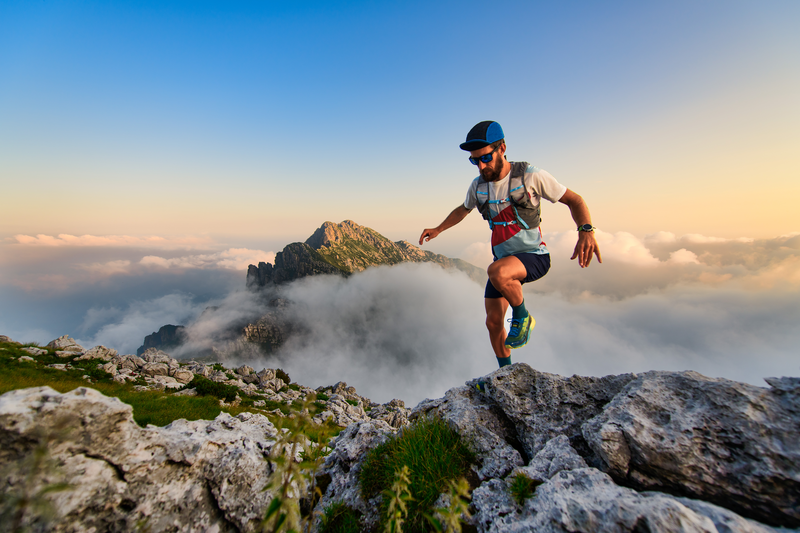
94% of researchers rate our articles as excellent or good
Learn more about the work of our research integrity team to safeguard the quality of each article we publish.
Find out more
SYSTEMATIC REVIEW article
Front. Pharmacol. , 02 November 2022
Sec. Neuropharmacology
Volume 13 - 2022 | https://doi.org/10.3389/fphar.2022.1021653
Objective: This network meta-analysis aimed to explore the effect of different drugs on mortality and neurological improvement in patients with traumatic brain injury (TBI), and to clarify which drug might be used as a more promising intervention for treating such patients by ranking.
Methods: We conducted a comprehensive search from PubMed, Medline, Embase, and Cochrane Library databases from the establishment of the database to 31 January 2022. Data were extracted from the included studies, and the quality was assessed using the Cochrane risk-of-bias tool. The primary outcome measure was mortality in patients with TBI. The secondary outcome measures were the proportion of favorable outcomes and the occurrence of drug treatment–related side effects in patients with TBI in each drug treatment group. Statistical analyses were performed using Stata v16.0 and RevMan v5.3.0.
Results: We included 30 randomized controlled trials that included 13 interventions (TXA, EPO, progesterone, progesterone + vitamin D, atorvastatin, beta-blocker therapy, Bradycor, Enoxaparin, Tracoprodi, dexanabinol, selenium, simvastatin, and placebo). The analysis revealed that these drugs significantly reduced mortality in patients with TBI and increased the proportion of patients with favorable outcomes after TBI compared with placebo. In terms of mortality after drug treatment, the order from the lowest to the highest was progesterone + vitamin D, beta-blocker therapy, EPO, simvastatin, Enoxaparin, Bradycor, Tracoprodi, selenium, atorvastatin, TXA, progesterone, dexanabinol, and placebo. In terms of the proportion of patients with favorable outcomes after drug treatment, the order from the highest to the lowest was as follows: Enoxaparin, progesterone + vitamin D, atorvastatin, simvastatin, Bradycor, EPO, beta-blocker therapy, progesterone, Tracoprodi, TXA, selenium, dexanabinol, and placebo. In addition, based on the classification of Glasgow Outcome Scale (GOS) scores after each drug treatment, this study also analyzed the three aspects of good recovery, moderate disability, and severe disability. It involved 10 interventions and revealed that compared with placebo treatment, a higher proportion of patients had a good recovery and moderate disability after treatment with progesterone + vitamin D, Bradycor, EPO, and progesterone. Meanwhile, the proportion of patients with a severe disability after treatment with progesterone + vitamin D and Bradycor was also low.
Conclusion: The analysis of this study revealed that in patients with TBI, TXA, EPO, progesterone, progesterone + vitamin D, atorvastatin, beta-blocker therapy, Bradycor, Enoxaparin, Tracoprodi, dexanabinol, selenium, and simvastatin all reduced mortality and increased the proportion of patients with favorable outcomes in such patients compared with placebo. Among these, the progesterone + vitamin D had not only a higher proportion of patients with good recovery and moderate disability but also a lower proportion of patients with severe disability and mortality. However, whether this intervention can be used for clinical promotion still needs further exploration.
Traumatic brain injury (TBI) is defined as a change in brain function or other brain pathologies caused by external forces (Capizzi et al., 2020). The severity of this disease varies from mild to moderate and severe; among these, moderate and severe TBI are more likely to lead to mortality and poor functional outcomes than mild TBI (Jochems et al., 2021). It is a growing public health problem of major proportions. Statistics show that the incidence of TBI among the elderly people in high-income countries has increased more than expected, mainly due to falls (Brazinova et al., 2021). However, the increase in the use of motor vehicles in low- and middle-income countries has led to an increase in TBI caused by road traffic accidents, mainly affecting young people (Abdelmalik et al., 2019). TBI accounts for 30%–40% of all injury-related deaths in all age groups, indicating that neurological injury will remain the most crucial reason for disability caused by neurological diseases until 2030 (Maas et al., 2017).
The acquisition and reporting of TBI epidemiological data vary greatly worldwide, leading to significant differences in robust data reliability. The prediction of research data has revealed that more than 50 million new cases every year and more than 90% of these are mild TBIs (RennieRibecco-Lutkiewicz et al., 2018). However, in Europe, 2.5 million people suffer from TBI every year, 75,000 people die of the disease, and the incidence is about 200 per 100,000 people every year. In the United States, TBI leads to more than 280,000 hospitalizations, 2.2 million emergency department visits, and more than 52,000 deaths annually. Several large-scale, population-based studies conducted in the 1980s in China showed that the incidence of TBI was 55.4–64.1 cases per 100,000 people per year, equivalent to about 770,060–890,990 new cases per year (Ban et al., 2010; Gilchrist et al., 2011; Jiang et al., 2019; Khellaf et al., 2019). Although TBI treatment strategies have evolved significantly over the past 30 years, the mortality rate remains high. In addition, this disease costs the international economy about $400 billion annually, accounting for approximately 0.5% of the annual global output, given an evaluated standardized gross world product of $73.7 trillion (Humphreys et al., 2013). This undoubtedly brings a serious economic burden to their families, society, and countries. Therefore, TBI is a medical and public health problem that must be urgently addressed.
TBI can be pathologically divided into two stages: primary and secondary injuries. Primary injury refers to direct injury to the brain, while secondary injury may be caused by changes in cerebral blood flow and intracranial pressure, hypoxemia, systemic hypotension, and cerebral edema within a few hours after the injury (Robinson, 2021). The surgical removal of intracranial hematoma and ruptured brain tissue can reduce mortality, incidence of high intracranial pressure (ICP), and length of hospital stay in patients with TBI (Hyuk and Han, 2018; Lu et al., 2019).
However, surgery may also worsen the clinical outcome of TBI, partly due to the presence of secondary injury. Based on this, several drugs with neuroprotective effects have been studied for secondary injury after brain injury with varying degrees of success, mainly involving corticosteroids, progesterone, erythropoietin, amantadine, tranexamic acid, and cytarabine (Maas et al., 2006; Wright et al., 2007; Zafonte et al., 2012; Yutthakasemsunt et al., 2013; Nichol et al., 2015; Fattahi et al., 2018). However, the clinical trials exploring the neuroprotective effects of different drugs in patients with TBI involved comparisons with placebo; no direct clinical trials comparing different drugs have been conducted.
Network meta-analysis is an extension of traditional meta-analysis. It is based on the principle that the analysis can be achieved by setting up a common control group for indirect comparison of the effectiveness and safety of multiple interventions in similar diseases when direct comparative evidence is less or lacking (Jansen and Naci, 2013; Catala-Lopez et al., 2014). Therefore, this research group attempted to use the indirect comparison principle of network meta-analysis. Data on all the direct or indirect comparative treatment interventions of neuroprotective drugs used to reduce mortality were collected to improve the prognosis and neurological function of patients with TBI. The summary analysis was conducted to explore the role of different drug treatment schemes in TBI, and to determine which drugs might be used as a more promising intervention for treating such patients by ranking.
The inclusion and exclusion criteria of this study were based on the PICOS strategy (P: Patient/population; I: Intervention; C: Comparison/control; O: Outcome; S: Study design). We included patients aged at least 15 years who suffered from TBI with a Glasgow coma score of 3–12 and the injury within 24 h. The treatment group used drugs such as corticosteroids, progesterone, erythropoietin, amantadine, tranexamic acid, citicoline, and so on; the control group received a placebo. The analysis included only randomized controlled clinical trials with a sample size of at least 10 people. The outcome indicators were mortality, and neurological function recovery rate (which were based on the GOS score).
The exclusion criteria were as follows: trials containing nontraumatic brain injury from other causes (e.g., cerebral hemorrhage, cerebral infarction, etc.), pregnant women, lactation, patients with a history of severe drug and food allergies, patients with severe heart, liver, or renal dysfunction, chronic alcohol or drug abuse, and severe psychiatric disorders; single case reports; single-arm trials without controls; and related trials without outcome indicators.
The primary outcome indicator was mortality in patients with TBI in each drug treatment group and control group. The secondary outcome indicators were GOS score (divided into five scales: 1 dead, 2 vegetative state, 3 severe disabilities, 4 moderate disability, and 5 Good recovery) used to evaluate the neurological function recovery rate of patients with TBI in different groups after each drug treatment, proportion of patients with favorable result (favorable result = good recovery + moderate disability), and adverse effects associated with different drug treatments.
The retrieval formula {[(traumatic brain injury) OR (traumatic head injury)] AND [(corticosteroids OR progesterone OR erythropoietin OR amantadine OR (tranexamic acid) OR citicoline OR (medical treatment) OR (drug treatment)] AND [(randomized controlled trial) OR random*)]} was used to conduct a comprehensive search in the databases of PubMed, Cochrane Library, Embase, and Medline from the establishment of the database to 31 January 2022. Meanwhile, relevant references, published systematic reviews, articles included in the meta-analysis, abstracts of conference papers, and ongoing or completed unpublished trials in the World Health Organization clinical registries were searched manually.
The inclusion and exclusion criteria were applied by two reviewers. They independently screened the literature-retrieval results and used the Cochrane quality evaluation method to assess all randomized trials from six aspects: randomization, allocation concealment, blind, selective bias, incomplete data, selective reporting, and other biases. In the case of randomization, the study was classified as having a “low” risk of bias if randomization was carried out with an appropriate method. The study could not be classified as having a high or low risk of bias, and if insufficient information regarding the implementation process was available, the study was defined instead as having an “unclear” risk of bias. The study was categorized as having a “high” risk of bias if randomization was not performed. Any problems or disagreements encountered during the screening of studies for inclusion in the analysis and quality assessment were resolved by two reviewers after consultation or by a third person through consultation.
Data were extracted from all included studies as follows: author, publication year, country, study type, age, intervention measures, number of people in each intervention group, details of drug treatment, mortality rates in patients with TBI in different treatment groups, rates of neurological recovery at the end of treatment, favorable outcome indicators, and incidence of side effects associated with each drug treatment. If data were missing, we contacted the corresponding author of the study wherever possible.
The heterogeneity test was carried out for all the included studies. When p > 0.1 and I2 < 50%, the results were considered non-heterogeneous, and the fixed-effects model was adopted. Otherwise, the random-effects model was adopted. We used the two-tailed statistical test and considered the difference statistically significant when p < 0.05. In the network meta-analysis, we used the surface under the cumulative ranking curve (SUCRA) to rank the treatment effects. In addition, before combining direct and indirect evidence, the node-splitting method was used to conduct a consistency test to determine whether the two could be combined. The statistical analyses were performed using RevMan 5.3 (Cochrane Collaboration, London, United Kingdom) and Stata 16.0 (StataCorp, TX, United States).
A total of 9,110 studies were retrieved. First, 7,854 duplicate studies were removed by reading titles and abstracts. Then, 1,256 studies were screened by reading the research objective and article type. Consequently, 1,129 studies were excluded (because they were irrelevant, did not involve a control group, or were reviews, animal studies, or case reports). Based on the inclusion and exclusion criteria, we screened 127 studies and excluded 53 of them (because they were second analyses, lacked main outcome indicators, or involved a sample size of at least 10). Finally, after excluding 44 (studies due to dual publication, or protocols, or inappropriate results, and so on), the remaining 30 studies were included for network meta-analysis. The screening flow chart is shown in Figure 1. The data including study design, publication years, types of interventions, and other details are shown in Tables 1, 2. The total sample size was 26,956, and the study included 13 interventions, in the order of TXA (Yutthakasemsunt et al., 2013; Chakroun-Walha et al., 2018; Collaborators, 2019; collaborators et al., 2010; Fakharian et al., 2018; Mojallal et al., 2020; Rowell et al., 2020; Perel et al., 2012), EPO (Aloizos et al., 2015; Nichol et al., 2015; Li et al., 2016; Hellewell et al., 2017; Bai and Gao, 2018; Skrifvars et al., 2018), progesterone (Wright et al., 2007; Xiao et al., 2007; Xiao et al., 2008; Shakeri et al., 2013; Skolnick et al., 2014; Wright et al., 2014; Soltani et al., 2017), progesterone + vitamin D (Aminmansour et al., 2012), atorvastatin (Farzanegan et al., 2017), beta-blocker therapy (Khalili et al., 2020), Bradycor (Marmarou et al., 1999), Enoxaparin (Baharvahdat et al., 2019), Tracoprodi (Yurkewicz et al., 2005), dexanabinol (Maas et al., 2006), selenium (Moghaddam et al., 2017), simvastatin (Shafiee et al., 2021) and placebo.
The 30 randomized controlled trials were included in the analysis. All the studies adopted the correct randomization method, and the data of the results were complete. Only Aloizos et al. (2015) had selective reports. For Aloizos et al. (2015), Aminmansour et al. (2012), Farzanegan et al. (2017), Bai and Gao, (2018), and Xiao et al. (2007), the correctness of the implementation of allocation concealment and blinding was uncertain. For Baharvahdat et al. (2019), Fakharian et al. (2018), Marmarou et al. (1999), Perel et al. (2012), Shafiee et al. (2021), (Skolnick et al. (2014), Wright et al. (2014), Xiao et al. (2008)), and Yutthakasemsunt et al. (2013), whether the correct blinding was applied in terms of outcome assessment was uncertain. Hellewell et al. (2017), Moghaddam et al. (2017), Mojallal et al. (2020), Wright et al. (2007), Rowell et al. (2020), and Shakeri et al. (2013) did not implement blinding for outcome assessment, and Chakroun-Walha et al. (2018) and Khalili et al. (2020) did not perform allocation concealment and implement blinding. This indicated that the quality of the randomized controlled studies included in the analysis was moderate (Figures 2A,B).
FIGURE 2. Quality assessment of identified randomized controlled trials; (A) Each risk of bias item presented as percentages across all included studies; (B) Each risk of bias item for each included study. Green indicates a low risk of bias, yellow indicates an unclear risk of bias, and red indicates a high risk of bias.
The subgroup analysis in terms of mortality in patients with TBI after drug treatment revealed (Supplementary Figure S1) no heterogeneity between subgroups (I2 < 50%, p > 0.1), and the fixed-effects model was adopted. The analysis showed that TXA and EPO treatment schemes significantly reduced mortality in patients with TBI compared with placebo treatment, with statistically significant differences (p = 0.009 and p = 0.003). However, compared with placebo treatment, progesterone, progesterone + vitamin D, Bradycor, Tracoprodil, dexanabinol, selenium, atorvastatin, simvastatin, Enoxaparin, and beta-blocker therapy did not reduce mortality in patients with TBI, and the differences were not statistically significant (all p > 0.05).
The subgroup analysis in terms of the proportion of patients with TBI having favorable outcomes after drug treatment revealed (Supplementary Figure S2) significant heterogeneity among the subgroups (I2 > 50%, p < 0.1), and the random-effect models was adopted. The study revealed that Enoxaparin and Progesterone + vitamin D treatment schemes significantly improved the prognosis of patients with TBI compared with placebo treatment, with statistically significant differences (p = 0.03 and p = 0.04). However, compared with placebo treatment, Bradycor, progesterone, selenium, TXA, EPO, dexanabinol, Tracoprodi, atorvastatin, simvastatin, and beta-blocker -therapy did not significantly improve the prognosis of patients with TBI, and the differences were not statistically significant (all p > 0.05).
A funnel plot analysis was performed on the mortality after the two interventions for assessing publication bias, which was drawn with the RR value of each outcome as the horizontal coordinates and SE (log[RR]) as the longitudinal coordinates. The funnel plot was basically symmetrical, indicating no evidence of publication bias for the comparison and the results were statistically robust (Figure 3).
A direct line between the two intervention groups indicated evidence of direct comparison, while no line indicated the lack of direct comparison evidence. The size of dots in the figure represents the sample size, and the thickness of lines represents the number of studies. No direct comparative evidence was found in the trials included in this study. The drug treatment schemes were indirectly compared using placebo as a medium, including 13 interventions: TXA, EPO, progesterone, progevitamin + vitamin D, atorvastatin, beta-blocker therapy, Bradycor, Enoxaparin, Tracoprodi, dexanabinol, selenium, simvastatin, and placebo (Figures 4A–E).
FIGURE 4. Network Chart; (A) Network chart based on the mortality of TBI; (B). Network chart based on the patient proportion of the favorable result of TBI; (C). Network chart based on the patient proportion of the good recovery of TBI; (D). Network chart based on the patient proportion of the moderate disability of TBI; (E). Network chart based on the patient proportion of the severe disability of TBI.
The analysis of the mortality of patients with TBI after different drug treatments, involving 13 different drug treatment regimens (Figure 5A). In this figure the larger area under the curve, this drug treatment regimen has a lower mortality, it revealed that each drug treatment intervention significantly reduced the mortality of patients with TBI compared with placebo treatment. The mortality rates were ranked from the lowest to the highest: progesterone + vitamin D, beta-blocker therapy, EPO, simvastatin, Enoxaparin, Bradycor, Tracoprodi, selenium, atorvastatin, TXA, progesterone, dexanabinol, and placebo. Further, the analysis in terms of the proportion of patients with TBI who achieved favorable outcomes (Figure 5B). In this figure, the larger area under the curve means that this drug treatment regimen has a better favorable outcome. It revealed that in patients with TBI, each drug treatment intervention significantly improved the prognosis of patients compared with placebo; the order from the highest to the lowest was Enoxaparin, progesterone + vitamin D, atorvastatin, simvastatin, Bradycor, EPO, beta-blocker therapy, progesterone, Tracoprodi, TXA, selenium, dexanabinol, and placebo.
FIGURE 5. The Rank Chart; (A) The rank chart based on the mortality of TBI; (B). The rank chart based on the patient proportion of the favorable result of TBI; (C). The rank chart based on the patient proportion of the good recovery of TBI; (D). The rank chart based on the patient proportion of the moderate disability of TBI; (E). The rank chart based on the patient proportion of the severe disability of TBI.
Subsequently, based on the classification of GOS score levels after each drug treatment, this research group performed analysis from three aspects: good recovery, moderate disability, and severe disability, involving 10 treatment schemes. The Figure 5C shows the network meta-analysis sequence diagram for the patient proportion of the good recovery of TBI. In this figure the larger area under the curve, this drug treatment regimen has a higher proportion of the good recovery. In terms of good recovery revealed that the proportion of patients recovering well after treatment with Enoxaparin, atorvastatin, progesterone + vitamin D, EPO, selenium, Bradycor, and progesterone increased compared with placebo. However, Tracoprodi and TXA regimens had a lower proportion of patients recovering well compared with placebo regimens; the order from the highest to the lowest was Enoxaparin, atorvastatin, progesterone + vitamin D, EPO, selenium, Bradycor, progesterone, placebo, Tracoprodi, and TXA. The Figure 5D shows the network meta-analysis sequence diagram for the patient proportion of the moderate disability of TBI. In this figure the larger area under the curve, this drug treatment regimen has a higher proportion of the moderate disability. In terms of moderate disability revealed that progesterone + vitamin D, Bradycor, Tracoprodi, EPO, TXA, and progesterone regimens had a higher proportion of patients with moderate disability compared with placebo. However, selenium, Enoxaparin, and atorvastatin had a lower proportion of patients with moderate disability compared with placebo; the order from the highest to the lowest was progesterone + vitamin D, Bradycor, Tracoprodi, EPO, TXA, progesterone, placebo, selenium, Enoxaparin, and atorvastatin. The Figure 5E shows the network meta-analysis sequence diagram for the patient proportion of the severe disability of TBI. In this figure the larger area under the curve, this drug treatment regimen has a higher proportion of the severe disability. This figure revealed that atorvastatin, EPO, TXA, progesterone, Tracoprodi, Enoxaparin, and selenium had a higher proportion of patients with severe disability compared with placebo. However, the progesterone + vitamin D and Bradycor regimens had a lower proportion of patients with severe disability compared with placebo; the sequence from high to low was atorvastatin, EPO, TXA, progesterone, Tracoprodi, Enoxaparin, selenium, placebo, progesterone + vitamin D, and Bradycor.
The lasting health effects of TBI can lead to damaging functional limitations and reduced quality of life, which may include cognitive decline as well as obstacles involving memory, attention, behavior, and emotion (Sulhan et al., 2020). TBI provides evidence of changes in brain function or brain pathology, mainly caused by car accidents, falls, and assaults. The severity of the resulting injury depends on many factors, including the nature of the initiating force, impact location, and magnitude (Flanagan, 2015; Zhou-Guang et al., 2015). However, the clinical outcome of TBI is not limited to the original event. In the following hours to days after TBI, the immune cells release a variety of signal molecules and inflammatory factors following neuronal and glial dysfunction, metabolic changes, nerve inflammation, and cerebral edema, leading to blood–brain barrier damage, cerebral hypoperfusion, mitochondrial dysfunction, and oxidative injury. This is known as secondary injury (Winkler et al., 2016; Dorsett et al., 2017; Simon et al., 2017; Sorby-Adams et al., 2017). With the continuous understanding of the pathophysiological evolution after TBI, the researchers discovered that secondary injury might be more destructive than primary injury. Therefore, finding effective treatment options for secondary injury may be a potential therapeutic target to improve the prognosis of such patients.
In recent years, neuroscientists have explored a number of neuroprotective medicines, but they have little effect. At present, a wide variety of neuroprotective drugs are used after TBI, but the evaluation and recognition of the therapeutic effects of these drugs are uneven. Also, which drugs are more suitable for these patients is unclear. This research group used the principle of indirect comparison of network meta-analysis to comprehensively search existing RCTs on drug therapy after TBI. Finally, 30 RCTs were included for analysis, including a total sample size of 26,956 cases and 13 kinds of intervention measures. The analysis revealed that each of these drugs significantly reduced mortality in patients with TBI and increased the proportion of patients with favorable outcomes after TBI compared with placebo. Subsequently, based on the classification of GOS after each drug treatment, this study also analyzed three aspects: good recovery, moderate disability, and severe disability, involving 10 treatment regimens. It found that progesterone + vitamin D, Bradycor, EPO, and progesterone all had a higher proportion of patients with good recovery and moderate disability compared with placebo, but a lower proportion of patients with a severe disability after progesterone + vitamin D and Bradycor treatment.
Among the interventions, progesterone + vitamin D, beta-blocker therapy, simvastatin, enoxaparin, Bradycor, Tracoprodi, selenium, atorvastatin and dexanabinol were used in only one RCT each. And the sample size of these was also very small (20, 99, 49, 26, 66, 198, 57, 21, and 428, respectively). Based on the aforementioned results, we speculated that progesterone + vitamin D treatment might be the most suitable treatment strategy for patients with TBI. However, we still believe that the result was unreliable due to the small sample size, and hence needs to be validated using a large number of high-quality RCTs.
This study examined the remaining RCTs for the effectiveness of TXA, EPO and progesterone in patients with TBI. The TXA was used in 8 RCTs, with a sample size of 10674. Our study showed that TXA reduced the mortality in these patients and improved their favorable outcomes. However, Lawati et al. (2021), showed an inconsistent result; they revealed that TXA probably had no effect on mortality or disability in these patients. The reason for this inconsistency that their study focused on ICU-related brain injury. Also, the study by CRASH-2 collaborators exploring the effectiveness of TXA in patients with moderate-severe TBI was not included. The intervention using progesterone was applied in 7 RCTs and with a sample size of 1,296. Our result showed that progesterone was reduced the mortality in these patients and improved their favorable outcomes. The result was similar to the findings of Pan et al. (2019) and Zhang et al. (2020), who indicated that progesterone administration was associated with a lower mortality and improved the clinical outcomes. Additionally, Lu et al. (2016), also revealed that progesterone improved neurologic outcome in these patients but did not decrease mortality. Therefore, the effect of this drug on mortality also needed further exploration. The last 6 RCTs explored the effectiveness of EPO in such patients, and the sample size was 789. Our result showed that EPO also reduced the mortality in these patients and improved their favorable outcomes. This was similar to the findings of Lee et al. (2019) and Liu et al. (2017), who showed that EPO might prevent the death of these patients. However, the improvement in neurological outcome(s) did not reach statistical significance. Hence, this effect of EPO remains unclear and needs further investigation.
Several limitations of this network meta-analysis need to be considered to better explain the results of this study and make this analysis provide some reference value in clinical treatment. First, only the EPO, TXA, and progesterone groups had a large sample size, and large-scale high-quality randomized controlled trials were included in the analysis. However, the remaining nine interventions involved only one RCT with a small sample size, and their results were unreliable. A further summary analysis is still needed after a large number of relevant large-scale high-quality RCTs are published. Second, the overall incidence of drug treatment–related adverse effects were not given for all interventions included in the analysis. Therefore, this network meta-analysis did not conduct a clustering analysis in terms of both effectiveness and safety. Whether the existence of adverse effects affected the ranking of their associated treatment effectiveness was not determined. In addition, whether blinding and allocation concealment were correctly implemented in some of the 30 RCTs included in this analysis was unclear. Moreover, blinding and allocation concealment were not implemented in two trials, which also reduced the strength of evidence in this analysis to some extent. Therefore, further exploration is still needed to remedy these deficiencies so as to draw more precise and convincing conclusions to guide clinical treatment.
The analysis of this study revealed that in patients with TBI, TXA, EPO, progesterone, progesterone + vitamin D, atorvastatin, beta-blocker therapy, Bradycor, Enoxaparin, Tracoprodi, dexanabinol, selenium, and simvastatin reduced mortality and increased the proportion of patients with favorable outcomes compared with placebo. The progesterone + vitamin D regimen had not only a high proportion of patients with good recovery and moderate disability but also a low proportion of patients with severe disability and mortality. However, the feasibility of this intervention for clinical promotion needs further exploration.
The original contributions presented in the study are included in the article/Supplementary Material, further inquiries can be directed to the corresponding authors.
HX and TC performed the study subject and design, data extraction, statistical analysis, interpretation of data and manuscript drafting. ML and XH contributed to the study design, statistical analysis and manuscript revising. YW and WL contributed to the study design, interpretation of data. LX performed statistical analysis. ZJ extracted the data. QH performed quality assessment. DS was involved in critical revision of manuscript.
The authors declare that the research was conducted in the absence of any commercial or financial relationships that could be construed as a potential conflict of interest.
All claims expressed in this article are solely those of the authors and do not necessarily represent those of their affiliated organizations, or those of the publisher, the editors and the reviewers. Any product that may be evaluated in this article, or claim that may be made by its manufacturer, is not guaranteed or endorsed by the publisher.
The Supplementary Material for this article can be found online at: https://www.frontiersin.org/articles/10.3389/fphar.2022.1021653/full#supplementary-material
Abdelmalik, P. A., Draghic, N., and Ling, G. S. F. (2019). Management of moderate and severe traumatic brain injury. Transfusion 59, 1529–1538. doi:10.1111/trf.15171
Aloizos, S., Evodia, E., Gourgiotis, S., Isaia, E. C., Seretis, C., and Baltopoulos, G. J. (2015). Neuroprotective effects of erythropoietin in patients with severe closed brain injury. Turk. Neurosurg. 25, 552–558. doi:10.5137/1019-5149.JTN.9685-14.4
Aminmansour, B., Nikbakht, H., Ghorbani, A., Rezvani, M., Rahmani, P., Torkashvand, M., et al. (2012). Comparison of the administration of progesterone versus progesterone and vitamin D in improvement of outcomes in patients with traumatic brain injury: A randomized clinical trial with placebo group. Adv. Biomed. Res. 1, 58. doi:10.4103/2277-9175.100176
Baharvahdat, H., Ganjeifar, B., Etemadrezaie, H., Farajirad, M., Zabihyan, S., and Mowla, A. (2019). Enoxaparin in the treatment of severe traumatic brain injury: A randomized clinical trial. Surg. Neurol. Int. 10, 10. doi:10.4103/sni.sni_112_18
Bai, X. F., and Gao, Y. K. (2018). Recombinant human erythropoietin for treating severe traumatic brain injury. Med. Baltim. 97, e9532. doi:10.1097/MD.0000000000009532
Ban, S. P., Son, Y. J., Yang, H. J., Chung, Y. S., Lee, S. H., and Han, D. H. (2010). Analysis of complications following decompressive craniectomy for traumatic brain injury. J. Korean Neurosurg. Soc. 48, 244–250. doi:10.3340/jkns.2010.48.3.244
Brazinova, A., Rehorcikova, V., Taylor, M. S., Buckova, V., Majdan, M., Psota, M., et al. (2021). Epidemiology of traumatic brain injury in Europe: A living systematic review. J. Neurotrauma 38, 1411–1440. doi:10.1089/neu.2015.4126
Capizzi, A., Woo, J., and Verduzco-Gutierrez, M. (2020). Traumatic brain injury: An overview of epidemiology, pathophysiology, and medical management. Med. Clin. North Am. 104, 213–238. doi:10.1016/j.mcna.2019.11.001
Catalá-López, F., Tobías, A., Cameron, C., Moher, D., and Hutton, B. (2014). Network meta-analysis for comparing treatment effects of multiple interventions: An introduction. Rheumatol. Int. 34, 1489–1496. doi:10.1007/s00296-014-2994-2
Chakroun-Walha, O., Samet, A., Jerbi, M., Nasri, A., Rekik, N., Kanoun, H., et al. (2018). Benefits of the tranexamic acid in head trauma with no extracranial bleeding: A prospective follow-up of 180 patients. Eur. J. Trauma Emerg. Surg. 45, 719–726. doi:10.1007/s00068-018-0974-z
collaborators, C.-t., Shakur, H., Roberts, I., Bautista, R., Caballero, J., Coats, T., et al. (2010). Effects of tranexamic acid on death, vascular occlusive events, and blood transfusion in trauma patients with significant haemorrhage (CRASH-2): A randomised, placebo-controlled trial. Lancet 376, 23–32. doi:10.1016/S0140-6736(10)60835-5
Collaborators, C. T. (2019). Effects of tranexamic acid on death, disability, vascular occlusive events and other morbidities in patients with acute traumatic brain injury (CRASH-3): A randomised, placebo-controlled trial. Lancet (London, Engl. 394, 1713–1723. doi:10.1016/S0140-6736(19)32233-0
Dorsett, C. R., McGuire, J. L., DePasquale, E. A., Gardner, A. E., Floyd, C. L., and McCullumsmith, R. E. (2017). Glutamate neurotransmission in rodent models of traumatic brain injury. J. Neurotrauma 34, 263–272. doi:10.1089/neu.2015.4373
Fakharian, E., Abedzadeh-Kalahroudi, M., and Atoof, F. (2018). Effect of tranexamic acid on prevention of hemorrhagic mass growth in patients with traumatic brain injury. World Neurosurg. 109, e748–e753. doi:10.1016/j.wneu.2017.10.075
Farzanegan, G. R., Derakhshan, N., Khalili, H., Ghaffarpasand, F., and Paydar, S. (2017). Effects of atorvastatin on brain contusion volume and functional outcome of patients with moderate and severe traumatic brain injury; a randomized double-blind placebo-controlled clinical trial. J. Clin. Neurosci. 44, 143–147. doi:10.1016/j.jocn.2017.06.010
Fattahi, A., Koohpayehzadeh, J., Khodadost, M., Fatahi, N., Taheri, M., Azimi, A., et al. (2018). The effects of amantadine on traumatic brain injury outcome: A double-blind, randomized, controlled, clinical trial. Brain Inj. 32, 1050–1055. doi:10.1080/02699052.2018.1476733
Flanagan, S. R. (2015). Invited commentary on "centers for disease control and prevention report to congress: Traumatic brain injury in the United States: Epidemiology and rehabilitation. Arch. Phys. Med. Rehabil. 96, 1753–1755. doi:10.1016/j.apmr.2015.07.001
Gilchrist, J., Thomas, K. E., Xu, L. K., Mcguire, L. C., and Coronado, V. (2011). Nonfatal traumatic brain injuries related to sports and recreation activities among persons aged ≤19 years--United States, 2001-2009. MMWR. Morb. Mortal. Wkly. Rep. 60, 1337–1342.
Hellewell, S. C., Mondello, S., Conquest, A., Shaw, G., Madorsky, I., Deng, J. V., et al. (2017). Erythropoietin does not alter serum profiles of neuronal and axonal biomarkers after traumatic brain injury: Findings from the Australian EPO-TBI clinical trial. Crit. Care Med. 1, 554–561. doi:10.1097/CCM.0000000000002938
Humphreys, I., Wood, R. L., Phillips, C. J., and Macey, S. (2013). The costs of traumatic brain injury: A literature review. Clin. Outcomes Res. 5, 281–287. doi:10.2147/CEOR.S44625
Hyuk, K., and Han, Y. (2018). Relationship between clinical outcomes and superior sagittal sinus to bone flap distance during unilateral decompressive craniectomy in patients with traumatic brain injury: Experience at a single trauma center. Korean Journal of Neurotrauma 14 (2), 99–104. doi:10.13004/kjnt.2018.14.2.99
Jansen, J. P., and Naci, H. (2013). Is network meta-analysis as valid as standard pairwise meta-analysis? It all depends on the distribution of effect modifiers. BMC Med. 11, 159. doi:10.1186/1741-7015-11-159
Jiang, J. Y., Gao, G. Y., Feng, J. F., Mao, Q., Chen, L. G., Yang, X. F., et al. (2019). Traumatic brain injury in China. Lancet. Neurol. 18, 286–295. doi:10.1016/S1474-4422(18)30469-1
Jochems, D., Rein, E. V., Niemeijer, M., Heijl, M. V., Es, M. A. V., Nijboer, T., et al. (2021). Incidence, causes and consequences of moderate and severe traumatic brain injury as determined by Abbreviated Injury Score in The Netherlands. Springer Science and Business Media LLC.
Khalili, H., Ahl, R., Paydar, S., Sjolin, G., Cao, Y., Abdolrahimzadeh Fard, H., et al. (2020). Beta-blocker therapy in severe traumatic brain injury: A prospective randomized controlled trial. World J. Surg. 44, 1844–1853. doi:10.1007/s00268-020-05391-8
Khellaf, A., Khan, D. Z., and Helmy, A. (2019). Recent advances in traumatic brain injury. J. Neurol. 266, 2878–2889. doi:10.1007/s00415-019-09541-4
Lawati, K. A., Sharif, S., Maqbali, S. A., Rimawi, H. A., Petrosoniak, A., Belley-Cote, E. P., et al. (2021). Efficacy and safety of tranexamic acid in acute traumatic brain injury: A systematic review and meta-analysis of randomized-controlled trials. Intensive Care Med. 47, 14–27. doi:10.1007/s00134-020-06279-w
Lee, J., Cho, Y., Choi, K. S., Kim, W., Jang, B. H., Shin, H., et al. (2019). Efficacy and safety of erythropoietin in patients with traumatic brain injury: A systematic review and meta-analysis. Am. J. Emerg. Med. 37, 1101–1107. doi:10.1016/j.ajem.2018.08.072
Li, Z. M., Xiao, Y. L., Zhu, J. X., Geng, F. Y., Guo, C. J., Chong, Z. L., et al. (2016). Recombinant human erythropoietin improves functional recovery in patients with severe traumatic brain injury: A randomized, double blind and controlled clinical trial. Clin. Neurol. Neurosurg. 150, 80–83. doi:10.1016/j.clineuro.2016.09.001
Liu, W. C., Wen, L., Xie, T., Wang, H., Gong, J. B., and Yang, X. F. (2017). Therapeutic effect of erythropoietin in patients with traumatic brain injury: A meta-analysis of randomized controlled trials. J. Neurosurg. 127, 8–15. doi:10.3171/2016.4.JNS152909
Lu, G., Zhu, L., Wang, X., Zhang, H., and Li, Y. (2019). Decompressive craniectomy for patients with traumatic brain injury: A pooled analysis of randomized controlled trials. World Neurosurg. 133, e135–e148. doi:10.1016/j.wneu.2019.08.184
Lu, X. Y., Sun, H., Li, Q. Y., and Lu, P. S. (2016). Progesterone for traumatic brain injury: A meta-analysis review of randomized controlled trials. World Neurosurg. 90, 199–210. doi:10.1016/j.wneu.2016.02.110
Maas, A. I. R., Menon, D. K., Adelson, P. D., Andelic, N., Bell, M. J., Belli, A., et al. In, and traumatic brain injury: Integrated approaches to improve prevention, clinical care, and research. Lancet. Neurol. 16 (2017) 987–1048. doi:10.1016/S1474-4422(17)30371-X
Maas, A., Murray, G., Henney, H., Kassem, N., Knoller, N., Mangelus, M., et al. (2006). Efficacy and safety of dexanabinol in severe traumatic brain injury: Results of a phase III randomised, placebo-controlled, clinical trial. Lancet. Neurol. 5, 38–45. doi:10.1016/S1474-4422(05)70253-2
Marmarou, A., Nichols, J., Burgess, J., Newell, D., Troha, J., Burnham, D., et al. (1999). Effects of the bradykinin antagonist bradycor (deltibant, CP-1027) in severe traumatic brain injury: Results of a multi-center, randomized, placebo-controlled trial. American brain injury consortium study group. J. Neurotrauma 16, 431–444. doi:10.1089/neu.1999.16.431
Moghaddam, O. M., Lahiji, M. N., Hassani, V., and Mozari, S. (2017). Early administration of selenium in patients with acute traumatic brain injury: A randomized double-blinded controlled trial. Indian J. Crit. Care Med. 21, 75–79. doi:10.4103/ijccm.IJCCM_391_16
Mojallal, F., Nikooieh, M., Hajimaghsoudi, M., Baqherabadi, M., Jafari, M., Esmaeili, A., et al. (2020). The effect of intravenous tranexamic acid on preventing the progress of cerebral hemorrhage in patients with brain traumatic injuries compared to placebo: A randomized clinical trial. Med. J. Islam. Repub. Iran. 34, 107. doi:10.34171/mjiri.34.107
Nichol, A., French, C., Little, L., Haddad, S., Presneill, J., Arabi, Y., et al. (2015). Erythropoietin in traumatic brain injury (EPO-TBI): A double-blind randomised controlled trial. Lancet 386, 2499–2506. doi:10.1016/S0140-6736(15)00386-4
Pan, Z. Y., Zhao, Y. H., Huang, W. H., Xiao, Z. Z., and Li, Z. Q. (2019). Effect of progesterone administration on the prognosis of patients with severe traumatic brain injury: A meta-analysis of randomized clinical trials. Drug Des. devel. Ther. 13, 265–273. doi:10.2147/DDDT.S192633
Perel, P., Al-Shahi Salman, R., Kawahara, T., Morris, Z., Prieto-Merino, D., Roberts, I., et al. (2012). CRASH-2 (clinical randomisation of an antifibrinolytic in significant haemorrhage) intracranial bleeding study: The effect of tranexamic acid in traumatic brain injury--a nested randomised, placebo-controlled trial. Health Technol. Assess. 16, 1–54. iii-xii. doi:10.3310/hta16130
Rennie, K., Ribecco-Lutkiewicz, M., Ly, D., Haukenfrers, J., Liu, Q., Nzau, M., et al. (2018). Traumatic brain injury: Classification, models, and markers. Biochem. Cell Biol. 96, 391–406. doi:10.1139/bcb-2016-0160
Robinson, C. P. (2021). Moderate and severe traumatic brain injury. Contin. Minneap. Minn. 27, 1278–1300. doi:10.1212/con.0000000000001036
Rowell, S. E., Meier, E. N., McKnight, B., Kannas, D., May, S., Sheehan, K., et al. (2020). Effect of out-of-hospital tranexamic acid vs placebo on 6-month functional neurologic outcomes in patients with moderate or severe traumatic brain injury. JAMA 324, 961–974. doi:10.1001/jama.2020.8958
Shafiee, S., Zali, A., Shafizad, M., Emami Zeydi, A., Ehteshami, S., Rezaii, F., et al. (2021). The effect of oral simvastatin on the clinical outcome of patients with severe traumatic brain injury: A randomized clinical trial. Ethiop. J. Health Sci. 31, 807–816. doi:10.4314/ejhs.v31i4.15
Shakeri, M., Boustani, M. R., Pak, N., Panahi, F., Salehpour, F., Lotfinia, I., et al. (2013). Effect of progesterone administration on prognosis of patients with diffuse axonal injury due to severe head trauma. Clin. Neurol. Neurosurg. 115, 2019–2022. doi:10.1016/j.clineuro.2013.06.013
Simon, D. W., McGeachy, M. J., Bayir, H., Clark, R. S., Loane, D. J., and Kochanek, P. M. (2017). The far-reaching scope of neuroinflammation after traumatic brain injury. Nat. Rev. Neurol. 13, 171–191. doi:10.1038/nrneurol.2017.13
Skolnick, B. E., Maas, A. I., Narayan, R. K., van der Hoop, R. G., MacAllister, T., Ward, J. D., et al. (2014). A clinical trial of progesterone for severe traumatic brain injury. N. Engl. J. Med. 371, 2467–2476. doi:10.1056/NEJMoa1411090
Skrifvars, M. B., French, C., Bailey, M., Presneill, J., Nichol, A., Little, L., et al. (2018). Cause and timing of death and subgroup differential effects of erythropoietin in the EPO-TBI study. J. Neurotrauma 35, 333–340. doi:10.1089/neu.2017.5135
Soltani, Z., Shahrokhi, N., Karamouzian, S., Khaksari, M., Mofid, B., Nakhaee, N., et al. (2017). Does progesterone improve outcome in diffuse axonal injury? Brain Inj. 31, 16–23. doi:10.1080/02699052.2016.1213421
Sorby-Adams, A. J., Marcoionni, A. M., Dempsey, E. R., Woenig, J. A., and Turner, R. J. (2017). The role of neurogenic inflammation in blood-brain barrier disruption and development of cerebral oedema following acute central nervous system (CNS) injury. Int. J. Mol. Sci. 18, E1788. doi:10.3390/ijms18081788
Sulhan, S., Lyon, K. A., Shapiro, L. A., and Huang, J. H. (2020). Neuroinflammation and blood-brain barrier disruption following traumatic brain injury: Pathophysiology and potential therapeutic targets. J. Neurosci. Res. 98, 19–28. doi:10.1002/jnr.24331
Winkler, E. A., Minter, D., Yue, J. K., and Manley, G. T. (2016). Cerebral edema in traumatic brain injury: Pathophysiology and prospective therapeutic targets. Neurosurg. Clin. N. Am. 27, 473–488. doi:10.1016/j.nec.2016.05.008
Wright, D. W., Kellermann, A. L., Hertzberg, V. S., Clark, P. L., Frankel, M., Goldstein, F. C., et al. (2007). ProTECT: A randomized clinical trial of progesterone for acute traumatic brain injury. Ann. Emerg. Med. 49, 391–402. doi:10.1016/j.annemergmed.2006.07.932
Wright, D. W., Yeatts, S. D., Silbergleit, R., Palesch, Y. Y., Hertzberg, V. S., Frankel, M., et al. (2014). Very early administration of progesterone for acute traumatic brain injury. N. Engl. J. Med. 371, 2457–2466. doi:10.1056/NEJMoa1404304
Xiao, G. M., Jing, W., Wu, Z. H., Wang, W. M., Fang, R., Cheng, J., et al. (2007). [Clinical study on the therapeutic effects and mechanism of progesterone in the treatment for acute severe head injury]. Zhonghua Wai Ke Za Zhi 45, 106–108.
Xiao, G., Wei, J., Yan, W., Wang, W., and Lu, Z. (2008). Improved outcomes from the administration of progesterone for patients with acute severe traumatic brain injury: A randomized controlled trial. Crit. Care 12 (2), R61. doi:10.1186/cc6887
Yurkewicz, L., Weaver, J., Bullock, M. R., and Marshall, L. F. (2005). The effect of the selective NMDA receptor antagonist traxoprodil in the treatment of traumatic brain injury. J. Neurotrauma 22, 1428–1443. doi:10.1089/neu.2005.22.1428
Yutthakasemsunt, S., Kittiwatanagul, W., Piyavechvirat, P., Thinkamrop, B., Phuenpathom, N., and Lumbiganon, P. (2013). Tranexamic acid for patients with traumatic brain injury: A randomized, double-blinded, placebo-controlled trial. BMC Emerg. Med. 1313 (11-22), 201–207. doi:10.1186/1471-227X-13-20
Zafonte, R. D., Bagiella, E., Ansel, B. M., Novack, T. A., Friedewald, W. T., Hesdorffer, D. C., et al. (2012). Effect of citicoline on functional and cognitive status among patients with traumatic brain injury-citicoline brain injury treatment trial (COBRIT). JAMA 308, 1993–2000. doi:10.1001/jama.2012.13256
Zhang, J., Wang, H., Li, Y., Zhang, H., and Dong, L. (2020). The efficacy of progesterone 1 mg kg(-1) every 12 hours over 5 days in moderate-to-severe traumatic brain injury: A meta-analysis of randomized controlled trials. Clin. Neurol. Neurosurg. 198, 106131. doi:10.1016/j.clineuro.2020.106131
Keywords: drug therapy, favorable outcome indicators, mortality, network metaanalysis, traumatic brain injury
Citation: Li M, Huo X, Wang Y, Li W, Xiao L, Jiang Z, Han Q, Su D, Chen T and Xia H (2022) Effect of drug therapy on nerve repair of moderate-severe traumatic brain injury: A network meta-analysis. Front. Pharmacol. 13:1021653. doi: 10.3389/fphar.2022.1021653
Received: 17 August 2022; Accepted: 19 October 2022;
Published: 02 November 2022.
Edited by:
Brijesh Sathian, Hamad Medical Corporation, QatarReviewed by:
Eileen Marie Foecking, Loyola University Chicago, United StatesCopyright © 2022 Li, Huo, Wang, Li, Xiao, Jiang, Han, Su, Chen and Xia. This is an open-access article distributed under the terms of the Creative Commons Attribution License (CC BY). The use, distribution or reproduction in other forums is permitted, provided the original author(s) and the copyright owner(s) are credited and that the original publication in this journal is cited, in accordance with accepted academic practice. No use, distribution or reproduction is permitted which does not comply with these terms.
*Correspondence: Tong Chen, Y3QuMTk3M0AxNjMuY29t; Hechun Xia, eGhlY2h1bkBueWZ5LmNvbS5jbg==
Disclaimer: All claims expressed in this article are solely those of the authors and do not necessarily represent those of their affiliated organizations, or those of the publisher, the editors and the reviewers. Any product that may be evaluated in this article or claim that may be made by its manufacturer is not guaranteed or endorsed by the publisher.
Research integrity at Frontiers
Learn more about the work of our research integrity team to safeguard the quality of each article we publish.