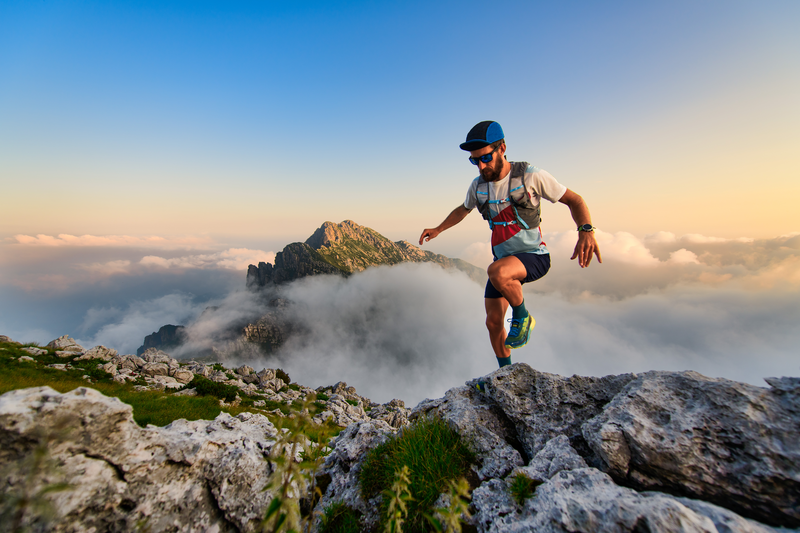
94% of researchers rate our articles as excellent or good
Learn more about the work of our research integrity team to safeguard the quality of each article we publish.
Find out more
REVIEW article
Front. Pharmacol. , 04 January 2023
Sec. Translational Pharmacology
Volume 13 - 2022 | https://doi.org/10.3389/fphar.2022.1018904
This clinical review presents what is known about the antiviral features of humic substances (HS) to the benefit of the clinical healthcare provider using available data in humeomics, the study of the soil humeome. It provides the reader with a working framework of historical studies and includes clinically relevant data with the goal of providing a broad appreciation of the antiviral potential of humic substances while also preparing for a translational leap into the clinical application of humic acid.
Humic substances include a variety of chromogenic, or pigmented, organic molecules that are primarily distributed in soils, rivers, oceans, and iterations of coal (Jung et al., 2021). They are also found in small quantities in Chaga (Inonotus obliquus), though this iteration contains almost no nitrogen in contrast to primary reservoirs of humic substances (Shashkina et al., 2006).
The soil humeome has the greatest abundance of humic substances and is fundamental to plant growth, carbon storage, and the management of environmental contaminants (Orsi, 2014). It, as well as other sources of humic substances, originated in the vast fauna which carpeted the biosphere 50–280 million years ago, between the Cenozoic and Paleozoic eras. Though the principal molecules of the soil humeome, namely humic acid, fulvic acid, and humin, are highly conserved across the environment, it is clinically valuable to regard humic acid as a molecular genus rather than a well-defined molecule with a static molecular formula and three-dimensional conformation. Differences in local fauna, micro-environments, and a time factor yield “variations on a theme” at the molecular level (Stevenson, 1994), which has a bearing on chemistry and ultimately clinical potential. Despite their common molecular superstructure, the highly iterative origin of the humic acids contrasts with conventional standards of pharmacology and our expectations in clinical practice (Murbach et al., 2020).
The conceptual model of humic acid has evolved dramatically over the past 20 years in tandem with new technologies that assay the soil humeome. The classical model of humic acid, that of a molecular polymer with an organized and predictable structure, has been superseded by a complex model based on a supramolecular aggregate of smaller molecules derived from the biotic and abiotic degradation of dead plant matter (Piccolo et al., 2018). Though the assembly of the supramolecular aggregate depends on an array of hydrophobic interactions and hydrogen bonds across multiple relatively low molecular mass sub-components, the dimensional behavior of these biomolecular components create unique molecular micro-environments that contribute to humic acid chemistry and the nuance between humic acid molecules. That these biomolecules are also indivisible from the larger humic molecule amends the functional paradigm of what it means to be a humic acid (Sutton and Sposito, 2005). It also makes it that much more difficult to conceptualize the three-dimensional conformation of humic acid given these added layers of complexity (Ghabbour et al., 2001). Regardless of these advancements, the building blocks of humic acid remain well established (Figure 1). The influence of carboxylic, alcoholic, and sulfhydryl groups to the supramolecule’s chemistry, in addition to fatty acids, amino acids and polypeptides, differentiate the functional potential of each humic acid iteration (Sutton and Sposito, 2005; Al-Faiyz, 2013; Orsi, 2014; Bondareva and Kudryasheva, 2021).
FIGURE 1. Essential building blocks of humic acid. Created with Biorender.com.
This complex backstory notwithstanding, the clinical value of the soil humeome was first identified by indigenous cultures living within the Himalayan regions of Bhutan, India, Nepal and Pakistan, in the form of shilajit, or mineral pitch, with a minor contribution emanating from Tibet and China (Meena et al., 2010). Shilajit, or a blend of metallo-humates, including humic and fulvic acids, low and medium molecular weight non-humic organic compounds, and medium and high molecular weight dibenzo-alpha-pyrones-chromoproteins, is still used today as an Ayurvedic medicinal food to mitigate a variety of physical ailments (Ghosal, 2006). Nearly 90-years of scientific research beginning in the 1930s has associated these molecules with anti-inflammatory (Junek et al., 2009), anti-oxidant (Zykova et al., 2018; Bondareva and Kudryasheva, 2021; Kulikova and Perminova, 2021), antiviral (See Table 1), anti-cancer, mycotoxin binding (De Mil et al., 2015) and gut-promoting properties (via optimization of the microbiota), though often without distinguishing between the relative contribution of humic acid versus fulvic acid to the net clinical effect (Vetvicka et al., 2010; Kuhnert, 2011; Vetvicka et al., 2013; Winkler and Ghosh, 2018; Lavrik and Ilyitcheva, 2019).
The potential of humic acid as an anti-viral is intrinsic to humic substances as a functional molecular class. These negatively charged polyanionic supramolecules rely on their net negative charge to bind positively charged viral glycoproteins, which ultimately inhibits viral fusion with susceptible cell membrane receptors (Figure 2) via a competitive inhibition mechanism. Numerous in vitro studies have established the antiviral capacity of humic acid molecules to influence Human Immunodeficiency Virus Types 1 and 2 (HIV-1 and HIV-2), Herpes Simplex Virus Types 1 and 2 (HSV-1 and HSV-2), Epstein Barr Virus (EBV), Varicella Zoster Virus (VZV), Influenza A, Influenza B, Respiratory Syncytial Virus (RSV), human Cytomegalovirus (hCMV), Severe Acute Respiratory Syndrome Coronavirus 2 (SARS-CoV-2), and other virus dynamics through an extracellular mechanism of action. Other studies have illuminated an intracellular antiviral contribution, particularly with HBV, HIV and influenza A (Lu et al., 2002; Pant et al., 2016). Other theoretical mechanisms to antiviral activity, such as cytotoxicity, have been reasonably excluded. Within the humic substance family, humic acid exhibits the greatest antiviral potential relative to its smaller sister molecule, fulvic acid, and shilajit. While multiple studies further conclude that humic substances exhibit no toxic features in vitro, others contend that pre-clinical studies are warranted to transition historical research into clinical practice, particularly in consideration of the potential downsides of using humic substances, such as poor bioavailability, heavy metal toxicity, inducement of hypercoagulability, and chelation of essential minerals.
FIGURE 2. Binding mechanism of humic acid with spike proteins. Created with Biorender.com.
The SARS-Cov-2 pandemic of 2019 illuminated the veracity of infectious disease, and viral infection in particular, to induce sub-clinical and clinical disease, sabotage patient recovery with a constellation of novel and residual symptoms, disrupt national economies, and impair local, national and geopolitical discourse. The inescapable requirement to reconcile public health needs against a granular, patient-based individual needs analysis requires a diversified toolbox that is considerate of both worlds. The ever-present danger of a SARS-CoV-2 mutation that eludes our antiviral repertoire is unfortunately real, particularly given its rate of antigenic shift and known parallels of drug-resistant viral infections, including influenza vs. adamantane derivatives (Dong et al., 2015), the herpes viradae vs. acyclovir (Pottage and Kessler, 1995; Bacon et al., 2003), and retroviruses vs. azithromycin (Jeeninga et al., 2001). The need to develop supplements and/or adjuncts to vaccines that are indifferent to viral mutation, exhibit prophylactic potential, reduce viral shedding and that modulate the depth and duration of clinical disease bear relevance now and in the future (Neuzil, 2021).
It has long been appreciated that humic substances exhibit antiviral activity, which is based on their molecular feature as carboxylated polyanions with a net negative charge that bind to positively charged viral glycoproteins (Helbig et al., 1997; Klöcking et al., 2002; Jooné et al., 2003). Humic acid is the primary molecule in this molecular family that exhibits antiviral activity, in contrast to other molecules in the HS fraction, such as fulvic acid and shilajit, a related natural product. This being said, the origin of humic acid is also relevant to its antiviral potential. Humic acid sourced from coal exhibits greater antiviral activity than samples from peloid and peat, as well as synthetic iterations of the molecule, such as caffeic acid or chlorogenic acid, versus select viral challenges (Zhernov et al., 2021). Zhernov et alia (2021) further identified the highest antiviral activity in the most hydrophobic and aromatic enriched humic acids. Zhernov et alia’s prior research in 2018 further identified a direct relationship between antiviral activity with the preponderance of CHO (carbohydrate) molecules in humic acid as well as the molecule’s lipophilicty, in addition to an inverse relationship with its density of carboxylic groups and total acidity (Zhernov, 2018). However, it seems that it was Meerbach, as early as 2001, who linked humic acid’s antiviral activity with the presence of carboxylic acid groups (Meerbach et al., 2001).
Numerous studies have established the activity of natural and synthetic humic substances as antivirals in vitro (Table 1). With the exception of Klöcking’s work with Coxsackie virus A9, ECHO-Virus Type 6 and Adenovirus Type 2 in the 1970s (Klöcking and Sprössig, 1972; Klöcking and Sprössig, 1975), these studies largely explored the binding affinity of negatively charged polyanionic substances, or humic substances, with viruses that include positively charged glycoproteins in their viral envelope. Though other studies also reference the binding potential of carboxylated polyanions with non-enveloped viruses, such as Human Papilloma Virus (HPV), the data is limited (Buck et al., 2006) and the relative efficacy still favors enveloped viruses in the absence of molecular enhancements. Others have identified clinical crossover potential of the carboxylated polyanion, poly (styrene-4-sulfonate) against the bacteria Chlamydia trachomatis and Neisseria gonorrhoeae (Anderson et al., 2000). This being said, relative to other antiviral biomaterials with comparable molecular features, the Selectivity Index (SI), or antiviral activity of natural and synthetic carboxylated polyanions, significantly prefers enveloped viruses over non-enveloped viruses (Terasawa et al., 2020).
In 2002, a contract lab through the National Institutes of Health (NIH) completed a comparative study that examined the relative antiviral activity of humic acid in vitro (NIH, 2002). The data from the original manuscript has been reorganized and is presented in Table 3 through six below.
The study performed through the NIH remains a benchmark in the landscape of in vitro research on humic substances. Though studies performed since 2002 have taken advantage of technological developments to provide more precise comparative data regarding the antiviral potential of humic substances, the NIH work is notable for its breadth of study, the quality of its data, and the lessons learned based on its conclusions. Subsequent work by others has verified specific conclusions of this study (Meerbach et al., 2001; Cagno et al., 2015; Zhernov et al., 2017; Zhernov, 2018; Zhernov et al., 2021).
For much of its report, the NIH study used IC50 and IC90 endpoints to quantify the antiviral efficacy of humic acid versus a reference antiviral compound in vitro. Table 2A and Table 2B itemize terminology utilized in the NIH report and other like-minded studies which warrant a definition or conceptual framework.
The results presented in Tables 3 and 4 established several key benchmarks relative to humic acid as an antiviral in vitro:
• Relative to the reference compound (acyclovir in Table 3 and ribavirin in Table 4), humic acid demonstrated IC50 and IC90 results consistent with antiviral activity.
• The IC50 and IC90 data generated by the studies illuminated the adaptability of the humic acid molecule as a non-specific antiviral.
• The antiviral activity of humic acid was greater for the influenza study (Table 4) than for the herpesviridae study (Table 3) in the aggregate. Within the herpesviridae study, the relative difference between the IC50 and IC90 findings for HSV-1 and HSV 2 versus VZV and EBV was notable, though meaningful IC50 and IC90 data was still generated for VZV and EBV.
TABLE 3. Effective inhibitory concentration at 50% (IC50) and 90% (IC90) of humic acid (HA) and acyclovir reference compound with herpesviridae (NIH, 2002).
TABLE 4. Effective inhibitory concentration at 50% (IC50) and 90% (IC90) of humic acid (HA) and ribavirin reference compound with influenza virus, Types A and B, in MDCK cells (NIH, 2002).
Contrary to the data generated for the Herpesviridae study summarized in Table 3, the influenza virus studies recruited a trifecta of assays to add credence to the dataset (Table 2B). Initial studies to evaluate the ability of natural humates to inhibit the influenza virus’ cytopathic effect (CPE) against cells in vitro were followed by neutral red (NR) studies that sought to validate the CPE data by quantifying the intensity of neutral red absorbed by surviving cells. In the final study, natural (and synthetic) humates that were regarded as active by CPE inhibition and confirmed by the NR assay were retested using the CPE method. These samples were then assessed for a reduction of virus yield (VY) relative to a positive control by assaying viral titers in the presence of susceptible cells. Development of CPE in the susceptible cell population was an indication of the presence of infectious virus and an ineffective natural humate.
The antiviral mechanism of humic acid is divided into a dominant extracellular component and a secondary cadre of intracellular mechanisms. In the extracellular framework, negatively charged carboxylated polyanions bind to positively charged viral envelop glycoproteins. Using HIV as a model, humic acid interacts with the positively charged V3 loop of the HIV-1 glycoprotein (gp120) or the glycoprotein 41 (gp41) complex (Zhernov et al., 2017). With SARS-CoV-2, it is theorized that humic acid binds to the positively charged M-glycoprotein of the viral envelop.
Historical studies aimed at deducing the antiviral mechanism of humic substances used assays for cytotoxicity in vitro to provide back door insight regarding humic acid’s mechanism(s) of action. In the absence of cytotoxicity, other rationales to explain the antiviral findings in vitro would advance to the forefront. Using a combination of toxicity and cell proliferation assays, the NIH’s 2002 study established that humic acid was not cytotoxic at levels at least as high as 100mcg/mL across a variety of cell lines (Table 5). Cagno et alia’s 2015 time-of-addition studies to demonstrate shilajit’s dose-dependent inhibitory activity against HSV-1, HSV-2, hCMV and RSV yielded that shilajit had no influence on cell viability at concentrations as high as 1500 mcg/mL (Cagno et al., 2015). This conclusion further narrowed the antiviral mechanism to something other than cytotoxicity. In 2017, Zhernov et alia similarly concluded that all humic pagination exhibited low cytotoxicity; however, their study design did not reach the CC50 for the humic polyanion studied. As a result, the CC50 for humic acid was estimated based on the largest concentration tested as > 200 mcg/mL (Zhernov et al., 2017). Zhernov et alia’s subsequent finding in 2021 that mitochondrial activity of cells in vitro remained greater than 80% in the presence of humic acid and shilajit samples at a concentration of 1000 mg/L reiterated his prior finding as well as the data published in the NIH study and provides further proof of concept that the mechanism driving the antiviral activity of humic acid and shilajit does not rely on cytotoxicity (NIH, 2002; Zhernov et al., 2021).
TABLE 5. Cytotoxicity assays: Humic acid with indicated cell lines (NIH, 2002).
Ultimately, the 2002 NIH study established the primary mechanism of action of humic polyanions using time-of-addition methodology (Table 6). Studies performed by Meerbach et al. (2001); Cagno et al. (2015); Zhernov et al. (2017), among others, yielded similar findings. In the aggregate, time-of-addition studies revealed that commingling viral particles with humic substances before adding cells to the mixture prevented viral fusion to cell membrane receptors; however, pre-treating cells with humic substances failed to influence the capacity of viral particles to adsorb to their target cell membrane receptors. Stated another way, (1) cells treated with humic substances before and after viral infection were found to be susceptible to viral adsorption; however, (2) cells exposed to humic substances at the time of infection do not experience the binding of viral particles to their cell surface membrane receptors, though viral entry is not otherwise inhibited; and (3) viral particles incubated with humic substances in advance of infection are not infectious due to viral inactivation and interference with the mechanics of viral attachment.
TABLE 6. In the Time of Addition study presented, humic acid or the reference compound, ribavirin, is added to virus-infected cells at discrete time points. Time point “0” represents prior to infection. The most effective antiviral effect is observed when cells were pretreated with humic acid. Though there was a decrease in the antiviral effect for humic acid between 1 h and 24 h relative to time “0,” ribavirin lost all activity by 24 h whereas humic acid remained active. Since the time from viral adsorption to the shedding of new influenza virus in vitro can begin after 6 h (World Health Organization, 2022), the IC50 data for humic acid at 24 h suggests that humic acid also limits new virus adsorption after a cycle of viral shedding. Given that cells in vitro were continuously exposed to humic acid material, this reasoned explanation is most likely.
By definition, humic acid is an opportunistic anti-viral molecule that inhibits viral adsorption to cell membrane receptors (1) during primary infection and (2) downstream of the replication cycle during viral shedding. Though the primary nexus for humic acid as an antiviral is extracellular, discreet observations have been reported for multiple intracellular activities. In studies evaluating Hepatits B virus, Pant et al. (2016) observed that humic acid (1) induces apoptosis of hepatic cancer cells via upregulation of caspase; (2) inhibits HBV-induced cell proliferation and autophagy via inhibition of HBx protein expression; (3) inhibits HBV DNA and HBsAg, and (4) inhibits HBV-induced autophagosome formation (Pant et al., 2016). The influence on cell cycle activities identified by Pant contrasts with mechanistic studies completed by Zhernov (2018) that further elaborated upon the influence of humic acid on viral replication. More specifically, Zhernov discovered that humic acid inhibits reverse transcriptase in an HIV-1 replication model, but not integrase. In 2002,Lu et alia, reported on the capacity of synthetic humate to disrupt viral RNA polymerase activity.
In 2000, Lüscher-Mattli noted that in their capacity as antiviral agents, anionic polymers such as humic acid inhibit syncytium formation between HIV-infected and normal CD4 T lymphocytes, which mirrored Meerbach’s parallel observation for a selection of 12 synthetic phenolic polymers (polyhydroxycarboxylates) (Meerbach et al., 2001; Lüscher-Mattli, 2000). Given findings that SARS-CoV-2 drives the formation of respiratory syncytia in those with severe respiratory disease (Lin et al., 2021), the potential of humic acid to limit the evolution of respiratory syncytia in vivo may be the subject of further study.
In unrelated assessments, Smirnova (2012) and Krezel (2016) conclude that humic acid behaves as an ionophore to facilitate the intracellular transport of zinc ions, which te Velthuis et al. (2010) showed have noteworthy antiviral effects against Sudden Acute Respiratory Syndrome (SARS), a clinical disease which some individuals developed during the 2003 coronavirus outbreak (Smirnova et al., 2012; Krężel and Maret, 2016; te Velthuis et al., 2010). Contemporary work regarding the antiviral influence of zinc on SARS-CoV-2 is substantial (Marreiro et al., 2021). Less well known is humic acid’s ability to stabilize zinc as well as selenium ions in chelate form, which enhances each ion’s bioavailability and anti-viral effect (Constantinescu-Aruxandei et al., 2018). Humic substances indirect protection of host systems from viral infection via the suppression of tumor necrosis factor alpha (TNF-α), prostaglandin E2 (PGE2) and cyclooxygenase 2 (COX-2) expression in human monocyte culture is also potentially clinically relevant (Hafez et al., 2020); as is its direct reduction of oxidative stress by complexing with intermediate free radicals and activation of the immune system via the promotion of IL-2 secretion (Vetvicka et al., 2013).
Humic substances have numerous potential clinical applications; however, the translation of what is known in vitro into clinical practice has been slow to mature. In the wake of SARS-CoV-2, the need for adjuvant antiviral therapies that are oral, deployable, and indifferent to viral antigenic shift is relevant. Translating the in vitro antiviral characteristics of humic acid into clinical practice would add to the clinician’s toolbox and the dialog within public health circles.
Pre-clinical studies have established two inflection points with humic substances. The first is that humic acid’s antiviral potential supersedes any contribution from fulvic acid, which in turn dwarfs the antiviral activity of shilajit. The second is that the translation of what is known regarding humic acid’s antiviral portfolio from pre-clinical studies to clinical application has been marred by concerns about efficacy, safety and tolerability.
Regardless of the working group, pre-clinical studies that assessed the antiviral potential of humic acid relied on a pure extract of humic polyanions that is not generally available. In the United States, humic products historically available to the consumer are 30% humic acid, with limited exception. To rival the antiviral efficacy of what has been measured in vitro, humic acid needs to be isolated from its sister molecule, fulvic acid. In the absence of this purity, fulvic acid competitively inhibits the binding sites of the humic acid polyanion, rendering it less effective as an antiviral (Figure 3).
FIGURE 3. Humic acid binds to viral spike protein receptor binding domains (RBD) and inhibits viral fusion with target cell membrane receptors. The hydrophilic properties of the humic acid molecule attract water to form a hydrogel which encapsulates spike protein RBD and suspends the viral lifecycle. In the presence of fulvic acid, humic acid’s potential to bind spike protein RBDs is impaired, which is the molecule’s primary mechanism of action. Created with Biorender.com.
Subsequent to this clinical review, clinical experience with a purified and further enhanced iteration of humic acid that has been used to mitigate SARS-CoV-2 infection will be presented in tandem with data regarding significant TNF-α suppression, CD4+ cell population augmentation, and outcome enhancements in a clinical model of influenza infection. Prior concerns that humic acid has low bioavailability and a short plasma half-life will be reconciled against favorable clinical outcomes. In addition, an argument in favor of using humic acid as a prophylactic antiviral will be postulated given the opportunistic antiviral mechanics of the humic acid molecule, with guidance from Zhernov et alia's 2017 observation that humic acid loses 50% of its efficacy relative to fusion inhibition 7–8 h after infection (which mirrors the findings for the anti-HIV medication, AZT, at 7.7 +/− 0.2 h).
The clinical data to be presented further intends to balance clinical experience with humic acid’s potential disadvantages as a source of heavy metal toxicity, mineral chelation, and pro-coagulant potential. The data yields that individuals using 500 mg of a purified and further enhanced humic acid iteration for a minimum of 6 months exhibit no abnormalities of serum lead levels or clinically abnormal levels of calcium, iron, magnesium or zinc. Further, regardless of humic acid’s potential influence on the coagulation cascade, specifically Factors IIa, VIIa and Xa via a serum protein-humic substance aggregate, unremarkable data on protime (PT) and partial thromboplastin time (PTT) will also be presented and contrasted with expectations based on thromboelastography studies (Klöcking et al., 2013; Hafez et al., 2020).
Humic substances, including humic acid, have been the subject of scientific inquiry since the early 1930s (Erdtman, 1933; Waksman, 1936). As a molecular class that has potential clinical relevance, they exhibit antiviral, anti-inflammatory, anti-oxidant, anti-cancer and gut promoting properties, but to date our understanding of this cadre of molecules has been limited by definitions, technology and clinical inquiry. The emergence of new technologies in the early 2000s reframed the concept of humic acid as a supramolecule rather than a molecular polymer. Numerous in vitro studies have chronicled the broad-spectrum antiviral capacity of humic substances against enveloped RNA and DNA viruses while also acknowledging their relative indifference to viral antigenic shift, among other features. These same studies established that humic substances, or polyanionic carboxylates, inhibit viral fusion to target cell membrane receptors as their primary mode of action, though other intracellular and broader indirect influence on the immune system and host viral response have also been reported. This being said, the translation of this body of in vitro research into clinical practice has been underwhelming based on concerns about bioavailability, a short plasma half-life and side effects. Future presentations will share insight regarding the successful deployment of humic acid as an antiviral in clinical practice and the basis for the pivot from pre-clinical studies into clinical practice.
DS researched, compiled and wrote the review article. He also created all figures in the article.
The author would like to thank Sharon Hausman-Cohen MD for her review of this manuscript.
DS is the CEO of Advanced Humeomics (R) and does have an ownership interest.
All claims expressed in this article are solely those of the authors and do not necessarily represent those of their affiliated organizations, or those of the publisher, the editors and the reviewers. Any product that may be evaluated in this article, or claim that may be made by its manufacturer, is not guaranteed or endorsed by the publisher.
Al-Faiyz, Y. S. S. (2013). CPMAS 13C NMR characterization of humic acids from composted agricultural Saudi waste. Arabian J. Chem. 1, S839–S853. doi:10.1016/j.arabjc.2012.12.018
American Society for Microbiology, (2007). Cytopathic effects of viruses protocols. Available at: https://asm.org/Protocols/Cytopathic-Effects-of-Viruses-Protocols (Accessed June 12, 2022).
Anderson, R. A., Feathergill, K., Diao, X., Cooper, M., Kirkpatrick, R., Spear, P., et al. (2000). Evaluation of poly(styrene-4-sulfonate) as a preventive agent for conception and sexually transmitted diseases. J. Androl. 21 (6), 862–875. doi:10.1002/j.1939-4640.2000.tb03417.x
Bacon, T. H., Levin, M. J., Leary, J. J., Sarisky, R. T., and Sutton, D. (2003). Herpes simplex virus resistance to acyclovir and penciclovir after two decades of antiviral therapy. Clin. Microbiol. Rev. 16 (1), 114–128. doi:10.1128/CMR.16.1.114-128.2003
Bondareva, L., and Kudryasheva, N. (2021). Direct and indirect detoxification effects of humic substances. Agronomy 11 (2), 198. doi:10.3390/agronomy11020198
Botes, M. E., Dekker, J., and van Rensburg, C. (2002). Phase I trial with oral oxihumate in HIV-infected patients. Drug Dev. Res. 57, 34–39. doi:10.1002/ddr.10117
Bruccoleri, A. G. (2013). Positional adaptability in the design of mutation-resistant nonnucleoside HIV-1 reverse transcriptase inhibitors: A supramolecular perspective. AIDS Res. Hum. Retroviruses 29 (1), 4–12. doi:10.1089/AID.2012.0141
Buck, C. B., Thompson, C. D., Roberts, J. N., Müller, M., Lowy, D. R., and Schiller, J. T. (2006). Carrageenan is a potent inhibitor of papillomavirus infection. PLoS Pathog. 2 (7), e69. doi:10.1371/journal.ppat.0020069
Cagno, V., Donalisio, M., Civra, A., Cagliero, C., Rubiolo, P., and Lembo, D. (2015). In vitro evaluation of the antiviral properties of Shilajit and investigation of its mechanisms of action. J. Ethnopharmacol. 166, 129–134. doi:10.1016/j.jep.2015.03.019
Constantinescu-Aruxandei, D., Frîncu, R., Capră, L., and Oancea, F. (2018). Selenium analysis and speciation in dietary supplements based on next-generation selenium ingredients. Nutrients 10 (10), 1466. doi:10.3390/nu10101466
Creative Diagnostics (2022). Virus yield reduction assay. Available at: https://antiviral.creative-diagnostics.com/virus-yield-reduction-assays.html (Accessed June 12, 2022).
CytoSmart, (2020). in Examples and readouts. Available at: https://cytosmart.com/resources/virus-induced-cytopathic-effect (Accessed May 4, 2022).Virus-induced cytopathic effect (CPE)
De Mil, T., Devreese, M., De Baere, S., Van Ranst, E., Eeckhout, M., De Backer, P., et al. (2015). Characterization of 27 mycotoxin binders and the relation with in vitro zearalenone adsorption at a single concentration. Toxins 7 (1), 21–33. doi:10.3390/toxins7010021
Dong, G., Peng, C., Luo, J., Wang, C., Han, L., Wu, B., et al. (2015). Adamantane-resistant influenza a viruses in the world (1902-2013): Frequency and distribution of M2 gene mutations. PloS One 10 (3), e0119115. doi:10.1371/journal.pone.0119115
Erdtman, H. G. H. (1933). Studies on the formation of complex oxidation and condensation products of phenols. A contribution to the investigation of the origin and nature of humic acid. Part I.—studies of the reactivity of simple monocyclic quinones. Proc. R. Soc. Lond. Ser. A Contain. Pap. A Math. Phys. Character 143, 177–191.
Ghabbour, E. A., Davies, G., Bruccoleri, A. G., Sorenson, B. T., and Langford, C. G. (2001). Humic substances: Structures, models and functions. United Kingdom: Royal Society of Chemistry.
Goebel, S., Snyder, B., Sellati, T., Saeed, M., Ptak, R., Murray, M., et al. (2016). A sensitive virus yield assay for evaluation of Antivirals against Zika Virus. J. Virological Methods 238, 13–20. doi:10.1016/j.jviromet.2016.09.015
Hafez, M., Popov, A., Zelenkov, V., Teplyakova, T., and Rashad, M. (2020). Humic substances as an environmental-friendly organic wastes potentially help as natural anti-virus to inhibit COVID-19. Sci. Arch. 1, 53–60. doi:10.47587/SA.2020.1202
Hajdrik, P., Pályi, B., Kis, Z., Kovács, N., Veres, D. S., Szigeti, K., et al. (2022). In vitro determination of inhibitory effects of humic substances complexing Zn and Se on SARS-CoV-2 virus replication. Foods 11 (5), 694. doi:10.3390/foods11050694
Helbig, B., Klöcking, R., and Wutzler, P. (1997). Anti-herpes simplex virus Type 1 activity of humic acid-like polymers and their O-diphenolic starting compounds. Antivir. Chem. Chemother. 8 (3), 265–273. doi:10.1177/095632029700800310
Jeeninga, R. E., Keulen, W., Boucher, C., Sanders, R. W., and Berkhout, B. (2001). Evolution of AZT resistance in HIV-1: The 41-70 intermediate that is not observed in vivo has a replication defect. Virology 283 (2), 294–305. doi:10.1006/viro.2001.0888
Jooné, G. K., Dekker, J., and van Rensburg, C. E. (2003). Investigation of the immunostimulatory properties of oxihumate. Zeitschrift fur Naturforschung. C, J. Biosci. 58 (3-4), 263–267. doi:10.1515/znc-2003-3-421
Junek, R., Morrow, R., Schoenherr, J. I., Schubert, R., Kallmeyer, R., Phull, S., et al. (2009). Bimodal effect of humic acids on the LPS-induced TNF-alpha release from differentiated U937 cells. Phytomedicine Int. J. Phytotherapy Phytopharm. 16 (5), 470–476. doi:10.1016/j.phymed.2008.10.003
Jung, H., Kwon, S., Kim, J. H., and Jeon, J. R. (2021). Which traits of humic substances are investigated to improve their agronomical value? Molecules 26 (3), 760. doi:10.3390/molecules26030760
Klöcking, H. P., and Klöcking, R. (2013). “Application of humic substances in medicine: Basic studies to assess pro- and anticoagulant properties of humic acids,” in Functions of natural organic matter in changing environment. Editors J. Xu, J. Wu, and Y. He (Dordrecht: Springer). doi:10.1007/978-94-007-5634-2_205
Klöcking, R., Helbig, B., Schötz, G., Schacke, M., and Wutzler, P. (2002). Anti-HSV-1 activity of synthetic humic acid-like polymers derived from p-diphenolic starting compounds. Antivir. Chem. Chemother. 13 (4), 241–249. doi:10.1177/095632020201300405
Klöcking, R., and Sprössig, M. (1972). Antiviral properties of humic acids. Experientia 28, 607–608. doi:10.1007/BF01931906
Klöcking, R., and Sprössig, M. (1975). Wirkung von Ammoniumhumat auf einige Virus-Zell-Systeme. Z. Allg. Mikrobiol. 15, 25–30. doi:10.1002/jobm.19750150105
Kornilaeva, G. V., Siniavin, A. E., Schultz, A., Germann, A., Moog, C., von Briesen, H., et al. (2019). The differential anti-HIV effect of a new humic substance-derived preparation in diverse cells of the immune system. Acta Naturae 11 (2), 68–76. doi:10.32607/20758251-2019-11-2-68-76
Krężel, A., and Maret, W. (2016). The biological inorganic chemistry of zinc ions. Archives Biochem. Biophysics 611, 3–19. doi:10.1016/j.abb.2016.04.010
Kuhnert, M. (2011). Effects of humic acid on animals and humans: An overview of literature and a review of current research. Arch. E-p. Veterinarmed 1982 (36), 169–177.
Kulikova, N. A., and Perminova, I. V. (2021). Interactions between humic substances and microorganisms and their implications for nature-like bioremediation technologies. Molecules 26 (9), 2706. doi:10.3390/molecules26092706
Lavrik, N. L., and Ilyitcheva, T. N. (2019) “Study of the influence of humic acid macromolecules on the structure of erythrocytes of some animals by the method of absorption”. In KhalidM. A. A (Ed.), Biophysical chemistry - advance applications. IntechOpen, London. doi:10.5772/intechopen.85321
Lin, L., Li, Q., Wang, Y., and Shi, Y. (2021). Syncytia formation during SARS-CoV-2 lung infection: A disastrous unity to eliminate lymphocytes. Cell Death Differ. 28 (6), 2019–2021. doi:10.1038/s41418-021-00795-y
Lu, F. J., Tseng, S. N., Li, M. L., and Shih, S. R. (2002). In vitro anti-influenza virus activity of synthetic humate analogues derived from protocatechuic acid. Archives Virology 147 (2), 273–284. doi:10.1007/s705-002-8319-5
Lüscher-Mattli, M. (2000). Polyanions-a lost chance in the fight against HIV and other virus diseases? Antivir. Chem. Chemother. 11 (4), 249–259. doi:10.1177/095632020001100401
Marreiro, D. D., Cruz, K. J., Oliveira, A. R., Morais, J. B., Freitas, B. D., Melo, S. R., et al. (2021). Antiviral and immunological activity of zinc and possible role in COVID-19. Br. J. Nutr. 1, 1172–1179. doi:10.1017/S0007114521002099
Meena, H., Pandey, H. K., Arya, M. C., and Shilajit, A. Z. (2010). Shilajit: A panacea for high-altitude problems. Int. J. Ayurveda Res. 1 (1), 37–40. doi:10.4103/0974-7788.59942
Meerbach, A., Neyts, J., Balzarini, J., Helbig, B., De Clercq, E., and Wutzler, P. (2001). In vitro activity of polyhydroxycarboxylates against herpesviruses and HIV. Antivir. Chem. Chemother. 12 (6), 337–345. doi:10.1177/095632020101200603
Murbach, T. S., Glávits, R., Endres, J. R., Clewell, A. E., Hirka, G., Vértesi, A., et al. (2020). A toxicological evaluation of a fulvic and humic acids preparation. Toxicol. Rep. 7, 1242–1254. doi:10.1016/j.toxrep.2020.08.030
Neuzil, K. M. (2021). Interplay between emerging SARS-CoV-2 variants and pandemic control. N. Engl. J. Med. 384 (20), 1952–1954. doi:10.1056/NEJMe2103931
NIH (2002). “National Institute of health, division of microbiology and infectious disease screening and testing program,” in Broad-spectrum antiviral efficacy of natural-product humates now trademarked viracillin. Available at: https://www.viracillin.com/pdfs/4214a_National_Institutes_of_Health.pdf (Accessed July 18, 2022).
Orlov, A. A., Zherebker, A., Eletskaya, A. A., Chernikov, V. S., Kozlovskaya, L. I., Zhernov, Y. V., et al. (2019). Examination of molecular space and feasible structures of bioactive components of humic substances by FTICR MS data mining in ChEMBL database. Sci. Rep. 9 (1), 12066. doi:10.1038/s41598-019-48000-y
Orsi, M. (2014). Molecular dynamics simulation of humic substances. Chem. Biol. Technol. Agric. 1, 10. doi:10.1186/s40538-014-0010-4
Pant, K., Yadav, A. K., Gupta, P., Rathore, A. S., Nayak, B., and Venugopal, S. K. (2016). Humic acid inhibits HBV-induced autophagosome formation and induces apoptosis in HBV-transfected Hep G2 cells. Sci. Rep. 6, 34496. doi:10.1038/srep34496
Piccolo, A., Spaccini, R., Drosos, M., Vinci, G., and Cozzolino, V. (2018). “The molecular composition of humus carbon: Recalcitrance and reactivity in soils,” in The future of soil carbon. Editors C. Garica, P. Nannipieri, and T. Hernandez (Academic Press), 87–124.
Pottage, J. C., and Kessler, H. A. (1995). Herpes simplex virus resistance to acyclovir: Clinical relevance. Infect. Agents Dis. 4 (3), 115–124.
Prichard, M. N., Turk, S. R., Coleman, L. A., Engelhardt, S. L., Shipman, C., and Drach, J. C. (1990). A microtiter virus yield reduction assay for the evaluation of antiviral compounds against human cytomegalovirus and herpes simplex virus. J. Virological Methods 28 (1), 101–106. doi:10.1016/0166-0934(90)90091-s
Rege, A., Ambaye, R., and Deshmukh, R. (2012). Evaluation of in vitro inhibitory effect of selected plants and Shilajit on HIV-Reverse Transcriptase. Indian J. Nat. Prod. Resour. 3, 145–151.
Repetto, G., del Peso, A., and Zurita, J. L. (2008). Neutral red uptake assay for the estimation of cell viability/cytotoxicity. Nat. Protoc. 3 (7), 1125–1131. doi:10.1038/nprot.2008.75
Schneider, J., Weis, R., Männer, C., Kary, B., Werner, A., Seubert, B. J., et al. (1996). Inhibition of HIV-1 in cell culture by synthetic humate analogues derived from hydroquinone: Mechanism of inhibition. Virology 218 (2), 389–395. doi:10.1006/viro.1996.0208
Shashkina, M. Y., Shashkin, P. N., and Sergeev, A. V. (2006). Chemical and medicobiological properties of chaga (review). Pharm. Chem. J. 40, 560–568. doi:10.1007/s11094-006-0194-4
Smirnova, O. V., Efimova, I. V., and Khil’ko, S. L. (2012). Antioxidant and pro-oxidant activity of ascorbic and humic acids in radical-chain oxidation processes. Russ. J. Appl. Chem. 85, 252–255. doi:10.1134/S1070427212020164
Stevenson, F. J. (1994). Humus chemistry: Genesis, composition, reactions. 2nd Ed. John Wiley & Sons, 512.
Sutton, R., and Sposito, G. (2005). Molecular structure in soil humic substances: The new view. Environ. Sci. Technol. 39 (23), 9009–9015. doi:10.1021/es050778q
te Velthuis, A. J., van den Worm, S. H., Sims, A. C., Baric, R. S., Snijder, E. J., and van Hemert, M. J. (2010). Zn(2+) inhibits coronavirus and arterivirus RNA polymerase activity in vitro and zinc ionophores block the replication of these viruses in cell culture. PLoS Pathog. 6 (11), e1001176. doi:10.1371/journal.ppat.1001176
Terasawa, M., Hayashi, K., Lee, J. B., Nishiura, K., Matsuda, K., Hayashi, T., et al. (2020). Anti-influenza A virus activity of rhamnan sulfate from green algae monostroma nitidum in mice with normal and compromised immunity. Mar. Drugs 18 (5), 254. doi:10.3390/md18050254
van Rensburg, C. E., Dekker, J., Weis, R., Smith, T. L., Janse van Rensburg, E., and Schneider, J. (2002). Investigation of the anti-HIV properties of oxihumate. Chemotherapy 48 (3), 138–143. doi:10.1159/000064919
Vetvicka, V., Baigorri, R., Zamarreño, A. M., Garcia-Mina, J. M., and Yvin, J. C. (2010). Glucan and humic acid: Synergistic effects on the immune system. J. Med. Food 13 (4), 863–869. doi:10.1089/jmf.2009.0178
Vetvicka, V., Vashishta, A., Fuentes, M., Baigorri, R., Garcia-Mina, J. M., and Yvin, J. C. (2013). The relative abundance of oxygen alkyl-related groups in aliphatic domains is involved in the main pharmacological-pleiotropic effects of humic acids. J. Med. Food 16 (7), 625–632. doi:10.1089/jmf.2012.0212
Vladimirovna, T. T., Viktorovich, P. O., Skarnovich, M. O., and Ivanovich, B. N. (2021). SARS-CoV-2 coronavirus replication inhibitor based on humic substances. Russian patent RU2752872C1.
Waksman, S. A. (1936). Humus origin, chemical composition, and importance in nature. Soil Sci. 41 (5), 395. doi:10.1097/00010694-193605000-00010
Winkler, J., and Ghosh, S. (2018). Journal of diabetes research. Hindawi Limited. doi:10.1155/2018/5391014Therapeutic potential of fulvic acid in chronic inflammatory diseases and diabetes
World Health Organization, (2022). Virology of human influenza. Available at: https://www.euro.who.int/en/health-topics/communicable-diseases/influenza/data-and-statistics/virology-of-human-influenza (Accessed June 12, 2022).
Zhernov, Y. (2018). Natural humic substances interfere with multiple stages of the replication cycle of human immunodeficiency virus. J. Allergy Clin. Immunol. 141, AB233. doi:10.1016/j.jaci.2017.12.737
Zhernov, Y. V., Konstantinov, A. I., Zherebker, A., Nikolaev, E., Orlov, A., Savinykh, M. I., et al. (2021). Antiviral activity of natural humic substances and shilajit materials against HIV-1: Relation to structure. Environ. Res. 193, 110312. doi:10.1016/j.envres.2020.110312
Zhernov, Y. V., Kremb, S., Helfer, M., Schindler, M., Harir, M., Mueller, C., et al. (2017). Supramolecular combinations of humic polyanions as potent microbicides with polymodal anti-HIV-activities. New J. Chem. 41, 212–224. doi:10.1039/C6NJ00960C
Keywords: Humic acid (HA), fulvic acid, shilajit, humic substances, carboxylated polyanions, antiviral, extracellular and intracellular mechanisms of action
Citation: Socol DC (2023) Clinical review of humic acid as an antiviral: Leadup to translational applications in clinical humeomics. Front. Pharmacol. 13:1018904. doi: 10.3389/fphar.2022.1018904
Received: 24 August 2022; Accepted: 05 December 2022;
Published: 04 January 2023.
Edited by:
Swaran J. S. Flora, National Institute of Pharmaceutical Education and Research, IndiaReviewed by:
Amal Hathout, National Research Centre, EgyptCopyright © 2023 Socol. This is an open-access article distributed under the terms of the Creative Commons Attribution License (CC BY). The use, distribution or reproduction in other forums is permitted, provided the original author(s) and the copyright owner(s) are credited and that the original publication in this journal is cited, in accordance with accepted academic practice. No use, distribution or reproduction is permitted which does not comply with these terms.
*Correspondence: David C. Socol, ZGF2aWRAaHVtZW8uaW8=
Disclaimer: All claims expressed in this article are solely those of the authors and do not necessarily represent those of their affiliated organizations, or those of the publisher, the editors and the reviewers. Any product that may be evaluated in this article or claim that may be made by its manufacturer is not guaranteed or endorsed by the publisher.
Research integrity at Frontiers
Learn more about the work of our research integrity team to safeguard the quality of each article we publish.