whole of the biological network. It has been widely used in the interpretation of the overall mechanism of action of traditional Chinese medicine (TCM) compounds, and in the analysis of drug combinations and formula compatibility law, providing new ideas for the study of complex systems of TCM (Zhang et al., 2019). In recent years, the number of articles on network pharmacology has rapidly increased every year, with hundreds of articles being published. According to a search with the subject term “network pharmacology,” more than 2,800 Chinese knowledge network studies were conducted in 2021.
Coronary heart disease (CHD), also known as coronary atherosclerotic heart disease, is caused by narrowing or obstruction of the vascular lumen due to atherosclerotic lesions in the coronary vessels, resulting in myocardial ischemia, hypoxia, or necrosis. Coronary atherosclerosis is a factor involved in the pathogenesis of CHD. It has been reported that the current number of patients with cardiovascular diseases in China is 290 million, of which the number of patients with CHD is as high as 11 million, and this has become a major public health problem in China (Benjamin et al., 2017; Hu et al., 2019).
At present, there have been satisfactory results in the use of TCM for the treatment of CHD. The combination of western medicine disease identification and TCM syndrome differentiation has become an important form of clinical diagnosis, treatment, and rehabilitation of CHD. In TCM, blood stasis is considered to be a factor leading to the dystrophy of the heart vessels, which leads to pain when the heart vessels are abnormal, thus causing “chest obstruction” and “heartache.” Its characteristics are basically consistent with the symptoms of modern CHD. Qi is the commander of the blood, and the normal operation of blood depends on the normal promotion of Qi. If the vitality is deficient and unable to circulate the blood, the blood flow will be slow and there will be blood stasis. Therefore, the treatment of CHD should focus on promoting blood circulation and invigorating Qi (China Association of Chinese Medicine Cardiovascular Disease Branch, 2019).
Huoxue Qingre (HXQR) decoction is the clinical experience of Guang’anmen Hospital in the treatment of CHD with significant therapeutic effect. HXQR consists of eight herbs, including Salvia miltiorrhiza Bunge (Danshen, DS), Panax ginseng C.A.Mey. (Renshen, RS), Coptis chinensis Franch. (Huanglian, HL), Pinellia ternata (Thunb.) Makino (Fabanxia, BX), Paeonia lactiflora Pall. (Chishao, CS), Panax notoginseng (Burk) F. H. Chen (Sanqi, SQ), Pueraria lobata (Willd.) Maesen & S.M. Almeida ex Sanjappa & Predeep (Gegen, GG), and Cistanche deserticola Ma (Roucongrong, RCR). It has the effects of invigorating Qi and activating blood circulation, clearing heat, and removing phlegm. In this formula, Salvia miltiorrhiza is the monarch drug. Panax ginseng, Coptis chinensis, and Pinellia ternata are the minister drugs. Paeonia lactiflora, Panax notoginseng, Puerariae Lobatae, and Cistanche deserticola are adjuvant drugs. Previously, our group found that HXQR improves the mitochondrial structure and function of cardiomyocytes, inhibits inflammatory factors and myocardial apoptosis, and thus improves ventricular remodeling after myocardial infarction (Chen et al., 2017; Chen et al., 2018; Wang et al., 2019).
Network pharmacology provides new methods and references for TCM research, but problems still remain such as single evaluation components and index methods, imperfect relevant databases, unscientific prediction results, and insufficient validation of results. Therefore, in the current study, we intend to adopt and improve the network pharmacology research method (Zhang and Li, 2015; Xie et al., 2019), explore the potential mechanism of HXQR in the treatment of critical coronary strain, and verify results through clinical trials. This study will provide a demonstration for the in-depth study of network pharmacology, promote the further development and application of network pharmacology, and provide a reference for the clinical application and mechanism research of HXQR decoction and other TCM compounds.
HXQR was provided by the Department of Pharmacy, Guang’anmen Hospital, China Academy of Chinese Medical Science. It consists of Salvia miltiorrhiza Bunge (Danshen, 20.8%, Collection bar code PE02053963, product batch number 15102502), Panax ginseng C.A.Mey. (Renshen, 8.3%, Collection bar code PE 01471910, product batch number 15102902), Coptis chinensis Franch. (Huanglian, 6.9%, Collection bar code PE01268317, product batch number 15111102), Pinellia ternata (Thunb.) Makino (Fabanxia, 8.3%, Collection bar code PE02240636, product batch number SAA171), Paeonia lactiflora Pall. (Chishao, 16.7%, Collection bar code PE02247249, product batch number 16011601), Panax notoginseng (Burk) F. H. Chen (Sanqi, 4.2%, Collection bar code PE 01976963, product batch number 15041601), Pueraria lobata (Willd.) Maesen & S.M. Almeida ex Sanjappa & Predeep (Gegen, 20.8%, Collection bar code PE02015760, product batch number 15121701), and Cistanche deserticola Ma (Roucongrong, 13.9%, Collection bar code PE 01542425, product batch number 160260541) (Table 1). All medicinal names have been unified using the Kew Medicinal plant names service. All specimens are deposited in Chinese National Herbarium, Institute of Botany, Chinese Academy of Sciences (20 Nanxincun, Xiangshan, Beijing, China). All medicinal materials are provided by Beijing Kangmei Pharmaceutical Co., Ltd. (Beijing, China).
The medicinal herbs of HXQR were extracted by 10 times of water through heating reflux for twice, each time for 1.0 h. The extracts were mixed and concentrated under reduced pressure. The relative density was about 1.12 (measured at 20°C). Ethanol was added to bring the ethanol content to 70%. After 24 h, the extracts were concentrated (density 1.20 at 50°C), and dried in vacuum at 60°C. Finally, all the extracts were crushed and mixed to get an extract of HXQR.
The dry extract yielding rate of HXQR was 26.00%. The contents of active ingredients in HXQR extract were 3.58% for salvianolic acid B, 0.76% for Coptis chinensis total alkaloids (berberine, epiberberine, coptisine and palmatine), 0.33% for ginsenoside Rb1, 1.60% for paeoniflorin, 1.95% for puerarin, 0.24% for echinacoside (Chen et al., 2016). All components are tested by HPLC (Aglient 1100, Agilent Technologies, Inc., CA, United States).
Based on the systematic database of TCM pharmacology and the analysis platform (Traditional Chinese Medicine Systems Pharmacology Database (TCMSP)), the components of the main chemicals in HXQR decoction were retrieved. S. miltiorrhiza, P. ginseng, C. chinensis, P. ternata, P. veitchii, P. notoginseng, P. lobata, and C. deserticola were searched to establish a chemical database for HXQR with oral bioavailability (OB) ≥30% and drug-like (DL) ≥0.18, combined with the TCM pharmacopoeia and published literature to supplement active ingredients. According to the TCMSP database and Swiss Target Prediction, the targets corresponding to the drug were queried, and the obtained target proteins were evaluated and corrected using the UniProt database, converted into the corresponding standard gene names, and saved in the drug target database for HXQR.
In the GeneCards database, with “coronary heart disease” as the keyword, a search was performed for CHD-related genes. These genes were exported to the Excel program, and gene evaluation was performed with the median, and the median was taken for four times to evaluate the genes with a relevance score greater than the median, and a CHD target database was then established.
Mapping targets of diseases and drugs were obtained. Cytoscape 3.6.2 software was used to associate each compound with the corresponding targets to construct an “active ingredients-common targets” network. The STRING database was used to select the “homo sapiens” identification, construct the PPI network, analyze its network topological parameters, and find the key targets with node degree as an important parameter.
HXQR and CHD mapping targets were imported into the DAVID bioinformatics platform for Gene Ontology (GO) function and Kyoto Encyclopedia of Genes and Genomes (KEGG) pathway enrichment analysis. The p value was used as the condition to analyze the pathway of target regulation biology. The main signaling pathways and biological processes of HXQR exerting pharmacological effects were analyzed to explore the possible mechanism of HXQR in the treatment of CHD.
The Uniprot database and RCSB PDB protein structure database (https://www.rcsb.org/) were used to find the PDB ID (Protein Data Bank Identification) of key targets, and obtain protein information, and utilized Pymol software to process molecules and saved them as pdb format. The active ingredient information of the drug corresponding to the target in the TCMSP database was downloaded and saved in mol2 format. Molecular docking was performed using SYBYL-X 2.0 software, and the results were analyzed by the obtained total score.
There is a comprehensive understanding of the purpose, methods, benefits, and possible risks of this study, which has been registered in the TCM Clinical Trials Registry (http://www.chictr.org.cn/index.aspx; Chi CTR-IOR-17013189). This trial has been approved by the ethics committee (Guang’anmen Hospital, China Academy of Chinese Medical Sciences, No. 2017-083-KY-01).
The clinical trial of HXQR for CHD was conducted by our group according to the registered trial protocol. Only the serum of patients in the control group and experimental group (9:11) was used for metabolomics analysis in the current study, and the metabolomics analysis method was performed according to the Fujisaka method (Fujisaka et al., 2018). The analytical instrument used for this experiment was a liquid chromatography-mass spectrometry system composed of a Waters ACQUITY UPLC ultra-high performance liquid chromatography-tandem AB Triple TOF 5600 high-resolution mass spectrometer.
Chromatographic conditions: chromatographic column ACQUITY UPLC BEH C18 (100 mm × 2.1 mm, 1.7 μm); column temperature 45°C; mobile phase: (A) water (containing 0.1% formic acid), (B) acetonitrile/methanol (2/3) (v/v) (containing 0.1% formic acid); flow rate: 0.4 ml/min; injection volume: 5 μl; elution conditions: A% (95%–0) gradient elution. Mass spectrometric conditions: electrospray ionization (ESI) ion source, the positive and negative ion scanning modes were used for sample mass spectrometric signal acquisition.
The chemical constituents of each drug in HXQR were searched by TCMSP, including 67 chemical constituents related to S. miltiorrhiza, 14 chemical constituents related to C. chinensis, 25 chemical constituents related to P. ginseng, 13 chemical constituents related to P. ternata, 27 chemical constituents related to P. veitchii, 11 chemical constituents related to P. notoginseng, five chemical constituents related to P. lobata, and eight chemical constituents related to C. deserticola. Combined with the results of the pharmacopoeia and literature analysis, nine components, salvianolic acid B, tanshinone I, ginsenoside Rg1, ginsenoside Re, ginsenoside Rb1, notoginsenoside R1, puerarin, echinacoside, and acteoside, were added to obtain a total of 172 active ingredients, and 151 compounds were obtained after deleting the duplicates.
Among them, MOL002714 is a common component of P. ternata and P. veitchii; MOL002879, MOL005341, MOL005344, and MOL007476 are common components of P. ginseng and P. notoginseng; MOL005320 and MOL005384 are common components of P. ginseng and C. deserticola; MOL000098 is a common component of C. chinensis, P. notoginseng, and C. deserticola; MOL002776 is a common component of S. miltiorrhiza, P. ternata, and P. ternata; MOL000449 is a common component of P. ginseng, P. veitchii, P. veitchii, and P. notoginseng; and MOL000358 is a common component of P. ginseng, P. ternata, P. veitchii, P. notoginseng, P. lobata, and C. deserticola. The four components with the highest OB were: MOL007064 przewalskin b, MOL007132 (2R)-3-(3,4-dihydroxyphenyl)-2-[(Z)-3-(3,4-dihydroxyphenyl)acryl]oxy-propionic acid, MOL002907 corchoroside aqt, and MOL005314 celabenzine. The four components with the highest DL were: MOL013352 obacunone, MOL012297 puerarin, MOL008875 echinacoside, and MOL008871 marckine. The results are shown in Table 2.
A total of 2166 constituent targets were retrieved through the TCMSP database and the UniProt website (https://www.uniprot.org/). Human genes corresponding to TCM drug targets were searched to remove duplicates, and finally, 286 genes were obtained. A network diagram of “HXQRs-active ingredient-drug targets” was drawn. The active ingredients in the figure are expressed according to the last five digits of the MOD ID. TCM drugs are represented by initials. There are a total of 445 nodes and 1734 edges, as shown in Figure 1.
A search was performed in the GeneCards database to find the median genes related to coronary heart disease, and the genes with a relevance score greater than the median were further analyzed. Subsequently, 426 targets were obtained that intersected with the targets of the main active components of HXQR, which yielded 81 common target genes, as shown in Figure 2. Cytoscape 3.7.2 was used to visualize the relationship between the targets of the active ingredients of HXQR in the treatment of CHD. The analysis showed that HXQR involved 110 active components in the treatment of CHD, with 190 nodes and 523 edges in the network diagram. The node degree reflects the importance of nodes in the network. The area of the network diagram was directly proportional to the area ratio using the degree reaction.
The results showed that prostaglandin G/H synthase 2 (PTGS2), β-2 adrenergic receptor (ADRB2), sodium channel protein type 5 subunit α (SCN5A), androgen receptor (AR), estrogen receptor (ESR1), inducible nitric oxide lyase (NOS2), sodium-dependent serotonin transporter (SLC6A4), endothelial nitric oxide synthase (NOS3), potassium voltage-gated channel subfamily H member 2 (KCNH2), peroxisome proliferator-activated receptor γ (PPARG), and other targets were the core nodes of the network, as shown in Figure 3. In terms of compounds, quercetin (MOL000098), puerarin (MOL012297), luteolin (MOL000006), kaempferol (MOL000422), tanshinone iia (MOL007154), and baicalein (MOL002714) were associated with multiple targets and may be the main active components of HXQR decoction in the treatment of CHD.
The drug and disease intersection targets from 81 mappings were analyzed by the STRING database to construct the potential target PPI network, and the results were shown in Figure 4. There were 81 targets in the PPI network that had the ability to interact with proteins, and 1514 edges represented the interactions between proteins. The average degree value was 37.383. There were 45 target proteins with a degree value greater than that of the average. The top 20 were IL6, AKT1, VEGFA, TNF, CCL2, IL1B, EGF, CASP3, CXCL8, MAPK1, MMP9, EDN1, PTGS2, NOS3, SERPINE1, IL10, TP53, EGFR, ICAM1, and MMP2, which suggested that these proteins might play a key role in the treatment of CHD with HXQR.
The common targets of HXQR and CHD were subjected to GO enrichment analysis and included three aspects: biological process (BP), cellular component (CC), and molecular function (MF), and at p < 0.01, 254 items were obtained for BP, 26 items for CC, and 41 items for MF. BPs mainly involve positive regulation of transcription by the RNA polymerase II promoter, positive regulation of transcription, DNA template, gene expression, signal transduction, inflammatory response, cell proliferation, aging, drug response, negative regulation of apoptotic process, and response to hypoxia; CCs mainly involve the plasma membrane, extracellular space, extracellular region, cytosol, and extracellular exosome; MFs mainly involve protein binding, identical protein binding, enzyme binding, protein homodimerization activity, and cytokine activity. Among them, three aspects are in the descending order of enrichment number, and the first 20 items were plotted. The p value is taken as the negative logarithm in the figure, and the significance is expressed by the depth of color. The number of enriched genes is expressed by the size of the bubbles, and the greater the number, the larger the bubble, as shown in Figures 5A–C.
FIGURE 5. (A) Biological process of GO function bubble chart of the targets of CHD treated by HXQR. (B) Cellular compoent of GO function bubble chart of the targets of CHD treated by HXQR. (C) Molecular function of GO function bubble chart of the targets of CHD treated by HXQR.
KEGG pathway enrichment analysis was performed on the common targets of HXQR and CHD, and it was determined that 105 pathways were associated with them. Evaluating with p < 0.05 and number of genes greater than or equal to 10 resulted in 32 pathways. Among them, the enrichment number is arranged in descending order, and the bubble is plotted as shown in Figure 6, in which the p value is taken as the negative logarithm, the color indicates the size, and the gene number is expressed as the bubble size.
Five key targets with high relevance in the PPI network: IL6, AKT1, VEGFA, TNF, and CCL2 were selected to obtain PDB IDs and target structures, and the five targets were docked to their corresponding active ingredients, respectively, and when the target was combined with the compound structure, the higher the docking score, the more stable the binding of the target with the chemical (Table 3). The average docking score was 4.9 points, which showed good binding strength, and 12 of them ≥5 points, suggesting that the core target has good binding ability with HXQR decoction active ingredients. Most of the compounds were linked to the core target in hydrogen-hydrogen bonds and arranged in descending order of docking score, and the docking structure diagram with a docking score greater than six is shown below (Figures 7A–E).
FIGURE 7. (A) Docking diagram of TNF- kaempferol. (B) Docking diagram of TNF- ginsenoside rh2. (C) Docking diagram of VEGFA-luteolin. (D) Docking diagram of VEGFA-quercetin. (E) Docking diagram of IL6-paeoniflorin.
The research group carried out a small sample randomized double-blind study in the early stage. 32 patients with borderline coronary artery disease were included and randomly divided into the experimental group and the control group according to the ratio of 1:1. Both groups received conventional western medicine treatment. On this basis, the test group was given HXQR, while the control group was given HXQR placebo. After 6 months of treatment, the study found that compared with the control group, the degree of stenosis of the target plaque area in the experimental group was significantly reduced after treatment (p = 0.033) (Figure 8).
The ratios were (38.42 ± 20.68)% and (52.00 ± 13.15)%, respectively, while there was no statistical difference between the average CT value of the target plaque and the calcification score of the target plaque (Figures 9, 10).
The TCM symptom score of angina pectoris of CHD showed a significantly higher decrease of the treatment score than that of the control group (p = 0.028) (Figure 11), and the symptoms of chest tightness and palpitations were significantly improved. Before and after the treatment, there was no significant difference in total cholesterol, triglyceride, high density lipoprotein, low density lipoprotein and very low-density lipoprotein between the two groups. The blood and urine routine, liver and kidney function and electrocardiogram were within the normal range before and after treatment in the two groups, with no significant difference. The study showed that HXQR for patients with borderline coronary artery disease could significantly improve chest tightness and palpitations, and had a good effect in stabilizing plaques and improving the degree of coronary stenosis (Huang, 2019).
Metabolomic analysis by clinical serum resulted in 2490 metabolites that were obtained, with 1180 in the negative mode and 1310 in the positive mode. The VIP value of the first principal component of the OPLS-DA model was >0.1, and the p-value of the t-test was <0.05 for differential metabolite identification, and a total of 98 differential metabolites were found (Figure 12). Metabolic pathway enrichment analysis of the differential metabolites based on the KEGG database revealed a total of 16 metabolic pathways (Figure 13). By comparing the metabolomics results of this clinical trial with the prediction results of network pharmacology, only the cancer pathway (hsa05200) was found to be its common pathway among the top 32 signaling pathways predicted by network pharmacology.
The results of extended network pharmacology prediction indicated that the sphingolipid signaling pathway (hsa04071) and prostate cancer pathway (hsa05215) matched the predicted metabolic pathway, indicating that HXQR may be involved in the treatment of CHD through three signaling pathways, including the cancer pathway (hsa05200), sphingolipid signaling pathway (hsa04071), and prostate cancer pathway (hsa05215). The differential metabolites may be N-oleoyl-D-sphingomyelin (d18:1/22:0) (SM, upregulated), 1-methyl-6-phenyl-1h-imidazole[4,5-b]pyridine-2-amine and 1-phenyl-6-phenyl-1H-imidazo[4,5-b]pyridine-2-amine (downregulated).
The metabolomics results showed that after treatment with HXQR, the key metabolites of the sphingolipid signaling pathway (hsa04071), Cer (d18:2/23:0), and Cer (t18:0/20:0 (2OH)), SM (d18:1/22:0), and SM (d18:1/24:1 (15Z)), were significantly increased (p < 0.01), and the level of S1P in serum was decreased (p = 0.08) (Figure 14). 1-Methyl-6-phenyl-1H-imidazo[4,5-b]pyridin-2-amine is a key differential metabolite of prostate cancer (hsa05200) and pathways in cancer (hsa05215), which may lead to DNA damage, but the specific cause is not clear and requires in-depth study.
FIGURE 14. The key metabolites of the sphingolipid signaling pathway (hsa04071) after treatment with HXQR.
The level of Cer (d18:2/23:0), Cer (t18:0/20:0 (2OH), SM (d18:1/22:0), and SM (d18:1/24:1 (15Z)), were significantly increased after treatment with HXQR (p < 0.01), and the level of S1P in serum was decreased after treatment with HXQR (p = 0.08).
Modern medical research indicates that the human body is an organism with a complex network. Cardiovascular and cerebrovascular diseases, cancer, tumors and other chronic diseases are not driven by a single cause. Only by analyzing a variety of signal transduction pathways of diseases can achieve a final ideal therapeutic effect. TCM compound preparations are widely used in clinical practice, with significant effect, and play an important role in disease prevention and treatment. However, how to deeply elucidate the complex chemical system and mechanism of action of TCM from the molecular level and establish a complete system including active ingredients and targets has become the focus and difficulty of modernization research of TCM. Network pharmacology provides a new method and reference for the study of TCM, which is consistent with the overall mechanism of action of TCM “multiple components and multiple targets” and has received the attention and favor of the majority of researchers. However, from our group’s analysis of numerous studies related to network pharmacology, we can conclude that the following problems may need to be solved in current network pharmacology research.
It is unreasonable to evaluate chemical compositions with only DL and OB values, and sometimes the conclusions drawn are unscientific or even incorrect. Network pharmacology generally uses OB ≥30% and DL ≥0.18 as conditions to evaluate active ingredients, ignoring some components with unqualified OB values or/and DL values such as tanshinones, ginsenosides, notoginsenosides, puerarin, verbascoside, and echinacoside that play a major role. Therefore, it would be helpful to perform a comprehensive analysis that fully considers the possible active ingredients recorded in the Chinese Pharmacopoeia and authoritative studies while using DL and OB values. In the current study, the project team adopted OB ≥30% and DL ≥0.18, and evaluated the active ingredients in HXQR in combination with the active ingredients indicated in the Chinese Pharmacopoeia.
There is still a space for improvement in the TCMSP and analysis platform. At present, the active ingredients and targets of some commonly used TCMs are missing, resulting in serious biases in the results and conclusions. For example, it is debatable that most of the network pharmacology literature will now conclude that β-sitosterol is the active ingredient. β-Sitosterol is widely presented in nature, and confers a variety of pharmacological effects, but the network pharmacological research literature that has used β-sitosterol as an active ingredient is worth pondering. The TCMSP database currently lacks commonly used active ingredients, and contains information errors and other circumstances that require continuous improvement by researchers for optimal and rational use.
Network pharmacological results need to undergo a series of validations to draw scientific conclusions. In this study, 151 active ingredients and 286 targets of HXQR in the treatment of CHD were obtained by network pharmacology research technology, and 81 common targets were obtained by mapping the active ingredient targets of HXQR with CHD targets. KEGG pathway enrichment analysis showed that 105 pathways were related to them, 32 pathways were obtained by analyzing with p < 0.05, and the number of genes was greater than or equal to 10. In terms of compounds, quercetin (MOL000098), puerarin (MOL012297), luteolin (MOL000006), kaempferol (MOL000422), tanshinone iia (MOL007154), and baicalein (MOL002714) were associated with multiple targets and may be the main active components of HXQR decoction in the treatment of CHD. In vitro and in vivo experiments have also confirmed that quercetin has the effects of antihypertensive, regulating blood lipid, lowering blood pressure, anti-atherosclerosis and inhibiting cardiotoxicity (Papakyriakopoulou et al., 2022). Kaempferol flavonoid compounds have antioxidant and antiplatelet potential and can improve cardiovascular disease by improving oxidative stress (Olas, 2020). Puerarin, as a natural isoflavone, has been developed into a variety of injectable dosage forms for widespread use. It can be used for a variety of diseases such as atherosclerosis, cardiac hypertrophy, heart failure, myocardial infarction and hypertension and other diseases (Zhou et al., 2021). Luteolin has antioxidant and anti-inflammatory effects, and has protective effects in various diseases such as ischemia/reperfusion (I/R) injury, heart failure (HF) and atherosclerosis (AS) and can inhibit apoptosis (Xu et al., 2012; Luo et al., 2017). Tanshinone iia is well studied, also has dosage forms such as injections, has anti-inflammatory and antioxidant activities, and can induce significant cardioprotection by enhancing angiogenesis (Guo et al., 2020). Baicalein can intervene signaling molecules and protect cardiomyocytes from ischemia/hypoxia injury by acting on PI3K/Akt, MAPKs, and NF-κB/p65 signaling pathways (He et al., 2015). The data from the above studies also indicate the intervention effect of the main active ingredients on cardiovascular disease and also provide support for docking.
Metabolomics of clinical trials of HXQR for the treatment of CHD showed that the differential metabolites were identified by VIP value of the first principal component of the OPLS-DA model >0.1 and p-value value of t-test < 0.05, with only 98 differential metabolites, which were enriched for metabolic pathways and could correspond to 16 pathways. In comparing these 16 clinically proven effective pathways with the network pharmacology prediction results, only three pathways were found to be identical, which may be related to the small sample size in this study. This result demonstrated that HXQR played a role in the treatment of CHD through three signaling pathways: the cancer pathway (hsa05200), the sphingolipid signaling pathway (hsa04071), and the prostate cancer pathway (hsa05215). However, we found that these three signaling pathways only had cancer pathway (hsa05200) in the top 32 predicted pathways, and prostate cancer pathway (hsa05215) and sphingolipid signaling pathway (hsa04071) in the 36th and 60th of the KEGG prediction results of network pharmacology, respectively, which also indicated that the unvalidated network pharmacology results may be severely biased and must be subject to the large sample size and strict scientific validation before arriving at any scientific conclusions.
SM is mainly located on the cell membrane, lipoproteins (especially LDL), and other lipid-rich tissue structures, which is very important for maintaining the micro-control function of the cell membrane structure so that it can regulate the activity of growth factor receptors and extracellular matrix proteins. Its degradation and anabolic intermediates are known as sphingomyelins, which have the effect of regulating cell biological functions (Cirillo et al., 2021). The metabolites of SM include ceramide (Cer) and sphingosine-1-phosphate (S1P), of which Cer is a central molecule in sphingomyelin metabolism, and its biological functions mainly include inducing apoptosis, and the regulation of cell differentiation, cellular immunity, and inflammatory responses. In this process, sphingomyelinase (SMase) is the key enzyme regulating SM metabolism, which can decompose SM to produce Cer and phosphorylcholine. Cer is cleaved to sphingosine (Sph) by ceramidase (CDase), and phosphatidylcholine generates S1P by sphingosine kinase (SphK), which then activates the downstream MAPK, BAX/BCL-2, and PI3K/AKT signaling pathways involved in the regulation of cell proliferation and apoptosis (Nishino et al., 2019; Cirillo et al., 2021; Green et al., 2021). The current study showed that higher plasma levels of Cer-16 and SM-16 were associated with increased risk of heart failure, and higher levels of Cer-22, SM-20, SM-22, and SM-24 with decreased risk of heart failure (Lemaitre et al., 2019; Fretts et al., 2021). Plasma S1P and sphingomyelin levels were significantly negatively correlated with the left ventricular ejection fraction and the severity of dyspnea (Polzin et al., 2017; Deshpande et al., 2018). This also demonstrates the consistency of the clinical trial evaluation with the network pharmacological analysis.
Through network analysis and metabolomic evaluation, there may be three signaling pathways that involve the Huoxue Qingre decoction in the treatment of CHD: pathways in cancer (hsa05200), sphingolipid signaling pathway (hsa04071), and prostate cancer pathway (hsa05215). Network pharmacology provides a method reference for the study of modernization of TCM. However, it is necessary to recognize the existing problems of network pharmacology and adopt a reasonable method to solve these problems in order to draw possible and correct conclusions.
The datasets presented in this study can be found in online repositories. The names of the repository/repositories and accession number(s) can be found in the article/Supplementary Material.
The studies involving human participants were reviewed and approved by Guang’anmen Hospital, China Academy of Chinese Medical Sciences. The patients/participants provided their written informed consent to participate in this study.
Y-QT: Methodology, Investigation, Formal analysis, Writing – original draft, Writing – review and editing. MJ: Conceptualization. X-HH: Methodology, Investigation. H-WC: Conceptualization, Supervision, Writing, Funding acquisition. All of the authors read and approved the submitted version.
This study was supported by the China Academy of Chinese Medical Sciences Innovation Fund-Major research project (CI 2021A05011; CI 2021A04619; CI 2021B017-05); and the National Natural Science Foundation of China (No. 82074396).
We thank LetPub (www.letpub.com) for its linguistic assistance during the preparation of this manuscript.
The reviewer HW declared a shared parent affiliation with the authors to the handling editor at the time of the review. The authors declare that the research was conducted in the absence of any commercial or financial relationships that could be construed as a potential conflict of interest.
All claims expressed in this article are solely those of the authors and do not necessarily represent those of their affiliated organizations, or those of the publisher, the editors and the reviewers. Any product that may be evaluated in this article, or claim that may be made by its manufacturer, is not guaranteed or endorsed by the publisher.
The Supplementary Material for this article can be found online at: https://www.frontiersin.org/articles/10.3389/fphar.2022.1025540/full#supplementary-material
ADRB2, β-2 adrenergic receptor; AR, androgen receptor; BP, biological process; CC, cellular component; CDase, ceramidase; Cer, ceramide; CHD, Coronary heart disease; DL, drug-like; ESR1, estrogen receptor; GO, Gene Ontology; HXQR, Huoxue Qingre decoction; KCNH2, potassium voltage-gated channel subfamily H member 2; KEGG, Kyoto Encyclopedia of Genes and Genomes; MF, molecular function; NOS2, inducible nitric oxide lyase; NOS3, endothelial nitric oxide synthase; OB, oral bioavailability; PPARG, peroxisome proliferator-activated receptor γ; PPI, protein-protein interaction; PTGS2, prostaglandin G/H synthase 2; S1P, sphingosine-1-phosphate; SCN5A, sodium channel protein type 5 subunit α; SLC6A4, sodium-dependent serotonin transporter; SM, N-oleoyl-D-sphingomyelin; SMase, sphingomyelinase; Sph, sphingosine; SphK, sphingosine kinase; TCM, traditional Chinese medicine; TCMSP, Traditional Chinese Medicine Systems Pharmacology Database.
Benjamin, E. J., Blaha, M. J., Chiuve, S. E., Cushman, M., Das, S. R., Deo, R., et al. (2017). Heart disease and stroke statistics-2017 update: A report from the American heart association. Circulation 135 (10), e146–e603. doi:10.1161/CIR.0000000000000485
Chen, H. W., Dong, Y., He, X., Li, J., and Wang, J. (2018). Paeoniflorin improves cardiac function and decreases adverse postinfarction left ventricular remodeling in a rat model of acute myocardial infarction. Drug Des. Dev. Ther. 12, 823–836. doi:10.2147/DDDT.S163405
Chen, H. W., Li, J., Lin, F., Li, J. P., and Wang, J. (2017). Effect of xuanbi antong formula in improving ventricular remodeling in myocardial infarction rats and its mechanism. Chin. J. Exp. Tradit. Med. Form. 23 (23), 71–78. doi:10.13422/j.cnki.syfjx.2017230071
Chen, H. W., Zhang, C. Y., Ren, W. G., Wang, J., and Wei, B. J. (2016). Multi-index integrated evaluation method optimizes water-extraction and ethanol precipitation of Xuanbi'antong formula (XBF). Chin. J. Chin. Mat. Med. 41, 70–74. doi:10.4268/cjcmm20160114
China Association of Chinese Medicine Cardiovascular Disease Branch (CACMDB) (2019). Guideline for diagnosis and treatment of Chinese medicine in stable angina coronary artery disease. J. Tradit. Chin. Med. 60 (21), 1880–1890. doi:10.13288/j.11-2166/r.2019.21.015
Cirillo, F., Piccoli, M., Ghiroldi, A., Monasky, M. M., Rota, P., La Rocca, P., et al. (2021). The antithetic role of ceramide and sphingosine-1-phosphate in cardiac dysfunction. J. Cell. Physiol. 236 (7), 4857–4873. doi:10.1002/jcp.30235
Deshpande, G. P., Imamdin, A., Lecour, S., and Opie, L. H. (2018). Sphingosine-1-phosphate (s1p) activates stat3 to protect against de novo acute heart failure (ahf). Life Sci. 196, 127–132. doi:10.1016/j.lfs.2018.01.023
Fretts, A. M., Jensen, P. N., Hoofnagle, A. N., McKnight, B., Sitlani, C. M., Siscovick, D. S., et al. (2021). Circulating ceramides and sphingomyelins and risk of mortality: The cardiovascular health study. Clin. Chem. 67 (12), 1650–1659. doi:10.1093/clinchem/hvab182
Fujisaka, S., Avila-Pacheco, J., Soto, M., Kostic, A., Dreyfuss, J. M., Pan, H., et al. (2018). Diet, genetics, and the gut microbiome drive dynamic changes in plasma metabolites. Cell Rep. 22 (11), 3072–3086. doi:10.1016/j.celrep.2018.02.060
Green, C. D., Maceyka, M., Cowart, L. A., and Spiegel, S. (2021). Sphingolipids in metabolic disease: The good, the bad, and the unknown. Cell Metab. 33 (7), 1293–1306. doi:10.1016/j.cmet.2021.06.006
Guo, R., Li, L., Su, J., Li, S., Duncan, S. E., Liu, Z., et al. (2020). Pharmacological activity and mechanism of tanshinone IIA in related diseases. Drug Des. devel. Ther. 14, 4735–4748. doi:10.2147/DDDT.S266911
He, X., Hao, C., and Li, Z. (2015). Advances in study on baicalein for regulation of signaling pathways in myocardial ischemia/hypoxia. Chin. Tradit. Herb. Drugs. 46 (11), 1685–1691. doi:10.7501/j.issn.0253-2670.2015.11.024
Hu, S. S., Gao, R. L., Liu, L. S., Zhu, M. L., Wang, W., and Wang, Y. J. (2019). Summary of the 2018 report on cardiovascular diseases in China. Chin. Circ. J. 34 (03), 209–220. doi:10.3969/j.issn.1000-3614.2019.03.001
Huang, M. Y. (2019). Clinical study on xuanbi antong formula in treatment of coronary borderline lesions with phlegm, blood stasis and heat syndrome. Chin. Acad. Chin. Med. Sci., 1–73. Master’s thesis.
Lemaitre, R. N., Jensen, P. N., Hoofnagle, A., Mcknight, B., Fretts, A. M., King, I. B., et al. (2019). Plasma ceramides and sphingomyelins in relation to heart failure risk: The cardiovascular health study. Circ. Heart Fail. 12 (7), e005708. doi:10.1161/CIRCHEARTFAILURE.118.005708
Luo, Y., Shang, P., and Li, D. (2017). Luteolin: A flavonoid that has multiple cardio-protective effects and its molecular mechanisms. Front. Pharmacol. 8, 692. doi:10.3389/fphar.2017.00692
Nishino, S., Yamashita, H., Tamori, M., Mashimo, M., Yamagata, K., Nakamura, H., et al. (2019). Translocation and activation of sphingosine kinase 1 by ceramide-1-phosphate. J. Cell. Biochem. 120 (4), 5396–5408. doi:10.1002/jcb.27818
Olas, B. (2020). Honey and its phenolic compounds as an effective natural medicine for cardiovascular diseases in humans? Nutrients 12 (2), 283. doi:10.3390/nu12020283
Papakyriakopoulou, P., Velidakis, N., Khattab, E., Valsami, G., Korakianitis, I., and Kadoglou, N. P. (2022). Potential pharmaceutical applications of quercetin in cardiovascular diseases. Pharm. (Basel, Switz. 15 (8), 1019. doi:10.3390/ph15081019
Polzin, A., Piayda, K., Keul, P., Dannenberg, L., Mohring, A., Gräler, M., et al. (2017). Plasma sphingosine-1-phosphate concentrations are associated with systolic heart failure in patients with ischemic heart disease. J. Mol. Cell. Cardiol. 110, 35–37. doi:10.1016/j.yjmcc.2017.07.004
Wang, L. H., He, X. H., Ma, T., Tian, P. P., Li, J., and Chen, H. W. (2019). Optimization of water extraction and alcohol precipitation process and improvement of cardiac function in rats with myocardial infarction (stasis-heat syndrome) for GCRF formula. Chin. J. New Drug. 28 (07), 862–870.
Xie, J., Gao, S., Li, L., Xu, Y. L., Gao, S. M., and Yu, C. Q. (2019). Research progress and application strategy on network pharmacology in Chinese materia medica. Chin. Tradit. Herb. Drug. 50 (10), 2257–2265. doi:10.7501/j.issn.0253-2670.2019.10.001
Xu, T., Li, D., and Jiang, D. (2012). Targeting cell signaling and apoptotic pathways by luteolin: Cardioprotective role in rat cardiomyocytes following ischemia/reperfusion. Nutrients 4 (12), 2008–2019. doi:10.3390/nu4122008
Zhang, R., Zhu, X., Bai, H., and Ning, K. (2019). Network pharmacology databases for traditional Chinese medicine: Review and assessment. Front. Pharmacol. 10, 123. doi:10.3389/fphar.2019.00123
Zhang, Y. Q., and Li, S. (2015). Progress in network pharmacology for modern research of traditional Chinese medicine. Chin. J. Pharmacol. Toxicol. 29 (06), 883–892. doi:10.3867/j.issn.1000-3002.2015.06.002
Keywords: network pharmacology, traditional Chinese medicine (TCM), Huoxue Qingre decoction, coronary heart disease (CHD), metabolomics
Citation: Tan Y-Q, Jin M, He X-H and Chen H-W (2022) Huoxue Qingre decoction used for treatment of coronary heart disease network analysis and metabolomic evaluation. Front. Pharmacol. 13:1025540. doi: 10.3389/fphar.2022.1025540
Received: 23 August 2022; Accepted: 11 October 2022;
Published: 20 October 2022.
Edited by:
Kuo Gao, Beijing University of Chinese Medicine, ChinaReviewed by:
Yong Wang, Beijing University of Chinese Medicine, ChinaCopyright © 2022 Tan, Jin, He and Chen. This is an open-access article distributed under the terms of the Creative Commons Attribution License (CC BY). The use, distribution or reproduction in other forums is permitted, provided the original author(s) and the copyright owner(s) are credited and that the original publication in this journal is cited, in accordance with accepted academic practice. No use, distribution or reproduction is permitted which does not comply with these terms.
*Correspondence: Xuan-Hui He, aGV4dWFuemkxNjQ2QHNpbmEuY29t; Heng-Wen Chen, Y2hlbmhlbmd3ZW5AMTYzLmNvbQ==
†These authors have contributed equally to this work
Disclaimer: All claims expressed in this article are solely those of the authors and do not necessarily represent those of their affiliated organizations, or those of the publisher, the editors and the reviewers. Any product that may be evaluated in this article or claim that may be made by its manufacturer is not guaranteed or endorsed by the publisher.
Research integrity at Frontiers
Learn more about the work of our research integrity team to safeguard the quality of each article we publish.