- 1Department of Periodical Press, West China Hospital, Sichuan University, Chengdu, China
- 2Chinese Evidence-based Medicine Center, West China Hospital, Sichuan University, Chengdu, China
- 3West China School of Medicine, Sichuan University, Chengdu, China
- 4Department of Nephrology, West China Hospital, Sichuan University, Chengdu, China
Objectives: Tumours remain a serious threat to human life. Following rapid progress in oncology research, tyrosine kinase inhibitors have been used to treat multiple tumour types. Given the great influence of kidneys on pharmacokinetics, renal toxicities associated with TKIs have attracted attention. However, the TKIs with the lowest risks of renal impairment are unclear. In this study, we conducted a Bayesian network meta-analysis to compare the incidence of renal impairment among different TKIs in patients with tumours.
Methods and analysis: Six databases (PubMed, EMBASE, The Cochrane Library, Chinese National Knowledge Infrastructure, Wanfang Data, and China Biomedical Literature Database) were electronically searched from inception to 1 November 2021 to identify randomized controlled trials on the incidence of renal impairment for different TKIs in patients with tumours. Two reviewers independently screened the literature, extracted data, and assessed the risk of bias of the included studies. Then, a pairwise meta-analysis was conducted using Stata version 13, and network meta-analysis within the Bayesian framework was conducted using R software version 3.5.3 with the package “gemtc 0.8–2” recalling JAGS (version 4.3.0).
Results: Overall, 34 randomized controlled trials were included in this study. Although renal toxicity was common among patients receiving TKIs, the incidence and severity greatly differed among the drugs and studies. Elevated creatinine and protein levels were the most common nephrotoxic events, whereas haematuria was relatively rare. Among TKIs, nintedanib and ripretinib carried the lowest risks of renal impairment.
Conclusion: TKIs displayed different profiles of renal toxicity because of their different targets and underlying mechanisms. Clinicians should be aware of the risks of renal impairment to select the optimal treatment and improve patient adherence to treatment.
Systematic Review Registration: [www.crd.york.ac.uk/prospero/], identifier [CRD42022295853].
1 Introduction
Cancer is a serious threat to human life. According to the World Health Organization, malignant tumours represent the leading cause of disease burden as estimated using cause-specific disability-adjusted life years in both men and women (Mattiuzzi and Lippi, 2019). Globally, an estimated 19.3 million new cancer cases and almost 10 million cancer deaths occurred in 2020. Nearly 28.4 million new cancer cases are expected to occur in 2040, representing a 47% increase vs. the number in 2020 (Sung et al., 2021). Because of the rapid progress in oncology research, the treatment paradigm for tumours has changed dramatically. Surgery and radiotherapy remain the primary treatments for local and non-metastatic cancers, whereas anticancer drugs (chemotherapy, hormone therapies, and biological therapies) are used for metastatic cancers. Compared with traditional chemotherapy, novel targeted therapy, a type of biological therapy, has significantly improved clinical outcomes and provided the foundation for precision medicine in cancer treatment (White Al-Habeeb et al., 2016). Targeted therapies are designed to block specific biologic transduction pathways or cancer proteins involved in tumour growth and differentiation, thereby minimizing the death of normal cells and avoiding undesirable side effects (Perez-Herrero and Fernandez-Medarde, 2015).
Tyrosine kinases (TKs) comprise a family of proteins that contribute to the development of cancer. This class of proteins catalyzes the transfer of phosphate groups on ATP to the tyrosine residues of several proteins, thereby phosphorylating proteins and then transferring signals to regulate cell growth, differentiation, death, and a series of physiological and biochemical processes (Jiao et al., 2018). More than 50% of proto-oncogenes and oncogene products have TK activity, and their abnormal expression leads to tumourigenesis (Drake et al., 2014). Therefore, TKs have emerged as valuable targets for drug development in oncology research. Based on their structure, function, and localization, TKs can be categorized into receptor tyrosine kinases (RTKs), non-receptor tyrosine kinases (NRTKs), and dual-specificity kinases that can phosphorylate serine, threonine, and tyrosine residues. Agents targeting these proteins, so-called tyrosine kinase inhibitors (TKIs), comprise a class of small-molecule, orally administered drugs (Hartmann et al., 2009; Patterson et al., 2009; Roskoski, 2020). There are two types of TKIs, namely cellular TKIs that target NRTKs and receptor TKIs that target single receptors, such as EGFR, or multiple targets. For example, sunitinib targets vascular endothelial growth factor receptors 1–3 (VEGFR1–3), PDGFR, KIT, Flt3, and RET (Broekman et al., 2011). TKIs have been used to treat multiple cancer types, including lung cancer, breast cancer, pancreatic cancer, and gastrointestinal stromal tumours. Compared with conventional chemotherapy, TKIs are associated with significantly reduced treatment-associated toxicity (Wu et al., 2016; Holleman et al., 2019; Huang et al., 2020). However, only a few of these drugs are strictly selective for one target. Because of the ubiquitous physiological roles of protein kinases in the body, adverse events affecting various organs have been identified during TKI treatment (Petrelli et al., 2012; Hamnvik et al., 2015; Fujita et al., 2017). Given that kidneys play an important role in pharmacokinetics, renal toxicities associated with TKIs have attracted attention (Grothey et al., 2013; Troxell et al., 2016; Ramachandran et al., 2018). Furthermore, the high rates of chronic kidney disease and concomitant nephrotoxic drug administration make patients with cancer susceptible to renal injuries from treatment (Launay-Vacher et al., 2015; Zhao et al., 2021a). Hypertension, electrolyte disturbance, and renal impairment are the most commonly reported renal adverse events (Jhaveri et al., 2016). Because the first two events probably result from multiple causes, it is difficult to ascribe them to renal toxicities. We only focused on renal impairment, which was reflected by abnormal urinalysis results and elevated serum creatinine levels in this study. These abnormal results of laboratory tests, including proteinuria, haematuria, and acute or chronic renal insufficiency, are also the main reasons for the referral of patients with tumours to the nephrology department. According to information available at NIH PubChem and FDA.gov, more than 50 TKIs have been approved by the FDA. The incidence, patterns, and severity of renal adverse effects are inconsistent among different TKIs. Information about these events is important for clinical decision-making. However, the renal toxicities of TKIs, especially newer TKIs, are limited to data from case reports, series, or simple pairwise comparisons. There is no network evidence chain among a variety of TKIs, and the TKIs with the lowest incidence of renal impairment are unclear. Therefore, a network analysis that includes all TKIs and systematically measures their comparative kidney safety is of great necessity. This study used network meta-analysis to systematically evaluate the renal adverse reactions of patients with tumours after receiving TKI treatment and rank the incidence of nephrotoxicity of different TKIs to provide a reference for clinicians to choose anti-cancer drugs.
2 Methods
This study has been registered on PROSPERO (www.crd.york.ac.uk/prospero/) under the number PROSPERO CRD42022295853. The protocol followed the Preferred Reporting Items for Systematic Reviews and Meta-analyses Protocol (S1 Checklist) (Moher et al., 2015).
2.1 Patient and public involvement
It was not appropriate or possible to involve patients or the public in the design, conduct, reporting, or dissemination plans of our research.
2.2 Eligibility criteria
2.2.1 Types of studies
Only randomized clinical trials (RCTs) that evaluated the renal adverse reactions of TKIs in the treatment of tumours were included. Observational, cohort, case–control, case series, qualitative, and laboratory studies were excluded. There were no limitations on the status, language, or year of publication.
2.2.2 Types of participants
Patients with malignant tumours diagnosed by clinicopathology were eligible. No limitations were implemented regarding age, sex, tumour type, and course of disease.
2.2.3 Types of interventions and comparator(s)/control
The experimental group was treated with at least one TKI, and the control group was treated with another (or additional) TKI, chemotherapy, or placebo (blank control). The experimental group was treated with at least one TKI combined with chemotherapy or routine treatment, and the control group was treated with another (or additional) TKI combined with the same chemotherapy or routine treatment.
2.2.4 Types of outcome measures
The outcome measures were the incidence of renal adverse reactions, grade 1–2 adverse reactions, grade 3–4 adverse reactions, elevated serum creatinine (Scr) levels, proteinuria, and haematuria.
2.2.5 Exclusion criteria
After multiple screenings, duplicate studies and studies for which the full text or complete data could not be obtained were excluded.
2.3 Data sources and search strategy
Six databases, namely PubMed, EMBASE, The Cochrane Library, Chinese National Knowledge Infrastructure (CNKI), Wanfang Data, and China Biomedical Literature Database, were electronically searched from inception to 1 November 2021 to identify RCTs of the incidence of renal impairment for different TKIs in patients with tumours from inception to 1 November 2021. In addition, the references of the identified studies were traced to supplement the relevant literature. Retrieval was performed using combinations of subject words and free words as follows: tyrosine kinase inhibitors, TKI, sunitinib, sorafenib, pazopanib, asitinib, cabozantinib, lenvatinib, vandetanib, lestartinib, tandutinib, afatinib, erlotinib, gefitinib, imatinib, nilotinib, ponatinib, dasatinib, bosutinib, ibrutinib, tivozanib, adverse reactions, side effects, renal injury, renal failure, elevated serum creatinine, proteinuria, haematuria, and randomization. An example of the search strategy is presented in Figure 1.
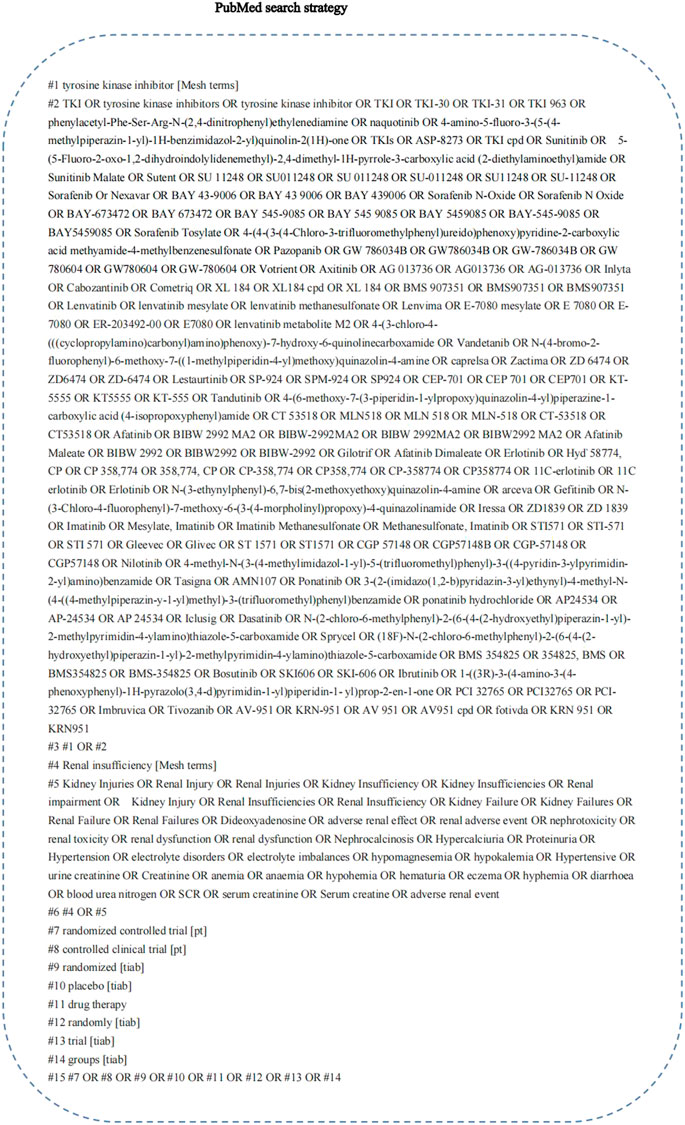
FIGURE 1. PubMed search strategy. A complete retrieval strategy of PubMed database which was used to search the literature in this study.
2.4 Literature screening and data extraction
Two evaluators independently screened the literature, extracted the data, and cross-checked the information. In case of differences, a third party was consulted to assist in judgment, and the authors of the included studies were contacted to supplement the missing data as needed. During literature screening, we first read the title and abstract and then read the full text after excluding obviously irrelevant studies to determine whether the research was suitable for inclusion. Data extraction mainly included basic information included in the study, including the research topic, first author, journal, and time of publication; the baseline characteristics of the subjects, including the number of samples in each group and the age, gender, and disease status of the patients; specific details about the intervention measures and follow-up duration key elements of bias risk assessment, and outcome indicators and outcome measurement data of concern.
2.5 Assessment of risk of bias
Two reviewers independently used the Cochrane Risk of Bias two tool (Sterne et al., 2019) to assess the risk of bias of each included study in terms of the following seven domains: random sequence generation (selection bias), allocation concealment (selection bias), blinding of participants and personnel (performance bias), blinding of outcome assessment (detection bias), incomplete outcome data (attrition bias), selective reporting (reporting bias), and other bias. Each domain was evaluated, and the risk of bias was graded as high, low, or unclear. The strength of the body of evidence was assessed using Grading of Recommendations Assessment, Development and Evaluation tools (Guyatt et al., 2011). The corresponding author was contacted if the data were unclear, and all disagreements were resolved by a third reviewer.
2.6 Statistical analysis
Conventional pairwise meta-analysis was initially performed considering the available head-to-head comparisons. We used the odds ratio (OR) or its logarithm and its 95% confidence interval (CI) to estimate the risk of renal adverse events for different regimens. A standard random-effects model was applied because of the expected variation among various regimens to provide more conservative estimated effects. Statistical heterogeneity was assessed using the I2 statistic (Higgins and Thompson, 2002). Bayesian network meta-analysis was conducted using random-effects generalized linear models based on the Markov chain Monte Carlo method (Gelman and Rubin, 1996). Each of the four chains was simultaneously run for 50,000 burn-ins and 100,000 inference iterations per chain to obtain the posterior distribution. The convergence of the model was detected using the Gelman–Rubin method combined with a density plot and tract plot (Brooks and Gelman, 1998). For all outcomes, we summarized the evidence by drawing a network relation graph. The renal adverse events of different treatment regimens was ranked according to the surface under the cumulative ranking (SUCRA) curve (Salanti et al., 2011). League tables were used to summarize all possible comparisons in the network, which indicated whether the estimated differences among different regimens were statistically significant. Model fit was assessed by calculating the deviance information criterion (DIC) as the sum of the posterior mean of the residual deviance and leverage pD. The transitivity assumption was evaluated by comparing the distribution of potential effect modifiers (mean age, sex ratio, sample size, and year) across treatment comparisons. In our analysis, global inconsistency was evaluated by the design-by-treatment interaction approach (Chaimani et al., 2013). To check the assumption of local consistency, the loop-specific approach and node-splitting method were used. We adopted the τ2 test to evaluate the extent of heterogeneity for each outcome. Additionally, meta-regression and sensitivity analyses were conducted to explore the sources of heterogeneity and ensure the validity and robustness of the findings. Furthermore, to probe the rankings of all treatment regimens for the secondary outcomes, we conducted subgroup analyses based on different outcome definitions (renal adverse event grade) and cancer types. Publication bias was assessed by examining the potential presence of small study effects via the visual inspection of comparison-adjusted funnel plots (Dias et al., 2010). Pairwise meta-analysis was conducted using Stata version 13 (StataCorp LP, College Station, TX, USA), and network meta-analysis within the Bayesian framework was conducted using R software version 3.5.3 (R Foundation for Statistical Computing, Vienna, Austria) with the package “BUGsnet” recalling JAGS (version 4.3.0) (Neupane et al., 2014). p < 0.05 indicated statistical significance.
3 Results
3.1 Document screening process and results
After database retrieval and supplementary retrieval, we obtained 2,115 documents, and after eliminating duplicate documents, we obtained 1969 documents. After reading the titles and abstracts, 168 studies were obtained. After reading the full text, 34 RCTs (Richardson et al., 2018; Zhao et al., 2021b; Motzer et al., 2013a; Motzer et al., 2013b; Sternberg et al., 2013; Rini et al., 2011; Kudo et al., 2018; Yang et al., 2017; Vilgrain et al., 2017; Spreafico et al., 2014; Sun et al., 2018; Symonds et al., 2015; Thornton et al., 2012; Qin et al., 2021; Schoffski et al., 2021; Sheng et al., 2019; Belani et al., 2014; Besse et al., 2017; Blay et al., 2020; Bukowski et al., 2007; Chi et al., 2021; Choueiri et al., 2020; Ellis et al., 2014; du Bois et al., 2014; Martin et al., 2017; Gravalos et al., 2018; Gross-Goupil et al., 2018; Zhou et al., 2019; Hall et al., 2020; Haas et al., 2021; Machiels et al., 2018; Langerbeins et al., 2015; Li et al., 2016; Schlumberger et al., 2015) were finally included (Figure 2).
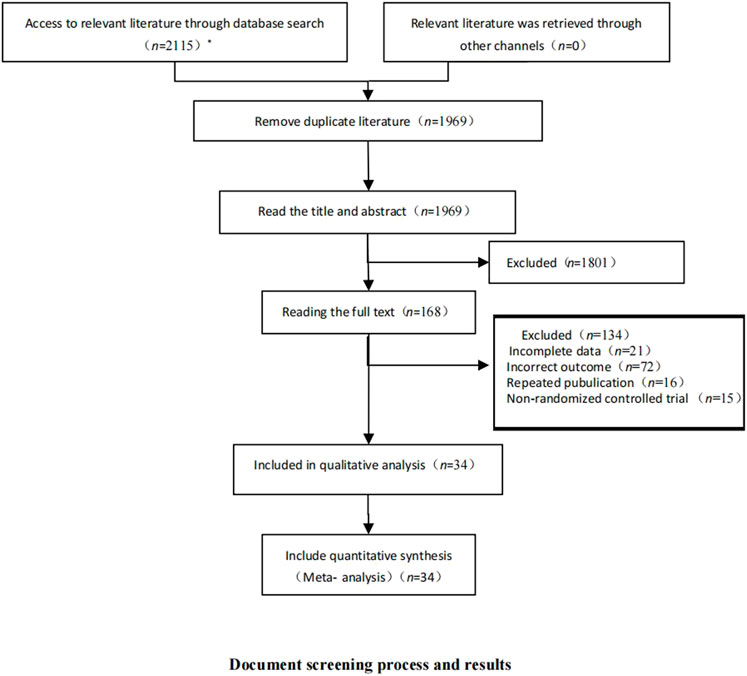
FIGURE 2. Document screening process and results. This figure shows the database and results retrieved by this study and the literature screening process. * The number of databases searched and documents checked were as follows: CNKI (n = 11); Wanfang Data (n = 45); PubMed (n = 998); Embase (n = 120); and The Cochrane Library (n = 941).
3.2 Basic characteristics of the included studies
Most of the included RCTs were published after 2010. In total, the studies included 13,398 patients with tumours, and the tumour types were widely distributed. The studies reported 26 different interventions, including TKI therapy alone and TKI therapy combined with chemotherapy or placebo. Table 1 presents the basic characteristics of the included studies.
3.3 Bias risk assessment results of the included studies
Among the 34 included RCTs, 22 studies (65%) clearly reported the randomization methods, 10 studies (29%) clearly reported the allocation concealment, and 19 studies (56%) clearly reported the blinding methods. Other studies did not clearly report these methods, but they were mentioned in the article, leading to an overall low-to-moderate risk of bias. Figure 3 and Table 2 present the bias risk assessment results of the included studies.
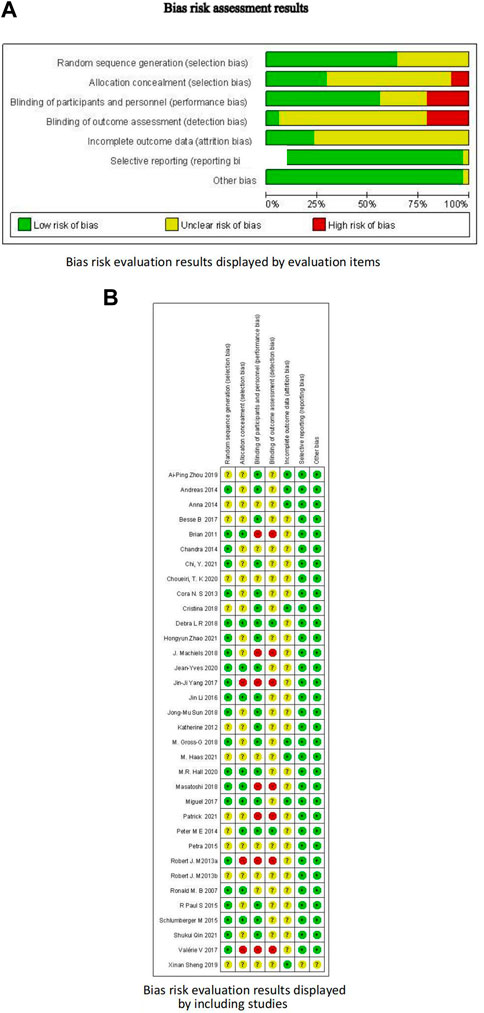
FIGURE 3. Bias risk assessment results. (A) Bias risk evaluation results displayed by evaluation items; (B) Bias risk evaluation results displayed by including studies.
3.4 Results of network meta-analysis
3.4.1 Network diagram
After using the data. prep () function to prepare the data, we used the net. plot () function to describe the research network graphically. The net. plot () function can output the network diagram of outcome indicators as required, the size of nodes is proportional to the number of studies included in each group, and the thickness of node connections is proportional to the number of studies directly compared at both ends of the nodes. If a closed loop is formed between nodes, this indicates that these studies can be simultaneously included in the comparison.
Using adverse events (AE) 1–2 as an example, it is apparent from Figure 4 that in addition to icotinib and WBI–chemotherapy, the other 24 interventions could form a closed loop. Among them, pazopanib, apatinib, and placebo have been more extensively studied, followed by lenvatinib, axitinib, and sorafenib.
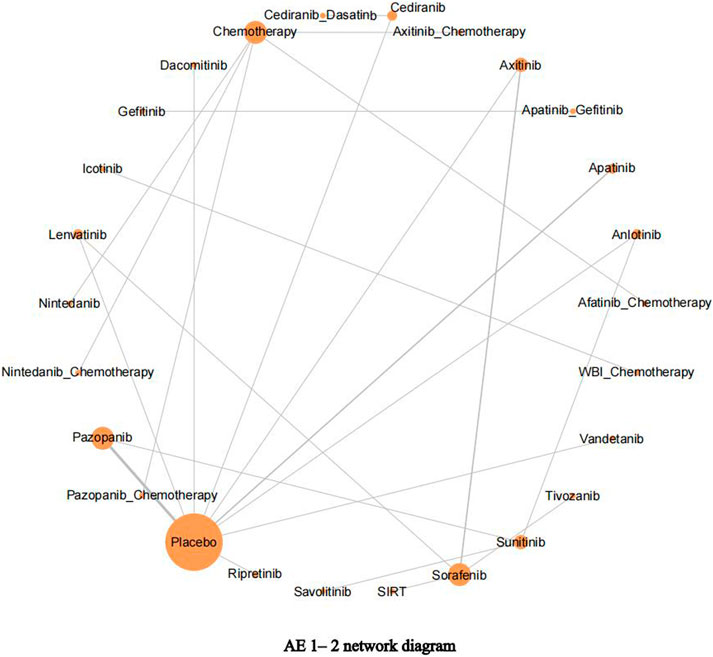
FIGURE 4. Adverse events (AE) 1–2 network diagram. The network diagram of outcome indicators AE 1–2, the size of nodes is proportional to the number of studies, and the thickness of node connections is proportional to the number of studies directly compared at both ends of the nodes.
3.4.2 Heterogeneity and consistency test
The nma. fit () function can perform model fitting and identify potential outliers. The lever plots and DIC values produced by this function can help determine the optimal effect model. The lever diagram presents the comparison between leverageik and Bayesian deviation residuals of all I tests and each of the K arms. This can highlight potential outliers when fitting the model; that is, if the data point is outside the purple arc (x2 + y = 3), it might lead to poor model fitting. nma. compare () compares the posterior mean deviation of each data group between consistency and the ume model to judge the consistency among the included research results. Using AE1–2 as an example, the lever diagram and consistency test of its model fitting are presented in Figures 5, 6. In general, the possibility of inconsistency in the included studies was small, and indirect comparisons could be made. It should be noted that because the outcome indicator of this study is adverse reactions, there was a zero value in the data set, and nma. fit () function cannot calculate the leverage ratio, PD, and DIC values of data points. However, it had no impact on subsequent data analyses.
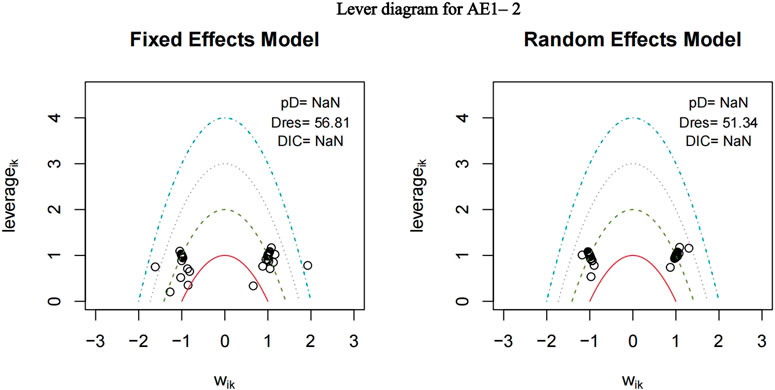
FIGURE 5. Lever diagram for adverse events (AE) 1–2. The lever diagram presents the comparison between leverageik and Bayesian deviation residuals of all I tests and each of the K arms.
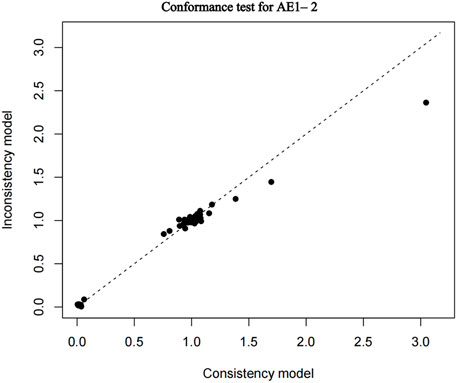
FIGURE 6. Conformance test for adverse events (AE) 1–2. Conformance test compares the posterior mean deviation of each data group between consistency and the ume m (b) Bias risk evaluation results displayed by including studies odel to judge the consistency among the included research results.
3.4.3 Direct comparison of meta-analysis results
The nma. forest () function can output forest maps, compare the combined results of drugs in different studies and different effector levels, and present effective intervention measures. Using placebo as the reference, the direct comparison forest map of each outcome indicator is presented in Figure 7. The meta-analysis revealed no significant difference between each intervention group and placebo concerning the incidence of grade 1–2 adverse events, whereas the incidence of grade 1–2 adverse events was higher in the chemotherapy group and TKI combined with chemotherapy group. Axitinib, lenvatinib, sorafenib, and tivozanib had higher risks of grade 3–4 adverse events than placebo. Nintedanib and ripretinib had lower risks of Scr elevation, but the difference between the two groups was not statistically significant. There was no significant difference in the incidence of proteinuria between the intervention groups and placebo.
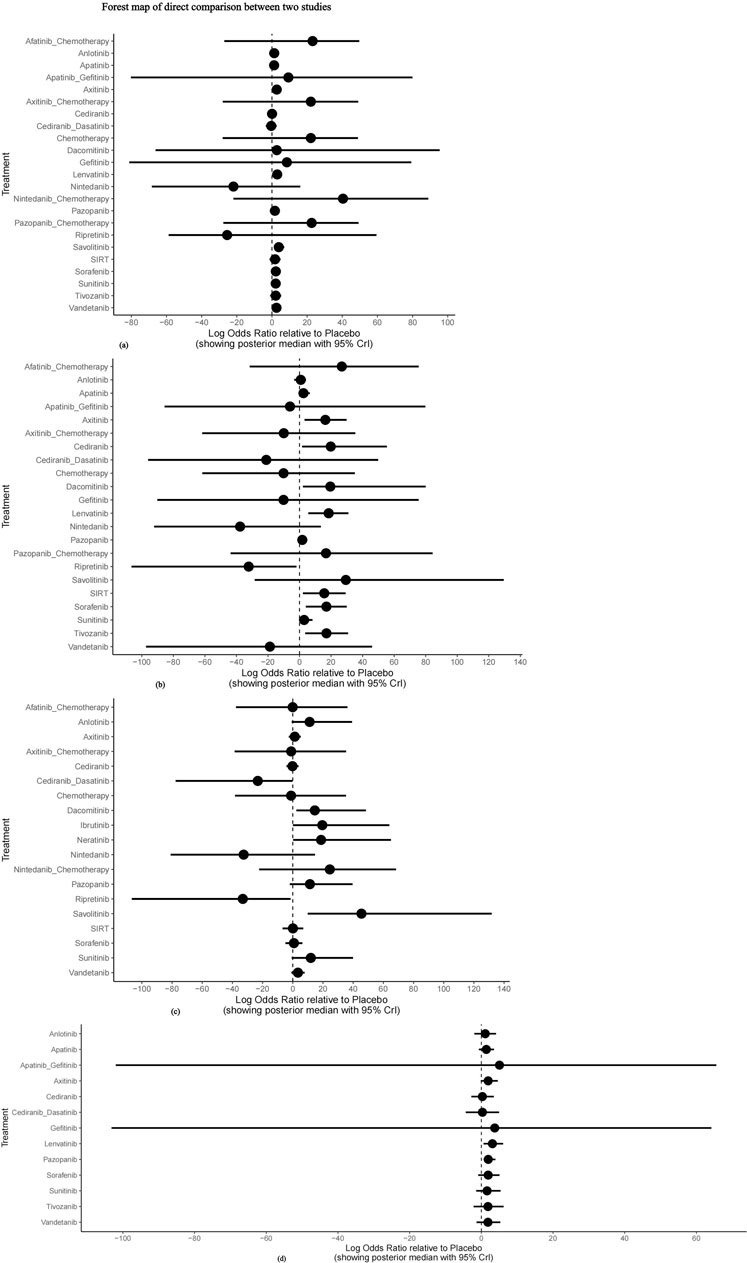
FIGURE 7. Forest map of direct comparison between two studies. (A) Forest map of direct comparison on adverse events (AE) 1–2; (B) Forest map of direct comparison on adverse events (AE) 3–4; (C) Forest map of direct comparison on elevated serum creatinine levels; (D) Forest map of direct comparison on proteinuria.
In conclusion, axitinib, lenvatinib, sorafenib, and tivozanib had higher probabilities of grade 3–4 adverse events than placebo, whereas nintedanib and ripretinib were the safest drugs compared with placebo.
3.4.4 Mesh meta-analysis results
Nma.rank () can compare the posterior probabilities of various intervention measures to achieve the ranking and comparison of NMA analysis results. The SUCRA and rankogram charts intuitively display the sorting probability of each intervention group in the form of curves and histogram. The function parameter larger better is set to FALSE, which means that the risk of outcome indicators increases as the input value increases.
As presented in Figure 8, consistent with the forest plot results, the nintedanib curve was always higher than that of other treatments. This drug had the smallest risk of grade 1–2 adverse events, whereas nintedanib combined with chemotherapy had the highest risk. Ripretinib and nintedanib carried the lowest risks of grade 3–4 adverse events, whereas lenvatinib had the highest risk. Ripretinib, cediranib in combination with dasatinib, and nintedanib had lower risks of Scr elevation, whereas other interventions had higher risks of Scr elevation than placebo. Compared with that for placebo, the probability of proteinuria was higher for all interventions, particularly lenvatinib, gefitinib, and apatinib combined with gefitinib.
The heat map of the ranking table generated by the nma. league () function provided an estimation of the relative effects and permitted comparisons of the relative effects between any pair of interventions. The heat map of each outcome index ranking table is presented in the figure, including the RR and 95% CI of each outcome index in all intervention groups.
As presented in Figure 9, nintedanib and ripretinib carried lower risks of grade 1–2 adverse events than placebo. Conversely, nintedanib combined with chemotherapy, afatinib combined with chemotherapy, and pazopanib combined with chemotherapy had high risks of such events. Whereas nintedanib, ripretinib, and vandetanib were linked to lower risks of grade 3–4 adverse events, afatinib combined with chemotherapy, dacomitinib, and cediranib had high risks of such events.
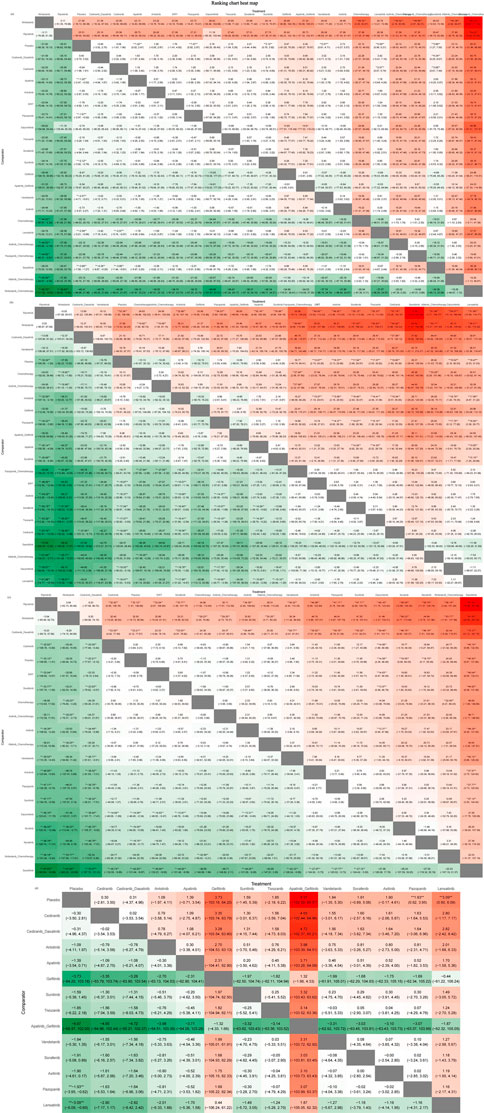
FIGURE 9. Ranking chart heat map. The heat map of each outcome index ranking table presented comparisons of the relative effects between any pair of interventions, including the RR and 95%CI of each outcome index in all intervention groups. (A) Adverse events (AE) 1–2 ranking chart heat map; (B) adverse events (AE) 3–4 ranking chart heat map; (C) Scr elevation ranking chart heat map; (D) proteinuria ranking chart heat map.
The interventions with lower risk of Scr elevation were ripretinib, nintedanib, and cediranib combined with dasatinib, whereas those with higher risks were savolitinib and pembrolizumab combined with axitinib. The interventions with lower risks of proteinuria were cediranib and cediranib combined with dasatinib, whereas apatinib combined with gefitinib and gefitinib monotherapy were associated with higher risks.
Among the included studies, four reported data for haematuria. Because of different intervention measures and a lack of evidence, meta-analysis could not be conducted. Therefore, the descriptive report of each research result was as follows (Table 3). In 2017, Yang et alreported the rates of haematuria in patients treated with icotinib (4/85) and WBI combined with chemotherapy (11/73) (Yang et al., 2017). Spreafico et alreported that the incidence of haematuria was lower for cediranib combined with dasatinib (0/11) than for cediranib monotherapy (1/11) (Spreafico et al., 2014). Chi et alstated that anlotinib (30/282) had a higher risk of haematuria than placebo (5/137) (Chi et al., 2021). Zhao et alrevealed that gefitinib combined with apatinib (40/157) had a higher risk of haematuria than gefitinib alone (24/154) (Zhao et al., 2021b).
4 Discussion
Because of their superior selectivity, efficacy, and safety compared with those of traditional chemotherapeutic drugs, some TKIs have become the first-line treatments for cancer in recent years. With the increasing use of these drugs, their renal toxicities are gradually being recognized. Although dose titration is not needed in most conditions and nearly all cases of renal impairment resolve after treatment cessation, therapy discontinuation or a switch to another medicine should be considered in severe cases. Therefore, it is important for clinicians to learn about the risk of kidney injury to select the optimal drug, especially for patients with diminished baseline renal function.
Our study found that renal adverse effects caused by TKIs are common. The highest reported incidence of grade 1–2 adverse events was nearly 70% (Motzer et al., 2013a), and the rate of grade 3–4 adverse events reached a maximum of 19.1% (Zhao et al., 2021b). It should be noted that the nephrotoxicity of TKIs varies greatly among different drugs and studies (the lowest reported incidence is 0%). Scr elevation and proteinuria were the most common events, whereas haematuria was relatively rare. Among the included studies, Scr elevation was recorded by 18 (47.4%) studies, and 23 (60.5%) studies reported proteinuria. Only four (10.5%) studies reported haematuria.
We further ranked the renal adverse reactions of TKIs during cancer treatment via network meta-analysis. Overall, TKIs combined with chemotherapy were linked to the highest incidence of renal injury, in which the chemotherapeutic agents played a major role. It has been demonstrated that chemotherapeutic drugs can impair the glomerulus, tubules, interstitium, and renal microvasculature (Malyszko et al., 2017). Nephrotoxicity caused by chemotherapy remains a significant complication limiting the application of these drugs. In terms of treatment with a single drug, savolitinib, dacomitinib, and ibrutinib were associated with greater rates of Scr elevation than other TKIs. Savolitinib is a highly selective mesenchymal epithelial transition factor (MET)–TKI. The underlying mechanism related to renal impairment is unknown. According to a study on the MET–TKI tepotinib, Scr elevation was the most common treatment-related adverse event (63.2%, all grade 1–2) (Sakai et al., 2021). The cause is considered to be reduced creatine secretion by renal tubules resulting from the direct inhibitory effect of the drug on renal tubular transporters. Therefore, the elevation of Scr caused by the reversible interaction with creatinine transporters differs from that caused by true renal failure. Drug adjustment could be unnecessary in this condition, although it is sometimes difficult to define clinically. Dacomitinib is a second-generation irreversible EGFR–TKI that is mainly used to treat non-small cell lung cancer. Because EGFR is also expressed in the kidneys, renal toxicity might be caused by anti-EGFR drugs. EGF is expressed in the ascending portion of Henle’s loop and distal tubules, but the role of EGFR in kidney function remains obscure. Studies on acute kidney injury (AKI) in animal models illustrated EGFR activation could promote renal recovery. However, another study demonstrated that EGFR activation is associated with renal fibrosis in chronic kidney disease (Tang et al., 2013). Recently, a few cases of glomerulonephritis and vasculitis secondary to EGFR–TKI therapy have been reported in the literature (Latcha et al., 2018; Oki et al., 2022), with AKI being most common. Regarding the mechanisms involved, renal hypoperfusion secondary to dehydration is believed to be a prime inducement. EGFR expressed in the gastrointestinal tract is responsible for mucosal integrity and the regulation of ionic transport. Anti-EGFR TKIs can block the negative regulation of chloride secretion and eventually cause diarrhoea (Crosnier et al., 2021). Among the three generations of anti-EGFR TKIs, the second generation, which includes dacomitinib, has the highest incidence of diarrhoea (Rugo et al., 2019). Therefore, AKI caused by these drugs is functional and actually an adverse effect in the context of digestive toxicity. Ibrutinib, which inhibits Bruton TK, is approved for the treatment of chronic lymphocytic leukaemia and mantle cell lymphoma. Scr elevation associated with this agent has been reported in a few previous studies. Dehydration and tumour lysis syndrome have been implicated in the pathogenesis of renal insufficiency (Byrd et al., 2013; Wang et al., 2015). Ripretinib and nintedanib were linked to the lowest incidence of Scr elevation. Both drugs target multiple TKs such as PDGFR and VEGFR (Dhillon, 2020; Schoffski et al., 2021). The reasons for their lower risks of adverse events are unclear.
Among the TKIs, gefitinib had the highest incidence of proteinuria. Similar to other anti-EGFR TKIs, gefitinib can elicit both proteinuria and Scr elevation during cancer treatment. According to a reported case, gefitinib caused nephrotic proteinuria, which was ascribed to an autoimmune response to the drug (Kumasaka et al., 2004). In another case, minimal change glomerulonephritis, the pathogenesis of which is related to an immune disorder, was proven by renal biopsy in a patient with proteinuria after gefitinib treatment (Maruyama et al., 2015). Lenvatinib and axitinib were associated with the second and third highest rates of proteinuria, respectively. Both drugs target VEGFR. Compared with other target therapies, proteinuria appears to be a common side effect on vascular endothelial growth factor (VEGF)-inhibiting agents (Gurevich and Perazella, 2009). The kidneys are rich in VEGF and VEGFR, and their interaction is critical for the maintenance of normal function and integrity of the glomerular basement membrane of kidneys. Thus, the kidneys are highly susceptible to the adverse effects of anti-VEGF medications. Both proteinuria and acute renal failure can occur in patients using these drugs (Izzedine et al., 2014). According to previous studies, the underlying pathologic changes involved proliferative glomerulopathies, thrombotic microangiopathy, and interstitial nephritis (Abbas et al., 2015). Interestingly, cediranib which is also an inhibitor of VEGFR tyrosine kinases showed the lowest risk of proteinuria. Haematuria related to TKIs has rarely been reported. In our study, only four RCTs recorded the incidence of haematuria. In patients treated with anlotinib or gefitinib alone, the rate of haematuria was approximately 10%. It is worth noting that the difference in the incidence of haematuria between the groups in the study was similar to that of proteinuria. This result indicated that haematuria arises from the same mechanism as proteinuria.
In conclusion, our present work provided insights into the previously unreported relative risks of nephrotoxicity associated with TKIs, and nearly all types of TKIs were covered. According to the results, all types of TKIs carry a risk of kidney injury although the underlying mechanisms may be different. Although direct injury, secondary autoimmune disorder, and dehydration have been regarded to contribute to kidney injury, the exact mechanisms remain to be clarified. The infeasibility of performing renal biopsy in patients with tumours in many cases has become the greatest obstacle to exploring this problem. Early studies have suggested that the occurrence of kidney injury depends both on the drug class and agent (Piscitani et al., 2020). Based on their lowest risks of renal adverse events, ripretinib and nintedanib are recommended for the treatment of kidney disease patients with advanced gastrointestinal stromal tumour and non-small cell lung cancer respectively. Cediranib can serve as the first choice in anti-VEGFR treatment when proteinuria is concomitant.
Our study had some limitations. First, some RCTs included in this study did not clarify the used randomization method, and blinding was not used or reported in some studies, which may have led to selection and performance bias. Second, the baseline characteristics of the patients included in the different RCTs, especially the tumour type and treatment strategies, were inconsistent, which may lead to heterogeneity among the studies. For example, patients with renal cell cancer were at higher risk of renal failure because of previous partial or radical nephrectomy (Launay-Vacher et al., 2011). Third, the severity of renal effects might be greatly affected by the different targets of TKIs, which is determined by underlying mechanisms that remain to be elucidated. However, because of the limited data, it is difficult to conduct further subgroup analysis at present. More evidence provided by high-quality studies is needed for future research.
Data availability statement
The original contributions presented in the study are included in the article/supplementary material, further inquiries can be directed to the corresponding author.
Author contributions
YX and XC conceived and designed the study. The protocol was drafted and revised by YX and XC. YX and JW designed the search strategies. QW, YL, YX, and XC performed searching, data curation, and assessment independently. QW, YL, YX, and JW analyzed and interpreted the data. YX and XC arbitrated disagreements during the review. All authors have read the study and approved its publication.
Acknowledgments
We thank Joe Barber Jr., PhD, from Liwen Bianji (Edanz) (www.liwenbianji.cn) for editing the English text of a draft of this manuscript.
Conflict of interest
The authors declare that the research was conducted in the absence of any commercial or financial relationships that could be construed as a potential conflict of interest.
Publisher’s note
All claims expressed in this article are solely those of the authors and do not necessarily represent those of their affiliated organizations, or those of the publisher, the editors and the reviewers. Any product that may be evaluated in this article, or claim that may be made by its manufacturer, is not guaranteed or endorsed by the publisher.
References
Abbas, A., Mirza, M. M., Ganti, A. K., and Tendulkar, K. (2015). Renal toxicities of targeted therapies. Target. Oncol. 10 (4), 487–499. doi:10.1007/s11523-015-0368-7
Belani, C. P., Yamamoto, N., Bondarenko, I. M., Poltoratskiy, A., Novello, S., Tang, J., et al. (2014). Randomized phase II study of pemetrexed/cisplatin with or without axitinib for non-squamous non-small-cell lung cancer. BMC Cancer 14, 290. doi:10.1186/1471-2407-14-290
Besse, B., Mazieres, J., Ribassin-Majed, L., BarlesiF., , Bennouna, J., GeRvais, R., et al. (2017). Pazopanib or placebo in completely resected stage I NSCLC patients: Results of the phase II IFCT-0703 trial. Ann. Oncol. 28 (5), 1078–1083. doi:10.1093/annonc/mdx070
Blay, J. Y., Serrano, C., Heinrich, M. C., Zalcberg, J., Bauer, S., Gelderblom, H., et al. (2020). Ripretinib in patients with advanced gastrointestinal stromal tumours (INVICTUS): A double-blind, randomised, placebo-controlled, phase 3 trial. Lancet. Oncol. 21 (7), 923–934. doi:10.1016/S1470-2045(20)30168-6
Broekman, F., Giovannetti, E., and Peters, G. J. (2011). Tyrosine kinase inhibitors: Multi-targeted or single-targeted? World J. Clin. Oncol. 2 (2), 80–93. doi:10.5306/wjco.v2.i2.80
Brooks, S. P., and Gelman, A. General methods for monitoring convergence of iterative simulations. J. Comput. Graph. Stat. 1998;7(4):434–455. doi: Doi doi:10.2307/1390675
Bukowski, R. M., Kabbinavar, F. F., Figlin, R. A., Flaherty, K., Srinivas, S., Vaishampayan, U., et al. (2007). Randomized phase II study of erlotinib combined with bevacizumab compared with bevacizumab alone in metastatic renal cell cancer. J. Clin. Oncol. 25 (29), 4536–4541. doi:10.1200/JCO.2007.11.5154
Byrd, J. C., Furman, R. R., Coutre, S. E., Flinn, I. W., Burger, J. A., Blum, K. A., et al. (2013). Targeting BTK with ibrutinib in relapsed chronic lymphocytic leukemia. N. Engl. J. Med. 369 (1), 32–42. doi:10.1056/NEJMoa1215637
Chaimani, A., Higgins, J. P. T., Mavridis, D., Spyridonos, P., and Salanti, G. (2013). Graphical tools for network meta-analysis in STATA. Plos One 8 (10), e76654. doi:10.1371/journal.pone.0076654
Chi, Y., Shu, Y., Ba, Y., Bai, Y., Qin, B., Wang, X., et al. (2021). Anlotinib monotherapy for refractory metastatic colorectal cancer: A double-blinded, placebo-controlled, randomized phase III trial (ALTER0703). Oncologist 26 (10), e1693–e1703. doi:10.1002/onco.13857
Choueiri, T. K., Heng, D. Y. C., Lee, J. L., Cancel, M., Verheijen, R. B., Mellemgaard, A., et al. (2020). Efficacy of savolitinib vs sunitinib in patients with MET-driven papillary renal cell carcinoma: The SAVOIR phase 3 randomized clinical trial. JAMA Oncol. 6 (8), 1247–1255. doi:10.1001/jamaoncol.2020.2218
Crosnier, A., Abbara, C., Cellier, M., Lagarce, L., Babin, M., Bourneau-Martin, D., et al. (2021). Renal safety profile of EGFR targeted therapies: A study from VigiBase® the WHO global database of individual case safety reports. Cancers 13 (23), 5907. doi:10.3390/cancers13235907
Dhillon, S. (2020). Ripretinib: First approval. Drugs 80 (11), 1133–1138. doi:10.1007/s40265-020-01348-2
Dias, S., Welton, N. J., Caldwell, D. M., and Ades, A. E. (2010). Checking consistency in mixed treatment comparison meta-analysis. Stat. Med. 29 (7-8), 932–944. doi:10.1002/sim.3767
Drake, J. M., Lee, J. K., and Witte, O. N. (2014). Clinical targeting of mutated and wild-type protein tyrosine kinases in cancer. Mol. Cell. Biol. 34 (10), 1722–1732. doi:10.1128/MCB.01592-13
du Bois, A., Floquet, A., Kim, J. W., Rau, J., del Campo, J. M., Friedlander, M., et al. (2014). Incorporation of pazopanib in maintenance therapy of ovarian cancer. J. Clin. Oncol. 32 (30), 3374–3382. doi:10.1200/JCO.2014.55.7348
Ellis, P. M., Shepherd, F. A., Millward, M., Perrone, F., Seymour, L., Liu, G., et al. (2014). Dacomitinib compared with placebo in pretreated patients with advanced or metastatic non-small-cell lung cancer (NCIC CTG BR.26): A double-blind, randomised, phase 3 trial. Lancet. Oncol. 15 (12), 1379–1388. doi:10.1016/S1470-2045(14)70472-3
Fujita, K. I., Ishida, H., Kubota, Y., and Sasaki, Y. (2017). Toxicities of receptor tyrosine kinase inhibitors in cancer pharmacotherapy: Management with clinical pharmacology. Curr. Drug Metab. 18 (3), 186–198. doi:10.2174/1389200218666170105165832
Gelman, A., and Rubin, D. B. (1996). Markov chain Monte Carlo methods in biostatistics. Stat. Methods Med. Res. 5 (4), 339–355. doi:10.1177/096228029600500402
Gravalos, C., Carrato, A., Tobena, M., Rodriguez-Garrote, M., Soler, G., Vieitez, J. M., et al. (2018). A randomized phase II study of axitinib as maintenance therapy after first-line treatment for metastatic colorectal cancer. Clin. Colorectal Cancer 17 (2), e323–e329. doi:10.1016/j.clcc.2018.02.004
Gross-Goupil, M., Kwon, T. G., Eto, M., Ye, D., Miyake, H., Seo, S. I., et al. (2018). Axitinib versus placebo as an adjuvant treatment of renal cell carcinoma: Results from the phase III, randomized ATLAS trial. Ann. Oncol. 29 (12), 2371–2378. doi:10.1093/annonc/mdy454
Grothey, A., Van Cutsem, E., Sobrero, A., Siena, S., Falcone, A., Ychou, M., et al. (2013). Regorafenib monotherapy for previously treated metastatic colorectal cancer (CORRECT): An international, multicentre, randomised, placebo-controlled, phase 3 trial. Lancet 381 (9863), 303–312. doi:10.1016/S0140-6736(12)61900-X
Gurevich, F., and Perazella, M. A. (2009). Renal effects of anti-angiogenesis therapy: Update for the internist. Am. J. Med. 122 (4), 322–328. doi:10.1016/j.amjmed.2008.11.025
Guyatt, G. H., Oxman, A. D., Schunemann, H. J., Tugwell, P., and Knottnerus, A. (2011). GRADE guidelines: A new series of articles in the journal of clinical epidemiology. J. Clin. Epidemiol. 64 (4), 380–382. doi:10.1016/j.jclinepi.2010.09.011
Haas, M., Waldschmidt, D. T., Stahl, M., Reinacher-Schick, A., Freiberg-Richter, J., Fischer von Weikersthal, L., et al. (2021). Afatinib plus gemcitabine versus gemcitabine alone as first-line treatment of metastatic pancreatic cancer: The randomised, open-label phase II ACCEPT study of the Arbeitsgemeinschaft Internistische Onkologie with an integrated analysis of the 'burden of therapy' method. Eur. J. Cancer 146, 95–106. doi:10.1016/j.ejca.2020.12.029
Hall, M. R., Dehbi, H. M., Banerjee, S., LoRd, R., ClAmp, A., Ledermann, J. A., et al. (2020). A phase II randomised, placebo-controlled trial of low dose (metronomic) cyclophosphamide and nintedanib (BIBF1120) in advanced ovarian, fallopian tube or primary peritoneal cancer. Gynecol. Oncol. 159 (3), 692–698. doi:10.1016/j.ygyno.2020.09.048
Hamnvik, O. P., Choueiri, T. K., Turchin, A., McKay, R. R., Goyal, L., Davis, M., et al. (2015). Clinical risk factors for the development of hypertension in patients treated with inhibitors of the VEGF signaling pathway. Cancer 121 (2), 311–319. doi:10.1002/cncr.28972
Hartmann, J. T., Haap, M., Kopp, H. G., and Lipp, H. P. (2009). Tyrosine kinase inhibitors - a review on pharmacology, metabolism and side effects. Curr. Drug Metab. 10 (5), 470–481. doi:10.2174/138920009788897975
Higgins, J. P., and Thompson, S. G. (2002). Quantifying heterogeneity in a meta-analysis. Stat. Med. 21 (11), 1539–1558. doi:10.1002/sim.1186
Holleman, M. S., van Tinteren, H., Groen, H. J., and Uyl-de Groot, C. A. (2019). First-line tyrosine kinase inhibitors in EGFR mutation-positive non-small-cell lung cancer: A network meta-analysis. Onco. Targets. Ther. 12, 1413–1421. doi:10.2147/OTT.S189438
Huang, L. L., Jiang, S. Y., and Shi, Y. K. (2020). Tyrosine kinase inhibitors for solid tumors in the past 20 years (2001-2020). J. Hematol. Oncol. 13 (1), 143. doi:10.1186/s13045-020-00977-0
Hutton, B., Salanti, G., Caldwell, D. M., Chaimani, A., Schmid, C. H., Cameron, C., et al. (2015). The PRISMA extension statement for reporting of systematic reviews incorporating network meta-analyses of Health Care interventions: Checklist and explanations. Ann. Intern. Med. 162 (11), 777–784. doi:10.7326/M14-2385
Izzedine, H., Escudier, B., Lhomme, C., Pautier, P., Rouvier, P., Gueutin, V., et al. (2014). Kidney diseases associated with anti-vascular endothelial growth factor (VEGF): An 8-year observational study at a single center. Med. Baltim. 93 (24), 333–339. doi:10.1097/MD.0000000000000207
Jhaveri, K. D., Sakhiya, V., Wanchoo, R., Ross, D., and Fishbane, S. (2016). Renal effects of novel anticancer targeted therapies: A review of the food and drug administration adverse event reporting system. Kidney Int. 90 (3), 706–707. doi:10.1016/j.kint.2016.06.027
Jiao, Q. L., Bi, L., Ren, Y. D., Song, S., Wang, Q., and Wang, Y. S. (2018). Advances in studies of tyrosine kinase inhibitors and their acquired resistance. Mol. Cancer 17, 36. doi:10.1186/s12943-018-0801-5
Kudo, M., Finn, R. S., Qin, S., Han, K. H., Ikeda, K., Piscaglia, F., et al. (2018). Lenvatinib versus sorafenib in first-line treatment of patients with unresectable hepatocellular carcinoma: A randomised phase 3 non-inferiority trial. Lancet 391 (10126), 1163–1173. doi:10.1016/S0140-6736(18)30207-1
Kumasaka, R., Nakamura, N., Shirato, K., Osawa, H., Takanashi, S., Hasegawa, Y., et al. (2004). Side effects of therapy: Case 1. Nephrotic syndrome associated with gefitinib therapy. J. Clin. Oncol. 22 (12), 2504–2505. doi:10.1200/JCO.2004.09.064
Langerbeins, P., Bahlo, J., Rhein, C., Cramer, P., Pflug, N., Fischer, K., et al. (2015). The CLL12 trial protocol: A placebo-controlled double-blind phase III study of ibrutinib in the treatment of early-stage chronic lymphocytic leukemia patients with risk of early disease progression. Future Oncol. 11 (13), 1895–1903. doi:10.2217/fon.15.95
Latcha, S., Jaimes, E. A., Gutgarts, V., and Seshan, S. (2018). Case of proteinuria, worsening hypertension, and glomerular endotheliosis with erlotinib and gefitinib. Kidney Int. Rep. 3 (6), 1477–1481. doi:10.1016/j.ekir.2018.07.005
Launay-Vacher, V., Aapro, M., De Castro, G., CohEn, E., Deray, G., DooleyM., , et al. (2015). Renal effects of molecular targeted therapies in oncology: A review by the cancer and the kidney international network (C-kin). Ann. Oncol. 26 (8), 1677–1684. doi:10.1093/annonc/mdv136
Launay-Vacher, V., Ayllon, J., Janus, N., Medioni, J., Deray, G., Isnard-Bagnis, C., et al. (2011). Evolution of renal function in patients treated with antiangiogenics after nephrectomy for renal cell carcinoma. Urol. Oncol. 29 (5), 492–494. doi:10.1016/j.urolonc.2009.07.023
Li, J., Qin, S., Xu, J., Xiong, J., Wu, C., Bai, Y., et al. (2016). Randomized, double-blind, placebo-controlled phase III trial of apatinib in patients with chemotherapy-refractory advanced or metastatic adenocarcinoma of the stomach or gastroesophageal junction. J. Clin. Oncol. 34 (13), 1448–1454. doi:10.1200/JCO.2015.63.5995
Machiels, J. P., Bossi, P., Menis, J., LiaM., , Fortpied, C., Liu, Y., et al. (2018). Activity and safety of afatinib in a window preoperative EORTC study in patients with squamous cell carcinoma of the head and neck (SCCHN). Ann. Oncol. 29 (4), 985–991. doi:10.1093/annonc/mdy013
Malyszko, J., Kozlowska, K., and Kozlowski, L., (2017). Nephrotoxicity of anticancer treatment. Nephrol. Dial. Transpl. 32 (6), 924–936. doi:10.1093/ndt/gfw338
Martin, M., Holmes, F. A., Ejlertsen, B., Delaloge, S., Moy, B., Iwata, H., et al. (2017). Neratinib after trastuzumab-based adjuvant therapy in HER2-positive breast cancer (ExteNET): 5-year analysis of a randomised, double-blind, placebo-controlled, phase 3 trial. Lancet. Oncol. 18 (12), 1688–1700. doi:10.1016/S1470-2045(17)30717-9
Maruyama, K., Chinda, J., Kuroshima, T., Kabara, M., Nakagawa, N., Fujino, T., et al. (2015). Minimal change nephrotic syndrome associated with gefitinib and a successful switch to erlotinib. Intern. Med. 54 (7), 823–826. doi:10.2169/internalmedicine.54.3661
Mattiuzzi, C., and Lippi, G. (2019). Current cancer epidemiology. J. Epidemiol. Glob. Health 9 (4), 217–222. doi:10.2991/jegh.k.191008.001
Moher, D., Shamseer, L., Clarke, M., Ghersi, D., Liberati, A., Petticrew, M., et al. (2015). Preferred reporting items for systematic review and meta-analysis protocols (PRISMA-P) 2015 statement. Syst. Rev. 4, 1. doi:10.1186/2046-4053-4-1
Motzer, R. J., Hutson, T. E., Cella, D., Reeves, J., Hawkins, R., Guo, J., et al. (2013). Pazopanib versus sunitinib in metastatic renal-cell carcinoma. N. Engl. J. Med. 369 (8), 722–731. doi:10.1056/NEJMoa1303989
Motzer, R. J., Nosov, D., Eisen, T., Bondarenko, I., Lesovoy, V., Lipatov, O., et al. (2013). Tivozanib versus sorafenib as initial targeted therapy for patients with metastatic renal cell carcinoma: Results from a phase III trial. J. Clin. Oncol. 31 (30), 3791–3799. doi:10.1200/JCO.2012.47.4940
Neupane, B., Richer, D., Bonner, A. J., Kibret, T., and Beyene, J. (2014). Network meta-analysis using R: A review of currently available automated packages. Plos One 9 (12), e115065. doi:10.1371/journal.pone.0115065
Oki, R., Hirakawa, Y., Oda, Y., Nakamura, M., Honda, K., Abe, H., et al. (2022). Renal-limited ANCA-associated vasculitis during erlotinib treatment for lung carcinoma. Cen. Case Rep. 11 (1), 67–72. doi:10.1007/s13730-021-00632-8
Patterson, K. I., Brummer, T., O'Brien, P. M., and Daly, R. J. (2009). Dual-specificity phosphatases: Critical regulators with diverse cellular targets. Biochem. J. 418 (3), 475–489. doi:10.1042/bj20082234
Perez-Herrero, E., and Fernandez-Medarde, A. (2015). Advanced targeted therapies in cancer: Drug nanocarriers, the future of chemotherapy. Eur. J. Pharm. Biopharm. 93, 52–79. doi:10.1016/j.ejpb.2015.03.018
Petrelli, F., Borgonovo, K., Cabiddu, M., Lonati, V., and Barni, S. (2012). Relationship between skin rash and outcome in non-small-cell lung cancer patients treated with anti-EGFR tyrosine kinase inhibitors: A literature-based meta-analysis of 24 trials. Lung Cancer 78 (1), 8–15. doi:10.1016/j.lungcan.2012.06.009
Piscitani, L., Sirolli, V., Di Liberato, L., Morroni, M., and Bonomini, M. (2020). Nephrotoxicity associated with novel anticancer agents (aflibercept, dasatinib, nivolumab): Case series and nephrological considerations. Int. J. Mol. Sci. 21 (14), E4878. doi:10.3390/ijms21144878
Qin, S., Li, Q., Gu, S., Chen, X., Lin, L., Wang, Z., et al. (2021). Apatinib as second-line or later therapy in patients with advanced hepatocellular carcinoma (AHELP): A multicentre, double-blind, randomised, placebo-controlled, phase 3 trial. Lancet. Gastroenterol. Hepatol. 6 (7), 559–568. doi:10.1016/S2468-1253(21)00109-6
Ramachandran, P., Morcus, R., Tahir, M., Onukogu, I., Spinowitz, B., and Wang, J. C. (2018). Alectinib (Alecensa)-induced reversible grade IV nephrotoxicity: A case report and review of the literature. J. Med. Case Rep. 12 (1), 303. doi:10.1186/s13256-018-1849-y
Richardson, D. L., Sill, M. W., Coleman, R. L., Sood, A. K., Pearl, M. L., Kehoe, S. M., et al. (2018). Paclitaxel with and without pazopanib for persistent or recurrent ovarian cancer: A randomized clinical trial. JAMA Oncol. 4 (2), 196–202. doi:10.1001/jamaoncol.2017.4218
Rini, B. I., Escudier, B., Tomczak, P., Kaprin, A., Szczylik, C., Hutson, T. E., et al. (2011). Comparative effectiveness of axitinib versus sorafenib in advanced renal cell carcinoma (AXIS): A randomised phase 3 trial. Lancet 378 (9807), 1931–1939. doi:10.1016/S0140-6736(11)61613-9
Roskoski, R. (2020). Properties of FDA-approved small molecule protein kinase inhibitors: A 2020 update. Pharmacol. Res. 152, 104609. doi:10.1016/j.phrs.2019.104609
Rugo, H. S., Di Palma, J. A., Tripathy, D., Bryce, R., Moran, S., Olek, E., et al. (2019). The characterization, management, and future considerations for ErbB-family TKI-associated diarrhea. Breast Cancer Res. Treat. 175 (1), 5–15. doi:10.1007/s10549-018-05102-x
Sakai, H., Morise, M., Kato, T., Matsumoto, S., Sakamoto, T., Kumagai, T., et al. (2021). Tepotinib in patients with NSCLC harbouring MET exon 14 skipping: Japanese subset analysis from the phase II VISION study. Jpn. J. Clin. Oncol. 51 (8), 1261–1268. doi:10.1093/jjco/hyab072
Salanti, G., Ades, A. E., and Ioannidis, J. P. A. (2011). Graphical methods and numerical summaries for presenting results from multiple-treatment meta-analysis: An overview and tutorial. J. Clin. Epidemiol. 64 (2), 163–171. doi:10.1016/j.jclinepi.2010.03.016
Schlumberger, M., Tahara, M., Wirth, L. J., Robinson, B., Brose, M. S., Elisei, R., et al. (2015). Lenvatinib versus placebo in radioiodine-refractory thyroid cancer. N. Engl. J. Med. 372 (7), 621–630. doi:10.1056/NEJMoa1406470
Schoffski, P., Toulmonde, M., Estival, A., Marquina, G., Dudzisz-Sledz, M., Brahmi, M., et al. (2021). Randomised phase 2 study comparing the efficacy and safety of the oral tyrosine kinase inhibitor nintedanib with single agent ifosfamide in patients with advanced, inoperable, metastatic soft tissue sarcoma after failure of first-line chemotherapy: EORTC-1506-STBSG "ANITA. Eur. J. Cancer 152, 26–40. doi:10.1016/j.ejca.2021.04.015
Sheng, X., Bi, F., Ren, X., Cheng, Y., Wang, J., Rosbrook, B., et al. (2019). First-line axitinib versus sorafenib in asian patients with metastatic renal cell carcinoma: Exploratory subgroup analyses of phase III data. Future Oncol. 15 (1), 53–63. doi:10.2217/fon-2018-0442
Spreafico, A., Chi, K. N., Sridhar, S. S., Smith, D. C., Carducci, M. A., Kavsak, P., et al. (2014). A randomized phase II study of cediranib alone versus cediranib in combination with dasatinib in docetaxel resistant, castration resistant prostate cancer patients. Invest. New Drugs 32 (5), 1005–1016. doi:10.1007/s10637-014-0106-5
Sternberg, C. N., Hawkins, R. E., Wagstaff, J., Salman, P., Mardiak, J., Barrios, C. H., et al. (2013). A randomised, double-blind phase III study of pazopanib in patients with advanced and/or metastatic renal cell carcinoma: Final overall survival results and safety update. Eur. J. Cancer 49 (6), 1287–1296. doi:10.1016/j.ejca.2012.12.010
Sterne, J. A. C., Savovic, J., Page, M. J., Elbers, R. G., Blencowe, N. S., Boutron, I., et al. (2019). RoB 2: A revised tool for assessing risk of bias in randomised trials. BMJ 366, l4898. doi:10.1136/bmj.l4898
Sun, J. M., Lee, K. H., Kim, B. S., Min, Y. J., and Yi, S. Y., (2018). Pazopanib maintenance after first-line etoposide and platinum chemotherapy in patients with extensive disease small-cell lung cancer: A multicentre, randomised, placebo-controlled phase II study (KCSG-LU12-07). Br. J. Cancer 118 (5), 648–653. doi:10.1038/bjc.2017.465
Sung, H., Ferlay, J., Siegel, R. L., Laversanne, M., Soerjomataram, I., Jemal, A., et al. (2021). Global cancer statistics 2020: GLOBOCAN estimates of incidence and mortality worldwide for 36 cancers in 185 countries. Ca. Cancer J. Clin. 71 (3), 209–249. doi:10.3322/caac.21660
Symonds, R. P., Gourley, C., Davidson, S., Carty, K., McCartney, E., Rai, D., et al. (2015). Cediranib combined with carboplatin and paclitaxel in patients with metastatic or recurrent cervical cancer (CIRCCa): A randomised, double-blind, placebo-controlled phase 2 trial. Lancet. Oncol. 16 (15), 1515–1524. doi:10.1016/S1470-2045(15)00220-X
Tang, J., Liu, N., and Zhuang, S. (2013). Role of epidermal growth factor receptor in acute and chronic kidney injury. Kidney Int. 83 (5), 804–810. doi:10.1038/ki.2012.435
Thornton, K., Kim, G., Maher, V. E., Chattopadhyay, S., Tang, S., Moon, Y. J., et al. (2012). Vandetanib for the treatment of symptomatic or progressive medullary thyroid cancer in patients with unresectable locally advanced or metastatic disease: U.S. Food and drug administration drug approval summary. Clin. Cancer Res. 18 (14), 3722–3730. doi:10.1158/1078-0432.CCR-12-0411
Troxell, M. L., Higgins, J. P., and Kambham, N. (2016). Antineoplastic treatment and renal injury: An update on renal pathology due to cytotoxic and targeted therapies. Adv. Anat. Pathol. 23 (5), 310–329. doi:10.1097/PAP.0000000000000122
Vilgrain, V., Pereira, H., Assenat, E., Guiu, B., Ilonca, A. D., Pageaux, G. P., et al. (2017). Efficacy and safety of selective internal radiotherapy with yttrium-90 resin microspheres compared with sorafenib in locally advanced and inoperable hepatocellular carcinoma (SARAH): An open-label randomised controlled phase 3 trial. Lancet. Oncol. 18 (12), 1624–1636. doi:10.1016/S1470-2045(17)30683-6
Wang, M. L., Blum, K. A., Martin, P., Goy, A., Auer, R., Kahl, B. S., et al. (2015). Long-term follow-up of MCL patients treated with single-agent ibrutinib: Updated safety and efficacy results. Blood 126 (6), 739–745. doi:10.1182/blood-2015-03-635326
White Al-Habeeb, N., Kulasingam, V., Diamandis, E. P., Yousef, G. M., Tsongalis, G. J., Vermeulen, L., et al. (2016). The use of targeted therapies for precision medicine in oncology. Clin. Chem. 62 (12), 1556–1564. doi:10.1373/clinchem.2015.247882
Wu, P., Nielsen, T. E., and Clausen, M. H. (2016). Small-molecule kinase inhibitors: An analysis of FDA-approved drugs. Drug Discov. Today 21 (1), 5–10. doi:10.1016/j.drudis.2015.07.008
Yang, J. J., Zhou, C., Huang, Y., Feng, J., Lu, S., Song, Y., et al. (2017). Icotinib versus whole-brain irradiation in patients with EGFR-mutant non-small-cell lung cancer and multiple brain metastases (BRAIN): A multicentre, phase 3, open-label, parallel, randomised controlled trial. Lancet. Respir. Med. 5 (9), 707–716. doi:10.1016/S2213-2600(17)30262-X
Zhao, D., Chen, J., Long, X., and Wang, J. (2021). Dose adjustment for tyrosine kinase inhibitors in nonsmall cell lung cancer patients with hepatic or renal function impairment (Review). Oncol. Rep. 45 (2), 413–426. doi:10.3892/or.2020.7870
Zhao, H., Yao, W., Min, X., Gu, K., Yu, G., Zhang, Z., et al. (2021). Apatinib plus gefitinib as first-line treatment in advanced EGFR-mutant NSCLC: The phase III ACTIVE study (CTONG1706). J. Thorac. Oncol. 16 (9), 1533–1546. doi:10.1016/j.jtho.2021.05.006
Keywords: Bayesian network meta-analysis, renal adverse reactions, tumours, tyrosine kinase inhibitors, treatment
Citation: Xiong Y, Wang Q, Liu Y, Wei J and Chen X (2022) Renal adverse reactions of tyrosine kinase inhibitors in the treatment of tumours: A Bayesian network meta-analysis. Front. Pharmacol. 13:1023660. doi: 10.3389/fphar.2022.1023660
Received: 20 August 2022; Accepted: 17 October 2022;
Published: 03 November 2022.
Edited by:
Yuanliang Yan, Central South University, ChinaCopyright © 2022 Xiong, Wang, Liu, Wei and Chen. This is an open-access article distributed under the terms of the Creative Commons Attribution License (CC BY). The use, distribution or reproduction in other forums is permitted, provided the original author(s) and the copyright owner(s) are credited and that the original publication in this journal is cited, in accordance with accepted academic practice. No use, distribution or reproduction is permitted which does not comply with these terms.
*Correspondence: Xiaolei Chen, irenecxl@163.com